- 1Department of Biological Sciences and Institute of Environment, Florida International University, Miami, FL, United States
- 2Department of Biological Sciences, Old Dominion University, Norfolk, VA, United States
- 3Department of Environment and Sustainability, University of Buffalo, Buffalo, NY, United States
Habitat degradation alters many ecosystem processes, and the potential for the reestablishment of ecosystem function through restoration is an area of active research. Among marine systems, coastal habitats are particularly vulnerable to anthropogenic degradation and, in response, are the focus of marine ecological restoration. One of the crucial functions of structurally complex coastal habitats (e.g., saltmarshes, seagrass meadows, kelp forests, coral reefs) are as nurseries to coastal and offshore species, many of whose larvae utilize sound to locate suitable nursery habitat. However, the effect of habitat degradation and subsequent restoration on underwater soundscapes and their function as navigational cues for larvae is unexplored. We investigated these phenomena in sponge-dominated hardbottom habitat in the waters surrounding the middle Florida Keys (Florida, United States) that have been degraded in recent decades by massive sponge die-offs caused by harmful algal blooms. One of the consequences of sponge die-offs are dramatic changes in underwater sounds normally produced by sponge-associated animals. We tested whether soundscapes from healthy hardbottom habitat influenced larval recruitment, and then examined how hardbottom degradation and restoration with transplanted sponges affected underwater soundscapes and the recruitment of larval fishes and invertebrates. Larval assemblages recruiting to healthy areas were significantly different than those assemblages recruiting to either degraded or restored hardbottom areas. Fewer larvae recruited to degraded and restored areas compared to healthy hardbottom, particularly during the full moon. Experimental playback of healthy hardbottom soundscapes on degraded sites did not promote larval community differences although some individual species responded to the playback of healthy habitat soundscapes. These results indicate that habitat-associated soundscapes have idiosyncratic effects on larval settlement, which is diminished by the degradation of nursery habitat but can be reestablished with appropriate habitat restoration.
Introduction
Environments unspoiled by humans no longer exist. The direct and indirect influences of anthropogenic disturbance have extensively altered earth’s environments for millennia, particularly since the onset of the Industrial Revolution (e.g., Boivin et al., 2016). For example, deforestation due to logging and agriculture in Brazil cleared between 4,571 and 7,989 km2 of Amazonian rainforest per year between 2012 and 2017 (Figure 1 in Rochedo et al., 2018), reef-building coral cover in the Caribbean has declined by 50% since the 1970s (Jackson et al., 2014), and industrial fishing fleets have depleted stocks of once plentiful fishes (Myers and Worm, 2003). Human population density is three times higher in coastal than inland areas (Small and Nicholls, 2003), which focuses and exacerbates the effects of human habitation on estuarine and near-shore environments (Kennish, 2002).
Worldwide, anthropogenic stressors have degraded marine ecosystems, with nearly half of which affected by multiple stressors (Halpern et al., 2008). Coastal ecosystems are particularly vulnerable (Lotze and Milewski, 2004), as are the vital ecological processes operating within them (Worm et al., 2006), such as productivity (Short and Wyllie-Echeverria, 1996) and the provisioning of shelter (Herrnkind et al., 1997). But few studies have examined the impact of habitat deterioration on the functioning of more enigmatic ecological phenomena - such as underwater soundscapes (Butler et al., 2016).
The pelagic larvae of many fishes, decapods (e.g., crabs and lobsters), bivalves (e.g., mussels and oysters), and corals use underwater sounds to locate nursery habitats (Tolimieri et al., 2000; Kingsford et al., 2002; Leis et al., 2002; Jeffs et al., 2005; Vermeij et al., 2010; Lillis et al., 2013). Unlike chemical cues carried by currents or visual cues that depend on the availability of light, underwater sound can carry biologically relevant information over long distances irrespective of water movement, turbidity, depth, or time of day (e.g., Myrberg, 1978).
Early studies of natural underwater sound in New Zealand and Australia determined that biogenic and geophysical noise generated along rocky coastal reefs could be detected 10-25 km offshore, providing a possible navigation cue for larvae searching for settlement habitat (Tait, 1962; Cato, 1978). More recent studies that broadcast reef noise at non-reef sites have revealed that some larval fish and decapods are attracted to underwater sounds produced at nursery habitats (Tolimieri et al., 2000, 2004). In fact, some reef fish and invertebrate larvae can discern habitat-associated differences in soundscapes and use specific components of reef noise to locate appropriate settlement sites, facilitating their recruitment. For example, Stanley et al. (2010, 2011, 2012) found that distinct acoustic spectra produced in natural rocky reefs altered the swimming behavior, induced settlement, and reduced the time to metamorphosis of larvae of five brachyuran crab species. Simpson et al. (2008) measured the response of a variety of settlement stage reef fish to the filtered components of reef noise (either 570 – 2,000 Hz or below 570 Hz) and found that the larvae preferred the 570 – 2,000 Hz frequency component of reef noise.
Because sound is used by many marine larvae as a navigational cue to locate nursery areas, habitat degradation has the potential to interrupt larval recruitment if it disrupts the acoustic properties of the habitat. Previously, we have described (Butler et al., 2016) how harmful algal blooms degraded hardbottom habitats in the Florida Keys (Florida, United States), which diminished soundscapes emanating from those habitats by killing large sponges that offer refuge to a variety of soniferous (i.e., sound-producing) animals. Healthy hardbottom habitat exhibits significantly higher spectral levels over the entire frequency bandwidth (0-24 kHz) as compared to degraded hardbottom; this was particularly evident during dusk on nights of the full moon, when healthy hardbottom spectra were up to 20 dB (re: 1 μPa/Hz) greater than degraded hardbottom spectra. Furthermore, that research also demonstrated that the reestablishment of sponges in those habitats restores the natural soundscape (see Figure 2 in Butler et al., 2016).
Therefore, we hypothesized that because habitat restoration can reestablish natural soundscapes, perhaps it also ameliorates the effect of soundscape deterioration on larval recruitment. To test this, we first conducted an experiment to determine whether playback of healthy hardbottom soundscapes within degraded areas prompted higher larval settlement. Then we quantified the settlement of larval and post-larval fish and invertebrates within areas affected and unaffected by habitat degradation (i.e., mass mortality of sponges), as well as areas undergoing habitat restoration via transplantation of sponges.
Materials and Methods
Study Area
Our studies were carried out in Florida Bay and the nearshore (< 3 m deep) waters north of the middle Florida Keys (United States) where there is a mosaic of underwater habitats such as seagrass meadows, sand-mud bottom, mangrove-fringed islands, and sponge-dominated hardbottom. Over the past few decades this region has experienced substantial ecological change, including massive blooms of cyanobacteria that caused sponge die-offs over ∼500 km2 of the central and southern portions of Florida Bay (Butler et al., 1995). Sponges dominate the animal biomass of hardbottom, filter the water column above these communities, and provide habitat and shelter for motile organisms (Butler et al., 1995). Thus, the near-complete loss of these crucial inhabitants of hardbottom has changed the ecological character of the environment (Valentine and Butler, 2019). To combat the loss of ecological functions, we have tested the feasibility of restoring sponge communities by transplanting portions of sponges taken from areas unaffected by the cyanobacteria blooms into areas degraded by the sponge die-offs (Butler et al., 2021). The present study takes advantage of those restoration efforts by utilizing some of the sites established in that project.
Does Settlement of Larvae Differ on Healthy, Degraded, and Restored Sites?
To test the effect of habitat degradation and subsequent habitat restoration on the settlement of larval fishes and invertebrates, nine sites were established in three different hardbottom areas: (1) three sites located in undisturbed, “healthy” hardbottom areas outside the region impacted by cyanobacteria blooms to serve as positive controls, (2) three sites within degraded hardbottom areas, and (3) three restored sites where sponges had been transplanted (in each 25 m × 25 m site: 9 species of sponge; 385 sponges) as part of our restoration study. These sites were selected from our previous work in which we characterized hardbottom soundscapes of Florida Bay and the Florida Keys and discovered that healthy hardbottom, degraded hardbottom, and restored hardbottom exhibited different acoustic characteristics (Butler et al., 2016).
At each site, three larval “shag” collectors were deployed in May 2013 and remained in the water until September 2013. The collectors were made of frayed rope woven into a mesh backing 50 cm wide by 100 cm long so as to mimic the physical structure of the bushy, macroalgae (mostly Laurencia spp.) that covers the substrate in hardbottom habitat and into which many types of fish and macroinvertebrate larvae settle. The collectors were secured by concrete blocks and suspended in the water column by buoys (Figure 1 inset). These collectors have been successfully used in previous larval settlement studies in the region (Herrnkind and Butler, 1994). We collected, preserved, and enumerated larvae from the collectors three days after new moon (n = 3) and full moon (n = 3) during that five-month period; thus, we had a total of 162 sampling units (9 sites x 3 collectors/sites x 6 moon phases). For sampling, the collectors were unclipped from their moorings and carefully slipped into a mesh bag (1 mm2 mesh) before being hauled aboard a vessel where they were shaken ten times to dislodge larvae into the mesh bag. Following IACUC approved protocols, larvae were immediately preserved in 70% ethanol and later identified to the lowest taxonomic level possible (at least to genus).
