- 1Natural Research Ltd., Brathens, United Kingdom
- 2Forestry and Land Scotland, Aberfoyle, United Kingdom
- 3RSPB Scotland, Inverness, United Kingdom
- 4Roy Dennis Wildlife Foundation, Forres, United Kingdom
- 5Consultant, Druminault, Invergordon, United Kingdom
With life-history traits involving high survival, low reproductive output, years of natal dispersal and deferred maturity, the population ecology and behaviour of large raptors which occur at low densities can be difficult to study. The age at which large raptors first settle on a prospective breeding territory receives relatively little attention, but is a key metric in population modelling including, for example, projections of reintroduction projects. It can also be a barometer of the “health” of populations and the availability of breeding opportunities. The advancement of GPS-telemetry has proved invaluable in gaining insights into several aspects of large raptor ecology and behaviour. Age of first territory settlement (AFTS) is one such aspect. AFTS is important in modelling population trajectories and considering individuals’ lifetime reproductive success. We used an algorithm based on GPS-records from dispersing Golden Eagles tagged as nestlings in Scotland to estimate AFTS. While the lifespan of GPS-tags can bias against settlement dates of older birds, they can also potentially reveal settlement ages difficult or impossible to discern from other methods. We found a range of ages for AFTS, including those in their second calendar year; much younger than previously documented by other methods. Ground-truthing – when possible and if inevitably slightly delayed – confirmed territory occupation on field-based survey criteria. We found that eagles settled younger in vacant territories and when older in occupied existing territories. Birds’ sex had no effect on AFTS. Birds which dispersed earlier from their natal territory (indicative of “quality” from some previous research) had no association with AFTS. Our results indicate that within technological temporal limits GPS-data can provide for accurate and precise estimations of AFTS including early settlement not consistently or precisely recorded by other methods. Within our study’s variable competitive landscape we found that AFTS was associated with the availability of territorial opportunities but not with the timing of dispersal. These findings have consequences for studying and understanding large raptor population dynamics.
Introduction
Dispersal is a critical but poorly understood behaviour in animal population dynamics (Clobert et al., 2009). The movement between a natal site to where reproduction first takes place is termed natal dispersal to distinguish it from any subsequent moves between breeding sites, termed breeding dispersal (Greenwood, 1980). Following a post-fledging dependence period (PFDP: Weston et al., 2013, 2018) three sequential behavioural phases of natal dispersal have been identified; emigration, transience, and immigration (Stenseth and Lidicker, 1992; Ims and Yoccoz, 1997), although other terms have been used: for example departure, transience and settlement, or start, transfer/wandering and stop (South and Kenward, 2001; Walls et al., 2005; Delgado and Penteriani, 2008; Clobert et al., 2009).
Breeding territory settlement, as the end point of natal dispersal, is a measure underlying natal dispersal distance (NDD; the linear distance between natal site and first location of entry to the breeding population: Greenwood and Harvey, 1982). It is consequently important to understanding gene flow, delimitation of “populations,” and basic population dynamics involving immigration or emigration. Thereby, determining its occurrence underlies an influential life-history feature, especially in long-lived birds such as large raptors (Newton, 1979; Clobert et al., 2009).
When modelling population dynamics, first territory settlement represents recruitment of dispersing birds to the prospective breeding population (Azpillaga et al., 2018) which affects measures of occupied territories and a population’s breeding productivity (Hayhow et al., 2017; Steenhof et al., 2017; Gjershaug et al., 2018). Age of first territory settlement (AFTS) is therefore an important variable in understanding several features of large raptor populations, not just NDD estimates.
Satellite tagging has been used recently to quantify several features of large raptor biology, such as PFDP and the onset of dispersal (Weston et al., 2013, 2018) and survival (Sergio et al., 2018). Millsap et al. (2014) and Murphy et al. (2019) have identified recent advances in satellite tagging, by technology and utilisation, as capable of providing invaluable insights into NDD. Most large raptors are tagged as nestlings to study natal dispersal phases (López-López, 2016). Hence, one difficulty can be that the expected duration of natal dispersal (Newton, 1979; Steenhof et al., 1983; Ferrer, 2001; Struwe-Juhl and Grünkorn, 2007; Whitfield et al., 2009a) may exceed the operational lifespan of satellite tags deployed; for “large raptor tags” either as given by manufacturers (typically ≤3 years: Whitfield and Fielding, 2017) or, as quantified in practice (about 3 years: Sergio et al., 2018). Telemetric data have documented first territory settlement (e.g., Urios et al., 2007; Murphy et al., 2019) but not via a quantified repeatable measure based solely on telemetric records.
In seeking a measure of natal dispersal termination and AFTS using remote telemetric data, however, there may be confusion with the use of temporary settlement areas (TSAs) during a “stop” phase of dispersal, because such movements can be similar to acquisition of a breeding territory (Delgado et al., 2009). Some authors have considered as equivalent the movement behaviour “stops” due to temporary home range use during natal dispersal and the later end of natal dispersal due to breeding territory settlement (Penteriani et al., 2005a,b; Delgado and Penteriani, 2008). For NDD and population dynamics, however, such “stops” are not equivalent: hence there is a need to differentiate. Subsequent short-term excursive movements by settled territorial birds (Watson et al., 2014) also need to be accounted for.
Our study used a novel algorithm, based on GPS satellite telemetry, accounting for these known features of movement behaviour, to determine when a dispersing Golden Eagle Aquila chrysaetos had settled on (occupied) a prospective breeding territory, to estimate AFTS. We cross-checked these remote estimates with field-based observations of territory occupancy when possible.
Using this tool, with field validation, and other data to estimate AFTS, we examined two hypotheses in a resident Scottish population. Our first hypothesis was that AFTS would be lower in territories which were new or unoccupied, as opposed to territories which were occupied (and thereby presumably defended). More youthful AFTS in an open (undefended) territorial landscape has been a notable feature of several reintroduction projects as these involve expanding populations (Morandini et al., 2019). Early AFTS is also, similarly, indicative of a territorial landscape free of occupants due to other causes. These causes typically involve persistent deaths of sub-adults and notably, adults, from persecution or other adverse anthropogenic influences (Balbontín et al., 2003; Whitfield et al., 2004a,b; Penteriani et al., 2005a,b). This can in turn indicate prospective population decline or unfavourable conservation status (Penteriani et al., 2005b; Whitfield et al., 2006).
Our second hypothesis was that birds which initiated dispersal earlier would be of higher quality (Ferrer, 1993a; Walls and Kenward, 1995; Wood et al., 1998), although see Balbontín and Ferrer (2005). As an influential measure in lifetime reproductive success (Newton, 1989) AFTS should consequently be earlier in birds which began dispersal earlier if they were of higher quality.
Materials and Methods
Study Site and Species
Scotland is a small country (c. 80,000 km2) on the north-western edge of temperate Europe, composed of a wide range of land forms and with numerous islands predominantly on its western edge. In 2015 there were about 500 occupied Golden Eagle territories in the Scottish uplands; mainly in regions known as the Highlands and Islands (Hayhow et al., 2017). Territorial Golden Eagles in Scotland are year-round residents (Watson, 2010). Young eagles, after departing from their natal territory, often travel thousands of kilometres during the subsequent dispersal (transience) phase, almost always remaining within Scotland (Watson, 2010; Weston, 2014; Whitfield and Fielding, 2017) on a non-migratory basis (cf higher latitude populations: Kochert and Steenhof, 2002; Sergio and Whitfield, 2021).
The natal dispersal phase (or juvenile dispersal: Ferrer, 1993b; Whitfield et al., 2009b; Weston et al., 2013) may last several years (Watson, 2010). Scotland presents a range of potential territory opportunities for young Golden Eagles from a high-density occupied territorial landscape in the west to – largely through persecution – several vacant potential territories in the east where live food supplies are also apparently greater (Whitfield et al., 2004a,b, 2006, 2007, 2008; Watson, 2010; Hayhow et al., 2017; Whitfield and Fielding, 2017).
Satellite Tagging
Transmitters were fitted to nestlings when they were 50–70 days old, as judged by plumage (Hoechlin, 1976; Peterson, 1997) under appropriate licences granted by Scottish Natural Heritage (SNH) and the British Trust for Ornithology (BTO). Golden Eagles weighed between 3.4 and 5.0 kg at time of tagging and transmitter weights and harnesses were always less than the 3% lower recommended maximum of body weight (Phillips et al., 2003; see also Kenward (2001), Sergio et al. (2015)).
A total of 161 nestlings were fitted with tags between 2007 and 2019. Telemetry data obtained up to mid-November 2020 were used in analyses. Tags were deployed at nests across several regions of Scotland (Fielding et al., 2012; Whitfield and Fielding, 2017). Nestlings were sexed on the basis of biometrics, supplemented by molecular techniques from an opportunistic sample, which confirmed biometric assignations (Weston et al., 2018).
Five Platform Transmitter Terminal (PTT) tag models were used in the present study, the majority manufactured by MTI (Microwave Telemetry Inc., Columbia, MD, United States):
• A total of 80 g North Star (n = 1, 2008). Lithium battery powered tags based on Argos transmission and manufacturer suggested a 3–5 year potential lifespan. Transmitters had a duty cycle that sent daily signals every 3–4 days. Even the best quality Argos locations are approximated c. 150 m accuracy but even these relatively coarse locations have sufficient accuracy to track landscape movements and settlement.
• A total of 105 g GPS/Argos lithium battery tags (n = 21, deployment years 2007–2015). These transmitters only provided one GPS fix per day and transmitted every 10 days. The battery life of transmitters was suggested by MTI to be 2.5 years.
• A total of 70 g solar powered GPS/Argos transmitters (n = 83, deployment years 2007–2019). GPS fixes and transmissions cycles adjusted by pre-programmed fix rate and transmission schedule (duty cycle): maximum fix rate was hourly during daylight hours. Longevity of transmitters was suggested at ≥3 years by MTI.
• A total of 70 g solar powered GPS/GSM transmitters (n = 46, deployment years 2014–2019). Transmission is over the mobile phone (GSM) network and GPS fix rate is dependent on battery charge (dynamic adjusted fix rate dependent on battery charge from 1 per minute to 1 every 2 h). Transmissions are attempted to GSM network twice daily. Longevity of transmitters was suggested at ≥3 years by MTI.
• A total of 95 g solar powered GPS/GSM transmitters (n = 11, deployment years 2016–2018): manufactured by Movetech Telemetry, Norfolk, United Kingdom. GPS fix rate was dynamic on battery charge and no tag longevity information was provided by manufacturer.
All transmitters were fitted using a harness of 13 mm Teflon Ribbon (Bally Ribbon Mills, Bally, PA, United States) using a “X harness method,” otherwise described as a “crossover wing harness” (Thaxter et al., 2016) or “Garcelon-type harness” (García et al., 2021). Fitting involved a breakaway feature within the harnesses by stitching through ribbons with either cotton or linen thread at the central point over the sternum (Kenward, 1987, 2001; García et al., 2021) intended to remain attached for the expected 3–5 year natal dispersal period of this species (Urios et al., 2007; Watson, 2010) and manufacturer’s expected transmitter lifespan.
