Histone H3 Methylation and Autosomal vs. Sex Chromosome Segregation During Male Meiosis in Heteroptera
- 1Facultad de Medicina, Instituto de Investigaciones Biomédicas, CONICET-Universidad de Buenos Aires, Universidad de Buenos Aires, Ciudad Autónoma de Buenos Aires, Argentina
- 2Departamento de Ecología, Genética y Evolución, Facultad de Ciencias Exactas y Naturales, Universidad de Buenos Aires, Ciudad Autónoma de Buenos Aires, Argentina
Heteropteran insects exhibit a remarkable diversity of meiotic processes, including coexistence of different chromosomes types with different behavior during the first meiotic division, non-chiasmatic segregation, and inverted meiosis. Because of this diversity they represent suitable models to study fundamental questions about the mechanisms of chromosome behavior during cell division. All heteropteran species possess holokinetic chromosomes and in most of them the autosomal chromosomes synapse, recombine, and undergoe pre-reductional meiosis. In contrast, the sex chromosomes are achiasmatic, behave as univalents at metaphase I and present an inverted or post-reductional meiosis. An exception to this typical behavior is found in Pachylis argentinus, where both the autosomes and the X-chromosome divide reductionally at anaphase I and then divide equationally at anaphase II. In the present report, we analyzed the distribution of histones H3K9me2 and H3K9me3 in P. argentinus and in five species that have simple and multiple sex chromosome systems with typical chromosome segregation, Belostoma elegans, B. oxyurum, Holhymenia rubiginosa, Phthia picta, and Oncopeltus unifasciatellus. We found that H3K9me3 is a marker for sex-chromosomes from early prophase I to the end of the first division in all the species. H3K9me2 also marks the sex chromosomes since early prophase but shows different dynamics at metaphase I depending on the sex-chromosome segregation: it is lost in species with equationally dividing sex chromosomes but remains on one end of the X chromosome of P. argentinus, where chromatids migrate together at anaphase I. It is proposed that the loss of H3K9me2 from the sex chromosomes observed at metaphase I may be part of a set of epigenetic signals that lead to the reductional or equational division of autosomes and sex chromosomes observed in most Heteroptera. The present observations suggest that the histone modifications analyzed here evolved in Heteroptera as markers for asynaptic and achiasmatic sex chromosomes during meiosis to allow the distinction from the chiasmatic autosomal chromosomes.
Introduction
Post-transcriptional modifications (PTMs) of histones are related to basic biological processes such as transcriptional activation/inactivation, chromosome packaging, mitosis, meiosis, apoptosis, and DNA damage/repair (Bannister and Kouzarides, 2011; Tessarz and Kouzarides, 2014). Several histone modifications participate in meiosis acting individually or collectively to regulate a variety of meiotic events (Ivanovska and Orr-Weaver, 2006; Wang et al., 2017; Lam et al., 2019). In many cases, these histone marks can be detected at cytogenetic acting on whole chromosome or large chromosome segments. Among histone PTMs, the methylation of histone H3 at lysine 9 (H3K9) is an important epigenetic mark of heterochromatin formation (Peters et al., 2001; García-Cao et al., 2004). In addition to its well-described role in somatic cells and mitotic chromosomes, H3K9 methylation also plays a key role during meiosis. In mouse meiotic cells its tri-methylated form (H3K9me3) is a constitutive marker of pericentromeric heterochromatin and it is essential for normal synapsis and segregation (Peters et al., 2001). Along with other histone H3 modifications, H3K9 can be methylated by PR domain-containing 9 (PRDM9) which is involved in meiotic recombination initiation in mammalian spermatocytes (Powers et al., 2016). Also in mammals, its di-methylated form (H3K9me2) is also present with other epigenetic markers on the heterochromatic “XY body” within the pachytene spermatocyte nucleus (Khalil et al., 2004; Sciurano and Solari, 2014). As shown in mice, the formation of the condensed XY body is essential for male fertility because it shields the non-synaptic X region from the checkpoint machinery and prevents the expression of a small number of Y-linked genes during male meiosis (Turner et al., 2004; Turner, 2007; Royo et al., 2010). Systematic investigations of histone H3 methylation in insect meiosis are scarce, with the exception of Drosophila melanogaster where the role of this epigenetic modification has been analyzed in mutants for different H3K9-specific histone methyltransferases (HMTs). Reduced methylation of H3K9 in Drosophila has variable effects ranging from early arrest of oogenesis to no effect in male and female meiosis depending of the mutated HMT (Clough et al., 2007; Ushijima et al., 2012). In Heteroptera immunostaining of H3K9me3 in two pentatomid species showed that this modification is present at heterochromatic regions of autosomal bivalents and also on the sex chromosomes X and Y throughout the first meiotic prophase, suggesting a role for meiotic silencing of the sex pair (Viera et al., 2009a; Viera et al., 2016).
The Heteroptera, or true bugs, are a diversified group of insects that have some unique cytogenetic features that make them attractive to study the meiotic chromosome behavior. In addition to the holokinetic nature of their chromosomes, autosomes, and sex chromosomes behave differently with respect to synapsis and crossing over during the male meiosis. In most Heteroptera, the autosomes synapse, recombine, and segregate reductionally during the first meiotic division (pre-reductional meiosis) (Ueshima, 1979; Papeschi, 1994; Gokhman and Kuznetsova, 2006; Papeschi and Bressa, 2006; Kuznetsova et al., 2011). Instead, the sex chromosomes are achiasmatic and behave as univalents at metaphase I. These chromosomes segregate equationally during anaphase I (inverted or post-reductional meiosis) and join in meiosis II by the so-called “touch-and-go pairing” to form a pseudo-bivalent or pseudo-multivalent (Ueshima, 1979; Manna, 1984; González-García et al., 1996; Suja et al., 2000). The pre-reduction of the sex chromosomes has been described in less than 70 species from different infraorders, representing only 4.3% percent of the cytologically studied heteropterans (approximately 1,600 species) (Papeschi et al., 2003; Grozeva et al., 2006, 2013; Papeschi and Bressa, 2006; Kuznetsova et al., 2011; Jauset et al., 2015; Golub et al., 2018). Another feature that makes the meiotic system unique in Heteroptera is the presence of a tiny pair of chromosomes, called m chromosomes, in many families of the infraorders Dipsocoromorpha (Dipsocoridae, Schizopteridae), Nepomorpha (Corixidae, Naucoridae, Notonectidae, Pleidae), Leptopodomorpha (Saldidae), Cimicomorpha (Miridae), and Pentatomomorpha (Colobathristidae, Lygaeidae, Largidae, Alydidae, Coreidae, Rhopalidae, and Stenocephalidae) (Nokkala and Nokkala, 1986; Grozeva and Nokkala, 1996; Papeschi and Bressa, 2006; Grozeva et al., 2009; Kuznetsova et al., 2021). These chromosomes are generally unpaired and achiasmatic during early meiosis and they come close together during late diakinesis. At metaphase I, they are often associated end-to-end (“touch-and-go pairing”) and form a pseudo-bivalent that segregates reductionally at anaphase I (Nokkala, 1986; Papeschi and Bressa, 2006; Toscani et al., 2008).
In addition to their differential behavior during meiotic divisions, autosomes and sex chromosomes show differences in their chromatin condensation and heterochromatin content. In the male germ line, sex chromosomes are often discernible as positively heteropyknotic, highly condensed “chromatin bodies” from early stages of meiotic prophase I to diakinesis (Henking, 1891; Ueshima, 1979; Rebagliati et al., 2005). This positive heteropyknosis suggests that the sex chromosomes are heterochromatic; the constitutive or facultative nature of the X and Y heterochromatin largely depends on the family, the genus and also the species (Bressa et al., 2009). Most early reports indicated that C-positive heterochromatin was restricted to one or more sex chromosomes, and/or to terminal positions on some or all autosomal pairs. However, a growing number of reports have described that the heterochromatin on autosomes and sex chromosomes are more variable within and between species than previously thought (Grozeva et al., 2004; reviewed in Papeschi and Bressa, 2006; Kuznetsova et al., 2007; Bressa et al., 2008; Rebagliati, 2009; Kaur et al., 2010; Panzera et al., 2010; Chirino et al., 2013; Chirino and Bressa, 2014). Thus, heterochromatin accumulation in the karyotype of Heteroptera is not be random process, but some constraints regulate its acquisition and/or accumulation in different karyotypes (reviewed in Papeschi and Bressa, 2006).
Considering the presence of chromosome types with different pyknosis during the first prophase and metaphase and the coexistence of chromosomes with different behaviors in terms of synapsis and segregation, we wanted to analyze the presence of H3K9me2 and H3K9me3 during male meiosis in heteropteran insects. We report our results in six species that differ in the content and distribution of constitutive heterochromatin, the type of sex chromosome system, and the presence/absence of m chromosomes. In addition, one of the species shows atypical (reductional) behavior of the single X chromosome. We show here that H3K9me2 and me3 are both associated with the formation of constitutive heterochromatin during prophase I through metaphase in all the species. However, in diakinesis/metaphase I, differences in histone H3 methylation patterns were found between the species with typical (equational) segregation of the sex chromosomes and P. argentinus, where the single X chromosome behaves similarly to autosomal bivalents during the meiotic divisions. It is suggested that histone H3 modifications may play a dual role in Heteroptera: first, as true markers of constitutive heterochromatin and, second, as signals to discriminate between autosomal bivalents from the sex chromosomes at anaphase I.
Materials and Methods
Insects
Our study includes six species with karyotypic features that are relevant to analyze: the behavior of autosomes, sex chromosomes, and m chromosomes during male meiosis, and the presence of different sex chromosome formulas. Nymphs and adults of Belostoma elegans Mayr, 1871, B. oxyurum (Dufour, 1863) (Nepomorpha, Belostomatidae), Holhymenia rubiginosa Breddin, 1904, Pachylis argentinus Berg, 1879, Phthia picta (Drury, 1770) (Pentatomomorpha, Coreidae), and Oncopeltus unifasciatellus Slater, 1964 (Pentatomomorpha, Lygaeidae) were collected in natural populations from several locations of Argentina (Supplementary Table 1).
Chromosome Preparations for Conventional Staining and Chromosome Bandings
All the analyzed specimens were brought alive to the laboratory. The male and female gonads were dissected out in physiological solution as earlier described for the pyralid moth, Ephestia sp., swollen in a hypotonic solution, and fixed as described in Bressa et al. (2009) and Poggio et al. (2011). Afterward, one of the gonads was fixed for 15–30 min in freshly prepared Carnoy fixative (ethanol:chloroform:acetic acid, 6:3:1) and kept at 4°C in 70% ethanol for meiotic studies. For conventional staining, gonads were squashed in a drop of 2% iron acetic haematoxylin following conventional procedures (Sáez, 1960). For C-banding, spread chromosome preparations were made from the other testis of adult and nymphs and the ovaries as described in Traut (1976) and Bressa et al. (2009). Briefly, cells were dissociated in a drop of 60% acetic acid with the help of tungsten needles and spread on the slide using a heating plate at 45°C. Immediately the preparations were dehydrated in an ethanol series (70, 80, and 96, 30 s each) and stored at −20°C until use.
Heterochromatin content, distribution and nucleotide composition were analyzed by means of C-banding and fluorochrome staining. Details of the methods used here are described in Papeschi (1988) and Poggio et al. (2011). The slides made for C-banding were stained with 4′,6-diamidino-2-phenylindole (DAPI; Fluka BioChemika, Sigma Aldrich Production GmbH, Buchs, Switzerland) for a better resolution of C-bands (Barros e Silva and Guerra, 2010).
Meiotic Cell Spreads for Immunostaining
In adult males, we used the anterior region of sperm tubes or follicles, from the germarium to the zones of growth and maturation, where cysts of spermatogonia divide mitotically and the spermatocytes undergo meiotic divisions to avoid the presence of or minimize the amount of spermatozoa. In nymphs, we used the entire testes. Testes were dissected and processed according to a surface-spreading procedure previously described (Toscani et al., 2008). Briefly, cells were spread on a glass slide covered with 1% PFA and 0.15% Triton X-100, pH 8. Chromosome preparations were incubated for 2 h in a humid chamber at room temperature, then rinsed in 0.08% Kodak Photoflo for 1 min, and air-dried. Slides were used immediately for immunostaining or kept at −70°C for later use. Immunostaining was performed as previously described (Pigozzi and Solari, 2003; Viera et al., 2009b). To detect the cohesin axes of prophase chromosomes, we employed a polyclonal rabbit anti-SMC3 antibody (Millipore, ab3914) at 1:500 dilution. Histone H3 trimethylated at lysine 9 (H3K9me3) was revealed with a rabbit polyclonal serum (Abcam, ab-8898) at 1:200 dilution and histone H3 dimethylated at lysine 9 (H3K9me2) was detected with a monoclonal antibody raised in mouse (Abcam, ab1289) at 1:100 dilution. The secondary antibodies were TRITC- or FITC-labeled goat anti-rabbit, or anti-mouse (Jackson) at 1:100 dilution. Chromosome preparations were mounted in Vectashield with DAPI as counterstain.
Microscopy and Image Processing
Haematoxylin stained and C-banded chromosome preparations were scanned and photographed using an epifluorescence microscope Leica DMLB equipped with a Leica DFC350 FX CCD camera and the Leica IM50 version 4.0 software (Leica Microsystems Imaging Solutions Ltd., Cambridge, United Kingdom). Black-and-white images of chromosomes were recorded separately for each dye. Images were pseudocoloured (light blue for DAPI) and processed with Adobe Photoshop, version 7.0. Individual images for red (SMC3) and green fluorescence (H3K9me2 and H3K9me3) were processed and merged using Adobe Photoshop, version 7.0. Immunostained chromosome spreads were scanned and photographed using a Zeiss Axiophot epifluorescence microscope equipped with a CCD color camera Olympus DP70 and DP Manager version 1.2.1.107 software (Olympus optical Co, Ltd., Japan).
