- 1Department of Ecology, Evolution and Behavior, University of Minnesota, Saint Paul, MN, United States
- 2Department of Biology & Monte L. Bean Museum, Brigham Young University, Provo, UT, United States
Flowers have evolved signals that exploit the sensory systems of insect visitors. In the case of visual cues, color signals are thought to have been shaped in large part by the spectral sensitivity of key pollinators, such as hymenopterans. Beetles were some of the first plant pollinators, pre-dating the angiosperm radiation but with the exception of a few well-studied species, the evolution of flower-visiting beetle visual systems is poorly understood. Thus, the ability of beetles to detect and distinguish flower color signals and perhaps their potential role in shaping flower coloration is not well understood. Traditional models of pollinator visual systems often assume a putative tri- or tetrachromatic flower-visitor, as is found in bees, flies and butterflies. Beetles are unique among modern pollinators as ancestrally they did not possess the machinery for trichromatic vision, lacking the blue-sensitive photoreceptor class. Research on the evolution of visual genes responsible for wavelength sensitivity (opsins) has revealed that beetles with putative tri- and tetrachromatic visual systems have evolved independently, along multiple lineages. We explore the evolution of beetle visual genes using newly generated and publicly available RNA-seq data from 25 species with flower associations, including previously unexplored key flower-visitor groups and 20 non-flower visiting relatives. Our findings serve as a resource to inform and guide future studies on beetle-flower interactions, where insight from both signal and receiver is needed to better understand these poorly explored systems.
Introduction
Beetles were some of the earliest pollinators and remain the primary pollinators of ancient plant group, gymnosperms (Toon et al., 2020), while also functioning as common pollinators of more recent angiosperm (flowering plant) radiations. Angiosperm radiations during the Cretaceous co-occurred with accelerated diversification among the holometabolous insects that comprise the dominant insect pollinators: hymenopterans, lepidopterans, dipterans and coleopterans (Doyle, 2012; Misof et al., 2014). This co-radiation is thought to be in part due to the establishment of close associations or mutualisms between pollinator and plant, which likely contributed to the diversification of floral pollinator signals seen in modern flowers (Bronstein et al., 2006; Cardinal and Danforth, 2013). It is estimated that there are over 77,000 extant beetle species with flower associations (Wardhaugh, 2015) and flower visitation in these species has arisen via various evolutionary routes. While some species have retained existing ancestral gymnosperm associations (e.g., Boganiidae) (Cai et al., 2018), pollination behavior likely transitioned in some beetle lineages from gymnosperms to angiosperms (e.g., Oedemeridae and Kateretidae) (Peris et al., 2017, 2020). In other beetle lineages, flower associations evolved without preexisting pollination behavior (e.g., Glaphyridae) (Sabatinelli et al., 2020), as in Anthophila (bees) (Cardinal and Danforth, 2013; Peters et al., 2017).
Traditionally, flowers visited by beetles have been described as dull in coloration and highly scented, suggesting that beetles do not use or are not reliant on spectral cues to detect flowers (Faegri and Van der Pijl, 1979). Perhaps unsurprisingly, considering the diversity of beetles, as more beetle-flower interactions have been described it has become clear that many beetle species do use spectral information for flower detection. Pollinators in the families Meloidae (blister beetles), Glaphyridae (bumble-bee scarabs), and Scarabaeidae (monkey beetles), use color alone as a cue for flower detection (Dafni et al., 1990; Steiner, 1998; Van Kleunen et al., 2007; Paudel et al., 2017). In carabids and fireflies, heavier investment in vision (larger eyes) rather than olfaction (reduced antennae) has been shown to be driven by visually mediated tasks (Bauer and Kredler, 1993; Stanger-Hall et al., 2018). It is not yet known whether there are similar trade-offs between flower visiting beetles that utilize predominantly visual or olfactory cues. Angiosperms that are pollinated primarily by beetles span at least 34 families (Figure 1; Bernhardt, 2000) thus there are likely many beetle-flower associations still to be described and the role of visual cues to be determined.

Figure 1. Flower-visiting beetle families included in this study. From left to right: Cetoniinae (Trichaulax philipsii), Buprestidae (Acmaeodera sp.), Lycidae (Lycus sp.), Curculionidae and Nitidulidae (Aethina concolor). Images by: Chris Moeseneder, GSP, Russ Anderson, Steven Marshall, GSP.
The role of insect pollinator visual systems in shaping floral color cues has been well studied in the context of hymenopteran pollination systems (e.g., Chittka, 1996; Dyer et al., 2012). The spectral sensitivities of hymenopteran UV-, blue- and green-sensitive photoreceptors are positioned for optimum wavelength discrimination of floral spectral cues (Chittka and Menzel, 1992). Rather than tuning of insect spectral sensitivity, this relationship is thought to have arisen from spectral tuning of floral reflectance, to existing photoreceptor sensitivities (Chittka, 1996; Dyer et al., 2012). The underlying sensitivity of a photoreceptor is determined by the structure of a GPCR protein (opsin), which is coupled to a light-absorbing chromophore pigment. Unlike the vast majority of insects studied, including bees and butterflies, beetles lack a key opsin (SW) that typically confers sensitivity to blue wavelengths of light (Jackowska et al., 2007), lost prior to the radiation of beetles (Sharkey et al., 2017). Based on spectral sensitivity measurements of beetles with this ancestral condition, it is assumed that the ancestor of all beetles had a dichromatic UV-green color visual system (Gribakin, 1981; Warrant and McIntyre, 1990).
The lack of a dedicated blue-sensitive photoreceptor channel impacts the discriminatory capabilities of certain wavelengths, particularly within the violet-blue region of the light spectrum where photon catch is low. The ancestral beetle visual system is less complex than bees, for example, which have retained all three insect opsin classes UV, SW and LW (long wavelength), resulting in UV- blue- and green-sensitivity. Opsin duplication events are the major route for acquiring additional spectral channels. Duplications alone do not lead to novel spectral sensitivities; selection for changes in function is required via mutations that lead to changes in protein (subfunctionalization). In a number of coleopteran lineages, duplications of the UV opsin and subsequent subfunctionalization has led to the ability of these taxa to perceive blue wavelengths (Sharkey et al., 2017) by essentially “re-evolving” a dedicated blue-sensitive photoreceptor, for example in coccinellids (Lin, 1993) and chrysomelids (Döring and Skorupski, 2007). There is also evidence that flower-visiting lineages of beetles in the families Scarabaeidae, Nitidulidae, and Curculionidae have expanded their opsin repertoire, likely expanding their wavelength sensitivities (Sharkey et al., 2017). It is not known if this is true across the diversity of beetle pollinators.
