- 1Burke Museum and Department of Biology, University of Washington, Seattle, WA, United States
- 2Roy M. Huffington Department of Earth Sciences, Southern Methodist University, Dallas, TX, United States
- 3Evolutionary Studies Institute, University of the Witwatersrand, Johannesburg, South Africa
- 4Iziko South African Museum, Cape Town, South Africa
A new burnetiamorph therapsid, Isengops luangwensis, gen. et sp. nov., is described on the basis of a partial skull from the upper Madumabisa Mudstone Formation of the Luangwa Basin of northeastern Zambia. Isengops is diagnosed by reduced palatal dentition, a ridge-like palatine-pterygoid boss, a palatal exposure of the jugal that extends far anteriorly, a tall trigonal pyramid-shaped supraorbital boss, and a recess along the dorsal margin of the lateral temporal fenestra. The upper Madumabisa Mudstone Formation was deposited in a rift basin with lithofacies characterized by unchannelized flow, periods of subaerial desiccation and non-deposition, and pedogenesis, and can be biostratigraphically tied to the upper Cistecephalus Assemblage Zone of South Africa, suggesting a Wuchiapingian age. Isengops is the second burnetiamorph recognized from Zambia and is part of a tetrapod assemblage remarkably similar to others across southern Pangea during the Wuchiapingian. A revised cladistic analysis of Biarmosuchia yielded over 500 most parsimonious trees that generally reaffirm the results of previous analyses for burnetiamorphs: Lemurosaurus is basal, Lobalopex and Isengops are proximate burnetiid outgroups, and Bullacephalus, Burnetia, Mobaceras, Niuksenitia, and Pachydectes are burnetiines. Furthermore, Russian biarmosuchians are scattered throughout the tree and do not form sister taxon relationships with each other. Burnetiamorphs display a wide disparity of cranial adornments and are relatively speciose (13 species), especially when compared to the number of specimens discovered to date (∼16 specimens). As has been suggested in some other tetrapod clades (e.g., ceratopsian dinosaurs), the burnetiamorph fossil record supports an inferred macroevolutionary relationship between cranial adornment and increased speciation rate.
Introduction
Among therapsids, biarmosuchians are known to be rare members of the assemblages in which they occur (Hopson, 1991; Sidor and Rubidge, 2006; Sidor and Smith, 2007; Sidor, 2015; Day et al., 2018). For example, in a review of the fossil record of the Beaufort Group of South Africa, Smith et al. (2012) noted that over 9,600 Permian fossils have been collected and identified, but Sidor (2015) documented only 22 biarmosuchian specimens in his comprehensive assessment of the same beds. Kammerer (2016) recently published on two additional specimens that were previously unreported collections and Day et al. (2016) described the first burnetiamorph from the Pristerognathus Assemblage Zone (now the Lycosuchus–Eunotosaurus subzone of the Endothiodon Assemblage Zone; Day and Smith, 2020), but it remains the case that the vast majority of biarmosuchian genera are known from only one or two specimens (Sidor, 2015). By comparison, there are at least seven Permian genera of other groups of therapsids that are known from at least a hundred specimens (e.g., Cistecephalus, Diictodon, Oudenodon; Smith et al., 2012), although all of these are herbivores. Interestingly, the biarmosuchian subclade Burnetiamorpha is known from only 16 specimens but was remarkably taxonomically diverse, with 13 species recognized (Table 1; see also Sidor, 2015). Indeed, Burnetiamorpha was among the first tetrapod clades to develop bony horn-like processes and crests, which in other extinct tetrapod lineages have been considered characteristics enhancing species recognition or mate competition (e.g., ceratopsian dinosaurs; Sampson, 1999; Padian and Horner, 2011).
Until relatively recently, African representatives of the Burnetiamorpha have been restricted to the Karoo Basin of South Africa (Broom, 1923; Boonstra, 1934; Rubidge and Sidor, 2002; Rubidge and Kitching, 2003; Sidor and Welman, 2003; Sidor et al., 2004; Rubidge et al., 2006; Smith et al., 2006; Sidor and Smith, 2007; Day et al., 2016, 2018; Kammerer, 2016). The first African burnetiamorph from outside of the Karoo Basin was discovered in the upper Permian Chiweta Beds of Malawi and was mentioned by Jacobs et al. (2005), although it went unnamed until the work of Kruger et al. (2015). Sidor et al. (2010) reported the first burnetiamorph material from the upper Permian of Tanzania, but did not name the single isolated skullcap. Likewise, Sidor et al. (2014) mentioned the presence of burnetiamorph fossils from the lower Madumabisa Mudstone Formation in southern Zambia, which they considered Guadalupian in age based on the co-occurrence of tapinocephalid dinocephalians (see Day et al., 2015), with one species recently described as Mobaceras zambeziense by Kammerer and Sidor (2021). From the same beds, Whitney and Sidor (2016) described Wantulignathus gwembensis as a probable burnetiamorph, although the type material allowed referral only to Biarmosuchia indet.
Here, we describe a new burnetiamorph species from the Luangwa Basin of Zambia, which represents the first occurrence of the clade in that basin. In addition, we compare the relationship between the diversity and abundance of burnetiamorphs to other clades of Permian therapsid carnivores as a preliminary test of the hypothesis that conspicuous cranial adornments are associated with enhanced rates of speciation (Vrba, 1984; Sampson, 1999).
Materials and Methods
Institution Abbreviations
BP, Evolutionary Studies Institute, University of the Witwatersrand, Johannesburg; CGS, Council for Geosciences, Pretoria; MAL, Malawi Department of Antiquities Collection, Lilongwe and Nguludi; NHCC, National Heritage Conservation Commission, Lusaka; NHMUK, Natural History Museum, London; NMQR, National Museum, Bloemfontein; NMT, National Museum of Tanzania, Dar es Salaam; PIN, Paleontological Institute, Moscow; SAM, Iziko South African Museum, Cape Town; UMZC, Cambridge University Museum of Zoology.
Geology
GPS coordinates of the start and end points of two overlapping measured sections of the Madumabisa Mudstone strata that are depicted Figure 1 are available from NJT on request. The Madumabisa stratigraphic section presented here was measured and described following standardized field geology methods (e.g., Compton, 1985). Beds were delineated by recognizable differences in color, composition, sedimentary grain size, sedimentary structure, sedimentary architecture, and were measured using a 150 cm high Jacob’s staff mounted with a topographic Abney level while paying particular attention to the upper contacts between beds as descriptions were made upward through the stratigraphic section. Attempts were made to provide a complete stratigraphic section of the rocks, but some areas were under grasses, modern sediments, and soils which were too extensive to remove and were marked as “covered section” and are represented by “X” within the schematic diagram of the measured section (Figure 1). The stratigraphic section in Figure 1 represents an approximation of the dominant sedimentary grain size, color, sedimentary structure and sedimentary architecture as well and the contact relationship of bedding contacts observed amongst the strata in this section.
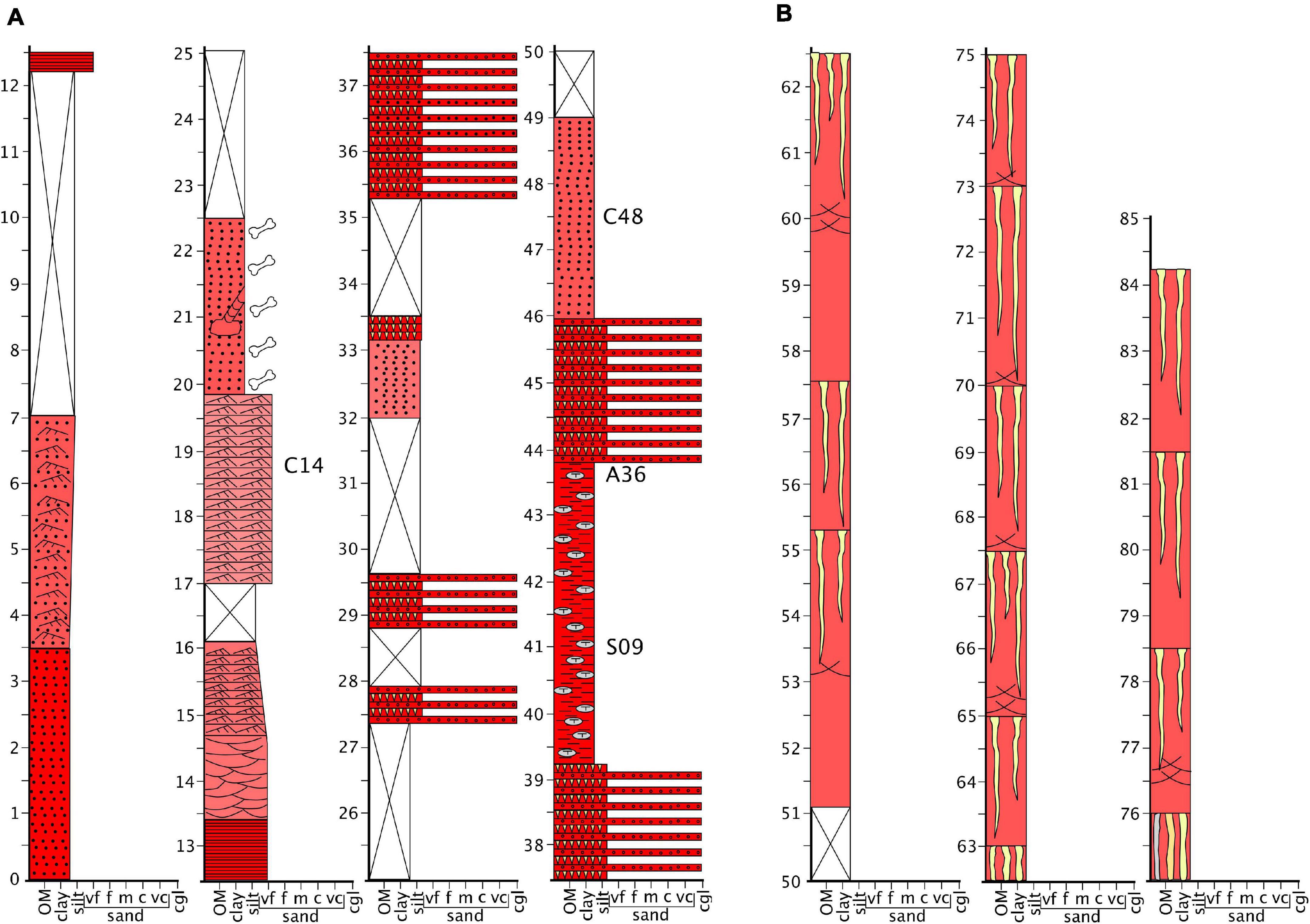
Figure 1. Representative stratigraphic section of the upper Madumabisa Mudstone Formation, Luangwa Basin. This section was taken near the southern boundary of North Luangwa National Park, in the vicinity of several vertebrate fossil localities (marked A36, C14, C48, S09 on section). (A) Lithofacies association 1 and (B) lithofacies association 2. The burnetiamorph skull described here was found away from the measured section, but likely within the lithofacies association 1 and probably very close to the transition between the two lithofacies. c, coarse; cgl, conglomerate; f, fine; m, medium; OM, organic matter; vc, very coarse; vf, very fine.
Fossil Preparation
The fossil described here was collected in 2014 by S. Nesbitt, as part of a long-term project to understand the geology and paleontology of the Permian and Triassic rocks of Zambia (see Sidor and Nesbitt, 2018). It was prepared at the University of Washington Burke Museum by G. Livingston and B. Crowley using airscribes and pin vices.
