- 1Departamento de Ecología, Facultad de Ciencias Biológicas, Pontificia Universidad Católica de Chile, Santiago, Chile
- 2Center of Applied Ecology and Sustainability (CAPES), Pontificia Universidad Católica de Chile, Santiago, Chile
- 3Instituto Milenio en Socio-Ecología Costera, Santiago, Chile
- 4Escuela de Agronomía, Pontificia Universidad Católica de Valparaíso, Quillota, Chile
- 5Department of Biology, Ecoinformatics and Biodiversity, Aarhus University, Aarhus, Denmark
- 6Department of Biology, Center for Sustainable Landscapes Under Global Change (SustainScapes), Aarhus University, Aarhus, Denmark
Urbanization effects have been studied all over the world, documenting impact in species richness, abundances and changes in species communities. Birds have been broadly used as study models. In general, urbanization affects birds, reducing species richness, especially in the urban core, and increasing species richness in areas with intermediate levels of disturbance, such as suburbs. Urbanization also changes species assemblages depending on urban characteristics and resources available, creating habitats for different species. Even when more than half of the cities in the world are on the coast, the effects of urbanization on habitat use of terrestrial and marine birds in coastal urban environments has received little attention. We hypothesized that coastal cities would present different bird diversity in modified marine areas and modified inland areas as terrestrial and marine ecosystems coexist. We predict that modified marine areas will have higher species richness than modified inland areas and natural marine areas. For bird assemblages, we expect to find similar species compositions between sites with similar habitat characteristics more than closeness. We compare habitat use of marine and terrestrial avifauna in the human-modified coastal city of Valparaiso, Chile, characterized by a range of urban developments within city boundaries. We specifically compare corrected bird abundance in six different possible habitats for birds, according to distance to the coast, and human influence. Bird counts (50 m fixed radius) were conducted in winter and spring of 2019. Bird species richness and abundances, corrected by the probability of detection, were estimated. Additionally, species composition and occupancy of bird species in those habitats were calculated. Results show that coastal urban cities can provide different habitats for bird species. Modified inland habitats differ from semi-natural inland habitats and from the modified beaches in species richness and species composition. Environmental heterogeneity in coastal cities seems to allow the coexistence of marine and terrestrial bird species, showing differences in species richness and bird assemblages for marine-inland environments and natural- modified habitats. Results highlight the need to consider these factors for urban planning in order to conserve bird diversity in coastal urban areas.
Introduction
Urbanization is continuously growing and, as an example of an extreme anthropogenic landscape transformation, represents a major driver of habitat loss and degradation (Grimm et al., 2008). This phenomena has brought profound changes in the ecological functioning of ecosystems such as the decline of species richness and diversity (Antrop, 2000; Pickett et al., 2011; Seress and Liker, 2015). Urbanization has been studied widely on birds given they are conspicuous species, and considered bioindicators of ecosystem functioning (Burger and Gochfeld, 2004; Rosenberg et al., 2019). Previous evidence indicates that bird communities respond to urbanization. They usually exhibit lower species richness in urban areas compared to more natural ones, with the lowest diversities in urban core areas (McKinney, 2002, 2008). Some levels of urbanization have shown an increase in avian diversity, for example in the suburbs, linked to intermediate levels of disturbance (Blair, 1996; Marzluff, 2001; Chace and Walsh, 2006; Lepczyk et al., 2008).
Bird assemblages are being affected by urbanization. As first described by Blair in 1996, different bird assemblages can be found along an urban gradient. Based on this, a new characterization deals with urbanization and ecological mechanisms. Here, species that rarely occur in developed areas are called urban avoiders, and they may persist in natural areas embedded in urbanized landscapes. Then, the urban utizers can be found making occasional use as non-breeders of urban resources to breeding in developing areas, only because of dispersal from adjacent natural areas. And urban dwellers, which can vary from having viable population in both natural and developed areas and persist in urbanized landscapes independently of natural areas, to being dependent on developed areas for survival (Fischer et al., 2015; González-Lagos and Quesada, 2017). Moreover, there are many studies that consider urban gradients incorporating the surroundings of the cities (e.g., Clergeau et al., 1998; Crooks et al., 2004; Melo et al., 2021). For bird assemblages, evidence shows that local habitat characteristics are more important than surrounding landscapes (Clergeau et al., 2001; Evans et al., 2009). Thus, bird communities in cities are more similar to urban communities nearby than communities in surrounding natural environments (McKinney and Lockwood, 1999; Clergeau et al., 2001; McKinney, 2006).
In South America, considered an area of high biodiversity, the impacts of urbanization have shown contradictory evidence. For example, high levels of urbanization have shown low species richness of birds (Leveau et al., 2017). In fact, some attributes of cities seem to be important in determining the presence of species, such as the land cover. For example, vegetation cover can positively correlate with species richness (Filloy et al., 2019) and specifically, can affect specialist species given vegetation structure in urban forest fragments would support their habitat and feeding (Campos-Silva and Piratelli, 2021). Additionally, three cities (La Paz in Bolivia, Mar del Plata in Argentina, and Osorno in Chile) showed that urbanization does not correlate with bird abundance, but it does with bird species composition (Leveau et al., 2017). Interestingly, a study in central Argentina showed that bird communities were more similar between urban centers than between adjacent semi-natural areas (Filloy et al., 2015; Bellocq et al., 2017). However, it was demonstrated that two kinds of biomes presented different spatial patterns of species richness. In an arid biome, the highly developed areas showed higher bird richness than the natural areas, and in the humid biome, the moderately developed areas showed lower species richness than natural areas (Filloy et al., 2019). Essentially, research has shown that the effects of urbanization on diversity depend on the urban developmental dynamics and the ecology of species within a given region (Chace and Walsh, 2006; Luck and Smallbone, 2010).
Cities in coastal areas have shown faster growth rates, given their attractiveness for human settlement. By 2017, about 40% of the world’s population lives within 100 km of the coast (United Nations [UN], 2017). Furthermore, by 2009, 67% of megacities corresponded to coastal cities (von Glasow et al., 2013). However, coastal urbanization research has been mainly focused on terrestrial aspects (Graells et al., 2021). There is a gap in studies that focus on the coastal ecotone and the influence of urban areas focusing on marine and terrestrial interaction of species in an urbanized area. Therefore it is important to explore what is happening on the coast where urban areas are influenced by terrestrial and marine ecosystems.
This research aims to identify the use of coastal urban habitats by birds as a way to explore the impact of urbanization in coastal cities and the response of terrestrial and marine bird communities. The study evaluated the response of bird communities to the anthropogenic impact in coastal urban environments characterizing the bird assemblage and species origin (marine or terrestrial). We hypothesized that coastal cities would present different bird diversity in modified marine areas and modified inland areas because the first are immersed in a marine-terrestrial ecotone where urban, terrestrial and marine ecosystems coexist. We are also interested in knowing how those species are assembled in those habitats. We predict that modified marine environments (beach and rocky shore) will have higher species richness than modified inland areas, and modified marine environments will present higher species richness than natural marine environments. In relation to bird assemblages, we expect to find similar species compositions between sites with similar habitat characteristics. We specifically identified species in contrasting habitats and described the spatial use of bird species that showed the greatest difference in those contrasts.
Materials and Methods
We conducted bird surveys in a coastal urban environment in the Valparaíso region. We estimated species composition and analyzed occupancy of those species that presented the largest differences between habitats. Greater Valparaíso is the largest metropolitan coastal area in Chile and the country’s second most populated urban area with 935,602 people (Instituto Nacional de Estadística [INE], 2017). It is composed of three coastal cities: Valparaíso, Viña del Mar, and Concón.
