- 1Canary Islands’ Ornithology and Natural History Group (GOHNIC), Buenavista del Norte, Spain
- 2Terrestrial Ecology Group (TEG-UAM), Department of Ecology, Universidad Autónoma de Madrid, Madrid, Spain
- 3Centro de Investigación en Biodiversidad y Cambio Global (CIBC-UAM), Universidad Autónoma de Madrid, Madrid, Spain
- 4Sociedad Española de Ornitología (SEO/BirdLife), Delegación de Canarias, La Laguna, Spain
- 5Estación Biológica de Doñana, Department of Evolutionary Ecology, Consejo Superior de Investigaciones Científicas, Seville, Spain
Light pollution causes attraction and/or disorientation of seabirds, leading to mortality events due to multiple threats. This is a poorly understood phenomenon, largely because of the challenge to track seabirds at night from their nests to the grounding light-polluted locations. New tracking technologies can inform about this phenomenon. Here, we used GPS transmitters with remote download to track the flights of Cory’s shearwater Calonectris borealis fledglings from an inland experimental releasing site to the ocean. We released birds assigned to three experimental groups: GPS tagged, tape-labelled, and control birds. We assessed how both intrinsic (such as body mass, body condition, body size, and down abundance) and extrinsic (i.e., flight descriptors, such as distance, straightness, and flight duration, wind speed, or moon luminance) factors influenced light-induced groundings by using two datasets: one including the three groups and another including just the GPS tagged birds (as GPS devices provide unique information). We tested whether the probability of being grounded by artificial lights was related to intrinsic factors. With the use of the whole dataset, we found that birds with a higher down abundance had a higher probability of being grounded. GPS data revealed that the probability of being grounded was positively related to the tortuosity of flights and the overflown light pollution levels. Also, birds with slower flights were more likely to be grounded than birds with fast flights. Tortuosity increased with light pollution levels but decreased with the ambient light of the moon. GPSs with remote data download provided information on birds reaching the ocean, this being a substantial improvement to previous studies requiring recapture of the individuals to retrieve the data. GPS tracks of birds reaching the ocean allowed us to know that some birds overflew coastal urban areas so light-polluted as the landing sites of grounded birds. We provide novel scientific-based information to manage seabird mortality induced by artificial lights.
Introduction
Light pollution is an important threat to biodiversity conservation because it can cause cascading effects on ecosystem functioning (Longcore and Rich, 2004; Hölker et al., 2010). Despite the numerous studies and reviews of the field, the underlying factors are far from being understood (Gaston et al., 2014, 2021), especially for secretive species. From a conservation point of view, mass mortality events of organisms are one of the most severe ecological consequences of light pollution, involving a wide range of taxa such as moths, sea turtles, passerine birds, and seabirds (Rich and Longcore, 2006).
Seabirds are one of the most endangered groups of birds (Croxall et al., 2012; Dias et al., 2019). Within seabirds, petrels and shearwaters (Order Procellariiformes; hereafter petrels) are mainly threatened by introduced predators in their nesting grounds and commercial fisheries at sea (Rodríguez et al., 2019). Petrels also suffer from mass mortality episodes caused by artificial night lighting (Rodríguez et al., 2017). For a long time, it has been known that petrel fledglings are attracted and/or disorientated by artificial lights when they are leaving their nests for the first time and fly towards the sea (Imber, 1975). More than 56 burrow-nesting petrel species, some of them critically endangered, are affected by lights (Rodríguez et al., 2017; Silva et al., 2020). Every fledging season, on islands where humans and petrels coexist, thousands of fledglings of different species are grounded by light pollution. This phenomenon called “fallout” exposes grounded birds to injuries or death by collision with human structures or vehicles, as well as predation by introduced or domestic animals (e.g., rats, cats, and dogs), but also plumage soiling, inanition, or dehydration. To mitigate light-induced mortality, rescue campaigns are conducted by local governments and NGOs, releasing into the ocean a high proportion of the admitted birds. Without human intervention (i.e., the rescue actions) it is assumed that most grounded birds would die (Le Corre et al., 2002; Rodríguez and Rodríguez, 2009; Fontaine et al., 2011). Around 10% of birds collected in the campaigns die before release back to the wild, although light-induced mortality could be higher as laypeople do not usually report dead birds, thus 40% is possibly a more accurate estimate (Podolskyi et al., 1998; Ainley et al., 2001; Rodríguez et al., 2014). Why petrels become disorientated by lights is far from being fully understood (Atchoi et al., 2020). The majority of our knowledge about the fallout comes from observational data from rescue campaigns and mainly consists of the reporting of species identification, individual numbers, dates, and locations (Rodríguez et al., 2017).
Analyses of rescue campaign data have uncovered the main factors determining the number of grounded birds. Thus, more fledglings are grounded during moonless, windy, and around peak fledging period nights (Telfer et al., 1987; Rodríguez et al., 2014; Syposz et al., 2018). Rescue campaign data have also described the spatial distribution of the fallout, showing the most dangerous areas. However, the highest numbers of grounded birds have not been reached in the most light-polluted areas according to satellite imagery (Troy et al., 2011, 2013; Rodrigues et al., 2012). It is probably because of the interaction with other influential factors, such as distribution of the breeding colonies and distance to artificial lights. Unfortunately, rescue campaigns cannot identify the colony of origin of birds grounded by artificial lights. Therefore, questions regarding the distance at which birds are attracted to lights and light intensity thresholds leading to fallout events, remains unanswered (Troy et al., 2011; Rodríguez et al., 2015b). In this sense, in Hawaii, it has been suggested that fledglings could be attracted to lights from a long-distance and a substantial number of birds are attracted back to land after reaching the sea (Troy et al., 2013).
Current technological advances in remote tracking systems can help to understand why petrels are attracted to lights. One of our previous studies using GPS data-loggers to track the flights of Cory’s shearwater fledglings from nest-burrows to light-polluted areas on Tenerife revealed that: (a) 50% of the birds were grounded within a 3 km radius from the nest-site; and (b) flight distance was positively related to light pollution levels (Rodríguez et al., 2015b). In this study, we conducted an experiment to assess the percentage of birds grounded by light pollution and to assess intrinsic and extrinsic factors related to the probability of grounding. We assessed intrinsic factors, such as body mass, body size, body condition, and down abundance, and extrinsic factors, such as moon luminance, wind speed, and overflown light pollution levels. We hypothesized that fledglings in a poor body condition have a higher chance of grounding due to lights and that light levels (from artificial light sources or the moon) play an important role in the probability of grounding. We expected that the higher moon illuminance and the lower overflown light pollution levels would result in a lower probability of groundings for shearwater fledglings. Given that shearwater fledgling flights are favoured by wind gusts; wind speed could help fledglings to keep on flight for longer. Consequently, we hypothesized that windy conditions reduce the probability of being grounded. This may seem contradictory to previous observational studies, which reported higher number of grounded birds during windy nights (e.g., Rodríguez et al., 2014; Syposz et al., 2018). However, the increase of groundings could be a consequence of a higher number of fledglings flying around and then being susceptible to artificial lights. To achieve these aims, we employed miniaturised GPS-GSM data loggers on Cory’s Shearwater fledglings from rescue campaigns. This technology enables downloading positional data remotely, with no need for recapturing the individuals. Further, we assessed light pollution levels by using high-resolution nocturnal satellite imagery. As precise knowledge of factors affecting fallout could have important implications for conservation and management, we discuss our findings in relation to previous studies.
Materials and Methods
Study Area and Species
The study was conducted on the south face of Tenerife Island, the largest and the highest of the Canary Islands (2,034 km2 and 3,718 m a.s.l.). The coastline (342 km) is predominantly rocky with boulder shores, and cliffs up to 300 m high. The climate is subtropical and oceanic. Oceanographic conditions are influenced by northeast trade winds and marine upwelling that occur off the northwest African coast (Barton et al., 1998). In 2019, the local human population was around 949,471 inhabitants. In addition, as the island’s economy is heavily dependent on tourism, several millions of visitors are received each year, and they mainly stay in touristic resorts in coastal areas (Martín-Ruiz, 2001; ISTAC, 2020).
Cory’s shearwater (Calonectris borealis) is a medium-sized pelagic seabird (body mass, 600–800 g; wingspan, 112–126 cm), that breeds underground in Azores, Berlengas, Madeira, Selvagems, and the Canary Islands. Its diet is composed of pelagic fishes, cephalopods, and crustaceans, and it spends the non-breeding period in the southern coasts of America and Africa (Reyes-González and González-Solís, 2016). Shearwaters are present for breeding in Canarian waters from February to November. Single-egg clutches are laid in early June, and thus only up to one individual may fledge per nest and year during late October to early November (Martín and Lorenzo, 2001). Adults visit the colonies only at night, and juvenile birds usually leave the nest in the first 3 h after sunset (Rodríguez et al., 2015b). Cory’s shearwater is the most abundant seabird species in the Canary Islands (Martín and Lorenzo, 2001). On Tenerife, its breeding population has been estimated at 8,200–16,600 pairs (Rodríguez et al., 2015b).
