- Department of Biology, Advanced Facility for Avian Research, Western University, London, ON, Canada
The influence of the social environment on the timing of the annual cycle is poorly understood. Seasonally migratory birds are under pressure to accurately time their spring migration, and throughout the annual cycle, they may experience variability of the local sex-ratio. A population-level male-biased sex ratio is predicted to advance spring migration timing in males and is attributed to the increased intra-specific competition for access to females and/or breeding territories. The present study had two goals. First, to develop a method that utilizes digitally coded radio-transmitters to quantify the activity of flocked individuals in captivity. Second, to use this method to test the hypothesis that the social environment influences the spring migration traits of male yellow-rumped warblers (Setophaga coronata coronata). To accomplish this, birds were captured in the fall in Long Point, Ontario, and transferred to the Advanced Facility for Avian Research, London, Ontario. In the winter, they were assigned to a slightly male- or female-biased treatment and housed in flocks in large free-flight rooms. Throughout the experimental period, we took body mass measurements and standardized photos to monitor body condition and molt progression. To measure locomotor activity, the birds were outfitted with digitally coded radio-transmitters in April and photo-triggered to enter a migratory phenotype. The tagged birds were released at their capture site in May and the Motus Wildlife Tracking System was used to determine stopover departure timing and migratory movements. Sex ratio did not influence body mass or molt progression. However, males from the male-biased treatment had significantly less locomotor movement than those from the female-biased treatment. Additionally, a lower proportion of males from the male-biased treatment initiated migratory restlessness, an indicator of the urge to migrate. Overall, these findings suggest that the social environment can influence behavior of songbirds, but do not support the hypothesis that a male-biased sex ratio accelerates migration.
Introduction
Migratory birds that traverse between geographically distinct breeding and non-breeding locations each year are under immense pressure to accurately time their seasonal events and processes. The temporal precision of successive events is essential for fitness maximization. For instance, early arrival at the breeding grounds allows for acquisition of higher quality territories, which results in better mating and increased breeding success (Møller, 1994; Hasselquist, 1998; Smith and Moore, 2005). This is, however, balanced by the potential hazards and costs associated with early spring arrival such as scarce resources and food supplies or severe weather conditions (Brown and Bomberger Brown, 2000; Pulido, 2007). Any rush or delay in the schedule of events may have consequences for the future timing of life history events and performance of individuals – so called carry-over effects (Catry et al., 2013; Saino et al., 2017; de Zwaan et al., 2019; Gow et al., 2019). Determining what drives the timing of seasonal events and processes has important implications for understanding how animals may respond to future environmental change (Helm et al., 2006).
Migratory birds exhibit adaptive phenotypic changes throughout the annual cycle, notably vocal communication, feather molt, fat gain, and other traits associated with migration (e.g., Figure 1). The initiation, sequence, and expression of such changes is believed to be endogenously programmed (e.g., Gwinner, 1986; Piersma et al., 2008; Maggini and Bairlein, 2010; Pedersen et al., 2018) because birds can sustain this cycle for over 10 years in a laboratory setting with no external timing cues (Gwinner, 1996). In nature, however, birds will additionally use components of the environment to precisely synchronize their seasonal behavior with the time of year (Åkesson and Helm, 2020). The annual change in photoperiod has been identified as a primary cue (Gwinner, 1989; Hau et al., 1998; Wingfield, 2008; Kumar et al., 2010). Individuals may then fine-tune their schedules in relation to other components of the physical environment such as food availability, weather, and temperature. For instance, poor winter food supply typically delays spring migration departure (Studds and Marra, 2011; Cooper et al., 2015), the presence of favorable winds may trigger departure (Åkesson et al., 2002; Dossman et al., 2016; Morbey et al., 2018; Beauchamp et al., 2020), and in autumn, increased temperature may delay departure (Berchtold et al., 2017) from breeding and stopover sites.
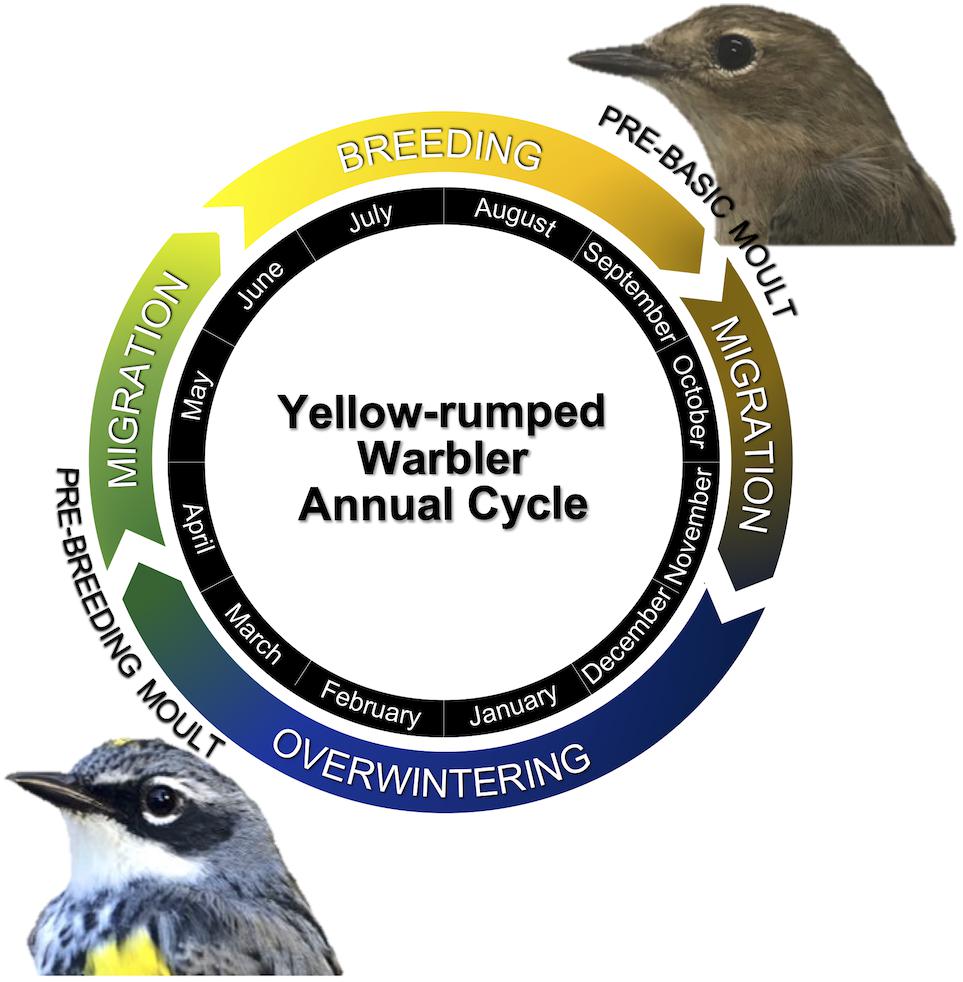
Figure 1. Schematic of the annual cycle of yellow-rumped warblers (Setophaga coronata coronata). Pictured is the typical appearance of a yellow-rumped warbler in breeding plumage and in pre-basic (non-breeding) plumage.
Besides the physical environment, birds may also extract predictive information from the social environment (reviewed by Helm et al., 2006). Birds seek and integrate information from others (“social information”) to make decisions. Social information arises from cues that are produced inadvertently by individuals and from direct signals (i.e., traits shaped by selection to modify the behavior of the receiver). Birds can use social cues in spatial decision making such as which stopover site or breeding site to choose (Nocera et al., 2006; Betts et al., 2008; Alessi et al., 2010; Ward et al., 2010). Conspicuous signals, on the other hand, are often used in temporal decision making such as coordinating migratory movements and synchronizing behaviors. For instance, individuals of some flocking species will signal in the form of vocal calls before initiating migration and during flight, which is believed to recruit conspecifics and to maintain cohesion (Hamilton, 1962; Black, 1988; Piersma et al., 1990). Signals can also be used to actively communicate seasonal disposition. Song, breeding plumage, and ornaments, for instance, are used by birds in courtship and territorial displays signaling their readiness to mate, social status, and/or competitive ability (Bortolotti et al., 2006). In all cases, variability in social information exchange may contribute to seasonal timing differences.
Sex ratio is an important aspect of the social environment that may influence migration decisions (Kokko et al., 2006). Throughout the annual cycle birds may experience different sex-biased ratios. For instance, males often precede females at stopover sites and at breeding destinations, termed protandry (e.g., Francis and Cooke, 1986; Spina et al., 1994; Wobker et al., 2021), which is believed to be the result of male-male competition for access to breeding territories (Kokko et al., 2006). There appear to be multiple non-mutually exclusive proximate causes of protandry (reviewed by Coppack and Pulido, 2009) including migration distance, where males may overwinter further north than female conspecifics (Ketterson and Nolan, 1976; Komar et al., 2005). Another proximate cause may be migration duration, where males have a shorter duration as a result of faster flight speed or shorter stopovers (Hedeström and Pettersson, 1986; Seewagen et al., 2013; Morbey et al., 2018). Earlier male departure from the non-breeding grounds may also contribute to protandrous spring arrival (Marra et al., 1998; Maggini and Bairlein, 2012; Briedis et al., 2019; Schmaljohann, 2019) and has an endogenous basis. This is demonstrated by the fact that in captivity, males will begin nocturnal migratory restlessness (“zugunruhe,” a suite of behaviors in captive individuals characterized by wing whirring and hopping at night that corresponds to migration departure timing in wild conspecifics) earlier on average than female conspecifics (Holberton, 1993; Deakin et al., 2019), even in the absence of environmental cues such as photoperiod (Maggini and Bairlein, 2012). Regardless of the proximate cause, the variation in the timing of migratory movements results in natural variation in sex ratio throughout spring migration.
The yellow-rumped warbler (“Myrtle warbler”; Setophaga coronata coronata) provides an ideal model organism to explore the influence of the social environment on spring migration timing. The lifecycle follows a typical pattern of seasonally migrating warblers (Figure 1). It is a small (10–12 g), socially monogamous, medium distance, nocturnally migrating songbird of the family Parulidae (“wood warblers”). It is perhaps the most common warbler inhabiting an expansive geographic range throughout North America. Yellow-rumped warblers occur in large flocks (sometimes >50 individuals) in winter in the south-eastern United States and into Central America, the Bahamas and Greater Antilles (Wunderle and Waide, 1993). Like many species of songbird, they exhibit latitudinal sexual segregation during the winter, with males found in greater proportions further north than females (Komar et al., 2005).
