Thyroid-Mediated Metabolic Differences Underlie Ecological Specialization of Extremophile Salmonids in the Arctic Lake El’gygytgyn
- 1A.N. Severtsov Institute of Ecology and Evolution, Russian Academy of Sciences, Moscow, Russia
- 2Kronotsky Nature Biosphere Reserve, Yelizovo, Russia
- 3The Faculty of Biology, Lomonosov Moscow State University, Moscow, Russia
- 4Koltzov Institute of Developmental Biology, Russian Academy of Sciences, Moscow, Russia
El’gygytgyn, the only “ancient lake” in the Arctic (3.6 MY), is a deep (176 m) and extremely cold (always ≤ 4°C) waterbody inhabited by unique salmonids, which colonized the ecosystem stepwise during the global fluctuations of the Quaternary climate. The descendant of the first-wave-invaders (long-finned charr) dwells in the deep waters and feeds on amphipods. The second-wave-invaders (smallmouth charr) consume copepods in the mid-waters. Recent third-wave-invaders (Boganida charr) are spread throughout the ecosystem and feed on insects when they are young shifting to piscivory at an older age. Here, we present the data on the charrs’ thyroid status and metabolic characteristics, confirming their ecological specialization. The long-finned charr exhibits an extremely low thyroid content, the substitution of carbohydrates for lipids in the cellular respiration, an increased hemoglobin level and a high antioxidant blood capacity. These traits are likely to be the legacy of anaerobic survival under perennial ice cover during several Quaternary glaciations. Moderate thyroid status and reduced metabolic rate of the smallmouth charr, along with an inactive lifestyle, could be regarded as a specialization to saving energy under the low food supply in the water column. The piscivorous Boganida charr could be sub-divided into shallow-water and deep-water groups. The former demonstrates a significantly elevated thyroid status and increased metabolism. The latter is characterized by a reduced thyroid level, metabolic rate, and lipid accumulation. Thus, the endemic El’gygytgyn charrs represent a wide spectrum of contrast physiological adaptation patterns essential to survive in sympatry under extremely cold conditions.
Introduction
Modern teleosts inhabit almost any kind of waterbody on the Earth, including such inhospitable habitats as hot springs in the deserts and subzero Antarctic waters, low-oxygen swamps, subterranean waterbodies, and lakes with extreme salinity (Nelson et al., 2016). The majority of fishes thriving under extreme conditions, i.e., extremophile fishes, exhibit remarkable physiological, morphological, life-history, and behavioral adaptations, so they are attractive models for investigating the causes and consequences of adaptive evolution (Plath et al., 2015). In the Arctic, freshwater salmonids represent multiple adaptations allowing them to flourish despite the long winter seasons coupled with darkness, ice shield, and low temperatures. Among the numerous Arctic salmonid communities, the unique fauna persisting in Lake El’gygytgyn (Western Beringia, Chukotka) through several glacial periods is of particular interest. This waterbody is the only “ancient” lake in the Arctic filling a meteorite crater formed 3.6 MY ago. The 176-m deep lake of a bowl-shaped morphology and the surface area of 110 km2 never warms up above 4°C and is covered with ice for 9–10 months per year (Nolan and Brigham-Grette, 2007; Melles et al., 2012). Given that the lake basin had escaped the continental-scale glaciation (Glushkova, 2001; Melles et al., 2012), it represents a unique model for paleoecological and evolutionary reconstructions.
Ichthyologists have discovered the endemic highly specialized El’gygytgyn charrs (g. Salvelinus, Figure 1), which stepwise colonized the lake during the global fluctuations of the Quaternary climate (Chereshnev et al., 2002; Osinov et al., 2015). The first invaders entered the lake between 3.6 and 1.7 MY ago. Their descendant, the long-finned charr, S. svetovidovi, dwells in the deep and demonstrates a very peculiar morphology with paedomorphic traits (Chereshnev and Skopets, 1990; Frolov, 1993; Alekseyev, 2000). The ancestors of two closely related but allopatric charrs: smallmouth, S. elgyticus, and Boganida, S. boganidae, invaded the lake much later, after the Last Glacial Maximum, 15–5 thousand years ago (Osinov et al., 2015; Oleinik et al., 2019, 2021; Osinov and Volkov, 2020). The former species is a gracile-bodied charr possessing planktivorous traits (Chereshnev et al., 2002). The latter, being the representatives of the Boganida charr, are found throughout the ecosystem and characterized by the phenotype typical of piscivorous salmonids (Chereshnev et al., 2002).
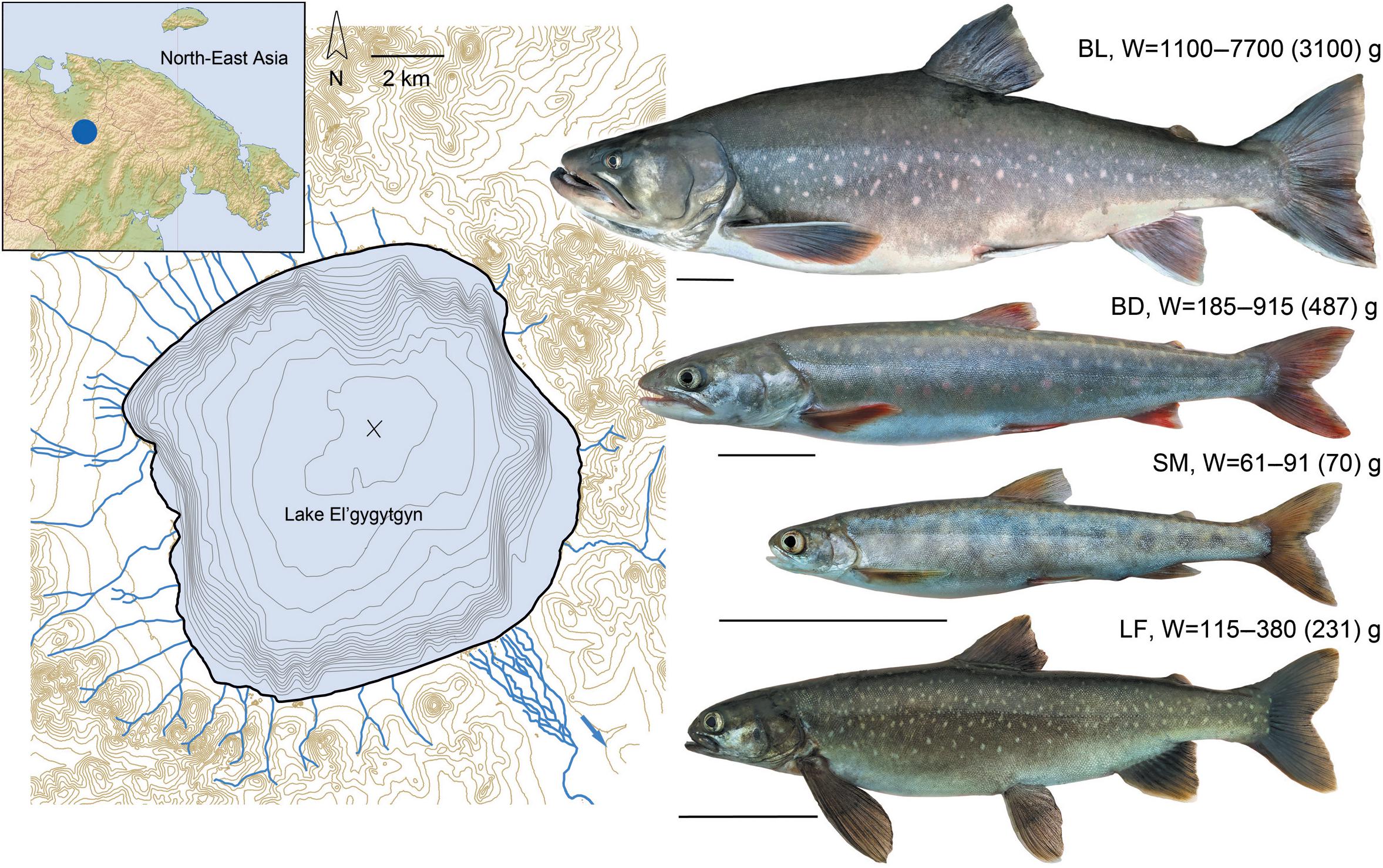
Figure 1. Lake El’gygytgyn map (on the left), its location (incut), and charr fauna discovered (on the right): BL, Boganida charr, large-sized epilimnetic; BD, Boganida charr, deepwater; SM, smallmouth charr; LF, long-finned charr. The weights (the range and mean value) of adult fish used for the analysis are presented. The lines under fish images correspond to 5 cm.