Results
We present the results of the cytogenetic analyses of the male meiosis in the six species, organized as follows: (1) karyotype and meiotic chromosome behavior, (2) amount and distribution of constitutive heterochromatin, and (3) immunolocalization of modified histone H3 (di- and trimethylated H3 at Lys9).
Karyotype and Meiotic Chromosome Behavior
The diploid chromosome number and male meiosis of Belostoma elegans, B. oxyurum, Holhymenia rubiginosa, Pachylis argentinus, and Phthia picta have been described in detail previously (Papeschi and Bidau, 1985; Papeschi, 1992; Papeschi et al., 2003; Bressa et al., 2005, 2008; Table 1). The male diploid chromosome number and meiosis of Oncopeltus unifasciatellus are described here for the first time.
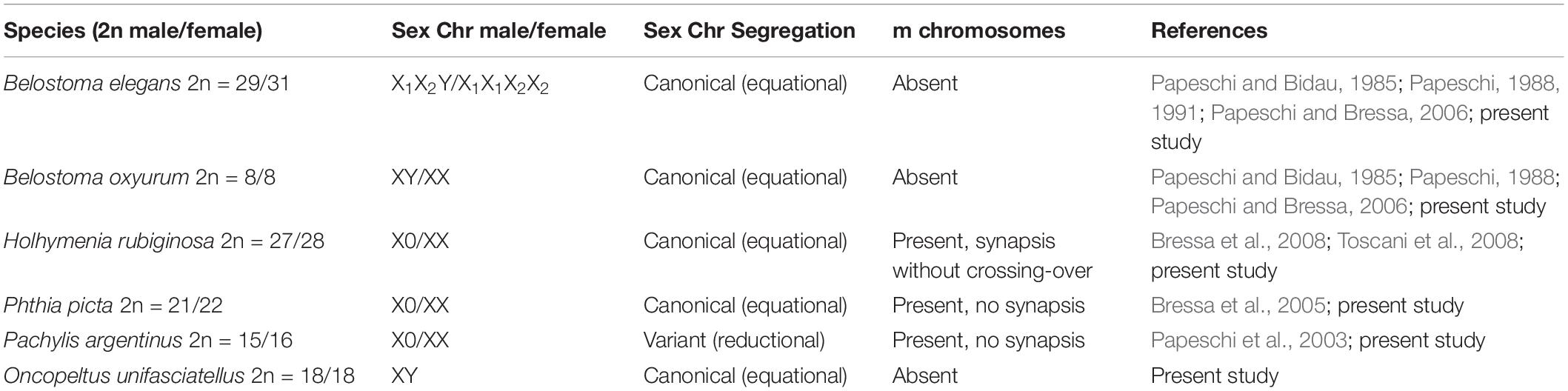
Table 1. Diploid chromosome complements, sex chromosome segregation in males, and meiotic behavior of the m chromosomes in the analyzed species.
Oncopeltus unifasciatellus has a male diploid chromosome number 2n = 16 + XY. Male meiotic karyotype is composed of eight autosomal bivalents of decreasing size and two sex chromosomes of similar size. During early prophase I, the X and Y were positively heteropyknotic and behaved as univalents throughout meiosis I (Figures 1A,B). However, they were also arranged close to each other until diakinesis. From leptotene up to the diffuse stage, a conspicuous nucleolus was observed immersed in autosomal chromatin. At the diffuse stage, autosomal bivalents did not completely decondense, whereas the X and Y chromosomes remained condensed and were positively heteropyknotic (Figures 1B,C). In diakinesis eight autosomal bivalents were recognized, along with the sex univalents that lay close to each other and became isopyknotic (Figure 1D). At metaphase I, the autosomal bivalents formed a ring, with the X and Y chromosomes in the center and apart from each other (Figure 1E). At anaphase I, the autosomes segregated reductionally whereas the sex chromosomes did so equationally and synchronously with the autosomes. All telophase I nuclei exhibited 10 chromosomes in each pole (8 + XY) (Figure 1F). At metaphase II, the autosomes formed a ring and the X and Y came close together and associated through “touch-and-go pairing” forming a pseudo-bivalent, which lay at the center of the ring (Figure 1G). At anaphase II, the autosomes divided equationally while the X and Y chromosomes did so reductionally. Females of O. unifasciatellus were not included in the analysis as no dividing cells were found in the ovaries.
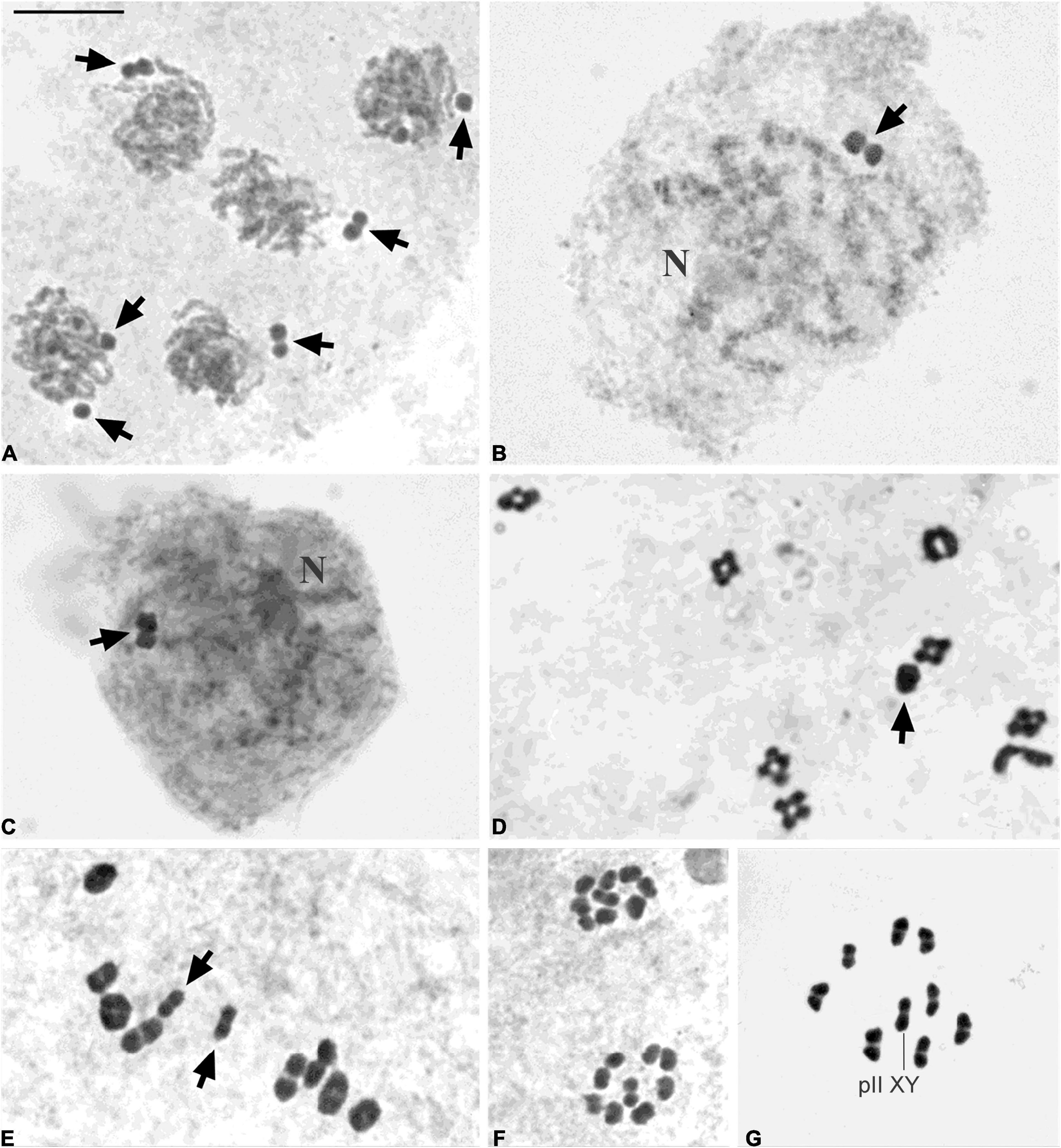
Figure 1. Male meiosis of Oncopeltus unifasciatellus (n = 8II + XY). (A) Leptotene-zygotene. (B) Pachytene. (C) Diffuse stage. (D) Diakinesis. (E) Metaphase I. (F) Telophase I. (G) Metaphase II. Arrows, sex chromosomes; N, nucleolus; pII XY, pseudo-bivalent XY. Chromosomes are stained with 2% acetic haematoxylin. Bar: 10 μm.
In P. picta, oogonia were cytogenetically analyzed for the first time to confirm the sex chromosome system of this species. The female chromosome complement comprises nine pairs of autosomes, one pair of sex chromosomes and one pair of m chromosomes, which are the smallest of the complement (2n = 18 + 2m + XX). In the oogonial and spermatogonial prometaphases, the m chromosomes pair was easily recognized, whereas the X chromosomes could not be identified (Figures 2E,F).
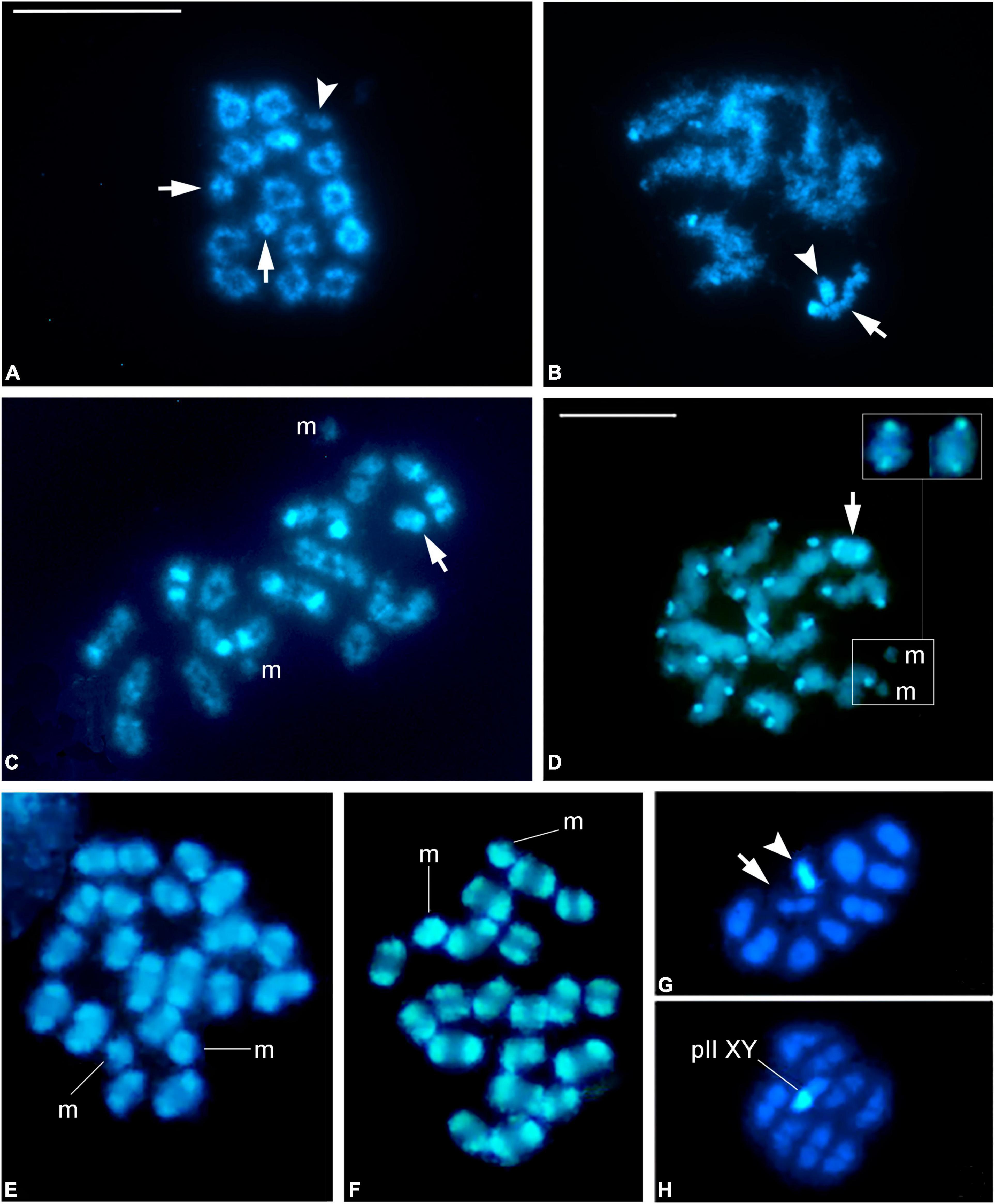
Figure 2. C-banding in male (A–D,F–H) and female (E) chromosome preparations. (A) Belostoma elegans (2n = 26 + X1X2Y, n = 13II + X1X2Y), diakinesis. (B) Belostoma oxyurum (2n = 6 + XY, n = 3II + XY), diplotene (C) Holhymenia rubiginosa (2n = 24 + 2m + X0, n = 12II + 2m + X0), diakinesis. (D) Pachylis argentinus (2n = 12 + 2m + X0, n = 6II + 2m + X0), spermatogonial prometaphase, and a detail of the minute C-bands of the m chromosomes on the upper left corner. (E,F) Phthia picta (2n = 18 + 2m + XX/X0, n = 9II + 2m + X0), oogonial prometaphase (E), and spermatogonial prometaphase (F). (G,H) Oncopeltus unifasciatellus (2n = 18 = 16 + XY, n = 8II + XY), metaphase I (G), and metaphase II (H). AII: autosomal bivalents. pII XY: pseudo-bivalent XY (H). Arrows point to X chromosomes (A–C,D,G). Arrowheads point to Y chromosomes (A,B,G). Chromosomes are stained with DAPI. Bar: 10 μm.
