- 1Behavioral and Molecular Ecology Group, Department of Biological Sciences, University of Wisconsin–Milwaukee, Milwaukee, WI, United States
- 2Unit of Evolutionary Biology/Systematic Zoology, Institute of Biochemistry and Biology, University of Potsdam, Potsdam, Germany
- 3GINVER, Facultad de Medicina Veterinaria, Corporación Universitaria Remington, Medellín, Colombia
- 4LAGE do Departamento de Ecologia, Instituto de Biociências, Universidade de São Paulo, São Paulo, Brazil
Many animals form aggregations with individuals of the same species (single-species aggregations, SSA). Less frequently, individuals may also aggregate with individuals of other species (mixed-species aggregations, MSA). Although the benefits and costs of SSA have been intensively studied, the same is not true for MSA. Here, we first review the cases of MSA in harvestmen, an arachnid order in which the records of MSA are more frequent than other arthropod orders. We then propose several benefits and costs of MSA in harvestmen, and contrast them with those of SSA. Second, using field-gathered data we describe gregariousness in seven species of Prionostemma harvestmen from Costa Rica. These species form MSA, but individuals are also found solitarily or in SSA. We tested one possible benefit and one possible cost of gregariousness in Prionostemma harvestmen. Regarding the benefit, we hypothesized that individuals missing legs would be more exposed to predation than eight-legged individuals and thus they should be found preferentially in aggregations, where they would be more protected from predators. Our data, however, do not support this hypothesis. Regarding the cost, we hypothesized that gregariousness increases the chances of parasitism. We found no support for this hypothesis either because both mite prevalence and infestation intensity did not differ between solitary or aggregated individuals. Additionally, the type of aggregation (SSA or MSA) was not associated with the benefit or the cost we explored. This lack of effect may be explained by the fluid membership of the aggregations, as we found high turnover over time in the number of individuals and species composition of the aggregations. In conclusion, we hope our review and empirical data stimulate further studies on MSA, which remains one of the most elusive forms of group living in animals.
Introduction
One of the simplest forms of group living in animals is gregariousness, defined as “the tendency of an animal to aggregate with others such that the animals are in contact with one another, or are nearly so, and that the distribution of the animals in the local environment is extremely patchy” (Vulinec, 1990). Although most theoretical and empirical studies on gregariousness focus on groups formed by individuals of the same species (reviewed in Ward and Webster, 2016), there is increasing evidence that aggregations composed of two or more species are common across the animal kingdom, including birds, mammals, fish, and arthropods (Rasa, 1990; Heymann and Buchanan-Smith, 2000; Zamon, 2003; Boulay et al., 2019; Goodale et al., 2019, 2020). Goodale et al. (2017) recognize two types of mixed-species associations: (a) mixed-species groups, which involve individuals of several species moving together, such as herds of herbivores in the African savanna (e.g., Kiffner et al., 2014), and (b) mixed-species aggregations, which involve individuals gathering around a resource or location, as occurs with many arthropods (reviewed in Boulay et al., 2019). Regardless of the type, the formation of mixed-species associations, especially among predatory species, is remarkable because it requires tolerance and the ability to exchange information with both conspecifics and heterospecifics (Box 1).
BOX 1. The challenges of aggregating with other species.
Aggregating with individuals of other species is a remarkable behavior because species differ in many phenotypic traits. These include physiological requirements, the way they deal with natural enemies, the type of food they consume, the level of aggressiveness they show towards conspecifics and heterospecifics, and how they communicate. Therefore, aggregating with other species requires that individuals overcome at least some of these differences, so that they can recognize, tolerate, and perhaps cooperate with each other (Cocroft, 2001; Boulay et al., 2019). For example, two species of web-building spiders, Hypochilus thorelli (Hypochilidae) and Achaearanea tepidariorum (Theridiidae), form mixed-species aggregations (MSA) in rock outcrops (Hodge and Storfer-Isser, 1997). Before grouping, individuals of one species need to use chemical and vibrational cues to recognize that silk threads were laid by individuals of another species. This task implies that these two non-closely related species share some communication channels for receiving cues and/or sending signals. Moreover, individuals must have the neural and cognitive mechanisms to interpret that the other species is not a potential predator. Finally, if individuals of one species build their own webs using the web of other species to anchor silk threads, some level of behavioral flexibility and tolerance are necessary.
Variation in phenotypic traits across species may either favor or prevent the formation of MSA (Cocroft, 2001; Gerhold et al., 2015; Perón, 2017; Boulay et al., 2019). Phenotypic variation is related, at least in part, to the phylogenetic relationship between the species that compose MSA. For instance, closely related species are more likely to have the same communication channels, which may favor both the recognition and exchange of information. This is the case of mixed-species bird flocks, which are thought to be maintained because species have similar communication channels. The vocal signals produced by one or more species in the presence of a potential predator (e.g., a hawk) are easily recognized and interpreted as an alarm signal by all species in the group (Goodale et al., 2020). However, closely related species may also have similar sizes and diets, so their trophic niches may overlap. In this situation, the close relationship between species may lead to intense interspecific competition, which ultimately may prevent the formation of MSA. In fact, members of mixed-species bird flocks that follow army-ants show clear differences in body size, gape size, and types of prey, suggesting that competition for food is an important factor to the composition of these flocks (Powell, 1985; Sridhar et al., 2009).
Research in evolutionary biology aims to understand why animals aggregate by contrasting the fitness benefits of group living with its costs (e.g., Ward and Zahavi, 1973; Parrish and Edelstein-Keshet, 1999; Greenfield, 2015). An overall balance toward benefits can explain the maintenance of gregariousness in a species. Here, we explore the less-studied question of whether the benefits and costs experienced by individuals in single-species aggregations (SSA) also apply to mixed-species aggregations (MSA). To address the benefits and costs of MSA, we first review the cases of MSA in a particular group of animals in which MSA are relatively frequent, the arachnids of the order Opiliones, commonly known as harvestmen. In our review, we compare potential benefits and costs individuals may have by joining MSA or SSA. Then, we present the first empirical investigation on the benefits and costs of MSA in harvestmen. Finally, we use our empirical findings to propose future directions to study group living among heterospecific individuals.
Mixed-Species Aggregations in Harvestmen: A Review
Arachnids are mostly solitary, but some cases of gregarious, communal, subsocial, and even social species have been described for mites (Saito, 1997), scorpions (Mashberg, 2001), pseudoscorpions (Del-Claro and Tizo-Pedroso, 2009), whip-spiders (Rayor and Taylor, 2006), spiders (Aviles, 1997; Whitehouse and Lubin, 2005; Yip and Rayor, 2014), and harvestmen (Machado and Macías-Ordóñez, 2007). Our review focuses on a particular type of group living, gregariousness. Most cases of gregariousness in arachnids refer to SSA composed of either kin or non-kin individuals (mites: Saito, 1997; spiders: Whitehouse and Lubin, 2005; harvestmen: Machado and Macías-Ordóñez, 2007). However, there are some cases of gregariousness among arachnids in which individuals of two or more species group together. These cases of MSA occur in mites and ticks (e.g., Tsunoda, 2007; Le Goff, 2011), scorpions (Warburg, 2000), spiders (e.g., Hodge and Uetz, 1996; Hodge and Storfer-Isser, 1997), and harvestmen (Machado and Macías-Ordóñez, 2007). Recently, Boulay et al. (2019) reviewed cases of MSA in arthropods, but the main focus of the paper was on insects and only a few examples in arachnids were mentioned. Hence, we aim to expand the topic by providing an in-depth account on the records of MSA in harvestmen.
Harvestman Aggregations
The order Opiliones includes nearly 6,650 species distributed in all continents, except Antarctica (Kury et al., 2020). There are four extant suborders: Cyphophthalmi, Dyspnoi, Eupnoi, and Laniatores. Most of the knowledge about harvestman ecology, behavior, and physiology is concentrated in the latter two suborders (Pinto-da-Rocha et al., 2007), which are also the most diverse, comprising together 90% of all Opiliones (Kury et al., 2020). All cases of gregariousness in harvestmen occur among representatives of Eupnoi and Laniatores (Machado and Macías-Ordóñez, 2007; Figure 1). The Eupnoi that form aggregations include exclusively small-bodied, long-legged species that are common in temperate regions, but also occur in tropical forests. In turn, the Laniatores that form aggregations include large-bodied species, with either short or long legs, which occur exclusively in the neotropics.
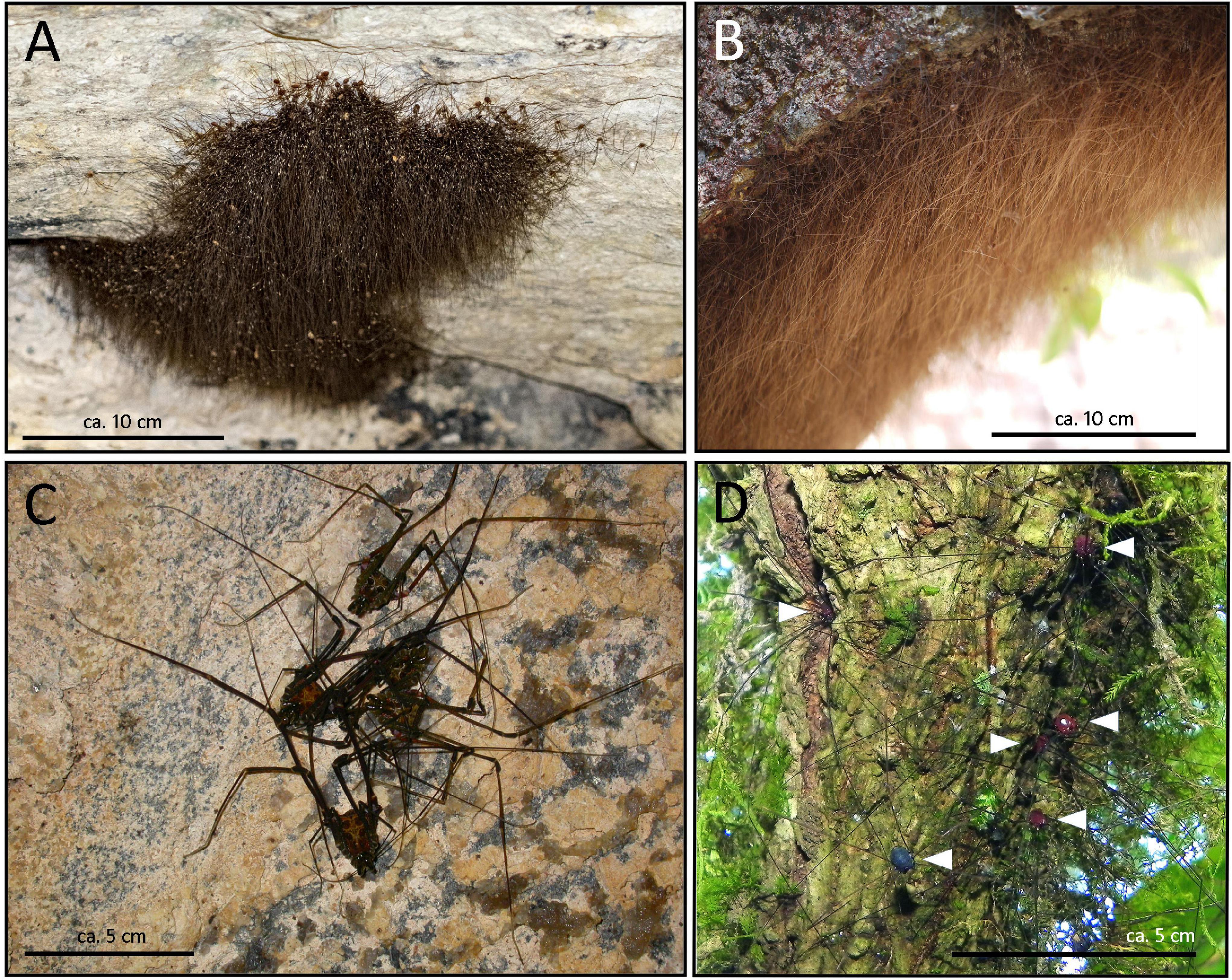
Figure 1. Types of aggregations recorded in harvestmen. (A) Dense single-species aggregation of an unidentified sclerosomatid (Eupnoi) in Texas, United States of America (photo: Aleksomber, Wikimedia Commons). (B) Dense single-species aggregation of an unidentified sclesomatid in Thane, India (photo: Dinesh Valke, Wikimedia Commons). (C) Loose single-species aggregation of Acutisoma longipes (Laniatores) inside a cave in southeastern Brazil (photo: G. Machado). (D) Loose mixed-species aggregations of three species of Prionostemma (Eupnoi) from Costa Rica (photo: I. Escalante). White arrows indicate individuals of different species that can be recognized based on body color.
Aggregations in harvestmen (SSA or MSA) consist of motionless individuals, with their bodies 0–5 cm apart from each other, and their legs usually overlapping or at least in close proximity (Machado et al., 2000). Holmberg et al. (1984) categorized harvestman aggregations into (i) dense or mass aggregations consisting of hundreds or thousands of individuals packed in high density, facing upward and with their legs hanging down or intertwined (Figures 1A,B), and (ii) loose aggregations of dozens or a few hundred individuals not densely packed, with bodies oriented in different directions and legs held outstretched or flexed, but never intertwined (Figures 1C,D). Both types of aggregations are composed mostly of non-kin subadults and adults in variable sex ratios (Machado and Macías-Ordóñez, 2007; Grether et al., 2014a). Because most harvestman species are nocturnal and sensitive to dehydration (Santos, 2007), aggregations are usually found during daytime and in humid, poorly illuminated places, including under rocks and rotting logs, inside caves, and under dense vegetation (e.g., Juberthie, 1972; Holmberg et al., 1984; Willemart and Gnaspini, 2004; Donaldson and Grether, 2007). The aggregations disperse at dusk, when individuals leave the roosting site to forage and then re-group at dawn (e.g., Machado et al., 2000; Wade et al., 2011; Proud et al., 2012). Finally, harvestman aggregations are more frequent during dry and cold periods, especially fall and winter, and/or in xeric environments (Machado and Macías-Ordóñez, 2007). In temperate regions, some species of Eupnoi form aggregations that remain quiescent inside caves throughout the winter and individuals disperse only at the beginning of spring (e.g., Holmberg et al., 1984; Novak et al., 2004).
Mixed-Species Aggregations
We performed a backward and forward literature search based on the papers contained in Table 11.2 of the chapter ‘Social behavior’ (Machado and Macías-Ordóñez, 2007) of the book Harvestmen: The Biology of Opiliones (Pinto-da-Rocha et al., 2007). Using Web of Science and Google Scholar, our backward and forward literature search resulted in 12 cases of MSA in harvestmen (Table 1, including the original data presented in this study). This frequency is higher than in any insect order reported in Boulay et al. (2019). The number of species found in MSA ranges from 2 to 7, and the number of individuals ranges from 2 to 356 (Table 1). In some cases, one or two species are consistently more frequent than the other species in the MSA (e.g., Machado and Vasconcelos, 1998; Elpino-Campos et al., 2001; Pereira et al., 2004), but it is not a general rule. Five cases of MSA involve only species of Eupnoi, six involve only species of Laniatores, and one case involves species of the two suborders (Table 1). These MSA are always loose, and the roosting sites are usually humid places protected from direct sunlight (Table 1).
All studies on MSA in harvestmen are descriptive and none of them investigate benefits or costs of gregariousness. To stimulate further research on this subject, we provide a comprehensive review of the possible benefits and costs of MSA. Some hypotheses on the benefits of MSA in harvestmen have already been proposed (e.g., Machado and Vasconcelos, 1998; Machado and Macías-Ordóñez, 2007), but here we include new ideas. Regarding the costs associated with MSA, we provide for the first time a set of hypotheses that may guide future empirical studies on the subject. Finally, we stress that there is no available information on whether harvestmen cooperate while forming and/or maintaining the aggregations. We know, however, that at least in one species of harvestman individuals mark the roosting sites, potentially by using recruitment pheromones (Donaldson and Grether, 2007; Grether et al., 2014b), and this type of chemical communication may allow cooperative behaviors to evolve (reviewed in Prokopy and Roitberg, 2001). Thus, although our review does not assume cooperation among individuals, we explore how chemical signals may favor the formation of MSA in the section “Gregariousness in Prionostemma harvestmen” below. Additionally, we invite future research to explore cooperation in harvestman SSA or MSA, which is beyond the scope of our project.
Benefits of Mixed-Species Aggregations
In general terms, the benefits of joining MSA can be divided into four main categories, which are similar to that already reported for SSA (Ward and Webster, 2016): physiological benefits, defense benefits, foraging benefits, and reproductive benefits (Table 2 and Figure 2). Although there are other types of benefits, we selected only those that are most frequently cited in the recent literature on MSA in arthropods and vertebrates (Boulay et al., 2019; Goodale et al., 2019, 2020). Moreover, we focused only on the benefits that can be supported by our knowledge on harvestman behavior, ecology, and physiology. For instance, we do not consider collective hunting as a possible benefit because aggregated harvestmen are always stationary and quiescent (Machado and Macías-Ordóñez, 2007). Additionally, after individuals disperse from the roosting site at night, they forage alone (Machado et al., 2000), and no coordinated collective hunting has ever been reported in harvestmen (Acosta and Machado, 2007).
