- 1College of Science and Engineering, James Cook University, Townsville, QLD, Australia
- 2School of Environmental and Rural Science, University of New England, Armidale, NSW, Australia
Both natural and artificial light at night can strongly influence animal behavior. Nocturnal animals often alter activity dependent on lunar light levels, to increase prey capture, minimize detection by predators, or both. Trade-offs among these ecological effects are likely to have a strong influence on behavior and fitness. Here, we examined the influence of light at night on nocturnal geckos that are both predators and prey, and use both natural and anthropogenic habitats. We tested the influence of illumination on the relative abundance and behavioral activity of native geckos in natural woodlands and under laboratory conditions. We hypothesized that Australian native house geckos (Gehyra dubia) would avoid activity on nights with high moon brightness, to minimize exposure to predators, consistent with the predation risk hypothesis. Counter to our prediction, we found a positive relationship between house gecko activity and moon brightness, i.e., house geckos were more active on bright nights. This behavior may allow house geckos to better see their prey while also increasing the visibility of approaching predators. In the laboratory, house geckos had shorter latency times to emerge from a shelter under low light conditions compared to darkness equivalent to a new moon, a trend consistent with higher activity under brighter conditions in the field. Light at night, from both natural and artificial sources, clearly influences the behavior and activity of geckos, but perhaps not in the ways we expect. Reducing the risk of attack from predators in darkness, and increasing prey capture success using vision, may increase the benefits of activity in lit conditions, compared to total darkness.
Introduction
Wildlife have adapted to cycles in environmental conditions by adjusting behavior. For example, animals can be grouped into at least four major activity categories based on diurnal changes in light levels: diurnal, crepuscular, cathemeral, or nocturnal (i.e., active in the day, at dawn and dusk, both day and night, or at night, respectively). Differences in activity among these groups is thought to increase fitness by reducing competition and predation risk (Kronfeld-Schor and Dayan, 1999, 2003; Kronfeld-Schor et al., 2017). Although we generally categorize 24-h periods into two major time periods, light (day) and dark (night), there are substantial variations in light levels within both these periods. Light levels are influenced primarily by weather, especially cloud cover, but also by planetary movements, such as moon phases and solar eclipses. Lunar illumination produces only a fraction of the light produced by the sun during the day, i.e., the sun is approx. 400,000 times brighter than a full moon (Williams, 2004, 2010), but the moon provides enough light for nocturnal species with evolutionarily adapted eyesight to function, and, when at its brightest, even diurnal species can see quite well in lunar light (Alonso et al., 2021). In addition, light levels at night are often increased by anthropogenic sources of artificial light (Kyba et al., 2011; Kyba and Hölker, 2013).
Even in the most remote parts of the world, far from human settlements, nocturnal landscapes are illuminated by the moon. The natural cycles of lunar illumination drive changes in species’ behavior (Jetz et al., 2003; Prugh and Golden, 2014). For example, many visually oriented predator species forage on bright nights (Lang et al., 2006; Lillywhite and Brischoux, 2012; Navarro-Castilla and Barja, 2014; Prugh and Golden, 2014) while many prey species avoid bright nights to minimize detection by predators (Kramer and Birney, 2001; Kotler et al., 2010; Weaver, 2011). Varying light levels create a complicated series of costs and benefits for small predators, such that they must balance the risks and rewards of using moonlight to detect both their prey and their predators (Daly et al., 1992; Bouskila, 1995; Penteriani et al., 2013). In addition, factors other than activity reduction can be important. For example, prey may decrease predation risk by changing their habitat selection to increase shelter use and minimize detection from predators, while minimizing food acquisition under bright lunar conditions (Skutelsky, 1996; Upham and Hafner, 2013; Prugh and Golden, 2014). Thus, nocturnal predators and prey, although adapted to low light conditions, could benefit from lunar illumination compared to complete darkness.
Prugh and Golden (2014) developed three theories to describe the response of animals to moonlight, and associated trade-offs. The first is the predation risk hypothesis, which posits that if the dominant effect of moonlight is to increase predation risk, we expect prey animals to reduce their activity to minimize this effect. Second, the habitat-mediated predation risk hypothesis, which suggests prey may offset increased predation risk under bright moonlight conditions by changing their habitat use, to include microhabitats with more shelter, thereby avoiding detection by predators. And finally, the visual acuity hypothesis states that moonlight should aid in foraging success of prey, and simultaneously aid in predator detection. This theory is relevant only to visually oriented species, such that groups that forage predominantly by other senses, e.g., smell or echolocation, should be unaffected by changes in moonlight. A challenge of testing the two first theories outlined above is placing the model group into the appropriate trophic category. Mesopredators are both predators of lower trophic groups, and prey to those in higher trophic groups. Therefore, while a mesopredator may be more active on bright nights to better detect their prey, they also risk higher visibility to their predators. Exploring their responses to increased light at night should reveal the primary risks driving their behavioral responses.
Geckos are mesopredators: both predators of arthropods (Nordberg et al., 2018b) and prey for birds and snakes (Nordberg and Schwarzkopf, 2019b), so they must balance complex cost-benefit trade-offs when active at night. Therefore, nocturnal geckos are an interesting model system in which to investigate the effects of lunar and artificial light on behavioral activity. Here we examined the influence of moon illumination on an abundant nocturnal gecko’s activity and behavior. We combined field surveys and a laboratory experiment to test two hypotheses described above (Prugh and Golden, 2014), examining if illumination (i.e., moon brightness) influenced the activity and behavior of geckos. First, we tested the predation risk hypothesis, by quantifying gecko and nocturnal bird detections on nights with different levels of moon illumination. We predicted (i) that geckos would be less abundant (detectable) on bright nights, to reduce detection by their predators, and (ii) that predators would be more abundant on bright nights. We also examined this hypothesis in the laboratory, by examining gecko willingness to emerge from shelter under dark, versus low light, conditions. We predicted geckos would be less likely to emerge from shelter when it was lighter, consistent with our prediction they would be less active in the field when it was lighter. Second, we tested the habitat-mediated predation risk hypothesis by specifically examining the complexity of the habitats used by geckos on nights with varying moon illumination. We predicted that geckos would alter their microhabitat use to favor trees with more shelter in response to increased predation risk under bright lunar conditions.
Materials and Methods
Study Species and Field Site
In northeastern Australia, house geckos (Gehyra dubia) are one of the most abundant nocturnal lizards (Neilly et al., 2018; Nordberg et al., 2018b; Nordberg and Schwarzkopf, 2019c). They occupy a variety of habitats, including bushland and urban environments (Zozaya et al., 2015; Nordberg and Schwarzkopf, 2019c). Given their small size (4.04 ± 0.07 g; Riedel et al., 2020) and high abundance, they are prey items for a variety of predators including birds, snakes, and even large invertebrates (Nordberg et al., 2018a; Nordberg and Schwarzkopf, 2019b). Field surveys were conducted within the Wambiana Grazing Trial (WGT) on Wambiana Station, a commercial cattle property 80 km southwest of Charters Towers, QLD, Australia (Figure 1). The WGT is composed of open savanna woodlands, dominated by Reid River box (Eucalyptus brownii) and Silver-leaf ironbark (E. melanofloia). For detailed site descriptions, see Neilly et al. (2017) and O’Reagain et al. (2011).
