- 1Department of Forestry and Wildlife Management, Faculty of Applied Ecology and Agricultural Sciences, Inland Norway University of Applied Sciences, Koppang, Norway
- 2Laboratory of Biometry and Evolutionary Biology, Université de Lyon, VetAgro Sup, CNRS, Marcy-l’Etoile, France
- 3Behavioural Ecology, Department of Biology, Ludwig Maximilian University of Munich, München, Germany
- 4Scandinavian Brown Bear Research Project, Orsa, Sweden
- 5Norwegian Institute for Nature Research, Trondheim, Norway
- 6Department of Wildlife, Fish and Environmental Studies, Faculty of Forest Sciences, Swedish University of Agricultural Sciences, Umeå, Sweden
Introduction: Climate change is altering the reproductive phenology of many organisms, but the factors that influence the timing of gestation in ursids are still poorly understood. Higher temperatures in spring are already causing an earlier den exit in some brown bear populations, and a temporal mismatch between hibernation and reproduction could have dramatic consequences for reproductive success. Therefore, understanding the factors that control the timing of these events is important to forecast the consequences of climate change on population growth rates.
Methods: In this study, we used abdominal temperature loggers and GPS collars with acceleration sensors on 23 free-ranging pregnant female brown bears living in two areas in Sweden (61°N and 67°N latitude) to pinpoint hibernation and reproductive events. We investigated how intrinsic and extrinsic factors influence the termination of embryonic diapause and parturition, as well as their impact on reproductive success.
Results: The termination of embryonic diapause was later in the northern area compared to the southern area and occurred earlier when ambient temperature at den entry was higher in both areas. In the southern area, young adults (i.e., females = 7 years old) had a delayed parturition when bilberry abundance was low the year of mating. Additionally, young adults had a lower reproductive success than adults and their probability to reproduce successfully was dependent on bilberry abundance, whereas adult females were not affected by this parameter.
Discussion: As den exit occurs later in the northern study area, we suggest that a later parturition might ensure that females lactate their cubs in the den for a reasonable amount of time while fasting. Similarly, a later parturition combined with an earlier emergence could allow young adults to spend less time in the den lactating if they could not reach an optimal body condition prior to hibernation. But as a result, their cubs are younger and more vulnerable when they leave the den leading to lower survival rates. Our results suggest that a decreased berry abundance in the fall could impact the reproductive and hibernation phenology of Scandinavian brown bear females and lead to a lower cub survival with potential consequences on the population dynamics.
Introduction
Seasonal reproduction is common among mammals living in temperate zones, where reproductive events are precisely timed during the annual cycle to maximize mating success and offspring survival (Bronson, 2009). In most species, environmental cues (i.e., photoperiod, ambient temperature, precipitation and/or food availability) are the principal factors that synchronize these events (Bronson, 2009). As climate change is altering the timing of some natural processes, including a rise in ambient temperatures and an earlier peak of plant productivity in spring (IPCC, 2021), animals’ reproduction might become out of synchrony with their environment (Root et al., 2003). An advancement of seasonal breeding has been reported in mammals and birds, but unequal shifts in the timing of births and peak of trophic resources can lead to a temporal mismatch between the two events with negative impacts on reproductive success (reviewed in Parmesan, 2007; Bronson, 2009). In particular, long-lived large mammals in Nordic areas are believed to be among the most vulnerable species. Indeed, higher latitudes have warmed the fastest in the past half century (Root et al., 2003), advantageous genetic traits might not be selected fast enough due to their slow reproduction (Hetem et al., 2014), and the timing of their reproductive events is believed to be mainly controlled by photoperiod and could lack flexibility (Spady et al., 2007; Bronson, 2009; Appleton et al., 2018). However, as the brown bear (Ursus arctos) is an opportunistic omnivore with a broad climatic niche, it is unclear if climate change will affect negatively this species.
To date, bears are the only mammals for which delayed implantation, active gestation, parturition, and lactation have been reported during hibernation, a period when they do not eat, drink, urinate or defecate for several months. This period is critical for pregnant females as they must provide energy for fetal development and milk production in addition to their own needs, which results in a greater loss of mass at den emergence than other reproductive status (Harlow et al., 2002; Keay et al., 2018). However, the reproductive physiology of bears, and in particular the mechanisms of delayed implantation, remains poorly understood. Because the literature for brown bear reproduction is incomplete, we have drawn inferences from other Ursinae species that share a similar physiology resulting from common ancestry and recurring gene flow across bear species during their evolutionary history (Kumar et al., 2017).
Brown bears are polygamous seasonal breeders with a mating season occurring in late spring-early summer in temperate zones (Spady et al., 2007). After mating, the female undergoes an obligate embryonic diapause during which the embryos stop their development at the blastocyst stage and remain free-floating in the uterus (Wimsatt, 1963; Kordek and Lindzey, 1980). During this period, each corpus luteum (CL) that formed after ovulation remains quiescent and synthetizes low levels of progesterone (Tsubota et al., 1987; Tsubota and Kanagawa, 1993). The embryonic diapause terminates with the reactivation of the CL causing a surge in progesterone (Tsubota et al., 1987; Tsubota and Kanagawa, 1993), and is followed by embryos’ implantation in November-December after the female has entered her den (Wimsatt, 1963; Kordek and Lindzey, 1980). In other carnivores with a delayed implantation, there is a time span of several days between the CL reactivation and the implantation of the blastocysts, referred to as secretory phase, and during which the uterus lining prepares itself to receive the fertilized eggs by producing a hospitable environment (6–10 days in the American mink Neovison vison, Murphy et al., 1981; Murphy and Fenelon, 2020). To our knowledge, the duration of the secretory phase remains unknown in ursids which precludes the exact determination of the active gestation length. However, with the omission of this phase, it has been suggested that the gestation of brown bears lasts between 56 and 60 days based on body temperature and progesterone levels, respectively (Foresman and Daniel, 1983; Tsubota et al., 1987; Friebe et al., 2014).
The active phase of gestation is associated with a metabolism rise due to the maintenance of high progesterone levels and the presence of fetuses (Shimozuru et al., 2013). In particular, the body temperature (Tb) is higher and more stable during gestation (37–38°C vs. 34–36°C) and drops to near hibernation levels around parturition time (Shimozuru et al., 2013; Friebe et al., 2014). The start of gestation is currently assumed to take place in November-December, at the time when the progesterone levels (Foresman and Daniel, 1983; Tsubota et al., 1987) and Tb (Shimozuru et al., 2013; Friebe et al., 2014) start to increase. But these events are most likely associated with CL reactivation as this structure synthesizes progesterone in bears (Tsubota et al., 2001) and increased plasma progesterone levels result in a rise of Tb within 24 h (de Mouzon et al., 1984). However, because different studies support the need of a high and stable Tb for embryo development during the active gestation phase in both hibernating and non-hibernating mammals (Laburn et al., 2002; McAllan and Geiser, 2014; Trethowan et al., 2016; Schmidt et al., 2020), it is unlikely that implantation occurs before Tb has reached a plateau near active levels.
Parturition occurs around January-February in the den where the female lactates for 3–4 months before emerging in spring (Friebe et al., 2014). Studies conducted on carnivores (Trethowan et al., 2016; Thiel et al., 2019) and hibernating rodents (Williams et al., 2011; Bieber et al., 2017) have shown that the day of parturition is associated with a sharp rise in Tb. Similarly, activity data has been used to determine parturition dates in the Scandinavian brown bear, with reduced activity levels reported during the postpartum period (Friebe et al., 2014). Furthermore, Rogers et al. (2020) reported increased movements during the labor and reduced movements after delivery using cameras placed inside the den of free-ranging female American black bears (Ursus americanus). Taken together, these observations provide a characteristic activity pattern that could facilitate the determination of parturition in free-ranging populations.
Delayed implantation is believed to be an important adaptation for species living in seasonal habitats to ensure birthing at the most beneficial time of the year and avoid resource demanding breeding behaviors in seasons of resource shortage (Heldstab et al., 2018). In bears, the reported length of embryonic diapause is highly variable in a given population, both between individuals and from year to year for a same individual (Spady et al., 2007; Friebe et al., 2014). Robbins et al. (2012) suggested that pregnant females may integrate intrinsic and external factors and modulate the timing of gestation long after conception to optimize their reproductive success. In this respect, the objective of this study was to pinpoint the termination of embryonic diapause and parturition in Scandinavian brown bears using Tb and activity data, and determine to what extent the timing of these events is influenced by intrinsic and extrinsic factors as well as the consequences for cubs’ survival.
In brown bears, the timing of parturition is related to latitude, with a shift of 6 months reported in captivity between the northern and the southern hemisphere (Spady et al., 2007). Latitude is associated with many environmental factors including ambient temperature, precipitation, food availability and photoperiod. Except for photoperiod, these parameters may vary greatly from year to year and are affected by climate change (IPCC, 2021). But to our knowledge, no study has so far investigated the impact of these factors on the brown bear reproduction, with the exception of Friebe et al. (2014) who found that favorable environmental conditions (formulated as a yearling body condition index as a proxy for food abundance) were correlated with a later parturition in Scandinavian brown bears in the southern range of their distribution. In contrast, the annual variation in daily light-dark cycles offers a highly predictive signal and is believed to be the main cue that synchronizes seasonal reproductive events in mammals, including the termination of embryonic diapause in non-hibernating carnivores (reviewed in Murphy and Fenelon, 2020). However, it is still unclear if this parameter plays a crucial role in the Scandinavian brown bear, as most females use under-ground den sites (Manchi and Swenson, 2005) that are snow covered from October to April (Swedish Meteorological and Hydrological Institute), which could reduce the perception of sun light in the den (Evernden and Fuller, 1972). Besides, Ware et al. (2013) reported an unusually small pineal gland and a weak melatonin rhythm in brown bears, which suggests that photoperiod might not be a cue as important for this species.
Similarly, several studies have highlighted the importance of intrinsic factors in the reproductive timing and success of female bears. A minimum of 19% fat is necessary for gestation (López-Alfaro et al., 2013), and Rogers (1976) suggested that blastocysts could be resorbed during the delay phase in case of poor physical status, although this is, to our knowledge, currently undocumented. Both offspring birth date and growth rate vary in response to the mother’s fat stores, with females in superior condition giving birth earlier and nursing their cubs longer in the den, which in turn accelerates the cubs’ growth (Robbins et al., 2012; Mesa-Cruz, 2018). As a result, cubs are heavier at den emergence and have higher chances of survival during their first year of life (Hertel et al., 2018; Keay et al., 2018). Maternal age may also influence the reproductive timing in ursids as Bridges et al. (2011) reported a later parturition date in American black bear females (Ursus americanus) aged less than 5 years old. However, this result was not found in Scandinavian brown bears, possibly due to a low number of young individuals included in that study (Friebe et al., 2014). Finally, because gestation and hibernation are two concurrent physiological states, one could expect them to be inextricably linked. Although implantation was not significantly correlated with the start of denning based on activity in Scandinavian brown bears (Friebe et al., 2014), the relatively similar age of American black bear cubs at den emergence (83, 16 days range) supports a relationship between parturition and the end of hibernation (Bridges et al., 2004).
Based on previous literature, we hypothesized and predicted that in brown bears: (1) The onset of gestation is influenced by environmental cues, in particular photoperiod, and would occur earlier at lower latitudes; (2) intrinsic factors play an important role in the reproductive timing, and parturition would be later in younger females and in females with poor body condition; and (3) the timing between parturition and den emergence impacts the reproductive success, and cubs spending less time in the den and/or emerging earlier would have a lower survival.
Materials and methods
Study area
The study was conducted in Sweden in two areas approximately 600 km apart (Supplementary Figure 1). The southern area is located in Dalarna and Gävleborg counties in central Sweden (61°N, 18°E) and the northern area in Norrbotten county in northern Sweden (67°N, 20°E). Snow cover usually lasts from the end of October to late April in the south and from the beginning of October to late May in the north. Mean ambient temperatures in January and July are –7°C/15°C in the south and –13°C/13°C in the north (Swedish Meteorological and Hydrological Institute).
