- 1Plant Ecology and Evolution, Uppsala University, Uppsala, Sweden
- 2Department of Integrative Biology, University of Guelph, Guelph, ON, Canada
- 3Department of Biology, University of New Brunswick, Fredericton, NB, Canada
Genome duplication in plants is thought to be a route to speciation due to cytotype incompatibility. However, to reduce cross-pollination between cytotypes in animal-pollinated species, distinctive floral phenotypes, which would allow pollinator-mediated assortative mating between flowers, are also expected. Chamerion angustifolium is a Holarctic species that forms a hybrid zone between diploid and tetraploid populations in the North American Rocky Mountains. Extensive research has shown that these cytotypes differ in many ways, including some floral traits, and that pollinators can discriminate between cytotypes, leading to assortative mating. However, two signals commonly used by insect pollinators have not been measured for this species, namely petal colour and floral scent. Using greenhouse-grown diploids and tetraploids of C. angustifolium from the ploidy hybrid-zone in the North American Rocky Mountains, we show that both floral scent signals and petal reflectance differ between cytotypes. These differences, along with differences in flower size shown previously, could help explain pollinator-mediated assortative mating observed in previous studies. However, these differences in floral phenotypes may vary in importance to pollinators. While the differences in scent included common floral volatiles readily detected by bumblebees, the differences in petal reflectance may not be perceived by bees based on their visual sensitivity across the spectra. Thus, our results suggest that differences in floral volatile emissions are more likely to contribute to pollinator discrimination between cytotypes and highlight the importance of understanding the sensory systems of pollinators when examining floral signals.
Introduction
Genome duplication is an important mechanism of speciation in plants (Rieseberg and Willis, 2007; Soltis et al., 2009; Barker et al., 2016) and is notable as one of the few roads to speciation that can occur in sympatry (e.g., Vallejo-Marín et al., 2016). Polyploidy can arise from the hybridisation of two progenitor species (allopolyploidy) or be the result of whole genome duplication from a single progenitor (autopolyploidy). In general allopolyploids have received relatively more attention than autopolyploids (Soltis et al., 2007; Parisod et al., 2010; Spoelhof et al., 2017), however, both types of polyploids must be reproductively isolated from their progenitors to coexist. Autopolyploids may face even larger challenges because they are often regarded as being morphologically similar to their diploid progenitors and as such have historically been lumped as the same species (Soltis et al., 2007; Spoelhof et al., 2017). Mixed populations of autopolyploids and diploid progenitors provide an opportunity to understand the evolution of polyploidy, however, many questions remain (Kolář et al., 2017). Moreover the ecological consequences of polyploidy are relatively unexplored despite the role of ecology in evolutionary processes (Ramsey and Ramsey, 2014; Segraves, 2017).
Newly formed autopolyploids are at a reproductive disadvantage relative to their progenitors due to their rarity, i.e., minority cytotype exclusion (Levin, 1975; Husband, 2000). If polyploids do not differ significantly in floral traits from progenitors, pollinator fidelity to a particular cytotype is unlikely. The resulting random visitation from pollinators across cytotypes means that polyploids are more likely to reproduce with diploids than other polyploids, resulting in reduced polyploid fitness and minority cytotype exclusion (Husband, 2000). Therefore, coexistence and spread of polyploids is likely driven by mechanisms of reproductive isolation. For example, increased selfing could facilitate separation between ploidies (Barringer, 2007; Husband et al., 2008; Segraves and Anneberg, 2016). For outcrossing species, mechanisms such as separation in flowering time (e.g., Husband and Schemske, 2000; Jersáková et al., 2010; Castro et al., 2011; Münzbergová et al., 2015; Münzbergová and Skuhrovec, 2017; Pegoraro et al., 2019) or geography/niche differentiation (e.g., Raabová et al., 2008; Maherali et al., 2009; Muñoz-Pajares et al., 2018; Decanter et al., 2020) could contribute to isolation. Natural selection could also act on floral traits for differentiated pollinator signals (e.g., Nuismer and Cunningham, 2005). For pollinators to act as a mechanism to facilitate coexistence of cytotypes (Kolář et al., 2017; Sutherland et al., 2020; Laport et al., 2021), the flowers of the cytotypes must differ in ways that pollinators can perceive (e.g., Segraves and Thompson, 1999) or have sufficient morphological differences to facilitate isolation (e.g., Borges et al., 2012). For Gymnadenia conopsea (Orchidaceae), cytotypes differ in floral signals, mainly floral scent (Jersáková et al., 2010; Gross and Schiestl, 2015), and assortative mating has also been observed in some populations (Gross and Schiestl, 2015), suggesting that these mechanisms may function as reproductive isolation mechanisms in mixed populations of autotetraploids. Alternatively, post-pollination mechanisms of isolation may be more important in keeping polyploids distinctive, as in Aster amellus (Asteraceae) (Castro et al., 2011) and for some species little evidence of reproductive isolation is observed (e.g., Barringer and Galloway, 2017). Although pollinator-mediated reproductive isolation between cytotypes has strong theorical support and empirical evidence for some species where it has been measured (e.g., Kennedy et al., 2006; Thompson and Merg, 2008; Roccaforte et al., 2015; Sutherland et al., 2020; Laport et al., 2021), few studies have examined differences in multifaceted floral signals between cytotypes making generalisations challenging.
Chamerion angustifolium has become a model system for studying autopolyploidy largely due to the work of Brian Husband’s group. Considerable advances have been made in the study of autopolyploidy using this system to measure reproductive isolation of the cytotypes (Husband and Sabara, 2004), assortative mating (Husband, 2000; Kennedy et al., 2006), fitness differences (Burton and Husband, 2000), climatic niche differences of the cytotypes (Thompson et al., 2014), and their physiology (Maherali et al., 2009). Reproductive isolation does occur between the cytotypes in wild populations (Kennedy et al., 2006) and experimental plots (Husband, 2000) and pollinator fidelity is an important isolating mechanism in the system (Husband and Sabara, 2004), suggesting that differences in floral traits may explain pollinator behaviour on these inflorescences. In natural mixed populations, tetraploids often display more and larger flowers than diploids, although greenhouse grown plants have shown the opposite pattern for flower number (Husband, 2000). However, a detailed examination of the floral phenotypes has not been undertaken for the species. In this study we examine floral signals pollinators could use to discriminate between diploid and tetraploid C. angustifolium. We compare flower size, spectral reflectance of petals, and scent between the two ploidies. These signalling traits can be important in driving pollinator preferences and fidelity, either individually or in an integrated fashion (Katzenberger et al., 2013; Schiestl and Johnson, 2013; Junker and Parachnowitsch, 2015). Signaling differences are likely for C. angustifolium cytotypes from mixed populations because pollinators do contribute to reproductive isolation in the species, however, which traits aid those decisions are largely unknown.
Materials and Methods
Study System
Chamerion angustifolium (formerly Epilobium angustifolium) is a protandrous herbaceous perennial native to temperate regions throughout the northern hemisphere (Mosquin, 1966). Tetraploid and diploid populations of C. angustifolium occur throughout North America, with diploids occupying regions at higher latitudes than tetraploids (Thompson et al., 2014) and adaptation to elevation of both cytotypes is observed (Martin and Husband, 2013). Although a contact zone between the two cytotypes exists in the Rocky Mountains, this contact zone features a patchy mosaic with small-scale variation in cytotype, rather than a smooth transition from tetraploid at low altitudes and diploids at higher altitudes (Husband and Schemske, 1998; Sabara et al., 2013) and mixed populations have been detected elsewhere in North America (Thompson et al., 2014). The history and phylogeography of populations is largely unknown but it can be assumed that diploids and tetraploids have had some opportunity to evolve in these populations (e.g., Maherali et al., 2009). Where diploids and tetraploids occur in sympatry, triploids can be found (Husband and Schemske, 1998; Sabara et al., 2013), suggesting that mating between cytotypes occurs. Reproduction happens both clonally and by seed (Baldwin and Husband, 2013). Hymenoptera serve as the primary pollinators throughout the range (Husband and Schemske, 2000; Kennedy et al., 2006; Ollerton et al., 2007) and Bombus spp. are the most common effective pollinators in North America (Galen and Plowright, 1985; Kennedy et al., 2006). While bees seem to be the more effective pollinators for C. angustifolium, their flowers can be visited by a diversity of additional animals such as Lepidoptera, syrphid flies, and occasionally hummingbirds.