Surviving in the extremely cold, deep, and low productive waters of El’gygytgyn, is an energy-balancing task requiring various physiological adaptations, foremost the consistent metabolic changes. We suggest that El’gygytgyn charrs possess common traits essential for life in the cold, such as enriching the cell membranes by the polyunsaturated fatty acids, changing the enzymes’ activity, respiration rate and glycogen metabolism (Cossins and Prosser, 1978; Guderley, 2004; White et al., 2012; DeLong et al., 2018). However, the endemic charrs varying in lifestyle should develop some specific metabolic features related to their ecological peculiarities.
To test this hypothesis, we compared the El’gygytgyn charrs’ metabolic phenotypes defined by a set of biochemical traits regulating the intensity of life processes. In particular, we assessed the blood glucose and liver glycogen levels as the circulating and stored source of the immediate energetic reserve (Masanori and Shizunori, 1971; Polakof et al., 2012). We evaluated the lipids in plasma lipoproteins and muscles, and especially triacylglycerides, as the circulating and stored source of deferred energetic reserve (Jobling, 1994b). As the indicator of the biochemical transport activity, we assessed plasma proteins (Rehulka et al., 2005) and phospholipids, which determine the cell membrane permeability, and outspread with lipoproteins throughout the body to intensify metabolism (Freeman et al., 1963). The oxidative balance was assessed via measuring the intensity of oxygen transport by hemoglobin and lipid oxidation. The latter was analyzed by the concentration of thiobarbituric acid active compounds in plasma (Debevec et al., 2017).
Finally, we compared the content of thyroid hormones as the primary endocrine factors regulating the energy balance, contributing to the thermal acclimatization, and acting as a mediator of adaptation to the changing environment in fish (Blanton and Specker, 2007; Little et al., 2013; McMenamin and Parichy, 2013; Campinho, 2019; Deal and Volkoff, 2020; Lema, 2020; Esin et al., 2021).
Materials and Methods
The lake was fished evenly at all depths using multipanel gillnets (18–40 mm mesh size) in August 2020. All the catches were carried out in accordance with the permission No. 412020032162 issued 07/21/2020 by the North-Eastern Territorial Administration of the Federal Agency for Fishery. The procedures with fish were approved by the Bioethics commission of the A.N. Severtsov Institute of Ecology and Evolution, Russian Academy of Sciences. The catch turned out to be represented by four and not three contrasting phenotypes separated on the spot by eye (Figure 1). Only the adult individuals of the deepwater slender-bodied Boganida charrs (BD) and long-finned charrs (LF) overlapped in size. Nevertheless, even these individuals were easily distinguished from each other, as well as from the large robust-bodied Boganida charrs (BL) and small-sized smallmouth charrs (SM) (Supplementary Figure 1) in a mixed catch.
To standardize the data on the physiological performance of the charrs for further comparison, only adult fish skipping the spawning of the appropriate year were subsampled for the analysis. The final set comprised 23 BL, 21 BD, 19 SM, and 26 LF individuals. All these fish were processed freshly caught following the standard protocol. The blood was collected from the caudal vessel with Vacuette serum tubes. The samples of muscle (cut behind the dorsal fin) and liver (distal lobe) were weighted on a balance AND HR-AZ with an accuracy of ±0.001 g. The set of biochemical parameters (see Supplementary Figure 2) was measured spectrophotometrically using the photometers Hatch DR2400 and StatFax 303 Plus. Centrifugation procedures were performed at 2,900 g (Velocity 6u, Dynamica).
Carbohydrate Analysis
The level of stored and circulated carbohydrates was analyzed in terms of liver glycogen and blood glucose content. The caudal fragment of the liver of 2 mg in weight was digested in 500 μl 30% NaOH at 100°C for 30 min. Then, 100 μl of 60% H2SO4 was added to the sample, and the volume was brought up to 200 μl with Milli-Q water. The sample was mixed with 1.5 mg of 0.2% anthrone reagent (Sigma) and incubated at 100°C for 20 min (Templeton, 1961). The optical density was read at 630 nm against glycogen standards. The glucose concentration was determined in blood by an automatic glucose-meter Contour TS (Harrison et al., 2011).
Blood Protein Analysis
The hemoglobin concentration was measured by the cyanmethemoglobin method (Gammon and Baker, 1977) with 5 ml of transforming reagent (Renam kit) added to 20 μl of whole blood. The plasma protein content was identified in 10 μl samples by the Biuret test (Smith et al., 1985) using the assembled Agat-Med kit. The absorbance of both components was measured at 540 nm against standard solutions.
Lipid Analysis
The level of circulated lipids was analyzed in terms of plasma lipoproteins’ content (excluding high-density fraction), which were precipitated using BioSystems kit. The samples of 20 μl plasma dissolved in 0.5 ml phosphovolframate-MgCl2 reactive were incubated at 100°C for 15 min followed by 1-h centrifugation (Burstein et al., 1980; Regerand et al., 1990). Then, following Folch et al. (1957) procedure, lipids were extracted from the resuspended precipitate and purified with 1% KCl solution employed as the aqueous phase. The Folch method was also used to extract lipids from 200-mg samples of shredded muscle.
The total lipids’ concentration was measured via sulfo-phospho-vanillin spectrophotometric approach, absorbance was measured at 520 nm (Knicht et al., 1972). The enzymatic hydrolysis reactions (Spinreact kits) provided triglyceride (TAG) and phospholipid (PHL) values (Trinder, 1969; Takeyama et al., 1977). The indicator substance kinonimin was measured at 505 nm. The lipid concentration was recalculated to the weight of muscle samples dried to constant weight.
Thiobarbituric Acid Reactive Substances
The intensity of lipid (per)oxidation in plasma was assessed by the content of thiobarbituric acid reactive substances (TBARS). Malondialdehyde was obtained from 0.2 ml plasma by adding 1 ml of thiobarbituric acid in phosphate buffer, subsequent incubation at 100°C for 45 min and 10-min centrifuging with 4 ml of butanol. The supernatant concentration was determined at 570 and 535 nm (Gavrilov et al., 1987).
Thyroid Hormone Content
The total triiodothyronine (T3, bioactive form) and thyroxine (T4, prohormone) concentrations in plasma were evaluated by enzyme-linked immunosorbent assay. We used commercially available Monobind kits and measured the hormones at 450 nm with a sensitivity of 0.04 pg ml–1 (tT3) and 3.2 pg ml–1 (T4).