In this area, a random stratified design was applied along three defined coastal environments: beach, area in the coastline with at least 80% sand on its surface; rocky shore, area in the coastline with at least 80% rock formations on its surface; and inland areas, between 200 and 1800 meters from the coast and with not a direct view to the sea. In each environment, two extremes of human intervention were selected: modified and natural/semi-natural areas. Modified areas had more than 50% human intervention on the surface (i.e., buildings and paved roads). Natural areas had less than 30% of human intervention on its surface (i.e., buildings or paved roads) and preserved wild vegetation. Because inland environments did not present enough natural areas, semi-natural areas were selected. These areas had more than 50% of vegetation (natural or planted) and are represented mainly by green areas and parks. In essence, six distinct areas were considered as potential habitats for birds: urbanized beach and natural beach, natural rocky shore and modified rocky shore, modified inland (urban center) and semi-natural inland (green area) (Figure 1). Two replicas for each habitat from each of the three coastal cities were selected, with a total of 36 sampling sites.
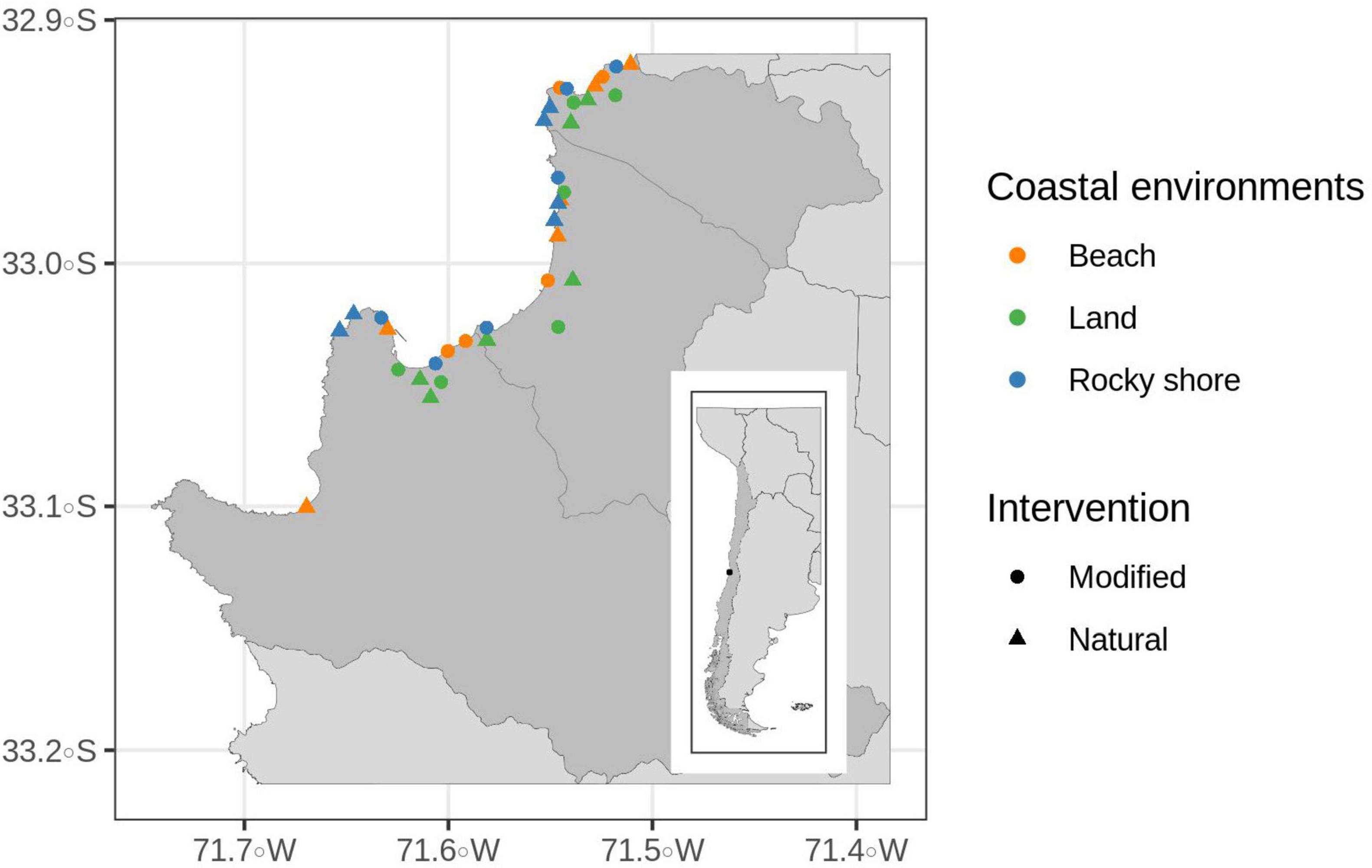
Figure 1. Valparaiso Metropolitan area in Central Chile and the 36 sampling points of this study. Each sample site shows its characterization based on coastal environments (beach, land or rocky shore) and human intervention (modified or natural).
Bird surveys were performed in the southern hemisphere winter and late spring of 2019 to determine resident and migratory species. We decided to sample in late spring instead of summer because of the possible influence of summer tourists on the beach. For both seasons, sampling was performed in each of the 36 sites randomly. Surveys were conducted daily during sunrise and 3 h later, managing to perform between four and six of them, depending on the distance between sites. We used fixed radius point counts with a range of 50 m from the observer. A short count period of 10 min was used to not over-count large, conspicuous species and to deal with the mobility assumption of point count methods (Bibby et al., 2000). All species that were seen and heard were registered.
To deal with differences in the detectability of birds, abundance obtained from the field was corrected using detection covariables (Royle and Nichols, 2003). We accounted for differences in precipitation, wind direction and speed, humidity, and temperature of the sampling point in a specific day and time (see Supplementary Table 1). To do this, we used repeated presence-absence data with the DiversityOcuppancy package for R software (Corcoran et al., 2017). Here, all the species detected in the sampling period were a part of the potential community. If the species was detected at least once during the point count, it was considered to be present (probability of 1 for that day and site). If it was not detected, it was supposed to be absent for that sampling date and site (probability of 0). When a species was observed once or twice in the 3 days sampling, the probability was according to the number of days present related to the 3 days of sampling (1/3 or 0.33 and 2/3 or 0.67, respectively). For all the further abundance analysis, we used abundance corrected by detection probability (MacKenzie and Nichols, 2004; Kendall and White, 2009; Latif et al., 2016).
Data Analysis
Species in Contrasting Habitats
To test what variables are the most important at determining species richness (λ) we made a Poisson GLM model. The general model included as predictor variables: human intervention (natural and modified habitats, I), the season (winter or spring, S), and the environment (beach, rocky shore or inland, E). The model included the interaction of variables (season and environment, intervention and environment) and the response variable was the observed species richness. We used a multimodel inference approach to select the best model (Burnham and Anderson, 2004). In order to do this, first we fitted the general model (Eq. 1), and we calculated the Variance Inflation Factor (VIF) for each variable (Belsley et al., 2005). Using a threshold of 5 (James et al., 2013), we recursively eliminated the variable with the highest VIF until all variables had a value that was lesser than the threshold. Then, we used the MuMIn package with the resulting equation to test all combinations of models from the new general model (Eq. 2) and limit the number of variables to the number of samples divided by 10. After that, we took into account models with a delta Akaike Information Criteria (AICc) of 2 from the model with the lowest AICc. Finally, we made a full model average taking AICc weights as the weight of the model for the final one. Post hoc comparisons between season, human intervention and coastal environments were obtained from estimated marginal means (EMMs) with Tukey adjustment using the “emmeans” package.
Bird communities for each habitat were analyzed using this abundance estimation through ordination methods with the Vegan package in R (Oksanen et al., 2019). Additionally, we determined dissimilarity distances among sample sites and tested significant differences among habitats by analyzing similarities (ANOSIM). To assess species contribution to the difference between habitats we used Bray–Curtis dissimilarities with SIMPER analysis.
