Competition Drives Group Formation and Reduces Within Nest Relatedness in a Facultatively Social Carpenter Bee
- Department of Biological Sciences, Brock University, St. Catharines, ON, Canada
Animals respond to competition among kin for critical breeding resources in two ways: avoidance of direct fitness costs via dispersal of siblings to breed separately, and formation of kin-based societies in which subordinates offset direct fitness costs of breeding competition via altruism and increased indirect fitness. In the facultatively social eastern carpenter bee, nests are a critical breeding resource in perpetually short supply, leading to strong competition among females. Observations of individually marked and genotyped females in conditions of high and low resource competition demonstrate that competition leads to resource sharing and group nesting. However, in contrast to almost all known animal societies, females avoid nesting with relatives, and disperse from their natal nests to join social groups of non-relatives. This is the first example of a structured insect society with cooperation nestmates, the majority of which are unrelated; thus cooperation is more likely based on selection for direct, rather than indirect fitness. By forming social groups of non-kin, females avoid the indirect fitness costs of kin competition among sisters, yet increase their chances of successful reproduction, and thus direct fitness, when forming colonies of non-relatives.
Introduction
Ultimately, the evolution of social behavior in animals is shaped by competition for crucial resources linked to reproduction, including such things as food or breeding sites. One way for animals to improve their access to breeding resources is by forming coalitions or groups of cooperative individuals that work together to obtain and share resources. Cooperative and helping behaviors are a major reason why groups of individuals can have higher per-capita fitness than solitary individuals (Rubenstein and Abbot, 2017). However, group-living does not shield animals from resource competition: dominant individuals often take proportionally greater shares of crucial breeding resources and get more breeding opportunities than subordinates (Ridley and Sutherland, 2002; Bach et al., 2006; Johnstone, 2008). As a result, while dominant individuals living groups may have significantly higher inclusive fitness than individuals breeding alone, the opposite may be true for non-breeding subordinates, whose inclusive fitness is lower than it would be if they bred independently (Richards et al., 2005). In social insects, subordinate females have several options that potentially improve their inclusive fitness by leaving their natal group to breed elsewhere: they can wait for opportunities to replace dominant reproductives (e.g., replacement queens in eusocial sweat bees; Awde and Richards, 2018), they can leave the natal nest and found their own nests elsewhere (Schwarz et al., 2007), or they can join a different breeding group which offers better reproductive opportunities (Leadbeater et al., 2010, 2011). The common thread here is that subordinates can increase the direct component of inclusive fitness by availing themselves of opportunities to lay eggs and raise brood, in their natal nest or elsewhere.
Facultatively social animals are ideal models for examining how resource competition influences the fitness balance between cooperation and competition, because they exemplify the costs and benefits of solitary vs. social living under varying ecological conditions (Schwarz et al., 2007; Wcislo and Fewell, 2017). Inter-individual competition should be most severe when critical resources are scarce, when population density is high, or both; competition for limited resources such as breeding sites should be particularly severe (Platt and Bever, 2009). In facultatively social bees, subordinate individuals with few opportunities for direct reproduction in their natal nests may be able to nest elsewhere, either by founding a new nest or joining a breeding group in which they will have more chances to lay eggs (Field et al., 2006).
The eastern carpenter bee, Xylocopa virginica, is a facultatively social species with a distribution that spans a large portion of eastern North America, from Florida in the south and southern Ontario in the north (Skandalis et al., 2011). They are floral generalists, but nest site specialists, and nests are costly to construct in terms of both time and energy. The value of the nest is demonstrated by the fact that frequently, they are occupied by successive generations of females, sometimes for decades (Rau, 1933). Eastern carpenter bees can nest solitarily or socially, and when social, females form small groups of two to five females, although there can be as many as eight (Gerling and Hermann, 1978; Vickruck and Richards, 2018). Females who are part of a social nest demonstrate one of three reproductive strategies (Richards, 2011). Dominant, primary females monopolize both foraging and reproduction and are first in a linear queue for reproductive opportunities, as only one female at a time is reproductive. Secondary females “wait” behind the dominant for opportunities to replace her, which can happen if she dies, becomes moribund, or occasionally, if the secondary aggressively usurps the dominant position (Vickruck and Richards, 2018). Finally, there are tertiary females, which are not a part of the reproductive queue and almost never leave the nest. These females do not reproduce even if primaries and secondaries are removed from the nest. By avoiding the physiological costs and risks of flight activity in their first year, they essentially double their life span and are able to successfully overwinter twice. They become primaries or secondaries in year two (Vickruck and Richards, 2018). From our experience, every social nest that was not newly constructed contains a tertiary female. Queue position is determined in early spring during a period referred to as the nestmate provisioning phase (NPP) when females often engage in aggressive in nest interactions as well as feeding each other (Richards and Course, 2015; Vickruck and Richards, 2017b). Females destined to be dominant and secondary females are often seen outside their natal nests at this time, briefly entering neighboring nests and presumably assessing whether it is best to remain in the reproductive queue in their natal nest or attempt to join a breeding group in another nest nearby. It is during this period that many females relocate to new nests (Peso and Richards, 2010). Thus, the breeding behavior of a facultatively social bee provides an ideal empirical model for evaluating the fitness consequences of cooperation and competition in shaping social behavior and group formation.
In this paper, we examine the behavioral consequences of high and low population density on Xylocopa virginica in two consecutive breeding seasons. We predicted that high population density would create more competition for nests and therefore more frequent social nesting and larger average colony size, as well as more competition for reproductive opportunities among females within breeding groups. We demonstrate that intense competition among females for nest sites increased both the frequency of cooperation (represented by nest-sharing) and the intensity of within-group competition for breeding opportunities. We genotyped females at 9 microsatellite loci to examine genetic relatedness among nestmates and used a randomization analysis to demonstrate that females were dispersing from their natal nests to ones that did not contain full siblings.