Results
Geological Context
Geological research on the Madumabisa Mudstone Formation of the Luangwa Basin has concentrated on rocks exposed in its northern part (Dixey, 1937; Drysdall and Kitching, 1962, 1963; Utting, 1978), with very little work focusing on the middle portion of the valley (Kemp, 1975; Banks et al., 1995). It is generally accepted that the formation can be subdivided into lower and upper members, with tetrapod fossils restricted to the upper member (Kitching, 1963; Drysdall and Kitching, 1963). Angielczyk et al. (2014) suggested that the vertebrate fossils collected from the northern and middle parts were best viewed as constituting a single assemblage and suggested correlation to the Cistecephalus Assemblage Zone (Wuchiapingian) of South Africa, however, more recent work by Peecook et al. (2020) suggests that two assemblages may be present and that the upper assemblage bears resemblance to the Dicynodon-Theriognathus Subzone of the Daptocephalus Assemblage Zone of South Africa (sensu Viglietti, 2020). In the Mid-Zambezi Basin of southern Zambia, Sidor et al. (2014) reported tetrapod fossils from the lower member of the Madumabisa Mudstone Formation and proposed a Guadalupian age, but corresponding fossils from the Luangwa Basin have yet to be discovered.
Stratigraphy and Sedimentology
Surface exposures of the upper Madumabisa Formation in North Luangwa National Park are limited to a series of disconnected low cliff sections along the eroded banks of ephemeral stream channels. We measured an approximately 85-meter composite stratigraphic section in which two lithofacies were recognized (Figure 1). The sedimentary strata strike N40°E and dip west between 2–5 degrees.
Lithofacies association 1
This association occurs from the base of the section up to ∼50 meters and is composed of intercalated mudstones, sandstones, and conglomerates (Figure 1A). Mudstones predominate and occur as dull reddish-brown (Munsell color ∼5YR 5/3), weakly-bedded to relict-laminated layers or as massive to blocky layers. These can occur with and without carbonate nodules and tubules and can range from a few centimeters to several meters thick. Many mudstone layers also include coarse silt to fine sand-filled desiccation cracks extending vertically into the mudstone and tapering downward as much as 10 centimeters. Sandstone beds occur as dull reddish-brown layers ranging from very-fine to fine-grained and range from <10 centimeters to slightly more than two-meters thick. Thinner beds of sandstone are typically ripple cross-laminated and commonly intercalated with thin mudstone beds (e.g., between 3.5 and 7 meters in Figure 1) or thin beds of carbonate-nodule conglomerates (e.g., between 35.5 and 39 meters, between 44 and 46 meters in Figure 1). Thicker beds and successions of sandstone exhibit general upward-fining textures with upward changes in sedimentary structures from planar bedding to trough-cross-bedding, into ripple-cross lamination (e.g., between 12 and 16 meters in Figure 1). Conglomerates occur only as thin beds of carbonate nodules similar morphologically to nodules found in the underlying blocky mudstone. They are typically clast-supported and have a fine muddy sandstone matrix. Relict-laminated muds tend to have the greatest concentrations of fossil vertebrate material and even preserve some large diameter burrows (∼30 cm wide), that are possibly vertebrate dwelling structures (e.g., between 20 and 22.5 meters in Figure 1). However, bone fragments tend also to be common to abundant in carbonate-nodule conglomerate layers.
Lithofacies association 2
This occurs from ∼50 to 85 meters in the stratigraphic section and is composed of dull reddish-brown (Munsell color ∼5YR 5/3) massive mudstones ranging from ∼2 meters (e.g., between 55.5 and 57.5 meters in Figure 1B) to ∼5 meters (e.g., between 57.5 and 63 meters in Figure 1B) in thickness. The upper surface of each bed is characterized by an abrupt and smooth-to-wavy contact with the overlying bed. Numerous vertically oriented, yellow-brown (Munsell color ∼2.5 YR 7/6) sandstone-filled polygonal desiccation cracks up to 10 cm wide taper downward from these surfaces up to two meters in the underlying strata. The lower parts of many of these mudstone beds display zones of slickensides up to 0.5 meters thick. Slickenplanes are long (∼1 meter), arcuate, and often occur as crosscutting co-sets in vertical exposures. In beds where sand-filled desiccation cracks and slickenplanes coexist, they are typically exclusive of each other in a vertical sense (e.g., between 53 and 55, 60 and 63, 65 and 67.5, 76 and 78.5 meters in Figure 1). To date, no vertebrate fossils have been recovered from lithofacies association 2.
Paleoenvironmental interpretation
The upward-fining sedimentary cycles in lithofacies association 1 are indicative of decreasing and shallowing unidirectional currents associated with fluvial systems. Plane-bed and trough-cross bedded sandstones represent the highest-velocity and/or deepest flows, whereas ripple cross-laminated sandstones, mixed ripple-cross-laminated sandstones and mudstones, and laminated mudstones represent decreasing flow depths, waning velocities, and in some instances slack-water conditions. Finally, massive and blocky mudstones with and without calcareous nodules are bioturbated layers that spent extended periods of time as hiatus surfaces within the vadose zone; they were soils and now are paleosols. Distinct channel structures, such as broad, lenticular sandstones with erosive bases, were not identified in the North Luangwa National Park stratigraphic section. Yet, the sedimentary structures in lithofacies association 1 appear closely matched to flood-type deposits in fluvial floodplains proximal to channel belt systems. In particular, the highest-velocity and deepest flow structures, and their upward shallowing/decreasing flow patterns are similar to those described in modern fluvial crevasse-splay deposits, and massive to blocky mudstones represent more distal positions and longer durations of sedimentary quiescence upon the floodplains.
Lithofacies association 2 is peculiar in that it is a monotonous sequence of bioturbated and pedoturbated (i.e., shrink-swell) mudrocks with no preserved evidence for flow. Yet, sand-sized particles clearly were transported into and through these systems because sandstone now fills the desiccation features within the mudstones, forming clastic dikes (Figure 1). This necessitated relatively high energy-flows to have brought sands into these parts of the depositional basin. However, it cannot be determined from the preserved sedimentary structures if these sandstone and mudstone elements in lithofacies association 2 represent products of aqueous or eolian transport. Nevertheless, blocky to massive mudstones with clastic dikes and slickensides all are common features associated with soil profiles with a high concentration of fine clay-sized particles in climates characterized by intense seasonal precipitation. Each bed in lithofacies association 2 is interpreted to have been a soil profile similar to modern Vertisols. The physiographic placement of lithofacies association 2 also is difficult to assess. We favor a very distal position upon a fluvial floodplain or possibly, a major facies shift from fluvial deposition in lithofacies 1 to lake-plain depositional systems. However, lithofacies association 2 also could represent eolian depositional systems, playa-type depositional systems, or even loess plateau-type depositional systems similar to those described from the Plio-Pleistocene of the south-central United States (e.g., Gustavson and Winkler, 1988).
Summary and comparisons
Within the mudrock-dominated basin fills associated with the East African rift system, the common association of vertebrate fossils with horizons of large smooth-surfaced calcareous nodules has been interpreted as the result of significant lowering of lake level (Yemane and Kelts, 1990; Smith, 2000; Catuneanu et al., 2005). In this view, the shrinking axial lake promotes an increased density of bones accumulating along its migrating shoreline. Bone burial is completed with the return of higher lake levels, and bone early diagenesis is promoted by alkaline groundwaters that preferentially precipitate micrite around the buried bones (Calvo et al., 1989).
The upper Madumbisa Mudstone vertebrate fauna in Zambia has been tentatively correlated with the Cistecephalus Assemblage Zone of the main Karoo Basin of South Africa (Angielczyk et al., 2014). Comparison of the host rocks suggests a different paleoenvironmental setting for the bone occurrences between these two basins. Superficially, the silt-dominated mudrocks are similar in color and both host calcareous nodules. However, the main difference is that, in the main Karoo Basin, well-confined channel sandstones are common and are clustered into sets of mappable lithostratigraphic units named the Oukloof Member in the west and the Oudeberg Member in the east. These members are interpreted as large, low-gradient fluvial distributary systems dominated by low sinuosity channels and seasonally dry floodplains (Viglietti et al., 2016). We have yet to recognize such stratigraphically distinctive sandstone units in the Madumabisa Mudstone sequence in North Luangwa National Park.
Systematic Paleontology
THERAPSIDA Broom (1905)
BIARMOSUCHIA Sigogneau-Russell (1989)
BURNETIAMORPHA Broom (1923)
Isengops luangwensis gen. et sp. nov.
Etymology – The generic name alludes to the tall, triangular supraorbital bosses by combining the local Bemba word for horn (isengo) with the Greek suffix commonly used for face (ops). The species name combines the geographic area of origin (Luangwa river valley) with “ensis,” which is Latin for “from” or “belonging to.”
Holotype – NHCC LB363, an isolated skull lacking most of the occiput, both zygomatic arches, the left temporal region, and the tip of the snout, as well as missing the lower jaws entirely.
Locality and Horizon – The holotype was surface collected at L322, one of a number of closely spaced localities in the upper Madumabisa Mudstone Formation near the southern border of North Luangwa National Park (Muchinga Province, Zambia; see also Sidor, 2015; Huttenlocker and Sidor, 2020). Based on the local tetrapod fauna, this portion of the formation can be biostratigraphically correlated with the Cistecephalus Assemblage Zone (AZ) of South Africa, and is therefore Wuchiapingian (late Permian) in age (Rubidge et al., 2013; Angielczyk et al., 2014; Smith et al., 2020). Detailed locality information is available to qualified researchers at the NHCC or by contacting CAS.
Diagnosis – Palatal dentition extremely reduced; palatine and pterygoid bosses ridge-like and oriented nearly anteroposteriorly; pterygoid midline suture absent; palatal exposure of jugal extends far anteriorly between maxilla and ectopterygoid; tall supraorbital boss (over 50% of orbit height), which is triangular in lateral view and has recessed area on its lateral surface; posterodorsal margin of lateral temporal fenestra with medially recessed shelf. Isengops can be distinguished from other burnetiamorphs by a taller nasal ridge than in Lemurosaurus (but one that is similarly unpachyostosed) and a supratemporal “horn” more robust than in Lobalopex, but not extremely pachyostosed as in Bullacephalus or Burnetia.
Description
Skull Roof
Septomaxilla – Most of the anterior portion of the skull was lost prior to discovery, but a small section of the facial process of the septomaxilla is preserved the right side (Figures 2A,B). The lateral surface of the septomaxilla is flat and featureless. It is preserved in the same plane as the maxilla, as both have been slightly dislodged along their common sutural contact with the nasal. As in several other biarmosuchians and other basal therapsids, the septomaxilla is relatively long, extending to a level posterior to that of the upper canine (Sidor, 2003).