Cory’s shearwater fledglings are attracted to artificial lights during their initial flights from their colonies to the Ocean. This attraction occurs in Azores, Madeira, and the Canary Islands causing the death of thousands of individuals every year (Rodríguez and Rodríguez, 2009; Fontaine et al., 2011; Rodrigues et al., 2012), but also in Mediterranean colonies of the related Scopoli’s shearwater (Calonectris diomedea; formerly considered a single species) (Baccetti et al., 2005; Rodríguez et al., 2015a). In Tenerife, there is an island-wide rescue programme implemented by La Tahonilla Wildlife Rehabilitation Centre (thereafter TWRC) and funded by the local Government (Cabildo Insular de Tenerife). According to the TWRC database, the number of fledglings recovered by this programme varied annually according to the moon cycle, but overall it has increased since its inception in 1990 (Rodríguez et al., 2012b). During the last five fledging seasons (2016–2020), the annual average of birds rescued on Tenerife by the programme after being grounded by artificial lights was 2,124 ± 526.1 (mean ± SD; range: 1,441–2,610).
Experimental Design
Tracking shearwater fledglings from their nests to grounding locations in Tenerife Island is a daunting task. First, Cory’s shearwater nests underground, in deep and inaccessible burrows (Rodríguez et al., 2022), located on high vertical cliffs or steep terrain. Therefore, extracting the nestlings for handling and marking is precluded for most nests. Second, being unable to monitor nestling growth, we cannot predict fledging dates with the accuracy needed. Batteries would get flat before birds fledge as energy consumption is higher when the GPSs are underground trying to connect with satellites. Third, sampling nestlings at their natural nests renders a smaller sample size as many devices would not work (see Rodríguez et al., 2015b for a complete discussion on the technical challenges). Given the above shortcomings, our sample size consisted of fledglings presumably affected by light pollution and later found by volunteers during the rescue campaign in 2017, 2018, and 2019. All birds were rescued the night before our handling took place and admitted to the programme conducted by TWRC. A visual inspection assessed the healthy status of birds. Birds underweight (<420 g), lethargic (with no perceptible reactions when handled), bleeding, with injuries, or with other clear evidence of violent collisions (e.g., damaged plumage or broken limbs) were excluded. Therefore, we only selected individuals among apparently healthy birds and thus able to fly.
We released the birds in a natural cave (simulating a natural burrow) situated 8 km inland and at 900 m a.s.l. on the south face of the island. In this part of the island, which concentrates the most largest and densest tourist urban areas of the island, more than 50% of the total number of stranded fledglings are reported annually (Rodríguez and Rodríguez, 2009). Before release, each bird was measured (see below) and randomly allocated to one of the three experimental treatments: GPS-tagged, tape-labelled, and control (see procedure details below). This procedure allowed us to quantify the percentage of birds attracted to artificial lights for each experimental treatment. Local authorities and environmental NGOs made a special effort on the media to involve the general public to rescue as many stranded birds as possible. The public was requested to collect and retain the birds, especially those wearing markings, and then call and inform TWRC. Rescued birds were examined by TWRC staff before their subsequent release into the sea.
Morphological Measurements
All birds were banded with metal rings and handled as described in Rodríguez et al. (2012a). For every fledgling, date, recovery location, body mass, wing length (W), tarsus length (T), skull length (S), and four bill morphometric measurements were recorded. Bill measurements were culmen (C, from the base of forehead feathers in centre of nasal tube to distant part of the curve of the hooked bill), bill length at nostril (BL, from centre of dorsomedial part of tube to distant part of the curve of the hooked bill), bill depth (BD, from the base of forehead feathers to ventral surface of lower mandible), and bill depth at nostril (BDN, from the base of nasal tube at nostrils to ventral surface of bill). The biometrics were taken by the same person (BR) using a spring balance (nearest 5 g), a rule (precise to 1 mm), and an electronic calliper (nearest 0.01 mm). The presence of down in the head and belly was assessed in an ordinal scale (0 = absence, 1 = presence of down, and 2 = entirely covered by down), and the sum of the two values (head and belly) was used as a down index (DI), ranging from 0 (down absence) to 4 (head and belly entirely covered by down).
Treatments
We randomly assigned birds to one of the three treatments: GPS-tagged, tape-labelled, and control birds. Birds of the first treatment (GPS-tagged) were tagged with customised GPS-GSM devices designed and provided by DigitAnimal1 (Móstoles, Spain). Each device was put in a heat-shrink tube for waterproofing, and its final size was 28 × 55 × 18 mm. The device weighed 23 g, which represented less than 4% of the body mass of Cory’s shearwater fledglings (mean ± SD = 3.4% ± 0.3; n = 87). Devices were attached to the mid-dorsal feathers of birds with TESA tape (Puitz et al., 1997). GPSs were programmed to record a position every 30 s from approximately one hour after sunset on the day of deployment. Data were remotely transmitted and downloaded to an internal server in spreadsheet files. To the just tape-labelled birds (second treatment), we attached a 4–5 cm long stripe of TESA tape to the mid-dorsal feathers. Then, we wrote a code number with a black permanent ink pen. This second treatment was intended as a control given that birds without marks on their back could be overlooked by rescue workers and volunteers (see section “Discussion”). Control birds (third treatment) were not GPS nor tape-labelled, but they were handled as long as the other treatment birds, and they were also measured and banded to allow identification.
Data Processing
The positional data recorded by the GPS devices were imported in Qgis (version 3.14.16.; Open Source Geospatial Foundation Project2). We built two databases: one of the complete tracks (n = 43), i.e., those containing all locations from the release point to the ending sites, and another containing environmental information of each GPS position of complete tracks (n = 1,985 locations). For each complete track, we calculated fifteen descriptive variables (Table 1). For each point location, we calculated land altitude and irradiance. We extracted the irradiance values of nighttime lights from nocturnal satellite imagery as a proxy of light pollution. Three cloud-free composite of VIIRS nighttime lights corresponding to November 2017–2019 and produced by the Earth Observation Group, NOAA National Geophysical Data Center3 were used. A Digital Elevation Model (DEM) with a cell size of 25 × 25 m and an accuracy of 1 m of horizontal and vertical resolution, respectively, was obtained from the Digital Atlas of Tenerife Cabildo de Tenerife4 and used to calculate the elevation of every GPS location. Sunset times in minutes were obtained from the NOAA Global Monitoring Laboratory.5 Moon luminance (continuous variable) was measured as the percentage of luminance at full moon at zenith at distance equal to the mean equatorial parallax (Austin et al., 1976). We calculated moon luminance for each 10-min period by using the moonlight Fortran software (Austin et al., 1976). Wind direction and wind speed were taken at 10-min periods from a meteorological station located at 10 km from the releasing site (Las Galletas, Agrocabildo, Cabildo de Tenerife6).