Study populations
The two study populations are connected through genetic exchanges due to the dispersal of males during the mating season (Taberlet et al., 1995). However, they differ in some demographic parameters including a younger age of primiparity in the south (Zedrosser et al., 2009). During hyperphagia in late summer and fall, bears forage mostly on berries (which comprise 68% of the autumn digestible energy intake) and especially bilberries (Vaccinium myrtillus) to gain fat prior to hibernation (Stenset et al., 2016). In our study areas, the denning period ranges from October to May depending on environmental conditions and reproductive status, with bears from the north and pregnant females denning earlier and emerging later (Manchi and Swenson, 2005). Based on Tb profiles, Friebe et al. (2014) reported that the mean dates for implantation and parturition in the southern study area were 1 December and 26 January, respectively, and the gestation length was 56 days on average. Females usually give birth to 1–4 cubs and begin lactation in the den (Zedrosser et al., 2009). In central Sweden, documented cubs-of-the-year losses after den emergence can be attributed to poor nutritional conditions before the 1st of May, but are mainly due to sexually selected infanticide afterward during the mating season (Zedrosser et al., 2009).
Bear captures and data collection
The study was conducted between 2011 and 2018 on 24 individuals and 31 reproductive events including 26 from the southern study area (20 individuals) and 5 from the northern study area (4 individuals). The number of analyzed reproductive events is thereafter referred to as the sample size N. Bears were captured by darting from a helicopter in spring shortly after den emergence following the biomedical protocol established by Arnemo and Evans (2017). All captures were carried out by the Scandinavian Brown Bear Research Project and approved by the Swedish Ethical Committee on Animal Research, Uppsala, Sweden (C18/15) and the Swedish Environmental Protection Agency, Stockholm, Sweden (NV-00741-18). Individuals were fitted with a Global Positioning System (GPS) collar equipped with a dual-axis motion sensor to record the bear’s activity (Vertex Plus, Vectronic Aerospace GmbH, Berlin), and for some of them (N = 18) with a temperature logger which was surgically implanted in the peritoneal cavity (DST Centi-T, Star Oddi, Iceland). GPS positions were recorded once a day from December to March and once an hour to every minute the rest of the year depending on the current project. Activity was recorded every 5 min at 6–8 Hz and individual indices (ranging from 0 to 510) were calculated by summing the acceleration values of both orthogonal axes for each 5-min interval (Gervasi et al., 2006; Friebe et al., 2014). Tb was recorded every 30 min. For further details on the marking procedures see Friebe et al. (2014) and Arnemo and Evans (2017).
Age category
The age of the bears was determined by following the offspring of marked females from their first capture as yearlings, or by counting the annuli of a cross-section of the root of one of the first premolars after surgical extraction (Harshyne et al., 1998). We then categorized a female as young adult if aged 7 years old or younger the year she got pregnant, and as adult otherwise to account for differences in terms of growth [95% of adult mass is reached at 7 years old, (Swenson et al., 2007)] and reproductive maturity [proportion of primiparous females reaches 0% at 7 and 8 years old in the southern and northern area, respectively (Zedrosser et al., 2009), and litter productivity stabilizes at 8 years old (Schwartz et al., 2003)].
We did not have the exact age of some females (N = 4) that were not followed from birth and for which a tooth was not extracted during the capture. In this case, we used the model established by Swenson et al. (2007) to classify a female based on body mass in spring and weight variation from one year to another. Those bears were categorized as young adults if body mass was ≤ 91.2 kg and annual weight variation was ≥ 3.5%, and as adults if the annual weight variation was < 3.5%, because some females never reach the asymptotic mass obtained by Swenson et al. (2007) during their lifetime. We excluded a female of unknown age that we could not classify using the previous definition (6.75% annual weight variation with a body mass exceeding 100 kg).
Annual berry availability
As brown bears in our study area forage mostly on bilberries (Vaccinium myrtillus) in late summer and fall (Stenset et al., 2016), we used an annual bilberry abundance index (referred thereafter as berry index) to account for food availability during the hyperphagia period when brown bears build fat stores prior to hibernation. This index reflects the annual production of bilberries in central Sweden compared to the average production over the last 15 years and ranges from 0 (2010) to 1 (2015). Because we did not capture bears in the fall, we did not have access to individual females’ body condition at den entry. However, the autumn populational nutritional status of female bears has been showed to be closely related to the annual bilberry abundance in the southern study area (Hertel et al., 2018; Langvall and Ottosson Löfvenius, 2021).
Reproductive status
The reproductive status was assessed based on visual interpretation of the mean daily Tb or activity profiles (Friebe et al., 2014). Based on the Tb profile, a female was considered pregnant when she exhibited a high Tb near summer normal (37–38°C) for several weeks during hibernation. Conversely, a female was considered non-pregnant when Tb remained low (30–36°C) throughout hibernation. Based on the activity profile, a female was considered pregnant when a drop in the activity level was noticed between late December and early February and as non-pregnant otherwise. A female was categorized as multiparous if she had exhibited a Tb or activity profile conform with pregnancy before or if she had been seen with cubs previously. Females that were followed from their first year of life were categorized as primiparous when such a profile was observed for the first time. We did not have this information for one female in the south that was not marked as a yearling and had never been observed with cubs. Because she was classified as an adult based the model established by Swenson et al. (2007) (i.e., ≥ 8 years old) and the proportion of primiparous females reaches 0% at 7 years old in this area (Zedrosser et al., 2009), we considered that this female was multiparous.
Gestation events
We used the mean daily Tb profile to determine reactivation and implantation dates (Figure 1), and the raw Tb and activity profiles to determine parturition date (Figure 2). To determine the timing of reproductive events, we applied a changepoint analysis method that detects the positioning of potential points at which the statistical properties of the distribution change in a time series (Killick and Eckley, 2014). This approach has already been used to detect parturition in wolverines (Gulo gulo, Thiel et al., 2019) and artic ground squirrels (Urocitellus parryii, Williams et al., 2011). Because Tb is higher and more stable during gestation than the rest of hibernation (Friebe et al., 2014), we identified changes in mean and variance using the “cpt.meanvar” function from the R-package “changepoint” (Killick and Eckley, 2014). We chose the pruned exact linear time algorithm (Killick et al., 2012) and set the arguments “test statistic” and “penalty,” respectively, to “normal” and “0.05.”
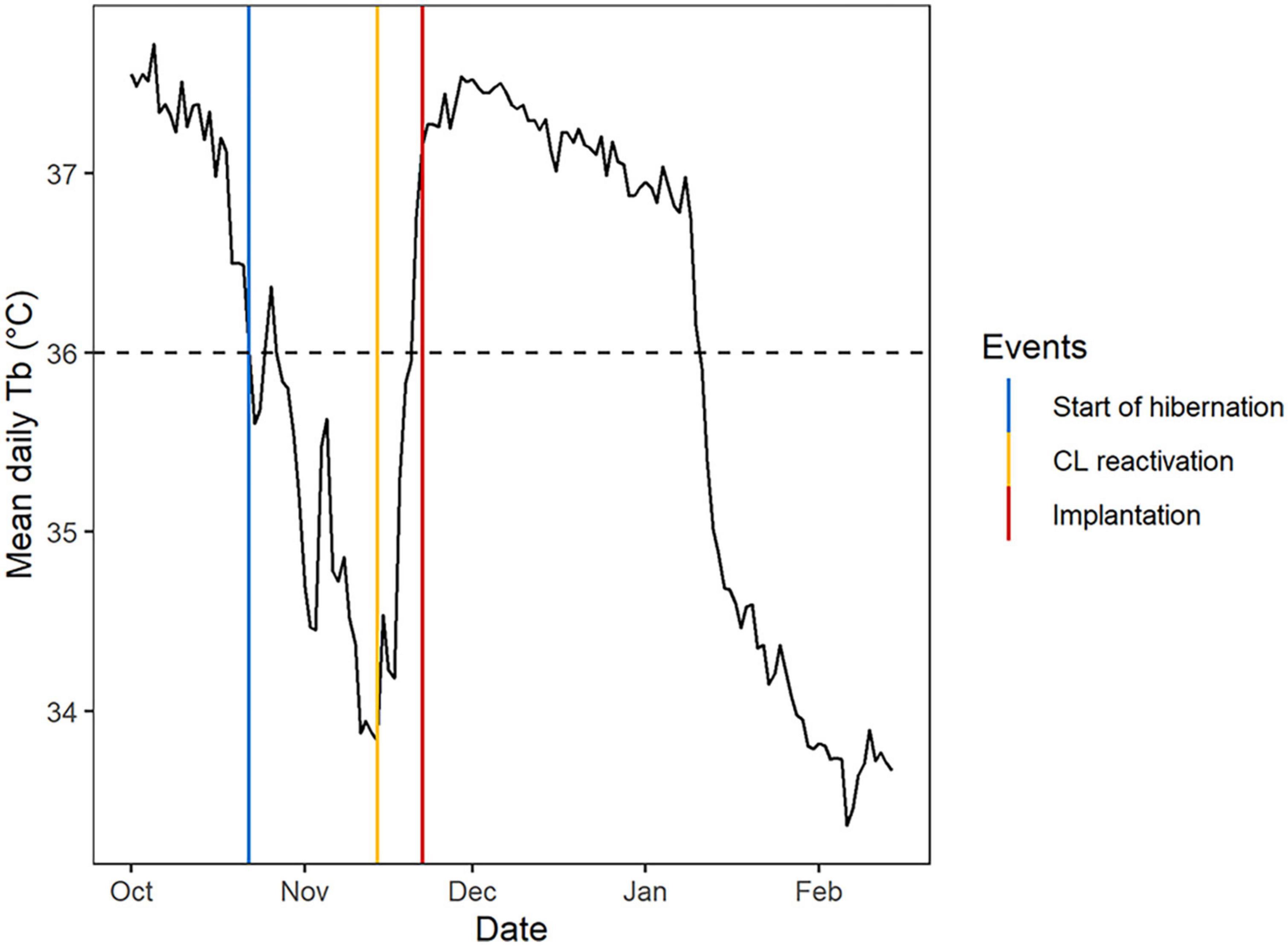
Figure 1. Example of the mean daily body temperature (Tb, °C) profile of a pregnant adult female Scandinavian brown bear in the southern area from October to March, and associated hibernation and reproductive events. The start of hibernation (vertical blue line) was defined when mean daily Tb decreased below 36°C for the first time in autumn (horizontal black dashed line). Corpus luteum (CL) reactivation (vertical yellow line) and embryo implantation (vertical red line) were defined, respectively, as a change point visually associated with the beginning and the end of the upward phase.