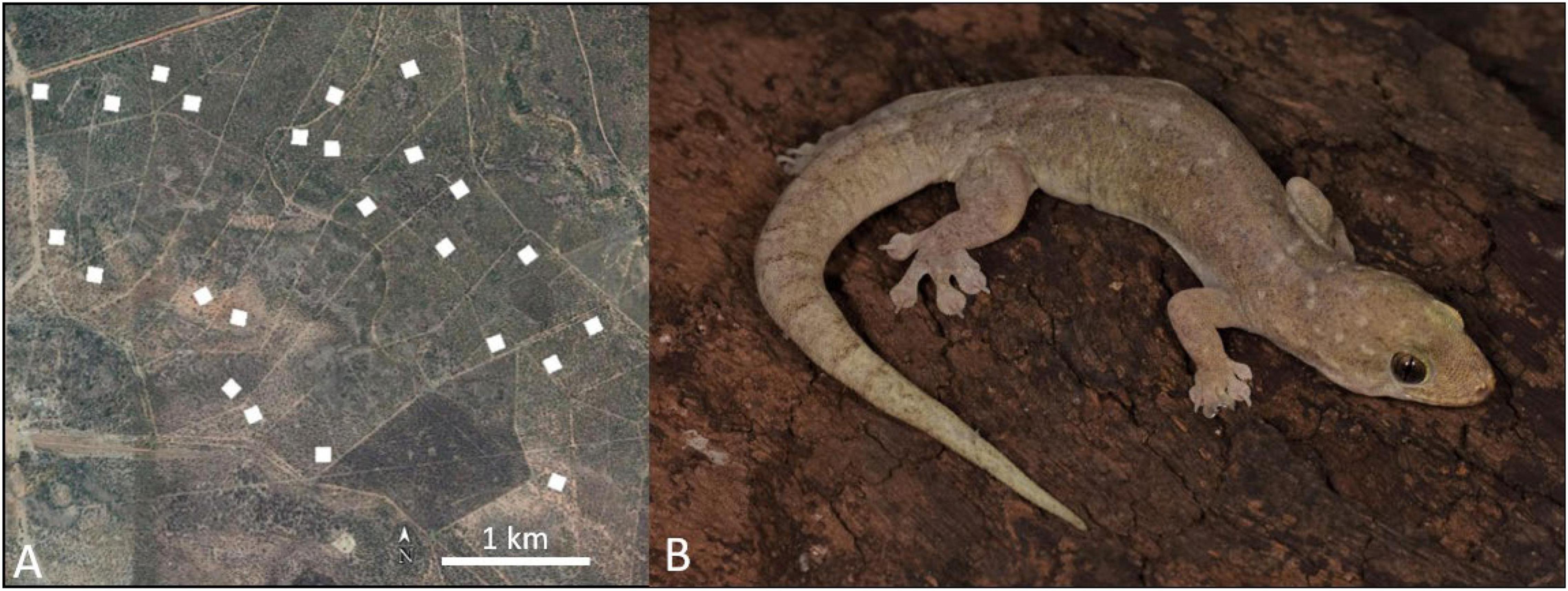
Figure 1. Field studies were conducted on the Wambiana Grazing Trail, a commercial cattle property located near Charters Towers, QLD, Australia. Twenty-four sites (white squares; 1 ha. each) were used to sample for geckos and predators (A). We used the Australian native house gecko, Gehyra dubia, as our study species. Gehyra dubia is abundant across eastern Australia and inhabits a wide range of habitats, including natural and anthropogenic environments (B). Photograph by R. Pillai, 2022.
Field Surveys
Predation risk hypothesis – Nocturnal geckos and their potential predators were surveyed using timed-constrained spotlight surveys to assess their relative abundances. Spotlighting at night is an ideal survey method because nocturnal animals can easily be observed from great distances using their eyeshine (light from a headtorch being reflected back off the animal’s tapetum lucidum). We used relative abundance as a proxy for activity, given that animals in shelters (e.g., in tree hollows, under bark, or other refugia) are not detectable during spotlight surveys, therefore all animals we detected were assumed to be active (e.g., exposed while moving or foraging). At each site (n = 24), two observers searched a 1 ha. site for geckos and their predators (predaceous birds, mammals and reptiles) on trees, logs, shrubs, and the ground. Surveys were conducted nightly for 1 week each in July and September 2014, August 2015, and May 2016.
Habitat-mediated predation risk hypothesis – For each gecko observation in the above described data, we assessed their microhabitat (tree bark) and categorised the structural complexity of the bark into one of three “flakiness” categories (Nordberg and Schwarzkopf, 2019c). Tree bark with a low flakiness score (bark index = 1) had tight, braided fissures, and few places for geckos to hide. Tree bark with high flakiness scores (bark index = 3) had loose and peeling tree bark and abundant shelter area (Figure 2A). In addition, we used a measure of tree branching complexity (i.e., the number of branching nodes coming off the main trunk) as a measure of structural complexity of each tree (Figure 2B). We followed the assumption that a more complex branching tree would provide more shelter for geckos while they were active, and more obstacles for predators, especially birds.
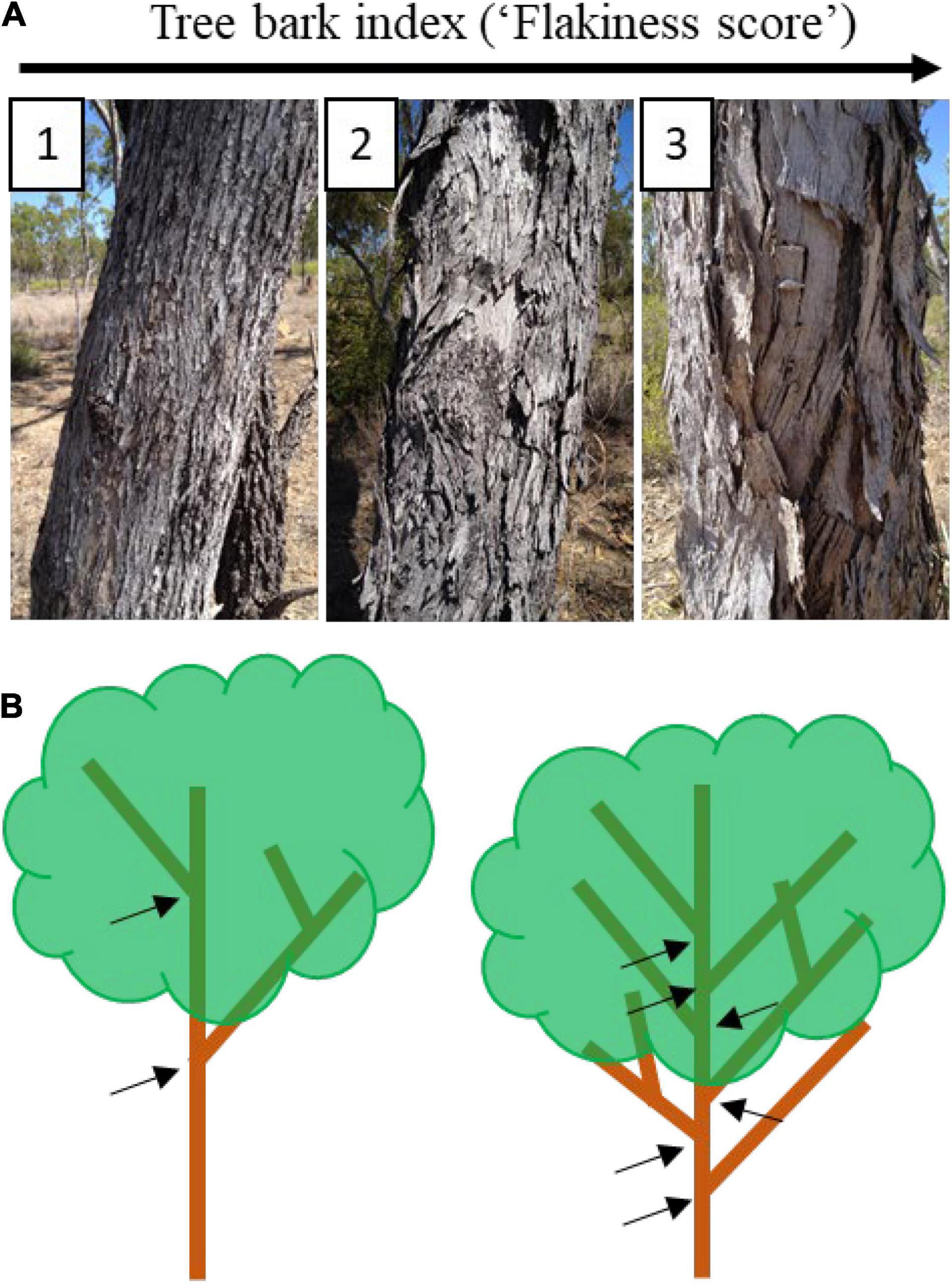
Figure 2. Microhabitat complexity measures, with tree bark index (A: “flakiness score”) ranging from 1 (not flaky, no peeling bark, tight braided fissures and very few sheltering options for geckos) to 3 (very loose and flaky or peeling tree bark with multiple areas for refugia; tree branch complexity (B), with only two major branches coming from the main trunk (black arrows; left) compared to a more complex tree with more branching complexity with six branches coming off the main trunk (right).
