- School of Life Sciences, Arizona State University, Tempe, AZ, United States
Kin selection theory has dominated our understanding of the evolution of group living. However, many animal groups form among non-relatives, which gain no indirect fitness benefits from cooperating with nestmates. In this study, we characterized the relatedness and inter-nest migration behavior of the facultatively social carpenter bee, Xylocopa sonorina. Nesting constraints due to costly nest construction in this species give rise to intense intraspecific competition over access to existing nests. We used mark-recapture techniques to characterize patterns of dispersal and nest relocation within a nesting aggregation of spatially clustered nests. Two-thirds of bees relocated at least once during the reproductive season, likely to seek reproductive opportunities in another nest. This fluid nest membership creates opportunities for association among non-relatives. To assess the effects of this dynamic nesting behavior on group relatedness, we used microsatellite analysis to estimate relative relatedness within and between nests in the aggregation. We found that relatedness was variable across sampling years, but that in many cases nestmates were no more related to one another than they were to non-nestmate bees in the population. Together, these results suggest that group composition in X. sonorina may result from strategies to maximize direct fitness. This study supports the hypothesis that factors beyond kinship, such as ecological constraints, are likely to drive group formation in this species.
Introduction
For many animal groups, kin selection theory has served as the central paradigm for understanding the evolution of social behavior (Hamilton, 1964; West-Eberhard, 1975; Trivers and Hare, 1976). Nevertheless, many animals form social groups with non-kin, and gain little to no indirect fitness benefits from cooperation (Bernasconi and Strassmann, 1999; Clutton-Brock, 2009; Riehl, 2013). Particularly within the Hymenoptera, these groups tend to be understudied relative to kin groups, but offer valuable opportunities to test hypotheses about drivers of social evolution while controlling for indirect fitness benefits (Ostwald et al., in review, this issue). However, the extent to which animals form alliances with non-relatives and the mechanisms by which these groups arise remain unknown for many social taxa.
Non-kin groups may arise through shared exploitation of limiting resources, especially nesting sites. These conditions may prompt individuals to disperse and seek reproductive opportunities by joining established groups or constructed nests. Nest joining by non-relatives is common within the cooperatively breeding birds, which may gain direct fitness benefits of cooperation even when relatedness is low (Piper et al., 1995; Young, 1998; Baglione et al., 2002; Riehl, 2011). Likewise, among the communal and polygynous wasps and bees, females may join nests established by non-relatives, where they may benefit from reduced costs of guarding, provisioning, and/or nest construction (Danforth et al., 1996; Johnson, 2004; Wcislo and Tierney, 2009; Mora-Kepfer, 2014; Ostwald et al., in review, this issue).
The large carpenter bees (genus Xylocopa) represent useful candidates for testing hypotheses about social evolution, particularly in the context of non-kin sociality. Carpenter bees may be solitary or may form small, fluid societies in which a single dominant female performs all or most of the egg laying, provisioning, and nest construction/maintenance (Gerling et al., 1989; Richards and Course, 2015; Buchmann and Minckley, 2019). Subordinate females may perform guarding duties but otherwise contribute little to the productivity of the nest (Hogendoorn and Velthuis, 1993; Richards, 2011; Prager, 2014). Instead, subordinates are likely waiting for opportunities to inherit existing nests (Velthuis and Gerling, 1983; Richards, 2011; Schwarz et al., 2011; Vickruck and Richards, 2018), which can be less costly than new-nest construction (Ostwald et al., 2021).
Because nests are costly and valuable resources, most females will breed in existing nests rather than undertaking new nest construction (Peso and Richards, 2011), which is energetically expensive (Ostwald et al., 2021). This limitation creates a shortage of available breeding space that can give rise to intense intraspecific competition for reproductive opportunities (Gerling et al., 1989; Buchmann and Minckley, 2019). Following emergence, adult Xylocopa often (but not always—see Gerling, 1982; Velthuis, 1987) overwinter with siblings in the natal nest in mutually tolerant pre-reproductive assemblages that become aggressive and break up at the onset of the reproductive season (Michener, 1990). These family groups become aggressive at the onset of the reproductive season, prompting dispersal and the formation of dominance hierarchies (Velthuis, 1987; Michener, 1990; Richards and Course, 2015). To secure reproductive opportunities, females may compete for dominance in their natal nests or may attempt to usurp reproductives in nearby nests (Hogendoorn and Leys, 1993; Hogendoorn, 1996; Richards, 2011). Alternatively, females may disperse from their natal nests to join neighboring nests, perhaps seeking to advance their position in a reproductive queue or to minimize competition with close kin (Vickruck and Richards, 2018, 2021).
Nest joining behavior has been observed in several Xylocopa species and is expected to create opportunities for association among non-relatives (Gerling, 1982; Velthuis, 1987; Camilo and Garofalo, 1989; Peso and Richards, 2011). Peso and Richards (2010) used mark-recapture techniques to examine the extent of nest joining in the eastern carpenter bee, Xylocopa virginica, and found that roughly half of recaptured females were found at a different nest from the one at which they were originally marked. The high rate of relocation can explain low within group relatedness in social groups of this species (Vickruck and Richards, 2021). Aside from this study, genetic relatedness of nesting groups is unknown for any other species of carpenter bee, despite ample behavioral observations indicating that carpenter bees tolerate non-relatives in their nests.
We examined nest joining behavior and relatedness in the facultatively social valley carpenter bee, Xylocopa sonorina. Like most carpenter bees, this species is characterized by high reproductive skew and intense nest-site competition (Gerling, 1982). Gerling (1982) observed adult females joining active nests during the reproductive season, and also found that some recently emerged offspring dispersed from their natal nests soon after emergence. We predicted that dispersal and nest relocation may dilute relatedness within nests, leading to mixed associations of kin and non-kin. Using complementary behavioral and genetic approaches, we characterized the dynamic group membership of X. sonorina and the consequences of these behaviors for relatedness within and among nesting groups. In doing so, we aim to highlight mechanisms of group formation that can evolve in the absence of helping behavior and indirect fitness returns.
