- 1Instituto Politécnico Nacional, Centro Interdisciplinario de Investigación para el Desarrollo Integral Regional (CIIDIR), Unidad Oaxaca, Oaxaca, México
- 2School of Life Sciences, University of Hawai’i at Mānoa, Honolulu, HI, United States
The destinies of epiphytic orchids (about 70% of all orchids) are linked to their host trees. However, there is little information on if differences in host trees characteristics can affect the long-term persistence of orchid populations, and how this might vary under different climatic conditions. We compared the population dynamics of two epiphytic orchid species, Alamania punicea and Oncidium brachyandrum growing on two host trees with contrasting leaf phenologies: the deciduous Quercus martinezii and the semideciduous Q. rugosa, over 3 years with varying levels of rainfall, in a montane tropical oak forest in Oaxaca, Mexico. Using data from > 500 individuals growing on 63 host trees, we applied linear mixed effects models, Integral Projection Models, and Life Table Response Experiments to identify the effects of host tree on orchid vital rates and population growth rates. For both orchid species, survival and growth did not differ between host species during wettest year. However, during the driest year both vital rates were higher on the semi-deciduous host Q. rugosa than on the deciduous Q. martinezii. Host species did not affect fecundity for A. punicea, but for O. brachyandrum fecundity was higher on the deciduous host. For A. punicea, λ values were similar between hosts during the wettest and intermediate years, but significantly lower (Δ λ = 0.28) on the deciduous than on the semi-deciduous host during the driest year. This was due primarily to lower survival on the deciduous host. For O. brachyandrum, λ was slightly higher (Δ λ = 0.03) on the deciduous than the semideciduous host during the wettest year, due to higher growth and reproduction. However, during the intermediate and driest years, λ values were significantly higher on the semi-deciduous than on the deciduous host (Δ λ = 0.13 and 0.15, respectively). This was due to higher survival and growth. A. punicea populations appear more vulnerable to dry conditions than O. brachyandrum, likely due to its smaller pseudobulbs, and hence lower water-storing capacity. Our results show that host tree species can both influence the vital rates and the long-term dynamics of orchid populations, and these effects vary across orchids species and over time. Our results highlight the importance of maintaining a diversity of host trees to ensure long-term population persistence.
Introduction
The orchid family is comprised of 850 genera with more than 30,000 species, and 50% of these species are concentrated in tropical areas of the world (Chase et al., 2015). In Mexico, there are approximately 1,200 species of orchids. Nearly 40% of Mexican orchids are endemic. Some species of Mexican orchids, including epiphytes, are very attractive to horticulturists, collectors, and public, and are extracted from their natural habitats and sold illegally, which can lead to them being threatened or extirpated (Halbinger and Soto, 1997; Merritt et al., 2014).
For vascular epiphytes, the presence of their host trees is essential for the establishment and permanence of their populations. However, due to differences in host traits, not all trees offer the same conditions for the establishment and development of epiphytic orchids (Wagner et al., 2015). For example, rough bark texture can affect the capture of seeds [rugose and scaly barks favor seed adherence compared to smooth barks (Adhikari and Fischer, 2011; Gowland et al., 2013; Timsina et al., 2016)], while an ability to retain and release water can favor the germination of seeds [barks with higher water retention capacity and slower release rates favor seed germination (Callaway et al., 2002; Einzmann et al., 2015)]. Similarly, the presence of allelopathic compounds in the bark of trees can limit seed germination and establishment of epiphytes (Callaway et al., 2002; Harshani et al., 2014); the rate of bark exfoliation and the fragility of branches can lead to differential mortality rates as a result of epiphyte falls (López-Villalobos et al., 2008); and the nutrient quality of stemflows and throughfalls could affect growth and fertility of the epiphytic orchids (higher amounts of nutrients could increase growth and fecundity rates). Finally, the leaf phenology of the host trees can affect the demography of epiphytes (Einzmann et al., 2015; Ticktin et al., 2016). In addition, these and other host trees characteristics can not only influence epiphytes directly, but also indirectly by providing different microclimatic conditions for the mycorrhizal fungal community, which are indispensable for the germination of the orchid seeds. Different trees may possess different communities of fungi that may or may not favor the germination of orchids (Otero et al., 2007; Rasmussen et al., 2015).
Although there is a large literature on the effects of host traits on orchid germination, establishment and survival, there is little information on whether differences in vital rates may scale up across the life cycle to differentially affect population persistence. Similarly, if and how these differences may shift with climatic conditions is largely unexplored. For example, annual variation in climatic conditions can influence the demographic behavior of epiphytes (Mondragón et al., 2004; Ticktin et al., 2016). Populations of epiphytes growing on host species that allow for higher humidity due to their phenology, architecture or bark water holding capacity might perform better during dry years, but not during average or wettest years. The one epiphyte study that has assessed this showed that the vital rates and population growth rates of an epiphytic bromeliad were different when growing on perennial pine vs. deciduous oak host trees (Ticktin et al., 2016). Populations on oaks had higher fecundity, but those on pines had higher survival and growth. Growth rates of populations on both host genera increased with increasing dry season rainfall, but the effect was larger for populations on oaks. The authors concluded that the presence of both pine and oak trees is very important for long-term conservation of these bromeliad populations.
Although we are unaware of other studies that have addressed this question for orchids, it is of great importance for developing conservation and management plans. Like many other wild species, populations of orchids are threatened by habitat loss and conversion to monocultures of timber species or other types of plantations (Boelter et al., 2011; Mondragón Chaparro et al., 2015), where diversity of host tree species is considerably diminished, for such as reforestation with pine species only (Jiménez-Bautista et al., 2014), or substitution of native shade trees from coffee or cocoa plantations by Inga spp. trees (Peeters et al., 2003; Valencia et al., 2016). These changes, in combination with changes in distribution of preferred hosts of species of epiphytes due to climate change (Hsu et al., 2012), could present problems for the long-term persistence of epiphytic orchid populations.
We carried out a demographic study to provide the first test of whether and how host species can affect the population dynamics of epiphytic orchids. We focus on two epiphytic orchid species Alamania punicea Lex. in La Llave and Lex. and Oncidium brachyandrum Lindl, growing on two congeneric host trees: the fully deciduous Q. martinezii Neé and the semi deciduous Quercus rugosa Neé. We addressed the following questions:
i) Do orchid vital rates (survival, growth, reproduction) vary between host tree species?
ii) Do differences in vital rates translate into differences in population growth rates?
iii) Do differences in vital rates and population growths rates vary with inter-annual climatic variation?
We hypothesized that:
(1) Survival and reproduction would be higher for both orchids on semi-deciduous Q. rugosa than fully deciduous Q. martinezii, due to lower light penetration and higher humidity in their treetops of the former, which can help orchids avoid photoinhibition (de la Rosa-Manzano et al., 2014; Einzmann et al., 2015) and increase flowering (Cervantes et al., 2005). In addition, we expected that branch fall, one of the main causes of mortality in epiphyte populations (Mondragón Chaparro et al., 2015; Cortes-Anzures et al., 2017), would be lower on Q. rugosa due to its thicker branches.
(2) Higher survival of individuals on the semideciduous Q. rugosa would translate into higher population growth rates (λ values), since population growth rates of long-lived species are highly sensitive to differences in survival (Franco and Silvertown, 2004). We also expected that the difference in λ values may be greater in drier years than in wettest years.
Materials and methods
Study area and species
This study was carried out in an oak forest in Tooxi, municipality of Yanhuitlán, Oaxaca, Mexico located in the Sierra Madre del Sur physiographical province (17°33′57.34″ N and 97°22′19.28″ W, elevation 2,579 m a.s.l; Figure 1) that encompasses the Mixteca Alta UNESCO Global Geopark (García-Sánchez et al., 2021). Mean annual precipitation is 753 ± 152 mm, with a dry season average of 86 ± 67 mm. The mean temperature of the dry season is 14.4 ± 1.3°C, and the average maximum and minimum temperatures are 23.3 ± 1.8°C and 7.0 ± 2.9, respectively (INIFAP, 2021; Table 1). Tree vegetation includes deciduous, semi-deciduous and evergreen trees and is comprised mainly of Quercus candicans Neé, Q. castanea Neé, Q. crassifolia Humb. and Bonpl., Q. rugosa Neé, Q. martinezii C.H. Müll., Juniperus flaccida Schltdl., and Arbutus xalapensis Kunth. The epiphytic vegetation includes Tillandsia bourgaei Baker, T. macdougallii, T. plumosa Baker, T. prodigiosa (Lem.) Baker, T. recurvata (L.) L., T. usneoides (L.) L., Pleopeltis conzatti (Weath.) R. M. Tryon and A.F.Tryon, Polypodium martensii Mett., Echeveria nodulosa (Baker) Otto, O. brachyandrum and A. punicea.