Environmental conditions were recorded during each survey, including moon phase and illumination, collated from www.timeanddate.com. We used an adjusted measure of moon illumination (herein referred to as “moon illumination”) because the moon was not always visible above the horizon during surveys (when the moonrise occurred after the spotlight survey period). We corrected for this by using an illumination value of zero when the moon was below the horizon. We used moon illumination (% illuminated) as a metric of moon brightness because our site had very low cloud cover (cloud cover reached a maximum of 5% during all surveys). A Sky Quality Meter (Sky Quality Meter SQM, Unihedron, Grimsby, Ontario, Canada) was used to measure the night brightness during a full moon (100% illuminated) and a new moon (0% illuminated) for comparison with our lab data (see 2.4 Emergence test below). We present the mean ± SD of five measures with the SQM under each condition (full moon, new moon, lab “full moon”, lab “new moon”) to capture variability. The SQM measures sky brightness in magnitudes per square arcsecond, with typical values ranging from 17 (a bright moon with artificial light pollution) to 23 (very dark night sky with no moon or light pollution). For comparability to other studies, we have converted the skyglow measures from the SQM (mag/arcsec2) to estimates of luminance (candelas) and illuminance (lux) following the approach outlined by Wilson et al. (2021) (Table 1).
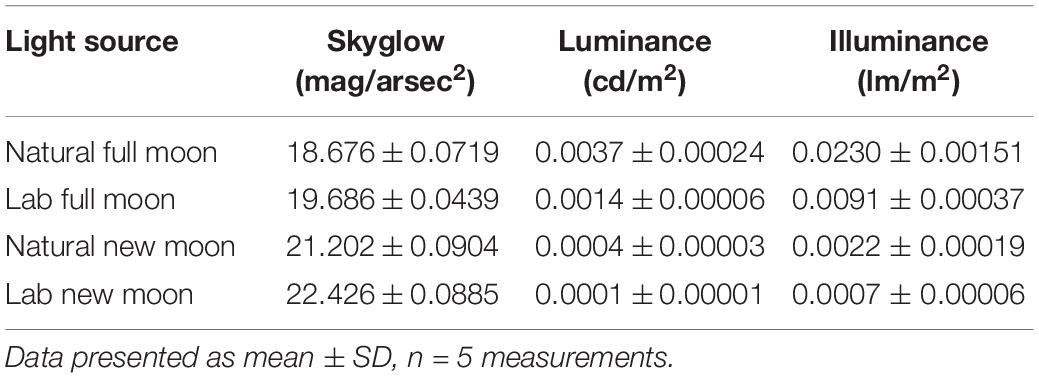
Table 1. Measure of skyglow (mag/arcsec2), luminance (candela [cd/m2]), and illuminance (lux [lm/m2]).
Gecko Collection and Husbandry for Lab Experiments
In March 2020, we collected 20 house geckos (Gehyra dubia) from the James Cook University campus (JCU), Townsville, Queensland, Australia. All geckos were hand-captured between 18:30 and 22:30 h from buildings, trees, and other vegetation around the campus. All geckos were maintained individually in plastic containers (300 × 225 × 115 mm L × W × H) containing a double layer of paper towel as substrate and shelter, a water dish, and a thermal gradient temperature strip along one side of the enclosure to allow for thermoregulation (Nordberg and Schwarzkopf, 2019a). Geckos were housed in a temperature-controlled room (25.5 ± 1.5°C) with a 12:12 h light cycle. Geckos were fed live European house crickets (Acheta domesticus) and provided fresh water twice weekly. All geckos were allowed two weeks to acclimate before any testing occurred.
Emergence Test
We designed a lab experiment to assess a behavior similar to what we measured in our field observations (to quantify the activity of geckos in response to varying light levels). To assess the activity of geckos in two light treatments, we used four identical glass terraria as testing arenas (500 × 400 × 300 mm; L × W × H; Figure 3). Each arena contained a 100 × 100 × 150 mm (L × W × H) ceramic tile shelter placed vertically against one glass wall, held in place with Blu-Tack adhesive (Blu-Tack, Bostik Australia Pty Ltd., Thomastown, VIC, Australia) as a refuge for the gecko. All trials used two infrared lights positioned behind the testing arena to increase the contrast between the gecko and the background of the arena for filming. In the light treatment, a 45-W bulb was placed inside a concrete block in the corner of the room, pointing at the wall, to provide the very low level of light (0.0091 lux compared to a full moon night of 0.0230 lux; Table 1). In the dark treatment, no room lights were used (0.0007 lux compared to a new moon night of 0.0019 lux; Table 1). All trials were recorded for 20 min with the video camera in night mode. To begin the experiment, geckos were placed in the center of the testing arenas and encouraged to take refuge under the tile shelter by chasing with an open hand, representing a simulated predation attempt to begin each trial. We then measured how long it took geckos to exit the shelter (emergence time) and to become active by exploring the testing arena. This behavior is similar to that in our field study as geckos under shelters would not be detectable during a spotlight survey, however, after the gecko emerged from the shelter to explore the arena (e.g., similar to crawling on the trunks of trees), they would be detectable via eyeshine. This experiment allowed us to test the activity of geckos in response to nocturnal light in the lab. The walls of the arena and the tile shelters were cleaned with 80% ethanol between trials to remove any chemical cues left by previous geckos. Each gecko was randomly tested twice per light treatment but was not tested more than once per 24 h.
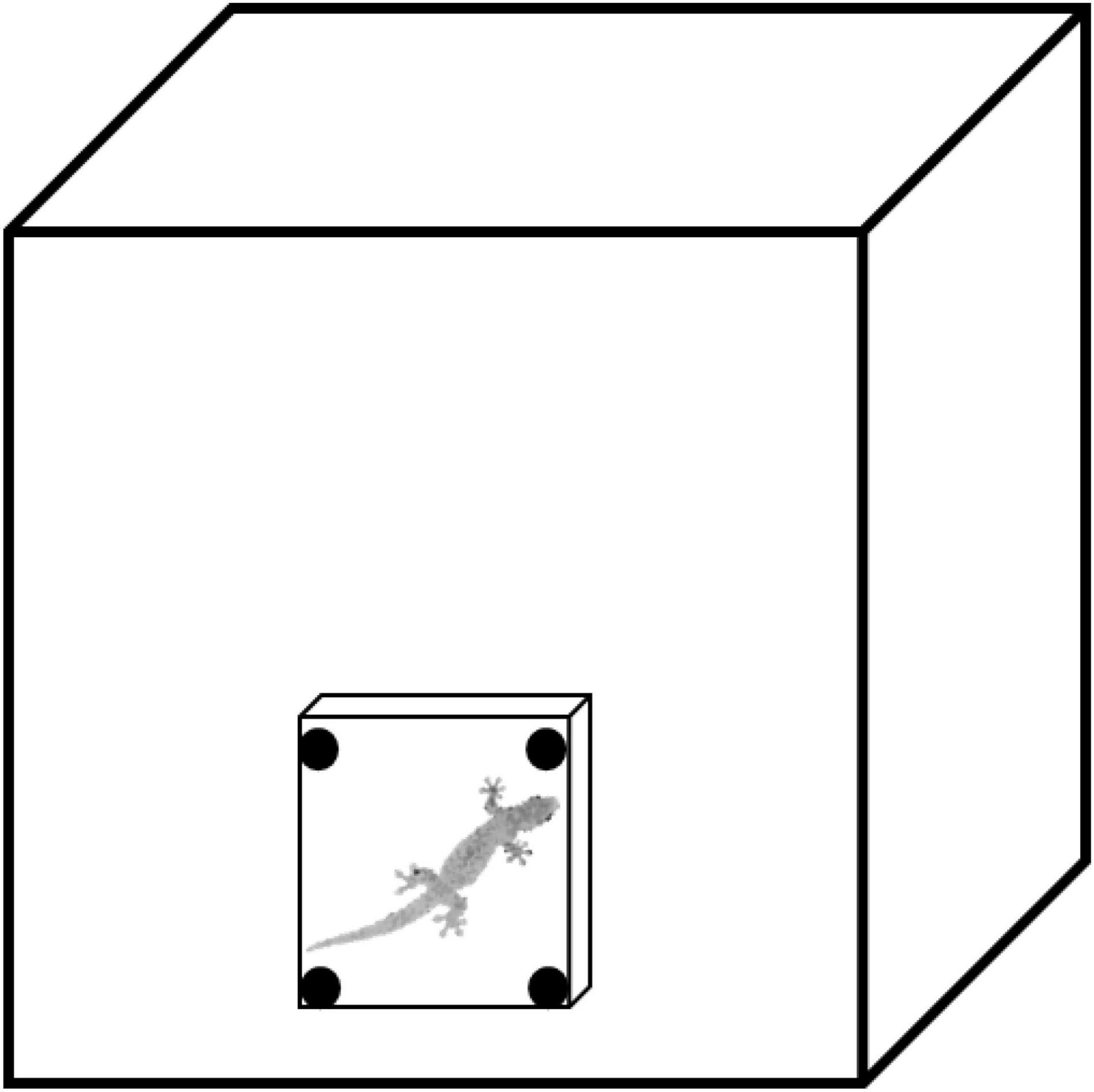
Figure 3. Experimental testing arena to test emergence time of Gehyra dubia in the laboratory. Testing arenas (glass terraria: 500 × 400 × 300 mm; L × W × H) have a ceramic tile shelter (100 × 100 × 150 mm; L × W × H) adhered to one vertical wall of the terrarium with BluTack adhesive (Blu-Tack, Bostik Australia Pty Ltd., Thomastown, VIC, Australia). Figure adapted from Nordberg et al., 2021.