The current study had two goals. The first was to test whether automated radio telemetry could be used to quantify individual diurnal and nocturnal activity of birds held in captivity in flocks. Activity of captive birds in individual cages has been measured effectively in a number of ways such as trip switches attached to perches (Wagner, 1930), infrared sensors (Helm and Gwinner, 2006; Coverdill et al., 2011; Vandermeer, 2013; Berchtold et al., 2017), video analysis (Ramenofsky and Agatsuma, 2006; Deakin et al., 2019), ultrasonic activity detectors and sound (Maggini and Bairlein, 2010; Eikenaar and Bairlein, 2014; Bulte et al., 2017). However, these technologies are less practical for tracking nocturnal activity for individual birds in flocks. For free living birds, activity has been measured with accelerometers which record for later download, however, we could not find an accelerometer logger that could continuously monitor activity in high resolution for weeks and was small and light enough for approximately 12 g birds. Radio telemetry tags can also be used to quantify activity using patterns of signal strength variation (Morbey et al., 2018, 2020). We chose to use digitally coded radio telemetry tags to measure activity because (1) they are very small and light (0.3 g), (2) all birds can be monitored continuously because the tags all transmit on a common radio frequency, (3) they do not require removal for download because the receiver logs activity data, and (4) they can remain on birds after release to the wild so that subsequent activity and migratory behavior can be tracked in the field. However, to our knowledge no previous study has used this methodology to detect migratory restlessness or measure activity of captive birds.
Our second goal was to explore whether modified social environments influence the spring migration traits of male yellow-rumped warblers. For this we experimentally manipulated the sex ratio of flocked birds in captivity during the winter and spring migration seasons. We measured traits associated with spring migration including feather molt, body mass gain, locomotor activity, and the onset of migratory restlessness, and then released the birds at the original capture site to measure stopover departure timing, and post-departure migratory movements. These traits were only measured in the males since biased-sex ratios are expected to influence the migratory timing of the sexes differently (Kokko et al., 2006). As a result of increased male-male competition, we predicted that birds inhabiting a male-biased environment would have a greater expression of traits associated with spring migration timing, represented as mass, molt, and locomotor activity. We also predicted that they would have an accelerated departure, represented by the onset of migratory restlessness and departure timing post-release.
Materials and Methods
Birds were captured under scientific collection permits from the Canadian Wildlife Service (SC-OR-2018-0256 and 10169BU). All animal methods complied with guidelines of the Canadian Council on Animal Care and were approved by the University of Western Ontario Animal Use Sub-committee (Protocol # 2018-092).
Study Site, Capture, and Housing Conditions
Yellow-rumped warblers were captured on Long Point, Ontario (43.0103°N, −81.2799°) with mist-nets in Sept–Oct 2018 and brought to the Advanced Facility for Avian Research at the University of Western Ontario. Upon arrival, they were kept in indoor mixed sex aviaries and on a light cycle of 12 h light and 12 h dark (12L:12D) to mimic the day length conditions experienced at their capture site during migration and to maintain them in a photorefractory stage (unresponsive to photoperiod or changes in photoperiod that normally elicit a behavioral and physiological response). The birds were used for different studies in the fall related to diet (Denommé et al., 2021). Blood samples were collected from the brachial vein to molecularly determine sex using a PCR approach followed by gel electrophoresis for visualization of the bands (Morbey et al., 2018), and age class was determined based on plumage characteristics (Pyle, 1997). Throughout the duration of their time in captivity, the birds were fed a synthetic diet ad libitum supplemented with mealworms (Tenebrio molitor) and experienced room temperatures of 20 ± 2°C.
Experimental Treatments and Triggering Spring Migration
We conducted our experiment over a 4-month period, from 16 January to 8 May 2019, which encompasses the non-breeding and spring migration life history stages. For our experiment, we had access to 19 males and 27 females and five, large, indoor rooms (240 cm × 360 cm). For this reason, we decided to place a similar number of male birds in the male and female biased treatment rooms, while maintaining relatively similar densities of birds in each room. The total sample size of males in the male biased social environment was 10 and the total sample size of males in the female biased social environment was 9. On the first day of the experiment, all birds were randomly assigned to slightly male-biased or female-biased experimental treatments; two male-biased rooms comprised of 5 males: 4 females, two female-biased rooms comprised of 3 males: 5 females, and an additional female-biased room comprised of 3 males: 9 females. Unfortunately, these social environments deviated from an initial study design which would have had additional room replicates. However, this was not considered a serious flaw, because differences in social environment were still present among rooms. Each room contained numerous natural tree branches as perching substrates, multiple food and water dishes, and were separated by thick concrete so the birds were not exposed to the social environments of the other rooms.
In the northern hemisphere in spring, for instance, lengthening days stimulate physiological and behavioral changes associated with migration and reproduction while shortening days delay such changes. In captivity, photoperiod can be used to stimulate birds to transition between life cycle stages. To do this, we reduced the light cycle to 9L:15D on 16 January. This photophase is shorter than that experienced at the most northern overwintering latitude of this species and thus acts as an unambiguous indicator of winter for all individuals, ensuring that all the birds became photosensitive. On 24 April 2019, the birds were photostimulated to enter a migratory state by increasing the light cycle to 15.28L:8.72D, which matches the photoperiod of the projected release date and median date of arrival (4 May) of male yellow-rumped warblers at Long Point over the past 10 years (Long Point Bird Observatory, 2008). The times of lights on and off corresponded to civil dawn and civil dusk of Long Point on the day of their planned release, 4 May 2019. All photoperiods used in this study were determined using an Excel VBA (Greg Pelletier, NOAA Sunrise/Sunset and Solar Position Calculators1).
Body Condition and Molt
After breeding and prior to southerly fall migration, yellow-rumped warblers undergo a pre-basic molt of their body plumage that results in feathers that are dull brown and gray in color (Figure 1; Pyle, 1997). They will then undergo a pre-breeding (“pre-alternate”) molt prior to departure for spring migration (Feb–Apr) that results in a breeding phenotype of brighter colors (Pyle, 1997), including a black patch on the cheeks. In addition, they become hyperphagic and considerably increase mass up to 4.5 g to fuel their migration (Moore and Simm, 1985). Consequently, we took body mass (±0.01 g; CS200, OHAUS, Parsippany, NJ, United States) measurements of the male birds approximately every 2 weeks from February until their release to monitor body mass over time. We also took standardized photographs (Canon EOS Rebel T3i2) once a month to monitor feather molt progression. We used the “Threshold Color” tool from the particle analysis software, ImageJ (Schneider et al., 2012), to measure amount of black plumage covering the face and throat of the birds. For this method, a threshold is set to tell the object of interest apart from the background. First, we measured the total number of pixels covering the face and throat and then we measured the number of pixels within that area that were specifically black. Dividing the number of black pixels by the total number of pixels gave us a % coverage of black plumage.
Locomotor Activity
Automated Very High Frequency telemetry was used in captivity to assess the day and night locomotor activity of the birds. On 21 April 2019, the males were weighed, photographed, and harnessed with a 0.29 g digitally encoded nano radio-transmitter (model NTQB2-1, 12.7 s burst rate, approximate battery life 62 days, Lotek Wireless3), and returned to their respective aviaries. The transmitters were attached via a modified over-the-leg loophole harness (Rappole and Tipton, 1991) made out of elastic material designed to fall off after prolonged exposure to field conditions. A Lotek SRX600 data logging receiver was placed in a hallway directly adjacent to the five aviary rooms and was used to collect locomotor activity for each bird by continuously and simultaneously detecting signal pulses (Figure 2A).
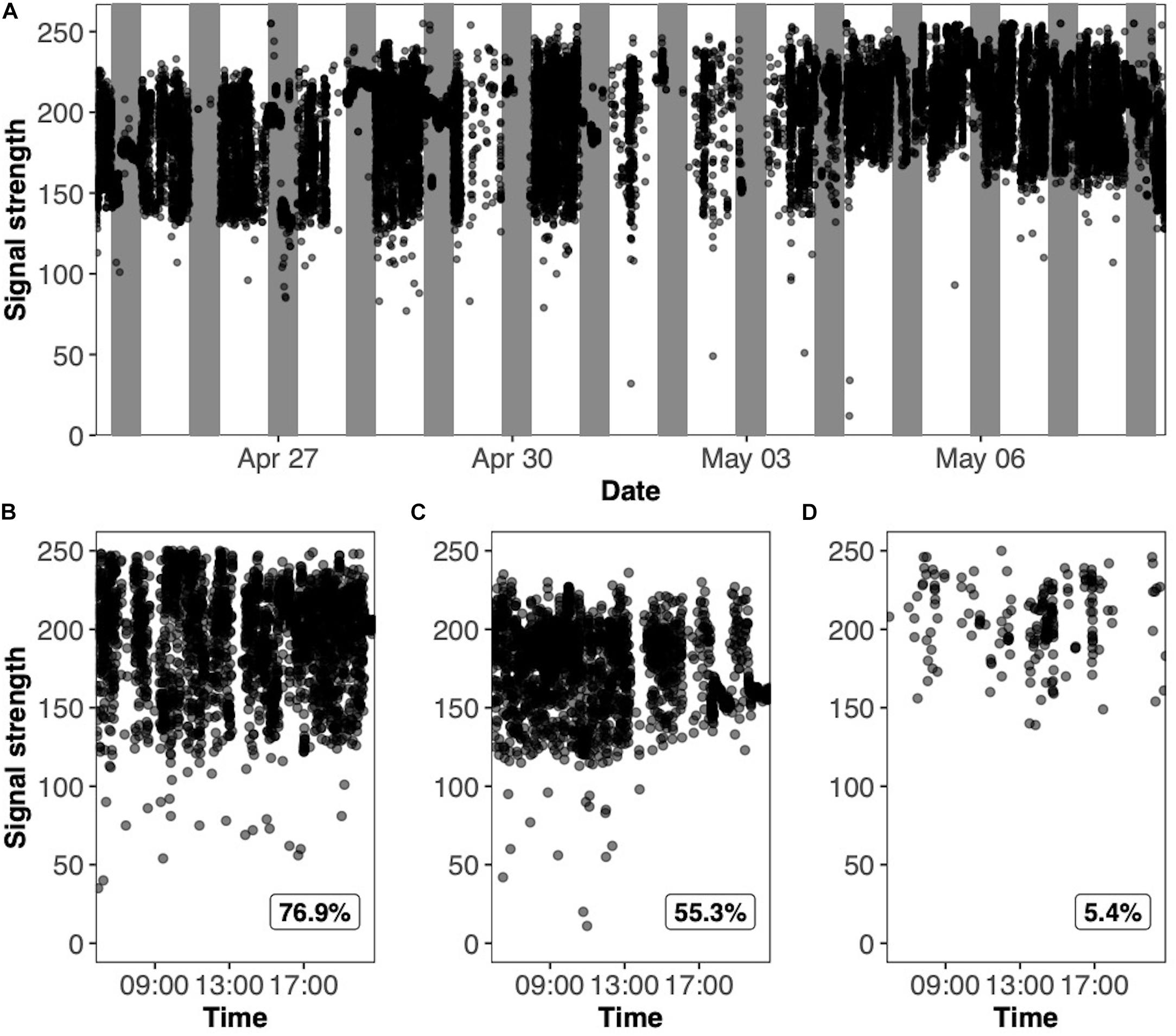
Figure 2. Activity traces of a radio-tagged yellow-rumped warbler (Setophaga coronata coronata) in captivity. Each data point represents a detection of the radio-tag by the Lotek receiver. The variation in consecutive detections indicates the movement pattern of the bird. High variation represents a bird that is highly active, while low variation represents no activity (i.e., sleeping or no locomotor movement). Panel (A) shows the activity trace of an individual throughout the duration of spring, where the white segments represent the day sections (lights-on), and the gray segments represent the night sections (lights-off). Panels (B–D) are activity traces demonstrating various signal coverages where the percent signal coverage is outlined in the bottom righthand corner of each plot.
