- 1Department of Forestry and Wildlife Management, Inland Norway University of Applied Sciences, Hamar, Norway
- 2Department of Ecology, Grimsö Wildlife Research Station, Swedish University of Agricultural Sciences, Uppsala, Sweden
Humans pose a major mortality risk to wolves. Hence, similar to how prey respond to predators, wolves can be expected to show anti-predator responses to humans. When exposed to a threat, animals may show a fight, flight, freeze or hide response. The type of response and the circumstances (e.g., distance and speed) at which the animal flees are useful parameters to describe the responses of wild animals to approaching humans. Increasing knowledge about behavioral responses of wolves toward humans might improve appropriate management and decrease conflicts related to fear of wolves. We did a pilot study by conducting 21 approach trials on seven GPS-collared wolves in four territories to investigate their responses to experimental human approaches. We found that wolves predominantly showed a flight response (N = 18), in a few cases the wolf did not flee (N = 3), but no wolves were seen or heard during trials. When wolves were downwind of the observer the flight initiation distance was significantly larger than when upwind, consistent with the hypothesis that conditions facilitating early detection would result in an earlier flight. Our hypothesis that early detection would result in less intense flights was not supported, as we found no correlation between flight initiation distances and speed, distance or straightness of the flight. Wolves in more concealed habitat had a shorter flight initiation distance or did not flee at all, suggesting that perceived risk might have been affected by horizontal visibility. Contrary to our expectation, resettling positions were less concealed (larger horizontal visibility) than the wolves’ initial site. Although our small number of study animals and trials does not allow for generalizations, this pilot study illustrates how standardized human approach trials with high-resolution GPS-data can be used to describe wolf responses at a local scale. In continuation, this method can be applied at larger spatial scales to compare wolf flight responses within and between populations and across anthropogenic gradients, thus increasing the knowledge of wolf behavior toward humans, and potentially improving coexistence with wolves across their range.
Introduction
In predator-prey systems, prey show anti-predator behaviors such as vigilance and altered foraging behavior to reduce the risk of being preyed upon (Ydenberg and Dill, 1986; Cooper and Frederick, 2007; Laundre et al., 2010). Detection is the first step in prey’s response to a predator (Bednekoff and Lima, 1998). Predation risk vary in time and space and vigilant behavior likely corresponds with the risk perception (Lima and Bednekoff, 1999; Gaynor et al., 2019). In low risk situations animals might spend less time on vigilant behavior and increase time feeding or resting (Lima and Bednekoff, 1999). In resting situation animals might select for protective cover, but potentially with an overview to detect any risks (Lazarus and Symonds, 1992).
Once a prey has detected a predator, it has four basic response options: flight, fight, hide, or freeze (Lima and Dill, 1990; Rupia et al., 2016; Roelofs, 2017). Different responses come with different energetic costs, which together with the perceived severity of risk (Cooper and Frederick, 2007) affects the response. Optimally, when the potential risk of staying exceeds the costs of fleeing, the animal should flee (Ydenberg and Dill, 1986; Lima and Dill, 1990; Cooper and Frederick, 2007). Additionally, the response in a given interaction can be affected by the animal’s personality and previous experience (Beale, 2007; Rupia et al., 2016; Found and Clair, 2018; Carricondo-Sanchez et al., 2020). Various disturbance intensities may affect the intensity and duration of the animal’s response (Beckmann et al., 2004; Ordiz et al., 2013; Petracca et al., 2019; Suraci et al., 2019).
Anti-predator behaviors are not limited to prey species. Even top predators might display similar behaviors to avoid intra-guild aggression (Holt and Polis, 1997; Swenson et al., 2001; Frid and Dill, 2002; Mech and Boitani, 2003; Wikenros et al., 2017) and as a response to human-induced disturbances (Gill et al., 1996; Frid and Dill, 2002; Moen et al., 2012). For wolves (Canis lupus), encountering humans is still not without risk. Lethal control, poaching, and traffic collisions are main sources of wolf mortality (Colino-Rabanal et al., 2011; Liberg et al., 2012, 2020; Recio et al., 2018), and human-related mortality currently limits the population growth of wolves in Europe (Kuijper et al., 2019; Liberg et al., 2020; Sunde et al., 2021). Hence, human-caused disturbances are expected to result in anti-predator behavior due to a potentially lethal risk for the wolf (Frid and Dill, 2002; Ordiz et al., 2011). In fact, even though wolves and other predators are known to make use of human-made structures (e.g., roads and bridges) (Blanco et al., 2005; Zimmermann et al., 2014; Dickie et al., 2017; Bojarska et al., 2020), they tend to avoid human activities, resulting in spatiotemporal segregation between wolf and human activities (Lesmerises et al., 2012; Milleret et al., 2019; Carricondo-Sanchez et al., 2020). The avoidance of human activity may have been shaped by the century-long history of wolf persecution by humans, as suggested for the brown bear (Ursus arctos) in Europe (Zedrosser et al., 2011).
Responses to approaching humans have been studied previously in wolves using information from VHF-collars (Karlsson et al., 2007; Wam et al., 2012, 2014), and more recently in brown bears using high-frequency GPS data (Moen et al., 2012, 2018; Ordiz et al., 2019). In wolves, flight initiation distance (FID, i.e., the distance at which an animal flees from an approaching threat) was affected by wind conditions, with shorter FID when the wind was blowing away from the wolf, but not by horizontal visibility at the wolf’s location (Karlsson et al., 2007). In contrast, reduced horizontal visibility at brown bear resting sites resulted in shorter FIDs when approached by humans (Moen et al., 2012). This indicates that the bear either made the decision to wait longer before fleeing (Beale, 2007; Cooper and Frederick, 2007), or it did not detect the observer (Moen et al., 2012), illustrating that the time of flight initiation does not necessarily equal the time of detection. After being disturbed by a human, VHF-collared wolves selected more concealed locations with lower horizontal visibility (Wam et al., 2012), and a recent study found that wolves select for more concealed resting sites during the day in response to increased human disturbances (Bojarska et al., 2021). Horizontal cover may benefit wolves more in terms of concealment more than it hampers their vigilance. Wolves have well developed auditory and olfactory systems, which may be more important in detection of threats over longer distances than visual detection (Mech, 1970; Harrington and Asa, 2003).
With this paper, we aimed to describe the flight response of seven wild GPS-collared wolves in Norway and Sweden by conducting experimental human approach trials. This is, to our knowledge, the first study assessing wolf flight responses toward humans with the use of high-resolution GPS data, which gives much more detailed and accurate information about flight intensity and flight patterns compared to VHF studies (Moen et al., 2012, 2018; Ordiz et al., 2019). High resolution GPS data makes it possible to re-construct details of the flight path without the need of snow tracking (Moen et al., 2012; Wam et al., 2012, 2014). With this advancement, fine-scaled studies of wolf flight responses no longer rely on snow cover, although supplementary snow-tracking may still have the potential to give additional behavioral information of importance.
Based on previous studies on wolves and bears (Karlsson et al., 2007; Moen et al., 2012) we hypothesized that wolves would avoid approaching humans (H1). Therefore, we predicted that during approach trials, wolves would show predominantly flight responses (P1) and flee before the observer would pass the wolf’s initial location (P2). If seen by the observer, we predicted that the wolves would retreat without signs of aggression (P3).
We hypothesized that wolves likelihood to flee would depend on the detectability of the approaching humans (H2). Previous studies have shown that FID during experimental human approaches can be affected by the number of observers for brown bears (Moen et al., 2012), and the wind condition for wolves (Karlsson et al., 2007). We predict larger FID with increasing number of observers (P4), observers walking though noisy vegetation (e.g., forest with dense regrowth of trees) (P5) and wind direction from observer to wolf (P6).
The wolf’s decision of whether and when to flee after detecting an observer may depend on the horizontal visibility at the individual’s location (i.e., wolf’s perception of risk) (H3). Previous study on bears found that individuals in more concealed resting site had shorter FIDs (Moen et al., 2012). We predicted that wolves resting at a more concealed location would wait for longer before fleeing (shorter FID) (P7) and have a higher occurrence of no flight (P8) compared to wolves at less concealed locations.
A higher response intensity is related to increased perceived risk (Ydenberg and Dill, 1986; Frid and Dill, 2002; Cooper and Frederick, 2007). Therefore, we hypothesized that an early retreat enabled by early detection would result in a lower perceived risk and thus a less intense flight (H4). From this hypothesis we predicted that larger FID would be associated with shorter (P9) and less straight (P10) flights at lower speed (P11).