As for autosomes and sex chromosomes during meiotic divisions, B. elegans, B. oxyurum, H. rubiginosa, P. picta, and O. unifasciatellus showed the typical meiotic behavior: autosomes were synaptic, chiasmatic, and segregated reductionally during anaphase I, whereas the sex chromosomes were achiasmatic, behaved as univalents until metaphase I and then segregated equationally during the first anaphase (Papeschi and Bidau, 1985; Bressa et al., 2005, 2008; present study). In P. argentinus, however, all the individuals showed the variant X-chromosome behavior previously described, i.e., it segregated undivided to one pole at anaphase I and divided equationally at anaphase II (Papeschi et al., 2003; present study).
Amount and Distribution of Constitutive Heterochromatin
C-banding revealed noticeable differences in the amount and location of heterochromatin among the six species analyzed. The C-banding patterns of B. elegans and B. oxyurum have been described in detail previously (Papeschi and Bidau, 1985; Papeschi, 1988, 1995). Briefly, B. elegans had conspicuous telomeric C-positive bands in all autosomal bivalents and the X chromosomes, whereas the Y chromosome was C-negative (Figure 2A). The longest bivalent of B. oxyurum had a very small C-positive band at only one telomere, the medium-sized bivalent had C-positive bands at both telomeres, and the smallest bivalent had conspicuous C-positive bands at both telomeres. The X chromosome had a moderately C-positive band at one telomere and a stronger C-positive band at the other, whereas the Y chromosome was completely C-positive (Figure 2B).
On the other hand, H. rubiginosa had C-positive bands located at terminal, subterminal or interstitial regions on 10–17 autosomes. The m chromosomes were always C-negative and the X chromosome had a small C-positive band at a subterminal position that was not always discernible (Figure 2C; Bressa et al., 2008). In P. argentinus mitotic prometaphases, all the autosomes and the X chromosome had telomeric C-positive bands, whereas the m chromosomes had tiny telomeric C-positive bands which were detectable only if they were not fully condensed (Figure 2D). From meiotic prophase I onward, only very large C-positive bands could be detected terminally on all the autosomal bivalents as well as the X chromosome. In oogonial (2n = 22) and spermatogonial (2n = 21) mitotic metaphases of P. picta all the autosomes and the X chromosome had prominent C-positive bands at telomeric regions, whereas the m chromosomes were wholly C-positive (Bressa et al., 2005; Figures 2E,F). In O. unifasciatellus, only one of the sex chromosomes was completely C-positive. No C-bands were detected in the autosomes and the other sex chromosome (Figures 2G,H).
Spermatocyte Staging and Immunolocalization of Di- and Trimethylated H3 at Lys9
Spermatocytes at prophase I were classified by the immunofluorescent staining profiles of the autosomes with antibodies against SMC3 (Figure 3 and Supplementary Figure 1). At leptotene, SMC3 appears as discontinuous threads or spots in the entire nucleus; during zygotene, the presence of thicker SMC3 axes indicates the onset of synapsis that finalizes at pachytene. Diffuse stage spermatocytes, do not show axes, but a cloud of SMC3 is observed. Likewise, SMC3 labeling was not observed on the condensed chromosomes from diakinesis onward in agreement with previous findings in other heteropterans (Toscani et al., 2008; Viera et al., 2016). In addition, two different types of synaptic behavior were observed in the autosomal bivalents of the analyzed species. In B. elegans, B. oxyurum, P. picta, and O. unifasciatellus, synapsis occurs simultaneously at several points of the bivalents, as shown by the presence of thick SMC3 threads labeled with similar intensity (Figure 3 and Supplementary Figure 1). In P. argentinus, synapsis begins at one or a few points of each bivalent with the zygotene stage characterized by the presence of thinner and thicker SMC3 signals that represent unsynapsed and synapsed regions of the bivalents, respectively (Figure 3). Regardless these variations, in all the examined, the autosomal bivalents are fully synapsed at pachytene.
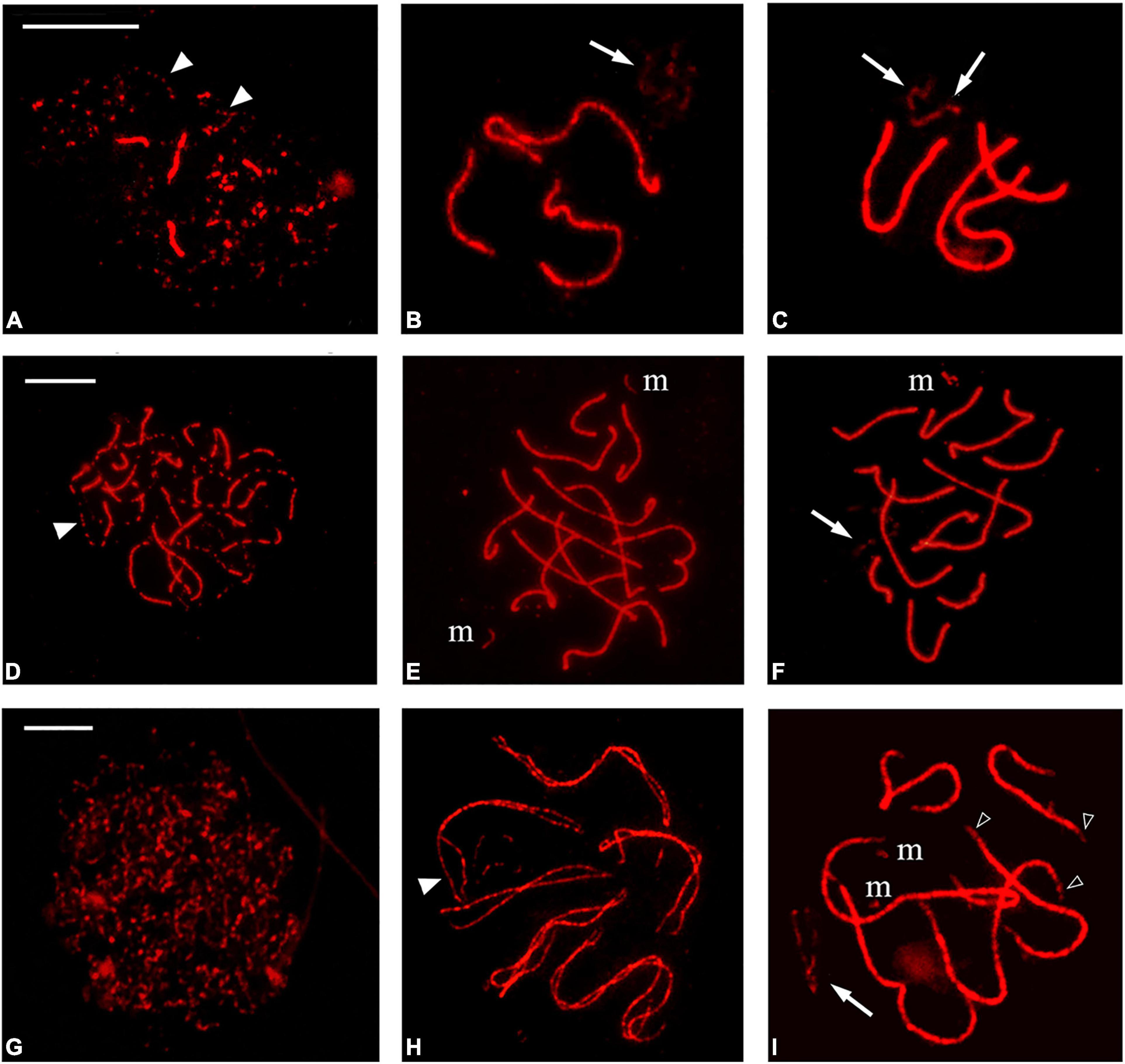
Figure 3. SMC3 distribution during male prophase I. In Belostoma oxyurum (A–C) and Holhymenia rubiginosa (D–F), SMC3 appears as discontinuous threads or spots that thicken as autosomal bivalents synapse. Non-synapsed segments are observed as discontinuous SMC3 threads (arrowheads). In Pachylis argentinus (G–I), synapsis progresses from different contact points along homologues and non-synapsed axes show continuous SMC3 labeling (white arrowheads). The end of the autosomal bivalents present thinner SMC3 axes (empty arrowheads). The m-chromosome axes were found separated in most nuclei of H. rubiginosa, but synapsis may occur (F). In P. argentinus, the m chromosomes were always observed separated. (A,D,G,H): zygotene stage. (B,C,E,F,I): pachytene stage. Arrows point to the SMC3 signal on the sex chromosomes. Bars: 10 μm.
Some species-specific features of the meiotic axes were detected, such as the thinning of the SMC3 signal at terminal positions of the autosomal bivalents in B. elegans and P. argentinus or the incomplete synapsis at terminal regions in P. picta (Figure 3I and Supplementary Figure 1). The m chromosomes could be identified as the shortest asynaptic axes in a variable proportion of pachytene nuclei of the three coreid species. In P. picta and P. argentinus the m chromosomes always remained separated; instead, in H. rubiginosa, the m chromosomes were found synapsed in 28% of the pachytene nuclei (15/54 nuclei), as assessed by the presence of a single SMC3 thread of similar thickness to autosomal bivalents (Figure 3 and Supplementary Figure 1). The SMC3 signal on the sex chromosome/s was variable between nuclei within the same species and also between species with the same chromosome system. As in the case of autosomal bivalents, SMC3 is no longer associated with the sex chromosomes at diakinesis, at least not at levels detectable by our methods.
We next examined the pattern of immunofluorescent signals of H3K9me3 and H3K9me2 (meH3 when we refer to both modified histones) on autosomes, sex chromosomes, and m chromosomes at prophase I. Both histone modifications are present in the regions identified as heterochromatic in C-banded autosomal chromosomes and also on the sex chromosome/s from early prophase I (Figure 4 and Supplementary Figures 2, 3). It should be mentioned that the labeling with meH3 seems to be influenced by the chromatin dispersion during the spreading procedure. As a consequence, constitutive heterochromatin blocks of similar size do not always have the same intensity in immunolabeled prophase I spreads. This is especially noticeable in both belostomatid species: B. elegans has large blocks of heterochromatin at telomeres compared to B. oxyurum but H3K9me3 signals are brighter and delimited more sharply in the later. In O. unifasciatellus a meH3 signal is detected on one of the autosomal bivalents with interstitial location, perhaps related to the nucleolus location (see Section “Discussion”) (Figures 4J–L).
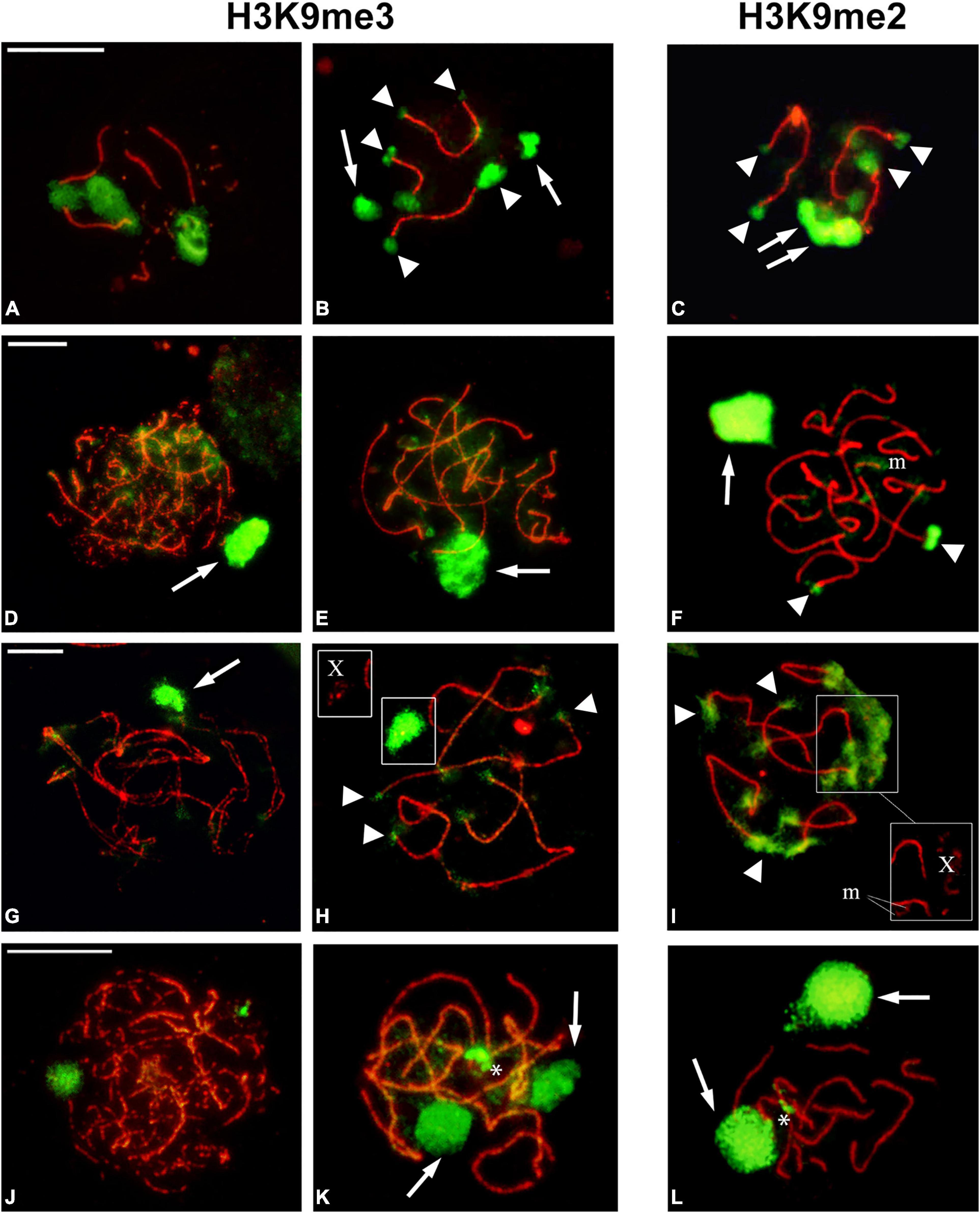
Figure 4. H3K9me2 and H3K9me3 distribution during male prophase I. (A–C) Belostoma oxyurum. (D–F) Holhymenia rubiginosa. (G–I) Pachylis argentinus. (J–L) Oncopeltus unifasciatellus. Methylated histones (green) label completely the sex chromosomes (arrows) and the heterochromatic blocks on the autosomal bivalents (arrowheads). The asterisks mark the interstitial heterochromatin in one of the autosomal bivalents of O. unifasciatellus. The insets in panels (H,I) show the SMC3 signal of the X chromosome. Meiotic axes were detected with an antibody against SMC3 (red). Bars: 10 μm.