Data Analyses
To account for differences in survey effort among spotlight surveys, we standardized our gecko relative abundances (herein referred to as ‘gecko abundance’) by dividing the total number of geckos observed by the total survey effort (cumulative time spent surveying) each night. To identify if gecko and predator abundances were correlated with moon illumination, we used Pearson’s correlations. In addition, we fit linear mixed-effects models (LME; Bates et al., 2015) with a Gaussian distribution, using gecko and predator abundance as response variables, moon illumination (%), sex, and predator group as fixed effects, and air temperature (°C) and site location as random factors in models to account for variation in temperature and site characteristics. Further, we calculated model fit values with pseudo R2 values (R2marginal = model fit for fixed effects; and R2conditional = model fit for fixed and random effects) for each model (Nakagawa and Schielzeth, 2013). Similarly, we fit linear mixed-effects models for our lab experiment to identify the influence of light treatment as a fixed effect on the emergence time (latency to emerge from shelter) of geckos from under the shelter tile. We used individual gecko IDs as a random factor to account for variation among individuals in our repeated-measures design. We conducted model selection using the dredge function in the package MuMIn (Barton, 2019) based on Akaike’s information criterion (AICc, corrected for small sample sizes). Models with the lowest AICc values had the greatest explanatory power, model fit, and model parsimony (Burnham and Anderson, 2004). Model averaging was used when no optimal model could be identified (i.e., there were multiple top models with ΔAICc < 2; Mazerolle, 2020). Final models were validated by examining deviance residual plots.
We compared the distribution of microhabitat use in relation to moon illumination to test if geckos used more complex microhabitats on the brightest nights. We examined two measures of tree complexity which would offer geckos more refugia. First, we used a measure of bark flakiness and used a Kolmogorov-Smirnov Tests (KS test; Arnold and Emerson, 2011) to compare the distribution of tree bark use with a series of paired KS tests (bark index 1 vs. 2; bark index 2 vs. 3; and bark index 1 vs. 3). We used Bonferroni adjusted p-values of 0.0167 for significance tests to account for multiple tests. Second, we used a measure of tree branching complexity as our response variable and used a linear regression model to test if geckos used more complex trees during brighter nights. All statistical analyses were performed in the program R (R Core Team, 2018). We inspected residual boxplots and determined if the assumptions of normality and homogeneity of variance were met. Means are presented as ± 1 SE.
Results
Field Surveys
Over four survey periods, we conducted a total of 800 person-hours of spotlight surveys of arboreal geckos, resulting in 644 observations of Australian house geckos (Gehyra dubia), 8 northern velvet geckos (Oedura castelnaui), and 7 eastern spiny-tailed geckos (Strophurus williamsi). The abundances of O. castelnaui and S. willaimsi were too low to adequately assess their relationship with moon illumination; herein ‘gecko’ refers to Gehyra dubia, unless otherwise stated. Moon illumination was 0.0022 lux during new moon nights and 0.0230 lux during full moon nights (Table 1). Our linear mixed-effects model indicated that moon illumination was an important predictor of gecko abundance and was included in the top model (Table 2). More geckos were detected when moon illumination was brighter, as gecko abundance was significantly and positively related to moon illumination (Pearson’s correlation test: r = 0.52; t = 4.98, df = 66, p < 0.001; Figure 4).

Table 2. Top models for linear mixed-effects results examining the effect of (adjusted) moon illuminations on (adjusted) gecko abundance during field surveys, and the effect of light treatment and species on latency to emerge from a shelter tile. Bold P-values indicate statistical significance.
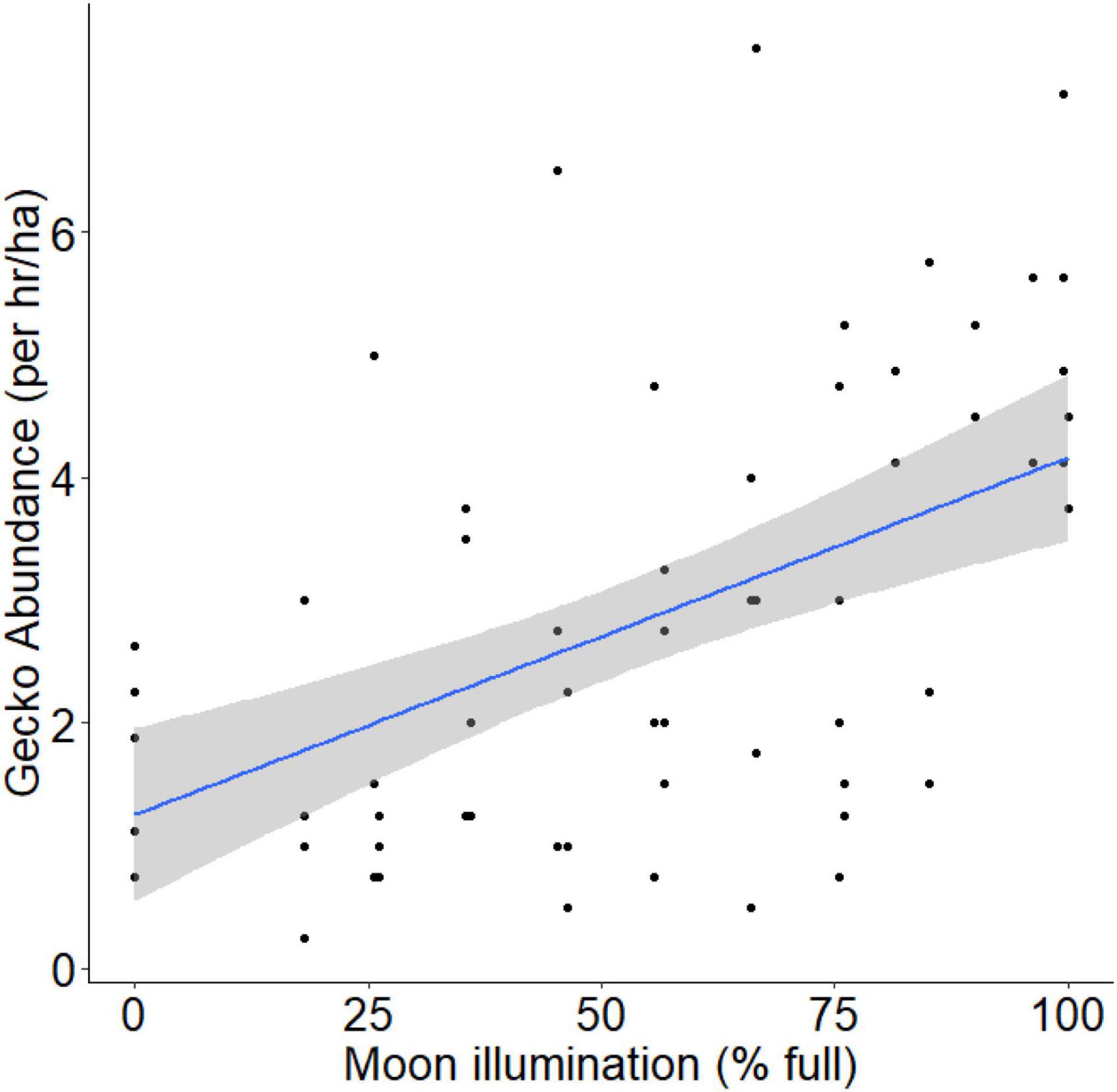
Figure 4. Relationship between relative gecko abundance (proxy for gecko activity) and moon illumination. Regression line represents the best fit line (blue line) and 95% confidence interval band (gray band) from our LME model incorporating gecko abundance (standardized for search-effort) and moon illumination (adjusted for visibility during the survey).