Materials and Methods
Xylocopa virginica Colony Cycle
Eastern carpenter bees overwinter as adults in their natal nests, with females emerging from hibernation in spring (late April or early May in southern Ontario). The activity patterns of eastern carpenter bees are unusual for social bees, with two foraging flight periods (Richards and Course, 2015). The first phase is the nestmate provisioning phase (NPP) in which females bring back pollen to feed adult nestmates. During this time, many females make flights outside the nest, as whether they become the dominant or secondary is yet to be determined. The exception are tertiary females, who will not leave the nest unless they are the last remaining female, and if they do, never make a pollen trip (Vickruck and Richards, 2018). This behavior allows us to mark the vast majority of the females in an aggregation during the NPP. The second phase is the brood provisioning phase (BPP) in which the dominant female (also known as the principal forager) provisions brood cells on which only she lays eggs. If the dominant female dies, disappears or becomes moribund, the next female in line replaces her as the principal forager and egg-layer (Richards and Course, 2015; Vickruck and Richards, 2018). During NPP and as late as BPP, subordinate females often left their natal nests to join neighboring nests in the same aggregation, other aggregations, or in another location. Females were additionally categorized as resident or transient based on behavioral observations over the course of the foraging season. Resident females did not disperse and were only ever seen entering and exiting one nest during the BPP, while transient females dispersed from the nest in which they overwintered and were observed in more than one nest across the season. Transient females were only classified as such if they left their natal nest and permanently moved to a new nest. It was common for females to “shop around” for nests in the aggregation, sticking their heads inside multiple nests before deciding on a new nest, or ultimately returning to their home nest. Brood provisioning ceases in early July, after which adult females remain inside their nests with developing brood. When brood eclose at the end of the summer, they may be fed by older adult females that leave the nests during a brief, late summer foraging phase (late August or early September in southern Ontario) or leave to feed themselves before returning to their natal nest. The newly eclosed adults, both male and female, remain in their natal nests throughout the winter until the following spring.
In our study populations in southern Ontario, most females live 1 year, breeding in their first spring and summer following eclosion. The exception are the tertiary females, who do not forage in their first season, and are able to overwinter a second time. In more than 7 years of intensive behavioral observations, no female has ever been observed to forage in 2 consecutive years, so lifetime reproductive success is equivalent to the number of brood produced in one foraging season.
Description of Field Sites
We studied five nesting aggregations of Xylocopa virginica, each one located in a wooden bridge at the Glenridge Quarry Naturalization Site (GQNS), in St. Catharines, Ontario, Canada (43.122, -79.236 decimal degrees). This location is close to the northern edge of the species’ range, with shorter flight seasons and much more severe winters than in southern locations where it has been previously studied (Gerling and Hermann, 1978). During the summers of 2012 and 2013, each bridge was home to between 10 and 22 nests. Bridges were constructed in 2003 and were available for the bees to use as nesting substrate beginning the spring of 2004. Eastern carpenter bees often reuse nests for many years. The term “new nest” refers to nests that were constructed in the current observation year, while “old nest” refers to nests that are being reused from previous years.
Bee Handling and Observations
In early spring, bees were trapped at their nest entrances using cup traps (Peso and Richards, 2011) on their first venture outside their natal nests. Cup traps are medium sized plastic cups that have a small hole cut out of the bottom while the top is covered with parafilm. The end with the small hole is then secured over the nest entrance with Velcro. Bees leave the nest but become trapped in the cup. Unmarked females were immediately placed on ice for approximately 10 min to allow for individual marking and measurements to be taken. Each female was individually marked on the thorax with a unique two-color combination using enamel model paint and her head width was measured across the widest part of her head, as a proxy for body size comparisons. The last tarsal segment of the left mesothoracic leg was removed and placed in chilled 100% redistilled ethanol for genotyping at a later date. Marked and measured bees were placed back outside of their nests to warm up and resume activity. Evidence from previous studies indicates that marking bees does not prevent them from relocating their nests (Richards and Course, 2015).
Observations of foraging females were used to determine which females were dominants (principal foragers) and which were subordinates. Foraging observations lasted from 08:00 h to 16:00 h during the NPP on days when there was no rain and the temperature was above 20°C. Time and nest of departure as well as the bee’s individual paint ID were recorded for each bee leaving the nest. Bees were then released and the trap was replaced over the nest entrance. When a bee returned to the nest, the trap was removed to allow her entry. The time of her return, the nest to which she returned, as well as whether or not she was carrying pollen was recorded. At the end of the day all cup traps were removed for the night. Observations ceased for the season when an entire observation day passed (8 h) without seeing a single pollen trip by a female bee, indicating that the BPP was complete.
Assigning Nest Status
Nest status (social or solitary) was determined by observations across the entire season. Each time a female departed from the nest a small, flexible plastic transfer pipette was inserted in the nest entrance. If there was still a female present in the nest she would buzz, bite the pipette tip or block the entrance with her abdomen. The presence or absence of a guarding female was recorded and used to determine if the nest was social or solitary. Nests were classified as solitary if during the BPP only one female was ever seen bringing pollen to the nest and a second bee was never observed guarding the nest entrance. Nests were classified as social if more than one female was recorded in the nest during the BPP.
Genetic Analyses and Relatedness Calculations
DNA extraction and genotyping procedures are described in Vickruck (2014). In 2012, 189 females from 71 nests were genotyped. In 2013, 101 females from 64 nests were genotyped. Sixteen females were excluded from analyses of relationships in 2012 and 8 in 2013 due to missing data at more than 2 loci. To assess the relatedness among female nestmates during the winter, 19 nests were destructively opened in March of 2012 to reveal natal nestmates prior to emergence from hibernation and spring dispersal. Nests were opened by carefully planning away layers of wood to expose overwintering bees. All individuals inside nests were measured, marked and a tarsal sample was taken using the same techniques as used for summer bees. Because this procedure is destructive, it was only done for one time point so as not to destroy too many nests in our focal population.
Relatedness among female nestmates was calculated using the method described by Queller (1989) as implemented in the program Kingroup V2 (Konovalov et al., 2004). Kingroup V2 allowed us to differentiate which pairs of bees within nests were significantly more likely to be full sisters than unrelated pairs. Hymenoptera are haplodiploid (females are diploid while males are haploid) therefore full sisters inherit one of two maternal alleles and must inherit the single paternal allele. When comparing full sisters, this means that full sisters must share the paternal allele at all loci. We examined relatedness among nestmates at three successive time points: in late winter when females were still in their natal nests; in spring during the nestmate provisioning period, when females establish reproductive queues in social nests; and in summer during the brood provisioning period when dominant females provision their brood and lay eggs.