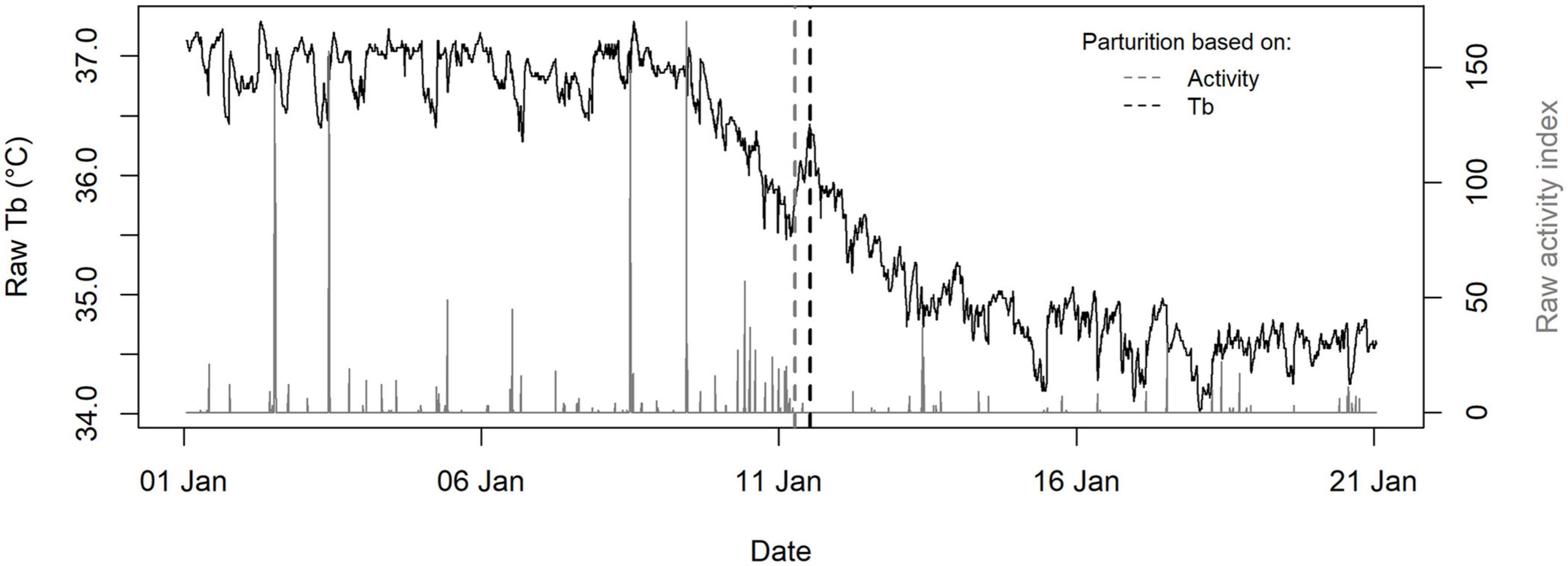
Figure 2. Example of the raw body temperature (Tb, °C, recorded every 30 min, black line) and activity (recorded every 5 min, gray line) profiles of a pregnant adult female Scandinavian brown bear from the southern area in January, and associated calculated parturition date and time (vertical dashed lines). Based on the Tb profile, parturition was defined as a change-point visually associated with a Tb surge during the downward phase (vertical black dashed line). Based on the activity profile, parturition was defined as a change-point visually associated with a drop in the activity level preceded by an increase in the activity level and/or frequency for a few hours between late December and early February (vertical gray dashed line).
We defined CL reactivation and implantation as a changepoint in November/December at which mean daily Tb began to increase and reached a plateau around 37°C, respectively (Figure 1). Based on raw Tb profiles, we defined parturition as a changepoint that was visually associated with an abrupt increase in Tb during the decreasing phase between late December and early February (Figure 2). Based on raw activity profiles, we defined parturition as a changepoint associated with a drop in the activity level preceded by a rise in the activity frequency and/or level for a few hours between late December and early February (Figure 2). We were unable to determine parturition date for one female who exited temporarily her den in early January, causing an abnormal Tb and activity profile. Additionally, we determined parturition date using activity data only for females that were not equipped with a Tb logger but whose GPS positions and offspring had been monitored. The secretory phase was defined as the time interval between CL reactivation and implantation. Gestation was defined as the time interval between implantation and parturition and was calculated using both activity and Tb profiles when available.
Hibernation events
We defined the start of hibernation as the first day in autumn when the mean daily Tb decreased below 36°C (Figure 1), which is considered as the clinical hypothermia threshold in active bears (Arnemo and Evans, 2017) and the maximum Tb observed in non-pregnant hibernating bears (Tøien et al., 2011). In addition, we determined den entry and exit dates based on GPS positions. In the southern study area, clusters of positions observed during the previous winter were visited in May after the bears had left the area and exact positions of the dens were taken with a handheld GPS (N = 21). For the dens which were not visited in spring (N = 5), we used the geographic mean of a cluster comprising at least 6 GPS positions within 100 m for at least 2 consecutive days between January and April. We chose a distance of 100 m based on the observation of individuals’ GPS data. This relatively long distance is due to low GPS accuracy when bears are underground and below snow cover, and occasional temporary den exits during the winter season. We considered that a bear had entered a denning area when it got closer than 100 m from the den position in the fall and never exited again before spring. Similarly, we considered that a bear had left a denning area when it moved further than 100 m from the den position in the following spring and never went back again. None of the bears changed den before CL reactivation or implantation, but some individuals changed den afterward (N = 4). For these females, we considered the first denning area for den entry and the denning area where parturition occurred for den exit. We were unable to determine the den exit date for one female due to lack of GPS positions in spring. The R packages « sp » (Pebesma and Bivand, 2005) et « mapview » (Appelhans, 2021) were used to analyze GPS data.
Reproductive success
In the southern study area, cubs-of-the-year presence and numbers were monitored on various occasions during the active season to determine the litter size at den emergence and subsequent cub losses. When denning sites were visited in spring after the family had left the area, signs of presence (i.e., tracks in the snow, scats and climbing marks on the trees) of cubs-of-the-year were reported. Additionally, aerial surveys were conducted from a helicopter and all females were observed at least twice during the active season (once toward the end of breeding season in June and once before the bear hunt in mid-August). In case of GPS collar failure, females were recaptured to replace the collar and the presence and number of cubs was also noted on this occasion. All families were immobilized the following year in spring for the need of other projects and the presence of cubs was also reported. Females that were not seen with cubs-of-the-year in spring were monitored again during the breeding season in order to capture unmarked males. As lactation induces anestrus in brown bears (Spady et al., 2007), the presence of a male attests that the female has reentered estrus following the loss of her entire litter. This monitoring was not conducted in the north, and consequently it was not possible to assess cub survival in this area. Following Hertel et al. (2018), a reproductive success in the south was defined when a female categorized as pregnant the previous winter managed to keep her cubs alive until the 1st of May the year they were born. Conversely, a reproductive failure was defined either when a female categorized as pregnant the previous winter was never seen with cubs after den emergence, or if she lost them before the 1st of May. All females in our dataset either lost their entire litter before the 1st of May or managed to keep them alive at least until this date. The age of cubs at den emergence was defined as the time interval between parturition and den exit.
Abiotic factors
For each bear, we determined the day length at den entry (defined as the number of hours of daylight in a 24-h period) using the mean latitude of our study areas (south: 61°N, north: 67°N) and the R packages “rgeos” (Hijmans et al., 2019) and “maptools”(Bivand and Lewin-Koh, 2021). We obtained ambient temperature (Ta) data of all Swedish weather stations from the Swedish Meteorological and Hydrological Institute (SE-601 76 Norrköpping, Sweden). We used inverse distance weighted interpolation from the R package “gstat” (Pebesma, 2004) and the function “krige” to spatially interpolate mean daily Ta to each den location based on the three closest weather stations. We then extracted the mean daily Ta at den entry for each bear in our dataset.
Statistical analysis
The statistical software R Version 1.2.5042 (R Development Core Team) was used for all statistical analyses. We used exact Wilcoxon signed rank tests to compare the calculated parturition dates and gestation lengths based on Tb or activity data (N = 13), and a Mann Whitney U-test to compare the calculated secretory phases and gestation periods between the two study areas (N = 10) (R-package “exactRankTest,” Hothorn and Hornik, 2021).
Due to the lack of information about bilberry abundance, primiparity and cub survival in the northern study area, we were not able to include all parameters in a single model selection. Therefore, we used two different models to test the effect of intrinsic and external factors on the timing of gestation, as well as a third model to evaluate their impact on reproductive success.
(1) We used linear models to assess the effect of the variables « area » (North/South), « photoperiod at den entry », « Ta at den entry », « den entry date » and « start of hibernation » on 10 reproductive events (5 from the south and 5 from the north) with CL reactivation date as response variable. We used CL reactivation instead of implantation because these variables were highly correlated (Pearson correlation coefficient: 0.9) and CL reactivation is the first event to occur in the timeline. Similarly, we used CL reactivation instead of parturition despite a lower sample size (N = 16 vs. N = 29) because we wanted to investigate whether latitude and associated environmental factors play a role in the termination of embryonic diapause of brown bears as seen in other carnivores (Heldstab et al., 2018). Therefore, we wanted to avoid any uncertainty around this date that could have been induced by the interindividual variation in gestation length. Because our dataset did not comprise young adults in the northern area and we suspected that the timing of gestation could be influenced by maternal age (Bridges et al., 2011), we included only adults in our model selection (N = 10).
(2) We used linear models to assess the effect of the variables « primiparity » (Yes/No), « age category » (Adult/Young adult) and « berry index » on 24 reproductive events from the south with parturition date as a response variable. We also included the year the female gave birth as a fixed variable to investigate any change in parturition dates over time. We used parturition instead of CL reactivation to increase our sample size (N = 29 vs. N = 16) and be able to build a model including more than one explanatory variable. Additionally, we suspected that the age and nutritional status of the female could impact to some extent the gestation length, and that the observed effect of these variables will be more pronounced on parturition date than CL reactivation date.
(3) We tested the influence of different factors on the reproductive success (Yes/No) using the previous data set and general linear models with a binomial distribution, and assessed the effects of « primiparity », « age category », « berry index » and « parturition date ». We tested the impact of parturition date rather than CL reactivation date on reproductive success because we suspected that the survival of cubs was linked to their date of birth and age at den emergence.
Dates were calculated as the day of the year with the origin set to 1 September the year the female got pregnant. Year and individual identities were not included as random factors due to the low number of repetitions (maximum 2). For each model selection, the maximum number of predictor variables included in candidate models was fixed in accordance with the sample size: one variable for the first model selection (N = 10) and two variables plus one interaction for the second and third model selections (N = 24). We did a model selection on all possible combinations of candidate models based on AICc (Akaike information criterion corrected for small sample size, R-package “AICcmodavg,” Mazerolle, 2020) and chose the model(s) for which ΔAICc with the lowest ranked model was less than 2. Finally, we inspected diagnostic plots to validate model assumptions.
Results
We were able to analyze 29 reproductive events (23 individuals) from 2011 to 2018 out of 31 (24 individuals) available. We determined den entry based on GPS positions as well as the start of hibernation, CL reactivation, implantation, and parturition based on the Tb for 16 reproductive events (15 individuals). We determined parturition date based on activity data for the 16 previous reproductive events and for 13 additional individuals. Den exit date and reproductive success were, respectively, determined for 23 and 24 reproductive events (19 individuals) from the south whose parturition date had been determined.
Estimation of reproductive events in free-ranging populations
We were able to determine parturition dates using both Tb and activity data in 13 bears (Figure 2). There was no significant difference in mean parturition date nor gestation length with the two methods (Wilcoxon signed rank test: p = 0.19, mean: 1 day, range: 2 days). Because the standard deviation (SD) was smaller with the method based on activity data (1.0 vs. 1.2 with the method based on Tb), we calculated the gestation length as ParturitionAct—ImplantationTb when possible and used ParturitionTb when activity data was not available.
CL reactivation occurred from early November to early December, implantation from mid-November to mid-December and parturition from early January to early February (Figures 1, 2 and Table 1). CL reactivation date was highly correlated with both implantation and parturition date (Pearson correlation coefficient: 0.9 in both cases). CL reactivation occurred within a range of 30 days and parturition within a range of 31 days (Table 1). However, CL reactivation and parturition occurred both within a range of 10 days in adults (Table 1). Mean gestation (± SD) was 51 days ± 1.1 and mean secretory phase (± SD) was 12 days ± 4.0 (75% between 9 and 14 days) (Table 2). In adult females, there was no significant difference in gestation and secretory phase between the northern and the southern area (Wilcoxon signed rank test, p = 0.66 in both cases). However, there was a tendency for young adults to have a more variable gestation length than adults (SD: 1.6 vs. 0.5, Table 2).