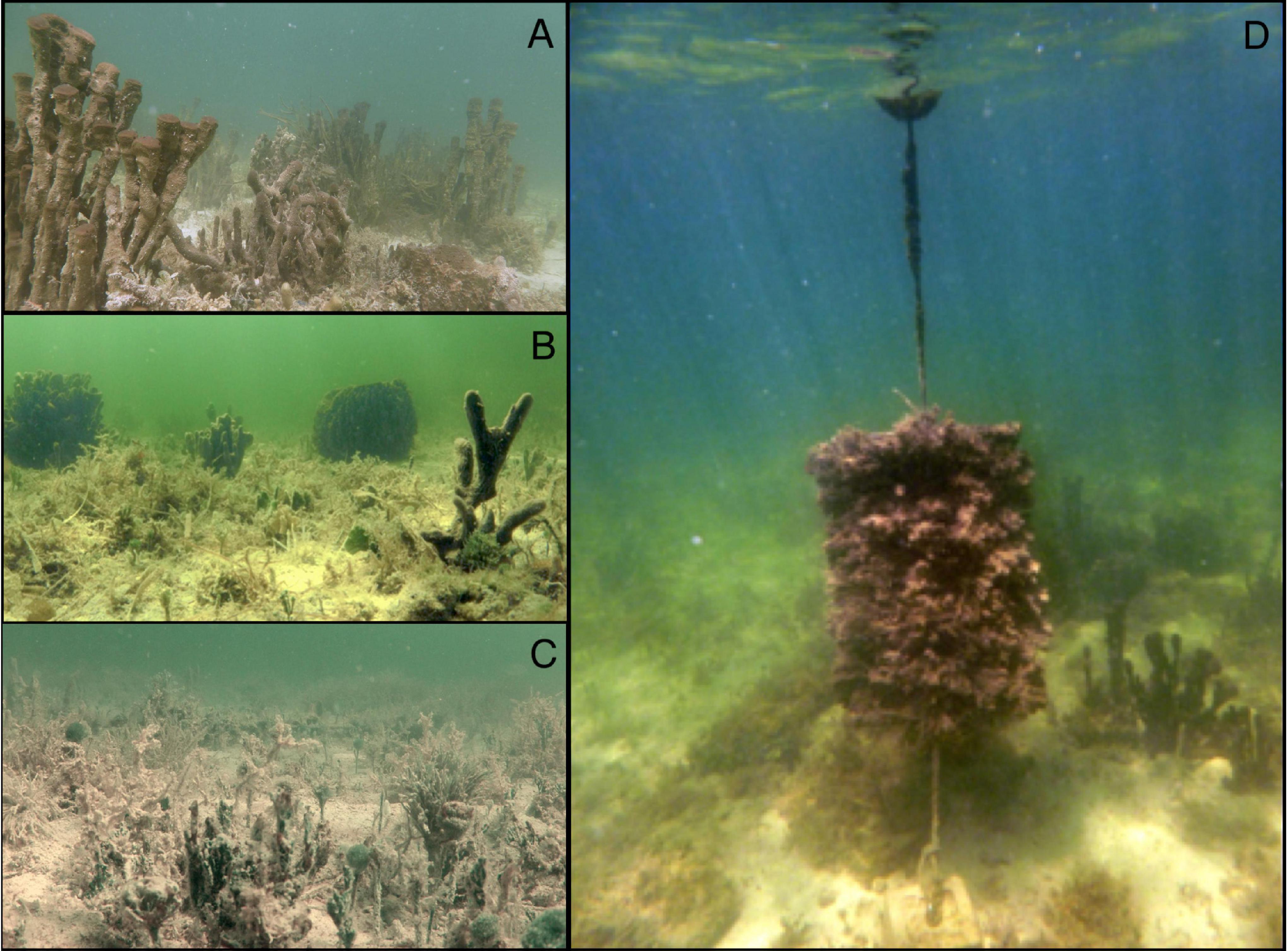
Figure 1. Example of healthy hardbottom habitat with many large sponges present, restored hardbottom habitat with sponge transplants attached to concrete pavers, and degraded hardbottom devoid of sponges (A–C, respectively). Panel (D) is a photo of a larval shag collector at an undisturbed hardbottom site; two of these were moored at each experimental site within each location.
Univariate ANOVAs were used to analyze the total number of larval recruits and number of species found within a site, where moon phase (new or full), habitat health (healthy, degraded, or restored), and their interaction were fixed factors. In addition, larval community assemblage data were Hellinger-transformed (Legendre and Gallagher, 2001) and analyzed using a PERMANOVA; moon phase, habitat health, and their interaction were fixed factors, and the random factor of site was nested within habitat health. The analysis was run using the vegan package (Oksanen et al., 2020) in R (R Core Team, 2020), and any significant differences attributed to fixed factors were analyzed using pairwise contrasts for levels of significant factors from the PERMANOVA, and p-values were Bonferroni-adjusted based on the number of contrasts.
Data were visually represented using 2-dimensional non-metric multidimensional scaling (2D-nMDS). The MDS algorithm relies on the rank order of samples, not the underlying dissimilarities, to create a map of relative distances between samples in a set number of dimensions that preserves the ranks among the samples. However, unlike metric ordination techniques such as principal components analysis that preserve the distance between samples, the axes of the nMDS plot represent only a coordinate system in ordination space onto which the samples are plotted, and not the components that drive sample differences (Pos et al., 2014).
Does Healthy Hardbottom Sound Prompt Higher Larval Settlement?
The “blank canvas” of barren hardbottom created by the sponge die-offs afforded us the opportunity to empirically test in situ whether settlement-stage larvae of fishes and invertebrates respond to the playback of hardbottom soundscapes. These hardbottom barrens were devoid of sponges and their associated soniferous fauna, hence the underwater sounds that normally emanate from healthy, sponge-dominated hardbottom (e.g., the din of snapping shrimp or the “hoots” of toadfish; Butler et al., 2016) were also absent. A sound playback device, consisting of a Lubell Labs 916C underwater loudspeaker (frequency response 200 Hz – 20 kHz, 180 dB re 1 μPa output @ 1 kHz) connected to a waterproof barrel that housed a solid-state WAV player, an amplifier to drive the speaker, and an AGM battery to power the speaker/amplifier combination, was used to broadcast healthy hardbottom soundscapes within degraded hardbottom areas. The healthy hardbottom sounds were recorded on hardbottom areas in the Florida Keys lying outside the region subject to sponge die-offs. Eight recordings (n = 4 during new moon, and n = 4 during full moon) from separate healthy hardbottom areas were used for playback, one for each trial and matched to the appropriate moon phase, to avoid pseudoreplication of the soundscape cue. These healthy hardbottom recordings were made using a calibrated hydrophone system described in Butler et al. (2016), wherein a calibrated Aquarian Audio H2a omnidirectional hydrophone (Aquarian Audio Products: sensitivity −180 dB re: 1 V/μPa; flat frequency response 10 Hz – 100 kHz) was connected to a Roland R-05 solid-state WAV recorder (Roland Corporation; 48 kHz, 16 bit) housed within a waterproof container. From each acoustic recording of healthy hardbottom, we extracted a two-hour sound clip beginning half an hour before sunset to one and a half hours after sunset to encompass the evening snapping shrimp chorus. Each two-hour sound clip was then broadcasted on a loop throughout each night of the experiment.
Our access to expensive underwater speakers was limited, so we tested whether we needed a control treatment that employed a speaker system to broadcast degraded sounds, or whether we could use a silent structural mimic. We conducted a preliminary experiment comparing the recruitment of fish and invertebrate larvae to both a silent structural mimic of the speaker system versus a speaker system broadcasting degraded soundscapes at sites established in a degraded hardbottom area. For that test of controls, three larval collectors were deployed at two sites in degraded hardbottom habitat for five nights: two nights before to two nights after three new (Aug, Oct, Nov) and two full moon phases (Oct, Nov). An underwater speaker broadcasting degraded hardbottom soundscapes was deployed each evening of the trial at one site and a structural mimic of the speaker system was deployed at the second site as a silent control. Collectors were retrieved the morning following the last night of the trial and larvae were preserved for enumeration and identification as above. Two separate 2-factor fixed effect ANOVAs were used to test the effects of treatment and moon phase on the number of larvae and number of larval taxa recruiting onto each site, and a holistic larval community analysis via PERMANOVA (as described above) was also performed to test for differences between these two types of controls.
