- 1Programa de Pós-Graduação em Ciências do Ambiente, Universidade Federal do Tocantins, Palmas, Brazil
- 2Coordenação de Zoologia, Museu Paraense Emílio Goeldi, Belém, Brazil
- 3Coordenação Geral de Projetos, Fundação Ecológica Cristalino, Alta Floresta, Brazil
- 4Programa de Pós-Graduação em Geografia, Universidade Federal do Tocantins, Porto Nacional, Brazil
- 5Instituto Tecnológico Vale, Belém, Brazil
- 6Finnish Museum of Natural History, University of Helsinki, Helsinki, Finland
Few phylogeographic studies have focused on understanding the role of the Tocantins–Araguaia Interfluve (TAI) in the diversification of Amazonian biodiversity. Herein we evaluate the phylogeographic relationships of 14 avian lineages present in the TAI and its two neighboring Amazonian Areas of Endemism: the Xingu (XAE) and Belém (BAE). Four alternative scenarios coupling degree of genetic differentiation and area relationships were tested: (1) populations distributed in TAI, BAE, and XAE are not genetically differentiated from each other (assumed as the null hypothesis); (2) populations from TAI are more closely related to those from BAE; (3) populations from TAI are more closely related to those from XAE; and (4) TAI populations represent independent or endemic lineages not present in either the BAE or XAE. Molecular analyses considered Bayesian Inference methods and Bayesian Information Criterion (BIC) evolutionary models, haplotype sharing and genetic differentiation estimates. We found three distinct phylogeographic patterns: (i) four lineages presented no population structure across XAE, TAI and BAE; (ii) six lineages were represented in the TAI with distinct phylogroups replacing each other between XAE and BAE, but with varying degrees of contact and evidence of gene-flow within the TAI; and (iii) for four lineages, the Tocantins River acted as a barrier isolating BAE phylogroups from those inhabiting both TAI and XAE. These different patterns demonstrate a heterogeneous response to the barrier effects posed by both the Tocantins and Araguaia rivers on the local fauna. Historical geomorphological and hydromorphological factors, such as the presence and absence of paleochannels and anastomosed channel stretches and variations in sedimentation rates support a dynamic history for the Araguaia-Tocantins floodplains, likely accounting for the observed heterogeneity in species’ specific responses. Finally, the scenario of phylogeographic breaks and population subdivision recovered herein along the Tocantins and Araguaia rivers, associated with the existence of contact zones and the occurrence of gene flow, define the TAI as hitherto unknown biogeographic suture zone, localized in southeasternmost Amazonia.
Introduction
Biotic diversification in the Amazon is the result of profound morpho-geo-climatic changes in time and space, promoting geographic isolation of populations and species formation (Haffer, 1969, 2008; Cracraft, 1985; Antonelli et al., 2010; Hoorn et al., 2010). These different diversification processes have been associated with one or more scenarios such as those predicted by the refuge (Haffer, 1969, 1992), river barrier (Wallace, 1853; Sick, 1967; Haffer, 1969, 1992, 2001; Cracraft, 1985), ecological gradients (Smith et al., 2001), and maritime incursion (Bates, 2001) hypotheses. More recently, Hoorn et al. (2010) and Silva et al. (2019) presented proposals integrating two of more of these hypotheses, clearly demonstrating the complexity of diversification scenarios across Amazonia.
Phylogeographic studies have helped define interspecific boundaries and shed light on the spatio-temporal patterns of diversification of several Amazonian taxa (Aleixo, 2002, 2004; Marks et al., 2002; Ribas et al., 2006, 2012; Lavergne et al., 2010; Avila-Pires et al., 2012; Carneiro et al., 2012; Batista et al., 2013; Fouquet et al., 2015; Mercês et al., 2015; Thom and Aleixo, 2015; Araújo-Silva et al., 2017; Sampaio et al., 2018; Capurucho et al., 2020; Dantas et al., 2021). These phylogeographic studies confirmed the effect imposed by major Amazonian rivers, inhibiting gene flow between allopatric populations and closely related species, and hence, delimiting interfluves as areas of endemism or endemism center (Silva et al., 2005; Ribas et al., 2012; Batista et al., 2013; Maldonado-Coelho et al., 2013; Thom and Aleixo, 2015). The phylogeographic pattern determined for the genus Psophia has been a recurrent example to illustrate the areas of endemism in the Amazon (Ribas et al., 2012).
In parallel, the identification of contact zones and hybridization among Amazonian birds has gained prominence with the phylogeographic approach. Comparative phylogeographic studies showed that pairs of selected parapatric taxa in the Tapajós Area of Endemism (TAE), between the Tapajós/Teles Pires and the Xingu rivers, in the central portion of the Amazon, presented some degree of gene flow around the headwaters of these Amazonian tributaries (Weir et al., 2015). Contact and hybridization zones were also identified between pairs of parapatric species in the headwaters of the Negro and Branco rivers, in the Guiana Shield in northern Amazonia (Naka et al., 2012). These phylogeographic studies confirmed the weakening of the isolation effect of major Amazonian rivers in their headwater portions, by facilitating gene flow between populations on opposite banks in these areas (Haffer, 1969, 1992).
Hence, phylogeography has played a fundamental role in understanding the diversification processes of the Amazonian avifauna. However, there are several regional gaps in Amazonian phylogeographic studies, including the Tocantins–Araguaia Interfluve (hereafter TAI). Haffer (1969, 1992) pointed to the lower Tocantins River as a geographic barrier, promoting the isolation of distinct lineages across their opposite banks, as recently corroborated by studies on Pyriglena leuconota (Maldonado-Coelho et al., 2013), Dendrocolaptes certhia (Batista et al., 2013), and Thamnophilus aethiops (Thom and Aleixo, 2015).
However, thus far, the evolutionary relationships of the TAI with other Amazonian areas of endemism remain obscure, with only a few studies sampling intensively populations in this sector of Amazonia (e.g., Hrbek et al., 2014, Rocha et al., 2015). The results found by Naka et al. (2012), Naka and Brumfield (2018), and Weir et al. (2015), in the northern and central regions of the Amazon, respectively, would also suggest a loss in power of any barrier effect exerted by the Tocantins River toward its headwaters, favoring a scenario of gene flow and little genetic differentiation between the TAI and neighboring areas of endemism. However, in contrast to this expectation, Rocha et al. (2015) showed that structured populations of small marsupials exist between the opposite banks of the middle Araguaia River.
To fill in these gaps, here we evaluate the phylogeographic relationships of at least 21 taxa belonging to 17 species of the avifauna of the TAI associated with humid and ecotonal forests occurring in its central-northern portion, with other Amazonian Areas of Endemism, mainly the neighboring Xingu (XAE) and Belém (BAE) (sensu Silva et al., 2005). Considering the relevant role played by major Amazonian rivers as geographic barriers, directly influencing the diversification of neotropical avifauna, we assume that the Araguaia and Tocantins rivers could act in such way, promoting genetic structuring of bird populations within the TAI.
Hence, we tested four alternative predictions concerning comparative levels of genetic differentiation and phylogeographic relationships (Figure 1) among the BAE, TAI, and XAE, as follows: (1) populations distributed in the TAI, BAE, and XAE are not genetically differentiated from each other, assumed as our null hypothesis; (2) populations from TAI are more closely related to those from XAE; (3) populations from TAI are more closely related to those from BAE; and (4) TAI populations represent genetically differentiated lineages not shared with the BAE and XAE. These hypotheses examine the possibility of the Tocantins and Araguaia rivers acting as geographical barriers to gene flow and the role of TAI as a contact, hybridization and introgression zone, or even as a new area of endemism for the Amazon. In addition, geological features of the Araguaia and Tocantins rivers are considered to support the discussion on how both rivers have influenced patterns of gene flow between lineages from their opposite banks.
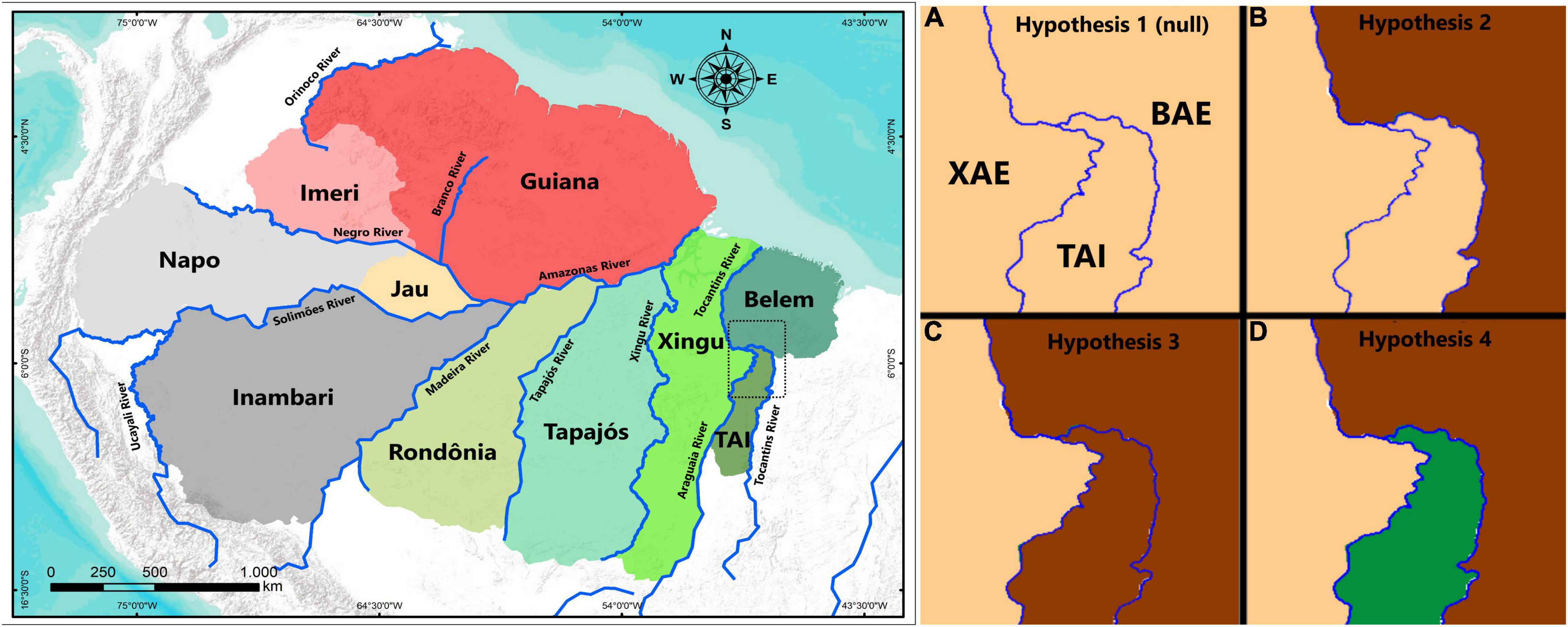
Figure 1. Left: Amazonian areas of endemism according Silva et al. (2019), with the TAI shown in southeasternmost Amazonia. Right and clockwise: frames (A–D) of the four hypotheses of expected phylogeographic patterns for the TAI and neighboring areas (Belém Area of Endemism – BAE and Xingu Area of Endemism – XAE). (A) Hypothesis 1, all three areas are inhabited by genetically undifferentiated populations (assumed as the null hypothesis). (B) Hypothesis 2, TAI and XAE are inhabited by sister populations to the exclusion of BAE populations. (C) Hypothesis 3, TAI and BAE are inhabited by sister populations to the exclusion of XAE populations. (D) Hypothesis 4, TAI populations are inhabited by reciprocally monophyletic populations with respect to those in both BAE and XAE.