Materials and Methods
Study Design and X. sonorina Seasonal Activity
To characterize nest relocation and relatedness patterns in X. sonorina, we collected genetic and behavioral data from a single nesting aggregation (an occupied log of Goodding’s willow, Salix goodingii, 206 cm length × 23 cm diameter) sourced from a riparian area in Phoenix, AZ, United States (33.41988 N, −112.07062 W). In central/southern Arizona, winter quiescence for X. sonorina typically ceases in March (Minckley, 1987). Mating activity occurs in March and April (Minckley and Buchmann, 1990), and female reproductive activities, including nest construction/renovation, egg laying, and offspring provisioning, occur primarily in April and May (Minckley, 1987; Ostwald et al., 2020), and offspring emerge in late May to June (Minckley, 1987; Ostwald et al., 2020). This species is univoltine and produces an average of 11.5 brood per nest (Ostwald et al., 2020), laid by a single reproductive female. Nests may be solitary or may contain as many as 9 adults during the spring (Ostwald et al., 2020), though the distribution of group sizes is expected to depend strongly on local factors such as nesting density.
To capture dynamic nest movement behavior across the reproductive season but prior to offspring emergence, we conducted behavioral observations from mid-March to early May of 2021. Likewise, to capture group relatedness at the onset of dispersal and reproductive activity we collected genetic samples in late March to early April of 2019 and 2020.
Behavioral Observations of Dispersal and Nest Joining
We used mark-recapture techniques to examine dispersal and nest joining by female bees over the course of the reproductive season. During spring 2021, we caught and/or observed bees entering and departing nest entrances in our focal log (as in Peso and Richards, 2010; Peso and Richards, 2011). Upon first capture of an individual, we recorded the nest of origin and marked each bee with a unique two-color paint marking on the thorax and abdomen using Testors enamel paint (Testors, Vernon Hills, IL). For all subsequent observations we recorded the identity of the bee and the nest of departure or arrival. These nests may have been natal nests or non-natal nests to which they had dispersed. To estimate total population size, we extrapolated from counts of the number of marked and unmarked female bees entering and exiting the log over the course of 1 h at the end of the spring, after all focal bees had been marked.
We observed nest entry and departure activity for 17 days between March 18 and May 2, 2021. On each sampling day we observed bees for 1–4 h within the daily window of peak flight activity, for a total observation period of 30 h 25 min over the course of the spring. We observed all entries and exits during these observation periods, and recorded the nests visited. Ambient temperatures at the time of observation ranged from approximately 20–34°C.
Genetic Analysis
We collected genetic samples by capturing bees upon departure from their nests. We anesthetized all females on ice then removed the most distal tarsal segment from one metathoracic leg using a sterile razor blade. Removal of this tarsal segment is not known or expected to significantly impair mobility (Vickruck and Richards, 2017). Tarsal samples were stored in ethanol at −20°C for later genetic analysis.
We extracted DNA from all tarsal samples using the DNeasy Blood & Tissue Kit (Qiagen, Valencia, CA, United States). We amplified DNA at 6 microsatellite regions characterized for the congener X. virginica (Vickruck, 2015: XV7, XV9, XV27, XV28, XV30, XV42), having previously confirmed the presence of these loci in X. sonorina using gel electrophoresis of amplified PCR products. Loci were amplified in three 12.5 μl PCR multiplex reactions of two or three primers per multiplex. Forward primers were tagged with a fluorescent probe (6FAM, TET, PET, HEX, or VIC) for fragment identification (Supplementary Table 1). Genotypes were analyzed by fragment analysis and scored by visual inspection of the tracefiles using Geneious R8 (Kearse et al., 2012).
Relatedness Calculation and Statistical Analysis
We estimated relative relatedness of sampled individuals using methods developed by Queller and Goodnight (1989), using the R package related (Pew et al., 2015). Data are presented as pairwise comparisons of the relative relatedness of all possible pairs of individual female bees in the sample. We used Wilcoxon tests to compare relative relatedness of nestmates (within nest comparison) vs. non-nestmates (between nest comparison) within each year of collection. We excluded from analysis any individuals that were missing genotype information at two or more loci (N = 6). In addition, we tested for adherence to Hardy-Weinberg equilibrium at all loci, and estimated FST and FIS between collection years, using the genepop package (Rousset, 2008). All statistical analyses were conducted in R 4.1.9 using the base and stats packages (R Core Team, 2021).
Results
Fluid Group Membership
Over the course of spring 2021, we marked a total of 75 unique female bees at 25 focal nests. We estimate that there were approximately 147 female bees residing in the log at this time based on the estimated ratio of marked to unmarked bees. Further, we estimate that there were approximately 40 active nests over the spring observation period. Of the 75 marked female bees, we observed 47 bees on more than one occasion, with an average of 2.57 ± 0.22 (range: 1–12) observations per individual. The bees that were only observed once may have dispersed to nests other than our 25 focal nests (including nests in other, distant aggregations) or may not have left the nest during our chosen sampling times. Of the 47 bees observed more than once, 16 (34.04%) were observed only at a single nest. The remaining 31 bees (66.96%) were observed at multiple nests: 19 bees (40.43%) were observed at 2 different nests, 7 bees (14.89%) were observed at 3 different nests, 3 were bees (6.38%) observed at 4 different nests, and 2 bees (4.26%) were observed at 5 different nests (Figure 1). No bee was observed re-visiting a nest she had previously occupied.