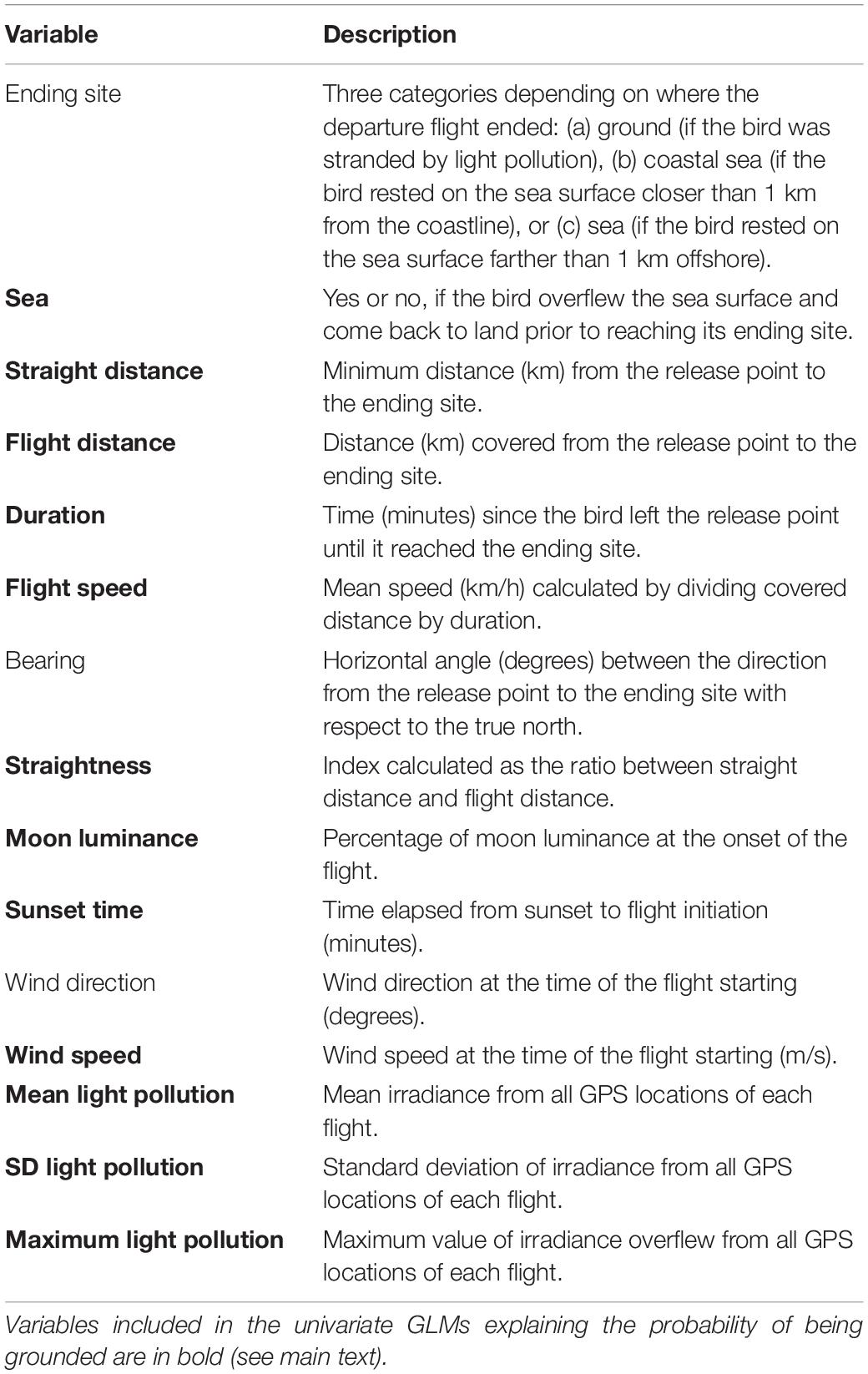
Table 1. Variables used to describe flights of GPS-tagged Cory’s Shearwater (Calonectris borealis) fledglings in Tenerife, Canary Islands, during 2017–2019.
To identify the areas where birds concentrated, we created kernel density estimations for the GPS positions of all tracked birds by using the heatmap algorithm (Interpolation) at the processing toolbox of Qgis. We also created animations of movement trajectories (Schwalb-Willmann et al., 2020; Supplementary Animations 1).
Data Analysis
To estimate bird size and bird body condition, we first ran a principal component analysis (PCA) on the seven centred and scaled morphometric variables (W, T, S, C, BL, BD, and BDN; see above for details). The first principal component was used as a body size index (BSI). The first principal component retained 67.8% of the variation (Supplementary Table 1). The seven morphometric variables showed positive factor loadings (factor loadings: 0.28, 0.35, 0.43, 0.41, 0.41, 0.38, and 0.36 for W, T, S, C, BL, BD, and BDN, respectively) and highly significant correlations to the first principal component (Supplementary Figure 1). Then, we run a linear model of body mass on BSI (the first principal component). This regression showed a R2 = 0.35 and it was statistically significant (F = 150.5, df = 1, 275, P < 0.001). Diagnostic plots indicated that model assumptions were not violated (see Supplementary Figure 2). Finally, we extracted the standardised residuals of this model and used them as a body condition index (BCI), where positive and negative values indicate that birds are heavier and lighter than the average in the population, respectively (Green, 2001; Rodríguez et al., 2012a).
We ran three linear models to test for potential differences in body mass, body size index (BSI), and body condition index (BCI) of birds allocated to the three treatments. We also tested for potential differences in body mass, BSI, and BCI among the study years (2017, 2018, and 2019). No significant differences were observed among treatments or years (see section “Results”). Chi-square tests were used to test for differences in the frequency of groundings among treatments and years.
We ran a generalised linear model (GLM) with binomial error and logit link function to model the probability of being grounded as a function of bird traits (body mass, BSI, BCI, and DI) and experimental treatments (categorical variable: GPS-tagged, tape-labelled, or control). As a response variable, we included the fate of each bird as 1, if the bird was grounded and reported in the rescue programme, or 0, if the bird was not reported by the rescue programme. For the latter case, we assumed the bird reached the ocean successfully. As explanatory variables, we included body mass, BSI, BCI, and DI, and the three-level factor treatment. To avoid multicollinearity, we calculated variance inflation factors (VIFs) for explanatory variables, and those with VIF values higher than 2 were excluded (Zuur et al., 2010). Body mass, which was positively related to BSI and BCI, reached the highest VIF value (>500), and, consequently, it was excluded from the analyses. After that, all VIF values were lower than 1.03.
We also assessed the probability of being grounded by using the dataset of complete tracks. We used GLMs with binomial errors, logit link functions, and the fate of birds (0 = non-reported in rescue programmes; 1 = grounded) as a response variable. Given our sample size (n = 43), we ran univariate GLMs to avoid overparameterization. Explanatory variables in univariate models were bird traits (i.e., body mass, BSI, BCI, and DI) and the variables describing flight traits in Table 1 (excepting the factor ending site, straight distance, and the two circular variables: bearing and wind direction). To assess the variables involved in the flight straightness, we ran univariate linear models, i.e., with an explanatory variable per model. As explanatory variables in univariate models, we used bird traits (i.e., body mass, BSI, BCI, and DI) plus the variables wind speed, time since sunset, moon luminance, and mean, standard deviation, and maximum of light pollution.
We ranked the models according to the AICc value to assess the most explaining variables (the lower the AICc, the better the model). Because variables were taken on different scales, we standardised continuous explanatory variables (mean = 0; SD = 1) prior to modelling. Statistical analyses were conducted in R (version 4.0.0; R Foundation for Statistical Computing, Vienna, Austria). We used the packages ggplot2, MASS, car, circular, and MuMIn.
Results
Recovery Rates and GPS Performance
We handled and released 277 fledglings, which were allocated to the three treatments: 87 GPS-tagged, 92 tape-labelled, and 98 control birds (Table 2). Body mass, body size index (BSI), and body condition index (BCI) did not differ among treatment groups (Body mass: F = 1.248, df = 2, 274, p-value = 0.289; BSI: F = 1.460, df = 2, 274, p-value = 0.234; BCI: F = 0.414, df = 2, 274, p-value = 0.662) nor among years (Body mass: F = 2.26, df = 2, 274, p-value: 0.106; BSI: F = 0.459, df = 2, 274, p-value = 0.632; BCI: F = 2.092, df = 2, 274, p-value = 0.125).

Table 2. Number of Cory’s Shearwater (Calonectris borealis) fledglings experimentally released, and those recovered during rescue campaigns (R) in Tenerife in 2017–2019.
Thirty-seven out of 277 fledglings were later recovered when they got stranded inland after release (Table 2). The percentage of grounded birds was slightly higher for GPS-tagged birds (19.5%) than for tape-labelled and control birds (13% and 8.2%, respectively), but differences were statistically non-significant (Pearson’s Chi-squared test = 5.166, df = 2, p-value = 0.076) (Table 2). However, we observed significant differences in grounding rate among the years, with higher rates in later years as follow: 2019 > 2018 > 2017 (Pearson’s Chi-squared test = 8.084, df = 2, p-value = 0.018). A majority of birds (18; 49%) were rescued within 24 h after release, while thirteen (35%), four (11%), and two (5%) birds were rescued 24–48 h, 3 days, and 5 days after release, respectively. Body mass decreased for all the rescue fledglings and this variation ranged from −103 to −5 g. No obvious differences among treatments were observed in days elapsed and body mass loss from release to rescue (Figure 1).