Using the models generated by Tamburello et al. (2015) and the body mass reported for the species in Wilman et al. (2014), we estimated the home range of the species selected by SIMPER. Because birds in urban environments had significantly broader environmental tolerance (Bonier et al., 2007), we created land-use proportions on a buffer of 2,200 m around the sampling points. For each sampling point, the percentage of cells belonging to the following environments was calculated: native forest, crops, gravel, shrubs, ocean, grasslands, sand, and paved surfaces. Land cover layers were extracted from Zhao et al. (2016). Thus, we obtained land cover buffer rasters to test environmental variables used by bird assemblages, using PERMANOVA and occupancy of bird species.
Spatial Use by Bird Species
Species selected by SIMPER analysis that showed differences between modified and natural habitats were analyzed separately to determine species occurrence. In order to do this, we used occupancy models with the package DiversityOccupancy in R (Corcoran et al., 2017) with previously prepared environmental variables from land cover layers. For each of the 36 sampling points, a specific previously selected diameter circle raster of the proportion of land cover was used. The percentage of cells belonging to the following environments was calculated: Native forest, Crops, Gravel, Shrubs, Ocean, Grasslands, Sand or Paved surfaces (for a complete description of variables see Supplementary Table 1). To select the best model of occurrence, corrected AICc was used. Models selected were projected to the study area showing the probability of use of habitat for each species in the area.
Results
A total of 56 bird species were found in the sampled six coastal urban habitats. Observed species richness was similar between winter and late spring (45 and 48 species, respectively); however, only 37 species were common in both seasons (see the complete species list in Supplementary Table 2).
Species in Contrasting Habitats
GLM indicated that species richness should be analyzed separately in both seasons, as the season was one of the selected variables under the Multimodel Inference approach (see in Supplementary Table 3). Predicted species richness obtained from GLM showed for both modified and natural areas that inland has the least richness and beach has the highest. Specifically, modified beach areas had significantly more species richness than modified inland areas in both seasons, but not modified rocky shore. When comparing marine environments, both beach and rocky shore did not show differences in species richness between modified and natural areas (Figure 2) (Complete results of post hoc paired analysis of the averaged GLM model can be found in Supplementary Table 4).
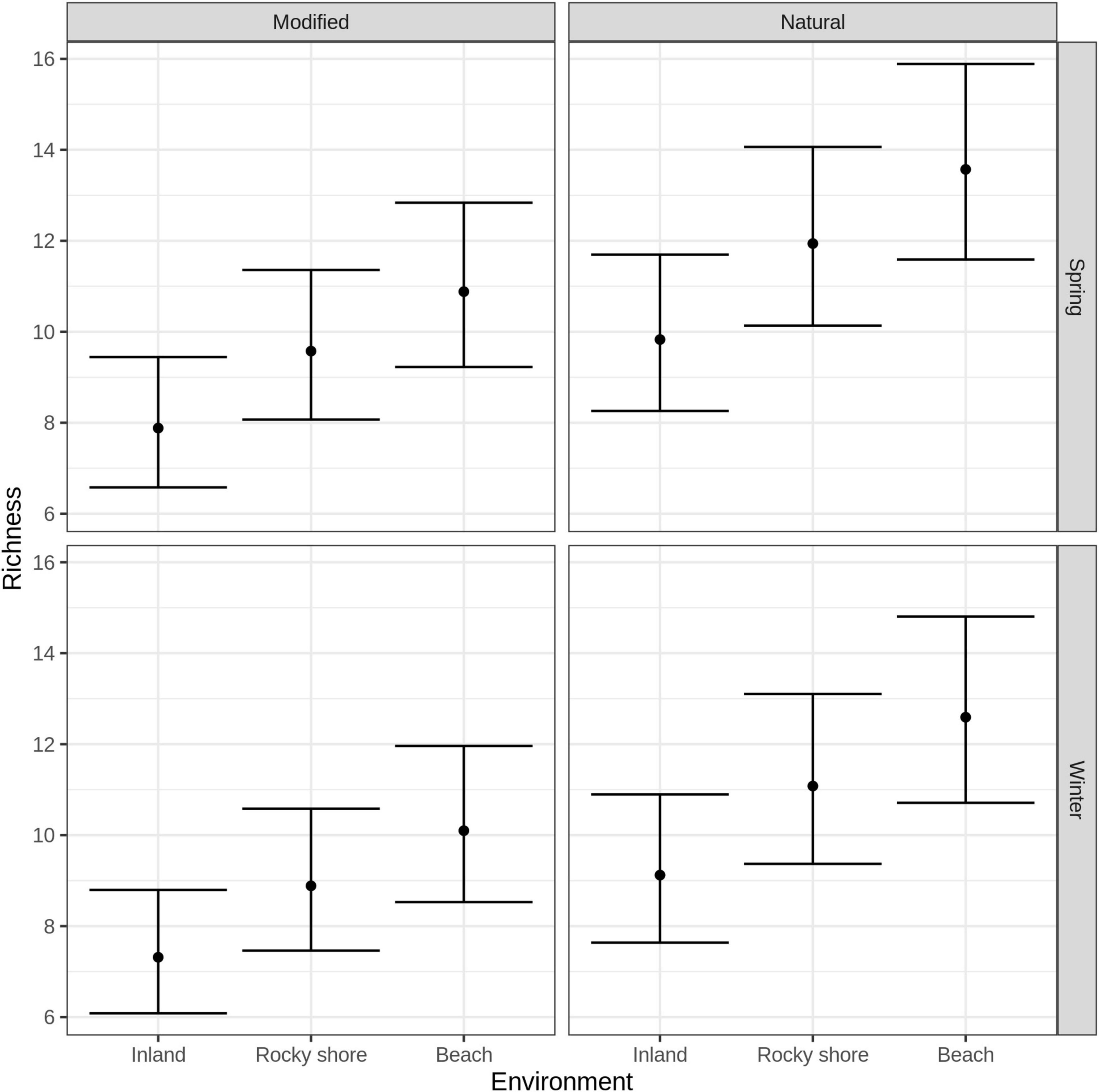
Figure 2. Species richness of birds estimated by GLM models, according to environment (inland, rocky shore and beach), human intervention (modified and natural) and sample season (winter and spring). Points represent the estimate and bars the 95% of CI.
Species assemblages also showed differences between human modification and environments. Figure 3 presents a multidimensional scaling (MDS) of sites of winter and spring seasons separately, according to the estimated species abundances. Both seasons showed that bird assemblages are different between habitats. Notably, distance to inland habitats are greatest. Also, marine modified sites, such as urbanized beaches and modified rocky shores, show more similarity to marine natural sites (natural beach and rocky shore) than to modified land (urban centers). Seasonal ANOSIM analysis shows that assemblages in each environment are different among studied seasons (R = 0.584, P < 0.01 in winter and R = 0.573, P < 0.01 in spring). Dissimilarity rank indicates with a 95% confidence interval, that dissimilarities within each habitat are lower than between them. There is one exception shown by natural beach habitat during winter, here the variability of species in the habitat is as high as we consider all habitats together. All of this seems to show that in most of the habitats there are specific conditions that allow different species compositions for the winter and spring seasons.