Satellite tagging should not have adverse effects on study individuals (Sergio et al., 2015, 2018). García et al. (2021) found no adverse effects of our tag harnessing method in many large raptors, including Golden Eagle. There was no evidence of adverse effects of tagging in our study under physiological, behavioural or demographic evaluations (Whitfield and Fielding, 2017).
Identifying Age of First Territory Settlement
Analytical rules, using satellite telemetry, were devised to determine when a dispersing Golden Eagle had settled on (occupied) a prospective breeding territory. This settlement metric conceptually aligns terminology and research concerned with natal dispersal, and broader population dynamics, notably recruitment to a breeding “population” via territory occupancy through settlement (Supplementary Appendix A). Our algorithm was based on three assumptions, which included accounting for possible confounding factors, such as use of TSAs and territorial excursion behaviour (further details in Supplementary Appendix A).
Assumption 1: If the bird is settled on a territorial home range, prospective for breeding, its movements should be relatively restricted spatially, but with temporal longevity, after accounting for possible confounding factors (see above, Supplementary Appendix A, and later Assumptions). This assumption should be particularly evident for nocturnal records when birds should more likely be within their prospective breeding territory when roosting, rather than records during excursive flights during daylight. This assumption was confirmed in the movements of known settled territorial birds (n = 14) satellite tagged in a separate study in Scotland (Supplementary Appendix A).
Assumption 2: If a bird is settled on a territory its movements will be constrained. This can be measured by a threshold distance moved since the last location. Each date, for which records are available, can be summarised by a median location. Median values are preferable to mean values, to account for occasional ex-territory exploratory movements. A value of 10 km as a threshold distance to the last median location was consistent with the movements of known settled territorial birds (n = 14) satellite tagged in a separate study in Scotland (Supplementary Appendix A). This value is also consistent with the typical range use of Scottish territorial birds derived from earlier less accurate methods (McLeod et al., 2002; Haworth et al., 2006). Additionally, 24 h median locations usually showed a greater spatial dispersion than nocturnal counterparts, and so were less reliable indications of average location (through greater standard errors). GSM tags can generate substantial data (maximum was 335,000 over 1,333 days). Although the algorithm works using each location record (e.g., the full 335,000), it is computationally much more efficient using median locations (e.g., the 1,333 median locations).
Assumption 3: Despite Assumption 2, settled birds have excursive flights outside their range (Watson et al., 2014; Whitfield, 2019), beyond the Assumption 2 distance threshold. However, these are of relatively short duration so distance travelled should be averaged over a period of days. A value of 10 days was consistent with the movements of 14 settled tagged adult birds, as referenced under prior Assumptions and in Supplementary Appendix A.
All data processing was undertaken in R (v3.4.0) (R Core Team, 2019). Tag data were pre-processed so that each date with location records was summarised by the median location. Dawn and dusk were estimated for each record according to its location and date using the R suncalc package (v 0.5.0) (Thieurmel and Elmarhraoui, 2019). A record between dawn and dusk was a day time record; after dusk and before dawn, a nocturnal record. Records between midnight and dawn were assigned to the previous day otherwise changes in roost locations between nights would result in spurious locations when calculating the median location from nocturnal locations. We preferred nocturnal data for several reasons, outlined under Assumption 1 and 2 above. Data processing time was also markedly reduced (Supplementary Appendix B).
The Algorithm
The AFTS algorithm has five stages, beginning with identifying the centre of a putative territory. If a bird is settled, its median location over the last 20 days should be a reasonable approximation. A better approximation is possible once settlement has been established.
The second step works backward in time from the last record finding, for each day, the distance from its median location to the median location 10 days earlier, as defined under Assumption 3. If one exists, a date is identified when the distance between days, 10 days apart, is less than the Assumption 2 threshold of 10 km. This is the putative settlement date. If no such date exists the bird has not settled yet.
The next two steps are checks on this putative settlement date. First, the settlement period must be at least 30 days in duration. Secondly, there is a check to ensure that the start of the settlement period is not part of prior TSA movement behaviour or a transient out-of-territory excursion, including possible mate-searching behaviour on mate loss. This check moves the putative settlement date backward in time, before the putative settlement date, to ascertain consistency. If the putative settlement date does not pass these checks then the bird has not yet settled.
Finally, the distances moved are plotted against date. Settlement is always clear as a sharp decrease in the average distance moved. All records are plotted, along with the Minimum Convex Polygon (MCP) for records after the putative settlement date, to verify visually that the conclusion appears sound as regards a bird settled on a specific space typical of the extent of a territory. While the algorithm intends a purely mechanistically objective tool, this final step provides visualisations to check that its outcomes are realistic to practitioners experienced in the biology of the study’s subject.
The R code for the algorithm and all associated steps is in Supplementary Appendix B, and is for nocturnal data, although it is possible to use 24 h data.
Field Validation of Territory Occupation
We used field observations to validate telemetric determinations of territory settlement through occupation. As a minimum, this required records of nest building activities involving a bird with a satellite tag (through visual confirmation of the tag’s aerial) which was apparently paired with a partner within the territory in question (i.e., “territory occupation”: Hardey et al., 2013; Hayhow et al., 2017; Steenhof et al., 2017; Gjershaug et al., 2018). Additional observations of breeding such as eggs laid, incubation behaviour, and/or chicks or fledgelings were also noted whenever possible ensuring minimal disturbance under licenced observations (Hardey et al., 2013).
Marshalling field observations across a country could not match the frequency of telemetric records, and consequently relevant field observations could inevitably only be undertaken later than any estimate of a territory settlement date derived from telemetry. Field observations were also typically undertaken at particular times of the year (Hardey et al., 2013; Hayhow et al., 2017). In addition, beyond nest building, young large raptors are typically less likely to reach any of the reproductive stages leading to fledging than in their later years or in older birds (Steenhof et al., 1983; Sánchez-Zapata et al., 2000; Pedrini and Sergio, 2001; Whitfield et al., 2004a; Azpillaga et al., 2018; Murgatroyd et al., 2018). Hence our analytical cut-off date could also reduce the availability of field evidence of egg-laying through to fledging, i.e., for young eagles which settled on a territory late in the study period there was a lower likelihood of field recording of reproductive activities beyond nest building.
Documenting Territories’ Prior Occupation Status and Dispersal Date
There is a rich historical database of known Golden Eagle territories in Scotland from several national censuses and prior records (Dennis et al., 1984; Green, 1996; Hayhow et al., 2017). Periodic censuses are supplemented by annual efforts undertaken by experienced surveyors, typically members of the Scottish Raptor Study Group (SRSG) who contribute data to national censuses and the Scottish Raptor Monitoring Scheme (SRMS: Challis et al., 2018). For territories which were deemed to be settled by tagged birds both sources were consulted, with an emphasis given to annual surveillance from local SRSG expertise to classify the occupied status immediately prior to the time when the algorithm had estimated AFTS. Blind to hypothesis 1 (see section “Introduction”), SRSG workers were asked to provide information on prior occupied status. Data on prior occupation were cast into two classes on the absence/presence of an occupied territorial opportunity: new/unoccupied and occupied:
1. New: a territory that was unknown from any prior data. Unoccupied: a territory that was known historically but which was not occupied prior to evidence of AFTS from telemetry data. Hereafter such territories are termed vacant.
2. Occupied: a territory that was known to be occupied prior to evidence of a new bird’s settlement through remote data on AFTS. On occasion, historical territories may also be amalgamated through occupants of one territory subsuming the neighbouring land of a former territory into their range use (Whitfield et al., 2007, 2008). If data indicated prior amalgamation, we also classed as occupied the territory identified by the algorithm with a newly settled bird.
Dispersal initiation date of tagged birds was estimated using “method 7” of Weston et al. (2013). Fledgeling date was crudely assigned as 1 August (Watson, 2010; Weston et al., 2018) which with estimated dispersal date initiation allowed an estimation of PFDP (Weston et al., 2018). We could not age nestlings precisely, due to a reluctance from licencing authorities to approve accessing nest sites at critical junctures early in the breeding season. Later, plumage features allowed crude ageing when birds were tagged (50–70 days old: see above). The imprecision in ageing (by a few days), however, was minimal in contrast to the variation in weeks and months which characterised the time spent on natal territories before dispersal initiation. Hence, because the end of PFDP (Weston et al., 2013, 2018) spanned a greater period than was possible at its beginning, our less precise assignation for its beginning (1 August) should not have been influential.
In preliminary analyses there was no correlation between PFDP and post-dispersal days to the estimated AFTS (r = −0.05, p > 0.1). Splitting the data into vacant or occupied did not change this result (vacant ranges r = −0.29, occupied ranges r = −0.21; p > 0.1 for both). Therefore, in statistical analyses we used the time since dispersal variable as of interest in representing AFTS.
Statistical Analyses
The variable of interest was the number of days taken to settle, since dispersal, and the three possible predictors were: sex of the bird; previous range status (vacant/occupied) and number of days from fledging to dispersal initiation (estimated PFDP). Seven general linear models (GLMs) were constructed using all combinations of the three predictors (single, double, and all three), without interactions. Interactions were not considered because the sample size did not justify a large number of coefficients. The best model was identified via the lowest Akaike’s Information Criterion (AIC) score and was subsequently subjected to tests of model assumptions. We used the lme function from the nlme package (3.1-145), in program R (3.6.1) (R Core Team, 2019; Pinheiro et al., 2020).
Results
The AFTS algorithm determined that 25 satellite tagged birds had occupied a presumptive breeding territory (Table 1 and Figure 1). There were no records of a bird settling in summer (Table 1). Visual checks for these settled birds via MCPs confirmed movements consistent with territory settlement (Figure 2). Within the wider data pool (see section “Materials and Methods”) there were several other birds older or of comparable age which were not assigned as settled by the algorithm (Figure 3). A summary of the ages and status of tagged birds which had not settled at the analytical cut-off of mid-November 2020 is in Table 2. Descriptive statistics on the longevity and status of tags on non-settled birds is in Supplementary Appendix C.