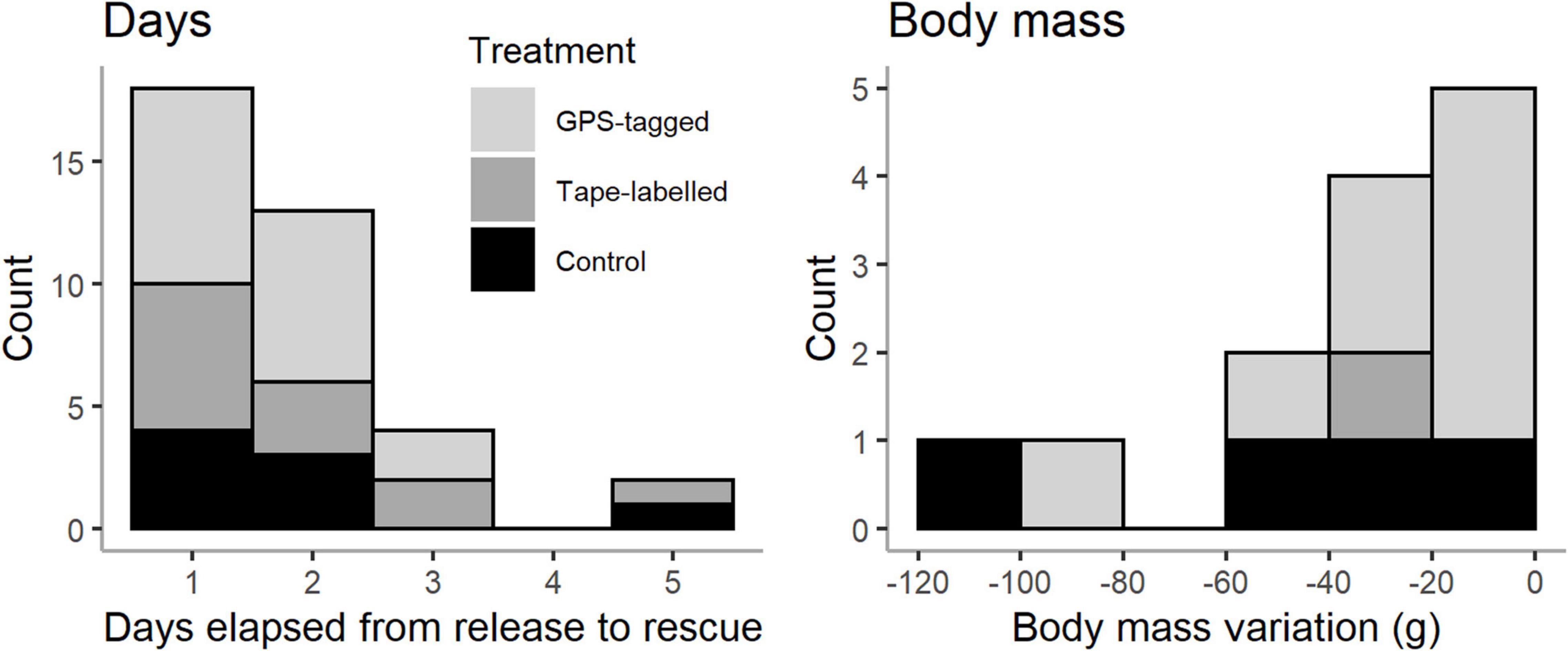
Figure 1. Histograms of the days elapsed from release to rescue and body mass loss between experimental release date and rescue date.
The probability of being grounded for control birds decreased in relation to the two other treatments (estimate ± SE = −1.114 ± 0.470, 95% CI = −2.085, −0.220). No differences in the probability of being grounded by artificial lights were detected between GPS-tagged and tape-labelled birds (estimate ± SE = −0.640 ± 0.427, 95% CI = −1.500, 0.187 using GPS-tagged birds as the reference value). The probability of being grounded was positively related to the down index (estimate ± SE = 0.485 ± 0.189, 95% CI = 0.123, 0.866), but BSI and BCI were not significant (BSI 95% CI = −0.607, 0.134; BCI 95% CI = −0.465, 0.285). The GLM including BSI, BCI, DI, and treatment was significant in relation to the null model (Deviance = 14.924, df = 5, p-value = 0.011; AICc = 215.2; null model AICc = 219.8).
Flight Characteristics
Seventy-one of the 87 deployed GPS devices recorded positions, but we got complete tracks, i.e., with GPS locations from the release site to the grounding location or the ocean, from just 43 birds. Additionally, we got incomplete tracks from nine birds (not included in the statistical analyses). Most incomplete tracks lack the first few meters of the flight, probably due to the difficulties the devices experienced to connect with satellites from inside the releasing cave. Thus, loggers unable to connect with satellites before flight initiation started to log data once the birds were flying.
Bird flights covered distances of 18.7 ± 10.8 km and lasted for 47.3 ± 45.3 min on average (mean ± SD; Table 3). According to the binomial GLMs, the explanatory variables that reached significance to explain the probability of grounding (i.e., 95% confidence intervals did not include the 0) were straightness, those related to light pollution, and flight speed (Table 4). The probability of being grounded decreased with the straightness and the speed of the bird flight, but it increased with light pollution levels (Table 4 and Figure 2). According to linear models, flight straightness was related to environmental light levels. Flights were more tortuous with high light pollution levels, but straightness increased with moon luminance (Table 5 and Figure 3).
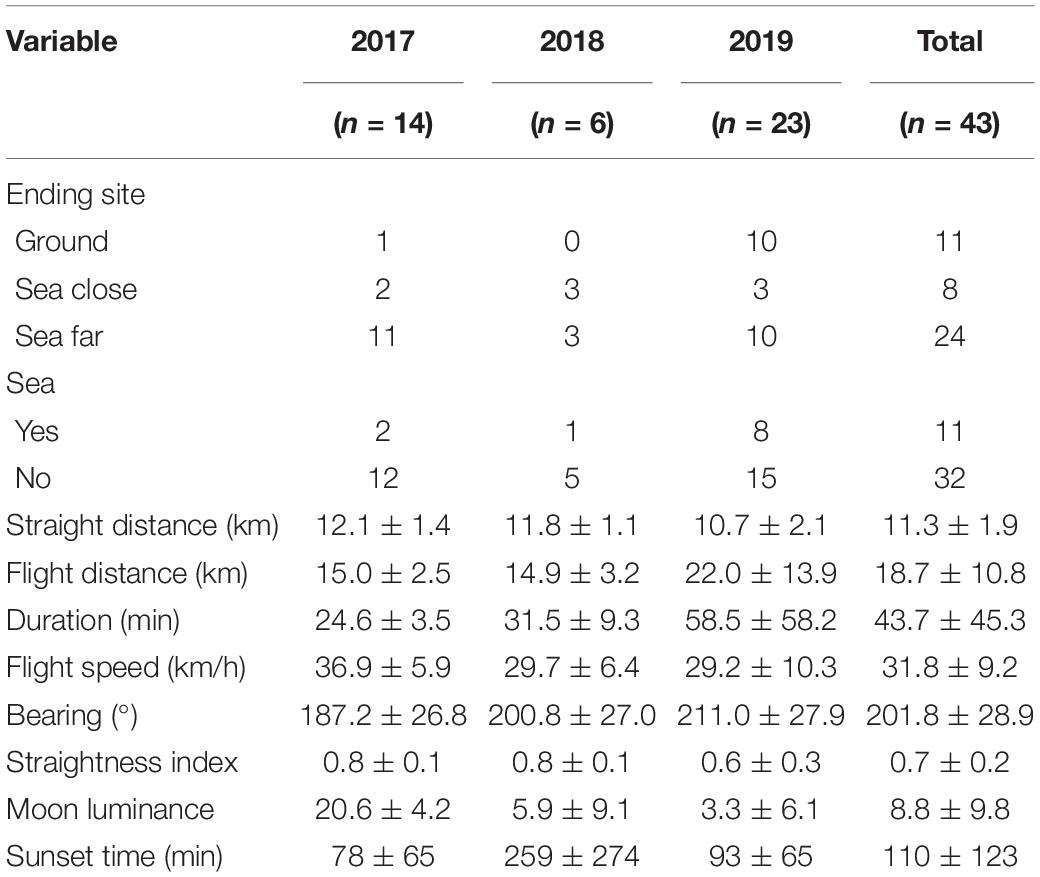
Table 3. Flight descriptors (mean ± SD) of GPS-tagged Cory’s Shearwater (Calonectris borealis) fledglings in Tenerife, Canary Islands, during 2017–2019.
