- 1Department of Integrative Biology, University of South Florida, St. Petersburg, FL, United States
- 2School of Marketing and Innovation, Muma College of Business, University of South Florida, St. Petersburg, FL, United States
Introduction: Along the coasts of Florida, United States, the nesting season of the loggerhead sea turtle, Caretta caretta, overlaps with the hurricane season. Nesting loggerhead females do not extend parental protection beyond depositing eggs in sandy, excavated nests in locations that provide a viable range of temperatures, moisture, and respiratory gas exchange. Thereafter, a female’s clutches are subjected to the uncertainties of desiccation, predation, flooding, or beach erosion.
Methods: Here, we used data from a 1996-2004 study of 94 tagged loggerhead females nesting on a small barrier island off the Gulf Coast of south Florida, United States. We tested the hypothesis that the distribution of nest sites by loggerhead females was a randomized response to unpredictable hatching failure.
Results: We show that nest site choice accounted for 19.2% of variation in hatching success whereas breeding year and breeding month accounted for the remaining 81.8% of variation in hatching success. We show that the emergence site along the beach-length axis, nest site choice along the beach-width axis, and distances between nest locations did not fit a uniform-random distribution or a normal distribution. Instead, we show that loggerhead females employed a “Goldilocks” distribution in which nest sites were “neither too clustered nor too dispersed.” Moreover, loggerhead females selected nest sites with limited overlap with nest sites from previous breeding seasons.
Discussion: We propose that nest site choice by this population of loggerhead females constitutes a significant maternal risk-management adaptation that deserves thoughtful consideration as we continue to assess the impacts of climate change on the future of loggerhead sea turtles.
Introduction
Mated females of the loggerhead sea turtle, Caretta caretta, engage in transoceanic migrations every 2–3 years to oviposit egg clutches on their natal beaches (Whitmore and Dutton, 1985; Mortimer, 1990; Hays et al., 1995; Miller et al., 2003; Pfaller et al., 2009; Tucker, 2010). Because loggerhead females return to land to oviposit their eggs, the survival of hatchlings based on nest site choice has become a primary focus of research exploring the impact of climate change on sea turtles (Esteban et al., 2017).
All populations of loggerhead sea turtles share a core set of nesting behaviors. After nesting females emerge from the surf, they ascend the beach in a straight-line perpendicular to the water line and toward the dune line, also called the vegetative line (Wood and Bjorndal, 2000). However, the details of nest site choice vary depending on the ecological characteristics of a beach. On the beaches of Sanibel and Captiva, Keewaydin Island, and the 10,000 Islands of southwest Florida, loggerhead females spread nests along the beach in close proximity to the vegetative line (Hays et al., 1995; Garmestani et al., 2000; Cassill, 2021). On the beaches of Arembepe Beach in the State of Bahia, Northeastern Brazil, and the Yakushima Island, Japan, loggerhead females nested on wider beaches with less slope, preferentially nesting on open sand, equidistance between the high water line and the vegetative line (Serafini et al., 2009; Hatase and Omuta, 2018). On the sandy beaches of Zakynthos Island, Greece, beach width, emergence site, organic content, sand texture, and clay layers were important factors affecting nest site selection by loggerhead females and hatching success after clutches are abandoned (Mazaris et al., 2006).
The spring-to-fall nesting and hatching season of the loggerhead sea turtle, Caretta caretta, overlaps with the spring-to-fall monsoon and severe storm seasons (Ross, 2005). Torrential thunderstorms and tidal surges suffocate eggs in flooded sediment (Kraemer and Bell, 1980). During hurricanes or cyclones, extreme tides, and currents erode beaches, washing clutches out to sea (van Houtan and Bass, 2007). Severe storms also expose clutches to desiccation and predators (Matsuzawa et al., 2002; Stewart and Wyneken, 2004). Nests located too close to the high water line are at risk of being flooded or washed out by storms (Papi et al., 1997; Ross, 2005; van Houtan and Bass, 2007; Ackerman, 2017; Cassill, 2021). Nests located too far from the high water line are at risk of predation or desiccation.
After a female leaves an egg clutch behind, offspring survival is a game-of-chance. To increase the probability of meeting replacement fitness, the survival of two sexually mature offspring to replace the female and her mate (Cassill, 2019), loggerhead females employ a game-of-numbers. Over her lifetime, each female will produce 4,000–5,000 eggs, divided among 40 clutches with each containing an average of 105 eggs. Clutches are spread over 10–12 breeding seasons (Cassill, 2021). Ambient temperature, moisture, and respiration gas concentrations within a subterranean nest have profound effects on the survival of hatchlings (Mortimer, 1990). Because loggerhead females do not extend parental care beyond burying eggs in excavated sand pits, clutches deposited close to the sea have a greater likelihood of hatching failure by flooding and erosion, whereas clutches deposited farther inland have a greater likelihood of desiccation, hatchling disorientation, and predation (Wood and Bjorndal, 2000; Hughes and Brooks, 2006). Breeding loggerhead females must locate nests at sites that are suitable for incubating eggs and for mitigating the loss of eggs to unpredictable storms and predators.