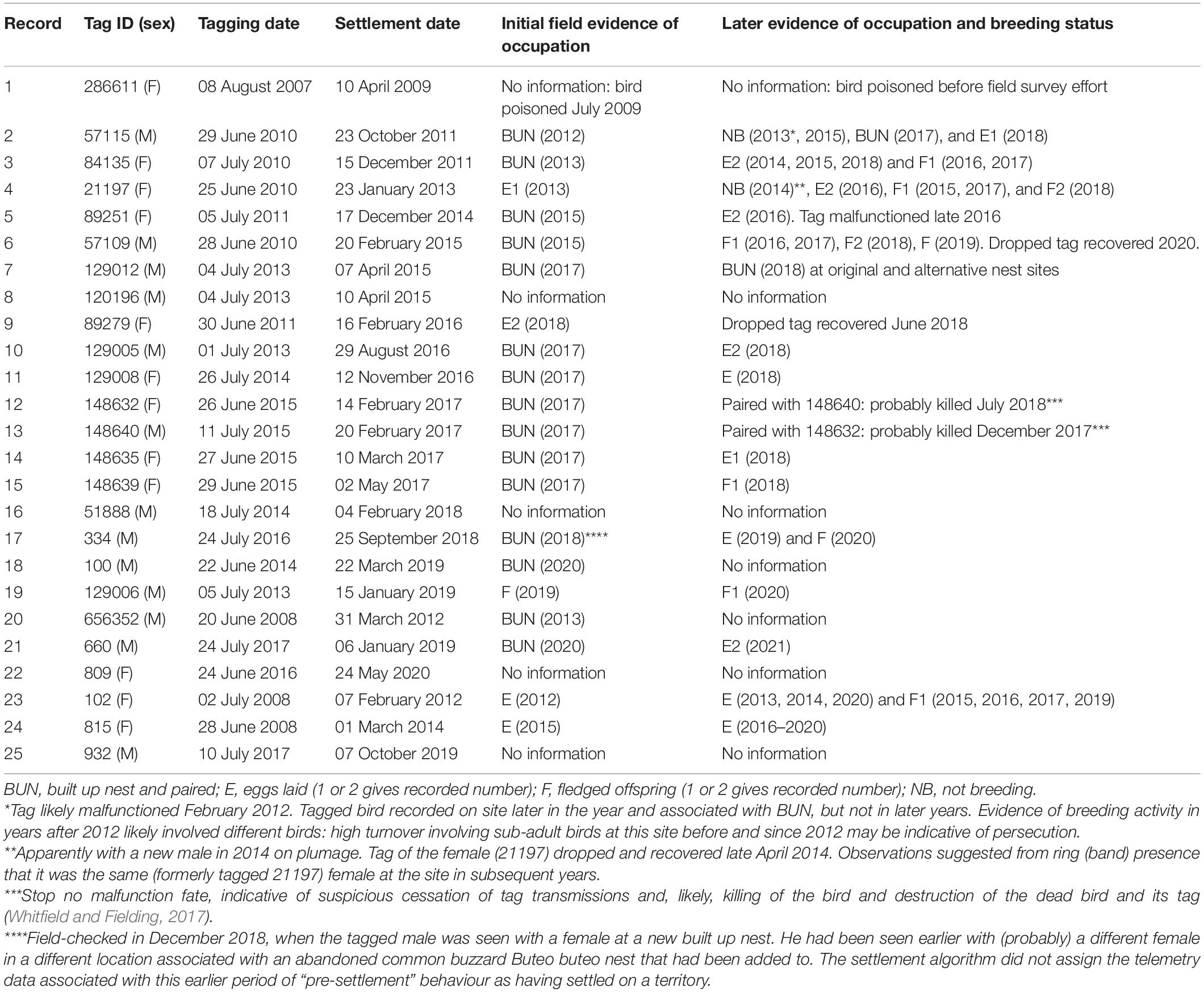
Table 1. The 25 satellite tagged birds deemed to have settled on a prospective breeding territory with a summary of subsequent field records at respective putative territories.
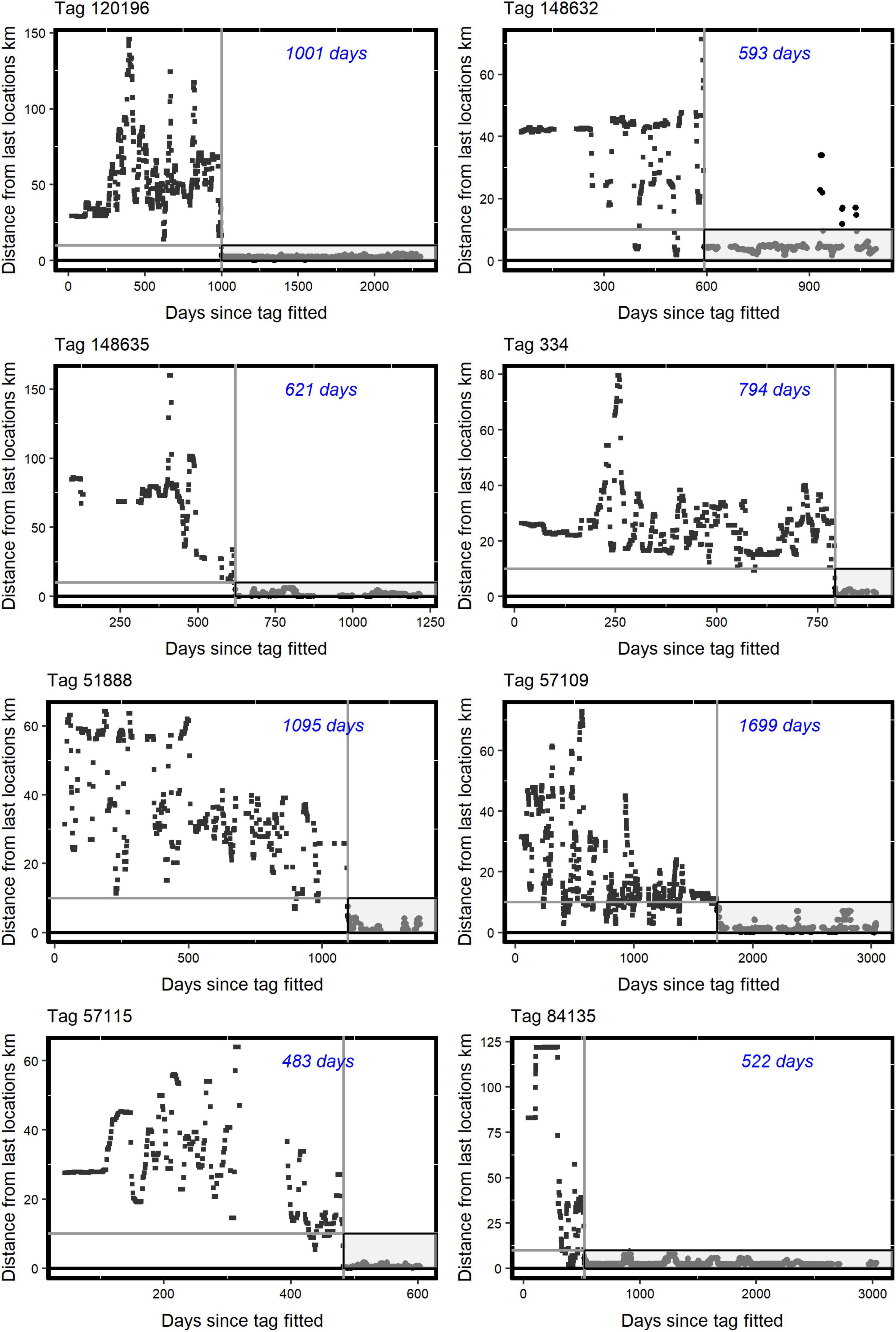
Figure 1. Examples for eight tagged birds (identified, upper left above each panel) deemed to have algorithmically settled on a putative breeding territory according to Distance from last locations (km) in the Y axes, against Days since tagged fitted in the X axes. The Y axes values were based on available median nocturnal locations and refer in distance to the median location 10 days earlier. The vertical grey line identifies the putative settlement date and the horizontal grey line shows the Assumption 2 threshold of 10 km (see main text). The “settlement period,” after the putative settlement date, and according to the Assumption 2 10 km threshold, is shown in greyscale. The italicised blue value (in days: top right within each panel) shows the time between tagging and putative territory settlement. The R code in Supplementary Appendix B provides for such plots.
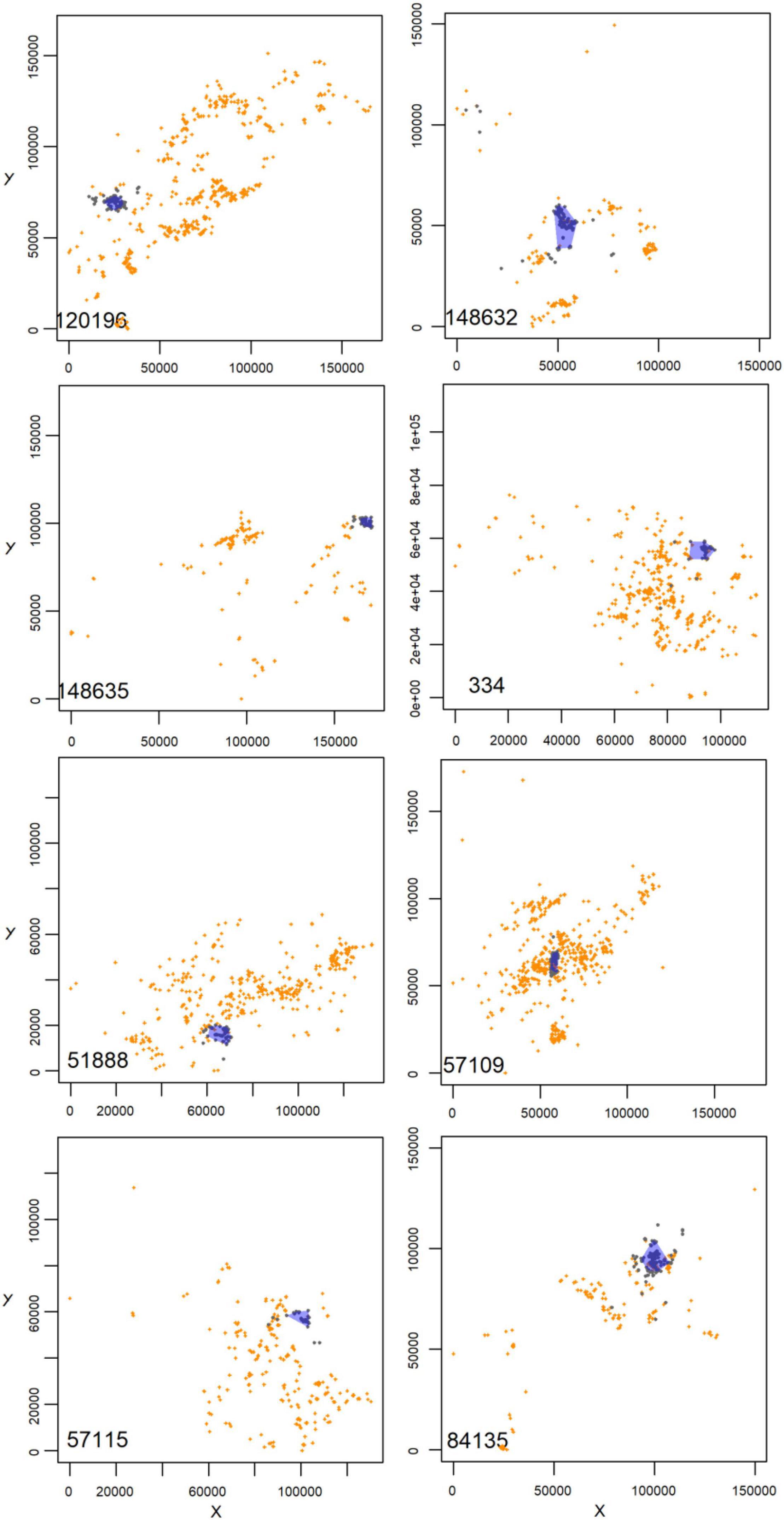
Figure 2. Example plots for eight tagged birds (identified, bottom left in each panel) of Minimum Convex Polygons (MCPs) (in blue transparency) for median nocturnal records post-putative settlement date (dark blue) and with comparable records pre-settlement date (orange) for tagged birds deemed to have settled according to the algorithm. Axes have been re-scaled to zero at origin so as not to reveal geographically explicit reference, for reasons of confidentiality on territory locations. The R code in Supplementary Appendix B provides for such plots.