We found no differences between these two control types (see Results), thus we used silent controls with a structural mimic for the formal test of larval recruitment in response to healthy hardbottom soundscapes. Four hardbottom locations separated by at least 5 km within the degraded sponge die-off area were selected, and at each location two sites (one designated as a sound playback site, and one designated as a silent control site) were established 1 km apart to allow paired comparisons of larval settlement (Figure 1). Anderson et al. (2021) conducted an acoustic propagation experiment within hardbottom habitats of the Florida Keys and found that the signal-to-noise ratio for broadcasted sounds from our speaker system was nearly 0 dB at 1 km. Each location was used as an experimental replicate twice: once during a full moon trial (n = 4) and once during a new moon trial (n = 4). At the sound playback treatment site, the sound playback device was deployed on the evening of each night of the trial and recovered the next morning; at the silent control site a structural mimic of the sound playback device was deployed. On both the sound playback site and silent control site, two larval “shag” collectors were deployed on the first day of the trial and were retrieved the final day of the trial; thus, we had a total of 64 sampling units (4 locations × 2 collectors/site × 8 moon phases).
On each site, the collectors were placed in opposing directions ∼ 10 m from either the playback device or the structural mimic. We ran a total of eight paired sound playback/silent control trials, wherein a single experimental trial lasted five nights: two nights prior to a moon phase of interest, either new moon or full moon, the night of the moon phase, and two nights post moon phase. After each five-day trial, the playback device and its mimic were retrieved as were the shag collectors.
Again, larval community assemblage data were Hellinger transformed (Legendre and Gallagher, 2001) and were analyzed via a two-way PERMANOVA (factor 1 = moon phase: new or full; factor 2 = treatment: sound playback or no sound control; n = 4 within each combination of factors). Data were also visually represented using 2-dimensional non-metric multidimensional scaling (2D-nMDS).
Results
Does Settlement of Larvae Differ on Healthy, Degraded, and Restored Sites?
We collected 10,391 recruits of 43 different species off the larval collectors deployed on the healthy, degraded, and restored hardbottom sites; crustaceans (40% of catch; 4,213 individuals of seven species) and mollusks (44% of catch; 4,547 individuals of 16 species) dominated the catch. Overall, more larval and post-larval fish and invertebrates recruited into healthy hardbottom habitat (4,079 total; 805 ± 165.0 [median ± m.a.d.]) than into degraded or restored hardbottom (3,278 and 3,034 total; 416 ± 157.0 and 366 ± 243.0, respectively) (Figure 2A); however, these differences were non-significant. Median (± m.a.d.) total numbers of recruits during the new moon (healthy: 849 ± 200.0; restored: 527 ± 255.0; degraded: 871 ± 590.0) were often greater than during the full moon (restored: 315 ± 239.0; degraded: 302 ± 17.8), except at healthy sites (776 ± 86.0) (Figure 2A). The two-way ANOVA indicated that moon phase significantly affected the number of recruits (F1,12 = 6.09, p = 0.029), whereas neither habitat health nor the interaction between moon phase and habitat health were significant (Supplementary Table 1).
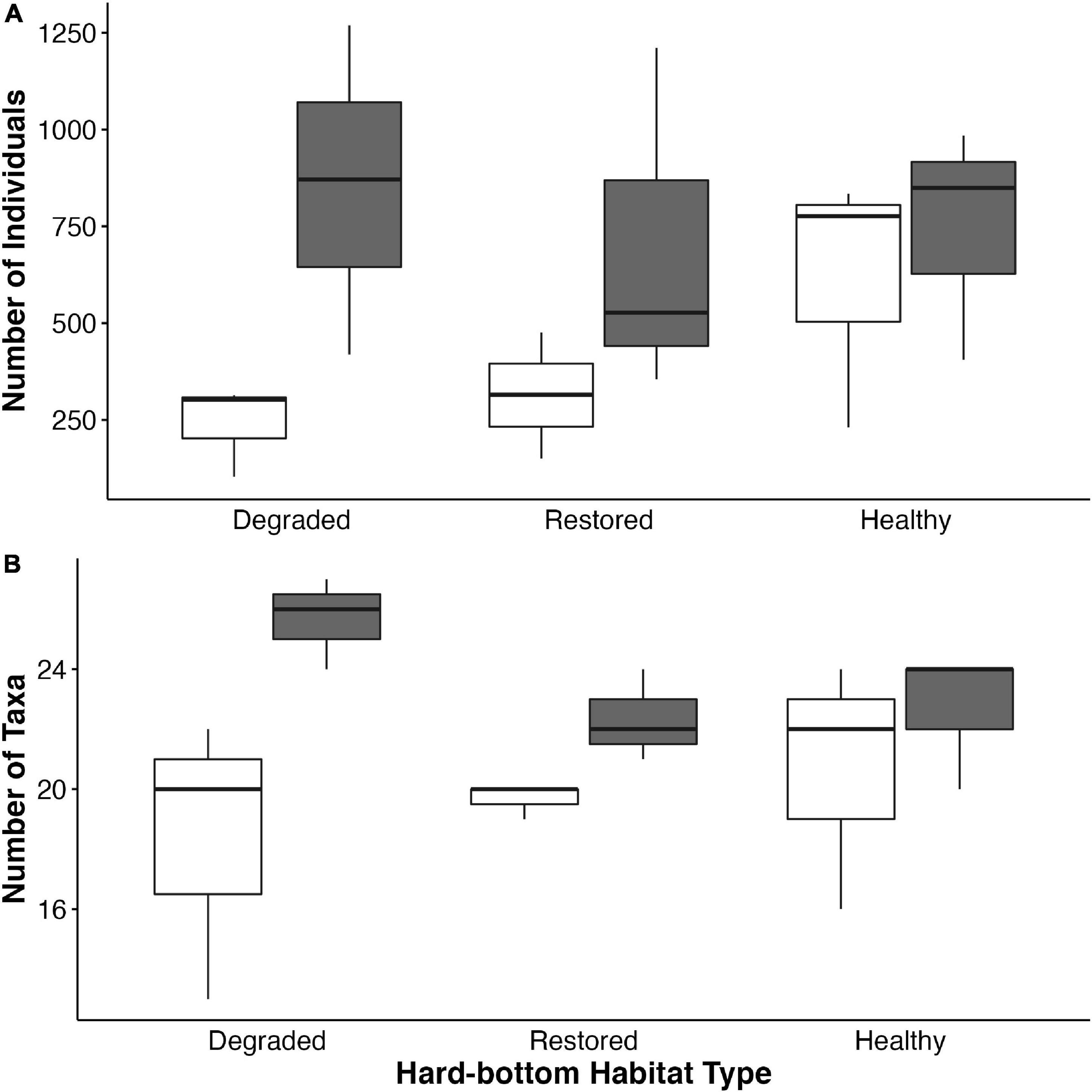
Figure 2. Boxplots of larval collections within the three hardbottom types. Number of larval fish and invertebrates (A) and number of larval fish and invertebrate taxa (B) that settled on larval shag collectors during full moon (white) or new moon (gray) deployed at three types of hardbottom sites: degraded, restored, and healthy.
In total, we collected 43 species of fishes and invertebrates. Thirty-four taxa recruited into healthy and degraded hardbottom (four species unique only to healthy hardbottom: Astrea americana, Hippocampus spp., Littorina spp., and Eoacmaea pustulata; two species unique only to degraded hardbottom: Balistes capriscus and Percnon gibbesi), and 32 taxa recruited into restored hardbottom (two unique species: Lachnolaimus maximus and Macrocoeloma trispinosum). The overall median number of taxa recruiting onto degraded, restored, and healthy hardbottom sites was 23 ± 4.4 (median ± m.a.d.), 20.5 ± 1.5, and 23 ± 1.5, respectively (Figure 2B). The median (± m.a.d.) number of taxa recruiting to all three hardbottom habitat types was greater during the new moon (degraded: 26 ± 1.5; restored: 22 ± 1.5; healthy: 24 ± 0.0, respectively) than during the full moon (20 ± 3.0, 20 ± 0.0, and 22 ± 3.0) (Figure 2B). Similarly to the ANOVA above, moon phase significantly affected the number of species recruiting to sites (F1,12 = 8.64, p = 0.012), whereas neither habitat health nor the interaction between habitat health and moon phase were significant (Supplementary Table 1).
The results of the PERMANOVA (Table 1) indicated that both habitat quality and moon phase significantly affected the assemblage of larvae that recruited to hardbottom sites. The a posteriori pair-wise PERMANOVA indicated that larval assemblages that recruited to healthy areas differed from those assemblages that recruited to degraded or restored habitat (F = 2.955; df = 2, 70; p = 0.030). In addition, larvae were more abundant during new moon than full moon (F = 9.199; df = 1, 70; p = 0.001). There were also significant differences in larval settlement among sites within a treatment, as indicated by the significance of that effect in the PERMANOVA (F = 2.3074; df = 6, 70; p = 0.036).