On the m chromosomes, meH3 signals correlate with the presence of heterochromatin (see Section Amount and Distribution of Constitutive Heterochromatin). In P. picta, the m chromosomes are relatively large and completely heterochromatic and could be identified in immunolabeled nuclei even when autosomal synapsis is not complete (Supplementary Figures 3D,H). The tiny m chromosomes of P. argentinus appeared completely covered by meH3 signals (Figure 4I), whereas in H. rubiginosa they showed faint or no labeling (Figure 4F). The sex chromosomes are labeled by both modified histones enabling their identification from early prophase (Figure 4 and Supplementary Figures 2, 3).
In H. rubiginosa, P. picta, and P. argentinus we also established the patterns of meH3 on diakinesis/metaphase I chromosomes. In H. rubiginosa and P. picta both histone modifications are present on autosomal bivalents, but the X chromosome is devoid of H3K9me2 (Figure 5). That is, only H3K9me3 is associated with the sex chromosome during prophase and metaphase I in these two species. In P. argentinus, instead, H3K9me3 is no longer observed on the autosomal bivalents during metaphase I but it is present on the X chromosome (Figures 6A–F). On the other hand, H3K9me2 persists at both ends of the autosomes and at one end of the X chromosome (Figures 6G–L). Figure 7 summarizes the distribution of both methylated histones on the autosomal bivalents and sex chromosomes at prophase I and metaphase I. The distribution of meH3 at prophase I is common to all species, that is, both methylated histones are present on the sex chromosomes and the heterochromatin of autosomes. The meH3 distribution at metaphase I corresponds to that in coreid species with typical (equational) and variant (reductional) segregation of the sex chromosome.
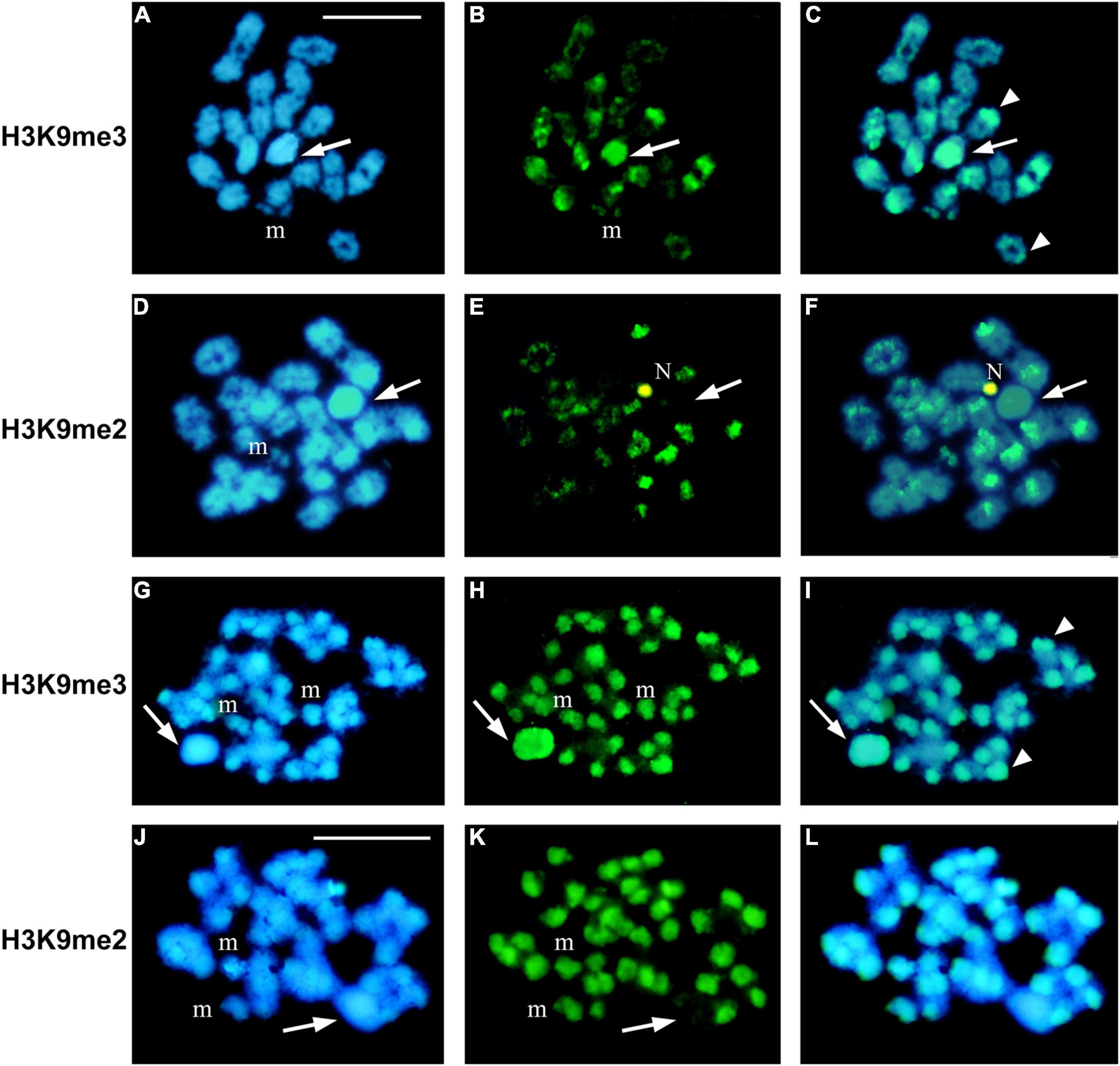
Figure 5. H3K9me2 and H3K9me3 distribution at metaphase I chromosomes in two species with typical sex chromosome segregation (equational division). (A–F) Holhymenia rubiginosa. (G–L) Phthia picta. Arrows point to the X chromosome and arrowheads to some heterochromatic blocks on the autosomal bivalents. In both species the sex chromosome shows clear labeling with H3K9me3 (A,B,G,H) while H3K9me2 is not detected (D,EJ,K). The m chromosomes (m) are associated in H. rubiginosa (A–F) and separated in P. picta (G,H). N, nucleolus. Blue color is for DAPI staining. Bars: 10 μm.
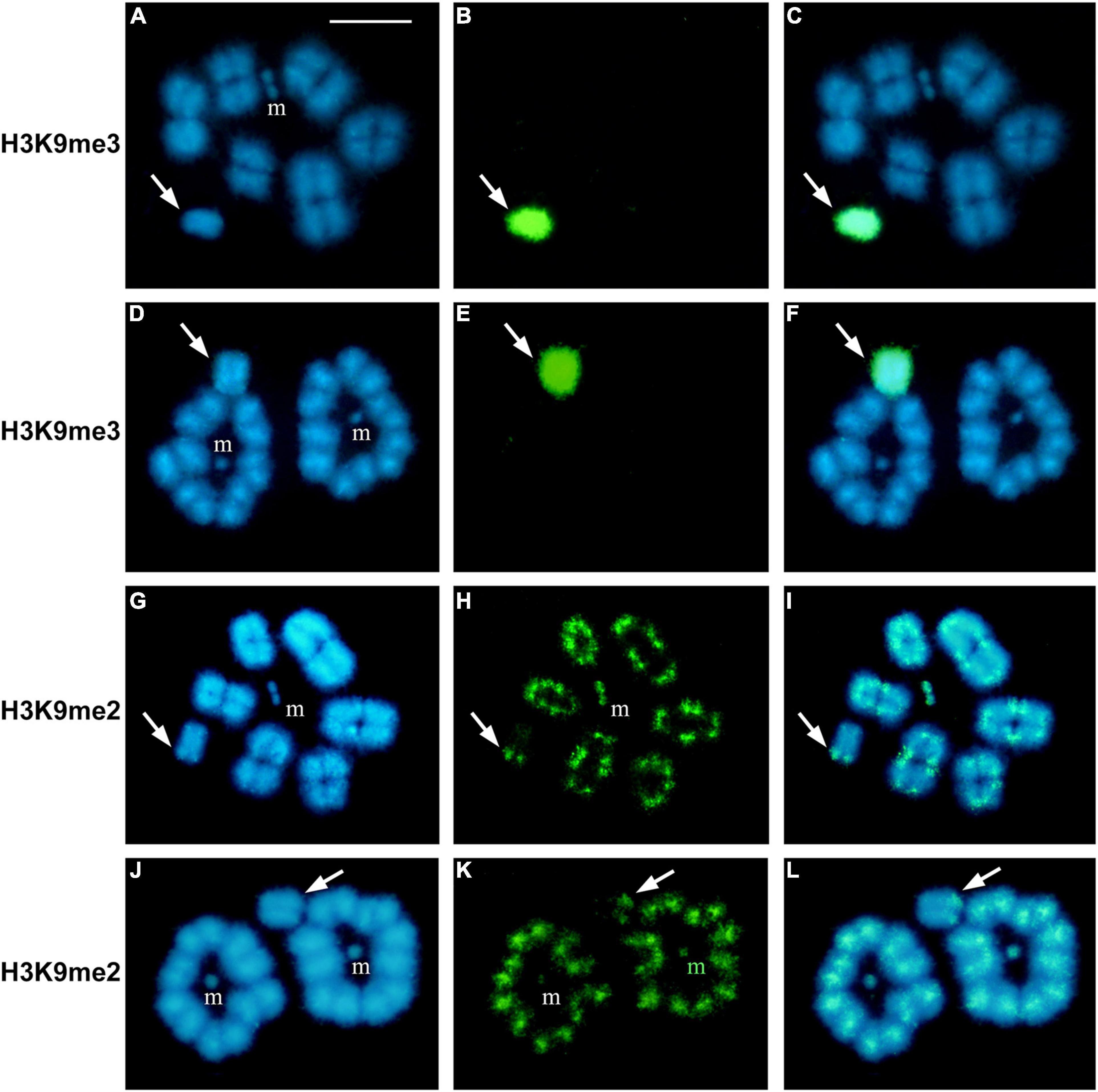
Figure 6. Immunostaining with antibodies against H3K9me2 and H3K9me3 in Pachylis argentinus showing reductional sex chromosome segregation during first meiotic division (variant). Meiotic chromosomes at metaphase I (A–C,G–I) and telophase I (D–F,J–L). The single X chromosome (arrows) is completely decorated with H3K9me3 while H3K9me2 is present at one end. Telophase nuclei show the reductional division of the X chromosome at first anaphase. Bars: 10 μm.