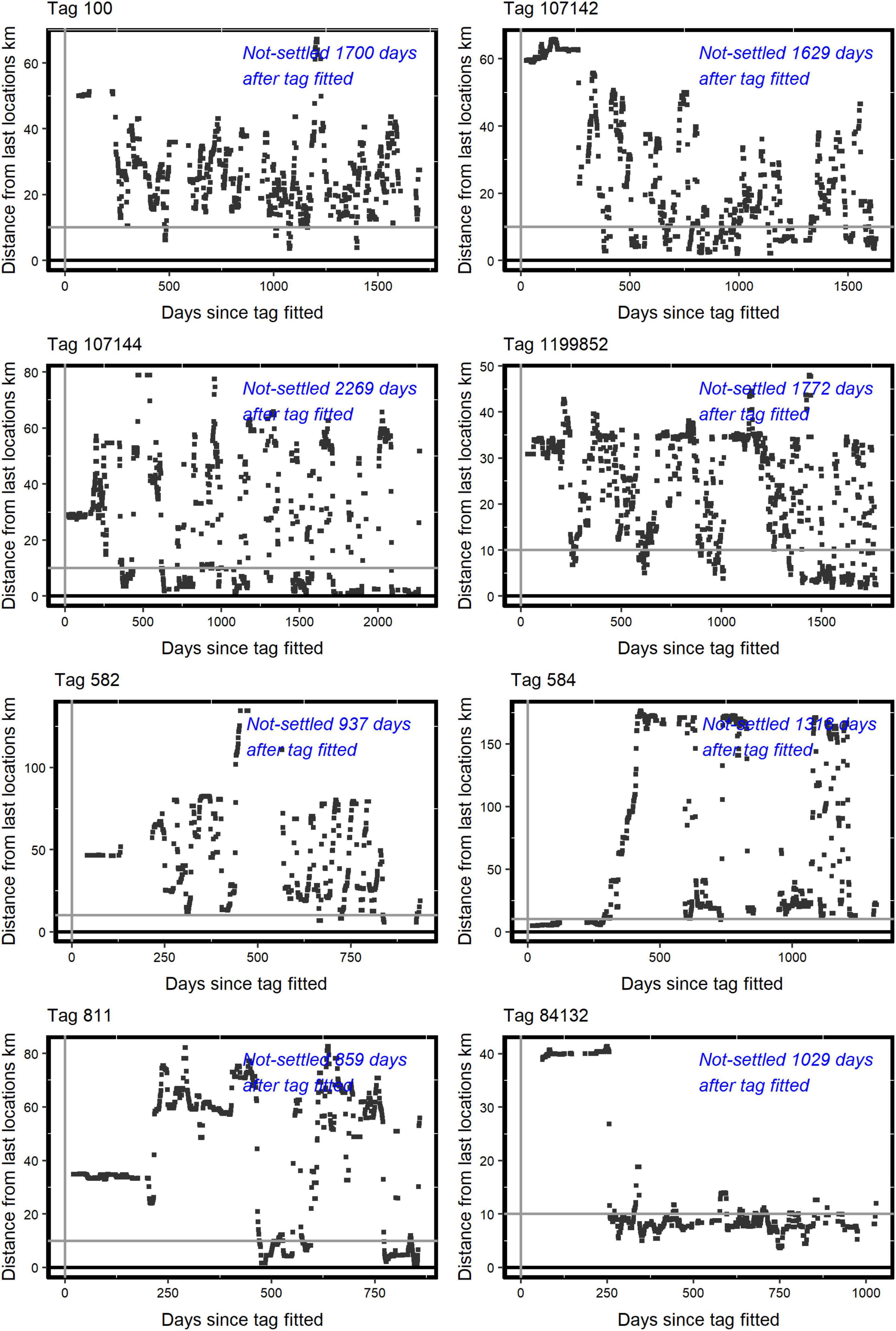
Figure 3. Examples for eight tagged birds (identified, upper left above each panel) deemed not to have algorithmically settled on a putative breeding territory according to Distance from last locations (km) in the Y axes, against Days since tagged fitted in the X axes. The Y axes values were based on available median nocturnal locations and refer in distance to the median location 10 days earlier. The horizontal grey line shows the Assumption 2 threshold of 10 km for territory settlement (see main text). The italicised blue text (in days: top right within each panel) shows the time between tagging and the study’s temporal data cut-off which were available to ascertain that the tagged bird had not yet settled on a putative breeding territory. The R code in Supplementary Appendix B provides for such plots.
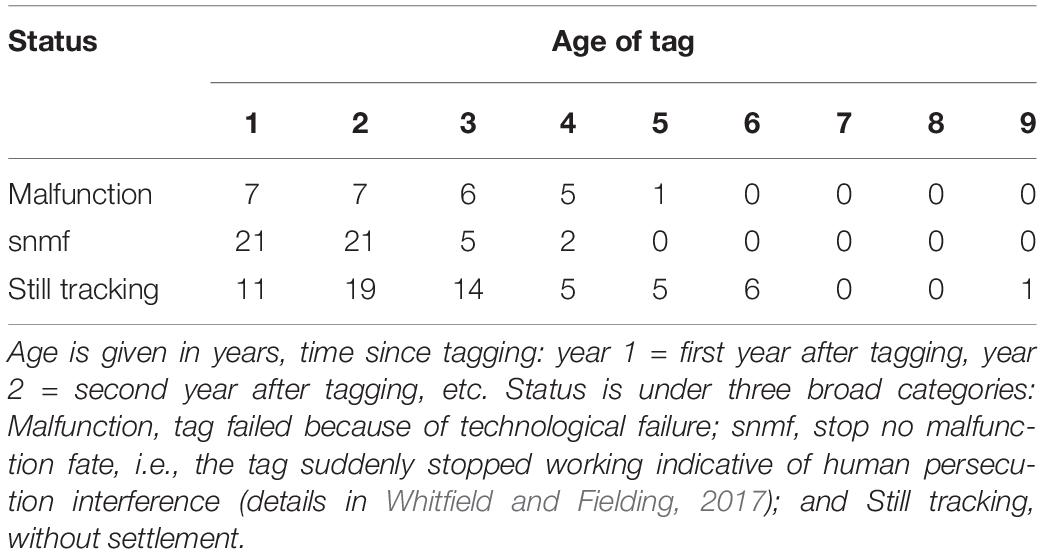
Table 2. Summary of the 136 tags not deemed as settled under the age of first territory settlement (AFTS) algorithm at mid-November 2020 (the study’s analytical cut-off date): according to age and status.
Field efforts on validation of the 25 birds deemed to have settled by the algorithm are summarised in Table 1. Photographic examples of field efforts are in Figure 4.
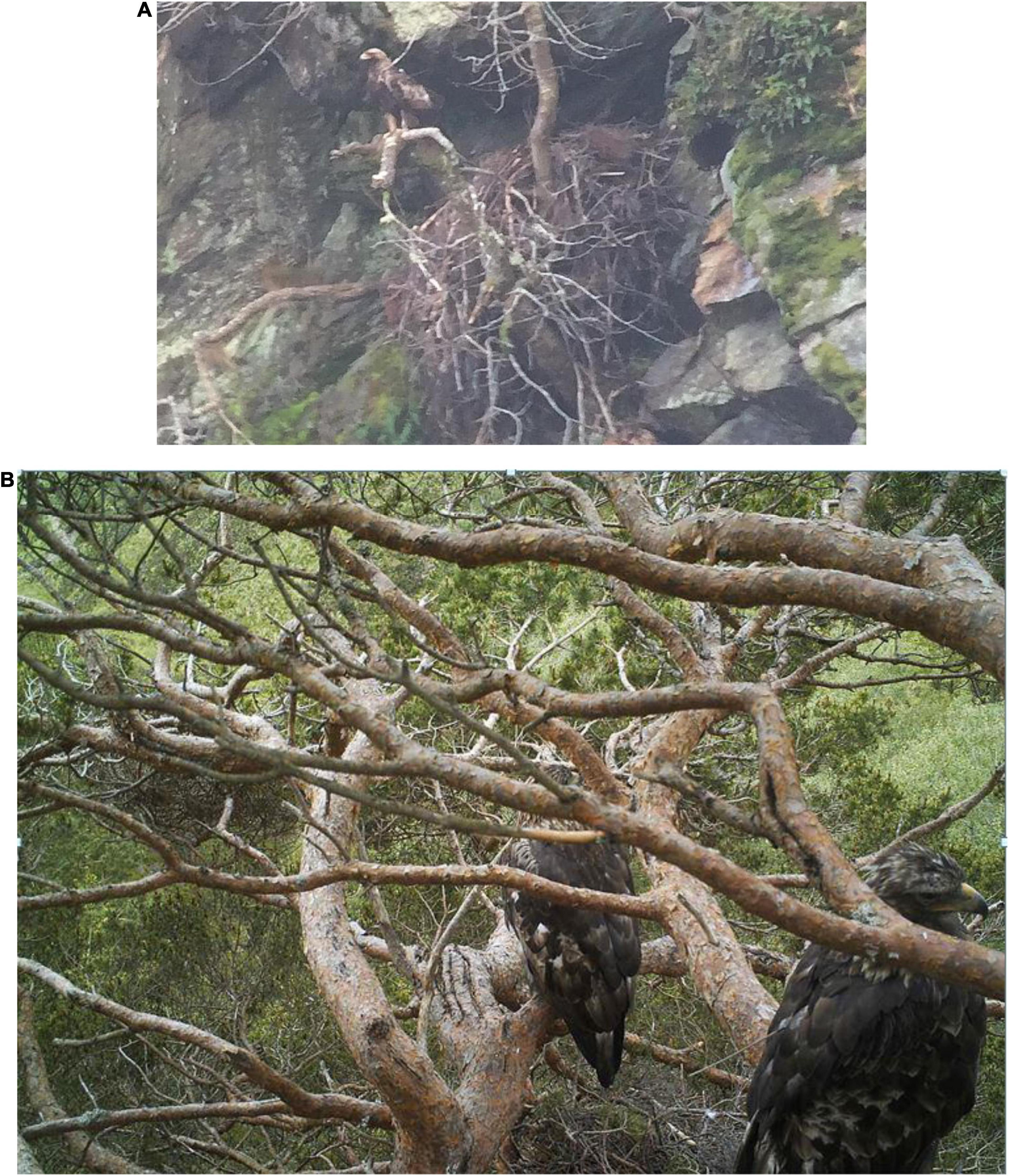
Figure 4. Photographic examples contributing to field validation of territory occupation: (A) tagged female 148632 attending a nest in central Scotland in early April 2017 where she was building up the nest and paired with male 148640, another tagged bird (Table 1) (Photo: Jonathan Clarke); (B) tagged sub-adult female 57115 (foreground) paired with an untagged sub-adult male in northeast Scotland in July 2017 (Photo: Ewan Weston).