For the activity analyses, we excluded data from the first 5 min and the last 1.5 min of the night. Data from the first 5 min was excluded to allow birds time to adjust to the abrupt light switch. Data from the last 1.5 min was excluded to ensure detections for daytime movement were not included in the night analysis. The time of lights on and lights off corresponded to the “day” and “night” periods. Signal coverage was determined by dividing the actual number of detections of radio pulses from individual birds by the theoretical maximum number of detections based on the tag pulse rate and the duration of a given period. For each period, a threshold for signal coverage was set to 5% in order for the data to be included in the statistical analyses. This threshold was chosen because coverages less than 5% would have too few datapoints and therefore would not be particularly meaningful for measuring activity changes (Figures 2B–D). We quantified the birds’ locomotor activities for each day and night period using two approaches which were intended to separately encompass relatively large and fine-temporal scale behaviors. First, the coefficient of variation (CV) was calculated as the ratio of the standard deviation of detections to the mean of detections for each bird and period. This is a common statistical metric used to measure the dispersion of data series around the mean of the population. A higher CV is indicative of more movement (more variability in strength of detection) over the course of an entire day or night period and a lower CV is indicative of less movement. Second, we calculated the change in signal strength between consecutive detections (hereafter referred to as “CSS”) (Morbey et al., 2020). Consecutive detections were those that occurred within at least 30 s from each other. This metric represents the general wakefulness of the bird and may encompass behaviors such as wing whirring during migratory restlessness. A higher CSS value represents greater movement, while a lower CSS represents less movement.
Post-release Departure Timing and Movement
On 8 May 2019, the radio-harnessed birds were photographed and weighed for the final time (minus the mass of their transmitter), transported for approximately 1.5 h to their original capture site on Long Point, and simultaneously released at 14:20 Eastern Daylight Time (EDT). We chose to delay the original planned release date by 4 days for two reasons. First, we examined the receiver data to evaluate how many birds had begun displaying migratory restlessness. We believed that delaying the release date would potentially allow for a greater proportion of the birds to begin exhibiting restlessness, which is indicative of their urge to migrate. Second, there was predicted severe weather on the original release date consisting of thunderstorms and high winds and we did not want the birds to experience this weather immediately upon release from their captive artificial environment. Following previous studies (Deakin et al., 2019; Seewagen et al., 2019), we considered the release to simulate arrival at a stopover site. Both previous studies captured birds during fall migration, held them in captivity over winter, and released them at the same site we used on Long Point in the spring. Upon release, the birds behaved similarly to wild conspecifics at stopover and migrated in the appropriate northward direction (Deakin et al., 2019; Seewagen et al., 2019). Motus Wildlife Tracking System (Motus), a network of automated telemetry receivers (Taylor et al., 2017), was used to determine the length of stay at the stopover site and to reconstruct the birds’ migratory trajectory paths post-departure. A bird was considered to be present at the site in Long Point if it was detected by the receiver station >50 times in a day and the length of stay (days) was calculated as the last day present minus the release day plus 1 (Seewagen et al., 2019).
Statistical Analysis
We used R version 3.6.2 for all analyses in this study. Significance was accepted for all tests when p < 0.05. A linear mixed effect model was used to determine whether body mass and body mass gain during the experimental period differed between treatments (male- and female-biased social environment) using the function lme from the package nlme (Pinheiro et al., 2018). In the model, treatment, date, and the treatment ∗ date interaction were included as fixed effects, where date was treated as a continuous covariate. Random intercepts and slopes among individuals and rooms were specified to allow for individual differences in mass gain over time and the model was fit using maximum likelihood (ML). If non-significant, the interaction was dropped before assessing the main effects. A linear mixed effect model was used to test whether overwintering sex ratio affected the molt (percent black coverage) and molt progression of the birds. In the model, treatment, date, and the treatment ∗ date interaction were included as fixed effects, where date was treated as a continuous covariate. Random intercepts and slopes among individuals and rooms were specified to allow for individual differences in molt progression over time and the model was fit using ML. For both the body mass and molt models, visual inspection of residual plots revealed no violations of linearity or normality and the absence of heteroskedasticity.
We used linear mixed effects models to determine if overwintering sex ratio affected the day or night activity of yellow-rumped warblers using both the CV and CSS activity metrics. The models included treatment (male- or female-biased sex ratio) as the fixed effect. As random effects, we had intercepts for bird ID and room. The models were fit using ML. Visual inspection of residual plots revealed no violations of linearity or normality and the absence of heteroskedasticity. To assess the significance of the fixed effects for the mass, molt, and activity models, we obtained p-values from likelihood ratio tests of the full model with the fixed effect in question against a model without the fixed effect in question. For the night activity, we also fit additional models with the CV and CSS activity metrics that only included data from birds prior to their onset of migratory restlessness (Supplementary Material).
In most cases, the onset of migratory restlessness is recognizable upon visual inspection of the individual activity graphs and/or CSS graphs. This is evident by the high variability in detections during a night period. The onset of migratory restlessness was estimated using the night period CSS values for each bird. We calculated a baseline level of CSS as the median 99th percentile on the first night under the assumption that the birds had not begun displaying migratory restlessness. The onset of migratory restlessness was defined for each bird as the first night it exceeded the baseline percent of CSS for greater than 4% of the night (∼20 min of the night). This threshold accurately matched the onset as determined by visual inspection of the graphs. We first compared the onset of migratory restlessness between treatment groups using Kaplan Meier survival analysis with the survdiff function from the survival package (Therneau, 2021). We then conducted Cox Proportional Hazard regression with the coxph function from the survival package (Therneau, 2021). In this model, we tested whether treatment and body mass measured on the day of photostimulation was associated with the onset of migratory restlessness. Birds that failed to reach the criteria for onset of migratory restlessness were assigned an onset date of 8 May and included in the models as right censored, which means that their true onset date is either equal to or greater than 8 May.
The length of stay (days) at the simulated stopover site was compared between the treatment groups using Kaplan Meier survival analysis. We also compared the length of stay between treatment groups using Cox Proportional Hazard regression so that body mass measured on the day of release could be in included in the survival model.
We used generalized linear models with binomial error distribution to test whether the post-release tag detection probability differed between treatment groups and over time using the function glmer from the package lme4 (Bates et al., 2015). Motus data from the first 5 h post-release was binned into 15-min intervals and for each interval, birds were assigned either 1 for present or 0 for absent. A bird was assumed to be present during a 15-min interval if it was detected by the receiver station at least 10 times. This conservative threshold was chosen to exclude any birds that may have been located near the edge of the detection area, flying into and out of range of the receiver. In the model, treatment, time, and a treatment ∗ time interaction were included as the fixed effects and tag ID as a random effect. Flight paths beyond the release site were plotted with the function ggmap from the package ggplot2 (Wickham, 2016). There were too few detections beyond the release site to statistically compare movement attributes between the treatment groups, so we characterized the movement paths qualitatively including a description of how many individuals were detected beyond the release site, which direction they had flown, time of detection, and length of detection.
Results
Locomotor Activity
We successfully quantified the individual locomotor activity of 19 radio-tagged male yellow-rumped warblers housed in captivity using automated radio telemetry. Visual observation of actograms demonstrates that periods of high activity are represented by large variation in signal strength while periods of low activity are represented by small variation in signal strength (Figure 2A). Eleven night periods and one daytime period were removed from the analysis for not meeting the 5% signal coverage criteria.
Yellow-rumped warblers’ day and night locomotor activity was influenced by sex ratio (Figure 3). During the day, the coefficient of variation (CV) of birds exposed to the male-biased treatment was 0.035 ± 0.016 (95% C.I.) lower than the birds exposed to the female-biased treatment [X2(1) = 6.77, P = 0.009; Figure 3A]. Likewise, the change in signal strength between consecutive detections (CSS) was 2.68 ± 1.97 (95% C.I.) lower in the birds exposed to the male-biased treatment [X2(1) = 5.25, P = 0.020]. The difference in locomotor activity was even greater at night, with birds exposed to the male-biased treatment having a 0.069 ± 0.040 (95% C.I) lower CV than those exposed to the female-biased treatment [X2(1) = 8.00, P = 0.005; Figure 3B]. Similarly, the birds exposed to the male biased treatment had a 1.58 ± 1.36 (95% C.I.) lower CSS at night [X2(1) = 4.52, P = 0.03]. The marginal coefficient of determination for the daytime activity was 0.29 and 0.16 for the CV and CSS models, respectively, and for the night activity was 0.12 and 0.11. The conditional coefficient of determination for the daytime activity was 0.52 and 0.54 for the CV and CSS models, respectively, and for the night activity was 0.24 and 0.45.