We created a randomization analysis to determine whether the observed numbers of sisters nesting together in each nest was different from the number of sisters that would be observed together if females were randomly distributed among nests. To do this we assigned all females marked in 2012 or 2013 to simulated nests at random. In each sample year, the number of nests as well as the size of each nest (the number of females recorded inside) was replicated exactly as observed in the sample population. After females were randomly assorted into nests, we used Kingroup V2 (Konovalov et al., 2004) to determine how many full sister pairs were present in simulated nests, as well as how many simulated nests contained full siblings. We then repeated this procedure 100 times for both the 2012 and 2013 datasets. Simulation results were used to create distributions for the expected number of siblings in nests and the number of nests that contained full siblings given the bees in the population for both 2012 and 2013. We then compared our observed values to the expected distributions of our randomization analysis to quantify the probability of our observations given the simulated data.
Results
Evidence for Resource Competition Among Females
Since we caught and individually marked almost every individual bee that emerged from our study nests, population density was measured as the total number of bees marked each year (summarized in Table 1). In 2012 the population contained 189 females that occupied 70 nests (high density), while in 2013 it contained only 101 females in 65 nests (low density).
Under crowded, high density conditions, female bees should be more likely to share nests. As predicted, group size was strongly associated with population density (Figure 1). In high density conditions (2012), 96% of overwintered females nested socially (in groups) and average group size was significantly higher than in 2013 when population density was much lower and only 70% of females nested socially (Table 1). In 2012, females were also more likely to relocate from their natal nest, either to another nest in the population, to a location outside of the study area, or disappear from the population entirely (Table 1). Also in 2012, 9 females initiated new nests, a rate of new nest construction unprecedented at this location over 7 years of observations (2011–2013, 2016–2019); no new nests were initiated in 2013. In every instance of nest initiation observed at our study sites over about 7 years (several dozen examples), a single female excavated the nest entrance and the first sections of tunnel by herself. Thus, nest initiation is a solitary activity, although additional females frequently join the new nest within a day or two of nest entrance completion. The significant differences in group size between 2012 and 2013 demonstrate density dependence of social group formation, with strong competition for nest sites inducing higher rates of dispersal, higher rates of nest construction, higher rates of social group formation, and increased social group size.
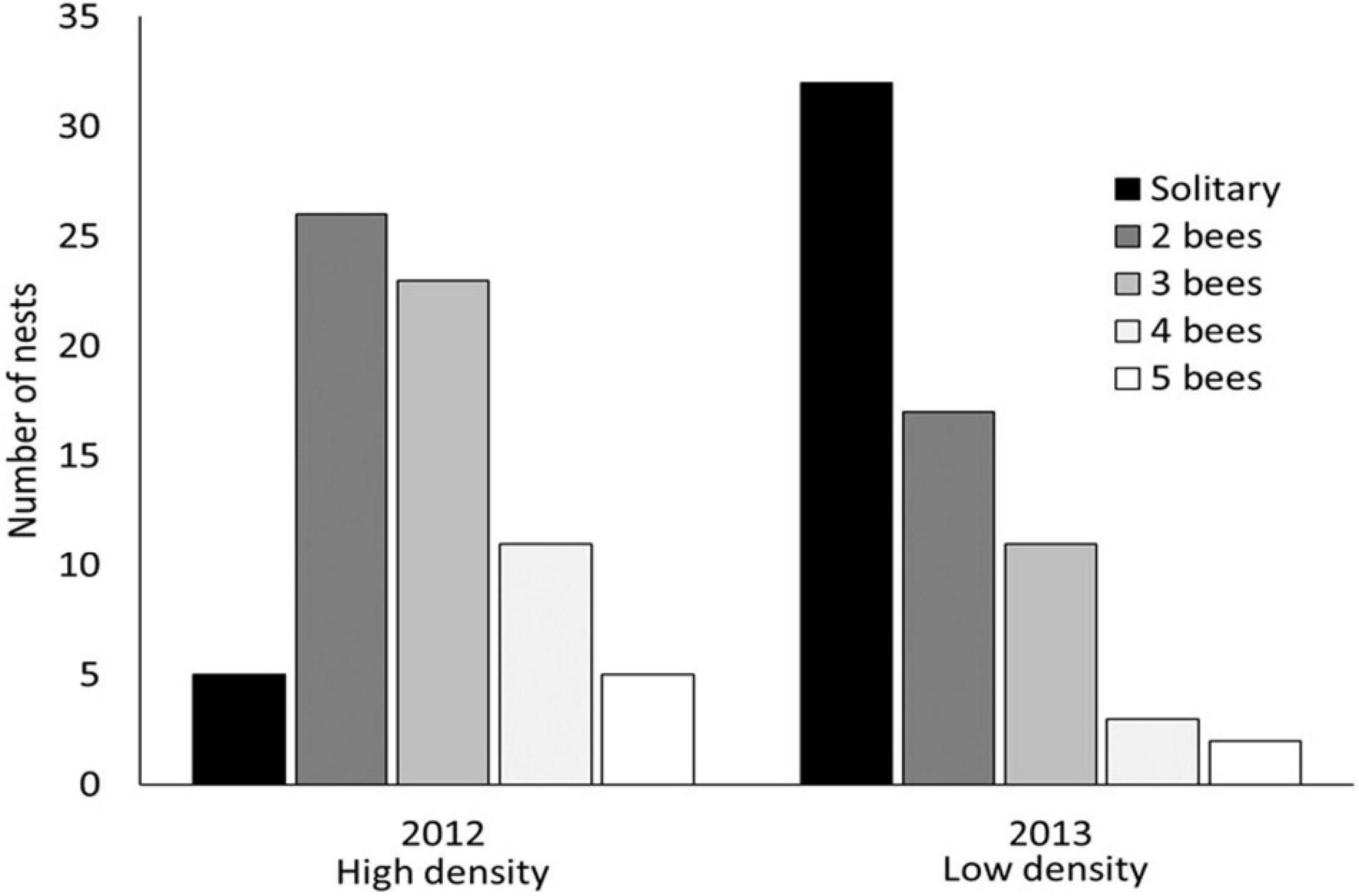
Figure 1. Variation in group size during the brood provisioning phase of the colony cycle in response to high (2012) and low (2013) population density. In low density, significantly more females nested solitarily (Table 1, P < 0.00001, also see Supplementary Table 1). In both 2012 and 2013, the maximum colony size during the brood provisioning phase was 5 females.