Greenhouse Conditions
We grew diploid and tetraploid C. angustifolium from seed in a greenhouse at Uppsala University in 2015. Seeds were collected from populations in the Rocky Mountains in North America, and were from a bulk collection of pure diploid, pure tetraploid and mixed populations as used in Thompson et al. (2015). Cytotype for the bulk collections was determined by estimating DNA content using flow cytometry in previous work and the classification was highly accurate [60/60 confirmed diploid, 59/60 confirmed tetraploid (Thompson et al., 2015)], however, we did not directly test the individuals used in our experiment. Seeds were planted in compost pellets (one seed per pellet). Germination success was lower in tetraploids, resulting in a sample size of 30 tetraploid and 50 diploid plants. Seedlings were transplanted into a 3:1 mixture of vegetable garden soil and expanded clay in 10 cm wide pots after 28 days and placed randomly on two self-watering greenhouse tables. The automated watering system delivered additional nutrients and water for 30 min every 48 h for the first month after transplant, then increased to 2 h of watering every 24 h to accommodate increased water demands as plants grew. Due to a technical problem with the automated watering system, one greenhouse table did not receive fertiliser and this was only discovered when plants showed signs of nutrient deficiency (e.g., chlorosis). To account for differences in pre-flowering fertiliser levels we include this factor in all analyses, however, both tables received the same water and fertiliser amounts during the flower sampling period and the deficient plants (N = 12 tetraploids, 26 diploids) quickly recovered.
Floral Morphology
We measured petal width, petal length, and style length on three haphazardly selected flowers on each plant following the protocols of Husband and Schemske (2000). We used the lower right petal of each flower for petal measurements, and all measurements were collected from female phase flowers to ensure petals were fully expanded. When possible, we selected flowers on the main stem. We measured style length as the distance from the base of the style to the point where stigmatic lobes separated. For each of the three morphological traits, we used plant mean values in analyses.
Floral Spectral Reflectance
During peak flowering, we haphazardly selected three female phase flowers from each plant for spectral reflectance measurements. As for petal size, we used the lower right petal of each flower using a UV-VIS spectrophotometer (Ocean Optics), however, these were not necessarily the same flowers used for morphology measurements. We measured reflectance spectra for 300–700 nm, taking at least three readings for each petal to account for slight variations in reflectance measures. We binned spectra readings to have one measure per nm and used the average reflectance of all flowers and readings for each plant (due to missing data of petal reflectance for four diploid plants, N = 30 tetraploids and 46 diploids).
Floral Volatile Sampling and Analyses
We collected floral volatiles using dynamic headspace sampling (Raguso and Pellmyr, 1998). To collect floral volatiles, the entire inflorescence was placed inside a 14.5 × 35 cm ICA® oven bag (ICA Sverige AB, Solna, Sweden) attached to a Teflon tube scent trap filled with 10 mg Tenax GR® filter (Sigma-Aldrich, St Louis, MO, United States). Scent traps were connected via a Cole-Parmer (Vernon Hills, IL, United States) 65-mm direct-reading flow meter to a custom-built air pump (GroTech, Gothenburg, Sweden) for 5 h at a flow rate of 200 ml/min (as in Friberg et al., 2013). For each sampling period, an air control was collected from an empty oven bag to assess background scent levels in our open sampling set-up and at least one diploid and one tetraploid were haphazardly chosen for a leaf sample to help determine floral-specific volatiles. Volatiles found in roughly equal amounts in air controls and plant samples were excluded from our analyses. For volatiles present in air controls but in greater amounts in plant samples, we subtracted the air control amounts from all samples taken that day (any negative numbers were converted to zero). At sampling, the number of open flowers sampled per plant was recorded and used to calculate the emission of floral volatiles in ng/h/flower. In order to assess temporal variation in volatile emissions, 10 plants (N = 6 tetraploid, 4 diploid with one sampled 3 times) were haphazardly selected for repeated sampling, however, we found no significant differences between repeated samples and so used the mean across multiple samples in our analysis. Compounds were extracted from scent traps using 200 μl of hexane and concentrated to 50 μl with nitrogen gas and stored at −20°C until analysis. We added 5 μl of a 0.03% toluene (1.3 μg) solution in hexane to samples for quantification. Samples were analyzed on a Finnigan Trace GC ultra 2000 gas chromatograph connected to a Finnigan Trace DSQ mass spectrometer (both Thermo Fisher Scientific, United States) with a 30 m × 0.250 mm × 0.25 μm DB-Wax column (Agilent Technologies, United States). Helium was used as carrier gas at a constant velocity of 1 ml/min. The temperature program was as follows: 3 min hold at 50°C, followed by a 10°C/min increase for 20 min until the oven reached a maximum temperature of 250°C. Chromatograms were integrated using XcaliburTM (version 1.4, ®Thermo Electron Corporation). Compounds were identified using NIST library searches, as well as available standards. Floral volatile emission in ng/h/flower was calculated following the equation in Svensson et al. (2005).
Statistical Analyses
All analyses were conducted in R (R Core Team, 2021).
Flower Size Analyses
We used a two-way multivariate analysis of variance (MANOVA) to compare petal length, petal width, and style length between diploids and tetraploids, while accounting for fertiliser level during growth. To determine differences for the individual traits, we used linear models (lm function) to conduct ANOVAs that included ploidy level and fertiliser and their interaction, followed by the Dunn–Šidák correction for multiple comparisons. All traits met the assumptions of the tests and so no transformations were used.
Reflectance Analyses
To identify differences in spectral reflectance between diploids and tetraploids, we first used the adonis function in the R package “vegan” (Oksanen et al., 2019) to conduct a permutational multivariate analysis of variance (PERMANOVA) on Bray-Curtis dissimilarities with 5,000 permutations, including fertiliser condition as an interaction term. This analysis considered the entire curve between 300 and 700 nm (spectral reflectance for each wavelength was treated as a single data point) to assess whether there was an overall difference. To test whether differences in reflectance are likely detected by pollinators, we applied the visual sensitivity model based on Chittka (1992) to the spectral reflectance data. The visual sensitivity model was implemented using the vismodel function in “Pavo2” R package using the recommended settings for the colour hexagon based on a green background, daylight illumination and for simplicity we used the built-in “Apis” model for visual sensitivity (Maia et al., 2019). The honeybee visual sensitivity is often used to represent Hymenoptera vision which is evolutionarily conserved (Briscoe and Chittka, 2001) and Apis mellifera can also be a common visitor to C. angustifolium. Similar results were found using the visual sensitivities of Bombus species (not shown), which are common C. angustifolium pollinators and also have very similar visual sensitivity (Skorupski and Chittka, 2010). We used the bootcoldist function of Pavo2 to calculate unweighted Euclidean distances between ploidies based on the hexagon to show the mean difference in colour contrast.
Scent Analyses
We first tested whether total scent emission differed between the two ploidies using a linear model with fertiliser condition included as an interaction term. We also compared scent profiles from the leaf and flower samples to determine floral specific volatiles; we considered volatiles as floral specific if they were at least twice as prevalent in the floral samples as in the leaf samples, as in Parachnowitsch et al. (2012). To assess whether variation in emission rates of specific compounds differed between the ploidies, we used a PERMANOVA on Bray–Curtis dissimilarities with 5,000 permutations. To determine which compounds contributed substantially to differences between diploid and tetraploids we used a similarity percentage (SIMPER) analysis using the simper function in “vegan” (Oksanen et al., 2019). To visualise these differences, we used non-metric multidimensional scaling ordination (NMDs) based on Bray–Curtis distances in “vegan.”
Results
Floral traits of C. angustifolium diploids and tetraploids differed across morphology, colour, and scent. Interestingly, petal size did not differ between ploidies. While the MANOVA detected differences between ploidies in morphology (P < 0.001), ANOVAs for petal length (F1,76 = 1.9, P = 0.17), petal width (F1,76 = 1.7, P = 0.20), and style length (F1,76 = 33.16, P < 0.001), showed that style length was responsible for these morphological differences (Figure 1). Style length differences remain significant after correction for multiple comparisons. Fertiliser during growth and its interaction with ploidy had no effect on morphology. We found significant differences in petal reflectance between diploids and tetraploids (Table 1), particularly in the 400–500 nm and 600–700 nm range (Figure 2). However, as the range 600–700 nm is not an important component of the Hymenoptera visual system, which could help explain why the signficant effect of ploidy on petal reflectance was lost when a bee vision model was applied (Figure 2). The differences detected in the 400–500 range were obviously not enough for bees to detect and this was not surprising given that the mean colour contrast in hexagon units between diploids and tetraploids was 0.002 (CI = 0.0008–0.012).