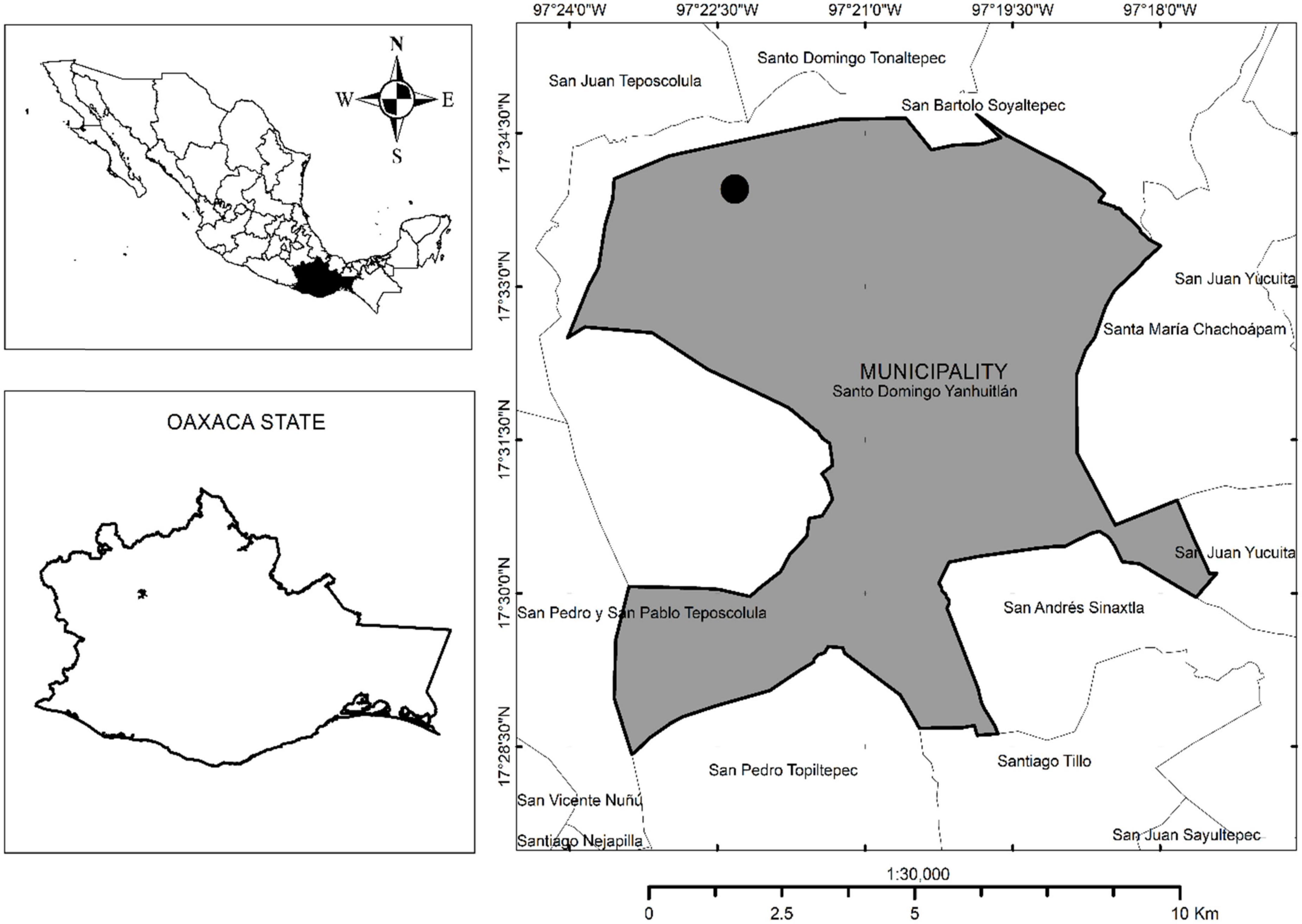
Figure 1. Geographic location of the study area within a seasonal oak forest in the municipality of Yanhuitlán, Oaxaca, Mexico.
We selected two species of tree hosts, Q. rugosa and Q. martinezii (Table 2) for this study. Quercus rugosa is distributed from Texas and Arizona, in the USA, to the Sierra Madre de Chiapas in Guatemala. Its populations are abundant in mountainous areas of western and central Mexico in temperate sub-humid climate, between the 1,800 and 2,800 m a.s.l. Quercus martinezii is endemic to Mexico and distributed in the states of Aguascalientes, Guanajuato, Hidalgo, Guerrero, Jalisco, Nuevo Leon, Nayarit, Puebla, Queretaro, San Luis Posoti, Tamaulipas, Veracruz, and Oaxaca from 2,000 to 2,500 m a.s.l (Valencia-A, 2004). The other species that hosted orchids (Q. crassifolia and Q. candicans, J. flaccida and A. xalapensis) had few individuals on them.
We focused on the two most abundant orchid species out of the three present at the study site. In addition, Alamania punicea is listed in CITES Appendix II, is the only species of a monotypic genus endemic to Mexico, and nothing is known about the demography of this species that could help to establish management and conservation strategies. Oncidium brachyandrum is harvested commercially species in Tlaxiaco, Oaxaca which is close to the study site (Ticktin et al., 2020). Nothing is known about the demography of this species; there is only one demographic study on another species of the genus (Oncidium poikilostalix Kraenzl.) M.W. Chase and N.H. Williams but in coffee plantations. We describe each orchid species as follows:
Alamania punicea Lex. is an epiphytic perennial orchid, 3–6 cm high including the inflorescence; ovoid pseudobulbs, slightly elongated, covered by translucent papyrus sheaths, 7–10 mm long; leaves 2, rarely 3, at the apex of the pseudobulb, elliptic to oblanceolate sheets, 1–4 cm long, 5–10 mm wide; flowers 7–14, red to pinkish reddish. Fruits are capsules with dust-like seeds. There is no report of A. punicea breeding system. Stpiczyńska et al. (2005) suggest that given its floral morphology, it could be pollinated by hummingbirds. Alamania punicea is an endemic species prevalent in cool and seasonally dry Quercus-Pinus forests on the Trans-Mexican Volcanic Belt and the Sierra Madre Oriental above 1,900 m a.s.l. (where oaks are dominant; García-Cruz et al., 2003; Soto Arenas, 2005; UNEP-WCMC, 2020).
Oncidium brachyandrum is an epiphytic perennial orchid, up to 20 cm high with clustered pseudobulbs, ovoid to ellipsoid or subglobose, somewhat laterally compressed, 2–3 cm long; 2 or 3 lateral leaves; flowers 2 or 3, simultaneous, showy, 25–30 mm in diameter, sepals, and petals brown or yellow with irregular brown spots and yellow lip. Fruits are capsules with dust-like seeds. This species probably is pollinated by oil-collecting or bombini bees and might be self-incompatible as reported for other members of the genera (Damon and Cruz-López, 2006; Pemberton, 2008). This orchid is distributed in Honduras, Guatemala, and in Mexico in the states of Durango, Guerrero, Jalisco, Michoacán de Ocampo, Morelos, Nayarit, Sinaloa, Zacatecas, and Oaxaca. It grows mainly in oak forests elevations of 2,000–2,500 m a.s.l. (Jiménez et al., 1998).
Precipitation, the most limiting factor for epiphytes (Zotz and Hietz, 2001; Laube and Zotz, 2003), varied across our three study years, especially during the dry season (Table 1). Although precipitation in all 3 years was above the mean of the past 20 years (Supplementary material), we refer to these years as the wettest year (2018), driest year (2019) where dry season precipitation was less than half that of the wettest year–and intermediate year (2020).