Materials and Methods
Study Area
The TAI is located in southeasternmost Amazonian Brazil, inserted predominantly in the limits of the state of Tocantins (Figure 1). It is characterized as an ecotonal region with two morpho-phyto-climatic domains, the Cerrado and the Amazon (Ab’sáber, 2003; Instituto Brasileiro de Geografia e Estatística [IBGE], 2019). The western and northern regions of the TAI harbor a predominantly Amazonian avifauna, while the eastern, southeastern and central portions support an avifauna typical of the Cerrado biome (Dornas et al., in press). Together, these regions present Amazonian forest formations characterized by tropical and ecotonal forests (i.e., forest enclaves surrounded by seasonal semideciduous forests) and savannah vegetation including the cerrado sensu stricto and cerradão phytophysiognomies (Secretaria e Planejamento do Estado do Tocantins [Seplan], 2012; Haidar et al., 2013; Marques et al., 2020).
Selection of Taxa
To detect broad patterns of avian taxa replacement across the TAI and neighboring interfluves (BAE and XAE) and select the taxa to be sampled in this study, we mapped localities and assessed subspecific taxonomies of specimens from these areas deposited at the Fernando C. Novaes Ornithological Collection at the Museu Paraense Emílio Goeldi (hereafter MPEG), as well as those obtained from the literature. For the BAE, the following references were considered: Roma (1996), Vasconcelos et al. (2007), Novaes and Lima (2009), Portes et al. (2011), Oren and Roma (2012), Lees et al. (2012), Henriques et al. (2021) and the Wikiaves portal.1 For the XAE, the following references were consulted: Graves and Zusi (1990), Aleixo et al. (2000), Oliveira et al. (2005), Valente (2006), Pacheco et al. (2007), Vasconcelos et al. (2007), Whittaker (2008); Aleixo et al. (2010), Somenzari et al. (2011), Aleixo et al. (2012), Henriques et al. (2021) and the Wikiaves portal. With respect to the TAI, the compilation made by Dornas et al. (in press) was considered. When reporting on our results, we followed the nomenclature and taxonomy contained in Pacheco et al. (2021), complemented by data on species and subspecies distributions found in the E-Bird portal.2
Our comparisons revealed which taxa are shared among the three different areas considering the following configuration (i) taxa with allopatric distributions replacing each other across the three areas; (ii) taxa with allopatric occurrences replacing each other between BAE/TAI or XAE/TAI; or (iii) taxa overlapping in distribution across all three areas. In all, 54 taxa belonging to 42 different species (of which 16 and 26 were, respectively, monotypic and polytypic) were identified as candidates for an initial screening (Supplementary Table 1). From these, a total of 21 taxa belonging to 17 species (8 monotypic and 9 polytypic) were selected as targets for this study due to tissue availability across all three major interfluves sampled. Together, these taxa comprise a total of 14 lineages, each corresponding to a monotypic/polytypic species or species complex (Table 1). Altogether, a total of 397 genetic samples belonging to these taxa were obtained from ornithological tissue collections (Supplementary Table 2) and sequenced herein.
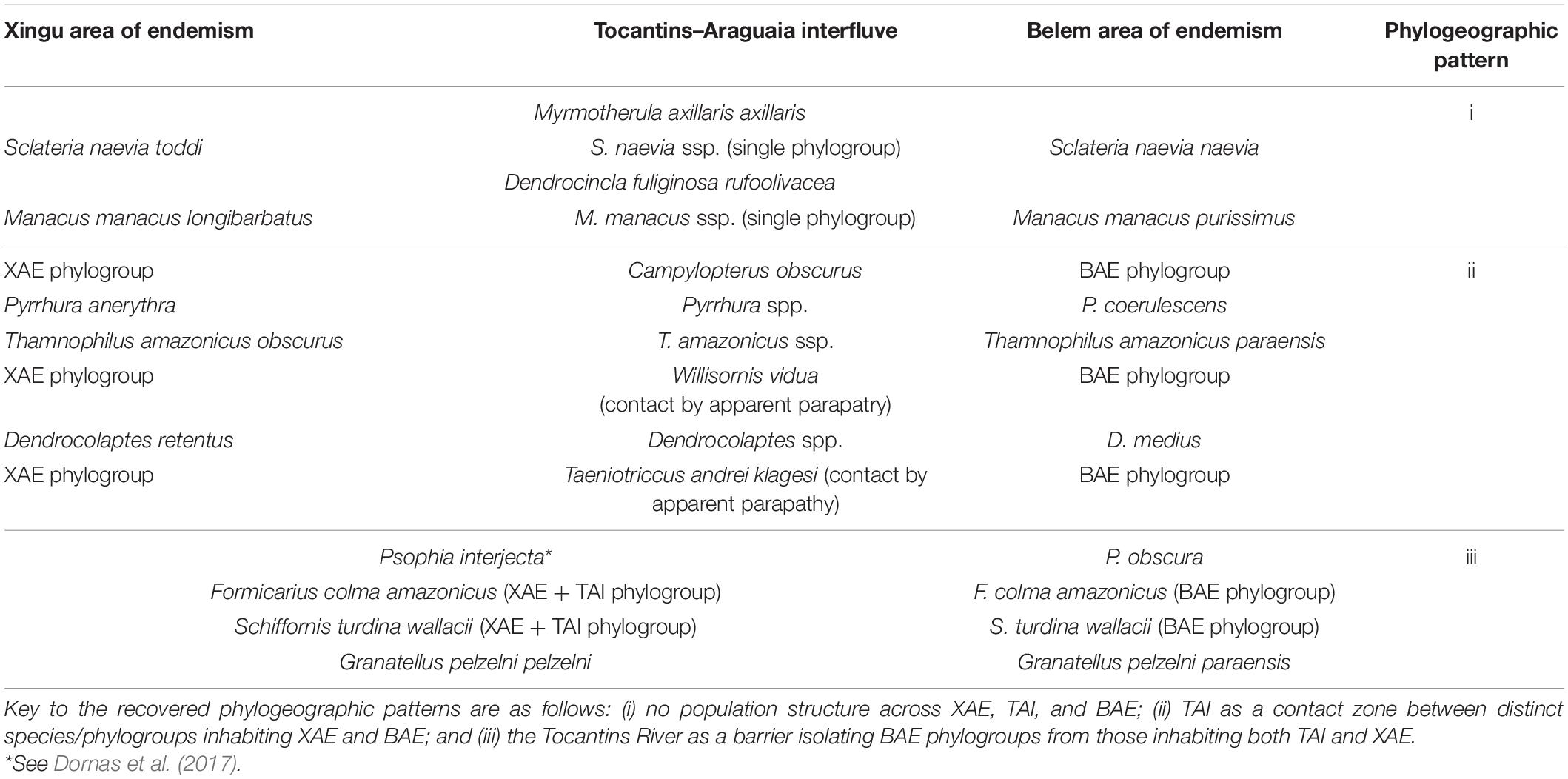
Table 1. List containing 14 avian lineages included in the molecular analyses and the taxon present in each of the major areas sampled in this study.
Molecular Data
The genetic marker chosen for the molecular analyses was the mitochondrial NADH dehydrogenase subunit 2 gene (ND2). In addition to the 397 samples sequenced, another 141 ND2 sequences belonging to the selected species and respective outgroups were obtained from the GenBank database (Supplementary Table 2).
Total DNA was extracted following standard procedures of the phenol-chloroform technique (Sambrook et al., 1989). The amplification of the ND2 gene occurred through the Polymerase Chain Reaction (PCR). Primers used for gene amplification were H6313: CCTTGAAGCACTTCTGGGAATCAGA (Sorenson et al., 1999) and L5215: TATCGGGCCCATACCCCGAAAAT (Hackett, 1996). The total volume of PCR reactions was 25 μL containing: 12.4 μL of Master Mix (50 U/mL, 400 μL of each dNTP and 3 mM of MgCl2), 9.8 μL of ultra-pure water, 0.4 μL of each of the primers (200 ng/μL) and, finally, 2 μL of genomic DNA.
The amplification profile proceeded in the following order: an initial 5 min step at 95°C for block temperature homogenization; followed by 35 cycles of 3 min each, separated by 1 min at 95°C, 1 min at the initiator association temperature for each selected species, and 1 min at 72°C; finally, a final step of 5 min, at 72°C, for the polymerization of eventual molecules, from which the polymerase has dissociated before the end of the total synthesis of the fragment. The association temperatures of the primers of each species were determined by a temperature gradient PCR (from 50 to 63°C). The amplified samples were checked in electrophoresis using a 1% agarose gel and purified following the Polyethylene Glycol (PEG-8000) protocol. The products of the amplifications and purifications were sequenced, directly and automatically, using the Kit-Big Dye Terminator Cycle Sequencing Standard Version 3.1||on the ABI 3130 Sequencer from Applied Biosystems according to the manufacturer’s specifications.
After DNA extraction, amplification and sequencing processes, the sequences were edited and aligned by the ClustalW method using the BioEdit software (Hall, 1999). The saturation in the number of mutations was evaluated through graphs of transitions plotted against transversions as a function of genetic distances using the DAMBE program (Xia and Xie, 2001).
Molecular Analyses
Phylogenetic analyses were carried out using Bayesian Inference (BI), as implemented in the MrBayes 3.1.2 program (Ronquist and Huelsenbeck, 2003). The molecular model of evolution best fitting each dataset was obtained based on the Bayesian Information Criterion (BIC) estimated with JModelTest (Posada, 2008). The BI proceeded with two independent runs of 10,000,000 generations each, with the sampling of one tree every 1,000 generations. The TRACER 1.5 software (Rambaut and Drummond, 2009) was used to verify whether posterior distributions of parameters reached the minimum threshold (ESS >200). Trees obtained before the Markov chain reached stable and convergent likelihood values were discarded. Haplotype networks were estimated for all species with HaploViewer (Salzburger et al., 2011).
The population genetics fixation index Fst was calculated using the Arlequin 3.0 program (Excoffier et al., 2005). Following Wright (1978), Hartl and Clark (2007), and Frankham et al. (2008), Fst values smaller than 0.49 were interpreted as representing low differentiation, between 0.5 and 0.69, moderate differentiation and above 0.7 high genetic differentiation.
Results
The superposition of distributions of the XAE, BAE, and TAI target taxa belonging to the 14 lineages selected for the phylogeographic analyses revealed the following patterns: (1) 7 pairs of taxa replace each other between XAE and BAE, with populations of unknown subspecific identity present in the TAI; and (2) 7 taxa occur indistinctly throughout all three areas (Table 1). Among the 14 lineages selected, the lower Tocantins River was a barrier separating 10 pairs of differentiated phylogroups belonging to thirteen species or species complexes (Table 1). In contrast, only four lineages belonging each to a distinct species did not show signs of geographic structuring across the Tocantins and Araguaia rivers. Below, we describe the results of the phylogeographic analyses in detail.