Data Processing
The obtained data were statistically checked for the distribution matching in different charr morphs using the Kolmogorov-Smirnov test (p > 0.05 in all cases). To investigate the differences among the morphs, we applied ANOVA complemented with post hoc Tukey HSD. Then, the biochemical parameters were tested for the correlation with each over and with the thyroid level. To elucidate the metabolic phenotypes of the charrs based on all eleven parameters, we used canonical variate analysis based on Wilks’ Lambda statistics launched in Statsoft v.10 (Hill and Lewicki, 2006).
Results
Distribution and Feeding
The charrs exhibited spatial segregation and different food preferences. BL was mostly caught in the epilimnetic zone (∼70% of catch). Its stomach contained fish and insects regardless of the fish size. BD was collected in the profundal zone (>90%), where it consumed fish. SM was represented mainly on the lake slope (∼70%), and their stomachs contained planktonic copepods. LF was found in the profundal zone and on the slope approximately in equal proportion. This species fed on deepwater amphipods and planktonic copepods.
Carbohydrate Characteristics
The content of blood glucose was interrelated with that of liver glycogen (r = 0.67; p = 0.0001) and morph-specific, ANOVA for the glucose/glycogen concentrations: F3;86 = 9.70; p = 0.0002/F3;86 = 187.42; p = 0.0001; p < 0.0001. LF exhibited the lowest content of both carbohydrates. SM showed a significantly reduced glycogen reserve and a slightly reduced glucose level relative to Boganida charrs. Among the latter, BL had a slightly increased glycogen reserve and a more stable glucose level than BD (Figures 2A,B and Supplementary Table 1 for pairwise p-values).
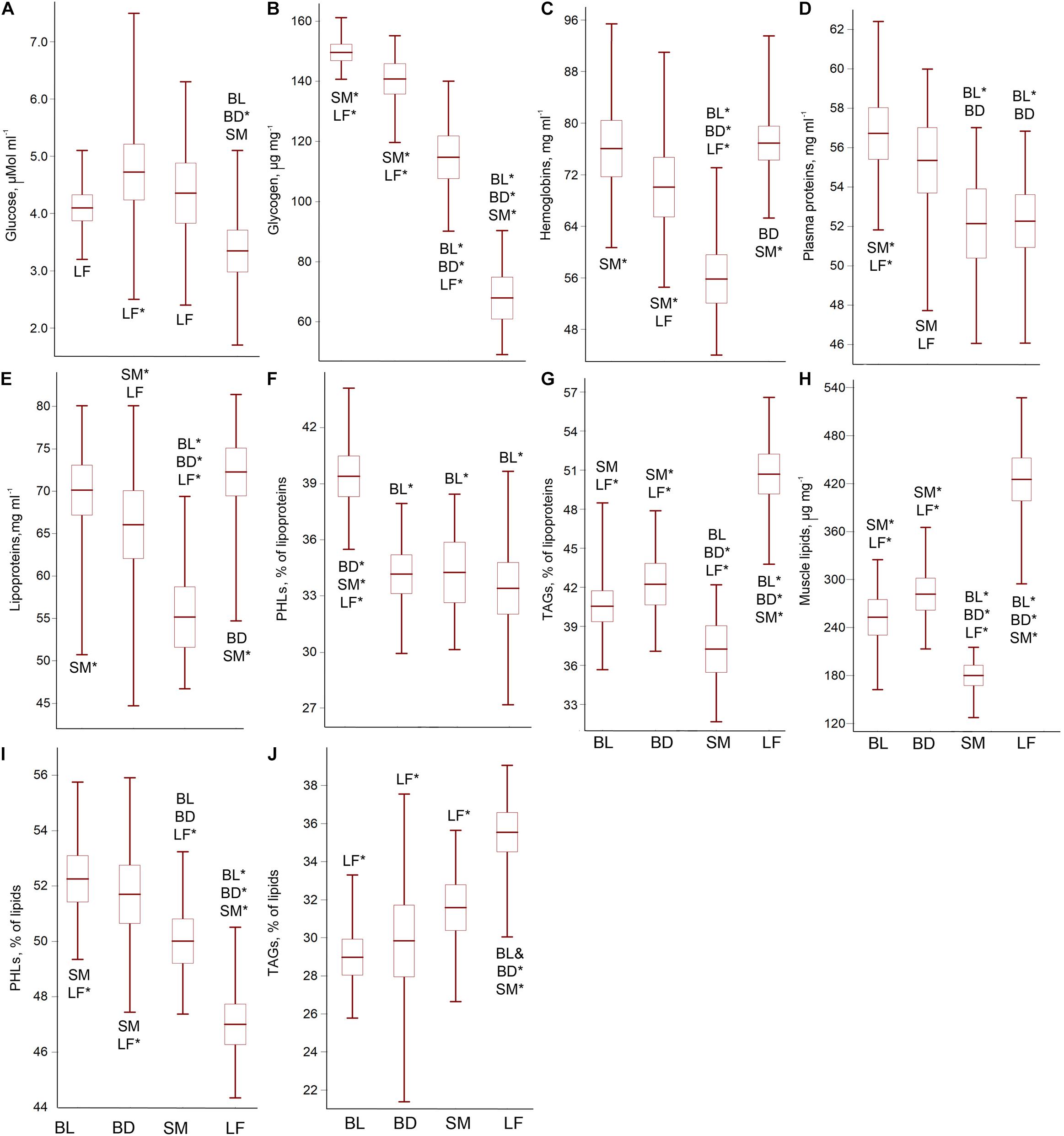
Figure 2. The blood glucose (A), liver glycogen (B), blood hemoglobin (C) and plasma protein (D) contents, as well as total plasma lipid content (E),% of PHL (F), and TAG (G) in the plasma lipids, total muscle lipid content (H), and% of PHL (I) and TAG (J) in the muscle lipids of the Lake El’gygytgyn charrs. Mean ± 95% confidence interval and min-max values are shown. Abbreviations with whisker boxes denote significantly differed groups (*p < 0.001; for the significance level of differences see Supplementary Tables 1–4). No correlation between the biochemical parameters and the fish weight (size) was found (r < 0.30; p > 0.05), except for the lipoproteins content in BD (r = 0.78; p = 0.0163) and muscle lipids’ content in BL (r = 0.96; p = 0.0111). In both cases, the fish accumulated lipids with the growth.
The liver glycogen reserve correlated with the plasma protein concentration (r = 0.53; p = 0.0005), as well as TAG reserve in the plasma (r2 = 0.51; p = 0.0006) and muscles (r = 0.86; p < 0.0001).
Blood Protein Characteristics
The morphs were defined by the specific ratio of hemoglobins and plasma proteins, ANOVA for the hemoglobin/plasma protein concentrations: F3;86 = 26.86; p < 0.0001/F3;86 = 10.31; p = 0.0001. SM possessed a reduced level of both parameters. LF demonstrated an increased level of hemoglobin but a decreased level of plasma proteins. A slight elevation in the blood protein content was revealed in BL compared to BD (Figures 2C,D and Supplementary Table 2 for pairwise p-values).
Lipid Characteristics
Lipoproteins
The charrs differed in the content of lipoproteins (ANOVA for the total lipid concentration in the plasma: F3;86 = 18.66; p < 0.0001). LF stood apart by the maximum lipid reserve, whereas SM demonstrated the lowest plasma lipid concentration (Figure 2E and Supplementary Table 3 for pairwise p-values). The plasma lipid composition also differed (ANOVA for% of PHL/TAG in plasma lipids: F3;86 = 23.34; p < 0.0001/F3;86 = 64.02; p < 0.0001). The PHL and TAG plasma contents displayed a significant inverse correlation (r = 0.56; p = 0.0003). The PHL level was significantly higher in BL (Figure 2F), TAG level – in LF; whereas SM showed a very low TAG plasma reserve (Figure 2G and Supplementary Table 3).
