- 1Maio Biodiversity Foundation (FMB), Cidade Porto Ingles, Cape Verde
- 2Department of Biology, University of Aveiro, Aveiro, Portugal
- 3Grupo de Investigación Geología Aplicada y Regional (GEOGAR), Universidad de Las Palmas de Gran Canaria, Las Palmas de Gran Canaria, Spain
We conducted a 5-year field (2017–2021) and laboratory study of the relationship between type of substrate and hatching success, embryonic development, and the quality of hatchlings in loggerhead turtle nests. Our study site, the island of Maio in the archipelago of Cabo Verde, one of the world’s largest loggerhead turtle nesting colonies, displays marked heterogeneity of sand colouration, with dark, mixed, and light sandy beaches. We experimentally incubated eggs, comparing different nesting substrates under standard temperature and humidity conditions. Females nest in all sand types without preference. However, both the field and experimental study revealed a significant difference in hatching success depending on the type of substrate. Substrate of volcanic origin, dark in colour, with a lower amount of calcium carbonate, had a lower hatching success (HS; 30.3 ± 20.2%) compared to substrates of mixed (HS = 46.1 ± 26.5%) or light (HS = 78.1 ± 18.2%) colour. Eggs experimentally incubated in substrate that was light-coloured, with a larger grain size and higher calcium carbonate concentration, produced significantly more and larger offspring. Incubation temperatures were significantly higher in dark substrate, which partially explains the lower hatching success in this type of sand. However, experimental incubation with controlled temperatures consistently showed lower hatching success in dark sand. Thus, we found that not only the temperature, but also the specific characteristics of each substrate determine hatching success. The main predator of eggs and hatchlings (the ghost crab Ocypode cursor) showed no significant differences in abundance or size between different substrate types. Our results indicate that nest site selection between beaches or even within the same beach with different substrate conditions affects hatching success, hatchling physical condition, and subsequently the reproductive success of each female. The results of this study can inform conservation programmes with nest management and controlled incubation in the field and optimise adaptive nest management under future scenarios of rising global temperatures.
Introduction
The absence of parental care in some oviparous species, such as sea turtles, makes nest site selection and incubation substrate vital for the success of their nests (Doody, 2011). Sea turtles are long-lived, late-maturing, ectothermic organisms and depend on sandy beaches to lay their eggs, which incubate at the mercy of environmental conditions. Sea turtle females exhibit nesting philopatry but use different beaches in the same area, which may vary in morphology, type of substrate or vegetation (Conrad et al., 2011; Ditmer and Stapleton, 2012). For eggs laid in each type of substrate, abiotic factors such as temperature, humidity and gas exchange, and biotic factors such as presence of roots or predators, determine their final embryonic success (Ackerman, 1997; Wallace et al., 2004; Chen et al., 2010). Dark-coloured sand, for example, absorbs more solar radiation and therefore eggs must withstand higher temperatures than in light-coloured sand (Hays et al., 2001). The temperature range at which turtle eggs develop is relatively narrow and even small changes in incubation temperatures can have dramatic effects on embryo mortality (Laloë et al., 2017). The different compaction, composition, or granulometry of the substrate may also be determining factors in the success of embryonic development in these species, but this is an area that needs further study (Mortimer, 1990; Chen et al., 2007; Yalçın-Özdilek et al., 2007; Salleh et al., 2018; Saito et al., 2019; Stewart et al., 2019). The different environmental incubation conditions in nests causes variation in the incubation time, hatchling phenotype, their sex, and their vitality (Patino-Martinez et al., 2014; Kobayashi et al., 2017; Jensen et al., 2018; Marco et al., 2018). As clutch survival may vary according to substrate type, e.g., dark versus light sandy beaches (Martins et al., 2020), nest site selection has the potential to enhance or reduce this parameter (Hawkes et al., 2010; Patrício et al., 2018). Understanding this behaviour and the consequence for clutch survival is therefore critical, especially for populations of conservation concern.
This study was conducted in Cabo Verde, an archipelago of volcanic origin located on the west coast of Africa, 500 km from Senegal. Recent findings suggest there are more loggerhead turtles nesting in Cabo Verde than previously estimated, and that this might be the largest nesting subpopulation of this species globally (Patino-Martinez et al., 2021).
The loggerhead turtle (Caretta caretta) is listed as globally “Vulnerable” by the International Union for the Conservation of Nature (IUCN) and its persistence is conservation dependent (Casale and Tucker, 2017). The subpopulation of Cabo Verde has been identified as a separate genetic stock (Monzon-Arguello et al., 2010; Wallace et al., 2010) with multiple nesting groups inside the rookery (Stiebens et al., 2013; Baltazar-Soares et al., 2020). It is listed as “Endangered” under the IUCN Red List criteria B2, due to the continuing decline in area, extent, and/or quality of its habitat (Casale and Marco, 2015).
Within Cabo Verde, the island of Maio hosts an important proportion of this subpopulation, with 4,063–14,364 nests per year between 2016 and 2019 (Patino-Martinez et al., 2021). The nesting beaches of Maio present a high heterogeneity in the colour and type of sand, varying in a spectrum from dark sands of volcanic origin to light-coloured sands of biogenic origin, and including beaches which are a mixture of the two. Such diversity of sandy beaches provides an excellent natural laboratory for studying the influence of substrate on the incubation of eggs and the reproductive success of sea turtles.
To understand the potential effect of incubation substrates on the reproductive success of the loggerhead turtle, we have quantified the inter-beach variability of sand type and evaluated its effect on (i) nesting success, (ii) nest density, (iii) abundance and size of predators, (iv) hatching success, and (v) physical condition of hatchlings, both in situ and in laboratory conditions.
Materials and Methods
Study Site
The study was conducted on the island of Maio (269 km2, 110 km of coastline and 38 km of sandy beaches), one of the ten islands of the archipelago of Cabo Verde (15°13′50′′N 23°09′22′′0; Figure 1).
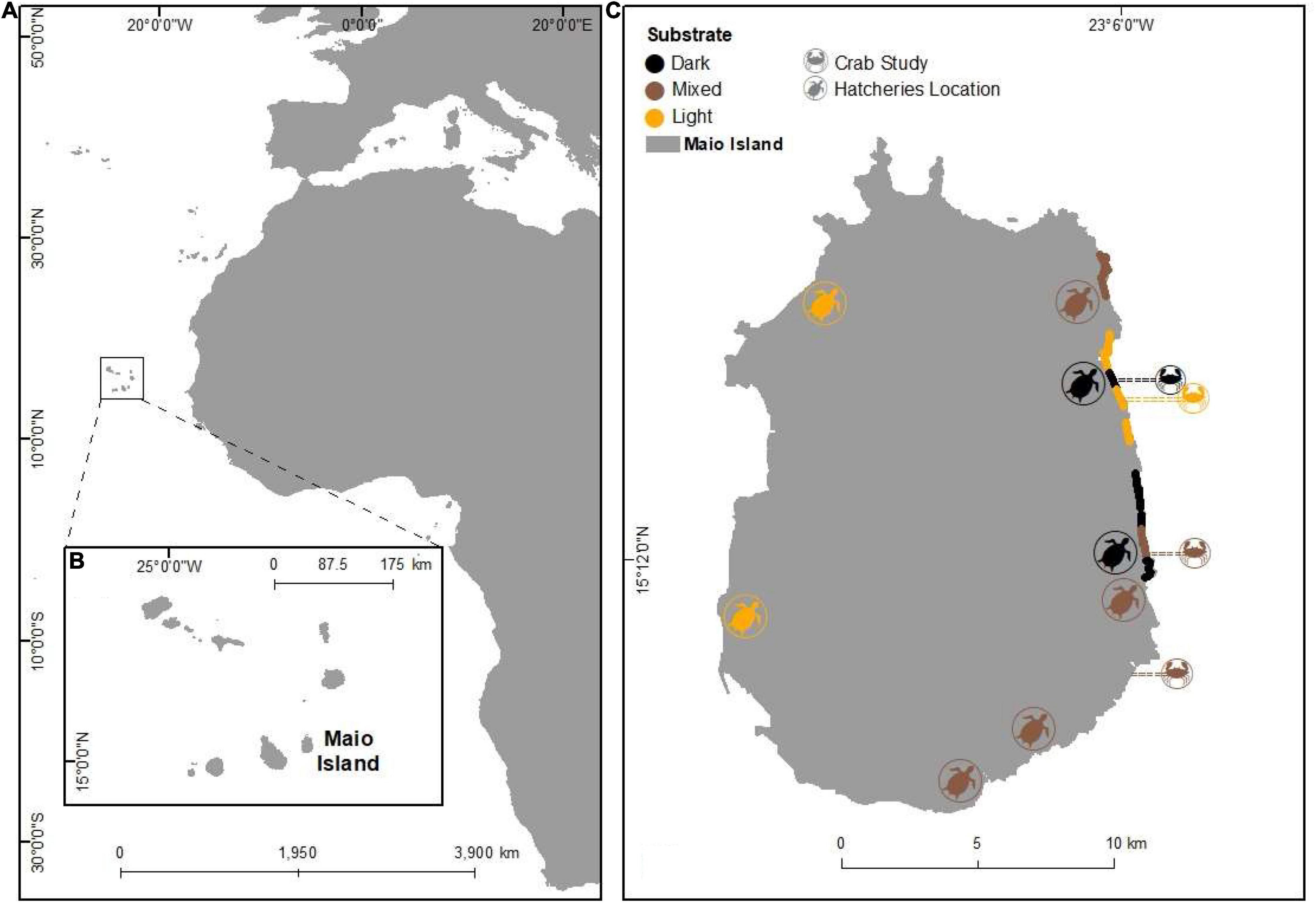
Figure 1. Overview of the study sites. (A) Image of the Cabo Verde archipelago, West Africa. (B) Detailed map of the Cape Verde archipelago (inset). (C) Island of Maio (C; 15°12′N—23°6′W) showing the study areas by sand types (by colours) where loggerhead turtles nest. The crab symbol represents the location of the predator survey. Coloured lines (east coast) illustrate nest density and nesting success study areas.