Following the results from Wam et al. (2012), we hypothesized that after a flight, wolves will seek a more concealed resting location (H5). Therefore, we predicted that the wolf end position will have a shorter horizontal visibility than the wolf starting position (P12).
Materials and Methods
Study Area and Animals
The study area is located along the Scandinavian border between Norway and Sweden. It included three wolf territories south of Trysil (Norway) (61°02′N, 12°18′E), and one wolf territory near Charlottenberg (Sweden) (59°55′N, 12°11′E). The study area is mainly dominated by Norway spruce (Picea abies), Scots pine (Pinus sylvestris), and a lower abundance of birch (Betula spp.) and aspen (Populous tremula). The forests are intensively managed consisting of a mosaic of age classes and an extensive network of forest roads (Sand et al., 2008; Zimmermann et al., 2014). The human population density within the study area varied from 2 to 10 inhabitants per km2 (Statistisk Sentralbyrå, 2020). The main prey of wolves in the study area is moose (Alces alces) (Sand et al., 2008; Zimmermann et al., 2015).
Seven wolves (five males, two females) were captured and equipped with VERTEX Plus GPS collars from VECTRONIC Aerospace GmbH. All wolves were scent-marking, territorial adults, and all were confirmed breeders, reproducing prior to or after the trial(s) (see Table 1 for the overview of all approach trials). The captures followed the ethically approved procedures as described by Arnemo and Evans (2017). The captures and experimental human approach trials were approved by the Norwegian Food Safety Authority (FOTS ID 15370) and the Animal Welfare Ethics Committee of Uppsala, Sweden (ref. 5.8.18-13246/2019). The GPS data were collected using GSM and Iridium communication into the Wireless Remote Animal Monitoring database system for data validation and management (Dettki et al., 2013).
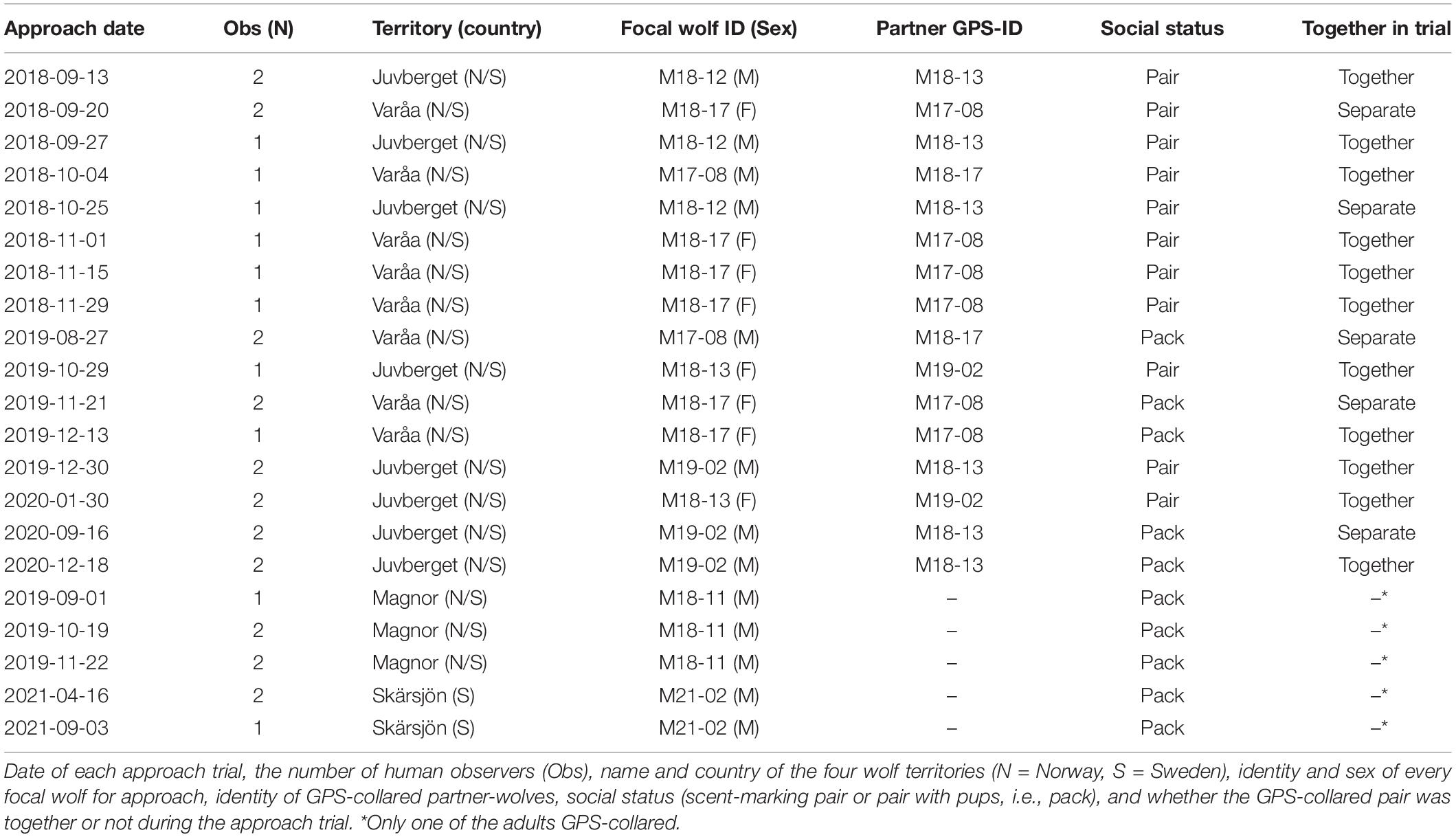
Table 1. Approach trials by humans toward seven territorial scent-marking, GPS-collared wolves along the Swedish-Norwegian border, 2018–2021.
Experimental Approach Trials
For the approach trials we followed the standardized protocols for collar schedule, approach method, field data collection and GPS data extraction1. Trials were conducted between mid-August and April in order to avoid disturbance during the denning and pup rearing period. We used minimum 14 days between consecutive trials on the same individuals (Table 1). Approach trials were only conducted when wolves were stationary at a resting site, which was determined based on their GPS positions before the trial started. We used a 4 h preparation period with 10-min positioning intervals to define the approach route. The observer(s) would start approaching the wolf from a minimum distance of 1,000 m from the wolf start position (WSP, last received GPS position before the trial), pass at a passing position (PP) 50 m from WSP, and continue walking for at least another 500 m. The approach route was as straight as possible and did not follow existing roads or paths. The actual passing distance might not have been exactly 50 m due to GPS error and small wolf movement after the last received GPS position.
We used 1-min GPS positioning intervals during the approach period (12:00–14:00 local time), which allowed us to extract the flight initiation at high precision, and provided fine-scale data for the initial flight response. The observer position was logged every second using a handheld GPS unit. In order to collect consistently a minimum of 10 min of flight data at 1-min resolution, all approach trials were started in time to reach the PP at least 10 min before the approach period ended.
During single-observer trials, the observer did not make an effort to be quiet but was not talking. During two-observer trials, the observers would talk to each other. In total 17 different observers (seven males and ten females) conducted the approach trials, in different combinations and avoiding the same observer(s) conducting consecutive trials on the same individual. The observer would register wind direction relative to a clock, where 12:00 o’clock related to the observer’s orientation toward the end of the approach route. Wind direction was later converged to head wind (wind from the wolf toward observer) when the wind came from 9:00 to 3:00 o’clock and tail wind (wind from observer toward the wolf) when the wind came from 3:00 to 9:00 o’clock. Furthermore, the observer estimated the noise made by walking through the vegetation at three levels: silent (e.g., mossy/peaty soil with no bushes), medium (e.g., crackling sound from leaves, some bushes scratching on observers clothes), and noisy (e.g., young forest with dense regrowth of trees). The level silent only occurred twice, therefore we pooled this level with medium and used two levels, noisy and not noisy, for further analyses.
We used 10-min positioning intervals during the post-disturbance period (14:00–17:00 local time) to capture the entire flight and to identify resettling. Post-trial we measured the horizontal visibility at the wolf’s initial location and at the resettling position by placing a cylinder (brightly colored with a length of 60 cm and diameter of 30 cm) at the coordinates of the positions. We measured the distance at which the cylinder was still visible in the four cardinal directions and calculated the average distance as a proxy for concealment, using the method described by Ordiz et al. (2009).