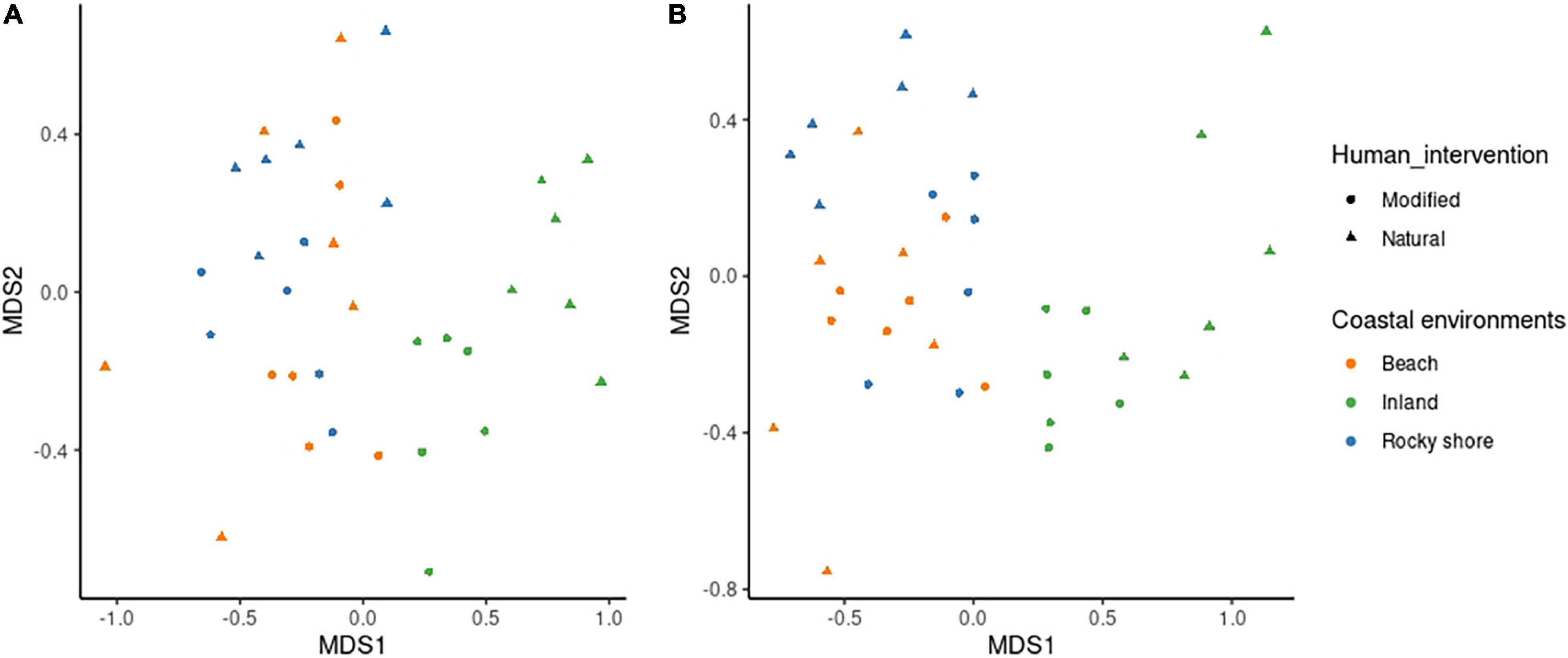
Figure 3. Multidimensional scaling (MDS) of bird species composition for all sites, according to environments and human intervention in panel (A) winter and panel (B) spring.
PERMANOVA indicated species assemblages were determined by the same two environmental variables: altitude and proportion of ocean cover 2,200 m around the sample point. However, two variables are different for each season: during the winter, vegetation cover also determines assemblages and during spring paved surfaces cover in 2,200 m around the sample point are also important.
Both sampled seasons showed that few species contribute to assemblage differences in paired habitat comparisons. According to the SIMPER analysis, in each habitat comparison, two species always contributed to the main differences (Table 1). In winter, six species showed to add to this difference between intervention states of environments. These species were Larus dominicanus, Larosterna inca, Pelecanus thagus, Coragyps atratus, Columba livia, and Sephanoides sephanoides. The cumulative sum of contribution to the difference between habitats went from 19.43 to 26.69%. In this season, Larus dominicanus presented differences between natural and modified beaches in spring. Even when Larus was present in the six studied habitats, in natural beaches its abundance was higher. Larosterna inca was more abundant in modified beaches, where they were seen flying and nesting in stone walls in front of the sea. Pelecanus thagus abundance was higher in modified rocky shore, mostly in places such as coves where there is a great amount of fishery waste. Coragyps atratus were more abundant in the natural rocky shore where they were seen flying above natural vegetation. Columba livia is the only exotic species described by SIMPER analysis, and they were more abundant in modified inland habitats. Lastly, Sephanoides sephanoides are winter visitors and were more abundant in green areas during this season (The complete SIMPER analysis result for winter can be found in Supplementary Table 5).
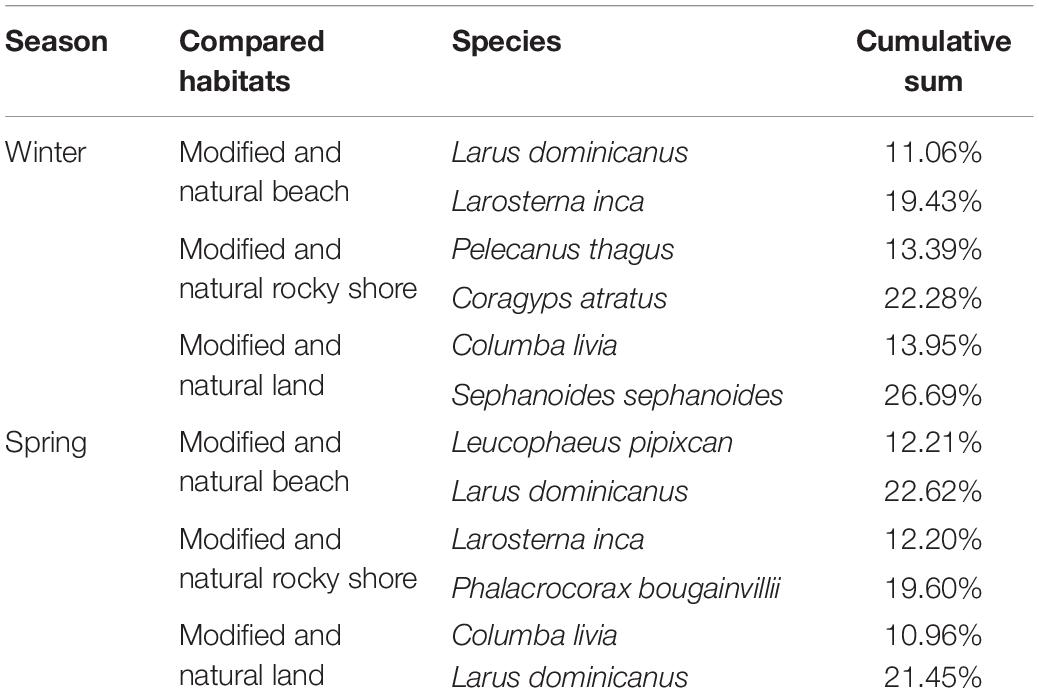
Table 1. Summary of SIMPER analysis results showing habitat compared in both seasons, species that contribute to the differences in this comparison and the cumulative sum of contribution to the difference between habitats of species mentioned.
In spring, five species are the most important at differentiating bird assemblages in different habitats: Leucophaeus pipixcan, Larus dominicanus, Larosterna inca, Phalacrocorax bougainvillii, Columba livia, and Larus dominicanus. Their contribution to the paired comparison in the cumulative sum went from 19.60 to 22.62%. In general, these cumulative sums are similar among paired habitat comparisons. However, species change between winter and spring. In this season, Leucophaeus pipixcan and Larus dominicanus were both more abundant in natural beaches. Larosterna inca was more abundant in the modified rocky shore. Phalacrocorax bougainvillii are spring visitors and they were more abundant in the natural rocky shore. Columba livia was also more abundant in modified inland habitats. And finally, Larus dominicanus showed a difference between modified and semi-natural inland areas, being more abundant in modified habitats in these environments (The complete SIMPER analysis result for spring can be found in Supplementary Table 6).