Muscle Lipids
The total lipid concentration in muscles correlated with the lipoprotein content in blood (r = 0.71; p = 0.0001). SM displayed a very low muscle lipid reserve, and LF had an extremely high one, ANOVA F3;86 = 98.25; p < 0.0001 (Figure 2H and Supplementary Table 4 for pairwise p-values). The ratio of negatively interrelated PHL and TAG (r = 0.95; p < 0.0001) differed between the charrs, ANOVA for% of PHL/TAG: F3;86 = 37.76; p < 0.0001/F3;86 = 22.35; p < 0.0001. The PHL level significantly declined, while the TAG level significantly increased in the row: Boganida charrs – SM – LF (Figures 2I,J and Supplementary Table 4). The PHL and TAG levels in the muscles and blood were correlated (r = 0.54; p = 0.0003 for both).
Plasma Thiobarbituric Acid Reactive Substances
The Boganida charrs demonstrated a plasma thiobarbituric acid reactive substances (TBARS) level equal to 1.33 ± 0.11 (from 0.8 to 2.8) nmol ml–1. A slight elevation of the parameter was revealed for SM, 1.61 ± 0.16 (1.0–2.8) nmol ml–1. At the same time, LF displayed significantly reduced TBARS levels (ANOVA F3;70 = 8.13; p = 0.0206) indicating a higher antioxidant capacity of the blood.
Thyroid Status
The charrs demonstrated morph-specific hormonal status, ANOVA for the T3/T4 concentrations: F3;77 = 49.32; p < 0.0001/F3;38 = 14.15; p < 0.0001. LF had a significantly reduced hormonal content, while BL demonstrated an increased hormonal level. BD and SM were characterized by a moderate T3 supply. However, SM differed by an extremely low T4 concentration (Figure 3 and Supplementary Table 5 for post hoc pairwise p-values).
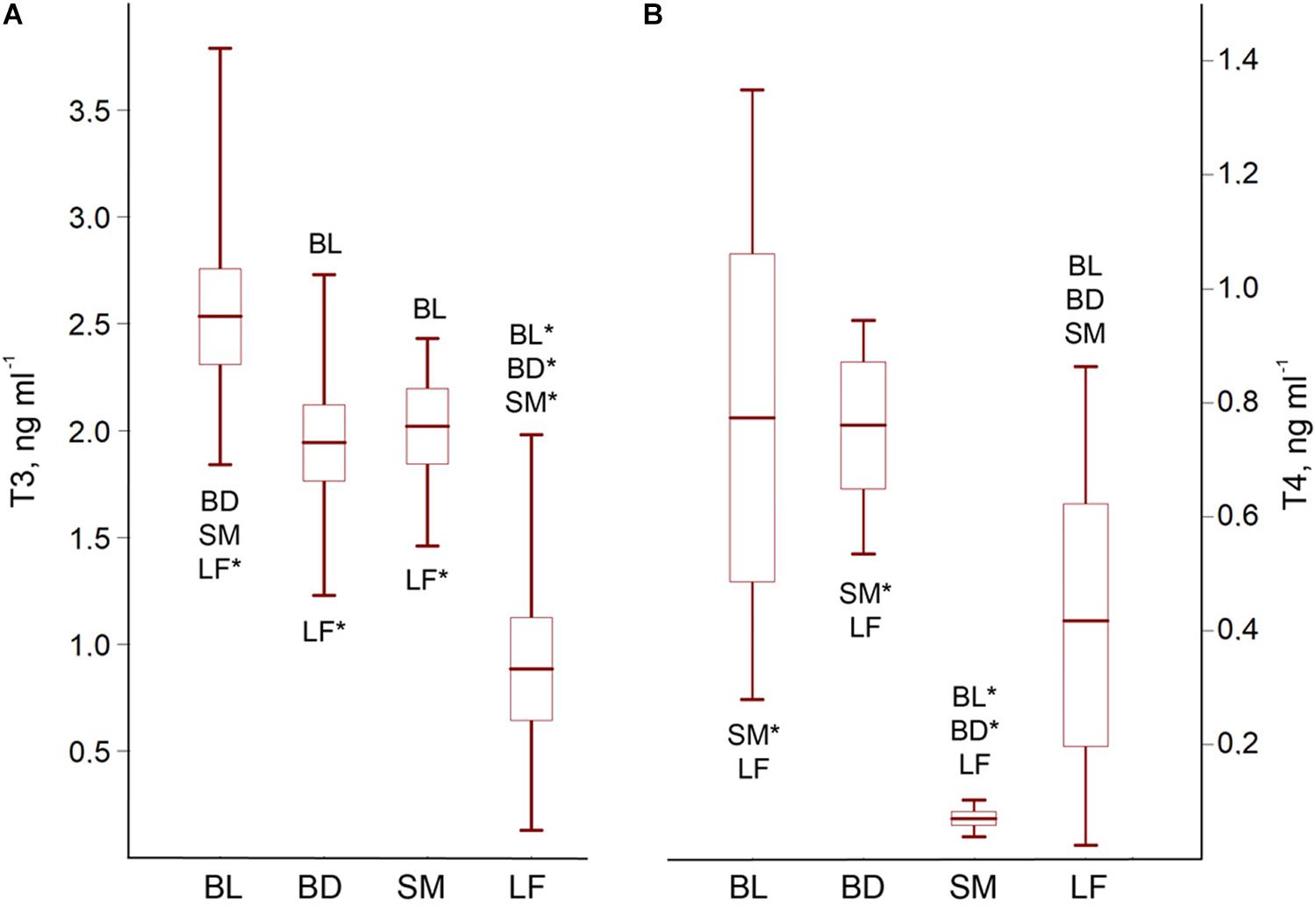
Figure 3. The T3 (A) and T4 (B) blood content of the El’gygytgyn charrs. Mean ± 95% confidence interval and min-max values are shown. Abbreviations with whisker boxes denote significantly differed groups (*p < 0.001; for the significance level of differences see Supplementary Table 5).
The T3 content manifested a significant correlation with the blood glucose (r = 0.58; p = 0.0002), liver glycogen (r2 = 0.95; p < 0.0001), TAG in the plasma (r = 0.85; p < 0.0001) and muscles (r2 = 0.68; p < 0.0001), plasma proteins (r = 0.52; p = 0.0010), as well as the PHL in the plasma (r = 0.60; p = 0.0001) and muscles (r2 = 0.72; p < 0.0001). Thus, the T3 level was associated with the biochemical parameters responsible for the energy storage and transport, but not with the levels of hemoglobin and TBARS (r2 = 0.09; p > 0.05).
Charr Metabolic Phenotypes
Using CV analysis for the complex of biochemical parameters, we identified four morph-specific physiological phenotypes (niches), F33;231 = 43.12; p < 0.0001, Wilks’ Lambda = 0.0027, canonical R = 0.9813. In the CV space, LF occupied a remote position separating by the main root mainly due to the specific glycogen and muscle lipid levels (Figure 4 and Supplementary Table 6). BL, BD, and SM were ordinated mainly along the second root following their discrete slowdown in the metabolic rate. The liver glycogen, blood hemoglobin and plasma lipid content were the most different parameters among these charrs. An additional multivariate comparison of BL and BD confirmed their distinct metabolic phenotypes (Figure 4, incut).