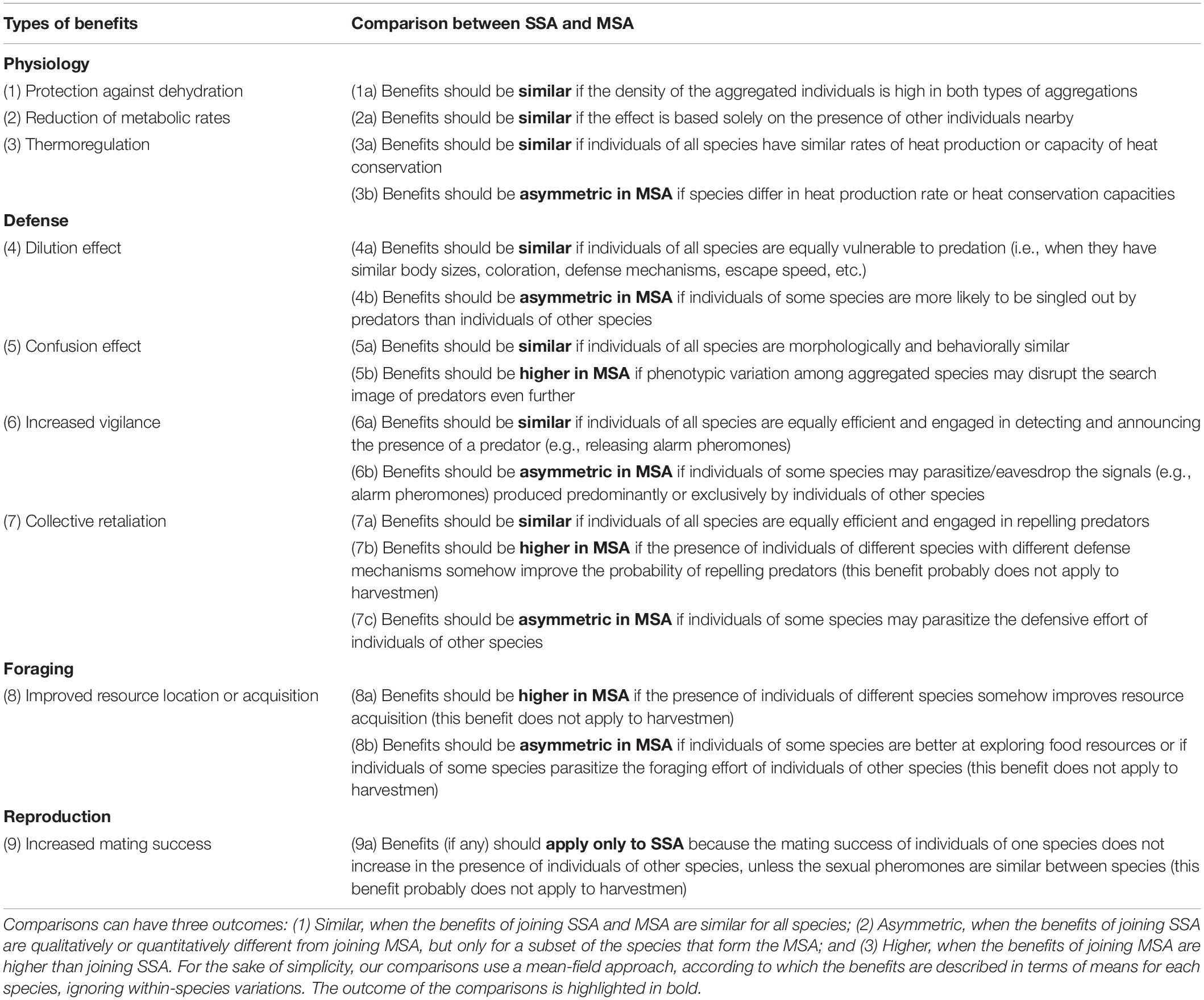
Table 2. Comparison of the benefits associated with single-species aggregations (SSA) and mixed-species aggregations (MSA).
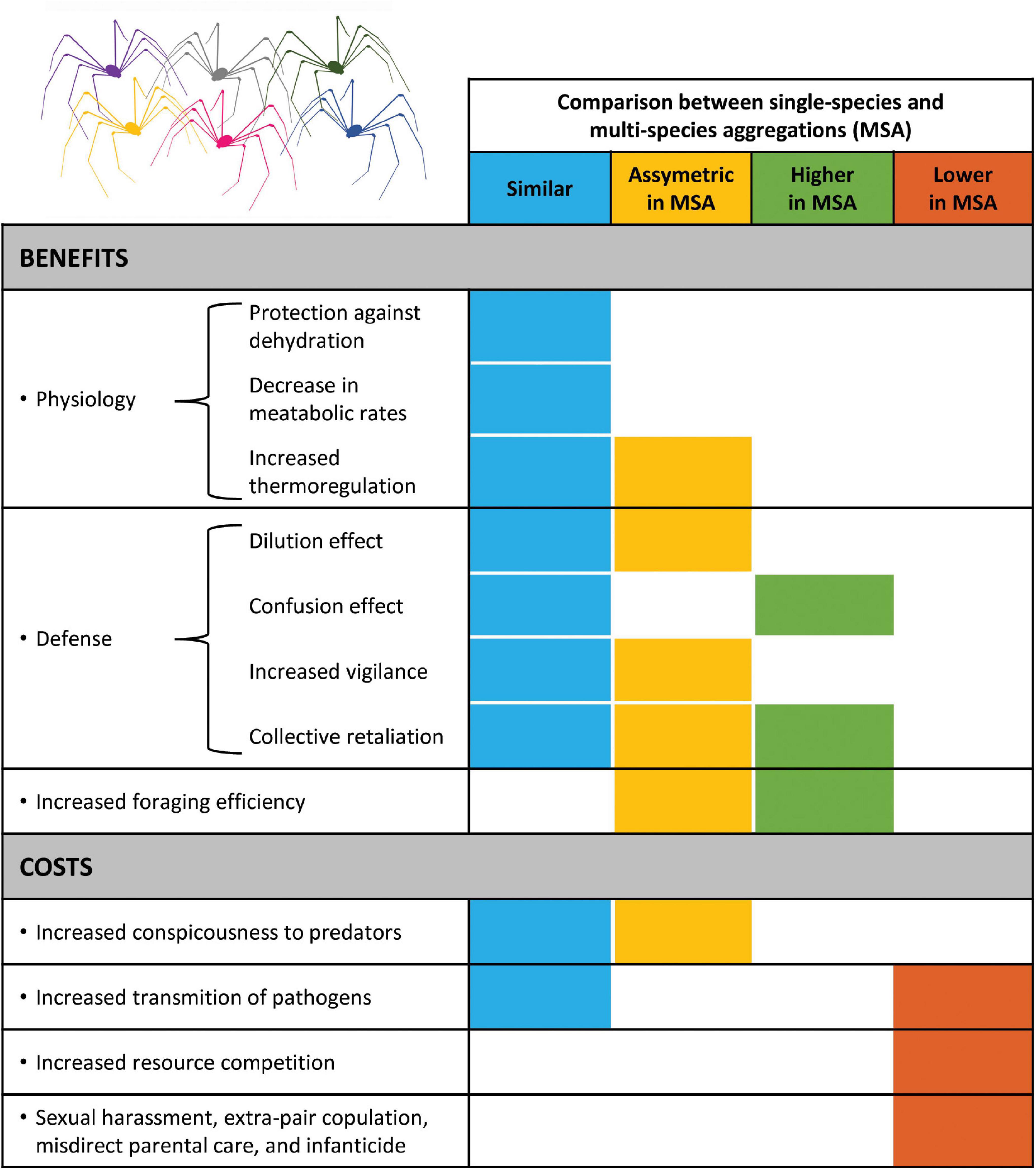
Figure 2. Comparison of benefits and costs of single-species aggregations (SSA) and mixed-species aggregations (MSA). This figure summarizes the reasoning presented in Tables 2, 3, where there are four possible outcomes: (1) Similar, when the benefits–costs of joining SSA and MSA are similar; (2) Asymmetric, when the benefits–costs of joining SSA are qualitatively or quantitatively different from joining MSA, but only for a subset of the species that form the MSA; (3) Higher, when the benefits–costs of joining MSA are higher than joining SSA; and (4) Lower, when the benefits–costs of joining MSA are lower than joining SSA.
Before expanding on the benefits, we stress that MSA may simply be a consequence of individuals of different species being independently attracted to places with specific features (Rasa, 1990). This explanation, known as the “similar habitat hypothesis” (Quinn et al., 2003), requires high tolerance to conspecifics and heterospecifics (Boulay et al., 2019). In this sense, harvestmen are unusual arachnids because cannibalism among subadults and adults has never been reported under field conditions. Most records of cannibalism include adults eating eggs or early instars (Acosta and Machado, 2007). Moreover, despite anecdotal records of heterospecific predation in harvestmen (Cokendolpher and Mitov, 2007), this behavior is rare and probably occurs when there is a great size difference between individuals of each species. Thus, contrary to spiders, whip-spiders, and scorpions, conspecifics and heterospecifics pose low risks of predation, which may have favored tolerance and the evolution of gregariousness in harvestmen (Machado, 2002).
Is there any evidence that the similar habitat hypothesis applies to MSA in harvestmen? As we mentioned earlier, most harvestmen have low tolerance to dehydration, and thus aggregations are usually found in humid places with low light incidence. Even in the cavernicolous habitat, which is humid and dark, SSA of Acutisoma longipes (Gonyleptidae) usually occur close to the river that crosses the cave and away from cave openings (Machado et al., 2000), suggesting that aggregations are not randomly distributed in the habitat. This pattern was not observed in two gonyleptid species (Serracutisoma proximum and S. spelaeum) closely related to A. longipes that also live inside caves and form MSA. Although these two species are congeneric, aggregations of S. proximum occur more frequently on walls close to cave openings (where individuals are exposed to light incidence, higher temperature, and humidity fluctuation) than aggregations of S. spelaeum (Chelini et al., 2012). This finding does not support the notion that MSA in harvestmen are a consequence of similar and specific habitat requirements by different species. However, MSA frequently involve species belonging to the same genus (Table 1), which are likely to have similar physiological and ecological requirements (Chown and Nicolson, 2004). This high frequency of congeneric species in MSA cannot be explained by the composition of the local harvestman communities, which usually include a great diversity of genera (Curtis and Machado, 2007). Thus, until a formal test of the similar habitat hypothesis is available, it may be premature to discard it as a possible (but certainly not the only) explanation for the formation of MSA in harvestmen.
Mixed-species aggregations can also be a consequence of limited resource availability, so different species are forced to share the same places (Rasa, 1990). For instance, individuals of several fish species are associated with sea anemones, which provide protection to small-bodied species or small individuals within species. Considering that colonies of sea anemones are highly clumped, the spatial distribution of different fish species follows the availability of the anemones, resulting in the formation of MSA (Brooker et al., 2019). This explanation, known as the “resource limitation hypothesis,” assumes that individuals of different species share similar requirements (e.g., protection) and that the risk of antagonistic interactions (i.e., cannibalism and predation) is low. The best candidate for limiting resources favoring the formation of MSA in harvestmen is the roosting site. Although biologically plausible, there is no evidence supporting that roosting sites are a limiting resource for harvestmen. Studies on two species of Prionostemma (Sclerosomatidae) that form SSA in Central America show no preference for different palm trees, which are the main roosting sites (Donaldson and Grether, 2007; Grether and Donaldson, 2007; Teng et al., 2012; Grether et al., 2014a,b). This finding contradicts the existence of suitable roosting sites with low availability in the field. Thus, we argue that the resource limitation hypothesis is unlikely to be an explanation for the existence of harvestman aggregations (SSA or MSA).
Physiological Benefits
Given the weak support for two simple explanations (i.e., similar habitat and resource limitation hypotheses) that require no individual benefit for the existence of MSA, we argue that this unusual form of group living evolved and is maintained in harvestmen because it provides benefits. Here we discuss three physiological benefits: protection against stressful abiotic conditions, reduction of metabolic rates, and thermoregulation (Table 2).
Gregariousness may act as a behavioral mechanism to reduce water loss in many arthropods (isopods: e.g., Friedlander, 1965; millipedes: e.g., Dangerfield, 1993; insects: e.g., Danks, 2002). In harvestmen, the close body contact and the intertwining of legs may reduce airflow and thus individual water loss (Machado and Macías-Ordóñez, 2007). This may be the case for the dense SSA observed in some Eupnoi from xeric regions composed of thousands of quiescent individuals (e.g., Wagner, 1954; Figures 1A,B). However, all cases of MSA in harvestmen are loose (Table 1), which is unlikely to protect the individuals from dehydration because they are not so densely packed together to prevent water loss. Thus, although loose aggregations may also promote changes in microclimatic conditions, we anticipate that the potential benefits they provide in terms of protection against dehydration should be lower than in dense aggregations.
Aggregations have also been found to reduce the resting metabolic rates of some insects (reviewed in Chown and Nicolson, 2004). Such reduction may be beneficial because it decreases energy expenditure and spiracular water loss in tracheate arthropods, including arachnids (Hadley, 1994). There is one single study showing that Vonones ornatus (Cosmetidae) harvestmen in small and loose aggregations have reduced metabolic rates (Anderson, 1993). The exact mechanism linking gregariousness and metabolic rates in harvestmen has not been explored yet and certainly deserves attention. Another important question is whether the reduction in metabolic rates reported for loose SSA of V. ornata also occurs in loose MSA of other harvestman species. Therefore, this potential benefit cannot be discarded and deserves further consideration.
Finally, gregariousness may favor thermoregulation by improving heat production and/or conservation, protecting the individuals from cold conditions. This benefit is particularly important among endothermic vertebrates living in high latitudes, where the temperature may reach extremely low values (Gilbert et al., 2010). Among insects that live in less harsh habitats, gregariousness may also play an important role in their thermal ecology (Danks, 2002; Chown and Nicolson, 2004). For instance, caterpillars maintain higher and more stable body temperatures when aggregated (e.g., Casey et al., 1988; Joos et al., 1988; Casey, 1993; Fitzgerald, 1993), which leads to higher growth rates (e.g., Scriber and Lederhouse, 1983; Knapp and Casey, 1986). Similarly, temperatures are higher and buffered in aggregations of the lady beetle Coleomegilla maculata (Benton and Crump, 1979). Although there is no information on the temperature inside harvestman aggregations, we argue that dense aggregations may conserve heat and buffer temperature variations, at least in its core. Heat control and temperature buffering would be particularly beneficial for individuals in overwintering aggregations from cold regions (e.g., Holmberg et al., 1984; Novak et al., 2004) and in diurnal aggregations from hot xeric regions (e.g., Wagner, 1954). Nonetheless, since all cases of MSA in harvestmen are loose (Table 1), we consider that a thermoregulation benefit is unlikely.
Defense Benefits
Aggregations in harvestmen may provide several defense benefits against predators. At least among Laniatores, scent gland secretions released by the individuals are a powerful chemical deterrent that repel different types of predators, including invertebrates and small vertebrates (e.g., Eisner et al., 2004; Machado et al., 2005). Thus, a first defense benefit of gregariousness is a higher amount of secretion released upon disturbance — a collective retaliation that may improve the efficiency of the chemical defenses (Machado and Macías-Ordóñez, 2007; Table 2). In the case of MSA, behavioral observations show that some species rarely release scent gland secretions (Elpino-Campos et al., 2001; Pereira et al., 2004). In one report, the most common species in the aggregation does not even produce scent gland secretions (Machado and Vasconcelos, 1998). This finding can be explained by the “protector species hypothesis,” according to which individuals of a poorly defended species benefit by associating with individuals of a well-defended species that aggressively repels potential predators of both species (Sullivan, 1984; Quinn et al., 2003; Goodale et al., 2014). The protector species hypothesis assumes that the benefits of MSA in terms of collective retaliation are necessarily asymmetric because not all species contribute to repel the predators (Table 2).
In known cases of MSA in harvestmen, the poorly defended species (e.g., Mischonyx cuspidatus and Encheiridium montanum) comprise most part of the individuals in the aggregation, whereas the well-defended species (e.g., Discocyrtanus oliverioi and Holoversia nigra) account for only a small number of individuals (Machado and Vasconcelos, 1998; Elpino-Campos et al., 2001; Pereira et al., 2004; Table 1). It is possible that the well-defended species are not even gregarious. In this case, well-defended species may serve as attractors (i.e., initiators) to poorly defended species (i.e., followers) that would aggregate around the former. This hypothesis does not imply that initiators are deliberately attracting followers using recruitment pheromones, although it may be the case. If initiators have some benefit when followers aggregate around them, it would be advantageous to release recruitment pheromones. An obvious advantage for the initiators of attracting followers is a decreased probability of being singled out by predators (Sridhar et al., 2009). This “dilution effect” (Turner and Pitcher, 1986; Table 2) is another defense benefit attributed to harvestman aggregations (Machado and Macías-Ordóñez, 2007). In MSA, both initiators and followers may enjoy this defense benefit; if the benefit is similar to all species in the aggregation, is an open question that deserves future studies.
Harvestmen also obtain the benefit of having an early alarm signal in aggregations. The scent gland secretions released by aggregated individuals and their movement to escape from an attack work as an alarm — one chemically and the other mechanically mediated (Machado et al., 2002). Experimental field evidence shows that aggregations of Serracutisoma gnaspinii (Gonyleptidae) with a greater number of individuals disperse faster to the chemical alarm promoted by the emission of scent gland secretions (Machado et al., 2002). Large harvestman aggregations have more sensory legs full of chemoreceptors to perceive the scent gland secretions released by other individuals. Due to the alarm communication, aggregations may increase both the escape capability of the individuals and their probability to survive a predatory attack (Machado and Macías-Ordóñez, 2007). In MSA, there is observational evidence showing that scent gland secretions released by one species may work as a chemical alarm to individuals of other species that do not release scent gland secretions (Machado and Vasconcelos, 1998). This suggests that the benefits promoted by the alarm communication are asymmetric: while individuals of species that do not release scent gland secretions benefit from the chemical alarm released by other species, the opposite does not happen (Table 2). Regarding the mechanically mediated alarm, however, all species in MSA may be favored because it only requires that individuals bump each other while they are dispersing from the aggregation (i.e., “Trafalgar effect”; Treherne and Foster, 1981).
Finally, it is possible that the fast dispersion of aggregated individuals causes a confusion effect in the search image of visually oriented predators (Goodale et al., 2019; Table 2). Most examples of confusion effect have been reported for vertebrate prey, but experiments with aggregations of the water flea Daphnia magna showed that stickleback attack rates decline when aggregated individuals are in larger aggregations, are closer together, have similar body size, and are moving parallel to each other (Ohguchi, 1981). After disturbance, individuals in harvestman aggregations quickly disperse, running away or falling from the roosting site (Newman, 1917; Holmberg et al., 1984). Certain species of Eupnoi also exhibit bobbing behavior (Holmberg et al., 1984), which is a fast up and down body movement (Gnaspini and Hara, 2007). The collective movement of dozens to thousands of individuals bobbing probably confuses the identification and precise location of a potential prey’s body by the predator (Escalante et al., 2019). This confusion effect may be more accentuated in the MSA of sclerosomatids in Central America because individuals of different species show great variation in body color (Figures 1D, 3), which may disrupt even more the search image of potential predators (Table 2).