Data Analyses
We used the software R, version 4.1.1 (R Core Team, 2021) for all data handling and analyses. When two GPS collared wolves were together during an approach trial (i.e., the adult territorial pair), only one was included in the analyses, as their responses could not be assumed to be independent of one another. If a flight was detected, we selected the wolf that moved first. In trials without detected flights, we chose the wolf which was passed closest by the observer.
For each trial the wolf and observer data were joined based on the timestamp, and the observer positions (originally 1-s intervals) were filtered to retain only those positions that matched the timestamps of the wolf positions (1-min intervals). The wolf moving speed was calculated as meters per minute by dividing the step length by the time difference between consecutive positions. The flight initiation was extracted by applying changepoint analysis for both change in mean and variance of the wolf speed at 1-min resolution using an MBIC (Modified Bayes Information System) penalty (Killick et al., 2016). In two cases in which no flight was detected with the MBIC penalty but visual inspection suggested that the wolf fled, the flight initiation was identified by rerunning changepoint analysis with the AIC penalty (see text footnote 1). We considered a flight when we detected the flight initiation within the 1-min resolution data. Additionally, we visually checked the wolf positions until the observer reached the end of the approach route. For 15 wolf flights, we identified the resettling position by applying changepoint analysis as described for flight initiation, but using GPS data at 10-min resolution.
Based on the obtained flight initiation and resettling positions, we extracted 11 variables to describe the wolf flight response for every interaction separately: We classified the wolf response as either Flight when flight initiation was identified, or No flight when no flight initiation was identified and the wolf remained stationary (1). Based on the 1-min positioning intervals, we calculated the Minimum wolf-observer distance as the minimum distance between simultaneous wolf and observer positions (2), FID as the wolf-observer distance at flight initiation (3), and Passing-flight time difference as the time difference between flight initiation and the observer passing the passing position (4). For the first 10 min after flight initiation and at 1-min resolution, we calculated Initial speed as the average speed (5) and Initial straightness as the sum of the step lengths divided by the linear displacement (6). We calculated Flight duration (7) and Flight displacement (8) as the time and distance from flight initiation to resettling, respectively. For the total flight (from flight initiation to resettling) and at 10-min resolution, we calculated Total distance traveled as the sum of the step lengths (9), Overall speed as the average speed (10), and Overall straightness as the average straightness index across the flight based on the straightness between every three consecutive positions (11) (see text footnote 1).
We did not test for consistent territory differences due to the small sample size. None of the variables showed visual differences between the territories (Supplementary Figure 1). Due to the small sample size and non-normality of the data we could not run multiple regression models. We used non-parametric tests to look at differences in the median of the response variables between categories (number of observers, noise level and wind direction, H2), we used the non-parametric Wilcoxon rank-sum test. We looked at the relationship between FID and horizontal visibility (H3), and between the different flight variables (H4) by using non-parametric Spearman’s rank-order correlation tests. Finally, we used Wilcoxon signed rank tests to compare concealment at flight initiation and resettling (H5). We visualized the results using the ggplot2 package (Wickham, 2009) and the ggcorplot package (Kassambara, 2019).
Results
We performed 21 successful experimental wolf approaches over the course of 4 years (8 in 2018, 8 in 2019, 3 in 2020, and 2 in 2021). Individual wolves were approached on average 4 times (range: 2–7, Table 1). Wolves fled in 18 out of 21 interactions, and did not initiate a flight in the other three interactions (P1). In two thirds (N = 12) of the cases, the flight initiation occurred before the observer(s) passed the passing position, and in one third (N = 6) shortly after the observer(s) passed (Figure 1, P2). Observers did not see or hear the wolves during any of the approach trials (P3).
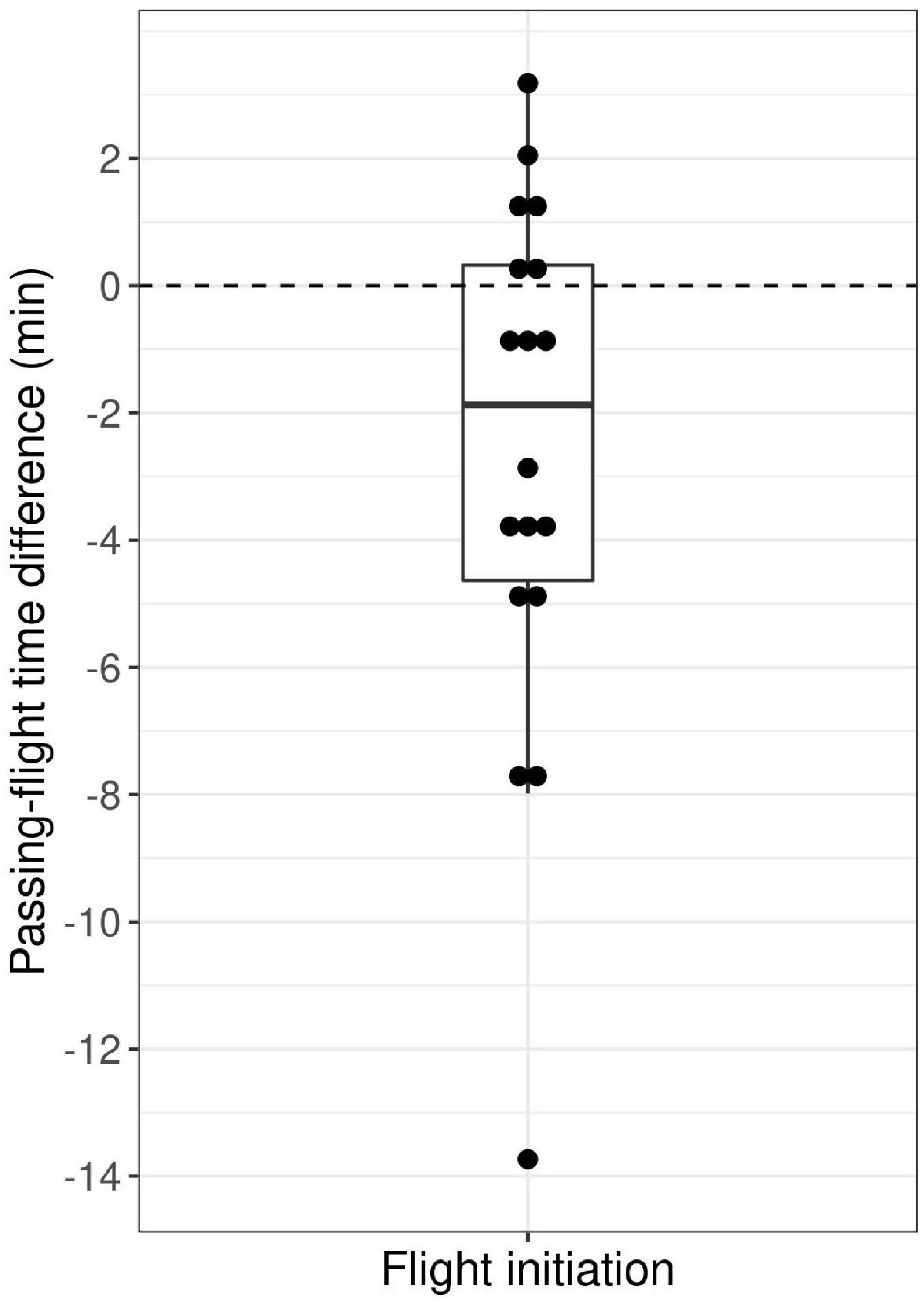
Figure 1. Time difference between the start of the wolf’s flight and the observer passing the passing position (PP) of wolves during experimental approach trials by humans in south-central Scandinavia, 2018–2021 (N = 18), where a negative time difference indicates a flight started before the observer passed the PP.
The flight initiation distance was significantly different between trials conducted with head wind and tail wind (Wilcoxon rank-sum test: W = 1, n = 11, p = 0.009, Figure 2, P6). However, the FID did not differ significantly between one or two observers present (Wilcoxon rank-sum test: W = 19, n = 18, p = 0.068, P4) or between noisy and not noisy conditions (Wilcoxon rank-sum test: W = 44, n = 17, p = 0.481, P5).