Phylogeographic Patterns
The phylogenetic and population genetic analyses obtained for the different lineages sampled uncovered three main distinct phylogeographic patterns (Figure 2), as follows: i) no population structure across XAE, TAI, and BAE, found in Mymotherula axillaris, Sclateria naevia, Manacus manacus, and Dendrocincla fuliginosa; (ii) TAI as a contact zone with evidence of gene-flow of lack thereof between distinct species/phylogroups replacing each other in the XAE and BAE, as in Pyrrhura anerythra/Pyrrhura coerulescens, Campylopterus obscurus, Dendrocolaptes medius/Dendrocolaptes retentus, Thamnophilus amazonicus, Willisornis vidua, and Taenotriccus andrei; and (iii) the Tocantins River as the barrier isolating BAE phylogroups from those inhabiting both TAI and XAE, as in Psophia interjecta/Psophia obscura, Formicarius colma, Granatellus pelzelni/Granatellus paraensis, and Schiffornis turdina. We found no support among the sampled taxa for two of our a priori hypotheses; i.e., no TAI lineages/populations grouped as sister to those in the BAE, to the exclusion of XAE phylogroups (as postulated by hypothesis 3), and no reciprocally monophyletic (endemic) phylogroups on the TAI were recovered (as predicted by hypothesis 4).
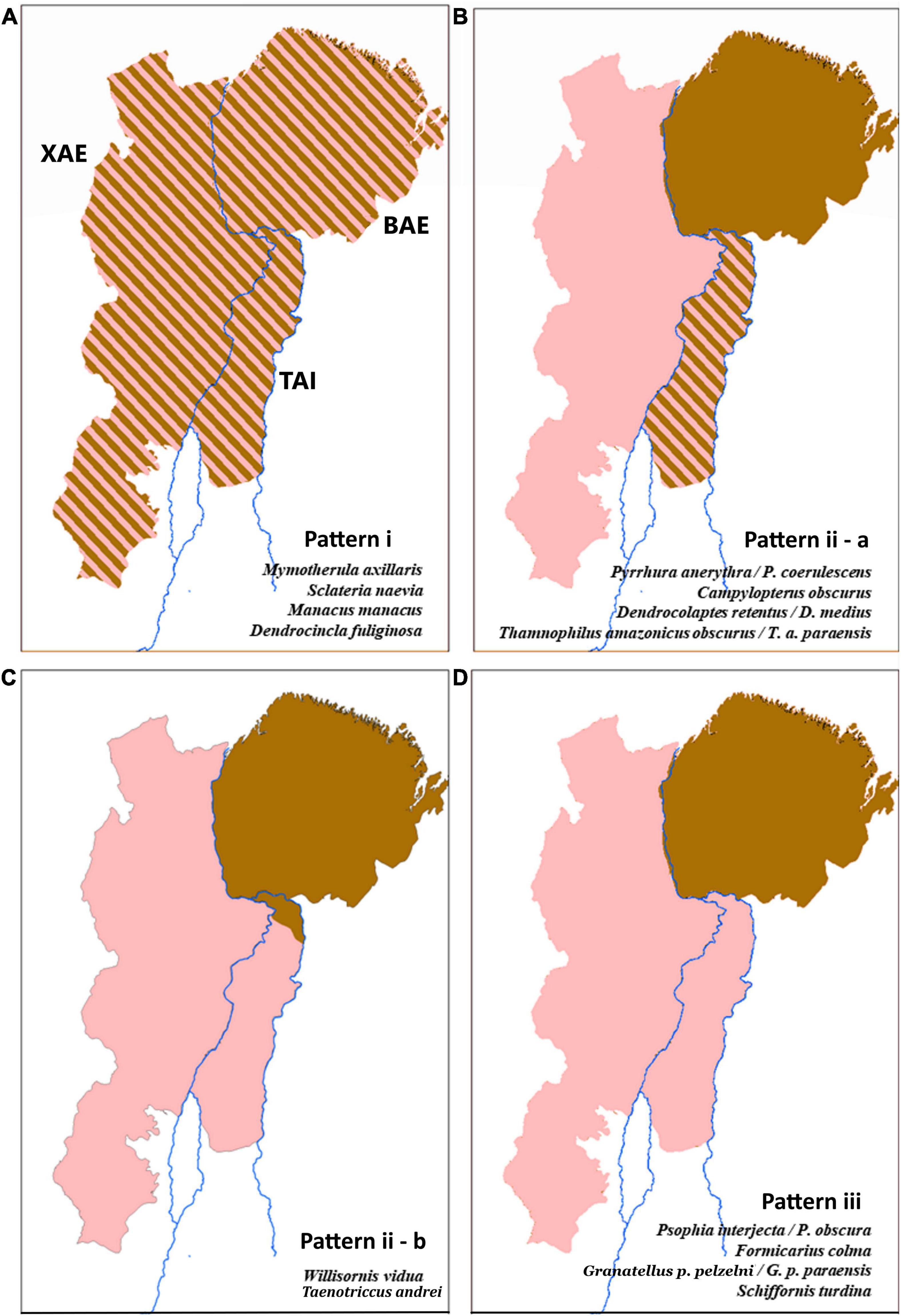
Figure 2. Phylogenetic and haplotype network analyses and Fst indexes revealed three distinct phylogeographic patterns shared by genetically sampled avian lineages in the TAI, as follows: pattern i (A), no population structure across the Xingu Area of Endemism (XAE), TAI, and Belem Area of Endemism (BAE); pattern ii, two clades associated each with XAE and BAE, with TAI populations grouping in both clades, but with two alternative spatial distributions, defined as “a” – TAI as a contact zone with evidence of gene-flow and sympatry between distinct species/phylogroups inhabiting XAE and BAE (B), and “b” – two distinct phylogroups without evidence of gene-flow, associated with XAE and BAE and distributed in a parapatric fashion within the TAI (C); and pattern iii (D), the Tocantins River as the barrier isolating BAE phylogroups from those inhabiting both TAI and XAE. See Supplementary Material for details.
Pattern “i” corroborated hypothesis 1, assumed as the null, that populations distributed in TAI, BAE, and XAE are not genetically differentiated, demonstrating no barrier effect posed by the Araguaia River or the Tocantins River on the gene flow between populations of species present in all sampled areas. The topologies of the phylogenetic trees indicated non-reciprocal monophyly among the TAI and the two neighboring endemic areas (Figure 3) which, added to the absence of population structure indicated by the extensive sharing of haplotypes and reduced Fst values (smaller than 0.49), demonstrated absence of genetic structure, supporting regular levels of gene flow between populations of M. axillaris, S. naevia, M. manacus, and D. fuliginosa across these three different areas (Supplementary Figures 1–4 and Supplementary Table 3). For D. fuliginosa, the absence of genetic differentiation seemed to also include the Tapajós Area of Endemism (TAE), suggesting a lack of phylogeographic structure across southeastern Amazonia as a whole, but strong genetic differentiation with respect to lineages occurring in western Amazonia and the Guiana Shield (see also Schultz et al., 2019).
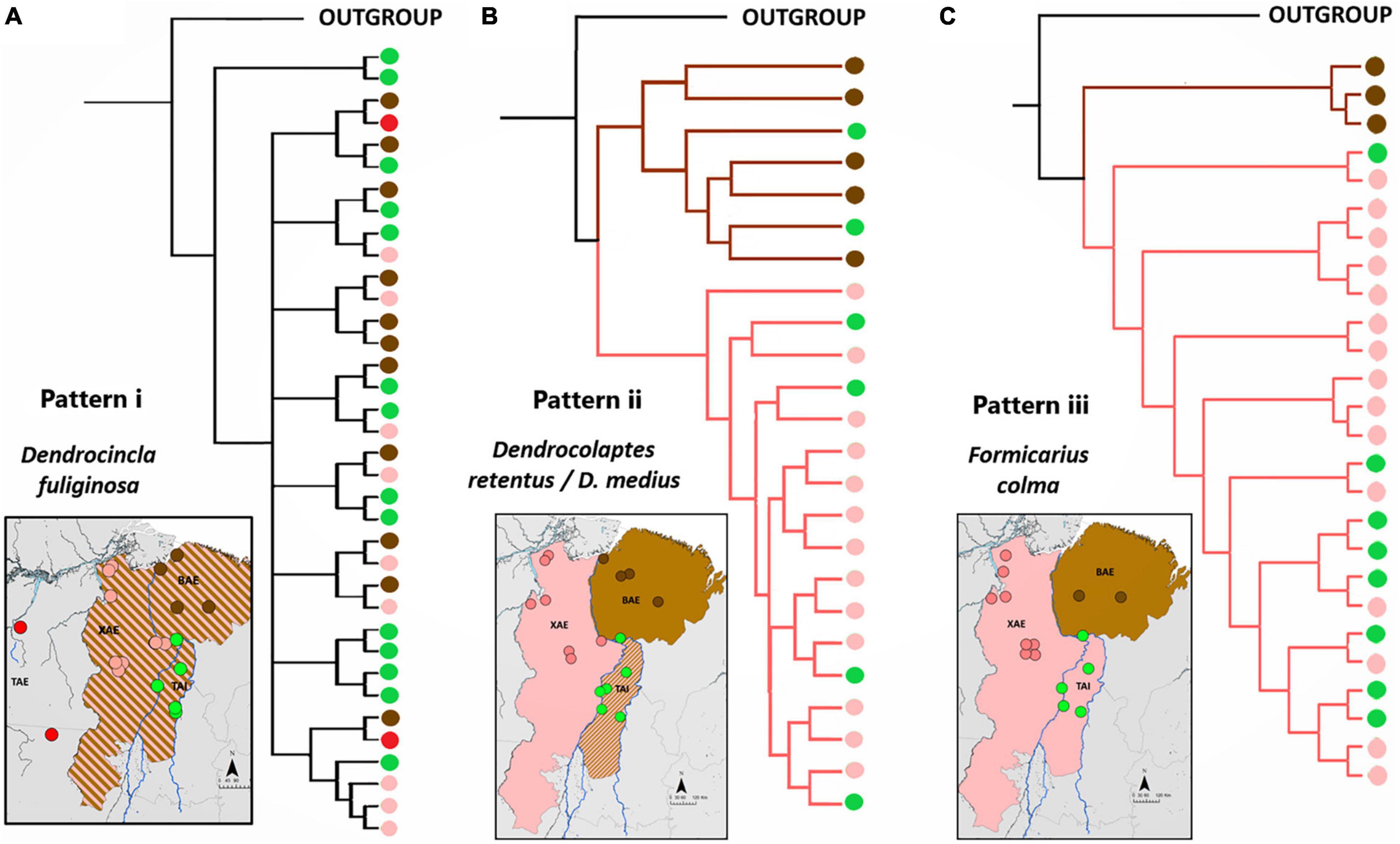
Figure 3. Topologies of phylogenetic trees representative of each of the three phylogeographic patterns recovered among lineages of the sampled taxa inhabiting the XAE, BAE, and TAI. Black circles represent outgroup samples, whereas red, green, pink, and brown circles refer respectively to the TAE, TAI, XAE, and BAE samples. (A) Phylogenetic tree obtained for Dendrocincla fuliginosa illustrating pattern i (null hypothesis), whereby no geographically structured was recovered. (B) Phylogenetic tree of samples of the allopatric Dendrocolaptes retentus (whose distribution is centered in the XAE) and D. medius (distributed mainly in the BAE) illustrating pattern ii, in which the riverine barrier effect is present only in the lower Tocantins River, with the TAI consisting a contact zone between otherwise allopatric lineages replacing each other in the XAE and BAE. (C) Phylogenetic tree recovered for Formicarius colma samples illustrating pattern iii, in which the barrier effect is present along the entire Tocantins River, implying in a sister relationship between XAE and TAI lineages to the exclusion of those in the BAE. See Supplementary Material for details on these and the other sampled species.