Spatial Use by Bird Species
Predictions of the occurrence of bird species in the studied area indicate differences in habitat use (Figure 4). Most of the predictions showed higher levels of occupancy on the coast than inland. In winter, two marine species (Pelecanus thagus and Larosterna inca) are present only in marine environments, one terrestrial species (Coragyps atratus) uses part of the coastline and terrestrial habitats, one species is present in the whole study area (Larus dominicanus), and two terrestrial species (Columba livia and Sephanoides sephaniodes) use the entire study area with probabilities over 50% both coastline and inland. In spring, three marine species (Larosterna inca, Leucophaeus pipixcan, and Phalacrocorax bougainvilii) use only the coastline and the other two (Columba livia, terrestrial exotic species, and Larus dominicanus, marine species) are present in the whole study area (Larus with some restrictions on the coast and inland). Specifically, Larus dominicanus presented the maximum probability of occupancy in the whole area in both winter and spring. In this case, there was no variable in the model that determined the occupancy. In the case of Columba livia, during the winter their occurrence is focussed on certain coastal areas, defined by the presence of sand. During the spring, its presence is high in almost the whole study area with exception of some small areas. According to the model selected, the presence of native forest and sand, and the absence of gravel and tree plantations allow this pattern. Larosterna inca shows a similar occurrence in both seasons, however during the spring it expands a little to the inland in some specific parts of the study area. The occurrence in winter is determined by covariables such as low altitude, low proportion of native forest, low proportion of sand, and in spring by high proportion of ocean and a high proportion of gardens on the coast.
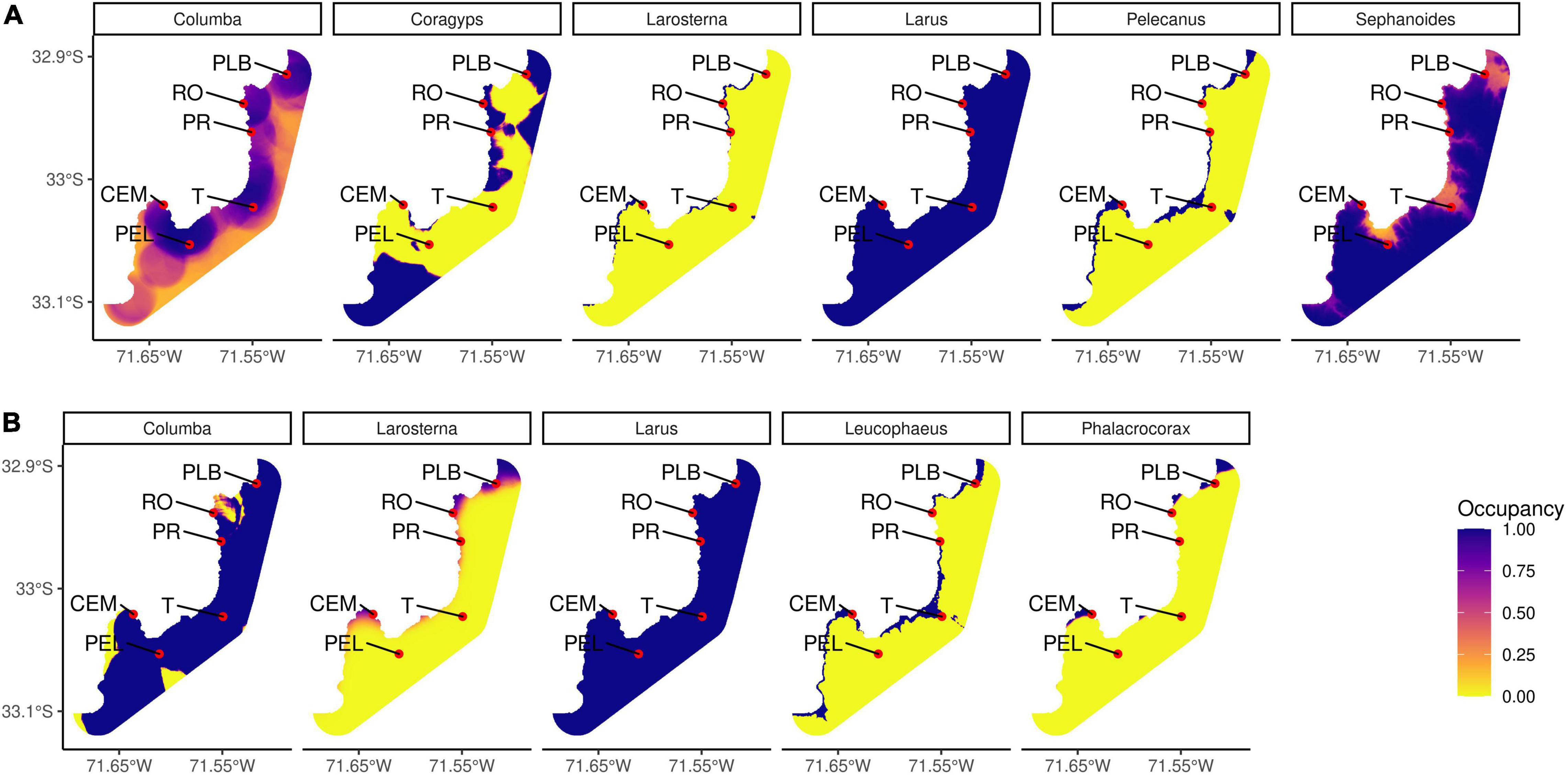
Figure 4. Predictions of occupancy models for those bird species that contributed the most to the differences between paired habitat comparisons during panel (A) winter and panel (B) spring. Some of the sample sites are PLB: La Boca Beach (natural beach), RO: Oceanic Rock (natural rocky shore), PR: Reñaca Beach (modified beach), T: Bus Terminal (modified inland), CEM: El Membrillo Cove (modified rocky shore), PEL: El Litre Park (semi-natural inland).
For the winter, Coragyps atratus has a specific presence in certain areas. Its model is determined by the presence of sand and the absence of paved areas. Pelecanus thagus appear only by the coastline and its model is determined by low altitude, absence of native forest and gravel and needs oceanic surrounding areas (all of this translates to being on the coast). Sephanoides sephaniodes are present on the coast and inland, but they have a marked preference for higher altitude and absence of sand surface. For the spring, both Leucophaeus pipixcan and Phalacrocorax bougainvilii are more selective of marine environments, with Leucophaeus being able to come to the inland to some extent. Leucophaeus pipixcan models indicate preferences of low altitude, absence of gravel and presence of oceanic surrounding and paved areas. Phalacrocorax bougainvilii also needs oceanic surroundings and the absence of gravel but showed a preference for more vegetation present in the area [Details of occupancy models can be found in Supplementary Table 7 (winter species) and Supplementary Table 8 (spring species)].
Discussion
Prior research has documented the effects of urbanization on bird richness (e.g., McKinney, 2002, 2008), abundance (e.g., Marzluff, 2001), and composition (e.g., Blair, 1996). However, evidence has been contradictory during the last decades, mostly from the southern hemisphere. Additionally, these studies have either been focused mostly on urban terrestrial habitats or in specific ecosystems within coastal areas. In this study, we evaluate species richness, abundance, and community assemblages of birds in modified and natural/semi-natural areas in a coastal city, including marine and inland environments. Our results match some of our predictions, indicating modified beach habitats can allow a higher bird species richness than modified inland habitats. However, modified marine areas do not show more species than their natural counterpart. Results highlight variations regarding species composition. Marine environments (beach and rocky shore) seem to be more similar among them compared to modified inland and semi-natural inland habitats for both seasons.
