- 1Ivy High School, Taichung, Taiwan
- 2Department of Biology, National Museum of Natural Science, Taichung, Taiwan
- 3Department of Life Sciences, National Chung Hsing University, Taichung, Taiwan
Eye size influences visual acuity, sensitivity, and temporal resolution and is a result of vertebrate adaptation to the environment. The habitats of snake species are diverse, ranging from fossorial, terrestrial, arboreal, to aquatic. They also demonstrate a variety of behavioral and physiological characteristics, such as activity time, feeding patterns, and prey detection. In this study, we comparatively investigated how the relative eye size (i.e., eye diameter vs. head width) associated with the ecological (i.e., habitat), behavioral (i.e., diel activity pattern, foraging strategy), and physiological traits (i.e., the presence of pits), respectively, across six snake families from Taiwan. Among the traits we examined, we found that terrestrial and/or diurnal snakes tended to exhibit the larger relative eye size, indicating the evolutionary responses of eye size to changes in habitat types and activity patterns, respectively, while no evidence of how foraging strategies and the presence of pits affected snake eye size was found. Our findings not only shed light on the adaptive significance of the visual system in diversifying the behaviors and the environments exploited in snakes, but also underline the interactive effects of multidimensional evolutionary attributes (e.g., behavior, ecology, physiology and phylogeny) on the evolution of optimal visual performance.
Introduction
Vision is a crucial sensory system connecting organisms to their environments. Eye size, which positively associates with the amount of photoreceptors in eyes (Land and Nilsson, 2002), determines visual acuity, sensitivity, and temporal resolution (Roaf, 1943; Land and Nilsson, 2002). Such a morphological trait can further account for the functional mechanisms of how species adapt to the environmental lights given their physical limitations. For example, organisms with the larger eyes may possess better foraging efficiency due to the improved visual resolution and/or the greater light sensitivity (Land and Nilsson, 2002; Thomas et al., 2006; Ausprey et al., 2021); yet, they may be imposed by higher energy expenditure simultaneously [e.g., the increased body weight (Laughlin, 1995), the development and maintenance of a large number of nerve cells (Laughlin et al., 1998), and/or susceptibility of eye (Harper, 1988)]. Thus, eye size may represent an ideal indicator of the visual requirements underlying the evolutionary tradeoffs between visual functions and energy expenditure.
In many vertebrates, the absolute eye size often varies allometrically with body size-associated traits [e.g., body length (Burton, 2008), body weight (Howland et al., 2004), head size (Kirk, 2006)], while the relative eye size tightly corresponds to ecological and behavioral attributes of species, such as habitat types (Liu et al., 2012; Thomas et al., 2020), diel activity patterns (Liu et al., 2012; Schmitz and Higham, 2018), and foraging strategies (Thomas et al., 2006; Lisney and Collin, 2007). Currently, the evolution of eye size has been studied thoroughly in many taxa [e.g., fishes (Caves et al., 2017; Vinterstare et al., 2020), frogs (Shrimpton et al., 2021), geckos (Werner and Seifan, 2006), birds (Thomas et al., 2006; Burton, 2008) and mammals (Kirk, 2006; Heard-Booth and Kirk, 2012; Nummela et al., 2013)]. However, it is relatively underexplored how the eye size across different snake species is shaped by the environment, facilitating their demands for behavioral activities and foraging strategies (Katti et al., 2019). For example, it is unclear whether the size of eyes is different in the snakes adopting active and sedentary foraging strategies. Also, do snakes modify the investment in eye size, given the high physiological costs incurred, if they are able to sense environmental cues using the additional sensory system (e.g., pit for infrared perception)?
Snakes have shown the remarkable ability to adapt to diverse environments (Greene, 1997). They heavily rely on visual cues when evaluating the environment (Danaisawadi et al., 2016; Schraft and Clark, 2019) and have been suggested to possess dichromatic vision (Bittencourt et al., 2019). Many of them possess rod and cone opsins (Simões et al., 2015, 2016) and thus are able to perceive different light intensities and colors (Bittencourt et al., 2019). In Colubridae, nocturnal species had larger eyes than diurnal species and species that inhabit arboreal habitats tend to have larger eyes compared with terrestrial or semiaquatic species (Liu et al., 2012). This suggested that the intensity and the propagation of lights provided by different environments can drive the evolution of snake eyes. In the watersnakes that heavily live on frogs, it has been found that the eye size was positively associated with the proportion of frogs in the diet, suggesting that larger eyes benefited the foraging ability of the snakes (Camp et al., 2020). Given the importance of vision in the foraging behavior of snakes, understanding the evolution of snake eyes can thus provide us deeper insights into the diversification of eye morphology and its role in regulating predator-prey interactions across different environmental gradients.
In this study, we examined how the habitat types, behavioral patterns (i.e., foraging strategies, diel activity patterns), and physiological conditions (i.e., the presence of pits) associate with the eye size across multiple snake families, respectively. Based on the potential visual requirements in the given environmental conditions and/or behavioral strategies, we predicted that (1) terrestrial and arboreal snakes should have larger eyes than aquatic snakes; (2) snakes that actively hunt prey have larger eyes than those that ambush; (3) diurnal snakes have larger eyes than nocturnal snakes; and (4) snakes with pits tend to have smaller eyes.
Materials and Methods
We examined the specimens of 33 snake species deposited in the National Museum of Natural Sciences (NMNS) in Taiwan. All of the species inhabit mainland Taiwan and the surrounding islands. The systematic classification included the earliest differentiation of the blind snake family (one species; Typhlopidae) and the Xenodermidae (one species), the Viperidae (five species), the Homalopsidae (two species), the Pseudaspididae (one species), the Elapidae (six species), and the latest differentiation of the Colubridae (17 species). The sample size of each species ranged from 3 to 207 individuals, for a total of 1,176 individuals.
We identified the habitat type, the diel activity pattern, the foraging strategy, and the presence of pits of each snake species based on Tu (2004) (Table 1). We measured the diameter of the left eye and head width of the specimens using a vernier scale (accurate to 0.1 mm; electronic vernier calipers; code number 500–138; model: CD-8″ BS, [Mitutoyo (Mitutoyo) Corporation, Japan]. We defined the eye diameter as the maximal diameter of the visible spectacle as the horizontal width of the inner edge of the eye contact from the nearest scale of the eye, and the head width as the widest maximal distance between the cheeks when looking down on the head (Supplementary Figure 1). Since the eyes of blind snakes are vestigial (Greene, 1997), we excluded the measurement of blind snakes from the subsequent analyses.