For pattern “ii,” the phylogenetic trees presented two statistically well-supported and reciprocally monophyletic clades associated with XAE and BAE, respectively, with TAI populations grouping in both clades (Figure 3 and Supplementary Figures 5–8). The haplotype networks also confirmed this genetic structure, indicating the presence of haplotypes otherwise restricted to both the XAE and BAE in the interior of the TAI (Supplementary Figures 5–9). These phylogeographic results showed, therefore, that there are representatives of both XAE and BAE in the TAI, which constitutes a wide contact zone between pairs of distinct taxa endemic to those areas of endemism. In addition to the contact zones, our phylogenetic trees and haplotypes networks also suggest the existence of hybrid individuals, at least between the closely related species pairs P. anerythra/P. coerulescens and D. retentus/D. medius (Supplementary Figures 5, 6). In other cases, such as in C. obscurus, specimens occurring in syntopy (e.g., AGUA 97 and AGUA 47) belonged to these two distinct mitochondrial clades, reinforcing the TAI as a wide contact zone between otherwise genetically structured populations across the lower Tocantins River, a situation clearly not maintained across the Araguaia River.
In Willisornis vidua, BAE populations clustered in a well-supported clade along with those from the northernmost part of the TAI, whereas XAE specimens did not cluster in a single clade, but with some of them being closely related to several individuals occurring throughout most of the TAI, as also supported by the haplotype networks (Supplementary Figure 9). These two genetically structured W. vidua populations within the TAI are apparently parapatric, and no evidence of widespread gene flow between them has been recovered so far (see Quaresma et al., 2022). Preliminary phylogeographic analyses focusing on T. andrei showed a similar north-south replacement across the TAI involving distinct phylogroups and haplotype network arrangements clustering with the BAE or XAE clades (Supplementary Figure 10). However, as we had access to few samples, this phylogeographic structure was not well supported statistically and could just be a sampling artifact. In this case, it is necessary to increase the sampling of T. andrei.
Pattern “iii” supports the Tocantins River as a barrier separating phylogroups restricted to the TAI and XAE from those in BAE (Figure 3), as predicted by hypothesis 2. The topologies of phylogenetic trees, the sharing of haplotypes and the Fst values recovered for F. colma, G. pelzelni, S. turdina, and P. interjecta/P. obscura are consistent with a stronger barrier effect played by the Tocantins River (Supplementary Figures 11–13 and Supplementary Table 3; see Dornas et al., 2017 for P. interjecta).
Discussion
The determination of phylogeographic patterns in the Amazon involving the sampling of the TAI area has been limited (Hrbek et al., 2014; Rocha et al., 2015) or completely absent (e.g. Aleixo, 2002; Ribas et al., 2006, 2012; Patané et al., 2009; Batista et al., 2013; Rodrigues et al., 2013; Soares et al., 2019). This lack of TAI samples in Amazonian phylogeographic studies precludes postulating any preliminary hypothesis regarding the historical relationships between this eastern Amazon interfluve and other regions.
Here, we assessed for the first time the comparative barrier effects posed by both the Tocantins and Araguaia rivers on 14 avian linages in the southeasternmost Amazonian biota. With the caveat that we sampled only one mitochondrial locus, which is insufficient to estimate accurate rates of gene flow and hybridization, our data showed the TAI as a contact zone between the rather differentiated avifauna of the neighboring BAE and XAE, providing further support to the overall view that the upper reaches of major Amazonian rivers are more permeable to gene flow and faunal exchange than their lowermost parts.
Effect of Geographic Barrier of the Tocantins and Araguaia Rivers
The effect of large Amazonian rivers as primary or secondary geographic barriers to the local avifauna, delimiting the distribution of independent evolutionary lineages among interfluves, is widespread (Wallace, 1853; Sick, 1967; Haffer, 1969, 1992; Naka, 2011; Fernandes et al., 2012; Naka et al., 2012; Ribas et al., 2012; Naka and Brumfield, 2018; Silva et al., 2019). The phylogeographic patterns documented herein demonstrated that TAI lineages are closely related to those in the neighboring XAE and BAE. However, the different patterns of genetic diversity and area relationships found revealed that taxa have had distinct evolutionary histories in the TAI.
Pattern “I,” consistent with the assumed null hypothesis, given the expected outcome of a lack of genetic differentiation in the absence of a river-barrier effect, was shared by four species and indicated an absence of genetic structuring between TAI, XAE, and BAE. This supports a lack of barrier effects imposed by the Araguaia and Tocantins rivers, consistent with gene flow between populations of the sampled lineages across the TAI, XAE, and BAE (Figure 2). Natural history attributes of the avian lineages sampled, alongside with the history of formation of the Araguaia and Tocantins rivers may explain these inferred high rates of gene flow. The four species included in pattern “i” inhabit environments of flooded forest or floodplain, typical of river banks (Remsen and Parker, 1983), as well as river islands (Sick, 1997; Ridgely and Tudor, 2009; Kirwan and Green, 2011; Sigrist, 2013), which may have facilitated their dispersal across both the Araguaia and Tocantins rivers. Species in this habitat are known to have different population genetic histories than those in terra firme habitats (Harvey et al., 2017), which may explain this pattern.
In contrast, pattern “ii” reflect an important biogeographic characteristic of the Amazon: the reduction of the barrier effect of Amazonian rivers toward their headwaters (see Weir et al., 2015). Disregarding the four species sharing the phylogeographic pattern “i” discussed above, the remaining thirteen species targeted for molecular analysis presented genetically structured populations along the opposite banks of the lower Tocantins River (Table 1). This role played by the lower Tocantins River in isolating populations of reciprocally monophyletic and allopatric taxa between the XAE and BAE had already been documented for several avian lineages associated mainly with upland terra-firme forest (Ribas et al., 2012; Batista et al., 2013; Maldonado-Coelho et al., 2013; Thom and Aleixo, 2015; Silva et al., 2019). This variation in strength of the barrier effect played by the Tocantins River is consistent with the narrowing of its course upstream from its confluence with the Araguaia River, which can be two to three times narrower compared to its lower course. This is clearly demonstrated by the phylogeographic patterns detected for the allopatric species pairs P. anerythra/P. coerulescens, D. retentus/D. medius, Campylopyerus obscurus, and Thamnophilus amazonicus.
This gradual reduction in the barrier effect between the lower Tocantins River and its middle and upper portions together with the Araguaia River is similar to the conditions described for the Negro and Branco rivers on the Guiana shield (Naka et al., 2012; Naka and Brumfield, 2018). The lower Rio Negro acts as a phylogeographic break for more than 40 pairs of allopatric taxa among those 69 sampled (Naka et al., 2012; Naka and Brumfield, 2018). For the region of the Tocantins–Araguaia basin, the lower Tocantins River isolated seven pairs of allopatric taxa between XAE and BAE and five more taxa whose populations showed genetic structure between those areas of endemism (patterns “ii” and “iii”). In addition to these allopatric populations and taxa for which we obtained genetic data, another nine pairs of taxa are separated by the lower Tocantins River (Supplementary Table 1), but were not sampled genetically by us.
In addition to its narrower course when compared to the lower Tocantins, glaciation cycles between 1 million and 20,000 years before present, when dry and cold periods alternated with hot and humid periods (Hoorn et al., 2010; Meyer et al., 2014) might be related to the lower intensity of the barrier effect exerted by the Araguaia River. Ecological niche modeling showed that during the driest and coldest glacial periods (such as the Last Glacial Maximum), forests were likely replaced by savanna and non-humid forest phytophysiognomies in southeastern Amazonia (Aleixo et al., 2014; Silva et al., 2019). If this scenario is correct, then it would have caused a reduction in the geographic distribution of bird species associated with humid forests in southeastern most Amazonia, probably accompanied by the extinction of several taxa, which nevertheless might have persisted in the central-western portions of the biome (Silva et al., 2019). Although in the wettest and hottest periods of these glacial cycles there were probably successive retakes of forest cover into the extreme east of the Amazon, it is assumed that on these occasions the Araguaia River acted as a secondary geographic barrier, preventing the transposition of many typical upland terra-firme species, which are present in the XAE but do not enter the TAI (such as Cercomacra cinerascens, T. aethiops, Monasa morphoeus, P. leuconota, Galbula dea, Thamnophilus palliatus, Myrmoborus myotherinus, Glyphorynchus spirurus, Vireolanius leucotis, Conopophaga aurita, Hylopezus paraensis, Synallaxis rutilans, Lamprospiza melanoleuca, Ramphocaenus melanurus, and Piprites chloris) (Ridgely and Tudor, 2009; Billerman et al., 2020; Birdlife International, 2020; Supplementary Figure 14).
The current patterns of geographic distributions of this set of bird species could reflect a response to paleoenvironmental conditions affecting southeastern Amazonia as a whole and the upper stretches of the Tocantins and Araguaia rivers in a similar way as discussed for the genera Hylexetastes and Xiphocolaptes, which are widely present in Amazonia, but absent in the BAE, the easternmost Amazonian area of endemism (Silva et al., 2002; Azuaje-Rodríguez et al., 2020). Therefore, the hypothesis 3 that postulated the Araguaia River as a geographic barrier impeding the gene flow between TAI and XAE, albeit not corroborated by our molecular analyses, appears nevertheless supported by the geographic distributions of Ortalis superciliaris, shared between TAI and BAE, but absent in XAE (Supplementary Figure 14; Grantsau, 2010; Pascoal et al., 2016; Del Hoyo and Kirwan, 2020), and Piculus paraensis, also absent in XAE, but confirmed in the BAE (Del-Rio et al., 2013) and suspected within the TAI (Dornas et al., in press).
The phylogeographic pattern documented here for W. vidua, whereby two parapatric genetic lineages exist in the TAI could also be related to Late Pleistocene climatic-vegetational changes, which could have resulted in the presence of large savanna blocks between the northern and central-southern parts of the TAI separating two patches of fully forested areas. The first to the north of the TAI, geographically close to the BAE and adjacent to the left (western) bank of the Tocantins River, and the second to the south, geographically close to the XAE and adjacent to the right (eastern) bank of the Araguaia River (Supplementary Figure 15). These genetically distinct populations of W. vidua are apparently not in direct contact and exchanging genes due to the presence of an intervening modern ecological barrier, at the same time that their establishment in different parts of the TAI reflects distinct origins and possible independent colonization events into this interfluve.