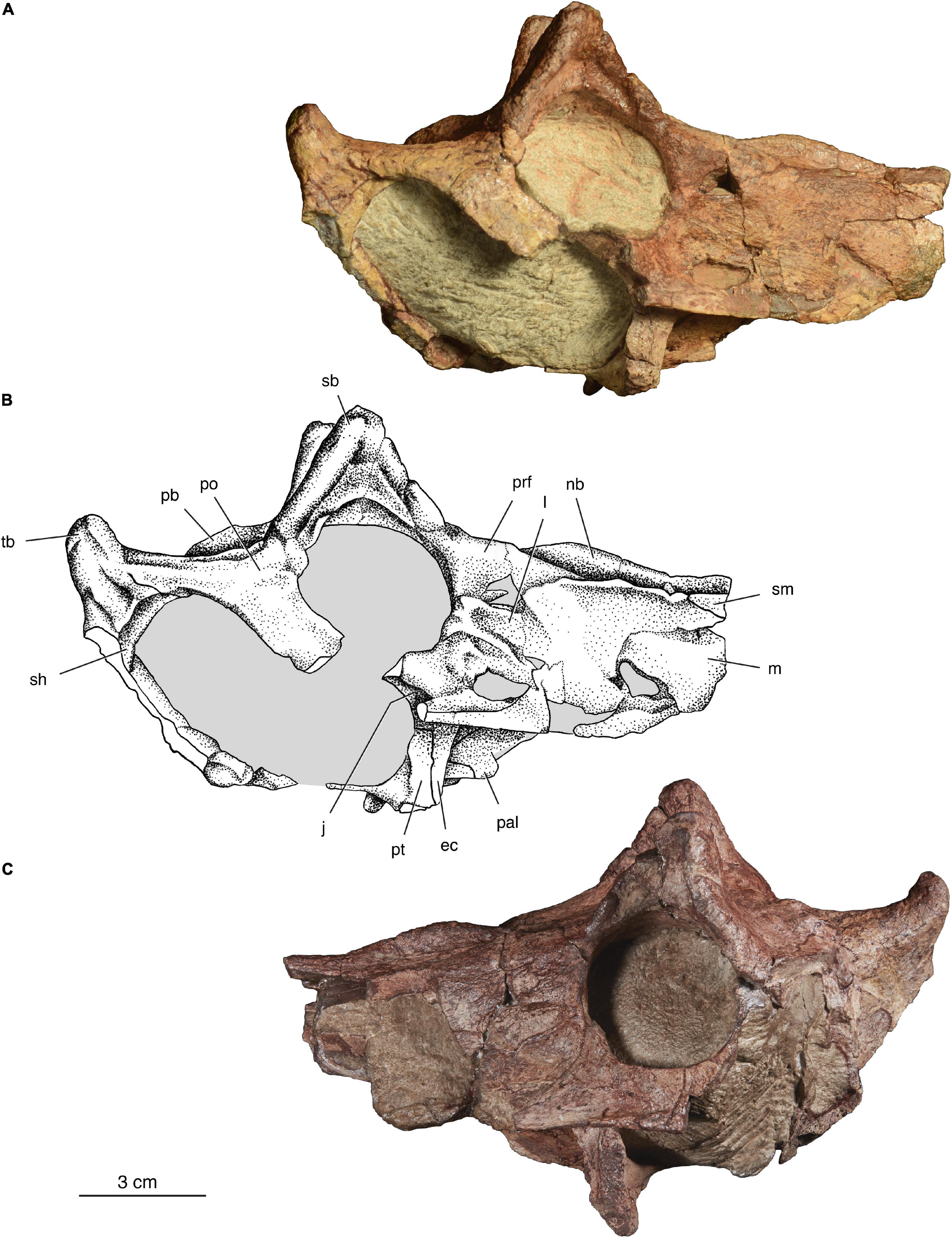
Figure 2. The holotype of Isengops luangwensis gen. et sp. nov. (NHCC LB363). Skull in right lateral (A,B) and left lateral (C) views. ec, ectopterygoid; j, jugal; m, maxilla; nb, median nasal boss, pal, palatine; pb, parietal boss; po, postorbital; prf, prefrontal; pt, pterygoid; sb, supraorbital boss; sh, shelf on lateral surface of temporal fenestra margin; sm, septomaxilla; tb, supratemporal “horn.”
Maxilla – This element is more completely preserved on the right side and its surface is in good condition, although a small opening is present anteroventrally, exposing internal matrix. In addition, two other openings are present in the vicinity of the prefrontal and lacrimal on the right side, but these openings are absent on the left side, suggesting they are artifactual. Such openings occur in many other burnetiamorph specimens and have been interpreted as fossae (e.g., Sidor and Welman, 2003). The lateral surface of the maxilla is textured, but it is not as rugose as in Bullacephalus or Pachydectes (Rubidge and Kitching, 2003; Rubidge et al., 2006). The right maxilla measures 33 mm in maximum height, which gives the snout of Isengops a lower and more gracile appearance than in other burnetiamorphs. Transverse compression of the skull has separated the maxilla along its dorsal contact with the nasal, but the corresponding contact with the prefrontal is more difficult to discern. Anterior to the orbit, the maxilla’s suture with the lacrimal and jugal is similarly difficult to discern, but a long posterior ramus of the maxilla is visible ventrally and forms part of the suborbital bar.
The ventral margin of the maxilla forms a shallow curve in lateral view, although anteriorly it becomes more horizontal just behind the position of the upper canine. Based on the geometry of the preserved root, the upper canine likely projected anteroventrally, as in Lemurosaurus and Lobalopex (Sidor and Welman, 2003; Sidor et al., 2004). Following a short post-canine diastema, the roots of seven small postcanine teeth are present in the right maxilla. In ventral view, the maxilla contacts the palatine, ectopterygoid, and jugal.
Nasal – The dorsal surface of the nasal is dominated by a median ridge or crest (Figure 3), which is similar in its degree of the development to that of Lobalopex or Lophorhinus (Sidor and Welman, 2003; Sidor et al., 2004) or the unnamed species from the Endothiodon Assemblage Zone (Day et al., 2016), although in the latter the ridge is more robust posteriorly. Importantly, the nasal crest is not transversely expanded as in Burnetia or Bullacephalus or pachyostosed as in Paraburnetia. In lateral view, the nasal crest is parabolic in outline, diminishing in height both anteriorly and posteriorly. The posterior margin of the nasal is difficult to determine, but based on the condition in other burnetiamorphs where sutures are visible, the nasal likely contributed to anterior portion of the frontal boss. The nasal crest is continuous with the median frontal ridge in Isengops, although the latter is very subdued in its expression, especially compared to what is seen in Lende, Leucocephalus, Paraburnetia, or Proburnetia.
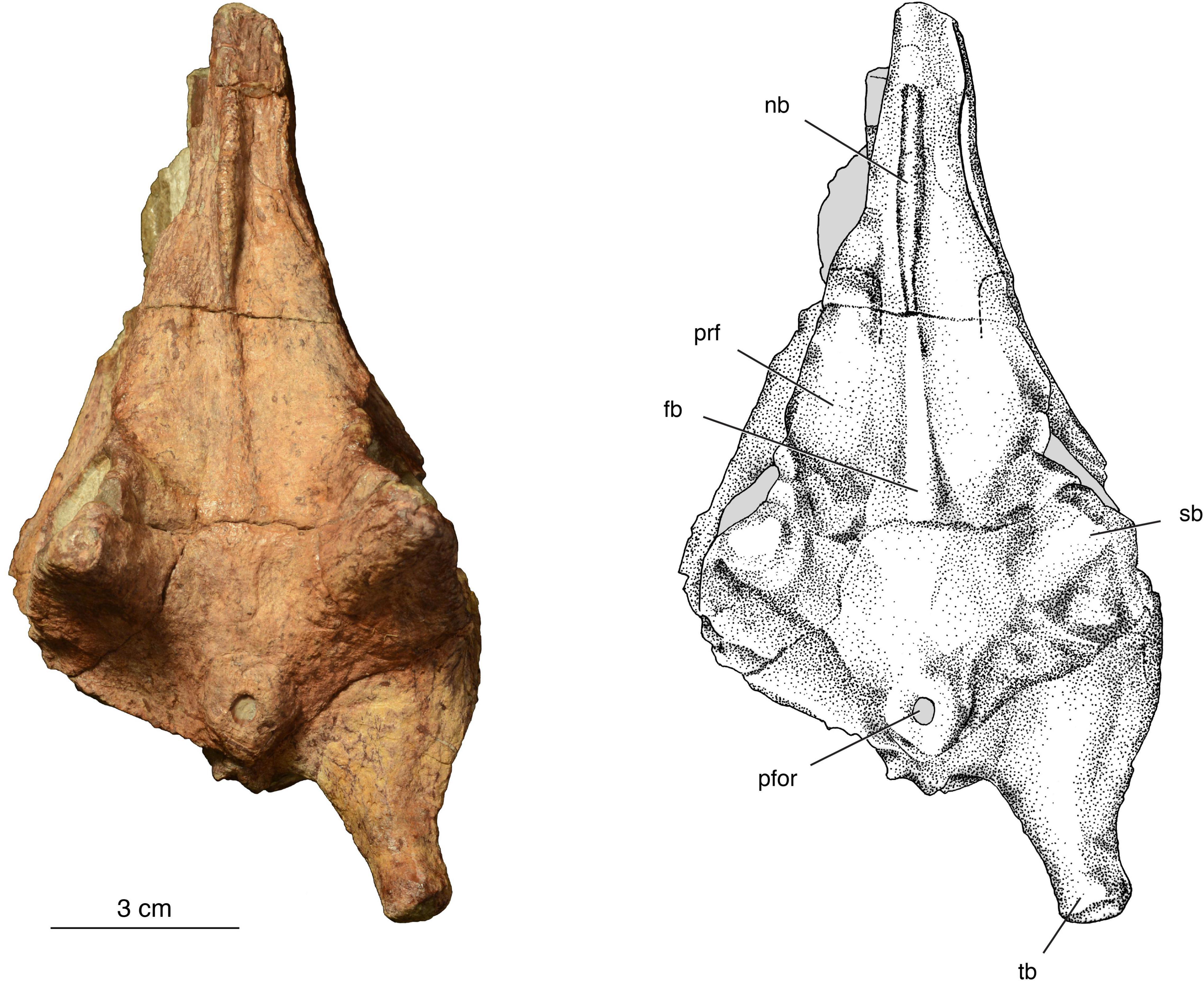
Figure 3. The holotype of Isengops luangwensis gen. et sp. nov. (NHCC LB363) in dorsal view. fb, median frontal boss; nb, median nasal boss, pfor, parietal foramen; prf, prefrontal; sb, supraorbital boss; tb, supratemporal “horn.”
Prefrontal – The sutures delimiting the prefrontal are difficult to make out, but this element most likely conforms to the anatomy reported by Sidor and Smith (2007) for Lophorhinus. The prefrontal contacts the nasal and frontal medially. Anteroventrally, it contacts the maxilla. On the left side, the suture between the prefrontal and lacrimal follows a raised area. The degree to which the prefrontal contributes to the supraorbital boss is unknown, but we suspect that it formed the anterior one-quarter of this feature.
Lacrimal – The lateral surface of the lacrimal is bounded by two subhorizontal ridges that radiate anterodorsally and anteroventrally, along the sutures with the prefrontal and jugal, respectively (Figure 2). Between these ridges, the lacrimal forms a mild depression. Anteriorly, the lacrimal contacts the maxilla, dorsally it contacts the prefrontal, and ventrally it contacts the jugal. Two lacrimal foramina are present on the anterior border of the orbit, as reported for Lophorhinus and Proburnetia (Rubidge and Sidor, 2001; Sidor and Smith, 2007).