We explore opsin expansions across the diversity of beetle pollinators using publicly available RNA-seq data, opsin sequences and an additional nine transcriptomes generated in this study. We aimed to investigate the evolution of the visual genes (opsins) that underpin sensitivity to spectral (color) information. Transcriptome data allow us to examine the diversity of opsin genes and hence putative wavelength sensitivities, from these flower-visiting species and their close relatives. In addition, we examine relative eye size in these species as an indicator of visual system investment.
Materials and Methods
RNA Extraction and Assembly
Seven flower-visiting beetle species (Trirhabda eriodictyonis, Sibinia setosa, Tychius meliloti, Anthrenus lepidus, Mordella albosuturalis, Nitops pallipennis, and Anaspis rufa) were collected from flowers in southern Utah into RNAlater and later frozen (–80°C) until processed. Multiple individuals were pooled for RNA extraction with the exception of T. eriodictyonis where one male was used. Total RNA was extracted from adult whole bodies using Nucleospin spin columns (Clontech) and reverse transcribed into cDNAs using the Illumina TruSeq RNA v2 kit. Sequencing was done on an Illumina HiSeq 2,500 generating 100 bp paired-end reads (BYU DNA sequencing center). RNA from two additional nitidulid species was extracted using the RNeasy Mini kit (Qiagen) and transcribed with the KAPA Stranded mRNA-Seq kit (Roche). Sequencing was completed on an Illumina HiSeq 2,500 generating 250 bp paired-end reads (BYU DNA sequencing center). Additionally, paired-end RNA-seq data from 36 species were downloaded from the Sequence Read Archive (SRA). Data were trimmed using Trimmomatic (Bolger et al., 2014), removing adapter sequences and poor-quality bases using the parameters: SLIDINGWINDOW:4:5 LEADING:5 TRAILING:5 MINLEN:25. Trinity (v2.11.0) (Grabherr et al., 2011; Haas et al., 2013) was used to assemble the remaining reads. Completeness of assemblies was estimated by searching each for the presence of 1367 insect Benchmarking Universal Single-Copy Orthologs using BUSCO v5 (Simão et al., 2015; Seppey et al., 2019). See Supplementary Table 1 for all species information. Opsin sequences have been deposited in GenBank with accession numbers MW885977—MW886096 and RNA-seq data have been uploaded to the Sequence Read Archive (PRJNA718629).
Opsin Search
Coding regions were predicted using TransDecoder v5.5.0,1 retaining the longest open reading frame (ORF). All predicted ORFs were also BLASTed2 against a database of known arthropod opsins (orthodb EOG8NKF98), with the addition of Lampyridae and Thermonectus marmoratus full-length opsin copies with an e-value of 0.001. All remaining ORFs were searched against our insect opsin database using hmmscan in HMMER (v3.3) (Eddy, 2011). Cross-contamination and pseudogenes were removed using phylogenies of DNA and protein opsin sequences and by examining alignments. Opsins with > 99% similarity in protein sequence, likely structurally and functionally identical, were removed (CD-hit v4.8.1; Li and Godzik, 2006; Fu et al., 2012). Duplicates with 100% sequence identity using local alignment (BLASTp) were considered to be one opsin gene (Anthocomus equestris and Pharaxonotha floridana; see asterisks Figure 2 and Supplementary Table 2).
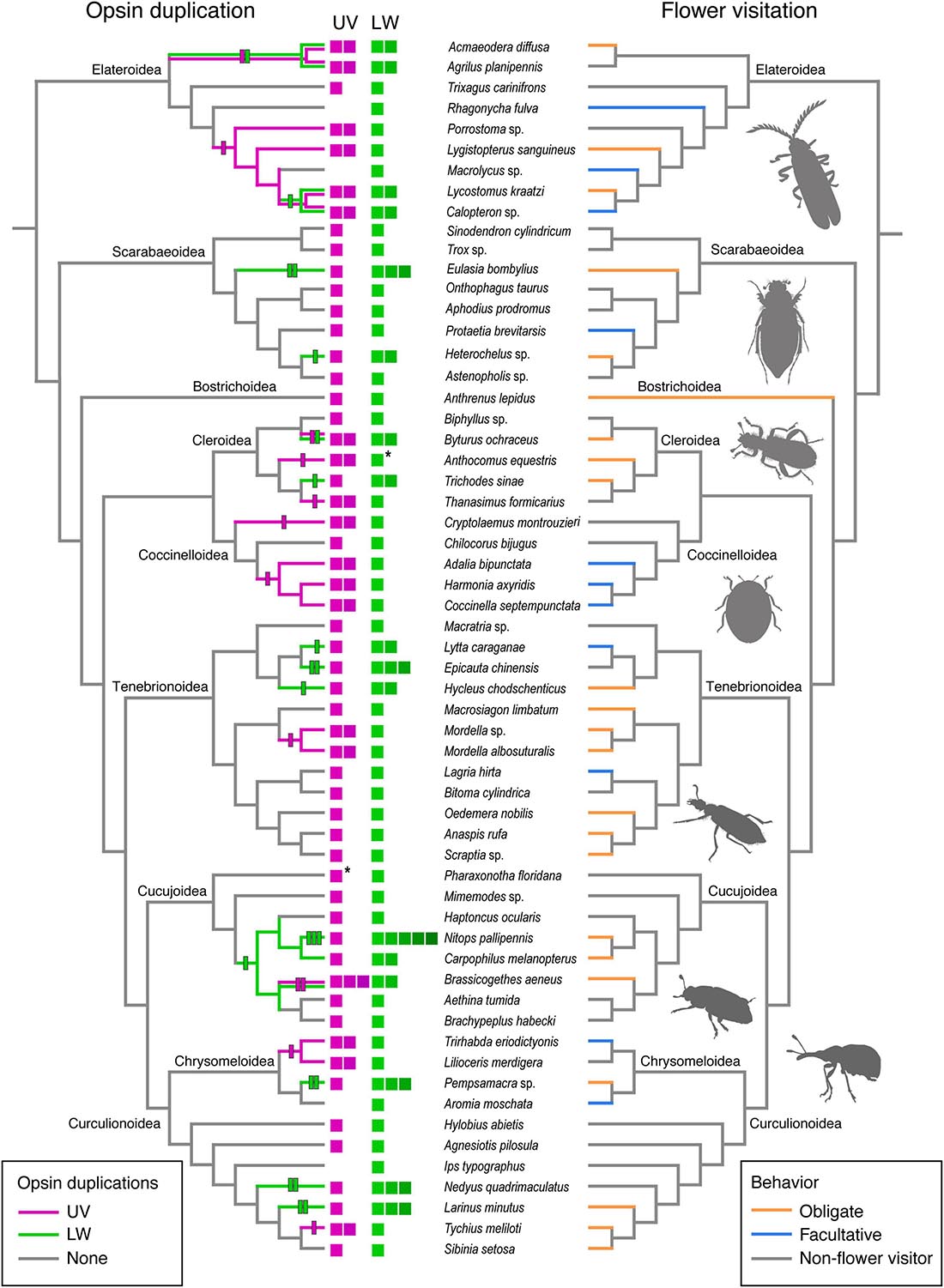
Figure 2. Patterns of opsin duplication and flower-visitation behavior for 59 beetle species. The cladograms represent a simplified version of beetle relationships after McKenna et al. (2019) with the position of superfamilies indicated. The left-hand cladogram shows opsin duplication events with opsin copy number at the terminals and duplication events indicated on the branches. Duplication events were estimated using the opsin tree (see Supplementary Figure 2). Asterisks (*) denote cases where additional duplication copies were found but determined not to be functionally distinct (see Opsin Search Methods text). The right-hand cladogram reflects known behavioral categories for the level of flower visitation. No attempt at ancestral reconstruction was made due to the available taxon sampling.
Final DNA sequences and additional insect opsins were subject to codon alignment (MAFFT v7.453; Katoh et al., 2002; Katoh and Standley, 2013) with automatic alignment strategy detection. Maximum likelihood opsin DNA gene trees were generated using IQ-tree (v1.6.12) (Minh et al., 2013; Nguyen et al., 2015). The substitution model GTR + F + I + G4 was selected automatically using ModelFinder (Kalyaanamoorthy et al., 2017). A species topology was generated in Mesquite 3.2 (Maddison and Maddison, 2018) based on McKenna et al. (2019). For statistical analyses, beetle species were categorized as obligate flower-visitors that require floral resources for food or reproduction (A), facultative flower visitors with known floral associations (e.g., facultative pollinators) but no reliance on floral resources for food or reproduction (B) and non-flower visitors with no known association with flowers (C).