In contrast to pattern “ii,” pattern “iii” documented herein for five species supported an extended barrier effect along the Tocantins River upstream from its confluence with the Araguaia River, highlighting the variability in inter-specific responses to a single riverine barrier, as verified for the middle and upper portions of the Negro and Branco rivers (Naka et al., 2012; Naka and Brumfield, 2018). Similarly, the proximity of middle and upper Tocantins River to the Amazon – Cerrado ecotone may enhance the barrier effect posed by the physical course of the river itself, which could “stabilize” the southeasternmost limits of ranges of several humid forest taxa within the TAI.
Finally, the phylogeographic analyses of the selected taxa did not demonstrate the presence of any genetically differentiated lineage restricted to the TAI. Evolutionarily, avian endemic lineages to the easternmost portion of the Amazon have shown very recent times of diversification coupled with smaller genetic distances separating them from other closely related south-central Amazonian lineages (Ribas et al., 2012; Batista et al., 2013; Aleixo et al., 2014; Thom and Aleixo, 2015; Silva et al., 2019). The continuing existence of gene flow together with the variation in the intensity of the barrier effect of the Tocantins and Araguaia rivers show that the time of diversification of these populations present in the TAI is still very recent, not favoring local coalescent processes.
Phylogenetic systematic studies supported lineages associated to the BAE and XAE, as single evolutionary units (e.g., Hylopezus macularius complex), hence, corroborating a scenario of reduced genetic diversification in the far east of the Amazon (Carneiro et al., 2012; Rodrigues et al., 2013; Silva et al., 2019). However, the presence of some species with populations showing high and significant Fst values between the TAI and XAE and also between TAI and BAE (Supplementary Table 3), demonstrate that genetic differentiation is underway inside the TAI. Until now, the only endemism of the TAI supported by molecular analyses is a didelphid marsupial described from the right bank of the Araguaia River (Rocha et al., 2015).
Contact Zones and Gene Flow
Contact zones represent areas where there is an overlap between parapatric populations of different taxa (Haffer, 1997; Aleixo, 2007). The recovered phylogeographic pattern “ii” demonstrated that within the TAI there is the concomitant occurrence of distinct populations or lineages otherwise endemic to the XAE and BAE, as verified for D. retentus/D. medius, P. anerythra/P. coerulescens, and C. obscurus.
The comparison of plumages of the specimens collected in the TAI for D. medius and D. retentus and P. anerythra and P. coerulescens, with those obtained from XAE and BAE indicated the apparent occurrence of hybridization events (Supplementary Table 2 and Supplementary Figures 5, 6). In Dendrocolaptes, the sharing of haplotypes from both endemic areas within the TAI, associated with the intermediate phenotypes between D. retentus and D. medius, support this area as a contact and hybridization zone between these species (Fst XAE and TAI = 0.23660/Fst BAE and TAI = 0.41681/P > 0.05), which nevertheless sort out completely across the lower Tocantins River (Batista et al., 2013).
The hybridization between P. anerythra and P. coerulescens had been initially suggested for the lower Tocantins River (Somenzari and Silveira, 2015) and later confirmed within the TAI by morphological comparisons among three specimens collected in the region (Brito et al., 2016). The three specimens collected in the TAI by Brito et al. (2016) were sampled in the molecular analyses (Supplementary Table 2) and had their hybridization also corroborated by genetic characters (Supplementary Figure 5). However, one specimen syntopic with the three hybrid specimens mentioned above (Dornas et al., in press), presented haplotype and morphological diagnoses of P. anerythra, supporting the phenotypic and genotypic occurrence of the species in the TAI. The confirmation of P. coerulescens records within the limits of the TAI is provided only through photographic records WA2759636 (Corrêa, 2017) and WA1882554 (Pacheco, 2005). The low and non-significant Fst values between XAE and TAI (Fst = 0.157), and between BAE and TAI (Fst = 0.368) P. anerythra/P. coerulescens lineages support a scenario of ongoing gene flow between them.
In turn, C. obscurus is a recently split species recognized by recent taxonomic revisions within the Campylopterus largipennis complex (Lopes et al., 2017). Our phylogenetic analyses recovered structure between XAE and BAE populations of this species, but which was not maintained in the TAI, where specimens of both lineages were found in syntopy (Supplementary Figure 7). Despite the marked molecular divergences observed between BAE and XAE C. obscurus populations, no apparent diagnosable morphological differences between were observed. Therefore, the extension of gene flow between these distinct populations must be assessed with a wider spectrum of genetic markers.
The occurrence of these contact and hybridization zones within the TAI (see also Areta et al., 2017) can be related to fluvial morphodynamic processes, such as the reduction of the isolation effect of the Tocantins and Araguaia rivers upstream from their confluence, due to geological and geomorphological characteristics. The widths of the Tocantins and Araguaia rivers gradually reduce upstream of their confluence (Supplementary Figure 16), being up to 10 times narrowed than the width of stretches of the Tocantins River downstream of the confluence with the Araguaia River (Agência Nacional das Águas [ANA], 2020). Moreover, the presence of extensive and forested river islands along the headwaters of the Tocantins and Araguaia rivers favors the establishment of several species of birds associated with humid forest habitats. These islands may facilitate the crossing between banks, promoting more frequent gene flow between populations on these upper stretches than in the Lower Tocantins area (Supplementary Figure 16).
Two other morphodynamic processes linked to fluvial systems in the Tocantins and Araguaia rivers can be related to the transposition of the banks by birds and consequent gene flow. The first is a succession of large oxbow lakes, with their surroundings taken over by floodplain forests. These lakes signal the old lines of the riverbed, known as paleochannels and characterize a meandrite fluvial system (Supplementary Figure 16), in which the riverbed is strongly sinuous, constituting an anastomosed fluvial system formed by numerous fluvial islands of varying sizes, generating a fluvial landscape with a highly ramified (Riccomini and Coimbra, 1993; Latrubesse and Stevaux, 2002, 2006; Morais et al., 2005; Rocha, 2011; Fryirs and Brierley, 2018). The second is the presence of straits, known also as pinched channels. Straits are drainage anomalies characterized by places where there is a narrowing of the river banks, with a marked reduction in the width of the riverbed, from the order of kilometers to a few hundred meters, usually resulting from local structural geological control, such as superimposition on dikes or embankments conditioned by geological faults (Howard, 1967; Summerfield, 2014; Barros and Magalhães Junior, 2020). Consequently, they can represent a relevant crossing point for the local biota between opposite river banks in closer proximity.
In the Tocantins River, one of the most remarkable straits is located in front of the municipality of Estreito. This river section presents an abrupt funneling of the riverbed from a predominant width of 1 km to about 150 m (6°33′42″S, 47°27′36″ W). On the Araguaia River, one of the main existing straits is located near the city of Xambioá (6°22′S; 48°23′W). The Pre-cambrian rock matrix of highly resistant quartzites, prominent in the region of Serra das Andorinhas (left bank) called the Morro Grande Formation, crosses the Araguaia River, entering the limits of the TAI (Figueiredo and Sousa, 2009). This geological continuity originates a very tapered strait, regionally called Remanso dos Botos (Supplementary Figure 16). The reduction in the width of the river in this stretch goes from 1.2 km to ca. 400–500 m. In sum, geomorphological fluvial processes support a strong physical historical connection between XAE and BAE biotas in the TAI, which is consistent with the documented contact zones and hybridization and gene flow events documented herein between lineages isolated in the BAE and XAE across the Lower Tocantins River.
Tocantins–Araguaia Interfluve as an Eastern Amazon Suture Zone
The disproportionate presence of a high number of phylogeographic breaks, contact zones and hybridization events configure the definition of biogeographic suture zones (Remington, 1968; Swenson and Howard, 2004, 2005). In the Amazon, the Negro–Branco interfluve has been characterized as a biogeographic suture zone for birds (Naka et al., 2012; Naka and Brumfield, 2018), as also verified for the upper reaches of the Tapajós River (Haffer, 1992).
Similarly, within the TAI, the recovered pattern “iii” supports the Tocantins River as a barrier separating genetically differentiated populations distributed in the XAE and TAI from those occurring in the BAE. In turn, pattern “ii” indicates the joint occurrence within the TAI of taxa otherwise distributed allopatrically in the XAE and BAE, hence, characterizing the existence of a contact zone. As discussed above, these contact zones involve either (1) an instance of apparent parapatry between two genetically distinct populations occurring in different parts of the TAI, and separated by the original presence of savanna formations (such as in W. vidua), or (2) taxa in apparent direct contact and which hybridize with each other within the TAI (such as in D. retentus/D. medius and P. anerythra/P. coerulescens).
Thus, the high number of phylogeographic breaks and contact zones within a single interfluve, as described for the Negro-Branco rivers by Naka et al. (2012) is equivalent to the one we documented herein for the TAI. Similarly, Weir et al. (2015) show several instances of hybridization between taxa distributed otherwise parapatrically across the headwaters of the Tapajós/Teles Pires and Xingu rivers, hence, further supporting a suture zone in south-central Amazonia. Both sutures zones documented by Naka et al. (2012) and Weir et al. (2015) overlap to some extent with ecotonal transitions between forest and savanna habitats located in the middle and upper portions of their respective hydrographic basins. These characteristics are also shared by the TAI, where another ecotonal transition between Amazonian forests and Cerrado savannas occur (Haidar et al., 2013; Marques et al., 2020). Therefore, all these characteristics combined support the TAI as a novel biogeographic suture zone, localized in the Eastern Amazon.
Data Availability Statement
The data presented in the study are deposited in the GenBank Nucleotide Database repository, accession numbers: ON137233–ON137450 and ON157084–ON157237.
Ethics Statement
Ethical review and approval was not required for the animal study because there was no handling of birds, just traditional laboratory work involving tissue aliquots, reagents, electronic machinery, and computer software. The source specimens of these aliquots were collected before the present study, by field expeditions of different teams from the consulted ornithological collections.
Author Contributions
TD and AA conceived the study. TD and SD analyzed the specimens in ornithological collection. TD, SD, and LA-S performed the laboratory work referring to molecular analyses. TD, LA-S, and AA applied and analyzed the data in computer applications. TD and FM analyzed the data under geological and fluvial morphology perspectives. TD wrote the manuscript. AA and SD reviewed and improved the English version of manuscript. All authors reviewed and approved the manuscript.
Funding
Laboratory work related to this manuscript were generously funded by CNPq (“INCT em Biodiversidade e Uso da Terra da Amazônia” # 574008/2008-0; # 563236/2010-8; and # 471342/2011-4) and FAPESPA (ICAAF 023/2011) to AA. TD was supported by Doctoral fellowship from CAPES to the Postgraduate Program in Biodiversity and Biotechnology of the Bionorte Network (PPG-BIONORTE) at Universidade Federal do Pará/Museu Paraense Emílio Goeldi, and PosDoctoral fellowship CAPES (88887.351860/2019-00) to the Postgraduate Program Science of Environment at Federal University of Tocantins.
Conflict of Interest
The authors declare that the research was conducted in the absence of any commercial or financial relationships that could be construed as a potential conflict of interest.
The handling editor declared a past collaboration with one of the authors, AA.