Jugal – The jugal is more complete on the left side (Figure 2C), which preserves a nearly complete suborbital bar. On the right side, this bar is broken and allows for the suture between the jugal and underlying maxilla to be seen in cross-section (Figures 2A,B). In ventral view (Figure 4), the jugal extends remarkably far anteriorly, wedging itself between the ectopterygoid and maxilla. This configuration has not been reported previously for a burnetiamorph, and is considered an autapomorphy of Isengops. The contact between the jugal and postorbital can be seen only in ventral view, on the lateral margin of the subtemporal fenestra.
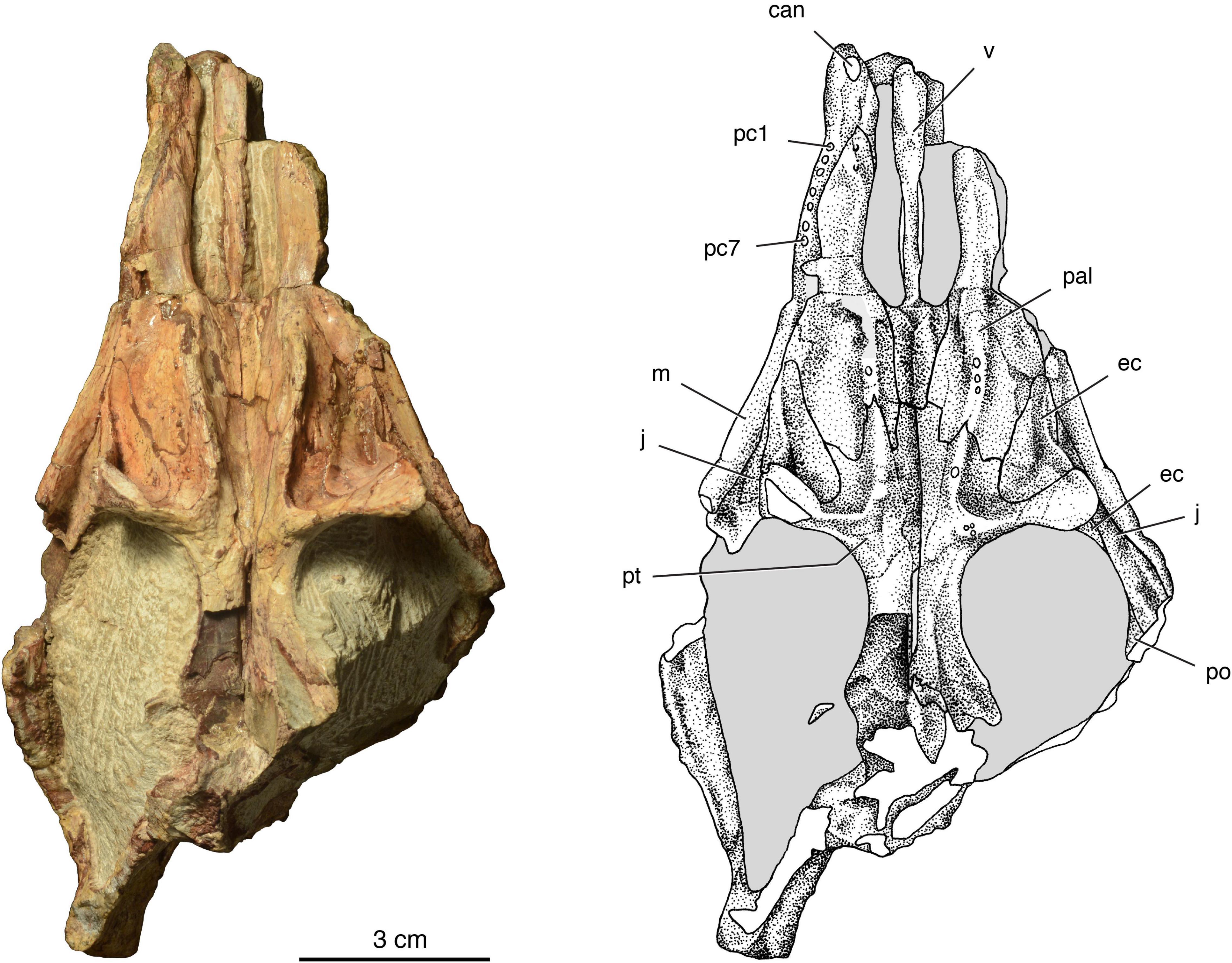
Figure 4. The holotype of Isengops luangwensis gen. et sp. nov. (NHCC LB363) in ventral view. 1, 7, tooth position number; can, upper canine; ec, ectopterygoid; j, jugal; m, maxilla; pal, palatine; pc, postcanine tooth; po, postorbital; pt, pterygoid; v, vomer.
Postorbital Bar – Based on the condition recorded for most other Permian therapsids, the postorbital bar was likely formed by the jugal and postorbital. Unfortunately, the limits of neither element can be identified on the lateral surface of the bar (Figure 2C). Compared to other burnetiamorphs, the postorbital bar is not pachyostosed and is much longer anteroposteriorly than thick mediolaterally. In contrast to the condition in burnetiines like Bullacephalus and Burnetia, Isengops shows no indication of a distinct thickening at the dorsal part of the postorbital bar, near its confluence with the skull roof. A small section of suture between the postorbital and squamosal appears above the lateral temporal fenestra on the right side.
Skull Table – The elements of the skull table are co-ossified and mildly pachyostotic. In posterior view, a cross-section of the dermal skull roof is visible along the broken surface between the parietal foramen and base of the left supraorbital boss (Figure 5). Two layers are apparent: a deeper, compact layer with trabecular structure and a more superficial layer that lacks clear internal organization. At a macroscopic level, this two-layered anatomy corresponds to what Kulik and Sidor (2019) described as zone B and zone C + D in thin-section. The degree of vascular pachyostosis in this region of the skull roof of Isengops is much reduced compared to what Kulik and Sidor (2019) described in the corresponding region of an unidentified burnetiamorph skull cap.
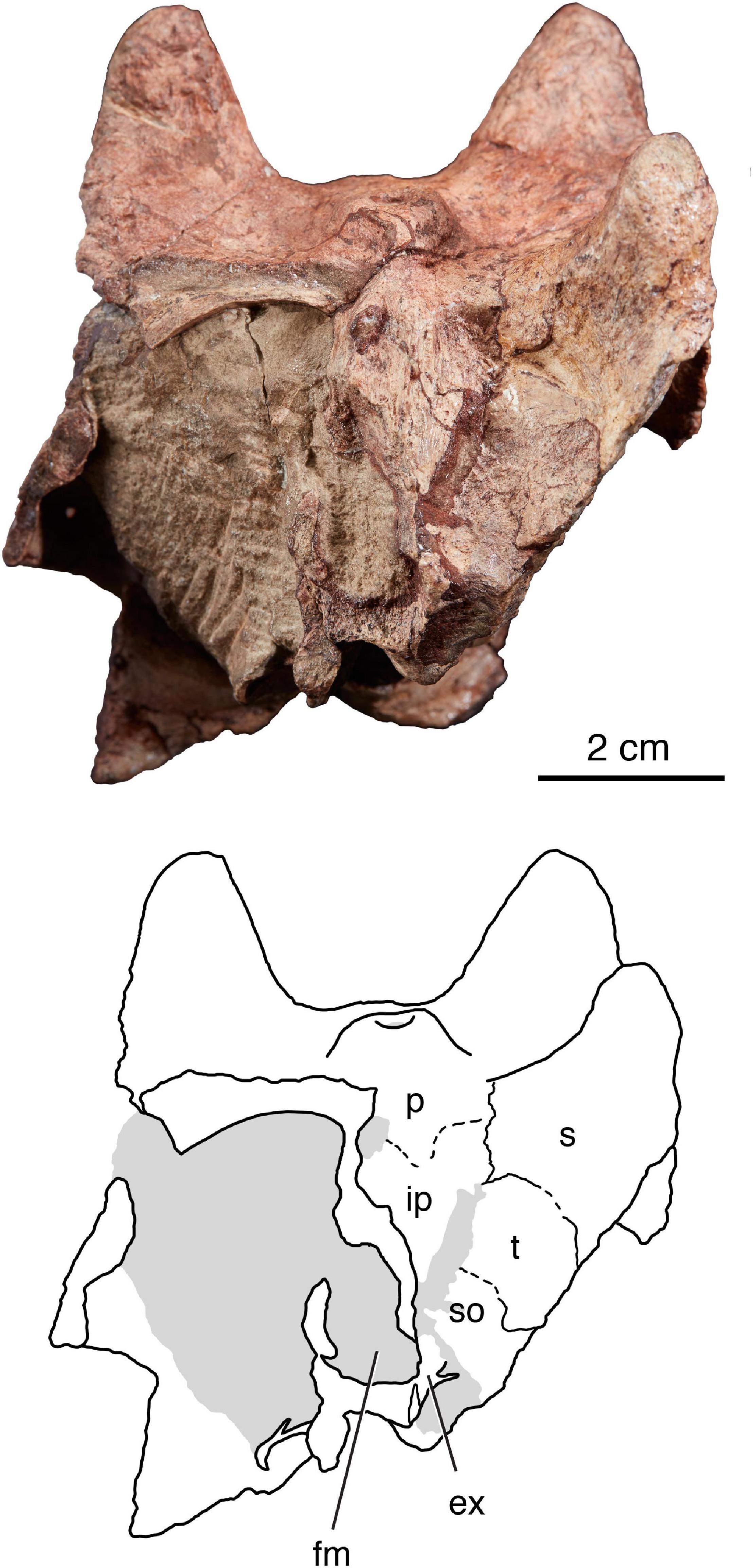
Figure 5. The holotype of Isengops luangwensis gen. et sp. nov. (NHCC LB363) in occipital view. ex, possible exoccipital; fm, foramen magnum; ip, postparietal; p, parietal; s, squamosal; so, supraoccipital; t, tabular.
Above each orbit, the most striking feature of the skull table is a tall supraorbital boss. It resembles a trigonal pyramid in geometry, with flat faces directed posteriorly and medially that converge at the apex. The remaining face is directed anterolaterally and is situated above the orbit. This configuration is most similar to that seen in Lemurosaurus (Sidor and Welman, 2003). In Isengops, however, ridges are present along the anterior and posterior edge of this face, and the apex is thickened to overhang a shallow fossa positioned just above the orbit. This anatomy is unlike that seen in any other burnetiamorph, with the possible exception of the left supraorbital boss in Lende, but the description of Kruger et al. (2015) does not make it clear if this feature is natural (because it is lacking on the other side). The supraorbital boss in Isengops is proportionately taller than in any other burnetiamorph, being over 50% of the height of the orbit.
The anatomy of the region surrounding the parietal foramen is also unique among burnetiamorphs. Anterior to the foramen, the pineal boss blends almost seamlessly into the skull roof. Laterally and posteriorly, however, the parietal foramen is raised above the surrounding elements, such that a shallow fossa is formed between the pineal boss, the supraorbital boss, and the base of the supratemporal horn. Importantly, the anatomy in Isengops does not conform to what Kammerer (2016) regarded as a dome-like anatomy, where the parietal boss and supraorbital boss become confluent. Although the occipital border is incompletely preserved, the parietal foramen was situated relatively posteriorly, which is the plesiomorphic condition within burnetiamorphs (Smith et al., 2006).