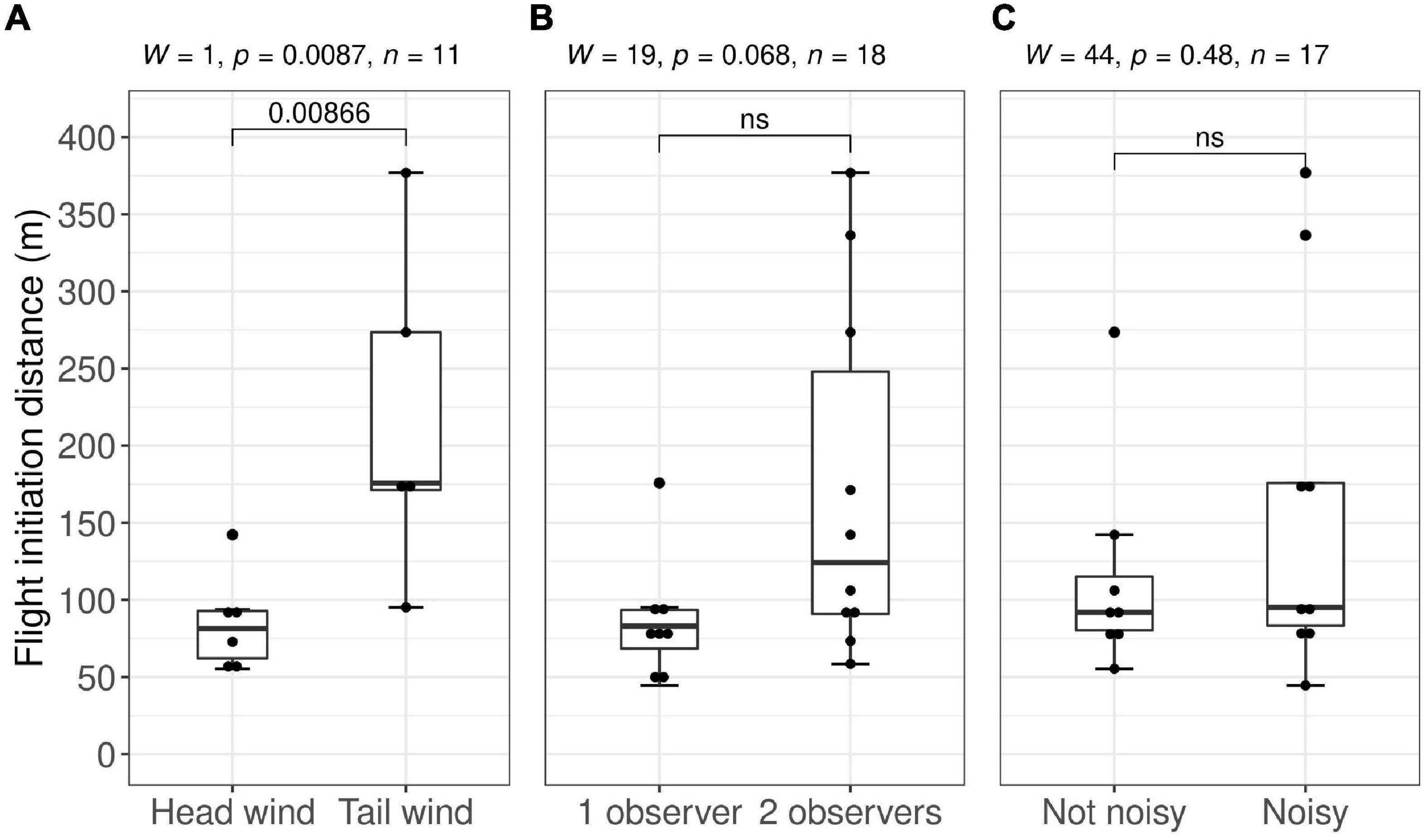
Figure 2. Distribution of flight initiation distances (meters) with (A) head wind (wind from the wolf toward observer, N = 6) vs. tail wind (wind from observer toward the wolf, N = 5), (B) single-observer approach (N = 8) vs. double-observer approach (N = 10), and (C) not noisy (N = 8) vs. noisy sounds (N = 9) by walking through the vegetation. Using the non-parametric Wilcoxon rank-sum test based on experimental approach trials by humans on wolves in south-central Scandinavia, 2018–2021.
Flight initiation distance and horizontal visibility were positively correlated (rs = 0.4), i.e., the FID increased when the wolf was less concealed (Figure 3, P7). Additionally, the horizontal visibility for three interactions where the wolf did not flee was low, with a mean visibility of 4, 6, and 14 m, respectively (P8), while the median horizontal visibility of the resting sites for fleeing wolves was 16 m (quartiles 7; 22 m).
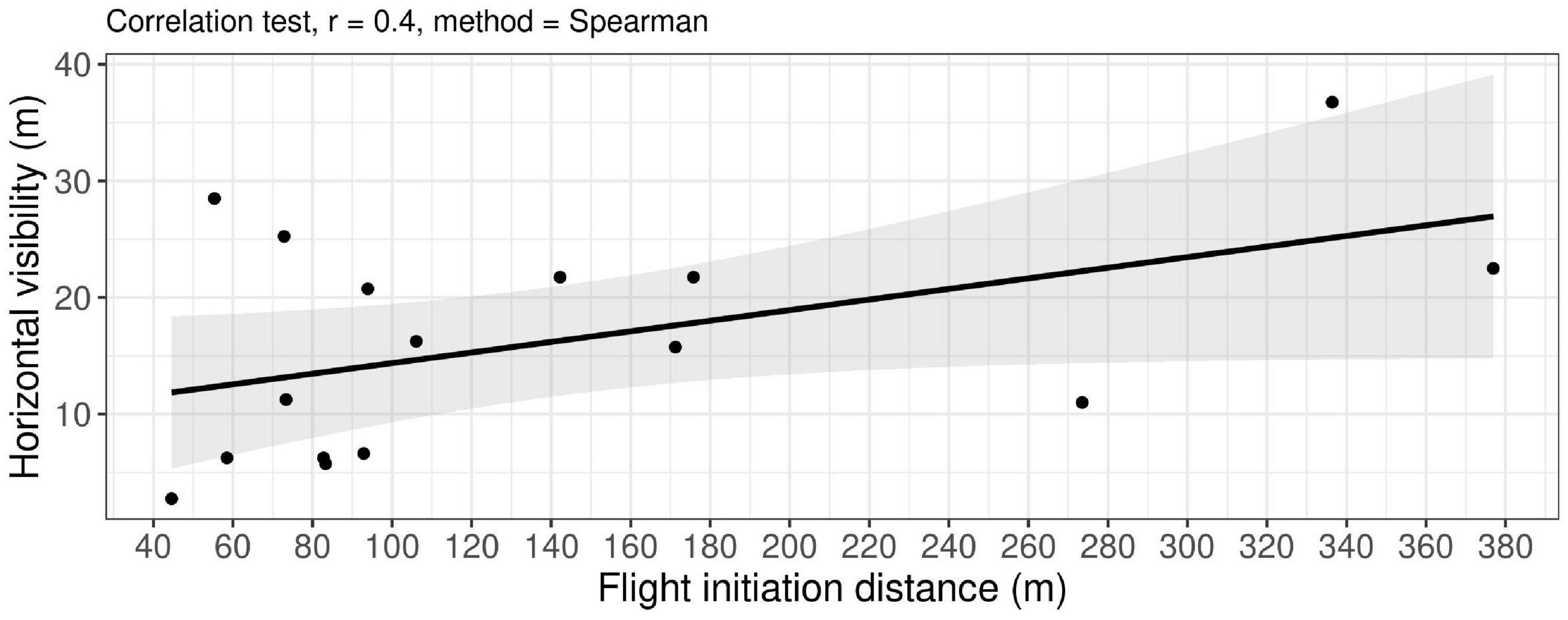
Figure 3. The relation between flight initiation distances (N = 16) and the horizontal visibility (both in meters). Using the non-parametric Spearman’s rank-order correlation test based on experimental approach trials by humans on wolves in south-central Scandinavia, 2018–2021. Wolves that did not fled are excluded (N = 3).
We found that flight duration, total distance traveled, and flight displacement were positively correlated (rs > 0.6, Figure 4 and Table 2). Therefore, we only analyzed the total distance traveled. Together with initial speed, initial straightness and overall straightness we considered those variables as a proxy representing flight intensity. We found no correlation between the FID and the total distance traveled (rs = 0.1, P9), initial straightness (rs = –0.12) and overall straightness (rs = –0.24) (P10), and initial speed (rs = 0.18, P11).