We selected 21 Q. martinezii and 42 Q. rugosa trees within a 1 ha plot and tagged and measured all the orchids of our study species on them. We selected a higher number of Q. rugosa trees since they had lower densities of orchids: on average there were 14 ± 11 O. brachyandrum plants/tree on Q. martinezii versus 6 ± 6 on Q. rugosa; these values were 8 ± 6 plants/tree vs. 7 ± 6, respectively, for A. punicea. For each of 3 years (2017–2020), we recorded plant status (alive, dead), size, and fecundity (number of capsules), and recorded the number of new seedlings. For both species of orchid, we measured height and width of the largest pseudobulb and counted the number of pseudobulbs. We also recorded causes of mortality distinguishing broadly between desiccation (the entire dead plant was still attached to the tree) and falling (the plant was missing). Although individuals that die due to desiccation are susceptible to falling, our monthly checks ensured that we were able to ascertain the correct cause of mortality. We did not include herbivory as a cause of death since we did not observe orchid plants attacked by herbivores at our study site. This is consistent with findings from other studies that rates of herbivory are mostly low in epiphytes (Benzing, 1990; Zotz, 1998; Winkler et al., 2005). Finally, we measured host characteristics on a subsample of individuals of each host species and tested for differences between hosts using ANOVAs (Table 2; Ramírez-Martínez, 2022).
Data analyses
Host effects on demographic rates (vital rates)
We tested differences in survival, growth, and reproduction of orchid individuals on the different Quercus species using generalized linear mixed models (GLMMs). Initial size (log-transformed), host species (Q. martinezii vs. Q. rugosa), and year were fixed effects and individual orchid nested within individual host tree were random effects. We used regression analyses to identify which measure of size (e.g., number of pseudobulbs, size of the pseudobulb, etc.) was the best predictor of growth, reproduction, and survival. We used Akaike’s information criterion (AICc) to compare model fit. We found that for A. punicea, the best predictor was an index of number of leaves times area of the longest leaf (calculated with the formula for an oval). For O. brachyandrum, the best predictor was pseudobulb area (calculated with the formula for an oval).
To model the probability of survival, reproduction, and probability of mortality due to desiccation, we used binomial distributions, and to model the number of capsules, we used a negative binomial model. To model growth (size at t + 1), we used Gaussian error structure with an exponential variance structure, where the variance increases as an exponential function of initial size (Zuur et al., 2009). We modeled the probability of reproduction with the minimum size observed for plant reproduction (sizes: A. punicea ≥ 1.44 cm2, and O. brachyandrum ≥ 0.79 cm2). We selected the best fit model based on the lowest Akaike (AICc). All analyses were performed using the glmmTMB package in R v. 4.1.1.
Host effects on population growth dynamics
Integral projection models
We used integral projection models [IPMs (Easterling et al., 2000)] to project the long-term (asymptotic) population growth rates (λ values) of each orchid species growing on each of the two host species. The IPMs are constructed from continuous functions that describe size-dependent growth, survivorship, and fecundity. The IPM kernel is the sum of two functions. One describes the survival, probability and growth (or shrinkage) of survivors (p kernel), and the second is the reproductive contribution of each individual and the size distribution of the new seedlings (f kernel). Our IPM took the form:
For both orchid species, the p(x,y) kernel was represented by the survival probability of individuals of size x to size y attributable to size-dependent survival, s(x), and growth g(x,y): p(x,y) = s(x) g(x,y). The fertility kernel f(x,y) denotes the production of new seedlings of size (x) produced from plants of size (y). This was calculated for plants of reproductive size as: f(x,y) = s(x) fn(x) pEfd(y), where s(x) is the survival of plants of size (x), fn(x) is the probability of producing capsules for plant size x times the number of capsules per plant size x; pE is the number of new seedlings produced per capsule, and fd(y) is the size distribution of new seedlings. For each host species, pE was calculated as the number of seedlings observed in the field divided by the total number of capsules produced. We calculated the asymptotic projected population growth rate (λ) for each IPM using the popbio 2.7 package in R (Stubben and Milligan, 2007) and obtained 95% confidence intervals by bootstrapping (N = 100).
We used life table response experiments (LTREs) (Caswell, 2001) to identify which vital rate contributed most to the observed differences in population growth rates between hosts, for each orchid species and year.
Results
Host effects on vital rates
Alamania punicea
Survival
For individuals on both host species, survival increased as a function of size and was highest during the wettest year and lowest during the driest year (Table 3 and Figure 2A). The best fit model included an interaction between host species and year such that difference in survival between individuals on semideciduous Q. rugosa and the deciduous Q. martinezii was least during the wettest year. The probability of mortality due to desiccation decreased significantly with (log) size (β = −0.69, SE = 0.09, z = −7.35, p < 0.001) but did not differ significantly between host trees.
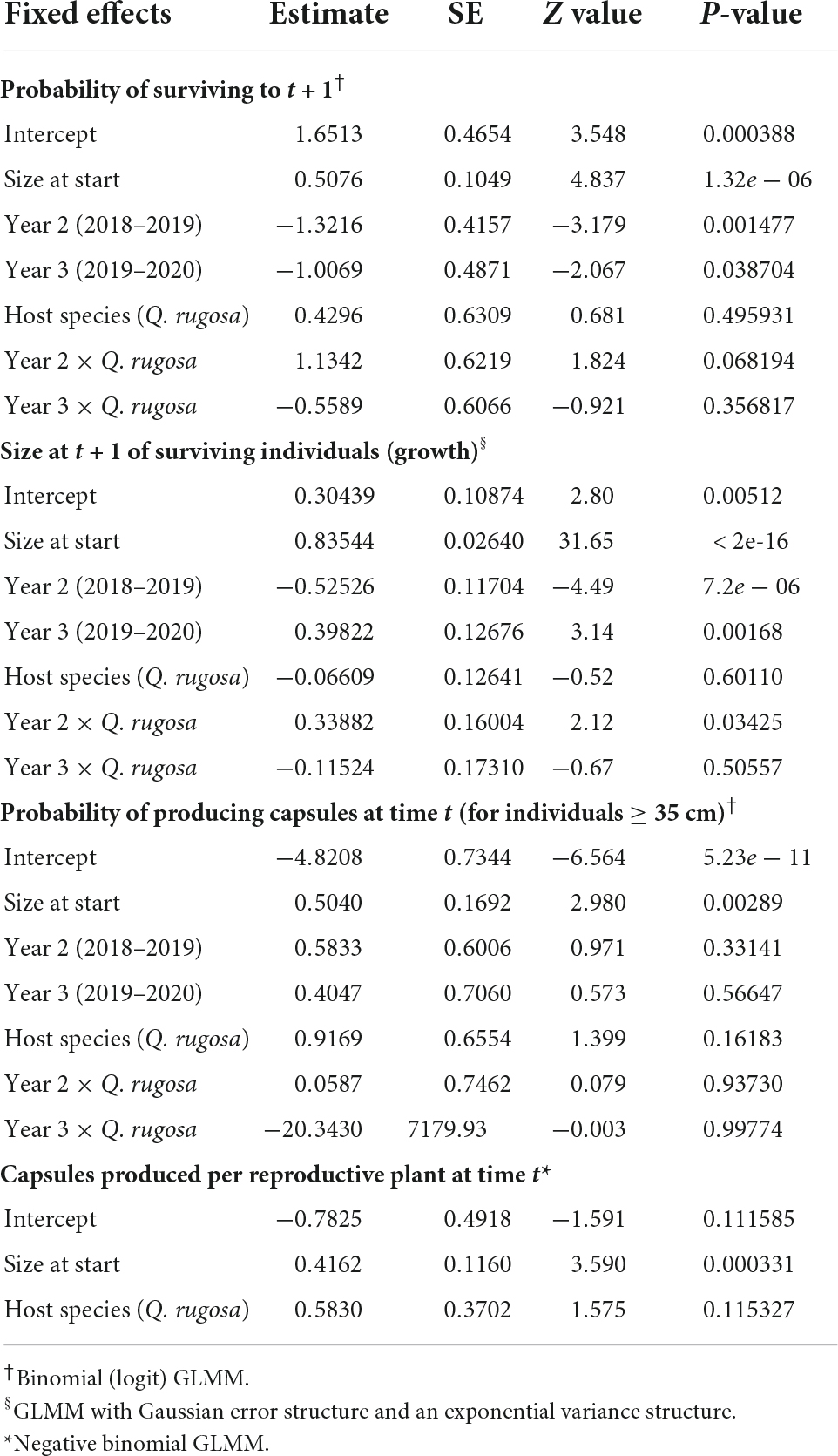
Table 3. Estimated coefficients from mixed-effect models of the probability of survival, growth, reproduction, and probability of producing capsules for Alamania punicea plants growing on two Quercus species.