The right supratemporal horn is well preserved and is directed posteriorly, dorsally, and slightly laterally (Figures 2, 3, 5). A supratemporal horn is present in all burnetiamorphs where this region is preserved, except for Lemurosaurus, although it takes a variety of forms. For example, in Bullacephalus, Burnetia, and Niuksenitia the entire posterior margin of the squamosal is thickened so that the horn is incorporated into the larger pachyostosis. In Isengops, the supratemporal horn is particularly well defined and upturned. In lateral view, the dorsal margin of the temporal fenestra shows a recess under supratemporal boss, which is seen also on the right side in Lende (Kruger et al., 2015: Figure 1C), but is absent in all other described burnetiamorphs.
Palate
Vomer – In ventral view (Figure 4), the choanal portion of the unpaired vomer has complex shape, narrowing slightly from anterior to posterior. Anteriorly, this region is flat to convex anteriorly, but is ventrally concave surface near its mid-length, as several other biarmosuchians (Sidor, 2003; Sidor and Smith, 2007). This troughed appearance is the product of the vomer having downturned lateral edges in the region of the choana, but these edges are weathered so the degree to which they curled together towards the midline is unknown. From what is preserved, the downturned edges of the vomer fail to coalesce on the midline posteriorly. Anteriorly, the connection between the vomer and premaxilla is not preserved.
The post-choanal portion of vomer is trapezoidal in ventral view and has a deep crack on its midline, making the recognition of a suture (or lack thereof) difficult to determine in this area. Posteriorly, the vomer contacts the palatine and pterygoid. As is characteristic for therapsids, the choana is relatively long and extends further posteriorly than the maxillary tooth row (Sidor, 2003).
Palatine – This element is better preserved on the right side and conforms to the general outline seen in most biarmosuchians (Sidor et al., 2004; Sidor and Smith, 2007). The palatine extends anteriorly to just behind the maxillary swelling that houses the upper canine. It forms the lateral margin of the choana posteriorly until it contacts the palatal portion of the vomer. The body of the palatine is deeply V-shaped in frontal section, with the apex formed by an anteriorly and slightly laterally oriented palatal ridge. The palatine ridge is more complete on the left side, where it preserves the roots of three small teeth. Uniquely among burnetiamorphs, the palatine teeth form a single row and the boss into which they implant is nearly straight and does not form a semicircular or triangular platform anteriorly. Laterally, the palatine contacts the maxilla and ectopterygoid, whereas most of its posterior border is with the pterygoid.
Ectopterygoid – The excellent preservation of the palate of NHCC LB363, combined with its lack of a lower jaw, has yielded new information about the anatomical relationships of the ectopterygoid in a burnetiamorph. In ventral view, the element has the outline of a right triangle, with the hypotenuse directly obliquely across the palate (Figure 4). A delicate vertical lamina is present and slightly inset from its lateral margin, connecting the palatal portion of the element to the lateral part of the transverse flange of the pterygoid. Lateral to the transverse flange of the pterygoid, a longitudinal trough for the adducted mandible is formed by the maxilla laterally, the jugal dorsally, and the ectopterygoid and pterygoid medially. In lateral view (Figure 2), the lack of a lower jaw affords a view of the ectopterygoid descending on the lateral surface of pterygoid wing, forming its anterior face.
Pterygoid – The pterygoid is more completely preserved on the left side. Anteriorly, the pterygoid forms a complex suture with the vomer and palatine. Surprisingly, there is no evidence for a midline suture connecting the pterygoids, so we must assume they are fused. As with the palatine, the pterygoid palatal ridge is directed anterolaterally and is remarkably narrow, housing a reduced complement of teeth (only one is visible on the left side, none on the right). The palatal ridge of the pterygoid is continuous with the transverse flange, which also occurs in Lobalopex (Sidor et al., 2004: Figure 3), although the frequency with which this connection has been accidentally damaged in other burnetiamorphs is uncertain. A cluster of three extremely small tooth roots is present near the medial extent of the transverse flange of the pterygoid on the left side, but corresponding evidence for teeth on the right side is absent. The transverse flange deepens laterally and bears a rugose, presumably cartilage-covered, lateral surface. Posteriorly, the basicranial ramus of the pterygoid is more complete on the left side, but only the anterior-most portion of the pterygoid ramus is preserved. Based on what is preserved, the basicranial ramus likely had a small parasagittal ridge that laterally bounded the interpterygoid vacuity, but this area is incomplete.
Occiput and Braincase
Compared to the palate, the occiput and braincase are poorly preserved in NHCC LB363, with much of the left side of the occiput missing so that matrix filling the temporal fenestra can be in posterior view (Figure 5). The postparietal is present and bears a midline nuchal crest, although the paired descending ridges highlighted by Kammerer (2016) in Paraburnetia and Bullacephalus are not apparent. The outline of the foramen magnum is recognizable and the opening appears shifted to the left of the midline, as most of surrounding bones are damaged on this side and seen in section. Sutures delimiting the medial and ventral extent of the right squamosal are visible in occipital view. In addition, the right tabular is mostly preserved, as is a good portion of the supraoccipital on that side. Ventral to the foramen magnum, we can more tentatively identify the basioccipital and right exoccipital, although for both of these only the internal anatomy of each bone is visible in cross-section.
Discussion
Phylogenetic Position of Isengops
Kammerer and Sidor (2021) recently published a comprehensive review of the characters used in most previous analyses of burnetiamorph phylogenetic relationships. Their paper was, in part, a response the results of Day et al. (2016), who found unorthodox phylogenetic results markedly at contrast to the majority of those since the initial studies of the group (e.g., Sidor, 2000; Sidor and Welman, 2003; Sidor et al., 2004; Kammerer, 2016). Day et al. (2016) were concerned with the lack of concordance between the stratigraphic and phylogenetic positions of burnetiamorph genera such as Bullacephalus and Pachydectes and based their study on the data set of Kammerer (2016), but added 12 characters. Surprisingly, they found currently recognized burnetiamorphs to be paraphyletic in some analyses, with the middle Permian Bullacephalus and Pachydectes forming a clade with Hipposaurus to the exclusion of other burnetiamorph taxa. Kammerer and Sidor (2021) reanalysis of the Day et al. (2016) data set suggested that: (1) some characters could be excluded because they were either parsimony uninformative or strongly tied to ontogenetic status of the specimens under study, (2) some character state attributions were mistaken and were corrected in the revised data matrix, and (3) some characters had to be reformulated in order to better describe and encompass the range of variation seen within the ingroup. After their revisions, the Kammerer and Sidor (2021) resulting data set included 27 characters and 21 operational taxonomic units (all biarmosuchians). In some analyses, three particularly incomplete specimens (BP/1/7098, NHMUK PV R871a, and TM 4305) were removed.
To determine the phylogenetic position of Isengops, we added it to the complete data set of Kammerer and Sidor (2021). Thus, the current analysis includes 27 characters assessed in 22 taxa. The line of character codings for Isengops is provided in Appendix 1. All other aspects of the analysis were identical to those of Kammerer and Sidor (2021), including character ordering and outgroup selection.
When the complete data set was analyzed using the heuristic analysis option of PAUP 4∗ (Swofford, 2002), 18,768 most parsimonious trees were found. When the three particularly incomplete specimens were removed, this number was reduced to 532 most parsimonious trees. The majority-rule consensus cladogram of the more restricted analysis is shown in Figure 6 and generally conforms to the results given by Sidor and Smith (2007) as well as by Kammerer (2016), with several burnetiamorph taxa (e.g., Lemurosaurus, Lobalopex, Lophorhinus) falling outside Burnetiidae, which is itself subdivided into Burnetiinae (Bullacephalus, Burnetia, Mobaceras, Niuksenitia, Pachydectes) and Proburnetiinae (Lende, Leucocephalus, Proburnetia, Paraburnetia). In all trees, Isengops was found to be more derived than Lemurosaurus and Lobalopex, falling just outside of Burnetiidae and it was not particularly closely related to either Mobaceras (the other burnetiamorph known from Zambia) or Lende, which is known from Malawi.
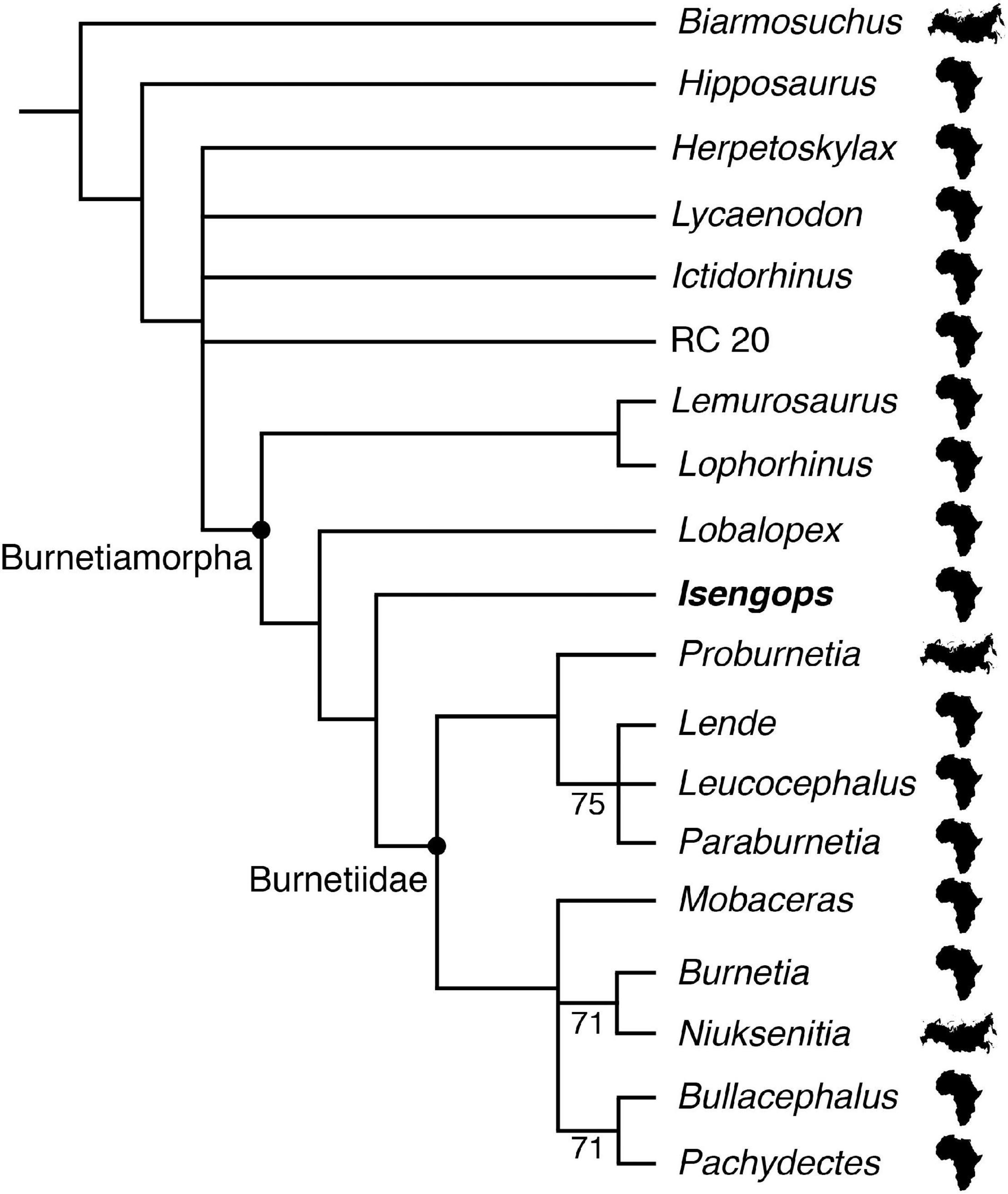
Figure 6. Phylogenetic relationships inferred among burnetiamorph biarmosuchians. The majority-rule consensus of 532 minimum-length trees of 49 steps is shown, with values above branches denoting the percentage of trees showing that clade (when less than 100%). Each tree had a Consistency Index of 0.735 and a Retention Index of 0.882. Of the 19 biarmosuchian taxa included, Biarmosuchus, Niuksenitia and Proburnetia are from Russia (modified Eurasian silhouette) whereas the remaining species from southern Africa (continental African silhouette).