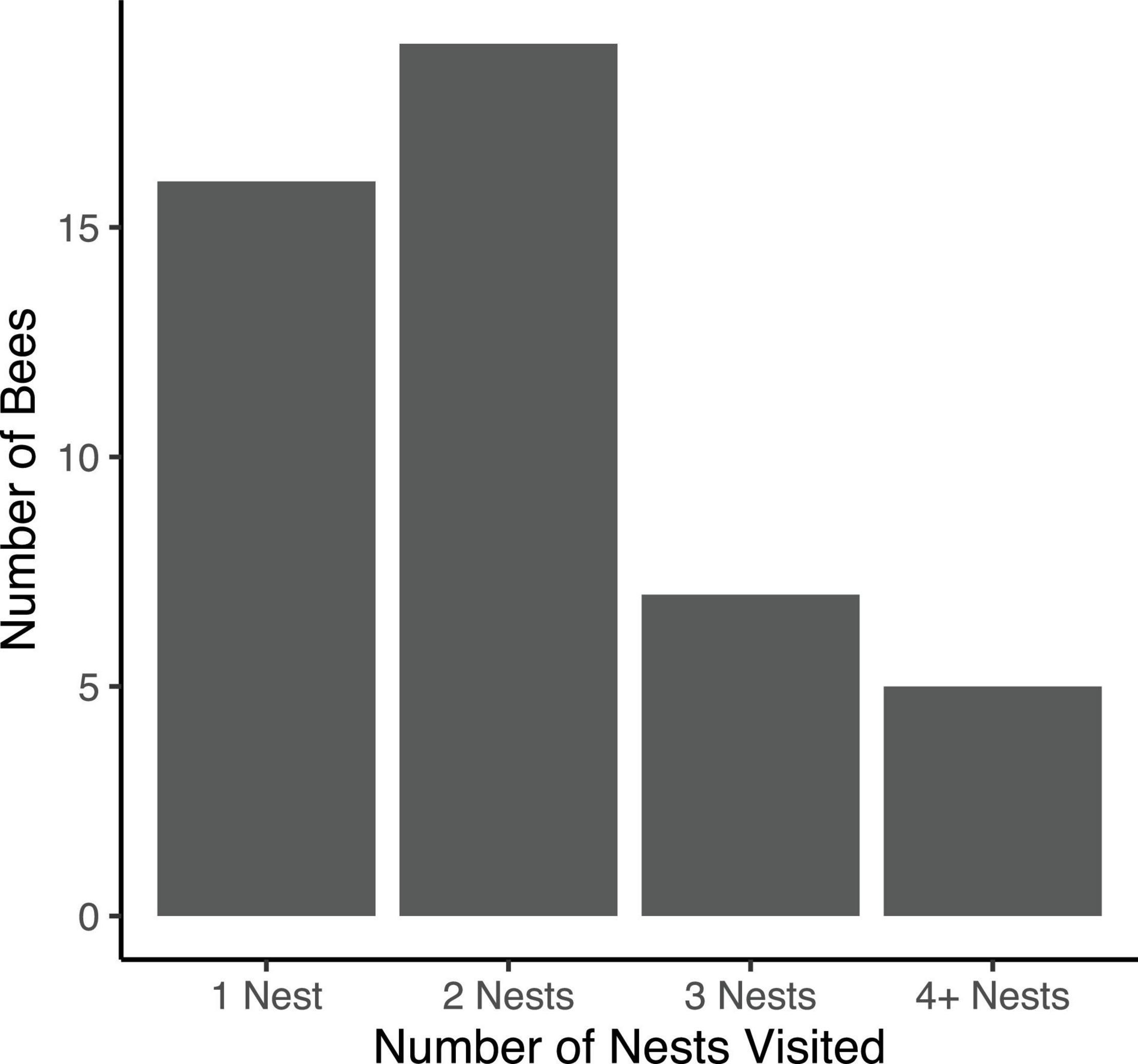
Figure 1. Counts of uniquely identified bees observed at 1, 2, 3, or ≥ 4 nests over the course of the 2021 reproductive season.
Importantly, the number of nests visited by each bee is likely to be greater than what we were able to observe during this limited observation period. Many of the 28 bees we marked but did not recapture may have relocated to non-focal nests. For all bees observed more than once, we observed a significant correlation between number of observations of each bee and the observed number of nests visited (r = 0.49; df = 44, P < 0.001). This correlation suggests that more intensive sampling would reveal even lower nest fidelity.
Relatedness Within and Between Groups
We sampled genetic material from 68 adult females in spring 2019 and 2020. In 2019 we sampled 29 females from 12 nests and in 2020 we sampled 39 females from 18 nests. We sampled between 1 and 7 females per nest (mean = 2.27, S.E. = 0.28). Population genetic analyses showed little genetic differentiation between years (across loci FST = 0.0488; Supplementary Table 2). Hardy-Weinberg equilibrium was verified for all but two loci (XS7 and XS30; Supplementary Table 2). In XS7 FIS analysis showed an extreme overabundance of heterozygotes (FIS = −0.97). We calculated the relative relatedness of all pairwise comparisons of individuals sampled (Queller and Goodnight, 1989). In this metric of relatedness, a value of 0 refers to the average relatedness of all individuals sampled. Positive values refer to above-average relatedness and negative values refer to below-average relatedness. A relative relatedness value of 1 indicates that the two individuals share alleles at all six loci tested. In 2019, the estimated relatedness (r) of nestmates (mean = −0.09, SE = 0.15, median = 0.10) was indistinguishable from the relatedness of non-nestmates (mean = 0.10, S.E. = 0.03, median = −0.023; Wilcoxon test: P = 0.500; Figure 2). In 2020, the relatedness of nestmates (mean = 0.35, S.E. = 0.07, median = 0.336) was significantly higher than the relatedness of non-nestmates (mean = −0.05, S.E. = 0.02, median = −0.01; Wilcoxon test: P < 0.001; Figure 2).