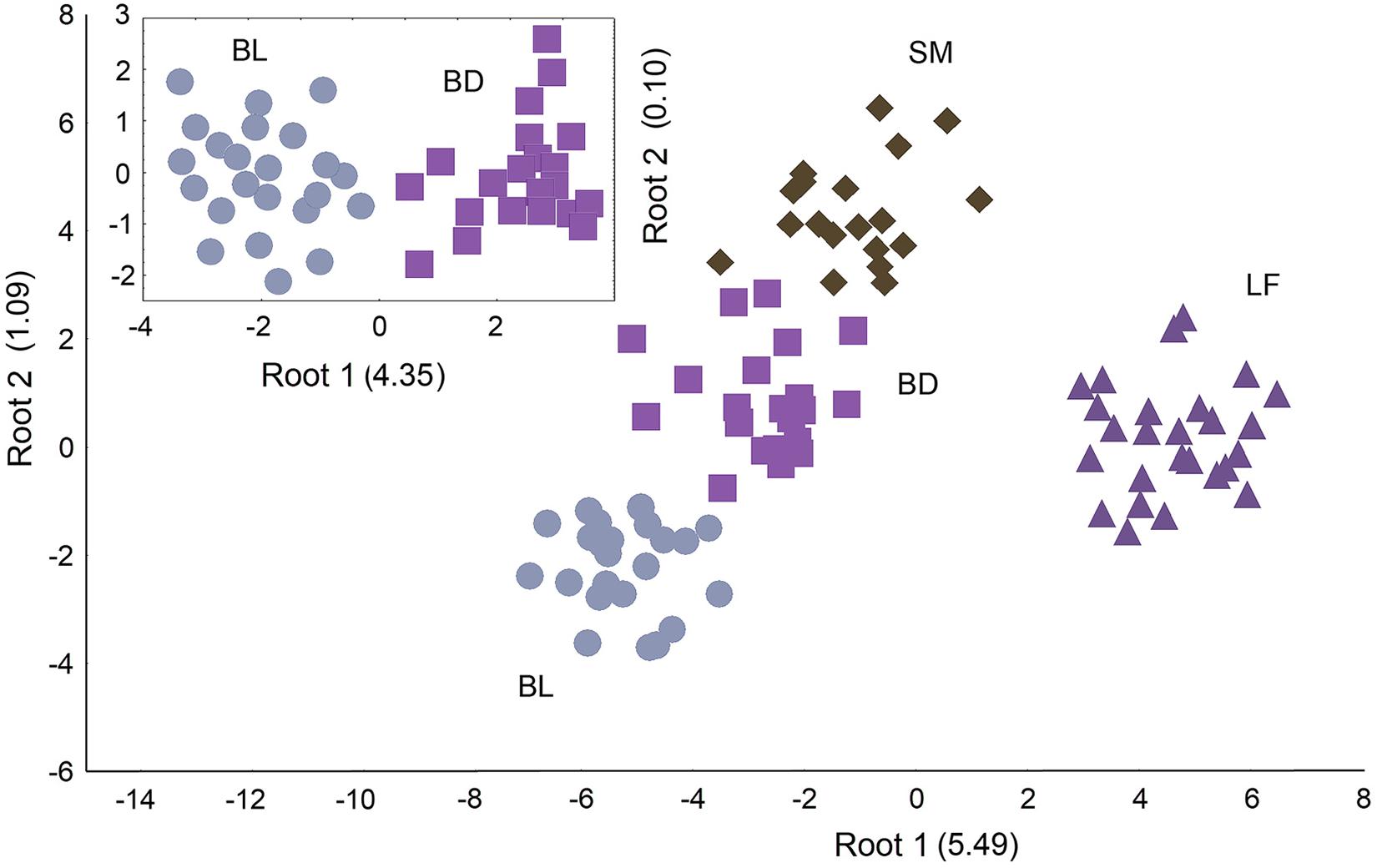
Figure 4. Results of physiological niche identification in the El’gygytgyn charrs via CV analysis of 11 biochemical parameters (the blood glucose, liver glycogen, blood hemoglobin, plasma protein, plasma lipid content,% of PHL and TAG in the plasma lipids, muscle lipid content,% of PHL and TAG in the muscle lipids, plasma TBARS). Incut figure represents the result of comparing two Boganida charrs separately from other species. The charr groups and the root eigen values are labeled.
Discussion
The Lake El’gygytgyn charrs survive at the edge of ecological tolerance for non-migratory freshwater fishes. The lake was covered with ice many times over the decades during the late Pleistocene – early Holocene (Melles et al., 2012). To date, the average annual air temperature over the lake surface is close to −9°C, which is lower than over Lake Hazen, the northernmost resident fish-inhabited lake, with the average surface temperature of approximately −5°C (France, 1993). Further to the north, non-annual ice breaking results in a sharp drop in the ecosystem productivity (Keatley et al., 2007), and the inability of lacustrine fishes to survive in these harsh conditions could be assumed.
A good indicator of fish fitness and performance in food acquisition is the proximate tissue composition (Beamish et al., 1989; Shearer, 1994; Heath, 1995; Speranza and Colombo, 2009). We found that all the El’gygytgyn charrs possess a specific common trait. They are very fatty: up to 37–53% (dry weight) and, therefore, adapted to the extremely cold conditions (White et al., 2012; DeLong et al., 2018). Lipids act as a resource store in ultra-oligotrophic ecosystems and also provide for the buoyancy essential for energy saving while swimming through the water column (Muir et al., 2014).
At the same time, the El’gygytgyn charrs differ in the ratio of biochemical parameters defining the dynamics of energy processing and storage, and biopolymer transport activity, which are related to the ecological differences in fish (Alexander, 1993). Following the authors describing the physiological discrepancies for the ecomorphs of various charr species (Proulx and Magnan, 2002; Goetz et al., 2014), we considered the revealed peculiarities of the El’gygytgyn charrs as the metabolic phenotypes corresponding to their ecological niches.
Long-finned charr (LF) occupies the unique niche – a deepwater amphipod-consumer capable of dwelling in anaerobic conditions. The increased hemoglobin level and decreased intensity of transporting lipid oxidation are inherent in this species. The last characteristic could be interpreted as the enhanced defense against oxidative stress, a likely adaptation to the low-oxygen conditions (Pollock et al., 2007; Debevec et al., 2017). Long-finned charr is also characterized by the substitution of carbohydrates for lipids in cellular respiration. The body fatness and TAG level in muscles and blood of this charr were out of scale.
The reduced metabolic rate and inactive lifestyle enable the energy saving necessary under the conditions of low food supply. Both features were characteristic of smallmouth charr (SM). Among the El’gygytgyn fish, this species possesses the lowest lipid level due to the planktivorous diet. In one respect, zooplankton contains approximately 21% (dry weight) of lipids as compared with about 14% in benthic insect larvae (Jobling, 1994b) and 15–20% in amphipods (Greze, 1977). Meanwhile, the main lipid storage product in many zooplankters is wax esters (Cavaletto et al., 1989; Brett et al., 2009), which are harder to digest than TAGs and PHLs from insects, amphipods, and fish prey. Thus, following Jobling (1994a), we suggest that the low lipid content found in the SM muscles is related to the presence of wax esters in its diet.
The piscivorous Boganida charrs are physiologically heterogeneous, but demonstrate an accelerated metabolism as compared with SM and LF. We found that Boganida charrs split into two discrete phenotypes related to the specific lifestyles: epilimnetic (BL) and deepwater (BD). The former displays high glycogen and PHL contents which allows considering it as more metabolically advanced than the latter. This finding goes along with the suggestion that metabolic level should be higher in fish that has to chase prey (Childress et al., 1990). At depth, where the visibility is reduced, the predator’s throw to prey is shorter, so the metabolism may be slower. Probably, BD hunts in an “ambush way,” and BL - in a “chasing way.” Moreover, the metabolic differences between the Boganida phenotypes were supported by the heterogeneity in the rate of their somatic growth (Chereshnev et al., 2002). Fast-growing BL is characterized by a higher plasma protein content, which can be associated with effective muscle anabolism (Houlihan et al., 1993).