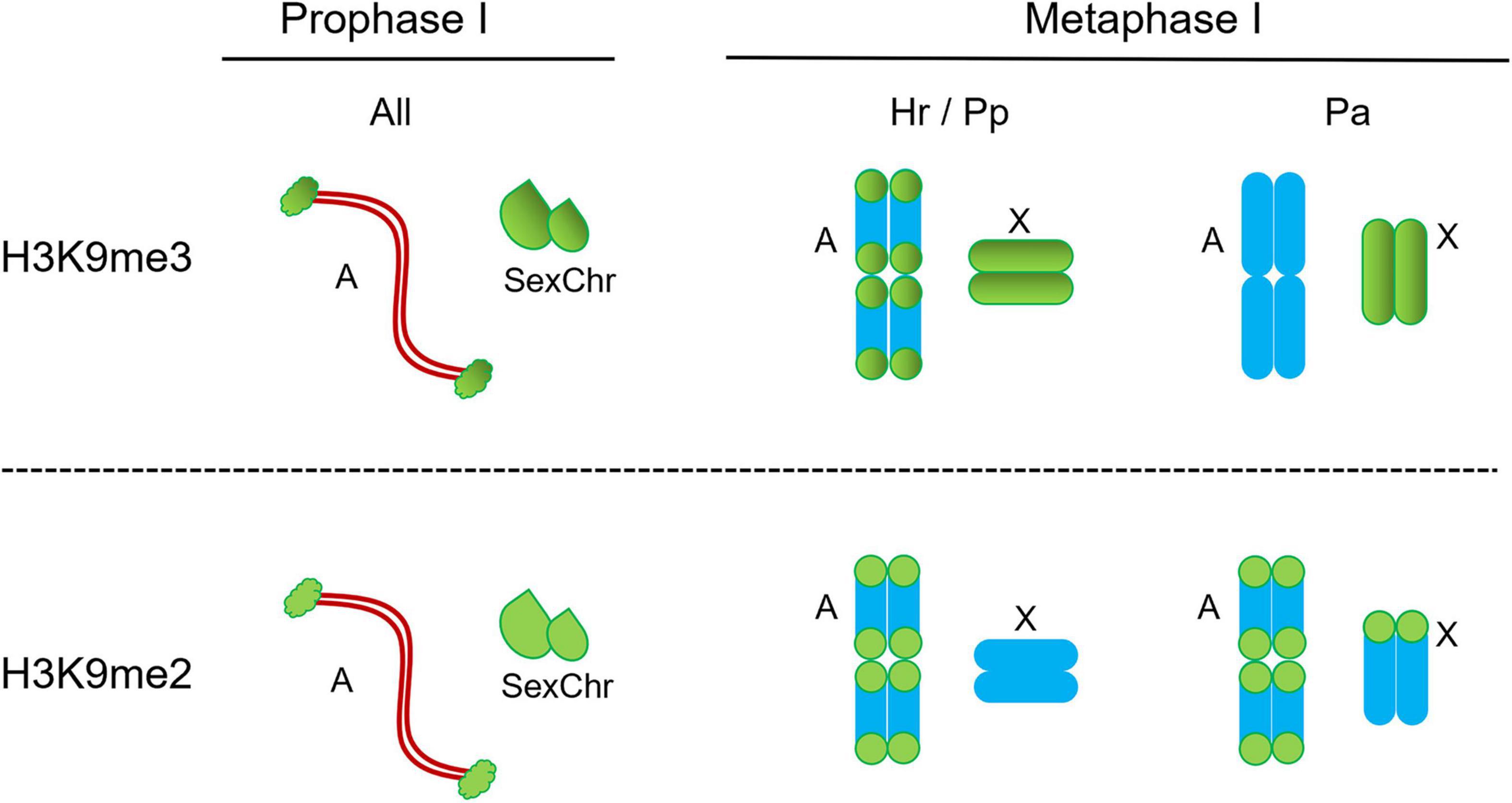
Figure 7. Schematic distribution of H3K9me2 and H3K9me3 on the autosomal bivalents and the sex chromosomes in species with typical and variant meiotic segregation. On the autosomal bivalents, the signals of methylated histone H3 are terminally located but they follow the distribution of C-banded heterochromatin in the examined species. Pp, Phthia picta; Hr, Holhymenia rubiginosa; Pa, Pachylis argentinus; AII, autosomal bivalents.
Discussion
Chromosome Features and Chromosome Evolution
Discussions on karyotype evolution in Heteroptera use the concept of modal chromosome number, i.e., the commonest chromosome number present in a group, and several times, the modal number is considered the ancestral one for the group under analysis (Ueshima, 1979; Manna, 1984; Papeschi and Bressa, 2006). This concept can be applied at a family level, but more often is applicable to lower categories such as tribes or genera (Ueshima, 1979). The variations of diploid number found have been mainly ascribed to dysploidy events, i.e., fragmentation and/or fusion, because other chromosome rearrangements, such as inversions and reciprocal translocations, have been rarely reported (Papeschi and Mola, 1990; Bressa et al., 1998; Pérez et al., 2004; Chirino et al., 2017). At the family level, karyotypes are highly homogeneous within certain groups, while others show intensive processes of karyotype variations.
Lygaeidae are a large and diverse heteropteran family in which the male diploid chromosome number ranges from 10 (Graphoralius novitus and Artheneidea tenuicornis) to 30 (Cymus sp., C. luridus, C. coriacipennis, and Cymodema sp.) of roughly 403 species cytogenetically analyzed (Ueshima, 1979; Ueshima and Ashlock, 1980; Grozeva and Kuznetsova, 1990, 1993; Bressa et al., 2002; Papeschi and Bressa, 2006; Souza et al., 2007; Kaur and Suman, 2009; Kaur et al., 2010; Bardella et al., 2014; Souza et al., 2014; Souza-Firmino et al., 2020). Within Lygaeidae, available data on 34 species of the subfamily Lygaeinae reveal that they are characterized by the lack m chromosomes, various male chromosome diploid numbers (12, 14, 15, 16, and 22), and an XY/XX (male/female) sex chromosome system (see references cited in Ueshima, 1979; Ueshima and Ashlock, 1980; Grozeva and Kuznetsova, 1993; Bressa et al., 2002; Bardella et al., 2014). Only two species have higher chromosome numbers, most probably due to fragmentation of autosomes: Lygaeus simulus (Parshad, 1957) and Oncopeltus famelicus (Ueshima and Ashlock, 1980) with 2n = 22 (20 + XY). Besides, Arocatus suboeneus is the only species of the subfamily that possesses a multiple sex chromosome system (2n = 15 = 12 + X1X2Y) that most probably originated through fragmentation of the ancestral X (Ueshima and Ashlock, 1980). Available cytogenetic reports of the genus Oncopeltus show two different male diploid chromosome numbers: 2n = 22 = 20 + XY in O. famelicus (Ueshima and Ashlock, 1980), and 2n = 16 = 14 + XY in O. fasciatus (Montgomery, 1901, 1906; Wilson, 1905; Wolfe and John, 1965; Ueshima and Ashlock, 1980), and O. nigriceps (Rao, 1955). In the present study, a novel chromosome complement found in specimens of O. unifasciatellus is described. The previous cytogenetic studies in Lygaeinae revealed that the most frequent chromosome complement is 2n = 14 with the simple system XY/XX (Ueshima, 1979; Ueshima and Ashlock, 1980; Grozeva and Kuznetsova, 1993; Bressa et al., 2002; Bardella et al., 2014). Thus, it is safe to assume that this modal chromosome number could be the ancestral complement of the subfamily, especially considering that it occurs in 25 species of 10 genera. Among the principal mechanisms of karyotype evolution, autosomal and autosomal-sex chromosome fusions and autosomal and sex chromosome fragmentations have seemingly played the most important role in Heteroptera (Ueshima, 1979; Manna, 1984; Thomas, 1987; Papeschi, 1994, 1996; Pérez et al., 2004; Papeschi and Bressa, 2006; Chirino and Bressa, 2014; Chirino et al., 2017). The diploid chromosomal number of O. unifasciatellus (2n = 16) can be considered as derived and would have originated from autosomal fragmentations whereas the sex chromosome system remained conserved. The presence of this novel chromosome complement allows deeper insight into the karyotype evolution of the other three species of Oncopeltus which could also have arisen through fragmentation of the autosomes.
The X and Y chromosomes have similar size and C-banding patterns; therefore, the analysis of the chromosome set in females did not help to identify them. However, in many heteropteran species with simple (XY, X0) and multiple sex chromosome systems, the X chromosome is facultatively heterochromatic and the Y chromosome is entirely constitutively heterochromatic (Papeschi, 1988; Panzera et al., 1995, 1998; Rebagliati et al., 2003; Cattani et al., 2004; Bressa et al., 2005, 2009; Poggio et al., 2007, 2014). Thus, we propose that the completely heterochromatic sex chromosome of O. unifasciatellus would be the Y chromosome.
The presence of an interstitial meH3 signal on one of the autosomal bivalents might be related to the presence of the nucleolus organizer region, since the nucleolus is associated to one autosomal bivalent in O. unifasciatellus (see Section “Results” and Figures 1B,C). The possibility of its occurrence is supported by the presence of only one interstitial DAPI-negative/CMA3-positive block on an autosomal pair in O. femoralis, which co-localizes with an 18S rDNA hybridization signal (Bardella et al., 2014). On the other hand, histone methylation is known to be associated with both transcriptionally active and repressive chromatin states (Briggs et al., 2001). It has been proposed that meH3 might have different functions depending on whether it occurs in coding regions or in promoters. Another possibility is that the outcome of meH3 could be gene dependent. For example, certain genes can be active despite presumably being enriched in meH3 (Weiler and Wakimoto, 1995; Vakoc et al., 2005). Considering the previous reports together with the results presented here, we suggest that in O. unifasciatellus H3K9me2 and H3K9me3 co-localization on the rDNA locus can help to program ribosomal genes in a transcriptionally competent configuration.
Heterochromatin, Chiasmata, and Meiotic Axis Formation
It has been proposed that constitutive heterochromatin has an important role in homologous chromosome pairing in meiosis and some negative effects on meiotic pairing and crossing-over (Sumner, 2003). In a large number of organisms, including plants, insects, and vertebrates, crossing-over is severely reduced or absent in heterochromatin and often associated with the absence of synaptonemal complex formation or changes in its structure (Stack, 1984; John, 1988, 1990). In Heteroptera, the presence of heterochromatic terminal blocks on the autosomal bivalents prevents synapsis at chromosome ends that are observed in repulsion in preparations made using classical cytogenetic techniques (Toscani et al., 2011; Poggio et al., 2014). A previous study has shown that constitutive heterochromatin is accumulated at terminal positions as a consequence of the lack of recombination near the chromosome ends in P. picta (Bressa et al., 2005). In this study, the immunolocalization of H3K9me2, H3K9me3, and SMC3 has allowed a detailed analysis of the relationship between meiotic axis and constitutive heterochromatin. Some species-specific features of the meiotic axes are detected, i.e., the thinning of the SMC3 signal at terminal positions of the autosomal bivalents in B. elegans and P. argentinus, or the incomplete synapsis at the telomeric regions in P. picta. These results suggest that constitutive heterochromatin can differentially affect meiotic axis formation and therefore the homologous chromosomes pairing. Besides, the large size of the terminal heterochromatic regions of P. picta would prevent their ends from synapsing. According to the results observed in the six species here analyzed, we suggest that constitutive heterochromatin would affect the formation and morphology of the meiotic axis.
In the spermatocytes of Heteroptera, the SMC3α and SMC3 subunits, as well as the meiosis-specific cohesin REC8 are part of the meiotic axes and follow similar association/dissociation dynamics throughout prophase I. From leptotene to late pachytene, these cohesins are present on meiotic axes, dissociate at the diffuse stage and are no longer detected during later meiotic stages (Pigozzi and Solari, 2003; Viera et al., 2009b). Despite the dissociation of these cohesins, the sister chromatids of autosomal bivalents remain together until they separate at the second division. A similar cycle of SMC3 can be observed on the X chromosome of P. argentinus where sister chromatids remain together after the first meiotic division. The molecular basis that holds chromatids together at anaphase I in Heteroptera remains undisclosed. Since there are no centromeres to protect cohesion in holokinetic chromosomes in meiosis I, the chiasma provides an alternative reference point. In Heteroptera, one chiasma persists on each autosomal bivalent until the end of metaphase I and holds the four chromatids in each autosomal bivalent. This helps to align the bivalents at the metaphase plate and ensures their axial orientation, i.e., with the long axes parallel to the polar axis (Ueshima, 1979; Papeschi, 1994; Pérez et al., 2004; Mola and Papeschi, 2006; Nokkala et al., 2006; Papeschi and Bressa, 2006). The gradual loss of sister chromatid cohesion in Caenorhabditis elegans and Luzula species depends on the orientation of the autosomal bivalents on the equatorial plate (Bureš et al., 2013). In the autosomal bivalents of Heteroptera, that are axially oriented, cohesion is released in those parts of the bivalent that lie along the equatorial plate, allowing segregation to opposite poles during meiosis I, while the chromatid parts that lie above and below the equatorial plate remain connected (Nokkala et al., 2004). Considering all these data, the orientation of the X chromosome in P. argentinus with its long axis parallel to the spindle at metaphase I, like each homolog of an autosomal bivalent, would mediate the release of sister chromatid cohesion during meiosis II.
The Relationship of Sex Chromosome Condensation and Synapsis
As described in the results, the labeling of H3K9me2 and H3K9me3 on the sex chromosomes is observed from early zygotene. This pattern throughout prophase I is common to all the analyzed species, irrespective of the sex chromosome system and the presence of constitutive heterochromatin on the sex chromosomes. The independence of C-positive heterochromatin and the condensed state of the sex chromosomes is illustrated by the example of the X chromosome of P. picta, which is heterochromatic only at one end but is completely decorated with meH3 during prophase I. Similarly, in O. unifasciatellus the X chromosome is euchromatic and the Y is completely heterochromatic, but both have meH3 labeling. The present results show that H3K9me2 and H3K9me3 are associated with the sex chromosomes from early meiosis in species with different sex chromosome systems and different amount of constitutive heterochromatin. These results are in agreement with observations in two pentatomid bugs where the staining of H3K9me3 increases from mid-zygotene on the X and Y chromosomes (Viera et al., 2016). Hypermethylation of histone H3 is a canonical mark of heterochromatin and gene silencing [reviewed in Bannister and Kouzarides (2011)], suggesting that asynaptic sex chromosomes are transcriptionally silenced in male bugs. Inactivation of unpaired DNA or unpaired chromosome segments, termed meiotic silencing of unpaired chromatin (MSUC) is evolutionarily conserved in eukaryotes and has been well characterized in filamentous fungi, nematodes, yeast and mammals (Shiu et al., 2001; Schimenti, 2005). In mice and humans, this meiotic silencing targets unsynapsed regions that may be present due to mutations or chromosome rearrangements and it is common to both males and females (Handel, 2004; Schimenti, 2005; Turner et al., 2005; Kelly and Aramayo, 2007). Another example of MSUC is the meiotic inactivation of the sex chromosomes (MSCI), which leads to the formation of the XY body in pachytene spermatocytes in eutherian mammals when the autosomal bivalents have synapsed (Solari, 1974; Turner, 2007). MSCI involves the transcriptional silencing of the unpaired segments of the X and Y chromosomes during pachytene when these segments are enriched in numerous epigenetic signals, including some modified histones and histone variants (Cowell et al., 2002; Fernandez-Capetillo et al., 2003; Khalil et al., 2004; Turner et al., 2004). In contrast to meiotic sex chromosome inactivation in mammals, repressive marks on heteropteran sex chromosomes are observed when autosomal bivalents still have substantial amounts of non-synapsed regions. This shows that the timing of meiotic silencing, despite its widespread occurrence in different organisms, is variable and probably depends on different mechanisms.