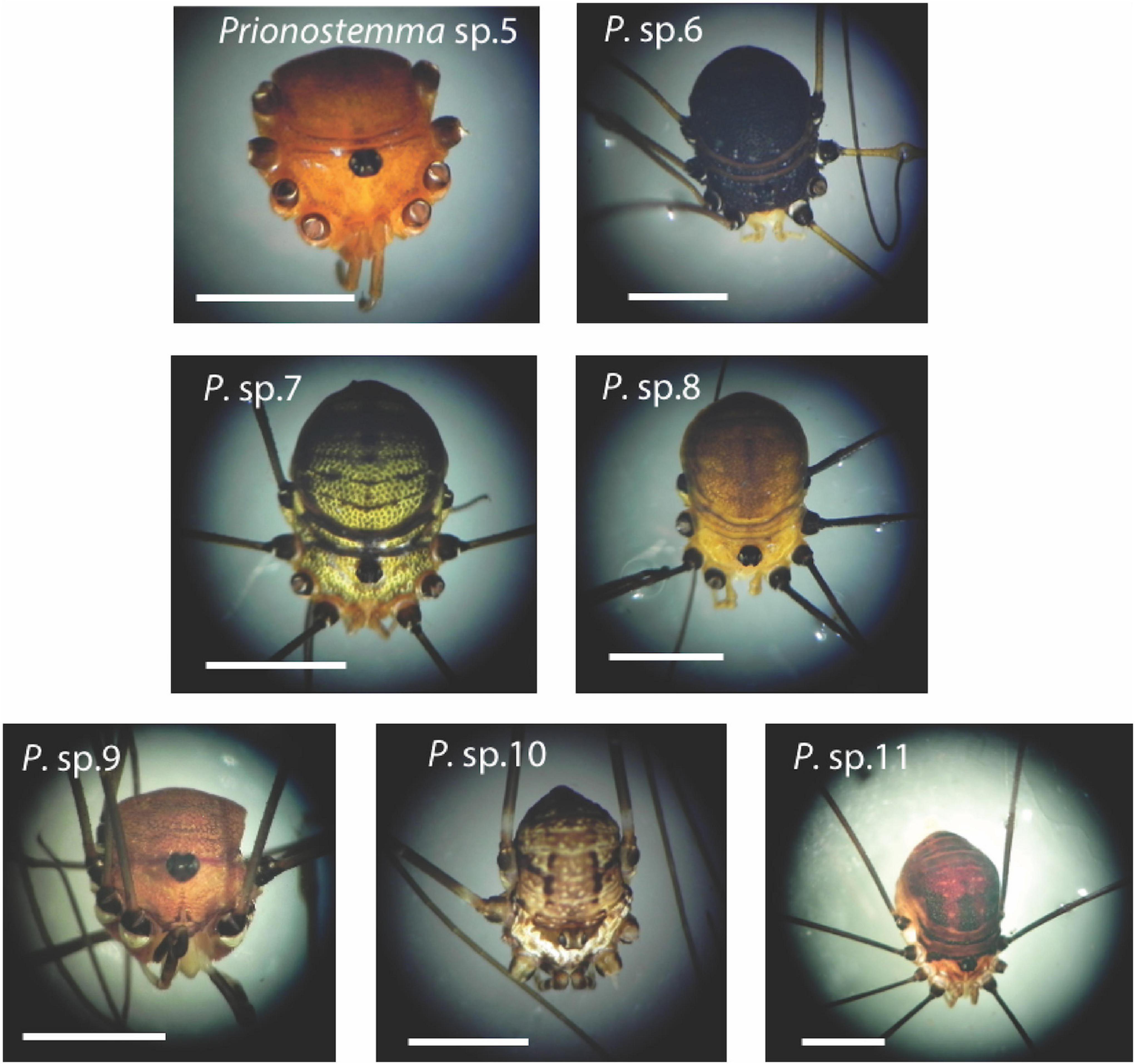
Figure 3. The seven species of Prionostemma harvestmen studied in a tropical forest of Costa Rica. These species are currently undescribed, but can be easily recognized based on external features, such as body size and color, as well as relative leg length and color. To reinforce our criteria of species recognition, we also found differences between species in the male genital morphology. Scale bars = 3 mm.
Reproductive Benefits
Aggregations during or immediately before the beginning of the breeding season may ensure that individuals of both sexes will be in close proximity. Thus, aggregations may provide mating benefits by increasing the opportunities to gain access to mates and by reducing the mating search costs (Ward and Webster, 2016). However, there is no evidence that harvestman aggregations are related to reproduction (Machado and Macías-Ordóñez, 2007). In many cases, aggregations occur during the winter, when no breeding activity occurs (e.g., Holmberg et al., 1984; Machado et al., 2000; Novak et al., 2004; Willemart and Gnaspini, 2004; Chelini et al., 2012). When aggregations occur during the breeding season, sexual interactions have not been observed close to them (e.g., Edgar, 1971; Cockerill, 1988; Grether et al., 2014a). In the case of MSA, the possibility of mating benefits is non-existent because individuals derive no reproductive advantage from aggregating with heterospecifics.
Costs of Mixed-Species Aggregations
In general terms, the costs of joining MSA can be divided into four main categories, which are similar to those already reported for SSA (Ward and Webster, 2016): increased conspicuousness, increased transmission of pathogens and parasites, increased resource competition, and costs related to reproduction, such as increased risk of sexual harassment and extra-pair copulation, misdirect parental care, and infanticide (Table 3 and Figure 2). This list includes only the costs that are most frequently explored in the recent literature on MSA in arthropods and vertebrates (Boulay et al., 2019; Goodale et al., 2019, 2020). Because harvestman aggregations have no connection with reproduction (Machado and Macías-Ordóñez, 2007), including parental care, we do not consider that MSA can impose reproductive costs. However, for species in which aggregations are somehow connected to reproduction, as occurs with many bird species (examples in Goodale et al., 2017; Boulay et al., 2019), we expect the costs of group living would be lower in MSA than in SSA (Figure 2).
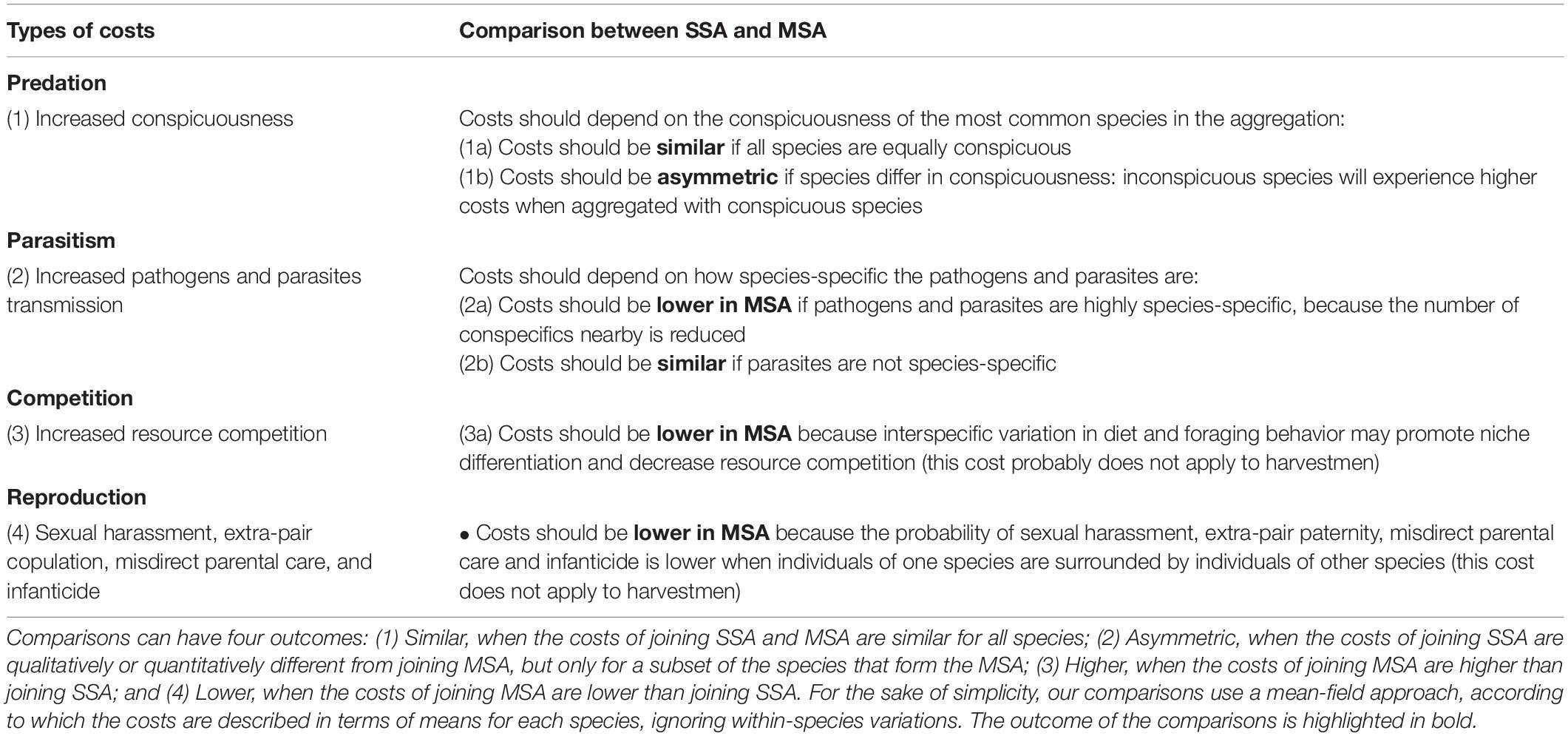
Table 3. Comparison of the costs associated with single-species aggregations (SSA) and mixed-species aggregations (MSA).
Increased Conspicuousness
Gregariousness may increase the chances of detection by predators because aggregations may be visually or chemically more conspicuous than isolated individuals (Vulinec, 1990; Table 3), especially if individuals are colorful, as it is the case of some Prionostemma species (Table 1 and Figures 1D, 3). To date, we lack information about whether harvestman aggregations (SSA or MSA) increase conspicuousness and consequently predatory attacks. However, if aggregations increase conspicuousness and the risk of predatory attacks, we predict that individuals of cryptic species should avoid joining MSA with conspicuous species. In this situation, individuals of cryptic species would derive more benefits by aggregating exclusively with conspecifics because they would make the aggregation less conspicuous to visually oriented predators. On the contrary, individuals of conspicuous species should infiltrate aggregations of cryptic species where they would be more protected from visually oriented predators than in aggregations composed only of conspecifics. By doing so, individuals of conspicuous species would ‘break’ the aposematic value of the aggregations. This conflict between individuals of different species is an interesting, yet unexplored, idea that may have important implications for the patterns of species co-occurrence in MSA.
Transmission of Pathogens and Parasites
One of the most obvious costs of gregariousness is the increased transmission of internal and external pathogens and parasites (Côté and Poulin, 1995; Kappeler et al., 2015). Harvestmen are exposed to a great sort of pathogens and parasites (reviewed in Cokendolpher and Mitov, 2007). The group of endoparasites most frequently reported for harvestmen are gregarines, which are apicomplexans that infect the digestive tract of their hosts. A harvestman infects itself when the small oocysts, present in the feces of other individuals, attach to the tip of its legs and then are ingested during leg grooming (Cokendolpher and Mitov, 2007). Considering that aggregated individuals share the same roosting sites and probably defecate in or close to these sites, it is likely that harvestman aggregations increase the chances of infection by gregarines. Unfortunately, there is no information on host specificity for the gregarines that infect harvestmen. If the host-parasite relationship is not species-specific (e.g., Cokendolpher, 1993), the risk of contamination may be equally high in SSA and MSA. On the contrary, if there is some degree of specialization in the host-parasite relationship, the risk of infection should be lower in MSA than in SSA (Table 3).
Harvestmen in aggregations are also exposed to external parasites, including many species of Leptus mites (Cokendolpher and Mitov, 2007). These mites deposit a cone of cementing material at the attachment site that, along with their mouthparts, form a tight anchorage on the tegument of the host (Åbro, 1988). Thus, while attached, it is very unlikely that one harvestman carrying mites will infect others. However, we observed mites moving around on the body of Prionostemma harvestmen that form MSA (see section “Costs of gregariousness” below). This indicates that mites do not attach their mouthparts immediately to their hosts, and thus may move from one host to another. Currently, there is no information on how long it takes for a Leptus mite to climb the host and attach its mouthparts. We know, however, that many Leptus mites prefer certain body parts on their harvestman hosts (e.g., McAloon and Durden, 2000; Townsend et al., 2008), which implies that they move around some time until they find a proper attachment site. The main implication of this movement is that horizontal transmission of mites among aggregated harvestmen is possible. It seems that Leptus mites do not show great host specialization because the same species may parasitize numerous harvestman species (Cokendolpher and Mitov, 2007). Therefore, the costs of infection are likely to be similar in SSA and MSA.
Resource Competition
When dozens, hundreds or even thousands of individuals aggregate in the same site, they may compete for resources, mainly food. However, when harvestman aggregations disperse at night, individuals visit different places and can move long distances (e.g., Grether and Donaldson, 2007). When individuals of Acutisoma longipes and Serracutisoma spelaeum leave the cave at night, each one follows a specific trail to forage on substrates as different as the external cave walls and the canopy of the forest surrounding the cave (Gnaspini, 1996; Machado et al., 2000). Since the foraging area shows little overlap between individuals, it is unlikely that they compete for food. We do not know if this also happens in large aggregations of Eupnoi that may have between 70,000 (Wagner, 1954) and 300,000 individuals (Mukherjee et al., 2010) in habitats with low productivity, such as deserts and cold forests. It is important to note, however, that harvestmen are highly generalist feeders and forage on many different items, including live and dead animals, as well as vegetal and fungi matter (Acosta and Machado, 2007). Thus, competition for food is not expected to have an important role in harvestmen ecology (Curtis and Machado, 2007). Supporting this claim, a long-term study on harvestman communities in deciduous woods from England found no evidence for resource competition among species (Adams, 1984). If competition is important, joining MSA may attenuate it, considering that differences in size, morphology, and microhabitat use between species somehow reflect differences in their food niche. This hypothesis has been originally proposed for MSA of birds that forage together (Powell, 1985; Sridhar et al., 2009), and to our knowledge, there is no demonstration that it also applies to invertebrates. Harvestmen offer an opportunity to test this hypothesis because of the numerous cases of SSA and MSA, which allow exploring how the intensity of competition for food varies according to the type of aggregation while controlling for aggregation size.
Study Case: Mixed-Species Aggregations in Prionostemma Harvestmen
Here, we provide empirical data on gregariousness in harvestmen of the genus Prionostemma, whose species form both SSA (e.g., Coddington et al., 1990; Grether and Donaldson, 2007; Teng et al., 2012) and MSA (e.g., Grether et al., 2014a). Our study system includes seven currently undescribed species that occur in primary and secondary forests in southwestern Costa Rica. Since a previous study refers to other four undescribed species from northeastern Costa Rica as Prionostemma sp.1 to sp.4 (Proud et al., 2012), we will refer to the species studied here as Prionostemma sp.5 to sp.11 (Figure 3). We are confident that the seven species studied are different taxonomic entities because they show clear differences in several traits (body size and color, relative leg length, and specially the morphology of male genitalia) commonly employed in the taxonomy of Neotropical sclerosomatids belonging to the subfamily Gagrellinae (e.g., Tourinho-Davis and Kury, 2003; Tourinho et al., 2015).
Natural History Background
The Prionostemma from Costa Rica are found during the day either solitarily or forming aggregations, mostly beneath moss, on tree buttresses, inside tree crevices, and on palm and ginger leaves. At dusk, individuals disperse and are found foraging and searching for mates on plants and on the forest floor (Domínguez et al., 2016; Escalante and Elias, 2021). Individuals of other Prionostemma species from Nicaragua can move long distances when they leave their diurnal roosts. For instance, Grether and Donaldson (2007) recaptured individuals 130 m from the roosting sites where they were originally marked. Although the roosting sites in Nicaragua were predictable, the membership of aggregations was fluid because individuals were recaptured in different roosting sites over time (Donaldson and Grether, 2007). Similar results were obtained for one of the Costa Rican species studied here, Prionostemma sp.5, which also showed low levels of roosting site fidelity (Escalante and Elias, 2021).
As occurs with many species of Eupnoi (e.g., Guffey, 1999; Houghton et al., 2011; Powell et al., 2021a,b), individuals of Prionostemma are frequently found missing legs in the field (Escalante et al., 2013, 2020, 2021; Domínguez et al., 2016). This is an indication of autotomy (i.e., the voluntary release of legs to escape predatory attempts) and to a much lesser extent the effect of failed molt (Gnaspini and Hara, 2007; Emberts et al., 2019). Autotomy affects the locomotor performance and the energetics of locomotion of sclerosomatid harvestmen (Escalante et al., 2013, 2020, 2021; Domínguez et al., 2016). This reduced locomotor performance may affect the chances of surviving future encounters with predators. Thus, the perception of predation risk is likely to change after autotomy (Emberts et al., 2019), and individuals may change their anti-predator behaviors (Fleming et al., 2007). For instance, in a laboratory experiment with the wolf spider Schizocosa avida, autotomized individuals showed increased avoidance of olfactory cues of predators (scorpions) when compared with intact individuals (Punzo, 1997). If aggregations of Prionostemma harvestmen indeed increase the protection of the individuals against predators, a possible behavioral response of autotomized individuals would be a tendency to roost more frequently in aggregations instead of solitarily.
Another conspicuous feature of the biology of many harvestman species is the high prevalence of erythraeid mites (Mesostigmata), which are ectoparasites of many arthropods (Cokendolpher and Mitov, 2007). In Prionostemma from Nicaragua, the prevalence of Leptus mites changed among roosting sites and species (Grether et al., 2014a), but no comparison between solitary and aggregated individuals was conducted. Although we know the prevalence of ectoparasite mites in several harvestman species (e.g., McAloon and Durden, 2000; Mitov, 2000; Townsend et al., 2006, 2008; Grether et al., 2014a), there is no information on the negative fitness-related effects these mites may have on their hosts. In other arthropod taxa, however, the infection by erythraeid mites has clear negative effects on their hosts. In Drosophila, for instance, mites extract hemolymph from the host, causing marked cuticular damage during feeding. The long-term nutrient extraction and mite-derived damages have negative effects on the reproductive tissues of males and females (Polak, 1996; Benoit et al., 2020). In harvestmen, Leptus mites extract hemolymph from their hosts, and the attachment of mites promotes intense immune response in their hosts (Åbro, 1988). Evidence from other arthropods indicates that activation of the immune system is costly, imposing several fitness trade-offs that may reduce reproductive performance and/or survival (Schmid-Hempel, 2005). Thus, it is reasonable to suppose that mite infestation is costly to harvestmen as well.