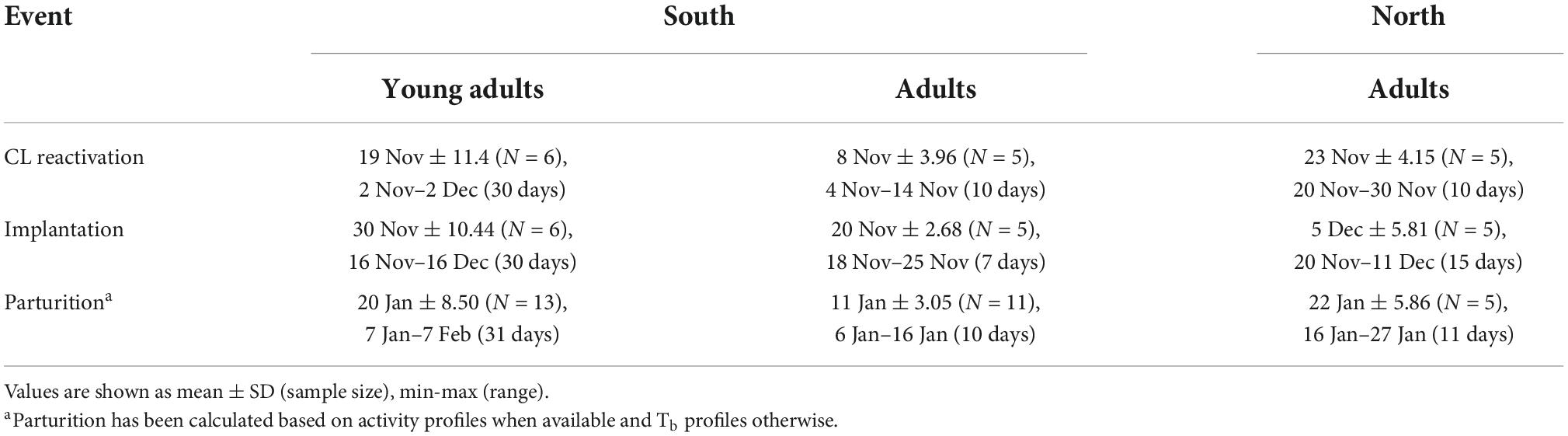
Table 1. Reproductive events of young adult and adult female brown bears in two study areas in Sweden, 2011–2018.
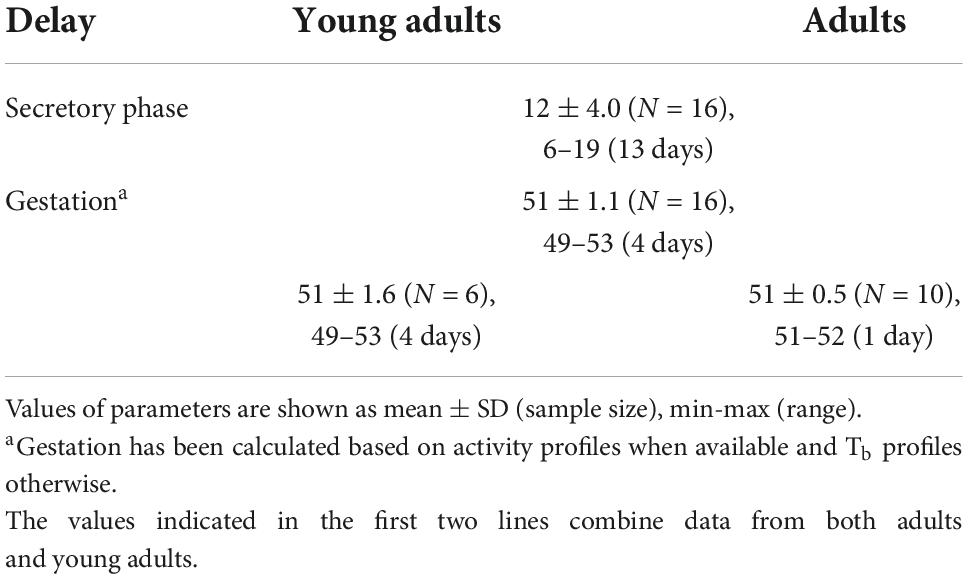
Table 2. Gestation and secretory phase periods of young adult and adult female brown bears in Sweden, 2011–2018.
Factors influencing the timing of gestation
The effects of latitude, photoperiod and Ta at den entry, as well as the behavioral and physiological start of hibernation were assessed on 10 reproductive events (5 from the south and 5 from the north) using CL reactivation as a response variable. The first two competing models were found to be within ΔAICc < 2. The top model included the latitude of the area and the second ranked model the Ta at den entry (Supplementary Table 1). Because Ta is linked to latitude and its general impact on CL reactivation appeared to be similar in both study areas (Figure 3), we decided to interpret both models separately. In the first ranked model, CL reactivation was predicted to be 14.4 days later in the north than in the south (Confidence interval (CI) = [–19.4; –9.4] days, mean ± SE: 22 November ± 2.0 vs. 8 November ± 1.7, Figure 3 and Table 3). In the second ranked model, CL reactivation was predicted to be earlier when Ta at den entry was higher, with an increase of 1°C leading to an advancement of 3.3 days in the start of gestation (CI = [–4.59; –2.04] days, Figure 3 and Table 3). Neither the start of hibernation, den entry date or day length at den entry were significantly correlated to CL reactivation (Supplementary Table 1). Furthermore, the time interval between den entry and CL reactivation was highly variable among individuals in both areas (range: 20 days in the north and 23 days in the south).
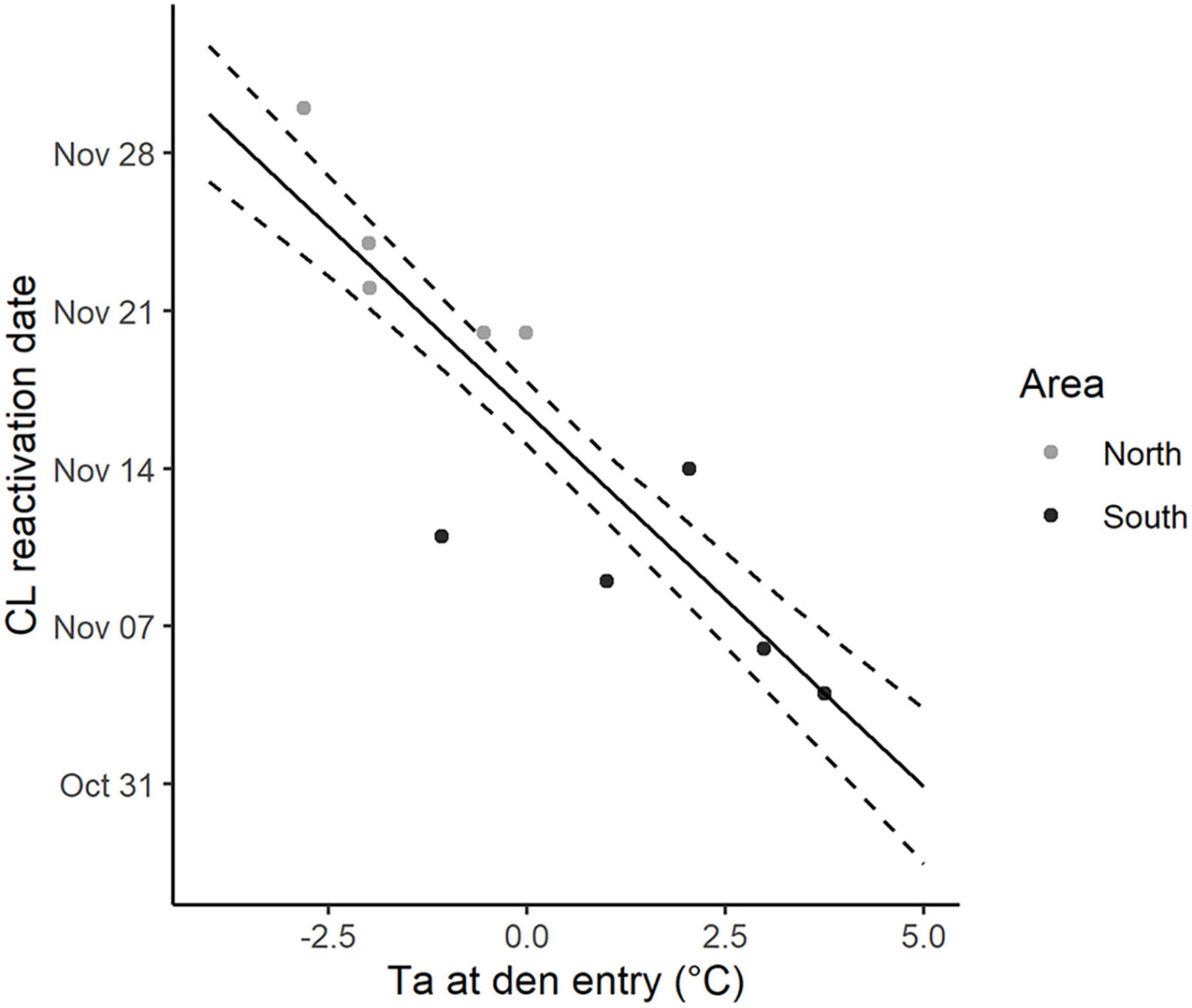
Figure 3. Dates of corpus luteum (CL) reactivation for pregnant female Scandinavian brown bears from two study areas in Sweden along the gradient of ambient temperature (Ta,°C) at den entry from 2011 to 2017. Observed values (filled dots) are colored according to the study area (northern area: light gray, N = 5; southern area: dark gray, N = 5). Predicted values (solid lines) are shown irrespective of the study area. Dashed lines represent the 95% confidence interval.
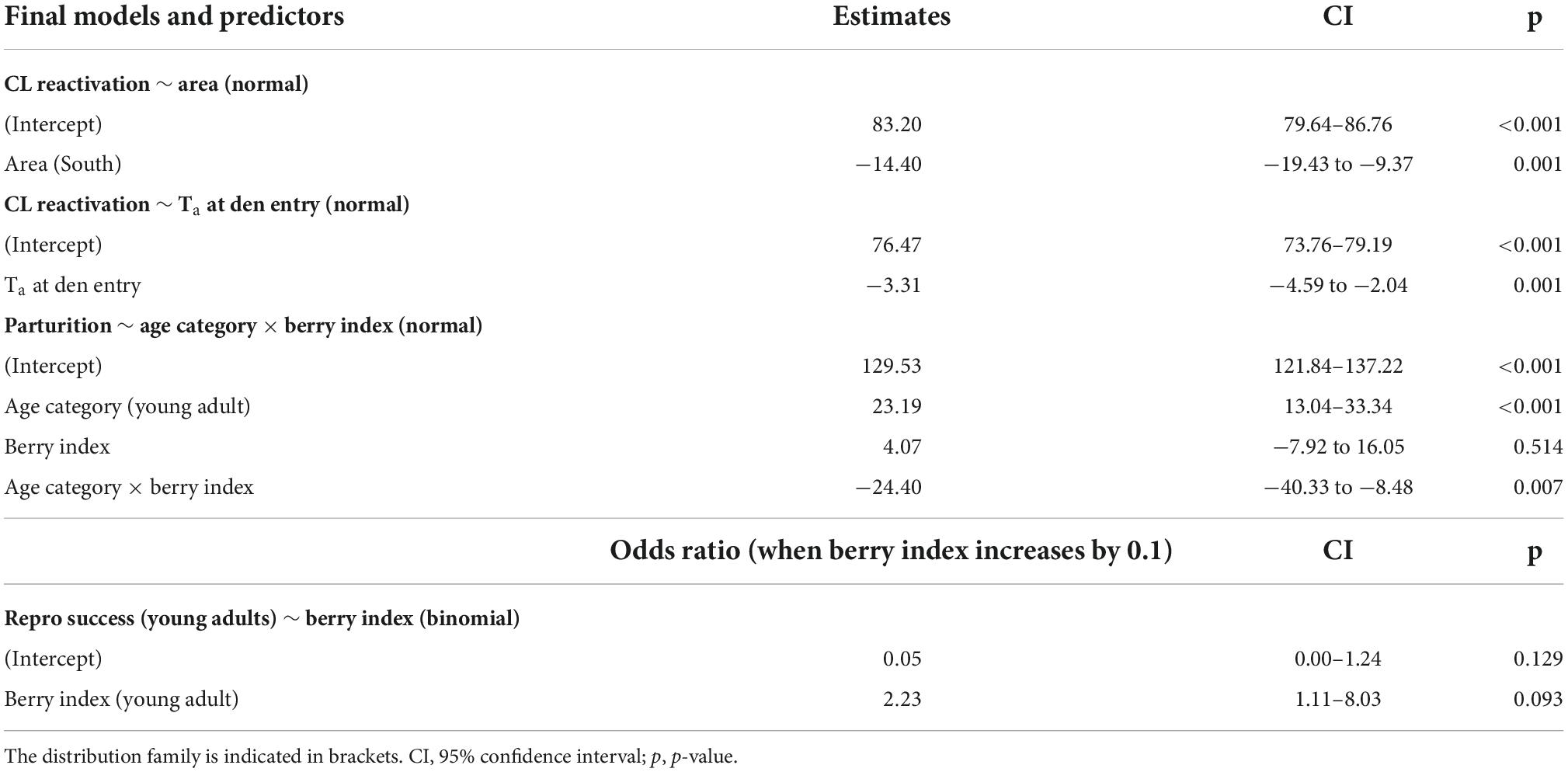
Table 3. Estimated parameters of the predictor variables included in the final models evaluating the factors influencing the timing of gestation and the reproductive success of Scandinavian brown bear females.
