Stoichiometry and stable isotopes of plants and their response to environmental factors in boreal peatland, Northeast China
- 1Key Laboratory of Sustainable Forest Ecosystem Management-Ministry of Education, School of Forestry, Northeast Forestry University, Harbin, China
- 2Key Laboratory of Wetland Ecology and Environment, Northeast Institute of Geography and Agroecology, Chinese Academy of Sciences, Changchun, China
- 3Heilongjiang Sanjiang Plain Wetland Ecosystem Research Station, Fuyuan, China
The alterations of plant composition and diversity pose a threat to the stability of the carbon pool in boreal peatland under climate change. We collected the samples of three plant functional types (deciduous shrubs, evergreen shrubs, and sedge) in seven permafrost peatlands of the Great Hing’an Mountains, China, and measured the properties of total carbon (TC), nitrogen (TN), and phosphorus (TP), their stoichiometric ratios (C:N, C:P, and N:P), and the stable isotope values (δ13C and δ15N) of six tissues (ranging from leaves to roots). For TC, TN, and TP, the contents had an average of 470.69 ± 1.56, 8.03 ± 0.23, and 1.71 ± 0.61 mg·g−1, respectively. TC contents of sedge were lower than those of shrubs for the whole plant. The allocations of N and P to shrub leaves were higher than to stems and roots. There was a similar trend of TN and TP contents, and stoichiometric ratios from leaves to roots between deciduous shrubs and evergreen shrubs. Shrubs and sedge have similar C: N in leaves and fine roots, while leaves of sedge C:P and N:P ratios were higher than shrubs, mainly showed that sedge is N and P co-limitation and shrubs are N limitation. The values of δ13C and δ15N were significantly higher in leaves and roots of sedge than those of shrubs, which means shrubs have higher nutrient acquisition strategies. These results support the shrubs are expanding in the boreal peatland under climate warming through nutrient competition. TC contents of all deciduous shrubs and sedge tissues were positively linear correlated to MAT and the values of δ13C and δ15N in sedge had significant relationships with MAT and MAP. Our results imply warming can increase plant photosynthesis in boreal peatland, and sedge was more sensitive to climate change. These findings would be helpful to understanding the responses of different plant tissues to climate changes in permafrost peatland.
Introduction
The global peatland area only accounts for 3% of the land area (Yu et al., 2010), but with the highest carbon content among all terrestrial ecosystems (Dise, 2009). It was an important global carbon sink and played a key role in mitigating climate change (Gorham, 1991; Beaulne et al., 2021). Plants are an important part of peatland systems, which fix carbon through photosynthesis (Bubier et al., 1999). However, peatland plants have been great changes under climate change (Sun et al., 2010a; Bragazza et al., 2013). Some studies find that shrubs have been expanded in the peatland with warming (Meier and Bowman, 2008; Lyons et al., 2020), while other studies show that graminoids are more sensitive to climate change (Sun et al., 2010b, 2011). These different feedbacks are dependent on vegetation types and research scales (Gao et al., 2006). Nevertheless, more studies focus on aboveground plant dynamics, few studies investigate the changes in plants belowground under climate change (Lyons and Lindo, 2020; Malhotra et al., 2020).
Plant functional types (PFTs) in peatland, which are deciduous shrubs (DS), evergreen shrubs (ES), and sedge, have different carbon cycle pathways and nutrient acquisition strategies (Aerts et al., 2009). Plant stoichiometric characteristics can reflect the utilization of nutrients among different plant species (Liu and Hu, 2020). The higher C: N and C: P ratios can show the higher utilization efficiency of N and P by plants (Herbert et al., 2003; Xu et al., 2022); the lower C: N ratio can characterize that plants have a shorter life span (especially for roots Yu et al., 2022), higher growth rate (Gusewell and Koerselman, 2002; Liu and Hu, 2020), and a fast decomposition rate (Liu et al., 2022); the N:P ratio is closely related to nutrient availability, and can act as an indicator of types of nutrient limitation (Gusewell, 2004). However, it is still few studies that present stoichiometry extent ranging from leaves to roots in peatland PFTs. It is essential to understand the relationships between belowground changes and nutrient cycling.
The stable carbon and nitrogen isotopes in plant different tissues can provide information on plant response to present environmental conditions (Dawson et al., 2002). Climate warming and environmental change can influence the stable isotope abundance of plants. More 13C could be consumed with increasing temperature (Smith et al., 1973; Welker et al., 2003; Rao et al., 2017) and humidity (Amesbury et al., 2015). On the one hand, higher temperature increases enzymatic activity in photosynthesis, thereby affecting carbon isotope fractionation (Farquhar et al., 1989). On the other hand, the opening of plant stomata in a humid environment results in increased stomatal conductance (Smith et al., 1973), leading to a decreased CO2 concentration gradient between the inside and outside of plants (Su et al., 2000), which ultimately reduces δ13C. There was a study found that plant δ15N values have been negatively correlated with temperature, which thought that lower temperature enhanced the viscosity of soil solution, leading to water stress of plants, thus increasing the δ15N value of plants (Liu et al., 2018). There are some research indicates that carbon and nitrogen stable isotopes can also reflect limiting nutrients for plant growth (Piao et al., 2004; Clarkson et al., 2005) and the sources of nutrient elements to a certain extent (Welker et al., 2003; Hobbie et al., 2009). However, carbon and nitrogen isotopes just have been conducted in peatland plant leaves. The belowground biomass of plants was much higher than aboveground in boreal peatland (Wang et al., 2016). Therefore, the relative study of plant roots would be helpful to understand the responses of plants to climate change.
In this study, we collected samples of different plant tissues (i.e., leaves and roots of sedge, leaves, green stems, brown stems, fine roots, medium roots, and coarse roots of shrubs) in five species falling into three plant functional types, namely deciduous shrubs, evergreen shrubs, and sedge in seven typical permafrost peatlands, and analyzed the total carbon (TC), nitrogen (TN), and phosphorus (TP) contents, δ13C, and δ15N values. This study aimed to clarify the different contents of TC, TN, and TP, and carbon and nitrogen isotopes among three PFTs and tissues (leaves, stems, and roots), and to explore the relationships between these plant properties and environmental factors. As mentioned above, a lower C: N ratio can be used as an indicator of a higher plant growth rate, so we hypothesized that the C: N ratio would be significantly negatively correlated with mean annual temperature (MAT). In addition, we hypothesized that the carbon and nitrogen isotopes of deciduous plants would be negatively correlated with MAT and mean annual precipitation (MAP).
Study area, materials, and methods
Study area and a description of plant species
The study area of the current study is in northern Great Hing’an Mountains, Northeast China (50°07′02″–53°33′42”N, 121°10′53″–127°01′21″E) and represents a typical permafrost peatland. This area has an average altitude, mean annual temperature, and mean annual precipitation of 573 m, −3°C, and 400 mm, respectively. The study area shows seasonal rainfall, with over 80% falling between June and August (Sun et al., 2011). Shrubs constitute the dominant vegetation type in the study area. Deciduous shrubs mainly include Betula fruticosa, Vaccinium uliginosum, and Salix myrtilloides. Evergreen shrubs include Ledum palustre, Chamaedaphne calyculata, and Rhododendron lapponicum. Sedges include Carex globularis and Eriophorum vaginatum, and forbs include Saussurea amurensis and Polygonatum odoratum. Bryophytes mainly include Sphagnum palustre and Bryum argenteum. Therefore, the peatland plant community in the study area consists of a shrub-sedge-moss structure, with an obvious and simple vertical structure.