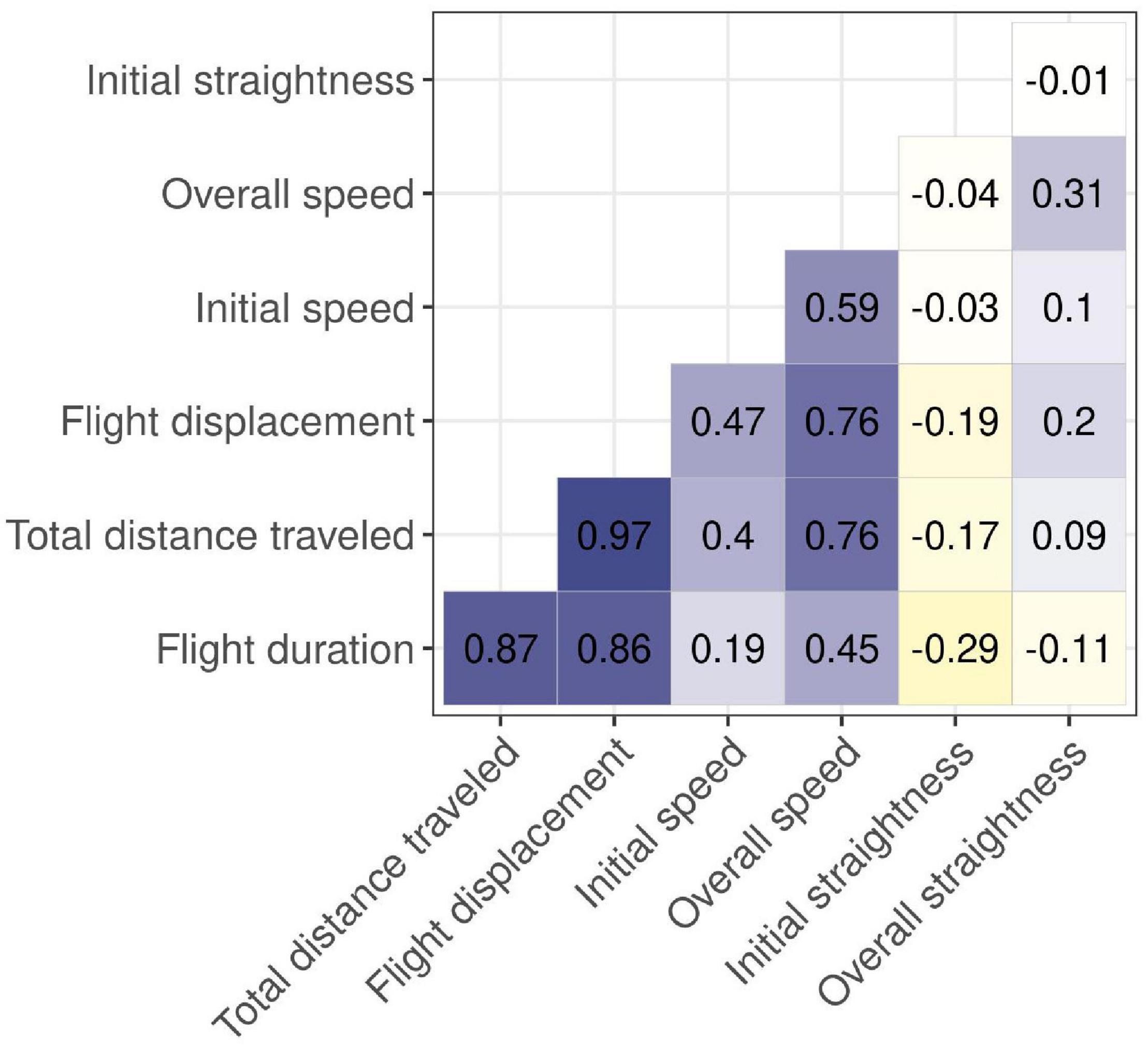
Figure 4. Correlation matrix with correlation coefficients showing relations between the variables, using non-parametric Spearman’s rank-order correlation test based on experimental approach trials by humans on wolves in south-central Scandinavia, 2018–2021.
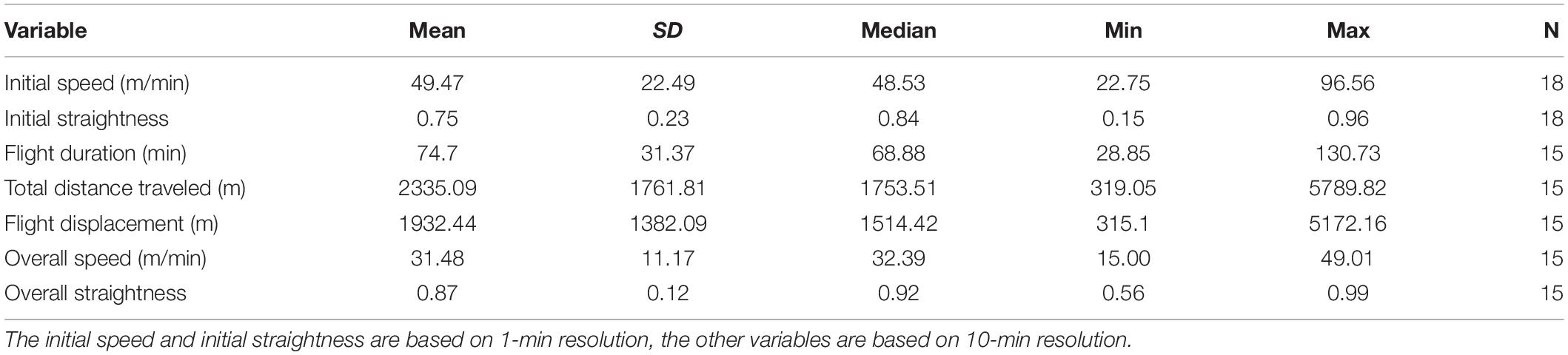
Table 2. Overview of the flight variables with the mean, standard deviation (SD), median, min, max, and number of observations (N).
The horizontal visibility showed a significant increase between the wolf start position and the wolf end position (Wilcoxon signed-ranked test: V = 78, p = 0.025, N = 13, Figure 5, P12).
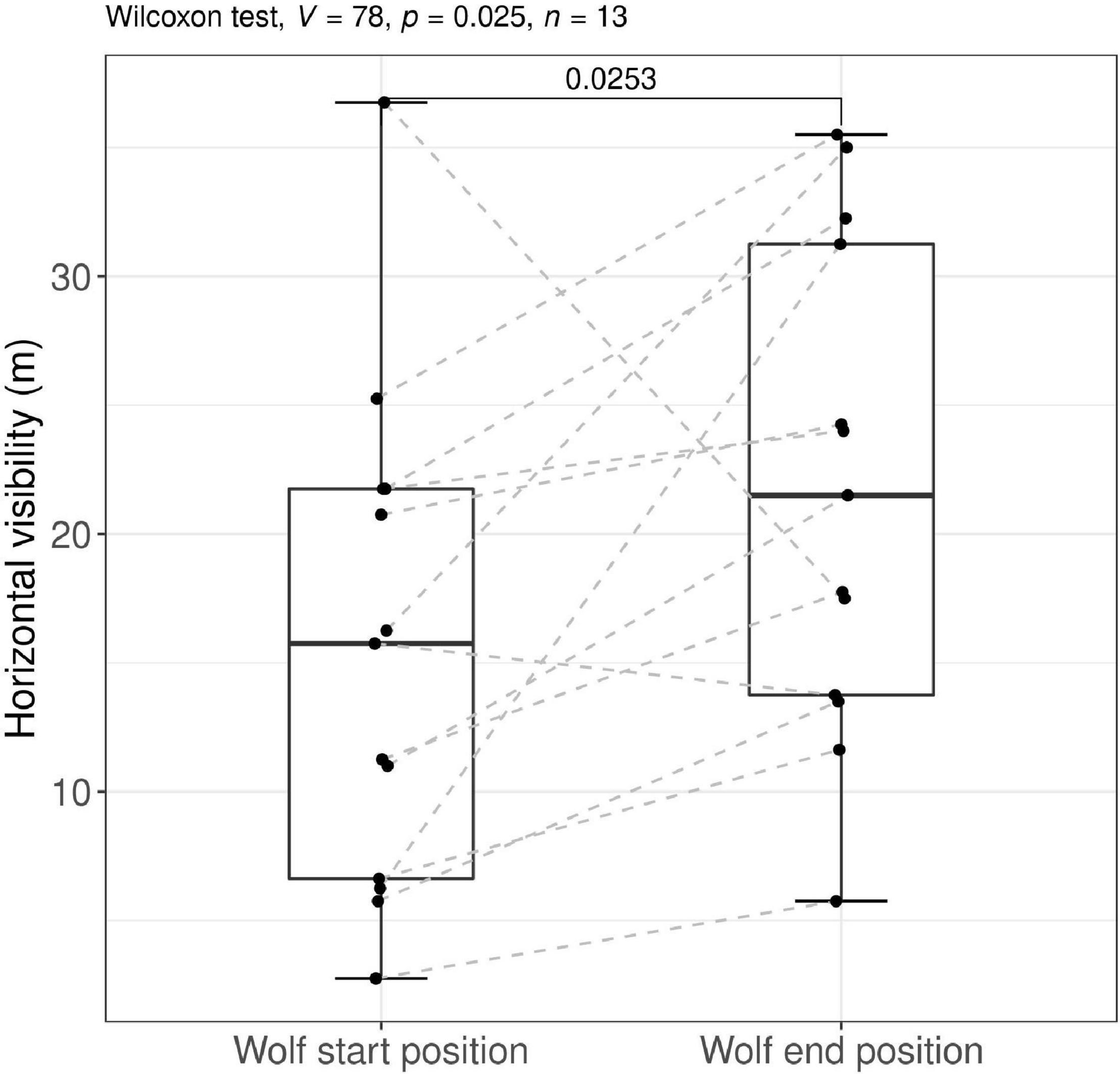
Figure 5. Distribution of the horizontal visibility (in meters) at the wolf start position (WSP) and the wolf end position. The difference in horizontal visibility tested by a non-parametric Wilcoxon signed rank tests. The gray dashed lines indicate paired observations. Observations are based on experimental approach trials by humans on wolves in south-central Scandinavia, 2018–2021.