Does H3K9me2 Play a Role in the Differential Behavior of Autosomes and Sex Chromosomes in Heteroptera?
In male Heteroptera with canonical chromosome segregation, autosomes restrict their kinetic activity to the bivalent ends (telokinetic meiosis), while the sex chromosomes undergo inverted meiosis (Hughes-Schrader and Schrader, 1961; Pérez et al., 2000; Mola and Papeschi, 2006; Viera et al., 2009a). The telokinetic ends face the poles in both the autosomal half-bivalents and the sex chromatids, and the spindle fibers attach directly to the chromatin in the absence of a kinetochore plate (Comings and Okada, 1972; Motzko and Ruthmann, 1984). This kinetic activity can alternatively occur at either chromosome end, regardless the position of the chiasma, and it changes its location from one chromosome end at the first meiotic division to the opposite end at the second meiotic division (González-García et al., 1996; Pérez et al., 1997; Poggio et al., 2011). As shown here, H3K9me2 is lost from the sex chromosomes in species with typical chromosome segregation (equational first meiotic division and reductional second meiotic division), but remains associated with autosomal bivalents (reductional first meiotic division and equational second meiotic division). We suggest that the loss of this histone modification only in sex chromosomes may be part of a machinery that enables the distinction of autosomes and sex chromosomes during anaphase I in Heteroptera. The differential distribution of H3K9me2 in the autosomal bivalents and the X chromosome of H. rubiginosa and P. picta may be part of an epigenetic signal to distinguish the reductional vs. equational division of half bivalents and the X chromatids. If H3K9me2-enriched heterochromatin is not located at the chromosome ends, as it is in some bivalents of H. rubiginosa, the signal should be able to act on terminal, cis-located sequences. The idea that H3K9me2 plays a role in distinguishing between reductional and equational segregation is supported by its prevalence in P. argentinus, a species in which both the autosomal bivalents and the X sex chromosome divide reductionally at the first anaphase. In this species, H3K9me2 is retained on autosomal bivalents and on one end of the X chromosome beyond the first anaphase. The undivided X chromosome of P. argentinus segregates toward one of the poles during first anaphase together with the autosomes and an m chromosome, resulting at metaphase II cells with and without the X chromosome (Papeschi et al., 2003). If the H3K9me2 signal present at one end of the X chromosome in this species is involved either directly or indirectly in microtubule binding, the timing of distribution of H3K9me2 could be related to the reductional behavior of the X chromosome at anaphase I. The absence of this modified histone on the X chromosome of H. rubiginosa and P. picta supports this assumption, as all the three species have telomeric C-positive bands (Bressa et al., 2005, 2008) but only in P. argentinus does the H3K9me2 mark persists at one end of the X chromosome at metaphase I.
Several lines of evidence indicate that kinetic activity is regulated by an epigenetic control rather than depending on a specific nucleotide sequence. Species with monocentric chromosomes show considerable variation in the architecture and scale of the centromeric repetitive elements, while the presence of a centromere-specific histone H3 variant (CenH3) is common to all functional centromeres. This H3 modification is widely distributed in eukaryotes, plays a key role in the segregation of chromosomes in mitosis and meiosis and, together with other factors, determines the identity of the centromere (Monen et al., 2005; Panchenko and Black, 2009; Lermontova et al., 2011; Prosée et al., 2020). Another conserved feature of centromeres is their localization at heterochromatic regions in organisms with heterologous centromere systems, both holokinetic or monocentric, suggesting that the heterochromatic state favors the localization of centromere-specific components (Henikoff et al., 2000). The role of chromatin architecture might also be central to the binding of microtubules bundles in meiotic divisions of heteropterans as these insects lack the typical trilaminar kinetochore plates (Comings and Okada, 1972; Motzko and Ruthmann, 1984). In contrast to most eukaryotes, the genome of insects with holokinetic chromosomes, including heteropterans, lacks the crucial centromere component CenH3 (Drinnenberg et al., 2014). This heterogenous trait involved in the kinetic identity of different holokinetic systems may be explained by the fact that holocentricity evolved several times in the tree of life from ancestral monocentric chromosomes (Mola and Papeschi, 2006; Melters et al., 2012). Insects with holokinetic chromosomes have found a solution for centromeric activity that is independent of CenH3. Given the prominent role of epigenetic modifications in the centromere function, and based on our present observations, we proposed that H3K9me2 may have been recruited to promote the meiotic chromosome segregation in Heteroptera in the absence of CenH3. Taking into account the diversity of this group of insects, other chromatin modifications are likely involved in the complex mechanisms behind chromosome segregation. Exploring the modifications of the chromatin during male meiotic divisions in other Heteroptera, especially from early divergent families, is necessary to strengthen our present conclusions.
The requirements for centromere identity and function in Heteroptera are one of many intriguing questions about chromosome behavior, as is the occurrence of reductional and equational segregation within the same meiotic nucleus. Concerted cytogenetic and genomic research on a few selected species could shed light on the molecular mechanisms underlying this basic issue.
Data Availability Statement
The raw data supporting the conclusions of this article will be made available by the authors, without undue reservation.
Author Contributions
MT collected the material, performed the experiments, analyzed the data, took microscope images, prepared figures and/or tables, and reviewed the manuscript. MP analyzed the data, prepared and wrote the manuscript, prepared figures and/or tables, discussed the results, and reviewed the literature. AP conducted the research work. MB analyzed the data, took microscope images, prepared and wrote the manuscript, prepared figures and/or tables, discussed the results, and reviewed the literature. All authors approved the final version of the manuscript.
Funding
This study was funded by Agencia Nacional de Promoción Científica y Tecnológica (ANPCyT) [Fondo para la Investigación Científica y Tecnológica, Grant Number PICT 2007-00635] and Universidad de Buenos Aires (UBA) [Secretaría de Ciencia y Técnica, Grant Numbers UBACyT X164 and UBACyT W917] from Argentina.
Conflict of Interest
The authors declare that the research was conducted in the absence of any commercial or financial relationships that could be construed as a potential conflict of interest.
Publisher’s Note
All claims expressed in this article are solely those of the authors and do not necessarily represent those of their affiliated organizations, or those of the publisher, the editors and the reviewers. Any product that may be evaluated in this article, or claim that may be made by its manufacturer, is not guaranteed or endorsed by the publisher.
Acknowledgments
Taxonomic determination of the specimens was performed by Axel Bachmann (Facultad de Ciencias Exactas y Naturales, Universidad de Buenos Aires) (Belostomatidae), Harry Brailovsky (Universidad Nacional Autónoma de México) (Coreidae), and Pablo Dellapé (Facultad de Ciencias Naturales y Museo, Universidad Nacional de La Plata) (Lygaeidae). MB and MP thank Consejo Nacional de Investigaciones Científicas y Técnicas (CONICET) and Universidad de Buenos Aires, Argentina.
Supplementary Material
The Supplementary Material for this article can be found online at: https://www.frontiersin.org/articles/10.3389/fevo.2022.836786/full#supplementary-material
Supplementary Figure 1 | SMC3 distribution during prophase I. (A–D) Belostoma elegans, leptotene (A), zygotene (B), early pachytene (C), pachytene (D). (E–H) Phthia picta, leptotene (E), zygotene (F), zygotene-pachytene (G), pachytene (H). The m chromosomes form single meiotic axes. (I–L) Oncopeltus unifasciatellus, leptotene (I), zygotene (J), late zygotene (K), pachytene (L). Arrows point to the SMC3 signal on the sex chromosomes. Arrowheads (white) point to synapsed meiotic axes. Arrowheads (yellow) point to simple discontinuous meiotic axes. N: nucleolus. Bars: 10 μm.
Supplementary Figure 2 | H3K9me3 and H3K9me2 distribution at pachytene in Belostoma elegans. Arrows point to the SMC3 axes of the sex chromosomes (A,C). Panels (B,D) show the same nuclei with meH3 labeling. (E) The inset shows in detail the thinning of the synaptic SMC3 axes at the end of one autosomal bivalent (asterisk) and their meH3 labeling.
Supplementary Figure 3 | H3K9me3 and H3K9me2 distribution at prophase I in Phthia picta. (A,E,I) Leptotene. (B,F,J) Pachytene. (C,G,K) Zygotene. (D,H,L) Pachytene. Arrows point to X chromosome. Arrowheads point to regions that stain brighter with DAPI and are labeled with H3K9me3 and H3K9me2. m: m chromosome axes. The inset shows an enlarged region in panel (H) corresponding to the unsynapsed SMC3 axes of an autosomal bivalent (asterisk). Bar (A–D): 10 μm. Bar (inset): 2 μm.
References
Bannister, A. J., and Kouzarides, T. (2011). Regulation of chromatin by histone modifications. Cell Res. 21, 381–395. doi: 10.1038/cr.2011.22
Bardella, V. B., Sampaio, T. R., Venturelli, N. B., Dias, A. L., Giuliano-Caetano, L., Fernandes, J. A., et al. (2014). Physical mapping of 18S rDNA and heterochromatin in species of family Lygaeidae (Hemiptera: Heteroptera). Genet. Mol. Res. 13, 2186–2199. doi: 10.4238/2014
Barros e Silva, A. E., and Guerra, M. (2010). The meaning of DAPI bands observed after C-banding and FISH procedures. Biotech. Histochem. 85, 115–125. doi: 10.1080/10520290903149596
Bressa, M. J., Franco, M. J., Toscani, M. A., and Papeschi, A. G. (2008). Heterochromatin heteromorphism in Holhymenia rubiginosa (Heteroptera: Coreidae). Eur. J. Entomol. 105, 65–72. doi: 10.14411/eje.2008.009
Bressa, M. J., Larramendy, M. L., and Papeschi, A. G. (2005). Heterochromatin characterization in five species of Heteroptera. Genetica 124, 307–317. doi: 10.1007/s10709-005-4524-3
Bressa, M. J., Papeschi, A. G., and Larramendy, M. L. (2002). Meiotic studies in Lygaeus alboornatus Blanchard 1852 (Heteroptera, Lygaeidae, Lygaeinae). Caryologia 55, 15–19. doi: 10.1080/00087114.2002.10589253
Bressa, M. J., Papeschi, A. G., Mola, L. M., and Larramendy, M. L. (1998). Meiotic studies in Largus rufipennis (Castelnau) (Largidae, Heteroptera). II. Reciprocal traslocation heterizygosity. Caryologia 51, 253–264. doi: 10.1080/00087114.1998.10797417
Bressa, M. J., Papeschi, A. G., Vítková, M., Kubícková, S., Fuková, I., Pigozzi, M. I., et al. (2009). Sex chromosome evolution in cotton stainers of the genus Dysdercus (Heteroptera: Pyrrhocoridae). Cytogenet. Genome Res. 125, 292–305. doi: 10.1159/000235936
Briggs, S. D., Bryk, M., Strahl, B. D., Cheung, W. L., Davie, J. K., Dent, S. Y., et al. (2001). Histone H3 lysine 4 methylation is mediated by Set1 and required for cell growth and rDNA silencing in Saccharomyces cerevisiae. Genes Dev. 15, 3286–3295. doi: 10.1101/gad.940201
Bureš, P., Zedek, F., and Marková, M. (2013). “Holocentric Chromosomes,” in Plant Genome Diversity, eds J. Greilhuber, J. Dolezel, and J. Wendel (Vienna: Springer), 187–208. doi: 10.1007/978-3-7091-1160-4_12
Cattani, M. V., Greizerstein, E. J., and Papeschi, A. G. (2004). Male meiotic behaviour and nucleolus organizer regions in Camptischium clavipes (Fabr.) (Coreidae, Heteroptera) analyzed by fluorescent banding and in situ hybridization. Caryologia 57, 267–273. doi: 10.1080/00087114.2004.10589403
Chirino, M. G., and Bressa, M. J. (2014). Karyotype evolution in progress: a new diploid number in Belostoma candidulum (Heteroptera: Belostomatidae) from Argentina leading to new insights into its ecology and evolution. Eur. J. Entomol. 111, 165–174. doi: 10.14411/eje.2014.027
Chirino, M. G., Dalíková, M., Marec, F., and Bressa, M. J. (2017). Chromosomal distribution of interstitial telomeric sequences as signs of evolution through chromosome fusion in six species of the giant water bugs (Hemiptera, Belostoma). Ecol. Evol. 7, 5227–5235. doi: 10.1002/ece3.3098
Chirino, M. G., Papeschi, A. G., and Bressa, M. J. (2013). The significance of cytogenetics for the study of karyotype evolution and taxonomy of water bugs (Heteroptera, Belostomatidae) native to Argentina. Comp. Cytogen. 7, 111–129. doi: 10.3897/compcytogen.v7i2.4462
Clough, E., Moon, W., Wang, S., Smith, K., and Hazelrigg, T. (2007). Histone methylation is required for oogenesis in Drosophila. Development 134, 157–165. doi: 10.1242/dev.02698
Comings, D. E., and Okada, T. A. (1972). Holocentric chromosomes in Oncopeltus: kinetochore plates are present in mitosis but absent in meiosis. Chromosoma 37, 177–192. doi: 10.1007/BF00284937
Cowell, I. G., Aucott, R., Mahadevaiah, S. K., Burgoyne, P. S., Huskisson, N., Bongiorni, S., et al. (2002). Heterochromatin, HP1 and methylation at lysine 9 of histone H3 in animals. Chromosoma 111, 22–36. doi: 10.1007/s00412-002-0182-8
Drinnenberg, I. A., deYoung, D., Henikoff, S., and Malik, H. S. (2014). Recurrent loss of CenH3 is associated with independent transitions to holocentricity in insects. Elife 3:e03676. doi: 10.7554/eLife.03676
Fernandez-Capetillo, O., Mahadevaiah, S. K., Celeste, A., Romanienko, P. J., Camerini-Otero, R. D., Bonner, W. M., et al. (2003). H2AX is required for chromatin remodeling and inactivation of sex chromosomes in male mouse meiosis Dev. Cell 4, 497–508. doi: 10.1016/s1534-5807(03)00093-5
García-Cao, M., O’Sullivan, R., Peters, A. H. F. M., Jenuwein, T., and Blasco, M. A. (2004). Epigenetic regulation of telomere length in mammalian cells by the Suv39h1 and Suv39h2 histone methyltransferases. Nat. Genet. 36, 94–99. doi: 10.1038/ng1278
Gokhman, V. E., and Kuznetsova, V. G. (2006). Comparative insect karyology: current state and applications. Entomol. Rev. 86, 352–368. doi: 10.1134/S0013873806030110
Golub, N. V., Golub, V. B., and Kuznetsova, V. G. (2018). New data on karyotypes of lace bugs (Tingidae, Cimicomorpha, Hemiptera) with analysis of the 18S rDNA clusters distribution. Comp. Cytogen. 12, 515–528. doi: 10.3897/CompCytogen.v12i4.30431
González-García, J. M., Antonio, C., Suja, J. A., and Rufas, J. S. (1996). Meiosis in holocentric chromosomes: kinetic activity is randomly restricted to the chromatid ends of sex univalents in Graphosoma italicum (Heteroptera). Chromosome Res. 4, 124–132. doi: 10.1007/BF02259705
Grozeva, S., and Kuznetsova, V. (1990). Karyotypes and some structural properties of the reproductive system of bugs of the subfamily Artheneinae (Heteroptera, Pentatomomorpha, Lygaeidae). Entomol. Rev. 69, 14–26.