Seven typical permafrost peatlands were examined in the present study (Figure 1; from north to south: Beijicun (BJC), Tuqiang (TQ), Amuer (AME), Pangu (PG), Tahe (TH), Huzhong (HZ), and Xinlin (XL)). A handheld global positioning system (GPS) was used to record the longitude, latitude, and altitude of each site. This study collected samples from common dominant plant species (i.e., C. globularis, B. fruticosa, V. uliginosum, L. palustre, and C. calyculata) in these sites.
Sampling and chemical analyses
During the sampling of sedge tissues, leaves and roots samples were collected, with three replicates of each sample taken from 1 × 1 m plots and 21 plots in total. During the sampling of shrub tissues, samples of leaves, green stems, brown stems, fine roots (0–2 mm), medium roots (2–5 mm), and coarse roots (>5 mm) were collected in triplicate in the above 21 plots. Each final sample was expressed as the mean of the three replicates ± standard error (SE). Leaf and stem samples were placed into kraft paper bags, marked, and dried in an oven at 65°C to a constant weight. Impurities (roots of other plants and peat soil) were removed from roots samples, after which the samples were placed in bags and dried. A ball mill was used to fully crush plant samples to be tested. The present study implemented a dispersed sampling regime to avoid as far as possible plants affected by pests and diseases.
Properties of C, N, and P
The total carbon (TC) content of plants was determined by the combustion method. Here, glucose (400 mg C·g−1) was used as the standard, 15 ± 2 mg of each plant to be tested was extracted, and a total organic carbon analyzer (TOC-Lcph SSM-5000A, Shimadzu, Japan) was used to determine the TC content.
A continuous flow analyzer (Seal AA3, Seal, Germany) was used to determine total nitrogen (TN) and total phosphorus (TP) contents. A 100 mg subsample of each plant to be analyzed was placed into a 50-mL digestion tube, three blank controls were set, and a catalyst was added along with 98% concentrated sulfuric acid. After 8 hours, the solution was boiled until achieving transparency and then allowed to cool to room temperature. Deionized water was then added to the sample to a volume of 50 ml to form the sample solution to be tested. From the sample solution to be tested, 2 ml was extracted and diluted five times using deionized water. Ammonium sulfate solution was then used as a standard to determine the sample N content. A sub-sample of the sample solution to be tested was mixed with 7.2 M sodium hydroxide at a ratio of 3:1, following which potassium dihydrogen phosphate solution was used as the standard to determine the content of P.
Stable isotopes of 13C and 15N
A 100 mg subsample of the sample to be tested was extracted for the measurement of 13C: 12C and 15N: 14N ratios of CO2 and N2 using an elemental analyzer gas stable isotope mass spectrometer (EA-IRMS, Thermo Fisher; Farquhar et al., 1989). Isotope ratios were converted to “δ” unit: δ (‰) = [(Rsample / Rstandard) − 1] × 1,000, where Rsample and Rstandard are the 13C: 12C or 15N: 14N molar abundance ratios of the sample and standard (PDB or atmospheric; Farquhar et al., 1982; Spasojevic and Weber, 2021).
Data analysis
One way analysis of variance (ANOVA) was used to test the differences in plant properties, stoichiometric ratios, and δ13C and δ15N among sites, PFTs, and tissues of plants. Pearson correlation analysis was used to analyze the correlation between C, N, and P contents, δ13C and δ15N, and environmental factors. The MAT and MAP data for each site were downloaded from the National Meteorological Science Data Center1. Dataanalysis and mapping tools used in the present study included ArcGIS 10.2, Excel 2010, Origin 2022, and SPSS 28.0.
All data at each site were expressed as the mean ± SE when analyzing the differences among sites, whereas the mean ± SE of all data of each category in each site was used when analyzing the differences among PFTs or tissues. Leaves and roots of sedge correspond to leaves and fine roots of shrubs, respectively, when the results were analyzed.
Results
Stoichiometry, δ13C and δ15N among all sites
The TC of boreal peatland plants in the Great Hing’an Mountains ranged from 381.28 to 547.40 mg·g−1, with an average of 470.69 ± 1.56 mg·g−1; TN ranged from 1.12 to 30.15 mg·g−1, with an average of 8.03 ± 0.23 mg·g−1; TP ranged from 0.36 to 12.98 mg·g−1, with an average of 1.71 ± 0.61 mg·g−1; C: N ranged from 16.49 to 382.57, with an average of 79.41 ± 1.92; C: P ranged from 39.37 to 1323.04, with an average of 388.89 ± 9.84; N: P ranged from 0.56 to 33.82, with an average of 5.89 ± 0.17; the δ13C value ranged from −31.62 to −25.19‰, with an average of −29.16 ± 0.05‰, and the δ15N value ranged from −7.66 to 2.83‰, with an average of −4.39 ± 0.08‰.
There were differences in plant properties, stoichiometric ratios, and isotope values among the different permafrost peatlands (Table 1). The TC and TN contents of plants in XL and TH sites exceeded those of other sites, whereas plants in XL and BJC sites had the highest TP content. The highest C:N ratio was found in TQ, whereas the highest C:P and N:P ratios were found in plants in PG and TH. The highest δ13C value was found in BJC, whereas the δ15N value in TH was significantly higher than those of other sites (p < 0.05).
Plant properties and stoichiometric ratios among PFTs
Sedge showed the smallest TC content both in leaves and roots (p < 0.05; Figure 2A), its TN content in leaves was significantly lower than that of DS (p < 0.05), and similar to that of ES (Figure 2B). The TP content of sedge was significantly lower than that of shrubs in leaves (p < 0.05), but slightly higher than that of shrubs in roots (Figure 2C). The C:P and N:P ratios of sedge were significantly higher than that of shrubs in leaves (p < 0.05), but lower in roots (Figures 2E,F).