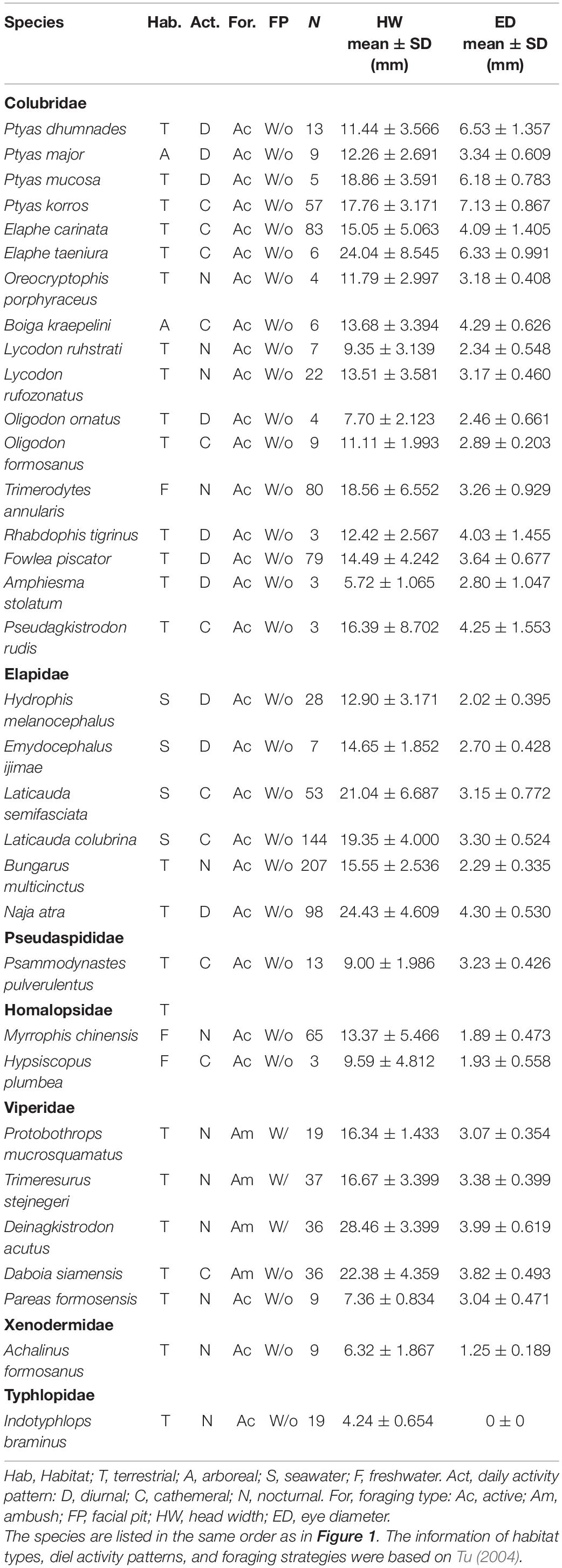
Table 1. Summary of the behavioral characteristics, head width and eye diameter of the 33 snake species from Taiwan used in this study.
We estimated the effect of the specific ecological/behavioral/physiological factors on the relative eye size (i.e., regressed by the head size) among the six snake families using Bayesian general linear mixed-effect models. The models allowed us to take accounts of the phylogeny and repeated measurements simultaneously. The model was derived as below:
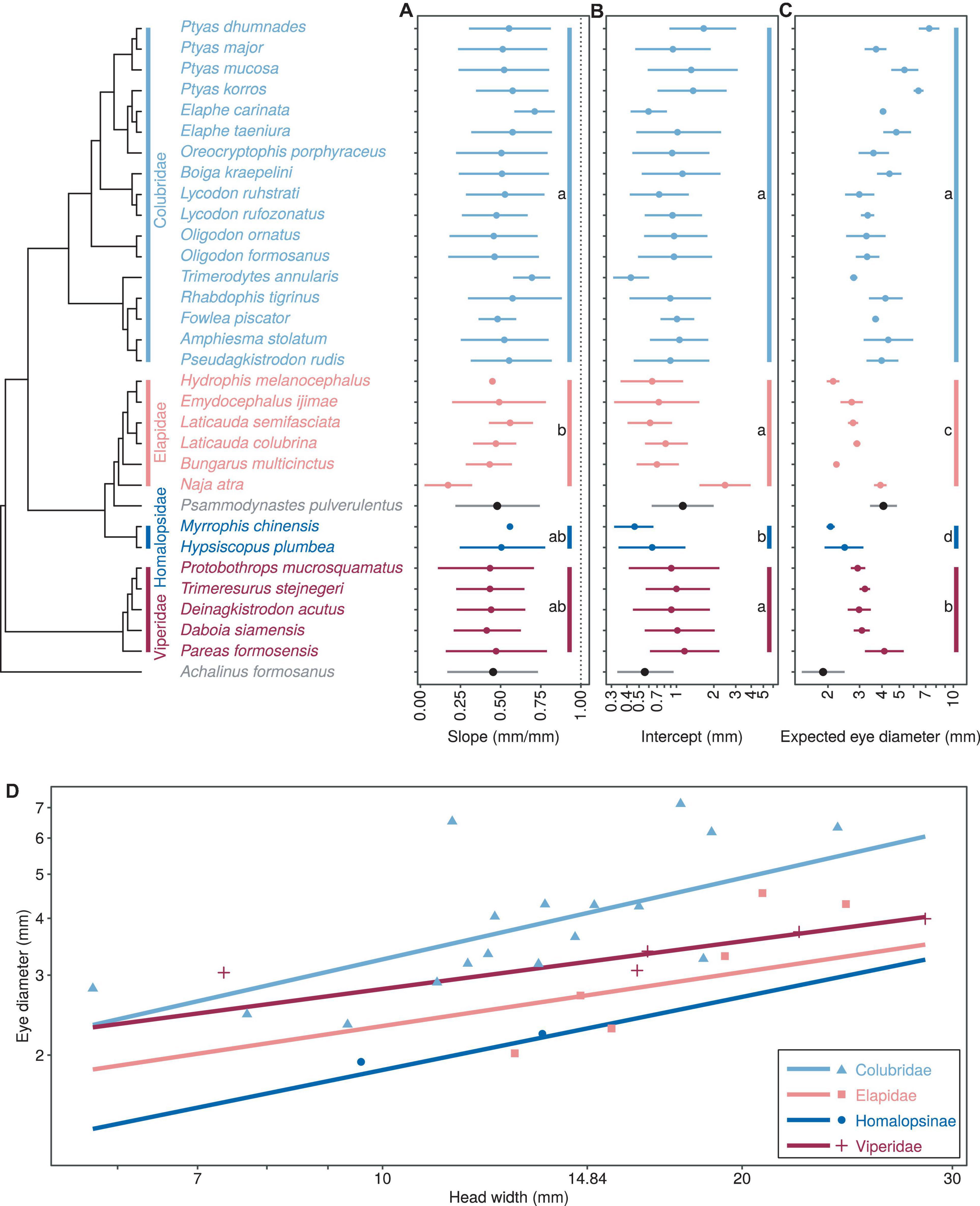
Figure 1. Comparison of growth patterns of eye diameter and head width for different taxa, including allometric growth index (A), proportionality coefficient (B), expected eye diameter (C) and scatter diagram (D). Different color dots and lines are used to represent the four major taxa. In subplots (A–C), the dots and horizontal lines represent the median and 95% HDI of the posterior distribution, respectively, and lowercase letters indicate multiple comparisons between groups (no identical letters indicate significant differences). In (D), the points indicate the mean head width and eye diameter within each species.
with the following priors:
where Y and X denote eye diameter and head width; i and j denote species and different samples within species, respectively, α and β denoted the overall intercept and slope, respectively, εij denoted the random errors; I and L denoted the random intercept and slope of ith species. The fixed effect, γp, including (1) snake family (i.e., Colubridae, Elapidae, Homalopsidae and Viperidae), (2) habitat types (i.e., seawater, freshwater, terrestrial and arboreal), (3) diel activity patterns (i.e., diurnal, cathemeral and nocturnal), (4) foraging strategies (i.e., active and ambush), or (5) the presence of pits (present and absent), were examined, respectively, in the models. We also included the interaction term between the fixed factor examined and the head width, cpln(Xij), where cp denoted the interaction effect.