Objectives
Our first goal in this empirical part of the study is to describe gregariousness in seven Prionostemma species. More specifically, we report: (a) the frequency of individuals belonging to each species that roost solitarily or in groups (SSA and MSA); (b) the natural variation in the number of individuals in SSA and MSA; (c) the species composition in MSA; (d) the patterns of species co-occurrence in MSA; and (e) the temporal variation in aggregation size and species composition in MSA. Our second goal is to examine one potential benefit and one potential cost of gregariousness in the seven Prionostemma species studied here, comparing the results between SSA and MSA. We tested the following hypotheses:
• If aggregations provide defense benefits, individuals roosting in aggregations would be in a safer condition than individuals roosting solitarily. Assuming that leg autotomy increases vulnerability to future predatory attacks and changes the perception of predation risk (Fleming et al., 2007; Emberts et al., 2019), we predict that autotomized individuals would be more prone to roost in groups because they would be more protected. Therefore, the probability of finding autotomized individuals roosting in aggregations would be higher than roosting solitarily. We also tested whether this probability differs between the types of aggregation. SSA and MSA confer several similar defense benefits, but at least one of these benefits is expected to be higher in MSA, the confusion effect (Table 2). Considering that the seven Prionostemma species show marked differences in color, and these differences may disrupt the search image of visually oriented predators, autotomized individuals could derive more defense benefits by joining MSA. Thus, the probability of finding autotomized individuals roosting in MSA would be higher than in SSA. Finally, assuming that most defense benefits of gregariousness are expected to be positively related to aggregation size, we predict that autotomized individuals would seek larger aggregations, where they would be safer.
• Given the close proximity of individuals, aggregations could increase the chance of horizontal transmission of ectoparasites. Therefore, we predict that individuals found in aggregations would be more likely parasitized by mites than solitary individuals. If the erythraeid mites that parasitize the Prionostemma species studied here have no host specificity, we expect similar costs of roosting in SSA and MSA. However, if the erythraeid mites have some level of host specificity, we expect the costs of roosting in SSA would be higher than in MSA.
Methods
Study Site
The research was conducted in Las Cruces Biological Station, Puntarenas, Costa Rica (8°47′ N; 82°57′W; 1,200 m. a.s.l.). The temperature in the study site ranges from 17 to 24°C, and the annual precipitation is about 3,600 mm, with a well-marked seasonality: a wet season between July and October, and a dry season between February and June. Data were collected in the understory of primary and secondary forests along the Jungle, Java, and Water trails.
Field Methods
We searched for Prionostemma harvestmen on three sampling occasions: January 2013, July 2013, and February 2014. Although the individuals were not marked, it is unlikely that they were resampled in different sampling occasions because Eupnoi harvestmen live only a few months as adults (Gnaspini, 2007). Moreover, we searched the trails only once and each individual and aggregation was sampled only once in the three sampling occasions. In all sampling occasions we searched for harvestmen during the day (08:00 to 14:00 h), when individuals are roosting motionless. On both sides of the trails, we looked for Prionostemma individuals roosting on the low vegetation (from 0 up to 2–3 m). For each individual, we recorded the species, which can be easily recognized based on the dorsal coloration (Figure 3), and the roosting status: (a) solitary, (b) in SSA, or (c) in MSA. For the purpose of this study, we defined aggregation as a group of two or more individuals, regardless of the species, resting in close proximity (<5 cm), with or without leg contact (Figure 1D). When we found isolated or aggregated individuals, we grabbed them by hand and quickly placed them inside plastic containers (30 cm diameter × 12 cm height). This allowed us to process one individual at a time while the others were caged in low density (2–4 individuals per container). After collecting the individuals, we carefully inspected each of them to record the number of missing legs, as well as the presence and number of mites on the body (including legs, dorsum, and venter).
We used the data collected in the three sampling occasions to describe the frequency of individuals belonging to each species that roost solitarily or in aggregations (SSA or MSA), the natural variation in the number of individuals in SSA and MSA, the species composition in MSA, and the patterns of species co-occurrence in MSA. Moreover, we used that data to test our hypotheses on benefits and costs of group living in harvestmen (see sections “Data analyses: benefits of gregariousness” and “Data analyses: cost of gregariousness” below).
In February-March 2014, we delimited a plot 1,100 m long and 6 m wide (3 m on each side of the above-mentioned trails). Inside this plot, we found 46 plants regularly used as roosting sites by Prionostemma individuals. These roosting sites were individually marked with numbered flags and inspected once every day (between 08:00 and 14:00 h) over 10.8 ± 1.3 days (range: 9–14 days). During each inspection, we recorded the number of individuals of each species in the roosting sites. We only used these data to describe the temporal variation in aggregation size and species composition.
Data Analyses: Benefits of Gregariousness
To test our predictions on the defense benefits of gregariousness, we ran generalized linear models (GLMs) in which leg loss (yes or no) was the predictor variable with multinomial distribution of errors and logit link function. The response variable was the roosting status with three levels: solitary, SSA, and MSA. This analysis was performed for each species separately, so that we could evaluate if the potential benefits of gregariousness are similar between them. Given that the number of individuals of Prionostemma sp.9, sp.10, and sp.11 was very low (15, 9, and 5, respectively), we could not perform the analysis for these species. Moreover, for Prionostemma sp.8 only MSA were found in the field, so that the response variable had only two levels (solitary and MSA). In this case, the GLM had a quasibinomial distribution of errors (to deal with data overdispersion) and the link function was logit. Based on the model for each species, we estimated the marginal means to calculate the necessary contrasts to test our predictions. We also ran models in which the predictor variable was the number of missing legs (ranging from 0 to 4). Given that the explanatory power of this model was similar to that of the leg loss model (Supplementary Table 1), we present here only the results obtained with the leg loss model.
Finally, we evaluated whether the number of individuals in the aggregations (i.e., aggregation size) and the type of aggregation (SSA or MSA) influenced the proportion of autotomized individuals in the aggregations. For this, we ran a GLM in which the response variable (i.e., the proportion of autotomized individuals in the aggregation) had a quasibinomial distribution of errors (to deal with data overdispersion) with logit link function. The full model included an interaction between aggregation size and type of aggregation.
Data Analyses: Cost of Gregariousness
To test our predictions on the costs of gregariousness, we ran two GLMs. In the first model, the response variable was the presence of mites (yes or no), with quasibinomial distribution of errors (to deal with data overdispersion) and logit link function. In the second model, the response variable was the number of mites per individual, with negative binomial distribution of errors (to deal with overdispersion of the data) and log link function. In both models, the predictor variable was the roosting status with three levels: solitary, SSA, and MSA. These analyses were performed for each species separately, so that we could evaluate if the potential costs of gregariousness are similar between them. Again, we excluded Prionostemma sp.9, sp.10, and sp.11 from the analyses because the number of individuals was very low. Based on the models for Prionostemma sp.5, sp.6, sp.7, and sp.8, we estimated the marginal means to calculate the necessary contrasts to test our predictions.
All statistical analyses were performed in the software R version 4.1.0 (R Core Team, 2021). We used the package nnet (Ripley et al., 2016) for the multinomial models, the package Stats (R Core Team, 2021) for the binomial models, the package MASS for the GLM with negative binomial distribution of errors (Venables and Ripley, 2002), and the package emmeans (Lenth, 2019) to calculate the contrasts. The complete datasets and the scripts used in the analyses are available on Dryad: https://datadryad.org/stash/share/TMMKprXz1Iji8hvzbumzaVDQi0g0jqxzLPfdl2btxpA.
Results
Gregariousness in Prionostemma Harvestmen
Taking together the three sampling occasions, we found 390 Prionostemma individuals, from which 73 (18.7%) were roosting solitarily and 317 (81.3%) in aggregations. The total number of aggregations was 78, being 56 (71.8%) MSA and 22 (28.2%) SSA. The aggregation size ranged from 2 to 9 in MSA and from 2 to 15 in SSA (Figure 4A). The number of species in MSA ranged from 2 to 5, with 92.3% of the aggregations containing 2 or 3 species.
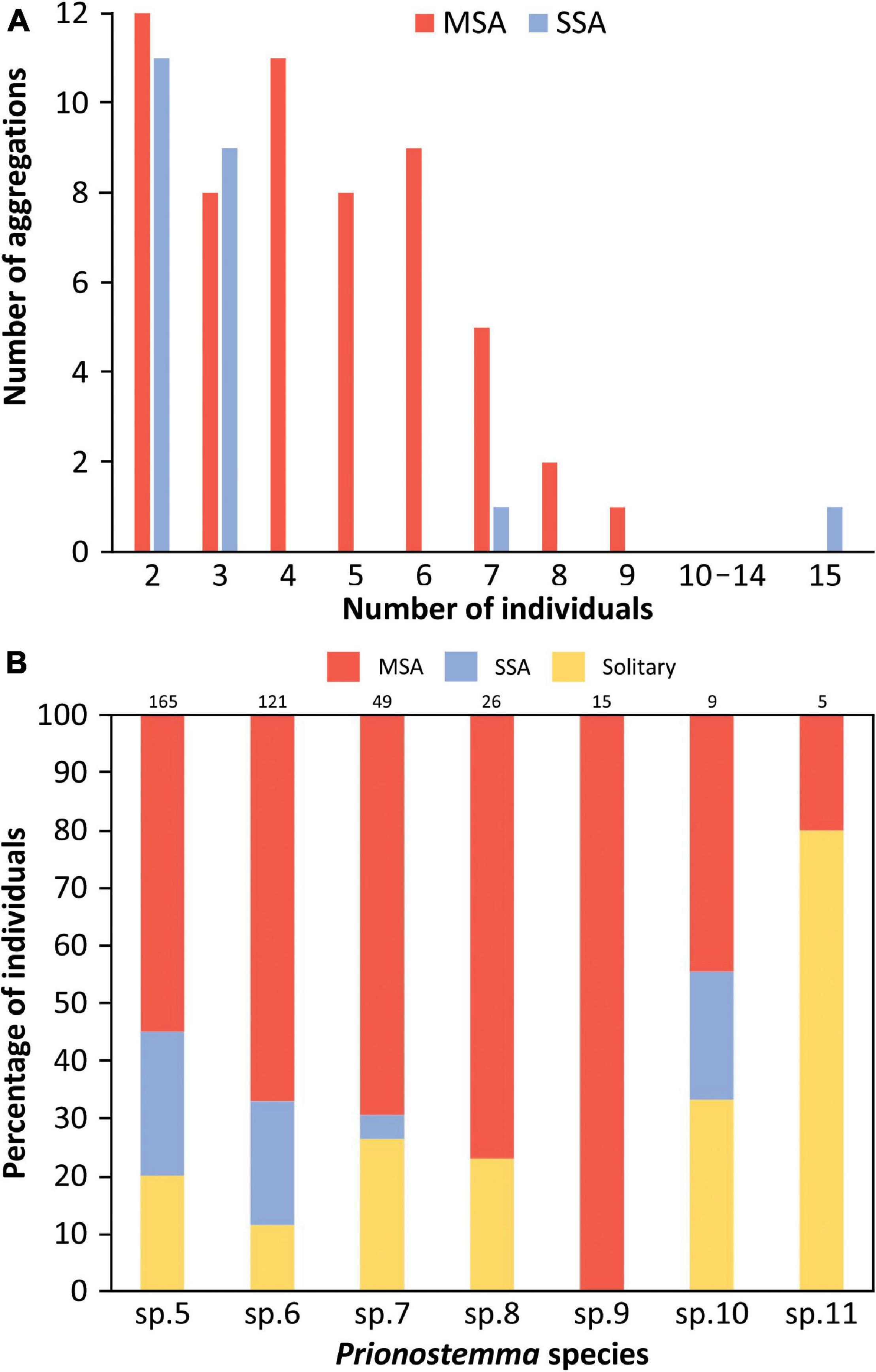
Figure 4. (A) Number of individuals (i.e., aggregation size) in single-species aggregations (SSA) and mixed-species aggregations (MSA) of seven species of Prionostemma harvestmen from Costa Rica. (B) Relative frequency of individuals of each Prionostemma species found in the field roosting solitarily or in aggregations (SSA or MSA). Numbers above the bars indicate the total number of individuals of each species.
The number of individuals was not evenly distributed among the seven Prionostemma species (Chi-square goodness-of-fit: X2 = 419.8, df = 6, P < 0.001, Figure 4B). The two most common species were Prionostemma sp.5 (42.2% of all individuals) and Prionostemma sp.6 (31.1%); the other five species comprised together 26.7% of all individuals (Figure 4B). The species differed in the proportion of individuals that were found roosting solitarily or in aggregations (Chi-square goodness-of-fit: X2 = 23.6, df = 6, P < 0.001, Figure 4B). For almost all species, individuals were more frequently found in aggregations than solitarily (Figure 4B). The only exception was Prionostemma sp.11, for which we collected only five individuals, one aggregated and four solitary (Figure 4B). When roosting in aggregations, individuals of Prionostemma sp.8, sp. 10, and sp. 11 were found only in MSA. In contrast, individuals of Prionostemma sp.5, sp.6, sp.7, and sp.9 were found both in MSA and SSA (Figure 4B).
To explore the co-occurrence of species in MSA we constructed an association matrix. This matrix quantified the number and percentage of times each species was found together with the other species in the same aggregation (Table 4). The three most common species (Prionostemma sp.5, sp.6, and sp.7) were commonly found with each other (Table 4). Each of the four other species (Prionostemma sp.8, sp.9, sp.10, and sp.11) were associated in roughly similar proportions with all species (Table 4). Additionally, three species combinations represented 54% (n = 30) of all species combinations observed in the field: (a) Prionostemma sp.5 + sp.6 (23%), (b) Prionostemma sp.5 + sp.6 + sp.7 (23%), and (c) Prionostemma sp.5 + sp. 7 (7%).
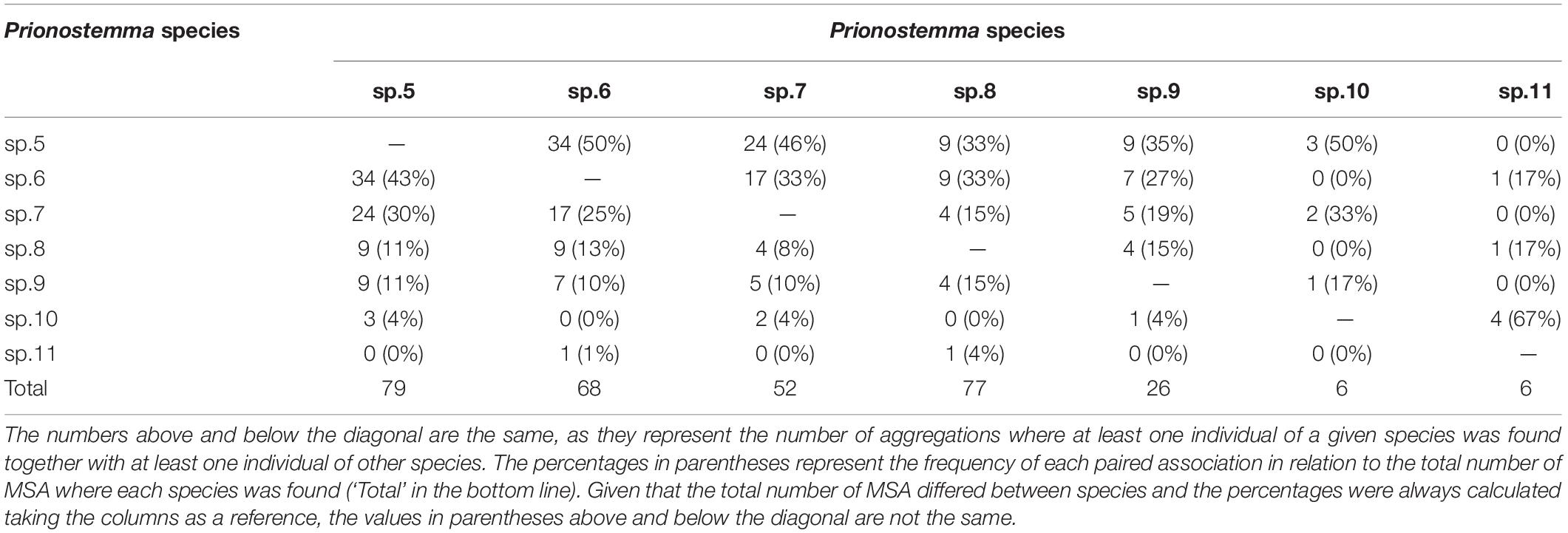
Table 4. Matrix of paired associations of all seven Prionostemma species that form mixed-species aggregations (MSA).
The number of individuals in the roosting sites was highly variable over time, ranging from 1 to 16. The mean (±SD) number of individuals was 3.3 ± 2.6 (n = 46), and the mean (±SD) coefficient of variation (CV) of the number of individuals across inspections was 55.2 ± 15.8% (range: 0–100%). The number of species in the roosting sites was also highly variable over time, ranging from 1 to 5. The mean (±SD) number of species was 1.8 ± 0.9 (n = 46), and the mean (±SD) CV across inspections was 43.3 ± 12.1% (range: 0–73.9%). Between two consecutive inspections, we recorded frequent transitions between all three categories of roosting status (Figure 5). The most frequent transition was from MSA to MSA, followed by MSA to solitary, and solitary to solitary (Figure 5). The least frequent transition was from solitary to SSA, followed by SSA to solitary, and MSA to SSA (Figure 5).