Current consensus is that the site of emergence on the beach fits a random distribution (Hays et al., 1995), suggesting that females select a “good” beach in which any nest site will produce a successful hatch (Wood and Bjorndal, 2000). Others suggest that patterns of nest site selection are nonrandom (Miller et al., 2003), suggesting that females select a “good” site on an ecologically diverse beach to ensure hatching success (Wood and Bjorndal, 2000).
Here, we compared the distribution of nest sites by loggerhead females along the beach-length and beach-width axes to normal and uniform-random distributions. If nest sites fit a normal distribution, then loggerhead females have a fine-tuned preference for selecting “good” areas on a beach for egg deposition. If nest sites fit a uniform random distribution, then loggerhead females have a broadly tuned ability to select a “good” beach with suitable sites anywhere on the beach. We tested three null hypotheses. (1) The emergence site of females along the beach-length axis is random. (2) The nest site along the beach-width axis is random. (3) The distances between and across nests within and over multiple breeding seasons are random.
In the following sections, we present our findings on the impact of breeding year, breeding month, and nest site choice on hatching success. We then present the pattern of nest-site choice by loggerhead females at population and individual levels. Lastly, we frame our findings as risk-management adaptations in light of the spatiotemporal unpredictability of floods, storms, desiccation, and predation.
Materials and methods
Data collection
Keewaydin Island is a primary barrier island located off the coast of Naples, in Collier County, Florida, United States (Figure 1). Keewaydin Island is managed by staff from the State of Florida’s Coastal Office, in cooperation with staff from NOAA, and the Rookery Bay National Estuarine Research Reserve. During the annual loggerhead sea turtle nesting season, staff and volunteers from The Conservancy of Southwest Florida Keewaydin Island monitor nesting females nightly. Staff and volunteers patrol the island nightly from 21:00 to 5:00 to record or tag new females and record information on the location and hatching success of their nest sites. Data included the female’s ID number, the location of nest sites along the beach-length axis from a permanent marker and along the beach-width axis from the high water line and relative to the vegetative line. The width of barrier-island beaches vary considerably along the beach length and are subject to dramatic change each year, depending on the frequency, intensity, and duration of storms and currents. Our analyses reflected nest site choice by loggerhead females against local conditions such as the high water line and the vegetative line, that were independent of past historical changes in the beach morphology. Each night, staff recorded evidence for predation, flooding, and partial or total washout. After the emergence of hatchlings, staff recorded the number of eggshells, the number of failed eggs, and the number of remaining hatchlings in the nest. Staff carried viable hatchlings that had not yet crawled out of the nest to the Gulf water. Conservancy staff provided data on 112 loggerhead females and their clutches nesting on Keewaydin Island in the southern Gulf coast of Florida from 1988 to 2004. From this information, we analyzed data on 389 nests produced by 94 females from 1996 through 2004. For a detailed analysis of individuals, we also selected six nesting females that produced 81 clutches over multiple breeding seasons.