Including the two parameters, I1 and L1, allowed us to take account of the intraspecific variation caused by repeatedly measuring multiple snake individuals within the same species. The other two parameters, I2 and L2, allowed us to adjust the proportionality coefficients and allometric growth indices of each species, eliminating the problem of false duplication of relatives. The covariance matrix adopted in this study was based on the most approximate affinity tree published in Figueroa et al. (2016) (see Supplementary Figure 2; Hadfield and Nakagawa, 2010). Given that four species from our study were not included in Figueroa et al. (2016), we substituted them by the most phylogenetically closest species (Supplementary Figure 2). We yielded the final results using the averaged models, where we were able to integrate the information of the posterior probabilities produced by the models including and excluding the interactive effects [i.e., cpln(Xij)].
To better illustrate the effect of each of the fixed factors on the eye size of snakes, we introduced the term, expected eye diameter, to allow us to directly compare the eye size. The magnitude of expected eye diameter was extracted, based on the linear regression of the allometry of eye size vs. head width, at the point where the head width equaled 14.84 mm (i.e., the mean head width among all the snake species). We excluded the blind snake, Indotyphlops braminus, from all of the analyses (except for morphological description) because the eye size was very close to zero. We also excluded the other two species, Achalinus formosanus and Psammodynastes pulverulentus, from the analysis of the history of eye size evolution due to the small species number within the respective family.
The models were estimated using the Bayesian Markov chain Monte Carlo method with the R-based brms suite (Bürkner, 2018). Each linear model was tuned with 10,000 warm-up Markov chains. Thereafter, we ran 10,000 Markov chains and used the results as the posterior probability distribution of each parameter. To conduct the significance tests, we compared the posterior distribution of each parameter among the categorical levels of the targeted fixed factor, and calculated the median (hereafter, M), 95% highest density interval (HDI), and two-tailed PMCMC. The significant statistical difference between the two categories was recognized if the corresponding PMCMC < 0.05, and vice versa.
Results
Phylogeny
There was a significant negative allometric growth of eye diameter vs. head width in snakes (Figures 1A,D), since the overall median (M = 0.469) and 95% HDI [i.e., (0.361, 0.562)] were significantly smaller than 1 (PMCMC < 0.001). The allometric growth (i.e., the slope) was the maximum in the Colubridae and the weakest in the Elapidae (Table 2A). Multiple comparisons indicated that the scaling coefficients were significantly higher in the Colubridae than in the Elapidae, but no significant differences existed between the other families (Table 2A). In the comparison of scaling coefficients, both Viperidae and Colubridae were significantly higher than the family Homalopsidae (Table 2B and Figure 1B). In comparing the expected eye diameters, significant differences were observed among the four groups (Table 2C and Figures 1C,D). The Colubridae have the largest eyes relative to head width, followed by the Viperidae, followed by the Elapidae, and the family Homalopsidae was the smallest (Table 2C). Taken together, the higher allometric growth index and proportionality coefficient of the later-emerging Colubridae resulted in a significantly larger increase in eye diameter with head width than the other three taxa, and a significantly larger expected eye diameter than the other three taxa (Figure 1D).
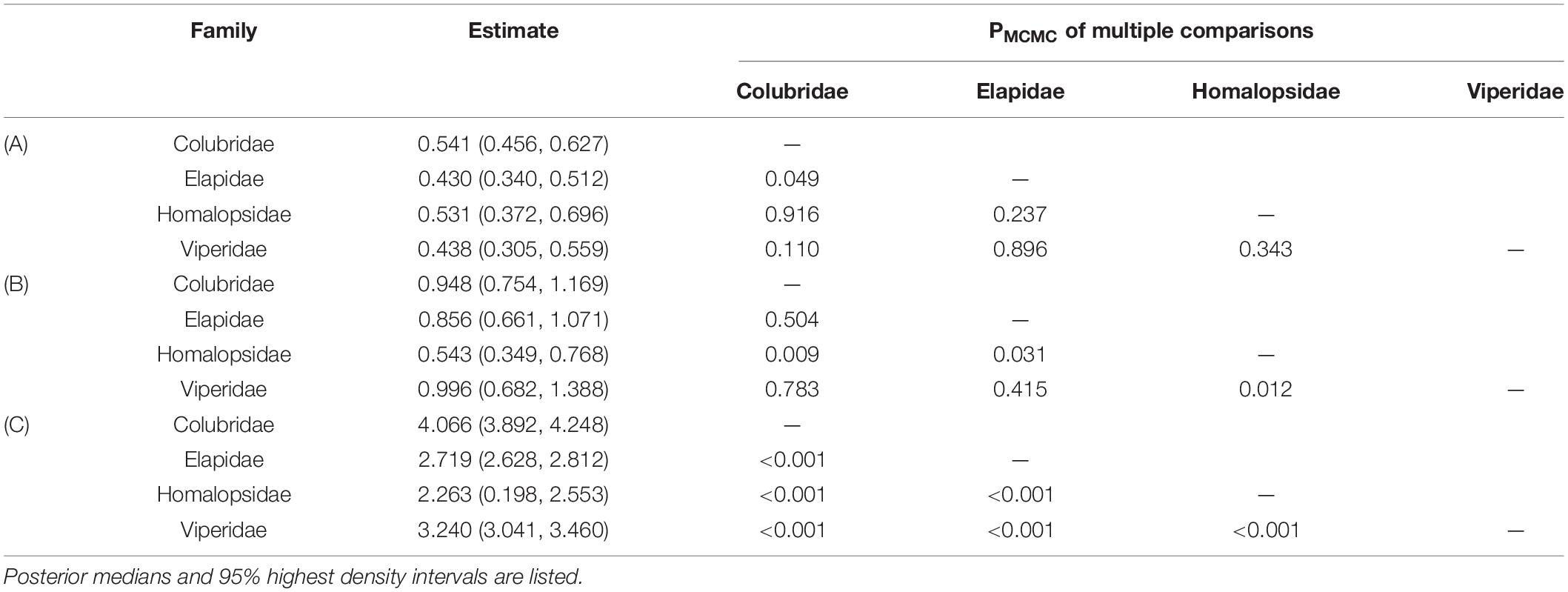
Table 2. Summary of posterior distributions and multiple comparisons of scaling coefficients (A), proportionality coefficients (B) and relative eye diameters (C) among four clades.
Habitat Types
In terms of the correlation between eye diameter and head width and habitat type, the median of the allometric growth index was very similar and not statistically significant for the four habitat types (PMCMC > 0.997; Figure 2A). In terms of the proportional coefficients, the magnitude of the terrestrial type was significantly higher compared with the freshwater type (PMCMC = 0.013; Figure 2B) and marginally higher compared with the seawater type, respectively (PMCMC = 0.055; Figure 2B). There was no significant difference in the proportional coefficients when comparing marine and freshwater types (PMCMC = 0.518; Figure 2B) or the arboreal type with the other three types (PMCMC = 0.624; Figure 2B). Similarly, in terms of the expected eye diameter, the magnitude of the terrestrial type was significantly higher compared with the freshwater type (PMCMC = 0.013; Figure 2C) and marginally higher compared with the seawater type, respectively (PMCMC = 0.055; Figure 2C). There was no significant difference in the expected eye diameter when comparing marine and freshwater types (PMCMC = 0.518; Figure 2C) or the arboreal type with the other three types (PMCMC = 0.624; Figure 2C). In summary, the relative eye diameter of aquatic snakes was significantly smaller than that of terrestrial snakes, and this phenomenon was mainly due to the difference in the ratio coefficients rather than the allometric growth index (Figure 2D).