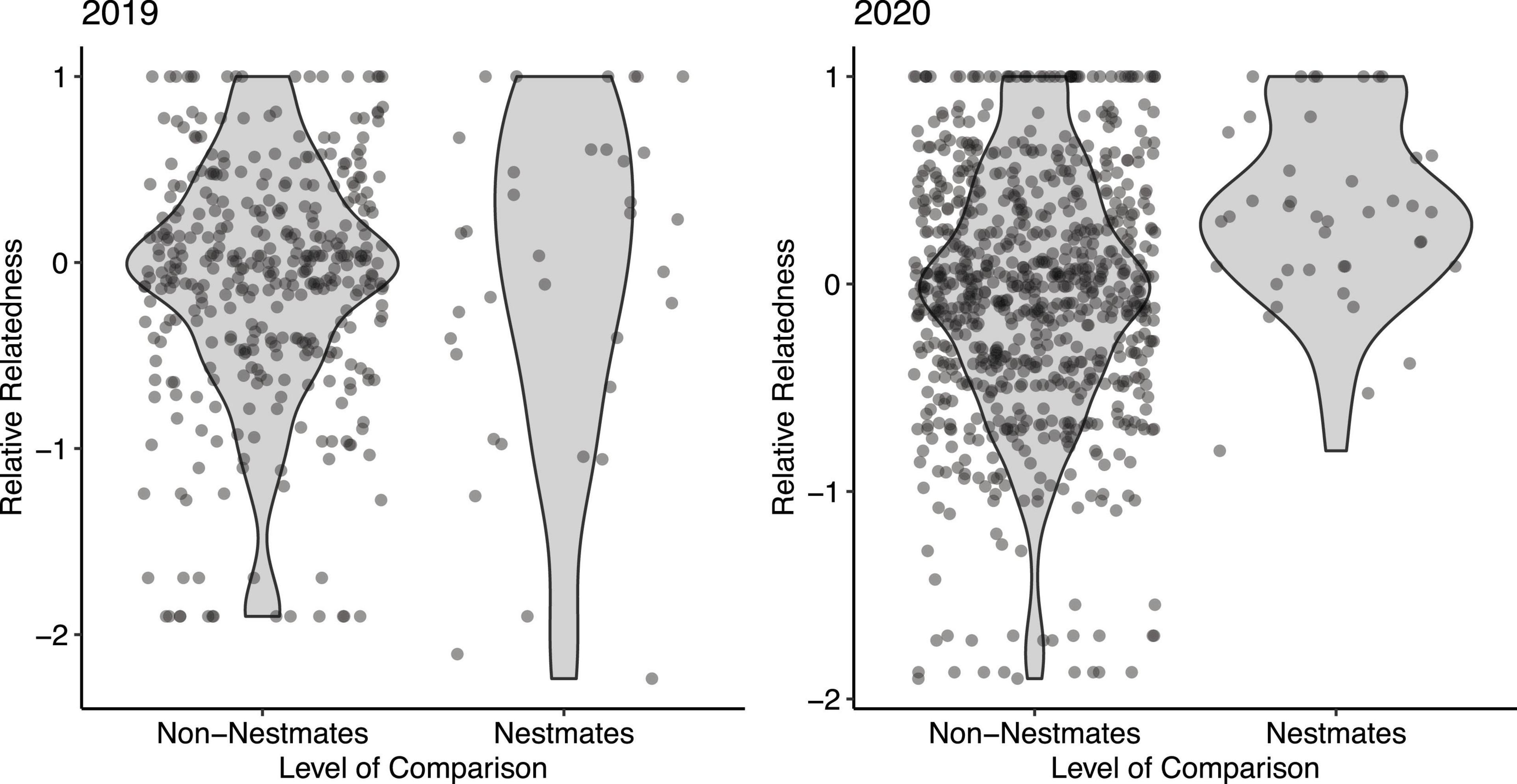
Figure 2. Estimated relative relatedness (r) of nestmate vs. non-nestmate females in a single nesting aggregation. Each point represents a single pairwise comparison between two unique individuals in the sample. In 2019 (left), nestmates were no more related than non-nestmates (Wilcoxon test: P = 0.500). In 2020, nestmates were significantly more related than non-nestmates (Wilcoxon test: P < 0.001). Shaded areas indicate probability density.
Discussion
Choosing whom to live with is one of the most consequential social decisions animals make. Kinship can factor strongly into this decision when individuals receive indirect fitness benefits from helping relatives reproduce. When helping behavior is limited, as for many carpenter bees (Gerling et al., 1989; Richards, 2011; Prager, 2014), incentives for nesting with kin may be likewise minimal. We explored nesting decisions in the valley carpenter bee (X. sonorina), which face severe intraspecific competition over nesting opportunities (Gerling, 1982; Ostwald et al., 2021). We demonstrated that nest joining is common throughout the reproductive season in this species. Joining behavior may prompt associations between non-relatives. Indeed, we present genetic evidence suggesting a mix of kin and non-kin in nesting groups, with many close relatives nesting apart, and many unrelated individuals nesting together. These highly dynamic social groups raise important questions about the costs and benefits of group living in different social contexts.