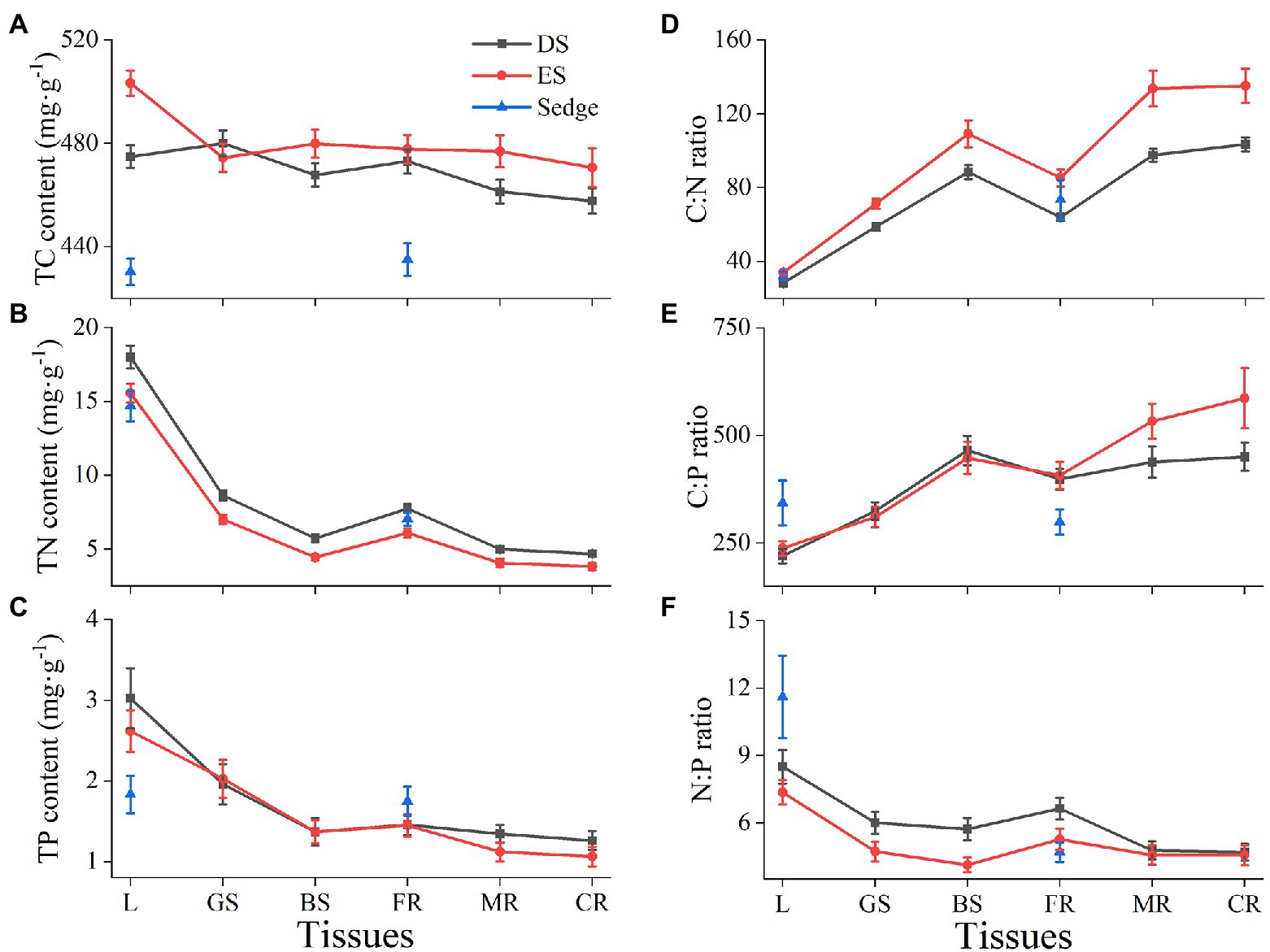
Figure 2. The TC (A), TN (B), and TP (C) content, C:N (D), C: P (E) and N: P (F) ratios among different plant functional types (PFTs) and tissues. Ds, deciduous shrubs; Es, evergreen shrubs; L, leaves; GS, green stems; BS, brown stems; FR, fine roots (0–2 mm); MR, medium roots (2–5 mm); CR, coarse roots (> 5 mm). Data shown are the mean value ± standard error (SE) of all same tissues within PFTs. The same below.
There was a similar change trend of different functional shrubs in properties and stoichiometric ratios among leaves, stems, and roots, but there were differences in quantity (Figure 2). The TC, TN, and TP contents and N: P ratio decreased from leaves to roots, except fine roots (Figures 2A–C,F). On the contrary, the C: N and C: P ratios of shrubs increased from leaves to roots, but appeared low values at fine roots, obviously (Figures 2D,E). In addition, the TC content of ES was significantly higher than that of DS, especially in leaves, except for green stems and fine roots (p > 0.05; Figure 2A). The TN content of DS was higher than that of ES from the whole plant (p < 0.05; Figure 2B). The C: N ratio of DS was lower than that of ES (p < 0.05), and the difference was larger at the roots (Figure 2D), indicating that deciduous shrubs had faster root growth. ES had a higher C: P ratio than DS in medium and coarse roots (p < 0.05; Figure 2E), while had a lower N: P ratio in green stems, brown stems, and fine roots (p < 0.05; Figure 2F).
δ13C and δ15N among PFTs
There were differences in δ13C and δ15N among different PFTs, especially the values of sedge were significantly higher than those of shrubs (p < 0.05; Figure 3).
The δ13C value of ES was lower than that of DS, except for coarse roots (Figure 3A). For sedge, the δ15N values in leaves were significantly higher than those of roots (p < 0.05), while the values of shrubs gradually increased from leaves to roots, with the largest value in fine roots (Figure 3B). The δ15N value of DS was higher than ES, but it decreased from fine roots to coarse roots, and the values were lower than that of ES in coarse roots eventually (Figure 3B).
Relationships between values of properties, stoichiometric ratios, and isotopes and environmental factors
The TC contents from the whole plants of DS and sedge were significantly positively correlated with MAT (p < 0.01), but these contents of leaves, green stems, and coarse roots were significantly positively correlated with MAT in ES (p < 0.05; Supplementary Table S1). The TN contents of leaves, fine roots, and medium roots in DS, brown stems and fine roots in ES, and leaves in sedge were significantly positively correlated with MAT (p < 0.05). There were negative correlations between TP contents of fine roots in DS and roots in sedge and MAT (p < 0.05). The MAP also has an effect on the plant properties (Supplementary Table S2), especially the TC content of DS was significantly positively correlated with MAP (p < 0.05).
There were no significant relationships between MAT and MAP and δ13C values of all tissues for DS (p > 0.05). The δ13C values of leaves, green stems, and fine roots in ES were significantly more negative with increasing MAT (p < 0.05; Figure 4). There were significant correlations between the δ13C values in leaves of sedge and MAT, but not in roots (p < 0.05; Figures 4A,C). δ15N values of leaves and roots in sedge, green stems and brown stems in deciduous shrubs were significantly positively correlated with MAT (p < 0.05; Figures 5A-D). δ13C values of leaves in sedge and medium roots in evergreen shrubs were significantly negatively correlated with MAP (p < 0.05; Figures 6A,B). δ15N values of leaves and roots in sedge, green stems and brown stems in deciduous shrubs were significantly positively correlated with MAP (p < 0.05; Figures 6C-F).