For the 25 birds which the settlement algorithm had deemed as settled on a territory, there were five with no data on field validation, either because of human persecution (Record 1: Table 1), or no available fieldwork effort (e.g., Records 8 and 16: Table 1). For the 20 birds where fieldwork was able to check, however, they were all observed to have occupied a territory (i.e., at minimum having built up a nest with a partner) (BUN: Table 1). These data validated, when validation was possible, the AFTS algorithm method: we found no field evidence which invalidated the algorithmic determinations.
Settled territories were approximately equally split between vacant (n = 13) and occupied (n = 12) with no difference in the mean distance from the natal nest to the settled range centroid [vacant 40.8 km (95% CL 19.9–61.7), occupied 44.3 km (95% CL 22.4–46.6)]. However, distances from natal sites to occupied ranges were less variable for occupied ranges (vacant SE = 9.6 km, occupied SE = 5.5 km). Although insignificant, there was weak evidence that females settled further from their natal sites than males (female mean 51.7 km, 95% CL 37.3–66.1; male mean 34.0 km, 95% CL 15.9–52.1). Geographically there was a tendency for previously vacant territories to be in the east of Scotland (Figure 5).
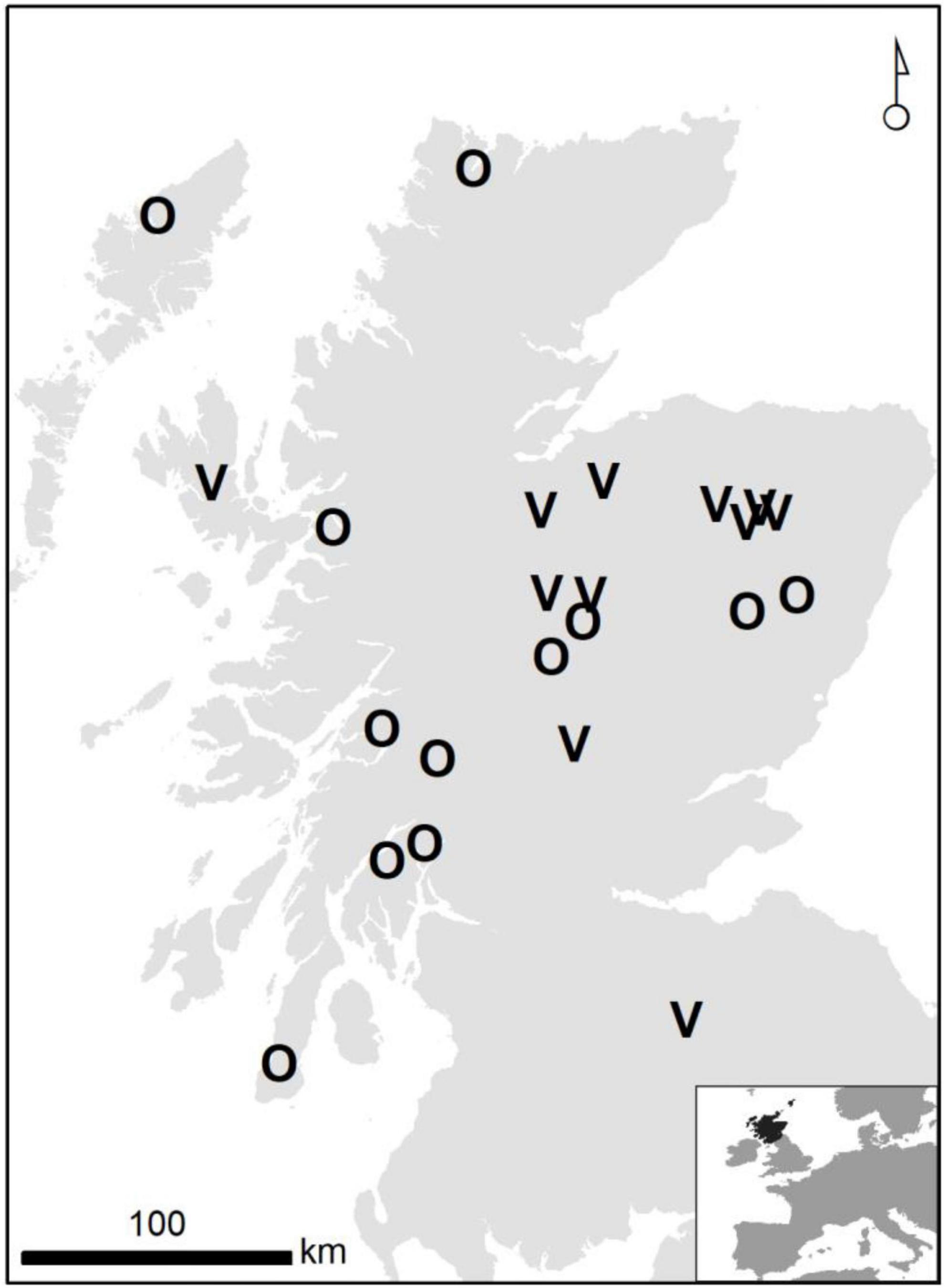
Figure 5. Geographical distribution of territories identified by the AFTS algorithm as newly settled by satellite tagged birds, according to the prior occupation status of the territory (V = vacant, O = occupied). The inset shows the location of Scotland in western Europe.
General linear model analyses showed that only intercepts and previous territory occupation status were significant predictors in any of the models (Table 3). Using AIC, model 2 was marginally better than model 6 (Table 3). There were no issues with the model diagnostics in the AIC preferred model: model 2 predicts that an occupied territory will be occupied 785 days (more than 2 years) later than a vacant territory (Figure 6). Therefore, on hypothesis 1 (see section “Introduction”), analyses indicated AFTS being later in an occupied territory than in a vacant territory (i.e., hypothesis support), and on hypothesis 2 (Introduction), the timing of birds’ dispersal had no influence on AFTS (i.e., hypothesis rejection).
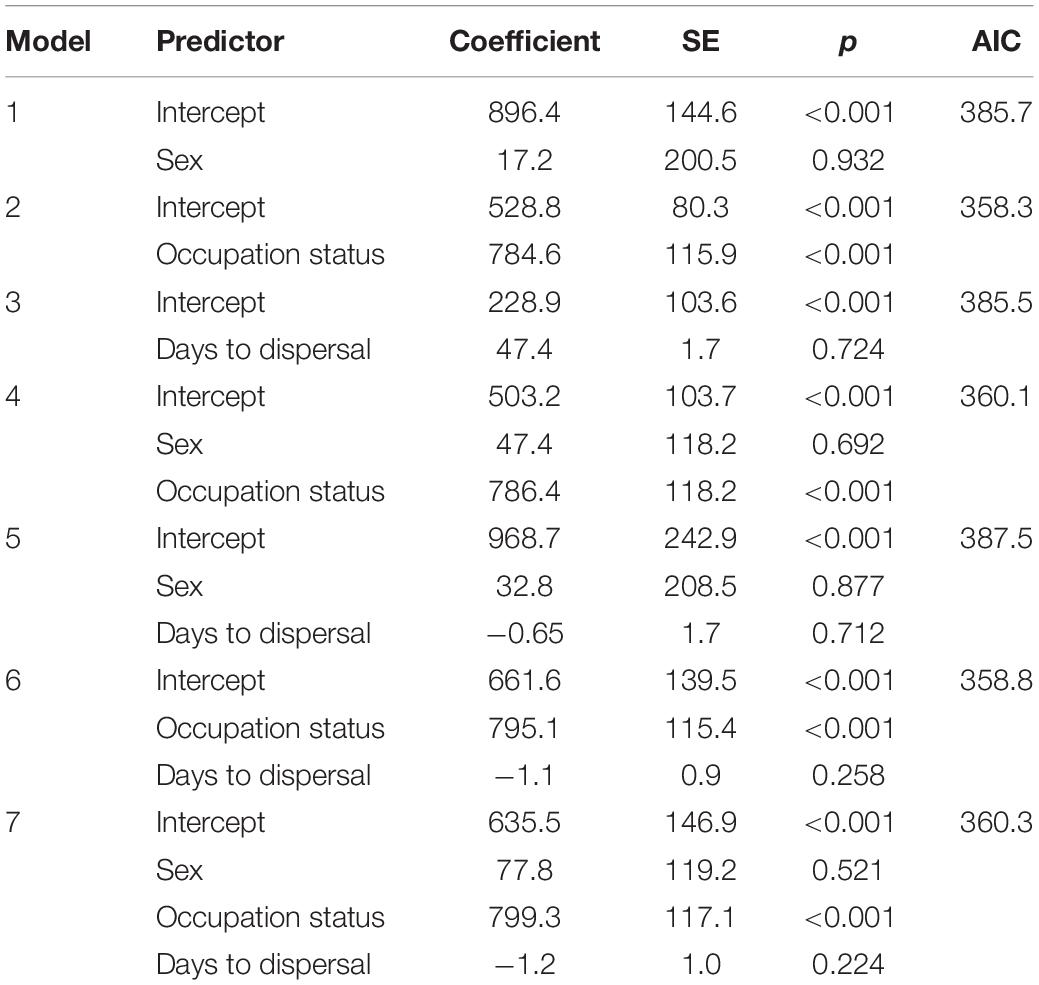
Table 3. Results of seven general linear model (GLM) models examining AFTS (days since dispersal to settlement) with three predictor variables: the bird’s sex, the prior occupation status of the territory where a bird settled (vacant or occupied), and the duration of the bird’s PFDP (days to dispersal).