Inter-nest migration creates opportunities for individuals to associate with non-relatives. Often, these movements reflect adaptive strategies to access limited reproductive opportunities through resource sharing or cooperation, as in many communal birds and insects (Abrams and Eickwort, 1981; Wcislo, 1993; Vehrencamp, 2000; Wcislo and Tierney, 2009; Riehl, 2011). In other cases, relocation may reflect usurpation (Klahn, 1988; Hogendoorn and Leys, 1993). In our study, a majority of bees (67%) relocated from the nests at which they were originally captured (similar to rates observed in other Hymenopteran species; Megachile rotundata, Goerzen et al., 1995; X. virginica, Peso and Richards, 2011; Polistes canadensis, Sumner et al., 2007), and 27% relocated more than once. These moves may be temporary or permanent, reflecting both relocation and perhaps inspection of possible nesting sites. Notably, however, we never observed a bee relocate and then return to her previous nest, suggesting that relocations are often long-term.
The high rates of nest relocation in our study likely represent attempts to seek out reproductive opportunities within saturated nesting space. Bees that relocate may be attempting to usurp dominant reproductives in nearby nests (Hogendoorn and Leys, 1993; Hogendoorn, 1996; Richards, 2011). Alternatively, they may join existing groups as subordinates, but perhaps with a greater chance of nest inheritance than they had in their natal nests (Richards and Course, 2015). Notably, nest relocation was common despite the fact that intruders are usually treated aggressively by resident bees (Velthuis and Gerling, 1983; Hogendoorn and Velthuis, 1995), suggesting that the potential benefits of relocation can outweigh the costs of physical conflict. The fitness outcomes of the relocation strategy compared with remaining in the natal nest remain to be investigated.
Often termed “drifting,” nest relocation behavior should not be conflated with navigational errors. For example, navigational errors are a well-documented apicultural phenomenon in which honey bees enter unfamiliar hives in crowded apiaries (Free, 1958; Pfeiffer and Crailsheim, 1998; Oliveira et al., 2021). Studies of inter-nest movements in the paper wasp Polistes canadensis and the eastern carpenter bee X. virginica found evidence that nest relocation in these species was not merely the result of navigational errors (Sumner et al., 2007; Peso and Richards, 2011). In a study of the navigational abilities of X. sonorina, females made very few navigational errors, even in treatments designed to disrupt homing cues (Ostwald et al., 2019). These observations, coupled with the high incidence (67%) of nest relocation in our study, suggest that nest relocation here represents an active strategy rather than simply a consequence of navigational errors.
Our behavioral data support the results of our genetic analysis, which suggests that nestmates are not always close relatives. In 2019, we found that nestmates were no more related to one another than they were to non-nestmates. Frequent inter-nest migration, as observed in our mark-recapture data, was likely to be the mechanism diluting relatedness in these nestmate groups. In 2020, however, we found nestmates to be significantly more related than non-nestmates, despite sampling at the same time of year across sampling years. This suggests that levels of relatedness vary, across years and likely seasonally. If females overwinter with siblings, then we would expect relatedness to progressively decrease over the reproductive season, as bees disperse and are driven from the nest by dominant bees (Velthuis, 1987; Richards and Course, 2015; Vickruck and Richards, 2021). Also, annual variation in the timing of environmental cues regulating carpenter bee social phenology (Minckley, 1987; Ostwald et al., 2020) could account for observed differences across years if, for example, bees began foraging and dispersing later in 2020 than in 2019.
Alternatively, the extent of dispersal and nest relocation across years may depend on factors such as population density and the degree of intraspecific competition. Further sampling throughout the year and across years would usefully clarify the extent to which relatedness changes over time and how these patterns are shaped by environmental factors. Our observed differences in relatedness may be, in part, an issue of limitations on genetic markers. We examined genetic loci characterized for another species, X. virginica (Vickruck, 2015), which we demonstrated to be present and variable in X. sonorina. However, developing species-specific genetic markers will enable greater resolution of relatedness estimates in future studies.
Why might bees leave their natal nests to join individuals to which they are not closely related? Many non-kin groups benefit from task sharing that improves survival or fitness by reducing the individual labor burden (Bernasconi and Strassmann, 1999; Tibbetts and Reeve, 2003; Cahan and Fewell, 2004; Wilkinson et al., 2016). Carpenter bees, however, do not share the labor costs of reproduction, with only the reproductively active female contributing meaningfully to foraging and nest construction (Richards, 2011; Richards and Course, 2015). As such, additional group members may not improve the productivity of the nest (Prager, 2014; Buchmann and Minckley, 2019). In the absence of helping behavior, the indirect fitness benefits of remaining in the natal nest with relatives are likely to be low or absent. Instead, females may prioritize seeking direct fitness opportunities wherever they may be available, with kin or non-kin.
At the same time, social decisions may not necessarily be made irrespective of kinship. Temporary matrifilial societies may arise from generation overlap between mothers and recently emerged offspring, in which offspring may guard the nest and receive food from their mother (Gerling, 1982; Gerling et al., 1983; Velthuis and Gerling, 1983). Conversely, non-kin nesting may actually represent a strategy to maximize reproductive opportunities among kin. Data from X. virginica even suggest that females may actively avoid nesting with relatives during the reproductive season to reduce kin competition (Vickruck and Richards, 2021). Indeed, our data show many instances of closely related non-nestmates. Further study is needed to determine whether females can benefit from associating with relatives, and if so, what conditions and life history stages favor these associations.
In conclusion, we found evidence for variable relatedness within carpenter bee nesting groups, suggesting that groups can consist of a dynamic mix of kin and non-kin nestmates. This study represents one of only two to quantify genetic relatedness in Xylocopa groups (Vickruck and Richards, 2021). Observations of nest relocation in an additional two Xylocopa species suggests that low relatedness may be common among the social species in the genus (X. pubescens, Gerling et al., 1983; X. sulcatipes, Velthuis, 1987). Nest membership in our study was highly fluid, with most females spending time in multiple nests over the course of the reproductive season. This nest relocation strategy likely reflects attempts to secure reproductive opportunities among strongly limited nest sites. Changes in nest membership demonstrate that relatedness is not a fixed condition, but rather may shift with seasonal and social variables. Instances of low relatedness among our sampled bees suggest limited indirect fitness benefits for nestmates, and instead emphasize the importance of ecological factors, especially nesting constraints, in facilitating sociality in X. sonorina (Ostwald et al., 2020, 2021). Though sociality is often interpreted through the lens of kin selection, systems such as these with low and dynamic relatedness highlight the complexity of social decisions beyond the role of kinship.