The high-energy beaches of the island of Maio are currently pristine and undeveloped. The colour of the sand and the size of the grain varies over the whole island. Beaches were classified by colour using the Munsell colour chart system (Fan et al., 2017), and all beaches were measured and georeferenced.
Nesting Success and Nest Density
We conducted 615 sampling nights (from 20:00 to 6:00; 44 fieldwork teams per night) during five consecutive nesting seasons (July–October) between 2017 and 2021, along 100% of the island’s sandy beaches (38 km). Each time a female turtle emerges from the sea to attempt to nest (nesting activity) she creates a distinctive set of tracks in the sand: with one track ascending to any aborted nesting attempt or a successful (camouflaged) nest, and another track descending to the sea. Following this pattern of tracks, all nesting activities (sometimes with direct observation of the females) were recorded and geolocated (Garmin e-trex Summit) and all turtle tracks were erased using wooden rakes or the ranger’s feet. To identify any nesting activity not recorded during the night, a daily early morning track count was carried out. Nesting success was estimated as the proportion of nesting activities resulting in effective nest placement. Nest density was calculated as the total number of nests per beach area (length × average width of three measurements along the beach). Nesting success and nest density were compared between beaches of different substrate (dark, mixed, and light) in the eastern part of the island (with similar wave conditions and accessibility from the sea), to reduce the spatial effect in the analysis.
Sand Type and Ghost Crab Abundance
In 2017, an observational study was undertaken to evaluate the influence of substrate type on the abundance and size of the ghost crab, the main predator of eggs, and offspring (Marco et al., 2015). Two infrared cameras (Browning BTC-5HD) were installed on three dark-coloured beaches (for six nights between July 5, 2017 and October 10, 2017), two mixed-sand beaches (for 10 nights between July 6, 2017 and September 16, 2017), and two light-sand beaches (for 13 nights between July 12, 2017 and October 8, 2017). Bait (unsuccessful eggs from excavated nests, and fresh fish heads) was placed in front of the cameras, and the number of crabs present in an area of 9 m2 (3 m × 3 m of sand) was counted with one photograph every hour (Supplementary Figure 1). The seven beaches were chosen in the eastern part of the island of Maio and are interspersed with each other (Figure 1). For the biometric study of the crabs, they were captured using drop traps (in 20-L plastic buckets with a high vertical wall) buried in the sand without a lid. The bait (fresh fish) was tied to a wire across the diameter of the top of the bucket. Three adjacent beaches (Figure 1) were chosen (one for each type of sand) and one trap was set per night from 22 to 6 h (dark sand – 10 nights between August 15, 2017 and October 13, 2017; mixed sand – eight nights between August 21, 2017 and September 30, 2017 and light sand – 14 nights between August 16, 2017 and October 11, 2017). Caught crabs were counted and the maximum carapace length and width of each crab were recorded (using Cen-Tech Digital callipers; Harbor Freight Tools, Pittsburgh, PA, United States; accuracy ± 0.01 mm).
Measuring the Effect of Sand Type on Hatchery Nests
To evaluate the influence of substrate type on nest incubation under standardised conditions, we conducted a field experiment. We moved 106 nests in 2017 to eight open beach hatcheries (two dark sand, four mixed substrate, and two light sand; Figure 1). In order to avoid any seasonal effect on hatching success the relocation was simultaneous in all three hatchery types (from 04 July to 17 August). The eggs were moved by walking with them in new plastic bags; it took a maximum of 3 h between oviposition and reburial. The relocated nests (28 in dark; 56 in mixed; 22 in light) were buried at a depth of 45 cm, in root-free substrate, and monitored daily during incubation. The hatcheries (enclosures surrounded by a 1 m high plastic mesh and wooden fence; 7 × 7 m; on a 0° slope) were in areas of the beach where tidal flooding does not occur and all of them prevented the entry of predators. Nests were in plots of 1 m2 for each nest. The number of eggs at the time of relocation was counted for each of the nests studied. The hatching success of each nest was determined as the proportion of eggs culminating in hatching. Incubation temperatures were recorded in three of the hatcheries simultaneously between 18 August and 18 November by one datalogger (Hobo Stow Away TidbiT v2 Onset,1 ±0.2°C accuracy, measuring 3.0 cm × 1.7 cm; programmed to record temperature at 30 min intervals) for each sand treatment at a depth of 0.37 m in the sand, resulting in 4,417 temperature data points from each sand type.
Temperature-Controlled Experiment
In 2018, we conducted a field experiment to assess the influence of sand type (dark, mixed, and light) on embryonic development, hatching success, and hatchling phenotype into a common temperature incubation environment. The experimental design corrected for spatial effects associated with the hatchery study and standardised the thermal regime as previously described in the study (Patino-Martinez et al., 2014). A total of 225 eggs were collected from five different females, laid during the same night (45 eggs per female). The eggs were collected directly from the female’s cloaca, ensuring that they did not touch the sand before the beginning of the experiment. Because females distribute their different nests among different beaches (Patino-Martinez et al., in press), nests were chosen randomly among beaches. Twenty-five eggs (5 per female) were placed in each one of nine plastic containers (0.37 m × 0.27 m × 0.23 m, sterile and dry). All eggs were weighed (using a microbalance Criacr Digital Pocket Scales, 500 g/accuracy ± 0.01 g. Stainless Steel. Energy Class A+), randomly distributed horizontally and completely covered with one of the types of sand. Each container was closed with a lid, with an air space (0.1 m) between the lid and the sand covering the eggs. The closed containers were buried in an open area of a mixed sand beach in three blocks of three containers, each block representing the three sand type treatments randomly distributed. The sand used in the experiment was collected at a depth of 0.45 m in the centre of natural nesting beaches of the different sand types and manually stirred for 3 min, before starting the experiment. All eggs were incubated at a depth of 45 cm (measured from the egg layer) without touching any other sand until hatching. From incubation day 45 onward, the experimental containers were inspected daily to verify hatching. To study temperature variability within the study treatments, data loggers [Hobo Stow Away TidbiT v2 Onset (see footnote 1), ±0.2°C accuracy, measuring 3.0 cm × 1.7 cm; programmed to record temperature at 30 min intervals] were placed in three containers (one per treatment) at the same depth as the eggs. The data loggers were placed at the start of the study, in the middle of each container and left untouched throughout incubation. To assess the effect of controlled incubation on temperatures, a temperature control was placed in the sand (one data every 30 min simultaneously with the experiment) (Supplementary Figure 2) on the beach, 1 m outside the experiment and at the same depth (45 cm).
To measure moisture, sand samples (approximately 300 g) were taken from each of the treatment at the beginning of the experiment. The fresh samples were weighed (using a microbalance Criacr Digital Pocket Scales, 500 g/accuracy ± 0.01 g. Stainless Steel. Energy Class A+) and then left to dry in high containers in a sunny location. The samples were reweighed periodically until a constant mass was reached (i.e., all water had been lost). The water content of the sand was calculated as the water loss divided by the total fresh (wet) weight, multiplied by 100. This experimental technique, using closed plastic containers, avoids flooding, and maintains initial moisture levels throughout the study (Patino-Martinez et al., 2014).
Temperature in situ
An observational study was conducted in 2019 and 2021 to compare in situ sand temperatures (dark, mixed, and light) at different depths (25, 35, 45, and 55 cm), to obtain baseline data on the normal ranges of incubation temperatures at these positions. The period studied was from 5/8 to 17/11 in both years. One temperature logger [Hobo Stow Away TidbiT v2 Onset (see footnote 1), ±0.2°C accuracy, measuring 3.0 cm × 1.7 cm] per depth and substrate type was placed in 2019 and two loggers per substrate type at 25 and 35 cm depth and three loggers per substrate type at 45 and 55 cm depth in 2021. Each year the dataloggers recorded data simultaneously at 30-min intervals. These data were used to calculate average temperatures per substrate and depth.
Measuring Hatchling Size and Performance
Morphology and locomotion of hatchlings affect their survival in the first hours after hatching (Ischer et al., 2009). Therefore, a random sample of eight hatchlings per nest was taken immediately after emergence and 100% of the hatchlings from the temperature-controlled experiment were taken for biometric analysis. For each hatchling, the length and straight width of the shell were recorded (using Cen-Tech Digital callipers; Harbor Freight Tools, Pittsburgh, PA, United States; accuracy ± 0.01 mm). All hatchlings were weighed (using a microbalance Criacr Digital Pocket Scales, 500 g/accuracy ± 0.01 g. Stainless Steel. Energy Class A+). The performance of the offspring was measured as the time taken to turn from the “face up” position to the normal “face down” run position (self-righting ability). These tests were done outdoors on moist, compact sand, repeated three times per individual and then averaged. Self-direction tests were mostly carried out before midnight (66.3%), therefore, in the present study, the possible effects of circadian rhythm on hatchling activity were not standardised. After the physical tests, the hatchlings were immediately released into the sea.