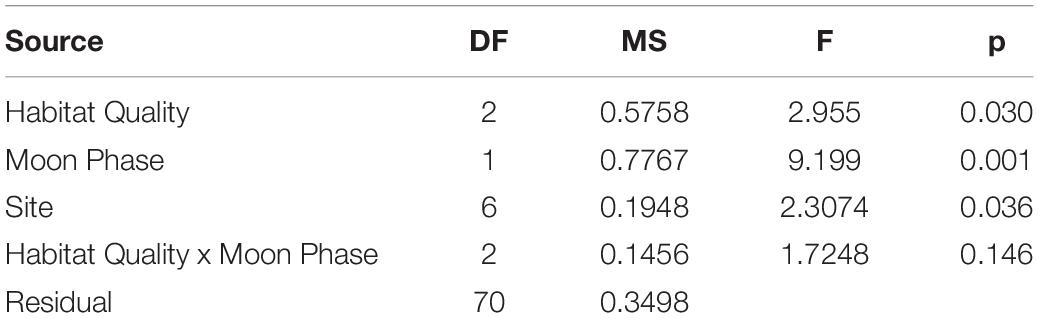
Table 1. PERMANOVA results testing the effects of habitat quality, moon phase, and site on larval community assemblage.
Examination of the larval assemblages shows that the largest recruitment differences occurred in thirteen species: seven mollusks, three crustaceans, two fishes, and one echinoderm (Supplementary Figure 1). The 2D-nMDS plot (Figure 3) illustrates how habitat quality and moon phase affected larval recruitment (stress; 0.096, r2 = 0.936). Restored hardbottom (blue) and degraded hardbottom (red) were more similar to each other than to healthy hardbottom (green). In addition, new moon collections (dark color shades) and full moon collections (light color shades) grouped separately for each habitat type. The top five species that influenced the ordination were a Sparid parrotfish, the Caribbean spiny lobster Panulirus argus, a Haemulid grunt, a bivalve clam Lima spp., and a pipefish Syngnathus spp.; the 13 most common species collected are presented in Supplementary Figure 1.
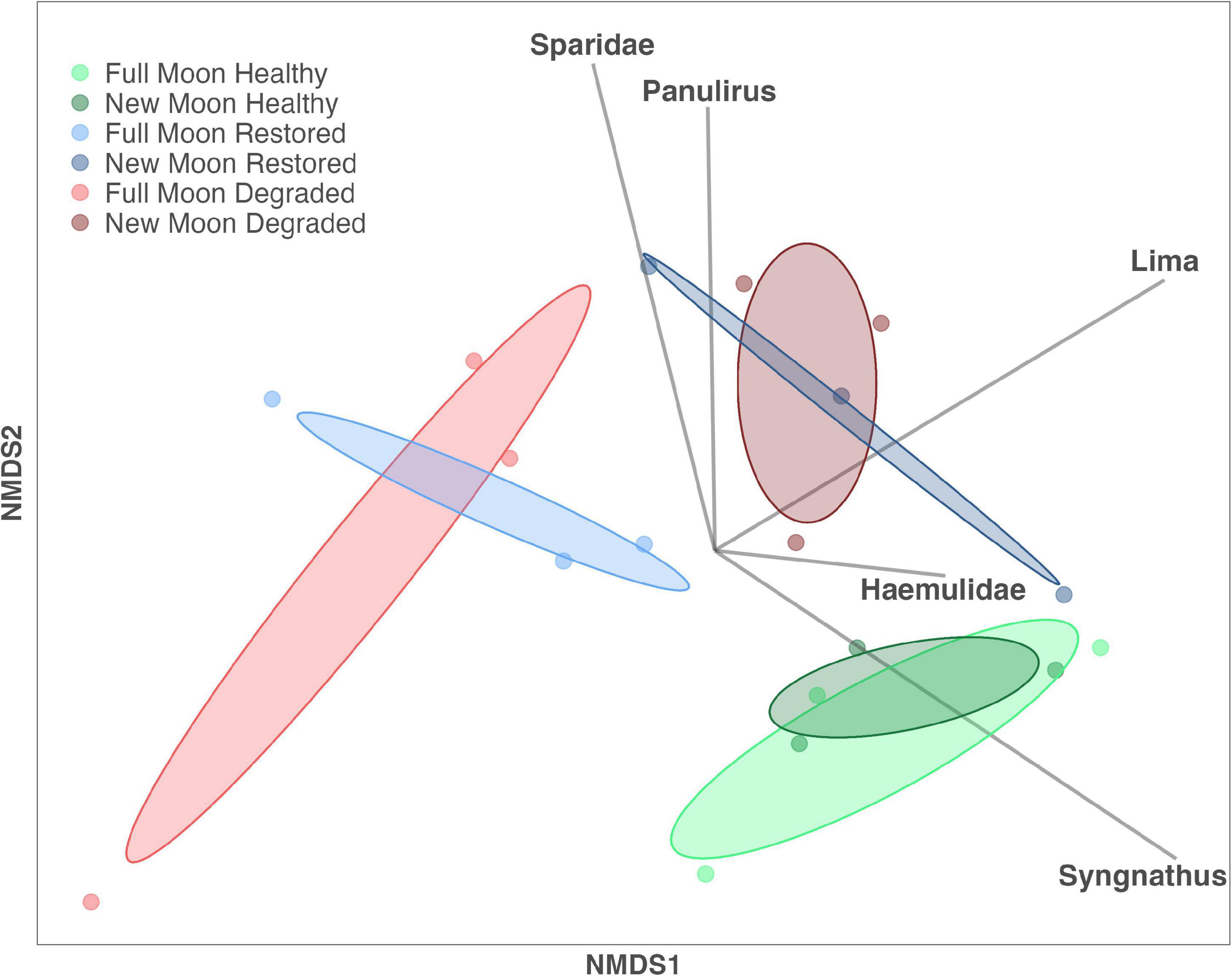
Figure 3. Two-dimensional non-metric multidimensional scaling plot showing groupings of healthy, restored, and degraded hardbottom sites. Healthy hardbottom is displayed in green, restored hardbottom is displayed in blue, and degraded hardbottom is displayed in red. Darker color shades indicate new moon and lighter color shades indicate full moon. Overlapping ellipsoids indicate similarity between groups, and species vectors indicate the top five species that influenced the ordination.
Does Healthy Hardbottom Sound Prompt Higher Larval Settlement?
In comparing two types of potential controls (i.e., silent control vs. control in which degraded hardbottom sound was played back) in our preliminary experiment, we found no difference in the number of larvae (F1,6 = 0.012, p = 0.917) or number of larval taxa (F1,6 = 0.137, p = 0.724) recruiting into degraded hardbottom sites with no sound addition (i.e., silent sites) versus those in which we played back the sound of degraded hardbottom sites (F1,6 = 0.386, p = 0.557; F1,6 = 0.863, p = 0.389; respectively for number of individuals versus larval species), regardless of moon phase. We also found no community composition differences between these two potential control types via PERMANOVA (F1,25 = 0.50, p = 0.921). We therefore used silent controls for the formal test of larval recruitment in response to healthy hardbottom sounds.
A total of 2,625 larvae of 19 species recruited onto the larval collectors deployed on the experimental sites over the eight trials. Seven species of mollusks accounted for roughly 76% (2,005 individuals) of the collections, most (73%) of those being a single bivalve clam (Lima sp.; 1,929 individuals). Overall, similar numbers of species recruited to both silent and sound playback treatments: 16 species recruited onto collectors at silent control sites (6 ± 1.5; median ± m.a.d.) and 12 species recruited onto healthy sound playback sites (5 ± 1.5) (Figures 4C,D). Median (± m.a.d.) larval abundances were also statistically similar given the high variance in the data, however settlement was 9 – 24% greater at sound playback sites (82.5 ± 43.7) than at silent control sites (44.5 ± 28.9) (Figures 4A,B and Supplementary Table 2). Though not statistically significant, during full moon greater numbers of larvae (58 ± 51.9; median ± m.a.d.) recruited onto sound playback sites than silent control sites (42.5 ± 25.2). Just the opposite occurred during new moon (silent control: 126 ± 136; sound playback: 88 ± 14.8), although the variance at the silent control sites was much higher than at the sound playback sites.