Burnetiamorph Biogeography
Burnetiamorph fossils are known from at least six geologic basins spanning the northern (Russia) to southern regions of Pangea (Malawi, South Africa, Tanzania, and Zambia). In all of the burnetiamorph phylogenies reported to date, the two Russian forms (Niuksenitia and Proburnetia) are not sister taxa, which implies independent dispersal events to northern Pangea (Figure 6). In addition, there is little consistency between stratigraphic appearance and phylogenetic position for taxa included in these phylogenies (see Wagner and Sidor, 2000). Two contrasting interpretations have been offered to explain these patterns. The first, typified by Sidor and Welman (2003), suggests that burnetiamorphs were, as a clade, likely widely distributed across Pangea and paleontologists have succeeded in sampling their diversity only rarely. Sidor and Welman (2003) suggested that the fossil record is not sufficient to answer questions like the area of origin of burnetiamorphs until adequate sampling – or at least equivalent sampling – can be demonstrated for all of the areas under consideration. In the second interpretation, the burnetiamorph cladograms and the stratigraphic position of their constituent taxa are viewed as straightforward guides to the biogeographic history of the group. For example, Rubidge and Kitching (2003:207) suggested that, “[Burnetiamorpha] had its origins in Gondwana” and Kruger et al. (2015:e1008698-8) stated, “what is now southern Africa was a potential area of origin for burnetiamorphs.” Below we analyze abundance data to assess the quality of the burnetiamorph fossil record.
Sidor and Welman (2003) suggested that burnetiamorphs have a poor fossil record, perhaps more so than other therapsids of the same geologic age. In terms of the number of specimens, the burnetiamorph fossil record is summarized in Table 1. These numbers can be readily compared to the numbers of other tetrapod fossils from the Karoo Basin of South Africa provided by Smith et al. (2012). Abundance data for other basins are more difficult to gather, but a preliminary comparison for Tapinocephalus and Cistecephalus-equivalent strata in three other southern Pangean basins is shown in Figure 7. It seems clear that Tanzanian and Zambian rocks have produced substantially fewer fossils than those of equivalent age in Karoo Basin of South Africa (Figure 7A). It is likewise apparent that biarmosuchian specimens, most of which are burnetiamorphs, are much less abundant in the fossil record than most of the other therapsid subclades (Figure 7B), including their nearest ecological equivalents, the gorgonopsians and therocephalians. Based on these data, Sidor and Welman’s (2003) worries that the burnetiamorph record might be insufficient to fruitfully assess biogeographic history seem justified.
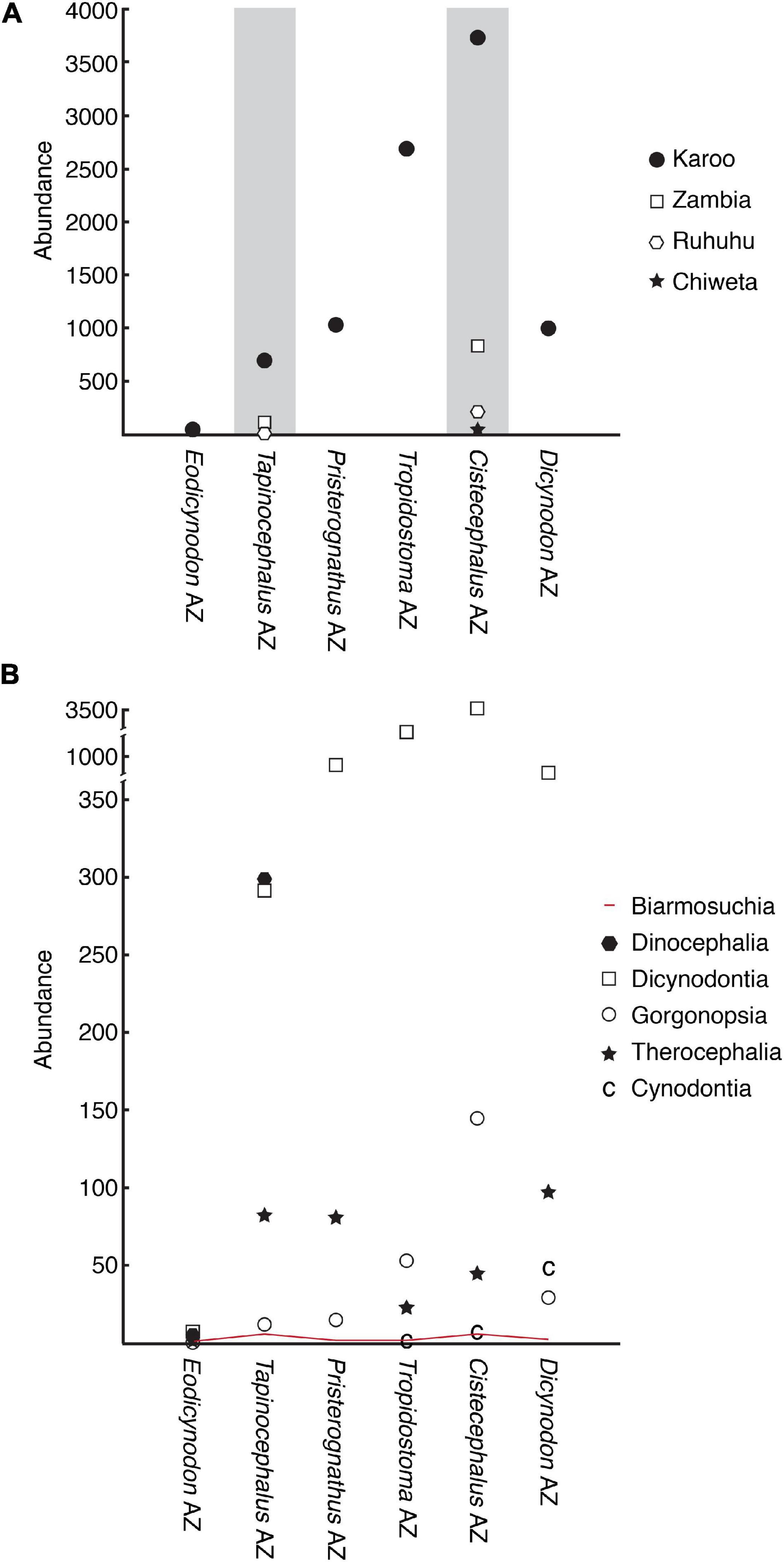
Figure 7. The fossil record of Permian therapsids from southern Pangea. Number of cataloged specimens in each geographic area (A) and number of cataloged specimens in each therapsid subgroup (B) are plotted against the biostratigraphic subdivisions of the Karoo Basin (Rubidge, 2005; Viglietti et al., 2016). Intervals where inter-basin comparisons are possible are highlighted in gray in (A). Abundance data are from Smith et al. (2012) for the Karoo and Peecook (2016) for Ruhuhu and Zambia (note that lower Madumabisa Mudstone Formation data from the Mid-Zambezi Basin are used for the Tapinocephalus Assemblage Zone and upper Madumabisa Mudstone Formation data from the Luangwa Basin are used for the Cistecephalus Assemblage Zone). Abundance data for the Chiweta beds of Malawi are based museum records at the SAM and UMZC as well as on the results of more recent collecting.
As part of their biogeographic argument, Kruger et al. (2015; see also Day et al., 2018) noted that both the oldest and the phylogenetically earliest diverging burnetiamorphs are from South Africa, as well as the majority of species. However, given the amount of paleontological effort devoted to the Karoo relative to other areas of mid-to-late Permian age (i.e., sampling noted above), one might predict all three of those observations to occur purely by chance. A more compelling scenario that contradicts the expectations of sampling would be if the earliest and most primitive members of a group occurred outside of the Karoo, and then only later did the group in question appear within South African rocks. Olroyd et al. (2018) found such an example for endothiodont dicynodonts. Another important consideration is the quality of the phylogenetic hypotheses available. The number of characters evaluated relative to the number of terminal taxa is relatively low in the current analysis, as well as in the analysis of Kruger et al. (2015), Day et al. (2018), Kammerer and Sidor (2021), and all previous studies. Relatedly, nearly all of the internal nodes recovered in the current analysis have weak support values. Indeed, adding a single extra step to the current analysis yields over four million most parsimonious trees, the strict consensus of which lacks any resolution within Burnetiamorpha. The pachyostosis seen in many burnetiamorphs can obscure the recognition of sutural contacts, thereby limiting the recognition of detailed homologies (Kulik and Sidor, 2019). It is possible that an over-reliance on characters related to cranial pachyostosis has led previous phylogenetic analyses astray and a greater variety of characters (e.g., postcranial) would yield a cladogram more consistent with the stratigraphic appearance of burnetiamorph taxa.
Like several previous authors, Day et al. (2018: 471) suggested that the, “origins of Burnetiidae probably lie in Gondwana” but they went further to suggest that the main Karoo Basin might have been on the fringe of the geographic range of burnetiamorphs, with greater numbers of species expected elsewhere (presumably at lower latitudes). The discovery of relatively numerous burnetiamorph skull caps in both the Guadalupian lower Madumabisa Mudstone Formation of Zambia as well as the Lopingian Usili Formation of Tanzania (Sidor et al., 2010, 2014 and unpublished data) is intriguing, but most of these specimens appear to represent one or two species/morphotypes. Therefore, while there is the suggestion that the abundance of individuals within a species is higher in Tanzania and Zambia, the low number of burnetiamorph species recognized per formation seems consistent regardless of geographic position.
Bosses, Horns, and Speciation
The discovery of a previously undescribed species of burnetiamorph in the upper Madumabisa Mudstone Formation of Zambia is somewhat surprising given that the faunal assemblage from these beds has been considered remarkably similar to others across southern Pangea. Indeed, Sidor et al. (2013) noted that of 21 Permian species recorded in Luangwa Basin of Zambia, only five were unique occurrences (i.e., endemic), with the 16 other taxa occurring in at least one other basin. However, it’s worth pointing out that Huttenlocker and Sidor (2020) have since reidentified one specimen as belonging to a new, endemic species for Zambia.
More generally, the discovery of an endemic burnetiamorph in Zambia conforms to the general pattern for the clade: almost every time a new burnetiamorph specimen is found, it is recognized as a new species. As seen in Table 1, only rarely are burnetiamorph species known from more than one specimen. At least three reasons might underlie this pattern. First, paleontologists using a morphological species concept might be compelled to recognize new species based on the differing forms of cranial ornamentation observed. In other words, because horns and crests elaborate through ontogeny, semaphoronts of the same species might be recognized as distinct species. Second, Day et al. (2018) proposed that burnetiamorphs might conform to the pattern, suggested by Stanley (1986) for Neogene bivalves, that rare species generally have high speciation rates.