We identified 24 nocturnal predators during spotlight surveys: 14 birds, 1 mammal, and 9 snakes (Supplementary Table 1). Mammal abundance was too low to analyze, but snake predator abundance was not correlated with moon illumination (Pearson’s correlation test: r = −0.38; t = −0.731, df = 3, p = 0.517) nor was bird predator abundance (Pearson’s correlation test: r = 0.08; t = 0.227, df = 8, p = 0.826). Similarly, our LME indicated that none of the variables we measured significantly influenced predator abundance, as the null model was the best predictor of predator abundance (Table 2).
We classified the bark flakiness of 181 different trees where geckos were located. Geckos preferred trees with flaky tree bark and complex branching systems, however they did not exploit tree bark with higher flakiness values (i.e., better shelter) during periods of high moon illumination (Kolmogorov-Smirnov tests: bark index 1 vs. 2: D = 0.069, p = 0.761; bark index 1 vs. 3: D = 0.124, p = 0.950; bark index 2 vs. 3: D = 0.069, p = 0.999). The selection of flaky tree bark was consistent across moon phases. In addition, geckos did not use more complex trees during bright nights (linear regression: F = 0.2311,193, p = 0.630, R2 = −0.004).
Lab Experiment: Emergence Test
In our lab set-up for the “full moon”, conditions were similar to the natural brightness of a full moon (lab “full moon” = 0.0091 ± 0.0004 lux; natural full moon = 0.0230 ± 0.0015 lux; Table 1). Similarly, our “new moon” conditions were similar to natural brightness levels of a new moon (lab “new moon” = 0.0007 ± 0.00006 lux; natural new moon = 0.0022 ± 0.0002 lux). Our top LME model indicated that light treatment and sex were important predictors of latency to emerge from the shelter (Table 2). The conditional R2 value in our model (0.39), which includes random effects (individual gecko ID), showed an important influence of individual behaviors. Geckos emerged significantly sooner from the shelter in the light treatment compared to the dark (Figure 5). We found no significant difference between sexes on latency to emerge from the shelter (Table 2).
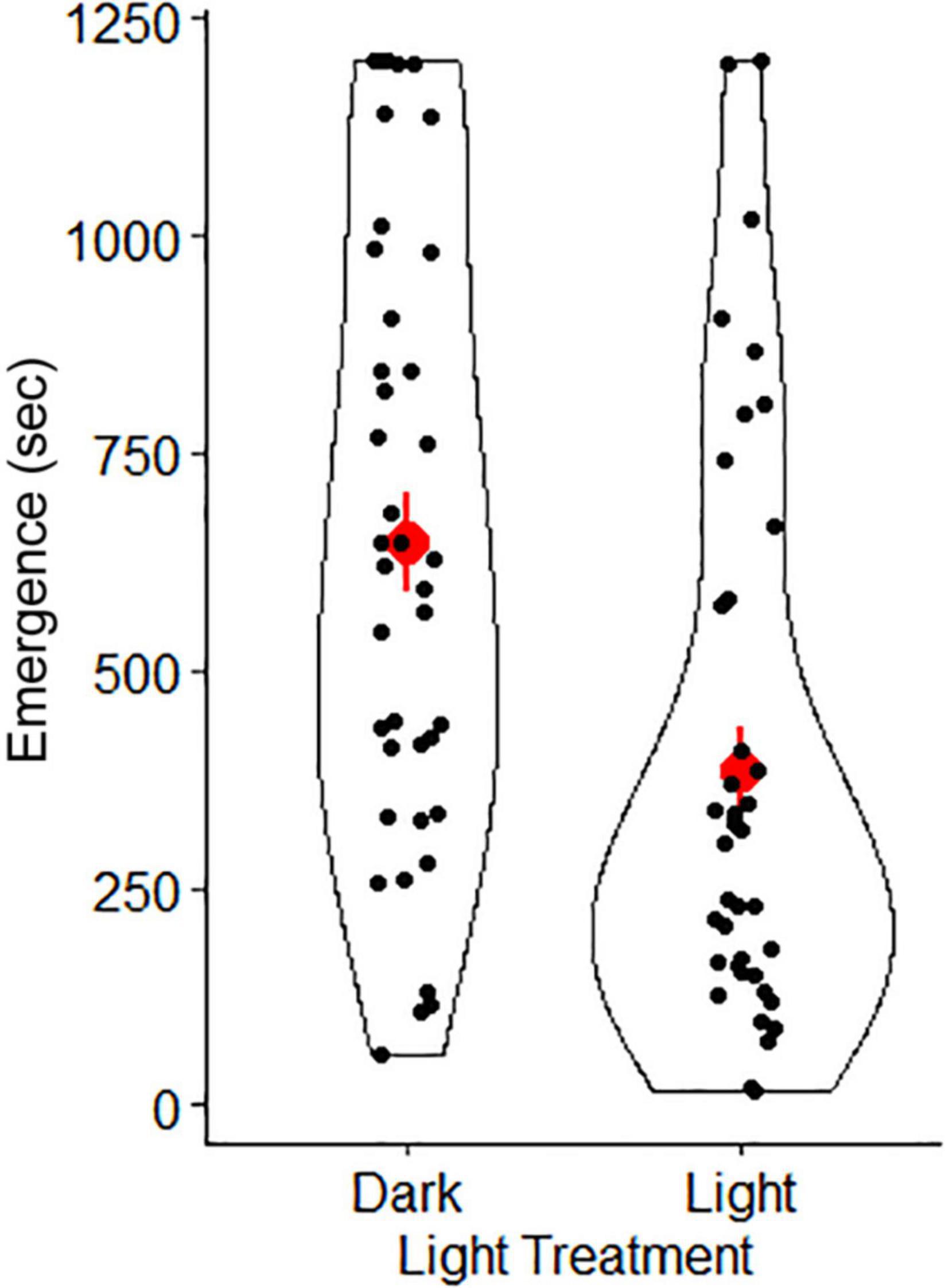
Figure 5. Mean (red points) ± SE (red lineranges) of emergence time for Australian house geckos (Gehyra dubia) in two light treatments. Black points represent the distribution of data. The dark treatment was completed at 0.0007 lux, representing the darkest nights (e.g., new moon conditions = 0.0022 lux). The light treatment was completed at 0.0091 lux and had low ambient light levels from a partially concealed light bulb in the corner of the testing room to represent the brightest nights (e.g., full moon conditions = 0.0230 lux).
Discussion
Both predators and prey rely on light from the moon to facilitate prey capture (predators) and or predator avoidance (prey). We predicted that house geckos would show reduced activity on bright nights, possibly to reduce predation risk, and show higher activity during darker moon phases, when predation risk was lower. However, our field data indicated the opposite trend: geckos were more active (abundant) on bright nights. A gecko that remains hidden in a shelter (e.g., under tree bark) would not be detectable via eyeshine, whereas animals that are on the surface of bark would be detectable. Therefore, our measure of abundance was a proxy measure of the activity of geckos in the environment, i.e., indicating they were not sheltering. In the lab, we examined this in a slightly different way, by testing the effect of light on the emergence time of geckos; determining how environmental light conditions influenced sheltering behavior. Our lab results corroborated our field results, as geckos remained in shelter longer in dark conditions compared to low light.