The ecologically determined metabolic phenotypes of the El’gygytgyn charrs are related to their specific hormonal phenotypes. The metabolically advanced BL displays the highest level of thyroid hormones. The piscivorous but metabolically retarded BD has a significantly lower concentration of T3. The planktivorous SM characterized by slow metabolism exhibits a moderate level of T3 and a very low level of T4. The metabolically unique LF is the most hypothyroid El’gygytgyn fish. We also found sharp differences in the blood plasma biochemistry among the charrs, which is known to strongly correlate with the thyroid hormone status (Abdollahpour et al., 2019).
Given that the thyroid hormones are the key regulators of metabolism (Blanton and Specker, 2007; Lanni et al., 2016; Lema, 2020), the assumption that this endocrine axis determines the metabolic phenotypes of the El’gygytgyn charrs seems plausible. Moreover, the knowledge that the thyroid signaling links the ontogeny and metabolism to the environmental variables (Holzer and Laudet, 2015) allows proposing that this endocrine axis played a decisive role during the lake colonization and subsequent specialization of the charrs. Above all, a life-history shift from anadromy to fresh-water residency is associated with the changes in endocrinology. The salt-water migration decline leads to a number of consequences. In particular, it results in the loss of a parr-smolt transformation (Ferguson et al., 2019), which is regarded as a “pan-hyperendocrine state” [sensu Bern (1978) in McCormick (2013)], when the hormone synthesis consistently increases. In resident salmonid populations, the activity of some of the pivotal smolting endocrine axes (growth hormone and hypothalamic-pituitary-interrenal hormones) significantly decreases. In contrast, the thyroid axis, which is also regarded as a crucial smolting endocrine axis, does not change the activity and enhances the influence on development and physiology (McCormick et al., 2019). This finding allows us to speculate that the thyroid axis took on the role of the main endocrine factor orchestrating the developmental and physiological changes in the El’gygytgyn charrs. Initially, the thyroid hormones could act as the mediators providing for the development of thermal acclimation (Little et al., 2013) and specific lipid metabolism (Plisetskaya et al., 1983; Lanni et al., 2016; Deal and Volkoff, 2020) common for all El’gygytgyn charrs. Further, the thyroid axis most likely participated in the evolution of the species-specific traits.
Particularly, after the lake colonization, LF have been surviving and specializing under the long-term ice cover during at least four global climatic coolings of Pleistocene (Osinov et al., 2015). Stepwise “evolutionary shifts” (sensu Szappanos et al., 2016) led to the formation of the unique phenotype under the pressure of sequential cooling and low-oxygen conditions, each time pushing the population toward a deeper ecological specialization. As a result, LF evolved into a hypothyroid fish with a low metabolic rate, which is regarded as an adaptation to the low-oxygen and poor food environment (Childress and Seibel, 1998; Kitano et al., 2010; Weidner et al., 2020). Low hormonal level, which often leads to paedomorphosis in low vertebrates (Laudet, 2011), also affected morphology. LF possesses neotenic morphological traits (Alekseyev, 2000).
Following Lema (2020) considering the endocrine regulation as a crucial proximate mechanism initiating evolutionary adaptations, we presume the thyroid status differences to be one of the key factors underpinning the radiation of the Boganida charrs. We suggest that BD is an evolutionary young ecological morph radiating from BL, a morph strongly resembling ancestral anadromous Salvelinus taranetzi (Chereshnev et al., 2002; Osinov et al., 2015). Occupying the new ecological niche (deep-water predator) required the life-style and physiological transformations, which seem to be associated with the decrease of the thyroid axis activity. Thus, we consider the development of the El’gygytgyn charr diversity as a stepwise adaptive evolution of metabolic phenotypes driven by the thyroid axis in response to the environmental challenges.
Data Availability Statement
The original contributions presented in the study are included in the article/Supplementary Material, further inquiries can be directed to the corresponding author.
Ethics Statement
The animal study was reviewed and approved by Ethics committee of A.N. Severtsov Institute of Ecology and Evolution RAS.
Author Contributions
EE and FS conceived the manuscript idea. All authors collected the materials, developed the theory, discussed the results, performed the computations, wrote, and revised the manuscript.
Funding
This work was supported by Russian Science Foundation, Grant No. 18-74-10085.
Conflict of Interest
The authors declare that the research was conducted in the absence of any commercial or financial relationships that could be construed as a potential conflict of interest.
Publisher’s Note
All claims expressed in this article are solely those of the authors and do not necessarily represent those of their affiliated organizations, or those of the publisher, the editors and the reviewers. Any product that may be evaluated in this article, or claim that may be made by its manufacturer, is not guaranteed or endorsed by the publisher.
Acknowledgments
We would like to thanks G. A. Kroshkin for the helicopter ride to the lake.
Supplementary Material
The Supplementary Material for this article can be found online at: https://www.frontiersin.org/articles/10.3389/fevo.2021.715110/full#supplementary-material
References
Abdollahpour, H., Falahatkar, B., Efatpanah, I., Meknatkhah, B., and Van Der Kraak, G. (2019). Hormonal and physiological changes in Sterlet sturgeon Acipenser ruthenus treated with thyroxine. Aquaculture 507, 293–300. doi: 10.1016/j.aquaculture.2019.03.063
Alekseyev, S. S. (2000). Reduction of skeletal elements in Arctic charr Salvelinus alpinus from Transbaikalia and the origin of some morphological features of Svetovidov’s long-finned charr Salvethymus svetovidovi. J. Ichthyol. 40, 495–504.
Alexander, R. M. (1993). “Buoyancy,” in The Physiology of Fishes, 2nd Edn, ed. D. H. Evans (Boca Raton, FL: CRC Press), 75–97.
Beamish, F. W. H., Howlett, J. C., and Medland, T. E. (1989). Impact of diet on metabolism and swimming performance in juvenile lake trout, Salvelinus namaycush. Can. J. Fish. Aquat. Sci. 46, 384–388. doi: 10.1139/f89-050
Bern, H. A. (1978). “Endocrinological studies on normal and abnormal salmon smoltification,” in Comp. Endocrinol. eds P. J. Gaillard and H. H. Boer (Amsterdam: Elsevier/North Holland Biomedical Press), 77–100.
Blanton, M. L., and Specker, J. L. (2007). The hypothalamic-pituitary-thyroid (HPT) axis in fish and its role in fish development and reproduction. Crit. Rev. Toxicol. 37, 97–115. doi: 10.1080/10408440601123529
Brett, M. T., Müller-Navarra, D. C., and Persson, J. (2009). “Crustacean zooplankton fatty acid composition,” in Lipids in Aquatic Ecosystems, eds M. Kainz, M. T. Brett, and M. T. Arts (New York, NY: Springer), 115–146. doi: 10.1007/978-0-387-89366-2_6
Burstein, M., Scholnick, H. R., and Morfin, R. (1980). Rapid method for the isolation of lipoproteins from human serum by precipitation with polyanions. Scand. J. Clin. Lab. Invest. 40, 583–595. doi: 10.1016/s0022-2275(20)42943-8
Campinho, M. A. (2019). Teleost metamorphosis: the role of thyroid hormone. Front. Endocrinol. 10:383. doi: 10.3389/fendo.2019.00383
Cavaletto, J. F., Vanderploeg, H. A., and Gardner, W. S. (1989). Wax esters in two species of freshwater zooplankton. Limnol. Oceanogr. 34, 785–789. doi: 10.4319/lo.1989.34.4.0785
Chereshnev, I. A., and Skopets, M. B. (1990). Salvethymus svetovidovi gen. et sp. nova – a new endemic fish of the subfamily Salmoninae from Lake El’gygytgyn (Central Chukotka). Voprosy Ikhtiologii 30, 201–213.