Data Availability Statement
The original contributions presented in the study are included in the article/Supplementary Material, further inquiries can be directed to the corresponding author/s.
Author Contributions
MO collected the data and wrote the manuscript. MO and RD analyzed the data. All authors contributed to study design and manuscript editing.
Funding
Publication of this study was supported by an ASU Graduate and Professional Student Association Publication Award to MO and by a Momental Foundation Mistletoe Research Fellowship to MO.
Conflict of Interest
The authors declare that the research was conducted in the absence of any commercial or financial relationships that could be construed as a potential conflict of interest.
Publisher’s Note
All claims expressed in this article are solely those of the authors and do not necessarily represent those of their affiliated organizations, or those of the publisher, the editors and the reviewers. Any product that may be evaluated in this article, or claim that may be made by its manufacturer, is not guaranteed or endorsed by the publisher.
Acknowledgments
We would like to thank Will Hillery and Sean Gutierrez for assistance with field observations and Christian Rabeling for laboratory protocols and equipment. We also thank the DNASU Core Facility and the University of Arizona Genetics core for fragment analysis services. Finally, we thank two reviewers for helpful comments that improved the manuscript.
Supplementary Material
The Supplementary Material for this article can be found online at: https://www.frontiersin.org/articles/10.3389/fevo.2021.767380/full#supplementary-material
Supplementary Table 1 | Amplified microsatellite loci with sequence, dye, and multiplex information. Loci were characterized for X. virginica in Vickruck, 2015.
Supplementary Table 2 | Summarized population genetic information across microsatellite loci, including FST, FIS, X2 test for Hardy-Weinberg Equilibrium with associated DF and P-values.
Supplementary Data Sheet 1 | Queller-Goodnight estimated relatedness values for all comparisons within and between colonies of the focal population presented in the paper, as well as for non-focal populations (“BetweenPop” comparisons).
Supplementary Data Sheet 2 | Mark recapture raw data including date of capture and nest identity.
References
Abrams, J., and Eickwort, G. C. (1981). Nest switching and guarding by the communal sweat bee Agapostemon virescens (Hymenoptera, Halictidae). Insectes Soc. 28, 105–116. doi: 10.1007/BF02223699
Baglione, V., Marcos, M., Canestrari, D., and Ekman, J. (2002). Direct fitness benefits of group living in a complex cooperative society of carrion crows Corvus corone corone. Anim. Behav. 64, 887–893. doi: 10.1006/anbe.2002.2007
Bernasconi, G., and Strassmann, J. E. (1999). Cooperation among unrelated individuals: the ant foundress case. Trends Ecol. Evol. 14, 477–482. doi: 10.1016/S0169-5347(99)01722-X
Buchmann, S. L., and Minckley, R. L. (2019). “Large Carpenter Bees (Xylocopa),” in Encyclopedia of Social Insects, ed. C. Starr (Springer Nature: Cham), 1–4. doi: 10.1007/978-3-319-90306-4_70-1
Cahan, S. H., and Fewell, J. H. (2004). Division of labor and the evolution of task sharing in queen associations of the harvester ant Pogonomyrmex californicus. Behav. Ecol. Sociobiol. 56, 9–17. doi: 10.1007/s00265-003-0746-5
Camilo, E., and Garofalo, C. (1989). Social organization in reactivated nests of three species of Xylocopa (Hymenoptera, Anthophoridae) in Southeastern Brazil. Insect Soc. 36, 92–105. doi: 10.1007/BF02225905
Clutton-Brock, T. (2009). Cooperation between non-kin in animal societies. Nature 461, 51–57. doi: 10.1038/nature08366
Danforth, B. N., Neff, J. L., and Barretto-k, P. (1996). Nestmate relatedness in a communal bee, perdita texana (Hymenoptera: Andrenidae), based on DNA fingerprinting. Evolution 50, 276–284. doi: 10.1111/j.1558-5646.1996.tb04491.x
Free, J. (1958). The drifting of honey-bees. J. Agric. Sci. 51, 294–306. doi: 10.1017/S0021859600035103
Gerling, D. (1982). Nesting biology and flower relationships of Xylocopa sonorina Smith in Hawaii. Pan-Pac. Entomol. 58, 336–351.