The effects of primiparity, age and bilberry abundance were assessed in 24 reproductive events from the south (11 adults and 13 young adults) using parturition date as a response variable. The top model included age category, berry index and their interaction (Supplementary Table 1). In young adults, a low berry abundance the previous year was associated with a later parturition, with a predicted delay of 21 days (CI = [–40.3; –8.5] days) when the berry index decreased from 1 to 0 (mean ± SE: 11 January ± 2.6 when berry index = 1 vs. 1st February ± 4.0 when berry index = 0, Figure 4 and Table 3). In contrast, adult females were not affected by this parameter (mean ± SE: 12 January ± 2.9 when berry index = 1 vs. 8 January ± 3.6 when berry index = 0, Figure 4). There was no significant change in parturition dates over time (Supplementary Table 1).
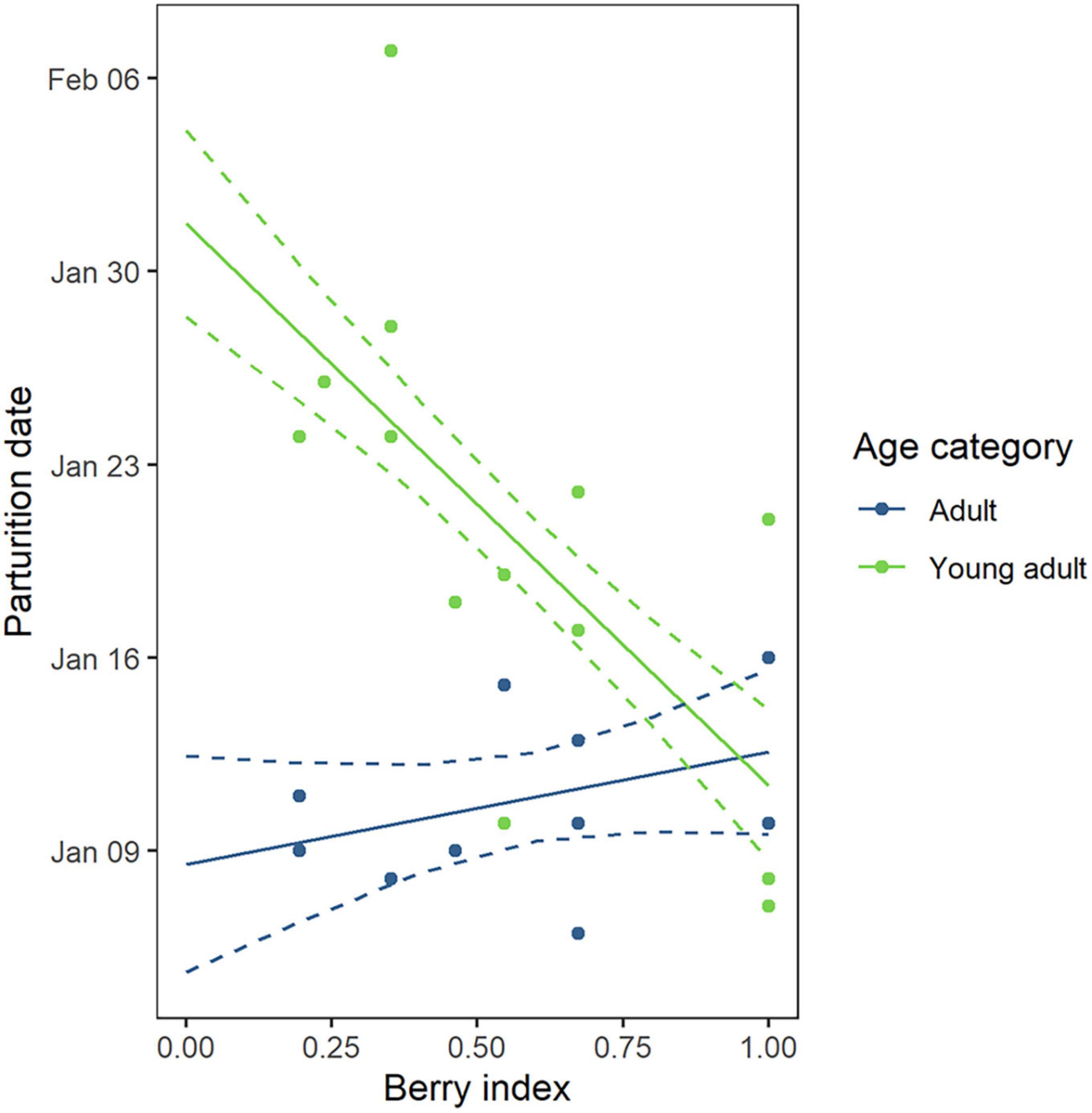
Figure 4. Predicted parturition date for female Scandinavian brown bears (N = 24) from the southern study area along the gradient of bilberry abundance in late summer. Estimates (solid lines) and observations (filled dots) during 2012–2018 are shown for young adults (green, N = 13) and adults (blue, N = 11). Dashed lines represent the 95% confidence interval.
Factors influencing the reproductive success
The factors influencing the reproductive success were tested in 24 reproductive events from the south (11 adults and 13 young adults). The top model included age, berry index and their interaction. However, the model coefficients were not estimable due to a lack of variability in adults, as all adults in our dataset successfully reproduced regardless of berry abundance the previous year (berry index ranging from 0.19 to 1, Supplementary Figure 2). We therefore proceeded in two stages:
(1) We compared the reproductive success of adults and young adults using an exact Wilcoxon signed rank test. Adults had a higher reproductive success than young adults regardless of bilberry abundance (100 vs. 61%, Wilcoxon signed rank test: p = 0.04).
(2) Then, we tested the effect of berry abundance in young adults only (N = 13) with a logistic regression (Supplementary Table 1). This model predicted a lower probability of successful breeding in young adults when bilberry production was low the previous year, with an odd ratio of 2.23 when berry index increases by 0.1 (CI = [1.1; 8.0], Table 3 and Figure 5).
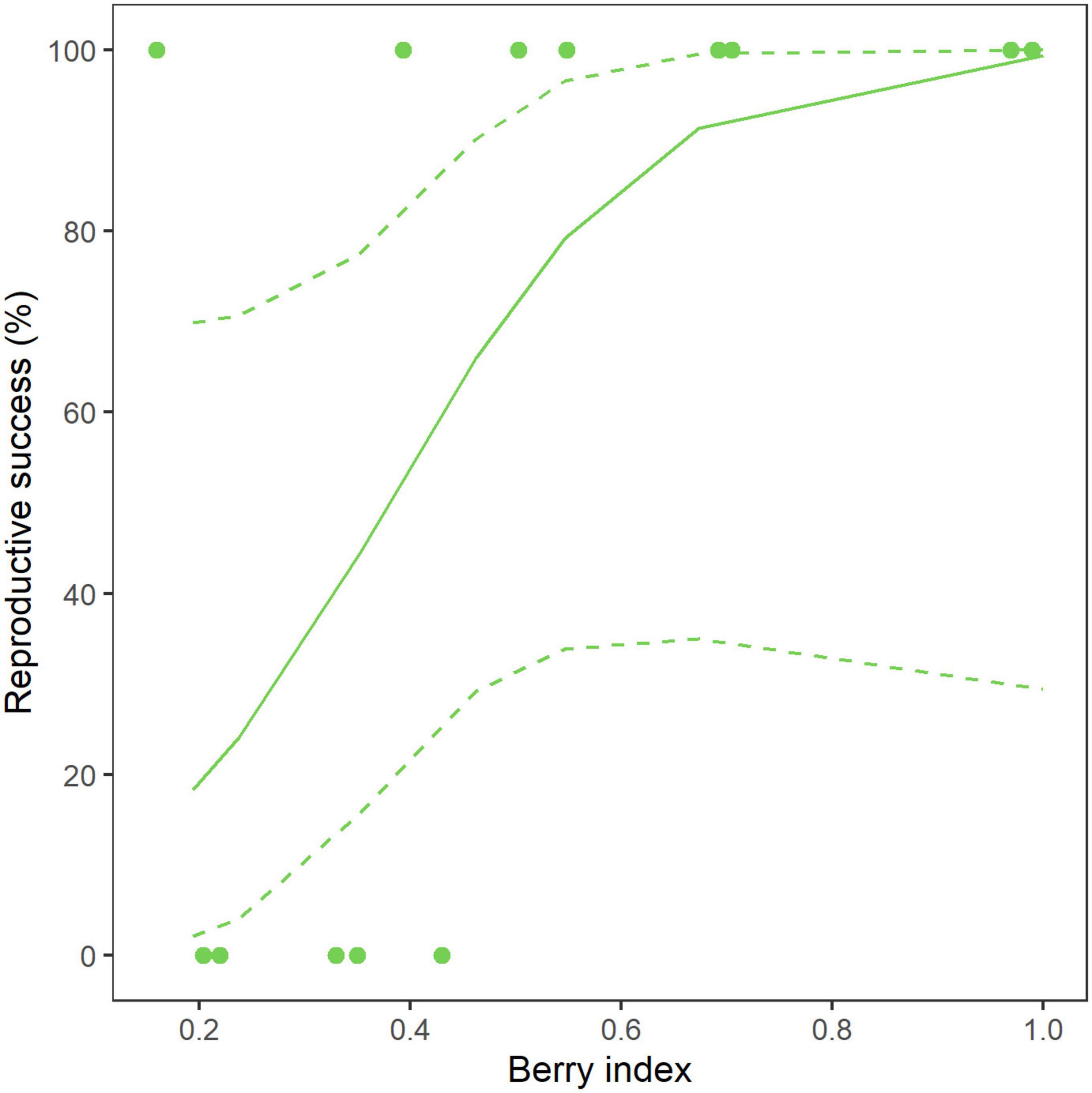
Figure 5. Predicted probability to reproduce successfully for young adult Scandinavian brown bears (N = 13) from the southern study area along the gradient of bilberry abundance in late summer during 2012–2018. The solid line represents the model predictions and the dashed lines the associated 95% confidence interval. The observations are shown as filled dots. We did not include adults in our model selection for predicting reproductive success because all adults had reproduced successfully in our dataset.