Our data suggest that nocturnal geckos in our system did not follow the behavioral pattern predicted by the predation risk hypothesis, that geckos should be less active on bright nights to avoid detection by predators. A different species of nocturnal gecko (Teratoscincus scincus) showed increased pause duration while foraging under moonlit conditions, suggesting that these geckos were minimizing predation risk by limiting their movement under bright conditions (Seligmann et al., 2007). We did not investigate specific behavioral changes in geckos in this study, but it may be worth examining. In our study, predators were not more abundant on bright nights. However, it is important to note that predator abundance is not necessarily a perfect predictor of predation risk (Nordberg and Schwarzkopf, 2019b), and we did not specifically quantify predation directly. Rather, we simply inferred that predator activity and behavior would also be influenced by moon illumination, such that more predators would be active when the moon was brightest. An experiment quantifying the success rates of predators in relation to moon brightness would contribute valuable data in this regard. While we cannot dismiss it completely, there was little support, in our study, for the predation risk hypothesis.
Another way to interpret our results, which is in direct opposition to the predictions of the predation risk hypothesis, is that bright nights may actually encourage gecko activity because the light facilitates predator detection. Geckos are vulnerable to predation by predators with exceptional night vision, such as owls (Clarke, 1983), and activity on brighter nights may help offset this risk. On dark nights, the visual acuity of both birds and geckos presumably decreases, possibly lowering the risk of predation by birds, but also the detection of birds by geckos. Our experiments were not designed to measure the visual acuity hypothesis, but studies that specifically identify how moonlight enhance or hinder prey or predator detection would be extremely valuable. Further, we note that arboreal ambush predators, particularly pale-headed snakes (Hoplocephalus bitorquattus), were abundant at this site. Reptilian ambush predators likely do not require moonlight to detect prey. The use of chemosensory abilities and olfaction allows some snakes to forage effectively regardless of light levels (Bouskila, 1995). So, geckos should be able to detect and avoid foraging snakes better on bright nights when their surroundings are illuminated by the moon. Therefore, if geckos are active on dark nights, their risk of failing to detect an ambush foraging snake is likely greater.
Consistent with the predation risk hypothesis, in our laboratory experiment, we expected geckos to be fearful of predators, and therefore emerge from the shelters more slowly when it was brighter. Instead, geckos in our lab experiment took much longer to emerge from shelters when it was dark. We also note that our lab experiment showed substantial individual variation, suggesting that individual personality traits of boldness or exploratory activity are important drivers (Nordberg et al., 2021). If geckos were hesitant to emerge from shelters in the wild when it was darker, this would reduce our detection of geckos in the field, and, indeed, we did observe fewer geckos when it was dark. Geckos may choose to be active under brighter conditions, first, because they may have greater foraging success, and second, because it may increase their ability to detect predators, however, both these suggestions should be tested with targeted experiments.
If we view house geckos as predators, they may be responding to moonlight consistent with that role, whereby they are using high moon illumination to increase their own predation success on invertebrates. There are two ways bright moon illumination might increase predation success. First, geckos may see better when it is brighter, potentially allowing greater predation success (Roth and Kelber, 2004; Roth et al., 2009). In addition, invertebrates are likely to be more active on moonlit nights (Meyer et al., 2004; Lang et al., 2006), facilitating prey encounter and capture by geckos. Finally, both these theories may act in synergy to greatly increase predation success during bright moon conditions. Further work should examine gecko prey capture success at different light levels, to test this hypothesis.
Many studies have identified habitat-mediated responses to predation pressure (Kotler et al., 1991; Bouskila, 1995; Brown et al., 1999; Lima and Bednekoff, 1999). For example, scorpions experience higher predation risk, and therefore have to lower activity levels, under bright moon conditions (Skutelsky, 1996). To counter this, scorpions forage under shrubs and bushes on bright nights, to remain concealed from predators. Geckos preferred trees with flaky tree bark and complex branching systems (Nordberg and Schwarzkopf, 2019c), however, we did not find evidence to suggest that geckos shifted their habitat use, in terms of bark flakiness or tree complexity, in response to moon illumination. The distribution and proportion of tree bark flakiness used did not vary significantly in response to moon illumination. If geckos were changing their habitat use according to the habitat-mediated predation risk hypothesis, we expected to see an increase in the proportion of flaky bark used, i.e., more instances of bark indices of 2 or 3, as moon illumination increased from 0 – 100%. Rather, geckos used the same range of trees with low (poor refugia) to high flakiness (good refugia), regardless of moon phase. Similarly, geckos did not use more structurally complex trees, i.e., trees with more complex branching, on bright nights.
In addition to moonlight, artificial light at night (ALAN) is a source of light influencing wildlife (Longcore and Rich, 2004; Hölker et al., 2010; Gaston et al., 2015; Russart and Nelson, 2018). Wildlife are adapted to temporally cyclical patterns of light and dark, but artificial light has now illuminated the night skies. ALAN and associated light pollution have significant impacts on nocturnal wildlife, altering activity (Becker et al., 2013; Bird et al., 2018; Baxter-Gilbert et al., 2021), physiology (Bedrosian et al., 2011; Dominoni et al., 2013), and increasing mortality (Rodríguez et al., 2012). In addition, ALAN can alter community composition of invertebrates attracted to artificial lights (Davies et al., 2012), and associated predation patterns (Komine et al., 2020). However, as with many ecological disturbances, and consistent with the benefits of low light to nocturnal animals, disturbance-tolerant species and urban-adapters can take advantage of ALAN. Reptiles and amphibians can exploit ALAN to their benefit: urban-adapted geckos, including Gehyra dubia, show increased foraging activity around artificial lights on buildings (Zozaya et al., 2015; Martín et al., 2018). Our study suggests these geckos may prefer the light levels provided in some urban areas. It would be interesting to determine the point at which brightness from ALAN becomes too great, and begins to act as a deterrent for these species.
The effects of light at night, either from artificial sources or moonlight, influences the behavior and activity of many animals. The associations between light levels and increased or decreased activity are of interest to behavioral ecologists, but also can be useful for conservation and management. Understanding the activity times of target species may enable researchers to survey or set traps at critical time periods, e.g., specific moon phases, that produce the highest activity measures or capture rates. Conservation managers could use activity associated with moonlight to increase their trapping success, for example, in controlling invasive species (Brivio et al., 2017; Muller et al., 2018; Komine et al., 2020). The effects of moonlight on wildlife is often overlooked, or assumptions are made about its effects on activity. It is important to quantify the influence of light on mesopredators to determine the costs and benefits of activity at particular times.
Data Availability Statement
The raw data supporting the conclusions of this article will be made available by the authors, without undue reservation.
Ethics Statement
The animal study was reviewed and approved by James Cook University Animal Ethics Committee.
Author Contributions
EN and LS conceived and designed the experiments and wrote the manuscript. EN performed the experiments and analyzed the data. Both authors contributed to the article and approved the submitted version.
Funding
This project was funded by the College of Science and Engineering at James Cook University and through a grant from Meat and Livestock Australia to LS (grant no. B.ERM.0088).
Conflict of Interest
The authors declare that the research was conducted in the absence of any commercial or financial relationships that could be construed as a potential conflict of interest.
Publisher’s Note
All claims expressed in this article are solely those of the authors and do not necessarily represent those of their affiliated organizations, or those of the publisher, the editors and the reviewers. Any product that may be evaluated in this article, or claim that may be made by its manufacturer, is not guaranteed or endorsed by the publisher.
Acknowledgments
We would like to acknowledge the Traditional Custodians of Townsville and Charters Towers, where this research was conducted, the Wulgurukaba of Gurrumbilbarra and Yunbenun, Bindal, and Gudjal People. We pay our respects to their Elders, past, present, and emerging. We would like to thank our many volunteers for their assistance in field and lab work. We would like to thank the Queensland Department of Agriculture and Fisheries, especially P. O’Reagain and J. Bushell for allowing us access to the Wambiana Grazing Trial. We also thank the Lyons family for their hospitality and accommodation at Wambiana Cattle Station.