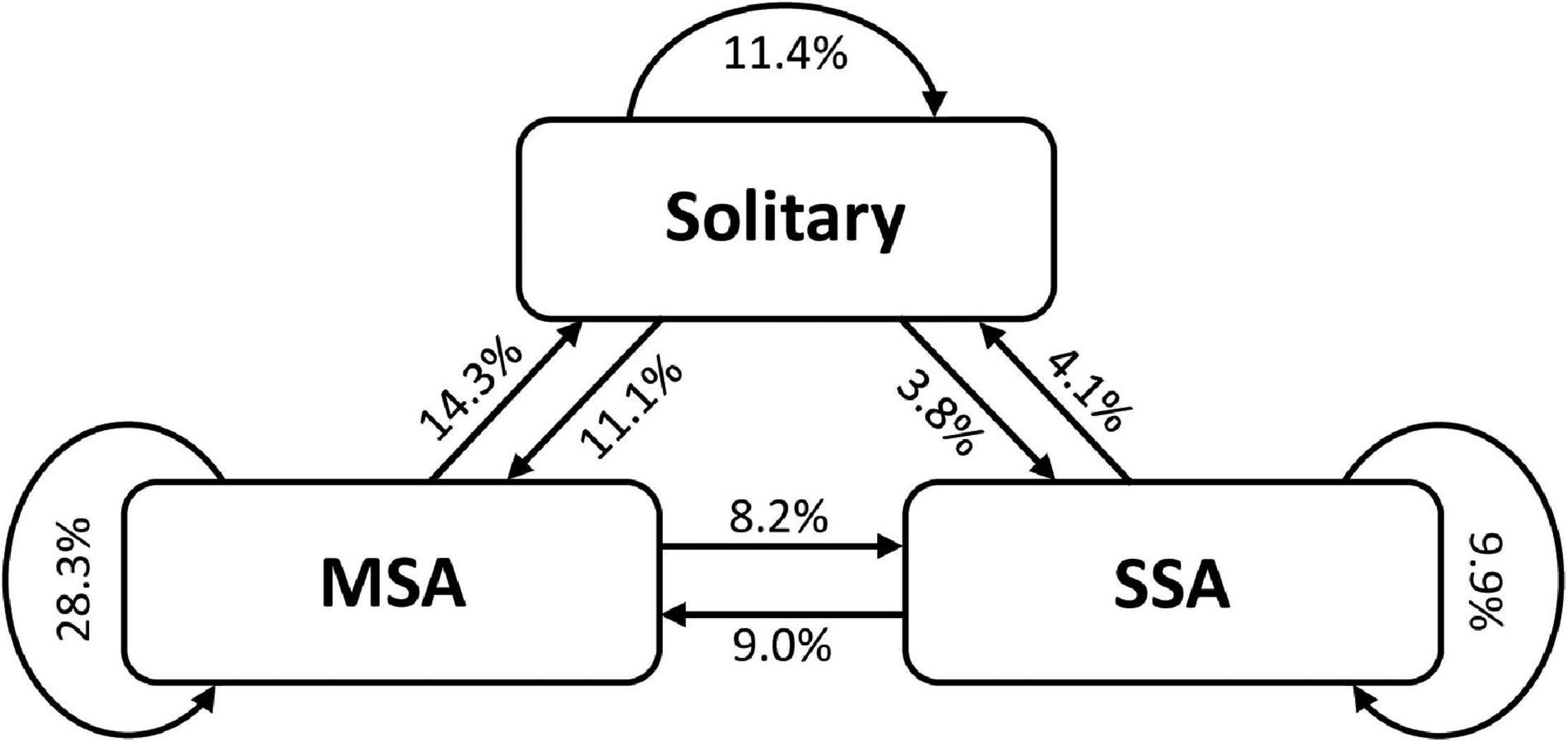
Figure 5. Transitions between three categories of roosting status: solitary, single-species aggregations (SSA), and mixed-species aggregations (MSA). Data are based on daily inspections of 46 roosting sites used by Prionostemma harvestmen.
Benefits of Gregariousness
We recorded leg loss for 390 Prionostemma individuals. A total of 194 individuals (all species together) were found missing at least one leg. Most of the autotomized individuals in all seven species were missing only one (63.9%) or two legs (25.3%), but some of them were missing three (8.3%) or even four legs (0.5%); for four individuals (2.1%) we do not have information on the number of missing legs. The frequency of autotomized individuals differed between species (Chi-square goodness-of-fit: X2 = 232.7, df = 6, P < 0.001), but the percentage of individuals missing at least one leg was always higher than 34% (Figure 6A).
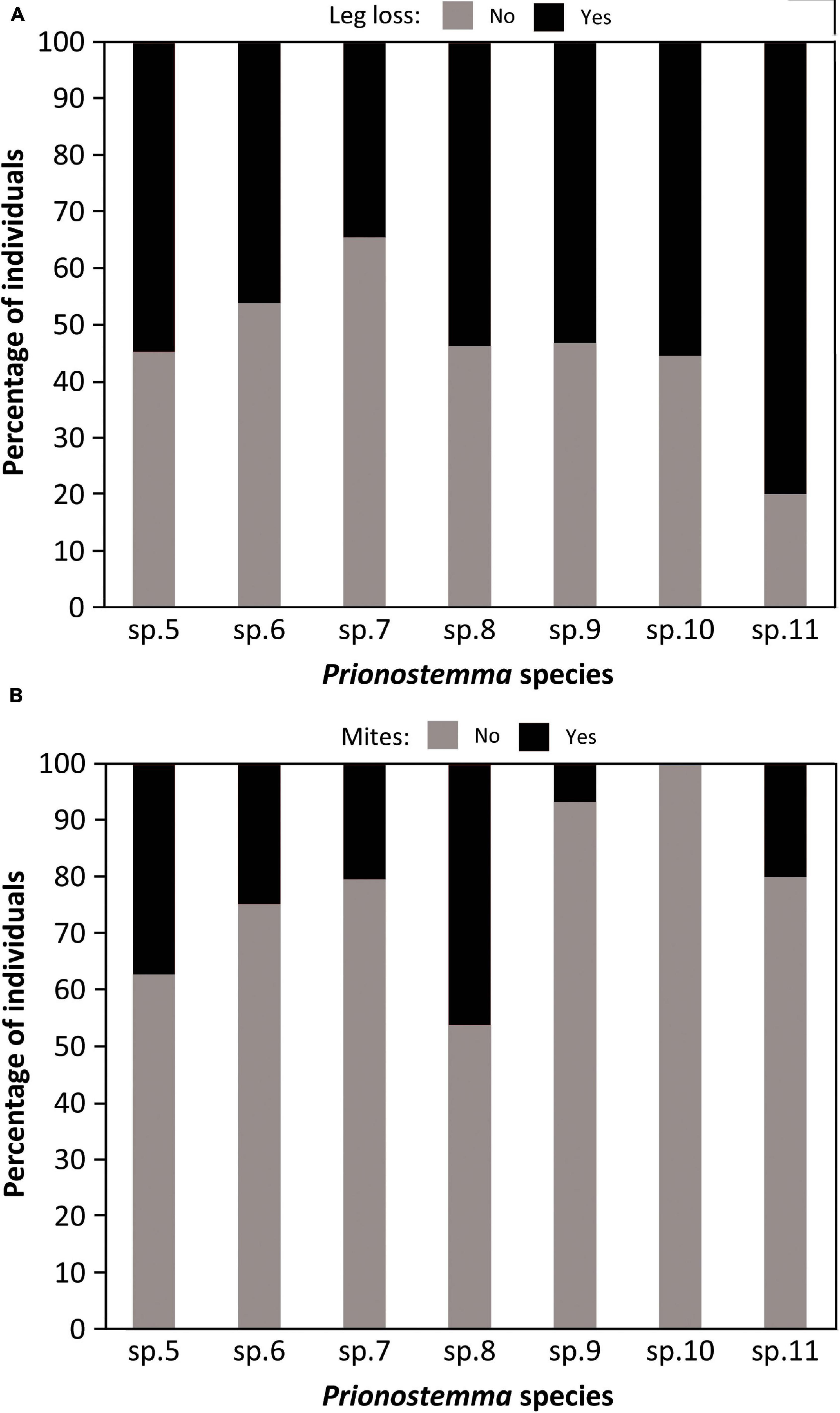
Figure 6. (A) Relative frequency of leg loss among individuals of seven Prionostemma species. (B) Relative frequency of individuals carrying at least one mite among seven Prionostemma species. Sample sizes for each species are the same presented in Figure 4B.
Leg loss was not associated with the roosting status of the individuals in any of the four species analyzed (i.e., Prionostemma sp.5, sp.6, sp.7, and sp.8). In Prionostemma sp.5, sp.6, and sp.7, individuals were more likely found in MSA than in SSA or solitary, but this pattern did not differ between intact (i.e., eight-legged) and autotomized individuals (Figures 7A–C, see also contrasts 1–3 in Figure 7E). In Prionostemma sp.8, individuals were more likely found solitary than in MSA, but again this pattern did not differ between intact and autotomized individuals (Figure 7D, see also contrasts 1-3 in Figure 7E).
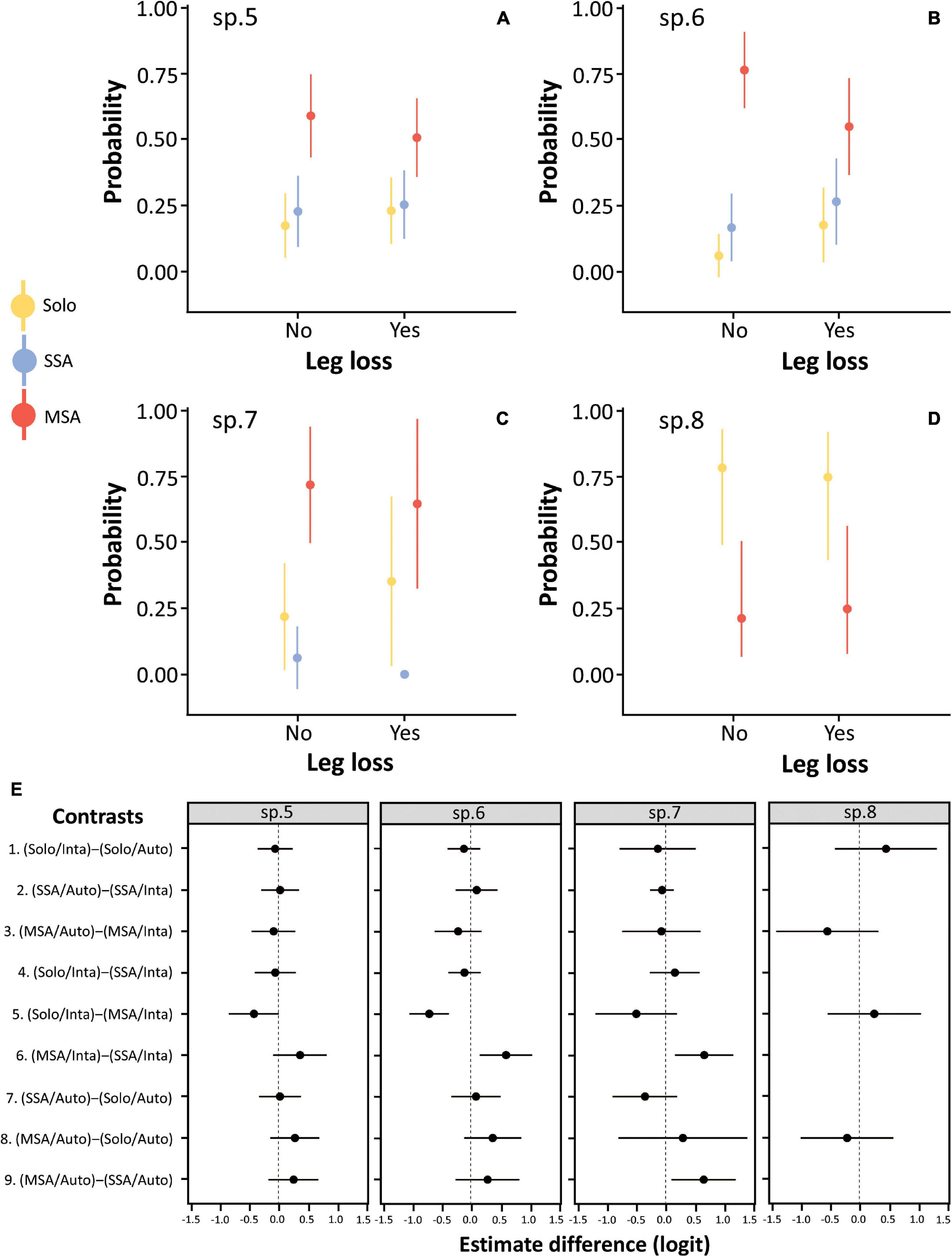
Figure 7. The probability of finding individuals of four harvestman species roosting solitary, in single-species aggregations (SSA), or in mixed-species aggregations (MSA) as a function of leg loss. (A) Prionostemma sp.5, (B) Prionostemma sp.6, (C) Prionostemma sp.7, and (D) Prionostemma sp.8 (without records of SSA). (E) Contrasts of the probabilities for combinations of roosting status (Solo, solitary; SSA and MSA) and leg loss (Inta, intact individuals and Auto, autotomized individuals). Each contrast is calculated as the probability value of the combination within the first parentheses minus the probability value of the combination within the second parentheses. Thus, a positive value indicates that the estimated probability for the combination within the first parentheses is higher than the combination within the second parentheses, whereas a negative value indicates the opposite. To facilitate visual interpretation, we arranged the parentheses so that positive values support our predictions, i.e., autotomized individuals will have a higher probability of being found in aggregations, especially in MSA, where they would be more protected from predators. Circles indicate mean values (A–D) or mean differences between probabilities (E). In all graphics, bars indicate 95% confidence intervals. In (E), contrast values with 95% confidence interval overlapping 0 were considered as non-significant.
For Prionostemma sp.5 and sp.6, the probability of finding intact individuals in MSA was higher than finding intact individuals solitarily (Figure 7, contrast 5 in Figure 7E). Moreover, for Prionostemma sp.6 and sp.7, the probability of finding intact individuals in MSA was higher than in SSA (Figure 7, contrast 6 in Figure 7E). For Prionostemma sp.5, sp.6, and sp.8, the probability of finding autotomized individuals solitary, in SSA, and in MSA was similar (Figure 7, contrasts 7–8 in Figure 7E). For Prionostemma sp.7, however, the probability of finding autotomized individuals was higher in MSA than in SSA (Figure 7, contrasts 9 in Figure 7E). Lastly, the proportion of autotomized individuals in the aggregations was not associated with aggregation size or type of aggregation (Table 5).
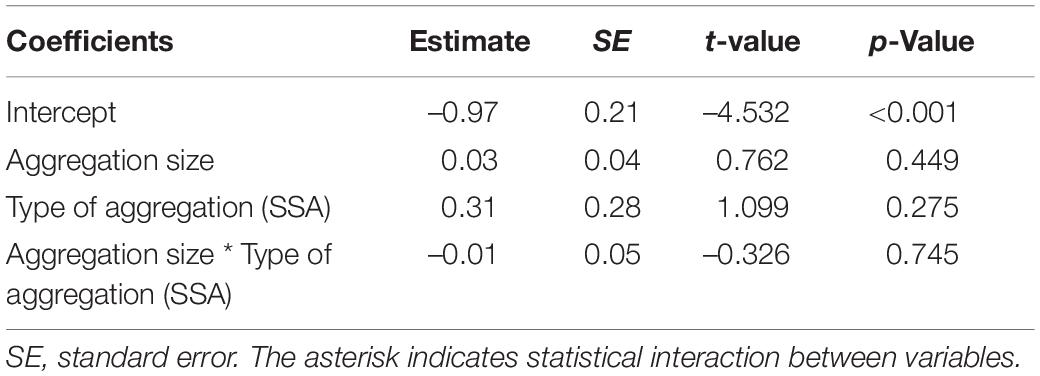
Table 5. Results of the models to investigate the effect of type of aggregation (SSA, single-species; MSA, mixed-species) and aggregation size on the percentage of autotomized individuals of Prionostemma harvestmen.
Costs of Gregariousness
We recorded the presence of parasitic mites for 390 Prionostemma individuals. A total of 115 individuals (all species together) had mites, and for 111 we have information on the number of mites they were carrying. Mites were present in different body parts (legs, dorsum, and venter), either motionless (probably sucking on the host’s hemolymph) or moving around (n = 12 observations). In 69.6% of all aggregations there was at least one individual carrying mites. The number of mites per individual ranged from 1 to 8, with the following relative frequencies: 1 = 57.7%, 2 = 25.2%, 3 = 9.9%, 4 = 2.7%, 5 = 2.7%, 6 = 0.9%, and 8 = 0.9%. The frequency of individuals carrying mites differed between species (Chi-square goodness-of-fit: X2 = 181.3, df = 6, P < 0.001, Figure 6B). However, for the four species we analyzed (i.e., Prionostemma sp.5, sp.6, sp.7, and sp.8), the roosting status had no effect on the probability of having mites (Figure 8) or the number of mites per individual (Figure 9).