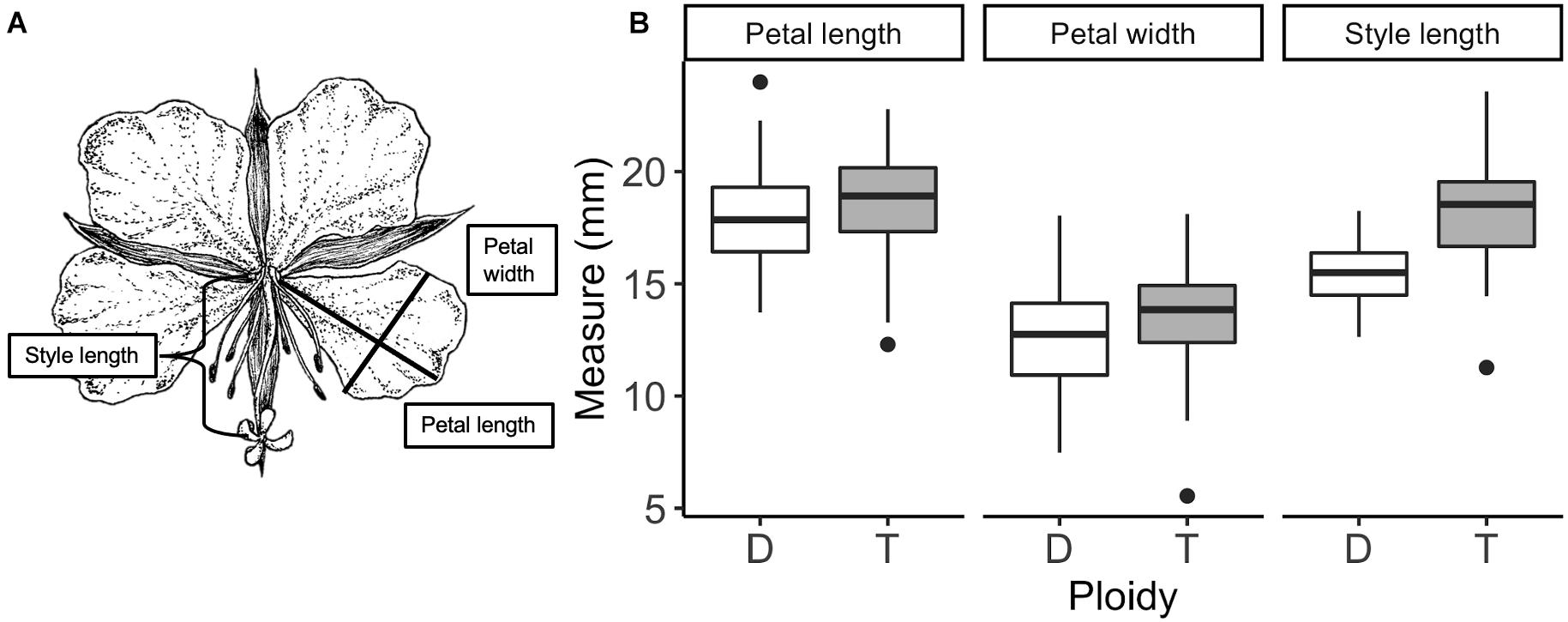
Figure 1. Drawing of Chamerion angustifolium with the three floral measures depicted (A) and (B) the variation in flower size measures of diploid (D) and tetraploid (T) plants. Petal measurements were taken from the lower right petal of female-phase flowers. Only style length showed significant differences between diploids (white) and tetraploids (grey). Boxplots show median and the spread of the data. Illustration by Lucie Vézina.
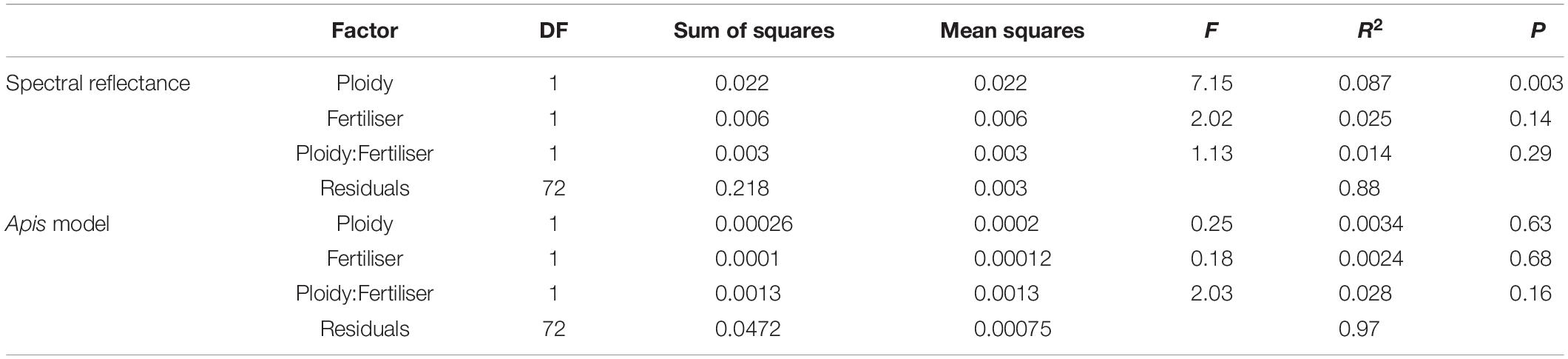
Table 1. PERMANOVA results for the spectral reflectance of Chamerion angustifolium flower petals before and after application of a Apis visual sensitivity model, testing for the effects of ploidy, fertiliser during growth, and their interaction.
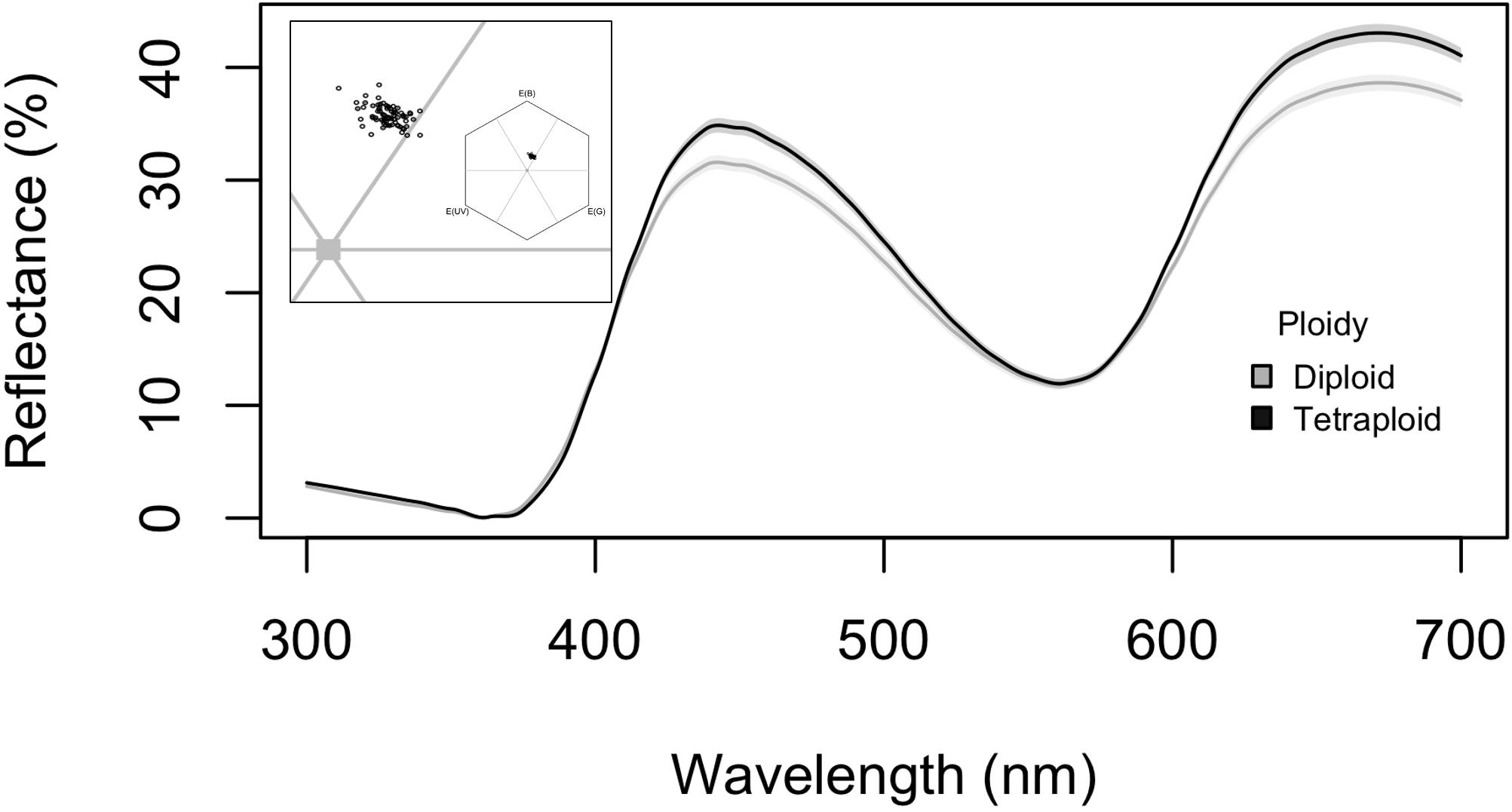
Figure 2. Flower reflectance differences for diploid and tetraploid Chamerion angustifolium plotted from 300 to 700 nm; lines are the treatment mean and shading represents the SE. Inset: flower colour plotted using the colour hexagon for Hymenoptera colour vision to illustrate the overlap of diploid and tetraploid reflectance (empty circles = diploid, filled circles = tetraploid), corners represent blue = E(B), green = E(G), and ultraviolet = E(UV).