All unhatched eggs were dissected 48 h after the last nest emergence and 60 days later in the eggs from the temperature-controlled experiment. The embryos were assigned to a developmental status category, adapting the criteria proposed by Bilinski et al. (2001). We assigned a value per category, as follows: 0 = undeveloped, 1 = early embryonic death, 2 = middle embryonic death, and 3 = late embryonic death. The success of embryonic development was calculated as the proportion of eggs reaching stages 3 and successful hatching.
Laboratory Analysis
Samples (500 g) of each type of sand were collected at the beginning of the temperature-controlled experiment and were sent for laboratory analysis.
Granulometry, composition and origin were analysed in the laboratory for each type of sand. Salts and impurities were initially removed to avoid errors in the analysis, by washing each sample with 2 L of distilled water. They were then dried in a stove at a moderate temperature (65°C). To obtain the representative fraction for each of the analyses to be carried out, each sample was split as many times as necessary (Folk and Ward, 1957).
The particle size of the sediment was determined by dry sieving of the sample, using a sieving tower composed of 17 sieves, covering the range from gravel to silt and clay (Wentworth, 1922).
The carbonate content was determined by Bernard’s Calcimeter (Lamas et al., 2005). This method is based on the reaction of hydrochloric acid (HCl) with calcium carbonate (CaCO3) causing release of carbon dioxide (CO2). The gas displaces the volume of a liquid contained in a burette that was quantified, indirectly obtaining the carbonate content.
The origin of the grains and their proportions was analysed by a provenance analysis. Each sand sample (dark, mixed, and light) was fixed in sheets with epoxy resin for 24 h. By cutting the sheets and using carborundum abrasive, three thin sections of between 20 and 30 microns were obtained. The characteristics of the grains were determined by observing the sheets under a petrographic microscope (Ortoplan-Leitz) with plane and polarised lights. Each sand grain was identified into subcategories: bioclasts (red algae meshes, molluscs, foraminifera, bryozoans, and echinoderms), lithoclasts (mafic volcanic rocks, intraclasts, olivine, clinopyroxene, opaques -Fe and Ti oxides, and volcanic glass), and carbonate aggregates (bioclastic and lithoclastic grains containing sparite and micrite carbonate cements).
Statistical Analyses
We used R Studio software, version 1.1.463 (Campbell, 2019) to perform non-parametric and parametric tests. When normality and heteroscedasticity were assumed, an ANOVA test was made. A Kruskal-Wallis test was performed once the assumptions were not assumed, while a Tukey’s Test was performed for multiple comparisons. To investigate if nesting success and nest density were significantly different between substrates, we fitted generalised linear models (GLM), with substrate type as predictor (fixed factor) with nesting success and nest density as response (dependent) variables, and year as a random factor. A Chi-squared test for given probabilities was also performed. We arcsine-transformed the percentages of hatching success and development success data. Alpha was set at 0.05.
The granulometric analysis was done through the Gradistat programme (Blott and Pye, 2001).
Results
Field Study
Over the 5 years of the study (2017–2021), on all sand types, we counted 12,806 nesting activities in 2017, 30,075 in 2018, 19,316 in 2019, 38,993 in 2020 and 68,789 in 2021, corresponding to 5,429, 14,364, 7,937, 23,185 and 46,161 clutches respectively (mean number of nests per year = 19415; SD = 16450). According to the Munsell colour charts, 9.1% of the beaches were dark (Munsell = 5Y 3/1), 65.4% mixed (Munsell = 5Y 8/3), and 25.5% light (Munsell = 5Y 8/4). Nesting success was not significantly different between substrates (GLM df = 2; F = 0.03; P = 0.97; n = 70; Table 1) and nest density did not differ statistically among the distinct types of sand (K-W df = 2; H = 310, P = 0.21, n = 70; Table 1).

Table 1. Nesting success, nest density, and crab biometric measurements among the three types of substrates on 14 beaches close to each other (5 in dark sand, 5 in mixed sand, and 4 in light sand) on the east coast of the island of Maio (Figure 1).
Crab abundance (No. of crabs per trap per night) did not differ significantly between beaches on different substrates. Light-coloured sand had an average of 4.6 crabs, which is higher than the averages of 2.1 and 1.5 for mixed and dark sand, respectively (Table 1). Crab biometrics were similar in all three sand types (length 37.7 mm in dark, 33.1 mm in mixed, and 35.7 mm in light; H(2) = 1.57, P = 0.456; Table 1).
The Effect of Sand Type on Hatchery Nests
Hatching success differed between incubation substrates, with a range between 30.3 and 78.1% and was significantly lower in dark sand (Figure 2). Nests incubated in light sand had the highest hatching success (Dunn test light vs mixed P < 0.0001 and light vs dark P < 0.0001). Hatchling mass varied as a function of incubation sand (Weight: light 16.8 g ± 2.0 vs mixed 15.1 g ± 1.3 and dark 15.2 g ± 1.1; H2 = 10.9, p < 0.01; Figure 3A). Both SCL and SCW were significantly higher for hatchlings from light-coloured substrate (SCL: light 41.5 mm ± 3.6 vs mixed 40.5 mm ± 1.2 and dark 40.4 mm ± 1.5, H2 = 9.1, p = 0.01, Figure 3B; SCW: light 31.8 mm ± 2.5 vs mixed 31.1 mm ± 1.1 and dark 30.3 mm ± 1.0, H2 = 14.2, p = 0.001, Figure 3C), indicating that embryo growth occurred differently between substrates and produced different phenotypes. Self-righting time showed that the hatchlings from the light substrate were faster (Self-righting time: light 13.2 s ± 7.4 vs mixed 24.2 s ± 11.4 and dark 26.8 s ± 10.6; F2–69 = 9.3, p < 0.001; Figure 4). There was a statistically significant difference in incubation temperatures between the different substrates, where the temperature decreased from dark to light sand (Table 2).
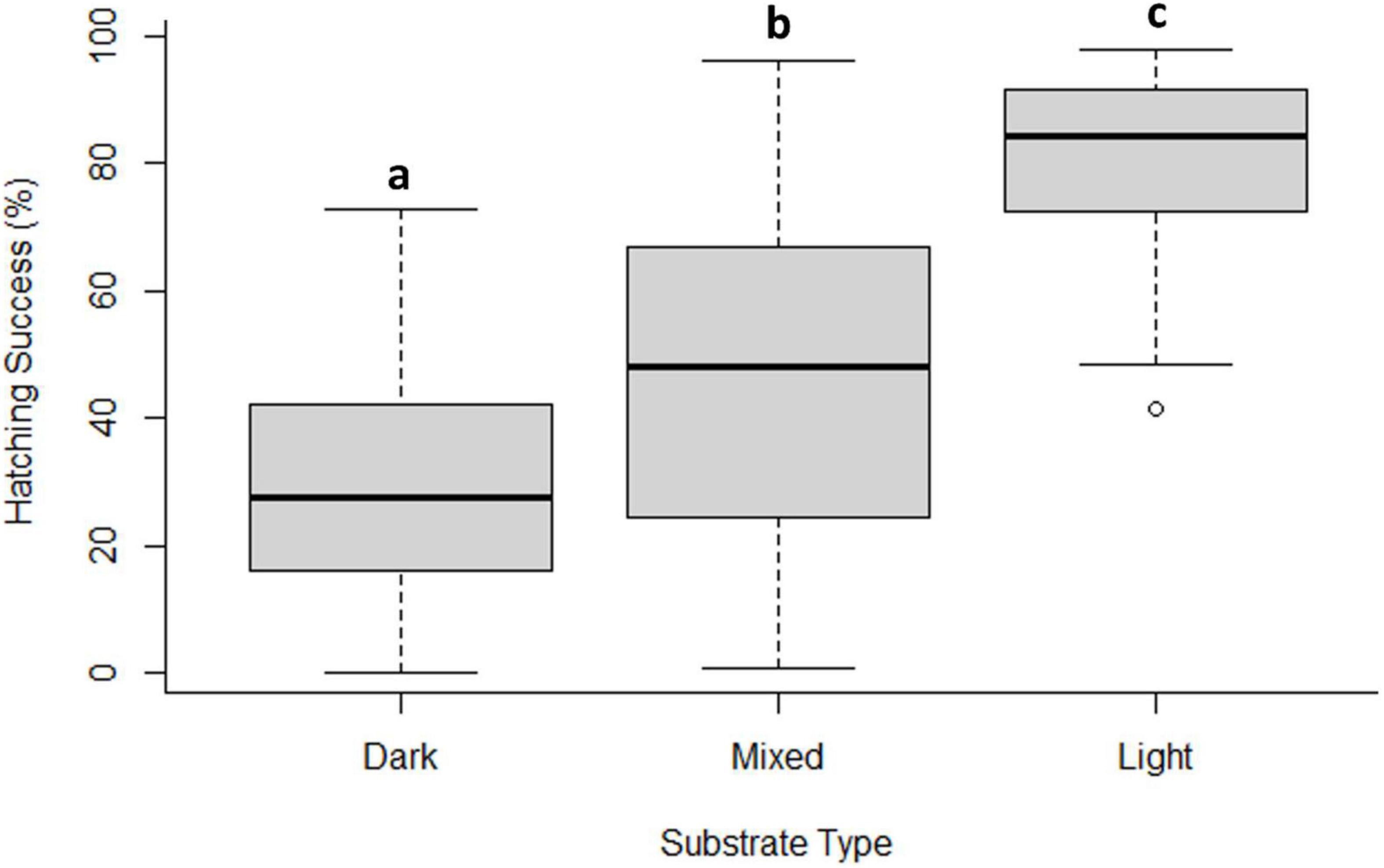
Figure 2. Hatching success in different substrates (dark, mixed, and light) under controlled incubation conditions in the hatchery. Different lowercase letters indicate statistically significant differences among treatments. The median is the bold line as a measure of central tendency, quartiles one (Q1) and three (Q3) marked by upper and lower box limits and maximum (max) and minimum (min) values marked with whiskers above and below.