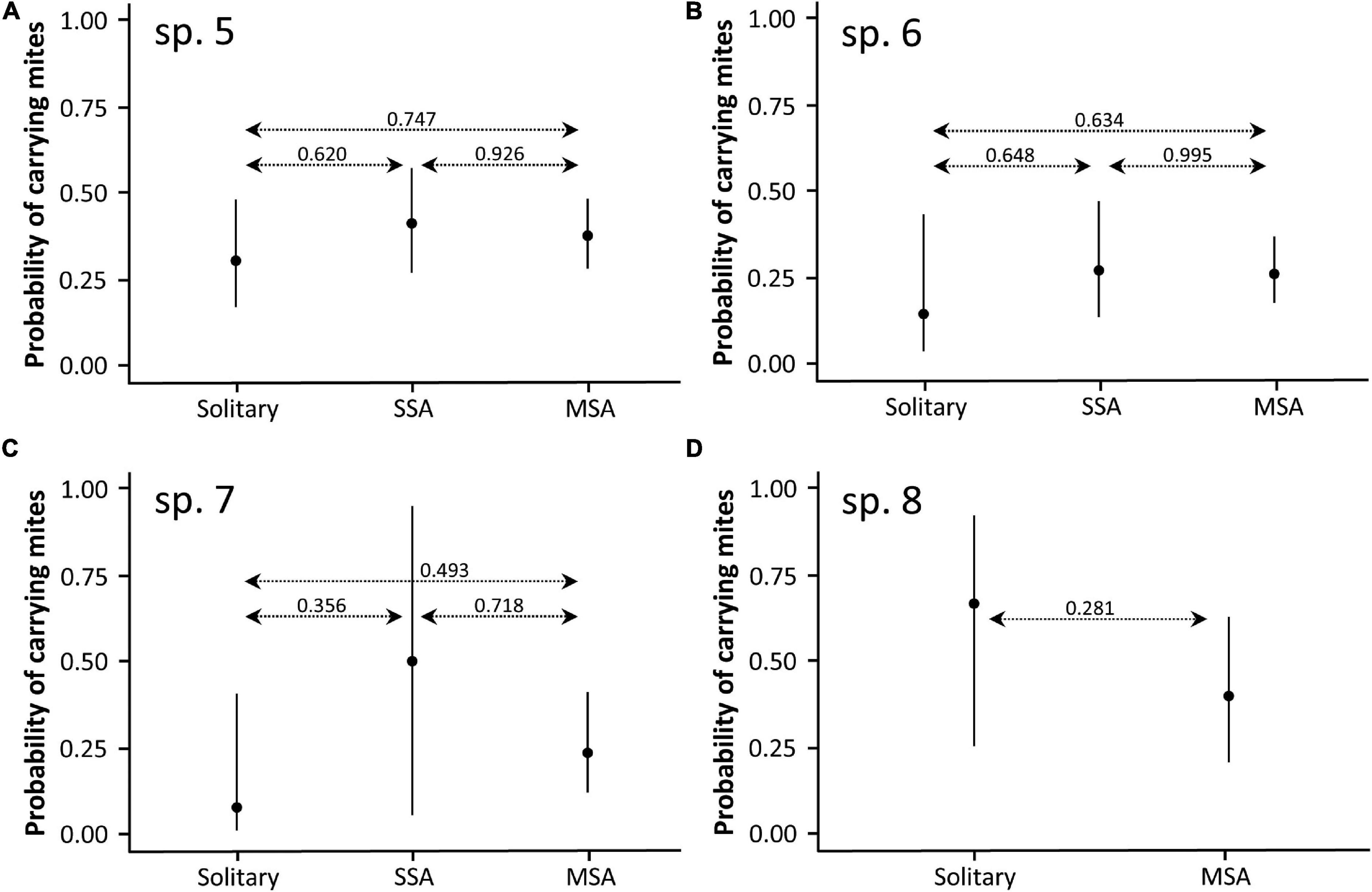
Figure 8. Probability of finding individuals of four harvestman species carrying parasitic mites in response to their roosting status (solitary;SA, single-species aggregation; MSA, mixed-species aggregation). (A) Prionostemma sp.5, (B) Prionostemma sp.6, (C) Prionostemma sp.7, and (D) Prionostemma sp.8 (without records of SSA). Dashed lines indicate pairwise P-values. Circles indicate mean values and bars indicate 95% confidence intervals. Values of all contrasts are presented in Supplementary Table 2.
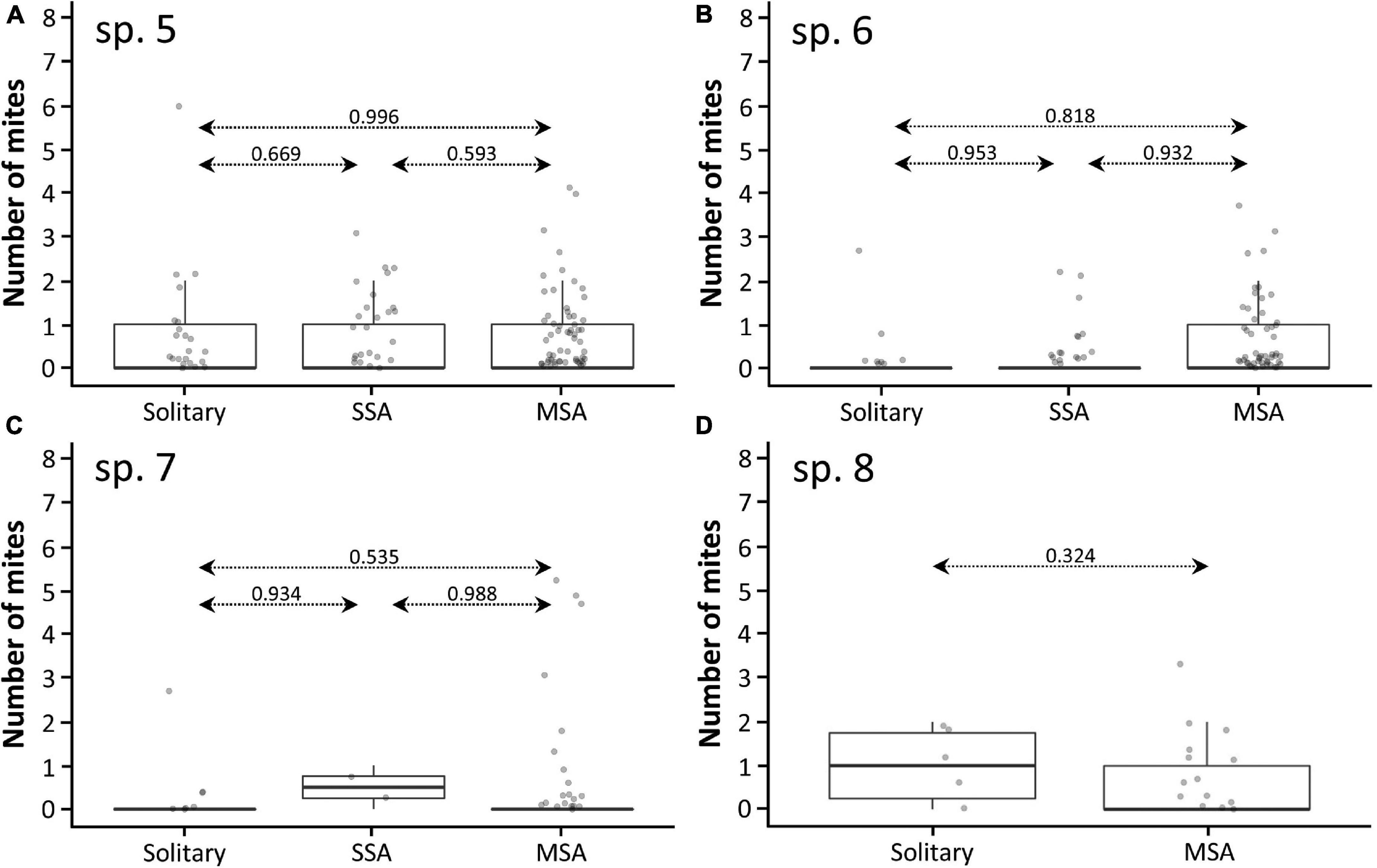
Figure 9. Box-plots showing the number of mites carried by individuals in response to their roosting status (solitary; SSA, single-species aggregation; MSA, mixed-species aggregation) of four harvestman species. (A) Prionostemma sp.5, (B) Prionostemma sp.6, (C) Prionostemma sp.7, and (D) Prionostemma sp.8 (without records of SSA). Dashed lines indicate pairwise P-values. Values of all contrasts are presented in Supplementary Table 3.
Discussion
The Costa Rican Prionostemma is an interesting study system to understand gregariousness in harvestmen because the individuals of each species are facultatively gregarious, and can be found solitarily or in aggregations, forming both SSA and MSA. Thus, we could evaluate one potential benefit and one potential cost of gregariousness and compare them between SSA and MSA. In what follows, we first explore the descriptive information we gathered in the field and then we discuss our findings on benefits and costs.
Gregariousness in Prionostemma Harvestmen
When compared with other species of Eupnoi, the Prionostemma aggregations described here are small, with no more than 16 individuals. Among sclerosomatids from temperate regions (e.g., Gyas, Leiobunum, and Nelima), aggregations are composed of hundreds to thousands of individuals, usually packed in high density (e.g., Wagner, 1954; Holmberg et al., 1984; Novak et al., 2004). One possible reason for this difference in the number and density of individuals may be related to the habitat: while Prionostemma species inhabit tropical forests, temperate sclerosomatid species inhabit cold and/or xeric places (Machado and Macías-Ordóñez, 2007). In tropical forests, where temperature is warm and pluviosity is high, aggregations may have no relevant role in protecting the individuals against harsh abiotic conditions. In turn, in cold and/or xeric places, a high number of individuals packed together may confer physiological benefits, buffering stressful abiotic conditions (Table 2; see also Danks, 2002).
The Prionostemma aggregations described here show the largest number of species among all MSA reported so far for harvestmen (Table 1), despite being relatively small aggregations compared to other harvestman species. Additionally, for six of the seven species we studied, individuals were found mainly aggregated (instead of solitary), and more frequently in MSA than in SSA. These findings suggest that Prionostemma harvestmen are mainly gregarious and highly tolerant to heterospecifics. How and why individuals from different species are brought together remains to be explored. Harvestmen use pheromones for different purposes (Raspotnig, 2012), and there is evidence for one species of Prionostemma from Nicaragua that individuals mark roosting sites with chemical compounds (which might function as pheromones) and come back to those roosting sites (Donaldson and Grether, 2007; Grether et al., 2014b). However, the attraction of heterospecifics to MSA requires that the compounds present in the chemical signal (i.e., infochemicals) to be recognized by all aggregating species. It is currently unknown if all the Prionostemma species studied here produce and deposit recruitment infochemicals. Perhaps Prionostemma sp.5 and sp.6 – the two most common species in the study site – may act as ‘initiators’ of the MSA depositing recruitment pheromones. The other species, which are rarer, may act as ‘followers’ and join the MSA by eavesdropping on the infochemical. Although speculative, a similar mechanism has already been reported for the formation of mixed-species flocks in birds by means of differential production of vocalizations (Sridhar et al., 2009; Magrath et al., 2015; Goodale et al., 2019, 2020). Future work on Prionostemma can provide novel insights on the function of chemical compounds in harvestman, as well as the role of chemical communication in group formation.
Three combinations of species in MSA represented almost 54% of the field records (Table 4). This is surprising as seven species can generate 120 possible combinations of species. Additionally, the three most common species tended to occur together. For visually oriented predators, two of these common species may be regarded as cryptic (Prionostemma sp.6: dark gray body; and Prionostemma sp. 7: green body), whereas one of them may be regarded as conspicuous (Prionostemma sp.5: red body) (Figure 3). In all three most frequent associations, there was a mixture of a conspicuous species and at least one cryptic species. This difference in conspicuity may promote asymmetries in the defense benefits obtained by individuals of each species. Two possible scenarios of these between-species asymmetries are plausible. First, individuals of conspicuous species may be safer in MSA composed mainly of individuals of cryptic species, because SSA of conspicuous species may attract more attention of visually oriented predators than SSA of cryptic species (Vulinec, 1990). Second, assuming that all Prionostemma are chemically defended, individuals of cryptic species may be safer in MSA composed mainly of individuals of conspicuous species if predators avoid attacking aggregations of aposematic prey (e.g., Rippi et al., 2001). Alternatively, individuals of both cryptic and conspicuous species may enjoy similarly higher defense benefits in MSA if the confusion effect they cause on potential predators is more intense than in SSA (see section ‘Benefits of mixed-species aggregations in harvestmen’ below).
The number of individuals and species in the roosting sites showed marked variation over time. All possible transitions between the categories of roosting status (solitary, SSA, and MSA) were frequently recorded (Figure 5). To our knowledge, this is the first time that the temporal dynamic of mixed-species aggregations is explored in harvestmen. Our data reinforces previous suggestions that Prionostemma individuals are constantly moving between aggregations (Donaldson and Grether, 2007). Why individuals do that is an open question that deserves investigation. One possibility is that suitable roosting sites are very common in the study site. After leaving a roosting site at dusk, an individual may go far away searching for food or mating partners. At dawn, instead of paying the costs of returning to the original roosting site, these individuals may simply go to the best roosting site nearby. An important implication of the constant movement of individuals is that one roosting site may have an aggregation in 1 day and a solitary individual in the next day. Moreover, frequent transitions between SSA and MSA suggest that the benefits and costs associated with these two types of aggregations are similar. Otherwise, we would expect higher temporal stability and low frequency of transitions.
Benefits of Mixed-Species Aggregations in Harvestmen
We found a high frequency of leg loss in all Prionostemma species studied here (Figure 6A). This is consistent with previous reports for many Eupnoi harvestmen (e.g., Guffey, 1999; Houghton et al., 2011; Escalante et al., 2013, 2020, 2021; Domínguez et al., 2016; Escalante and Elias, 2021; Powell et al., 2021a,b). However, leg loss had no effect on the roosting status of the individuals, i.e., whether they were found solitary or in aggregations (SSA or MSA). Our hypothesis relating leg loss to the defense benefits of gregariousness assumed that autotomized individuals are more exposed to predation than intact individuals. Therefore, a first explanation for the lack of relationship between leg loss and roosting status is that our assumption does not hold. Although leg loss hampers the locomotor performance of sclerosomatid harvestmen (Escalante et al., 2013, 2020, 2021; Domínguez et al., 2016), a recent study showed that the recapture rates of autotomized and intact individuals of Prionostemma sp.5 are similar, suggesting that autotomy does not negatively impact individual survival (Escalante and Elias, 2021). That study also showed that autotomy changed micro-habitat use so that individuals missing legs were more frequently recaptured roosting among moss than on exposed tree trunks (Escalante and Elias, 2021). This finding suggests that autotomized individuals seek protection in concealed micro-habitats and perhaps this behavior is more effective in preventing predation than joining an aggregation.
If autotomized individuals are indeed more exposed to predation and find protection against predators in aggregations, we hypothesized that MSA would confer higher defense benefits due to the confusion effect, and predicted the probability of finding autotomized individuals roosting in MSA would be higher than in SSA. Our results support this prediction only for Prionostemma sp.7: the percentage of autotomized individuals was higher in MSA than in SSA. For Prionostemma sp.5 and sp.6, although the results we found are in the expected direction (i.e., a positive value of contrast 9 in Figure 7E), they were not statistically significant. This finding suggests that any possible defense benefit caused by the presence of multiple species in the aggregation is asymmetric. Whereas individuals of Prionostemma sp.7 may have higher defense benefits in joining MSA, the same is probably not true for Prionostemma sp.5 and sp.6. The reasons for this asymmetry remain to be better understood, but are unlikely to be explained by the confusion effect. As we mentioned above, Prionostemma sp.5, sp.6, and sp. 7 are commonly found together and show marked difference in body color. Thus, if the confusion effect emerges because phenotypic variation among aggregated species disrupts the search image of predators (Table 2), autotomized individuals of the three species should prefer to join MSA, which was not found in the field.
Finally, we expected that autotomized individuals of all species would prefer to join larger aggregations, where they would be more protected (Vulinec, 1990). This prediction was not supported by our data as aggregation size was not associated with the percentage of autotomized individuals. Although gregariousness may decrease the individual chance of predation via the dilution effect, it may also increase the chances of detection by predators because aggregations may be visually or chemically more conspicuous than isolated individuals (Tables 2, 3). In sclerosomatid harvestmen, the main predators during daytime (when individuals are roosting) are probably birds (Cokendolpher and Mitov, 2007), which are visually oriented and may detect aggregations more easily than solitary individuals. Therefore, the encounter and dilution effects may operate in different directions (see Figure 1 in Wrona and Dixon, 1991), and both need to be considered simultaneously in future studies (i.e., the attack-abatement effect sensu Turner and Pitcher, 1986) to fully assess the net fitness benefit of gregariousness.
Costs of Aggregating With Other Species
Mite prevalence for most Prionostemma species studied here (Figure 6B) is within the range already reported for other sclerosomatids worldwide, which varies from 16.7% (Townsend et al., 2008) to 61% (McAloon and Durden, 2000). In a comparative study with six harvestman species from Trinidad, Townsend et al. (2008) suggested that differences between species in mite prevalence are mainly related to habitat use: species that live or forage on the leaf litter are more likely to be parasitized because erythraeid mites lay their eggs in the soil. In a similar way, a study on two gregarious Prionostemma species in Nicaragua showed that mite prevalence varied between roosting sites (Grether et al., 2014a). In some spiny palms, no mites were found infesting the aggregated individuals, but in one of them, mite prevalence was 31.1%. Here, we investigated whether the roosting status of the individuals affects mite parasitism in the four most common Prionostemma species in our study site. We found that mite prevalence differed between species (Figure 6B), which suggests some level of host specificity or preference by the erythraeid mites. However, within species mite prevalence was similar for solitary and aggregated individuals – regardless of whether they were in SSA or MSA (Figure 8). This finding refutes our hypothesis that gregariousness increases the chance of horizontal transmission of ectoparasites.
The intensity of parasitism in the seven Prionostemma species studied here ranged from 1 to 8 mites per individual. As reported in previous studies with sclerosomatids from the United States (McAloon and Durden, 2000) and phalangiids from Bulgaria (Mitov, 2000), most individuals carried few mites, whereas a few individuals were heavily parasitized. Clumped parasite distributions on hosts are a widespread pattern in animals (Leung, 1998). In the case of erythraeid mites, only the larval stages are parasitic, so that the high parasite load of few Prionostemma individuals may result from a host being exposed to an aggregation of larvae just after they hatch (McAloon and Durden, 2000). Despite the great inter-individual variation, we showed that the intensity of parasitism was not affected by the roosting status (Figure 9). This finding reinforces the conclusion that aggregating with conspecific or heterospecifics does not bring different costs in terms of mite parasitism in Prionostemma harvestmen.
The fluid membership of the Prionostemma aggregations, with marked daily turnovers in the number of individuals and species composition at the roosting sites (Figure 5), can have important implications for the transmission of mites between individuals. Given that individuals are likely changing their roosting status over time, a snapshot sample (as the one conducted here) does not provide a precise picture of their risk of being parasitized. A recent mathematical model has shown that aggregation size and roost site fidelity are key factors influencing pathogen spreading in populations of gregarious species (Laughlin et al., 2019). According to this model, pathogens spread faster among roosting sites when (i) individuals are distributed among a large number of small aggregations and (ii) exhibit low roost site fidelity. These two conditions apply to Prionostemma harvestmen (Figures 4A, 5), which may explain why nearly 70% of all aggregations had at least one individual carrying mites. However, to better understand the factors that influence the individual risk of being parasitized, future research should be conducted with individually marked harvestmen. The question to be explored is whether individuals with higher tendency of being found in aggregations are more likely to be parasitized than those with higher tendency of being found solitary. There is increasing evidence that individuals vary widely in their probability of contracting and spreading parasites (Barron et al., 2015), and Prionostemma harvestmen offer an opportunity to explore this subject in species that form both SSA and MSA.