Because some of the cubs that were never observed in spring might have died in the den, we were unable to test the impact of den exit and age of cubs at den emergence on reproductive success in the previous model. However, we observed that when berry abundance was below-average the previous year, young adults spent less time in the den after parturition (mean ± SD: 88.4 days ± 18.7 vs. 117.0 days ± 7.8) and emerged earlier (mean ± SD: 27 April ± 14.0 vs. 7 May ± 14.9) than other females. In addition, those that emerged before the 1st of May were not seen with cubs in spring (Supplementary Figure 3). The cubs observed in spring were minimum 105 days old at den emergence, but this age varied about 1 month (mean: 117 days, SD: 7.7, range: 26, min: 105, max: 131, Supplementary Figure 3). Finally, we observed that there was a tendency for den exit to be later when parturition was later (Supplementary Figure 4).
Discussion
To date, the reproductive biology and phenology of ursids remain poorly understood, and few studies have investigated these aspects in the wild (but see Friebe et al., 2014; Appleton et al., 2018; Mesa-Cruz, 2018; García-Rodríguez et al., 2020). The goal of this study was to provide new insights into the factors influencing the timing of gestation and the reproductive success of free-ranging Scandinavian brown bears as well as the potential impacts of climate change. Our analyses produced three main results. First, CL reactivation was later in the northern area than in the southern area and occurred earlier when ambient temperature at den entry was higher. Second, parturition was delayed in young adults when bilberry abundance was low the previous year. Third, young adults had a lower reproductive success than adults and their probability to reproduce successfully was dependent on bilberry abundance during the year of mating.
Estimation of reproductive events in free-ranging populations
Our study confirms that parturition can be determined using both Tb and activity data, as no significant difference was found between the two methods. Whereas Friebe et al. (2014) found a significantly longer gestation length using activity data compared to Tb data (activity: 63.3 days, Tb: 55.8 days), we did not find any significant difference between the two methods and obtained the same mean gestation length (i.e., 51 days, Table 2). Because the vast majority of placental mammals have a limited intra-specific gestation variation, the small standard errors that we obtained with Tb and activity data suggests that both of these methods are reliable.
CL reactivation occurred from early November to early December, implantation from mid-November to mid-December, and parturition from early January to early February (Table 1) in accordance with previous findings (Wimsatt, 1963; Tsubota et al., 1987; Friebe et al., 2014). However, the average gestation length in our study (i.e., 51 days, Table 2) was shorter than what was previously reported in the literature (i.e., 56–60 days, Foresman and Daniel, 1983; Tsubota et al., 1987; Friebe et al., 2014). This difference is most likely due to the definitions that we used. The duration of gestation in bears has been mainly estimated using changes in progesterone levels, and the sharp increase in progesterone in late November-early December has been recurrently associated with implantation (Foresman and Daniel, 1983; Tsubota et al., 1987). However, concomitant changes in CL morphology and volume (Wimsatt, 1963; Tsubota and Kanagawa, 1993; Tsubota et al., 2001) suggest that this hormonal increase is associated with CL reactivation rather than embryo implantation. Therefore, previous studies did not consider the existence of a secretory phase during which the uterus prepares for implantation. Similarly, Friebe et al. (2014) defined implantation as the date when Tb increased above the mean daily Tb during the denning period, approximately mid-way of the upward phase in early December, in the middle of the supposed secretory phase. This phase was on average 12 days in our study (range: 6–19 days, Table 2). Accounting for this phase, our calculated gestation length is in line with previous studies and associated with a lower standard error [SD: 1.1 days vs. 2.0 days in Friebe et al. (2014)].
Factors influencing the timing of gestation
The termination of embryonic diapause was on average 14.4 days later in the north than in the south (Table 3 and Figure 3), a result in line with previous reports in which high latitudes were associated with a longer embryonic diapause in carnivores (Heldstab et al., 2018). Because females in the northern study area tend to emerge later from their den than in the southern study area (Manchi and Swenson, 2005), a later parturition might ensure that they do not compromise their own survival while both nursing their cubs in the den and fasting.
Latitude is associated with many environmental factors including photoperiod, Ta, precipitation, and food availability. Our results suggest that Ta around den entry influences the end of the embryonic diapause in Scandinavian brown bears, with an increase of 1°C leading to an advancement of 3.3 days (Table 3 and Figure 3), although this value should be interpreted with caution as our sample size was limited (N = 10, 5 from the south and 5 from the north). A similar impact has been reported in wild ungulates, with an increase in Ta in autumn leading to an advancement of calving dates (reviewed in Kerby and Post, 2013; Paoli et al., 2020). However, these correlations do not mean that animals use Ta as a proximal cue and may rather represent an energetic constraint or an indirect impact on other environmental factors. Kerby and Post (2013) identified two ways in which the interannual variation in climatic conditions could influence calving timing in wild ungulates: (1) Through a direct impact on the physical condition of reproducing females before conception and during pregnancy (i.e., limited access to food under snow and increased amount of energy spent in thermoregulation and locomotion) and (2) through an indirect impact on the timing of plant growth and forage availability when females build fat stores to support gestation and lactation costs. In Scandinavia, brown bears build fat stores from late summer to den entry in the fall mostly foraging on bilberries (Stenset et al., 2016), and Hertel et al. (2018) identified that a longer growing season had a positive effect on bilberry production and therefore maternal body mass in the fall. Furthermore, it has been reported that parturition (and therefore implantation) occurs earlier in female bears in better physical condition (Robbins et al., 2012; Mesa-Cruz, 2018). Therefore, our result could reflect the impact of thermal conditions on forage availability in the fall and thus maternal fat stores that will support gestation and lactation costs. Milder weather conditions in autumn could increase berry availability for bears and thus maternal body condition prior to hibernation, leading to an advancement in parturition dates by shortening the embryonic diapause. There was no significant change in parturition dates over time in our dataset (Supplementary Table 1). But as our study was only conducted over 7 years, it is possible that a longer period of monitoring would have yielded to a different result.
Although photoperiod is recognized as the main driver of the termination of embryonic diapause in non-hibernating carnivore species (Murphy and Fenelon, 2020), neither day length at den entry (the last day the female had potentially full access to this cue) nor the date of den entry itself (which is associated with a sharp decrease in perceived light) had a significant effect on CL reactivation (Supplementary Table 1). Furthermore, if photoperiod was the main cue synchronizing the end of embryonic diapause in ursids, implantation and parturition dates should occur in a narrow time range. However, these two events ranged over 1 month in our dataset (Table 2). This result supports the fact that photoperiod may not play a major role in the timing of implantation in contrast to mating (Appleton et al., 2018; García-Rodríguez et al., 2020).
We did not consider relevant to test the impact of snow depth on the start of gestation as most females entered their den before the first snow fall and the layer covering the den between den entry and CL reactivation was too thin to have an ecological impact in terms of thermal insulation and light-shielding [mean: 1.76 cm whereas a layer of 30 cm is necessary to block sunlight and create an insulated layer (Evernden and Fuller, 1972; Baltensperger et al., 2017)].
Neither the date of den entry nor the date of the physiological start of hibernation influenced CL reactivation in our study (Supplementary Table 1). Although this lack of significance might be attributed to our sample size, this result is in accordance with Friebe et al. (2014) who did not find any correlation between parturition and the start of hibernation in 46 females based on activity data. Therefore, these two physiological events appear to be independent. This is supported by the existence of a delayed implantation in bear species that do not hibernate (i.e., the spectacle bear Tremactos ornatus and the sloth bear Melursus ursinus).
Finally, parturition was later in young adults (i.e., females ≤ 7 years old) when berry production was low the previous year (Table 3 and Figure 4), which is consistent with earlier studies reporting a later parturition in young females (Bridges et al., 2011; Friebe et al., 2014) and an earlier parturition in females with superior body condition (Robbins et al., 2012; Mesa-Cruz, 2018). Young adults may lack experience to build sufficient fat stores when berry abundance is low and must allow energy for their own growth during hyperphagia in addition to storing fat. Therefore, in case of low berry production, they may not reach an optimal body condition prior to hibernation and the diapause could be lengthened leading to a later parturition. Robbins et al. (2012) proposed a similar explanation and hypothesized that the wide inter-individual variation in implantation dates reported in the European brown bear (Friebe et al., 2014) and American black bear (Mesa-Cruz, 2018) might be a strategy for pregnant females to adapt the onset of gestation in response to their fat stores. In our study, implantation range was 30 days in the southern area in accordance with Friebe et al. (2014) but occurred within a 10-day period in adults (Table 2). As previous studies were conducted on individuals of various age, young adult females in below average body condition might have widened the implantation range (Friebe et al., 2014; Mesa-Cruz, 2018). We suggest that in such case, a later parturition could be a consequence of insufficient fat stores rather than an energy strategy, as most of these females lost their cubs early in spring in our dataset. However, in females with adequate fat stores, CL reactivation occurs in a narrow time range due to a trade-off between the time spent in the den lactating while fasting and sufficient age of cubs at den emergence.
Factors influencing the reproductive success
Zedrosser et al. (2009) reported a higher probability of cub loss for primiparous females compared to multiparous females in our population, but we did not find this effect in our dataset comprising 6 primiparous females (Supplementary Table 1). However, we observed an effect of age with young adults having a lower reproductive success than adults. Young adults may be less experienced at keeping their cubs alive, and a more variable gestation length (Table 2) suggests that they could be prone to reproductive abnormalities (e.g., abortion, preterm delivery or prolonged gestation). Furthermore, the characteristics of the den could influence the reproductive success. Older bears excavate better-fitting and more energy-efficient dens compared to younger and less experienced bears (Shiratsuru et al., 2020), and the probability for a pregnant female to use an excavated anthill, which provides a better thermal insulation than other den types, increases with age (Mannaart, 2016). Therefore, adult females could have reduced heat and fat losses during hibernation compared to young adults, leading to a better body condition at den emergence (Shiratsuru et al., 2020) and therefore a higher reproductive success.
In addition, young adults had a lower reproductive success when berry abundance was low the previous summer (Table 3 and Figure 5) and emerged on average 29 days earlier than other females when bilberry abundance was below average (Supplementary Figure 3). Using body mass in spring in the same population, Hertel et al. (2018) found that lightweight females (≤ 80 kg) had a lower reproductive success when berry abundance was low the year of mating. This finding is in line with our result since all young adults weighed less than 80 kg in our dataset. We suggest that young adults’ fat stores might not be sufficient after a low berry production in autumn to support lactation until early May despite a later parturition. Therefore, they may have to emerge sooner to forage while their cubs are still young and vulnerable to harsh environmental conditions, predation, and infanticide. This hypothesis is consistent with previous studies reporting that grizzly females that lost a least one cub during their first summer had a lower amount of fat at den entry but a similar fat mass at den emergence when compared to females that successfully raised an entire litter (Keay et al., 2018). Our study did not identify an effect of bilberry production on adults’ parturition date and reproductive success, and we hypothesize that fully grown adult females are less susceptible to yearly variations in food abundance and can store enough energy for reproduction in most years. However, we did not have direct access to the individual’s body condition at den entry nor during gestation as pregnant females are not captured during these periods both for ethical and safety reasons. And although bilberries account for most of the brown bear diet in the fall (Stenset et al., 2016) and this metric has been shown to be a good proxy for the populational nutritional status of female bears during this period (Hertel et al., 2018), other factors could potentially impact the individuals’ body condition.
When analyzing the GPS positions to determine den exit dates in spring, we noticed two different movements patterns in unsuccessful females. Some females stayed in the vicinity of the den (i.e., within a few hundred meters) for several days, whereas others traveled more than 1 km on the day of den emergence. As reported in female grizzlies (Gardner et al., 2014), we suggest that the first category emerged with cubs early in spring and that these cubs died soon after due to harsh environmental conditions, malnutrition or infanticide, whereas the second lost their cubs in the den and emerged earlier as a consequence. The latter situation could be due to multiple reasons including gestation abnormalities (e.g., fetal loss, late abortion), neonatal death (e.g., due to insufficient milk production), den abandonment (due to natural or anthropic disturbances) or pseudopregnancy, a physiological state that has been reported in captive bears (Shimozuru et al., 2013).