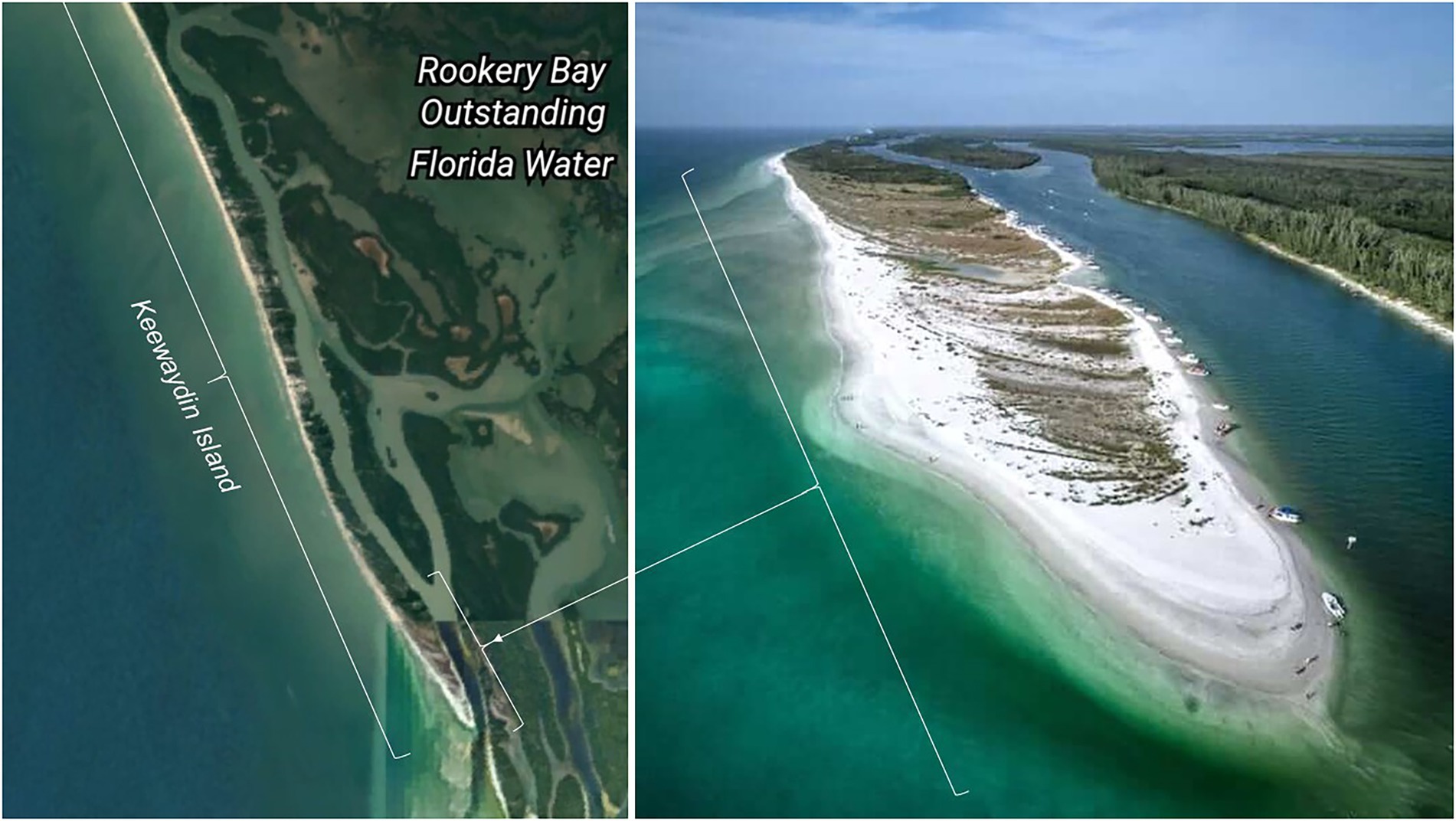
Figure 1. Keewaydin Island Preserve, Collier County, Florida, United States. Photographs are copyright free under the website https://linkrelated.com/terms-of-use/. This website is an integral part of Florida Media’s digital internet media assets and solutions.
Simulation
The simulation was undertaken using the following software: RStudio 2021.09.2 + 382 “Ghost Orchid” for Windows with “R-4.1.2 for Windows” using the function runif() from the package “stats” version 4.1.2 for uniform-random number generation. We produced a uniform-random distribution for a total of 400 nests by 10 simulated females. We compared the simulated distribution of nest sites to nests by loggerhead females. Variables were: percent hatching success per nest; emergence of females along the beach-length axis (11,000 m); nest site along the beach-width axis (40 m); distance between nearest-neighbor nest within a breeding season per female; longest distance across nests within a breeding season per female; and longest distance across all nests over multiple breeding seasons per female.
Analyses
To compare distances between and among nests within and over multiple breeding seasons per loggerhead female, we used the Shapiro–Wilk test to check for normality. Because our data did not meet the assumptions for normality and homogeneity, we used the nonparametric Kruskal-Wallis test and the Wilcoxon Each Pair. To compare nest site distance by loggerhead females versus simulated nest site distances, we used a nonparametric one-way test, Chi square approximation. We used a mixed model to analyze hatching success per nest (percent of hatched eggs) by five independent variables: breeding year, breeding month, nest site distance from the vegetative line, nest site along the beach-width axis from the high water line to the vegetative line, and emergence sites along the beach-length axis. Data analyses and graphics were generated using JMP Pro Statistical Software (16.1) and PowerPoint. Data from this study are available in Excel format upon request.
Results
During the 1996–2004 period of this study, Hurricane George struck in September 1998; Irene struck in October 1999; and Gabrielle struck in September 2001. Hurricanes Charley, Frances, Ivan, and Jeanne struck in August and September 2004. During this period, mean hatching success per nest for loggerhead females was 65.3% (SE = 1.9; n = 389). Uniform-random hatching success for simulated females as 50.4%. Did nest-site choice by loggerhead females mitigate hatching failure? By modeling breeding year and breeding month as “confounding variables,” we show that the closeness of nest sites to the vegetative line and the female’s emergence sites along the beach-length axis were significant predictors of hatching success per nest, but not beach width (Figure 2; Mixed Model: R2 = 0.44; p < 0.0001). Breeding year accounted for 71.4% of explained variation in hatching success; breeding month accounted for 10.4%; nest distance from the vegetative line accounted for 9.7%; and distance along the beach-length axis accounted for 9.1%. Beach width accounted for 0.4% of explained variation.