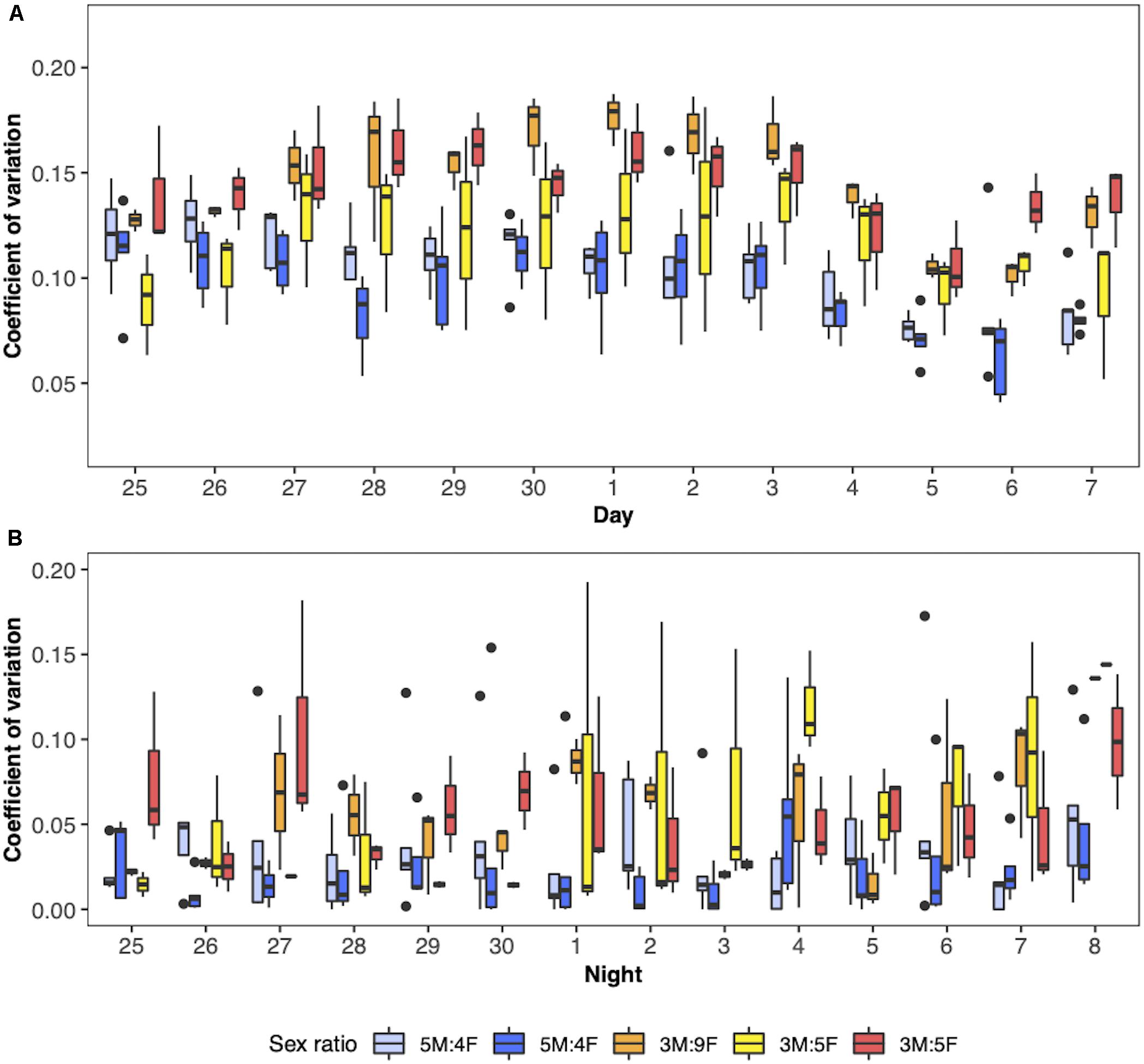
Figure 3. Boxplots showing the activity of male yellow-rumped warblers (Setophaga coronata coronata) in the spring. Data for day activity is shown in panel (A) and for the night in panel (B). Activity is represented as the coefficient of variation where a higher value indicates more movement. The x-axis labels correspond to dates between 25 April and 8 May 2019. Different colors represent the different rooms and the legend outlines the corresponding sex ratio treatment as males: females (M:F). The lower and upper hinges of each boxplot represent the 25th and 95th percentiles, a line is shown at the median value, and individual points fall outside of these values.
Onset of Migratory Restlessness
During the 2 weeks the birds were exposed to a simulated spring light cycle, many reached our criteria for displaying migratory restlessness. From the male-biased treatment 60% of the birds reached the criteria, while 89% reached the criteria from the female-biased treatment. Including the right censored birds, the median onset of migratory restlessness was 13.5 days for the male-biased treatment and 7 days for the female-biased treatment (Figure 4). However, the onset of migratory restlessness was not significantly different between treatments (βmale–biased = − 1.1 ± 0.6, hazard ratio = 0.34, P = 0.057) and did not depend on body mass measured on the day the birds were photostimulated (β = 0.1 ± 0.5, hazard ratio = 1.1, P = 0.85).
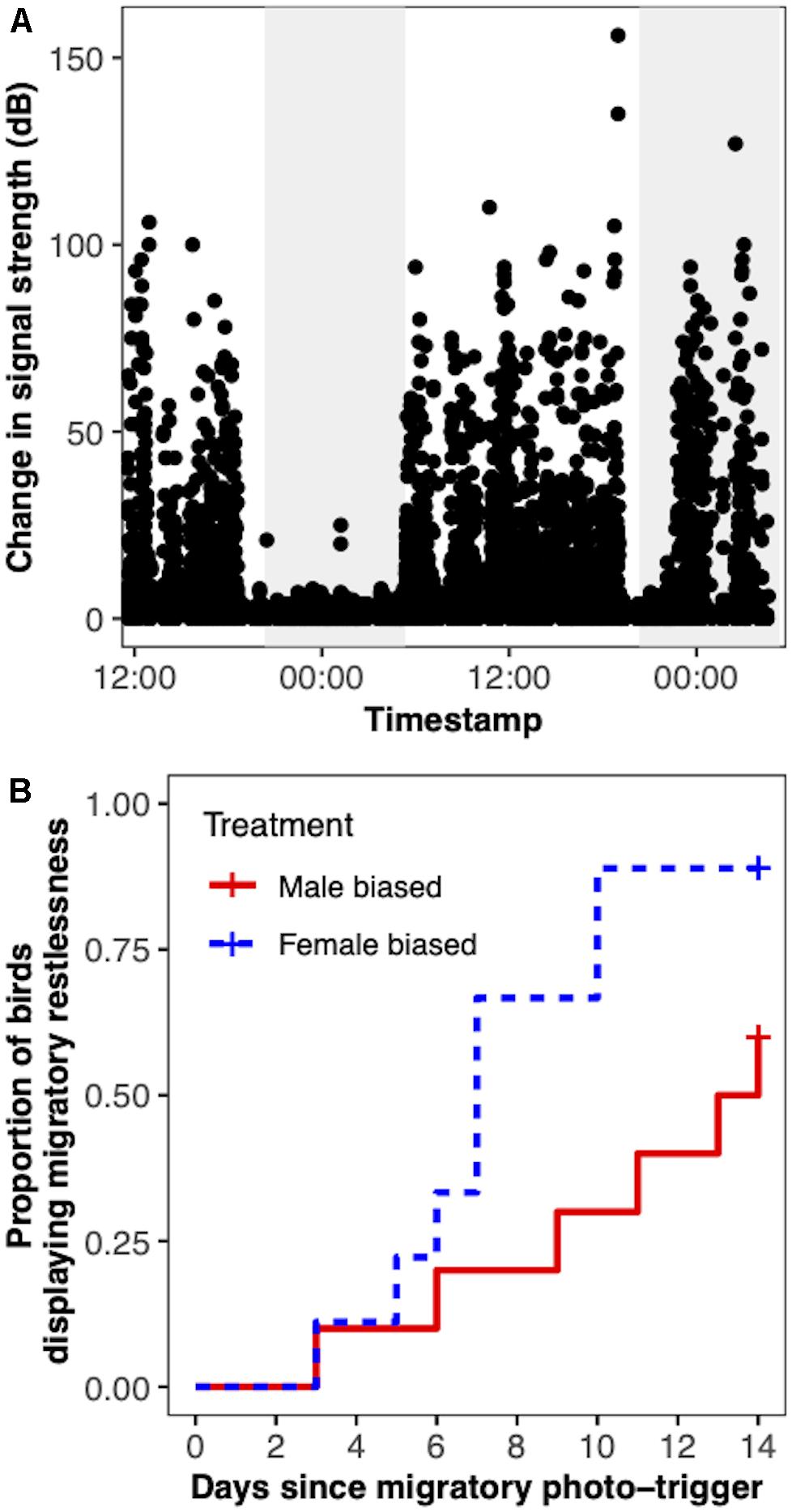
Figure 4. Calculating onset of Zugunruhe in yellow-rumped warblers (Setophaga coronata coronata) using change in signal strength (CSS). Panel (A) demonstrates the pattern of CSS that arises in a bird that is not moving (e.g., sleeping) versus when it is moving. A higher value of CSS corresponds to more movement. The gray panel represents the night section (lights-off), and the white represents the day section (lights-on). In this example, the bird is likely sleeping during the night section labeled with a (−) because CSS remains low throughout the night. The bird is likely displaying migratory restlessness in the night section labeled with a (+) because CSS remains high throughout the night. Panel (B) shows the survival curve of the onset of Zugunruhe between birds exposed to a female- or male-biased social environment.