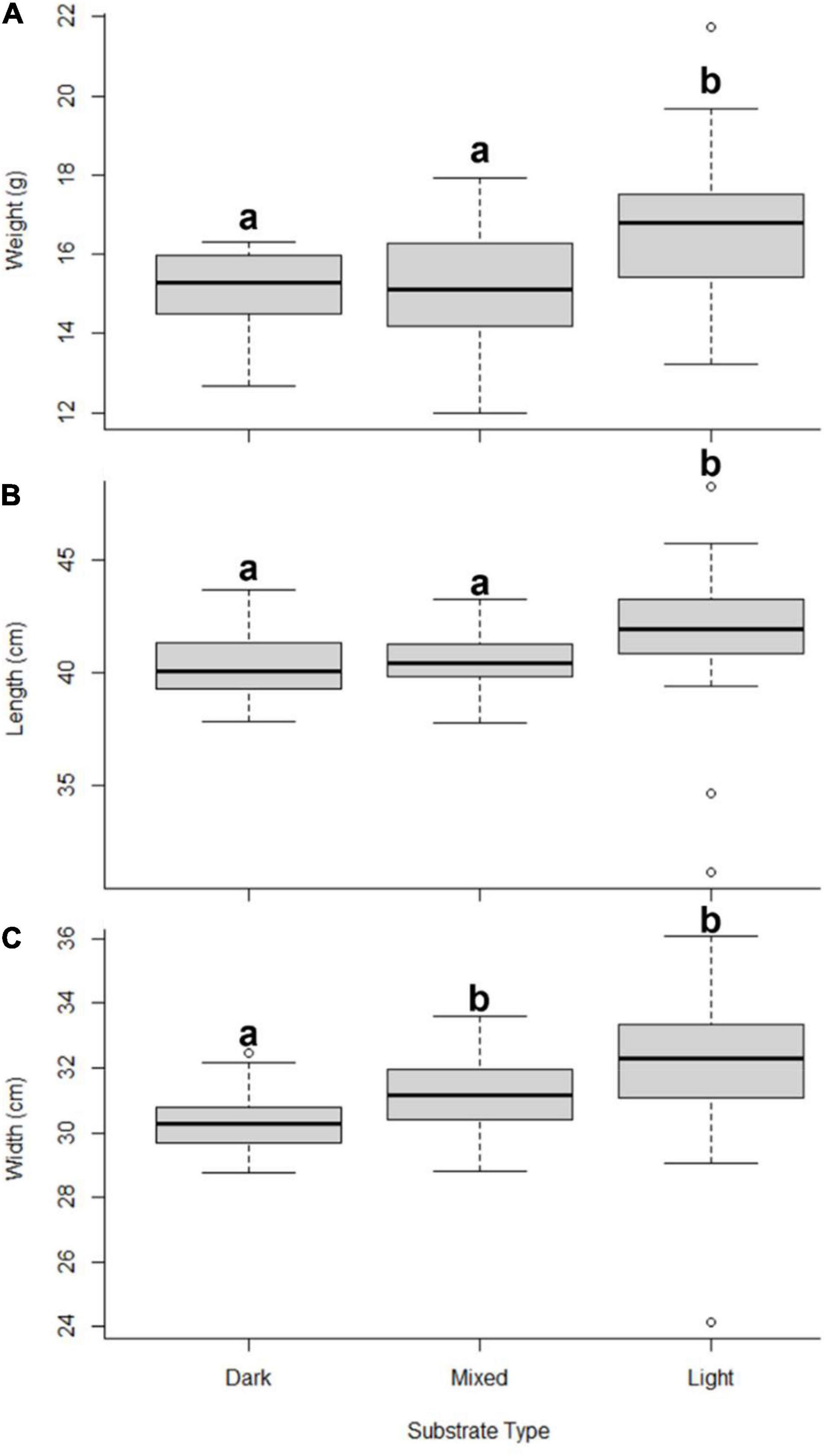
Figure 3. Hatchling phenotype (A) weight, (B) straight carapace length, and (C) straight carapace width in the different incubation substrates (dark, mixed, and light) under controlled conditions in the hatchery. Different lowercase letters indicate statistically significant differences among treatments. The median is the bold line as a measure of central tendency, quartiles one (Q1) and three (Q3) marked by upper and lower box limits and maximum (max) and minimum (min) values marked with whiskers above and below.
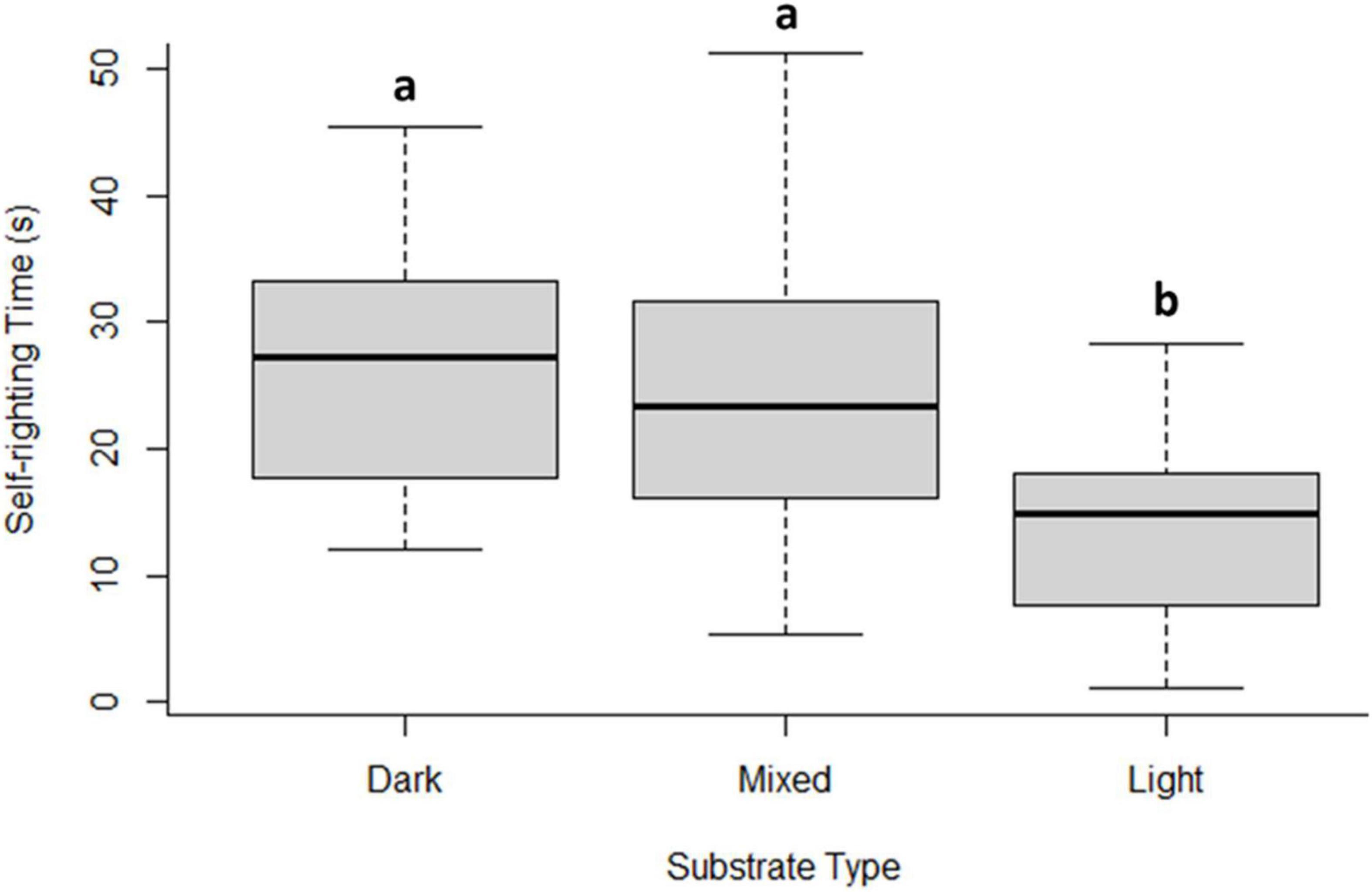
Figure 4. Hatchling self-righting time (time needed to recover the face-down position) in different substrates (dark, mixed, and light) under controlled incubation conditions in the hatchery. Different lowercase letters indicate statistically significant differences among treatments. The median is the bold line as a measure of central tendency, quartiles one (Q1) and three (Q3) marked by upper and lower box limits and maximum (max) and minimum (min) values marked with whiskers above and below.