Modified beach areas show more bird richness than modified inland areas (Figure 2), probably because modified beaches are immersed in a marine-terrestrial ecotone. This ecotone provides more resources to species because it mixes terrestrial, oceanic, and atmospheric processes (Ray and Hayden, 1992). At the same time, modified inland areas present a high level of urbanization with high percentages of paved surfaces such as roads and buildings. And even when human intervention has been demonstrated to facilitate the presence of birds (Cereghetti et al., 2019; Carmona et al., 2021), urban areas also can generate ecological traps for bird nesting (Reynolds et al., 2019), increase bird mortality by collisions with moving vehicles (Erritzoe et al., 2003), and increased competition for good quality food and native trees for nesting (Seress and Liker, 2015).
If human intervention have been shown to increase ecosystem variability and the availability of resources for birds (Cereghetti et al., 2019; Carmona et al., 2021), this could potentially be also true for modified beach areas that correspond to an intermediate level of human intervention (Doxa et al., 2012). However, rocky shores could present higher intervention than beaches, and that reflects on non-differences with modified inland areas richness (Figure 2). Modified marine environments did not show higher species richness than natural marine environments as we had predicted. Both observations could represent that human modification on the coast is not an intermediate level of perturbation and present areas with higher intervention.
There are differences in bird assemblages between marine (beach and rocky shore) and inland environments. MDS results show marine (natural and modified) habitats are more similar than those inland (Figure 3). Notably, specific differences between modified and natural habitat species composition could be described with SIMPER results. Table 1 shows the species that add the most to the dissimilarity between those habitats in the beach, rocky shore, and inland environments. In winter there were six species (Larus dominicanus, Larosterna inca, Pelecanus thagus, Coragyps atratus, Columba livia, and Sephanoides sephanoides), and in spring, five (Leucophaeus pipixcan, Larus dominicanus, Larosterna inca, Phalacrocorax bougainvillii, Columba livia, and Larus dominicanus). Considering this, it seems that some species can use modified habitats to get what they need when resources are scarce or available. For example, in winter Larosterna inca was more abundant in modified beaches than natural beaches. We found Larosterna nesting in stone walls in front of the sea built as part of the roads. Similar to habitats that they naturally use in order to reduce predation risk, selecting natural walls with cavities and crevices (Velando and Márquez, 2002). Moreover, this happens in winter probably because there is a low presence of tourists on the beach. During spring, people can affect the foraging behavior, formerly described for birds (Yasué, 2005). Other species can be generalistic and opportunistic, such as Larus dominicanus. They are present in all habitats but more abundant on natural beaches. These differences could be linked to preferences for feeding areas (Kasinsky et al., 2018) or breeding sites (García-Borboroglu and Yorio, 2004).
There are differences in the abundance of those species for modified and natural habitats. This could indicate differences in nesting possibilities, shelter (protection from predators) and food availability. Mediterranean climate seasonality with cold/wet winters and hot/dry summers (Aschmann, 1973) can make a difference in terms of resources present in different habitats. This is demonstrated by PERMANOVA, which indicates that in winter, vegetation cover is an important variable to determine species composition. Vegetation cover also had been positively correlated to species richness (e.g., Filloy et al., 2019), and could be possible given evergreen trees, shrubs and grasses provide food and shelter during this season for some species. As an example of the studied habitats, we found a large number of Sephanoides sephanoides feeding on nectar from the hemiparasitic Tristerix corymbosus (Smith-Ramirez, 1993; Aizen, 2005; Abrahamczyk et al., 2017), mainly in green areas. Urban green areas have been studied in detail, and it is known that they can sustain bird and plant biodiversity, and this could also explain the difference in species richness between modified inland and semi-natural inland areas (Figure 2). Specifically, urban green space management plays an important role (Aronson et al., 2017). For example, native trees for specialist seed feeder birds or keeping leaf litter for foraging birds. In spring, paved surface cover is a variable that determines species composition. Possibly for the human presence and food subsidies for birds (Cereghetti et al., 2019; Carmona et al., 2021).
Coastal urban areas present specific characteristics that allow the presence of marine and terrestrial bird species. The occupancy model analysis shows that most of the marine birds (defined by literature) are using only marine areas. Most terrestrial species get closer to the ocean, specifically in areas with native vegetation such as natural beaches and natural rocky shores (Figure 4). It seems that coastal cities present several variables and closeness to the coast that may generate conditions that allow the subsistence of specific species of birds in different habitats. This is also demonstrated by PERMANOVA results, which indicate that ocean cover is an important variable that determines species composition for the six habitats in both seasons.
There are specific bird assemblages in modified marine habitats (beach and rocky shore). This has been described for beaches in the coast of Australia, where human activities modify habitat selection by birds (Meager et al., 2012). Additionally, modified marine habitats assemblages are more similar to natural marine assemblages than modified inland habitat, probably because of the effect of the ocean. Contrary to what has been described, where species present in cities are more similar among them and less with those in natural areas surrounding them (Clergeau et al., 2001), these areas can possibly keep certain environmental heterogeneity that allows new species assemblages where marine and terrestrial bird species can coexist. Additionally, there is a low rate of invasiveness, contrary to what has been found in other urban areas (González-Lagos et al., 2021). Our study found a few non-native species, where only Columba livia was selected in our analysis. The presence of exotic species tends to create more homogeneous environments (McKinney and Lockwood, 1999; Bilyaminu et al., 2020). Furthermore, coastal urban areas, being a land-sea ecotone, are environmentally more diverse (Ray and Hayden, 1992), and they can provide more resources to native species.
Human intervention in coastal urban areas such as modified beaches and rocky shores show nuances in the effects over species richness, abundance and species composition. Here, modified beaches show higher species richness than modified inland areas. However, natural and modified marine areas did not show differences in the number of species. Additionally, bird species assemblages can be particular depending on the combination of environmental variables presented. Results suggest that modified marine environments are more similar to their natural counterparts, whereas modified inland areas are very different from semi-natural inland areas (green spaces). One consequence of this is that green areas might have to be placed more strategically since they are potentially important to maintain the composition of species.
The study of coastal urban areas makes an excellent opportunity to understand their complexity, considering the land-sea ecotone where they are located and how bird communities respond to habitat interventions. This study highlights the importance of species diversity in marine modified habitats. Under this scenario, efforts directed toward conserving coastal areas should focus on both modified and natural habitats. Future research should focus on evaluating marine urbanization and its effects on bird communities, given sea construction creates a new habitat modification.
Data Availability Statement
The datasets presented in this study can be found in online repositories. The names of the repository/repositories and accession number(s) can be found below: https://github.com/GiorgiaGraells/MonitoreoVisualGit.
Ethics Statement
Ethical review and approval was not required for the animal study because research only included visual monitoring of birds.
Author Contributions
GG, SG, and JLC-D contributed to the design of the study. GG and DC made the bird surveys and performed the statistical analysis. GG wrote the first draft of the manuscript. DC, JLC-D, and SG wrote sections of the manuscript. All authors contributed to manuscript revision, read, and approved the submitted version.
Funding
This work was supported by the National Agency for Research and Development (ANID) Scholarship Program, National Graduate Scholarship (21171829, 2017), ANID—Millennium Science Initiative Program (Code ICN2019_015), and Center of Applied Ecology and Sustainability (CAPES) (ANID PIA/BASAL FB0002).
Conflict of Interest
The authors declare that the research was conducted in the absence of any commercial or financial relationships that could be construed as a potential conflict of interest.
Publisher’s Note
All claims expressed in this article are solely those of the authors and do not necessarily represent those of their affiliated organizations, or those of the publisher, the editors and the reviewers. Any product that may be evaluated in this article, or claim that may be made by its manufacturer, is not guaranteed or endorsed by the publisher.