A third, and more thought-provoking explanation for the relatively high number of burnetiamorph species given their low sample size, is that cranial ornamentation has a macroevolutionary relationship with speciation rate. As proposed for other clades like ceratopsian dinosaurs, horns and other visual displays might promote speciation by allowing for social selection or enhanced species recognition (Vrba, 1984; Sampson, 1999; Padian and Horner, 2011). One prediction made by this hypothesis is that a clade with adorned species should be more speciose than a closely related, but unadorned clade, with similar ecology. Although not sister-taxa, burnetiamorphs can be compared with gorgonopsians and therocephalians, as all three clades first appear in Guadalupian of southern Pangea, overlap in inferred body size, and share similar carnivorous ecologies. Remarkably, there are 13 burnetiamorph species known from 16 specimens (Table 1). By comparison, in the Cistecephalus Assemblage Zone of South Africa, there are 18 gorgonopsians based on 146 specimens, and nine therocephalians based on 46 specimens (based on values reported in Smith et al., 2012). Although preliminary, this suggests that burnetiamorphs were more speciose than would be expected given their sample size (Sidor et al., 2017).
A more systematic analysis of cranial adornment and its relationship to speciation would need to address the function of burnetiamorph horns and crests. Hieronymus et al. (2009) compared the osteological and histological correlates of various skin structures in a variety of extant tetrapods to the anatomy seen in centrosaurine ceratopsians as a way to infer the function of the latter (e.g., for head-butting). Kulik and Sidor (2019) applied the criteria developed by Hieronymus et al. (2009) to two burnetiamorph skull caps from the middle Permian of Zambia, but were unable to make definitive inferences about what covered them in life because of imperfect surficial preservation. Future work addressing the function of burnetiamorph cranial adornments will need to focus on comparative biomechanics, as has been previously proposed for some other groups with thickened, pachyostotic crania (e.g., tapinocephalids and pachycephalosaurs; Barghusen, 1975; Sues, 1978).
Data Availability Statement
The original contributions presented in the study are included in the article/Supplementary Material, further inquiries can be directed to the corresponding author.
Ethics Statement
Ethical review and approval was not required for the animal study because these are fossil vertebrates.
Author Contributions
CS conceptualized the study. NT and RS analyzed the geological data. CS analyzed the paleontological data. CS, NT, and RS drafted the manuscript and prepared the figures. All authors contributed to the article and approved the submitted version.
Funding
This research in Zambia has been supported by the National Geographic Society (CRE 8571-08 to J. S. Steyer, CRE 8962-11 to CS), the National Science Foundation (NSF EAR-1337569 to CS and EAR-1337291 to K. Angielczyk), and The Grainger Foundation and the Field Museum/IDP, Inc., African Partner’s Program (to K. Angielczyk).
Conflict of Interest
The authors declare that the research was conducted in the absence of any commercial or financial relationships that could be construed as a potential conflict of interest.
The reviewer MD declared a past co-authorship with one of the authors RS to the handling editor.
Acknowledgments
We thank K. Mwamulowe and J. Museba (NHCC) for assistance in arranging and carrying out the fieldwork. The entire 2014 field team (K. Angielczyk, N. Barbolini, C. Beightol, S. Myers, B. Peecook, S. Steyer, and S. Tolan) contributed to a tremendously successful expedition, but S. Nesbitt deserves special thanks for finding NHCC LB363. Fossil preparation was skillfully performed by G. Livingston, with final detailing by B. Crowley (both Burke Museum). We acknowledge the fine illustrations made by R. Whitehead and also C. Knaub for her contribution to the initial stages of this project. C. Abraczinskas provided helpful input on the layout of the figures. Finally, we thank M. Day and K. Angielczyk for the helpful reviews they provided on an earlier version of this manuscript as well as the two reviews for this Frontiers research topic.
Supplementary Material
The Supplementary Material for this article can be found online at: https://www.frontiersin.org/articles/10.3389/fevo.2021.685244/full#supplementary-material
References
Angielczyk, K. D., Steyer, J. S., Sidor, C. A., Smith, R. M. H., Whatley, R. L., and Tolan, S. (2014). “Permian and Triassic dicynodont (Therapsida: Anomodontia) faunas of the Luangwa Basin, Zambia: taxonomic update and implications for dicynodont biogeography and biostratigraphy,” in Early Evolutionary History of the Synapsida, eds C. F. Kammerer, K. D. Angielczyk, and J. Fröbisch (Dordrecht: Springer), 93–138. doi: 10.1007/978-94-007-6841-3_7
Banks, N. L., Bardwell, K. A., and Musiwa, S. (1995). “Karoo rift basins of the Luangwa Valley, Zambia,” in Hydrocarbon Habitat in Rift Basins, ed. J. J. Lambiase (London: Geological Society Special Publication), 285–295. doi: 10.1144/gsl.sp.1995.080.01.13
Barghusen, H. R. (1975). A review of fighting adaptation in dinocephalians (Reptilia, Therapsida). Paleobiology 1, 295–311. doi: 10.1017/s0094837300002542
Boonstra, L. D. (1934). On an aberrant gorgonopsian, Burnetia mirabilis Broom. S. Afr. J. Sci. 31, 462–470.
Broom, R. (1923). On the structure of the skull in the carnivorous dinocephalian reptiles. Proc. Zool. Soc. Lond. 2, 661–684. doi: 10.1111/j.1096-3642.1923.tb02203.x
Calvo, J. P., Alonso-Zarza, A. M., and García del Cura, M. A. (1989). Different models of marginal lacustrine sedimentation as a response to depositional regimes and source rocks in the Madrid Basin. Palaeogeogr. Palaeoclimatol. Palaeoecol. 70, 199–214. doi: 10.1016/0031-0182(89)90090-4
Catuneanu, O., Wopfner, H., Eriksson, P. G., Cairncross, B., Rubidge, B. S., Smith, R. M. H., et al. (2005). The Karoo basins of south-central Africa. J. Afr. Earth Sci. 43, 211–253.
Day, M. O., Ramezani, J., Bowring, S. A., Sadler, P. M., Erwin, D. H., Abdala, F., et al. (2015). When and how did the terrestrial mid-Permian mass extinction occur? Evidence from the tetrapod record of the Karoo Basin, South Africa. Proc. R. Soc. B Biol. Sci. 282:20150834. doi: 10.1098/rspb.2015.0834
Day, M. O., Rubidge, B. S., and Abdala, F. (2016). A new mid-Permian burnetiamorph therapsid from the main Karoo Basin of South Africa and a phylogenetic review of Burnetiamorpha. Acta Palaeontol. Pol. 61, 701–719.
Day, M. O., and Smith, R. M. H. (2020). Biostratigraphy of the Endothiodon Assemblage Zone (Beaufort Group, Karoo Supergroup), South Africa. Sou. Afr. J. Geol. 123, 165–180. doi: 10.25131/sajg.123.0011
Day, M. O., Smith, R. M. H., Benoit, J., Fernandez, V., and Rubidge, B. S. (2018). A new species of burnetiid (Therapsida: Burnetiamorpha) from the early Wuchiapingian of South Africa and implications for the evolutionary ecology of the family Burnetiidae. Pap. Palaeontol. 2018, 1–23.
Dixey, F. (1937). The geology of part of the Upper Luangwa Valley, North-Eastern Rhodesia. J. Geol. Soc. London 93, 52–74. doi: 10.1144/gsl.jgs.1937.093.01-04.05
Drysdall, A. R., and Kitching, J. W. (1962). The Karroo succession of the Upper Luangwa Valley, Northern Rhodesia. Trans. Geol. Soc. Sou. Afr. 45, 75–90.
Drysdall, A. R., and Kitching, J. W. (1963). A re-examination of the Karroo succession and fossil localities of part of the Upper Luangwa Valley. Memoir Geol. Surv. 1, 1–62.
Gustavson, T. C., and Winkler, D. A. (1988). Depositional facies of the Miocene-Pliocene Ogallala Formation, northwestern Texas and eastern New Mexico. Geology 16, 203–206. doi: 10.1130/0091-7613(1988)016<0203:dfotmp>2.3.co;2
Hieronymus, T. L., Witmer, L. M., Tanke, D. H., and Currie, P. J. (2009). The facial integument of Centrosaurine Ceratopsids: morphological and histological correlates of novel skin structures. Anat. Rec. 292, 1370–1396. doi: 10.1002/ar.20985
Hopson, J. A. (1991). “Systematics of the nonmammalian Synapsida and implications for patterns of evolution in synapsids,” in Origins of the Higher Groups of Tetrapods: Controversy and Consensus, eds H.-P. Schultze and L. Trueb (Ithaca: Comstock Publishing Associates), 635–693. doi: 10.7591/9781501718335-020
Huttenlocker, A. K., and Sidor, C. A. (2020). A basal nonmammalian cynodont from the Permian of Zambia and the origins of mammalian endocranial and postcranial anatomy. J. Vertebr. Paleontol. 40:e1827413. doi: 10.1080/02724634.2020.1827413
Jacobs, L. L., Winkler, D. A., Newman, K. D., Gomani, E. M., and Deino, A. (2005). Therapsids from the Permian Chiweta Beds and the age of the Karoo Supergroup in Malawi. Palaeontol. Electronica 8:23.
Kammerer, C. F. (2016). Two unrecognized burnetiamorph specimens from historical Karoo collections. Palaeontol. Africana 50, 64–75.
Kammerer, C. F., and Sidor, C. A. (2021). A new burnetiid from the middle Permian of Zambia and a reanalysis of burnetiamorph relationships. Pap. Palaeontol. doi: 10.1002/spp2.1341
Kemp, T. S. (1975). Vertebrate localities in the Karroo System of the Luangwa Valley, Zambia. Nature 254, 415–416. doi: 10.1038/254415a0
Kitching, J. W. (1963). The fossil localities and mammal-like reptiles of the upper Luangwa Valley, Northern Rhodesia. Sou. Afr. J. Sci. 59, 259–264.
Kruger, A., Rubidge, B. S., Abdala, F., Chindebvu, E. G., and Jacobs, L. L. (2015). Lende chiweta, a new therapsid from Malawi, and its influence on burnetiamorph phylogeny and biogeography. J. Vertebr. Paleontol. 35:e1008698. doi: 10.1080/02724634.2015.1008698
Kulik, Z. T., and Sidor, C. A. (2019). The original boneheads: histologic analysis of the pachyostotic skull roof in Permian burnetiamorphs (Therapsida: Biarmosuchia). J. Anat. 255, 151–166.
Olroyd, S. L., Sidor, C. A., and Angielczyk, K. D. (2018). New materials of the enigmatic dicynodont Abajudon kaayai (Therapsida, Anomodontia) from the lower Madumabisa Mudstone Formation, middle Permian of Zambia. J. Vertebr. Paleontol. 37:e1403442. doi: 10.1080/02724634.2017.1403442
Padian, K., and Horner, J. R. (2011). The evolution of ‘bizarre structures’ in dinosaurs: biomechanics, sexual selection, social selection or species recognition. J. Zool. 283, 3–17. doi: 10.1111/j.1469-7998.2010.00719.x
Peecook, B. R. (2016). Ecological Dynamics of Vertebrate Assemblages Across the End-Permian Mass Extinction in Tanzania and Zambia. Ph. D. thesis. Washington: University of Washington.
Peecook, B. R., Angielczyk, K. D., Kammerer, C. F., Lungmus, J. K., McIntosh, J. A., Sidor, C. A., et al. (2020). A new stratigraphic framework for the Permo-Triassic strata of the Luangwa Basin, Zambia: upper Permian turnover and Middle-Upper Triassic vertebrate assemblages. J. Vertebr. Paleontol. 40 (electronic supplement):266.