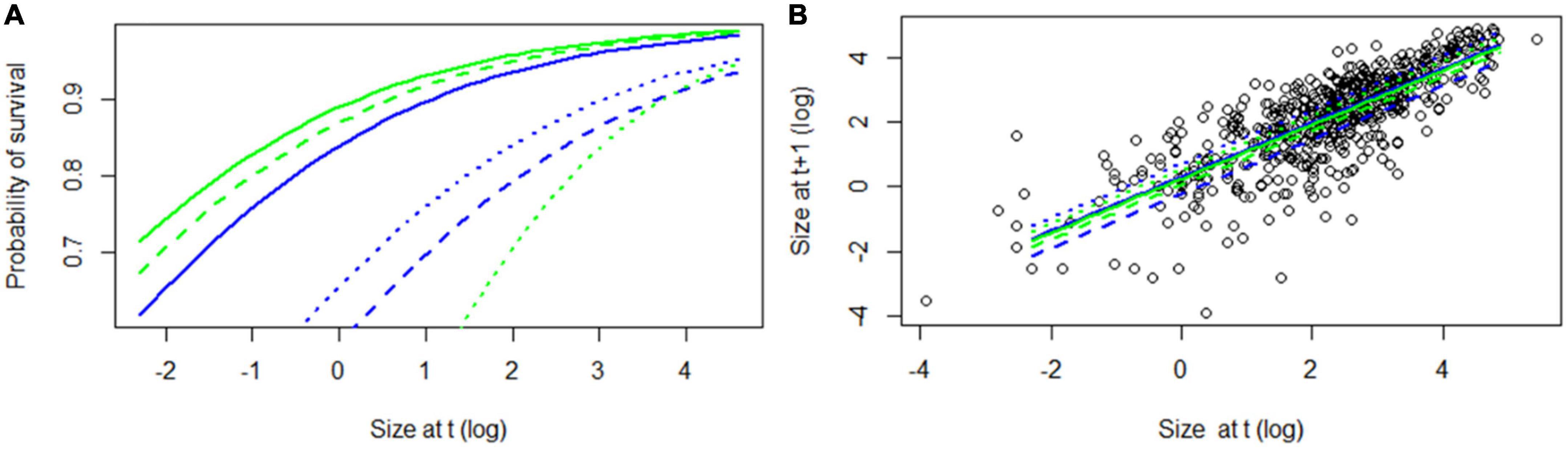
Figure 2. (A) Survival and (B) growth as function of size, for Alamania punicea plants growing on two oak species Quercus martinezii (blue) and Q. rugosa (green). Solid lines indicate year 2017–2018, dashed lines year 2018–2019, and dotted lines year 2019–2020.
Growth
For individuals on both species, growth was lowest in the driest year and highest in the intermediate year. During the dry year, growth was higher on the semi-deciduous Q. martinezii than on the deciduous Q. martinezii (Table 3 and Figure 2B).
Reproduction
Plants began to reproduce once they reached 1.08 cm2 size (number of leaves times area of the longest leaf). Only 1.4% of plants flowered and 100% those produced capsules. The probability of reproduction increased as a function of size, as did the number of capsules produced by reproducing plants (Table 3).
Oncidium brachyandrum
Survival
For plants on both host species, survival increased as a function of plant size. There was no difference in survival between host species during the wettest year, but survival on the semi-deciduous Q. rugosa was higher than on the deciduous Q. martinezii during the dry and intermediate years (Table 4 and Figure 3A). The probability of mortality due to desiccation decreased significantly with (log) size (β = −0.64, SE = 1.07, z = −6.00, p < 0.001) and was significantly higher in the intermediate year than in the wettest year (β = −1.63, SE = 0.57, z = −2.89, p = 0.004). It did not differ significantly between host trees.
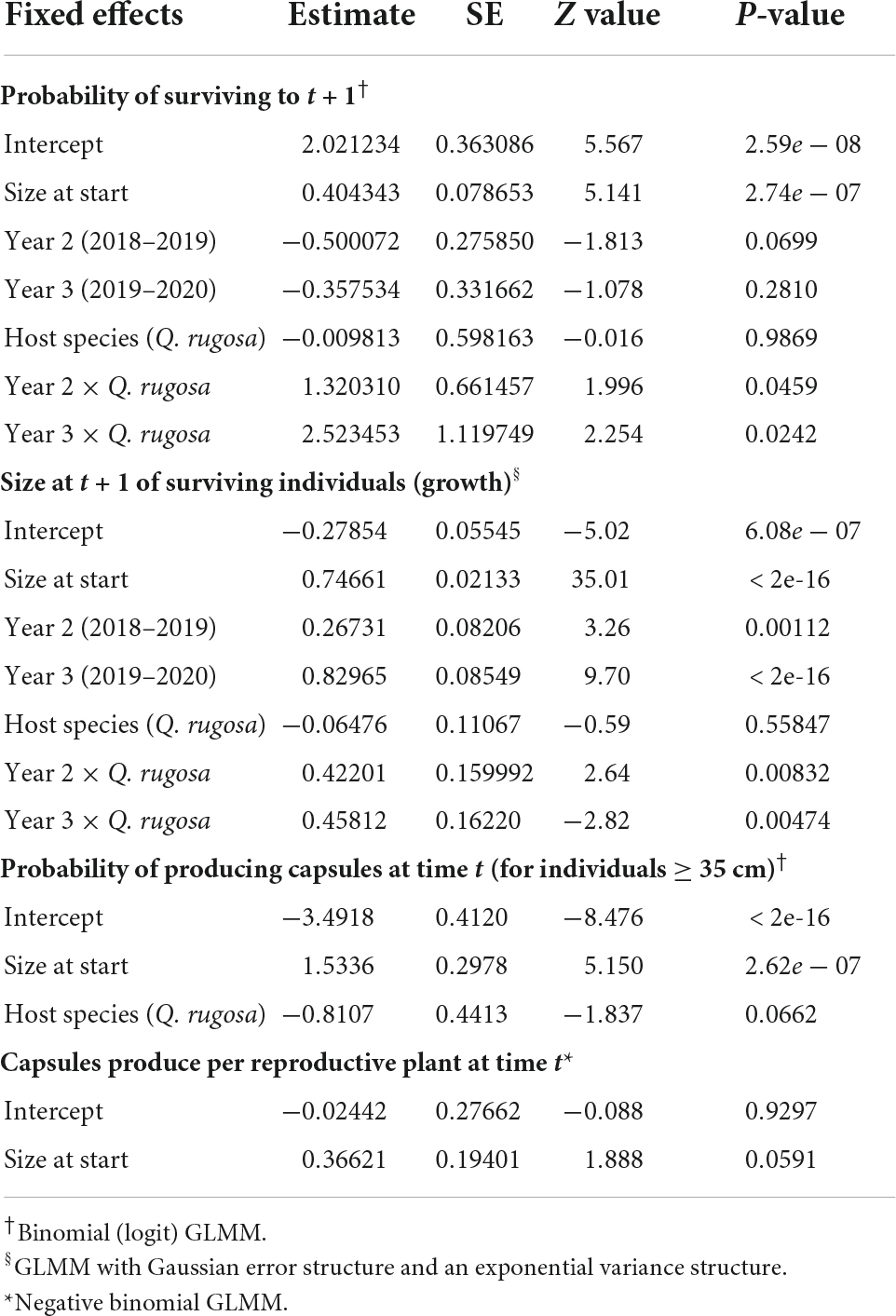
Table 4. Estimated coefficients from mixed-effect models of the probability of survival, growth, reproduction, and probability of producing capsules for Oncidium brachyandrum plants growing on two Quercus species.