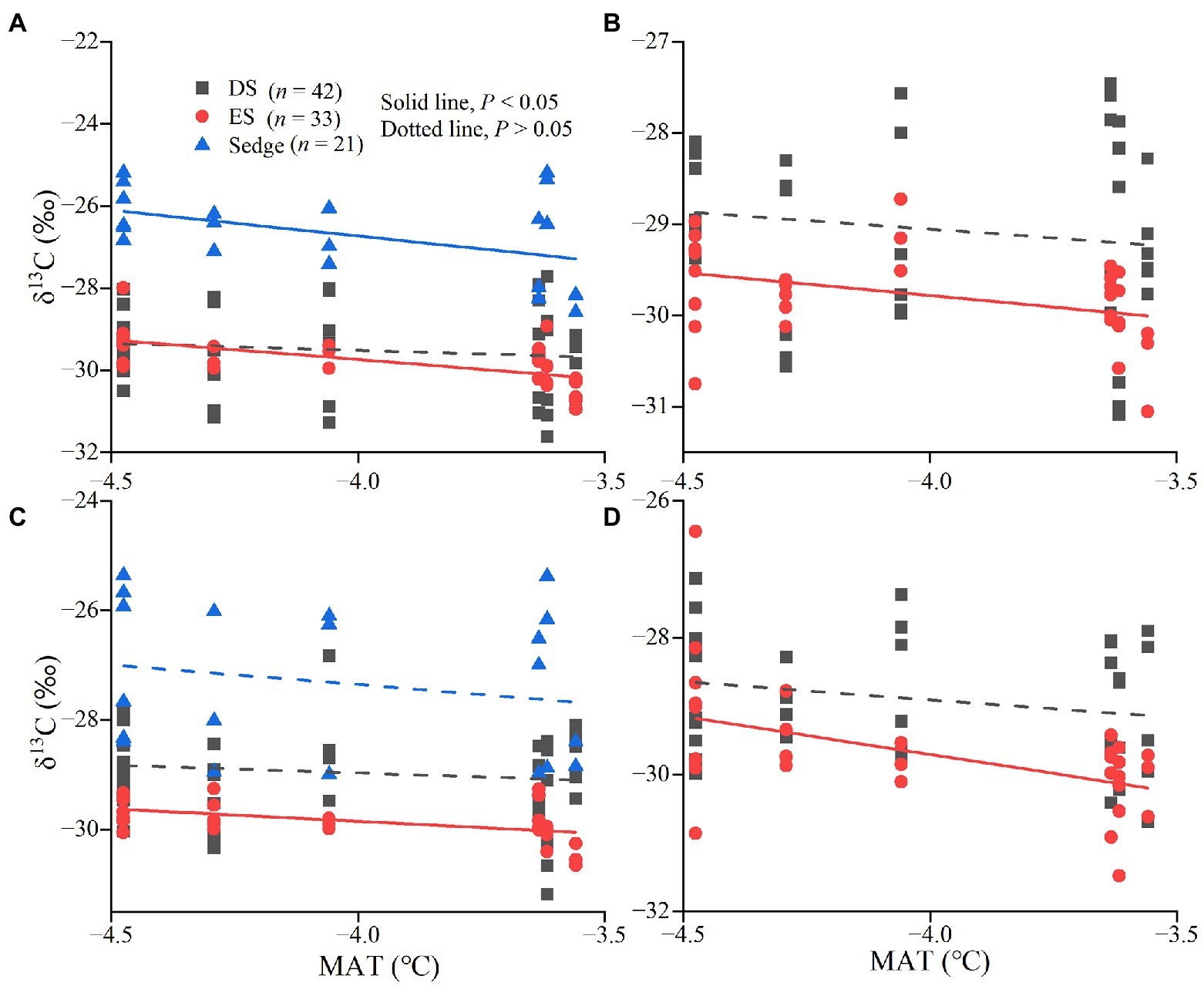
Figure 4. Linear fitting between δ13C values and mean annual temperature (MAT). The relationship between δ13C values of leaves (A), green stems (B), fine roots (C), and medium roots (D) and MAT.
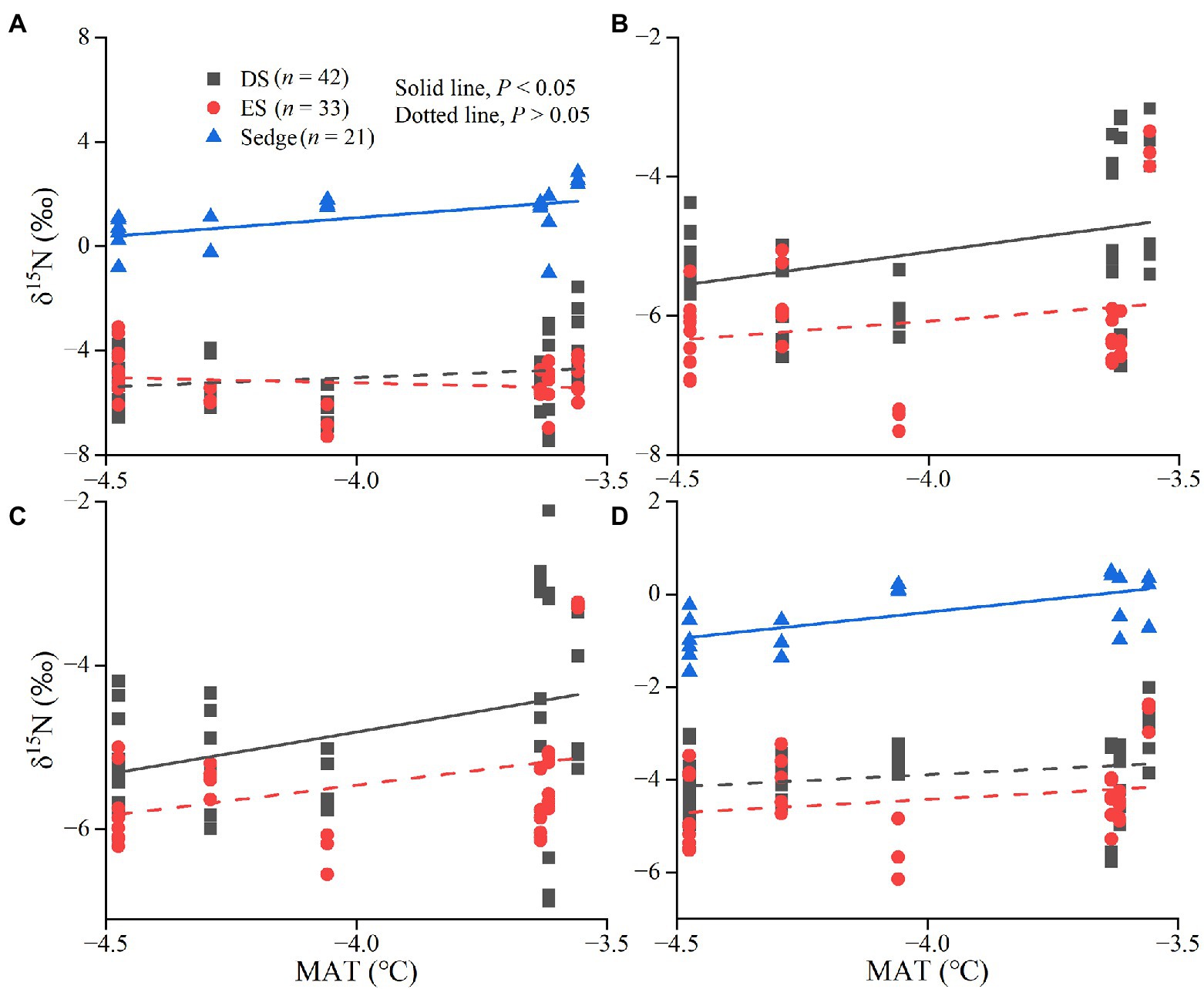
Figure 5. Linear fitting between δ15N value and mean annual temperature (MAT). The relationship between δ15N values of leaves (A), green stems (B), brown stems (C), and fine roots (D) and MAT.
Discussion
Properties and stoichiometric ratios in peatland plants
In this study, the TC contents of sedge tissues were lower than those of shrubs. A similar result was found in the ombrotrophic peatland of Switzerland (Gavazov et al., 2016). Shrubs have more lignin, which may result in higher TC content than sedge (Kastovska et al., 2018; Yu et al., 2022). All tissues of deciduous shrubs showed higher TN content and lower C: N ratio than evergreen shrubs, which means that deciduous shrubs have high resource acquisition strategies and growth rates (Liu and Hu, 2020), especially for the roots. TP content was not changed greatly for whole plants between deciduous and evergreen shrubs, but the C:P ratio of evergreen shrubs was significantly higher than that of deciduous shrubs in the medium and coarse roots. This was also confirmed that deciduous roots grow faster. In our study, the TN, and TP contents decreased from leaves to roots, except for fine roots. It means that the fine roots have a high metabolic rate, which needs higher N and P to maintain normal physiological activities (Yu et al., 2022). Fine roots are the main tissues that absorb mineral nutrients from soil, so the TN and TP contents are higher than those of coarse roots (Nadelhoffer, 2000; Xu et al., 2012; Yu et al., 2022). Fine roots absorb nutrients from soil, then most nutrients are transported to aboveground tissues and accumulated in the stems and leaves (Piao et al., 2004; Wang and Moore, 2014). This causes higher TN and TP contents in leaves.