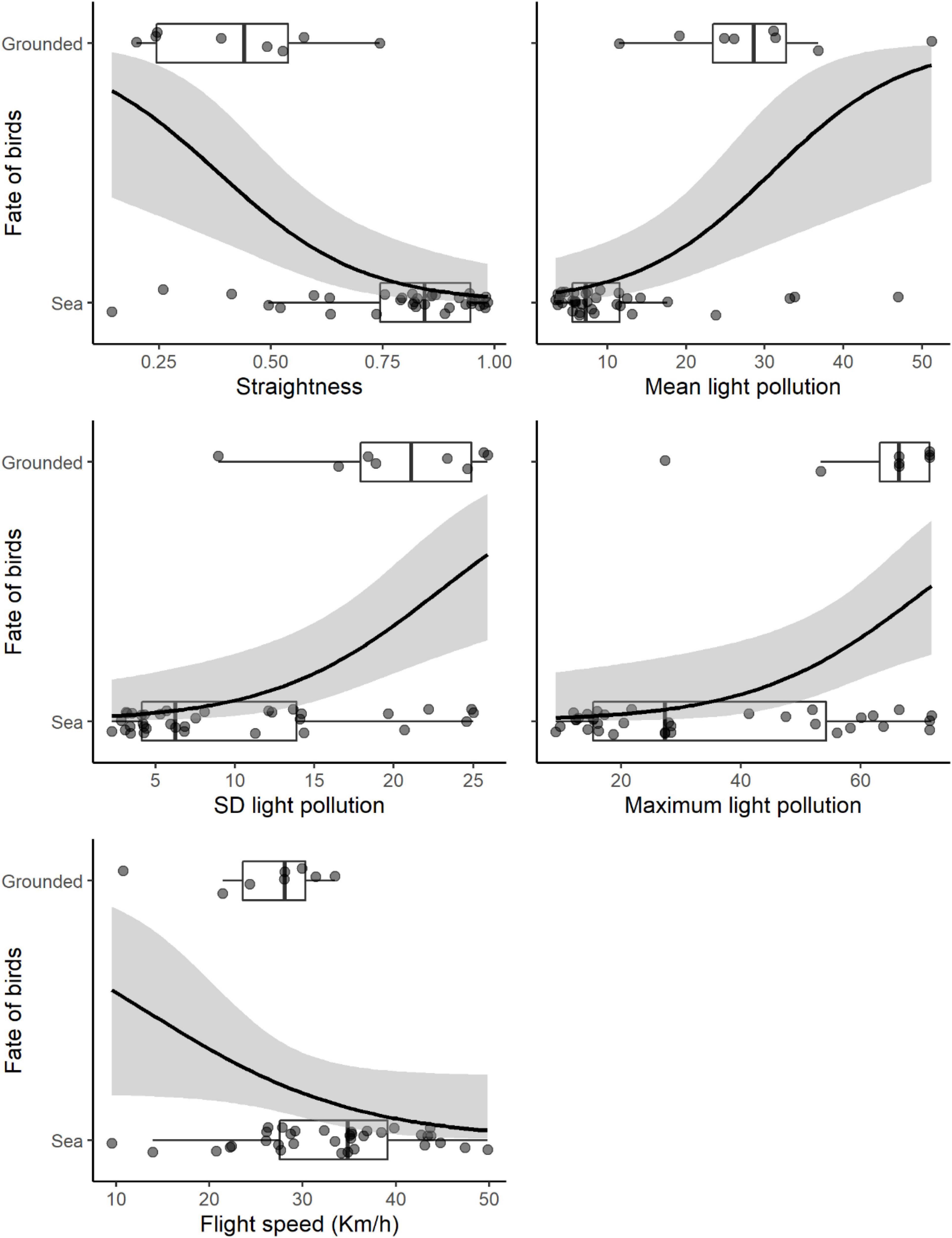
Figure 2. Relation of the fate of birds (grounded birds vs. birds which reached the ocean successfully) with the significant explanatory variables (see Table 4). Black lines and grey areas indicate the predicted effects and the 95% confidence intervals according to the GLMs (Table 4). Dots have been jittered for better visualization. The line within boxes indicated the median, the right and the left edges of the boxes represent the first and third quartiles, and the whiskers extend 1.5 times the interquartile range. Straightness is the ratio between straight distance and flight distance.
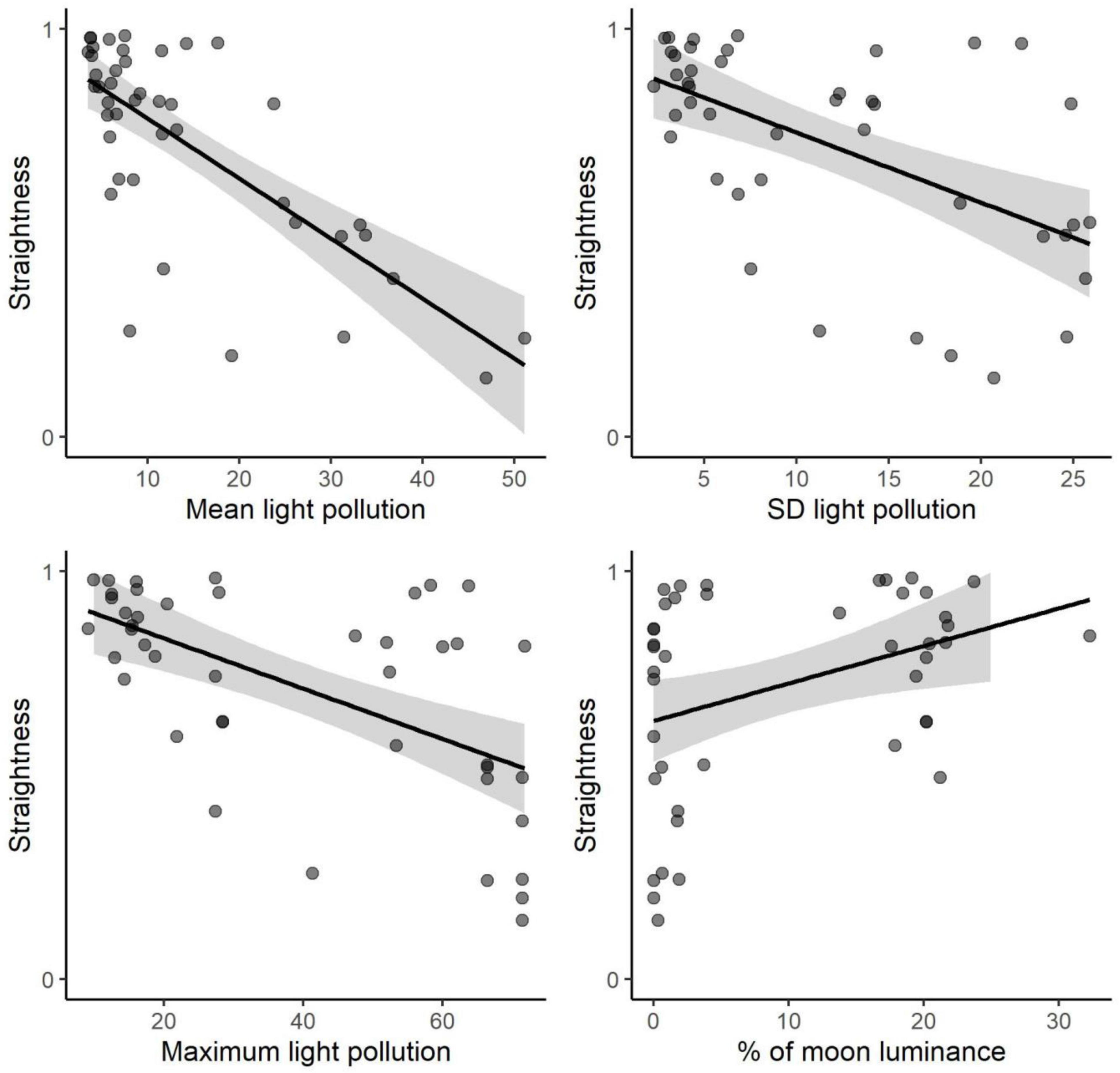
Figure 3. Relationships between flight straightness (calculated as the ratio between straight distance and flight distance) and significantly correlated variables according to the linear models (see Table 5). Black lines and grey areas indicate the predicted effects and the 95% confidence intervals of univariate linear models (Table 5).
Hotspots
All birds left the release site towards low altitude areas following a southern heading, irrespective of wind direction at flight initiation (Figure 4). The directions birds followed corresponded to the most light-polluted areas in the south of our study area (Figure 5). The areas highlighted by the kernel density estimation (heatmap) overlapped with light-polluted and urban areas in coastal sectors (Figure 5 and Supplementary Animations 1).