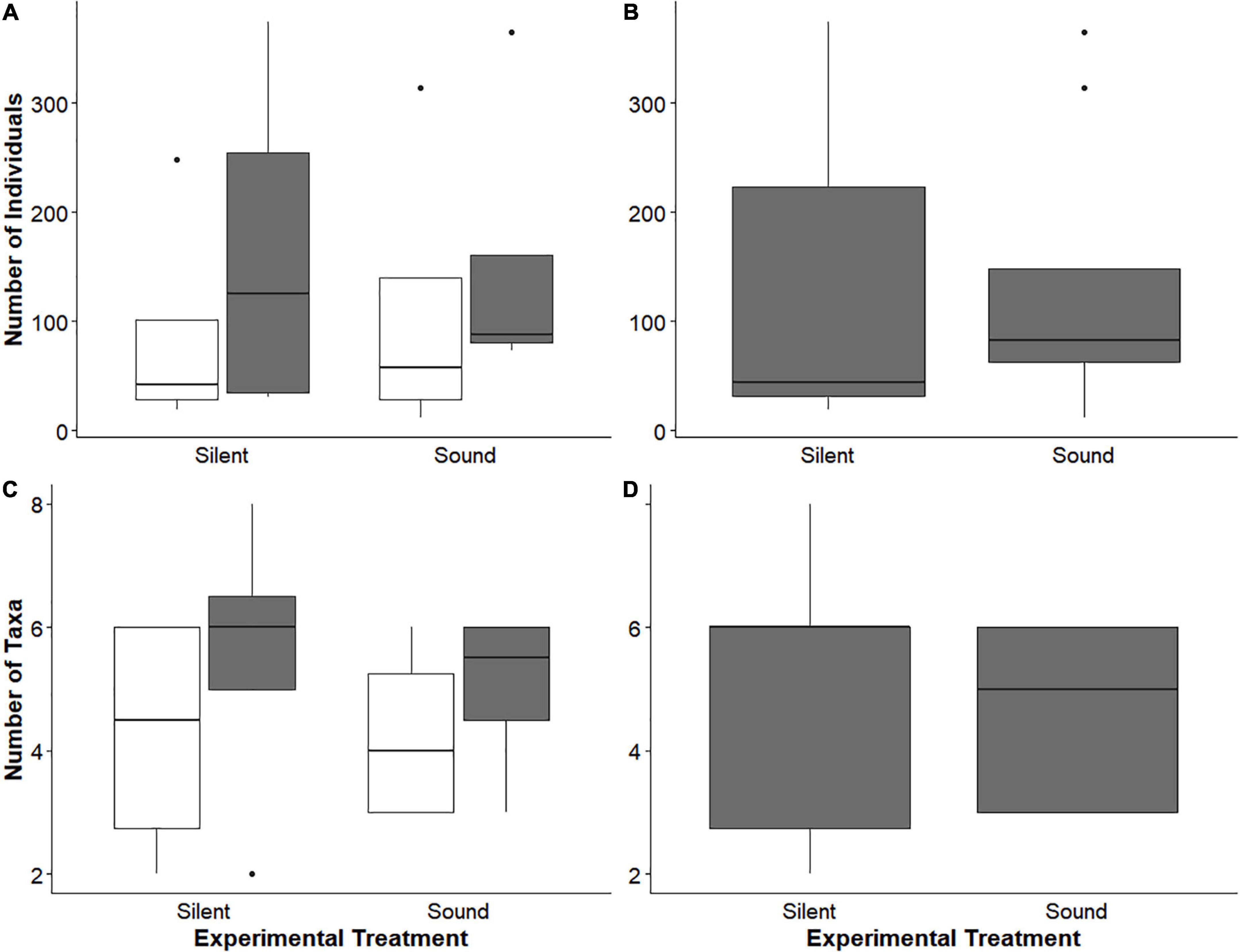
Figure 4. Boxplots of larval collections during experimental sound playback. Number of larval fish and invertebrates (A) and number of larval fish and invertebrate taxa (C) that settled on larval shag collectors during full moon (light colors) or new moon (dark colors) at degraded hardbottom sites when the soundscape of healthy hardbottom was played (blue) versus a nearby site where no sound was played (silent control; red). Overall number of larval fish and invertebrates and number of taxa that settled into sound payback treatments and silent controls are shown in panels (B,D), respectively.
The results of the two-way PERMANOVA indicate that neither sound playback (F1,12 = 0.514, p = 0.81, 1,000 permutations) nor moon phase (F1,12 = 0.838, p = 0.54, 1,000 permutations) significantly affected the larval assemblages that recruited onto shag collectors at the degraded hardbottom sites. Figure 5A shows the grouping of treatment combinations in 2D-nMDS plots (stress: 0.037, r2 = 0.997), and Figure 5B shows the species locations within the ordination space with the environmental vectors (moon phase and sound treatment) overlaid to show how each species responds.
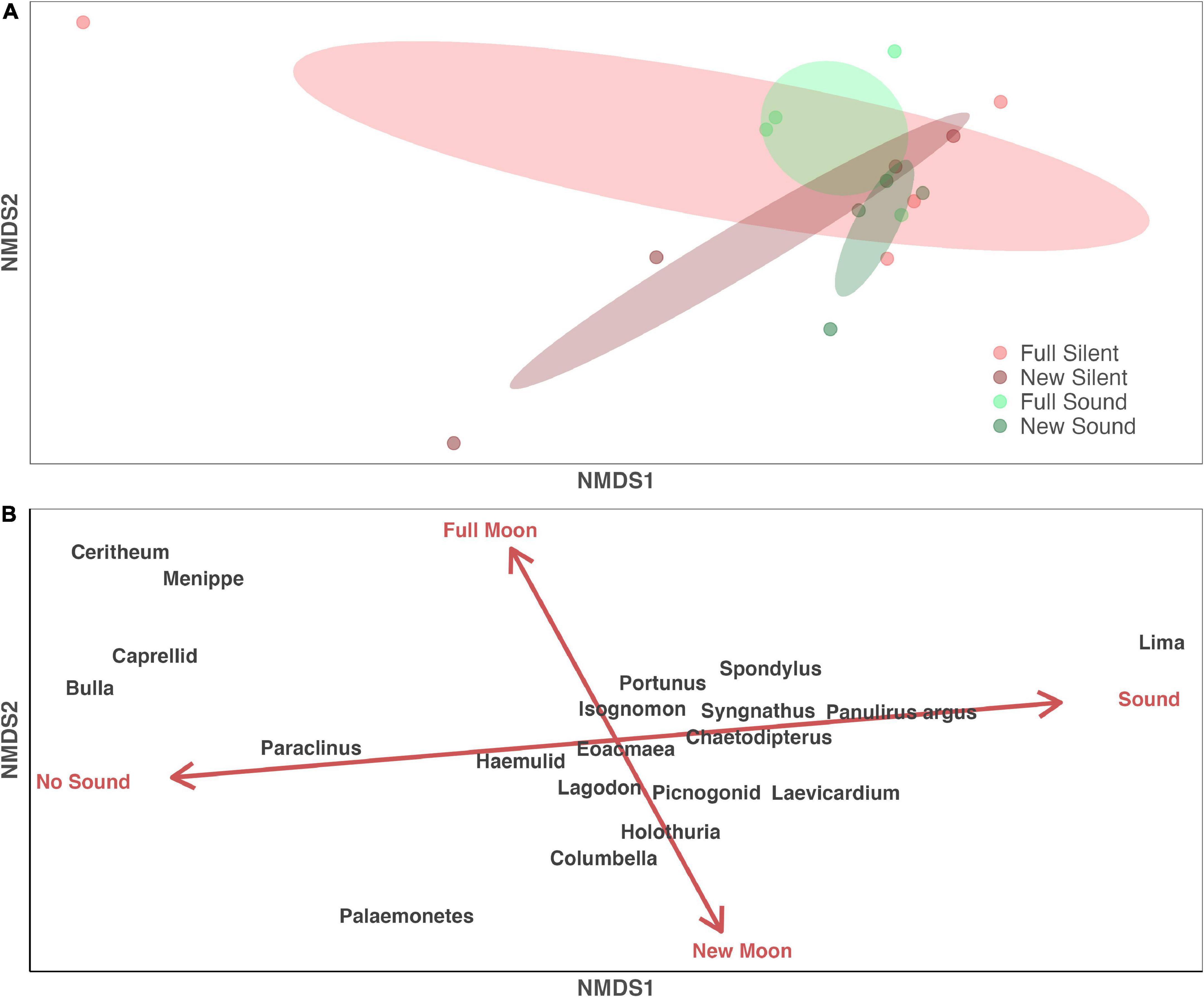
Figure 5. Two-dimensional non-metric multidimensional scaling plot showing the relationships among the lunar and sound-playback treatment groups. Sound playback groups are shown in green, whereas no sound playback control groups are shown in red; darker color shades indicate new moon, and lighter color shades indicate full moon. Top panel (A) shows site groupings, and bottom panel (B) shows species locations in the ordination space with treatment vectors (in red).
Discussion
We examined if habitat degradation and its restoration influenced larval recruitment into tropical hardbottom habitat and whether differences in underwater sound play a role in that process. The loss of large, structure-forming sponges in central Florida Bay due to harmful algal blooms (HABs) provided a “natural experiment” that permitted us to compare the assemblages of fish and invertebrate larvae that settled within degraded hardbottom areas, healthy hardbottom areas unaffected by sponge die-offs, and hardbottom sites undergoing sponge community restoration. Though there were no statistically significant differences in total larval abundance or number of taxa that settled on artificial collectors at the three types of study sites, different larval assemblages recruited into healthy hardbottom habitat than those recruited to degraded or restored habitat. This was particularly evident during full moon when collections of larvae in healthy hardbottom habitat were double those of degraded or restored habitats. Broadcasting the sound of healthy hardbottom in the midst of large, degraded areas did not alter overall larval communities; however, the recruitment of some types of larvae, particularly during the full moon, did respond to sound playback. This confirms the use of sound as a navigational cue by the larvae of some, but not all taxa that recruit into tropical hardbottom habitats.