The plant TN contents of the study area were lower than those of plants in forest peatlands of the Great Hing’an Mountains (Liu and Hu, 2020), in marsh of Sanjiang Plain (Lou et al., 2012), and in wetlands of Europe (Gusewell and Koerselman, 2002). Nitrogen was in general the nutrient limiting the growth of peat plants in permafrost of the Great Hing’an Mountains (Liu et al., 2022), as shown by the plant stoichiometric ratios of peatlands in Canada (Wang and Moore, 2014). It is generally believed that an N: P ratio < 10 or > 20 represents N or P limitation respectively, whereas a ratio of between 10 and 20 represents co-limitation of N and P (Gusewell, 2004). Our study showed a mean N: P ratio for all plants and tissues was 5.86 (<10), representing a strong N limitation. Furthermore, the N: P ratio in leaves of sedge (11.61 ± 1.83) was much higher than that of deciduous and evergreen shrubs (8.50 ± 0.74 and 7.36 ± 0.55, respectively), indicating that the growth of sedge was limited by N and P, while shrubs were limited by N. Shrubs have been expended in boreal peatland under climate warming and permafrost degradation by increasing N availability (Bragazza et al., 2013; Lyons et al., 2020; McCabe, 2020; Song et al., 2021). Only N limitation of shrubs could be one of the reasons that climate change promotes shrub growth, while sedges would continue to be limited by P.
δ13C And δ15N in peatland plants
There was little difference in δ13C values among tissues but varies greatly among different PFTs in our study. The δ13C values of leaves and roots were the highest in sedge (p < 0.05), which might be due to the low content of lignin and lipid (Hobbie and Werner, 2004). The δ13C values of all shrubs tissues were lower than those of sedge tissues, especially for roots, which indicated that shrubs had higher internal water use efficiency and lower stomatal conductance (Brooks et al., 1997). In addition, the high nutrient contents in plants could also improve the photosynthetic capacity of plants (Li and Huang, 2009), thus making the δ13C value more negative. Those indicated that the nutrient flow in shrubs was faster, and could also support that shrubs in peatlands have higher nutrient acquisition strategies (Li, 2015). Furthermore, variations in the carbon isotope composition of CO2 available for photosynthesis may have an impact on this disparity between species (Galimov, 2000). Some studies have found that differences in growth morphology among species, such as plant stature and canopy density of vegetation, will cause changes in δ13C value (Brooks et al., 1997; Amesbury et al., 2015). We found that C: N ratios were negatively correlated with δ13C in deciduous leaves (p < 0.01; Supplementary Table S3), which may be explained by the hardly decomposable compounds (cellulose, etc.) in shrubs (Werth and Kuzyakov, 2010). There was also a significant positive correlation between δ13C and TN of deciduous leaves (Supplementary Table S3), which may be partly related to the photosynthetic process (Welker et al., 2003; Moore and Bubier, 2020).
In our study, the δ15N values of sedge were significantly higher than those of shrubs (p < 0.05). The sedge has long roots which could absorb stable organic nitrogen (Makarov et al., 2014; Feng et al., 2016) or inorganic nitrogen in deep soil (Hobbie and Hobbie, 2006). This led to higher δ15N values for the whole plant in sedge. Furthermore, it may be that sedges use ammonium salts in a larger proportion than nitrate, which will also cause a slight enrichment of plant δ15N values (Welker et al., 2003). Shrub in peatland is the mycorrhizal plant. 15N could be consumed by mycorrhizal fungi during plant nitrogen transport and depleted by mycorrhizal fungi, resulting in lower 15N in the shrubs (Michelsen et al., 1998; Asada et al., 2005; Clarkson et al., 2005). Our results showed that δ15N values of fine roots were higher than the other tissues for shrubs. Mycelium is surrounded in the tips of fine roots with rich 15N (Michelsen et al., 1998; Welker et al., 2003), which leads to high δ15N values in the roots (especially in fine roots) of shrubs. In deciduous brown stems, the δ15N was positively correlated with TN (p < 0.01), but negatively correlated with C: N ratio (p < 0.01; Supplementary Table S4). Skrzypek et al. (2008) showed a similar result, indicating that δ15N reflects the isotopic composition of nitrate assimilated from water. Gavazov et al. (2016) reported that the content of exchangeable nitrogen in soil can affect δ15N abundance. Future studies should combine soil properties and PFTs.
In brief, different PFTs and tissues had different ways of obtaining and using nutrients. For both δ13C and δ15N values, there was a clear change in the trend at the coarse roots (Figure 3), which may be due to root depth (Nadelhoffer et al., 1996; Kohzu et al., 2003) or other reasons. The belowground studies are still limited in peatland and relative studies would be helpful to assess the N cycling and C dynamics in boreal peatlands.
Response to the environment
Our study indicated that all tissues of deciduous shrubs and sedge showed positive linear correlations between TC contents and MAT (p < 0.01), whereas the TC contents of leaves, green stems, and coarse roots in evergreen shrubs were significantly positively correlated with MAT (p < 0.05). This means that warming can increase plant photosynthesis, thereby promoting plant growth (Wu et al., 2019; Zhang et al., 2019), especially for deciduous plants. In addition, we found that the TN content and N:P ratios of deciduous shrubs and sedge were more positively correlated with MAT than evergreen shrubs. This indicated that deciduous plants might become the dominant species of the community, fundamentally altering the peatland ecosystem function (Moore and Bubier, 2020), and even affecting the carbon pool balance of peatland. There was a negative correlation between C: N ratio and temperature in a warming experiment (Liu et al., 2022). But in our study, with the increase in temperature, the C:N ratio has a downward trend but has not reached a significant level, which was different from our first hypothesis. The plant growth in peatlands would be more complex under climate warming. The TC contents of all tissues in deciduous plants were also positively correlated with MAP (p < 0.01). While other properties and stoichiometric ratios did not respond as sensitively to MAP, it means precipitation mainly influenced the plant C fixation in the peatlands.
The δ13C of leaves and green stems in evergreen shrubs, and leaves in sedge showed a negative correlation with MAT in our study. One direct explanation for this observation was that plants close their stoma to avoid excessive evaporation due to a temperature rise, resulting in a decrease in δ13C (Rao et al., 2017). Another explanation was that temperature indirectly affects δ13C by using N (Welker et al., 2003). Previous studies had shown that the melting of permafrost would release a large amount of available nitrogen for plants and increased the nutrient circulation rate (Keuper et al., 2012). These changes could influence stomatal density and leaf thickness, thereby reducing 13C of plant tissue (Piao et al., 2004). Rao et al. (2017) showed that more negative 13C values occurred in wetter environments, whereas some studies believed that there was no correlation between 13C and humidity (Skrzypek et al., 2007; Amesbury et al., 2015). The latter point was similar to our study, there was no significant correlation between δ13C of leaves in shrubs and MAP. It is probably because the permafrost peatland may have sufficient water conditions and shrubs have higher absorption capacity. MAP did not significantly influence the δ13C of aboveground tissues in shrubs.