Eye Size Measurements and Statistics
Relative eye size was generated using the measurement tools in Adobe Photoshop v.19.1.6 from high resolution habitus photos (available upon request due to copyright) with an unimpeded dorsal view of the head. Two measurements were taken for each photo: total head width including eyes, and interocular distance (see Supplementary Figure 1 for example image). These two measurements were used to generate the total width of the eyes in the dorsal view and used to calculate a percentage of lateral head space dedicated to eye tissue as viewed dorsally. We tested for a relationship between UV and LW opsin copy number (Pearson’s correlation test) and if flower visitation behavior predicted relative eye-size (one-way ANOVA). Both were included as predictors in the final MANOVA. Because LW and UV were not correlated, we chose to test the effects of relative eye size and flower visitation on both LW and UV opsin copy number (MANOVA). For the purpose of this analysis, species without UV opsin copies were omitted. All data met required assumptions, and no data transformations were performed. All eye size statistical tests were executed in SPSS 27 (IBMCorp.).
Results
Opsin Duplications
A transcriptomics approach was taken to explore the putative wavelength sensitivities of flower visiting and non-flower visiting beetle species. To determine possible wavelength sensitivity expansions, RNA-seq data from 45 species spanning 26 families were mined for opsins and data were analyzed for the presence of duplication events. Nine transcriptomes from flower-visiting species were generated for this study to expand the diversity of sampled lineages. We recovered 120 opsin copies, 92 of which were full length copies with a minimum length of 101 amino acids. We classified 18 beetle species as obligate flower visitors (category A), seven as facultative flower visitors (category B), and 20 with no known floral associations (category C). Additionally, we included 18 previously published beetle opsins from five category A species, 11 opsins from four category B species and 14 opsins from five category C species (Sharkey et al., 2017; Supplementary Table 1).
In our dataset both obligate (A) and facultative (B) flower visitors had 2-fold higher proportions of UV duplications present, 39.1 and 45.5%, respectively, than non-flower visitors (C) with only 20%. LW duplications were more common in obligate flower visitors (52.2%) than either facultative (18.2%) or non-flower visitors (12%). The prevalence of either opsin duplication was highest in obligate flower visitors (73.9%), lower in flower-associated species (54.5%) and lowest in non-flower visitors sampled (28%). We report 33 independent opsin duplication events (12 UV and 21 LW), which have occurred across the diversity of Coleoptera (Figure 2). Duplication events occurred within all but one superfamily, Bostrichoidea, which has only a single flower-visiting lineage (the Dermestidae) represented by Anthrenus lepidus. Percent identity (sequence similarity) of aligned duplicate opsins (BLASTp, see text footnote 2) ranged from 64 to 98% sequence identity and a mean of 80% (see Supplementary Table 2).
Within Scarabaeoidea only flower visiting scarabs exhibit opsin duplications. These duplications were independent and solely among LW opsins in Heterochelus sp. and Eulasia bombylius with one and two duplications, respectively (Figure 2). Notably, Protaetia brevitarsis, which can commonly be found on flowers (B) but is not considered an obligate flower visitor, did not have opsin duplicates. Among the five Lycidae (Elateroidea) species sampled, the UV opsin duplicates present were orthologous (Figure 2 and Supplementary Figure 2) and therefore represent an early duplication event in this group. Two LW opsin copies were present in two lycid species Lycostomus kraatzi and Calopteron sp. However, this was not linked to flower visiting behavior with the former likely an obligate (A) and the latter only facultatively associated with flowers (B). Rather, these species are among the most derived members of this group and therefore highlight a potential ancestral LW opsin duplication event that occurred during the lycid radiation. All flower visiting members (A) of Cleroidea have either UV or LW duplicates. Each duplication event was independent, including within the subfamily Clerinae where a UV duplication occurred in Thanasimus formicarius and a LW duplication in the flower visitor Trichodes sinae.