Acknowledgments
We appreciate their assistance in the field to Francisca Cuevas, Gabriel Brito, Fiorella Gonzáles, and Daniela Moraga. We are also grateful to Pilar Veas, Port Captain of the Chilean Navy and Sebastián Cordero for providing us with access to carry out bird monitoring.
Supplementary Material
The Supplementary Material for this article can be found online at: https://www.frontiersin.org/articles/10.3389/fevo.2022.807280/full#supplementary-material
References
Abrahamczyk, S., Poretschkin, C., and Renner, S. S. (2017). Evolutionary flexibility in five hummingbird/plant mutualistic systems: testing temporal and geographic matching. J. Biogeogr. 44, 1847–1855. doi: 10.1111/jbi.12962
Aizen, M. (2005). Breeding system of Tristerix corymbosus (Loranthaceae), a winter-flowering mistletoe from the Southern Andes. Aust. J. Bot. 53, 357–361. doi: 10.1071/BT04088
Antrop, M. (2000). Changing patterns in the urbanized countryside of Western Europe. Landsc. Ecol. 15, 257–270.
Aronson, M., Lepczyk, C., Evans, K., Goddard, M., Lerman, S., MacIvor, J. S., et al. (2017). Biodiversity in the city: key challenges for urban green space management. Front. Ecol. Environ. 15, 189–196. doi: 10.1002/fee.1480
Aschmann, H. (1973). “Distribution and peculiarity of mediterranean ecosystems,” in Mediterranean Type Ecosystems: Origin and Structure. Ecological Studies, eds F. di Castri and H. A. Mooney (Berlin: Springer), 11–19. doi: 10.1007/978-3-642-65520-3_2
Bellocq, I., Leveau, L. M., and Filloy, J. (2017). “Urbanization and bird communities: spatial and temporal patterns emerging from southern South America,” in In Ecology and Conservation of Birds in Urban Environments, eds E. Murgui and M. Hedblom (Cham: Springer), 35–54. doi: 10.1007/978-3-319-43314-1_3
Belsley, D. A., Kuh, E., and Welsch, R. E. (2005). Regression Diagnostics: Identifying Influential Data and Sources of Collinearity, Vol. 571. Hoboken: John Wiley & Sons.
Bibby, C. J., Burgess, N. D., Hillis, D. M., Hill, D. A., and Mustoe, S. (2000). Bird Census Techniques. Amsterdam: Elsevier.
Bilyaminu, H., Abdulrashid, I., Wada, A. F., and Gambo, M. S. (2020). Biotic homogenization and its potential drivers: a review abstract - science range publications. Int. Res. J. Biol. Sci. 2, 1–11.
Blair, R. B. (1996). Land use and avian species diversity along an urban gradient. Ecol. Appl. 6, 506–519.
Bonier, F., Martin, P. R., and Wingfield, J. C. (2007). Urban birds have broader environmental tolerance. Biol. Lett. 3, 670–673. doi: 10.1098/rsbl.2007.0349
Burger, J., and Gochfeld, M. (2004). Marine birds as sentinels of environmental pollution. EcoHealth 1, 263–274. doi: 10.1007/s10393-004-0096-4
Burnham, K. P., and Anderson, D. R. (2004). Multimodel inference: understanding AIC and BIC in model selection. Sociol. Methods Res. 33, 261–304. doi: 10.1177/0049124104268644
Campos-Silva, L. A., and Piratelli, A. J. (2021). Vegetation structure drives taxonomic diversity and functional traits of birds in urban private native forest fragments. Urban Ecosyst. 24, 375–390.
Carmona, M., Aymí, R., and Navarro, J. (2021). Importance of predictable anthropogenic food subsidies for an opportunistic gull inhabiting urban ecosystems. Eur. J. Wildl. Res. 67:9. doi: 10.1007/s10344-020-01446-2
Cereghetti, E., Scherler, P., Fattebert, J., and Grüebler, M. U. (2019). Quantification of anthropogenic food subsidies to an avian facultative scavenger in urban and rural habitats. Landsc. Urban Plan. 190:103606. doi: 10.1016/j.landurbplan.2019.103606
Chace, J. F., and Walsh, J. J. (2006). Urban effects on native avifauna: a review. Landsc. Urban Plann. 74, 46–69.
Clergeau, P., Jokimäki, J., and Savard, J.-P. L. (2001). Are urban bird communities influenced by the bird diversity of adjacent landscapes? J. Appl. Ecol. 38, 1122–1134.
Clergeau, P., Savard, J. P. L., Mennechez, G., and Falardeau, G. (1998). Bird abundance and diversity along an urban-rural gradient: a comparative study between two cities on different continents. Condor 100, 413–425. doi: 10.2307/1369707
Corcoran, D., Kesler, D., Webb, L., and Graells, G. (2017). DiversityOccupancy: Building Diversity Models from Multiple Species Occupancy Models. Available online at: https://CRAN.R-project.org/package=DiversityOccupancy (accessed March 2021).
Crooks, K. R., Suarez, A. V., and Bolger, D. T. (2004). Avian assemblages along a gradient of urbanization in a highly fragmented landscape. Biol. Conserv. 115, 451–462. doi: 10.1016/s0006-3207(03)00162-9
Doxa, A., Paracchini, M. L., Pointereau, P., Devictor, V., and Jiguet, F. (2012). Preventing biotic homogenization of farmland bird communities: the role of High Nature Value farmland. Agric. Ecosyst. Environ. 148, 83–88. doi: 10.1016/j.agee.2011.11.020
Erritzoe, J., Mazgajski, T. D., and Rejt, Ł (2003). Bird casualties on European roads—a review. Acta Ornithol. 38, 77–93. doi: 10.3161/068.038.0204
Evans, K. L., Newson, S. E., and Gaston, K. J. (2009). Habitat influences on urban avian assemblages. Ibis 151, 19–39.
Filloy, J., Grosso, S., and Bellocq, M. I. (2015). Urbanization altered latitudinal patterns of bird diversity-environment relationships in the southern Neotropics. Urban Ecosyst. 18, 777–791.
Filloy, J., Zurita, G. A., and Bellocq, M. I. (2019). Bird diversity in urban ecosystems: the role of the biome and land use along urbanization gradients. Ecosystems 22, 213–227. doi: 10.1007/s10021-018-0264-y
Fischer, J. D., Schneider, S. C., Ahlers, A. A., and Miller, J. R. (2015). Categorizing wildlife responses to urbanization and conservation implications of terminology. Conserv. Biol. 29, 1246–1248. doi: 10.1111/cobi.12451
García-Borboroglu, P., and Yorio, P. (2004). Effects of microhabitat preferences on kelp gull Larus dominicanus breeding performance. J. Avian Biol. 35, 162–169. doi: 10.1111/j.0908-8857.2004.03149.x
González-Lagos, C., and Quesada, J. (2017). “Stay or leave? Avian behavioral responses to urbanization in Latin America,” in Avian Ecology in Latin American Cityscapes, eds I. MacGregor-Fors and J. F. Escobar-Ibáñez (Cham: Springer), 99–123. doi: 10.1007/978-3-319-63475-3_6
González-Lagos, C., Cardador, L., and Sol, D. (2021). Invasion success and tolerance to urbanization in birds. Ecography 44, 1642–1652.
Graells, G., Nakamura, N., Celis-Diez, J. L., Lagos, N. A., Marquet, P. A., Pliscoff, P., et al. (2021). A Review on coastal urban ecology: research gaps, challenges, and needs. Front. Mar. Sci. 8:561. doi: 10.3389/fmars.2021.617897
Grimm, N. B., Faeth, S. H., Golubiewski, N. E., Redman, C. L., Wu, J., Bai, X., et al. (2008). Global change and the ecology of cities. Science 319, 756–760. doi: 10.1126/science.1150195
Instituto Nacional de Estadística [INE] (2017). Censo Chile año 2017. Available online at: http://resultadoscenso2017cl (accessed November 9, 2020).