Most previous studies focused on the influence of mycorrhizal types on δ15N values (Michelsen et al., 1998; Clarkson et al., 2005; Moore and Bubier, 2020), but only a few studies had shown the relationship between δ15N values and environmental factors. We found that δ15N of deciduous stems and sedge were significantly positively correlated with MAT, which was contrary to our second hypothesis. The activity of nitrifying bacteria increased with warming (Kang et al., 2011), and the higher temperature increased the net nitrification potential of soil or increased the soil nitrogen cycle, and finally lead to 15N enrichment in plants (Liu et al., 2007). In our study, the δ15N value of leaves and roots in sedge was positively correlated with MAP. Fewer ammonium ions (NH4+) will be created in humid soil, and more dissolved organic nitrogen will be absorbed by the action of ectomycorrhiza, promoting 15N enrichment in plants (Kang et al., 2011).
Conclusion
Climate warming has changed plant distribution and community structure, which will threaten the carbon pool balance of boreal peatland. We explore the nutrient utilization of different plant functional types and allocation among different tissues (ranging from leaves to roots), and reveal the adaptive survival strategies of different functional plants in boreal peatland to the environment comprehensively. Compared with sedge, shrubs have higher TC contents, and shrubs have a large allocation of nutrients to leaves. Deciduous shrubs have high resource acquisition strategies and growth rates than evergreen shrubs, especially in roots. The growth of sedge is limited by N and P whereas shrubs are limited by N. And shrubs have been proven to have a higher nutrient acquisition strategy in our study, which also explains the shrub expansion of boreal peatland. The rising temperature can increase plant photosynthesis in boreal peatland, and sedge is more responsive to climate change. To better understand the effects of climate change on the carbon pool of boreal peatland, however, future research still needs to incorporate plant properties with litter, soil, and even surface water research.
Data availability statement
The original contributions presented in the study are included in the article/Supplementary material, further inquiries can be directed to the corresponding authors.
Author contributions
SW: writing-original draft preparation. XW and XS: funding acquisition. XW: conceptualization. XS: methodology. GM and YD: investigation. JJ: data curation. All authors contributed to the article and approved the submitted version.
Funding
The work was supported by National Natural Science Foundation of China No. 41971143 and 42271111, and National Science & Technology Fundamental Resources Investigation Program of China No. 2019FY100600.
Acknowledgments
We wish to acknowledge Zhenling Gao for the field work and Jinli Gao for the laboratory analyses.
Conflict of interest
The authors declare that the research was conducted in the absence of any commercial or financial relationships that could be construed as a potential conflict of interest.
The AE BN declared a shared affiliation with the authors XW, NC, and YD at the time of review.
Publisher’s note
All claims expressed in this article are solely those of the authors and do not necessarily represent those of their affiliated organizations, or those of the publisher, the editors and the reviewers. Any product that may be evaluated in this article, or claim that may be made by its manufacturer, is not guaranteed or endorsed by the publisher.
Supplementary material
The Supplementary material for this article can be found online at: https://www.frontiersin.org/articles/10.3389/fevo.2022.1071947/full#supplementary-material
Footnotes
References
Aerts, R., Callaghan, T. V., Dorrepaal, E., van Logtestijn, R. S. P., and Cornelissen, J. H. C. (2009). Seasonal climate manipulations result in species-specific changes in leaf nutrient levels and isotopic composition in a sub-arctic bog. Funct. Ecol. 23, 680–688. doi: 10.1111/j.1365-2435.2009.01566.x
Amesbury, M. J., Charman, D. J., Newnham, R. M., Loader, N. J., Goodrich, J. P., Royles, J., et al. (2015). Carbon stable isotopes as a palaeoclimate proxy in vascular plant dominated peatlands. Geochim. Cosmochim. Acta 164, 161–174. doi: 10.1016/j.gca.2015.05.011
Asada, T., Warner, B. G., and Aravena, R. (2005). Nitrogen isotope signature variability in plant species from open peatland. Aquat. Bot. 82, 297–307. doi: 10.1016/j.aquabot.2005.05.005
Beaulne, J., Garneau, M., Magnan, G., and Boucher, E. (2021). Peat deposits store more carbon than trees in forested peatlands of the boreal biome. Sci. Rep. 11:2657. doi: 10.1038/s41598-021-82004-x
Bragazza, L., Parisod, J., Buttler, A., and Bardgett, R. D. (2013). Biogeochemical plant-soil microbe feedback in response to climate warming in peatlands. Nat. Clim. Chang. 3, 273–277. doi: 10.1038/nclimate1781
Brooks, J. R., Flanagan, L. B., Buchmann, N., and Ehleringer, J. R. (1997). Carbon isotope composition of boreal plants: functional grouping of life forms. Oecologia 110, 301–311. doi: 10.1007/s004420050163
Bubier, J. L., Frolking, S., Crill, P. M., and Linder, E. (1999). Net ecosystem productivity and its uncertainty in a diverse boreal peatland. J. Geophys. Res.-Atmos. 104, 27683–27692. doi: 10.1029/1999jd900219
Clarkson, B. R., Schipper, L. A., Moyersoen, B., and Silvester, W. B. (2005). Foliar N-15 natural abundance indicates phosphorus limitation of bog species. Oecologia 144, 550–557. doi: 10.1007/s00442-005-0033-4
Dawson, T. E., Mambelli, S., Plamboeck, A. H., Templer, P. H., and Tu, K. P. (2002). Stable isotopes in plant ecology. Annu. Rev. Ecol. Syst. 33, 507–559. doi: 10.1146/annurev.ecolsys.33.020602.095451
Dise, N. B. (2009). Peatland response to global change. Science 326, 810–811. doi: 10.1126/science.1174268
Farquhar, G. D., Ehleringer, J. R., and Hubick, K. T. (1989). Carbon isotope discrimination and photosynthesis. Annu. Rev. Plant Physiol. Plant Mol. Biol. 40, 503–537. doi: 10.1146/annurev.pp.40.060189.002443
Farquhar, G. D., Oleary, M. H., and Berry, J. A. (1982). On the relationship between carbon isotope discrimination and the inter-cellular carbon-dioxide concentration in leaves. Aust. J. Plant Physiol. 9, 121–137. doi: 10.1071/pp9820121
Feng, W., Xu, L., Wang, X., Li, H., and Jiang, J. (2016). Response of Carex cinerascens populations to groundwater level gradients in the Poyang Lake wetland. Acta Ecol. Sin. 36, 5109–5115. doi: 10.5846/stxb201501220180
Galimov, E. M. (2000). Carbon isotope composition of Antarctic plants. Geochim. Cosmochim. Acta 64, 1737–1739. doi: 10.1016/s0016-7037(99)00328-2
Gao, J., Ma, K., and Feng, Z. (2006). Effects of landscape composition, structure and gradient pattern on plant diversity. Chin. J. Ecol. 25, 1087–1094. doi: 10.1016/S1872-2032(06)60052-8