Gerling, D., Hurd, P. D., and Hefetz, A. (1983). Comparative behavioral biology of two Middle East species of carpenter bees (Xylocopa Latreille) (Hymenoptera:Apoidea). Smithson. Contrib. Zool. 369, 1–33. doi: 10.5479/si.00810282.369
Gerling, D., Velthuis, H. H. W., and Hefetz, A. (1989). Bionomics of the large carpenter bees of the genus Xylocopa. Annu. Rev.Entomol. 34, 163–190. doi: 10.1146/annurev.en.34.010189.001115
Goerzen, D. W., Richards, K. W., and Rank, G. H. (1995). Drifting in populations of the alfalfa leafcutting bee, Megachile rotundata (Fab.) (Hym., Megachilidae). J. Appl. Ent. 119, 575–579. doi: 10.1111/j.1439-0418.1995.tb01338.x
Hamilton, W. D. (1964). The genetical evolution of social behaviour.I. J. Theor. Biol. 7, 1–16. doi: 10.1016/0022-5193(64)90038-4
Hogendoorn, K. (1996). Socio-economies of brood destruction during supersedure in the carpenter bee Xylocopa pubescens. J. Evol. Biol. 9, 931–952. doi: 10.1046/j.1420-9101.1996.9060931.x
Hogendoorn, K., and Leys, R. (1993). The superseded female’s dilemma: ultimate and proximate factors that influence guarding behaviour of the carpenter bee Xylocopa pubescens. Behav. Ecol. Sociobiol. 33, 371–381. doi: 10.1007/BF00170252
Hogendoorn, K., and Velthuis, H. (1995). The role of young guards in Xylocopa pubescens. Insectes Soc. 42, 427–448. doi: 10.1007/BF01242171
Hogendoorn, K., and Velthuis, H. H. W. (1993). The sociality of Xylocopa pubescens: does a helper really help? Behav. Ecol. Sociobiol. 32, 247–257. doi: 10.1007/BF00166514
Johnson, R. A. (2004). Colony founding by pleometrosis in the semiclaustral seed-harvester ant Pogonomyrmex californicus (Hymenoptera: Formicidae). Anim. Behav. 68, 1189–1200. doi: 10.1016/j.anbehav.2003.11.021
Kearse, M., Moir, R., Wilson, A., Stones-Havas, S., Cheung, M., Sturrock, S., et al. (2012). Geneious Basic: an integrated and extendable desktop software platform for the organization and analysis of sequence data. Bioinformatics 28, 1647–1649. doi: 10.1093/bioinformatics/bts199
Klahn, J. (1988). Intraspecific Comb Usurpation in the Social Wasp Polistes fuscatus. Behav. Ecol. Sociobiol. 23, 1–8. doi: 10.1007/BF00303051
Michener, C. (1990). “Castes in xylocopine Bees,” in Social Insects: An Evolutionary Approach to Castes and Reproduction, ed. W. Engel (Berlin: Springer Verlag), 123–146. doi: 10.1007/978-3-642-74490-7_7
Minckley, R. L. (1987). Aspects of the Reproductive Biology of two Carpenter Bees (genus Xylocopa) in Southern Arizona. Tucson, ARI: University of Arizona.
Minckley, R. L., and Buchmann, S. L. (1990). Territory site selection of male Xylocopa (Neoxylocopa) varipuncta Patton (Hymenoptera: Anthophoridae). J. Kans. Entomol. Soc. 63, 329–339.
Mora-Kepfer, F. (2014). Context-dependent acceptance of non-nestmates in a primitively eusocial insect. Behav. Ecol. Sociobiol. 68, 363–371. doi: 10.1007/s00265-013-1650-2
Oliveira, R. C., Andrés, F., Contrera, L., Arruda, H., Jaffé, R., Costa, L., et al. (2021). Foraging and drifting patterns of the highly eusocial neotropical stingless bee melipona fasciculata assessed by radio-frequency identification tags. Front. Ecol. Evol. 9:708178. doi: 10.3389/fevo.2021.708178
Ostwald, M. M., Fox, T. P., Harrison, J. F., and Fewell, J. H. (2021). Social consequences of energetically costly nest construction in a facultatively social bee. Proc. R. Soc. B 288:20210033. doi: 10.1098/rspb.2021.0033
Ostwald, M. M., Lyman, B. R., Shaffer, Z., and Fewell, J. H. (2020). Temporal and spatial dynamics of carpenter bee sociality revealed by CT imaging. Insectes Soc. 67, 203–212. doi: 10.1007/s00040-020-00761-w
Ostwald, M. M., Shaffer, Z., Pratt, S. C., and Fewell, J. H. (2019). Multimodal cues facilitate nest recognition in carpenter bee aggregations. Anim. Behav. 155, 45–51. doi: 10.1016/j.anbehav.2019.06.023
Peso, M., and Richards, M. H. (2010). Knowing who’s who: nestmate recognition in the facultatively social carpenter bee, Xylocopa virginica. Anim. Behav. 79, 563–570. doi: 10.1016/j.anbehav.2009.11.010
Peso, M., and Richards, M. H. (2011). Not all who wander are lost: nest fidelity in Xylocopa virginica examined by mark recapture. Insectes Soc. 58, 127–133. doi: 10.1007/s00040-010-0125-y
Pew, J., Muir, P. H., Wang, J., and Frasier, T. R. (2015). related: an R package for analysing pairwise relatedness from codominant molecular markers. Mol. Ecol. Resour. 15, 557–561. doi: 10.1111/1755-0998.12323
Pfeiffer, K. J., and Crailsheim, K. (1998). Drifting of honeybees. Insectes Soc. 45, 151–167. doi: 10.1007/s000400050076
Piper, W. H., Parker, P. G., and Rabenold, K. N. (1995). Facultative dispersal by juvenile males in the cooperative stripe-backed wren. Behav. Ecol. 6, 337–342. doi: 10.1093/beheco/6.3.337
Prager, S. M. (2014). Comparison of social and solitary nesting carpenter bees in sympatry reveals no advantage to social nesting. Biol. J. Linn. Soc. 113, 998–1010. doi: 10.1111/bij.12395
Queller, D. C., and Goodnight, K. F. (1989). Estimating relatedness using genetic markers. Evolution 43, 258–275. doi: 10.1111/j.1558-5646.1989.tb04226.x
R Core Team (2021). R: A Language and Environment for Statistical Computing. Vienna: R Foundation for Statistical Computing.