We detected 21 compounds emitted from C. angustifolium from a range of compound classes such as terpenes, aromatics, fatty acid derivatives, and aliphatics (Table 2). Most compounds were emitted in much greater amounts in the floral samples than the leaf samples, suggesting that these were floral-specific compounds. The exceptions were the fatty acid derivatives cis-3-hexenyl butyrate, cis-3-hexenyl acetate, and 3-hexen-1-ol benzoate, the monoterpenes ocimene and linalool, as well as methyl salicylate and nonadecane. No compounds were exclusively detected only in diploids or tetraploids, suggesting that these scent bouquets generally overlap in composition (Figure 3, inset). Moreover, total scent emission did not differ with ploidy (F1,76 = 1.6, P = 0.21), and nutrient deficiency during growth or its interaction with ploidy did not affect total scent emission (Ps > 0.5). However, despite the overlap in scent bouquet, there was significant differences between diploids and tetraploids when emission amounts of all compounds were considered, as shown with the PERMANOVA (Table 3), suggesting scent ratios within the floral scent bouquet differed between ploidies. This remains true if we limit our analyses to only volatiles that are found in greater abundances in the floral samples (PERMANOVA: ploidy F1,76 = 6.63, P = 0.0002) suggesting floral-specific bouquet differs between ploidies. However, because floral visitors experience the whole plant scent, we focus on the analyses that included all volatiles. Generally, diploids emit more 2-nonanone and 2-hexen-1-ol while tetraploids emit more phenyl acetaldehyde and cis-3-hexenyl acetate (Figure 3). Together these four compounds explain ∼55% of the difference in scent between diploids and tetraploids (Supplementary Table 1) and were the most abundant volatiles in the floral bouquet.
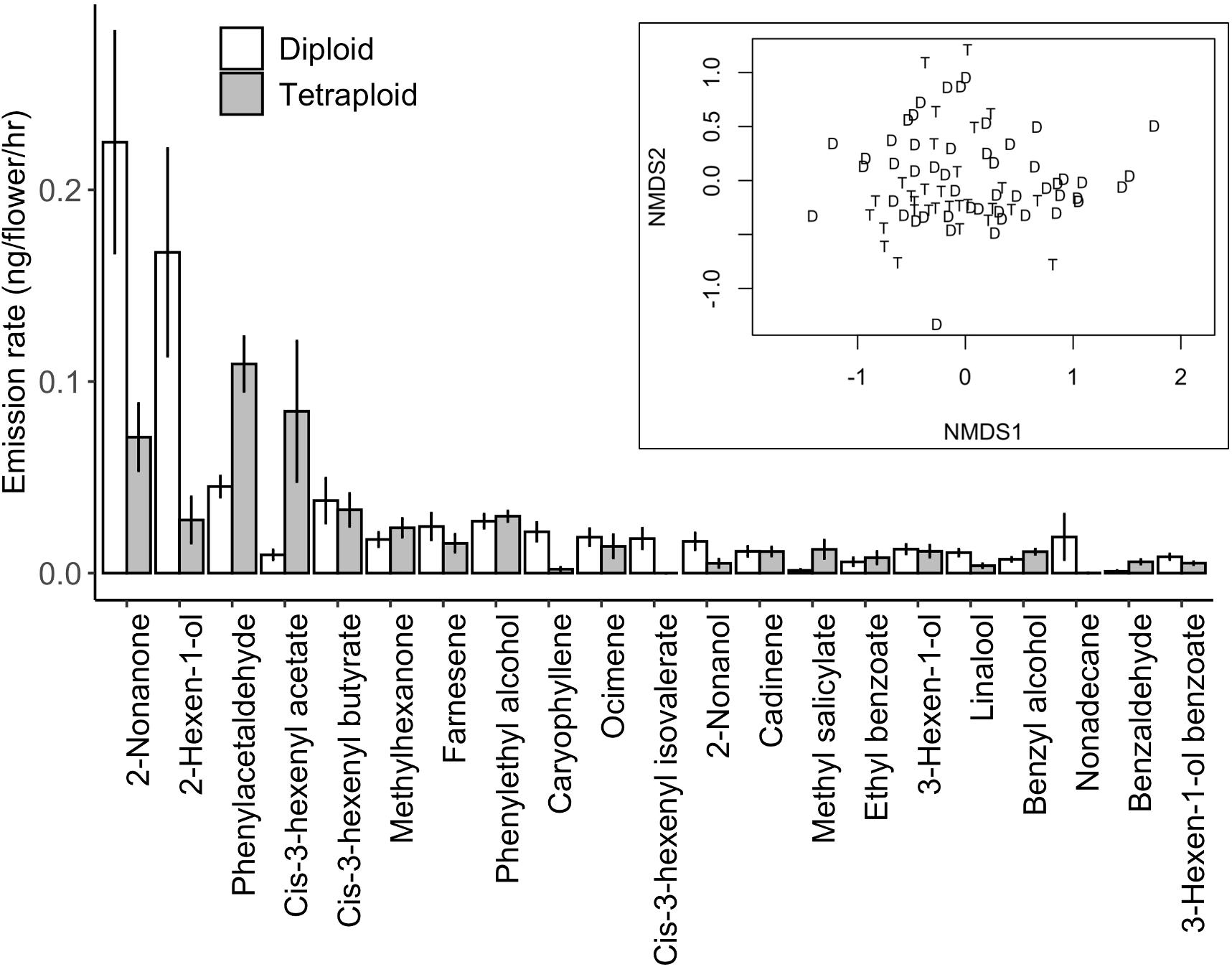
Figure 3. Floral volatile compound mean emission rate (+/− SE) from diploid and tetraploid Chamerion angustifolium. Compounds are ordered by their contributions to differences between diploids (white bars) and tetraploids (grey bars) based on SIMPER (Supplementary Table 1). Inset: Overlap of floral scent bouquets for diploids (D) and tetraploids (T) based on all compounds emitted.
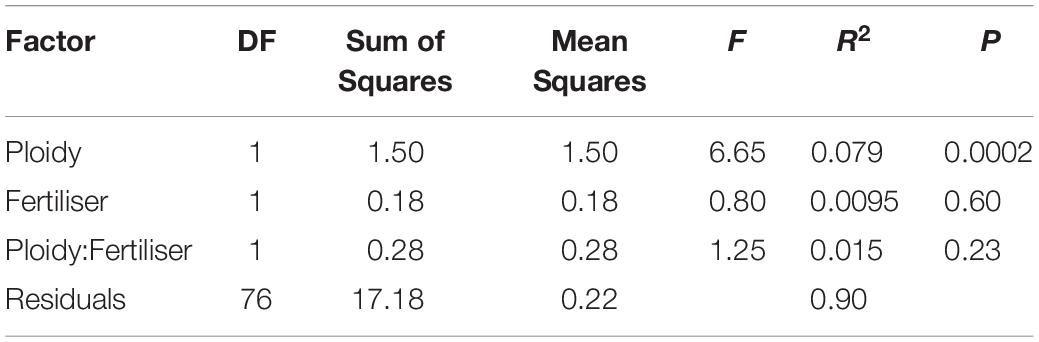
Table 3. PERMANOVA results for the 21 floral volatiles emitted by Chamerion angustifolium individuals, testing for the effects of ploidy, fertiliser during growth, and their interaction.