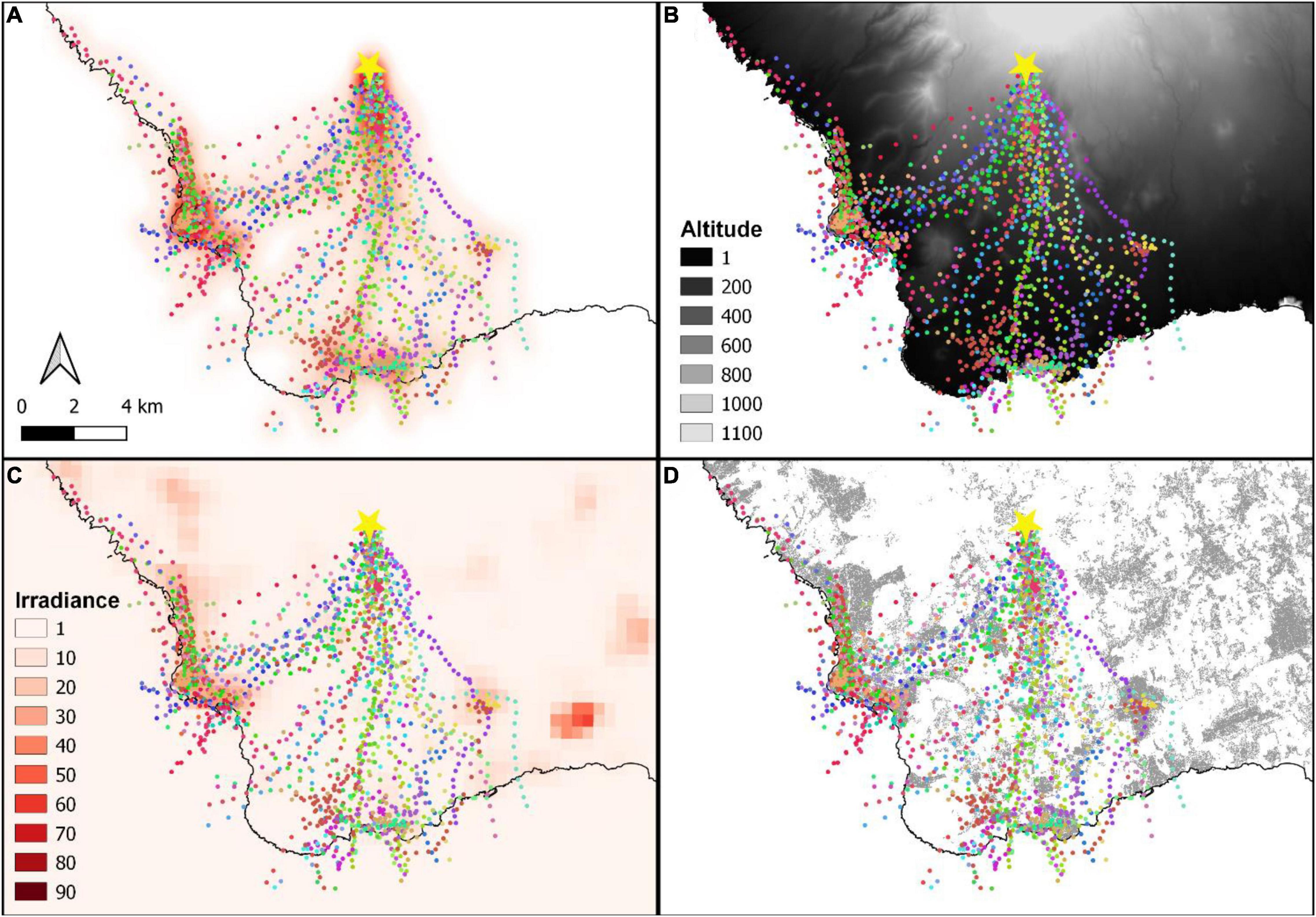
Figure 5. GPS locations of Cory’s shearwater Calonectris borealis fledglings tracked in the south of Tenerife, Canary Islands, during the fledging seasons of 2017–2019. (A) Heat map of all GPS locations. (B) Digital elevation model. (C) Irradiance levels taken as a proxy of light pollution from a monthly composite of November 2019 VIIRS. (D) Urban areas are depicted in grey. Yellow star indicates the release site.
Discussion
Several methodologies, i.e., radar, night-vision scopes, and GPS data-loggers, have been employed to study the attraction of seabirds to artificial lights (Day and Cooper, 1995; Day et al., 2015; Rodríguez et al., 2015b). Radar and night-vision scopes do not allow identifying the individual, so that intrinsic information is not provided. Formerly used GPS data-loggers involved recapturing tagged individuals to retrieve the information. As such, information was only available for grounded individuals (Rodríguez et al., 2015b). Our GPS-GSM devices with remote data download let us obtain information from all fledglings, even those not grounded and reaching the sea. They provide substantially more data. However, our approach used a non-random sample of birds, i.e., they had been previously exposed to light pollution and rescued in the programme. Uncertainty in predicting the fledging date and battery lifespan preclude the use of birds fledging from their natural nests (see a full discussion in Rodríguez et al., 2015b). In addition, the narrow and long entrances of the underground nests, as well as the steep nesting colonies in cliffs, make studying fledglings at their natural nests a challenging, even life-risky, task (Supplementary Figure 3). Despite this shortcoming, our experimental design has shed light on the grounding risk of young seabirds in relation to their exposure to light pollution, as well as their pathways to the sea, two pieces of information critical for the management of artificial lights around seabird colonies. We also identified the most dangerous light-polluted areas in the south of Tenerife, where more than 50% of birds rescued on the whole island are grounded (Rodríguez and Rodríguez, 2009).
All tracked birds oriented their flights towards low altitude, coastal areas, which is consistent with previous research (Rodríguez et al., 2015b; Syposz et al., 2021). The rate of grounding, including all birds, was similar to that reported in a previous tracking study (this study 13.4 vs. 14% in Rodríguez et al., 2015b). Interestingly, according to the GLM, the probability of being grounded differed among treatments. The significant differences detected in the frequencies of grounding for control birds and tape-labelled or GPS-tagged birds, but not between tape-labelled or GPS-tagged birds, suggest that control birds are overlooked during the rescue programme. Small metal rings are harder to spot than artificial marks on the back of the birds (whether labels or GPSs), particularly for shearwaters which hide the legs under their belly when they are on land. In fact, we, the authors, noticed two ringed birds, which had been overlooked by the rescue volunteers, among the birds we handled at the main rescue station (note we just checked and handled a minority of rescued birds). Another non-exclusive potential explanation is that the labels and GPSs could affect the flight performance of birds by disturbing aerodynamic. This is supported by our finding on the positive correlation between down abundance and the probability of being grounded. Fluffy down protruding from feathers may negatively affect the aerodynamics of the birds (Aldheeb et al., 2016). Therefore, the flight performance of younger or less developed fledglings may diminish. In this sense, the probability of releasing a rescued fledgling back to the wild decreases with the abundance of down, but not with body condition (Rodríguez et al., 2012a; Cuesta-García et al., 2022). Dedicated rehabilitation centres that are equipped with facilities to provide food, liquid and shelter in a safe place, could additionally consider allowing birds to stay up until they grow the plumage completely to increase their chances of survival.
Regarding the differences in grounding rates among years, they may be related to the ambiance light level (moon illuminance) according to the moon cycle. It is well known that seabird groundings are reduced during the moon-lit nights probably related to the less conspicuousness of focal artificial lights (Imber, 1975; Telfer et al., 1987; Ainley et al., 2001; Le Corre et al., 2002; Rodríguez and Rodríguez, 2009; Miles et al., 2010). The full moon of November 2017 coincided with the emancipation peak of Cory’s shearwater fledglings on Tenerife (Rodríguez and Rodríguez, 2009). Thus, the recaptured birds were fewer in 2017 in comparison with the two following years (see Table 2), which is in line with the number of rescued birds during the whole campaigns during the three study years (2017 = 1,692; 2018 = 2,610; 2019 = 2,539 birds).
Most birds were rescued during the first 24 h after grounding, but some of them were rescued up to 5 days after their experimental release. All birds lost body mass, as expected given that no food or water was provided. Body mass at fledging is a crucial trait for the future survival and recruitment into the breeding population for seabirds (Becker and Bradley, 2007; Meathrel and Carey, 2007; Maness and Anderson, 2013; Perrins, 2014), and this is also true for our model species (Mougin et al., 2000). Thus, rescuing and delivering the birds to the sea as soon as possible increases survival during the first weeks, and consequently, increases the probability of recruitment into the breeding population (Cuesta-García et al., 2022).
The flight trajectories provided by our GPS devices are particularly useful as they provided basic descriptive statistics for the birds grounded by light pollution, but also for those birds who reached the ocean successfully (Figure 2). Straightness and flight speed are two flight traits related to grounding. Birds with more tortuous and slower flights were more susceptible to grounding. The observed correlation between the chance to be grounded and the above-mentioned traits could be due to the necessity the birds had to land. In other words, landing birds might need to slow down their flight speed to avoid abrupt collisions. Similarly, because they were seemingly forced to do what we may call an “emergency landing” (because they were, for example, blinded by the light, or dazzled, they went exhausted, they perceived the urban matrix as an unsafe place, or whatever the reason made them land), they changed their flight directions multiple times.