Supplementary Material
The Supplementary Material for this article can be found online at: https://www.frontiersin.org/articles/10.3389/fevo.2022.821335/full#supplementary-material
References
Alonso, J. C., Abril-Colón, I., and Palacín, C. (2021). Moonlight triggers nocturnal display in a diurnal bird. Anim. Behav. 171, 87–98. doi: 10.1016/J.ANBEHAV.2020.11.011
Arnold, T. B., and Emerson, J. W. (2011). Nonparametric goodness-of-fit tests for discrete null distributions. R J. 3, 34–39. doi: 10.32614/rj-2011-016
Barton, K. (2019). MuMIn: Multi-model Inference. Available online at: https://cran.r-project.org/package=MuMIn (accessed January 2022).
Bates, D., Mächler, M., Bolker, B. M., and Walker, S. C. (2015). Fitting linear mixed-effects models using lme4. J. Stat. Softw. 67, 1–48. doi: 10.18637/jss.v067.i01
Baxter-Gilbert, J., Baider, C., Florens, F. B. V., Hawlitschek, O., Mohan, A. V., Mohanty, N. P., et al. (2021). Nocturnal foraging and activity by diurnal lizards: six species of day geckos (Phelsuma spp.) using the night-light niche. Austral. Ecol. 46, 501–506. doi: 10.1111/AEC.13012
Becker, A., Whitfield, A. K., Cowley, P. D., Järnegren, J., and Næsje, T. F. (2013). Potential effects of artificial light associated with anthropogenic infrastructure on the abundance and foraging behaviour of estuary-associated fishes. J. Appl. Ecol. 50, 43–50. doi: 10.1111/1365-2664.12024
Bedrosian, T. A., Fonken, L. K., Walton, J. C., and Nelson, R. J. (2011). Chronic exposure to dim light at night suppresses immune responses in Siberian hamsters. Biol. Lett. 7, 468–471. doi: 10.1098/rsbl.2010.1108
Bird, B. L., Branch, L. C., Miller, D. L., Bird, B. L., Branch, L. Y. N. C., and Mitter, D. L. (2018). Effects of coastal lighting on foraging behavior of beach mice. Conserv. Biol. 18, 1435–1439. doi: 10.1111/j.1523-1739.2004.00349.x
Bouskila, A. (1995). Interactions between predation risk and competition: a field study of kangaroo rats and snakes. Ecology 76, 165–178. doi: 10.2307/1940639
Brivio, F., Grignolio, S., Brogi, R., Benazzi, M., Bertolucci, C., and Apollonio, M. (2017). An analysis of intrinsic and extrinsic factors affecting the activity of a nocturnal species: the wild boar. Mamm. Biol. 84, 73–81. doi: 10.1016/j.mambio.2017.01.007
Brown, J. S., Laundré, J. W., and Gurung, M. (1999). The ecology of fear: optimal foraging, game theory, and trophic interactions. J. Mammal. 80, 385–399. doi: 10.2307/1383287
Burnham, K. P., and Anderson, D. R. (2004). Multimodel inference: understanding AIC and BIC in model selection. Sociol. Methods Res. 33, 261–304. doi: 10.1177/0049124104268644
Clarke, J. A. (1983). Moonlight’s influence on predator/prey interactions between short-eared owls (Asio flammeus) and Deermice (Peromyscus maniculatus). Behav. Ecol. Sociobiol. 13, 205–209. doi: 10.1007/bf00299924
Daly, M., Behrends, P. R., Wilson, M. I., and Jacobs, L. F. (1992). Behavioural modulation of predation risk: moonlight avoidance and crepuscular compensation in a nocturnal desert rodent, Dipodomys merriami. Anim. Behav. 44, 1–9. doi: 10.1016/S0003-3472(05)80748-80741
Davies, T. W., Bennie, J., and Gaston, K. J. (2012). Street lighting changes the composition of invertebrate communities. Biol. Lett. 8, 764–767. doi: 10.1098/rsbl.2012.0216
Dominoni, D., Quetting, M., and Partecke, J. (2013). Artificial light at night advances avian reproductive physiology. Proc. R. Soc. B Biol. Sci. 280:20123017. doi: 10.1098/rspb.2012.3017
Gaston, K. J., Visser, M. E., and Hölker, F. (2015). The biological impacts of artificial light at night: the research challenge. Philos. Trans. R. Soc. B Biol. Sci. 370:20140133. doi: 10.1098/rstb.2014.0133
Hölker, F., Wolter, C., Perkin, E. K., and Tockner, K. (2010). Light pollution as a biodiversity threat. Trends Ecol. Evol. 25, 681–682. doi: 10.1016/j.tree.2010.09.007
Jetz, W., Steffen, J., and Linsenmair, K. E. (2003). Effects of light and prey availability on nocturnal, lunar and seasonal activity of tropical nightjars. Oikos 103, 627–639. doi: 10.1034/j.1600-0706.2003.12856.x
Komine, H., Koike, S., and Schwarzkopf, L. (2020). Impacts of artificial light on food intake in invasive toads. Sci. Rep. 10, 3–5. doi: 10.1038/s41598-020-63503-63509
Kotler, B. P., Brown, J. S., and Hasson, O. (1991). Factors affecting gerbil foraging behavior and rates of owl predation. Ecology 72, 2249–2260. doi: 10.2307/1941575
Kotler, B. P., Brown, J., Mukherjee, S., Berger-Tal, O., and Bouskila, A. (2010). Moonlight avoidance in gerbils reveals a sophisticated interplay among time allocation, vigilance and state-dependent foraging. Proc. R. Soc. B Biol. Sci. 277, 1469–1474. doi: 10.1098/rspb.2009.2036
Kramer, K. M., and Birney, E. C. (2001). Effect of light intensity on activity patterns of Patagonian leaf-eared mice, Phyllotis xanthopygus. J. Mammal. 82, 535–544. doi: 10.1093/jmammal/82.2.535
Kronfeld-Schor, N., and Dayan, T. (1999). The dietary basis for temporal partitioning: food habits of coexisting Acomys species. Oecologia 121, 123–128. doi: 10.1007/s004420050913
Kronfeld-Schor, N., and Dayan, T. (2003). Partitioning of time as an ecological resource. Annu. Rev. Ecol. Evol. Syst. 34, 153–181. doi: 10.1146/132435
Kronfeld-Schor, N., Visser, M. E., Salis, L., and van Gils, J. A. (2017). Chronobiology of interspecific interactions in a changing world. Philos. Trans. R. Soc. B Biol. Sci. 372:20160248. doi: 10.1098/rstb.2016.0248
Kyba, C. C. M., and Hölker, F. (2013). Do artificially illuminated skies affect biodiversity in nocturnal landscapes? Landsc. Ecol. 28, 1637–1640. doi: 10.1007/s10980-013-9936-9933
Kyba, C. C. M., Ruhtz, T., Fischer, J., and Hölker, F. (2011). Cloud coverage acts as an amplifier for ecological light pollution in urban ecosystems. PLoS One 6:e17307. doi: 10.1371/journal.pone.0017307
Lang, A. B., Kalko, E. K. V., Römer, H., Bockholdt, C., and Dechmann, D. K. N. (2006). Activity levels of bats and katydids in relation to the lunar cycle. Oecologia 146, 659–666. doi: 10.1007/s00442-005-0131-133
Lillywhite, H. B., and Brischoux, F. (2012). Is it better in the moonlight? nocturnal activity of insular cottonmouth snakes increases with lunar light levels. J. Zool. 286, 194–199. doi: 10.1111/j.1469-7998.2011.00866.x
Lima, S. L., and Bednekoff, P. A. (1999). Temporal variation in danger drives antipredator behavior: the predation risk allocation hypothesis. Am. Nat. 153, 649–659. doi: 10.1086/303202
Longcore, T., and Rich, C. (2004). Ecological light pollution. Front. Ecol. Environ. 2:191–198. doi: 10.1890/1540-92952004002[0191:ELP]2.0.CO;2
Martín, B., Pérez, H., and Ferrer, M. (2018). Effects of natural and artificial light on the nocturnal behaviour of the wall gecko. Anim. Biodivers. Conserv. 41, 209–215. doi: 10.32800/abc.2018.41.0209
Mazerolle, M. J. (2020). AICcmodavg: Model Selection and Multimodel Inference Based on (Q)AIC(c). Available online at: https://cran.r-project.org/package=AICcmodavg (accessed January 2022).