Gavazov, K., Hagedorn, F., Buttler, A., Siegwolf, R., and Bragazza, L. (2016). Environmental drivers of carbon and nitrogen isotopic signatures in peatland vascular plants along an altitude gradient. Oecologia 180, 257–264. doi: 10.1007/s00442-015-3458-4
Gorham, E. (1991). Northern peatlands-role in the carbon-cycle and probable responses to climatic warming. Ecol. Appl. 1, 182–195. doi: 10.2307/1941811
Gusewell, S. (2004). N: P ratios in terrestrial plants: variation and functional significance. New Phytol. 164, 243–266. doi: 10.1111/j.1469-8137.2004.01192.x
Gusewell, S., and Koerselman, M. (2002). Variation in nitrogen and phosphorus concentrations of wetland plants. Perspect. Plant Ecol. Evol. Syst. 5, 37–61. doi: 10.1078/1433-8319-0000022
Herbert, D. A., Williams, M., and Rastetter, E. B. (2003). A model analysis of N and P limitation on carbon accumulation in Amazonian secondary forest after alternate land-use abandonment. Biogeochemistry 65, 121–150. doi: 10.1023/a:1026020210887
Hobbie, J. E., and Hobbie, E. A. (2006). N-15 in symbiotic fungi and plants estimates nitrogen and carbon flux rates in Arctic tundra. Ecology 87, 816–822. doi: 10.1890/0012-9658(2006)87[816,Nisfap]2.0.Co;2
Hobbie, J. E., Hobbie, E. A., Drossman, H., Conte, M., Weber, J. C., Shamhart, J., et al. (2009). Mycorrhizal fungi supply nitrogen to host plants in Arctic tundra and boreal forests: N-15 is the key signal. Can. J. Microbiol. 55, 84–94. doi: 10.1139/w08-127
Hobbie, E. A., and Werner, R. A. (2004). Intramolecular, compound-specific, and bulk carbon isotope patterns in C-3 and C-4 plants: a review and synthesis. New Phytol. 161, 371–385. doi: 10.1111/j.1469-8137.2004.00970.x
Kang, H., Liu, C., Yu, W., Wu, L., Chen, D., Sun, X., et al. (2011). Variation in foliar delta N-15 among oriental oak (Quercus variabilis) stands over eastern China: patterns and interactions. J. Geochem. Explor. 110, 8–14. doi: 10.1016/j.gexplo.2011.02.002
Kastovska, E., Strakova, P., Edwards, K., Urbanova, Z., Barta, J., Mastny, J., et al. (2018). Cotton-grass and blueberry have opposite effect on peat characteristics and nutrient transformation in peatland. Ecosystems 21, 443–458. doi: 10.1007/s10021-017-0159-3
Keuper, F., van Bodegom, P. M., Dorrepaal, E., Weedon, J. T., van Hal, J., van Logtestijn, R. S. P., et al. (2012). A frozen feast: thawing permafrost increases plant-available nitrogen in subarctic peatlands. Glob. Chang. Biol. 18, 1998–2007. doi: 10.1111/j.1365-2486.2012.02663.x
Kohzu, A., Matsui, K., Yamada, T., Sugimoto, A., and Fujita, N. (2003). Significance of rooting depth in mire plants: evidence from natural (15) N abundance. Ecol. Res. 18, 257–266. doi: 10.1046/j.1440-1703.2003.00552.x
Li, S. (2015). Mutual Influence Between Santalum Album and Its Host on Water and Nutrient. Bejing, China: Chinese Academy of Forestry.
Li, Y., and Huang, J. (2009). Photosynthetic physiological responses of glycyrrhiza uralensis under different water and nutrient supplies in kubuqi desert, China. Acta Phytoecol. Sin. 33, 1112–1124. doi: 10.3773/i.issn.1005-264x.2009.06.011
Liu, X., and Hu, Y. (2020). C:N:P stoichiometry of leaves and fine roots in typical forest swamps of the greater Hinggan Mountains, China. J. Appl. Ecol. 31, 3385–3394. doi: 10.13287/j.1001-9332.202010.007
Liu, Z., Song, Y., Wang, X., Tan, W., Zhang, H., Gao, J., et al. (2022). Effects of simulated warming on plant growth and carbon and nitrogen characteristics in permafrost peatland. Ecol. Environ. Sci. 31, 1765–1772. doi: 10.16258/j.cnki.1674-5906.2022.09.006
Liu, X., Zhang, Y., Su, Q., Li, Z., Feng, T., and Song, Y. (2018). Gradient variation of delta~(15) N values in herbs and its indication to environmental information in the agro-pastoral ecotone in the north of China. J. Univ. Chin. Acad. Sci. 35, 749–760. doi: 10.7523/i.issn.2095-6134.2018.06.006
Liu, X., Zhao, L., Gasaw, M., Gao, D., Qin, D., and Ren, J. (2007). Foliar delta C-13 and delta N-15 values of C-3 plants in the Ethiopia Rift Valley and their environmental controls. Chin. Sci. Bull. 52, 1265–1273. doi: 10.1007/s11434-007-0165-5
Lou, Y., Wang, G., Lu, X., and Jiang, M. (2012). Nitrogen and phosphorus content of vascular plant species in herbaceous marshes in the SANJIANG PLAIN, Northeast China. Fresenius Environ. Bull. 21, 3766–3772.
Lyons, C. L., Branfireun, B. A., McLaughlin, J., and Lindo, Z. (2020). Simulated climate warming increases plant community heterogeneity in two types of boreal peatlands in north-Central Canada. J. Veg. Sci. 31, 908–919. doi: 10.1111/jvs.12912
Lyons, C. L., and Lindo, Z. (2020). Above- and belowground community linkages in boreal peatlands. Plant Ecol. 221, 615–632. doi: 10.1007/s11258-020-01037-w
Makarov, M. I., Onipchenko, V. G., Malysheva, T. I., van Logtestijn, R. S. P., Soudzilovskaia, N. A., and Comelissen, J. H. C. (2014). Determinants of N-15 natural abundance in leaves of co-occurring plant species and types within an alpine lichen heath in the northern Caucasus. Arct. Antarct. Alp. Res. 46, 581–590. doi: 10.1657/1938-4246-46.3.581
Malhotra, A., Brice, D. J., Childs, J., Graham, J. D., Hobbie, E. A., Stel, H. V., et al. (2020). Peatland warming strongly increases fine-root growth. Proc. Natl. Acad. Sci. U. S. A. 117, 17627–17634. doi: 10.1073/pnas.2003361117
McCabe, S.J. (2020). Permafrost Thaw Drives Changes in Plant Community Characteristics and Nutrient Stoichiometry, with Increases in the Magnitude of c-cycling in an Arctic Peatland. Columbus, OH: The Ohio State University.
Meier, C. L., and Bowman, W. D. (2008). Links between plant litter chemistry, species diversity, and below-ground ecosystem function. Proc. Natl. Acad. Sci. U. S. A. 105, 19780–19785. doi: 10.1073/pnas.0805600105
Michelsen, A., Quarmby, C., Sleep, D., and Jonasson, S. (1998). Vascular plant N-15 natural abundance in heath and forest tundra ecosystems is closely correlated with presence and type of mycorrhizal fungi in roots. Oecologia 115, 406–418. doi: 10.1007/s004420050535
Moore, T. R., and Bubier, J. L. (2020). Plant and soil nitrogen in an Ombrotrophic peatland, southern Canada. Ecosystems 23, 98–110. doi: 10.1007/s10021-019-00390-w
Nadelhoffer, K. J. (2000). The potential effects of nitrogen deposition on fine-root production in forest ecosystems. New Phytol. 147, 131–139. doi: 10.1046/j.1469-8137.2000.00677.x
Nadelhoffer, K., Shaver, G., Fry, B., Giblin, A., Johnson, L., and McKane, R. (1996). N-15 natural abundances and N use by tundra plants. Oecologia 107, 386–394. doi: 10.1007/bf00328456
Piao, H. C., Zhu, J. M., Zhu, S. F., Yu, D. L., and Ran, J. C. (2004). Altitudinal variations of nutrient concentrations and carbon isotope compositions in a C_3 plant and the effects of nutrient interactions on carbon isotope discrimination in limestone areas of Southwest China. Advance in Earth Sciences 19, 412–418.