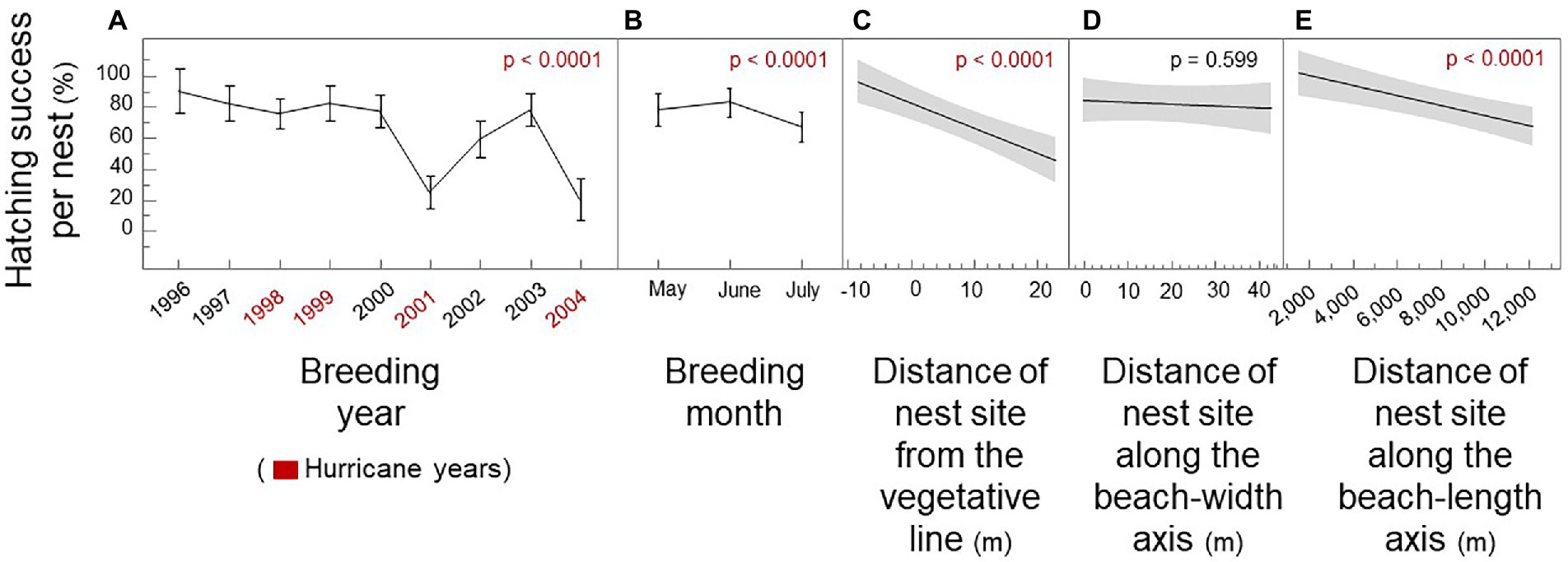
Figure 2. Hatching success per nest by spatiotemporal nest site choice of loggerhead females. Breeding year, breeding month, nest sites, and emergence sites. (A) Hatching success by breeding year (B) Hatching success by breeding month, independent of year. (C) Hatching success by the distance of the nest site from the line of vegetation. (D) Hatching success by the distance of the nest site along the beach-width axis starting from the high water line. (E) Hatching success by the distance of the nest site along the beach-length axis from a permanent marker. Hurricane years are shown as blue; mean shows as breaks in the line; SE shows as whiskers; regression line with 95% confidence intervals are shown in gray.
We graphically illustrate how the impact of nest site choice by breeding loggerhead females on hatching success per nest differed depending on the breeding year (Figure 3). In 1997, the impact of nest site choice on hatching success was insignificant. Hatching success was high for most nests. In 1998, emergence sites by loggerhead females at the far end of the island improved hatching success. In 2001, nest site choices closer to the vegetative line and further from the high water line improved hatching success. In 2002, nest site choices closer to the vegetative line, closer to the high water line, and furthest from the end of the island improved hatching success (see Appendix A for interaction effects).