Conclusion
Our review highlights that most of the potential benefits of MSA in harvestmen are similar to those reported for SSA in other taxa (Figure 2; see also Boulay et al., 2019; Goodale et al., 2020). For the physiological benefits, for instance, aggregations may reduce metabolic rates regardless of whether they are composed of one or multiple species. Most of the defense benefits — dilution effect, confusion effect, increased vigilance, and collective retaliation — should also work in SSA and MSA. However, there are circumstances in which the physiological and defense benefits in MSA are expected to be asymmetric, with some species obtaining more benefits than others (Figure 2). In harvestmen, an extreme example of this asymmetry probably occurs when only one species is chemically defended. In this case, individuals of the non-defended species may be regarded as parasites of both the alarm signal and the defensive compounds released by individuals of the chemically defended species (Machado and Vasconcelos, 1998). Lastly, in some circumstances, MSA may confer higher benefits than SSA. For instance, future studies should experimentally address the potential increase in the confusion effect in MSA, which should be higher than in SSA if variation in color between species disrupts the search image of predators even further.
Contrary to the benefits, our review suggests that most of the potential costs of MSA for harvestmen differ from those reported for SSA in other taxa (Figure 2; see also Goodale et al., 2020). For instance, if pathogens and parasites are species-specific, the likelihood of transmission may be lower when individuals are aggregated with heterospecifics — a pattern already reported for birds (e.g., Poulin, 2010; González et al., 2014). Moreover, if aggregated individuals fiercely compete for resources when they leave the aggregations, MSA may attenuate competition because interspecific variation is also expected in their diet and microhabitats used for foraging (e.g., Krasnov et al., 2006; but see Kaplan and Denno, 2007). Finally, if aggregations are related to reproduction, reproductive costs, such as sexual harassment, extra-pair copulation, misdirect parental care, and infanticide, should be lower in MSA than in SSA due to the lower density of conspecifics nearby (Figure 2). In all examples mentioned so far, the costs of MSA are lower than SSA, but there are two exceptions: the costs related to increased conspicuousness to predators and increased risk of pathogens and parasites transmission. In these two cases, if individuals of different species are equally conspicuous to predators and susceptible to non-specific parasites, the costs of MSA should be similar to those of SSA (Figure 2).
In our empirical study, we investigated one potential benefit and one potential cost of gregariousness in seven Prionostemma species that roost either solitarily or in groups, forming SSA or MSA. Although our data provide an observational snapshot of the study system, they are the first formal test of the hypotheses that gregariousness confers defense benefits but incurs costs in terms of increased parasitism. We found that intact and autotomized individuals of the four most common Prionostemma species have similar probability of being found solitarily or in aggregations. This result refutes our hypothesis that autotomized individuals would be found preferentially in aggregations, where they would be more protected from predators. We stress, however, that our findings do not discard the possibility that harvestman aggregations improve defense since our test does not directly address any of the specific defense benefits in Table 2. Regarding the costs, we found that mite prevalence or infestation intensity were similar between solitary or aggregated individuals. This result refutes our hypothesis that gregariousness would increase the chances of mite parasitism in harvestmen. We suggest that the fluid membership of the aggregations, with great variation over time in the number of individuals and species composition, may explain the lack of relationship between roosting status and mite parasitism.
Overall, we expect that both our review and the empirical results stimulate further investigation on group living in harvestmen. We highlighted many questions to be answered in fields of knowledge as diverse as physiology, chemical ecology, parasitology, and behavioral ecology. Moreover, the frequency of species that form MSA in harvestmen is higher than any other arthropod taxa (see Table 1 in Boulay et al., 2019 and Table 1 in the present study). In the same species that form MSA, individuals are also found solitarily or forming SSA. As we showed here, the genus Prionostemma offers a unique opportunity to quantify the benefits and costs of gregariousness and to compare them between different types of aggregation.
Data Availability Statement
The datasets presented in this study can be found in online repositories. The names of the repository/repositories and accession number(s) can be found below: Dryad (https://datadryad.org/stash/share/TMMKprXz1Iji8hvzbumzaVDQi0g0jqxzLPfdl2btxpA).
Author Contributions
All authors contributed equally to the conceptualization, data curation, analysis, and review and editing of the manuscript. IE, MD, and DAG-R conducted the field-data collection. IE and GM led the writing of the original draft. IE led the funding acquisition and project administration.
Funding
Funding for this study came from the Research Fellowship Program (Christiane & Christopher Tyson Fellowship, and the Don & Beverly Stone Fellowship) from the Organization for Tropical Studies (OTS) to IE. GM was supported by research grants from the National Council for Technological and Scientific Development (CNPq 302743/2019-7) and the São Paulo Research Foundation (FAPESP 2021/00915-5).
Conflict of Interest
The authors declare that the research was conducted in the absence of any commercial or financial relationships that could be construed as a potential conflict of interest.
Publisher’s Note
All claims expressed in this article are solely those of the authors and do not necessarily represent those of their affiliated organizations, or those of the publisher, the editors and the reviewers. Any product that may be evaluated in this article, or claim that may be made by its manufacturer, is not guaranteed or endorsed by the publisher.
Acknowledgments
We are grateful to R. Quirós, Y. Blanco, and E. Triana for their support during fieldwork, to R. L. Rodríguez, K. Fowler-Finn, R. Willemart, E. Hebets, G. Höebel, L. Whittingham, V. R. Townsend, A. G. Farji, F. Chinchilla, and the two reviewers for their feedback on the ideas presented in the manuscript, and D. Solano-Brenes and S. García-Hernández for helping with the statistical analyses.
Supplementary Material
The Supplementary Material for this article can be found online at: https://www.frontiersin.org/articles/10.3389/fevo.2021.766323/full#supplementary-material
References
Åbro, A. (1988). The mode of attachment of mite larvae (Leptus spp.) to harvestmen (Opiliones). J. Nat. Hist. 22, 123–130. doi: 10.1080/00222938800770091
Acosta, L. E., and Machado, G. (2007). “Diet and foraging,” in Harvestmen: The Biology Of Opiliones, eds R. Pinto-da-Rocha, G. Machado, and G. Giribet (Cambridge: Harvard University Press), 309–338.
Adams, J. (1984). The habitat and feeding ecology of woodland harvestmen (Opiliones) in England. Oikos 42, 361–370. doi: 10.2307/3544406
Anderson, J. F. (1993). Respiratory energetics of two Florida harvestmen. Comp. Biochem. Physiol. A 105, 67–72. doi: 10.1016/0300-9629(93)90174-3
Aviles, L. (1997). “Causes and consequences of cooperation and permanent-sociality in spiders,” in The Evolution Of Social Behavior In Insects And Arachnids, eds J. C. Choe and B. J. Crespi (Cambridge: Cambridge University Press), 476–498. doi: 10.1186/1471-2148-9-257
Barron, D. G., Gervasi, S. S., Pruitt, J. N., and Martin, L. B. (2015). Behavioral competence: how host behaviors can interact to influence parasite transmission risk. Curr. Opin. Behav. Sci. 6, 35–40. doi: 10.1016/j.cobeha.2015.08.002
Benoit, J. B., Bose, J., Bailey, S. T., and Polak, M. (2020). Interactions with ectoparasitic mites induce host metabolic and immune responses in flies at the expense of reproduction-associated factors. Parasitology 147, 1196–1205. doi: 10.1017/S0031182020000918
Benton, A. H., and Crump, A. J. (1979). Observations on aggregation and overwintering in the coccinellid beetle Coleomegilla maculata (DeGeer). J. N.Y. Entomol. Soc. 87, 154–159.
Boulay, J., Aubernon, C., Ruxton, G. D., Valéry, H., Deneubourg, J. L., and Charabidzé, D. (2019). Mixed-species aggregations in arthropods. Insect Sci. 26, 2–19. doi: 10.1111/1744-7917.12502
Brooker, R. M., Feeney, W. E., Sih, T. L., Maud-Ferrari, C. O., and Chivers, D. P. (2019). Comparative diversity of anemone-associated fishes and decapod crustaceans in a Belizean coral reef and seagrass system. Mar. Biodiv. 49, 2609–2620. doi: 10.1007/s12526-019-00993-5
Capocasale, R. M., and Bruno-Trezza, L. (1964). Biología de Acanthopachylus aculeatus (Kirby, 1819) (Opiliones: Pachylinae). Rev. Soc. Uruguaya Entomol. 6, 19–32.
Casey, T. M. (1993). “Effects of temperature on foraging of caterpillars,” in Caterpillars: Ecological And Evolutionary Constraints On Foraging, eds N. E. Stamp and T. M. Casey (New York, NY: Chapman and Hall), 5–28.
Casey, T. M., Joos, B., Fitzgerald, T. D., Yurlona, M., and Young, P. (1988). Synchronized group feeding, thermoregulation, and growth of eastern tent caterpillars in relation to microclimate. Physiol. Zool. 61, 372–377.
Chelini, M. C., Willemart, R. H., and Gnaspini, P. (2012). Gregarious behavior of two species of Neotropical harvestmen (Arachnida: Opiliones: Gonyleptidae). J. Arachnol. 40, 256–258. doi: 10.1636/hi11-12.1
Chown, S., and Nicolson, S. W. (2004). Insect Physiological Ecology: Mechanisms And Patterns. Oxford: Oxford University Press.
Cockerill, J. J. (1988). Notes on aggregations of Leiobunum (Opiliones) in the Southern USA. J. Arachnol. 16, 123–126.
Cocroft, R. B. (2001). Vibrational communication and the ecology of group-living, herbivorous insects. Am. Zool. 41, 1215–1221. doi: 10.1093/icb/41.5.1215
Coddington, J., Horner, M., and Soderstrom, E. (1990). Mass aggregations in tropical harvestmen (Opiliones, Gagrellidae: Prionostemma sp.). Rev. Arachnol. 8, 213–219.
Cokendolpher, J. C. (1993). Pathogens and parasites of Opiliones (Arthropoda: Arachnida). J. Arachnol. 21, 120–146.
Cokendolpher, J. C., and Mitov, P. G. (2007). “Natural enemies,” in Harvestmen: The Biology Of Opiliones, eds R. Pinto-da-Rocha, G. Machado, and G. Giribet (Cambridge: Harvard University Press), 339–373.
Côté, I. M., and Poulin, R. (1995). Parasitism and group size in social animals: a meta-analysis. Behav. Ecol. 6, 159–165.
Curtis, D. J., and Machado, G. (2007). “Ecology,” in Harvestmen: The Biology Of Opiliones, eds R. Pinto-da-Rocha, G. Machado, and G. Giribet (Cambridge: Harvard University Press), 280–308.
Dangerfield, J. M. (1993). Aggregation in the tropical millipede Alloporus uncinatus (Diplopoda: Spirostreptidae). J. Zool. 230, 503–511.
Danks, H. V. (2002). Modification of adverse conditions by insects. Oikos 99, 10–24. doi: 10.1034/j.1600-0706.2002.990102.x
Del-Claro, D., and Tizo-Pedroso, E. (2009). Ecological and evolutionary pathways of social behavior in Pseudoscorpions (Arachnida: Pseudoscorpiones). Acta Ethol. 12, 13–22.
Domínguez, M., Escalante, I., Carrasco-Rueda, F., Figuerola-Hernández, C. E., Ayup, M. M., Umaña, M. N., et al. (2016). Losing legs and walking hard: effects of autotomy and different substrates in the locomotion of harvestmen in the genus Prionostemma. J. Arachnol. 44, 76–82. doi: 10.1636/j15-08.1
Donaldson, Z. R., and Grether, G. F. (2007). Tradition without social learning: scent-mark-based communal roost formation in a Neotropical harvestman (Prionostemma sp.). Behav. Ecol. Sociobiol. 61, 801–809. doi: 10.1007/s00265-006-0311-0
Edgar, A. L. (1971). Studies on the biology and ecology of Michigan Phalangida (Opiliones). Misc. Pub. Museum Zool. Univ. Michigan 144, 1–64.
Eisner, T., Rossini, C., González, A., and Eisner, M. (2004). Chemical defense of an opilionid (Acanthopachylus aculeatus). J. Exp. Zool. 207, 1313–1321. doi: 10.1242/jeb.00849
Elpino-Campos, A., Pereira, W., Del-Claro, K., and Machado, G. (2001). Behavioural repertory and notes on natural history of the Neotropical harvestman Discocyrtus oliverioi (Opiliones: Gonyleptidae). Bull. Br. Arachnol. Soc. 12, 144–150.
Emberts, Z., Escalante, I., and Bateman, P. W. (2019). The ecology and evolution of autotomy. Biol. Rev. 94, 1881–1896. doi: 10.1111/brv.12539
Escalante, I., Albín, A., and Aisenberg, A. (2013). Lacking sensory (rather than locomotive) legs affects locomotion but not food detection in the harvestman Holmbergiana weyenberghi. Can. J. Zool. 91, 726–731. doi: 10.3390/ijms21020405
Escalante, I., and Elias, D. O. (2021). The type of leg lost affects habitat use but not survival in a non-regenerating arthropod. Ecol. Evol. 11, 10672–10685. doi: 10.1002/ece3.7879
Escalante, I., Badger, M. A., and Elias, D. O. (2019). Variation in movement: multiple locomotor gaits in neotropical harvestmen. Biol J Linn Soc 127, 493–507. doi: 10.1093/biolinnean/blz047
Escalante, I., Badger, M. A., and Elias, D. O. (2020). Rapid recovery of locomotor performance after leg loss in harvestmen. Sci. Rep. 10, 1–13. doi: 10.1038/s41598-020-70557-2
Escalante, I., Ellis, V. R., and Elias, D. O. (2021). Leg loss decreases endurance and increases oxygen consumption during locomotion in harvestmen. J. Comp. Physiol. A 207, 257–268. doi: 10.1007/s00359-020-01455-1
Fitzgerald, T. D. (1993). “Sociality in caterpillars,” in Caterpillars: Ecological And Evolutionary Constraints On Foraging, eds N. E. Stamp and T. M. Casey (New York, NY: Chapman and Hall), 372–404.
Fleming, P. A., Muller, D., and Bateman, P. W. (2007). Leave it all behind: a taxonomic perspective of autotomy in invertebrates. Biol. Rev. 82, 481–510. doi: 10.1111/j.1469-185X.2007.00020.x
Gerhold, P., Cahill, J. F., Winter, M., Bartish, I. V., and Prinzing, A. (2015). Phylogenetic patterns are not proxies of community assembly mechanisms (they are far better). Funct. Ecol. 29, 600–614.
Gilbert, C., McCafferty, D., Maho, Y. L., Martrette, J. M., Giroud, S., Blanc, S., et al. (2010). One for all and all for one: the energetic benefits of huddling in endotherms. Biol. Rev. 85, 545–569. doi: 10.1111/j.1469-185X.2009.00115.x
Gnaspini, P. (1996). Population ecology of Goniosoma spelaeum, a cavernicolous harvestmen from South-eastern Brazil (Arachnida: Opiliones: Gonyleptidae). J. Zool. 239, 417–435.
Gnaspini, P. (2007). “Development,” in Harvestmen: The Biology Of Opiliones, eds R. Pinto-da-Rocha, G. Machado, and G. Giribet (Cambridge: Harvard University Press), 455–472.
Gnaspini, P., and Hara, M. R. (2007). “Defense mechanisms,” in Harvestmen: The Biology Of Opiliones, eds R. Pinto-da-Rocha, G. Machado, and G. Giribet (Cambridge: Harvard University Press), 374–399.
González, A. D., Matta, N. E., Ellis, V. A., Miller, E. T., Ricklefs, R. E., and Gutierrez, H. R. (2014). Mixed species flock, nest height, and elevation partially explain avian haemoparasite prevalence in Colombia. PLoS One 9:e100695. doi: 10.1371/journal.pone.0100695
Goodale, E., Beauchamp, G., and Ruxton, G. (2017). Mixed-Species Groups Of Animals: Behavior, Community Structure, And Conservation. Cambridge, MA: Academic Press.
Goodale, E., Ratnayake, C. P., and Kotagama, S. W. (2014). Vocal mimicry of alarm associated sounds by a drongo elicits flee and mobbing responses from other species that participate in mixed species bird flocks. Ethology 120, 266–274. doi: 10.1111/eth.12202
Goodale, E., Ruxton, G. D., and Beauchamp, G. (2019). Predator eavesdropping in a mixed-species environment: How prey species may use grouping, confusion, and the cocktail party effect to reduce predator detection. Front. Ecol. Evol. 7:141. doi: 10.3389/fevo.2019.00141
Goodale, E., Sridhar, H., Sieving, K. E., Bangal, P., Colorado, G. J., Farine, D. R., et al. (2020). Mixed company: a framework for understanding the composition and organization of mixed-species animal groups. Biol. Rev. 95, 889–910. doi: 10.1111/brv.12591
Greenfield, M. D. (2015). Signal interactions and interference in insect choruses: singing and listening in the social environment. J. Comp. Physiol. A 201, 143–154. doi: 10.1007/s00359-014-0938-7
Grether, G. F., Aller, T. L., Grucky, N. K., Levi, A., Antaky, C. C., and Townsend, V. R. Jr. (2014a). Species differences and geographic variation in the communal roosting behavior of Prionostemma harvestmen in Central American rainforests. J. Arachnol. 42, 257–267. doi: 10.1636/j14-27.1
Grether, G. F., Levi, A., Antaky, C., and Shier, D. M. (2014b). Communal roosting sites are potential ecological traps: experimental evidence in a Neotropical harvestman. Behav. Ecol. Sociobiol. 68, 1629–1638.
Grether, G. G., and Donaldson, Z. R. (2007). Communal roost site selection in a Neotropical harvestman: habitat limitation vs tradition. Ethology 113, 290–300. doi: 10.1111/j.1439-0310.2006.01328.x
Guffey, C. (1999). Costs associated with leg autotomy in the harvestmen Leiobunum nigripes and Leiobunum vittatum (Arachnida: Opiliones). Can. J. Zool. 77, 824–830.
Harvey, B. D., Vanni, K. N., Shier, D. M., and Grether, G. F. (2017). Experimental test of the mechanism underlying sexual segregation at communal roosts of harvestmen (Prionostemma spp.). Ethology 123, 516–525.