Grozeva, S., and Kuznetsova, V. (1993). Notes on the karyotypes of some lygaeid bugs (Heteroptera, Pentatomomorpha, Lygaeidae). Folia Biol. 41, 65–75.
Grozeva, S., Kuznetsova, V., and Nokkala, S. (2004). Patterns of chromosome banding in four nabid species (Heteroptera, Cimicomorpha, Nabidae) with high chromosome number karyotypes. Hereditas 140, 99–104. doi: 10.1111/j.1601-5223.2004.01782.x
Grozeva, S., Kuznetsova, V. G., Simov, N., Langourov, M., and Dalakchieva, S. (2013). “Sex chromosome pre-reduction in male meiosis of Lethocerus patruelis (Stål, 1854) (Heteroptera, Belostomatidae) with some notes on the distribution of the species,” in Advances in Hemipterology, Vol. 319, eds A. Popov, S. Grozeva, N. Simov, and E. Tasheva (Bulgaria: ZooKeys), 119–135. doi: 10.3897/zookeys.319.4384
Grozeva, S., and Nokkala, S. (1996). Chromosomes and their meiotic behaviour in two families of the primitive infraorder Dipsocoromorpha (Heteroptera). Hereditas 125, 31–36. doi: 10.1111/j.1601-5223.1996.t01-1-00031.x
Grozeva, S., Nokkala, S., and Simov, N. (2006). First evidence of sex chromosome pre-reduction in male meiosis in the Miridae bugs (Heteroptera). Folia Biol. 54, 9–12. doi: 10.3409/173491606777919166
Grozeva, S., Nokkala, S., and Simov, N. (2009). Chiasmate male meiosis in six species of water bugs from infraorders Nepomorpha and Gerromorpha (Insecta: Heteroptera). Comp. Cytogen. 3, 125–130. doi: 10.3897/compcytogen.v3i2.19
Handel, M. A. (2004). The XY body: a specialized meiotic chromatin domain. Exp. Cell. Res. 296, 57–63. doi: 10.1016/j.yexcr.2004.03.008
Henikoff, S., Ahmad, K., Platero, J. S., and van Steensel, B. (2000). Heterochromatic deposition of centromeric histone H3-like proteins. Proc. Natl. Acad. Sci. U. S. A. 97, 716–721. doi: 10.1073/pnas.97.2.716
Henking, H. (1891). Untersuchungen über die ersten Entwicklungsvorgänge in den Eiern der Insekten. II. Über Spermatogenese und deren Beziehung zur Eientwicklung bei Pyrrhocoris apterus L. Z. wiss. Zoology 51, 685–736.
Hughes-Schrader, S., and Schrader, F. (1961). The kinetochore of the Hemiptera. Chromosoma 12, 327–350. doi: 10.1007/BF00328928
Ivanovska, I., and Orr-Weaver, T. L. (2006). Histone modifications and the chromatin scaffold for meiotic chromosome architecture. Cell Cycle 5, 2064–2071. doi: 10.4161/cc.5.18.3253
Jauset, A. M., Edo-Tena, E., Castañé, C., Agustí, N., Alomar, O., and Grozeva, S. (2015). Comparative cytogenetic study of three Macrolophus species (Heteroptera, Miridae). Comp. Cytogen. 9, 613–623. doi: 10.3897/CompCytogen.v9i4.5530
John, B. (1988). “The biology of heterochromatin,” in Heterochromatin: Molecular and Structural Aspects, ed. R. S. Verma (Cambridge: Cambridge University Press), 1–147. doi: 10.1101/pdb.top079889
Kaur, H., and Suman, V. (2009). Chromosomes and their meiotic behaviour in two species of Dieuches Dohrn, 1860 (Heteroptera: Lygaeidae: Rhyparochromini). Comp. Cytogen. 3, 43–50. doi: 10.3897/compcytogen.v3i1.7
Kaur, H., Suman, V., and Kaur, R. (2010). First report on C-banding and fluorescent banding in species of Dieuches (Rhyparochrominae: Lygaeidae: Heteroptera). Entomol. Res. 40, 1–7. doi: 10.1111/j.1748-5967.2009.00255.x
Kelly, W. G., and Aramayo, R. (2007). Meiotic silencing and the epigenetics of sex. Chromosome Res. 15, 633–651. doi: 10.1007/s10577-007-1143-0
Khalil, A. M., Boyar, F. Z., and Driscoll, D. J. (2004). Dynamic histone modifications mark sex chromosome inactivation and reactivation during mammalian spermatogenesis. Proc. Natl. Acad. Sci. U. S. A. 101, 16583–16587. doi: 10.1073/pnas.0406325101
Kuznetsova, V. G., Gavrilov-Zimin, I. A., Grozeva, S. M., and Golub, N. V. (2021). Comparative analysis of chromosome numbers and sex chromosome systems in Paraneoptera (Insecta). Comp. Cytogen. 15, 279–327. doi: 10.3897/compcytogen.v15.i3.71866
Kuznetsova, V. G., Grozeva, S. M., Nokkala, S., and Nokkala, C. (2011). Cytogenetics of the true bug infraorder Cimicomorpha (Hemiptera, Heteroptera): a review. ZooKeys 154, 31–70. doi: 10.3897/zookeys.154.1953
Kuznetsova, V. G., Grozeva, S. M., Sewlal, N., and Nokkala, S. (2007). Cytogenetic Characterization of the Trinidad Endemic, Arachnocoris trinitatus Bergroth: the First Data for the Tribe Arachnocorini (Heteroptera: Cimicomorpha: Nabidae). Folia Biol. 55, 17–26. doi: 10.3409/173491607780006344
Lam, K. G., Brick, K., Cheng, G., Pratto, F., and Camerini-Otero, R. D. (2019). Cell-type-specific genomics reveals histone modification dynamics in mammalian meiosis. Nat. Commun. 10:3821. doi: 10.1038/s41467-019-11820-7
Lermontova, I., Koroleva, O., Rutten, T., Fuchs, J., Schubert, V., Moraes, I., et al. (2011). Knockdown of CENH3 in Arabidopsis reduces mitotic divisions and causes sterility by disturbed meiotic chromosome segregation. Plant J. 68, 40–50. doi: 10.1111/j.1365-313X.2011.04664.x
Manna, G. K. (1984). “Chromosomes in evolution in Heteroptera,” in Chromosomes in Evolution of Eukaryotic Groups, eds A. K. Sharma and A. Sharma (Boca Raton, FL: CRC Press), 189–225.
Melters, D. P., Paliulis, L. V., Korf, I. F., and Chan, S. W. L. (2012). Holocentric chromosomes: convergent evolution, meiotic adaptations, and genomic analysis. Chromosome Res. 20, 579–593. doi: 10.1007/s10577-012-9292-1
Mola, L. M., and Papeschi, A. G. (2006). Holokinetic chromosomes at a glance. J. Basic Appl. Genet. 17, 17–33.
Monen, J., Maddox, P. S., Hyndman, F., Oegema, K., and Desai, A. (2005). Differential role of CENP-A in the segregation of holocentric C. elegans chromosomes during meiosis and mitosis. Nat. Cell Biol. 7, 1248–1255. doi: 10.1038/ncb1331
Montgomery, T. H. (1901). A study of the chromosomes of the germ cells of Metazoa. T. Am. Philos. Soc. 20, 154–236. doi: 10.2307/1005428
Montgomery, T. H. (1906). Chromosomes in the spermatogenesis of the Hemiptera-Heteroptera. T. Am. Philos. Soc. 21, 97–173.
Motzko, D., and Ruthmann, A. (1984). Spindle membranes in mitosis and meiosis of the heteropteran insect Dysdercus intermedius. A study of the interrelationship of spindle architecture and the kinetic organization of chromosomes. Eur. J. Cell Biol. 33, 205–216.
Nokkala, S. (1986). The mechanisms behind the regular segregation of the m-chromosomes in Coreus marginatus L. (Coreidae, Hemiptera). Hereditas 105, 73–85. doi: 10.1111/j.1601-5223.1986.tb00645.x
Nokkala, S., Kuznetsova, V., Maryanska-Nadachowska, A., and Nokkala, C. (2006). Holocentric chromosomes in meiosis. II. The modes of orientation and segregation of a trivalent. Chromosome Res. 14, 559–565. doi: 10.1007/s10577-006-1053-6
Nokkala, S., Kuznetsova, V. G., Maryanska-Nadachowska, A., and Nokkala, C. (2004). Holocentric chromosomes in meiosis. I. Restriction of the number of chiasmata in bivalents. Chromosome Res. 12, 733–739. doi: 10.1023/B:CHRO.0000045797.74375.70
Nokkala, S., and Nokkala, C. (1986). Achiasmatic male meiosis of collochore type in the heteropteran family Miridae. Hereditas 105, 193–197. doi: 10.1111/j.1601-5223.1986.tb00661.x
Panchenko, T., and Black, B. E. (2009). “The epigenetic basis for centromere identity,” in Centromere, Progress in Molecular and Subcellular Biology, Vol. 48, ed. D. Ugarkovic (Berlin: Springer), 1–32. doi: 10.1007/978-3-642-00182-6_1
Panzera, F., Pérez, R., Panzera, Y., Álvarez, F., Scvortzoff, E., and Salvatella, R. (1995). Karyotype evolution in holocentric chromosomes of three related species of triatomines (Hemiptera-Reduviidae). Chromosome Res. 3, 143–150. doi: 10.1007/BF00710707
Panzera, F., Pérez, R., Panzera, Y., Ferrandis, I., Ferreiro, M. J., and Calleros, L. (2010). Cytogenetics and genome evolution in the subfamily Triatominae (Hemiptera, Reduviidae). Cytogenet. Genome Res. 128, 77–87. doi: 10.1159/000298824
Panzera, F., Scvortzoff, E., Pérez, R., Panzera, Y., Hornos, S., Cestau, R., et al. (1998). “Cytogenetics of triatomines,” in Atlas of Chagas’ Disease Vectors in the American, eds R. Carcavallo, I. Galindez Girón, J. Jurberg, and H. Lent (Río de Janeiro: Fiocruz), 621–664.
Papeschi, A. G. (1988). C-banding and DNA content in three species of Belostoma (Heteroptera) with large differences in chromosome size and number. Genetica 76, 43–51. doi: 10.1007/bf00126009
Papeschi, A. G. (1991). DNA content and heterochromatin variation in species of Belostoma (Heteroptera). Hereditas 115, 109–114. doi: 10.1111/j.1601-5223.1991.tb03543.x
Papeschi, A. G. (1992). Estudios Citogenéticos y Evolutivos en Heteroptera. Doctoral thesis. Buenos Aires: Universidad de Buenos Aires.
Papeschi, A. G. (1994). Chromosome rearrangements in Belostoma plebejum (Stål) (Belostomatidae, Heteroptera). Caryologia 47, 223–230.