Whereas group size was significantly higher in 2012 than in 2013, the duration of the BPP of the colony cycle was the same (Table 1). As a result, fewer subordinates would have achieved egg-layer status under high density, compared to low density.
Relatedness Among Nestmates
Relatively high relatedness among cohabitating females in winter suggests that many females could have formed kin groups in spring by remaining together in their natal nests, yet the proportion of full sisters in colonies declined significantly from winter through spring to summer (Figure 2 and Table 2), indicating that sibships were broken up as females dispersed to nests in spring. We therefore identified all possible full sisters for each genotyped female in the population and investigated whether they nested together or apart (Figure 3). Of 266 genotyped females that were still alive at the time of brood provisioning and egg-laying, only 30 sisters (11%) nested together, 178 sisters (67%) nested apart (in different nests), and 58 individuals (22%) did not have a full sister in the population (Figure 3). There was no effect between years (Figure 3). The proportion of sisters nesting together in summer was compared to the number that would be expected under a null hypothesis in which females were randomly distributed among nests (Supplementary Figure 1). The observed proportions of co-nesting sisters were not significantly different from random expectation.
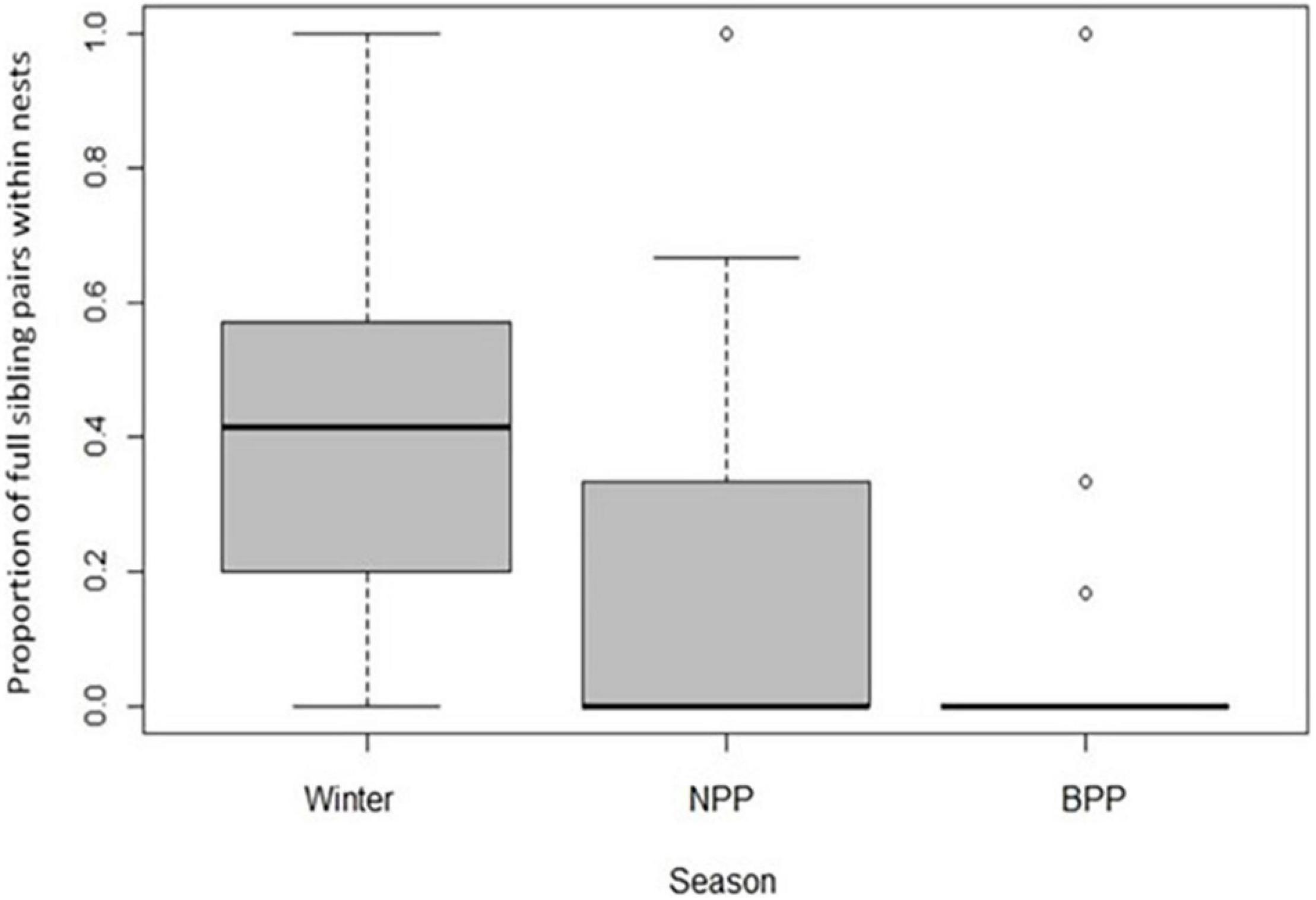
Figure 2. Decline in kinship among social nestmates prior to the formation of breeding associations. Winter associations represent natal nestmates, since bees overwinter in their natal nests. The proportion of nest mate pairs that were full sisters decreased significantly from winter, through the spring nestmate provisioning phase (NPP) to the summer brood provisioning phase (BPP) (Kruskal-Wallis X2 = 13.01, d.f. = 2, P = 0.001). Boxes-and-whiskers represent median and quartile ranges, while open circles represent outliers.