Rubidge, B. S. (2005). Re-uniting lost continents — Fossil reptiles from the ancient Karoo and their wanderlust. Sou. Afr. J. Geol. 108, 135–172. doi: 10.2113/108.1.135
Rubidge, B. S., Erwin, D. H., Ramezani, J., Bowring, S. A., and de Klerk, W. J. (2013). High-precision temporal calibration of Late Permian vertebrate biostratigraphy: U-Pb zircon constraints from the Karoo Supergroup, South Africa. Geology 41, 363–366. doi: 10.1130/g33622.1
Rubidge, B. S., and Kitching, J. W. (2003). A new burnetiamorph (Therapsida: Biarmosuchia) from the lower Beaufort Group of South Africa. Palaeontology 46, 199–210. doi: 10.1111/1475-4983.00294
Rubidge, B. S., and Sidor, C. A. (2001). Evolutionary patterns among Permo-Triassic therapsids. Annu. Rev. Ecol. Syst. 32, 449–480. doi: 10.1146/annurev.ecolsys.32.081501.114113
Rubidge, B. S., and Sidor, C. A. (2002). On the cranial morphology of the basal therapsids Burnetia and Proburnetia (Therapsida: Burnetiidae). J. Vertebr. Paleontol. 22, 257–267. doi: 10.1671/0272-4634(2002)022[0257:otcmot]2.0.co;2
Rubidge, B. S., Sidor, C. A., and Modesto, S. P. (2006). A new burnetiamorph (Therapsida: Biarmosuchia) from the middle Permian of South Africa. J. Paleontol. 80, 740–749. doi: 10.1666/0022-3360(2006)80[740:anbtbf]2.0.co;2
Sampson, S. D. (1999). Sex and destiny: the role of mating signals in speciation and macroevolution. Hist. Biol. 13, 173–197. doi: 10.1080/08912969909386580
Sidor, C. A. (2000). Evolutionary Trends and Relationships within the Synapsida. Ph. D. thesis. Chicago: University of Chicago.
Sidor, C. A. (2003). The naris and palate of Lycaenodon longiceps (Therapsida: Biarmosuchia), with comments on their early evolution in the Therapsida. J. Paleontol. 77, 153–160.
Sidor, C. A. (2015). The first biarmosuchian from the upper Madumabisa Mudstone Formation (Luangwa Basin) of Zambia. Palaeontol. Africana 49, 1–7.
Sidor, C. A., Angielczyk, K. D., Nesbitt, S. J., Peecook, B. R., Smith, R. M. H., Tabor, N. J., et al. (2017). Burnetiamorphs did it first: cranial adornment and rates of speciation in a Permian lineage of therapsids. J. Vertebr. Paleontol. 37(electronic supplement):194.
Sidor, C. A., Angielczyk, K. D., Smith, R. M. H., Goulding, A. K., Nesbitt, S. J., Peecook, B. R., et al. (2014). Tapinocephalids (Therapsida: Dinocephalia) from the Permian Madumabisa Mudstone Formation (Lower Karoo, Mid-Zambezi Basin) of southern Zambia. J. Vertebr. Paleontol. 34, 980–986. doi: 10.1080/02724634.2013.826669
Sidor, C. A., Angielczyk, K. D., Weide, D. M., Smith, R. M. H., Nesbitt, S. J., and Tsuji, L. A. (2010). Tetrapod fauna of the lowermost Usili Formation (Songea Group, Ruhuhu Basin) of southern Tanzania, with a new burnetiid record. J. Vertebr. Paleontol. 30, 696–703. doi: 10.1080/02724631003758086
Sidor, C. A., Hopson, J. A., and Keyser, A. W. (2004). A new burnetiamorph therapsid from the Teekloof Formation, Permian, of South Africa. J. Vertebr. Paleontol. 24, 938–950. doi: 10.1671/0272-4634(2004)024[0938:anbtft]2.0.co;2
Sidor, C. A., Knaub, C. R., Angielczyk, K. D., Beightol, C. V., Nesbitt, S. J., Smith, R. M. H., et al. (2015). Tanzania and Zambia yield an unprecedented fossil record of burnetiamorph therapsids. J. Vertebr. Paleontol. Program Abstracts. 2015:213.
Sidor, C. A., and Nesbitt, S. J. (2018). Introduction to vertebrate and climatic evolution in the Triassic rift basins of Tanzania and Zambia. J. Vertebr. Paleontol. 37, 1–7. doi: 10.1080/02724634.2017.1420661
Sidor, C. A., and Rubidge, B. S. (2006). “Herpetoskylax hopsoni, a new biarmosuchian (Therapsida: Biarmosuchia) from the Beaufort Group of South Africa,” in Amniote Paleobiology: Perspectives on the Evolution of Mammals, Birds, and Reptiles, eds M. T. Carrano, T. Gaudin, R. Blob, and J. Wible (Chicago: University of Chicago Press), 76–113.
Sidor, C. A., and Smith, R. M. H. (2007). A second burnetiamorph from the Permian Teekloof Formation of South Africa and its associated fauna. J. Vertebr. Paleontol. 27, 420–430. doi: 10.1671/0272-4634(2007)27[420:asbtft]2.0.co;2
Sidor, C. A., Vilhena, D. A., Angielczyk, K. D., Huttenlocker, A. K., Nesbitt, S. J., Peecook, B. R., et al. (2013). Provincialization of terrestrial faunas following the end-Permian mass extinction. Proc. Natl. Acad. Sci. U. S. A. 110, 8129–8133. doi: 10.1073/pnas.1302323110
Sidor, C. A., and Welman, J. (2003). A second specimen of Lemurosaurus pricei (Therapsida: Burnetiamorpha). J. Vertebr. Paleontol. 23, 631–642. doi: 10.1671/0272-4634(2003)023[0631:assolp]2.0.co;2
Sigogneau-Russell, D. (1989). “Theriodontia I,” in Encyclopedia of Paleoherpetology, Part 17B, ed. P. Wellnhofer (Stuttgart: Gustav Fischer), 1–127. doi: 10.1206/3738.2
Smith, R., Rubidge, B., and van der Walt, M. (2012). “Therapsid biodiversity patterns and paleoenvironments of the Karoo Basin, South Africa,” in Forerunners of Mammals: Radiation, Histology, Biology, ed. A. Chinsamy-Turan (Indianapolis: Indiana University Press), 30–62.
Smith, R. M. H. (2000). Sedimentology and taphonomy of Late Permian vertebrate fossil localities in southwestern Madagascar. Palaeontol. Africana 36, 25–41.
Smith, R. M. H., Rubidge, B. S., Day, M. O., and Botha, J. (2020). Introduction to the tetrapod biozonation of the Karoo Supergroup. Sou. Afr. J. Geol. 123, 131–140. doi: 10.25131/sajg.123.0009
Smith, R. M. H., Rubidge, B. S., and Sidor, C. A. (2006). A new burnetiid (Therapsida: Biarmosuchia) from the Upper Permian of South Africa and its biogeographic implications. J. Vertebr. Paleontol. 26, 331–343. doi: 10.1671/0272-4634(2006)26[331:anbtbf]2.0.co;2
Stanley, S. M. (1986). Population size, extinction, and speciation: the fission effect in Neogene Bivalvia. Paleobiology 12, 89–110. doi: 10.1017/s0094837300003006
Sues, H.-D. (1978). Functional morphology of the dome in pachycephalosaurid dinosaurs. Neues Jahrb. Geol. Paläontol. Monatsh. 1978, 459–472.
Swofford, D. L. (2002). PAUP∗. Phylogenetic Analysis Using Parsimony (∗and Other Methods). Version 4. Sunderland: Sinauer Associates.
Utting, J. (1978). The Karroo stratigraphy of the northern part of the Luangwa Valley. Memoir Geol. Surv. 4, 1–64.
Viglietti, P. A. (2020). Biostratigraphy of the Daptocephalus Assemblage Zone (Beaufort Group, Karoo Supergroup), South Africa. Sou. Afr. J. Geol. 123, 191–206. doi: 10.25131/sajg.123.0014
Viglietti, P. A., Smith, R. M. H., Angielczyk, K. D., Kammerer, C. F., Fröbisch, J., and Rubidge, B. S. (2016). The Daptocephalus Assemblage Zone (Lopingian), South Africa: a proposed biostratigraphy based on a new compilation of stratigraphic ranges. J. Afr. Earth Sci. 113, 1–12.
Vrba, E. S. (1984). “Evolutionary pattern and process in the sister-group Alcelaphini-Aepycerotini (Mammalia: Bovidae),” in Living Fossils, eds N. Eldredge and S. M. Stanley (New York: Springer-Verlag), 62–79. doi: 10.1007/978-1-4613-8271-3_7
Wagner, P. J., and Sidor, C. A. (2000). Age rank/clade rank metrics-sampling, taxonomy, and the meaning of “stratigraphic consistency”. Syst. Biol. 49, 463–479. doi: 10.1080/10635159950127349
Whitney, M. R., and Sidor, C. A. (2016). A new therapsid from the Permian Madumabisa Mudstone Formation (Mid-Zambezi Basin) of southern Zambia. J. Vertebr. Paleontol. 36:e1150767. doi: 10.1080/02724634.2016.1150767
Yemane, K., and Kelts, K. (1990). A short review of paleoenvironments for the Lower Beaufort (Upper Permian) Karoo sequences from southern to central Africa: a major Gondwana lacustrine episode. J. Afr. Earth Sci. 10, 169–185. doi: 10.1016/0899-5362(90)90053-h
Appendix 1
This line of data was added to the cladistic analysis of biarmosuchian relationships presented by Kammerer and Sidor (2021). The complete list of characters and the remainder of the data matrix can be found in that paper or at Morphobank publication #3785 (https://morphobank.org/index.php/Projects/ProjectOverview/project_id/3785).
Isengops luangwenesis ?0111001?1010?2??1001?0?111.
Keywords: Lopingian, Permian, Luangwa Basin, Zambia, Africa, speciation, Burnetiamorpha, Therapsida
Citation: Sidor CA, Tabor NJ and Smith RMH (2021) A New Late Permian Burnetiamorph From Zambia Confirms Exceptional Levels of Endemism in Burnetiamorpha (Therapsida: Biarmosuchia) and an Updated Paleoenvironmental Interpretation of the Upper Madumabisa Mudstone Formation. Front. Ecol. Evol. 9:685244. doi: 10.3389/fevo.2021.685244
Received: 24 March 2021; Accepted: 18 May 2021;
Published: 24 June 2021.
Edited by:
Mark Joseph MacDougall, Museum of Natural History Berlin (MfN), GermanyReviewed by:
Sean P. Modesto, Cape Breton University, CanadaMichael Oliver Day, Natural History Museum, United Kingdom
Copyright © 2021 Sidor, Tabor and Smith. This is an open-access article distributed under the terms of the Creative Commons Attribution License (CC BY). The use, distribution or reproduction in other forums is permitted, provided the original author(s) and the copyright owner(s) are credited and that the original publication in this journal is cited, in accordance with accepted academic practice. No use, distribution or reproduction is permitted which does not comply with these terms.
*Correspondence: Christian A. Sidor, Y2FzaWRvckB1dy5lZHU=
†ORCID: Christian A. Sidor, orcid.org/0000-0003-0742-4829; Roger M. H. Smith, orcid.org/0000-0001-6806-1983