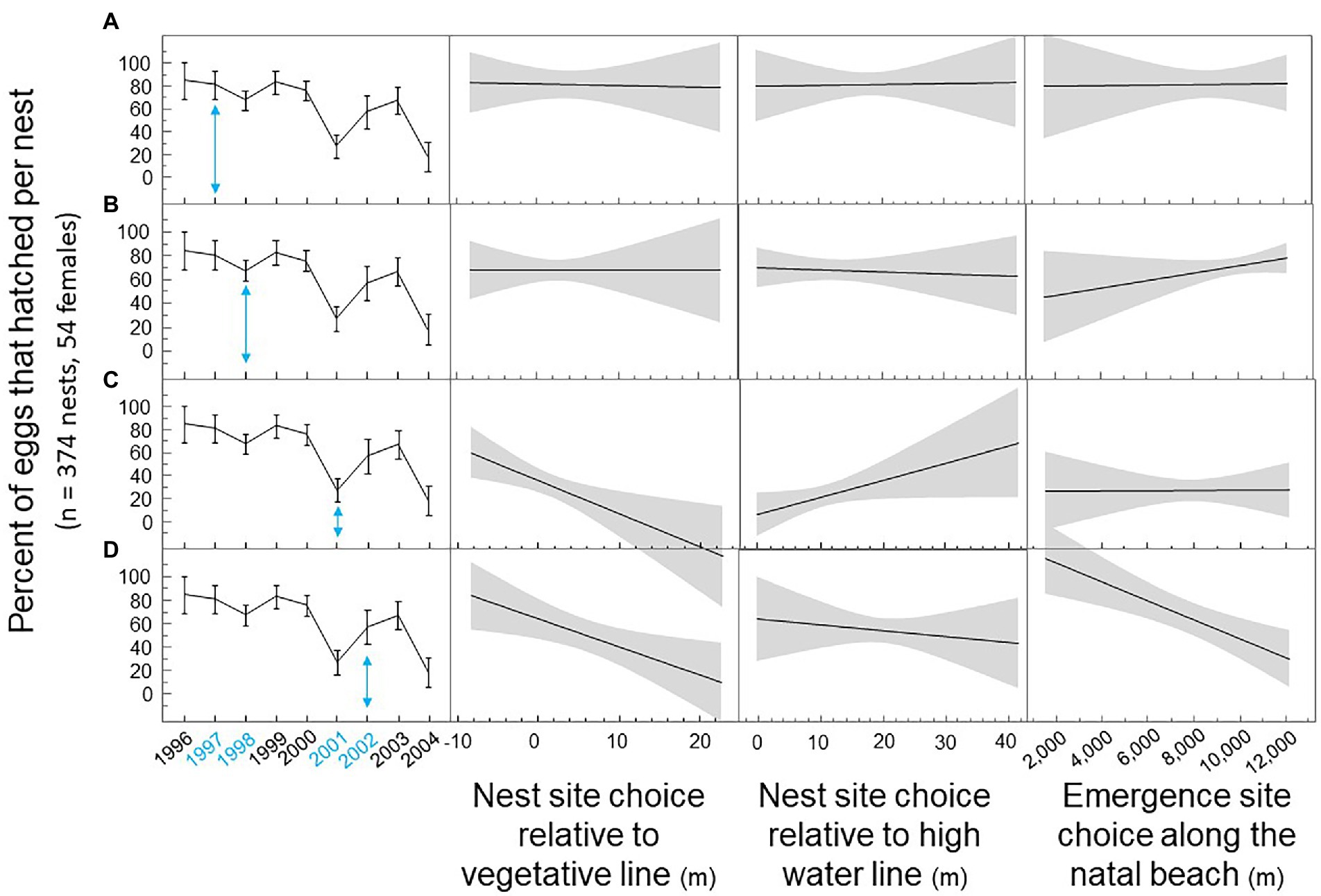
Figure 3. Nest site choice on Keewaydin Island by loggerhead females during multiple breeding seasons from 1996 through 2004. (A) Hatching success by nest site choice in 1997. (B) Hatching success by nest site choice in 1998. (C) Hatching success by nest site choice in 2001. (D) Hatching success by nest site choice in 2002.
Do loggerhead females randomize nest site selection to offset hatching failure by unpredictable storms and predators? To address this question, we plotted the distribution of 389 nest sites by a population of 94 nesting loggerhead females on the beach of Keewaydin Island from 1996 through 2004 (Figures 4A,B). We also plotted the uniform-random distribution of 400 nests divided among simulated females (Figure 4C). The vast majority of nests (94%) were located between 6,000 and 12,000 m along the 13,000 m beach-length axis. Beach width was a significant predictor of nest site choice for loggerhead females, but not for simulated females (Figures 4D–F; Regression: R2vegetative line = 0.16; p < 0.0001; R2high water line = 0.62; p < 0.0001; R2uniform-random simulation = 0.0004; p = 0.712). The majority (69%) of nest sites were located on narrow beach areas (<20 m) such that clutches were closer to both the vegetative line and the high water line that nests on wider beach areas (Figures 4A,B).