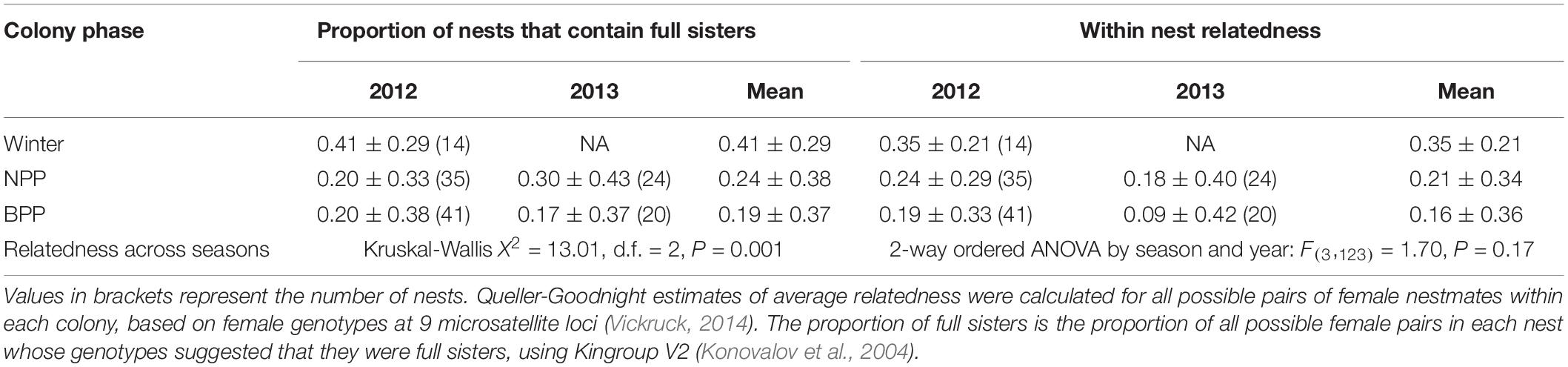
Table 2. Decline in mean relatedness among nestmates from the late winter hibernation phase to nestmate provisioning phase (NPP) to brood provisioning phase (BPP).
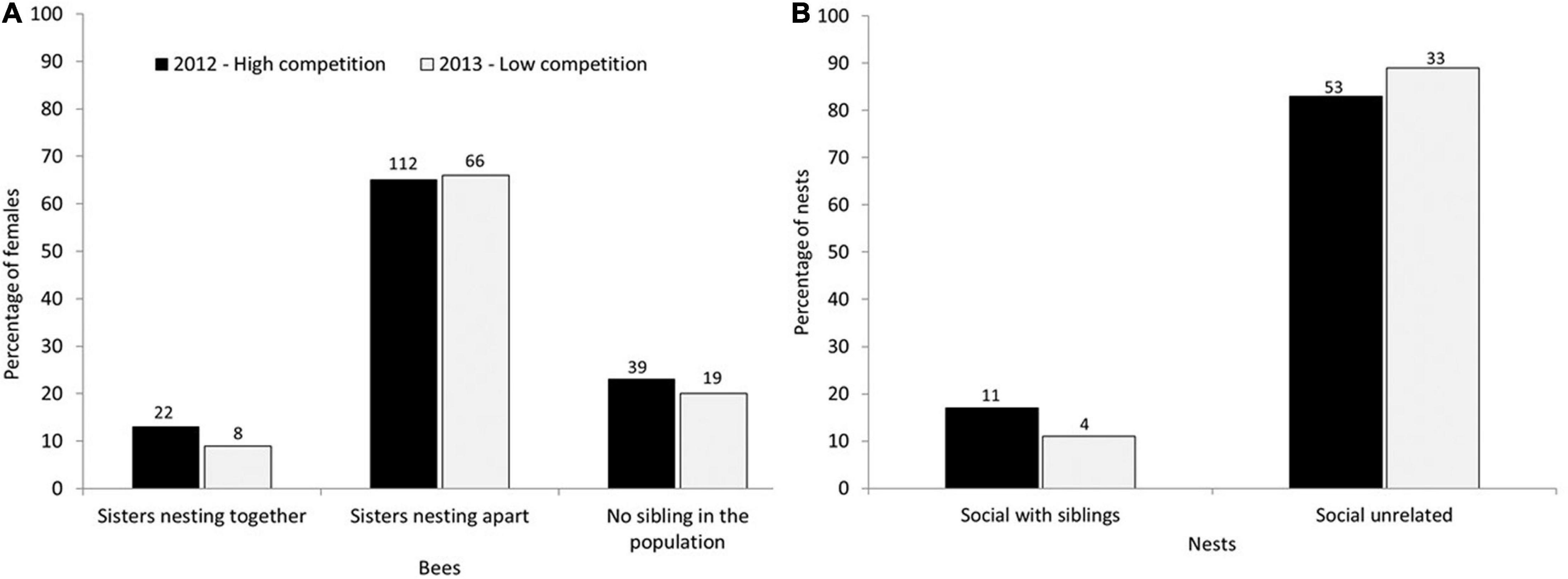
Figure 3. Evidence for avoidance of competition by adult females during the brood provisioning period. (A) Although many females had at least one full sister in the population, most females nested apart from their sisters, rather than remaining together in the natal nest or dispersing together to a new nest. The proportions of sisters nesting together or apart did not differ between 2012 and 2013 (X2 = 1.38, d.f. = 2, P = 0.50), suggesting that changes in population density did not influence the relatedness structure of colonies. (B) The proportion of social colonies that contained full sisters was also similar in 2012 and 2013 (Fisher’s exact test, P = 0.48). Numbers above the bars represent the number of individuals (A) or nests (B).
Discussion
Resource Competition Influences the Frequency of Social Nesting
In general, competition for resources is higher when population densities increase or when resources become scarcer (Moore et al., 2006; Platt and Bever, 2009). For large carpenter bees in general, and eastern carpenter bees in particular, nests are a critical breeding resource, costly to produce and in perpetually short supply (Ostwald et al., 2021a). Eastern carpenter bees forage on a wide variety of blossom types, but their nests are almost always found in structures built of milled lumber, especially pine and spruce (Hurd, 1978); thus they are foraging generalists but nesting substrate specialists (Vickruck and Richards, 2017a). They are strongly philopatric and nesting aggregations persist for years or even decades, as successive generations of females reuse nests (Rau, 1933; Gerling and Hermann, 1978; Richards and Course, 2015). Nests are very costly to construct; a female that initiates nest construction may take up to a week to construct a nest with a single tunnel, during a BPP that lasts only 3–6 weeks (note that nests are never founded jointly). As a result, most females attempt to breed in their natal nest or relocate to existing nests close by, usually in the same aggregation (Peso and Richards, 2011).