In successful females, cubs were ≥ 105 days old at den emergence (Supplementary Figure 3) and we observed that there was a tendency for den exit to be later when parturition was later (Supplementary Figure 4). These results suggest that females with sufficient fat stores exit the den when their cubs are at least 3.5 months old. However, the age of cubs at den emergence was variable with a range of 26 days in our dataset (Supplementary Figure 3). Females in superior body condition could lactate longer in the den as a strategy for their cubs to be older and heavier at den emergence, two factors that have been associated with a better survival (Hertel et al., 2018; Keay et al., 2018). Another explanation would be that the age of cubs is not the only driver of den exit. González-Bernardo et al. (2020a) reported that den exit was influenced by maximum Ta in spring in European brown bear females with cubs-of-the-year. Similarly, Keay et al. (2018) reported a similar fat content in parturient grizzly females at den emergence, which suggests that a minimum threshold of fat stores could drive den exit.
Potential impacts of climate change on the Scandinavian brown bear population
Previous studies have reported an earlier den exit in several bear populations due to increasing Ta in spring (González-Bernardo et al., 2020a,b). Similarly, our results suggest that global warming could impact the reproductive phenology of Scandinavian brown bears, as a rise in Ta in the fall could lead to an earlier start of gestation and therefore an advancement in parturition dates. But these events may not shift at the same pace, which could result in a temporal mismatch between hibernation, reproduction, and food availability in spring with dramatic consequences for reproductive success (Parmesan, 2007). A faster advancement in parturition dates might force parturient females to leave their den sooner to forage again, leading to an earlier start of the active period when protein food sources used to replenish maternal reserves and produce milk are scarce (López-Alfaro et al., 2013) and environmental conditions are severe for cubs. Conversely, a slower advancement in parturition dates would reduce the period inside the den after parturition and cause cubs to be younger and thus more vulnerable to predation or infanticide at den emergence.
Furthermore, recent studies suggest that bilberry abundance will be affected by global changes in Sweden, but it is still unclear if it will be in a positive or negative manner (Hertel et al., 2018). As Scandinavian brown bears rely heavily on this resource to build fat stores prior to hibernation (Stenset et al., 2016), future changes in bilberry production could negatively affect the reproductive success of females aged under 7 years old. But older females could also be affected by this parameter in the future if berry production was to fall below current minimum standards. Therefore, a decrease in berry production combined with a mismatch between parturition and hibernation could impact the Scandinavian brown bear population dynamic in the future.
However, it remains difficult to predict the impact of climate change on free-ranging populations and brown bears could potentially adapt to these environmental pressures by phenotypic plasticity (Hetem et al., 2014). As an opportunistic omnivore, the brown bear could find another food source during hyperphagia to store sufficient fat reserves before hibernation despite a decrease in berry production. Additionally, delayed implantation may allow brown bears to track at least partially the rapid changes in their environment (Robbins et al., 2012) in contrast to other mammals for which parturition time might be less flexible due to constraints on gestation length (Kerby and Post, 2013). Some authors (summarized in González-Bernardo et al., 2020b) even suggest that bears could benefit from climate change. An earlier peak in plant productivity in spring and/or a higher or longer bilberry productivity in the fall would lengthen the active period and result in better body condition pre- and post-hibernation, with a positive impact on females’ reproductive success (Ozgul et al., 2010). Therefore, the duration of hibernation could be reduced without a cost for offspring. Future studies will be necessary to determine to what extent the brown bear is able to adapt to rapid and unpredictable changes in its environment.
Data availability statement
The raw data supporting the conclusions of this article will be made available by the authors, without undue reservation.
Ethics statement
The animal study was reviewed and approved by the Swedish Ethical Committee on Animal Research, Uppsala, Sweden (C18/15) and the Swedish Environmental Protection Agency, Stockholm, Sweden (NV-00741-18).
Author contributions
AE, AT, BF, and LL conceived the ideas and designed the methodology. AE, AF, AT, BF, JA, JK, and O-GS (bear captures) as well as AGH (berry abundance) contributed to the fieldwork and data collection. AF, JA, and O-GS provided equipment. AF, AT, and BF did data management and submission to WRAM (wireless remote animal monitoring, SLU). AGH (berry index), AT (environmental variables), and LL carried out data analysis. AT, BF, and EG-F provided the supervision and advice in statistical modeling. LL did preparation of figures and drafting of the manuscript. All authors participated in revisions of the manuscript and gave final approval for publication.
Funding
The Scandinavian Brown Bear Research Project was funded by the Norwegian Directorate for Nature Management, the Swedish Environmental Protection Agency, the Swedish Association for Hunting and Wildlife Management, and the Research Council of Norway. LL, AT, BF, AE, and JA were supported by the Inland Norway University of Applied Sciences. AGH was supported by the German Science Foundation (HE 8857/1-1).
Conflict of interest
The authors declare that the research was conducted in the absence of any commercial or financial relationships that could be construed as a potential conflict of interest.
Publisher’s note
All claims expressed in this article are solely those of the authors and do not necessarily represent those of their affiliated organizations, or those of the publisher, the editors and the reviewers. Any product that may be evaluated in this article, or claim that may be made by its manufacturer, is not guaranteed or endorsed by the publisher.
Supplementary material
The Supplementary Material for this article can be found online at: https://www.frontiersin.org/articles/10.3389/fevo.2022.1045331/full#supplementary-material
References
Appleton, R. D., Van Horn, R. C., Noyce, K. V., Spady, T. J., Swaisgood, R. R., and Arcese, P. (2018). Phenotypic plasticity in the timing of reproduction in Andean bears. J. Zool. 305, 196–202. doi: 10.1111/jzo.12553
Arnemo, J. M., and Evans, A. L. (2017). Biomedical protocols for free-ranging brown bears, wolves, wolverines and lynx. Norway: Inland Norway University of Applied Sciences, Evenstad.
Baltensperger, A. P., Morton, J. M., and Huettmann, F. (2017). Expansion of American marten (Martes americana) distribution in response to climate and landscape change on the Kenai Peninsula. Alaska. J. Mammal. 98, 703–714. doi: 10.1093/jmammal/gyx011
Bieber, C., Cornils, J. S., Hoelzl, F., Giroud, S., and Ruf, T. (2017). The costs of locomotor activity? Maximum body temperatures and the use of torpor during the active season in edible dormice. J. Comp. Physiol. B. 187, 803–814. doi: 10.1007/s00360-017-1080-y
Bridges, A. S., Fox, J. A., Olfenbuttel, C., and Vaughan, M. R. (2004). American black bear denning behavior: Observations and applications using remote photography. Wildl. Soc. Bull. 32, 188–193. doi: 10.2193/0091-7648200432[188:ABBDBO]2.0.CO;2
Bridges, A. S., Vaughan, M. R., and Fox, J. A. (2011). American black bear estrus and parturition in the alleghany mountains of Virginia. Ursus 22, 1–8. doi: 10.2192/URSUS-D-10-00008.1
Bronson, F. H. (2009). Climate change and seasonal reproduction in mammals. Philos. Trans. R. Soc. B 364, 3331–3340. doi: 10.1098/rstb.2009.0140
de Mouzon, J., Testart, J., Lefevre, B., Pouly, J.-L., and Frydman, R. (1984). Time relationships between basal body temperature and ovulation or plasma progestins. Fertil. Steril. 41, 254–259. doi: 10.1016/S0015-0282(16)47600-4
Evernden, L. N., and Fuller, W. A. (1972). Light alteration caused by snow and its importance to subnivean rodents. Can. J. Zool. 50, 1023–1032. doi: 10.1139/z72-137
Foresman, K. R., and Daniel, J. C. (1983). Plasma progesterone concentrations in pregnant and nonpregnant black bears (Ursus americanus). Reproduction 68, 235–239. doi: 10.1530/jrf.0.0680235
Friebe, A., Evans, A. L., Arnemo, J. M., Blanc, S., Brunberg, S., Fleissner, G., et al. (2014). Factors affecting date of implantation, parturition, and den entry estimated from activity and body temperature in free-ranging brown bears. PLoS One 9:e101410. doi: 10.1371/journal.pone.0101410
García-Rodríguez, A., Rigg, R., Elguero-Claramunt, I., Bojarska, K., Krofel, M., Parchizadeh, J., et al. (2020). Phenology of brown bear breeding season and related geographical cues. Eur. Zool. J. 87, 552–558. doi: 10.1080/24750263.2020.1801866
Gardner, C. L., Pamperin, N. J., and Benson, J. F. (2014). Movement patterns and space use of maternal grizzly bears influence cub survival in Interior Alaska. Ursus 25, 121–138. doi: 10.2192/URSUS-D-14-00015.1
Gervasi, V., Brunberg, S., and Swenson, J. E. (2006). An individual-based method to measure animal activity levels: A test on brown bears. Wildl. Soc. Bull. 34, 1314–1319. doi: 10.2193/0091-7648200634[1314:AIMTMA]2.0.CO;2
González-Bernardo, E., Bombieri, G., del Mar Delgado, M., and Penteriani, V. (2020a). The role of spring temperatures in the den exit of female brown bears with cubs in southwestern Europe. Ursus 31, 1–11. doi: 10.2192/URSUS-D-19-00015.1
González-Bernardo, E., Russo, L. F., Valderrábano, E., Fernández, Á, and Penteriani, V. (2020b). Denning in brown bears. Ecol. Evol. 10, 6844–6862. doi: 10.1002/ece3.6372
Harlow, H. J., Lohuis, T., Grogan, R. G., and Beck, T. D. I. (2002). Body mass and lipid changes by hibernating reproductive and non-reproductive black bears (Ursus Americanus). J. Mammal. 83:6. doi: 10.1644/1545-15422002083<1020:BMALCB<2.0.CO;2
Harshyne, W., Diefenbach, D., Alt, G., and Matson, G. (1998). Analysis of error from cementum-annuli age estimates of known-age Pennsylvania black bears. J. Wild. Manag. 62, 1281–1291. doi: 10.2307/3801992
Heldstab, S. A., Müller, D. W. H., Graber, S. M., Bingaman Lackey, L., Rensch, E., Hatt, J.-M., et al. (2018). Geographical origin, delayed implantation, and induced ovulation explain reproductive seasonality in the carnivora. J. Biol. Rhythms 33, 402–419. doi: 10.1177/0748730418773620
Hertel, A. G., Bischof, R., Langval, O., Mysterud, A., Kindberg, J., Swenson, J. E., et al. (2018). Berry production drives bottom-up effects on body mass and reproductive success in an omnivore. Oikos 127, 197–207. doi: 10.1111/oik.04515
Hetem, R. S., Fuller, A., Maloney, S. K., and Mitchell, D. (2014). Responses of large mammals to climate change. Temperature 1, 115–127. doi: 10.4161/temp.29651
Hijmans, R., Williams, E., and Vennes, C. (2019). Geosphere: Spherical trigonometry. Vienna: R Foundation for Statistical Computing.
Hothorn, T., and Hornik, K. (2021). Exact distributions for rank and permutation tests. R package, version 0.8-32.
IPCC (2021). “Climate change 2021: The physical science basis,” in Contribution of working group I to the sixth assessment report of the intergovernmental panel on climate change, eds V. Masson-Delmotte, P. Zhai, A. Pirani, S. L. Connors, C. Péan, S. Berger, et al. (Cambridge: Cambridge University Press).