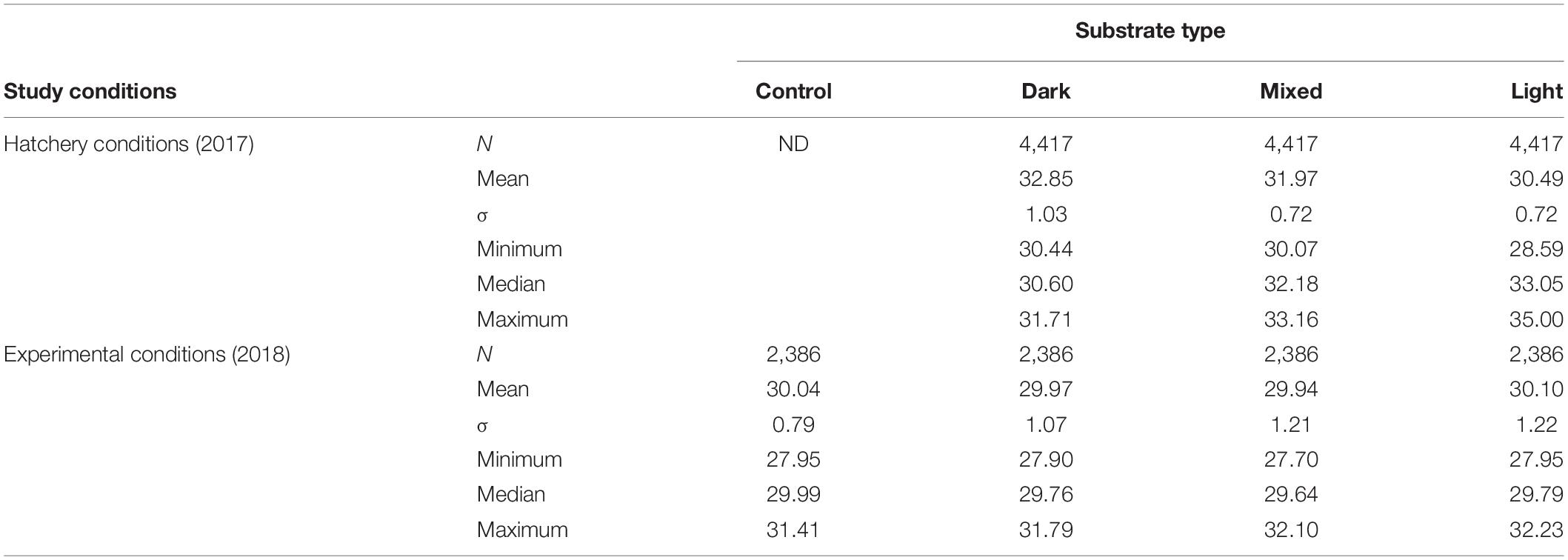
Table 2. Temperatures recorded in three types of substrates in two study conditions (hatchery and experimental).
Temperature-Controlled Experiment
Hatching success differed among substrates (ANOVA: F2,6 = 30.6, P < 0.001), ranging from 16 to 81% and was significantly lower in the dark sand (16.0% ± 4.0 s.d., 95% CI = 0.2–32) compared to mixed (81.3% ± 15.1 s.d., 95% CI = 65–97) or light (77.3% ± 12.2 s.d, 95% CI = 61–93). Embryonic mortality in the dark substrate occurred earlier than in mixed and light (ANOVA: F2,222 = 16.1, P < 0.0001). While 24% of the eggs experienced early embryonic mortality in dark substrate, only 6.7% of the embryos died in initial stages in light substrate.
The dark substrate produced significantly smaller offspring in shell length (SCL: WS = 40.6 mm ± 1.9 s.d, MS = 40.9 ± 2.1 s.d, BS = 38.3 ± 3.7 s.d ANOVA: F2,119 = 4.9, P < 0.01). Shell width of experimental hatchlings were similar among treatments (SCW: P = 0.13). The hatchlings from dark substrate were significantly slower in the self-righting ability test, at 84.8 s ± 42.1s.d (mixed = 28.6 s ± 38.4s.d, light 58.6 s ± 42.4s.d; ANOVA: F2,119 = 11.78, P < 0.0001). The apparent shell malformations of offspring were extremely rare and there was no relationship with the incubation substrate.
The mean incubation temperatures in the experimental treatments were highly and significantly correlated with the control temperatures (Pearson correlation: dark = 0.96, mixed = 0.94, light = 0.95, P < 0.0001, Table 2 and Supplementary Figure 2) and the mean difference between treatments was 0.03°C. The eggs were incubated at the natural humidity of the substrate and had a maximum difference of less than 1% between substrate types, and therefore were considered to be within a similar range (Patino-Martinez et al., 2014). The natural humidity of the sand was 2.4% in dark substrate, 1.5% in mixed, and 2.1% in light substrate.
Temperatures in situ
Mean incubation temperatures differed significantly between sand types (dark, 32.5°C ± 1.08, mixed 32.38°C ± 1.10, and light 30.40°C ± 1.13; KW H2 = 71,999, p = 0.000). Temperatures in light sand were on average 2.2°C cooler than black sand (Table 3). There was a significant sand type × depth interaction for temperatures (GLM, random factor year: substrate type × depth, F = 67,881, p = 0.000). Temperature decreased with increasing depth in all sand types, although not to the same extent (mean decrease every 10 cm: dark = 0.18°C, range = 0.17–0.19; mixed = 0.19, range = 0.13–0.25; light = 0.25, range = 0.19–0.31).
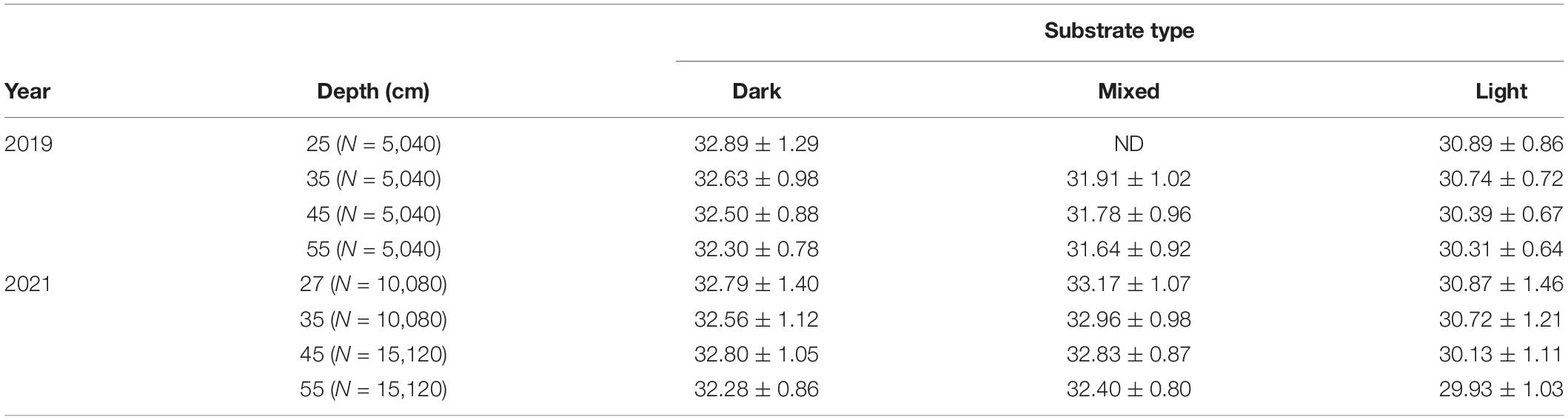
Table 3. Temperatures recorded at four depths (25, 35, 45, and 55 cm) between August 05 and November 17 during two nesting seasons (2019 and 2021), under three different substrate types.
Analysis of Substrates in the Laboratory
The substrates analysed were composed mostly of medium sand (between 98.9 and 99.9%), with little size variability (sorting; Table 4), and with small proportions of mud or gravel (between 0.01 and 0.3%) (Table 4).
The proportion of coarse:fine sand was markedly different, with a of 3.7:23.2% in dark, 7.8:27.4% in mixed, and 22.3:13.7% in light substrate.
The proportion of CO3 was markedly different with a percentage of 17.8 ± 0.4 in dark, 44.8 ± 1.85 in mixed, and 78.5 ± 0.5 in light sand. This indicates that the composition of light-coloured sand was comparatively much higher in grains of biogenic origin (red algal and mollusc meshes predominated; foraminifera, bryozoans, and echinoderms were scarce) (Supplementary Figure 3). Volcanic lithoclasts (clinopyroxenes, Fe and Ti oxides, and the fragments of mafic volcanic rocks and glasses) were major components of the dark-coloured sand (Figure 5 and Supplementary Figure 3).