Richards, M. H. (2011). Colony social organisation and alternative social strategies in the eastern carpenter bee, Xylocopa virginica. J. Insect Behav. 24, 399–411. doi: 10.1007/s10905-011-9265-9
Richards, M. H., and Course, C. (2015). Ergonomic skew and reproductive queuing based on social and seasonal variation in foraging activity of eastern carpenter bees (Xylocopa virginica). Can. J. Zool. 93, 615–625. doi: 10.1139/cjz-2014-0330
Riehl, C. (2011). Living with strangers: direct benefits favour non-kin cooperation in a communally nesting bird. Proc. R. Soc. B 278, 1728–1735. doi: 10.1098/rspb.2010.1752
Riehl, C. (2013). Evolutionary routes to non-kin cooperative breeding in birds. Proc. R. Soc. B 280:20132245. doi: 10.1098/rspb.2013.2245
Rousset, F. (2008). genepop’007: a complete re-implementation of the genepop software for Windows and Linux. Mol. ecol. resour. 8, 103–106. doi: 10.1111/j.1471-8286.2007.01931.x
Schwarz, M. P., Tierney, S. M., Rehan, S. M., Chenoweth, L. B., and Cooper, S. J. B. (2011). The evolution of eusociality in allodapine bees: workers began by waiting. Biol. Lett. 7, 277–280. doi: 10.1098/rsbl.2010.0757
Sumner, S., Lucas, E., Barker, J., and Isaac, N. (2007). Radio-tagging technology reveals extreme nest-drifting behavior in a eusocial insect. Curr. Biol. 17, 140–145. doi: 10.1016/j.cub.2006.11.064
Tibbetts, E. A., and Reeve, H. K. (2003). Benefits of foundress associations in the paper wasp Polistes dominulus: increased productivity and survival, but no assurance of fitness returns. Behav. Ecol. 14, 510–514. doi: 10.1093/beheco/arg037
Trivers, R. L., and Hare, H. (1976). Haplodiploidy and the evoluti of the social insects. Science 191, 249–263. doi: 10.1126/science.1108197
Vehrencamp, S. L. (2000). Evolutionary routes to joint-female nesting in birds. Behav. Ecol. 11, 334–344. doi: 10.1093/beheco/11.3.334
Velthuis, H. H. W. (1987). The evolution of sociality: ultimate and proximate factors leading to primitive social behavior in carpenter bees. Exp. Suppl. 54, 405–430.
Velthuis, H. H. W., and Gerling, D. (1983). At the brink of sociality: interactions between adults of the carpenter bee Xylocopa pubescens spinola. Behav. Ecol. Sociobiol. 12, 209–214. doi: 10.1007/BF00290773
Vickruck, J. L. (2015). Development of sixteen novel microsatellite markers for the eastern carpenter bee, Xylocopa virginica (Hymenoptera: Apidae), through paired-end illumina sequencing. Conserv. Genet. Resour. 7, 427–429. doi: 10.1007/s12686-014-0387-1
Vickruck, J. L., and Richards, M. H. (2017). Nestmate discrimination based on familiarity but not relatedness in eastern carpenter bees. Behav. Process. 145, 73–80. doi: 10.1016/j.beproc.2017.10.005
Vickruck, J. L., and Richards, M. H. (2018). Linear dominance hierarchies and conditional reproductive strategies in a facultatively social carpenter bee. Insectes Soc. 64, 619–629. doi: 10.1007/s00040-018-0653-4
Vickruck, J. L., and Richards, M. H. (2021). Competition Drives Group Formation and Reduces Within Nest Relatedness in a Facultatively Social Carpenter Bee. Front. Ecol. Evol. 9:738809. doi: 10.3389/fevo.2021.738809
Wcislo, W. T. (1993). Communal nesting in a North American pearly-banded bee, Nomia tetrazonata, with notes on nesting behavior of Dieunomia heteropoda (Hymenoptera: Halictidae: Nomiinae). Ann. Entomol. Soc. Am. 86, 813–821. doi: 10.1093/aesa/86.6.813
Wcislo, W. T., and Tierney, S. M. (2009). “The evolution of communal behavior in bees and wasps: an alternative to eusociality,” in Organization of Insect Societies: From Genome to Sociocomplexity, eds J. Gadau and J. Fewell (Cambridge, MA: Harvard University Press), 148–169. doi: 10.2307/j.ctv228vr0t.11
West-Eberhard, M. J. (1975). The evolution of social behavior by kin selection. Q. Rev. Biol. 50, 1–33. doi: 10.1086/408298
Wilkinson, G. S., Carter, G. G., Bohn, K. M., Adams, D. M., and Wilkinson, G. S. (2016). Non-kin cooperation in bats. Phil. Trans. R. Soc. B 371:20150095. doi: 10.1098/rstb.2015.0095
Keywords: carpenter bees, Xylocopa, microsatellite, dispersal, social evolution, non-kin, relocation, drifting
Citation: Ostwald MM, Dahan RA, Shaffer Z and Fewell JH (2021) Fluid Nest Membership Drives Variable Relatedness in Groups of a Facultatively Social Bee. Front. Ecol. Evol. 9:767380. doi: 10.3389/fevo.2021.767380
Received: 30 August 2021; Accepted: 17 November 2021;
Published: 07 December 2021.
Edited by:
Miriam H. Richards, Brock University, CanadaReviewed by:
Simon M. Tierney, Western Sydney University, AustraliaAdam R. Smith, George Washington University, United States
Copyright © 2021 Ostwald, Dahan, Shaffer and Fewell. This is an open-access article distributed under the terms of the Creative Commons Attribution License (CC BY). The use, distribution or reproduction in other forums is permitted, provided the original author(s) and the copyright owner(s) are credited and that the original publication in this journal is cited, in accordance with accepted academic practice. No use, distribution or reproduction is permitted which does not comply with these terms.
*Correspondence: Madeleine M. Ostwald, bW9zdHdhbGRAYXN1LmVkdQ==