Discussion
Most wolves that were approached in this pilot study showed what we interpret as an avoidance response, consistent with our first hypothesis (H1). In the majority of trials, the wolf left its initial resting site before the observer(s) passed the passing position (PP). During the remaining trials, the wolf fled shortly after the observer passed, or did not flee at all. No wolves were seen or heard during the approach trials, even when the observer(s) passed the wolf at less than 50 m. Kuijper et al. (2019) described getting closer than 100 m to wolves as “risk-enhancing human behavior”. However, similar to previous studies on wolves as well as brown bears (Karlsson et al., 2007; Moen et al., 2012; Wam et al., 2014; Ordiz et al., 2019), the wolves did not show any aggressive response to our approach trials.
A wolf’s decision to flee from an approaching human is contingent on the human being detected by the wolf. Despite their acute senses, Karlsson et al. (2007) demonstrated that it is possible to walk up to a resting wolf undetected. Hence, we hypothesized that FID would be larger under conditions that increase the detectability of humans (H2). Olfactory and auditory cues are important for a wolf’s ability for communication, tracking prey, and social interactions (Mech, 1970; Harrington and Asa, 2003). Therefore, it is likely that sound and smell might be important for detecting threats as well. Karlsson et al. (2007) found that a combination of wind direction and wind speed affected wolf FID during earlier approach trials and hypothesized that noise made by the observer likely affected the wolf’s FID. We found a significant difference between tail wind and head wind, where tail wind resulted in larger flight initiation distances. Unfortunately, we did not have sufficient observations of wind speed to make a similar comparison. The FID did not vary significantly with number of observers or the level of noise. Potentially, the noise made by the observer may have been masked by noise created by the wind. Exploring, this and other interactions between the explanatory variables will require a larger sample size and multiple regression analysis.
Once a wolf has detected an approaching human, the decision to flee should reflect the perception of risk (Ydenberg and Dill, 1986; Cooper and Frederick, 2007). We found a weak positive correlation between FID and the horizontal visibility at the wolf start position (H3). Furthermore, during three interactions without a flight, the horizontal visibility was low (<15 m). This is partly consistent with our hypothesis that wolves would perceive the risk as lower when they were more concealed, and allow the human to get closer before fleeing, or choose to not flee at all. However, GPS data alone does not allow us to identify the moment of detection, but rather shows the moment when the wolf responds spatially by dislocation. Hence, we cannot conclude whether the shorter FID or no flight at lower visibility was due to the wolf’s perception of being less detectable by the observer (i.e., lower perceived risk) or because the wolf did not detect the observer. This was also described by Moen et al. (2012) who found that the FID for brown bears increased as the horizontal vegetation structure became less dense. Potentially, the wolf’s social status, season, and habituation to human presence might affect the decision to flee, though the effect of habituation were found to be minor (Wam et al., 2014). Fine-scale accelerometer data or heart rate data from the wolves could provide additional information to distinguish between detection and risk perception by potentially measuring changes in heart rate, posture or fine-scale movement before the wolf flees (Græsli et al., 2020; Williams et al., 2020).
We expected that a shorter FID would be related with a more intense flight (larger distance traveled, higher speeds) due to a flush effect if the observer was not detected, or not perceived as a threat, until it was close by (H4). This could result in an energetically more costly flight (Ydenberg and Dill, 1986; Cooper and Frederick, 2007). However, we did not find a correlation between FID and the different flight variables. Other variables, such as landscape composition, road density and human population density might also have an effect on the initiation and path of the flight (Moen et al., 2018), but were not included in our study due to low sample size.
Wam et al. (2012) showed that wolves can adjust their strategy in choosing a resting site in a more concealed location after being disturbed. After centuries of persecution wolves might choose hiding and therefore sacrificing visual vigilance for better concealment to avoid human encounters (Wam et al., 2012). Similar strategies are found in lynx (Lynx lynx) and brown bear (Sunde et al., 1998; Ordiz et al., 2011). Therefore, we hypothesized that wolves would select for a more concealed (i.e., shorter horizontal visibility) resting site after being approached by a human (H5). Interestingly, we found the opposite effect where wolves chose resettling locations which were slightly more open and less concealed compared to their resting sites prior to the disturbance. It is likely that resting site selection after a disturbance might lead wolves to select places with a better overview on approaching humans, so they can initiate a new flight earlier. This would follow earlier results from a study on vigilance and protective cover on birds (Lazarus and Symonds, 1992). Additionally, concealed areas are available throughout the wolf’s territory, and there is no lack of available sites. An increased sample size would give the possibility to explore whether this pattern is consistent and to what degree other factors (e.g., elevation and vegetation types) affect the resettling site selection.
The sample size in this study was small and we could not account for individual differences between wolves, but we are aware that individual choices may exist (Beale, 2007). However, we did not perceive visually obvious differences in response variables between wolf territories. Such potential differences should be considered, and for future studies with larger sample size, we would advise considering the effects of individuals and territories, together with variables as e.g., social status and sex.
Moreover, the small sample size does not allow us to generalize the results across the Scandinavian wolf population, let alone to wolves in general. This first study rather serves to illustrate the application of standardized human approach trials with high-resolution GPS-data for describing wolf responses at a local scale. In continuation, the protocol (see text footnote 1) can be applied at larger spatial scales to compare wolf flight responses within and between populations and across anthropogenic gradients. Potential applications are to establish the range of responses by wolves in a given area, allowing the identification of individual wolves that show atypical response patterns, or to identify consistent differences between wolves inhabiting different habitat types in different parts of their range. This may lead a better understanding of how wolves can be expected to behave toward humans, and thus improve the potential for wolf-human coexistence across their range. If future research confirms what the current and previous studies suggest (Karlsson et al., 2007), i.e., that wolves generally avoid approaching humans, and that increased detectability increases the likelihood of an early flight by the wolf, people who wish to avoid a wolf encounter when walking in wolf areas should maximize their possibility of being detected.
Data Availability Statement
The raw data supporting the conclusions of this article will be made available by the authors, without undue reservation.
Ethics Statement
The animal study was reviewed and approved by the Norwegian Food Safety Authority (FOTS ID 15370) and the Animal Welfare Ethics Committee of Uppsala, Sweden (ref. 5.8.18-13246/2019).
Author Contributions
PW, BZ, AE, and EV conceived the study. AE, PW, BF, CW, HS, and BZ secured the funding. EV, BF, AE, BZ, and PW conducted field trials. EV conducted the analyses and drafted the manuscript. All authors contributed ideas and edits to the manuscript and approved the submitted version.
Funding
This study was funded by the Innlandet Regional Research Fund and the County Governors of Hedmark, Innlandet, and Oslo and Viken. The Norwegian Environment Agency, the Interreg Sweden-Norway, and the Inland Norway University of Applied Sciences funded wolf-captures and GPS-collaring through the Scandinavian Wolf Research Project (SKANDULV). EV worked on this manuscript funded by the Erasmus + post-graduate grant.