Body Condition and Molt
The body mass of the birds significantly changed over the course of the experimental period (F(1,92) = 24.07, P < 0.0001; Figure 5) and this change was similar for both treatments (F(1,92) = 0.23, P > 0.5). There was no overall difference between the treatments (F(1,17) = 0.37, P > 0.5). When we compared the body mass of the birds on the day of their release, we found no difference between the treatment groups (t17 = 1.14, P = 0.27). The amount of black plumage covering the face of the yellow-rumped warblers changed throughout the experimental period (F(1,73) = 40.6, P < 0.0001; Figure 6). The change over time was similar for both treatments (F(1,73) = 0.46, P = 0.5) and overall, the treatment groups did not differ in amount of black plumage (F(1,17) = 1.31, P = 0.27).
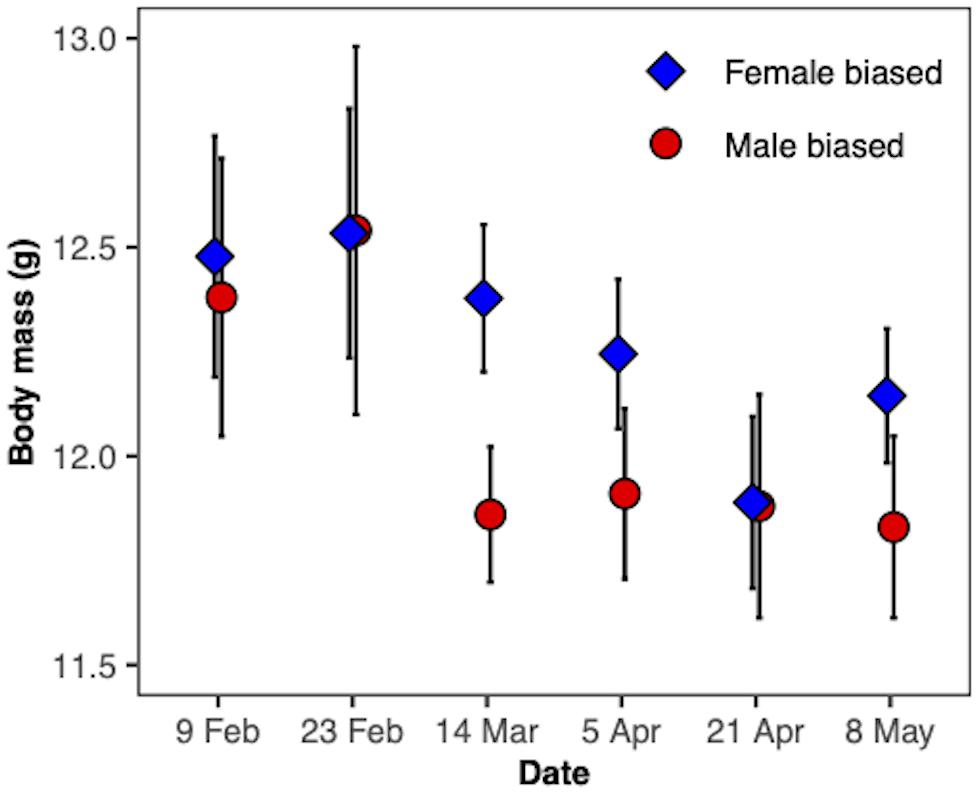
Figure 5. Mean body mass ± SE (g) of male yellow-rumped warblers (Setophaga coronata coronta). The birds were photostimulated to enter a migratory state on 21 April and released back to the wild on 8 May 2019.
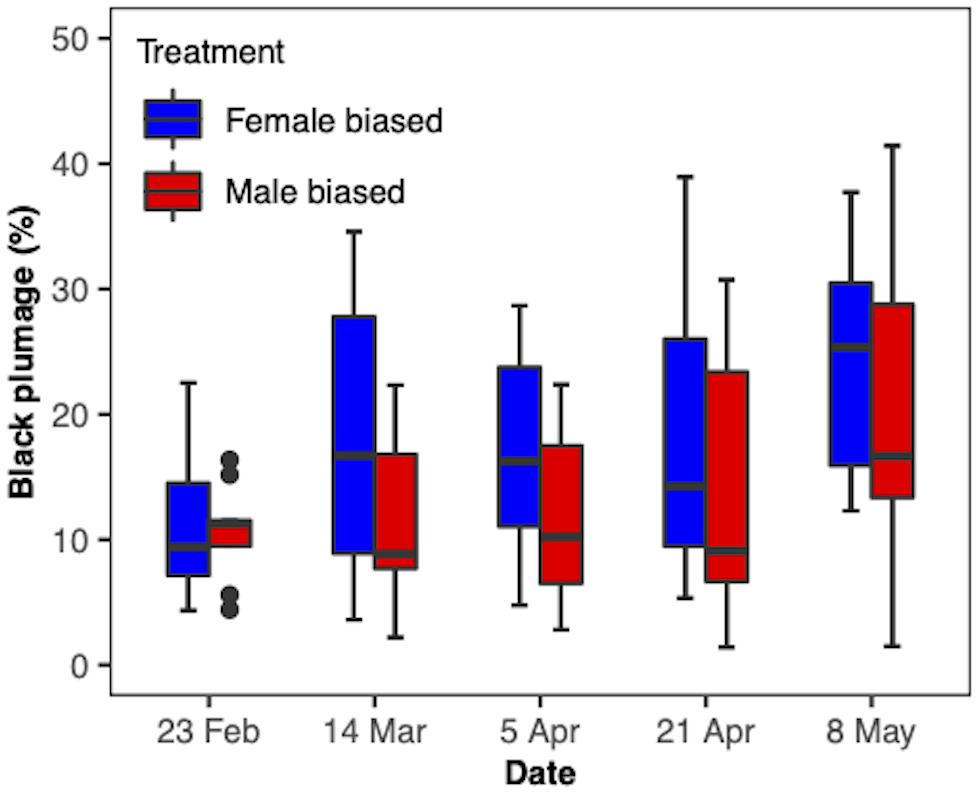
Figure 6. Amount of black plumage covering the face and neck of male yellow-rumped warblers (Setophaga coronata coronata) over time.
Post-release Movement
Immediately upon release the birds stayed in the vicinity of the Old Cut tower and were observed exploring the area and gleaning for insects. As time proceeded, the birds began to move away from the vicinity of the tower. Over the first 5 h post-release the odds of detection in a 15-min period by the Old Cut tower decreased by 29.0 ± 0.2% (95% C.I. = 0.6–0.8%) and this decrease was similar for both treatments. More than half of the birds departed on the first day of release (5 from female-biased and 5 from male-biased) and an additional 5 had departed within the first week (2 female-biased and 3 male-biased). The remaining 3 birds departed 9 (female-biased), 11 (female-biased), and 16 (male-biased) days post-release. One of the birds from the female-biased treatment was removed from the analysis because it had too many dubious detections (low probability of being a true detection) which was determined using the motusFilter function from the motus package (Brzustowski and LePage, 2021). Overall, the length of stay was the same between the treatment groups (βmale–biased = −0.13 ± 0.49, hazard ratio = 0.88, P > 0.5) and the distribution of the length of stay at the release site was the same (P > 0.05). The length of stay was negatively associated with mass measured on the release date (β = 1.02 ± 0.43, hazard ratio = 2.79, P = 0.02). We detected 22% of the birds from the male-biased treatment group (N = 2) and 75% (N = 6) of the birds from the female-biased treatment beyond the release site (Figure 7). All the detections beyond the release site were in the appropriate northward direction. Most of these detections (22/24) represent a nocturnal migratory flight because the detection time was very short, ranging from 50 s to 9.1 min. The remaining 2 detections were from two birds from the male-biased treatment and lasted 61 min and 1.75 days. Moreover, most of the detections beyond the release site (20/24) occurred at night between 21:58 and 04:05 h EDT with peak detections occurring between 22:00 and 01:34 h EDT. The fate of the 11 birds that were not detected beyond the release site could not be determined.
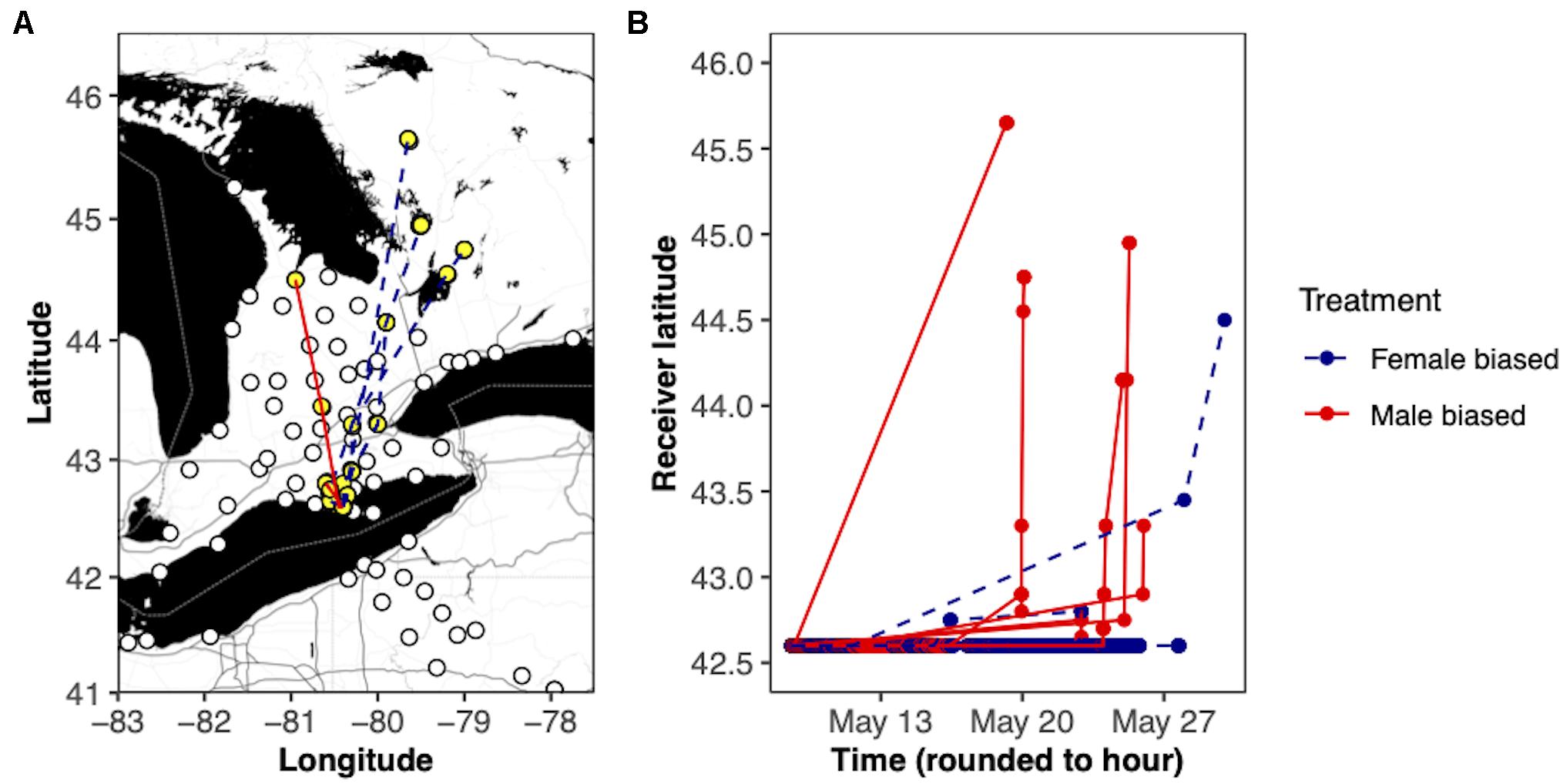
Figure 7. Movement of yellow-rumped warblers (Setophaga coronata coronata) after being released at simulated stopover on Long Point, ON, on 8 May 2019. Panel (A) shows the movement paths of the birds after departing Long Point. The white circles represent active receiver stations in the region at the time of the study. The yellow circles and associated connected lines represent receiver stations with detections and the individual tracks. Panel (B) shows the movement timing of the birds. For both panels, birds from the female biased treatment are represented by the blue dashed lines and the birds from the male biased treatment by the red solid lines.