Meyer, C. F. J., Schwarz, C. J., and Fahr, J. (2004). Activity patterns and habitat preferences of insectivorous bats in a West African forest-savanna mosaic. J. Trop. Ecol. 20, 397–407. doi: 10.1017/S0266467404001373
Muller, B. J., Cade, B. S., and Schwarzkopf, L. (2018). Effects of environmental variables on invasive amphibian activity: using model selection on quantiles for counts. Ecosphere 9:e02067. doi: 10.1002/ecs2.2067
Nakagawa, S., and Schielzeth, H. (2013). A general and simple method for obtaining R2 from generalized linear mixed-effects models. Methods Ecol. Evol. 4, 133–142. doi: 10.1093/sysbio/syy060
Navarro-Castilla, Á, and Barja, I. (2014). Does predation risk, through moon phase and predator cues, modulate food intake, antipredatory and physiological responses in wood mice (Apodemus sylvaticus)? Behav. Ecol. Sociobiol. 68, 1505–1512. doi: 10.1007/s00265-014-1759-y
Neilly, H., Nordberg, E. J., VanDerWal, J., and Schwarzkopf, L. (2018). Arboreality increases reptile community resistance to disturbance from livestock grazing. J. Appl. Ecol. 55, 786–799. doi: 10.1111/1365-2664.12982
Neilly, H., Reagain, P. O., Vanderwal, J., and Schwarzkopf, L. (2017). Profitable and sustainable cattle grazing strategies support reptiles in tropical savanna rangeland. Rangel. Ecol. Manag. 71, 205–212. doi: 10.1016/j.rama.2017.09.005
Nordberg, E. J., and Schwarzkopf, L. (2019a). Heat seekers: a tropical nocturnal lizard uses behavioral thermoregulation to exploit rare microclimates at night. J. Therm. Biol. 82, 107–114. doi: 10.1016/j.jtherbio.2019.03.018
Nordberg, E. J., and Schwarzkopf, L. (2019b). Predation risk is a function of alternative prey availability rather than predator abundance in a tropical savanna woodland ecosystem. Sci. Rep. 9:7718. doi: 10.1038/s41598-019-44159-44156
Nordberg, E. J., and Schwarzkopf, L. (2019c). Reduced competition may allow generalist species to benefit from habitat homogenization. J. Appl. Ecol. 56, 305–318. doi: 10.1111/1365-2664.13299
Nordberg, E. J., Edwards, L., and Schwarzkopf, L. (2018a). Terrestrial invertebrates: an underestimated predator guild for small vertebrate groups. Food Webs 15:e00080. doi: 10.1016/j.fooweb.2018.e00080
Nordberg, E. J., Murray, P., Alford, R., and Schwarzkopf, L. (2018b). Abundance, diet and prey selection of arboreal lizards in a grazed tropical woodland. Austral. Ecol. 43, 328–338. doi: 10.1111/aec.12570
Nordberg, E., Denny, R., and Schwarzkopf, L. (2021). Testing measures of boldness and exploratory activity in native versus invasive species: geckos as a model system. Anim. Behav. 177, 215–222. doi: 10.1016/j.anbehav.2021.05.013
O’Reagain, P. O., Bushell, J., and Holmes, B. (2011). Managing for rainfall variability: long-term profi tability of different grazing strategies in a northern Australian tropical savanna. Anim. Prod. Sci. 51, 210–224. doi: 10.1071/an10106
Penteriani, V., Kuparinen, A., del Mar, Delgado, M., Palomares, F., López-Bao, J. V., et al. (2013). Responses of a top and a meso predator and their prey to moon phases. Oecologia 173, 753–766. doi: 10.1007/s00442-013-2651-2656
Prugh, L. R., and Golden, C. D. (2014). Does moonlight increase predation risk? meta-analysis reveals divergent responses of nocturnal mammals to lunar cycles. J. Anim. Ecol. 83, 504–514. doi: 10.1111/1365-2656.12148
Riedel, J., Nordberg, E., and Schwarzkopf, L. (2020). Ecological niche and microhabitat use of Australian geckos. Isr. J. Ecol. Evol. 66, 209–222. doi: 10.1163/22244662-bja10002
Rodríguez, A., Rodríguez, B., Curbelo, ÁJ., Pérez, A., Marrero, S., and Negro, J. J. (2012). Factors affecting mortality of shearwaters stranded by light pollution. Anim. Conserv. 15, 519–526. doi: 10.1111/j.1469-1795.2012.00544.x
Roth, L. S. V., and Kelber, A. (2004). Nocturnal colour vision in geckos. Proc. R. Soc. B Biol. Sci. 271, 485–487. doi: 10.1098/rsbl.2004.0227
Roth, L. S. V., Lundström, L., Kelber, A., Kröger, R. H. H., and Unsbo, P. (2009). The pupils and optical systems of gecko eyes. J. Vis. 9:27.1–11. doi: 10.1167/9.3.27
Russart, K. L. G., and Nelson, R. J. (2018). Artificial light at night alters behavior in laboratory and wild animals. J. Exp. Zool. Part A Ecol. Integr. Physiol. 329, 401–408. doi: 10.1002/jez.2173
Seligmann, H., Anderson, S. C., Autumn, K., Bouskila, A., Saf, R., Tuniyev, B. S., et al. (2007). Analysis of the locomotor activity of a nocturnal desert lizard (Reptilia: Gekkonidae: Teratoscincus scincus) under varying moonlight. Zoology 110, 104–117. doi: 10.1016/j.zool.2006.11.005
Skutelsky, O. (1996). Predation risk and state-dependent foraging in scorpions: effects of moonlight on foraging in the scorpion Buthus occitanus. Anim. Behav. 52, 49–57. doi: 10.1006/anbe.1996.0151
Upham, N. S., and Hafner, J. C. (2013). Do nocturnal rodents in the great basin desert avoid moonlight? J. Mammal. 94, 59–72. doi: 10.1644/12-MAMM-A-076.1
Weaver, R. E. (2011). Effects of simulated moonlight on activity in the desert nightsnake (Hypsiglena chlorophaea). Northwest Sci. 85, 497–500. doi: 10.3955/046.085.0308
Williams, D. R. (2004). Sun Fact Sheet. NASA (National Sp. Sci. Data Center). Available online at: https://nssdc.gsfc.nasa.gov/planetary/factsheet/sunfact.html (accessed November 2020).
Williams, D. R. (2010). Moon Fact Sheet. NASA (National Sp. Sci. Data Center). Available online at: https://nssdc.gsfc.nasa.gov/planetary/factsheet/moonfact.html (accessed November 2020).
Wilson, A. A., Seymoure, B. M., Jaeger, S., Milstead, B., Payne, H., Peria, L., et al. (2021). Direct and ambient light pollution alters recruitment for a diurnal plant-pollinator system. Integr. Comp. Biol. 61, 1122–1133. doi: 10.1093/icb/icab010
Keywords: ALAN, boldness, cost-benefit, moonlight, movement, predation
Citation: Nordberg EJ and Schwarzkopf L (2022) Afraid of the Dark? The Influence of Natural and Artificial Light at Night on the Behavioral Activity of a Nocturnal Gecko. Front. Ecol. Evol. 10:821335. doi: 10.3389/fevo.2022.821335
Received: 24 November 2021; Accepted: 04 March 2022;
Published: 28 March 2022.
Edited by:
Rebecca Rimbach, Duke University, United StatesReviewed by:
Nitya Prakash Mohanty, Stellenbosch University, South AfricaAnne Aulsebrook, Max Planck Institute of Ornithology, Germany
Brett Michael Seymoure, Colorado State University, United States
Copyright © 2022 Nordberg and Schwarzkopf. This is an open-access article distributed under the terms of the Creative Commons Attribution License (CC BY). The use, distribution or reproduction in other forums is permitted, provided the original author(s) and the copyright owner(s) are credited and that the original publication in this journal is cited, in accordance with accepted academic practice. No use, distribution or reproduction is permitted which does not comply with these terms.
*Correspondence: Eric J. Nordberg, RXJpYy5Ob3JkYmVyZ0B1bmUuZWR1LmF1