Coccinellid opsin UV duplicates have been previously examined (Sharkey et al., 2017). We add the species Chilocorus bijugus (tribe: Chilocorini), which has no known associations with flowers. No UV duplicate was recovered for this species making this species unique among the coccinellids sampled thus far. Our opsin phylogeny (Supplementary Figure 2) suggest that two separate UV duplication events occurred, one prior to the diversification of the tribe Coccinellini and the other along lineage sister to the rest of the coccinellids, Cryptolaemus montrouzieri (tribe: Coccidulini). We note only two opsin duplication events within the Tenebrionoidea, within the blister beetles Meloidae (Hycleus chodschenticus) (LW duplication) and tumbling flower beetles Mordellidae (Mordella sp. and Mordella albosuturalis) (UV duplication). Opsin orthologs are present in both Mordella species, suggesting that this duplicate may be shared among other members of this genus.
A close relationship between flower visitation and opsin copy number can be clearly seen among the cucujoids (Figure 2). Single UV and LW opsin copies are present in all non-flower visiting species but UV and/or LW opsin duplications are present among all flower-visiting nitidulids sampled (Figure 2). The LW opsin duplicates form two clades shared among these species suggesting an early LW duplication event in Nitidulidae that has potentially been lost in the three non-flower visiting lineages. Two of the three obligate flower visiting weevils (Curculionidae) species possess additional opsin copies.
While duplications more commonly occurred amongst flower visiting species (categories A and B), than in non-flower visitors (C), 26% of obligate flower visitors have retained the ancestral UV-LW opsin condition. Among the obligate flower visitors, four of the seven tenebrionid species sampled have single opsin copies, including both members of Scraptiidae (Scraptia sp. and Anaspis rufa), Oedemera nobilis (Oedemeridae), and Macrosiagon limbatum (Ripiphoridae) (Figure 2). Carpet beetle Anthrenus lepidus (Dermestidae: Bostrichoidea), Biphyllus sp. (Biphyllidae: Cleroidea) and two weevil species, Tychius meliloti and Sibinia setosa (Curculionidae: Curculionoidea) also did not have opsin duplications. We did not recover UV opsins from four non-obligate flower visiting species, Rhagonycha fulva, Macrolycus sp., Aromia moschata or Ips typographus but LW opsins were recovered from all of these species.
Eye Size, Flower Visitation and Opsin Copy Number
Relative eye size was used as an approximate measure of visual investment across the species in this study. We found no significant correlation between UV and LW opsin copy number (p = 0.893). Flower visitation category (A, B or C) did significantly predict relative eye size (F = 8.52, df = 2, p < 0.001). Post hoc tests revealed obligate flower visiting species (A) have significantly larger eyes than those without any floral association (C) (p < 0.001, mean difference = 11.15%). Flower visitation category in conjunction with relative eye size was a significant predictor of LW copy number (F = 3.895, df = 32, p = 0.015), but not UV copy number (F = 2.06.11, df = 32, p = 0.122). While the overall model showed evidence for the combination of both flower visitation and eye size influencing LW copy number, neither was significant individually.
Discussion
A previous study (Sharkey et al., 2017) found opsin duplications in four of five flower-visiting beetle species. While LW opsin duplications were not common generally, in the coleopterans sampled, they were ubiquitous among these flower-visitors. These findings suggested that there may be a selective advantage for duplications, such as an expansion of wavelength sensitivity, among species that may use floral cues. Our opsin analysis across numerous flower-visiting species of coleopterans suggests that associations with flowers has often led to or precedes the expansion of wavelength sensitivity through opsin duplication. We cannot attribute an opsin duplication event strictly to the evolution of flower visitation behavior due to associated factors, such as diurnality (Sondhi et al., 2021) and other visually guided behaviors (e.g., host-plant seeking), for which expanded wavelength sensitivity may be beneficial. However, by including closely related non-flower visiting relatives in this study, we show that duplication events and particularly LW opsin duplications, do commonly occur along lineages associated with flowers, more so than those that have no floral associations. The frequency of duplications varies among other pollinator groups. Hymenopterans exhibit relatively stable opsin copy numbers (Spaethe and Briscoe, 2004; Oeyen et al., 2020) but in contrast the opsin repertoire of lepidopterans is highly variable, particularly within the butterflies, Papilionoidea (Sondhi et al., 2021). Our finding that there have been many independent duplication events in flower-visiting coleopterans may be attributed to the ancestral loss of the SW opsin in this group, or as in butterflies, may suggest increased spectral richness of the visual system.
Opsin duplications do not in all cases lead to new photoreceptor sensitivities, as is the extreme case in odonates, where there is a large excess of opsin copies (Futahashi et al., 2015; Suvorov et al., 2016) compared to measured photoreceptor sensitivities (Laughlin, 1976; Yang and Osorio, 1991). In contrast, it has been shown that beetles are relatively conservative in opsin copy number (Sharkey et al., 2017). Therefore, opsin duplicates can be used as a guide for photoreceptor sensitivity diversity. For example, all sampled jewel beetles (Buprestidae) and Carabus sp. (Carabidae) have 2 UV and 2 LW opsin copies (Lord et al., 2016; Sharkey et al., 2017) that underpin UV-, blue-, green-, and red-sensitive photoreceptors (Hasselmann, 1962; Meglič et al., 2020), demonstrating increased function of photoreceptor sensitivity for these groups as a result of opsin duplication. Further, the presence of blue-sensitive photoreceptors also aligns well with the presence of a UV opsin duplication, in coccinellids (Lin and Wu, 1992) and chrysomelids (Döring and Skorupski, 2007). Additionally, species with ancestral UV-green sensitivity and complementary opsin data only possess a single UV and a single LW opsin copy (e.g., Lampyridae and Dendroctonus) (Groberman and Borden, 1982; Lall et al., 2010; Martin et al., 2015; Sander and Hall, 2015).