Publisher’s Note
All claims expressed in this article are solely those of the authors and do not necessarily represent those of their affiliated organizations, or those of the publisher, the editors and the reviewers. Any product that may be evaluated in this article, or claim that may be made by its manufacturer, is not guaranteed or endorsed by the publisher.
Acknowledgments
We are grateful to the two reviewers and editor LN for improvements provided to our manuscript. We thank the staff of the MPEG (Museu Paraense Emílio Goeldi, Belém, Brazil) for allowing us to borrow tissues and study specimens under their care. We also thank LGEMA-USP (Laboratory of Genetics and Molecular Evolution of Birds, University of São Paulo) and Department of Ornithology of the Museu Nacional do Rio de Janeiro for sending tissue samples requested from their respective cryogenic collections. TD thanks all the team of the ECOAVES/UFT Research Group and Renato Torres Pinheiro for all the logistical support. TD is also grateful the Postgraduate Program Science of Environment at Federal University of Tocantins (in Portuguese PPG-Ciamb/UFT) for the post-doctoral internship.
Supplementary Material
The Supplementary Material for this article can be found online at: https://www.frontiersin.org/articles/10.3389/fevo.2022.826394/full#supplementary-material
Footnotes
References
Ab’sáber, A. N. (2003). Os Domínios de Natureza no Brasil: Potencialidades Paisagísticas. São Paulo: Ateliê Editorial.
Agência Nacional das Águas [ANA] (2020). Mapa da Região Hidrográfica Tocantins-Araguaia. Available online at: https://www.gov.br/ana/pt-br/todos-os-documentos-do-portal/documentos-spr/mapas-regioes-hidrograficas/tocantins-araguaia-para-site-ana-a0.pdf (accessed on July 14, 2021)
Aleixo, A. (2002). Molecular systematics and the role of the várzea-terra firme ecotone in the diversification of Xiphorhynchus woodcreepers (Aves: Dendrocolaptidae). Auk 119, 621–640. doi: 10.2307/4089963
Aleixo, A. (2004). Historical diversification of a terra-firme forest bird superspecies: a phylogeographic perspective on the role of different hypotheses of Amazonian diversification. Evolution 58, 1303–1317. doi: 10.1111/j.0014-3820.2004.tb01709.x
Aleixo, A. (2007). Conceitos de espécie e o eterno conflito entre continuidade e operacionalidade: uma proposta de normatização de critérios para o reconhecimento de espécies pelo Comitê Brasileiro de Registros Ornitológicos. Revista Brasileira de Ornitologia 15, 229–242.
Aleixo, A., Carneiro, L., and Dantas, S. M. (2012). “Aves,” in Fauna da Floresta Nacional de Carajás: Estudos Sobre Vertebrados Terrestres, 1ed Edn, eds F. D. Martins, A. F. Castilho, J. Campos, F. M. Hatano, and S. G. Rolim (São Paulo: Nitro Imagens), 102–141.
Aleixo, A., Peterson, A. T., Araujo-Silva, L. E., Bandeira, C. H. M. M., Batista, R. S. S., Burlamaqui, T., et al. (2014). “Instabilidade climaìtica e diversificaçaϸo de espeìcies na Amazônia,” in Cenários para a Amazônia: Clima, Biodiversidade e Uso da Terra, 1ed Edn, eds T. Emilio and F. Luizão (Manaus: Editora INPA), 43–53.
Aleixo, A., Poletto, F., Portes, E., and Lima, M. F. (2010). “Aves,” in Os Animais da Tanguro, Mato Grosso: Diversidade na Zona de Transição entre a Floresta Amazônica e o Cerrado, eds A. C. M. Oliveira, J. Santos, and M. C. S. Costa (Belém, PA: MPEG, UFPA, IPAM), 53–56.
Aleixo, A., Whitney, B. M., and Oren, D. C. (2000). Range extensions of birds in southeastern Amazonia. Wilson Bull. 112, 137–142. doi: 10.1676/0043-5643(2000)112[0137:REOBIS]2.0.CO;2
Antonelli, A., Quijada-Mascareñas, Q., Crawford, A. J., Bates, J. M., Velazco, P. M., and Wuster, W. (2010). “Molecular studies and phylogeography of Amazon tetrapods and their relation to geological and climatic models,” in Amazonia: Landscape and Species Evolution, a look into the Past, eds C. Hoorn and F. Wesseling (Oxford: Willey-Blackwell), 386–404. doi: 10.1002/9781444306408.ch24
Araújo-Silva, L. E., Miranda, L. S., Carneiro, L., and Aleixo, A. (2017). Phylogeography and diversification of an Amazonian understorey hummingbird: paraphyly and evidence for widespread cryptic speciation in the Plio-Pleistocene. Ibis 159, 778–791. doi: 10.1111/ibi.12500
Areta, J. I., Dornas, T., Kirwan, G. M., Araújo-Silva, L. E., and Aleixo, A. (2017). Mixing the waters: a linear hybrid zone between two riverine Neotropical cardinals (Paroaria baeri and P. gularis). Emu - Austral Ornithol. 117, 40–50. doi: 10.1080/01584197.2016.1266447
Avila-Pires, T. C. S., Mulcahy, D. G., Werneck, F. P., and Sites, J. W. Jr. (2012). Phylogeography of the teiid lizard Kentropyx calcarata and the sphaerodactylid Gonatodes humeralis (Reptilia: squamata): testing a geological scenario for the lower Amazon-Tocantins Basins, Amazonia, Brazil. Herpetologica 68, 272–287. doi: 10.1655/HERPETOLOGICA-D-11-00021.1
Azuaje-Rodríguez, R. A., Weckstein, J. D., Dispoto, J. H., Patel, S., Cacioppo, J. A., Bates, J. M., et al. (2020). Molecular systematics of the Amazonian endemic genus Hylexetastes (Aves: Dendrocolaptidae): taxonomic and conservation implications. Ibis 162, 119–136. doi: 10.1111/ibi.12693
Barros, L. F. P., and Magalhães Junior, A. P. (2020). “Morfogênese Fluvial,” in Hidrogeomorfologia: Formas, Processos e Registros Sedimentares Fluviais, 1 Edn. eds L. F. P. Barros and A. P. Magalhães Junior (Rio de Janeiro: Bertrand Brasil), 163–174.
Bates, J. M. (2001). “Avian diversification in Amazonia: evidence for historical complexity and a vicariance model for a basic diversification pattern,” in Diversidade Cultural e Biológica da Amazônia, eds I. C. G. Vieira, J. M. C. Silva, D. C. Oren, and M. A. D’incao (Belém: Museu Paraense Emílio Goeldi), 119–138.
Batista, R., Aleixo, A., Vallinoto, M., Azevedo, L., Rêgo, P. S., Silveira, L. F., et al. (2013). “Molecular systematics and taxonomic revision of the Amazonian Barred Woodcreeper complex (Dendrocolaptes certhia: Dendrocolaptidae), with description of a new species from the Xingu–Tocantins interfluve,” in Handbook of the Birds of the World, Special Volume: New Species and Global Index, eds J. Del Hoyo, A. Elliott, and D. Christie (Barcelona, Spain: Lynx Edicions), 245–247.
Billerman, S. M., Keeney, B. K., Rodewald, P. G., and Schulenberg, T. S. (2020). Birds of the World. Ithaca, USA: Cornell Laboratory of Ornithology.
Birdlife International (2020). Species Distribution Data Request Version 2020. Available online at: http://datazone.birdlife.org/species/requestdis (accessed on Sep 10, 2021)
Brito, G. R. R., Kirwan, G. M., Assis, C. P., Firme, D. H., Figueira, D. M., Neto, N. B., et al. (2016). A collection of birds from Presidente Kennedy and adjacent areas, Tocantins: a further contribution to knowledge of Amazonian avifauna between the Araguaia and Tocantins rivers. Revista Brasileira de Ornitologia 24, 168–184.
Capurucho, J. M. G., Borges, S. H., Cornelius, C., Vicentini, A., Prata, E. M. B., Costa, F. M., et al. (2020). “Patterns and Processes of Diversification in Amazonian White Sand Ecosystems: Insights from Birds and Plants,” in Neotropical Diversification: Patterns and Processes, eds V. Rull and A. Carnaval (Cham: Fascinating Life Sciences), 245–270. doi: 10.1007/978-3-030-31167-4_31
Carneiro, L. S., Gonzaga, L. P., Rêgo, P. S., Sampaio, I., Schneider, H. E., and Aleixo, A. (2012). Systematic revision of the Spotted Antpitta (Grallariidae: Hylopezus macularius), with description of a cryptic new species from Brazilian Amazonia. Auk 129, 338–351.
Corrêa, A. G. (2017). WA2759636, Pyrrhura coerulescens Neumann, 1927. Available online at: http://www.wikiaves.com/2759636 (accessed on Sep 21, 2021)
Cracraft, J. (1985). Historical biogeography and patterns of differentiation within the South American avifauna: areas of endemism. Ornithol. Monogr. 36, 49–84. doi: 10.2307/40168278
Dantas, S. M., Weckstein, J. D., Bates, J., Oliveira, J. N., Catanach, T., and Aleixo, A. (2021). Multi-character taxonomic review, systematics, and biogeography of the Black-capped/Tawny-bellied Screech Owl (Megascops atricapilla M. watsonii) complex (Aves: Strigidae). Zootaxa 4949, 401–444. doi: 10.11646/zootaxa.4949.3.1
Del Hoyo, J., and Kirwan, G. M. (2020). “Buff-browed Chachalaca (Ortalis superciliaris), version 1.0,” in Birds of the World, eds J. del Hoyo, A. Elliott, J. Sargatal, D. A. Christie, and E. de Juana (Ithaca, NY, USA: Cornell Lab of Ornithology), doi: 10.2173/bow.bubcha1.01
Del-Rio, G., Silveira, L. F., Cavarzere, V., and Rêgo, M. A. (2013). A taxonomic review of the Golden-green Woodpecker, Piculus chrysochloros (Aves: Picidae) reveals the existence of six valid taxa. Zootaxa 3626, 531–542. doi: 10.11646/zootaxa.3626.4.7
Dornas, T., Marcelino, D., Dantas, S., Pinheiro, R., and Aleixo, A. (2017). Range extension and conservation of Psophia interjecta Griscom & Greenway, 1937 (Aves: Psophidae) in the Tocantins-Araguaia interfluve, state of Tocantins, Brazil. Check List 13, 141–146. doi: 10.15560/13.4.141
Dornas, T., Marcelino, D. G., Dantas, S. M., Prado, A. D., Pinheiro, R. T., Pascoal, W., et al. (in press). “Aves da Amazônia Tocantinense e zonas ecotonais: composição, riqueza, endemismos, biogeografia e conservação,” in Avanços nos Estudos da Biodiversidade e Biotecnologia na Amazçnia, eds C. Neto, E. Andrade, and M. L. Ruivo (Belem: UFRA).
Excoffier, L., Laval, G., and Schneider, S. (2005). Arlequin (version 3.0): an integrated software package for population genetics data analysis. Evol. Bioinform. 1, 47–50. doi: 10.1177/117693430500100003
Fernandes, A. M., Wink, M., and Aleixo, A. (2012). Phylogeography of the Chestnut-tailed Antbird (Myrmeciza hemimelaena) clarifies the role of rivers in Amazonian biogeography. J. Biogeogr. 39, 1524–1535. doi: 10.1111/j.1365-2699.2012.02712.x
Figueiredo, S. L., and Sousa, G. P. S. (2009). Análise Geológica, Geomorfológica e Turística do Parque Estadual da Serra dos Martírios-Andorinhas: potencial para o geoturismo. Pesquisas em Turismo e Paisagens Cársticas 2, 41–56.