Discussion
Males exposed to a male-biased sex ratio had lower day and night locomotor activity than males exposed to a female-biased sex ratio, implying that the social environment can influence behavior. Sex is an important component of the social spacing system of songbirds during the non-breeding season. In many species of Parulidae wood warblers, for instance, males and females have often been shown to occupy different habitats (Lynch et al., 1985; Marra, 2000; Bennett et al., 2019). In some species, males and females remain in mixed-sex flocks but maintain a difference in microhabitat use. Such is the case in black-throated blue warblers (Setophaga caerulescens), where males forage more frequently in mid-canopy to canopy while females forage more often near the ground (Cooper et al., 2021). Sometimes sexual habitat segregation is driven by choice (e.g., Morton et al., 1987), but oftentimes it is driven by dominance hierarchies where dominant males actively exclude females from superior habitats (i.e., greater quality and abundance of food, lower risk of predation, and/or better shelter) (e.g., Marra, 2000). In our study, the intrasexual competition for “superior habitat” locations within our treatment rooms may have been greater for the males exposed to the male-biased sex ratio. This could result in an overall smaller territory size for each male compared to the males exposed to the female-biased sex ratios, whom would presumably have comparably reduced intrasexual competition for territories. A reduction in territory size would explain the reduced locomotor movement in the male-biased treatment groups assuming the birds had no- or minimal-overlap of territories.
Fewer birds from the male-biased sex ratio treatment reached the criteria for the onset of migratory restlessness, suggesting that the males in the male-biased treatment did not have a relatively increased urge to migrate compared to males from the female-biased treatment as was predicted. Perhaps more interesting is that males from the female-biased treatment displayed migratory restlessness on average 7.5 days after photostimulation, which is relatively quick. Previous studies have found that yellow-rumped warblers can take up to 3 weeks to develop migratory restlessness after a change in photoperiod in captivity (Moore and Simm, 1985). There are two potential hypotheses that could explain our results. The female-biased sex ratio may have been a cue of being “late.” Yellow-rumped warblers display protandry during spring migration (Long Point Bird Observatory, 2008). As a result, males will naturally experience a male-biased sex ratio during migration which would continue as they migrate north and persist until the females arrive at the breeding grounds. Thus, it is possible that being exposed to excess females would act as a cue of being late in the progression of their northward migration. Correspondingly, the male-biased sex ratio may act as a cue of being “on-time” because the males would normally experience these male-biased ratios in the wild. This could explain why many of the birds from the male-biased treatment group had not begun displaying migratory restlessness within the 2 weeks we examined; they were simply not ready to migrate.
Both treatment groups had similar body mass throughout the experiment and the gain in mass for migration was also similar. The birds also had a similar progression of molt. In the wild, birds occupying female-biased habitats typically have reduced body mass (e.g., Studds and Marra, 2005; Cooper et al., 2021), lower muscle scores (Latta and Faaborg, 2002), and higher baseline corticosterone levels (Marra and Holberton, 1998) than birds from male-biased habitats. These results suggest that birds from male-biased habitats are in better condition than birds from female-biased habitats. The birds in our study, however, experienced ad libitum food and had zero threat of predation. The ad libitum food allowed the birds to maintain a relatively high body mass throughout the simulated winter and spring seasons. Thus, it is possible that the influence of sex ratio on body condition was masked in our captive experiment.
In our study, there was no relationship between body mass and the onset of migratory restlessness. However, there was a negative relationship between body mass measured on the release date and length of stay at the simulated stopover. Birds with a higher body mass had a shorter length of stay. This is similar to previous studies that have demonstrated a positive relationship between body mass and probability of departing (Goymann et al., 2010; Seewagen and Guglielmo, 2010; Lupi et al., 2016), measured using migratory restlessness (Maggini and Bairlein, 2010) and stopover departure timing (Goymann et al., 2010; Seewagen and Guglielmo, 2010).
All the birds departed or moved away from the release site on Long Point, despite some of the birds being released prior to exhibiting migratory restlessness. This suggests that there was no negative implication of being released at the simulated stopover despite not exhibiting migratory restlessness. Both treatments had similar length of stay and the probability of detection within the first 5 h from the Old Cut Motus tower was also similar. Within the first hour post-release, however, the birds from the male-biased treatment had a constant probability of detection while the males from the female-biased treatment had a 33% decrease in probability of detection. The detectable range of the Old Cut tower for forest dwelling birds, including the yellow-rumped warbler, is approximately 600 m (Crewe et al., 2019). This suggests that within the first hour, birds from the female-biased treatment departed or moved away from the release site by at least 600 m and those from the male-biased treatment remained within this detectable range. The Old Cut tower is situated in the middle of a peninsula and so the birds that were not detected by the tower would either have had to make a flight north/northeast over Lake Erie or follow the peninsula to the mainland toward the west. Both situations would represent a departure or movement away from the release site. Overall, these results are comparable to our results we found in captivity, where males from the female-biased treatment groups had greater locomotor activity. It is likely that the sex-biased treatment was alleviated shortly after time of release since wild yellow-rumped warblers were present at the release site. This could explain why we saw a difference between the treatments in movement immediately (within an hour) post-release but not afterward.
We detected 8 out of 19 birds beyond the release site. Although this detection rate appears low, it is similar to a previous study with yellow-rumped warblers at our study site that had a detection rate of approximately 41% (10 out of 24 birds) (Seewagen et al., 2019). In our study, all the detections of birds beyond the release site were in the appropriate northward direction despite being held in captivity overwinter. Furthermore, most detections beyond the release site occurred at night which is comparable to a previous study on yellow-rumped warblers (Seewagen et al., 2019). Overall, these results suggest that we were successful at photostimulating the birds to enter a spring migratory phenotype and that they behaved in a comparable manner to wild conspecifics.
Adult sex ratio (proportion of males in the population) is poorly described for most bird species. However, a review by Donald (2007), suggests that skewed adult sex ratios are common and averages 0.57 (males/females). Measuring sexual segregation in wild populations is a challenging task because of the large spatial scales involved, the difficulty in distinguishing the sexes using external morphology, and other potential sampling bias (Donald, 2007; Amrhein et al., 2012). Males are often more visually conspicuous and more vocal and can therefore be detected more readily than females. More captive experiments with known sex ratios are needed to advance our understanding of the role of the social environment on spring migration. Further experimentation should include studies with increased sample sizes and the inclusion of additional sex ratios, such as more extreme male-biased treatments and a male only treatment.
Here, we used a method that streamlines the process of quantifying locomotor activity of flocked individuals in a controlled environment followed by the assessment of timing and movement attributes in the wild. Using our novel method, we tested whether the spring migration traits of male yellow-rumped warblers are influenced by the social environment. Overall, our findings suggest that the social environment can influence migratory behavior but our hypothesis that male-biased sex ratios would advance spring migration was largely unsupported.
Data Availability Statement
The original contributions presented in the study are included in the article/Supplementary Material, further inquiries can be directed to the corresponding author.
Ethics Statement
The animal study was reviewed and approved by University of Western Ontario Animal Use Sub-committee.
Author Contributions
JD conceived and designed the study, collected and analyzed the data, and drafted the manuscript. YM and CG supervised the work and reviewed and edited the manuscript. All authors contributed to the article and approved the submitted version.
Funding
Contributions from YM and CG were supported by NSERC Discovery Grants. Contributions from JD were supported by Queen Elizabeth II Scholarship in Science and Technology and NSERC PGS-D.
Conflict of Interest
The authors declare that the research was conducted in the absence of any commercial or financial relationships that could be construed as a potential conflict of interest.
Publisher’s Note
All claims expressed in this article are solely those of the authors and do not necessarily represent those of their affiliated organizations, or those of the publisher, the editors and the reviewers. Any product that may be evaluated in this article, or claim that may be made by its manufacturer, is not guaranteed or endorsed by the publisher.
Acknowledgments
We thank Long Point Bird Observatory for supplying Canadian Migration Monitoring Network data, and all the volunteer participants who helped in gathering data for the project. We would also like to acknowledge the staff and volunteers at Long Point Bird Observatory for their help in collection of the birds used in this study. Thank you to Greg Mitchell and Environment and Climate Change Canada for providing the radio-tags used in this study. Finally, we thank Dana Nguyen, Melanie Denommé, and Michela Rebuli for their very appreciated help with bird care and Adriana del Pilar Caicedo Argülles for assistance with radio-tagging.