Light pollution levels, measured as the mean, the standard deviation, and the maximum irradiance from satellite imagery, increased the probability of being grounded. These findings are in line with our predictions and previous research (Rodríguez et al., 2015b). However, some birds safely reached the ocean having flown over more light-polluted areas than those overflown by some grounded birds (Figure 2 and Supplementary Animations 1). How these birds managed to reach the ocean is still an open question that deserves further research. It would be interesting to know, for instance, if there is a positive selection of birds able to negotiate a lighted nightscape, and whether we should expect less grounded birds in the future. Although certain variables, such as the increasing light pollution levels and the rescue awareness by the general public, could mask a decline, the number of admitted birds into the rescue programme increases annually (Rodríguez et al., 2012b). Therefore, the information available does not seem to support the latter hypothesis.
Conclusion
The attraction and disorientation of seabirds by artificial lights is a poorly understood phenomenon causing mass mortality events of seabirds (Rodríguez et al., 2017). Contrary to other marine animals stranded by light pollution, e.g., sea turtles that leave tracks on beach sand (Hirama et al., 2021), tracking the fledgling flights from their nests to grounding locations is challenging. Our experimental study has revealed that intrinsic factors, such as down abundance in the plumage, are associated with the probability of being grounded by artificial lights. Our GPS devices with remote downloads have provided new insights into this poorly known conservation issue, as they rendered information on flight characteristics for both birds that successfully reached the ocean and those that got stranded inland. However, given that battery life was limited to a few hours, we had to resort to deploying GPS tags and tracking individual birds that had previously been grounded once after leaving their natal nests. Longer-lasting GPS devices that may be deployed on birds that have never experienced flight would be the next technological development needed to provide novel information to better understand the process of seabird fallout.
Data Availability Statement
The datasets generated for this study can be found in the article/Supplementary Material and the Digital.CSIC repository (Rodríguez et al., 2021). Further inquiries can be directed to the corresponding author.
Ethics Statement
The animal study was reviewed and approved by insular and regional governments (Cabildo de Tenerife: AFF82/17, AFF94/18, and AFF142/19; Gobierno de Canarias: 2017/10464 and 2018/6842).
Author Contributions
AR and BR conceived and designed the study, and conducted the fieldwork. AR analysed the data and wrote the first draft of the manuscript. YA and JN contributed materials and tools. YA managed and coordinated the research project. All authors improved the manuscript and approved the submitted version.
Funding
This study was funded by European Commission through the project INTERREG MAC/4.6d/157 LuMinAves.
Conflict of Interest
The authors declare that the research was conducted in the absence of any commercial or financial relationships that could be construed as a potential conflict of interest.
Publisher’s Note
All claims expressed in this article are solely those of the authors and do not necessarily represent those of their affiliated organizations, or those of the publisher, the editors and the reviewers. Any product that may be evaluated in this article, or claim that may be made by its manufacturer, is not guaranteed or endorsed by the publisher.
Acknowledgments
We are grateful to the people who kindly rescue grounded birds, contributing both to save birds and to collect data. We thank the special collaboration and help of Enrique Sacramento, M. Nazaret Carrasco, José Juan Hernández, Acaimo Martínez, María del Carmen Martín, Felipe Rodríguez, and the staff of La Tahonilla Wildlife Rehabilitation Center (Cabildo Insular de Tenerife). Special mention deserves the staff and the volunteers of Asociación para el Desarrollo de Actuaciones en Emergencias (ADAE), who allowed us to use its installations in Arona. The work was coordinated by the Canary Islands regional SEO/BirdLife’s office, then our special thanks to its staff, Juan Antonio Lorenzo and Elena Ramos. We also thank Rubén Blanco of DigitAnimal for customizing the GPS loggers. Permits to conduct fieldwork were granted by insular and regional governments (Cabildo de Tenerife: AFF82/17, AFF94/18, and AFF142/19; Gobierno de Canarias: 2017/10464 and 2018/6842).
Supplementary Material
The Supplementary Material for this article can be found online at: https://www.frontiersin.org/articles/10.3389/fevo.2021.786557/full#supplementary-material
Footnotes
- ^ www.digitanimal.com
- ^ http://qgis.osgeo.org
- ^ https://eogdata.mines.edu/products/vnl/#monthly
- ^ http://atlastenerife.es/portalweb
- ^ https://www.esrl.noaa.gov/gmd/grad/solcalc
- ^ www.agrocabildo.org
References
Ainley, D. G., Podolsky, R., Deforest, L., and Spencer, G. (2001). The status and population trends of the Newell’s Shearwater on Kaua’i: insights from modeling. Stud. Avian Biol. 22, 108–123.
Aldheeb, M. A., Asrar, W., Sulaeman, E., and Omar, A. A. (2016). A review on aerodynamics of non-flapping bird wings. J. Aerosp. Technol. Manag. 8, 7–17.
Atchoi, E., Mitkus, M., and Rodríguez, A. (2020). Is seabird light-induced mortality explained by the visual system development? Conserv. Sci. Pract. 2:e195. doi: 10.1111/csp2.195
Austin, R. H., Phillips, B. F., and Webb, D. J. (1976). A method for calculating moonlight illuminance at the Earth’s surface. J. Appl. Ecol. 13, 741–748.
Baccetti, N., Sposimo, P., and Giannini, F. (2005). Artificial lights and mortality of Cory’s shearwater Calonectris diomedea on a Mediterranean island. Avocetta 29, 89–91.
Barton, E. D., Aristegui, J., Tett, P., Canton, M., García-Braun, J., Hernández-León, S., et al. (1998). The transition zone of the canary current upwelling region. Prog. Oceanogr. 41, 455–504. doi: 10.1016/S0079-6611(98)00023-8
Becker, P. H., and Bradley, S. J. (2007). The role of intrinsic factors for the recruitment process in long-lived birds. J. Ornithol. 148, 377–384. doi: 10.1007/s10336-007-0157-x
Croxall, J. P., Butchart, S. H. M., Lascelles, B., Stattersfield, A. J., Sullivan, B., Symes, A., et al. (2012). Seabird conservation status, threats and priority actions: a global assessment. Bird Conserv. Int. 22, 1–34. doi: 10.1017/S0959270912000020
Cuesta-García, M., Rodríguez, A., Martins, A. M., Neves, V., Magalhães, M., Atchoi, E., et al. (2022). Targeting efforts in rescue programmes mitigating light-induced seabird mortality: first the fat, then the skinny. J. Nat. Conserv. 65:126080. doi: 10.1016/j.jnc.2021.126080
Day, R. H., and Cooper, B. A. (1995). Patterns of movement of dark-rumped petrels and Newell’s shearwaters on Kauai. Condor 97, 1011–1027. doi: 10.2307/1369540
Day, R. H., Rose, J. R., Prichard, A. K., and Streever, B. (2015). Effects of gas flaring on the behavior of night-migrating birds at an artificial oil-production island, Arctic Alaska. Arctic 68, 367–379. doi: 10.14430/arctic4507
Dias, M. P., Martin, R., Pearmain, E. J., Burfield, I. J., Small, C., Phillips, R. A., et al. (2019). Threats to seabirds: a global assessment. Biol. Conserv. 237, 525–537. doi: 10.1016/j.biocon.2019.06.033
Fontaine, R., Gimenez, O., and Bried, J. (2011). The impact of introduced predators, light-induced mortality of fledglings and poaching on the dynamics of the Cory’s shearwater (Calonectris diomedea) population from the Azores, northeastern subtropical Atlantic. Biol. Conserv. 144, 1998–2011. doi: 10.1016/j.biocon.2011.04.022
Gaston, K. J., Ackermann, S., Bennie, J., Cox, D. T. C., Phillips, B. B., Sánchez de Miguel, A., et al. (2021). Pervasiveness of biological impacts of artificial light at night. Integr. Comp. Biol. 61, 1098–1110. doi: 10.1093/icb/icab145
Gaston, K. J., Duffy, J. P., Gaston, S., Bennie, J., and Davies, T. W. (2014). Human alteration of natural light cycles: causes and ecological consequences. Oecologia 176, 917–931. doi: 10.1007/s00442-014-3088-2
Green, A. J. (2001). Mass/length residuals: measures of body condition or generators of spurious results? Ecology 82, 1473–1483.
Hirama, S., Witherington, B., Kneifl, K., Sylvia, A., Wideroff, M., and Carthy, R. (2021). Environmental factors predicting the orientation of sea turtle hatchlings on a naturally lighted beach: a baseline for light-management goals. J. Exp. Mar. Bio. Ecol. 541:151568. doi: 10.1016/J.JEMBE.2021.151568
Hölker, F., Wolter, C., Perkin, E. K., and Tockner, K. (2010). Light pollution as a biodiversity threat. Trends Ecol. Evol. 25, 681–682. doi: 10.1016/j.tree.2010.09.007
Imber, M. J. (1975). Behaviour of petrels in relation to the moon and artificial lights. Notornis 22, 302–306.