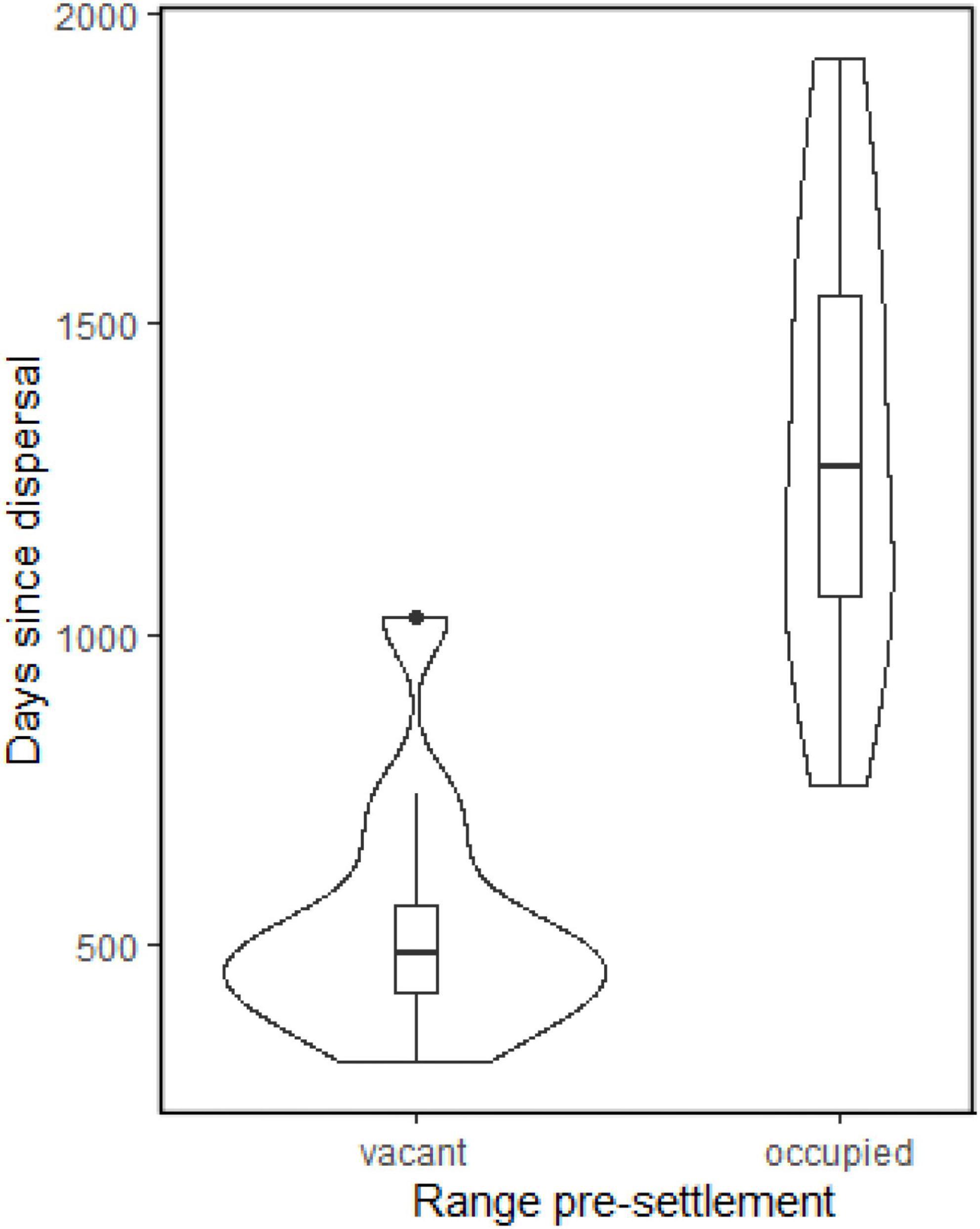
Figure 6. Violin plots of days to settle (AFTS: days since dispersal initiation) with boxplot inserts according to the prior occupation status of the territory where a bird settled (vacant or occupied).
Discussion
Many of the tagged birds we algorithmically determined as having occupied a territory were relatively young, compared to prior expectations (Steenhof et al., 1983; Whitfield et al., 2004b; Murphy et al., 2019). Young Golden Eagles in occupied territories are less likely to reproduce or attempt to reproduce beyond basic occupancy (Steenhof et al., 1983; Sánchez-Zapata et al., 2000; Pedrini and Sergio, 2001; Whitfield et al., 2004a), and presumably thereby spend further years building to reproduction, like the White-tailed Eagle Haliaeetus albicilla (Murgatroyd et al., 2018), which rarely switches territories once settled (Whitfield et al., 2009a). Our dataset was consistent with this post-settlement progression toward reproduction and a shortage of breeding dispersal movements (see also Supplementary Appendix A).
Other studies have used satellite telemetry data when examining AFTS in large raptors (Urios et al., 2007; Cadahía et al., 2009; Murphy et al., 2019). Away from the present study, however, there are no directly comparable studies which have sought to use a set of generic analytical rules based only on telemetric data; without pre-conceptions on when settlement should be more or less likely (Murphy et al., 2019).
Millsap et al. (2014) in analyses of Golden Eagle and Bald Eagle Haliaeetus leucocephalus NDD noted inherent biases in their source ringing (banding) datasets and posited that satellite telemetry data could provide an ideal in overcoming such biases. Murphy et al. (2019) cited this idealised prospect in their research on NDD, based on telemetric data. As noted earlier (see section “Introduction”), however, a potential bias in a telemetric method to assign a date or location to breeding settlement (including our method) lies in the prospect of satellite tags failing mechanically/technically in lifespan before the still-living tagged bird has settled on a territory.
Consequently, as a tool in the study of natal dispersal termination and age of recruitment to “breeding populations” in large raptors, our (or any current telemetric) method is inherently biased by skew away from older settlement ages. This skew opposes that inherent in other non-telemetric methods (including ringing/banding datasets), however. This opposing skew is becoming apparent through telemetric methods which can be independent of traditional field efforts: hence the foresight of Millsap et al. (2014). With continued technological advancement the manufactured lifespan of satellite tags should extend, as it has over recent decades (López-López, 2016) and then the idealised nature of the technology as optimistically espoused by Millsap et al. (2014) and Murphy et al. (2019) could be realised fully.
While necessarily acknowledging a current temporal bias through technological limitation, we suggest that our AFTS method nevertheless provides for satellite telemetry data to document objectively a key juncture in the life history of large raptors, adding to other studies which have also used telemetric data to estimate objectively other critical life history features (Weston et al., 2013; Sergio et al., 2018). The AFTS algorithm is free from pre-conceptions on when putative breeding territory settlement is more or less likely, either by time of year or by age (cf Murphy et al., 2019). It is objectively repeatable, and also adaptable within its assumptions and chosen values, according to biological features of Golden Eagles elsewhere and other large raptors.
Greenwood (1980) suggested that natal dispersal could be classified as either gross (the permanent movement of individuals to a new location irrespective of whether or not they reproduce after settling) or effective (an individual reproduces following dispersal). In our study population, gross dispersal was probably equivalent to effective dispersal. Even though reproduction (the production of fledgelings) was confirmed in a few tagged birds, our study nevertheless suggested equivalency. This was because reproduction beyond settlement/occupation is age-related and may not or rarely occur for some Scottish Golden Eagles even when old (Whitfield et al., 2008) and, notably, breeding dispersal appears unusual (Supplementary Appendix A).
There was strong evidence that prior status of territorial occupancy was highly influential as an opportunity in association with AFTS, such that birds settled younger in vacant territories than in occupied territories. This supported hypothesis 1. The relationship between AFTS and prior territory status where settlement occurred was predictable from previous research (e.g., Balbontín et al., 2003; Whitfield et al., 2004a,b). This research includes reintroduction studies (Evans et al., 1999; Muriel et al., 2010, 2011; Morandini et al., 2019).
Our algorithm allowed documentation of AFTS earlier than most other field-based research when this typically involves large well-established populations. In such large populations, where field monitoring is inevitably spread thin and with few individually identifiable birds, AFTS has been based primarily on observation-only records of settlement age (crudely, by plumage features or – at best occasional - any reading or recovery of metal rings/bands or colour rings/bands or patagial tags) (Steenhof et al., 1983; Whitfield et al., 2004b,2009a,2009b; Murphy et al., 2019). Documenting AFTS by such thinly spread field methods is inevitably delayed often through the timing of field efforts (Hardey et al., 2013; Hayhow et al., 2017; Steenhof et al., 2017; Gjershaug et al., 2018). Such field efforts provided important validation of our AFTS algorithmic approach; but were always later in confirmation than the algorithmic AFTS. Our study has also shown, consequently, the delay in documenting AFTS by field-based methods in the Scottish breeding population.
By contrast to typical field-based monitoring of large established populations, reintroduction projects typically involve a greater capacity to document early AFTS, through smaller population size, greater funding of monitoring (including tagging of all translocated birds), and incentive with expectation that early AFTS should be apparent. Reintroduction projects are typically set up to detect AFTS early, because it is an early indicator of prospective project success. Early AFTS is expected and has been shown (Muriel et al., 2010, 2011; Morandini et al., 2019). Our algorithm has its temporal flaws as acknowledged earlier. However, it allows for detection of AFTS remotely, and independent of differing field-based efforts or the size and status of the monitored population.
Within Scotland, illegal persecution is typically associated with management for driven shooting of Red Grouse Lagopus lagopus scotica and is more prevalent in eastern Scotland (Whitfield et al., 2003, 2004a,2004b, 2006, 2007, 2008; Whitfield and Fielding, 2017). The regional variation in illegal persecution can suppress the number of occupied territories in such eastern areas (Whitfield et al., 2004a,b, 2006, 2007, 2008; Whitfield and Fielding, 2017). With a substantive absence of such persecution in the west (and hence a higher density of occupied territories), this results in a landscape with variable competitive territorial opportunities for non-territorial young eagles. Hence, several open opportunities (vacant territories) occur in parts of the east, but many fewer in the west. Accordingly, in the present study there was an indication that younger settlement was more likely in eastern Scotland than in the west. There were also several examples in our study of how progression beyond AFTS in tagged birds was curtailed by known or likely illegal killing of tagged individuals. These examples occurred in areas previously documented as being prone to illegal persecution (Whitfield et al., 2004a,2007, 2008; Whitfield and Fielding, 2017).
Rejecting hypothesis 2, we found that birds which initiated dispersal earlier (and with a shorter PFDP) did not have an earlier AFTS. Early dispersal initiation has been equated with a bird’s “quality” in some previous studies (Ferrer, 1993a; Walls and Kenward, 1995; Wood et al., 1998) but not all (Balbontín and Ferrer, 2005). AFTS can be a key contributor to future lifetime reproductive success and birds’ fitness (e.g., Newton, 1985, 1989; Brommer et al., 1998). The basis of our second hypothesis was such that early dispersal initiation should consequently be associated with early AFTS.
Our study’s rejection of this hypothesis could have several reasons:
(1) Early entry to the dispersal phase is not indicative of quality in terms of ability to find a territorial opportunity when younger.
(2) Related, several young eagles in Scotland have a prolonged PFDP up to, at extreme, when their parents are actively involved in the following year’s breeding attempt (present study and Weston et al., 2013, 2018: see also Murphy et al., 2017). Extended PFDP may be a mechanism by which, in staying in a safe haven (natal territory), inherently poorer quality young are better prepared to enter the competitive dispersal landscape (even if later), where survival and gaining a territorial foothold should be priorities, and/or.
(3) Our study involved regional variation in vacant territorial opportunities (hence support for hypothesis 1). The capacity for birds’ settling on such opportunities was probably at least partially regionally based, and probably partially related to an inherent “philopatric pull” as revealed by NDD measures we recorded, which are not dissimilar to those documented elsewhere (Millsap et al., 2014). In other words, in our study system a bird’s “quality” insofar as AFTS may also have been influenced by where it started dispersal and how it dispersed geographically.
Hence, while our rejection of hypothesis 2 superficially dispelled a notion that an early dispersal initiation is equivalent to high quality of the disperser regarding AFTS, we urge further research on this issue. This should include that a prolonged PFDP, rather than a rapid initiation of natal (juvenile) dispersal, may be adaptive in bolstering young eagles’ capacity to cope with a competitive environment during natal (juvenile) dispersal.