Rao, Z., Guo, W., Cao, J., Shi, F., Jiang, H., and Li, C. (2017). Relationship between the stable carbon isotopic composition of modern plants and surface soils and climate: a global review. Earth Sci. Rev. 165, 110–119. doi: 10.1016/j.earscirev.2016.12.007
Skrzypek, G., Kaluzny, A., Wojtun, B., and Jedrysek, M.-O. (2007). The carbon stable isotopic composition of mosses: a record of temperature variation. Org. Geochem. 38, 1770–1781. doi: 10.1016/j.orggeochem.2007.05.002
Skrzypek, G., Paul, D., and Wojtun, B. (2008). Stable isotope composition of plants and peat from Arctic mire and geothermal area in Iceland. Polish Polar Res. 29, 365–376. doi: 10.1007/s11104-007-9321-6
Smith, B. N., Herath, H. M. W., and Chase, J. B. (1973). Effect of growth temperature on carbon isotopic ratios in barley, pea and rape. Plant Cell Physiol. 14, 177–178. doi: 10.1104/pp.14.1.177
Song, Y., Jiang, L., Song, C., Wang, X., Ma, X., Zhang, H., et al. (2021). Microbial abundance and enzymatic activity from tussock and shrub soil in permafrost peatland after 6-year warming. Ecol. Indic. 126:107589. doi: 10.1016/j.ecolind.2021.107589
Spasojevic, M. J., and Weber, S. (2021). Variation in delta C-13 and delta N-15 within and among plant species in the alpine tundra. Arct. Antarct. Alp. Res. 53, 340–351. doi: 10.1080/15230430.2021.2000567
Su, B., Han, X. G., Li, L. H., Huang, J. H., Bai, Y. F., and Qu, C. M. (2000). Responses of δ13C value and water use efficiency of plant species to environmental gradients along the grassland zone of Northeast China transect. Chin. J. Plant Ecol. 24, 648–655.
Sun, J., Li, X., Wang, X., Lv, J., and Li, Z. (2010a). Plant species distribution in permafrost wetlands of the great Hing'an mountain valleys and its response to global climate change. J. Earth Sci. 21, 266–270. doi: 10.1007/s12583-010-0232-8
Sun, J., Li, X., Wang, X., Lv, J., Li, Z., and Hu, Y. (2010b). Analysis of structures of permafrost wetland plant communities along environmental gradients in the Da Hinggan Mountains, China. Acta Phytoecol. Sin. 34, 1165–1173. doi: 10.3724/SP.J.1088.2010.00432
Sun, J., Li, X., Wang, X., Lv, J., Li, Z., and Hu, Y. (2011). Latitudinal pattern in species diversity and its response to global warming in permafrost wetlands in the great Hing'an mountains, China. Russ. J. Ecol. 42, 123–132. doi: 10.1134/s1067413611020111
Wang, P., Heijmans, M. M. P. D., Mommer, L., van Ruijven, J., Maximov, T. C., and Berendse, F. (2016). Belowground plant biomass allocation in tundra ecosystems and its relationship with temperature. Environ. Res. Lett. 11:055003. doi: 10.1088/1748-9326/11/5/055003
Wang, M., and Moore, T. R. (2014). Carbon, nitrogen, phosphorus, and potassium stoichiometry in an Ombrotrophic peatland reflects plant functional type. Ecosystems 17, 673–684. doi: 10.1007/s10021-014-9752-x
Welker, J. M., Jonsdottir, I. S., and Fahnestock, J. T. (2003). Leaf isotopic (delta C-13 and delta N-15) and nitrogen contents of Carex plants along the Eurasian coastal Arctic: results from the Northeast passage expedition. Polar Biol. 27, 29–37. doi: 10.1007/s00300-003-0562-4
Werth, M., and Kuzyakov, Y. (2010). C-13 fractionation at the root-microorganisms-soil interface: a review and outlook for partitioning studies. Soil Biol. Biochem. 42, 1372–1384. doi: 10.1016/j.soilbio.2010.04.009
Wu, H., Gao, Q., Ganjurjav, H., Li, Y., Yan, Y., Hu, G., et al. (2019). Effects of grazing and simulated warming on plant community structure and productivity of alpine grassland in northern Xizang, China. Chin. J. Plant Ecol. 43, 853–862. doi: 10.17521/cjpe.2018.0288
Xu, G., Fan, X., and Miller, A. J. (2012). “Plant nitrogen assimilation and use efficiency” in Annual review of plant biology. ed. S. S. Merchant, PALO ALTO, CA :ANNUAL REVIEWS vol. 63, 153–182.
Xu, R., Liu, J., Wang, L., Yan, Y., Ma, X., and Li, M. (2022). Analysis of root and leaf functional traits and C, N, P stoichiometry of Cunninghamia lanceolate from different provenances. Acta Ecol. Sin. 42, 6298–6310. doi: 10.5846/stxb202103230765
Yu, H., Gao, R., Yang, W., Yang, L., Li, S., Lin, Y., et al. (2022). Carbon, nitrogen, and phosphorus contents of leaf, root, and soil and their relationships in dominant herbaceous plants in dry-hot valley. Chin. J. Appl. Environ. Biol. 28, 727–735. doi: 10.19675/.cnki.1006-687x.2021.01008
Yu, Z., Loisel, J., Brosseau, D. P., Beilman, D. W., and Hunt, S. J. (2010). Global peatland dynamics since the last glacial maximum. Geophys. Res. Lett. 37:L13402. doi: 10.1029/2010gl043584
Keywords: boreal peatland, ecological stoichiometry, plant functional types, stable carbon isotopic, 15N natural abundance
Citation: Wang S, Wang X, Sun X, Ma G, Du Y and Jiang J (2022) Stoichiometry and stable isotopes of plants and their response to environmental factors in boreal peatland, Northeast China. Front. Ecol. Evol. 10:1071947. doi: 10.3389/fevo.2022.1071947
Edited by:
Ben Niu, Institute of Geographic Sciences and Natural Resources Research (CAS), ChinaReviewed by:
Jiang Peipei, Shandong Normal University, ChinaTao Zhang, Shenyang Agricultural University, China
Copyright © 2022 Wang, Wang, Sun, Ma, Du and Jiang. This is an open-access article distributed under the terms of the Creative Commons Attribution License (CC BY). The use, distribution or reproduction in other forums is permitted, provided the original author(s) and the copyright owner(s) are credited and that the original publication in this journal is cited, in accordance with accepted academic practice. No use, distribution or reproduction is permitted which does not comply with these terms.
*Correspondence: Xianwei Wang, wangxianwei@iga.ac.cn; Xiaoxin Sun, sunxiaoxin@nefu.edu.cn