Keay, J. A., Robbins, C. T., and Farley, S. D. (2018). Characteristics of a naturally regulated grizzly bear population. J. Wild. Manag. 82, 789–801. doi: 10.1002/jwmg.21425
Kerby, J., and Post, E. (2013). “Chapter 25: Reproductive phenology of large mammals,” in Phenology: An integrative environmental science, ed. M. D. Schwartz (Springer: University of Wisconsin-Milwaukee Milwaukee), 467–479. doi: 10.1007/978-94-007-6925-0
Killick, R., and Eckley, I. A. (2014). changepoint: An R package for changepoint analysis. J. Stat. Softw. 58, 1–19.
Killick, R., Fearnhead, P., and Eckley, I. A. (2012). Optimal detection of changepoints with a linear computational cost. J. Am. Stat. Assoc. 107, 1590–1598.
Kordek, W. S., and Lindzey, J. S. (1980). Preliminary analysis of female reproductive tracts from Pennsylvania black bears. Bears Biol. Manag. 4:159. doi: 10.2307/3872861
Kumar, V., Lammers, F., Bidon, T., Pfenninger, M., Kolter, L., Nilsson, M. A., et al. (2017). The evolutionary history of bears is characterized by gene flow across species. Sci. Rep. 7:46487. doi: 10.1038/srep46487
Laburn, H. P., Faurie, A., Goelst, K., and Mitchell, D. (2002). Effects on fetal and maternal body temperatures of exposure of pregnant ewes to heat, cold, and exercise. J. Appl. Physiol. 92, 802–808. doi: 10.1152/japplphysiol.00109.2001
Langvall, O., and Ottosson Löfvenius, M. (2021). Long-term standardized forest phenology in Sweden: A climate change indicator. Int. J. Biometeorol. 65, 381–391. doi: 10.1007/s00484-019-01817-8
López-Alfaro, C., Robbins, C. T., Zedrosser, A., and Nielsen, S. E. (2013). Energetics of hibernation and reproductive trade-offs in brown bears. Ecol. Modell. 270, 1–10. doi: 10.1016/j.ecolmodel.2013.09.002
Manchi, S., and Swenson, J. E. (2005). Denning behaviour of Scandinavian brown bears Ursus arctos. Wildl. Biol. 11, 123–132. doi: 10.2981/0909-6396200511[123:DBOSBB]2.0.CO;2
Mannaart, A. H. I. (2016). Denning ecology of Scandinavian brown bears (Ursus arctos) in a dynamic landscape. [Master’s thesis]. Ås, Norway: Norwegian University of Life Sciences.
Mazerolle, M. J. (2020). Model selection and multimodel inference based on (Q)AIC(c). R package, version 2.3-1.
McAllan, B. M., and Geiser, F. (2014). Torpor during reproduction in mammals and birds: Dealing with an energetic conundrum. Integr. Comp. Biol. 54, 516–532. doi: 10.1093/icb/icu093
Mesa-Cruz, J. B. M. (2018). Assessment of physiological challenges in overwintering American black bears (Ursus americanus): Active gestation, neonatal growth, and skeletal muscle conservation. [Doctoral dissertation]. Blacksburg (VA): Faculty of the Virginia Polytechnic Institute and State University.
Murphy, B. D., Concannon, P. W., Travis, H. F., and Hansel, W. (1981). Prolactin: The hypophyseal factor that terminates embryonic diapause in mink. Biol. Reprod. 25, 487–491. doi: 10.1095/biolreprod25.3.487
Murphy, B., and Fenelon, J. (2020). “Models of embryonic diapause in Carnivora,” in Pregnancy in waiting: Embryonic diapause in mammals, Proceedings of the III international symposium on embryonic diapause, eds S. Ulbrich, K. Jewgenow, B. D. Murphy, and M. B. Renfree (Ascona). doi: 10.1530/biosciprocs.10.006
Ozgul, A., Childs, D. Z., Oli, M. K., Armitage, K. B., Blumstein, D. T., Olson, L. E., et al. (2010). Coupled dynamics of body mass and population growth in response to environmental change. Nature 466, 482–485. doi: 10.1038/nature09210
Paoli, A., Weladji, R. B., Holand, Ø, and Kumpula, J. (2020). The onset in spring and the end in autumn of the thermal and vegetative growing season affect calving time and reproductive success in reindeer. Curr. Zool. 66, 123–134. doi: 10.1093/cz/zoz032
Parmesan, C. (2007). Influences of species, latitudes, and methodologies on estimates of phenological response to global warming. Glob. Change Biol. 13, 1860–1872. doi: 10.1111/j.1365-2486.2007.01404.x
Pebesma, E. J. (2004). Multivariable geostatistics in S: The gstat package. Comput. Geosci. 30, 683–691.
Pebesma, E. J., and Bivand, R. S. (2005). Classes and methods for spatial data: The sp package. R package, version 1.4-5.
Robbins, C. T., Ben-David, M., Fortin, J. K., and Nelson, O. L. (2012). Maternal condition determines birth date and growth of newborn bear cubs. J. Mammal. 93, 540–546. doi: 10.1644/11-MAMM-A-155.1
Rogers, L. (1976). “Effects of mast and berry crop failures on survival, growth, and reproductive success of black bears,” in Proceedings of the 41st North American Wildlife and Natural Resources Conference, (Washington, D.C: Wildlife Management Institute).
Rogers, L. L., McColley, L., Dalton, J., Stroner, J., Hajicek, D., Partin, A., et al. (2020). Behavior in free-living American black bear dens: Parturition, maternal care, and cub behavior. Animals 10:1123. doi: 10.2192/URSUS-D-10-00008.1
Root, T. L., Price, J. T., Hall, K. R., Schneider, S. H., Rosenzweig, C., and Pounds, J. A. (2003). Fingerprints of global warming on wild animals and plants. Nature 421, 57–60. doi: 10.1038/nature01333
Schmidt, N. M., Grøndahl, C., Evans, A. L., Desforges, J.-P., Blake, J., Hansen, L. H., et al. (2020). On the interplay between hypothermia and reproduction in a high arctic ungulate. Sci. Rep. 10:1514. doi: 10.1038/s41598-020-58298-8
Schwartz, C. C., Keating, K. A., Reynolds, H. V., Barnes, V. G., Sellers, R. A., Swenson, J. E., et al. (2003). Reproductive maturation and senescence in the female brown bear. Ursus 14, 109–119.
Shimozuru, M., Iibuchi, R., Yoshimoto, T., Nagashima, A., Tanaka, J., and Tsubota, T. (2013). Pregnancy during hibernation in Japanese black bears: Effects on body temperature and blood biochemical profiles. J. Mammal. 94, 618–627. doi: 10.1644/12-MAMM-A-246.1
Shiratsuru, S., Friebe, A., Swenson, J. E., and Zedrosser, A. (2020). Room without a view - Den excavation in relation to body size in brown bears. Evol. Ecol. 10, 8044–8054. doi: 10.1002/ece3.6371
Spady, T. J., Lindburg, D. G., and Durrant, B. S. (2007). Evolution of reproductive seasonality in bears. Mamm. Rev. 37, 21–53. doi: 10.1111/j.1365-2907.2007.00096.x
Stenset, N. E., Lutnæs, P. N., Bjarnadóttir, V., Dahle, B., Fossum, K. H., Jigsved, P., et al. (2016). Seasonal and annual variation in the diet of brown bears Ursus arctos in the boreal forest of southcentral Sweden. Wild. Biol. 22, 107–116. doi: 10.2981/wlb.00194
Swenson, J. E., Adamič, M., Huber, D., and Stokke, S. (2007). Brown bear body mass and growth in northern and southern Europe. Oecologia 153, 37–47. doi: 10.1007/s00442-007-0715-1
Taberlet, P., Swenson, J. E., Sandegren, F., and Bjarvall, A. (1995). Localization of a contact zone between two highly divergent mitochondrial DNA lineages of the brown bear Ursus arctos in Scandinavia. Conserv. Biol. 9, 1255–1261. doi: 10.1046/j.1523-1739.1995.951255.x
Thiel, A., Evans, A. L., Fuchs, B., Arnemo, J. M., Aronsson, M., and Persson, J. (2019). Effects of reproduction and environmental factors on body temperature and activity patterns of wolverines. Front. Zool. 16:21. doi: 10.1186/s12983-019-0319-8
Tøien, Ø, Blake, J., Edgar, D. M., Grahn, D. A., Heller, H. C., and Barnes, B. M. (2011). Hibernation in black bears: Independence of metabolic suppression from body temperature. Science 331, 906–909. doi: 10.1126/science.1199435
Trethowan, P. D., Hart, T., Loveridge, A. J., Haw, A., Fuller, A., and Macdonald, D. W. (2016). Improved homeothermy and hypothermia in African lions during gestation. Biol. Lett. 12:20160645. doi: 10.1098/rsbl.2016.0645
Tsubota, T., and Kanagawa, H. (1993). Morphological characteristics of the ovary, uterus and embryo during the delayed implantation period in the Hokkaido brown bear (Ursus arctos yesoensis). J. Reprod. Dev. 39, 325–331. doi: 10.2307/3872643
Tsubota, T., Takahashi, Y., and Kanagawa, H. (1987). Changes in serum progesterone levels and growth of fetuses in Hokkaido brown bears. Bears Biol. Manag. 7:355.
Tsubota, T., Taki, S., Nakayama, K., Mason, J. I., Kominami, S., Harada, N., et al. (2001). Immunolocalization of steroidogenic enzymes in the corpus luteum and placenta of the Japanese black bear, Ursus thibetanus japonicus, during pregnancy. Reproduction 121, 587–594. doi: 10.1530/rep.0.1210587
Ware, J. V., Nelson, O. L., Robbins, C. T., Carter, P. A., Sarver, B. A. J., and Jansen, H. T. (2013). Endocrine rhythms in the brown bear (Ursus arctos): Evidence supporting selection for decreased pineal gland size. Physiol. Rep 1:e00048. doi: 10.1002/phy2.48
Williams, C. T., Sheriff, M. J., Schmutz, J. A., Kohl, F., Tøien, Ø, Buck, C. L., et al. (2011). Data logging of body temperatures provides precise information on phenology of reproductive events in a free-living arctic hibernator. J. Comp. Physiol. B 181, 1101–1109. doi: 10.1007/s00360-011-0593-z
Wimsatt, W. A. (1963). “Delayed implantation in the Ursidae, with particular reference to the black bear (Ursus americanus Pallus),” in Delayed implantation, ed. A. C. Enders (Chicago: Chicago Press), 49–76.
Keywords: biologging, embryonic diapause, reproductive phenology, reproductive success, Ursus arctos
Citation: Lemière L, Thiel A, Fuchs B, Gilot-Fromont E, Hertel AG, Friebe A, Kindberg J, Støen O-G, Arnemo JM and Evans AL (2022) Extrinsic and intrinsic factors drive the timing of gestation and reproductive success of Scandinavian brown bears. Front. Ecol. Evol. 10:1045331. doi: 10.3389/fevo.2022.1045331
Received: 15 September 2022; Accepted: 25 November 2022;
Published: 07 December 2022.
Edited by:
Todd Jason McWhorter, The University of Adelaide, AustraliaReviewed by:
Vicente Urios, University of Alicante, SpainLoren Buck, Northern Arizona University, United States
Sarah Cubaynes, Université Paris Sciences et Lettres, France
Marwan Naciri, CEFE, University of Montpellier, CNRS, EPHE, IRD, France, in collaboration with reviewer SC
Copyright © 2022 Lemière, Thiel, Fuchs, Gilot-Fromont, Hertel, Friebe, Kindberg, Støen, Arnemo and Evans. This is an open-access article distributed under the terms of the Creative Commons Attribution License (CC BY). The use, distribution or reproduction in other forums is permitted, provided the original author(s) and the copyright owner(s) are credited and that the original publication in this journal is cited, in accordance with accepted academic practice. No use, distribution or reproduction is permitted which does not comply with these terms.
*Correspondence: Lucie Lemière, bHVjaWUubGVtaWVyZUBpbm4ubm8=