Conflict of Interest
The authors declare that the research was conducted in the absence of any commercial or financial relationships that could be construed as a potential conflict of interest.
Publisher’s Note
All claims expressed in this article are solely those of the authors and do not necessarily represent those of their affiliated organizations, or those of the publisher, the editors and the reviewers. Any product that may be evaluated in this article, or claim that may be made by its manufacturer, is not guaranteed or endorsed by the publisher.
Acknowledgments
We are very grateful to Henrike Hensel for collar programming, and to Morten Heim and Henrike Hensel for technical assistance and troubleshooting for GPS data access. We would also like to thank Giorgia Ausilio, Doris Friedrich, Malena Diaz Gómez, Emma den Hartog, Rick Heeres, Theresa Kirchner, Kristoffer Nordli, Erling Maartmann, Roberto Lo Monaco, Irene Garcia Noa, Ilka Reinhard, and Juuli Vänni for participation in field trials.
Supplementary Material
The Supplementary Material for this article can be found online at: https://www.frontiersin.org/articles/10.3389/fevo.2022.792916/full#supplementary-material
Footnotes
- ^ Eriksen, A., Versluijs, E., Fuchs, B., Zimmermann, B., Wabakken, P., Ordiz, A. (2022). A standardized method for experimental human approach trials on wild wolves. Front. Ecol. Evol.
References
Arnemo, J. M., and Evans, A. L. (2017). Biomedical Protocols for Free-ranging Brown Bears, Wolves, Wolverines and Lynx. Inland Nor. Univ. Appl. Sci. 16, 1–16. Available online at: http://bearproject.info/wp-content/uploads/2014/07/E-55-Biomedical-protocols-brown-bears-wolves_2005.pdf
Beale, C. M. (2007). The Behavioral Ecology of Disturbance Responses. Int .J. Comp. Psychol. 20, 111–120.
Beckmann, J. P., Lackey, C. W., and Berger, J. (2004). Evaluation of deterrent techniques and dogs to alter behavior of “nuisance” black bears. Wildl. Soc. Bull. 32, 1141–1146. doi: 10.2193/0091-7648(2004)032[1141:eodtad]2.0.co;2
Bednekoff, P. A., and Lima, S. L. (1998). Randomness, chaos and confusion in the study of antipredator vigilance. Trends Ecol. Evol. 13, 284–287. doi: 10.1016/s0169-5347(98)01327-5
Blanco, J. C., Cortés, Y., and Virgós, E. (2005). Wolf response to two kinds of barriers in an agricultural habitat in Spain. Can. J. Zool. 83, 312–323. doi: 10.1139/z05-016
Bojarska, K., Maugeri, L., Kuehn, R., Król, W., Theuerkauf, J., Okarma, H., et al. (2021). Wolves under cover: the importance of human-related factors in resting site selection in a commercial forest. For. Ecol. Manag. 497:119511. doi: 10.1016/j.foreco.2021.119511
Bojarska, K., Sulich, J., Bachmann, S., Okarma, H., Theuerkauf, J., and Gula, R. (2020). Opportunity and peril: how wolves use a dense network of forest roads. Mamm. Biol. 100, 203–211. doi: 10.1007/s42991-020-00014-0
Carricondo-Sanchez, D., Zimmermann, B., Wabakken, P., Eriksen, A., Milleret, C., Ordiz, A., et al. (2020). Wolves at the door? Factors influencing the individual behavior of wolves in relation to anthropogenic features. Biol. Conserv. 244:108514. doi: 10.1016/j.biocon.2020.108514
Colino-Rabanal, V. J., Lizana, M., and Peris, S. J. (2011). Factors influencing wolf Canis lupus roadkills in Northwest Spain. Eur. J. Wildl. Res. 57, 399–409. doi: 10.1007/s10344-010-0446-1
Cooper, W. E., and Frederick, W. G. (2007). Optimal flight initiation distance. J. Theor. Biol. 244, 59–67. doi: 10.1016/j.jtbi.2006.07.011
Dettki, H., Ericsson, G., Giles, T., and Norrsken-Ericsson, M. (2013). “Wireless Remote Animal Monitoring (WRAM)-A new international database e-infrastructure for telemetry sensor data from fish and wildlife,” in Proceedings Etc. 2012: convention for telemetry, test instrumentation and telecontrol, (Norderstedt, Germany: Books on Demand)
Dickie, M., Serrouya, R., McNay, R. S., and Boutin, S. (2017). Faster and farther: wolf movement on linear features and implications for hunting behaviour. J. Appl. Ecol. 54, 253–263. doi: 10.1111/1365-2664.12732
Found, R., and Clair, C. C. S. (2018). Personality influences wildlife responses to aversive conditioning. J. Wildl. Manag. 82, 747–755. doi: 10.1002/jwmg.21449
Frid, A., and Dill, L. (2002). Human-caused Disturbance Stimuli as a Form of Predation Risk. Conserv. Ecol. 6:11.
Gaynor, K. M., Brown, J. S., Middleton, A. D., Power, M. E., and Brashares, J. S. (2019). Landscapes of Fear: spatial Patterns of Risk Perception and Response. Trends Ecol. Evol. 34, 355–368. doi: 10.1016/j.tree.2019.01.004
Gill, J. A., Sutherland, W. J., and Watkinson, A. R. (1996). A Method to Quantify the Effects of Human Disturbance on Animal Populations. J. Appl. Ecol. 33, 786–792. doi: 10.2307/2404948
Græsli, A. R., Le Grand, L., Thiel, A., Fuchs, B., Devineau, O., Stenbacka, F., et al. (2020). Physiological and behavioural responses of moose to hunting with dogs. Conserv. Physiol. 8:coaa122. doi: 10.1093/conphys/coaa122
Harrington, F. H., and Asa, C. S. (2003). “Wolf communication,” in Wolves: behavior, ecology, and conservation, eds M. L. David and L. Boitani (Chicago, IL: University of Chicago).
Holt, R. D., and Polis, G. A. (1997). A Theoretical Framework for Intraguild Predation. Am. Nat. 149, 745–764. doi: 10.1086/286018
Karlsson, J., Eriksson, M., and Liberg, O. (2007). At what distance do wolves move away from an approaching human? Can. J. Zool. 85, 1193–1197. doi: 10.1139/z07-099
Kassambara, A. (2019). ggcorrplot: visualization of a Correlation Matrix using “ggplot2.” Package Version 0.1.3
Killick, R., Haynes, K., and Eckley, I. A. (2016). changepoint: an R package for changepoint analysis R package version 2.2.2
Kuijper, D. P. J., Churski, M., Trouwborst, A., Heurich, M., Smit, C., Kerley, G. I. H., et al. (2019). Keep the wolf from the door: how to conserve wolves in Europe’s human-dominated landscapes? Biol. Conserv. 235, 102–111. doi: 10.1016/j.biocon.2019.04.004
Laundre, J. W., Hernandez, L., and Ripple, W. J. (2010). The Landscape of Fear: ecological Implications of Being Afraid~!2009-09-09~!2009-11-16~!2010-02-02~! Open Ecol. J. 3, 1–7. doi: 10.2174/1874213001003030001
Lazarus, J., and Symonds, M. (1992). Contrasting effects of protective and obstructive cover on avian vigilance. Anim. Behav. 43, 519–521. doi: 10.1016/s0003-3472(05)80110-1
Lesmerises, F., Dussault, C., and St-Laurent, M.-H. (2012). Wolf habitat selection is shaped by human activities in a highly managed boreal forest. For. Ecol. Manag. 276, 125–131. doi: 10.1016/j.foreco.2012.03.025
Liberg, O., Chapron, G., Wabakken, P., Pedersen, H. C., Hobbs, N. T., and Sand, H. (2012). Shoot, shovel and shut up: cryptic poaching slows restoration of a large carnivore in Europe. Proc. R. Soc. B. Biol. Sci. 279, 910–915. doi: 10.1098/rspb.2011.1275
Liberg, O., Suutarinen, J., Åkesson, M., Andrén, H., Wabakken, P., Wikenros, C., et al. (2020). Poaching-related disappearance rate of wolves in Sweden was positively related to population size and negatively to legal culling. Biol. Conserv. 243:108456. doi: 10.1016/j.biocon.2020.108456
Lima, S. L., and Bednekoff, P. A. (1999). Temporal Variation in Danger Drives Antipredator Behavior: the Predation Risk Allocation Hypothesis. Am. Nat. 153, 649–659. doi: 10.1086/303202
Lima, S. L., and Dill, L. M. (1990). Behavioral decisions made under the risk of predation: a review and prospectus. Can. J. Zool. 68, 619–640. doi: 10.1139/z90-092
Mech, L. D. (1970). The Wolf: the Ecology and Behavior of an Endangered Species. New York: Doubleday.