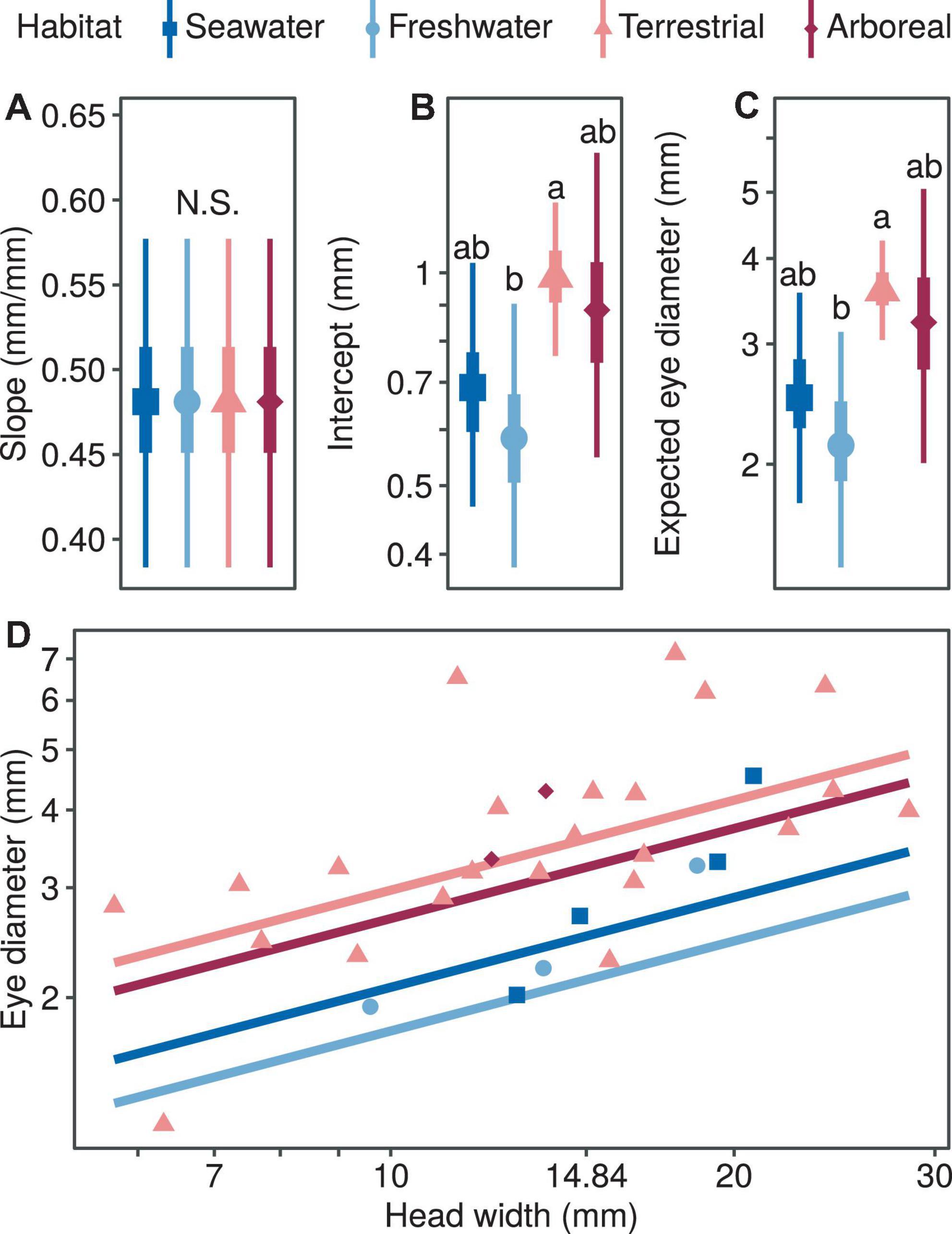
Figure 2. Comparison of growth patterns of eye diameter and head width for different taxa, including allometric growth index (A), proportionality coefficient (B), expected eye diameter (C) and scatter diagram (D). In subplots (A–C), the symbols, vertical thick lines and vertical thin lines indicate the median, 50 and 95% HDI of the posterior distribution, respectively, and lowercase letters indicate multiple comparisons between groups (not having the same letter indicates a significant difference), while N.S. indicates no statistical difference. In (D), the points indicate the mean head width and eye diameter within each species.
Diel Activity Pattern
With regard to the correlation between eye diameter and head width and diel activity pattern, the median allometric growth index was higher for the diurnal type, but there was no statistically significant difference among the three types (Figure 3A). In terms of the proportionality coefficient, the coefficient for the diurnal type was the highest and significantly higher than that for the nocturnal type (PMCMC = 0.043; Figure 3B), while the cathemeral type was at the intermediate level and did not differ significantly from the other two types (Figure 3B). In comparing the expected eye diameter, the median coefficient was the lowest for the nocturnal type (PMCMC = 0.038; Figure 3C) and intermediate for the diurnal type (PMCMC = 0.038; Figure 3C), which was not significantly different from the other two types (Figure 3C). In summary, the relative eye diameters of nocturnal snakes were significantly smaller than those of diurnal snakes, and this was mainly due to the difference in the scaling coefficients (Figure 3D).