UV opsins were absent in four species. The UV opsin typically has lower expression levels as often UV-sensitive photoreceptors are less numerous than long wavelength-sensitive receptors in insects. Thus, we cannot be sure whether this finding reflects inadequate sequencing depth in these species or a true opsin loss. The use of head or eye tissue rather than whole body specimens, or deeper sequencing, may be necessary for future studies. Additionally, opsin expressed in photoreceptors with low abundance, such as those used in highly specialized regions (e.g., the polarization-sensitive dorsal rim area) may also have low signal. Further study to determine opsin abundance and expression patterns is required to better understand how well opsin copy number is estimated from whole body specimens, in particular those with reduced eyes.
Flowers commonly frequented by beetles have been traditionally described as dull in coloration (white, green or yellow) but highly scented, thought to exploit existing attraction to certain volatile compounds, such as skatole, attractive to coprophagous beetles (Schiestl and Dötterl, 2012). Despite compelling evidence that many beetles use floral visual cues for flower visitation, the study of associated visual adaptations is lacking. Scarab pollinators provide compelling evidence for vision as the primary cue for flower detection. Bumble-bee scarabs (Glaphyridae) and monkey beetles (Hopliini) use the dark center of a flower as a visual cue, termed “beetle marks” (Dafni et al., 1990; Johnson and Midgley, 2001). Other non-scarab beetle pollinators are also attracted to dark spots on flowers, e.g., nitidulids (Free and Williams, 1978) and mordellids (Westmoreland and Muntan, 1996).
In scarab beetles, anthophagy (flower feeding) has evolved at least seven times (Ahrens et al., 2014). Three of these lineages have been sampled in this study: Glaphyridae, Hopliini and Cetoniinae. We found LW opsin duplications among the two lineages that are known to have preferences for red and orange flowers, Glaphyridae (Sabatinelli et al., 2020) and Hopliini (Johnson and Midgley, 2001). The bumble-bee scarab Pygopleurus israelitus (Glaphyridae) has both green- and red-sensitive photoreceptors (λmax: 631 nm) the latter of which increase the conspicuousness of red bowl-shaped flowers they visit (Martínez-Harms et al., 2012). This suggests a role for LW opsin duplication and subfunctionalization to expand long-wavelength sensitivity for floral detection. Electrophysiological measurements of Protaetia brevitarsis (Cetoniinae), a facultative flower visitor, are in agreement with our finding that this species only has UV and green sensitivity (Lin and Wu, 1992) but curiously this species exhibits attraction to red over green stimuli (Cai et al., 2021). We have yet to find any opsin duplications in the eight additional scarabs examined thus far (Sharkey et al., 2017), highlighting flower-visiting scarabs as an interesting group to study visual systems and signals in the context of anthophily.
Flower visitation is not always a predictor of an opsin duplication event; opsin duplications were absent from six flower-visiting lineages. It is possible that these species rely more heavily on olfaction than vision, and we may expect to see greater investment in these sensory structures (e.g., larger antennae), rather than vision (Bauer and Kredler, 1993; Stanger-Hall et al., 2018). Such sensory adaptations can be seen in the flabellate (fan-shaped) antennae of Macrosiagon limbatum (Ripiphoridae). Additionally, if odor was a primary cue in ancient cycads, as in modern species (Toon et al., 2020), lineages such as the false oil beetles (Oedemeridae) may have initially established olfactory rather than visual specializations that persisted after they transitioned to angiosperm hosts (Peris et al., 2017). A UV-green color channel system may be adequate to detect floral cues commonly attractive to beetles, e.g., white and yellow (Reverté et al., 2016), but wavelength sensitivity expansion may be advantageous to detect floral cues that fall outside this spectral range (e.g., pink or violet).
In this study we measured eye size as a proportion of the head (i.e., relative eye size), which has been used in prior studies of beetles to test relationships between visual investment and behavior (Bauer and Kredler, 1993; Stanger-Hall et al., 2018). This does not give a perfect estimation of eye volume due to the variation in eye shape across coleopterans with extreme morphological diversity. However, using relative eye size to examine the potential link between flower visitation, opsin copy number and investment in vision reveals some interesting findings. For the species examined in this study, eye size was found to be predicted by flower visitation behavior with obligate flower visitors having larger eyes as a proportion of the head. This suggests that flower-visitation or associated visual ecology may have driven selection for greater investment in vision. Similarly, flower visitors were also more likely to have a greater LW opsin copy number, suggesting that an expansion of long-wavelength sensitivity is advantageous for behaviors associated with flower visitation in the species we have examined. This is certainly true among beetles that prefer orange and red flowers and have dedicated red-sensitive photoreceptors, but red flower visitation is not commonplace among other species with LW duplications examined herein. Interestingly, among all species, LW opsin copy number was not correlated with eye size suggesting that it is not eye size alone that predicts the number of opsin duplicates. This may point to greater visual specialization in flower visiting beetles.
Our aim was to demonstrate that there is much more exciting work that needs to be done to better understand the evolution of beetle visual systems. This is particularly true for flower visitors, which have multiple origins within the majority of coleopteran superfamilies. Equally exciting is the large visual system diversity, morphological, molecular and certainly functional, among the anthophilous Coleoptera. In short, beetles represent a largely untapped area to study insect plant interactions at both the fine and coarse scales of evolution.
Data Availability Statement
The datasets presented in this study can be found in online repositories. The names of the repository/repositories and accession number(s) can be found below: https://www.ncbi.nlm.nih.gov/, PRJNA718629.
Author Contributions
CS conceived the study, ran transcriptomic and phylogenetic analyses, and wrote the manuscript. GP advised on beetle taxa, collected and analyzed eye size measurements. SB advised on beetle taxa and generated the species topology. All authors edited the manuscript and approved the submitted version.
Funding
This project was made possible by funding from the National Science Foundation DEB-1655981 to SB.
Conflict of Interest
The authors declare that the research was conducted in the absence of any commercial or financial relationships that could be construed as a potential conflict of interest.