Discussion
We found differences in floral signals between diploid and tetraploid C. angustifolium grown in controlled greenhouse conditions that suggest pollinators might distinguish between these cytotypes. However, not all signals showed the same pattern of variation. Flower petal size did not differ between the ploidies, but we did see a trend that flowers in tetraploids were generally bigger with longer styles (Figure 1). Significant flower size differences are detectable in field populations of C. angustifolium and are a common result of genome wide duplication (Porturas et al., 2019). While floral petal reflectance showed a significant statistical difference between ploidies, using a bee vision model suggests that these differences are unlikely to be perceived by Hymenopteran pollinators (Figure 2). Instead our results suggest that floral scent may be the most likely signal to differentiate between cytotypes in mixed populations (Figure 3).
Neither diploid nor tetraploid C. angustifolium emitted unique floral volatiles and the overall scent bouquets are not distinctive (Figure 3) suggesting that these two cytotypes strongly overlap in their scent. However, insect pollinators can respond to differences in volatile ratios to distinguish between flowers (Raguso, 2008) and we did detect differences in the specific compounds in the volatile bouquets (Figure 3). Both 2-nonanone and 2-hexen-1-ol were emitted in higher amounts from diploids and are common floral scents (Knudsen et al., 2006). Interestingly, 2-nonanone is also a component of the Bombus terrestris queen pheromone (Krieger et al., 2006) and can be produced by nectar fungi (Rering et al., 2018). Because our plants were grown in the greenhouse without access to pollinators to inoculate the nectar, it is unlikely that 2-nonanone was nectar fungi-produced. It would be interesting to see if these differences remain in wild plants where nectar microbes could more readily interact with the flowers and influence the floral scent.
Tetraploids tended to emit more phenylacetaldehyde and cis-3-hexenyl acetate than diploids (Figure 3). Phenylacetaldehyde is also a common floral volatile (Knudsen et al., 2006), detected by a range of insects such as bees and moths (Huber et al., 2005; Dötterl and Vereecken, 2010; Knauer and Schiestl, 2015) and has a significant positive effect on the number of bumble bee visits to Brassica rapa inflorescences (Knauer and Schiestl, 2017). Together these suggest a strong attractive role for the compound in floral scent bouquets. Conversely, cis-3-hexenyl acetate is more commonly associated with green leaf volatiles (GLVs) released after herbivore damage (Kessler and Baldwin, 2001; Frost et al., 2008), although that can also be true for most of the aliphatics detected here. For C. angustifolium, this compound was not floral specific and was found in similar amounts in leaf and floral samples. While GLVs can be associated with handling plants to sample for scent, there is no reason to think tetraploids experienced different damage levels during our sampling. Variation in response to plant damage is detected in many plant species (Karban, 2020) and ploidy can influence herbivory (Halverson et al., 2008; Boalt et al., 2010; Segraves and Anneberg, 2016), however, differences in herbivore-induced volatiles between ploidies should be specifically tested in C. angustifolium to be conclusive. Furthermore, while our results suggest that the two cytotypes differ in scent emission of compounds that are common to floral bouquets and likely readily detected by the wide range of insect pollinators to C. angustifolium, behaviour tests are needed to determine if these signals are used in foraging decisions such as in Husband (2000) and Kennedy et al. (2006).
Flower size differences between cytotypes have been found for C. angustifolium in the field (Kennedy et al., 2006), and although we saw a trend for tetraploids to have larger flowers, we did not detect the same statistical differences as previous work. As pollinators can learn to distinguish between flowers based on size, although possibly not as easily as colour and floral scent signals (e.g., Blarer et al., 2002), further behavioural studies examining foraging behaviour could prove illuminating. Interestingly, the reflectance differences we observed are unlikely to be perceived by bee pollinators to C. angustifolium (Figure 2). While Hymenoptera do show sensitivity between the 400 and 500 nm wavelengths where tetraploids have higher reflectance than diploids (Figure 2), these differences were presumably not pronounced enough to detect and the mean difference in hexagon units between ploidy is likely below the discrimination level for bees (Dyer and Chittka, 2004; Aguiar et al., 2020). Lepidoptera can also visit these flowers and their vision incorporates the 600–700 nm range (van der Kooi et al., 2021), so perhaps they could better distinguish between these cytotypes. However, Lepidoptera visits to C. angustifolium are unlikely to drive reproductive isolation between cytotypes due to their relatively infrequent visits (Lack, 1982) and behaviour on the flowers (personal observation, ALP). Similarly, Diptera can be common visitors to C. angustifolium but based on pollen removal and deposition rates compared to bees, Diptera are poor pollinators of this species (Ollerton et al., 2007). Interestingly, the difference in amplitude in reflectance suggests that the reason these reflectances differ is due to tissues or cells changing the backscattering structure rather than pigment amount or placement (e.g., Figure 3 in van der Kooi et al., 2019). Larger cell size and nuclear volume are common with polyploidy and might help explain the reflectance patterns. An unanswered question for the system is whether any C. angustifolium visitors perceive the reflectance variation from the petals or if it matters. Additionally, it is possible pollinators may respond to visual signals that were not represented by our point measure of spectral reflectance, such as ultraviolet patterns (Koski and Ashman, 2014; Trunschke et al., 2021). While we only measured a single petal per flower, to our knowledge there are no UV patterns on C. angustifolium petals (Kaz Ohashi, personal communication) and the low reflectance in the 300–400 nm range also supports this interpretation. Regardless of whether the reflectance differences drive behavioral differences on the flowers, it could be interesting to examine the effects of genome duplication on floral pigments in autotetraploids and whether these are similar to somatic ploidy seen in the petals of some species (Schepper et al., 2001).
The two C. angustifolium cytotypes have different physiological responses (e.g., to water stress, Maherali et al., 2009), and so tend to occupy different microclimates (Martin and Husband, 2013; Thompson et al., 2014). These microclimatic differences could also drive phenotypic variation in wild plants, for example scent emission can vary with temperature (Farré-Armengol et al., 2014). Thus, wild plants may show greater, or at least different, variation than the greenhouse grown ones we measured here. However, the controlled greenhouse environment does mean the differences we detected are more likely to have a genetic basis than are due to phenotypic plasticity. Our bulked seeds mean that our study represents general differences with ploidy in the Rocky Mountains. Evolutionary history, especially in mixed populations where reproductive isolation likely plays a larger role, could mean wild populations show different patterns of variation. Furthermore, plants in this experiment were not directly tested for ploidy, so it is possible that we have misclassified some individuals or included triploids. Although given the accuracy for other individuals in the bulk seed collections (Thompson et al., 2015), it seems unlikely that this would play a large role in the results we found.
Our experiment unintentionally included differences in nutrient availability during development that could have impacted the floral traits and differences between cytotypes. However, generally this ‘treatment’ was not significant in our comparisons, perhaps because it was corrected before flowering began. Nutrients can of course have strong effects on plant growth (e.g., in C. angustifolium Bennett et al., 2004; Pinno et al., 2013), and these effects can impact diploid and tetraploid C. angustifolium differently (Bales and Hersch-Green, 2019). Nutrients can also impact floral traits. These effects are often seen as increases in display size (e.g., Friberg et al., 2017) directly related to an increase of overall biomass in high nutrient environments. Nutrients can also affect floral scent for some species (Majetic et al., 2017), but scent may be less affected than other floral traits (Friberg et al., 2017; Luizzi et al., 2021). Timing of nutrient deficits could be important, especially for physiologically plastic traits such as scent emission, and may explain the lack of differences with fertiliser seen here. Although our experiment cannot be seen as a definitive test of fertiliser effects on floral traits in C. angustifolium, it does suggest that these weedy plants can recover quickly from nutrient deficits.