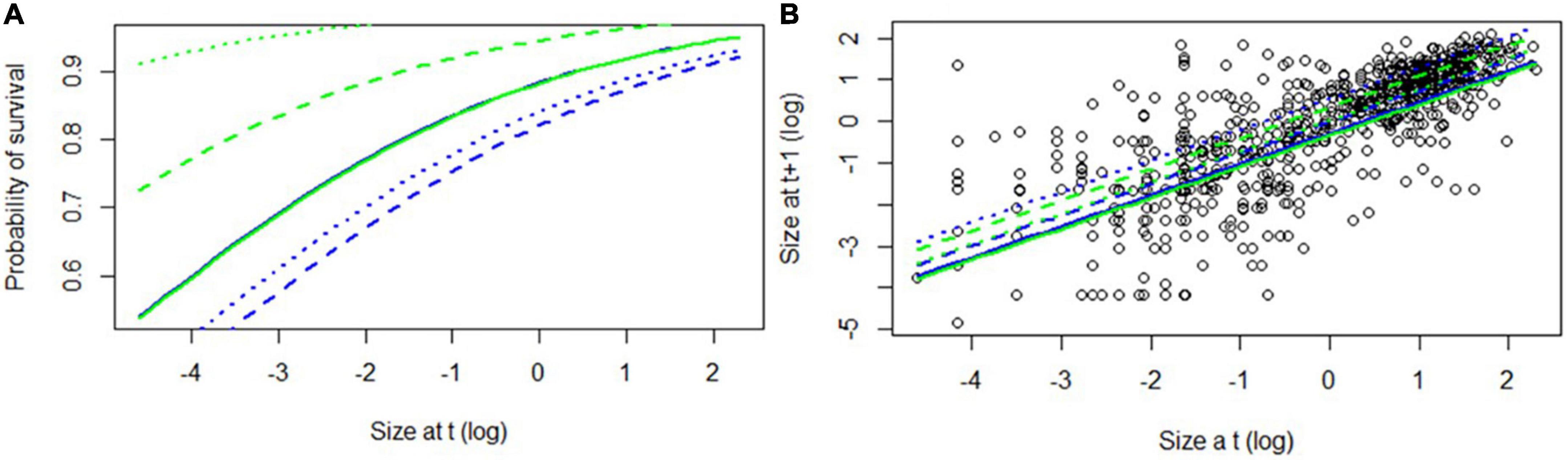
Figure 3. (A) Survival and (B) growth as function of size, for Oncidium brachyandrum plants growing on two oak species Quercus martinezii (blue) and Q. rugosa (green). Solid lines indicate year 2017–2018, dashed lines year 2018–2019, and dotted lines year 2019–2020.
Growth
For plants growing on both host species, growth was higher in dry and intermediate years than in wettest year. Growth was higher on the semi-deciduous Q. rugosa than on the deciduous Q. martinezii only during the dry year (Table 4 and Figure 3B).
Reproduction
Plants began to reproduce once they reached 0.78 cm2 (pseudobulb area); 14% of plants flowered and 100% of these produced capsules. The probability of reproduction and the number of capsules produced per reproductive plant both increased as a function of size (Table 4). Host species was included in the best fit model for the probability of reproduction, with reproduction higher on the deciduous Q. martinezii than the semideciduous Q. rugosa (Table 4).
Host tree effect on population dynamics
Population growth rates of A. punicea on the two host species were similar in the wettest year and the intermediate years (Δλ between hosts = 0.01). However, population growth rates were significantly higher on the semideciduous host than the deciduous host during the driest year ((Δ λ = 0.28; Figure 4A). For O. brachyandrum, λ was slightly lower on Q. martinezii than on Q. rugosa during the wettest year (Δ λ = 0.03). However, the reverse was true during the driest and intermediate years, where λ values were significantly higher on Q. rugosa than on Q. martinezii (Δ λ = 0.15 and 0.13, respectively) (Figure 4B). For O. brachyandrum, λ values were higher in the dry and intermediate years, than in the wettest year.
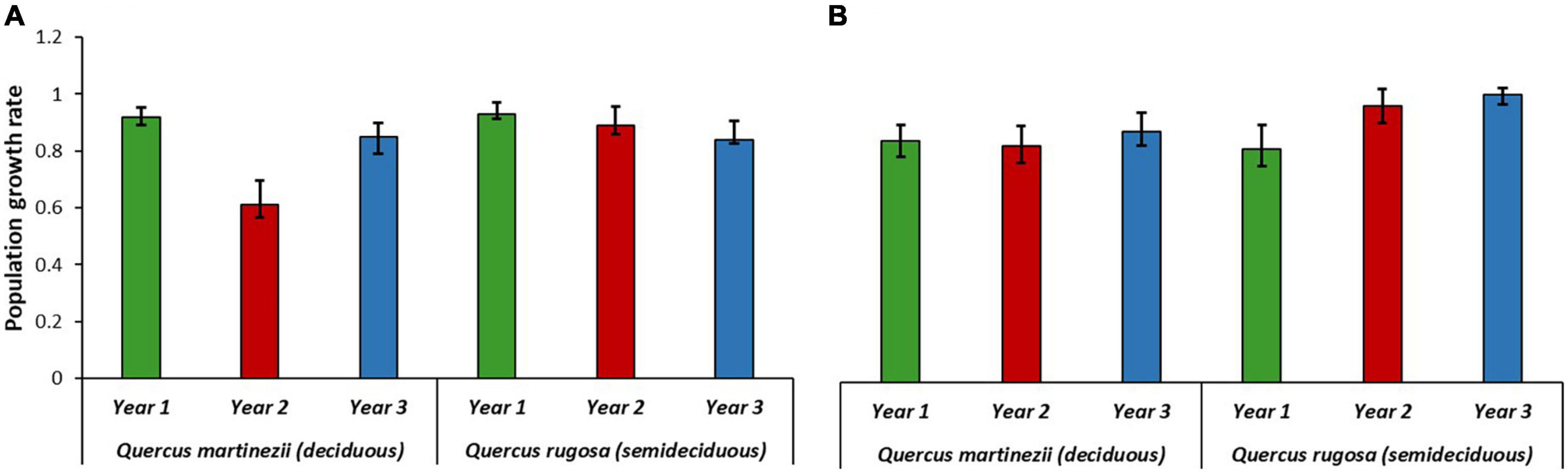
Figure 4. Population growth rates (λ values) of (A) Alamania punicea and (B) Oncidium brachyandrum growing on two Quercus species, from 2018 to 2020 in an oak forest in Oaxaca, Mexico. Error bars represent 95% confidence intervals.
LTRE analyses
For A. punicea, the higher λ value observed for populations on Q. rugosa in the driest year was mainly due to higher survival on that host tree. For the wettest and the intermediate rainfall year, there was little differences in λ values across hosts, but differences were due to higher survival on Q. rugosa during the wettest year growth, and higher growth on Q. martinezii during the intermediate year (Figures 5A–C). For O. brachyandrum higher growth and reproduction contributed the most to the higher λ value of Q. martinezii in wettest year. The higher λ values observed for populations in Q. rugosa during the two drier years was due to higher growth (driest year), and higher survival (intermediate year) (Figures 5D–F).