Acknowledgments
We thank all the researchers who generated RNA-seq data that was used in this study. We are grateful to Nathan Lord and Gavin Martin for help with sample collection and processing. We also thank Alexandra Duffy for statistics advice.
Supplementary Material
The Supplementary Material for this article can be found online at: https://www.frontiersin.org/articles/10.3389/fevo.2021.676369/full#supplementary-material
Footnotes
References
Ahrens, D., Schwarzer, J., and Vogler, A. P. (2014). The evolution of scarab beetles tracks the sequential rise of angiosperms and mammals. Proc. R. Soc. B Biol. Sci. 281:20141470. doi: 10.1098/rspb.2014.1470
Bauer, T., and Kredler, M. (1993). Morphology of the compound eyes as an indicator of life-style in carabid beetles. Can. J. Zool. 71, 799–810. doi: 10.1139/z93-105
Bernhardt, P. (2000). Convergent evolution and adaptive radiation of beetle-pollinated angiosperms. Plant Syst. Evol. 222, 293–320. doi: 10.1007/BF00984108
Bolger, A. M., Lohse, M., and Usadel, B. (2014). Trimmomatic: a flexible trimmer for Illumina sequence data. Bioinformatics 30, 2114–2120. doi: 10.1093/bioinformatics/btu170
Bronstein, J. L., Alarcón, R., and Geber, M. (2006). The evolution of plant – insect mutualisms. New Phytol. 172, 412–428. doi: 10.1111/j.1469-8137.2006.01864.x
Cai, C., Escalona, H. E., Li, L., Yin, Z., Huang, D., and Engel, M. S. (2018). Beetle pollination of cycads in the mesozoic. Curr. Biol. 28, 2806–2812. doi: 10.1016/j.cub.2018.06.036
Cai, H., Zhang, T., Su, Y., Wang, Z., Zhang, X., Wang, S., et al. (2021). Influence of trap color, type, and placement on capture efficacy for Protaetia brevitarsis (Coleoptera: Scarabaeidae). J. Econ. Entomol. 114, 225–230. doi: 10.1093/jee/toaa259
Cardinal, S., and Danforth, B. N. (2013). Bees diversified in the age of eudicots. Proc. R. Soc. B Biol. Sci. 280:20122686. doi: 10.1098/rspb.2012.2686
Chittka, L. (1996). Does bee color vision predate the evolution of flower color? Naturwissenschaften 83, 136–138. doi: 10.1007/s001140050263
Chittka, L., and Menzel, R. (1992). The evolutionary adaptation of flower colours and the insect pollinators’ colour vision. J. Comp. Physiol. A 171, 171–181. doi: 10.1007/BF00188925
Dafni, A., Bernhardt, P., Shmida, A., Ivri, Y., Greenbaum, S., O’Toole, C. H., et al. (1990). Red bowl-shaped flowers: convergence for beetle pollination in the Mediterranean region. Isr. J. Bot. 39, 81–92.
Döring, T. F., and Skorupski, P. (2007). Host and non-host leaves in the colour space of the Colorado potato beetle (Coleoptera: Chrysomelidae). Entomol. Gen. 29, 81–95. doi: 10.1127/entom.gen/29/2007/81
Doyle, J. A. (2012). Molecular and fossil evidence on the origin of angiosperms. Annu. Rev. Earth Planet. Sci. 40, 301–326. doi: 10.1146/annurev-earth-042711-105313
Dyer, A. G., Boyd-Gerny, S., McLoughlin, S., Rosa, M. G. P., Simonov, V., and Wong, B. B. M. (2012). Parallel evolution of angiosperm colour signals: common evolutionary pressures linked to hymenopteran vision. Proc. Biol. Sci. 279, 3606–3615. doi: 10.1098/rspb.2012.0827
Eddy, S. R. (2011). Accelerated profile HMM searches. PLoS Comput. Biol. 7:e1002195. doi: 10.1371/journal.pcbi.1002195
Faegri, K., and Van der Pijl, L. (1979). The principles of pollination ecology. Oxford, UK: Pergamon Press.
Free, J. B., and Williams, I. H. (1978). The responses of the pollen beetle, Meligethes aeneus, and the seed weevil, Ceuthorhynchus assimilis, to oil-seed rape, Brassica napus, and other plants. J. Appl. Ecol. 15, 761–774. doi: 10.2307/2402773
Fu, L., Niu, B., Zhu, Z., Wu, S., and Li, W. (2012). CD-HIT: Accelerated for clustering the next-generation sequencing data. Bioinformatics 28, 3150–3152. doi: 10.1093/bioinformatics/bts565
Futahashi, R., Kawahara-Miki, R., Kinoshita, M., Yoshitake, K., Yajima, S., Arikawa, K., et al. (2015). Extraordinary diversity of visual opsin genes in dragonflies. Proc. Natl. Acad. Sci. 112, E1247–E1256. doi: 10.1073/pnas.1424670112
Grabherr, M. G., Haas, B. J., Yassour, M., Levin, J. Z., Thompson, D. A., Amit, I., et al. (2011). Full-length transcriptome assembly from RNA-Seq data without a reference genome. Nat. Biotechnol. 29, 644–652. doi: 10.1038/nbt.1883
Gribakin, F. G. (1981). Automatic spectrosensitometry of photoreceptors in Lethrus (Coleoptera, Scarabaeidae). J. Comp. Physiol. A 142, 95–102. doi: 10.1007/BF00605481
Groberman, L. J., and Borden, J. H. (1982). Electrophysiological response of Dendroctonus pseudotsugae and Ips paraconfusus (Coleoptera: Scolytidae) to selected wavelength regions of the visible spectrum. Can. J. Zool. 60, 2180–2189. doi: 10.1139/z82-279
Haas, B. J., Papanicolaou, A., Yassour, M., Grabherr, M., Blood, P. D., Bowden, J., et al. (2013). De novo transcript sequence reconstruction from RNA-seq using the Trinity platform for reference generation and analysis. Nat. Protoc. 8, 1494–1512. doi: 10.1038/nprot.2013.084
Hasselmann, E. M. (1962). Über die relative spektrale Empfindlichkeit von Käfer- und Schmetterlingsaugen bei verschiedenen Helligkeiten. Zool. Jahrbucher 69, 537–576.