Coexistence of polyploids with their diploid progenitors requires reproductive isolation to maintain separation of the cytotypes. Pollinator fidelity is estimated to play a significant role in reproductive isolation of C. angustifolium (Husband and Sabara, 2004) and therefore traits that allow pollinators to distinguish between cytotypes are expected. Flower size and display size may play a role in these decisions in wild populations (Kennedy et al., 2006), however, our results suggest that floral scent may also be an important trait to distinguish between cytotypes. Floral trait differences may be a direct result of genome duplication but could also be the result of subsequent evolution in the cytotypes. For our study, we cannot distinguish these effects but other work with neo-tetraploid C. angustifolium, shows that differences in response to water stress between the cytotypes is likely due to evolution rather than the duplication event (Maherali et al., 2009). Presumably, the pollinators are also motivated by more than just subtle differences in floral signals and an important next step would be to compare rewards such as nectar and pollen in C. angustifolium. The connection with rewards and signals is generally understudied in pollination biology, especially in an evolutionary ecology context (Parachnowitsch et al., 2019), therefore evaluating reward differences between cytotypes could have broad value to understanding assortative mating. Our results are similar to those in the orchid G. conopsea where floral scent, but not petal reflectance, differs between cytotypes (Gross and Schiestl, 2015). Mixed autoploidy systems provide opportunities for furthering our understanding of ploidy (Kolář et al., 2017), however, many more of these systems will need to be measured for floral traits to know whether the pattern of floral scent differences between cytotypes in G. conopsea and C. angustifolium is common or rare. These two examples also incorporate evolutionary history after genome-wide duplication and testing synthesised neo-polyploids would help determine whether the difference observed in these species are likely the result of the duplication or natural selection to reinforce reproductive isolation.
Data Availability Statement
The original contributions presented in the study are included in the article/Supplementary Material, further inquiries can be directed to the corresponding author.
Author Contributions
BP and AP conceived and designed the study. BP collected all data and wrote the first draft of the manuscript for his masters thesis. BP, HB, and AP performed the statistical analysis. All authors contributed to manuscript revision, read, and approved the submitted version.
Funding
The Parachnowitsch lab is supported by a Natural Sciences and Engineering Research Council of Canada Discovery Grant (2018-04148) as well as funding from the Swedish Research Council (VR 2016-04508).
Conflict of Interest
The authors declare that the research was conducted in the absence of any commercial or financial relationships that could be construed as a potential conflict of interest.
Publisher’s Note
All claims expressed in this article are solely those of the authors and do not necessarily represent those of their affiliated organizations, or those of the publisher, the editors and the reviewers. Any product that may be evaluated in this article, or claim that may be made by its manufacturer, is not guaranteed or endorsed by the publisher.
Acknowledgments
We thank Brian Husband for the seed that allowed this study to happen, Paul Kron for information on the seed source, Kaz Ohashi for sharing observations on the UV patterns (or rather lack of) of the flowers, and Robert Junker for sharing R code and advice for floral reflectance analyses. CV and three reviewers provided helpful feedback that improved the manuscript. We acknowledge that the universities in which we work and the land from which these seeds were harvested are the traditional lands of First Nations People.
Supplementary Material
The Supplementary Material for this article can be found online at: https://www.frontiersin.org/articles/10.3389/fevo.2021.734128/full#supplementary-material
References
Aguiar, J. M. R. B. V., Giurfa, M., and Sazima, M. (2020). A cognitive analysis of deceptive pollination: associative mechanisms underlying pollinators’ choices in non-rewarding colour polymorphic scenarios. Sci. Rep. 10:9476. doi: 10.1038/s41598-020-66356-4
Baldwin, S. J., and Husband, B. C. (2013). The association between polyploidy and clonal reproduction in diploid and tetraploid Chamerion angustifolium. Mol. Ecol. 22, 1806–1819. doi: 10.1111/mec.12217
Bales, A. L., and Hersch-Green, E. I. (2019). Effects of soil nitrogen on diploid advantage in fireweed, Chamerion angustifolium (Onagraceae). Ecol. Evol 9, 1095–1109. doi: 10.1002/ece3.4797
Barker, M. S., Husband, B. C., and Pires, J. C. (2016). Spreading Winge and flying high: the evolutionary importance of polyploidy after a century of study. Am. J. Bot. 103, 1139–1145. doi: 10.3732/ajb.1600272
Barringer, B. C. (2007). Polyploidy and self-fertilization in flowering plants. Am. J. Bot. 94, 1527–1533. doi: 10.3732/ajb.94.9.1527
Barringer, B. C., and Galloway, L. F. (2017). The reproductive ecology of diploid and tetraploid Galax urceolata. Am. Midl. Nat. 177, 299–308. doi: 10.1674/0003-0031-177.2.299
Bennett, J. N., Lapthorne, B. M., Blevins, L. L., and Prescott, C. E. (2004). Response of Gaultheria shallon and Epilobium angustifolium to large additions of nitrogen and phosphorus fertilizer. Can. J. For. Res. 34, 502–506. doi: 10.1139/x03-219
Blarer, A., Keasar, T., and Shmida, A. (2002). Possible mechanisms for the formation of flower size preferences by foraging bumblebees. Ethology 108, 341–351.
Boalt, E., Arvanitis, L., Lehtilä, K., and Ehrlén, J. (2010). The association among herbivory tolerance, ploidy level, and herbivory pressure in Cardamine pratensis. Evol. Ecol. 24, 1101–1113. doi: 10.1007/s10682-010-9364-7
Borges, L. A., Souza, L. G. R., Guerra, M., Machado, I. C., Lewis, G. P., and Lopes, A. V. (2012). Reproductive isolation between diploid and tetraploid cytotypes of Libidibiaferrea (= Caesalpinia ferrea) (Leguminosae): ecological and taxonomic implications. Plant Syst. Evol. 298, 1371–1381. doi: 10.1007/s00606-012-0643-3
Briscoe, A. D., and Chittka, L. (2001). The evolution of color vision in insects. Annu. Rev. Entomol. 46, 471–510. doi: 10.1146/annurev.ento.46.1.471
Burton, T. L., and Husband, B. C. (2000). Fitness differences among diploids, tetraploids, and their triploid progeny in Chamerion angustifolium: mechanisms of inviability and implications for polyploid evolution. Evolution 54, 1182–1191. doi: 10.1111/j.0014-3820.2000.tb00553.x
Castro, S., Münzbergová, Z., Raabová, J., and Loureiro, J. (2011). Breeding barriers at a diploid–hexaploid contact zone in Aster amellus. Evol. Ecol. 25, 795–814. doi: 10.1007/s10682-010-9439-5
Chittka, L. (1992). The colour hexagon: a chromaticity diagram based on photoreceptor excitations as a generalized representation of colour opponency. J. Comp. Physiol. A 170, 533–543. doi: 10.1007/BF00199331
Decanter, L., Colling, G., Elvinger, N., Heiðmarsson, S., and Matthies, D. (2020). Ecological niche differences between two polyploid cytotypes of Saxifraga rosacea. Am. J. Bot. 107, 423–435. doi: 10.1002/ajb2.1431
Dötterl, S., and Vereecken, N. J. (2010). The chemical ecology and evolution of bee–flower interactions: a review and perspectives. Can. J. Zool. 88, 668–697. doi: 10.1139/Z10-031
Dyer, A. G., and Chittka, L. (2004). Biological significance of distinguishing between similar colours in spectrally variable illumination: bumblebees (Bombus terrestris) as a case study. J. Comp. Physiol. A 190, 105–114. doi: 10.1007/s00359-003-0475-2
Farré-Armengol, G., Filella, I., Llusià, J., Niinemets, Ü, and Peñuelas, J. (2014). Changes in floral bouquets from compound-specific responses to increasing temperatures. Glob. Change Biol. 20, 3660–3669. doi: 10.1111/gcb.12628
Friberg, M., Schwind, C., Raguso, R. A., and Thompson, J. N. (2013). Extreme divergence in floral scent among woodland star species (Lithophragma spp.) pollinated by floral parasites. Ann. Bot. 111, 539–550. doi: 10.1093/aob/mct007
Friberg, M., Waters, M. T., and Thompson, J. N. (2017). Nutrient availability affects floral scent much less than other floral and vegetative traits in Lithophragma bolanderi. Ann. Bot. 120, 471–478. doi: 10.1093/aob/mcx069
Frost, C. J., Mescher, M. C., Dervinis, C., Davis, J. M., Carlson, J. E., and Moraes, C. M. D. (2008). Priming defense genes and metabolites in hybrid poplar by the green leaf volatile cis-3-hexenyl acetate. New Phytol. 180, 722–734. doi: 10.1111/j.1469-8137.2008.02599.x
Galen, C., and Plowright, R. C. (1985). The effects of nectar level and flower development on pollen carry-over in inflorescences of fireweed (Epilobium angustifolium) (Onagraceae). Can. J. Bot. 63, 488–491.