Supplementary Material
The Supplementary Material for this article can be found online at: https://www.frontiersin.org/articles/10.3389/fevo.2021.715418/full#supplementary-material
Footnotes
References
Åkesson, S., and Helm, B. (2020). Endogenous Programs and Flexibility in Bird Migration. Front. Ecol. Evol. 8:78. doi: 10.3389/fevo.2020.00078
Åkesson, S., Walinder, G., Karlsson, L., and Ehnbom, S. (2002). Nocturnal migratory flight initiation in reed warblers Acrocephalus scirpaceus: effect of wind on orientation and timing of migration. J. Avian Biol. 33, 349–357.
Alessi, M. G., Benson, T. J., and Ward, M. P. (2010). Nocturnal social cues attract migrating Yellow-breasted Chats. Wilson J. Ornithol. 122, 780–783. doi: 10.1676/10-040.1
Amrhein, V., Scaar, B., Baumann, M., Minéry, N., Binnert, J., and Korner-Nievergelt, F. (2012). Estimating adult sex ratios from bird mist netting data. Methods Ecol. Evol. 3, 713–720.
Bates, D., Maechler, M., Bolker, B., and Walker, S. (2015). Fitting linear mixed-effects models using lme4. J. Stat. Softw. 67, 1–48. doi: 10.18637/jss.v067.i01
Beauchamp, A. T., Guglielmo, C. G., and Morbey, Y. E. (2020). Stopover refuelling, movement and departure decisions in the white-throated sparrow: the influence of intrinsic and extrinsic factors during spring migration. J. Anim. Ecol. 89, 2553–2566. doi: 10.1111/1365-2656.13315
Bennett, R. E., Rodewald, A. D., and Rosenberg, K. V. (2019). Overlooked sexual segregation of habitats exposes female migratory landbirds to threats. Biol. Conserv. 240:108266. doi: 10.1016/j.biocon.2019.108266
Berchtold, A., Nightingale, I., Vandermeer, C., and MacDougall-Shackleton, S. A. (2017). Experimental temperature manipulations alter songbird autumnal nocturnal migratory restlessness. Anim. Migr. 4, 1–7. doi: 10.1515/ami-2017-0001
Betts, M. G., Hadley, A. S., Rodenhouse, N., and Nocera, J. J. (2008). Social information trumps vegetation structure in breeding-site selection by a migrant songbird. Proc. R. Soc. B Biol. Sci. 275, 2257–2263. doi: 10.1098/rspb.2008.0217
Black, J. M. (1988). Preflight signaling in swans: a mechanism for group cohesion and flock formation. Ethology 79, 143–157.
Bortolotti, G. R., Blas, J., Negro, J. J., and Tella, J. L. (2006). A complex plumage pattern as an honest social signal. Anim. Behav. 72, 423–430. doi: 10.1016/j.anbehav.2006.01.016
Briedis, M., Bauer, S., Adamík, P., Alves, J. A., Costa, J. S., Emmenegger, T., et al. (2019). A full annual perspective on sex-biased migration timing in long-distance migratory birds. Proc. R. Soc. B Biol. Sci. 286:20182821. doi: 10.1098/rspb.2018.2821
Brown, C. R., and Bomberger Brown, M. (2000). Weather-mediated natural selection on arrival time in cliff swallows (Petrochelidon pyrrhonota). Behav. Ecol. Sociobiol. 47, 339–345. doi: 10.1007/s002650050674
Brzustowski, J., and LePage, D. (2021). motus: Fetch and use data from the Motus Wildlife Tracking System. R package version 4.0.6.
Bulte, M., Heyers, D., Mouritsen, H., and Bairlein, F. (2017). Geomagnetic information modulates nocturnal migratory restlessness but not fueling in a long distance migratory songbird. J. Avian Biol. 48, 75–82. doi: 10.1111/jav.01285
Catry, P., Dias, M. P., Phillips, R. A., and Granadeiro, J. P. (2013). Carry-over effects from breeding modulate the annual cycle of a long-distance migrant: an experimental demonstration. Ecology 94, 1230–1235. doi: 10.1890/12-2177.1
Cooper, N. W., Sherry, T. W., and Marra, P. P. (2015). Experimental reduction of winter food decreases body condition and delays migration in a long-distance migratory bird. Ecology 96, 1933–1942. doi: 10.1890/14-1365.1
Cooper, N. W., Thomas, M. A., and Marra, P. P. (2021). Vertical sexual habitat segregation in a wintering migratory songbird. Ornithology 138, 1–11. doi: 10.1093/ornithology/ukaa080
Coppack, T., and Pulido, F. (2009). Proximate control and adaptive potential of protandrous migration in birds. Integr. Comp. Biol. 49, 493–506. doi: 10.1093/icb/icp029
Coverdill, A. J., Clark, A. D., Wingfield, J. C., and Ramenofsky, M. (2011). Examination of nocturnal activity and behaviour in resident white-crowned sparrows (Zonotrichia leucophrys nuttalli). Behaviour 148, 859–876. doi: 10.1163/000579511X579038
Crewe, T. L., Deakin, J. E., Beauchamp, A. T., and Morbey, Y. E. (2019). Detection range of songbirds using a stopover site by automated radio-telemetry. J. Field Ornithol. 90, 176–189. doi: 10.1111/jofo.12291
de Zwaan, D. R., Wilson, S., Gow, E. A., and Martin, K. (2019). Sex-specific spatiotemporal variation and carry-Over effects in a migratory alpine songbird. Front. Ecol. Evol. 7:285. doi: 10.3389/fevo.2019.00285
Deakin, J. E., Guglielmo, C. G., and Morbey, Y. E. (2019). Sex differences in migratory restlessness behavior in a Nearctic–Neotropical songbird. Auk 136:ukz017. doi: 10.1093/auk/ukz017
Denommé, M., Deakin, J. E., Morbey, Y. E., and Guglielmo, C. G. (2021). Using breath δ13C analysis to determine the effects of dietary carbohydrate and protein on glucose and leucine oxidation at rest in the yellow-rumped warbler (Setophaga coronata). Comp. Biochem. Physiol. A Mol. Integr. Physiol. 256:110936. doi: 10.1016/j.cbpa.2021.110936
Donald, P. F. (2007). Adult sex ratios in wild bird populations: adult sex ratios in birds. Ibis 149, 671–692. doi: 10.1111/j.1474-919X.2007.00724.x
Dossman, B. C., Mitchell, G. W., Norris, D. R., Taylor, P. D., Guglielmo, C. G., Matthews, S. N., et al. (2016). The effects of wind and fuel stores on stopover departure behavior across a migratory barrier. Behav. Ecol. 27, 567–574. doi: 10.1093/beheco/arv189
Eikenaar, C., and Bairlein, F. (2014). Food availability and fuel loss predict Zugunruhe. J. Ornithol. 155, 65–70. doi: 10.1007/s10336-013-0987-7
Francis, C. M., and Cooke, F. (1986). Differential timing of spring migration in wood warblers (Parulidae). Auk 103, 548–556.
Gow, E. A., Burke, L., Winkler, D. W., Knight, S. M., Bradley, D. W., Clark, R. G., et al. (2019). A range-wide domino effect and resetting of the annual cycle in a migratory songbird. Proc. R. Soc. B Biol. Sci. 286:20181916. doi: 10.1098/rspb.2018.1916
Goymann, W., Spina, F., Ferri, A., and Fusani, L. (2010). Body fat influences departure from stopover sites in migratory birds: evidence from whole-island telemetry. Biol. Lett. 6, 478–481. doi: 10.1098/rsbl.2009.1028
Gwinner, E. (1989). Photoperiod as a modifying and limiting factor in the expression of avian circannual rhythms. J. Biol. Rhythms 4, 125–138. doi: 10.1177/074873048900400210
Gwinner, E. (1996). Circadian and circannual programmes in avian migration. J. Exp. Biol. 199, 39–48.
Hamilton, W. J. (1962). Evidence concerning the function of nocturnal call notes of migratory birds. Condor 64, 390–401. doi: 10.2307/1365547
Hasselquist, D. (1998). Polygyny in great reed warblers: a long-term study of factors contributing to male fitness. Ecology 79, 2376–2390.
Hau, M., Wikelski, J. C., and Wingfield, J. C. (1998). A neotropical forest bird can measure the slight changes in tropical photoperiod. Proc. R. Soc. B 265, 89–95.
Hedeström, A., and Pettersson, J. (1986). Differences in fat deposits and wing pointedness between male and female willow warblers caught on spring migration at Ottenby, SE Sweden. Ornis Scandinavica 17, 182–185. doi: 10.2307/3676868
Helm, B., and Gwinner, E. (2006). Migratory Restlessness in an Equatorial Nonmigratory Bird. PLoS Biol. 4:e110. doi: 10.1371/journal.pbio.0040110
Helm, B., Piersma, T., and van der Jeugd, H. (2006). Sociable schedules: interplay between avian seasonal and social behaviour. Anim. Behav. 72, 245–262. doi: 10.1016/j.anbehav.2005.12.007
Holberton, R. L. (1993). An endogenous basis for differential migration in the dark-eyed junco. Condor 95, 580–587. doi: 10.2307/1369602
Ketterson, E. D., and Nolan, V. (1976). Geographic variation and its climatic correlates in the sex ratio of eastern-wintering Dark-Eyed Juncos (Junco Hyemalis Hyemalis). Ecology 57, 679–693. doi: 10.2307/1936182
Kokko, H., Gunnarsson, T. G., Morrell, L. J., and Gill, J. A. (2006). Why do female migratory birds arrive later than males? J. Anim. Ecol. 75, 1293–1303. doi: 10.1111/j.1365-2656.2006.01151.x
Komar, O., O’Shea, B. J., Peterson, A. T., Navarro-Sigüenza, A. G., and Murphy, M. T. (2005). Evidence of latitudinal sexual segregation among migratory birds wintering in mexico. Auk 122, 938–948.
Kumar, V., Wingfield, J. C., Dawson, A., Ramenofsky, M., Rani, S., and Bartell, P. (2010). Biological Clocks and Regulation of Seasonal Reproduction and Migration in Birds. Physiol. Biochem. Zool. 83, 827–835. doi: 10.1086/652243
Latta, S. C., and Faaborg, J. (2002). Demographic and population responses of Cape May warblers wintering in multiple habitats. Ecology 83, 2502–2515. doi: 10.2307/3071810
Long Point Bird Observatory (2008). Canadian Migration Monitoring Network - Banding. Data accessed from NatureCounts, a node of the Avian Knowledge Network, Bird Studies Canada. Available online at: http://www.naturecounts.ca/ (Accessed February 8, 2019).