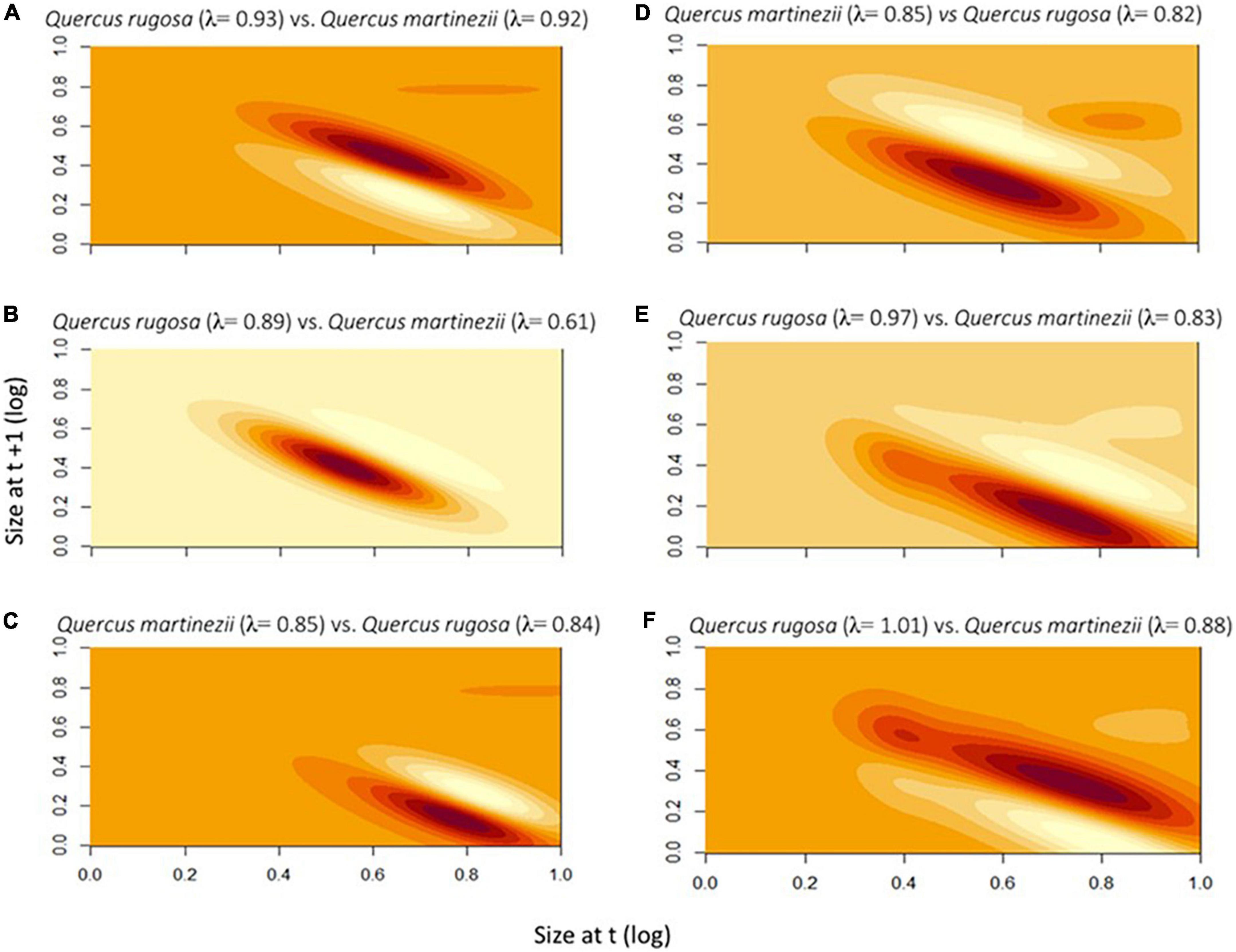
Figure 5. Life table response experiment contributions for Alamania punicea (A–C) and Oncidium brachyandrum (D–F) plants growing on two Quercus species. Darker colors represent life-history transitions that make greater contributions to higher λ values observed. Values across the diagonal represent contributions from survival, and those below diagonal represent contributions from growth. Fecundity contributions are represented in the top right corner.
Discussion
The aim of our paper was to test if and how host species can affect the population dynamics of epiphytic orchids. Our results show that rates of survival, growth and reproduction of epiphytic orchids can vary across host tree species, and this can translate into difference in population growth rates. They also suggest that the direction and magnitude of these differences appears to depend on climatic conditions.
Our finding that both orchid species had higher growth and survival on the semi-deciduous host than on the deciduous host, during the driest year, but not during the wettest year, is consistent with other studies. Einzmann et al. (2015) found higher growth and survival rates of two epiphytic species growing on perennial trees than on deciduous trees, due to the sunnier and drier microclimates during dry season on the latter, which increased mortality due to desiccation. The same has been reported for an epiphytic bromeliad, where individuals growing on perennial pines had higher survival and growth rates that those growing on deciduous oak (Ticktin et al., 2016). Similarly, Callaway et al. (2002) reported higher growth rates of epiphytic individuals growing on their preferred host and attributed it to the higher water holding capacity its bark. Species with greater water holding capacity provide a more humid environment, and allow epiphytes access to water for a longer period. Humidity is recognized as the more limiting factor within epiphytism (Benzing, 1990; Laube and Zotz, 2003; Zotz, 2013).
Our results suggest potential lag effects of drought, since for the semi-deciduous host, a higher number of orchid individuals died the year after the driest year (intermediate year), than during the driest year itself. According to Zotz and Tyree (1996) there can be long-term drought stress effects on the physiology of orchids that can be perceived afterward forcing plants to show die back on some of their parts (like leaves), and finally die.
Our finding that fecundity of O. brachyandrum was also higher on the deciduous host could be related to the low photosynthetic efficiency of epiphytic orchids due to drought adaptation (Sahagun-Godinez, 1996); they therefore need higher light (as in deciduous trees in our case) to perform photosynthesis efficiently and produce photosynthates for flower production. The lack of effect that we observed for A. punicea could be related to sample size, since very few individuals flowered during our study. The low probability of flowering is typical for many epiphytic orchids (Tremblay, 2006).
The differences we found in vital rates scaled up to differences in population growth rates, with λ values much lower on the deciduous host during the dry years than in the wettest year. Our finding that Δλ between the wettest and dry year was greater for A. punicea than for O. brachyandrum is likely related to differences between the species in adaptation related with drought tolerance. A. punicea has thick leaves and cuticles, and small pseudobulbs (7–10 mm long), while O. brachyandrum has thin leaves and pseudobulbs 2–3 cm long. These represent two of the main strategies of orchids for drought tolerance (Stancato et al., 2001; Yang et al., 2016): thick cuticles avoid water loss, while pseudobulbs store water. In our study, the species with pseudobulbs better buffered the effect of host tree on its growth and survival rates. Pseudobulbs play an important role in the growth and survival of epiphytic orchids since they not only store water but are also responsible for the partition of assimilates, carbohydrate and minerals and can perform photosynthesis (Ng and Hew, 2000). Further research is needed to identify how morphological and physiological variation in epiphytic orchids (Dressler, 1993; Yang et al., 2016; Zhang et al., 2018) shapes demographic responses. In addition, translocation experiments could further disentangle differences in demographic rates across hosts.
Our results highlight the importance of preserving tree species diversity to foster the long-term persistence of populations of vascular epiphytes. This is especially true if we consider that vascular epiphytes function as metapopulations (Winkler et al., 2009; Valverde and Bernal, 2010), where individuals growing on one host tree represent a sub-population interconnected with other sub-populations (on other host trees) by seed dispersal, and where trees that support growing sub-populations determine the growth of the metapopulation (Winkler et al., 2009). Thus, metapopulation growth may be maximized when there is a diversity of host species that allow for growth under varying climatic conditions. In addition, variation among genotypes of the same tree species could potentially affect demographic rates, given that Zytynska et al. (2011) found a positive correlation between the genetic distances among host trees and similarity among the community vascular epiphytes growing on them. Further research is needed to better understand how host tree traits shape the persistence of populations of epiphytic orchids. On the ground, working with local communities to help identify land-use options that maintain tree diversity will be key.
Data availability statement
The raw data supporting the conclusions of this article will be made available by the authors, without undue reservation.
Author contributions
AR-M conducted fieldwork and wrote most of the manuscript. DM conceived the study, provided the advice, financial support, conducted fieldwork, and contributed to the manuscript. TT provided the advice, helped with data analyses, contributed to the manuscript, and reviewed the English. All authors contributed to the article and approved the submitted version.
Funding
We are grateful for the financial support provided by the Instituto Politécnico Nacional (projects SIP-20170823, SIP-20180674, SIP-20195449, and SIP-20200025). AR-M received a studentship from Consejo Nacional de Ciencia y Tecnología (CONACYT) to carry out her Ph.D. studies.