Gross, K., and Schiestl, F. P. (2015). Are tetraploids more successful? Floral signals, reproductive success and floral isolation in mixed-ploidy populations of a terrestrial orchid. Ann. Bot. 115, 263–273. doi: 10.1093/aob/mcu244
Halverson, K., Heard, S. B., Nason, J. D., and Stireman, J. O. (2008). Differential attack on diploid, tetraploid, and hexaploid Solidago altissima L. by five insect gallmakers. Oecologia 154, 755–761. doi: 10.1007/s00442-007-0863-3
Huber, F. K., Kaiser, R., Sauter, W., and Schiestl, F. P. (2005). Floral scent emission and pollinator attraction in two species of Gymnadenia (Orchidaceae). Oecologia 142, 564–575. doi: 10.1007/s00442-004-1750-9
Husband, B. C. (2000). Constraints on polyploid evolution: a test of the minority cytotype exclusion principle. Proc. R. Soc. Lond. B Biol. Sci. 267, 217–223. doi: 10.1098/rspb.2000.0990
Husband, B. C., and Sabara, H. A. (2004). Reproductive isolation between autotetraploids and their diploid progenitors in fireweed, Chamerion angustifolium (Onagraceae). New Phytol. 161, 703–713. doi: 10.1046/j.1469-8137.2004.00998.x
Husband, B. C., and Schemske, D. W. (1998). Cytotype distribution at a diploid–tetraploid contact zone in Chamerion (Epilobium) angustifolium (Onagraceae). Am. J. Bot. 85, 1688–1694.
Husband, B. C., and Schemske, D. W. (2000). Ecological mechanisms of reproductive isolation between diploid and tetraploid Chamerion angustifolium. J. Ecol. 88, 689–701. doi: 10.1046/j.1365-2745.2000.00481.x
Husband, B. C., Ozimec, B., Martin, S. L., and Pollock, L. (2008). Mating consequences of polyploid evolution in flowering plants: current trends and insights from synthetic polyploids. Int. J. Plant Sci. 169, 195–206. doi: 10.1086/523367
Jersáková, J., Castro, S., Sonk, N., Milchreit, K., Schödelbauerová, I., Tolasch, T., et al. (2010). Absence of pollinator-mediated premating barriers in mixed-ploidy populations of Gymnadenia conopsea s.l. (Orchidaceae). Evol. Ecol. 24, 1199–1218. doi: 10.1007/s10682-010-9356-7
Junker, R. R., and Parachnowitsch, A. L. (2015). Working towards a holistic view on flower traits – How floral scents mediate plant–animal interactions in concert with other floral characters. J. Indian Inst. Sci. 95, 43–68.
Karban, R. (2020). The ecology and evolution of induced responses to herbivory and how plants perceive risk. Ecol. Entomol. 45, 1–9. doi: 10.1111/een.12771
Katzenberger, T., Lunau, K., and Junker, R. (2013). Salience of multimodal flower cues manipulates initial responses and facilitates learning performance of bumblebees. Behav. Ecol. Sociobiol. 67, 1587–1599. doi: 10.1007/s00265-013-1570-1
Kennedy, B. F., Sabara, H. A., Haydon, D., and Husband, B. C. (2006). Pollinator-mediated assortative mating in mixed ploidy populations of Chamerion angustifolium (Onagraceae). Oecologia 150, 398–408. doi: 10.1007/s00442-006-0536-7
Kessler, A., and Baldwin, I. T. (2001). Defensive function of herbivore-induced plant volatile emissions in nature. Science 291, 2141–2144.
Knauer, A. C., and Schiestl, F. P. (2015). Bees use honest floral signals as indicators of reward when visiting flowers. Ecol. Lett. 18, 135–143. doi: 10.1111/ele.12386
Knauer, A. C., and Schiestl, F. P. (2017). The effect of pollinators and herbivores on selection for floral signals: a case study in Brassica rapa. Evol. Ecol. 31, 285–304. doi: 10.1007/s10682-016-9878-8
Knudsen, J. T., Eriksson, R., Gershenzon, J., and Ståhl, B. (2006). Diversity and distribution of floral scent. Bot. Rev. 72, 1–120.
Kolář, F., Čertner, M., Suda, J., Schönswetter, P., and Husband, B. C. (2017). Mixed-ploidy species: progress and opportunities in polyploid research. Trends Plant Sci. 22, 1041–1055. doi: 10.1016/j.tplants.2017.09.011
Koski, M. H., and Ashman, T.-L. (2014). Dissecting pollinator responses to a ubiquitous ultraviolet floral pattern in the wild. Funct. Ecol. 28, 868–877. doi: 10.1111/1365-2435.12242
Krieger, G. M., Duchateau, M.-J., Van Doorn, A., Ibarra, F., Francke, W., and Ayasse, M. (2006). Identification of queen sex pheromone components of the bumblebee Bombus terrestris. J. Chem. Ecol. 32:453. doi: 10.1007/s10886-005-9013-8
Lack, A. J. (1982). The ecology of flowers of chalk grassland and their insect pollinators. J. Ecol. 70, 773–790. doi: 10.2307/2260104
Laport, R. G., Minckley, R. L., and Pilson, D. (2021). Pollinator assemblage and pollen load differences on sympatric diploid and tetraploid cytotypes of the desert-dominant Larrea tridentata. Am. J. Bot. 108, 297–308. doi: 10.1002/ajb2.1605
Levin, D. A. (1975). Minority cytotype exclusion in local plant populations. TAXON 24, 35–43. doi: 10.2307/1218997
Luizzi, V. J., Friberg, M., and Petrén, H. (2021). Phenotypic plasticity in floral scent in response to nutrient, but not water, availability in the perennial plant Arabis alpina (Brassicaceae). Funct. Ecol. 35, 1655–1665. doi: 10.1111/1365-2435.13866
Maherali, H., Walden, A. E., and Husband, B. C. (2009). Genome duplication and the evolution of physiological responses to water stress. New Phytol. 184, 721–731. doi: 10.1111/j.1469-8137.2009.02997.x
Maia, R., Gruson, H., Endler, J. A., and White, T. E. (2019). pavo 2: New tools for the spectral and spatial analysis of colour in R. Methods Ecol. Evol. 10, 1097–1107. doi: 10.1111/2041-210X.13174
Majetic, C. J., Fetters, A. M., Beck, O. M., Stachnik, E. F., and Beam, K. M. (2017). Petunia floral trait plasticity in response to soil nitrogen content and subsequent impacts on insect visitation. Flora 232, 183–193. doi: 10.1016/j.flora.2016.08.002
Martin, S. L., and Husband, B. C. (2013). Adaptation of diploid and tetraploid Chamerion angustifolium to elevation but not local environment. Evolution 67, 1780–1791. doi: 10.1111/evo.12065
Mosquin, T. (1966). A new taxonomy for Epilobium angustifolium L. (Onagraceae). Brittonia 18, 167–188. doi: 10.2307/2805200
Muñoz-Pajares, A. J., Perfectti, F., Loureiro, J., Abdelaziz, M., Biella, P., Castro, M., et al. (2018). Niche differences may explain the geographic distribution of cytotypes in Erysimum mediohispanicum. Plant Biol. 20, 139–147. doi: 10.1111/plb.12605
Münzbergová, Z., and Skuhrovec, J. (2017). Contrasting effects of ploidy level on seed production in a diploid–tetraploid system. AoB Plants 9:lw077. doi: 10.1093/aobpla/plw077
Münzbergová, Z., Skuhrovec, J., and Maršík, P. (2015). Large differences in the composition of herbivore communities and seed damage in diploid and autotetraploid plant species. Biol. J. Linn. Soc. 115, 270–287. doi: 10.1111/bij.12482
Nuismer, S. L., and Cunningham, B. M. (2005). Selection for phenotypic divergence between diploid and autotetraploid Heuchera grossulariifolia. Evolution 59, 1928–1935. doi: 10.1111/j.0014-3820.2005.tb01062.x
Oksanen, J., Blanchet, F. G., Friendly, M., Kindt, R., Legendre, P., McGlinn, D., et al. (2019). vegan: Community Ecology Package. R package version 2.5–6. Available online at: https://CRAN.R-project.org/package=vegan
Ollerton, J., Killick, A., Lamborn, E., Watts, S., and Whiston, M. (2007). Multiple meanings and modes: on the many ways to be a generalist flower. TAXON 56, 717–728. doi: 10.2307/25065855
Parachnowitsch, A. L., Manson, J. S., and Sletvold, N. (2019). Evolutionary ecology of nectar. Ann. Bot. 123, 247–261.