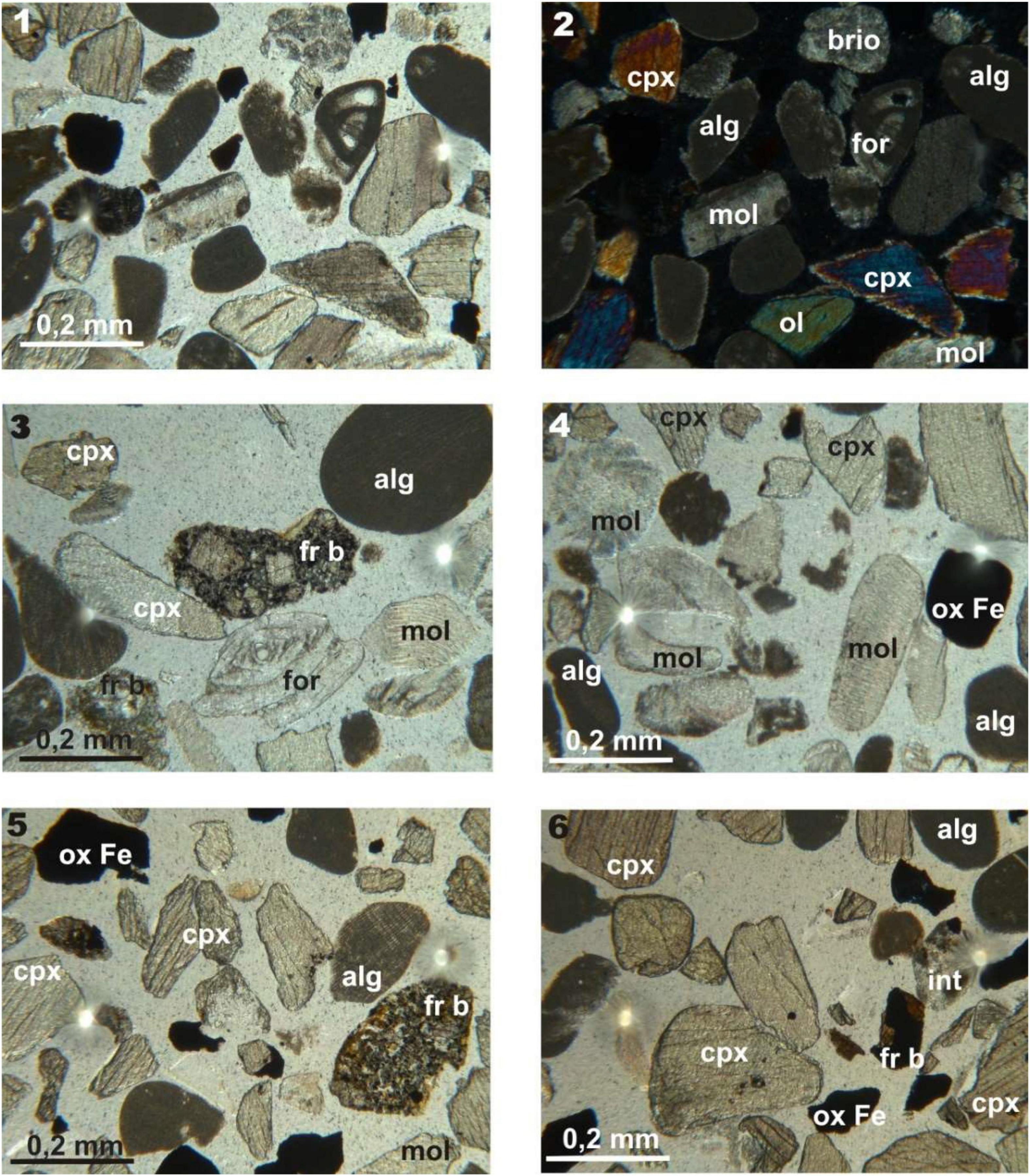
Figure 5. Petrographic microscope photographs at 400× magnification of the samples from the light (1 and 2), mixed (3 and 4), and dark (5 and 6) sands. The difference between polarised light (parallel nicols, Photo 1) and plane light (crossed nicols, Photo 2) is illustrated. Alg, meshes of red algae; mol, molluscs; brio, bryozoans; for, foraminifera; cpx, clinopyroxene; ol, olivine; Fe ox, Fe and Ti oxides; fr g, basalt rock fragment; int, intraclasts.
Discussion
Field and Experimental Study
Through a comprehensive 5-year field (2017–2021) and laboratory study we have demonstrated the effect of the different types of sand selected by loggerhead sea turtle nesting females on their embryonic development, hatching success, and hatchling phenotype. This information is especially relevant for species that depend on the conservation and protection of their nests, such as sea turtles (Casale and Tucker, 2017). Incubation of eggs in dark-coloured, volcanic, and fine-grained sands may have negative effects on loggerhead reproduction. Dark sand caused significantly lower hatching success and thus may lead to reproductive loss of some populations. Embryo death tended to occur at an earlier stage of development in the dark-coloured substrate, whereas in the light-coloured sand embryo death occurred mostly at the end of development. The reason for this difference could be desiccation and late death by dehydration, which has been described in coarser sand substrates (Mortimer, 1990; Warner et al., 2011). However, our experimental results, with controlled temperature and humidity, do not support this theory. Dark-coloured substrates have been associated with negative impacts for embryo survival by direct association with high incubation temperatures (Weber et al., 2012; Martins et al., 2020). Interestingly, divergence in the thermal tolerance of female green turtles with philopatry to both dark and light-coloured beaches has also been demonstrated (Weber et al., 2012). Here we have demonstrated experimentally at controlled temperatures that an inherent effect of the substrate determines differences in embryonic development, hatching success, and hatchling performance regardless of temperature. The fundamental differences between the substrates analysed, other than their colour, were the grain size and the composition of calcium carbonate (different origins; dark substrate from volcanic material and light substrate from biogenic material). A higher fraction of fine sand, as in our dark coloured substrates, could hinder gas diffusion and generate lethal hypoxia during embryonic development. On the other hand, the lower percentage of calcium carbonate in this type of sand generates questions about the optimal composition for sea turtle nest incubation substrates. It is possible that the volcanic substrate composition itself may cause dehydration, leading to the death of the embryos (Marco et al., 2017). The mechanism responsible for this lower hatching success and the association with biotic factors linked to each substrate, e.g., microflora, is still under study for sea turtles (Elshafie et al., 2007).
Adaptive Substrate Selection
The incubation substrate on the island of Maio is dynamic, varies temporally and spatially, and consequently it would be difficult for females to choose a specific substrate before their arrival to the beach. The results of this study suggest that females select all sand types for nesting without preference, as no significant difference was found in either nesting success or nest density between beaches with different substrates. Nesting success indicates the number of nesting attempts on the beach that culminate in the effective laying of a nest (there is a proportion of unsuccessful attempts). Nesting success was surprisingly very similar for the different substrates (56% on dark sand, 56% on mixed sand, and 57% on nearby beaches light sand). This suggests that the abandonment of the laying process is not due to the type of substrate present on the beach chosen by the female. Other factors, not sand type, appear to be more important determinants for the choice of the laying site (Wood and Bjorndal, 2000). Therefore, extreme philopatric adaptation to one type of substrate or beach seems unlikely for loggerhead turtles in Maio. The most plausible adaptive mechanism to ensure the survival of some of their embryos seems to be the selection of different nesting beaches for different clutches, with their inherent differences in substrate type, to spread the risk of nest failure (Patino-Martinez et al., in Press).
Hatchling Phenotype and Performance
We found inter-substrate differences in hatchling biometrics and performance. Hatchlings from dark substrates had smaller carapace dimensions than hatchlings from light sand, suggesting that more yolk was converted to hatchling tissue (Ischer et al., 2009) during embryonic development in light sand nests. The relationship between substrate type and hatchling survival may be complex. Previous work has suggested that larger, more vigorous offspring may be better prepared for survival than smaller, weaker offspring (Miller et al., 1987; McGehee, 1990; Packard, 1999; Reece et al., 2002).
Hatchlings from light sand tended to be faster self-righters than hatchlings from dark sand, which could have a further effect on their survival. The amount of time required for a hatchling to self-right is negatively correlated with its physiological condition (Kobayashi et al., 2017). Globally, sea turtle hatchlings are vulnerable to predation by ghost crabs, mammalian predators, and shorebirds (Antworth et al., 2006; Ischer et al., 2009; Marco et al., 2015), and the time of exposure to these predators is inversely related to the ability to self-right when necessary. Assuming a fixed amount of energy to share between embryonic development, nest emergence, beach crawling and swimming offshore (Rusli et al., 2016), those hatchlings that take longer and expend more energy to reach the sea will reduce their subsequent swimming performance (van de Merwe et al., 2013). Interestingly, recent studies found significant correlations between self-righting propensity, crawling speed, and swimming performance in sea turtle hatchlings (Saito et al., 2019; Stewart et al., 2019). It is crucial to have a strong swimming performance once they enter the ocean because of their vulnerability to predation (Pereira et al., 2011; van de Merwe et al., 2013). Therefore, substrates that optimise the performance attributes of sea turtle hatchlings, as light sand did in our study, increase the likelihood of survival in this critical phase of high mortality.
Sand Type and Ghost Crab Abundance
In Maio, about 42% of loggerhead turtle nests suffer from ghost crab predation (Patino-Martinez et al., 2021), making it one of the most important threats to reproductive success on the island, and a strong selection pressure (Skelhorn et al., 2011). In this study, crabs that came to our bait (non-viable eggs from hatched nests, and fish) were of equivalent size and abundance between substrate types. Therefore, if we assume that these two factors determine predation rate, we could conclude that predation risk is similar between substrates. However, here there may be a benefit linked to the dark colour of the hatchlings on dark-coloured substrates where they may be more difficult to detect. One of the anti-predatory traits of some species is to resemble, sometimes almost imperceptibly, the local environment (Skelhorn et al., 2011). On the other hand, the benefit of resembling the environment has been shown to diminish as local prey density increases relative to their camouflage environments (Skelhorn et al., 2011). In this case the decrease in offspring density, resulting from lower hatching success, could decrease predator motivation and provide a density-dependent benefit. Further experimental studies are needed to evaluate predator density and their activity in microhabitats with thermal variability (due to sand type) and assess the effect on nest and hatchling predation.
Incubation Substrates and Climate Change
The Republic of Cabo Verde hosts one of the two largest loggerhead nesting populations in the world (Patino-Martinez et al., 2021), hosting up to 100,000 nests per season during the period of this study. All of the islands in Cabo Verde have some proportion of nesting beaches with dark-coloured sand (Tanner et al., 2019). Assuming an equal distribution, we estimate that 10% of nests currently show a strong decrease in hatching success due to dark incubation substrates. Under probable emission scenarios, lethal temperatures in dark incubation substrates on the islands will be reached before 2100 (Tanner et al., 2019). Furthermore, dark-coloured substrates, and therefore higher incubation temperatures, will only support the globally female-biassed hatchling sex ratios due to anthropogenic global warming (Hays et al., 2014).