James, G., Witten, D., Hastie, T., and Tibshirani, R. (2013). An Introduction to Statistical Learning. New York: springer.
Kasinsky, T., Suárez, N., Marinao, C., and Yorio, P. (2018). Kelp Gull (Larus dominicanus) use of alternative feeding habitats at the bahía san blas protected area, Argentina. Waterbirds 41, 285–294. doi: 10.1675/063.041.0308
Kendall, W. L., and White, G. C. (2009). A cautionary note on substituting spatial subunits for repeated temporal sampling in studies of site occupancy. J. Appl. Ecol. 46, 1182–1188.
Latif, Q. S., Ellis, M. M., and Amundson, C. L. (2016). A broader definition of occupancy: comment on Hayes and Monfils. J. Wildl. Manage. 80, 192–194.
Lepczyk, C. A., Flather, C. H., Radeloff, V. C., Pidgeon, A. M., Hammer, R. B., and Liu, J. (2008). Human impacts on regional avian diversity and abundance. Conserv. Biol. 22, 405–416. doi: 10.1111/j.1523-1739.2008.00881.x
Leveau, L. M., Leveau, C. M., Villegas, M., Cursach, J. A., and Suazo, C. G. (2017). Bird communities along urbanization gradients: a comparative analysis among three Neotropical cities. Ornitol. Neotrop. 28, 77–87.
Luck, G. W., and Smallbone, L. T. (2010). “Species diversity and urbanisation: patterns, drivers and implications,” in Urban Ecology, ed. K. J. Smallbone (Cambridge: Cambridge University Press), 88–119. doi: 10.1017/cbo9780511778483.006
MacKenzie, D. I., and Nichols, J. D. (2004). Occupancy as a surrogate for abundance estimation. Anim. Biodivers. Conserv. 27, 461–467. doi: 10.1111/mec.15042
Marzluff, J. M. (2001). “Worldwide urbanization and its effects on birds,” in Avian Ecology and Conservation in an Urbanizing World, eds J. M. Marzluff, R. Bowman, and R. Donnelly (Boston: Springer), 19–47.
McKinney, M. L. (2002). Urbanization, Biodiversity, and Conservation: the impacts of urbanization on native species are poorly studied, but educating a highly urbanized human population about these impacts can greatly improve species conservation in all ecosystems. BioScience 52, 883–890.
McKinney, M. L. (2006). Urbanization as a major cause of biotic homogenization. Biol. Conserv. 127, 247–260.
McKinney, M. L. (2008). Effects of urbanization on species richness: a review of plants and animals. Urban Ecosyst. 11, 161–176. doi: 10.1016/j.envint.2015.09.013
McKinney, M. L., and Lockwood, J. L. (1999). Biotic homogenization: a few winners replacing many losers in the next mass extinction. Trends Ecol. Evol. 14, 450–453. doi: 10.1016/s0169-5347(99)01679-1
Meager, J. J., Schlacher, T. A., and Nielsen, T. (2012). Humans alter habitat selection of birds on ocean-exposed sandy beaches. Divers. Distrib. 18, 294–306.
Melo, M. A., Sanches, P. M., Silva Filho, D. F., and Piratelli, A. J. (2021). Influence of habitat type and distance from source area on bird taxonomic and functional diversity in a Neotropical megacity. Urban Ecosyst. 1–16. doi: 10.1007/s11252-021-01169-5
Oksanen, J., Blanchet, F. G., Friendly, M., Kindt, R., Legendre, P., McGlinn, D., et al. (2019). vegan: Community Ecology Package. Available online at: https://CRAN.R-project.org/package=vegan (accessed March 2021).
Pickett, S. T., Cadenasso, M. L., Grove, J. M., Boone, C. G., Groffman, P. M., Irwin, E., et al. (2011). Urban ecological systems: scientific foundations and a decade of progress. J. Environ. Manage. 92, 331–362. doi: 10.1016/j.jenvman.2010.08.022
Ray, G. C., and Hayden, B. P. (1992). “Coastal zone ecotones,” in Landscape Boundaries: Consequences for Biotic Diversity and Ecological Flows, eds A. J. Hansen and F. di Castri (New York, NY: Springer), 403–420. doi: 10.1007/978-1-4612-2804-2_21
Reynolds, S. J., Ibáñez Álamo, J. D., Sumasgutner, P., and Mainwaring, M. C. (2019). Urbanisation and nest building in birds: a review of threats and opportunities. J. Ornithol. 160, 841–860.
Rosenberg, K. V., Dokter, A. M., Blancher, P. J., Sauer, J. R., Smith, A. C., Smith, P. A., et al. (2019). Decline of the North American avifauna. Science 366, 120–124. doi: 10.1126/science.aaw1313
Royle, J. A., and Nichols, J. D. (2003). Estimating abundance from repeated presence–absence data or point counts. Ecology 84, 777–790.
Seress, G., and Liker, A. (2015). Habitat urbanization and its effects on birds. Acta Zool. Acad. Sci. Hung. 61, 373–408. doi: 10.17109/AZH.61.4.373.2015
Smith-Ramirez, C. (1993). Los picaflores y su recurso floral en el bosque templado de la isla de Chiloé. Chile. Rev. Chil. Hist. Nat. 66, 65–73.
Tamburello, N., Côté, I. M., and Dulvy, N. K. (2015). Energy and the scaling of animal space use. Am. Nat. 186, 196–211. doi: 10.1086/682070
United Nations [UN] (2017). Factsheet: People and Oceans. Available online at: https://oceanconference.un.org/documents (accessed June 2021).
Velando, A., and Márquez, J. C. (2002). Predation risk and nest-site selection in the Inca tern. Can. J. Zool. 80, 1117–1123. doi: 10.1139/z02-091
von Glasow, R., Jickells, T. D., Baklanov, A., Carmichael, G. R., Church, T. M., Gallardo, L., et al. (2013). Megacities and large urban agglomerations in the coastal zone: interactions between atmosphere, land, and marine ecosystems. Ambio 42, 13–28. doi: 10.1007/s13280-012-0343-9
Wilman, H., Belmaker, J., Simpson, J., de la Rosa, C., Rivadeneira, M. M., and Jetz, W. (2014). EltonTraits 1.0: species-level foraging attributes of the world’s birds and mammals: ecological Archives E095-178. Ecology 95:2027.
Yasué, M. (2005). The effects of human presence, flock size and prey density on shorebird foraging rates. J. Ethol. 23, 199–204. doi: 10.1007/s10164-005-0152-8
Keywords: coastal urban biodiversity, coastal urbanization, coastal birds, human-modified habitats, urban birds
Citation: Graells G, Celis-Diez JL, Corcoran D and Gelcich S (2022) Bird Communities in Coastal Areas. Effects of Anthropogenic Influences and Distance From the Coast. Front. Ecol. Evol. 10:807280. doi: 10.3389/fevo.2022.807280
Received: 02 November 2021; Accepted: 14 February 2022;
Published: 08 March 2022.
Edited by:
Lucas Leveau, Consejo Nacional de Investigaciones Científicas y Técnicas (CONICET), ArgentinaReviewed by:
Viviana Fernandez, National University of San Juan, ArgentinaAugusto Joao Piratelli, Federal University of São Carlos, Brazil
Copyright © 2022 Graells, Celis-Diez, Corcoran and Gelcich. This is an open-access article distributed under the terms of the Creative Commons Attribution License (CC BY). The use, distribution or reproduction in other forums is permitted, provided the original author(s) and the copyright owner(s) are credited and that the original publication in this journal is cited, in accordance with accepted academic practice. No use, distribution or reproduction is permitted which does not comply with these terms.
*Correspondence: Giorgia Graells, Z3lncmFlbGxAdWMuY2w=