Data Availability Statement
The original contributions presented in the study are included in the article/Supplementary Material, further inquiries can be directed to the corresponding author.
Ethics Statement
Ethical review and approval was not required for the animal study because such an ethical committee was not required. The study was reviewed and approved by the relevant authorities. Nests were visited and chicks tagged with transmitters under appropriate licences for permission of disturbance, handling, ringing and tagging from the relevant authority managed by Scottish Natural Heritage and British Trust for Ornithology.
Author Contributions
AF analysed the data. DPW and AF conceived the study, wrote the manuscript with co-author contributions. DA, SB, RD, and EW undertook and collated, with additional SRSG assistance, fieldwork validation, and prior territory occupation status data. DA, RD, JG, and EW undertook essential tagging of birds (with SRSG assistance). All authors contributed to the article and approved the submitted version.
Funding
Funding of tags and data download costs came from many sources, notably Natural Research, the Royal Society for the Protection of Birds, Scottish Natural Heritage (SNH), Roy Dennis Wildlife Foundation, Ruth Tingay (RPUK), and SSE. We are grateful to Emma Ahart (SSE) and Peter Robson (Scottish Power Renewables) for gaining permission to use data and to encourage tag funding; and to Des Thompson and Chris Donald for tags funded by SNH. Several individual tags were also funded by private donations. SSE funded analyses and manuscript production as part of their support for the RECMP. Despite this support, SSE had no influence or commentary in the production of this manuscript.
Conflict of Interest
DPW, AF, and EW was employed by Natural Research Ltd.
The remaining authors declare that the research was conducted in the absence of any commercial or financial relationships that could be construed as a potential conflict of interest.
Publisher’s Note
All claims expressed in this article are solely those of the authors and do not necessarily represent those of their affiliated organizations, or those of the publisher, the editors and the reviewers. Any product that may be evaluated in this article, or claim that may be made by its manufacturer, is not guaranteed or endorsed by the publisher.
Acknowledgments
Many people made this study possible. Mike McGrady initiated satellite tagging of Golden Eagles in Scotland along with his unbounded enthusiasm for the species and a desire to understand its biology. Brian Etheridge tagged several birds and we are also extremely grateful too for the considerable supporting fieldwork from many members of SRSG. Staff at MTI and Movetech (tag manufacture and support) and the British Trust for Ornithology (BTO: licencing) were very helpful. We thank Emma Ahart, George Baxter, Andy Jacobs, Iain McMyn, and Thomas Plant of SSE for previous and ongoing assistance in the Regional Eagle Conservation Management Plan’s Golden Eagle research programme (RECMP), which was substantially initiated by our much-missed colleague and friend Paul Haworth who sadly died in July 2018.
Supplementary Material
The Supplementary Material for this article can be found online at: https://www.frontiersin.org/articles/10.3389/fevo.2022.743598/full#supplementary-material
References
Azpillaga, M., Real, J., and Hernández-Matías, A. (2018). Effects of rearing conditions on natal dispersal processes in a long-lived predator bird. Ecol. Evol. 8, 6682–6698. doi: 10.1002/ece3.4165
Balbontín, J., and Ferrer, M. (2005). Factors affecting the length of the postfledging period in the Bonelli’s Eagle Hieraaetus fasciatus. Ardea 93, 189–198.
Balbontín, J., Penteriani, V., and Ferrer, M. (2003). Variations in the age of mates as an early warning signal of changes in population trends? The case of Bonelli’s eagle in Andalusia. Biol. Conserv. 109, 417–423.
Belthoff, J. R., and Ritchison, G. (1989). Natal dispersal of eastern screech owls. Condor 91, 254–265. doi: 10.2307/1368302
Belthoff, J. R., and Ritchison, G. (1990). Natal dispersal: Greenwood (1980) revisited. Condor 92, 803–804.
Brommer, J. E., Pietiäinen, H., and Kolunen, H. (1998). The effect of age of first breeding on Ural owl lifetime reproductive success and fitness under cyclic food conditions. J. Anim. Ecol. 67, 359–369.
Cadahía, L., López-López, P., Urios, V., Soutullo, V., and Negro, J. J. (2009). Natal dispersal and recruitment of two Bonelli’s Eagles Aquila fasciata: a four-year satellite tracking study. Acta Ornithol. 44, 193–198.
Challis, A., Edwards, C., Heavisides, A., Holling, M., Kortland, K., Mattingley, W., et al. (2018). The scottish raptor monitoring scheme: recent developments in good practice monitoring. Bird Study 65, S21–S34.
Clobert, J., Le Galliard, J. F., Cote, J., Meylan, S., and Massot, M. (2009). Informed dispersal, heterogeneity in animal dispersal syndromes and the dynamics of spatially structured populations. Ecol. Lett. 12, 197–209. doi: 10.1111/j.1461-0248.2008.01267.x
Delgado, M. M., and Penteriani, V. (2008). Behavioral states help translate dispersal movements into spatial distribution patterns of floaters. Am. Nat. 172, 475–485. doi: 10.1086/590964
Delgado, M. M., Penteriani, V., Nams, V. O., and Campioni, L. (2009). Changes of movement patterns from early dispersal to settlement. Behav. Ecol. Sociobiol. 64, 35–43.
Dennis, R. H., Ellis, P. M., Broad, R. A., and Langslow, D. R. (1984). The status of the golden eagle in britain. Br. Birds 77, 592–607.
Evans, I. M., Summers, R. W., O’Toole, L., Orr-Ewing, D. C., Evans, R., Snell, N., et al. (1999). Evaluating the success of translocating red kites Milvus milvus to the UK. Bird Study 46, 129–144.
Ferrer, M. (1993a). Ontogeny of dispersal distances in young Spanish imperial eagles. Behav. Ecol. Sociobiol. 32, 259–263.
Ferrer, M. (1993b). Juvenile dispersal behavior and natal philopatry of a long-lived raptor, the Spanish Imperial Eagle Aquila adalberti. Ibis 135, 132–138.
Fielding, A. H., Anderson, D., Benn, S., Dennis, R., Geary, M., Weston, E., et al. (2012). Responses of dispersing GPS-tagged golden eagles Aquila chrysaetos to multiple wind farms across Scotland. Ibis 164, 102–117. doi: 10.1111/ibi.12996
García, V., Iglesias-Lebrija, J. J., and Moreno-Opo, R. (2021). Null effects of Garcelon harnessing method and transmitter type on soaring raptors. Ibis 163, 899–912. doi: 10.1111/ibi.12942
Gjershaug, J. O., Brøseth, H., Kleven, O., Kålås, J.-A., Mattisson, J., and Tovmo, M. (2018). Monitoring methods for the Golden Eagle Aquila chrysaetos in Norway. Bird Study 65, S43–S51.
Greenwood, P. J. (1980). Mating systems, philopatry and dispersal in birds and mammals. Anim. Behav. 28, 1140–1162. doi: 10.1016/s0003-3472(80)80103-5
Greenwood, P. J., and Harvey, P. H. (1982). The natal and breeding dispersal of birds. Annu. Rev. Ecol. Syst. 13, 1–21. doi: 10.1146/annurev.es.13.110182.000245
Hardey, J., Crick, H. Q. P., Wernham, C. V., Riley, H., Etheridge, B., and Thompson, D. B. A. (2013). Raptors. A Field Guide for Surveys and Monitoring, 3rd Edn. Edinburgh: The Stationery Office.
Haworth, P. F., McGrady, M. J., Whitfield, D. P., Fielding, A. H., and McLeod, D. R. A. (2006). Ranging behaviour of golden eagles Aquila chrysaetos in western Scotland according to season and breeding status. Bird Study 53, 265–273.
Hayhow, D. B., Benn, S., Stevenson, A., Stirling-Aird, P. K., and Eaton, M. A. (2017). Status of Golden Eagle Aquila chrysaetos in Britain in 2015. Bird Study 64, 281–294. doi: 10.1080/00063657.2017.1366972
Hoechlin, D. R. (1976). Development of golden eaglets in southern California. West. Birds 7, 137–152.
Ims, R. A., and Yoccoz, N. G. (1997). “Studying transfer processes in metapopulations: emigration, migration, and colonization,” in Metapopulation Biology, Ecology, Genetics and Evolution, eds I. A. Hanski and M. E. Gilpin (San Diego, CA: Academic Press), 247–265. doi: 10.1016/b978-012323445-2/50015-8
Katzner, T. E., Bragin, E. A., and Milner-Gulland, E. J. (2006). Modelling populations of long-lived birds of prey for conservation: a study of imperial eagles (Aquila heliaca) in Kazakhstan. Biol. Conserv. 132, 322–335.
Kenward, R. E. (1987). Wildlife Radio Tagging. Biological Techniques Series. London: Academic Press.
Kochert, M. N., and Steenhof, K. (2002). Golden Eagles in the U.S. and Canada; status, trends, and conservation challenges. J. Raptor Res. 36, (Suppl. 1), 32–40.
Krüger, O., and Lindström, J. (2001). Lifetime reproductive success in common buzzard, Buteo buteo: from individual variation to population demography. Oikos 93, 260–273. doi: 10.1034/j.1600-0706.2001.930209.x
López-López, P. (2016). Individual-based tracking systems in ornithology: welcome to the era of big data. Ardeola 63, 103–136.
McLeod, D. R. A., Whitfield, D. P., Fielding, A. H., Haworth, P. F., and McGrady, M. J. (2002). Predicting home range use by golden eagles Aquila chrysaetos in western Scotland. Avian Sci. 2, 183–198.
Millsap, B. A., Harmata, A. R., Stahlecker, D. W., and Mikesic, D. G. (2014). Natal dispersal distance of bald and golden eagles originating in the coterminous United States as inferred from band encounters. J. Raptor Res. 48, 13–23. doi: 10.3356/jrr-13-00005.1
Morandini, V., Dietz, S., Newton, I., and Ferrer, M. (2019). The role of age of first breeding in modelling raptor reintroductions. Ecol. Evol. 9, 2978–2985. doi: 10.1002/ece3.4979
Morrison, J. L., and Wood, P. B. (2009). Broadening our approaches to studying dispersal in raptors. J. Raptor Res. 43, 81–89. doi: 10.3356/jrr-08-33.1
Murgatroyd, M., Roos, S., Evans, R., Sansom, A., Whitfield, D. P., Sexton, D., et al. (2018). Sex-specific patterns of reproductive senescence in a long-lived reintroduced raptor. J. Anim. Ecol. 87, 1587–1599. doi: 10.1111/1365-2656.12880
Muriel, R., Ferrer, M., Casado, E., and Pérez Calabuig, C. (2010). First successful breeding of reintroduced ospreys Pandion haliaetus in mainland Spain. Ardeola 57, 175–180.
Muriel, R., Ferrer, M., Casado, E., Madero, A., and Calabiug, C. (2011). Settlement and successful breeding of reintroduced Spanish imperial eagles Aquila adalberti in the province of Cádiz (Spain). Ardeola 58, 323–333.