ISTAC (2020). ISTAC. Instituto Canario de Estadística. Gobierno de Canarias. Available online at: www.gobiernodecanarias.org/istac/ (accessed September 15, 2020)
Le Corre, M., Ollivier, A., Ribes, S., and Jouventin, P. (2002). Light-induced mortality of petrels: a 4-year study from Réunion Island (Indian Ocean). Biol. Conserv. 105, 93–102. doi: 10.1016/S0006-3207(01)00207-5
Maness, T. J., and Anderson, D. J. (2013). Predictors of juvenile survival in birds. Ornithol. Monogr. 78, 1–55. doi: 10.1525/om.2013.78.1.1.1
Martín, A., and Lorenzo, J. A. (2001). Aves del Archipiélago Canario. La Laguna: Francisco Lemus Editor.
Martín-Ruiz, J.-F. (2001). Geografía de Canarias. Sociedad y Medio Natural. Las Palmas de Gran Canaria: Ediciones Cabildo de Gran Canaria.
Meathrel, C. E., and Carey, M. J. (2007). How important are intrinsic factors to natal recruitment in short-tailed shearwaters Puffinus tenuirostris? J. Ornithol. 148, 385–393. doi: 10.1007/s10336-007-0204-7
Miles, W., Money, S., Luxmoore, R., and Furness, R. W. (2010). Effects of artificial lights and moonlight on petrels at St Kilda. Bird Study 57, 244–251. doi: 10.1080/00063651003605064
Mougin, J.-L., Jouanin, C., Roux, F., and Zino, F. (2000). Fledging weight and juvenile survival of Cory’s shearwaters Calonectris diomedea on selvagem grande. Ringing Migr. 20, 107–110. doi: 10.1080/03078698.2000.9674231
Perrins, C. M. (2014). Factors affecting survival of fledgling manx shearwaters Puffinus puffinus. Seabird 27, 62–71.
Podolskyi, R., Ainley, D. G., Spencer, G., Leah, D., and Nur, N. (1998). Mortality of Newell’s shearwaters caused by collisions with urban structures on Kauai. Colon. Waterbirds 21, 20–34.
Puitz, K., Wilson, R. P., Culik, B., Scolaro, J. A., and Charrassin, J. (1997). Long-term attachment of transmitting and recording devices to penguins and other seabirds. J. Wildl. Manage. 25, 101–106.
Reyes-González, J. M., and González-Solís, J. (2016). “Pardela cenicienta atlántica – Calonectris borealis,” in Enciclopedia Virtual de los Vertebrados Españoles, eds A. Salvador and M. B. Morales (Madrid: Museo Nacional de Ciencias Naturales).
Rich, C., and Longcore, T. (2006). Ecological Consequences of Artificial Night Lighting. Washington, DC: Island Press.
Rodrigues, P., Aubrecht, C., Gil, A., Longcore, T., and Elvidge, C. (2012). Remote sensing to map influence of light pollution on Cory’s shearwater in São Miguel Island, Azores Archipelago. Eur. J. Wildl. Res. 58, 147–155. doi: 10.1007/s10344-011-0555-5
Rodríguez, A., Arcos, J. M., Bretagnolle, V., Dias, M. P., Holmes, N. D., Louzao, M., et al. (2019). Future directions in conservation research on petrels and shearwaters. Front. Mar. Sci. 6:94. doi: 10.3389/fmars.2019.00094
Rodríguez, A., Burgan, G., Dann, P., Jessop, R., Negro, J. J., and Chiaradia, A. (2014). Fatal attraction of short-tailed shearwaters to artificial lights. PLoS One 9:e110114. doi: 10.1371/journal.pone.0110114
Rodríguez, A., Rodríguez, B., and Negro, J. J. (2015b). GPS tracking for mapping seabird mortality induced by light pollution. Sci. Rep. 5:10670. doi: 10.1038/srep10670
Rodríguez, A., García, D., Rodríguez, B., Cardona, E., Parpal, L., and Pons, P. (2015a). Artificial lights and seabirds: is light pollution a threat for the threatened Balearic petrels? J. Ornithol. 156, 893–902. doi: 10.1007/s10336-015-1232-3
Rodríguez, A., Holmes, N. D., Ryan, P. G., Wilson, K.-J., Faulquier, L., Murillo, Y., et al. (2017). Seabird mortality induced by land-based artificial lights. Conserv. Biol. 31, 986–1001. doi: 10.1111/cobi.12900
Rodríguez, A., and Rodríguez, B. (2009). Attraction of petrels to artificial lights in the Canary Islands: effects of the moon phase and age class. Ibis 151, 299–310. doi: 10.1111/j.1474-919X.2009.00925.x
Rodríguez, A., Rodríguez, B., Acosta, Y., and Negro, J. J. (2021). GPS and Intrinsic Data of Cory’s Shearwater Fledglings of Tenerife, Canary Islands [dataset]. Digital.CSIC. doi: 10.20350/digitalCSIC/14132
Rodríguez, A., Rodríguez, B., and Lucas, M. P. (2012b). Trends in numbers of petrels attracted to artificial lights suggest population declines in Tenerife, Canary Islands. Ibis 154, 167–172. doi: 10.1111/j.1474-919X.2011.01175.x
Rodríguez, A., Rodríguez, B., Curbelo, ÁJ., Pérez, A., Marrero, S., and Negro, J. J. (2012a). Factors affecting mortality of shearwaters stranded by light pollution. Anim. Conserv. 15, 519–526. doi: 10.1111/j.1469-1795.2012.00544.x
Rodríguez, B., Siverio, F., Acosta, Y., and Rodríguez, A. (2022). Breeding success of Cory’s shearwater in relation to nest characteristics and predation by alien mammals. Ardeola 69, 1–14. doi: 10.13157/arla.69.1.2022.sc1
Schwalb-Willmann, J., Remelgado, R., Safi, K., and Wegmann, M. (2020). moveVis: animating movement trajectories in synchronicity with static or temporally dynamic environmental data in R. Methods Ecol. Evol. 11, 664–669. doi: 10.1111/2041-210X.13374
Silva, R., Medrano, F., Tejeda, I., Terán, D., Peredo, R., Barros, R., et al. (2020). Evaluación del impacto de la contaminación lumínica sobre las aves marinas en chile: diagnóstico y propuestas. Ornitol. Neotrop. 31, 13–24.
Syposz, M., Gonçalves, F., Carty, M., Hoppitt, W., and Manco, F. (2018). Factors influencing manx shearwater grounding on the west coast of Scotland. Ibis 160, 846–854. doi: 10.1111/ibi.12594
Syposz, M., Padget, O., Wynn, J., Gillies, N., Fayet, A. L., and Guilford, T. (2021). An assay to investigate factors influencing initial orientation in nocturnally fledging seabirds. J. Avian Biol. 52:e02613. doi: 10.1111/JAV.02613
Telfer, T. C., Sincock, J. L., Byrd, G. V., and Reed, J. R. (1987). Attraction of hawaiian seabirds to lights: conservation efforts and effects of moon phase. Wildl. Soc. Bull. 15, 406–413.
Troy, J., Holmes, N., Veech, J., and Green, M. (2013). Using observed seabird fallout records to infer patterns of attraction to artificial light. Endanger. Species Res. 22, 225–234. doi: 10.3354/esr00547
Troy, J. R., Holmes, N. D., and Green, M. C. (2011). Modeling artificial light viewed by fledgling seabirds. Ecosphere 2:art109. doi: 10.1890/ES11-00094.1
Keywords: Canary Islands, coastal environment, conservation, management, mortality, light pollution, phototaxy
Citation: Rodríguez A, Rodríguez B, Acosta Y and Negro JJ (2022) Tracking Flights to Investigate Seabird Mortality Induced by Artificial Lights. Front. Ecol. Evol. 9:786557. doi: 10.3389/fevo.2021.786557
Received: 30 September 2021; Accepted: 16 December 2021;
Published: 18 January 2022.
Edited by:
Davide M. Dominoni, University of Glasgow, United KingdomReviewed by:
Martyna Syposz, University of Gdańsk, PolandJacopo Cecere, Istituto Superiore per la Protezione e la Ricerca Ambientale (ISPRA), Italy
Copyright © 2022 Rodríguez, Rodríguez, Acosta and Negro. This is an open-access article distributed under the terms of the Creative Commons Attribution License (CC BY). The use, distribution or reproduction in other forums is permitted, provided the original author(s) and the copyright owner(s) are credited and that the original publication in this journal is cited, in accordance with accepted academic practice. No use, distribution or reproduction is permitted which does not comply with these terms.
*Correspondence: Airam Rodríguez, YWlyYW1yZ3VlekBnbWFpbC5jb20=