Papeschi, A. G. (1995). Correspondence between C-banding and Ag-NOR in the sex chromosomes of Belostoma oxyurum (Belostomatidae, Heteroptera). Cytologia 60, 291–295.
Papeschi, A. G. (1996). Sex chromosome polymorphism in species of Belostoma (Belostomatidae, Heteroptera). Hereditas 124, 269–274.
Papeschi, A. G., and Bidau, C. J. (1985). Chromosome complement and male meiosis in four species of Belostoma Latreille (Heteroptera-Belostomatidae). Braz. J. Genet. 8, 249–261.
Papeschi, A. G., and Bressa, M. J. (2006). Evolutionary cytogenetics in Heteroptera. J. Biol. Res. 5, 3–21. doi: 10.1186/jbiol30
Papeschi, A. G., and Mola, L. M. (1990). Meiotic studies in Acanonicus hahni (Stål) (Coreidae, Heteroptera) I. Behaviour of univalents in desynaptic individuals. Genetica 80, 31–38.
Papeschi, A. G., Mola, L. M., Bressa, M. J., Greizerstein, E. J., Lia, V., and Poggio, L. (2003). Behaviour of ring bivalents in holokinetic systems: alternative sites of spindle attachment in Pachylis argentinus and Nezara viridula (Heteroptera). Chromosome Res. 11, 725–733. doi: 10.1023/b:chro.0000005740.56221.03
Parshad, R. (1957). Cytological studies in Heteroptera. II. Chromosome complement and meiosis in the males of three pyrrhocorid bugs. Cytologia 22, 127–135.
Pérez, R., Calleros, L., Rose, V., Lorca, M., and Panzera, F. (2004). Cytogenetic studies on Mepraia gajardoi (Heteroptera: Reduviidae). Chromosome behaviour in a spontaneous translocation mutant. Eur. J. Entomol. 101, 211–218.
Pérez, R., Panzera, F., Page, J., Suja, J. A., and Rufas, J. S. (1997). Meiotic behaviour of holocentric chromosomes: orientation and segregation of autosomes in Triatoma infestans (Heteroptera). Chromosome Res. 5, 47–56. doi: 10.1023/A:1018493419208
Pérez, R., Rufas, J. S., Suja, J. A., Page, J., and Panzera, F. (2000). Meiosis in holocentric chromosomes: orientation and segregation of an autosome and sex chromosomes in Triatoma infestans (Heteroptera). Chromosome Res. 8, 17–25. doi: 10.1023/A:1009266801160
Peters, A. H., O’Carroll, D., Scherthan, H., Mechtler, K., Sauer, S., Schöfer, C., et al. (2001). Loss of the Suv39h histone methyltransferases impairs mammalian heterochromatin and genome stability. Cell 107, 323–337. doi: 10.1016/s0092-8674(01)00542-6
Pigozzi, M. I., and Solari, A. J. (2003). Differential immunolocalization of a putative Rec8p in meiotic autosomes and sex chromosomes of triatomine bugs. Chromosoma 112, 38–47. doi: 10.1007/s00412-003-0240-x
Poggio, M. G., Bressa, M. J., and Papeschi, A. G. (2007). Karyotype evolution in Reduviidae (Insecta: Heteroptera) with special reference to Stenopodainae and Harpactorinae. Comp. Cytogen. 1, 159–168. doi: 10.3897/CompCytogen.v8i4.8430
Poggio, M. G., Bressa, M. J., and Papeschi, A. G. (2011). Male meiosis, heterochromatin characterization and chromosomal location of rDNA in Microtomus lunifer (Berg, 1900) (Hemiptera: Reduviidae: Hammacerinae). Comp. Cytogen. 5, 1–22. doi: 10.3897/compcytogen.v5i1.1143
Poggio, M. G., Di Iorio, O., Turienzo, P., Papeschi, A. G., and Bressa, M. J. (2014). Heterochromatin characterization and ribosomal gene location in two monotypic genera of bloodsucker bugs (Cimicidae, Heteroptera) with holokinetic chromosomes and achiasmatic male meiosis. Bull. Entomol. Res. 104, 788–793. doi: 10.1017/S0007485314000650
Powers, N. R., Parvanov, E. D., Baker, C. L., Walker, M., Petkov, P. M., and Paigen, K. (2016). The meiotic recombination activator PRDM9 trimethylates both H3K36 and H3K4 at recombination hotspots in vivo. PLoS Genet. 12:e1006146. doi: 10.1371/journal.pgen.1006146
Prosée, R. F., Wenda, J. M., and Steiner, F. A. (2020). Adaptations for centromere function in meiosis. Essays Biochem. 64, 193–203. doi: 10.1042/EBC20190076
Rao, S. R. V. (1955). Chromosomes of Oncopeltus nigriceps (Dall) (Lygaeidae: Heteroptera). J. Zool. Soc. India 7, 104–106.
Rebagliati, P. (2009). Citogenética Básica, Molecular y Evolutiva en Pentatómidos de Interés Agrícola en la Argentina (Pentatomidae, Heteroptera). Doctoral thesis. Buenos Aires: Universidad de Buenos Aires.
Rebagliati, P., Papeschi, A. G., and Mola, L. M. (2003). Meiosis and fluorescent banding in Edessa meditabunda and E. rufomarginata (Heteroptera: Pentatomidae: Edessinae). Eur. J. Entomol. 100, 11–18.
Rebagliati, P. J., Mola, L. M., Papeschi, A. G., and Grazia, J. (2005). Cytogenetic studies in Pentatomidae (Heteroptera): A review. J. Zool. Syst. Evol. Res. 43, 199–213. doi: 10.1111/j.1439-0469.2005.00312.x
Royo, H., Polikiewicz, G., Mahadevaiah, S. K., Prosser, H., Mitchell, M., Bradley, A., et al. (2010). Evidence that meiotic sex chromosome inactivation is essential for male fertility. Curr. Biol. 20, 2117–2123. doi: 10.1016/j.cub.2010.11.010
Sáez, F. A. (1960). El empleo de la hematoxilina acética o propiónica para el estudio de los cromosomas con la técnica de aplastamiento. Comunicaciones de la Sociedad de Biología de Montevideo Mimeographed 1.
Sciurano, R. B., and Solari, A. J. (2014). Ultrastructural and immunofluorescent methods for the study of the XY body as a biomarker. Method. Mol. Biol. 1094, 137–149. doi: 10.1007/978-1-62703-706-8_11
Shiu, P. K., Raju, N. B., Zickler, D., and Metzenberg, R. L. (2001). Meiotic silencing by unpaired DNA. Cell 107, 905–916. doi: 10.1016/s0092-8674(01)00609-2
Solari, A. J. (1974). The behaviour of the xy pair in mammals. Int. Rev. Cell Mol. Biol. 38, 273–317. doi: 10.1016/s0074-7696(08)60928-6
Souza, H. V., Bicudo, H. E., and Itoyama, M. M. (2007). Study of chromosomal and nucleolar aspects in testes of Nysius californicus (Heteroptera: Lygaeidae). Genet. Mol. Res. 6, 33–40.
Souza, H. V. D., Castanhole, M. M. U., Gomes, M. O., Murakami, A. S., Souza Firmino, T. S. D., Saran, P. S., et al. (2014). Meiotic behaviour of 18 species from eight families of terrestrial Heteroptera. J. Insect Sci. 14:149. doi: 10.1093/jisesa/ieu011
Souza-Firmino, T. S., Alevi, K. C. C., and Itoyama, M. M. (2020). Chromosomal divergence and evolutionary inferences in Pentatomomorpha infraorder (Hemiptera, Heteroptera) based on the chromosomal location of ribosomal genes. PLoS One 15:e0228631. doi: 10.1371/journal.pone.0228631
Stack, S. M. (1984). Heterochromatin, the synaptonemal complex and crossing over. J. Cell Sci. 71, 159–176. doi: 10.1242/jcs.71.1.159
Suja, J. A., del Cerro, A. L., Page, J., Rufas, J. S., and Santos, J. L. (2000). Meiotic sister chromatid cohesion in holocentric sex chromosomes of three heteropteran species is maintained in absence of axial elements. Chromosoma 109, 35–43. doi: 10.1007/s004120050410
Tessarz, P., and Kouzarides, T. (2014). Histone core modifications regulating nucleosome structure and dynamics. Nat. Rev. Mol. Cell Biol. 15, 703–708. doi: 10.1038/nrm3890
Thomas, D. B. J. (1987). Chromosome evolution in the Heteroptera (Hemiptera): agmatoploidy versus aneuploidy. Ann. Entomol. Soc. Am. 80, 720–730.
Toscani, M. A., Papeschi, A. G., and Pigozzi, M. I. (2011). La formación de ejes meióticos y su relación con regiones heterocromáticas en los heterópteros (Hemiptera, Insecta) (in Spanish). J. Basic Appl. Genet. 21, 152.
Toscani, M. A., Pigozzi, M. I., Bressa, M. J., and Papeschi, A. G. (2008). Synapsis with and without recombination in the male meiosis of the leaf-footed bug Holhymenia rubiginosa (Coreidae, Heteroptera). Genetica 132, 173–178. doi: 10.1007/s10709-007-9159-0
Traut, W. (1976). Pachytene mapping in the female silkworm Bombyx mori L. (Lepidoptera). Chromosoma 58, 275–284. doi: 10.1007/BF00292094
Turner, J. M., Aprelikova, O., Xu, X., Wang, R., Kim, S., Chandramouli, G. V. R., et al. (2004). BRCA1, histone H2AX phosphorylation, and male meiotic sex chromosome inactivation. Curr. Biol. 14, 2135–2142. doi: 10.1016/j.cub.2004.11.032
Turner, J. M., Mahadevaiah, S. K., Fernandez-Capetillo, O., Nussenzweig, A., Xu, X., Deng, C. X., et al. (2005). Silencing of unsynapsed meiotic chromosomes in the mouse. Nat. Genet. 37, 41–47.
Ueshima, N. (1979). “Hemiptera II: Heteroptera,” in Animal Cytogenetics. 3. Insecta 6, ed. B. John (Stuttgart: Gebrüder Bornträger), 1–117. doi: 10.1007/978-94-007-0705-4_1
Ueshima, N., and Ashlock, P. D. (1980). Cytotaxonomy of the Lygaeidae (Heteroptera). Univ. Kansas Sci. Bull. 51, 717–801.
Ushijima, Y., Inoue, Y. H., Konishi, T., Kitazawa, D., Yoshida, H., Shimaji, K., et al. (2012). Roles of histone H3K9 methyltransferases during Drosophila spermatogenesis. Chromosome Res. 20, 319–331. doi: 10.1007/s10577-012-9276-1
Vakoc, C. R., Mandat, S. A., Olenchock, B. A., and Blobel, G. A. (2005). Histone H3 Lysine 9 methylation and HP1γ are associated with transcription elongation through mammalian chromatin. Mol. Cell 19, 381–391. doi: 10.1038/nrm1761
Viera, A., Page, J., and Rufas, J. S. (2009a). Inverted meiosis: the true bugs as a model to study. Genome Dyn. 5, 137–156. doi: 10.1159/000166639
Viera, A., Santos, J. L., Parra, M. T., Calvente, A., Gómez, R., de la Fuente, R., et al. (2009b). Cohesin axis maturation and presence of RAD51 during first meiotic prophase in a true bug. Chromosoma 118, 575–589. doi: 10.1007/s00412-009-0218-4
Viera, A., Parra, M. T., Rufas, J. S., and Page, J. (2016). Transcription reactivation during the first meiotic prophase in bugs is not dependent on synapsis. Chromosoma 126, 179–194. doi: 10.1007/s00412-016-0577-6
Wang, L., Xu, Z., Khawar, M. B., Liu, C., and Li, W. (2017). The histone codes for meiosis. Reproduction 154, R65–R79. doi: 10.1530/rep-17-0153
Weiler, K. S., and Wakimoto, B. T. (1995). Heterochromatin and gene expression in Drosophila. Annu. Rev. Genet. 29, 577–605. doi: 10.1146/annurev.ge.29.120195.003045
Wilson, E. B. (1905). Studies on chromosomes. I. The behaviour of the idiochromosomes in Hemiptera. J. Exp. Zool. 2, 371–405.
Keywords: histone modifications, meiosis, holokinetic chromosomes, sex chromosomes, autosomes, m chromosomes, Heteroptera
Citation: Toscani MA, Pigozzi MI, Papeschi AG and Bressa MJ (2022) Histone H3 Methylation and Autosomal vs. Sex Chromosome Segregation During Male Meiosis in Heteroptera. Front. Ecol. Evol. 10:836786. doi: 10.3389/fevo.2022.836786
Received: 15 December 2021; Accepted: 11 February 2022;
Published: 07 March 2022.
Edited by:
Valentina G. Kuznetsova, Zoological Institute of Russian Academy of Science, RussiaReviewed by:
Snejana Grozeva, Bulgarian Academy of Sciences (BAS), BulgariaFrancisco Panzera, Universidad de la República, Uruguay
David Sadílek, General University Hospital in Prague, Czechia
Copyright © 2022 Toscani, Pigozzi, Papeschi and Bressa. This is an open-access article distributed under the terms of the Creative Commons Attribution License (CC BY). The use, distribution or reproduction in other forums is permitted, provided the original author(s) and the copyright owner(s) are credited and that the original publication in this journal is cited, in accordance with accepted academic practice. No use, distribution or reproduction is permitted which does not comply with these terms.
*Correspondence: María José Bressa, mjbressa@ege.fcen.uba.ar
†Deceased