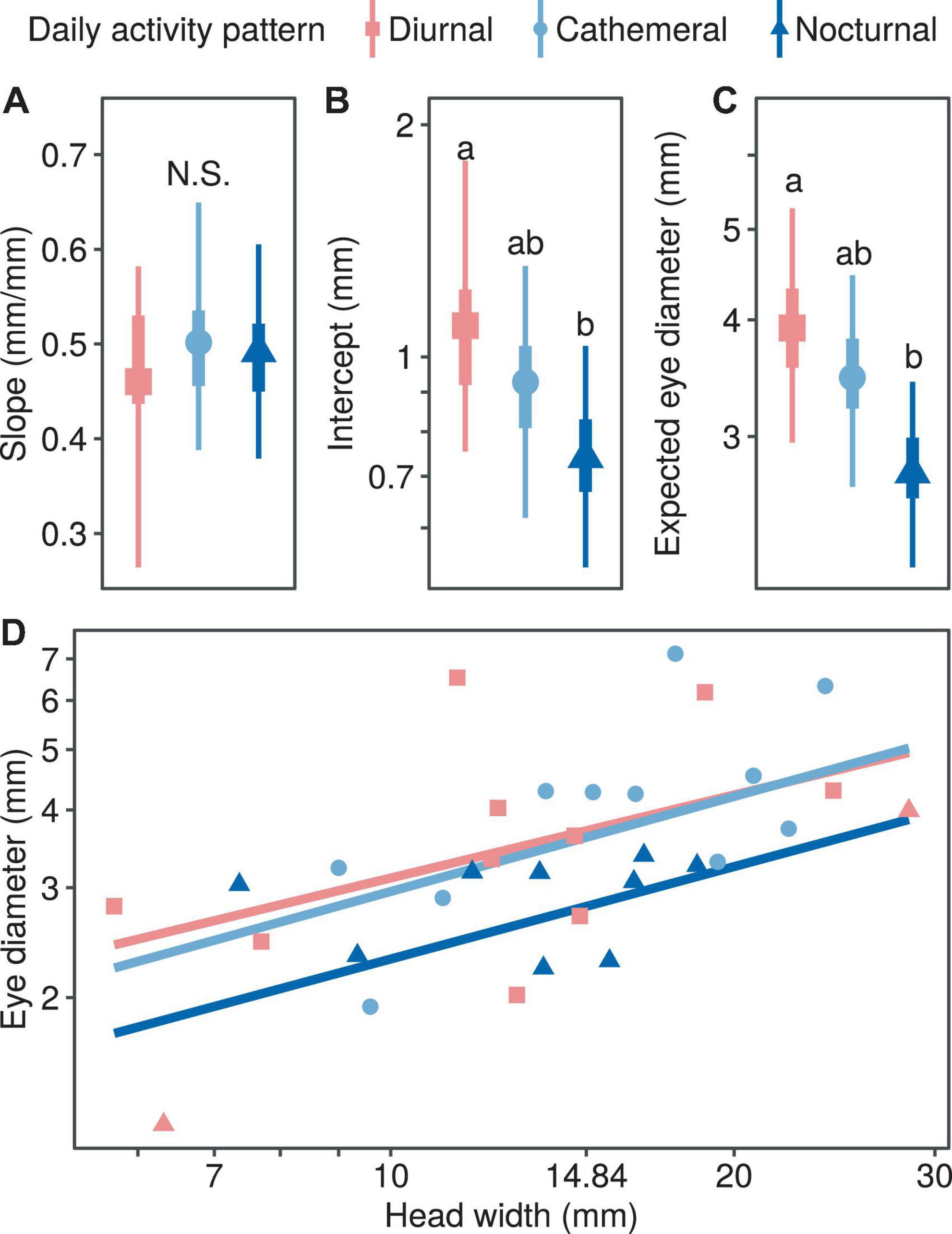
Figure 3. Comparison of growth patterns of eye diameter and head width at different activity times, including allometric growth index (A), proportionality coefficient (B), expected eye diameter (C) and scatter diagram (D). In subplots (A–C), the symbols, vertical thick lines and vertical thin lines indicate the median, 50 and 95% HDI of the posterior distribution, respectively, and lowercase letters indicate multiple comparisons between groups (not having the same letter indicates a significant difference), while N.S. indicates no statistical difference. In (D), the points indicate the mean head width and eye diameter within each species.
Foraging Strategy and the Presence of Pits
We found no significant difference in the regression coefficients of the eye diameter when comparing the two foraging types (allometric growth index, PMCMC = 0.391; proportionality coefficient, PMCMC = 0.469; expected eye diameter, PMCMC = 0.581; Figure 4). Also, we found no significant difference in the regression coefficients of the eye diameter when comparing the snakes with and without pit (allometric growth index, PMCMC = 0.350; proportionality coefficient, PMCMC = 0.496; expected eye diameter, PMCMC = 0.610; Figure 5).
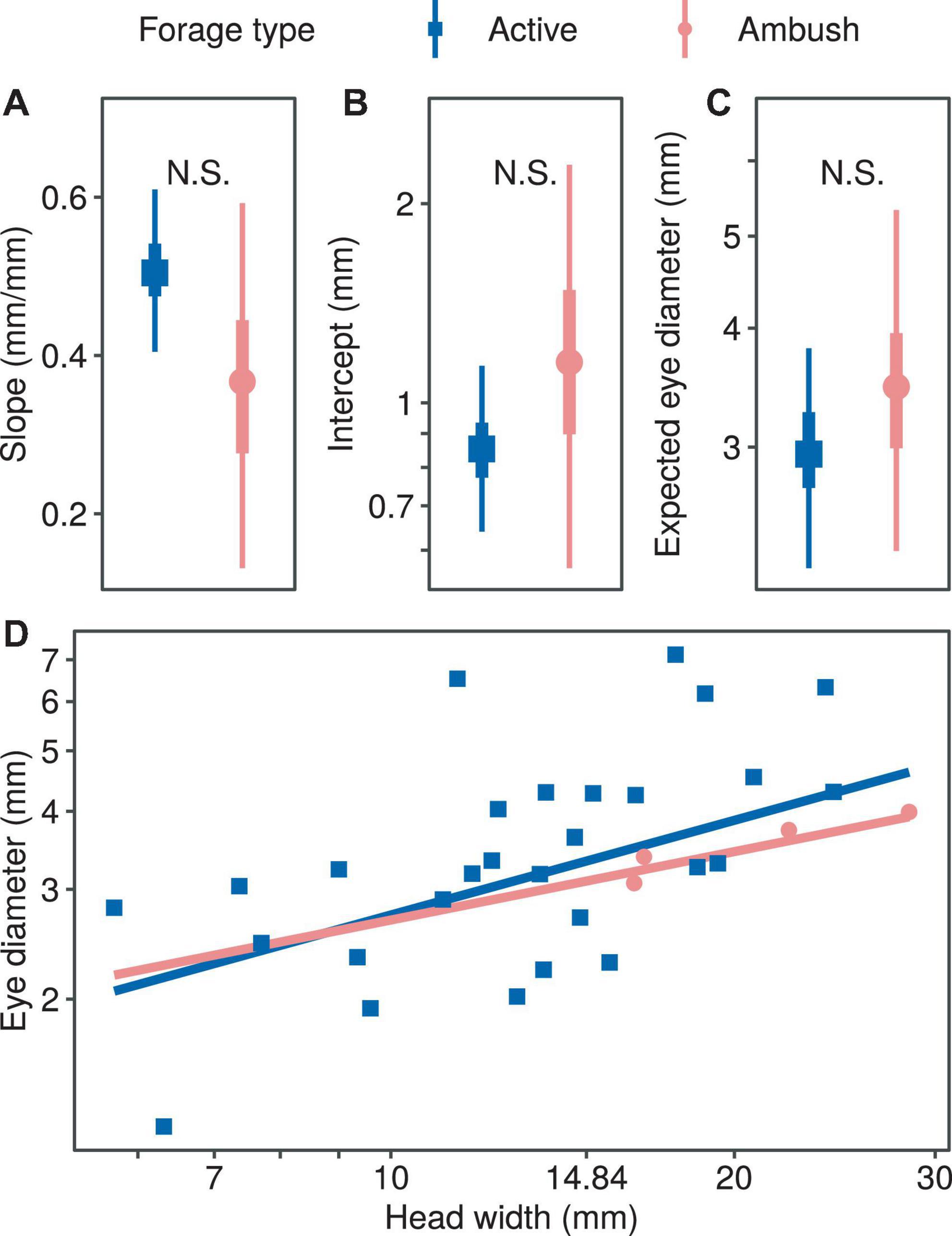
Figure 4. Comparison of growth patterns of eye diameter and head width for different foraging modes, including allometric growth index (A), scaling factor (B), expected eye diameter (C) and scatter diagram (D). In subplots (A–C), the symbols, vertical thick lines and vertical thin lines indicate the median, 50 and 95% HDI of the posterior distribution, respectively, and lowercase letters indicate multiple comparisons between groups (not having the same letter indicates a significant difference), while N.S. indicates no statistical difference. In (D), the points indicate the mean head width and eye diameter within each species.