Jackowska, M., Bao, R., Liu, Z., McDonald, E. C., Cook, T. A., and Friedrich, M. (2007). Genomic and gene regulatory signatures of cryptozoic adaptation: Loss of blue sensitive photoreceptors through expansion of long wavelength-opsin expression in the red flour beetle Tribolium castaneum. Front. Zool. 4:24. doi: 10.1186/1742-9994-4-24
Johnson, S. D., and Midgley, J. J. (2001). Pollination by monkey beetles (Scarabaeidae: Hopliini): Do color and dark centers of flowers influence alighting behavior? Environ. Entomol. 30, 861–868. doi: 10.1603/0046-225X-30.5.861
Kalyaanamoorthy, S., Minh, B. Q., Wong, T. K. F., Von Haeseler, A., and Jermiin, L. S. (2017). ModelFinder: Fast model selection for accurate phylogenetic estimates. Nat. Methods 14, 587–589. doi: 10.1038/nmeth.4285
Katoh, K., Misawa, K., Kuma, K., and Miyata, T. (2002). MAFFT: a novel method for rapid multiple sequence alignment based on fast Fourier transform. Nucleic Acids Res. 30, 3059–3066. doi: 10.1093/nar/gkf436
Katoh, K., and Standley, D. M. (2013). MAFFT multiple sequence alignment software version 7: Improvements in performance and usability. Mol. Biol. Evol. 30, 772–780. doi: 10.1093/molbev/mst010
Lall, A. B., Cronin, T. W., Carvalho, A. A., de Souza, J. M., Barros, M. P., Stevani, C. V., et al. (2010). Vision in click beetles (Coleoptera: Elateridae): pigments and spectral correspondence between visual sensitivity and species bioluminescence emission. J. Comp. Physiol. A 196, 629–638. doi: 10.1007/s00359-010-0549-x
Laughlin, S. B. (1976). The sensitivities of dragonfly photoreceptors and the voltage gain of transduction. J. Comp. Physiol. A 111, 221–247. doi: 10.1007/BF00606466
Li, W., and Godzik, A. (2006). Cd-hit: A fast program for clustering and comparing large sets of protein or nucleotide sequences. Bioinformatics 22, 1658–1659. doi: 10.1093/bioinformatics/btl158
Lin, J. T. (1993). Identification of photoreceptor locations in the compound eye of Coccinella septempunctata Linnaeus (Coleoptera, Coccinellidae). J. Insect Physiol. 39, 555–562. doi: 10.1016/0022-1910(93)90037-R
Lin, J. T., and Wu, C. Y. (1992). A comparative study on the color vision of four coleopterous insects. Bull. Inst. Zool. Acad. Sin. 31, 81–88.
Lord, N. P., Plimpton, R. L., Sharkey, C. R., Suvorov, A., Lelito, J. P., Willardson, B. M., et al. (2016). A cure for the blues: opsin duplication and subfunctionalization for short-wavelength sensitivity in jewel beetles (Coleoptera: Buprestidae). BMC Evol. Biol. 16:107. doi: 10.1186/s12862-016-0674-4
Maddison, W., and Maddison, D. (2018). Mesquite: a modular system for evolutionary analysis. Version 3.2. Available Online at: http://www.mesquiteproject.org
Martin, G. J., Lord, N. P., Branham, M. A., and Bybee, S. M. (2015). Review of the firefly visual system (Coleoptera: Lampyridae) and evolution of the opsin genes underlying color vision. Org. Divers. Evol. 15, 513–526. doi: 10.1007/s13127-015-0212-z
Martínez-Harms, J., Vorobyev, M., Schorn, J., Shmida, A., Keasar, T., Homberg, U., et al. (2012). Evidence of red sensitive photoreceptors in Pygopleurus israelitus (Glaphyridae: Coleoptera) and its implications for beetle pollination in the southeast Mediterranean. J. Comp. Physiol. A 198, 451–463. doi: 10.1007/s00359-012-0722-5
McKenna, D. D., Shin, S., Ahrens, D., Balke, M., Beza-Beza, C., Clarke, D. J., et al. (2019). The evolution and genomic basis of beetle diversity. Proc. Natl. Acad. Sci. U. S. A. 116, 24729–24737. doi: 10.1073/pnas.1909655116
Meglič, A., Ilić, M., Quero, C., Arikawa, K., and Belušič, G. (2020). Two chiral types of randomly rotated ommatidia are distributed across the retina of the flathead oak borer Coraebus undatus (Coleoptera: Buprestidae). J. Exp. Biol. 223:225920. doi: 10.1242/jeb.225920
Minh, B. Q., Nguyen, M. A. T., and Von Haeseler, A. (2013). Ultrafast approximation for phylogenetic bootstrap. Mol. Biol. Evol. 30, 1188–1195. doi: 10.1093/molbev/mst024
Misof, B., Liu, S., Meusemann, K., Peters, R. S., Donath, A., Mayer, C., et al. (2014). Phylogenomics resolves the timing and pattern of insect evolution. Science 346, 763–767. doi: 10.1126/science.1257570
Nguyen, L. T., Schmidt, H. A., Von Haeseler, A., and Minh, B. Q. (2015). IQ-TREE: A fast and effective stochastic algorithm for estimating maximum-likelihood phylogenies. Mol. Biol. Evol. 32, 268–274. doi: 10.1093/molbev/msu300
Oeyen, J. P., Baa-Puyoulet, P., Benoit, J. B., Beukeboom, L. W., Bornberg-Bauer, E., Buttstedt, A., et al. (2020). Sawfly genomes reveal evolutionary acquisitions that fostered the mega-radiation of parasitoid and eusocial Hymenoptera. Genome Biol. Evol. 12, 1099–1188. doi: 10.1093/GBE/EVAA106
Paudel, B. R., Shrestha, M., Dyer, A. G., and Li, Q.-J. (2017). Ginger and the beetle: Evidence of primitive pollination system in a Himalayan endemic alpine ginger (Roscoea alpina, Zingiberaceae). PLoS One 12:e0180460. doi: 10.1371/journal.pone.0180460
Peris, D., Labandeira, C. C., Barrón, E., Delclòs, X., Rust, J., and Wang, B. (2020). Generalist pollen-feeding beetles during the Mid-Cretaceous. iScience 23:100913. doi: 10.1016/j.isci.2020.100913