Eggs incubated in light-coloured, biogenic substrates with high carbonate levels, larger grain size and lower temperatures are more successful than those on other substrates. They also experience better embryonic development and produce hatchlings in better physical condition. Therefore, in spatially heterogeneous environments, such as the island of Maio, sea turtles may be exposed to contrasting selective regimes that may cause divergence in traits affecting survival and reproduction (Mickelson and Downie, 2010). For example, given predictions of future climate change, adaptive variation in enhanced heat tolerance may have important adaptive consequences in an increasingly warmer world (Weber et al., 2012). Many conservation programmes consider the translocation of turtle nests on or between beaches, or to hatcheries, as a useful technique. In the face of foreseeable climate warming scenarios, we propose adaptive management of nests using light-coloured substrates, and at depths slightly deeper than the natural average (for a further decrease in incubation temperature). The aim is to favour a higher primary production of males, which can ideally become reproductive adults and to favour a future colonisation of new, thermally appropriate nesting areas, as an adaptive mechanism.
Conclusion
This study investigates (i) the selection of different incubation substrates by loggerhead turtle nesting females and (ii) the reproductive effects associated with incubation. We provide convincing evidence that the benefit of incubation on light-coloured, biogenic-derived sands is greater than on dark-coloured, and volcanic-derived sands. Our results strongly suggest that greater embryonic development and hatching success (a direct measure of fitness), with larger and more physically fit hatchlings (a possible determinant of early survival), implies a reproductive advantage. We recommend replicating this study with other sea turtle species and in different ecosystems. The effect of substrate on predator activity and the intrinsic components of substrate that affect embryonic development are aspects that we suggest investigating in the future. We propose an adaptive management technique of nest incubation on light-coloured substrates (beach or hatchery) and at a greater depth, to favour hatching success, hatchling quality, and to slightly lower incubation temperatures to help mitigate the effects of feminisation linked to global warming.
Data Availability Statement
The raw data supporting the conclusions of this article will be made available by the authors, without undue reservation.
Ethics Statement
Ethical review and approval was not required for the animal study because this research submitted to Frontiers in Ecology and Evolution comply with the journal’s Code of Conduct for authors contributing articles. This study does not involve human subjects, experimentation with animals, and/or collection of specimens. All necessary DNA (Direcçao Nacional do Ambiente) authorisations were received. The animal experimentation standards used to protect the species were followed.
Author Contributions
JP-M: conceptualization, writing-original draft, and supervision. JP-M, JV, IA, KY, JM-V, and GC: methodology and writing-review and editing. JP-M, JV, JM-V, and IA: formal analysis. JP-M, JV, IA, KY, and GC: investigation. JM-V: lab analysis. JP-M, JV, and GC: project administration. All authors contributed to the article and approved the submitted version.
Funding
Financial support came from MAVA Fondation pour la Nature and US Fish and Wildlife Service.
Conflict of Interest
The authors declare that the research was conducted in the absence of any commercial or financial relationships that could be construed as a potential conflict of interest.
Publisher’s Note
All claims expressed in this article are solely those of the authors and do not necessarily represent those of their affiliated organizations, or those of the publisher, the editors and the reviewers. Any product that may be evaluated in this article, or claim that may be made by its manufacturer, is not guaranteed or endorsed by the publisher.
Acknowledgments
We would like to thank the FMB local rangers, team leaders, supervisors, and volunteers for their continuing efforts to collect important data. We also thank the environmental national authority, DNA (Direcçao Nacional do Ambiente), for authorisation and their assistance. The manuscript was improved by the review of Matthew Godfrey, Samir Harshad Patel and the editor J. Sean Doody.
Supplementary Material
The Supplementary Material for this article can be found online at: https://www.frontiersin.org/articles/10.3389/fevo.2022.823118/full#supplementary-material
Footnotes
References
Ackerman, R. A. (1997). “The nest environment and embryonic development of sea turtles,” in The Biology of Sea Turtles, eds P. L. Lutz and J. A. Musick (Boca Raton, FL: CRC Press).
Antworth, R. L., Pike, D. A., and Stiner, J. C. (2006). Nesting ecology, current status, and conservation of sea turtles on an uninhabited beach in Florida. USA. Biol. Conserv. 130, 10–15. doi: 10.1016/j.biocon.2005.11.028
Baltazar-Soares, M., Klein, J. D., Correia, S. M., Reischig, T., Taxonera, A., Roque, S. M., et al. (2020). Distribution of genetic diversity reveals colonization patterns and philopatry of the loggerhead sea turtles across geographic scales. Sci. Rep. 10:18001. doi: 10.1038/s41598-020-74141-6
Bilinski, J. J., Reina, R. D., Spotila, J. R., and Paladino, F. V. (2001). The effects of nest environment on calcium mobilization by leatherback turtle embryos (Dermochelys coriacea) during development. Comp. Biochem. Physiol. Mol. Integr. Physiol. 130, 151–162. doi: 10.1016/s1095-6433(01)00374-9
Blott, S. J., and Pye, K. (2001). GRADISTAT: a grain size distribution and statistics package for the analysis of unconsolidated sediments. Earth Surf. Process. Landf. 26, 1237–1248. doi: 10.1002/esp.261
Campbell, M. (2019). Installing RStudio. in: Learn RStudio IDE. Berlin: Springer. Database Name 2015.
Casale, P., and Marco, A. (2015). Caretta Caretta (North East Atlantic Subpopuulation). The IUCN Red List of Threatened Species. T83776383A83776554. doi: 10.2305/IUCN.UK.2015-4.RLTS.T83776383A83776554.en (accessed January 24, 2020).
Casale, P., and Tucker, A. D. (2017). Caretta Caretta (Amended version of 2015 Assessment). The IUCN Red List of Threatened Species 2017: e.T3897A119333622. doi: 10.2305/IUCN.UK.2017-2.RLTS.T3897A119333622.en (accessed May 31, 2020).
Chen, C.-L., Wang, C.-C., and Cheng, I. J. (2010). Effects of biotic and abiotic factors on the oxygen content of green sea turtle nests during embryogenesis. J. Comp. Physiol. B-Biochem. Systemic Environ. Physiol. 180, 1045–1055. doi: 10.1007/s00360-010-0479-5
Chen, H.-C., Cheng, I.-J., and Hong, E. J. (2007). The influence of the beach environment on the digging success and nest site distribution of the green turtle, Chelonia mydas, on Wan-an Island, Penghu Archipelago, Taiwan. J. Coastal Res. 23, 1277–1286. doi: 10.2112/05-0455.1
Conrad, J. R., Wyneken, J., Garner, J. A., and Garner, S. (2011). Experimental study of dune vegetation impact and control on leatherback sea turtle Dermochelys coriacea nests. Endanger. Spec. Res. 15, 13–27. doi: 10.3354/esr00361
Ditmer, M. A., and Stapleton, S. P. (2012). Factors affecting hatch success of hawksbill sea turtles on long island, antigua, west indies. PLoS One 7:e38472. doi: 10.1371/journal.pone.0038472
Doody, J. S. (2011). Environmentally cued hatching in reptiles. Integr. Comp. Biol. 51, 49–61. doi: 10.1093/icb/icr043
Elshafie, A., Al-Bahry, S. N., Alkindi, A. Y., Ba-Omar, T., and Mahmoud, I. (2007). Mycoflora and aflatoxins in soil, eggshells, and failed eggs of Chelonia mydas at Ras Al-Jinz. Oman. Chelonian Conserv. Biol. 6, 267–270. doi: 10.2744/1071-8443(2007)6[267:maaise]2.0.co;2
Fan, Z., Herrick, J. E., Saltzman, R., Matteis, C., Yudina, A., Nocella, N., et al. (2017). Measurement of soil color: a comparison between smartphone camera and the munsell color charts. Soil. Sci. Soc. Am. J. 81, 1139–1146. doi: 10.2136/sssaj2017.01.0009
Folk, R. L., and Ward, W. C. (1957). Brazos River bar [Texas]; a study in the significance of grain size parameters. J. Sediment Petrol. 27, 3–26.
Hawkes, L. A., Broderick, A. C., Godfrey, M. H., and Godley, B. J. (2010). Climate change and marine turtles. Endanger. Spec. Res. 7, 137–154. doi: 10.1016/S0065-2881(09)56002-6
Hays, G. C., Ashworth, J. S., Barnsley, M. J., Broderick, A. C., Emery, D. R., Godley, B. J., et al. (2001). The importance of sand albedo for the thermal conditions on sea turtle nesting beaches. Oikos 93, 87–94.
Hays, G. C., Mazaris, A. D., and Schofield, G. J. (2014). Different male vs. female breeding periodicity helps mitigate offspring sex ratio skews in sea turtles. Front. Mar. Sci. 1:43. doi: 10.3389/fmars.2014.00043
Ischer, T., Ireland, K., and Booth, D. T. (2009). Locomotion performance of green turtle hatchlings from the Heron Island Rookery, Great Barrier Reef. Mar. Biol. 156, 1399–1409.
Jensen, M. P., Allen, C. D., Eguchi, T., Bell, I. P., LaCasella, E. L., Hilton, W. A., et al. (2018). Environmental warming and feminization of one of the largest sea turtle populations in the world. Curr. Biol. 28, 154–159.e4. doi: 10.1016/j.cub.2017.11.057
Kobayashi, S., Wada, M., Fujimoto, R., Kumazawa, Y., Arai, K., Watanabe, G., et al. (2017). The effects of nest incubation temperature on embryos and hatchlings of the loggerhead sea turtle: implications of sex difference for survival rates during early life stages. J. Exp. Mar. Biol. Ecol. 486, 274–281.