Chereshnev, I. A., Volobuev, V. V., Shestakov, A. V., and Frolov, S. V. (2002). Salmonid fishes in Russian North-East. Vladivostok: Dal’nauka Press.
Childress, J. J., Price, M. H., Favuzzi, J., and Cowles, D. (1990). Chemical composition of midwater fishes as a function of depth of occurrence off the Hawaiian Islands: food availability as a selective factor? Mar. Biol. 105, 235–246. doi: 10.1007/bf01344292
Childress, J. J., and Seibel, B. A. (1998). Life at stable low oxygen levels: adaptations of animals to oceanic oxygen minimum layers. J. Exp Biol. 201(Pt 8), 1223–1232. doi: 10.1242/jeb.201.8.1223
Cossins, A. R., and Prosser, C. L. (1978). Evolutionary adaptation of membranes to temperature. Proc. Natl. Acad. Sci. U.S.A. 75, 2040–2043. doi: 10.1073/pnas.75.4.2040
Deal, C. K., and Volkoff, H. (2020). The role of the thyroid axis in fish. Front. Endocrinol. 11:596585. doi: 10.3389/fendo.2020.596585
Debevec, T., Grégoire, P. M., and Pialoux, V. (2017). Hypoxia-induced oxidative stress modulation with physical activity. Front. Physiol. 8:84. doi: 10.3389/fphys.2017.00084
DeLong, J. P., Bachman, G., Gibert, J. P., Luhring, T. M., Montooth, K. L., Neyer, A., et al. (2018). Habitat, latitude and body mass influence the temperature dependence of metabolic rate. Biol. Lett. 14:0442. doi: 10.1098/rsbl.2018.0442
Esin, E. V., Markevich, G. N., Melnik, N. O., Kapitanova, D. V., and Shkil, F. N. (2021). Natural toxic impact and thyroid signaling interplay orchestrates riverine adaptive divergence of salmonid fish. J. Anim. Ecol. 90, 1004–1019. doi: 10.21203/rs.3.rs-15718
Ferguson, A., Reed, T. E., Cross, T. F., McGinnity, P., and Prodöhl, P. A. (2019). Anadromy, potamodromy and residency in brown trout Salmo trutta: the role of genes and the environment. J. Fish. Biol. 95, 692–718. doi: 10.1111/jfb.14005
Folch, J., Lees, M., and Sloan-Stanley, G. H. (1957). A simple method for the isolation and purification of total lipides from animal tissues. J. Biol. Chem. 226, 497–509. doi: 10.1016/s0021-9258(18)64849-5
France, R. L. (1993). The Lake Hazen trough: a late winter oasis in a polar desert. Biol. Conserv. 63, 149–151. doi: 10.1016/0006-3207(93)90503-s
Freeman, N. K., Lindgren, F. T., and Nichols, A. V. (1963). “The chemistry of serum lipoproteins,” in Progress in the Chemistry of Fats and Other Lipids, eds R. T. Holman, W. O. Lundberg, and T. Malkin (NewYork, NY: The MacMillan Comp.), 215–250.
Frolov, S. V. (1993). Unique karyotype and constitutive heterochromatin in the endemic Salmoninae fish Salvethymus svetovidovi. J. Ichthyol. 33, 42–49.
Gammon, A., and Baker, S. J. (1977). Studies in methods of hemoglobin estimation suitable for use in public health programmes. Ind. J. Med. Res. 65, 150–156.
Gavrilov, V. B., Gavrilova, A. R., and Mazhul’, L. M. (1987). Analysis of methods for determination of lipid peroxidation products in blood serum in the thiobarbituric acid test. Vopr. Med. Khim. 1, 118–121.
Glushkova, O. Y. (2001). Geomorphological correlation of Late Pleistocene glacial complexes of Western and Eastern Beringia. Quat. Sci. Rev. 20, 405–417. doi: 10.1016/S0277-3791(00)00108-6
Goetz, F., Jasonowicz, A., Johnson, R., Biga, P., Fischer, G., and Sitar, S. (2014). Physiological differences between lean and siscowet Lake trout morphotypes: are these metabolotypes? Can. J. Fish. Aquat. Sci. 71, 427–435. doi: 10.1139/cjfas-2013-0463
Greze, I. I. (1977). Amfipody Chernogo Morya Ikh Biologiya [Amphipods of the Black Sea and Their Biology]. Kiev: Noukova Dumka.
Guderley, H. (2004). Metabolic responses to low temperature in fish muscle. Biol. Rev. 79, 409–427. doi: 10.1017/s1464793103006328
Harrison, B., Leazenby, C., and Halldorsdottir, S. (2011). Accuracy of the CONTOUR® blood glucose monitoring system. J. Diabetes Sci. Technol. 5, 1009–1013. doi: 10.1177/193229681100500425
Holzer, G., and Laudet, V. (2015). Thyroid hormones: a triple-edged sword for life history transitions. Curr. Biol. 25, R344–R347. doi: 10.1016/j.cub.2015.02.026
Houlihan, D. F., Mathers, E. M., and Foster, A. (1993). “Biochemical correlates of growth rate in fish,” in Fish Ecophysiology, eds J. C. Rankin and F. B. Jensen (London: Chapman & Hall), 45–71. doi: 10.1007/978-94-011-2304-4_2
Keatley, B. E., Douglas, M. S. V., and Smol, J. P. (2007). Limnological characteristics of a High Arctic oasis and comparisons across northern Ellesmere Island. Arctic 60, 294–308.
Kitano, J., Lema, S. C., Luckenbach, J. A., Mori, S., Kawagishi, Y., Kusakabe, M., et al. (2010). Adaptive divergence in the thyroid hormone signaling pathway in the stickleback radiation. Curr. Biol. 20, 2124–2130. doi: 10.1016/j.cub.2010.10.050
Knicht, J. A., Anderson, S., and Rawle, J. M. (1972). Chemical basis of the sulfo-phospho-vanillin reaction for estimating total serum lipids. Clin. Chem. 18, 199–202. doi: 10.1093/clinchem/18.3.199
Lanni, A., Moreno, M., and Goglia, F. (2016). Mitochondrial actions of thyroid hormone. Compr. Physiol. 6, 1591–1607. doi: 10.1002/cphy.c150019
Laudet, V. (2011). The origins and evolution of vertebrate metamorphosis. Curr. Biol. 21, R726–R737.
Lema, S. C. (2020). Hormones, developmental plasticity, and adaptive evolution: endocrine flexibility as a catalyst for ‘plasticity-first’ phenotypic divergence. Mol. Cell. Endocrinol. 502:110678. doi: 10.1016/j.mce.2019.110678
Little, A. G., Kunisue, T., Kannan, K., and Seebacher, F. (2013). Thyroid hormone actions are temperature-specific and regulate thermal acclimation in zebrafish (Danio rerio). BMC Biol. 11:26. doi: 10.1186/1741-7007-11-26
Masanori, N., and Shizunori, I. (1971). Effects of sarvation and dietary composition on the blood glucose level and the hepatopancreatic glycogen and lipid contents in carp. Nippon Suisan Gakkaishi. 37, 404–409.