While population-level competition for available nest sites is the critical factor driving group formation in X. virginica, it also intensified within-group competition for breeding opportunities. When groups are larger, reproductive queues are longer, and subordinates in lower queue positions are less likely to achieve egg-layer status (Richards and Course, 2015). In our study population, subordinate females in queue positions 2 and 3 may eventually become principal foragers, as primary females (rank 1) often die before the end of the BPP (Richards and Course, 2015). However, females in ranks 4–6 never moved up to rank 1. Since dominant and secondary females virtually never forage in two consecutive breeding seasons (Vickruck and Richards, 2018), direct fitness for these two reproductive strategies is completely predicated upon raising brood in the current breeding season. Therefore, in a social nest of full sisters, individuals ranked lower in the queue would have no direct fitness. By relocating to a new nest and hopefully improving their ranks, a female would increase her direct fitness, while still maintaining the indirect fitness benefits of her egg laying sister in another nest. The significantly longer reproductive queues of 2012 would have led to significantly lower average direct fitness of subordinate females that year.
Unrelated Females Form Breeding Groups
Our results indicate that siblings overwintered together but rarely nested together. Kin relationships were broken up in spring as many females relocated to new nests. In low density conditions, such as those of 2013, females often were able to nest alone, either by relocating to a new nest or forcing their nestmates out, but in high density conditions, relocating females joined breeding groups of non-relatives. Most females take at least one flight during the NPP at the beginning of the season, which affords them the opportunity to assess the level of competition both within their home nest, as well as nests in the surrounding aggregation. This suggests that dispersal from the natal nest is a mechanism by which females solve the problem of severe competition for reproductive opportunities. This competitive cost is also mediated by the potential increase in direct fitness benefits from a female improving her position in the reproductive queue. A previous study demonstrated that transient females were as likely as resident females to become dominants in social nests, which means that transient females can successfully usurp primary queue positions (Richards and Course, 2015). The phenomenon of females joining nests of unrelated individuals, once thought to be an exception to the rule, is being noted more and more among social insects (Sumner et al., 2007; Peso and Richards, 2010; Leadbeater et al., 2011; Grinsted and Field, 2018). Indeed, relatedness among nestmates in Xylocopa sonorina is also highly variable, with many social nests comprised of unrelated individuals (Ostwald et al., 2021a, this issue). Recent modeling has demonstrated that species may be best to maximize high relatedness or low relatedness (social heterosis) to increase overall fitness (Nonacs, 2017). We would like to emphasize that we are not claiming that females are choosing new colonies randomly, but that their distribution among breeding groups appears to be random with respect to familial patterns.
While nesting with non-relatives means that transient females still incur the direct fitness costs of breeding competition within groups, it does allow them to reduce the indirect fitness costs of breeding competition with relatives. A female that manages to improve her queue position by moving to a new nest potentially increases her own direct fitness, but also avoids the cost of competing with siblings for reproductive opportunities. On average then, inclusive fitness should be higher for females that avoid sibling competition in their natal nests and move into nests occupied by non-relatives. Behavioral evidence demonstrates that females frequently investigate multiple new nests and groups before permanently relocating; even dominant breeders occasionally “visit” neighboring nests, sometimes spending several hours inside (Richards and Course, 2015). Another means by which females might avoid competing with siblings is by breeding in separate years. Tertiary females postpone reproduction until their second spring (Vickruck and Richards, 2018), perhaps avoiding competition with sisters that bred in their first year.
Since most social insects live in kin groups, most cooperative and helpful behaviors are directed at relatives. Eastern carpenter bees provide evidence of cooperation and helping behavior in non-kin groups, although the extent of their cooperative behavior is certainly more limited than in eusocial bees. Female carpenter bees recognize and are more tolerant toward nestmates and more aggressive to non-nestmates (Peso and Richards, 2011; Vickruck and Richards, 2017b). Both dominant and subordinate females have been observed to guard nest entrances against conspecific intruders. Perhaps the most dramatic example of cooperation occurs in early spring, prior to the onset of egg-laying and brood provisioning, when dominant females forage for pollen that they feed to subordinate nestmates, especially the lowest-ranking tertiary females that rarely leave the nest (Richards, 2011; Vickruck and Richards, 2017b). Such observations demonstrate that cooperative behavior does exist in insect societies in which most of the social group are unrelated.
Although this is a rare example of a structured insect society in which group members are mostly unrelated, there are intriguing hints in other social insects that non-kin-based sociality may often have been overlooked. To start with, many carpenter bees are facultatively social, nesting either solitarily or in small groups, sometimes as few as two females, in which only one female lays eggs, while other females await their turn to become the primary reproductive (Hogendoorn and Velthius, 1999; Ostwald et al., 2021b). Another carpenter bee, Xylocopa sonorina, also forms social groups comprised mostly of unrelated individuals (Ostwald et al., 2021a, this issue). Accumulating behavioral evidence suggests that some euglossine bees are facultatively social, forming social groups that resemble reproductive queues and which may include non-kin (Nascimento and Andrade-Silva, 2012). Recent phylogenetic evidence suggests that societies based on reproductive queues in which subordinates wait for their own reproductive opportunities could represent an early stage in evolutionary transitions to caste-based sociality, in which subordinates, sacrifice their own reproduction to aid kin (Schwarz et al., 2011; Richards, 2019). In recent years, considerable evidence has accumulated that subordinate individuals, variously known as “drifters,” “aliens,” or “joiners” leave their natal colonies and join unrelated colonies (Field et al., 2006; Brahma et al., 2019). Some wait for opportunities to inherit the role of dominant egg-layer (the “queen” role in eusocial colonies). Whether such joiners actively avoid moving to nests with relatives should be investigated. In many ants, unrelated gynes cooperate to initiate new nests, but once the first workers emerge, they aggressively and often violently, compete for dominance, with a single female becoming the colony’s egg-laying queen (Haney and Fewell, 2018).