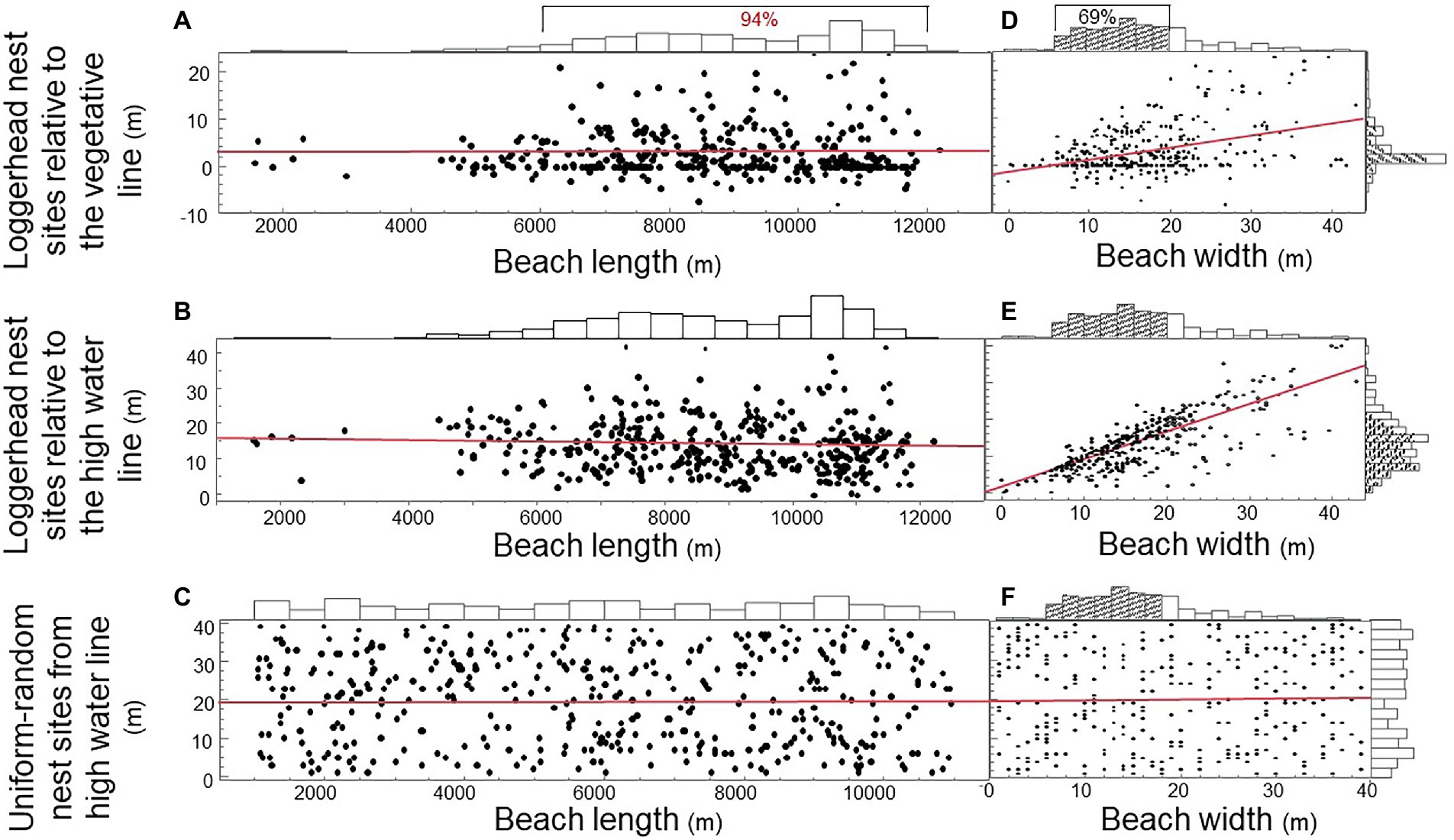
Figure 4. Distributions and predictors of loggerhead sea turtle nest sites on Keewaydin Island beach, Naples Florida, United States. (A) Emergence sites of loggerhead females along the beach-length axis by the distance of nest sites from the vegetative line. (B) Emergence sites along the beach-length axis by the distance of nest sites from the high water line. (C) Uniform-random emergence sites along the beach-length axis and nest site locations along the beach-width axis. (D) Nest site location by loggerhead females along the beach-width axis by the distance of nest sites from the vegetative line. (E) Nest sites along the beach-width axis by the distance of nest sites from the high water line. (F) Uniform-random nest sites along the beach-length axis by the beach-width axis.
We show that nest site choice for loggerhead females relative to the vegetative line fits a leptokurtic distribution with double-exponential skew. Hence, nest site choice relative to the vegetative line differed significantly from a normal distribution and from a uniform random distribution (Figure 5A; Shapiro–Wilk: Wnormal = 0.84; p < 0.0001; Wuniform-random = 0.94; p < 0.0001; n = 100; one-way Chi Square Approximation: χ2 = 119.75; DF = 1; p < 0.0001). Nest site choice for loggerhead females relative to the high water line fits a platykurtic distribution with moderate skew. Thus, nest site choice relative to the high water line differed significantly from a normal distribution and from a uniform-random distance (Figure 5B; Shapiro–Wilk: Wnormal = 0.95; p = 0.010; n = 71; Wuniform-random = 0.94; p < 0.0001; and one-way Chi Square Approximation: χ2 = 20.31; DF = 1; p < 0.0001). The emergence sites by loggerhead females along the beach-length axis fit a platykurtic distribution with moderate skew. Thus, emergence sites along the beach-length axis differed significantly from a normal distribution and from a uniform-random distribution (Figure 5C; Shapiro–Wilk: Wnormal = 0.97; p = 0.016; n = 389; Wuniform-random = 0.95; p < 0.0001; and one-way Chi Square Approximation: χ2 = 63.98; DF = 1; p < 0.0001).