Acknowledgments
We acknowledged the contributions of specific colleagues, institutions, or agencies that aided the efforts of the authors.
Conflict of interest
The authors declare that the research was conducted in the absence of any commercial or financial relationships that could be construed as a potential conflict of interest.
Publisher’s note
All claims expressed in this article are solely those of the authors and do not necessarily represent those of their affiliated organizations, or those of the publisher, the editors and the reviewers. Any product that may be evaluated in this article, or claim that may be made by its manufacturer, is not guaranteed or endorsed by the publisher.
Supplementary material
The Supplementary Material for this article can be found online at: https://www.frontiersin.org/articles/10.3389/fevo.2022.1059136/full#supplementary-material
References
Adhikari, Y. P., and Fischer, A. (2011). Distribution pattern of the epiphytic orchid Rhynchostylis retusa under strong human influence in Kathmandu valley, Nepal. Bot. Orient. J. Plant Sci. 8, 90–99. doi: 10.3126/botor.v8i0.5956
Benzing, D. H. (1990). Vascular epiphytes: general biology and related biota, 1st Edn. Cambridge: Cambridge University Press, doi: 10.1017/CBO9780511525438
Boelter, C. R., Zartman, C. E., and Fonseca, C. R. (2011). Exotic tree monocultures play a limited role in the conservation of Atlantic Forest epiphytes. Biodivers. Conserv. 20, 1255–1272. doi: 10.1007/s10531-011-0026-z
Callaway, R. M., Reinhart, K. O., Moore, G. W., Moore, D. J., and Pennings, S. C. (2002). Epiphyte host preferences and host traits: mechanisms for species-specific interactions. Oecologia 132, 221–230. doi: 10.1007/s00442-002-0943-3
Caswell, H. (2001). Matrix population models: construction, analysis, and interpretation, 2nd Edn. New York, NY: Oxford University Press.
Cervantes, S. E., Graham, E. A., and Andrade, J. L. (2005). Light microhabitats, growth and photosynthesis of an epiphytic bromeliad in a tropical dry forest. Plant Ecol. 179, 107–118. doi: 10.1007/s11258-004-5802-3
Chase, M. W., Cameron, K. M., Freudenstein, J. V., Pridgeon, A. M., Salazar, G., Van den Berg, C., et al. (2015). An updated classification of Orchidaceae. Bot. J. Linn. Soc. 177, 151–174. doi: 10.1111/boj.12234
Cortes-Anzures, B. O., Corona-López, A. M., Toledo-Hernández, V. H., Valencia-Díaz, S., and Flores-Palacios, A. (2017). Branch mortality influences phorophyte quality for vascular epiphytes. Botany 95, 709–716. doi: 10.1139/cjb-2017-0023
Damon, A. A., and Cruz-López, L. (2006). Fragrance in Relation to Pollination of Oncidium sphacelatum and Trichocentrum oerstedii (Orchidaceae) in the Soconusco Region of Chiapas, Mexico. Selbyana 27, 186–194.
de la Rosa-Manzano, E., Andrade, J. L., Zotz, G., and Reyes-García, C. (2014). Epiphytic orchids in tropical dry forests of Yucatan, Mexico – Species occurrence, abundance and correlations with host tree characteristics and environmental conditions. Flora 209, 100–109. doi: 10.1016/j.flora.2013.12.002
Dressler, R. (1993). Phylogeny and classification of orchid family. Cambridge: Cambridge University Press.
Easterling, M. R., Ellner, S. P., and Dixon, P. M. (2000). Size-specific sensitivity: applying a new structured population model. Ecology 81, 694–708. doi: 10.1890/0012-9658(2000)081[0694:SSSAAN]2.0.CO;2
Einzmann, H. J. R., Beyschlag, J., Hofhansl, F., Wanek, W., and Zotz, G. (2015). Host tree phenology affects vascular epiphytes at the physiological, demographic and community level. AoB Plants 7:lu073. doi: 10.1093/aobpla/plu073
Franco, M., and Silvertown, J. (2004). A comparative demography of plants based upon elasticities of vital rates. Ecology 85, 531–538.
García-Cruz, P. J., Saldaña, S. L. M., Jiménez, M. R., and Solano, G. R. (2003). Orchidaceae. Tribu epidendreae. Flora Bajío Regiones Adyacentes 119, 1–173.
García-Sánchez, L., Canet, C., Cruz-Pérez, M. Á, Morelos-Rodríguez, L., Salgado-Martínez, E., and Corona-Chávez, P. (2021). A comparison between local sustainable development strategies based on the geoheritage of two post-mining areas of Central Mexico. Int. J. Geoheritage Parks 9, 391–404.
Gowland, K. M., van der Merwe, M. M., Linde, C. C., Clements, M. A., and Nicotra, A. B. (2013). The host bias of three epiphytic Aeridinae orchid species is reflected, but not explained, by mycorrhizal fungal associations. Am. J. Bot. 100, 764–777. doi: 10.3732/ajb.1200411
Harshani, H. C., Senanayake, S. P., and Sandamali, H. (2014). Host tree specificity and seed germination of Dendrobium aphyllum (Roxb.) C.E.C. Fisch. in Sri Lanka. J. Natl. Sci. Found. 42, 71–86. doi: 10.4038/jnsfsr.v42i1.6682
Hernández-Álvarez, G. R. (2021). Efecto del forofito sobre la germinación de alamania punicea y oncidium brachyandrum. Master dissertation. Oaxaca: Instituto Politécnico Nacional.
Hsu, R. C.-C., Tamis, W. L. M., Raes, N., de Snoo, G. R., Wolf, J. H. D., Oostermeijer, G., et al. (2012). Simulating climate change impacts on forests and associated vascular epiphytes in a subtropical island of East Asia. Divers. Distrib. 18, 334–347. doi: 10.1111/j.1472-4642.2011.00819.x
INIFAP (2021). Sistema de base de datos meteorológicos. Available online at: https://clima.inifap.gob.mx/lnmysr/DatosIndirectos/Essenger (accessed September 30, 2020).
Jiménez, R. M., Sánchez, L. S., and García-Cruz, J. (1998). Orchidaceae. Flora del bajío y de regiones adyacentes. Fascículo 67, 38.
Jiménez-Bautista, L., Damon, A., Ochoa-Gaona, S., and Tapia, R. C. (2014). Impact of silvicultural methods on vascular epiphytes (ferns, bromeliads and orchids) in a temperate forest in Oaxaca, Mexico. For. Ecol. Manage. 329, 10–20. doi: 10.1016/j.foreco.2014.05.053
Laube, S., and Zotz, G. (2003). Which abiotic factors limit vegetative growth in a vascular epiphyte? Funct. Ecol. 17, 598–604. doi: 10.1046/j.1365-2435.2003.00760.x
López-Villalobos, A., Flores-Palacios, A., and Ortiz-Pulido, R. (2008). The relationship between bark peeling rate and the distribution and mortality of two epiphyte species. Plant Ecol. 198, 265–274. doi: 10.1007/s11258-008-9402-5
Merritt, D. J., Hay, F. R., Swarts, N. D., Sommerville, K. D., and Dixon, K. W. (2014). Ex situ conservation and cryopreservation of orchid germplasm. Int. J. Plant Sci. 175, 46–58. doi: 10.1086/673370
Mondragón, D., Durán, R., Ramírez, I., and Valverde, T. (2004). Temporal variation in the demography of the clonal epiphyte Tillandsia brachycaulos (Bromeliaceae) in the Yucatán Peninsula. Mexico. J. Trop. Ecol. 20, 189–200. doi: 10.1017/S0266467403001287
Mondragón Chaparro, D. M., Valverde Valdés, M. T., and Hernández Apolinar, M. (2015). Population ecology of epiphytic angiosperms: A review. Trop. Ecol. 56, 1–39.
Ng, C. K. Y., and Hew, C. S. (2000). Orchid pseudobulbs–false’bulbs with a genuine importance in orchid growth and survival! Sci. Hortic. 83, 165–172.