A rarely considered aspect of competition within social groups for resources critical to reproduction, is the effect of kin competition. Competition within breeding groups has different consequences for kin and non-kin. When individuals acquire disproportionately large shares of critical resources, they increase their own direct fitness at the expense of less competitive individuals. Competition among kin adds another layer of complexity: the increase in direct fitness experienced by dominant individuals may be obtained at the cost of lower direct fitness for related subordinates (Bach et al., 2006; Johnstone, 2008), so dominant individuals that harm kin achieve lower inclusive fitness than dominant individual that harm non-kin. Some theory predicts that the consequences of competition for resources within kin groups can be severe enough to “totally negate” the inclusive fitness benefits of kin cooperation (West et al., 2001; Bourke, 2011). The costs of kin competition can be avoided if related individuals disperse; indeed most empirical studies of kin competition and its effects have focused on how kin competition influences pre-breeding dispersal (Moore et al., 2006). However, dispersal has the additional effects of lowering opportunities for cooperative interactions that might increase access to breeding resources, as well as decreasing population viscosity and relatedness among potential cooperators. Therefore, dispersal in response to kin competition decreases the inclusive fitness costs of kin competition, but also decreases the inclusive fitness payoffs of cooperation and altruism (Bourke, 2011).
Eastern carpenter bees demonstrate alternative solutions to ways of coexisting in social groups when there is a division of labor. When the availability of nests is high, females can avoid competition by nesting solitarily, and when availability is low, they can nest in group while increasing their direct and indirect fitness benefits. Future research into the reproductive success of resident and transient females at different ranks in the reproductive queue across multiple years would help us to assign costs and benefits to each strategy under varying population densities. Further understanding patterns of paternity across the population will also be critical to assigning direct and indirect fitness benefits by reproductive strategy for this species.
Data Availability Statement
The original contributions presented in the study are included in the article/Supplementary Material, further inquiries can be directed to the corresponding author/s.
Author Contributions
JV and MR designed the experiment, performed statistical analysis, and wrote the manuscript. JV collected the data. Both authors have approved the final version of this manuscript.
Funding
This research was funded by the Natural Sciences and Engineering Research Council (NSERC) of Canada Discovery Grant to MR (RGPIN 2018-04078) and an NSERC Post-graduate Scholarship to JV, as well as internal funding and resources from Brock University.
Conflict of Interest
The authors declare that the research was conducted in the absence of any commercial or financial relationships that could be construed as a potential conflict of interest.
Publisher’s Note
All claims expressed in this article are solely those of the authors and do not necessarily represent those of their affiliated organizations, or those of the publisher, the editors and the reviewers. Any product that may be evaluated in this article, or claim that may be made by its manufacturer, is not guaranteed or endorsed by the publisher.
Acknowledgments
We sincerely thank Nigel Raine, Liette Vasseur, Glenn Tattersall, and especially Graham Thompson and Peter Nonacs for their comments and input on this research.
Supplementary Material
The Supplementary Material for this article can be found online at: https://www.frontiersin.org/articles/10.3389/fevo.2021.738809/full#supplementary-material
References
Awde, D. N., and Richards, M. H. (2018). Investigating queen influence on worker behaviour using comparisons of queenless and queenright workers. Insectes Soc. 65, 367–79.
Bach, L. A., Thomsen, R., Pertoldi, C., and Loeschcke, V. (2006). Kin competition and the evolution of dispersal in an individual-based model. Ecol. Modell. 192, 658–666. doi: 10.1016/j.ecolmodel.2005.07.026
Brahma, A., Mandal, S., and Gadagkar, R. (2019). To leave or to stay: direct ftness through natural nest foundation in a primitively Eusocial wasp. Insectes Soc. 66, 335–342. doi: 10.1007/s00040-019-00702-2
Field, J., Cronin, A., and Bridge, C. (2006). Future fitness and helping in social queues. Nature 441, 214–217. doi: 10.1038/nature04560
Gerling, D., and Hermann, H. (1978). Biology and mating behaviour of Xylocopa virginica L. (Hymenoptera: anthophoridae). Behav.l Ecol. Sociobiol. 3, 99–111. doi: 10.1007/bf00294984
Grinsted, L., and Field, J. (2018). Predictors of nest growth: diminishing returns for subordinates in the paper wasp Polistes dominula. Behav. Ecol. Sociobiol. 72:88. doi: 10.1007/s00265-018-2502-x
Haney, B. R., and Fewell, J. H. (2018). Ecological drivers and reproductive consequences of non-kin cooperation by ant queens. Oecologia 187, 643–655. doi: 10.1007/s00442-018-4148-9
Hogendoorn, K., and Velthius, H. H. W. (1999). Task allocation and reproductive skew in social mass provisioning carpenter bees in relation to age and size. Insectes Soc. 46, 198–207. doi: 10.1007/s000400050135
Hurd, P. D. J. (1978). An Annotated Catalog of the Carpenter Bees (Genus Xylocopa Latreille) of the Western Hemisphere (Hymenoptera: Anthophoridae). Washington, DC: Smithsonian Institution Press.
Johnstone, R. A. (2008). Kin selection, local competition and reproductive skew. Evolution 62, 2592–2599. doi: 10.1111/j.1558-5646.2008.00480.x
Konovalov, D. A., Manning, C., and Henshaw, M. T. (2004). kingroup: a program for pedigree relationship reconstruction and kin group assignments using genetic markers. Mol. Ecol. Notes 4, 779–782. doi: 10.1111/j.1471-8286.2004.00796.x
Leadbeater, E., Carruthers, J. M., Green, J. P., Rosser, N. S., and Field, J. (2011). Nest inheritance is the missing source of direct fitness in a primitively Eusocial insect. Science 333, 874–876. doi: 10.1126/science.1205140
Leadbeater, E., Carruthers, J. M., Green, J. P., van Heusden, J., and Field, J. (2010). Unrelated helpers in a primitively Eusocial wasp: is helping tailored towards direct fitness? PLoS One 5:e11997. doi: 10.1371/journal.pone.0011997
Moore, J. C., Loggenberg, A., and Greeff, J. M. (2006). Kin competition promotes dispersal in a male pollinating fig wasp. Biol. Lett. 2, 17–19. doi: 10.1098/rsbl.2005.0370
Nascimento, F., and Andrade-Silva, A. (2012). Multifemale nests and social behavior in Euglossa melanotricha (Hymenoptera, Apidae, Euglossini). J. Hymenopt. Res. 26, 1–16. doi: 10.3897/jhr.26.1957
Nonacs, P. (2017). Go High or Go Low? Adaptive evolution of high and low relatedness societies in social Hymenoptera. Front. Ecol. Evol. 5:87. doi: 10.3389/fevo.2017.00087
Ostwald, M. M., Dahan, R. A., Shaffer, Z., and Fewell, J. H. (2021a). Fluid nest memebership drives variable relatdness in groups of a facultatively social bee. Front. Ecol. Evol.