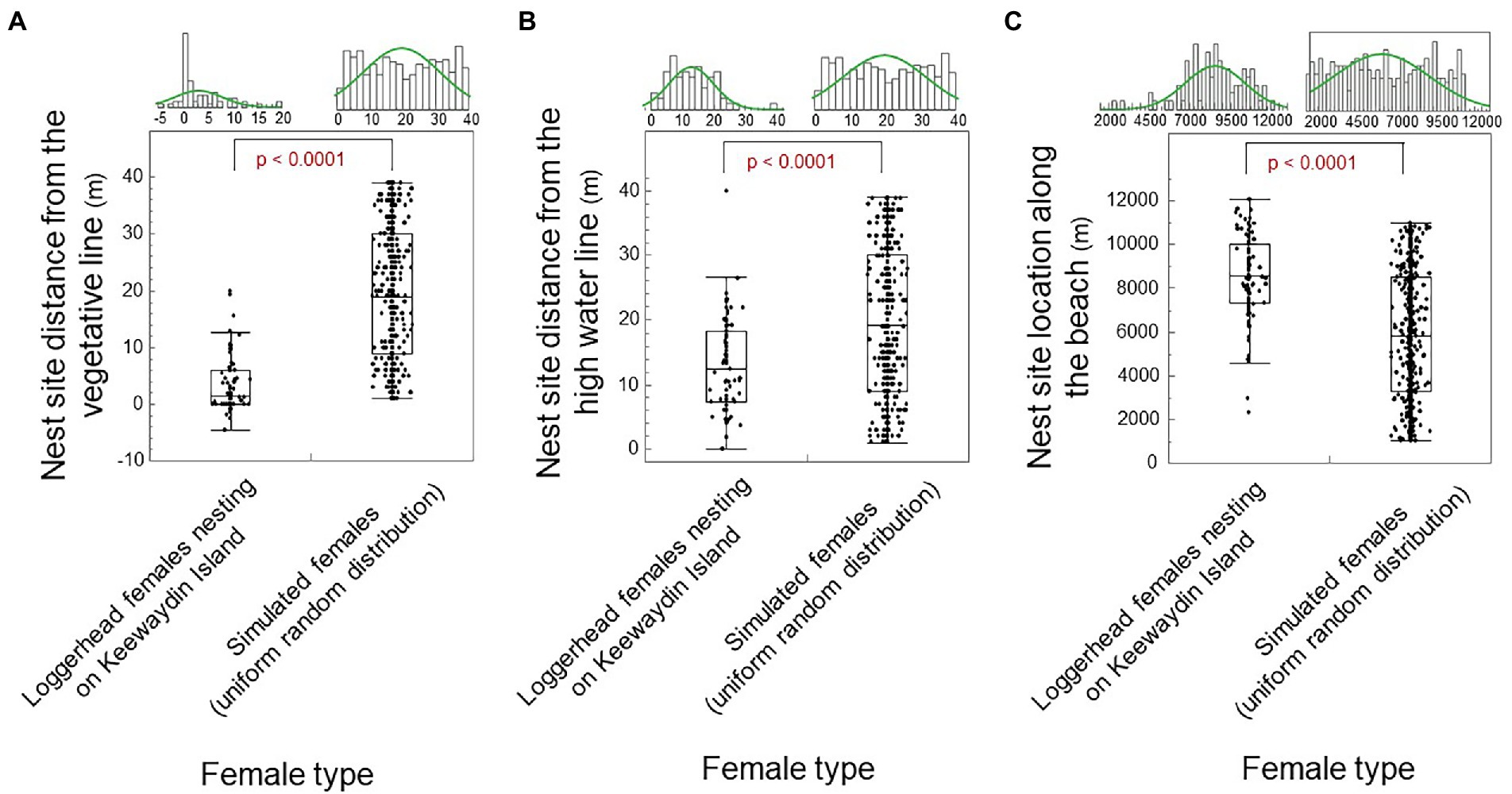
Figure 5. Nest site choice by female type (loggerhead females versus simulated females). (A) Nest site distance from the vegetative line by female type. (B) Nest site distance from the high water line by female type. (C) Nest site distance along the beach-length axis by female type. The median shows as a line in the box. The box ends are the 25 and 75th quantiles. Whiskers enclose all data except outliers. Significant differences show as red.
The distance among nest sites along the beach-length axis differed significantly within and across breeding seasons for loggerhead females (Figure 6A; Wilcoxon Kruskal-Wallas: χ2 = 25.12; DF = 2, p < 0.0001). Relative to a uniform random distribution of nest sites by simulated females, the distance between neighboring nest sites within a breeding season per loggerhead female was significantly smaller (Figure 6B; one-way test, Chi Square Approximation: χ2 = 46.53; DF = 1, p < 0.0001; median: 989 vs. 3,069 m). The longest distance among nests within a breeding season for loggerhead females was significantly smaller than the longest distance for simulated females (Figure 6C: χ2 = 13.13; DF = 1, p = 0.0003; medium: 2,315 vs. 5,943 m). The longest distance among all nests over multiple breeding seasons per loggerhead females was significantly smaller than the longest distance per simulated female (Figure 6D: χ2 = 4.25; DF = 1, p = 0.039; medium: 5,169 vs. 8,518 m). In short, loggerhead females selected nest sites that were one-third the distance to the nearest neighbor, 40% the distance across nests within a breeding season, and 60% the distance across all breeding seasons.