Laloë, J. O., Cozens, J., Renom, B., Taxonera, A., and Hays, G. C. (2017). Climate change and temperature-linked hatchling mortality at a globally important sea turtle nesting site. Glob. Change Biol. 23, 4922–4931. doi: 10.1111/gcb.13765
Lamas, F., Irigaray, C., Oteo, C., and Chacon, J. (2005). Selection of the most appropriate method to determine the carbonate content for engineering purposes with particular regard to marls. Eng. Geol. 81, 32–41.
Marco, A., Abella, E., Martins, S., López, O., and Patino-Martinez, J. (2018). Female nesting behaviour affects hatchling survival and sex ratio in the loggerhead sea turtle: implications for conservation programmes. Ethol. Ecol. Evol. 30, 141–155.
Marco, A., Abella-Perez, E., and Tiwari, M. J. (2017). Vulnerability of loggerhead turtle eggs to the presence of clay and silt on nesting beaches. J. Exp. Marine Biol. 486, 195–203.
Marco, A., da Graça, J., García-Cerdá, R., Abella, E., and Freitas, R. (2015). Patterns and intensity of ghost crab predation on the nests of an important endangered loggerhead turtle population. J. Exp. Mar. Biol. Ecol. 468, 74–82.
Martins, S., Silva, E., Abella, E., Loureiro, N. S., and Marco, A. (2020). Warmer incubation temperature influences sea turtle survival and nullifies the benefit of a female-biased sex ratio. Climatic Change 163, 689–704.
McGehee, M. A. (1990). Effects of moisture on eggs and hatchlings of loggerhead sea-turtles (Caretta-Caretta). Herpetologica 46, 251–258.
Mickelson, L. E., and Downie, J. R. (2010). Influence of incubation temperature on morphology and locomotion performance of Leatherback (Dermochelys coriacea) hatchlings. Canadian J. Zoology-Revue Canadienne Zool. 88, 359–368.
Miller, K., Packard, G. C., and Packard, M. J. (1987). Hydric conditions during incubation influence locomotor performance of hatchling snapping Turtles. J. Exp. Biol. 127, 401–412.
Monzon-Arguello, C., Rico, C., Naro-Maciel, E., Varo-Cruz, N., Lopez, P., Marco, A., et al. (2010). Population structure and conservation implications for the loggerhead sea turtle of the Cape Verde Islands. Conserv. Genet. 11, 1871–1884.
Mortimer, J. A. (1990). The influence of beach sand characteristics on the nesting-behavior and clutch survival of Green Turtles (Chelonia-Mydas). Copeia 19, 802–817. doi: 10.2307/1446446
Packard, G. C. (1999). Water relations of chelonian eggs and embryos: is wetter better? Am. Zool. 39, 289–303. doi: 10.1093/icb/39.2.289
Patino-Martinez, J., Dos Passos, L., Afonso, I. O., Teixidor, A., Tiwari, M., Székely, T., et al. (2021). Globally important refuge for the loggerhead sea turtle: Maio Island. Cabo Verde. Oryx 56, 54–62. doi: 10.1017/S0030605320001180
Patino-Martinez, J., Dos Passos, L., Amador, R., Teixidor, A., Cardoso, S., Marco, A., et al. (in press). Strategic nest site selection in one of the world’s largest loggerhead turtle nesting colonies, Maio Island, Cabo Verde. Oryx.
Patino-Martinez, J., Marco, A., Quiñones, L., and Hawkes, L. A. (2014). The potential future influence of sea level rise on leatherback turtle nests. J. Exp. Mar. Biol. Ecol. 461, 116–123.
Patrício, A. R., Varela, M. R., Barbosa, C., Broderick, A. C., Airaud, M. B. F., Godley, B. J., et al. (2018). Nest site selection repeatability of green turtles, Chelonia mydas, and consequences for offspring. Anim Behav. 139, 91–102. doi: 10.1016/j.anbehav.2018.03.006
Pereira, C. M., Booth, D. T., and Limpus, C. J. (2011). Locomotor activity during the frenzy swim: analysing early swimming behaviour in hatchling sea turtles. J. Exp. Biol. 214, 3972–3976. doi: 10.1242/jeb.061747
Reece, S. E., Broderick, A. C., Godley, B. J., and West, S. A. (2002). The effects of incubation environment, sex and pedigree on the hatchling phenotype in a natural population of loggerhead turtles. Evol. Ecol. Res. 4, 737–748.
Rusli, M. U., Booth, D. T., and Joseph, J. J. (2016). Synchronous activity lowers the energetic cost of nest escape for sea turtle hatchlings. J. Exp. Biol. 219, 1505–1513. doi: 10.1242/jeb.134742
Saito, T., Wada, M., Fujimoto, R., Kobayashi, S., and Kumazawa, Y. (2019). Effects of sand type on hatch, emergence, and locomotor performance in loggerhead turtle hatchlings. J. Exp. Mar. Biol. Ecol. 511, 54–59.
Salleh, S. M., Nishizawa, H., Ishihara, T., Sah, S. A. M., and Chowdhury, A. J. (2018). Importance of sand particle size and temperature for nesting success of green turtles in Penang Island. Malaysia. Chelonian Conserv. Biol. 17, 116–122.
Skelhorn, J., Rowland, H. M., Delf, J., Speed, M. P., and Ruxton, G. D. (2011). Density-dependent predation influences the evolution and behavior of masquerading prey. Proc. Natl. Acad. Sci. U S A. 108, 6532–6536. doi: 10.1073/pnas.1014629108
Stewart, T. A., Booth, D. T., and Rusli, M. U. (2019). Influence of sand grain size and nest microenvironment on incubation success, hatchling morphology and locomotion performance of green turtles (Chelonia mydas) at the Chagar Hutang Turtle Sanctuary, Redang Island, Malaysia. Australian J. Zool. 66, 356–368.
Stiebens, V. A., Merino, S. E., Roder, C., Chain, F. J. J., Lee, P. L. M., and Eizaguirre, C. (2013). Living on the edge: how philopatry maintains adaptive potential. Proc. R. Soc. B-Biol. Sci. 22:280. doi: 10.1098/rspb.2013.0305
Tanner, C., Marco, A., Martins, S., Perez, E. A., and Hawkes, L. (2019). Highly feminised sex ratio estimations for the world’s third largest nesting aggregation of the loggerhead sea turtle. Mar. Ecol. Prog. Series (MPES) 621, 209–219.
van de Merwe, J. P., Ibrahim, K., and Whittier, J. M. (2013). Post-emergence handling of green turtle hatchlings: improving hatchery management worldwide. Animal Conserv. 16, 316–323.
Wallace, B. P., DiMatteo, A. D., Hurley, B. J., Finkbeiner, E. M., Bolten, A. B., Chaloupka, M. Y., et al. (2010). Regional management units for marine turtles: a novel framework for prioritizing conservation and research across multiple scales. PLoS One 5:e15465. doi: 10.1371/journal.pone.0015465
Wallace, B. P., Sotherland, P. R., Spotila, J. R., Reina, R. D., Franks, B. F., and Paladino, F. V. (2004). Biotic and abiotic factors affect the nest environment of embryonic leatherback turtles, Dermochelys coriacea. Physiol. Biochem. Zool. 77, 423–432. doi: 10.1086/420951
Warner, D. A., Moody, M. A., and Telemeco, R. S. (2011). Is water uptake by reptilian eggs regulated by physiological processes of embryos or a passive hydraulic response to developmental environments? Comp. Biochem. Physiol. Mol. Integr. Physiol. 160, 421–425. doi: 10.1016/j.cbpa.2011.07.013
Weber, S. B., Broderick, A. C., Groothuis, T. G. G., Ellick, J., Godley, B. J., and Blount, J. D. (2012). Fine-scale thermal adaptation in a green turtle nesting population. Proc. R. Soc. B-Biol. Sci. 279, 1077–1084. doi: 10.1098/rspb.2011.1238
Wentworth, C. K. (1922). A scale of grade and class terms for clastic sediments. J. Geol. 30, 377–392.
Wood, D. W., and Bjorndal, K. A. (2000). Relation of temperature, moisture, salinity, and slope to nest site selection in Loggerhead Sea Turtles. Copeia 1, 119–128.
Keywords: egg incubation, Maio Island, sea turtles, incubation substrate, (Caretta caretta), conservation, global warming, climate change
Citation: Patino-Martinez J, Veiga J, Afonso IO, Yeoman K, Mangas-Viñuela J and Charles G (2022) Light Sandy Beaches Favour Hatching Success and Best Hatchling Phenotype of Loggerhead Turtles. Front. Ecol. Evol. 10:823118. doi: 10.3389/fevo.2022.823118
Received: 26 November 2021; Accepted: 28 February 2022;
Published: 25 March 2022.
Edited by:
J. Sean Doody, University of South Florida, United StatesReviewed by:
Samir Harshad Patel, Coonamessett Farm Foundation, United StatesMatthew H. Godfrey, North Carolina Wildlife Resources Commission, United States
Copyright © 2022 Patino-Martinez, Veiga, Afonso, Yeoman, Mangas-Viñuela and Charles. This is an open-access article distributed under the terms of the Creative Commons Attribution License (CC BY). The use, distribution or reproduction in other forums is permitted, provided the original author(s) and the copyright owner(s) are credited and that the original publication in this journal is cited, in accordance with accepted academic practice. No use, distribution or reproduction is permitted which does not comply with these terms.
*Correspondence: Juan Patino-Martinez, anVhbnBhdGlub21AZ21haWwuY29t