Lupi, S., Goymann, W., Cardinale, M., and Fusani, L. (2016). Physiological conditions influence stopover behaviour of short-distance migratory passerines. J. Ornithol. 157, 583–589. doi: 10.1007/s10336-015-1303-5
Lynch, J. F., Morton, E. S., and Van der Voort, M. E. (1985). Habitat segregation between the sexes of wintering Hooded Warblers (Wilsonia citrina). Ornithology 102, 714–721. doi: 10.1093/auk/102.4.714
Maggini, I., and Bairlein, F. (2010). Endogenous rhythms of seasonal migratory body mass changes and nocturnal restlessness in different populations of northern wheatear Oenanthe oenanthe. J. Biol. Rhythms 25, 268–276. doi: 10.1177/0748730410373442
Maggini, I., and Bairlein, F. (2012). Innate sex differences in the timing of spring migration in a songbird. PLoS One 7:e31271. doi: 10.1371/journal.pone.0031271
Marra, P. P. (2000). The role of behavioral dominance in structuring patterns of habitat occupancy in a migrant bird during the nonbreeding season. Behav. Ecol. 11, 299–308. doi: 10.1093/beheco/11.3.299
Marra, P. P., Hobson, K. A., and Holmes, R. T. (1998). Linking winter and summer events in a migratory bird by using stable-carbon isotopes. Science 282, 1884–1886.
Marra, P. P., and Holberton, R. L. (1998). Corticosterone levels as indicators of habitat quality: effects of habitat segregation in a migratory bird during the non-breeding season. Oecologia 116, 284–292.
Møller, A. P. (1994). Phenotype-dependent arrival time and its consequences in a migratory bird. Behav. Ecol. Sociobiol. 35, 115–122. doi: 10.1007/BF00171501
Moore, F. R., and Simm, P. A. (1985). Migratory disposition and choice of diet by the Yellow-Rumped Warbler (Dendroica coronata). Auk 102, 820–826. doi: 10.1093/auk/102.4.820
Morbey, Y. E., Beauchamp, A. T., Bonner, S. J., and Mitchell, G. W. (2020). Evening locomotor activity during stopover differs on pre-departure and departure days in free-living songbirds. J. Avian Biol. 51:e02448. doi: 10.1111/jav.02448
Morbey, Y. E., Guglielmo, C. G., Taylor, P. D., Maggini, I., Deakin, J., Mackenzie, S. A., et al. (2018). Evaluation of sex differences in the stopover behavior and postdeparture movements of wood-warblers. Behav. Ecol. 29, 117–127. doi: 10.1093/beheco/arx123
Morton, E. S., Lynch, J. F., Young, K., and Mehlhop, P. (1987). Do Male Hooded Warblers Exclude Females from Nonbreeding Territories in Tropical Forest? Auk 104, 133–135. doi: 10.2307/4087245
Nocera, J. J., Forbes, G. J., and Giraldeau, L.-A. (2006). Inadvertent social information in breeding site selection of natal dispersing birds. Proc. R. Soc. B Biol. Sci. 273, 349–355. doi: 10.1098/rspb.2005.3318
Pedersen, L., Jackson, K., Thorup, K., and Tøttrup, A. P. (2018). Full-year tracking suggests endogenous control of migration timing in a long-distance migratory songbird. Behav. Ecol. Sociobiol. 72:139. doi: 10.1007/s00265-018-2553-z
Piersma, T., Brugge, M., Spaans, B., and Battley, P. F. (2008). Endogenous circannual rhythmicity in body mass, molt, and plumage of great knots (Calidris Tenuirostris). Auk 125, 140–148. doi: 10.1525/auk.2008.125.1.140
Piersma, T., Zwarts, L., and Bruggemann, J. H. (1990). Behavioural aspects of the departure of waders before long-distance flights: flocking, vocaliations, flight paths and diurnal timing. Ardea 78, 157–184.
Pinheiro, J., Bates, D., DebRoy, S., and Sarkar, D. R Core Team (2018). nlme: Linear and Nonlinear Mixed Effects Models. Vienna: R Foundation for Statistical Computing.
Pulido, F. (2007). Phenotypic changes in spring arrival: evolution, phenotypic plasticity, effects of weather and condition. Clim. Res. 35, 5–23. doi: 10.3354/cr00711
Ramenofsky, M., and Agatsuma, R. (2006). Migratory behaviour of captive white-crowned sparrows, Zonotrichia leucophrys gambelii differs during autumn and spring migration. Behaviour 143, 1219–1240. doi: 10.1163/156853906778691586
Rappole, J. H., and Tipton, A. R. (1991). New harness design for attachment of radio transmitters to small passerines. J. Field Ornithol. 62, 335–337.
Saino, N., Ambrosini, R., Caprioli, M., Romano, A., Romano, M., Rubolini, D., et al. (2017). Sex-dependent carry-over effects on timing of reproduction and fecundity of a migratory bird. J. Anim. Ecol. 86, 239–249. doi: 10.1111/1365-2656.12625
Schmaljohann, H. (2019). The start of migration correlates with arrival timing, and the total speed of migration increases with migration distance in migratory songbirds: a cross-continental analysis. Mov. Ecol. 7:25. doi: 10.1186/s40462-019-0169-1
Schneider, C. A., Rasband, W. S., and Eliceiri, K. W. (2012). NIH Image to ImageJ: 25 years of image analysis. Nat. Methods 9, 671–675.
Seewagen, C. L., and Guglielmo, C. G. (2010). Effects of Fat and Lean Body Mass on Migratory Landbird Stopover Duration. Wilson J. Ornithol. 122, 82–87. doi: 10.1676/09-088.1
Seewagen, C. L., Guglielmo, C. G., and Morbey, Y.E. (2013). Stopover refueling rate underlies protandry and seasonal variation in migration timing of songbirds. Behav. Ecol. 24, 634–642. doi: 10.1093/BEHECO/ARS225
Seewagen, C. L., Ma, Y., Morbey, Y. E., and Guglielmo, C. G. (2019). Stopover departure behavior and flight orientation of spring-migrant Yellow-rumped Warblers (Setophaga coronata) experimentally exposed to methylmercury. J. Ornithol. 160, 617–624. doi: 10.1007/s10336-019-01641-2
Smith, R. J., and Moore, F. R. (2005). Arrival timing and seasonal reproductive performance in a long-distance migratory landbird. Behav. Ecol. Sociobiol. 57, 231–239. doi: 10.1007/s00265-004-0855-9
Spina, F., Massi, A., and Montemaggiori, A. (1994). Back from Africa: who’s running ahead? Aspects of differential migration of sex and age classes in Palearctic-African spring migrants. Ostrich 65, 137–150. doi: 10.1080/00306525.1994.9639676
Studds, C. E., and Marra, P. P. (2005). Nonbreeding habitat occupancy and population processes: an upgrade experiment with a migratory bird. Ecology 86, 2380–2385. doi: 10.1890/04-1145
Studds, C. E., and Marra, P. P. (2011). Rainfall-induced changes in food availability modify the spring departure programme of a migratory bird. Proc. R. Soc. B. 278, 3437–3443. doi: 10.1098/rspb.2011.0332
Taylor, P., Crewe, T., Mackenzie, S., Lepage, D., Aubry, Y., Crysler, Z., et al. (2017). The Motus Wildlife Tracking System: a collaborative research network to enhance the understanding of wildlife movement. Avian Conserv. Ecol. 12:8. doi: 10.5751/ACE-00953-120108
Therneau, T. (2021). A package for survival analysis in R. R package version 3.2-10. Available online at: <URL:https://CRAN.R-project.org/package=survival> (accessed June 25, 2020).
Vandermeer, C. L. (2013). The effect of testosterone on the spring migratory phenotype of a North American songbird (Zonotrichia albicollis). Available online at: http://ir.lib.uwo.ca/cgi/viewcontent.cgi?article=3235&context=etd (Accessed June 19, 2017).
Wagner, H. O. (1930). Über Jahres- und Tagesrhythmus bei Zugvögeln. Z. f. vergl. Physiol. 12, 703–724. doi: 10.1007/BF00337903
Ward, M. P., Benson, T. J., Semel, B., and Herkert, J. R. (2010). The use of social cues in habitat selection by wetland birds. Condor 112, 245–251. doi: 10.1525/cond.2010.090238
Wingfield, J. C. (2008). Organization of vertebrate annual cycles: implications for control mechanisms. Philos. Trans. R. Soc. Lond. B Biol. Sci. 363, 425–441. doi: 10.1098/rstb.2007.2149
Wobker, J., Heim, W., and Schmaljohann, H. (2021). Sex, age, molt strategy, and migration distance explain the phenology of songbirds at a stopover along the East Asian flyway. Behav. Ecol. Sociobiol. 75:25. doi: 10.1007/s00265-020-02957-3
Keywords: social environment, protandry, sex ratio, migration timing, automated radio-telemetry, migratory restlessness, sexual segregation
Citation: Deakin JE, Guglielmo CG and Morbey YE (2021) Effect of the Social Environment on Spring Migration Timing of a Songbird. Front. Ecol. Evol. 9:715418. doi: 10.3389/fevo.2021.715418
Received: 26 May 2021; Accepted: 14 September 2021;
Published: 04 October 2021.
Edited by:
Simeon Lisovski, Polar Terrestrial Environmental Systems, Alfred Wegener Institute Helmholtz Centre for Polar and Marine Research (AWI), GermanyReviewed by:
Maggie MacPherson, Louisiana State University, United StatesHelen Chmura, University of Alaska Fairbanks, United States
Copyright © 2021 Deakin, Guglielmo and Morbey. This is an open-access article distributed under the terms of the Creative Commons Attribution License (CC BY). The use, distribution or reproduction in other forums is permitted, provided the original author(s) and the copyright owner(s) are credited and that the original publication in this journal is cited, in accordance with accepted academic practice. No use, distribution or reproduction is permitted which does not comply with these terms.
*Correspondence: Jessica E. Deakin, amRlYWtpbjRAdXdvLmNh