McCormick, S. D. (2013). “Smolt physiology and endocrinology,” in Euryhaline Fishes, eds S. D. McCormick, A. P. Farrell, and C. J. Brauner (Cambridge, MA: Academic Press), 199–251. doi: 10.1016/b978-0-12-396951-4.00005-0
McCormick, S. D., Regish, A. M., Ardren, W. R., Björnsson, B. T., and Bernier, N. J. (2019). The evolutionary consequences for seawater performance and its hormonal control when anadromous Atlantic salmon become landlocked. Sci. Rep. 9:968. doi: 10.1038/s41598-018-37608-1
McMenamin, S. K., and Parichy, D. M. (2013). Metamorphosis in teleosts. Curr. Top. Dev. Biol. 103, 127–165. doi: 10.1016/b978-0-12-385979-2.00005-8
Melles, M., Brigham-Grette, J., Minyuk, P. S., Nowaczyk, N. R., Wennrich, V., DeConto, R. M., et al. (2012). 2.8 Million years of Arctic climate change from Lake El’gygytgyn, NE Russia. Science 337, 315–320. doi: 10.1126/science.1222135
Muir, A. M., Bronte, C. R., Zimmerman, M. S., Quinlan, H. R., Glase, J. D., and Krueger, C. C. (2014). Ecomorphological diversity of Lake charr Salvelinus namaycush at Isle Royale, Lake Superior. Trans. Am. Fish. Soc. 143, 972–987.
Nelson, J. S., Grande, T. C., and Wilson, M. V. (2016). Fishes of the World, 5th Edn. London: John Wiley & Sons.
Nolan, M., and Brigham-Grette, J. (2007). Basic hydrology, limnology, and meterology of modern Lake El‘gygytgyn, Siberia. J. Paleolimnol. 37, 17–35. doi: 10.1007/s10933-006-9020-y
Oleinik, A. G., Skurikhina, L. A., Kukhlevsky, A. D., and Bondar, E. I. (2019). Genetic differentiation of the Arctic phylogenetic group of charr from Northeast Asia and North America. Rus. J. Genet. 55, 349–359. doi: 10.1134/s1022795419030116
Oleinik, A. G., Skurikhina, L. A., Kukhlevsky, A. D., and Semenchenko, A. A. (2021). The first complete mitochondrial genomes of two species of charr, Salvelinus boganidae and Salvelinus elgyticus, from Lake El’gygytgyn (Chukotka). Polar Biol. 44, 1209–1217. doi: 10.1007/s00300-021-02861-0
Osinov, A. G., Senchukova, A. L., Mugue, N. S., Pavlov, S. D., and Chereshnev, I. A. (2015). Speciation and genetic divergence of three species of charr from ancient Lake El’gygytgyn (Chukotka) and their phylogenetic relationships with other representatives of the genus Salvelinus. Biol. J. Linn. Soc. 116, 63–85. doi: 10.1111/bij.12559
Osinov, A. G., and Volkov, A. A. (2020). On the origin of two species of charrs (Salvelinus, Salmonidae) in Lake El’gygytgyn: data on the ATPase6-NADH4L region of mitochondrial DNA. J. Ichthyol. 60, 618–625. doi: 10.1134/S003294522004013X
Plath, M., Tobler, M., and Rüdiger, R. (2015). “Extremophile fishes: an introduction,” in Extremophile Fishes. Ecology, Evolution, and Physiology of Teleosts in Extreme Environments, eds R. Riesch, M. Tobler, and P. Martin (Switzerland: Springer International Publishing), 1–7. doi: 10.1007/978-3-319-13362-1_1
Plisetskaya, E., Woo, N. Y. S., and Murat, J.-C. (1983). Thyroid hormones in cyclostomes and fish and their role in regulation of intermediary metabolism. Comp. Biochem. Physiol. A Physiol. 74, 179–187. doi: 10.1016/0300-9629(83)90586-8
Polakof, S., Panserat, S., Soengas, J. L., and Moon, T. W. (2012). Glucose metabolism in fish: a review. J. Compar. Physiol. Part B 182, 1015–1045. doi: 10.1007/s00360-012-0658-7
Pollock, M. S., Clarke, L. M. J., and Dube, M. G. (2007). The effects of hypoxia on fishes: from ecological relevance to physiological effects. Environ. Rev. 15:6. doi: 10.1139/a06-006
Proulx, R., and Magnan, P. (2002). Physiological performance of two forms of lacustrine brook charr, Salvelinus fontinalis, in the open-water habitat. Environ. Biol. Fish. 64, 127–136. doi: 10.1023/a:1016049820846
Regerand, T. I., Lizenko, E. I., Petrovsky, V. I., and Sidorov, V. S. (1990). Isolation of serum lipoproteins by precipitation and determination of their lipid composition. Lab. Delo. 4, 48–52.
Rehulka, J., Minarik, B., Adamec, V., and Rehulkova, E. (2005). Investigations of physiological levels of total plasma protein in Rainbow trout, Oncorhynchus mykiss (Walbaum). Aquac. Res. 36, 22–32.
Shearer, K. D. (1994). Factors affecting the proximate composition of cultured fishes with emphasis on salmonids. Aquaculture 119, 63–88. doi: 10.1016/0044-8486(94)90444-8
Smith, P. K., Krohn, R. I, Hermanson, G. T., Mallia, A. K., Gartner, F. H., Provenzano, M. D., et al. (1985). Measurement of protein using bicinchoninic acid. Anal. Biochem. 150, 76–85. doi: 10.1016/0003-2697(85)90442-7
Speranza, E. D., and Colombo, J. C. (2009). Biochemical composition of a dominant detritivorous fish Prochilodus lineatus along pollution gradients in the Paraná-Río de la Plata Basin. J. Fish. Biol. 74, 1226–1244. doi: 10.1111/j.1095-8649.2009.02191.x
Szappanos, B., Fritzemeier, J., Csörgő, B., Lázár, V., Lu, X., Fekete, G., et al. (2016). Adaptive evolution of complex innovations through stepwise metabolic niche expansion. Nat. Commun. 7:11607. doi: 10.1038/ncomms/11607
Takeyama, M., Itoh, S., Nagasaki, T., and Tanimizu, I. (1977). A new enzymatic method for determination of serum choline-containing phospholipids. Clin. Chem. Acta 79, 93–98. doi: 10.1016/0009-8981(77)90465-x
Templeton, M. C. (1961). Microdetermination of Glycogen with Anthrone Reagent. Evanston, IL: Northwestern University, Dept. Medical Anatomy, 670–672.
Trinder, P. (1969). Estimation of triglyceride in blood GPO-PAP enzymatic method. Am. Clin. Biochem. 6, 24–27.
Weidner, J., Håkonsrud, J. C., Giske, J., Eliassen, S., and Jørgensen, C. (2020). Hormones as adaptive control systems in juvenile fish. Biol. Open 9:bio046144. doi: 10.1242/bio.046144
Keywords: metabolic phenotype, evolutionary divergence, thyroid status, Arctic, salmonids, charrs
Citation: Esin EV, Markevich GN, Zlenko DV and Shkil FN (2021) Thyroid-Mediated Metabolic Differences Underlie Ecological Specialization of Extremophile Salmonids in the Arctic Lake El’gygytgyn. Front. Ecol. Evol. 9:715110. doi: 10.3389/fevo.2021.715110
Received: 26 May 2021; Accepted: 15 October 2021;
Published: 10 November 2021.
Edited by:
Marco António Campinho, Universidade do Algarve, PortugalReviewed by:
Paolo Sordino, Stazione Zoologica Anton Dohrn Napoli, ItalyRodrigo Nunes Da Fonseca, Federal University of Rio de Janeiro, Brazil
Copyright © 2021 Esin, Markevich, Zlenko and Shkil. This is an open-access article distributed under the terms of the Creative Commons Attribution License (CC BY). The use, distribution or reproduction in other forums is permitted, provided the original author(s) and the copyright owner(s) are credited and that the original publication in this journal is cited, in accordance with accepted academic practice. No use, distribution or reproduction is permitted which does not comply with these terms.
*Correspondence: Evgeny V. Esin, evgesin@gmail.com