Peris, D., Pérez-de la Fuente, R., Peñalver, E., Delclòs, X., Barrón, E., and Labandeira, C. C. (2017). False blister beetles and the expansion of gymnosperm-insect pollination modes before angiosperm dominance. Curr. Biol. 27, 897–904. doi: 10.1016/j.cub.2017.02.009
Peters, R. S., Krogmann, L., Mayer, C., Donath, A., Gunkel, S., Meusemann, K., et al. (2017). Evolutionary history of the Hymenoptera. Curr. Biol. 27, 1013–1018. doi: 10.1016/j.cub.2017.01.027
Reverté, S., Retana, J., Gómez, J. M., and Bosch, J. (2016). Pollinators show flower colour preferences but flowers with similar colours do not attract similar pollinators. Ann. Bot. 118, 249–257. doi: 10.1093/aob/mcw103
Sabatinelli, G., Eberle, J., Fabrizi, S., and Ahrens, D. (2020). A molecular phylogeny of Glaphyridae (Coleoptera: Scarabaeoidea): evolution of pollination and association with ‘Poppy guild’ flowers. Syst. Entomol. 45, 838–848. doi: 10.1111/syen.12429
Sander, S. E., and Hall, D. W. (2015). Variation in opsin genes correlates with signalling ecology in North American fireflies. Mol. Ecol. 24, 4679–4696. doi: 10.1111/mec.13346
Schiestl, F. P., and Dötterl, S. (2012). The evolution of floral scent and olfactory preferences in pollinators: Coevolution or pre-existing bias? Evolution 66, 2042–2055. doi: 10.1111/j.1558-5646.2012.01593.x
Seppey, M., Manni, M., and Zdobnov, E. M. (2019). BUSCO: assessing genome assembly and annotation completeness. Methods Mol. Biol. 1962, 227–245. doi: 10.1007/978-1-4939-9173-0_14
Sharkey, C. R., Fujimoto, M. S., Lord, N. P., Shin, S., McKenna, D. D., Suvorov, A., et al. (2017). Overcoming the loss of blue sensitivity through opsin duplication in the largest animal group, beetles. Sci. Rep. 7:8. doi: 10.1038/s41598-017-00061-7
Simão, F. A., Waterhouse, R. M., Ioannidis, P., Kriventseva, E. V., and Zdobnov, E. M. (2015). BUSCO: Assessing genome assembly and annotation completeness with single-copy orthologs. Bioinformatics 31, 3210–3212. doi: 10.1093/bioinformatics/btv351
Sondhi, Y., Ellis, E. A., Bybee, S. M., Theobald, J., and Kawahara, A. (2021). Light environment drives evolution of color vision genes in butterflies and moths. Commun. Biol. 4:177. doi: 10.1038/s42003-021-01688-z
Spaethe, J., and Briscoe, A. D. (2004). Early duplication and functional diversification of the opsin gene family in insects. Mol. Biol. Evol. 21, 1583–1594. doi: 10.1093/molbev/msh162
Stanger-Hall, K. F., Lower, S. E. S., Lindberg, L., Hopkins, A., Pallansch, J., and Hall, D. W. (2018). The evolution of sexual signal modes and associated sensor morphology in fireflies (Lampyridae, Coleoptera). Proc. R. Soc. B Biol. Sci. 285:20172384. doi: 10.1098/rspb.2017.2384
Steiner, K. E. (1998). The evolution of beetle pollination in a South African orchid. Am. J. Bot. 85, 1180–1193. doi: 10.2307/2446351
Suvorov, A., Jensen, N. O., Sharkey, C. R., Fujimoto, M. S., Bodily, P., Wightman, H. M. C., et al. (2016). Opsins have evolved under the permanent heterozygote model: insights from phylotranscriptomics of Odonata. Mol. Ecol. 26, 1306–1322. doi: 10.1111/mec.13884
Toon, A., Terry, L. I., Tang, W., Walter, G. H., and Cook, L. G. (2020). Insect pollination of cycads. Austral. Ecol. 45, 1033–1058. doi: 10.1111/aec.12925
Van Kleunen, M., Nänni, I., Donaldson, J. S., and Manning, J. C. (2007). The role of beetle marks and flower colour on visitation by monkey beetles (Hopliini) in the greater cape floral region, South Africa. Ann. Bot. 100, 1483–1489. doi: 10.1093/aob/mcm256
Wardhaugh, C. W. (2015). How many species of arthropods visit flowers? Arthropod. Plant. Interact. 9, 547–565. doi: 10.1007/s11829-015-9398-4
Warrant, E. J., and McIntyre, P. D. (1990). Limitations to resolution in superposition eyes. J. Comp. Physiol. A 167, 785–803. doi: 10.1007/BF00189768
Westmoreland, D., and Muntan, C. (1996). The influence of dark central florets on insect attraction and fruit production in Queen Anne’s Lace (Daucus carota L.). Am. Midl. Nat. 135, 122–129. doi: 10.2307/2426878
Keywords: opsin, insect vision, pollinators, gene duplication, coleoptera (beetles)
Citation: Sharkey CR, Powell GS and Bybee SM (2021) Opsin Evolution in Flower-Visiting Beetles. Front. Ecol. Evol. 9:676369. doi: 10.3389/fevo.2021.676369
Received: 05 March 2021; Accepted: 14 May 2021;
Published: 14 June 2021.
Edited by:
Casper J. Van Der Kooi, University of Groningen, NetherlandsReviewed by:
Markus Friedrich, Wayne State University, United StatesJair E. Garcia, RMIT University, Australia
Copyright © 2021 Sharkey, Powell and Bybee. This is an open-access article distributed under the terms of the Creative Commons Attribution License (CC BY). The use, distribution or reproduction in other forums is permitted, provided the original author(s) and the copyright owner(s) are credited and that the original publication in this journal is cited, in accordance with accepted academic practice. No use, distribution or reproduction is permitted which does not comply with these terms.
*Correspondence: Camilla R. Sharkey, Y2FtaWxsYS5yLnNoYXJrZXlAZ21haWwuY29t