Otero, J. T., Aragón, S., and Ackerman, J. D. (2007). Site variation in spatial aggregation and phorophyte preference in Psychilis monensis (Orchidaceae). Biotropica 39, 227–231. doi: 10.1111/j.1744-7429.2006.00258.x
Peeters, L. Y. K., Soto-Pinto, L., Perales, H., Montoya, G., and Ishiki, M. (2003). Coffee production, timber, and firewood in traditional and Inga-shaded plantations in Southern Mexico. Agric. Ecosyst. Environ. 95, 481–493. doi: 10.1016/S0167-8809(02)00204-9
Pemberton, R. W. (2008). Pollination of the ornamental orchid Oncidium sphacelatum by the naturalized oil-collecting bee (Centris nitida) in Florida. Selbyana 29, 87–91. doi: 10.1653/0015-4040(2008)091[0101:NOTOCB]2.0.CO;2
Ramírez-Martínez, A. (2022). Host tree effect on demography and phenology of epiphytic species. Doctoral dissertation. Oaxaca: Instituto Politécnico Nacional.
Rasmussen, H. N., Dixon, K. W., Jersáková, J., and Těšitelová, T. (2015). Germination and seedling establishment in orchids: a complex of requirements. Ann. Bot. 116, 391–402. doi: 10.1093/aob/mcv087
Sahagun-Godinez, E. (1996). Trends in the phenology of flowering in the Orchidaceae of Western Mexico. Biotropica 28, 130–136. doi: 10.2307/2388778
Soto Arenas, M. A. (2005). “Alamania,” in Genera orchidacearum. Epidendroideae (Part one), Vol. 4, eds A. M. Pridgeon, P. J. Cribb, M. W. Chase, and F. N. Rasmussen (Oxford: Oxford University Press).
Stancato, G. C., Mazzafera, P., and Buckeridge, M. S. (2001). Effect of a drought period on the mobilisation of non-structural carbohydrates, photosynthetic efficiency and water status in an epiphytic orchid§1Part of the Ph.D. thesis of Giulio Cesare Stancato (Instituto de Biologia, UNICAMP). Plant Physiol. Biochem. 39, 1009–1016. doi: 10.1016/S0981-9428(01)01321-3
Stpiczyńska, M., Davies, K. L., and Gregg, A. (2005). Comparative account of nectary structure in Hexisea imbricata (Lindl.) Rchb.f. (Orchidaceae). Ann. Bot. 95, 749–756. doi: 10.1093/aob/mci081
Stubben, C., and Milligan, B. (2007). Estimating and analyzing demographic models using the popbio package in R. J. Stat. Softw. 22, 1–23. doi: 10.18637/jss.v022.i11
Ticktin, T., Mondragón, D., and Gaoue, O. G. (2016). Host genus and rainfall drive the population dynamics of a vascular epiphyte. Ecosphere 7:e01580. doi: 10.1002/ecs2.1580
Ticktin, T., Mondragón, D., Lopez-Toledo, L., Dutra-Elliott, D., Aguirre-León, E., and Hernández-Apolinar, M. (2020). Synthesis of wild orchid trade and demography provides new insight on conservation strategies. Conserv. Lett. 13:e12697. doi: 10.1111/conl.12697
Timsina, B., Rokaya, M. B., Münzbergová, Z., Kindlmann, P., Shrestha, B., Bhattarai, B., et al. (2016). Diversity, distribution and host-species associations of epiphytic orchids in Nepal. Biodivers. Conserv. 25, 2803–2819. doi: 10.1007/s10531-016-1205-8
Tremblay, R. L. (2006). The effect of population structure, plant size, herbivory and reproductive potential on effective population size in the temperate epiphytic orchid, Sarcochilus australis. Cunninghamia 9, 529–535.
UNEP-WCMC (2020). CITES Secretariat, Geneva, Switzerland. Compiled by UNEP-WCMC, Cambridge, UK. The checklist of CITES species website. Available online at: https://efloramex.ib.unam.mx/cdm_dataportal/taxon/35a4cfa8-6d5e-48cd-a5f7-b3dd3b380c81#distribuci%C3%B3n (accessed September 15, 2022).
Valencia, V., Naeem, S., García-Barrios, L., West, P., and Sterling, E. J. (2016). Conservation of tree species of late succession and conservation concern in coffee agroforestry systems. Agric. Ecosyst. Environ. 219, 32–41. doi: 10.1016/j.agee.2015.12.004
Valencia-A, S. (2004). Diversidad del género Quercus (Fagaceae) en México. Bot. Sci. 75, 33–53. doi: 10.17129/botsci.1692
Valverde, T., and Bernal, R. (2010). ¿Hay asincronía demográfica entre poblaciones locales de Tillandsia recurvata?: Evidencias de su funcionamiento metapoblacional. Bol. Soc. Bot. Mex. 23–36.
Wagner, K., Mendieta-Leiva, G., and Zotz, G. (2015). Host specificity in vascular epiphytes: a review of methodology, empirical evidence and potential mechanisms. AoB Plants 7:lu092. doi: 10.1093/aobpla/plu092
Winkler, M., Hülber, K., and Hietz, P. (2009). Population dynamics of epiphytic orchids in a metapopulation context. Ann. Bot. 104, 995–1004. doi: 10.1093/aob/mcp188
Winkler, M., Hülber, K., Mehltreter, K., Franco, J. G., and Hietz, P. (2005). Herbivory in epiphytic bromeliads, orchids and ferns in a Mexican montane forest. J. Trop. Ecol. 21, 147–154. doi: 10.1017/S0266467404002081
Yang, S.-J., Sun, M., Yang, Q.-Y., Ma, R.-Y., Zhang, J.-L., and Zhang, S.-B. (2016). Two strategies by epiphytic orchids for maintaining water balance: thick cuticles in leaves and water storage in pseudobulbs. AoB Plants 8:lw046. doi: 10.1093/aobpla/plw046
Zhang, S., Yang, Y., Li, J., Qin, J., Zhang, W., Huang, W., et al. (2018). Physiological diversity of orchids. Plant Divers. 40, 196–208.
Zotz, G. (1998). Demography of the epiphytic orchid, Dimerandra emarginata. J. Trop. Ecol. 14, 725–741. doi: 10.1017/S0266467498000534
Zotz, G. (2013). The systematic distribution of vascular epiphytes – a critical update. Bot. J. Linn. Soc. 171, 453–481. doi: 10.1111/boj.12010
Zotz, G., and Hietz, P. (2001). The physiological ecology of vascular epiphytes: current knowledge, open questions. J. Exp. Bot. 52, 2067–2078. doi: 10.1093/jexbot/52.364.2067
Zotz, G., and Tyree, M. T. (1996). Water stress in the epiphytic orchid, Dimerandra emarginata (G. Meyer) Hoehne. Oecologia 107, 151–159.
Zuur, A. F., Ieno, E. N., Walker, N. J., Saveliev, A. A., and Smith, G. M. (2009). Mixed effects models and extensions in ecology with R. 574. New York, NY: Springer. doi: 10.1007/978-0-387-87458-6
Keywords: Orchidaceae, population dynamics, host preference, integral projection models, life table response experiment, Quercus, Oncidium, Alamania
Citation: Ramírez-Martínez A, Ticktin T and Mondragon D (2022) Host tree species effects on long-term persistence of epiphytic orchid populations. Front. Ecol. Evol. 10:1059136. doi: 10.3389/fevo.2022.1059136
Received: 30 September 2022; Accepted: 21 November 2022;
Published: 13 December 2022.
Edited by:
Dennis Whigham, Smithsonian Institution, United StatesReviewed by:
Spyros Tsiftsis, International Hellenic University, GreeceBertrand Schatz, Centre National de la Recherche Scientifique (CNRS), France
Copyright © 2022 Ramírez-Martínez, Ticktin and Mondragon. This is an open-access article distributed under the terms of the Creative Commons Attribution License (CC BY). The use, distribution or reproduction in other forums is permitted, provided the original author(s) and the copyright owner(s) are credited and that the original publication in this journal is cited, in accordance with accepted academic practice. No use, distribution or reproduction is permitted which does not comply with these terms.
*Correspondence: Demetria Mondragon, ZG1vbmRyYWdvbkBpcG4ubXg=