Habitat Degradation and Larval Settlement: A Role for Sound?
Mounting evidence suggests that habitat degradation alters the properties of marine soundscapes, just as it does natural sounds in terrestrial habitats (Brumm, 2010; Shannon et al., 2015; Sordello et al., 2019; Williams et al., 2021). Piercy et al. (2014) compared coral-reef soundscapes across a quality gradient and found that higher quality coral reefs are louder, suggesting that sounds may propagate farther from healthier reefs. Butler et al. (2016) found that degraded backreef hardbottom soundscapes were significantly quieter than healthy hardbottom soundscapes, but that restoring hardbottom habitat with transplanted sponges returned natural soundscape spectra to levels indistinguishable from healthy hardbottom. The degradation of habitat soundscapes in turn has ramifications for the larvae that use them to locate settlement habitat. For example, 8% more fish were attracted to playbacks of pre-degradation coral reef soundscapes as opposed to quieter post-degradation soundscapes after severe damage to Australia’s Great Barrier Reef (Gordon et al., 2018). Similarly, more coral larvae settle on Caribbean reefs in response to high-quality reef soundscapes than to soundscapes of low-quality reefs (Lillis et al., 2016).
Our results indicate that larval recruitment also differs between healthy and degraded hardbottom habitats. For instance, larvae from two families of fishes (Syngnathidae and Bleniidae) showed distinct differences in recruitment among habitats in this study and are known to respond to sound (Amorim, 1996), some of which may be cues produced by conspecifics (Colson et al., 1998). Recent laboratory work by Lillis et al. (2013) suggests that larvae of the oyster Crassostrea virginica settle in response to habitat-associated sound cues, and our study indicates that the bivalve clam Lima spp. may do the same. Testing the response of individual species to underwater sound cues has been limited but would be useful in confirming the taxon-specific results of experimental field studies such as ours. Moreover, information on the navigational cues that drive settlement behavior of marine larvae is crucial to understanding the patterns of recruitment of coastal species, many of commercial importance, and the potential disruption of their recruitment to coastal nurseries through direct (e.g., underwater anthropogenic noise) or indirect influences (e.g., habitat degradation).
One aspect of our results is perplexing. Our previous research (Butler et al., 2016) demonstrated that soundscapes emanating from restored sites were virtually identical to those soundscapes emanating from healthy sites; however, the present study found that natural recruitment differed between healthy hardbottom sites versus restored hardbottom and degraded hardbottom sites. Though not statistically different, we also found a trend that more larvae were attracted to collectors placed within degraded hardbottom areas near a speaker broadcasting sounds of healthy hardbottom versus collectors placed within degraded hardbottom areas where sounds were not broadcast (510 larvae collected at sound playback sites versus 386 larvae collected at silent control sites). Thus, we would have expected recruitment of similar larval communities into healthy and restored hardbottom areas.
Several factors can explain why the larval assemblages collected on restored hardbottom sites were more similar to communities collected within degraded hardbottom areas versus healthy areas. The hardbottom restoration sites that we used are small (25 m × 25 m) and have only been in existence <5 years, so the sponge communities are not yet fully recovered, and we do not know how far sounds from these restored areas travel through the water. Perhaps the sound produced by small, restored sponge habitat “islands” does not propagate far enough to attract settling larvae. In contrast, our previous work in the area (Anderson et al., 2021) found that tones broadcast from our playback systems at 115 dB re: 1 μPa were detectable nearly half a kilometer away, thus perhaps the healthy hardbottom soundscapes broadcast during this experiment were transmitted (in both the pressure and particle motion domains) much farther than the sounds emanating from the small, restored hardbottom sites. If so, the experimental broadcasting of sounds probably attracted larvae from further away than did the sponges transplanted onto restoration sites, thus explaining the observed differences in larval recruitment between restoration sites and experimental sound playback sites.
Habitat-associated soundscapes likely work in concert with other cues used by larvae to find suitable settlement sites. Perhaps incoming larvae searching for settlement sites use underwater sounds to orient toward the appropriate habitat at distances relevant to underwater sound propagation [e.g., hundreds of meters to several kilometers (Jeffs et al., 2003; Radford et al., 2010)], yet once these larvae home in on the appropriate habitat, they might use visual or chemical cues to find their exact settlement sites. In this respect, our small hardbottom restoration sites might not produce the visual, chemical, or other cues necessary to induce settlement of incoming larvae, which could explain why larval assemblages on restored hardbottom sites were more similar to assemblages found on degraded sites.
Habitat-Associated Sounds and Larval Attraction
Underwater sound is unique among the host of cues that the larvae of marine organisms use during settlement (see Kingsford et al. (2002) for review) because it propagates up to tens of kilometers, depending upon acoustic frequency, water depth, and physical barriers and does so regardless of current. Thus, underwater sound can carry biologically relevant information to distant larvae (Jeffs et al., 2003; Radford et al., 2010). Still, a larva must possess the sensory abilities to detect and respond to different information within the soundscape. Studies more recent than Kingsford et al. (2002) have extended the list of species that possess the sensory abilities necessary to use underwater sound to respond to acoustic stimuli (Vermeij et al., 2010; Stanley et al., 2011, 2012; Lillis et al., 2013).
Sound appears to be an important cue to larvae recruiting into a variety of temperate coastal habitats (Colson et al., 1998; Tolimieri et al., 2004; Radford et al., 2010; Stanley et al., 2012; Lillis et al., 2013). In tropical systems, most studies of underwater sound and larval recruitment have focused on coral-reef habitats (Vermeij et al., 2010; Staaterman et al., 2013; Lillis et al., 2016; Gordon et al., 2018; Salas et al., 2018), where sound again appears to be an important navigational cue for many species, particularly fishes. However, we are aware of only one other publication on underwater sound in tropical backreef environments besides our own – the work by Wilson et al. (2013) measuring sound in seagrass meadows.
The backreef habitats in south Florida are a mosaic of inter-linked tropical habitats (i.e., seagrass, mud-sand bottom, hardbottom, mangrove) among which animals at various life stages interact and energy is transferred. Seagrass and mangroves are well known nurseries for coral-reef associated species (Herrnkind et al., 1997; Nagelkerken et al., 2000; Heck et al., 2003). But back-reef hardbottom habitats dominated by sponges are also crucial nursery habitats for many of the same reef-species, as well as other ecologically and commercially important species such as spiny lobster, stone crab, and commercial sponges (Herrnkind and Butler, 1994; Butler et al., 2017). We previously characterized the soundscapes of mangrove, seagrass, and hardbottom habitats including “healthy” sites unaffected by HABs and HAB degraded sites (Butler et al., 2016). Mangrove, healthy hardbottom, and restored hardbottom habitats had higher soundscape spectra levels than seagrass and degraded hardbottom regardless of moon phase or time of day. The soundscapes in healthy hardbottom with high sponge density and mangroves contained high levels of high frequency sounds (> 1,000Hz), large numbers of snapping shrimp snaps, and more low-frequency sounds in the ∼300 Hz frequency range that are most likely fish calls. In contrast, seagrass beds were far quieter, as were degraded hardbottom sites whose soundscape spectra were similar to that of seagrass beds. Clearly, nearshore tropical habitats have unique soundscapes, but how important is sound as a cue for larvae that seek those habitats?
Though the PERMANOVA on the larval assemblages recruiting to our sound playback versus silent control sites did not indicate an overall difference in the community, our results indicate that the response of larvae to underwater soundscape cues is idiosyncratic for each species. It appears that some taxa, especially molluscs and crustaceans, use sounds associated with hardbottom habitat, likely in conjunction with other cues (e.g., chemical or visual cues) to find settlement sites. Examination of the species locations in the ordination space compared to the treatment vectors (Figure 5) shows trends of how individual species responded to moon phase and treatment. For example, species that lie close to the ends of the Sound/No Sound vector showed a trend of attraction to healthy hardbottom soundscapes (e.g., Lima or Panulirus argus) when close to the “Sound” end of the vector, or conversely showed a trend of aversion to the healthy hardbottom soundscapes (e.g., Paraclinus or Bulla). Similarly, along the moon phase axis the gastropod molluscs Columbella exhibited higher recruitment during the new moon versus the full moon (i.e., its location is closer to the “New Moon” end of the vector). Species lying in the quadrants created by the treatment vectors exhibit influences from both moon phase and sound treatment. For example, Cerithium (a gastropod) and Menippe (a crab) lie within the quadrant created by the Full Moon x No Sound treatment and exhibit higher recruitment during the full moon at our silent control sites.