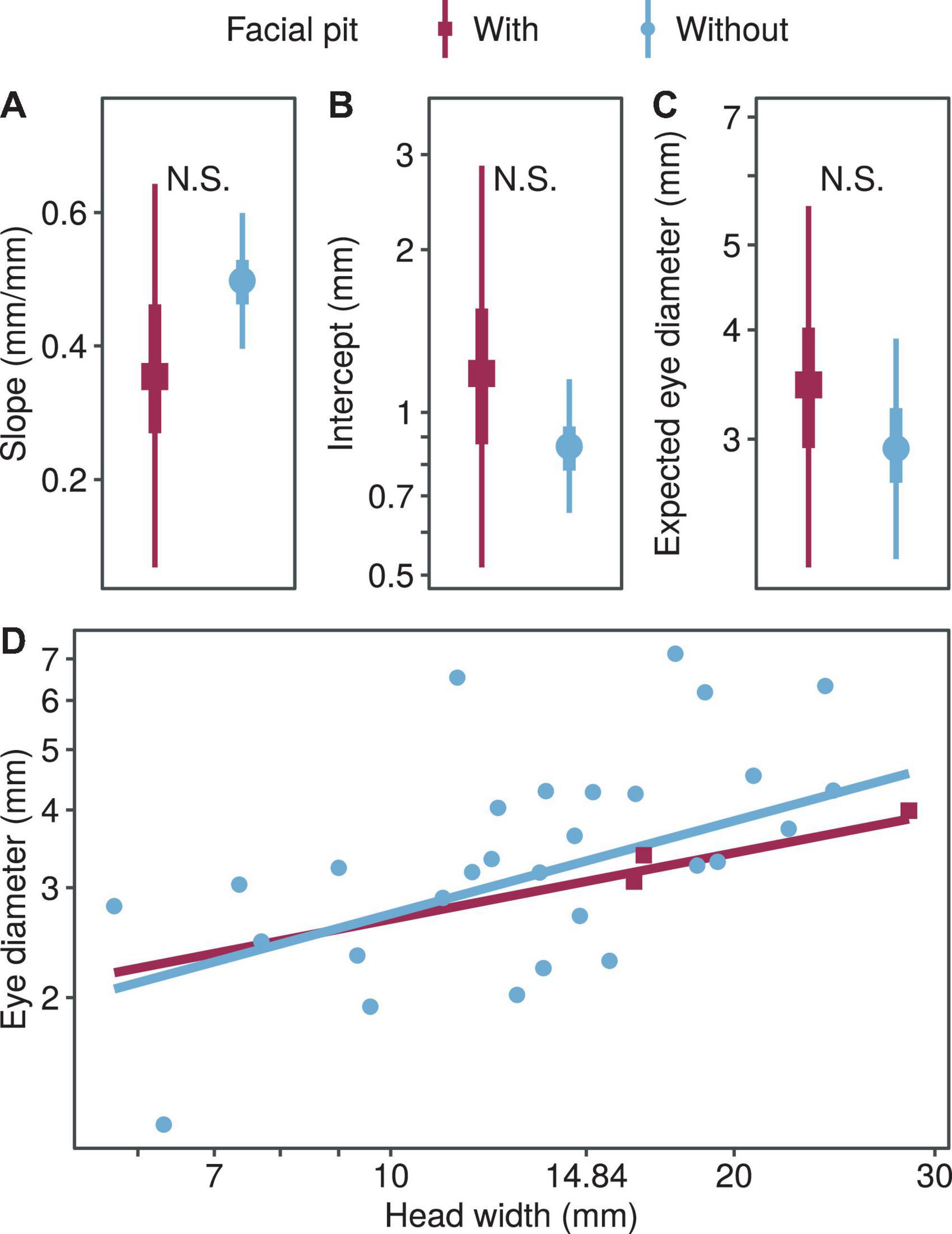
Figure 5. Comparison of growth patterns of eye diameter and head width with and without pit, including allometric growth index (A), proportionality coefficient (B), expected eye diameter (C) and scatter diagram (D). In subplots (A–C), the symbols, vertical thick lines and vertical thin lines indicate the median, 50 and 95% HDI of the posterior distribution, respectively, and lowercase letters indicate multiple comparisons between groups (not having the same letter indicates a significant difference), while N.S. indicates no statistical difference. In (D), the points indicate the mean head width and eye diameter within each species.
Discussion
In this study, we demonstrated the important role of environmental pressures in driving the evolution of the eye size in snakes. Among the four families we examined, Colubridae exhibited the most apparent trend of evolutionary allometry of eyes. In addition, we showed that terrestrial and/or diurnal snakes have larger eyes, based on our comparative analyses, whereas foraging strategies and the presence of pits did not correlate with the relative eye size of the snakes we examined.
Phylogeny
Colubridae, the latest differentiated family examined in this study, have the larger relative eye size. This suggests that they may have developed better vision and/or relied more on the visual cues in engaging in the daily activities compared with the rest of more ancestral snake families. Such implications comply with the conventional thought regarding the evolutionary direction of snake vision. Snakes have improved the visual ability along with the evolutionary history. There might be up to five visual opsins present in the ancestral vertebrate (i.e., RH1, RH2, SWS1, SWS2, and LWS; Simões et al., 2015). While the snakes that belong to the basal lineage, scolecophidians, only express RH1 genes likely as an adaptation of fossorial habits, most of the stem snakes express the additional two opsin genes that enable dichromatic color vision (i.e., SWS1 and LWS) (Tu, 2004; Davies et al., 2009; Simões et al., 2015; Katti et al., 2019). The better visual ability, as the result of the development of larger eyes (Corral-López et al., 2017), could have further advanced the performance of how they explore and utilize resources in the environment. Snakes have generally been considered to evolve from slow, passive cavity-dwelling predators to agile, aggressive predators (Underwood, 1967). The larger eye size associated with the extremely high proportion of the active hunting strategy, at least among the Colubridae species examined here, may thus provides a hint on how the visual system could have facilitated the evolution of foraging strategies in this organism.
Habitat Type
Terrestrial snakes have a larger relative eye size than that inhabit the freshwater environments, while there was no difference in the relative eye size among the comparisons across other habitat types. Our findings are inconsistent with the previous study that concluded that arboreal snakes had larger eyes than the terrestrial and semiaquatic snakes (Liu et al., 2012). This inconsistence may have occurred because the phylogenetic effects were not included in the analyses of the previous study.
Notably, the difference in the eye size between terrestrial and freshwater snakes were not generated by the allometry of eye size vs. head width (i.e., the slope), but primarily by the absolute eye size (i.e., the intercept; Figure 2). Given that it is the absolute eye size that directly determines the visual functions and optical limits (Heesy and Hall, 2010; Veilleux and Kirk, 2014), the results suggests that it would require the freshwater snakes to either expand the body size dramatically or suppress the development of other vital organs in skulls in order to employ vision in the same level as terrestrial species. Such evolutionary strategies may not be as beneficial for the freshwater snakes, considering the high energetic costs potentially incurred and that information may be delivered more efficiently by other sensory systems (e.g., olfactory) than the visual system in aquatic environments due to limited illuminance.