Fouquet, A., Courtois, E., Baudain, D., Lima, J., Souza, S., Noonan, B., et al. (2015). The trans-riverine genetic structure of 28 Amazonian frog species is dependent on life history. J. Tropic. Ecol. 31, 361–373. doi: 10.1017/S0266467415000206
Frankham, R., Ballou, J. D., and Briscoe, D. A. (2008). Fundamentos da Genética da Conservação. Ribeiro Preto: Sociedade Brasileira de Genética.
Fryirs, K. A., and Brierley, G. J. (2018). What’s in a name? A naming convention for geomorphic river types using the River Styles Framework. PLoS One 13:e0201909. doi: 10.1371/journal.pone.0201909
Grantsau, R. K. H. (2010). Guia completo para identificação das Aves do Brasil. São Carlos: Vento Verde.
Graves, G. R., and Zusi, R. L. (1990). Avian body weights from the lower Xingu. Bull. Br. Ornitol. Club. 110, 20–25.
Hackett, S. J. (1996). Molecular Phylogenetics and Biogeography of Tanagers in the Genus Ramphocelus (Aves). Mol. Phylogen. Evol. 5, 68–382. doi: 10.1006/mpev.1996.0032
Haffer, J. (1969). Speciation in Amazonian forest birds. Science 165, 131–137. doi: 10.1126/science.165.3889.131
Haffer, J. (1992). On the river effect in some forest birds of southern Amazonia. Boletim do Museu Paraense Emílio Goeldi, Série Zoologia 8, 217–245.
Haffer, J. (2001). “Hypotheses to explain the origin of species in Amazonia,” in Diversidade Cultural e Biológica da Amazônia, eds I. C. G. Vieira, J. M. C. Silva, D. C. Oren, and M. A. D’incao (Belém: Museu Paraense Emílio Goeldi), 45–118.
Haffer, J. (2008). Hypotheses to explain the origin of species in Amazonia. Brazil. J. Biol. 68, 917–947. doi: 10.1590/s1519-69842008000500003
Haidar, R. F., Fagg, J. M. F., Pinto, J. R. R., Dias, R. R., Damasco, G., Silva, L. C. R., et al. (2013). Florestas estacionais e áreas de ecótono no estado do Tocantins, Brasil: parâmetros estruturais, classificação das fitofisionomias florestais e subsídios para conservação. Acta Amazon. 43, 261–290. doi: 10.1590/S0044-59672013000300003
Hall, T. A. (1999). BioEdit: a user-friendly biological sequence alignment editor and analysis program for Windows 95/95/NT. Nucleic Acids Symp. Ser. 41, 95–98.
Hartl, D. L., and Clark, A. G. (2007). Principles of Population Genetics, 4rd Edn. Sunderland, MA: Sinauer Associates.
Harvey, M. G., Aleixo, A., Ribas, C. C., and Brumfield, R. T. (2017). Habitat Association Predicts Genetic Diversity and Population Divergence in Amazonian Birds. Am. Nat. 190, 631–648. doi: 10.1086/693856
Henriques, L. M. P., Dantas, S., Santos, L. B., Bueno, A. S., and Peres, C. A. (2021). Avian extinctions induced by the oldest Amazonian hydropower mega dam: evidence from museum collections and sighting data spanning 172 years. PeerJ 9:e11979. doi: 10.7717/peerj.11979
Hoorn, C., Wesselingh, F. P., Ter Steege, H., Bermudez, M. A., Mora, A., Sevink, J., et al. (2010). Amazonia through time: Andean Uplift, climate change, landscape evolution, and biodiversity. Science 330, 927–931. doi: 10.1126/science.1194585
Howard, A. D. (1967). Drainage analysis in geologic interpretation: summation. Bull. Am. Assoc. Petrol. Geol. 5, 2246–2259.
Hrbek, T., Silva, V. M. F., Dutra, N., Gravena, W., Martin, A. R., and Farias, I. P. (2014). A new species of river dolphin from Brazil or: how little do we know our biodiversity. PLoS One 9:e83623. doi: 10.1371/journal.pone.0083623
Instituto Brasileiro de Geografia e Estatística [IBGE] (2012). Manual Técnico da Vegetação Brasileira. Brazil: Instituto Brasileiro de Geografia e Estatística.
Instituto Brasileiro de Geografia e Estatística [IBGE] (2019). Biomas e Sistema Costeiro-Marinho do Brasil: Compatível Com a Escala 1:250 000. Available online at: https://biblioteca.ibge.gov.br/index.php/biblioteca-catalogo?view=detalhes&id=2101676 (accessed on Oct 16, 2021).
Latrubesse, E., and Stevaux, J. C. (2002). Geomorphology and Environmental aspects of the Araguaia fluvial basin, Brazil. Zeitschrift Fur Geomorphologie 129, 109–127.
Latrubesse, E., and Stevaux, J. C. (2006). Características físico-bióticas e problemas ambientais associados à planície aluvial do Rio Araguaia, Brasil Central. Revista UnG-Geociências 5, 65–73.
Lavergne, A., Ruiz-García, M., Catzeflis, F., Lacote, S., Contamin, H., Mercereau-Puijalon, O., et al. (2010). Phylogeny and phylogeography of squirrel monkeys (genus Saimiri) based on cytochrome b genetic analysis. Am. J. Primatol. 72, 242–253. doi: 10.1002/ajp.20773
Lees, A. C., Moura, N. G., Silva, A. S., Aleixo, A. L. P., Barlow, J., Berenguer, E., et al. (2012). Paragominas: a quantitative baseline inventory of an Eastern Amazonian avifauna. Revista Brasileira de Ornitologia 20, 93–118.
Lopes, L. E., Vasconcelos, M. F., and Gonzaga, L. P. (2017). A cryptic new species of hummingbird of the Campylopterus largipennis complex (Aves: Trochilidae). Zootaxa 4268, 1–33. doi: 10.11646/zootaxa.4268.1.1
Maldonado-Coelho, M., Blake, J., Silveira, L., Batalha-Filho, H., and Ricklefs, R. (2013). Rivers, refuges and population divergence of fire-eye antbirds (Pyriglena) in the Amazon Basin. J. Evol. Biol. 26, 1090–1107. doi: 10.1111/jeb.12123
Marks, B. D., Hackett, S. J., and Capparella, A. P. (2002). Historical relationships among Neotropical lowland forest areas of endemism as determined by mitochondrial DNA sequence variation within the Wedge-billed Woodcreeper (Aves: Dendrocolaptidae: Glyphorynchus spirurus). Mol. Phylogen. Evol. 24, 153–167. doi: 10.1016/s1055-7903(02)00233-6
Marques, E. Q., Marimon-Junior, B. H., Marimon, B. S., et al. (2020). Redefining the Cerrado–Amazonia transition: implications for conservation. Biodiv. Conservat. 29, 1501–1517. doi: 10.1007/s10531-019-01720-z
Mercês, M. P., Lynch-Alfaro, J. W., Ferreira, W. A. S., Harada, M. L., and Silva Júnior, J. S. (2015). Morphology and mitochondrial phylogenetics reveal that the Amazon River separates two eastern squirrel monkey species: Saimiri sciureus and S. collinsi. Mol. Phylogen. Evol. 82, 426–435. doi: 10.1016/j.ympev.2014.09.020
Meyer, K. E. B., Cassino, R. F., Lorente, F. L., Raczka, M., and Parizzi, M. G. (2014). “Paleoclima e Paleoambiente do Cerrado Durante o Quaternário com Base em Análises Palinológicas,” in Paleontologia: Cenário de Vida – Paleoclimas, Vol. 5, eds I. S. Carvalho, M. J. Garcia, C. C. Lana, and O. Strohschoen Jr. (Rio de Janeiro: Interciência), 397–414.
Morais, R. P., Oliveira, L. G., Latrubesse, E. M., and Pinheiro, R. C. D. (2005). Morfometria de sistemas lacustres da planície aluvial do médio rio Araguaia. Acta Scient. Biol. Sci. 27, 203–213. doi: 10.4025/actascibiolsci.v27i3.1278
Naka, L. N. (2011). Avian distribution patterns in the Guiana Shield: Implications for the delimitation of Amazonian areas of endemism. J. Biogeogr. 38, 681–696. doi: 10.1111/j.1365-2699.2010.02443.x
Naka, L. N., and Brumfield, R. T. (2018). The dual role of Amazonian rivers in the generation and maintenance of avian diversity. Sci. Adv. 4:eaar8575. doi: 10.1126/sciadv.aar8575
Naka, L. N., Bechtoldt, C. L., Henriques, L. M., and Brumfield, R. T. (2012). The role of physical barriers in the location of avian suture zones in the Guiana Shield, northern Amazonia. Am. Nat. 179, E115–E132. doi: 10.1086/664627
Novaes, F. C., and Lima, M. F. C. (2009). Aves da Grande Belém. Municípios de Belém e Ananindeua, Pará, 2.ed Edn. Belém: Museu Paraense Emilio Goeldi.
Oliveira, D. M. M., Oliveira, C. R. A., França, M. S. A., and Gonini, C. (2005). “Avifauna do norte do Araguaia, Mato Grosso, Brasil,” in Inventário Zoobotânico no Rio das Mortes, MT. Fauna e flora do Pantanal do Mortes-Araguaia, MT, ed. H. S. R. Cabette (Nova Xavatina: Universidade do Estado de Mato Grosso), 52–71.
Oren, D. C., and Roma, J. C. (2012). “Composição e vulnerabilidade da avifauna da Amazônia maranhense, Brasil,” in Amazônia Maranhense: Diversidade e Conservação, eds M. B. Martins and T. G. Oliveira (Belém: Museu Paraense Emilio Goeldi), 220–248.
Pacheco, J. F. (2005). WA1882554, Pyrrhura coerulescens Neumann, 1927]. Available online at: http://www.wikiaves.com/1882554 (accessed on Sep 22, 2021)
Pacheco, J. F., Kirwan, G. M., Aleixo, A., Whitney, B. M., Whittaker, A., Minns, J., et al. (2007). An avifaunal inventory of the CVRD Serra dos Carajás project, Pará, Brazil. Cotinga 27, 15–30.
Pacheco, J. F., Silveira, L. F., Aleixo, A., Agne, C. A., Bencke, G. A., Bravo, G. A., et al. (2021). Annotated checklist of the birds of Brazil by the Brazilian Ornithological Records Committee. Ornithol. Res. 29, 94–105. doi: 10.1007/s43388-021-00058-x
Pascoal, W., Dantas, S., Weber, L., and Duks, C. (2016). Levantamento preliminar da avifauna do Campus da EMVZ da Universidade Federal do Tocantins, Araguaína-TO, com observações sobre a reprodução de algumas espécies. Atualidades Ornitológicas 189, 45–56.