Additionally, we did not measure the particle motion component of underwater sound at our playback or silent control sites. Because larvae of marine organisms – particularly invertebrates – likely sense this component of underwater sound, we hope to measure both acoustic pressure and particle motion in playback experiments during the next phase of our research. We will also compare larval recruitment when playing back degraded soundscapes versus healthy soundscapes so we can further rule out whether an increase in particle motion produced from the underwater speaker increased recruitment at playback sites rather than larvae selecting healthy soundscapes based on their spectral composition.
It is also important to know whether playback was representative of in situ healthy habitats, especially given the sites were relatively shallow (< 3 m depth) which could lead to scattering/distortion of the broadcast sound (Urick, 1983). Unfortunately, we do not have recordings of the playback system as it broadcasted pre-recorded hardbottom soundscapes in situ. Within such a shallow water environment with complex seabed features (e.g., rugose limestone bedrock) the acoustic propagation would likely be affected by scattering and attenuation. However, some of our similar work in the area (Anderson et al., 2021) used the same acoustic playback devices and measured the attenuation of sine-wave tones (100, 500, 1000, 1500, and 2,000 Hz) at an amplitude of 115 dB re: 1 uPa, and we found little distortion.
Regardless, our study highlights that habitat degradation alters larval recruitment and that the loss of habitat-associated sounds likely plays a role in driving differences in larval settlement of some species. As the science of restoration ecology expands, setting realistic and attainable goals for restoration efforts will become increasingly important. There have been numerous calls for research that quantifies not only how restoration returns habitat structure, but also ecological function to degraded areas (French-McCay et al., 2003; Nilsson et al., 2016; Wortley et al., 2016). Such information will provide a fuller understanding of the key ecological drivers of ecosystem function and perhaps more clarity as to the most effective means to restore those functions - even enigmatic ecological characteristics like soundscapes.
Data Availability Statement
The raw data supporting the conclusions of this article will be made available by the authors, without undue reservation.
Ethics Statement
The animal study was reviewed and approved by Old Dominion University Institutional Animal Care and Use Committee.
Author Contributions
JB and MB designed the study. MB funded the research. JB and EA performed the research and analysed, with guidance from MB. JB wrote the initial version of the manuscript, with revisions made by EA and MB. All authors contributed to the article and approved the submitted version.
Conflict of Interest
The authors declare that the research was conducted in the absence of any commercial or financial relationships that could be construed as a potential conflict of interest.
Publisher’s Note
All claims expressed in this article are solely those of the authors and do not necessarily represent those of their affiliated organizations, or those of the publisher, the editors and the reviewers. Any product that may be evaluated in this article, or claim that may be made by its manufacturer, is not guaranteed or endorsed by the publisher.
Acknowledgments
We are grateful to many people who provided logistical support and advice for this project, including: J. Anderson, I. Bartol, D. Behringer, C. Butler, H. Gaff, A. Spadaro, M. Valentine, J. Vincent, and J. Wisman. We are especially indebted to J. Stanley for her guidance and comments on an earlier draft of this manuscript. Permission to conduct research in Florida Bay and the near-shore waters of the Florida Keys was granted by Everglades National Park (Permit #: EVER-2012-SCI-0034) and the Florida Keys National Marine Sanctuary (Permit #: FKNMS-2011-100). This is contribution # 1390 from the Coastlines and Oceans Division of the Institute of Environment at Florida International University.
Supplementary Material
The Supplementary Material for this article can be found online at: https://www.frontiersin.org/articles/10.3389/fevo.2022.785986/full#supplementary-material
Supplementary Figure 1 | Summary of the species with the largest differences among habitats.
References
Amorim, M. C. P. (1996). Acoustic Communication in Triglids and Other Fishes. PhD thesis, Aberdeen: University of Aberdeen.
Anderson, E. R., Butler, J., and Butler, M. J. I. V. (2021). Response of fish and invertebrate larvae to backreef sounds at varying distances: implications for habitat restoration. Front. Mar. Sci. 8:663887. doi: 10.3389/fmars.2021.663887
Boivin, N. L., Zeder, M. A., Fuller, D. Q., Crowther, A., Larson, G., Erlandson, J. M., et al. (2016). Ecological consequences of human niche construction: examining long-term anthropogenic shaping of global species distributions. Proc. Natl. Acad. Sci. U S A. 113, 6388–6396. doi: 10.1073/pnas.1525200113
Brumm, H. (2010). “Anthropogenic noise: implications for conservation,” in Encyclopedia of Animal Behavior, eds M. D. Breed and J. Moore (Oxford: Academic Press), 89–93. doi: 10.1016/B978-0-08-045337-8.00289-8
Butler, M. J. IV, Hunt, J. H., Herrnkind, W. F., Childress, M. J., Bertelsen, R., Sharp, W., et al. (1995). Cascading disturbances in Florida Bay, USA: cyanobacteria blooms, sponge mortality, and implications for juvenile spiny lobsters Panulirus argus. Mar. Ecol. Prog. Series 129, 119–125. doi: 10.3354/meps129119
Butler, J., Sharp, W. C., Hunt, J. H., and Butler, M. J. IV (2021). Setting the foundations for renewal: restoration of sponge communities to aid the ecological recovery of Florida Bay. FL (USA). Ecosphere 12:e03876. doi: 10.1002/ecs2.3876
Butler, J., Stanley, J., and Butler, M. J. IV (2016). Underwater soundscapes in near-shore tropical habitats and the effects of environmental degradation and habitat restoration. J. Exp. Mar. Biol. Ecol. 479, 89–96. doi: 10.1016/j.jembe.2016.03.006
Butler, M. J. IV, Behringer, D. C., and Valentine, M. M. (2017). Commercial sponge fishery impacts on the population dynamics of sponges in the Florida Keys, FL (USA). Fish. Res. 190, 113–121. doi: 10.1016/j.fishres.2017.02.007
Cato, D. H. (1978). Marine biological choruses observed in tropical waters near Australia. J. Acoustical Soc. Am. 64, 736–743. doi: 10.1121/1.382038
Colson, D. J., Patek, S. N., Brainerd, E. L., and Lewis, S. M. (1998). Sound production during feeding in Hippocampus seahorses (Syngnathidae). Environ. Biol. Fishes 51, 221–229. doi: 10.1023/A:1007434714122
French-McCay, D. P., Peterson, C. H., DeAlteris, J. T., and Catena, J. (2003). Restoration that targets function as opposed to structure: replacing lost bivalve production and filtration. Mar. Ecol. Prog. Series 264, 197–212. doi: 10.3354/meps264197
Gordon, T. A. C., Harding, H. R., Wong, K. E., Merchant, N. D., Meekan, M. G., McCormick, M. I., et al. (2018). Habitat degradation negatively affects auditory settlement behavior of coral reef fishes. Proc. Natl. Acad. Sci U S A. 115, 5193–5198. doi: 10.1073/pnas.1719291115
Halpern, B. S., Walbridge, S., Selkoe, K. A., Kappel, C. V., Micheli, F., D’Agrosa, C., et al. (2008). A global map of human impact on marine ecosystems. Science 319, 948–952. doi: 10.1126/science.1149345
Heck, K. L. Jr., Hays, G., and Orth, R. J. (2003). Critical evaluation of the nursery role hypothesis for seagrass meadows. Mar. Ecol. Prog. Series 253, 123–136. doi: 10.3354/meps253123
Herrnkind, W. F., and Butler, M. J. IV (1994). Settlement of spiny lobster, Panulirus argus (Latreille, 1804), in Florida: pattern without predictability? Crustaceana 67, 46–64. doi: 10.1163/156854094X00288
Herrnkind, W. F., Butler, M. J. IV, Hunt, J. H., and Childress, M. J. (1997). The role of physical refugia: implications from a mass sponge die-off in a lobster nursery. New Zealand J. Mar. Freshwater Res. 48, 759–770. doi: 10.1071/MF97193
Jackson, J. B. C., Donovan, M. K., Cramer, K. L., and Lam, V. Y. Y. (2014). Status and Trends of Caribbean Coral Reefs: 1970–2012. Global Coral Reef Monitoring Network. Gland: IUCN.
Jeffs, A. G., Montgomery, J. C., and Tindle, C. T. (2005). How do spiny lobster post-larvae find the coast? New Zealand J. Mar. Freshwater Res. 39, 605–617. doi: 10.1080/00288330.2005.9517339
Jeffs, A. G., Tolimieri, N., and Montgomery, J. C. (2003). Crabs on cue for the coast: the use of underwater sound for orientation by pelagic crab stages. Mar. Freshwater Res. 54, 841–845. doi: 10.1071/mf03007
Kennish, M. J. (2002). Environmental threats and environmental future of estuaries. Environ. Conserv. 29, 78–107. doi: 10.1017/S0376892902000061
Kingsford, M. J., Leis, J. M., Shanks, A., Lindeman, K. C., Morgan, S. G., and Pineda, J. (2002). Sensory environments, larval abilities, and local self-recruitment. Bull. Mar. Sci. 70, 309–340.