Diel Activity Pattern
Our results showed that the eyes of nocturnal snakes appeared to be smaller than diurnal snakes, indicating that the diel activity pattern acts as the causal, behavioral mechanism, shaping the evolution of snake vision. Such a statement aligns with the conclusion derived by the two previous studies of Colubrid snakes (Liu et al., 2012; Hauzman et al., 2018) and one of them further showed that nocturnal snakes have reduced visual acuity and poorer spatial resolution power (Hauzman et al., 2018). Despite the difficulty in navigating the environment in the dim-light condition, some nocturnal, highly mobile animals (e.g., geckos; Werner and Seifan, 2006), waterfowls (Thomas et al., 2006), or primates (Kirk, 2006) have shown to overcome the obstacles with the large eyes evolved. Distinctively, in our case, we showed how the less mobile animals (e.g., snakes) could have adopted the other evolutionary trajectory by adapting to the nocturnal activity pattern with other sensory systems complemented (Chen and Wiens, 2020). The majority of Viperidae are classified as nocturnal species in this study; this family has demonstrated the remarkable ability of exploiting the vomeronasal and the infrared sensing systems for discriminating prey species (Yang and Mori, 2021) and thermoregulatory (Krochmal et al., 2004). Our findings, regarding the eye size reduction in nocturnal snakes, thus yield a new hypothesis in terms of the evolutionary origin of multiple sensory modalities associated with the diel activity in snakes. Meanwhile, such a bipolar adaptive response of eye size across a broader taxonomic scale to the nocturnal environments also stresses the complexity of optimal sensory performance as the product of multidimensional evolutionary attributes (e.g., behavior, ecology, physiology, and phylogeny).
Foraging Strategy and the Presence of Pits
Both foraging strategies and the presence of pits showed no effect on the eye size based on the analyses, suggesting that snakes may have similar visual demands when exploiting either active hunting or ambush strategy. It also implies that the information perceived through the visual system is as important as that through the infrared system and, therefore, the presence of pits does not necessary relax the selection intensity in the evolution of snake eyes. Despite that we did not detect the significant effect of the two respective traits on the eye size, the results indeed generate some research directions worthwhile to examine subsequently. For example, considering the strong association between foraging strategies and the spatial structure of feeding habitats (Gilmour et al., 2018), as well as the evolutionary directions of the foraging strategy and optical ability cohered in snakes (Underwood, 1967; Tu, 2004; Simões et al., 2015; Katti et al., 2019), one may test whether the arboreal snakes tend to possess smaller eyes and adopt the ambush strategy disproportionally. By exploring how the behavioral, physiological, and ecological traits contribute to the evolution of eye size interactively, we are able to foster our ability to account for the evolution of innovative sensory systems and their role in promoting species adaptation across a broader geographical landscape.
Data Availability Statement
The original contributions presented in the study are included in the article/Supplementary Material, further inquiries can be directed to the corresponding author/s.
Ethics Statement
The animal study was reviewed and approved by NMNSH002.
Author Contributions
MC-WH and W-SH conceived the study. MC-WH measured all of the specimens. C-PL performed the statistical analyses. C-PL, C-CC, and W-SH led the writing. J-WL revised several sections with critical inputs in research discussions. All authors gave final approval for the publication.
Funding
The study was funded by the research grant from the Ministry of Science and Technology to W-SH (MOST 109-2621-B-178-001-MY3) and J-WL (MOST 110-2311-B-178-001-MY3).
Conflict of Interest
The authors declare that the research was conducted in the absence of any commercial or financial relationships that could be construed as a potential conflict of interest.
Publisher’s Note
All claims expressed in this article are solely those of the authors and do not necessarily represent those of their affiliated organizations, or those of the publisher, the editors and the reviewers. Any product that may be evaluated in this article, or claim that may be made by its manufacturer, is not guaranteed or endorsed by the publisher.
Acknowledgments
We appreciated R-C. Cheng for his kind advisements on analyses. We appreciated C-H. Chang for his kind helps of museum specimen collections.
Supplementary Material
The Supplementary Material for this article can be found online at: https://www.frontiersin.org/articles/10.3389/fevo.2021.821965/full#supplementary-material
Supplementary Figure 1 | The measurement of snake eye diameter (A) and head width (B).
Supplementary Figure 2 | The most approximate relatives of 31 snake species from Taiwan used in this study. The phylogenetic tree is adapted from Figueroa et al. (2016) based on the most approximate phylogenetic tree. The numbers to the right of the nodes indicate the support of the bootstrap method. Red texts indicate that species in this study could not be matched with Figueroa et al. (2016) and should be synonyms. Blue texts indicate species were not included in Figueroa et al. (2016), so they are represented by similar species.
References
Ausprey, I. J., Newell, F. L., and Robinson, S. K. (2021). Adaptations to light predict the foraging niche and disassembly of avian communities in tropical countrysides. Ecology 102:e03213. doi: 10.1002/ecy.3213
Bittencourt, G. B., Hauzman, E., Bonci, D. M. O., and Ventura, D. F. (2019). Photoreceptors morphology and genetics of the visual pigments of Bothrops jararaca and Crotalus durissus terrificus (Serpentes, Viperidae). Vision Res. 158, 72–77. doi: 10.1016/j.visres.2019.02.006
Bürkner, P.-C. (2018). Advanced Bayesian multilevel modeling with the R package brms. R J. 10, 395–411. doi: 10.32614/RJ-2018-017
Burton, R. F. (2008). The scaling of eye size in adult birds: relationship to brain, head and body sizes. Vision Res. 48, 2345–2351. doi: 10.1016/j.visres.2008.08.001
Camp, C. D., Wooten, J. A., Pepper, M. K., Austin, R. M. Jr., and Whitfield Gibbons, J. (2020). Eye size in North American watersnakes (genus Nerodia) correlates with variation in feeding ecology. Biol. J. Linnean. Soc. 131, 774–784. doi: 10.1093/biolinnean/blaa163
Caves, E. M., Sutton, T. T., and Johnsen, S. (2017). Visual acuity in ray-finned fishes correlates with eye size and habitat. J. Exp. Biol. 220, 1586–1596. doi: 10.1242/jeb.151183
Chen, Z., and Wiens, J. J. (2020). The origins of acoustic communication in vertebrates. Nat. Commun. 11:369. doi: 10.1038/s41467-020-14356-3
Corral-López, A., Garate-Olaizola, M., Buechel, S. D., Kolm, N., and Kotrschal, A. (2017). On the role of body size, brain size, and eye size in visual acuity. Behav. Ecol. Sociobiol. 71:179. doi: 10.1007/s00265-017-2408-z
Danaisawadi, P., Asami, T., Ota, H., Sutcharit, C., and Panha, S. (2016). A snail-eating snake recognizes prey handedness. Sci. Rep. 6:23832. doi: 10.1038/srep23832
Davies, W. L., Cowing, J. A., Bowmaker, J. K., Carvalho, L. S., Gower, D. J., and Hunt, D. M. (2009). Shedding light on serpent sight: the visual pigments of henophidian snakes. J. Neurosci. 29, 7519–7525. doi: 10.1523/jneurosci.0517-09.2009
Figueroa, A., Mckelvy, A. D., Grismer, L. L., Bell, C. D., and Lailvaux, S. P. (2016). A species-level phylogeny of extant snakes with description of a new colubrid subfamily and genus. PLoS One 11:e0161070. doi: 10.1371/journal.pone.0161070
Gilmour, M. E., Castillo-Guerrero, J. A., Fleishman, A. B., Hernández-Vázquez, S., Young, H. S., and Shaffer, S. A. (2018). Plasticity of foraging behaviors in response to diverse environmental conditions. Ecosphere 9:e02301. doi: 10.1002/ecs2.2301
Greene, H. W. (1997). Snakes: The Evolution of Mystery in Nature. Berkeley, CA: University of California Press.