Murphy, R. K., Dunk, J. R., Woodbridge, R., Stahlecker, D. W., LaPlante, D. W., Millsap, B. A., et al. (2017). First-year dispersal of Golden Eagles from natal areas in the southwestern United States and implications for second-year settling. J. Raptor Res. 51, 216–233. doi: 10.3356/jrr-16-80.1
Murphy, R. K., Stahlecker, D. W., Millsap, B. A., Jacobson, K. V., Johnson, A., Smith, C. S., et al. (2019). Natal dispersal distance of Golden Eagles in the southwestern United States. J. Fish Wildl. Manag. 10, 213–218.
Newton, I. (1985). Lifetime reproductive output of female sparrowhawks. J. Anim. Ecol. 54, 241–253. doi: 10.2307/4634
Ogden, R., Heap, E., McEwing, R., Tingay, R., and Whitfield, D. P. (2015). Population structure and dispersal history in Scottish Golden Eagles Aquila chrysaetos revealed by molecular genetic analysis of territorial birds. Ibis 157, 834–848.
Pedrini, P., and Sergio, F. (2001). Density, productivity, diet, and human persecution of golden eagles (Aquila chrysaetos) in the central-eastern Italian Alps. J. Raptor Res. 35, 40–48.
Penteriani, V., Otalora, F., and Ferrer, M. (2005a). Floater survival affects population persistence. The role of prey availability and environmental stochasticity. Oikos 108, 523–534. doi: 10.1111/j.0030-1299.2005.13514.x
Penteriani, V., Otalora, F., Sergio, F., and Ferrer, M. (2005b). Environmental stochasticity in dispersal areas can explain the ‘mysterious’ disappearance of breeding populations. Proc. R. Soc. Lond. B 272, 1265–1269. doi: 10.1098/rspb.2005.3075
Penteriani, V., Otalora, F., and Ferrer, M. (2008). Floater mortality within settlement areas can explain the Allee effect in breeding populations. Ecol. Modell. 213, 98–104. doi: 10.1016/j.ecolmodel.2007.11.009
Phillips, R. A., Xavier, J. C., and Croxall, J. P. (2003). Effects of satellite transmitters on albatrosses and petrels. Auk 120, 1082–1090. doi: 10.2307/4090279
Pinheiro, J., Bates, D., DebRoy, S., Sarkar, D., and R Core Team (2020). nlme: Linear and Nonlinear Mixed Effects Models. R Package Version 3.1-145.
R Core Team (2019). R: A Language and Environment for Statistical Computing. Vienna: R Foundation for Statistical Computing.
Sánchez-Zapata, J. A., Calvo, J. F., Carrete, M., and Martínez, J. E. (2000). Age and breeding success of a golden eagle Aquila chrysaetos population in southeastern Spain. Bird Study 47, 235–237.
Sergio, F., and Whitfield, D. P. (2021). “Golden eagle Aquila chrysaetos,” in European Bird Atlas 2: Distribution, Abundance and Change, eds V. Keller, S. Herrando, P. Vořŕšek, M. Franch, M. Kipson, P. Milanesi, et al. (Madrid: EBCC and Lynx Edicions), 450–451.
Sergio, F., Tanferno, A., Blas, J., Blanco, G., and Hiraldo, F. (2018). Reliable methods for identifying animal deaths in GPS - and satellite-tracking data: review, testing and calibration. J. Appl. Ecol. 56, 562–572. doi: 10.1111/1365-2664.13294
Sergio, F., Tavecchia, G., Tanferna, A., López Jiménez, L., Blas, J., De Stephanis, R., et al. (2015). No effect of satellite tagging on a raptor survival, recruitment, longevity, productivity, social dominance, and its offspring provisioning and condition. J. Appl. Ecol. 52, 1665–1675. doi: 10.1111/1365-2664.12520
South, A. B., and Kenward, R. E. (2001). Mate finding, dispersal distances and population growth in invading species: a spatially explicit model. Oikos 95, 53–58. doi: 10.1034/j.1600-0706.2001.950106.x
Steenhof, K., Kochert, M. N., and Doremus, J. H. (1983). Nesting of subadult golden eagles in southwestern Idaho. Auk 100, 743–747.
Steenhof, K., Kochert, M. N., McIntyre, C. L., and Brown, J. L. (2017). Coming to terms about describing golden eagle reproduction. J. Raptor Res. 51, 378–390. doi: 10.3356/jrr-16-46.1
Stenseth, N. C., and Lidicker, W. Z. (1992). “The study of dispersal: a conceptual guide,” in Animal Dispersal: Small Mammals as a Model, eds N. C. Stenseth and W. Z. Lidicker <suffix>Jr.</suffix> (London: Chapman and Hall), 5–20.
Struwe-Juhl, B., and Grünkorn, T. (2007). Results of colour-ringing white-tailed sea Eagles Haliaeetus albicilla in Schleswig-Holstein: site fidelity, movements, dispersal, age of first breeding, age structure and breeding of siblings. Vogelwelt 128, 117–129.
Thaxter, C. B., Ross-Smith, V. H., Clark, J. A., Clark, N. A., Conway, G. J., Masden, E. E., et al. (2016). Contrasting effects of GPS device and harness attachment on adult survival of Lesser Black-backed Gulls Larus fuscus and Great Skuas Stercorarius skua. Ibis 158, 279–290.
Thieurmel, B., and Elmarhraoui, A. (2019). suncalc: Compute Sun Position, Sunlight Phases, Moon Position and Lunar Phase. R Package Version 0.5.0.
Urios, V., Soutullo, A., López-López, P., Cadahía, L., Limiñana, R., and Ferrer, M. (2007). The first case of successful breeding of a Golden Eagle Aquila chrysaetos tracked from birth by satellite telemetry. Acta Ornithol. 42, 205–209.
Walls, S. S., and Kenward, R. E. (1995). Movements of radio-tagged common buzzards Buteo buteo in their first year. Ibis 137, 177–182.
Walls, S. S., Kenward, R. E., and Holloway, G. J. (2005). Weather to disperse? Evidence that climatic conditions influence vertebrate dispersal. J. Anim. Ecol. 74, 190–197. doi: 10.1111/j.1365-2656.2005.00914.x
Warkentin, I. G., and James, P. C. (1990). Dispersal terminology: changing definitions in mid-flight? Condor 92, 802–803. doi: 10.2307/1368709
Watson, J. E., Duff, A. A., and Davies, R. W. (2014). Home range and resource selection by GPS-monitored adult Golden Eagles in the Columbia plateau ecoregion: implications for wind power development. J. Wildl. Manag. 78, 1012–1021. doi: 10.1002/jwmg.745
Weston, E. D. (2014). Juvenile Dispersal Behaviour in the Golden Eagle (Aquila chrysaetos). PhD thesis. Scotland: University of Aberdeen.
Weston, E. D., Whitfield, D. P., Travis, J. M. J., and Lambin, X. (2013). When do young birds disperse? Tests from studies of golden eagles in Scotland. BMC Ecol. 13:42. doi: 10.1186/1472-6785-13-42
Weston, E. D., Whitfield, D. P., Travis, J. M. J., and Lambin, X. (2018). The contribution of flight capability to the post-fledging dependence period of golden eagles. J. Avian Biol. 49:e01265.
Whitfield, D. P. (2019). Satellite Tagging of Territorial Golden Eagles: A Joint Natural Research and Forest Enterprise Project. Report from Natural Research. Banchory: Natural Research.
Whitfield, D. P., and Fielding, A. H. (2017). Analyses of the Fates of Satellite Tracked Golden Eagles in Scotland. Scottish Natural Heritage Commissioned Report No. 982. Battleby: SNH.
Whitfield, D. P., Douse, A., Evans, R. J., Grant, J., Love, J., McLeod, D. R. A., et al. (2009a). Natal and breeding dispersal in a reintroduced population of White-tailed Eagles Haliaeetus albicilla. Bird Study 56, 177–186. doi: 10.1080/00063650902792023
Whitfield, D. P., Duffy, K., McLeod, D. R. A., Evans, R. J., MacLennan, A. M., and Reid, R. (2009b). Juvenile dispersal of White-tailed Eagles in western Scotland. J. Raptor Res. 43, 110–120. doi: 10.3356/jrr-08-54.1
Whitfield, D. P., Fielding, A. H., McLeod, D. R. A., and Haworth, P. F. (2004a). The effects of persecution on age of breeding and territory occupation in golden eagles in Scotland. Biol. Conserv. 118, 249–259.
Whitfield, D. P., Fielding, A. H., McLeod, D. R. A., and Haworth, P. F. (2004b). Modelling the effects of persecution on the population dynamics of golden eagles in Scotland. Biol. Conserv. 119, 319–333.
Whitfield, D. P., Fielding, A. H., McLeod, D. R. A., and Haworth, P. F. (2008). A Conservation Framework for Golden Eagles: Implications for their Conservation and Management in Scotland. Scottish Natural Heritage Commissioned Report No. 193. Battleby: SNH.
Whitfield, D. P., Fielding, A. H., McLeod, D. R. A., Haworth, P. F., and Watson, J. (2006). A conservation framework for the golden eagle in Scotland: refining condition targets and assessment of constraint influences. Biol. Conserv. 130, 465–480. doi: 10.1016/j.biocon.2006.01.008
Whitfield, D. P., Fielding, A. H., McLeod, D. R. A., Morton, K., Stirling-Aird, P., and Eaton, M. (2007). Factors constraining the distribution of Golden Eagles Aquila chrysaetos in Scotland. Bird Study 54, 199–211.
Whitfield, D. P., MacLeod, D. R. A., Watson, J., Fielding, A. H., and Haworth, P. F. (2003). The association of grouse moor in Scotland with the illegal use of poisons to control predators. Biol. Conserv. 114, 157–163. doi: 10.1016/s0006-3207(03)00019-3
Keywords: GPS telemetry, juvenile dispersal, natal dispersal distance, raptor population dynamics, satellite tracking, territory occupation
Citation: Whitfield DP, Fielding AH, Anderson D, Benn S, Dennis R, Grant J and Weston ED (2022) Age of First Territory Settlement of Golden Eagles Aquila chrysaetos in a Variable Competitive Landscape. Front. Ecol. Evol. 10:743598. doi: 10.3389/fevo.2022.743598
Received: 18 July 2021; Accepted: 25 January 2022;
Published: 03 March 2022.
Edited by:
Des Thompson, Scottish Natural Heritage, United KingdomReviewed by:
Tricia Miller, Conservation Science Global, Inc., United StatesIan Newton, UK Centre for Ecology and Hydrology (UKCEH), United Kingdom
Miguel Ferrer, Spanish National Research Council (CSIC), Spain
Copyright © 2022 Whitfield, Fielding, Anderson, Benn, Dennis, Grant and Weston. This is an open-access article distributed under the terms of the Creative Commons Attribution License (CC BY). The use, distribution or reproduction in other forums is permitted, provided the original author(s) and the copyright owner(s) are credited and that the original publication in this journal is cited, in accordance with accepted academic practice. No use, distribution or reproduction is permitted which does not comply with these terms.
*Correspondence: D. Philip Whitfield, phil.whitfield@natural-research.org; orcid.org/0000-0003-4255-7782