Legendre, P., and Gallagher, E. D. (2001). Ecologically meaningful transformations for ordination of species data. Oecologia 129, 271–280. doi: 10.1007/s004420100716
Leis, J. M., Carson-Ewart, B. M., and Webley, J. (2002). Settlement behaviour of coral-reef fish larvae at subsurface artificial-reef moorings. Mar. Freshwater Res. 53, 319–327. doi: 10.1071/mf01082
Lillis, A., Bohnenstiehl, D. R., Peters, J. W., and Eggleston, D. (2016). Variation in habitat soundscape characteristics influences settlement of a reef-building coral. PeerJ 4:e2557. doi: 10.7717/peerj.2557
Lillis, A., Eggleston, D. B., and Bohnenstiehl, D. R. (2013). Oyster larvae settle in response to habitat-associated underwater sounds. PLoS One 8:e79337. doi: 10.1371/journal.pone.0079337
Lotze, H. K., and Milewski, I. (2004). Two centuries of multiple human impacts and successive changes in a North Atlantic food web. Ecol. Appl. 14, 1428–1447. doi: 10.1890/03-5027
Myers, R. A., and Worm, B. (2003). Rapid worldwide depletion of predatory fish communities. Nature 423, 280–283. doi: 10.1038/nature01610
Myrberg, A. A. (1978). “Ocean noise and the behavior of marine animals: relationships and implications,” in Effects of Noise on Wildlife, eds J. L. Fletcher and R. G. Busnel (Cambridge, MA: Academic Press). doi: 10.1016/B978-0-12-260550-5.50012-2
Nagelkerken, I., van der Velde, G., Gorissen, M. W., Meijer, G. J., Van’t Hof, T., and den Hartog, C. (2000). Importance of mangroves, seagrass beds and the shallow coral reef as a nursery for important coral reef fishes, using a visual census technique. Estuarine Coastal Shelf Sci. 51, 31–44. doi: 10.1006/ecss.2000.0617
Nilsson, C., Aradottir, A. L., Hagen, D., Halldórsson, G., Høegh, K., Mitchell, R. J., et al. (2016). Evaluating the process of ecological restoration. Ecol. Soc. 21:41. doi: 10.5751/ES-08289-210141
Oksanen, J., Blanchet, G., Friendly, M., Kindt, R., Legendre, P., McGlinn, D., et al. (2020). vegan: Community Ecology Package. R Package Version 2.5-7. Available online at: https://CRAN.R-project.org/package=vegan (accessed November 28, 2020).
Piercy, J. C., Hill, A., Smith, D., and Simpson, S. (2014). Habitat quality affects sound production and likely distance of detection on coral reefs. Mar. Ecol. Prog. Series 516, 35–47. doi: 10.3354/meps10986
Pos, E., Guevara-Andino, J. E., Sabatier, D., Molino, J. F., Pitman, N., Mogollón, H., et al. (2014). Are all species necessary to reveal ecologically important patterns? Ecol. Evol. 4, 4626–4636. doi: 10.1002/ece3.1246
R Core Team (2020). R: A Language and Environment for Statistical Computing. Vienna: R Foundation for Statistical Computing.
Radford, C., Stanley, J., Tindle, C., Montgomery, J. C., and Jeffs, A. G. (2010). Localised coastal habitats have distinct underwater sound signatures. Mar. Ecol. Prog. Series 401, 21–29. doi: 10.3354/meps08451
Rochedo, P. R., Soares-Filho, B., Schaeffer, R., Viola, E., Szklo, A., Lucena, A. F., et al. (2018). The threat of political bargaining to climate mitigation in Brazil. Nat. Climate Change 8, 695–698. doi: 10.1038/s41558-018-0213-y
Salas, A. K., Altieri, A. H., Wilson, P. S., and Keitt, T. H. (2018). Predicting the reef acoustic cuescape from the perspective of larval fishes across a habitat quality gradient. Mar. Ecol. Prog. Series 605, 173–193. doi: 10.3354/meps12737
Shannon, G., McKenna, M. F., Angeloni, L. M., Crooks, K. R., Fristrup, K. M., Brown, E., et al. (2015). A synthesis of two decades of research documenting the effects of noise on wildlife. Biol. Rev. 91, 982–1005. doi: 10.1111/brv.12207
Short, F. T., and Wyllie-Echeverria, S. (1996). Natural and human-induced disturbance of seagrasses. Environ. Conserv. 23, 17–27. doi: 10.1017/S0376892900038212
Simpson, S. D., Meekan, M. G., Jeffs, A., Montgomery, J., andMcCauley, R. (2008). Settlement-stage coral reef fish prefer the higher-frequency invertebrate-generated audible component of reef noise. Animimal Behav. 75, 1861–1868. doi: 10.1016/j.anbehav.2007.11.004
Small, C., and Nicholls, R. J. (2003). A global analysis of human settlement in coastal zones. J. Coastal Res. 19, 584–599.
Sordello, R., Flamerie, De Lachapelle, F., Livoreil, B., and Vanpeene, S. (2019). Evidence of the environmental impact of noise pollution on biodiversity: a systematic map protocol. Environ. Evidence 8:8. doi: 10.1186/s13750-019-0146-6
Staaterman, E., Rice, A. N., Mann, D. A., and Paris, C. B. (2013). Soundscapes from a tropical Eastern Pacific reef and a Caribbean Sea reef. Coral Reefs 32, 553–557. doi: 10.1007/s00338-012-1007-8
Stanley, J. A., Radford, C. A., and Jeffs, A. G. (2010). Induction of settlement in crab megalopae by ambient underwater reef sound. Behav. Ecol. 21, 113–120. doi: 10.1093/beheco/arp159
Stanley, J. A., Radford, C. A., and Jeffs, A. G. (2011). Behavioural response thresholds in New Zealand crab megalopae to ambient underwater sound. PLoS One 6:e28572. doi: 10.1371/journal.pone.0028572
Stanley, J. A., Radford, C. A., and Jeffs, A. G. (2012). Location, location, location: finding a suitable home among the noise. Proc. R. Soc. Biol. 279, 3622–3631.
Tait, R. I. (1962). The Evening Chorus: a Biological Noise Investigation. Aukland: Naval Research Laboratory.
Tolimieri, N., Haine, O., Jeffs, A., McCauley, R., and Montgomery, J. (2004). Directional orientation of Pomacentrid larvae to ambient reef sound. Coral Reefs 23, 184–191. doi: 10.1007/s00338-004-0383-0
Tolimieri, N., Jeffs, A., and Montgomery, J. C. (2000). Ambient sound as a cue for navigation by the pelagic larvae of reef fishes. Mar. Ecol. Prog. Series 207, 219–224. doi: 10.3354/meps207219
Valentine, M. M., and Butler, M. J. IV (2019). Sponges structure water column characteristics in a shallow, tropical coastal ecosystem. Mar. Ecol. Prog. Series 608, 133–147. doi: 10.3354/meps12758
Vermeij, M. J., Marhaver, K. L., Huijbers, C. M., Nagelkerken, I., and Simpson, S. D. (2010). Coral larvae move toward reef sounds. PLoS One 5:e10660. doi: 10.1371/journal.pone.0010660
Williams, B. R., McAfee, D., and Connell, S. D. (2021). Repairing recruitment processes with sound technology to accelerate habitat restoration. Ecol. Appl. 31:e02386. doi: 10.1002/eap.2386
Wilson, C. J., Wilson, P. S., Greene, C. A., and Dunton, K. H. (2013). Seagrass meadows provide an acoustic refuge for estuarine fish. Mar. Ecol. Prog. Series 472, 117–127. doi: 10.3354/meps10045
Worm, B., Barbier, E. B., Beaumont, N., Duffy, J. E., Folke, C., Halpern, B. S., et al. (2006). Impacts of biodiversity loss on ocean ecosystem services. Science 314, 787–790. doi: 10.1126/science.1132294
Keywords: habitat restoration, marine soundscapes, acoustic enrichment, larval recruitment, PERMANOVA
Citation: Butler J, Anderson ER and Butler MJ IV (2022) Habitat Restoration Restores Underwater Soundscapes and Larval Recruitment. Front. Ecol. Evol. 10:785986. doi: 10.3389/fevo.2022.785986
Received: 29 September 2021; Accepted: 17 February 2022;
Published: 10 March 2022.
Edited by:
Mariana Mayer Pinto, University of New South Wales, AustraliaReviewed by:
Dominic Mcafee, University of Adelaide, AustraliaValeriya Komyakova, Norwegian Institute of Marine Research (IMR), Norway
Copyright © 2022 Butler, Anderson and Butler. This is an open-access article distributed under the terms of the Creative Commons Attribution License (CC BY). The use, distribution or reproduction in other forums is permitted, provided the original author(s) and the copyright owner(s) are credited and that the original publication in this journal is cited, in accordance with accepted academic practice. No use, distribution or reproduction is permitted which does not comply with these terms.
*Correspondence: Jack Butler, amJ1dGxlckBmaXUuZWR1