Parachnowitsch, A. L., Raguso, R. A., and Kessler, A. (2012). Phenotypic selection to increase floral scent emission, but not flower size or colour in bee-pollinated Penstemon digitalis. New Phytol. 195, 667–675. doi: 10.1111/j.1469-8137.2012.04188.x
Parisod, C., Holderegger, R., and Brochmann, C. (2010). Evolutionary consequences of autopolyploidy: research review. New Phytol. 186, 5–17. doi: 10.1111/j.1469-8137.2009.03142.x
Pegoraro, L., De Vos, J. M., Cozzolino, S., and Scopece, G. (2019). Shift in flowering time allows diploid and autotetraploid Anacamptis pyramidalis (Orchidaceae) to coexist by reducing competition for pollinators. Bot. J. Linn. Soc. 191, 274–284. doi: 10.1093/botlinnean/boz043
Pinno, B. D., Landhäusser, S. M., Chow, P. S., Quideau, S. A., and MacKenzie, M. D. (2013). Nutrient uptake and growth of fireweed (Chamerion angustifolium) on reclamation soils. Can. J. For. Res. 44, 1–7. doi: 10.1139/cjfr-2013-0091
Porturas, L. D., Anneberg, T. J., Curé, A. E., Wang, S., Althoff, D. M., and Segraves, K. A. (2019). A meta-analysis of whole genome duplication and the effects on flowering traits in plants. Am. J. Bot. 106, 469–476. doi: 10.1002/ajb2.1258
R Core Team (2021). R: A Language and Environment for Statistical Computing. Vienna: R Foundation for Statistical Computing. Available online at: https://www.R-project.org/.
Raabová, J., Fischer, M., and Münzbergová, Z. (2008). Niche differentiation between diploid and hexaploid Aster amellus. Oecologia 158, 463–472. doi: 10.1007/s00442-008-1156-1
Raguso, R. A. (2008). Wake up and smell the roses: the ecology and evolution of floral scent. Annu. Rev. Ecol. Evol. Syst. 39, 549–569. doi: 10.1146/annurev.ecolsys.38.091206.095601
Raguso, R. A., and Pellmyr, O. (1998). Dynamic headspace analysis of floral volatiles: a comparison of methods. Oikos 81:238. doi: 10.2307/3547045
Ramsey, J., and Ramsey, T. S. (2014). Ecological studies of polyploidy in the 100 years following its discovery. Philos. Trans. R. Soc. B Biol. Sci. 369:20130352. doi: 10.1098/rstb.2013.0352
Rering, C. C., Beck, J. J., Vannette, R. L., and Willms, S. D. (2018). Quantitative assessment of nectar microbe-produced volatiles. ACS Symp. Ser. 1294, 127–142. doi: 10.1021/bk-2018-1294.ch010
Rieseberg, L. H., and Willis, J. H. (2007). Plant speciation. Science 317, 910–914. doi: 10.1126/science.1137729
Roccaforte, K., Russo, S. E., and Pilson, D. (2015). Hybridization and reproductive isolation between diploid Erythronium mesochoreum and its tetraploid congener E. albidum (Liliaceae). Evolution 69, 1375–1389. doi: 10.1111/evo.12666
Sabara, H. A., Kron, P., and Husband, B. C. (2013). Cytotype coexistence leads to triploid hybrid production in a diploid–tetraploid contact zone of Chamerion angustifolium (Onagraceae). Am. J. Bot. 100, 962–970. doi: 10.3732/ajb.1200583
Schepper, S., Leus, L., Mertens, M., Debergh, P., Van Bockstaele, E., and Loose, M. (2001). Somatic polyploidy and its consequences for flower coloration and flower morphology in azalea. Plant Cell Rep. 20, 583–590. doi: 10.1007/s002990100372
Schiestl, F. P., and Johnson, S. D. (2013). Pollinator-mediated evolution of floral signals. Trends Ecol. Evol. 28, 307–315. doi: 10.1016/j.tree.2013.01.019
Segraves, K. A. (2017). The effects of genome duplications in a community context. New Phytol. 215, 57–69. doi: 10.1111/nph.14564
Segraves, K. A., and Anneberg, T. J. (2016). Species interactions and plant polyploidy. Am. J. Bot. 103, 1326–1335. doi: 10.3732/ajb.1500529
Segraves, K. A., and Thompson, J. N. (1999). Plant polyploidy and pollination: floral traits and insect visits to diploid and tetraploid Heuchera grossulariifolia. Evolution 53, 1114–1127. doi: 10.1111/j.1558-5646.1999.tb04526.x
Skorupski, P., and Chittka, L. (2010). Photoreceptor spectral sensitivity in the Bumblebee, Bombus impatiens (Hymenoptera: Apidae). PLoS One 5:e12049. doi: 10.1371/journal.pone.0012049
Soltis, D. E., Albert, V. A., Leebens-Mack, J., Bell, C. D., Paterson, A. H., Zheng, C., et al. (2009). Polyploidy and angiosperm diversification. Am. J. Bot. 96, 336–348. doi: 10.3732/ajb.0800079
Soltis, D. E., Soltis, P. S., Schemske, D. W., Hancock, J. F., Thompson, J. N., Husband, B. C., et al. (2007). Autopolyploidy in angiosperms: have we grossly underestimated the number of species? Taxon 56, 13–30. doi: 10.2307/25065732
Spoelhof, J. P., Soltis, P. S., and Soltis, D. E. (2017). Pure polyploidy: closing the gaps in autopolyploid research. J. Syst. Evol. 55, 340–352. doi: 10.1111/jse.12253
Sutherland, B. L., Miranda-Katz, T., and Galloway, L. F. (2020). Strength in numbers? Cytotype frequency mediates effect of reproductive barriers in mixed-ploidy arrays. Evolution 74, 2281–2292. doi: 10.1111/evo.14077
Svensson, G. P., Hickman, M. O. Jr., Bartram, S., Boland, W., Pellmyr, O., and Raguso, R. A. (2005). Chemistry and geographic variation of floral scent in Yucca filamentosa (Agavaceae). Am. J. Bot. 92, 1624–1631.
Thompson, J. N., and Merg, K. F. (2008). Evolution of polyploidy and the diversification of plant-pollinator interactions. Ecology 89, 2197–2206.
Thompson, K. A., Husband, B. C., and Maherali, H. (2014). Climatic niche differences between diploid and tetraploid cytotypes of Chamerion angustifolium (Onagraceae). Am. J. Bot. 101, 1868–1875. doi: 10.3732/ajb.1400184
Thompson, K. A., Husband, B. C., and Maherali, H. (2015). No influence of water limitation on the outcome of competition between diploid and tetraploid Chamerion angustifolium (Onagraceae). J. Ecol. 103, 733–741. doi: 10.1111/1365-2745.12384
Trunschke, J., Lunau, K., Pyke, G. H., Ren, Z.-X., and Wang, H. (2021). Flower color evolution and the evidence of pollinator-mediated selection. Front. Plant Sci. 12:617851. doi: 10.3389/fpls.2021.617851
Vallejo-Marín, M., Cooley, A. M., Lee, M. Y., Folmer, M., McKain, M. R., and Puzey, J. R. (2016). Strongly asymmetric hybridization barriers shape the origin of a new polyploid species and its hybrid ancestor. Am. J. Bot. 103, 1272–1288. doi: 10.3732/ajb.1500471
van der Kooi, C. J., Dyer, A. G., Kevan, P. G., and Lunau, K. (2019). Functional significance of the optical properties of flowers for visual signalling. Ann. Bot. 123, 263–276. doi: 10.1093/aob/mcy119
Keywords: fireweed, assortative mating, floral scent, volatiles, reflectance, Apis visual model
Citation: Palmqvist B, Brazeau HA and Parachnowitsch AL (2021) Differences in Floral Scent and Petal Reflectance Between Diploid and Tetraploid Chamerion angustifolium. Front. Ecol. Evol. 9:734128. doi: 10.3389/fevo.2021.734128
Received: 30 June 2021; Accepted: 13 September 2021;
Published: 04 October 2021.
Edited by:
Casper J. Van Der Kooi, University of Groningen, NetherlandsReviewed by:
Karin Gross, University of Salzburg, AustriaJoão Marcelo Robazzi Bignelli Valente Aguiar, University of São Paulo, Brazil
Zong-Xin Ren, Kunming Institute of Botany, Chinese Academy of Sciences (CAS), China
Copyright © 2021 Palmqvist, Brazeau and Parachnowitsch. This is an open-access article distributed under the terms of the Creative Commons Attribution License (CC BY). The use, distribution or reproduction in other forums is permitted, provided the original author(s) and the copyright owner(s) are credited and that the original publication in this journal is cited, in accordance with accepted academic practice. No use, distribution or reproduction is permitted which does not comply with these terms.
*Correspondence: Amy L. Parachnowitsch, YXBhcmFjaG5AdW5iLmNh