Mech, L. D., and Boitani, L. (2003). “Wolf social ecology,” in Wolves: behavior, ecology, and conservation, eds M. L. David and L. Boitani (Chicago, IL: University of Chicago).
Milleret, C., Ordiz, A., Sanz-Pérez, A., Uzal, A., Carricondo-Sanchez, D., Eriksen, A., et al. (2019). Testing the influence of habitat experienced during the natal phase on habitat selection later in life in Scandinavian wolves. Sci. Rep. 9:6526. doi: 10.1038/s41598-019-42835-1
Moen, G. K., Ordiz, A., Kindberg, J., Swenson, J. E., Sundell, J., and Støen, O.-G. (2018). Behavioral reactions of brown bears to approaching humans in Fennoscandia. Écoscience 26, 23–33. doi: 10.1080/11956860.2018.1513387
Moen, G. K., Støen, O.-G., Sahlén, V., and Swenson, J. E. (2012). Behaviour of Solitary Adult Scandinavian Brown Bears (Ursus arctos) when Approached by Humans on Foot. PLoS One 7:e31699. doi: 10.1371/journal.pone.0031699
Ordiz, A., Moen, G. K., Saebo, S., Stenset, N., Swenson, J. E., and Stoena, O.-G. (2019). Habituation, sensitization, or consistent behavioral responses? Brown bear responses after repeated approaches by humans on foot. Biol. Conserv. 232, 228–237. doi: 10.1016/j.biocon.2019.01.016
Ordiz, A., Støen, O.-G., Delibes, M., and Swenson, J. E. (2011). Predators or prey? Spatio-temporal discrimination of human-derived risk by brown bears. Oecologia 166, 59–67. doi: 10.1007/s00442-011-1920-5
Ordiz, A., Støen, O.-G., Langebro, L. G., Brunberg, S., and Swenson, J. E. (2009). A practical method for measuring horizontal cover. Ursus 20, 109–113. doi: 10.2192/08sc031.1
Ordiz, A., Støen, O.-G., Sæbø, S., Sahlén, V., Pedersen, B. E., Kindberg, J., et al. (2013). Lasting behavioural responses of brown bears to experimental encounters with humans. J. Appl. Ecol. 50, 306–314. doi: 10.1111/1365-2664.12047
Petracca, L. S., Frair, J. L., Bastille-Rousseau, G., Hunt, J. E., Macdonald, D. W., Sibanda, L., et al. (2019). The effectiveness of hazing African lions as a conflict mitigation tool: implications for carnivore management. Ecosphere 10:e02967.
R Core Team (2021). R: a Language and Environment for Statistical Computing. Vienna: R Foundation for Statistical Computing.
Recio, M. R., Zimmermann, B., Wikenros, C., Zetterberg, A., Wabakken, P., and Sand, H. (2018). Integrated spatially-explicit models predict pervasive risks to recolonizing wolves in Scandinavia from human-driven mortality. Biol. Conserv. 226, 111–119. doi: 10.1016/j.dib.2018.08.060
Roelofs, K. (2017). Freeze for action: neurobiological mechanisms in animal and human freezing. Philos. Trans. R. Soc. B Biol. Sci. 372:20160206. doi: 10.1098/rstb.2016.0206
Rupia, E. J., Binning, S. A., Roche, D. G., and Lu, W. (2016). Fight-flight or freeze-hide? Personality and metabolic phenotype mediate physiological defence responses in flatfish. J. Anim. Ecol. 4, 927–937. doi: 10.1111/1365-2656.12524
Sand, H., Wabakken, P., Zimmermann, B., Johansson, Ö, Pedersen, H. C., and Liberg, O. (2008). Summer kill rates and predation pattern in a wolf–moose system: can we rely on winter estimates? Oecologia 156, 53–64. doi: 10.1007/s00442-008-0969-2
Statistisk Sentralbyrå (2020). Areal og Befolkning, Etter Region, Statistikk Variabel og år. Statistikkbanken. Available online at: https://www.ssb.no/statbank/table/11342/tableViewLayout1/
Sunde, P., Collet, S., Nowak, C., Thomsen, P. F., Hansen, M. M., Schulz, B., et al. (2021). Where have all the young wolves gone? Traffic and cryptic mortality create a wolf population sink in Denmark and northernmost Germany. Conserv. Lett. 14:e12812.
Sunde, P., Stener, S. Ø, and Kvam, T. (1998). Tolerance to humans of resting lynxes Lynx lynx in a hunted population. Wildl. Biol. 4, 177–183. doi: 10.2981/wlb.1998.020
Suraci, J. P., Smith, J. A., Clinchy, M., Zanette, L. Y., and Wilmers, C. C. (2019). Humans, but not their dogs, displace pumas from their kills: an experimental approach. Sci. Rep. 9:12214. doi: 10.1038/s41598-019-48742-9
Swenson, J. E., Dahle, B., and Sandegren, F. (2001). Intraspecific Predation in Scandinavian Brown Bears Older than Cubs-of-the-Year. Ursus 12, 81–91.
Wam, H. K., Eldegard, K., and Hjeljord, O. (2012). From overlooking to concealed: predator avoidance in an apex carnivore. Eur. J. Wildl. Res. 58, 1001–1003. doi: 10.1007/s10344-012-0670-y
Wam, H. K., Eldegard, K., and Hjeljord, O. (2014). Minor habituation to repeated experimental approaches in Scandinavian wolves. Eur. J. Wildl. Res. 60, 839–842. doi: 10.1007/s10344-014-0841-0
Wikenros, C., Jarnemo, A., Frisén, M., Kuijper, D. P. J., and Schmidt, K. (2017). Mesopredator behavioral response to olfactory signals of an apex predator. J. Ethol. 35, 161–168. doi: 10.1007/s10164-016-0504-6
Williams, H. J., Taylor, L. A., Benhamou, S., Bijleveld, A. I., Clay, T. A., Grissac, S., et al. (2020). Optimizing the use of biologgers for movement ecology research. J. Anim. Ecol. 89, 186–206. doi: 10.1111/1365-2656.13094
Ydenberg, R. C., and Dill, L. M. (1986). The economics of fleeing from predators. Adv. Study Behav. 16, 229–249. doi: 10.1016/j.jtbi.2006.07.011
Zedrosser, A., Steyaert, S. M. J. G., Gossow, H., and Swenson, J. E. (2011). Brown bear conservation and the ghost of persecution past. Biol. Conserv. 144, 2163–2170. doi: 10.1016/j.biocon.2011.05.005
Zimmermann, B., Nelson, L., Wabakken, P., Sand, H., and Liberg, O. (2014). Behavioral responses of wolves to roads: scale-dependent ambivalence. Behav. Ecol. 25, 1353–1364. doi: 10.1093/beheco/aru134
Keywords: experimental human disturbances, flight responses, Canis lupus, animal behavior, flight initiation distance
Citation: Versluijs E, Eriksen A, Fuchs B, Wikenros C, Sand H, Wabakken P and Zimmermann B (2022) Wolf Responses to Experimental Human Approaches Using High-Resolution Positioning Data. Front. Ecol. Evol. 10:792916. doi: 10.3389/fevo.2022.792916
Received: 11 October 2021; Accepted: 09 March 2022;
Published: 06 April 2022.
Edited by:
Attila D. Sándor, University of Agricultural Sciences and Veterinary Medicine of Cluj-Napoca, RomaniaReviewed by:
Janne Sundell, University of Helsinki, FinlandFriederike Range, University of Veterinary Medicine Vienna, Austria
Adrian Treves, University of Wisconsin-Madison, United States
Copyright © 2022 Versluijs, Eriksen, Fuchs, Wikenros, Sand, Wabakken and Zimmermann. This is an open-access article distributed under the terms of the Creative Commons Attribution License (CC BY). The use, distribution or reproduction in other forums is permitted, provided the original author(s) and the copyright owner(s) are credited and that the original publication in this journal is cited, in accordance with accepted academic practice. No use, distribution or reproduction is permitted which does not comply with these terms.
*Correspondence: Erik Versluijs, ZXJpay52ZXJzbHVpanNAaW5uLm5v