Patané, J. S. L., Weckstein, J. D., Aleixo, A., and Bates, J. M. (2009). Evolutionary history of Ramphastos toucans: molecular phylogenetics, temporal diversification, and biogeography. Mol. Phylogen. Evol. 53, 923–934. doi: 10.1016/j.ympev.2009.08.017
Portes, C. E. B., Carneiro, L. S., Schunck, F., Silva, M. S. S., Zimmer, K. J., Whittaker, A., et al. (2011). Annotated checklist of birds recorded between 1998 and 2009 at nine areas in the Belém area of endemism, with notes on some range extensions and the conservation status of endangered species. Revista Brasileira de Ornitologia 19, 167–184.
Posada, D. (2008). JModelTest: Phylogenetic model averaging. Mol. Biol. Evol. 25, 1253–1256. doi: 10.1093/molbev/msn083
Quaresma, T. F., Cronemberger, A. A., Batista, R., and Aleixo, A. (2022). Diversification and species limits in scale-backed antbirds (Willisornis, Thamnophilidae), an Amazonian endemic lineage. Zool. J. Linnean Soc. zlac011. doi: 10.1093/zoolinnean/zlac011
Rambaut, A., and Drummond, A. J. (2009). Tracer version 1.5. Available online at http://beast.bio.ed.ac.uk/Tracer (accessed on April 05, 2015)
Remington, C. L. (1968). “Suture-zones of hybrid interaction between recently joined biotas,” in Evolution Biology, Vol. 2, eds T. Dobzhansky, M. K. Hecht, and W. C. Steere (Boston, MA: Springer), 321–428.
Remsen, J. V. Jr., and Parker, T. A. III (1983). Contribution of river-created habitats to bird species richness in Amazonia. Biotropica 15, 223–231. doi: 10.2307/2387833
Ribas, C. C., Aleixo, A., Nogueira, A. C. R., Miyaki, C. Y., and Cracraft, J. (2012). A paleobiogeographic model for biotic diversification within Amazonia over the past three million years. Proc. Biol. Soc. 279, 681–689. doi: 10.1098/rspb.2011.1120
Ribas, C. C., Joseph, L., and Miyaki, C. Y. (2006). Molecular systematics and patterns of diversification in Pyrrhura (Psittacidae), with special reference to the picta-leucotis complex. Auk 123, 660–680. doi: 10.1093/auk/123.3.660
Riccomini, C., and Coimbra, A. M. (1993). Sedimentação em rios entrelaçados e anastomosados. Bol. Inst. Geociênc. IGUSP Série Didática 6, 1–44. doi: 10.11606/issn.2316-896X.v0i6p1-37
Ridgely, R. S., and Tudor, G. (2009). Songbirds of South America. The Passerines. Austin: University of Texas Press.
Rocha, P. C. (2011). Sistemas rio-planície de inundação: geomorfologia e conectividade hidrodinâmica. Caderno Prudentino de Geografia 1, 50–67.
Rocha, R. G., Ferreira, E., Loss, A. C., Heller, R., Fonseca, C., and Costa, L. P. (2015). The Araguaia River as an Important Biogeographical Divide for Didelphid Marsupials in Central Brazil. J. Heredity 106, 593–607. doi: 10.1093/jhered/esv058
Rodrigues, E. B., Aleixo, A., Whittaker, A., and Naka, L. N. (2013). “Molecular systematics and taxonomic revision of the Lineated Woodcreeper complex (Lepidocolaptes albolineatus: Dendrocolaptidae), with description of a new species from southwestern Amazonia,” in Handbook of the Birds of the World, Special Volume: New Species and Global Index, eds J. Del Hoyo, A. Elliott, and D. Christie (Barcelona, Spain: Lynx Edicions), 248–252.
Roma, J. C. (1996). Composição e Vulnerabilidade da Avifauna do Leste do Estado do Pará, Brasil. Ph.D.thesis. Belém(Br): Museu Paraense Emílio Goeldi.
Ronquist, F., and Huelsenbeck, J. P. (2003). MrBayes 3: Bayesian Phylogenetic Inference Under Mixed Models. Bioinformatics 19, 1572–1574. doi: 10.1093/bioinformatics/btg180
Salzburger, W., Ewing, G. B., and Von Haeseler, A. (2011). The performance of phylogenetic algorithms in estimating haplotype genealogies with migration. Mol. Ecol. 20, 1952–1963. doi: 10.1111/j.1365-294X.2011.05066.x
Sambrook, J., Fritsch, E. F., and Maniatis, T. (1989). Molecular Cloning: A Laboratory Manual. New York: Cold Spring Harbor.
Sampaio, L., Aleixo, A., Schneider, H., Sampaio, I., Araripe, J., and Rêgo, P. S. D. (2018). Molecular and plumage analyses indicate the incomplete separation of two woodpeckers (Aves, Picidae). Zoo. Scripta 47, 418–427. doi: 10.1111/zsc.12287
Schultz, E. D., Perez-Eman, J., Aleixo, A., Miyaki, C. Y., Brumfield, R. T., Cracraft, J., et al. (2019). Diversification history in the Dendrocincla fuliginosa complex (Aves: Dendrocolaptidae): Insights from broad geographic sampling. Mol. Phylogen. Evol. 140:106581. doi: 10.1016/j.ympev.2019.106581
Secretaria e Planejamento do Estado do Tocantins [Seplan] (2012). Atlas do Tocantins: Subsídios ao Planejamento da Gestão Territorial. Available online at: https://www.to.gov.br/seplan/atlas-do-tocantins/1asvwxbj530d (accessed on Sep 3, 2021)
Sick, H. (1967). Rios e enchentes na Amazônia como obstáculo para a avifauna. Atlas do Simpósio sobre a Biota Amazônica 5, 495–520.
Sick, H. (1997). Ornitologia Brasileira, versão revista e atualizada por José Fernando Pacheco. Rio de Janeiro: Nova Fronteira.
Sigrist, T. (2013). Guia de Campo Avis Brasilis: Avifauna Brasileira, 3a Edn. São Paulo: Editora Avis Brasilis.
Silva, J. M. C., Novaes, F. C., and Oren, D. C. (2002). Differentiation of Xiphocolaptes (Dendrocolaptidae) across the river Xingu, Brazilian Amazonia: recognition of a new phylogenetic species and biogeographic implications. Bull. Br. Ornitol. Club 122, 185–194.
Silva, J. M. C., Rylands, E., and Fonseca, G. A. B. (2005). O destino das áreas de endemismo da Amazônia. Megadiversidade 1, 124–131.
Silva, S. M., Peterson, A. T., Carneiro, L., Burlamaqui, T. C. T., Ribas, C. C., Sousa-Neves, T., et al. (2019). A dynamic continental moisture gradient drove Amazonian bird diversification. Sci. Adv. 5:eaat5752. doi: 10.1126/sciadv.aat5752
Smith, T. B., Schneider, C. J., and Holder, K. (2001). Refugial isolation versus ecological gradients. Genetica 112, 383–398. doi: 10.1023/A:1013312510860
Soares, L. M. D. S., Bates, J., Carneiro, L. S., Santos, M. P. D., and Aleixo, A. (2019). Molecular systematics, biogeography and taxonomy of forest-falcons in the Micrastur ruficollis species complex (Aves: Falconidae). J. Avian Biol. 50:e01943. doi: 10.1111/jav.01943
Somenzari, M., and Silveira, L. F. (2015). Taxonomy of the Pyrrhura perlata-coerulescens complex (Psittaciformes: Psittacidae) with description of a hybrid zone. J. Ornithol. 156, 1049–1060. doi: 10.1007/s10336-015-1216-3
Somenzari, M., Silveira, L. F., Piacentini, V. Q., Rego, M. A., Schunck, F., and Cavarzere, V. (2011). Birds of an Amazonia-Cerrado ecotone in southern Pará, Brazil, and the efficiency of associating multiple methods in avifaunal inventories. Revista Brasileira de Ornitologia 19, 260–275.
Sorenson, M. D., Ast, J. C., Dimchev, D. E., Yuri, T., and Mindell, D. P. (1999). Primers for a PCR-Based Approach to Mitochondrial Genome Sequencing in Birds and Other Vertebrates. Mol. Phylogen. Evol. 12, 105–114. doi: 10.1006/mpev.1998.0602
Summerfield, M. A. (2014). Global Geomorphology: An Introduction to the Lanforms, 2° Edn. London: Routtledge.
Swenson, N. G., and Howard, D. J. (2005). Clustering of contact zones, hybrid zones, and phylogeographic breaks in North America. Am. Nat. 166, 581–591.
Thom, G., and Aleixo, A. (2015). Cryptic speciation in the White-shouldered Antshrike (Thamnophilus aethiops, Aves-Thamnophilidae): the tale of a transcontinental radiation across rivers in lowland Amazonia and the northeastern Atlantic Forest. Mol. Phylogen. Evol. 82, 95–110. doi: 10.1016/j.ympev.2014.09.023
Valente, R. M. (2006). Padrões Espaciais em Comunidades de Aves Amazônicas. Ph.D.thesis. Belém(Br): Museu Paraense Emílio Goeldi/Universidade Federal do Pará.
Vasconcelos, M. F., Pacheco, J. F., and Parrini, R. (2007). Levantamento e conservação da avifauna na zona urbana de Marabá, Pará, Brasil. Cotinga 28, 45–51.
Weir, J., Faccio, M., Pulido-Santa Cruz, P., Barrera-Guzmán, A. O., and Aleixo, A. (2015). Hybridization in headwater regions, and the role of rivers as drivers of speciation in Amazonian birds. Evolution 69, 1823–1834. doi: 10.1111/evo.12696
Whittaker, A. (2008). A rapid ornithological assesment from the kayapó indigenous reserve, east of the rio Xingu at aldeia kubenkrankei, Pará, Brazil. Manaus: Bird Brazil Tours.
Wright, S. (1978). Evolution and the Genetics of Populations. Vol. 4. Variability within and among Natural Populations. Chicago: University of Chicago Press.
Keywords: Amazonia, contact zones, gene flow, hybridization, river barrier, river dynamics
Citation: Dornas T, Dantas SM, Araújo-Silva LE, Morais F and Aleixo A (2022) Comparative Phylogeography of Birds Across the Tocantins–Araguaia Interfluve Reveals a New Biogeographic Suture in the Amazon Far East. Front. Ecol. Evol. 10:826394. doi: 10.3389/fevo.2022.826394
Received: 30 November 2021; Accepted: 20 May 2022;
Published: 24 June 2022.
Edited by:
Luciano N. Naka, Federal University of Pernambuco, BrazilReviewed by:
Andre Moncrieff, Louisiana State University, United StatesOscar Johnson, The University of Texas at El Paso, United States
Copyright © 2022 Dornas, Dantas, Araújo-Silva, Morais and Aleixo. This is an open-access article distributed under the terms of the Creative Commons Attribution License (CC BY). The use, distribution or reproduction in other forums is permitted, provided the original author(s) and the copyright owner(s) are credited and that the original publication in this journal is cited, in accordance with accepted academic practice. No use, distribution or reproduction is permitted which does not comply with these terms.
*Correspondence: Alexandre Aleixo, YWxleGFuZHJlLmFsZWl4b0BoZWxzaW5raS5maQ==