Ostwald, M. M., Fox, T. P., Harrison, J. F., and Fewell, J. H. (2021b). Social consequences of energetically costly nest construction in a facultatively social bee. Proc. Biol. Sci. 288:20210033. doi: 10.1098/rspb.2021.0033
Peso, M., and Richards, M. H. (2010). Not all who wander are lost: nest fidelity in Xylocopa virginica examined by mark recapture. Insectes Soc. 58, 127–133. doi: 10.1007/s00040-010-0125-y
Peso, M., and Richards, M. H. (2011). Knowing who’s who: nestmate recognition in the facultatively social carpenter bee, Xylocopa virginica. Anim. Behav. 79, 563–570. doi: 10.1016/j.anbehav.2009.11.010
Platt, T. G., and Bever, J. D. (2009). Kin competition and the evolution of cooperation. Trends Ecol. Evol. 24, 370–377. doi: 10.1016/j.tree.2009.02.009
Queller, D. C. (1989). Estimating relatedness using genetic markers. Evolution 43, 258–275. doi: 10.2307/2409206
Rau, P. (1933). The Jungle Bees and Wasps of Barro Colorado Island. St. Louis, MO: Von Hoffman Press.
Richards, M. (2011). Colony social organisation and alternative social strategies in the eastern carpenter bee, Xylocopa virginica. J. Insect Behav. 24, 399–411. doi: 10.1007/s10905-011-9265-9
Richards, M. H. (2019). Social trait definitions influence evolutionary inferences: a phylogenetic approach to improving social terminology for bees. Curr. Opin. Insect Sci. 34, 97–104. doi: 10.1016/j.cois.2019.04.006
Richards, M., and Course, C. (2015). Ergonomic skew and reproductive queuing based on social and seasonal variation in foraging activity of eastern carpenter bees (Xylocopa virginica). Can. J. Zool. 93, 615–625. doi: 10.1139/cjz-2014-0330
Ridley, J., and Sutherland, W. J. (2002). Kin competition within groups: the offspring depreciation hypothesis. Proc. R. Soc. B 269, 2559–2564. doi: 10.1098/rspb.2002.2208
Rubenstein, D. R., and Abbot, P. (2017). Comparative Social Evolution. Cambridge: Cambridge University Press.
Richards, M. H., French, D., and Paxton, R. J. (2005). It’s good to be queen: classically eusocial colony structure and low worker fitness in an obligately social sweat bee. Mol. Ecol. 14, 4123–4133. doi: 10.1111/j.1365-294X.2005.02724.x
Schwarz, M. P., Richards, M. H., and Danforth, B. N. (2007). Changing paradigms in insect social evolution: insights from halictine and allodapine bees. Annu. Rev. Entomol. 52, 127–150. doi: 10.1146/annurev.ento.51.110104.150950
Schwarz, M. P., Tierney, S. M., Rehan, S. M., Chenoweth, L. B., and Cooper, S. J. (2011). The evolution of eusociality in allodapine bees: workers began by waiting. Biol. Lett. 7, 277–280. doi: 10.1098/rsbl.2010.0757
Skandalis, D. A., Richards, M. H., Sformo, T. S., and Tattersall, G. J. (2011). Climate limitations on the distribution and phenology of a large carpenter bee, Xylocopa virginica (Hymenoptera: apidae). Can. J. Zool. 89, 785–795. doi: 10.1139/z11-051
Sumner, S., Lucas, E., Barker, J., and Isaac, N. (2007). Radio-tagging technology reveals extreme nest-drifting behavior in a Eusocial insect. Curr. Biol. 17, 140–145. doi: 10.1016/j.cub.2006.11.064
Vickruck, J. L. (2014). Development of sixteen novel microsatellite markers for the eastern carpenter bee, Xylocopa virginica (Hymenoptera: apidae), through paired-end Illumina sequencing. Conserv. Genet. Resour. 7, 427–429.
Vickruck, J. L., and Richards, M. H. (2017a). Nesting habits influence population genetic structure of a bee living in anthropogenic disturbance. Mol. Ecol. 26, 2674–2686. doi: 10.1111/mec.14064
Vickruck, J. L., and Richards, M. H. (2017b). Nestmate discrimination based on familiarity but not relatedness in eastern carpenter bees. Behav. Process. 145, 73–80. doi: 10.1016/j.beproc.2017.10.005
Vickruck, J. L., and Richards, M. H. (2018). Linear dominance hierarchies and conditional reproductive strategies in a facultatively social carpenter bee. Insectes Soc. 65, 619–629.
Wcislo, W. T., and Fewell, J. H. (2017). “Sociality in bees,” in Comparative Social Evolution, eds D. R. Rubenstein and P. Abbott (Cambridge: Cambridge University Press), 50–83. doi: 10.1017/9781107338319.004
Keywords: social evolution, Xylocopa virginica, nesting resources, behavioral plasticity, bee
Citation: Vickruck JL and Richards MH (2021) Competition Drives Group Formation and Reduces Within Nest Relatedness in a Facultatively Social Carpenter Bee. Front. Ecol. Evol. 9:738809. doi: 10.3389/fevo.2021.738809
Received: 09 July 2021; Accepted: 02 November 2021;
Published: 24 November 2021.
Edited by:
Peter Nonacs, University of California, Los Angeles, United StatesReviewed by:
Patrick Abbot, Vanderbilt University, United StatesSara Helms Cahan, University of Vermont, United States
Copyright © 2021 Vickruck and Richards. This is an open-access article distributed under the terms of the Creative Commons Attribution License (CC BY). The use, distribution or reproduction in other forums is permitted, provided the original author(s) and the copyright owner(s) are credited and that the original publication in this journal is cited, in accordance with accepted academic practice. No use, distribution or reproduction is permitted which does not comply with these terms.
*Correspondence: Jess L. Vickruck, jess.vickruck@agr.gc.ca
†Present address: Jess L. Vickruck, Agriculture and Agri-Food Canada, Fredericton Research and Development Centre, Fredericton, NB, Canada