Hadfield, J. D., and Nakagawa, S. (2010). General quantitative genetic methods for comparative biology: phylogenies, taxonomies and multi-trait models for continuous and categorical characters. J. Evol. Biol. 23, 494–508. doi: 10.1111/j.1420-9101.2009.01915.x
Harper, D. G. C. (1988). “Robin Erithacus rubecula species account,” in Handbook of Birds of Europe, the Middle East and North Africa, ed. S. Cramp (Oxford: Oxford University Press), 605.
Hauzman, E., Bonci, D. M. O., and Ventura, D. S. F. (2018). “Retinal topographic maps: a glimpse into the animals’ visual world,” in Sensory nervous system, (London: IntechOpen). Available online at: https://www.intechopen.com/chapters/59768
Heard-Booth, A. N., and Kirk, E. C. (2012). The influence of maximum running speed on eye size: a test of Leuckart’s law in mammals. Anat. Rec. 295, 1053–1062. doi: 10.1002/ar.22480
Heesy, C. P., and Hall, M. I. (2010). The nocturnal bottleneck and the evolution of mammalian vision. Brain Behav. Evol. 75, 195–203. doi: 10.1159/000314278
Howland, H. C., Merola, S., and Basarab, J. R. (2004). The allometry and scaling of the size of vertebrate eyes. Vision Res. 44, 2043–2065. doi: 10.1016/j.visres.2004.03.023
Katti, C., Stacey-Solis, M., Coronel-Rojas, N. A., and Davies, W. I. L. (2019). The diversity and adaptive evolution of visual photopigments in reptiles. Front. Ecol. Evol. 7:352. doi: 10.3389/fevo.2019.00352
Kirk, E. C. (2006). Effects of activity pattern on eye size and orbital aperture size in primates. J. Hum. Evol. 51, 159–170. doi: 10.1016/j.jhevol.2006.02.004
Krochmal, A. R., Bakken, G. S., and Laduc, T. J. (2004). Heat in evolution’s kitchen: evolutionary perspectives on the functions and origin of the facial pit of pitvipers (Viperidae: Crotalinae). J. Exp. Biol. 207, 4231–4238. doi: 10.1242/jeb.01278
Laughlin, S. B. (1995). “Towards the cost of seeing,” in Nervous Systems and Behaviour, eds M. Burrows, T. Matheson, P. L. Newland, and H. Schuppe (New York, NY: Thieme), 290.
Laughlin, S. B., De Ruyter Van Steveninck, R. R., and Anderson, J. C. (1998). The metabolic cost of neural information. Nat. Neurosci. 1, 36–41. doi: 10.1038/236
Lisney, T. J., and Collin, S. P. (2007). Relative eye size in Elasmobranchs. Brain Behav. Evol. 69, 266–279. doi: 10.1159/000100036
Liu, Y., Ding, L., Lei, J., Zhao, E., and Tang, Y. (2012). Eye size variation reflects habitat and daily activity patterns in colubrid snakes. J. Morphol. 273, 883–893. doi: 10.1002/jmor.20028
Nummela, S., Pihlström, H., Puolamäki, K., Fortelius, M., Hemilä, S., and Reuter, T. (2013). Exploring the mammalian sensory space: co-operations and trade-offs among senses. J. Comp. Physiol. A 199, 1077–1092. doi: 10.1007/s00359-013-0846-2
Roaf, H. E. (1943). The vertebrate eye and its adaptive radiation. Nature 151, 236–236. doi: 10.1038/151236a0
Schmitz, L., and Higham, T. E. (2018). Non-uniform evolutionary response of gecko eye size to changes in diel activity patterns. Biol. Lett. 14:20180064. doi: 10.1098/rsbl.2018.0064
Schraft, H. A., and Clark, R. W. (2019). Sensory basis of navigation in snakes: the relative importance of eyes and pit organs. Anim. Behav. 147, 77–82. doi: 10.1016/j.anbehav.2018.11.004
Shrimpton, S. J., Streicher, J. W., Gower, D. J., Bell, R. C., Fujita, M. K., Schott, R. K., et al. (2021). Eye-body allometry across biphasic ontogeny in anuran amphibians. Evol. Ecol. 35, 337–359. doi: 10.1007/s10682-021-10102-3
Simões, B. F., Sampaio, F. L., Douglas, R. H., Kodandaramaiah, U., Casewell, N. R., Harrison, R. A., et al. (2016). Visual pigments, ocular filters and the evolution of snake vision. Mol. Biol. Evol. 33, 2483–2495. doi: 10.1093/molbev/msw148
Simões, B. F., Sampaio, F. L., Jared, C., Antoniazzi, M. M., Loew, E. R., Bowmaker, J. K., et al. (2015). Visual system evolution and the nature of the ancestral snake. J. Evol. Biol. 28, 1309–1320. doi: 10.1111/jeb.12663
Thomas, K. N., Gower, D. J., Bell, R. C., Fujita, M. K., Schott, R. K., and Streicher, J. W. (2020). Eye size and investment in frogs and toads correlate with adult habitat, activity pattern and breeding ecology. Proc. R. Soc. B Biol. Sci. 287:20201393. doi: 10.1098/rspb.2020.1393
Thomas, R., Székely, T., Powell, R., and Cuthill, I. (2006). Eye size, foraging methods and the timing of foraging in shorebirds. Funct. Ecol. 20, 157–165. doi: 10.1111/j.1365-2435.2006.01073.x
Underwood, G. (1967). A Contribution to the Classification of Snakes. London: British Museum Natural History Publications.
Veilleux, C. C., and Kirk, E. C. (2014). Visual acuity in mammals: effects of eye size and ecology. Brain Behav. Evol. 83, 43–53. doi: 10.1159/000357830
Vinterstare, J., Hulthén, K., Nilsson, D. E., Nilsson, P. A., and Brönmark, C. (2020). More than meets the eye: predator-induced pupil size plasticity in a teleost fish. J. Anim. Ecol. 89, 2258–2267. doi: 10.1111/1365-2656.13303
Werner, Y., and Seifan, T. (2006). Eye size in geckos: asymmetry, allometry, sexual dimorphism, and behavioral correlates. J. Morphol. 267, 1486–1500. doi: 10.1002/jmor.10499
Keywords: snake eye size, diel activity patterns, habitat type, allometric growth, phylogenetic regression
Citation: Huang MC-W, Liao C-P, Chou C-C, Lin J-W and Huang W-S (2022) Size of Snake Eyes Correlates With Habitat Types and Diel Activity Patterns. Front. Ecol. Evol. 9:821965. doi: 10.3389/fevo.2021.821965
Received: 25 November 2021; Accepted: 27 December 2021;
Published: 24 January 2022.
Edited by:
Michael Hrncir, University of São Paulo, BrazilReviewed by:
Jessica Wooten, Piedmont University, United StatesEinat Hauzman, University of São Paulo, Brazil
Copyright © 2022 Huang, Liao, Chou, Lin and Huang. This is an open-access article distributed under the terms of the Creative Commons Attribution License (CC BY). The use, distribution or reproduction in other forums is permitted, provided the original author(s) and the copyright owner(s) are credited and that the original publication in this journal is cited, in accordance with accepted academic practice. No use, distribution or reproduction is permitted which does not comply with these terms.
*Correspondence: Wen-San Huang, d3NodWFuZy4zODBAZ21haWwuY29t
†These authors have contributed equally to this work