Heymann, E. W., and Buchanan-Smith, H. M. (2000). The behavioural ecology of mixed-species troops of callitrichine primates. Biol. Rev. Camb. Philos. Soc. 75, 169–190. doi: 10.1017/s0006323199005460
Hodge, M. A., and Storfer-Isser, A. (1997). Conspecific and heterospecific attraction: a mechanism of web-site selection leading to aggregation formation by web-building spiders. Ethology 103, 815–826. doi: 10.1111/j.1439-0310.1997.tb00123.x
Hodge, M. A., and Uetz, G. W. (1996). Foraging advantages of mixed- species association between solitary and colonial orb-weaving spiders. Oecologia 107, 578–587. doi: 10.1007/BF00333951
Holmberg, R. G., Angerilli, N. P. D., and LaCasse, L. J. (1984). Overwintering aggregations of Leiobunum paessleri in caves and mines (Arachnida, Opiliones). J. Arachnol. 12, 195–204.
Houghton, J. E., Towsend, V. R. Jr., and Proud, D. N. (2011). The ecological significance of leg autotomy for climbing temperate species of harvestmen (Arachnida, Opiliones, Sclerosomatidae). Southeast. Nat. 10, 579–590.
Joos, B., Casey, T. M., Fitzgerald, T. D., and Buttermer, W. A. (1988). Roles of the tent in behavioral thermoregulation of eastern tent caterpillars. Ecology 69, 2004–2011.
Juberthie, C. (1972). Reproduction et développement d’un opilion Cosmetidae, Cynorta cubana (Banks), de Cuba. Ann. Spéléol. 27, 773–785.
Kaplan, I., and Denno, R. F. (2007). Interspecific interactions in phytophagous insects revisited: a quantitative assessment of competition theory. Ecol. Lett. 10, 977–994. doi: 10.1111/j.1461-0248.2007.01093.x
Kappeler, P. M., Cremer, S., and Nunn, C. L. (2015). Sociality and health: impacts of sociality on disease susceptibility and transmission in animal and human societies. Phil. Trans. R. Soc. B 370:20140116. doi: 10.1098/rstb.2014.0116
Kiffner, C., Kioko, J., Leweri, C., and Krause, S. (2014). Seasonal patterns of mixed species groups in large East African mammals. PLoS One 9:e113446. doi: 10.1371/journal.pone.0113446
Knapp, R., and Casey, T. M. (1986). Thermal ecology, behaviour, and growth of gypsy moth and eastern tent caterpillars. Ecology 67, 598–608.
Krasnov, B. R., Stanko, M., Khokhlova, I. S., Mošanský, L., Shenbrot, G. I., Hawlena, H., et al. (2006). Aggregation and species coexistence in fleas parasitic on small mammals. Ecography 29, 159–168. doi: 10.1186/s13071-020-04492-6
Kury, A., Cruz Mendes, A., Cardoso, L., Souza Kury, M., and Granado, A. (2020). WCO-Lite: Online World Catalogue Of Harvestmen (Arachnida, Opiliones): Version 1.0 — Checklist of All Valid Nomina In Opiliones With Authors And Dates Of Publication Up to 2018. Rio de Janeiro: Ed. do Autor.
Laughlin, A. J., Hall, R. J., and Taylor, C. M. (2019). Ecological determinants of pathogen transmission in communally roosting species. Theor. Ecol. 12, 225–235. doi: 10.1007/s12080-019-0423-6
Le Goff, G. (2011). Benefits of Aggregation In Tetranychus Urticae. Ph.D. Dissertation. Ottignies-louvain-la-neuve: University of Louvain-la-Neuve.
Lenth, R. (2019). emmeans: Estimated Marginal Means, Aka Least-Squares Means (R Package Version 1.4.2). Available online at: https://CRAN.R-project.org/package=emmeans (accessed November 2021).
Leung, B. (1998). Aggregated parasite distributions on hosts in a homogeneous environment: examining the Poisson null model. Internat. J. Parasitol. 28, 1709–1712. doi: 10.1016/s0020-7519(98)00128-3
Machado, G. (2002). Maternal care, defensive behavior, and sociality in Neotropical Goniosoma harvestmen (Arachnida, Opiliones). Insect. Soc. 49, 388–393. doi: 10.1007/pl00012663
Machado, G., and Macías-Ordóñez, R. (2007). “Social behavior,” in Harvestmen: The Biology Of Opiliones, eds R. Pinto-da-Rocha, G. Machado, and G. Giribet (Cambridge: Harvard University Press), 400–413.
Machado, G., and Vasconcelos, C. H. F. (1998). Multi-Species aggregations in Neotropical harvestmen (Opiliones, Gonyleptidae). J. Arachnol. 26, 389–391.
Machado, G., Bonato, V., and Oliveira, P. S. (2002). Alarm communication: a new function for the scent-gland secretion in harvestmen (Arachnida: Opiliones). Naturwissenschaften 89, 357–360. doi: 10.1007/s00114-002-0337-8
Machado, G., Carrera, P. C., Pomini, A. M., and Marsaioli, A. J. (2005). Chemical defense in harvestmen (Arachnida, Opiliones): do benzoquinone secretions deter invertebrate and vertebrate predators? J. Chem. Ecol. 31, 2519–2539. doi: 10.1007/s10886-005-7611-0
Machado, G., Raimundo, R. L. G., and Oliveira, P. S. (2000). Daily activity schedule, gregariousness, and defensive behaviour in the Neotropical harvestman Goniosoma longipes (Opiliones: Gonyleptidae). J. Nat. Hist. 34, 587–596. doi: 10.1080/002229300299453
Magrath, R. D., Haff, T. M., Fallow, P. M., and Radford, A. N. (2015). Eavesdropping on heterospecific alarm calls: from mechanisms to consequences. Biol. Rev. 90, 560–586. doi: 10.1111/brv.12122
Martínez, S. V. (1974). Consideraciones ecológicas sobre algunas especies de opiliones (Arachnida) hallados en el Departamento Capital (Santa Fe, Argentina). Comun. Museo Prov. Cien. Nat. Florentino Ameghino (Zool.) 7:11.
Mashberg, D. (2001). “Brood care and social behavior,” in Scorpion Biology and Research, eds P. Brownell and G. A. Polis (New York, NY: Oxford University Press), 257–277.
McAloon, F. M., and Durden, L. A. (2000). Attachment sites and frequency distribution of erythraeid mites, Leptus indianensis (Acari: Prostigmata), ectoparasitic on harvestmen, Leiobunum formosum (Opiliones). Exp. Appl. Acarol. 24, 561–567. doi: 10.1023/a:1026554308826
Mestre, L. A. M., and Pinto-da-Rocha, R. (2004). Population dynamics of an isolated population of the harvestman Ilhaia cuspidata (Opiliones, Gonyleptidae), in Araucaria Forest (Curitiba, Paraná, Brazil). J. Arachnol. 32, 208–220. doi: 10.1636/m02-61
Mitov, P. (2000). Faunistic, Biological And Ecological Investigations On The Opiliones From Vitosha Mt. With Some Zoogeographical Notes. Ph.D. dissertation. Plovdiv: University of Plovdiv Paissi Hilendarski.
Mukherjee, A., Wilske, B., and Jin, C. (2010). First report on mass aggregation of Opiliones in China. J. Threat. Taxa 2, 892–893. doi: 10.11609/jott.o2296.892-3
Newman, H. H. (1917). A case of synchronic behavior in Phalangidae. Science 45:44. doi: 10.1126/science.45.1150.44
Novak, T., Lipovsek, S., Sencic, L., Pabst, M. A., and Janzekovic, F. (2004). Adaptations in phalangiid harvestmen Gyas annulatus and G. titanus to their preferred water current adjacent habitats. Acta Oecol. 26, 45–53. doi: 10.1016/j.actao.2004.03.004
Ohguchi, O. (1981). Prey density and selection against oddity by three-spined sticklebacks. Zeitschr. Tierpsychol. Suppl. 23, 1–79.
Parrish, J. K., and Edelstein-Keshet, L. (1999). Complexity, pattern, and evolutionary trade-offs in animal aggregation. Science 284, 99–101. doi: 10.1126/science.284.5411.99
Pereira, W., Elpino-Campos, A., Del-Claro, K., and Machado, G. (2004). Behavioral repertory of the neotropical harvestman Ilhaia cuspidata (Opiliones, Gonyleptidae). J. Arachnol. 32, 22–30. doi: 10.1636/s02-35
Perón, G. (2017). Multicontinental community phylogenetics of avian mixed-species flocks reveal the role of the stability of associations and of kleptoparasitism. Ecography 40, 1267–1273. doi: 10.1111/ecog.02574
Pinto-da-Rocha, R., Machado, G., and Giribet, G. (2007). Harvestmen: The Biology Of Opiliones. Cambridge, MA: Harvard University Press.
Polak, M. (1996). Ectoparasitic effects on host survival and reproduction: the Drosophila–Macrocheles association. Ecology 77, 1379–1389.
Poulin, R. (2010). Decay of similarity with host phylogenetic distance in parasite faunas. Parasitology 137, 733–741. doi: 10.1017/S0031182009991491
Powell, E. C., Painting, C. J., Hickey, A. J., Machado, G., and Holwell, G. I. (2021a). Diet, predators, and defensive behaviors of New Zealand harvestmen (Opiliones: Neopilionidae). J. Arachnol. 49, 122–140.
Powell, E. C., Willmott, N. J., Selleck, C. J., Painting, C. J., Hickey, A. J., Machado, G., et al. (2021b). No risk to scrambling? Mating tactic does not affect the frequency of leg autotomy in a New Zealand harvestman. Anim. Behav. 177, 99–106. doi: 10.1016/j.anbehav.2021.04.015
Powell, G. V. N. (1985). Sociobiology and adaptive significance of interspecific foraging flocks in the neotropics. Neotrop. Ornithol. 36, 713–732.
Prokopy, R. J., and Roitberg, B. D. (2001). Joining and avoidance behavior in nonsocial insects. Annu. Rev. Entomol. 46, 631–665. doi: 10.1146/annurev.ento.46.1.631
Proud, D. N., Felgenhauer, B. E., Towsend, V. R. Jr., Osula, D. O., Gilmore, I. I. I. W. O., Napier, Z. L., et al. (2012). Diversity and habitat use of Neotropical harvestmen (Arachnida: Opiliones) in a Costa Rican rainforest. ISRN Zool. 2012:549765. doi: 10.5402/2012/549765
Punzo, F. (1997). Leg autotomy and avoidance behavior in response to a predator in the wolf spider, Schizocosa avida (Araneae, Lycosidae). J. Arachnol. 25, 202–205.
Quinn, J. L., Prop, J., Kokorev, Y., and Black, J. M. (2003). Predator protection or similar habitat selection in red-breasted goose nesting associations: extremes along a continuum. Anim. Behav. 65, 297–307. doi: 10.1006/anbe.2003.2063
R Core Team (2021). R: A Language And Environment For Statistical Computing. Vienna: R Foundation for Statistical Computing.
Rasa, O. A. E. (1990). Interspecific defence aggregations: a model for the evolution of sociality and kin selection. Netherlands J. Zool. 40, 711–728.
Raspotnig, G. (2012). Scent gland chemistry and chemosystematics in harvestmen. Biol. Serbica 34, 5–18.
Rayor, L. S., and Taylor, L. A. (2006). Social behavior in amblypygids, and a reassessment of arachnid social patterns. J. Arachnol. 34, 399–421.
Ripley, B., Venables, W., and Ripley, M. B. (2016). Package ‘nnet’ (R Package Version, 7.3–14). Available online at: https://cran.r-project.org/web/packages/nnet/nnet.pdf (accessed November 2021).
Rippi, M., Alatalo, R. V., Lindstrom, L., and Mappes, J. (2001). Multiple benefits of gregariousness cover detectibility costs in aposeamtic aggregations. Nature 413, 512–514. doi: 10.1038/35097061
Saito, Y. (1997). “Sociality and kin selection in Acari,” in The Evolution Of Social Behavior In Insects And Arachnids, eds J. C. Choe and B. J. Crespi (Cambridge: Cambridge University Press), 443–457. doi: 10.1017/cbo9780511721953.022
Santos, F. H. (2007). “Ecophysiology,” in Harvestmen: The Biology Of Opiliones, eds R. Pinto-da-Rocha, G. Machado, and G. Giribet (Cambridge: Harvard University Press), 473–488.
Schmid-Hempel, P. (2005). Evolutionary ecology of insect immune defenses. Annu. Rev. Entomol. 50, 529–551. doi: 10.1146/annurev.ento.50.071803.130420
Scriber, J. M., and Lederhouse, R. C. (1983). Temperature as a factor in the development andfeeding ecology of tiger swallowtail caterpillars, Papilio glaucus (Lepidoptera). Oikos 40, 95–102. doi: 10.2307/3544203
Sridhar, H., Beauchamp, G., and Shanker, K. (2009). Why do birds participate in mixed-species foraging flocks? A large-scale synthesis. Anim. Behav. 78, 337–347. doi: 10.1016/j.anbehav.2009.05.008
Sullivan, K. A. (1984). The advantages of social foraging in downy woodpeckers. Anim. Behav. 32, 16–22. doi: 10.1016/s0003-3472(84)80319-x
Teng, B., Dao, S., Donaldson, Z. R., and Grether, G. F. (2012). New communal roosting tradition established through experimental translocation in a Neotropical harvestman. Anim. Behav. 84, 1183–1190.
Tourinho, A. L., Pinto-Da-Rocha, R., and Bragagnolo, C. (2015). Taxonomic notes on Holcobunus Roewer, 1910, with descriptions of three new species, and new records for Holcobunus nigripalpis Roewer, 1910 (Opiliones: Eupnoi: Sclerosomatidae). Zootaxa 4027, 425–436. doi: 10.11646/zootaxa.4027.3.6
Tourinho-Davis, A. L., and Kury, A. B. (2003). A review of Jussara Mello-Leitão 1935, with a description of six new species from Brazil (Arachnida Opiliones Sclerosomatidae). Trop. Zool. 16, 209–275.
Townsend, V. R., Mulholland, K. A., Bradford, J. O., Proud, D. N., and Parent, K. M. (2006). Seasonal variation in parasitism by Leptus mites (Acari, Erythraeidae) upon the harvestman, Leiobunum formosum (Opiliones, Sclerosomatidae). J. Arachnol. 34, 492–494. doi: 10.1636/t05-44.1
Townsend, V. R., Proud, D. N., Moore, M. K., Tibbetts, J. A., Burns, J. A., Hunter, R. K., et al. (2008). Parasitic and phoretic mites associated with Neotropical harvestmen from Trinidad, West Indies. Ann. Entomol. Soc. Am. 101, 1026–1032.
Townsend, V. R., Rana, N. J., Proud, D. N., Moore, M. K., Rock, P., and Felgenhauer, B. E. (2009). Morphological changes during postembryonic development in two species of neotropical harvestmen (Opiliones, Laniatores, Cranaidae). J. Morphol. 270, 1055–1068. doi: 10.1002/jmor.10742
Treherne, J. E., and Foster, W. A. (1981). Group transmission of predator avoidance-behavior in a marine insect – the Trafalgar Effect. Anim. Behav. 29, 911–917.
Tsunoda, T. (2007). Interspecific and intraspecific associations of two species of hard ticks, Haemaphysalis longicornis and Haemaphysalis megaspinosa, in relation to questing site. J. Parasitol. 93, 531–541. doi: 10.1645/GE-982R.1
Turner, G. F., and Pitcher, T. J. (1986). Attack abatement: a model for group protection by combined avoidance and dilution. Am. Nat. 128, 228–240.
Venables, W. N., and Ripley, B. D. (2002). Modern Applied Statistics With S, 4th Edn. New York, NY: Springer.
Vulinec, K. (1990). “Collective security: aggregation by insects as a defense,” in Insect Defenses, eds D. L. Evans and J. O. Schmidt (Albany, NY: State University of New York Press), 251–288.
Wade, R. R., Loaiza-Phillips, E. M., Townsend, V. R., and Proud, D. N. (2011). Activity patterns of two species of neotropical harvestmen (Arachnida: Opiliones) from Costa Rica. Ann. Entomol. Soc. Am. 104, 1360–1366. doi: 10.1603/an11018
Wagner, H. O. (1954). Massenansammlungen von Weberknechten in Mexiko. Z. Tierpsychol. 11, 349–352. doi: 10.1111/j.1439-0310.1954.tb02163.x
Warburg, M. R. (2000). Intra- and interspecific cohabitation of scorpions in the field and the effect of density, food, and shelter on their interactions. J. Ethol. 18, 59–63. doi: 10.1007/s101640070026
Ward, P., and Zahavi, A. (1973). The importance of certain assemblages of birds as “information-centres” for food-finding. IBIS 115, 517–534.
Whitehouse, M. E. A., and Lubin, Y. (2005). The functions of societies and the evolution of group living: spider societies as a test case. Biol. Rev. 80, 347–361. doi: 10.1017/s1464793104006694
Willemart, R. H., and Gnaspini, P. (2004). Spatial distribution, mobility, gregariousness, and defensive behaviour in a Brazilian cave harvestman Goniosoma albiscriptum (Arachnida, Opiliones, Gonyleptidae). Anim. Biol. 54, 221–236.
Wrona, F. J., and Dixon, R. W. J. (1991). Group size and predation risk: a field analysis of encounter and dilution effects. Am. Nat. 137, 186–201. doi: 10.1086/285153
Yip, E. C., and Rayor, L. S. (2014). Maternal care and subsocial behaviour in spiders. Biol. Rev. 89, 427–449. doi: 10.1111/brv.12060
Keywords: alarm signals, aggregation size, autotomy, chemical defenses, dilution effect, ectoparasitism, group living, roosting site
Citation: Escalante I, Domínguez M, Gómez-Ruiz DA and Machado G (2022) Benefits and Costs of Mixed-Species Aggregations in Harvestmen (Arachnida: Opiliones). Front. Ecol. Evol. 9:766323. doi: 10.3389/fevo.2021.766323
Received: 28 August 2021; Accepted: 14 December 2021;
Published: 11 January 2022.
Edited by:
Leticia Aviles, University of British Columbia, CanadaReviewed by:
Guy Gilles Beauchamp, Concordia University, CanadaPeter Schausberger, University of Vienna, Austria
Copyright © 2022 Escalante, Domínguez, Gómez-Ruiz and Machado. This is an open-access article distributed under the terms of the Creative Commons Attribution License (CC BY). The use, distribution or reproduction in other forums is permitted, provided the original author(s) and the copyright owner(s) are credited and that the original publication in this journal is cited, in accordance with accepted academic practice. No use, distribution or reproduction is permitted which does not comply with these terms.
*Correspondence: Ignacio Escalante, ZXNjYWxhbjNAdXdtLmVkdQ==, orcid.org/0000-0003-1919-4303