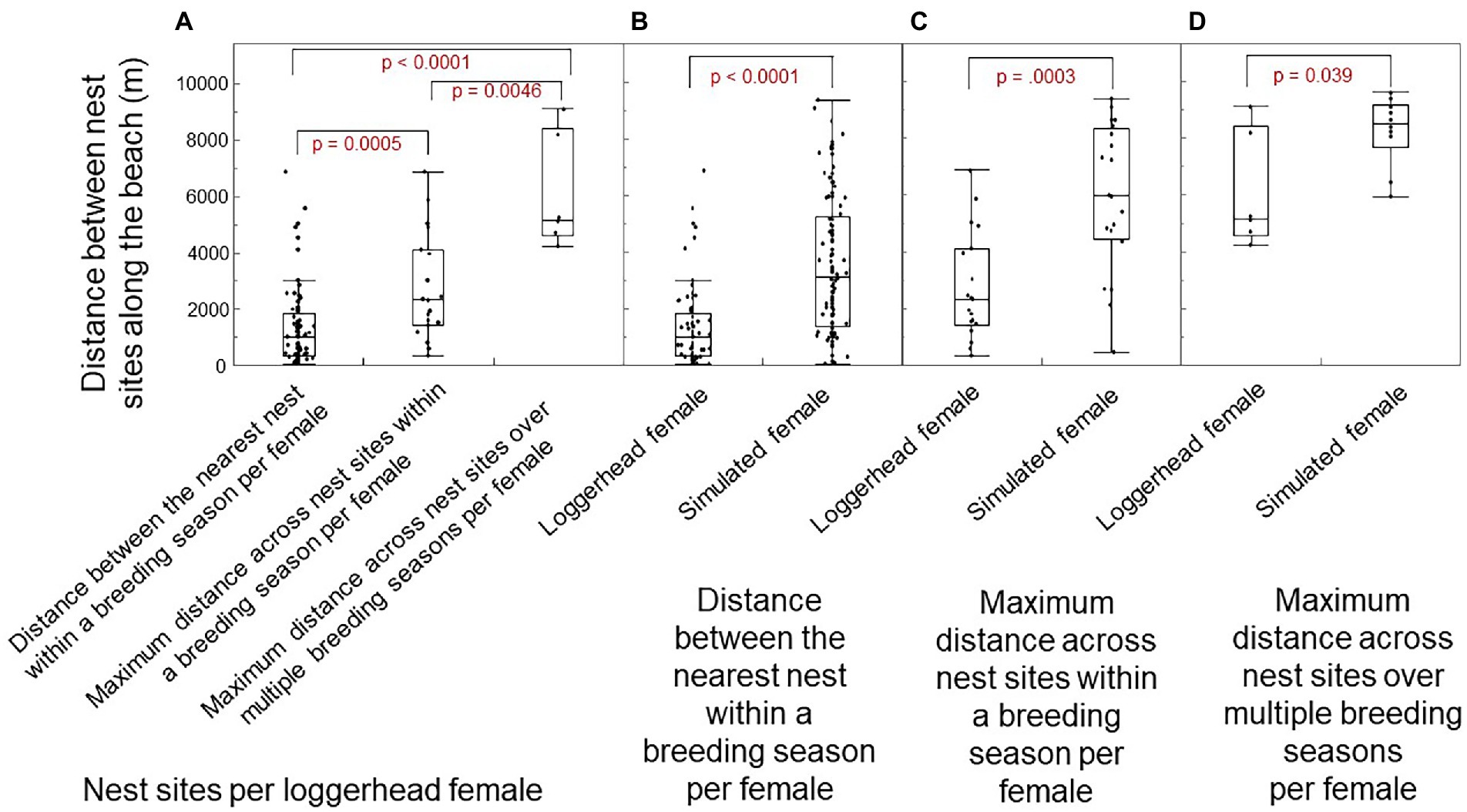
Figure 6. Distances among nests along the beach-length axis of Keewaydin Island for loggerhead females and simulated females. (A) Distance among nests per loggerhead female within and across breeding seasons. (B) Distance between the nearest-neighbor nests within a breeding season by female type. (C) Longest distance across nests within a breeding season by female type. (D) Longest distance across all nests over multiple breeding seasons by female type. The median shows as a line across the box. The ends of the box are the 25 and 75th quantiles (i.e., quartiles). Whiskers enclose all data except outliers. Significant differences show as red.
Because not every female in the current population of breeders will achieve lifetime replacement fitness (Cassill, 2019), we detailed hatching success and failure individually for six loggerhead females who produced at least nine nests over at least two breeding seasons on Keewaydin Island beach from 1996 through 2004. Mean hatching success was 68.3% for Female I with a range of 0–99% (Figure 7A; n = 18 nests). Mean hatching success was 66.4% for Female II with a range of 0–100% (Figure 7B; n = 10 nests). Hatching success was 63.1% for Female III (Figure 7C; range = 0–98%; n = 16 nests). Hatching success was 72.0% for Female IV (Figure 7D; range = 0–99%; n = 12 nests). Mean hatching success was 61.1% for Female V (Figure 7E; range = 0–100%; n = 9 nests); and mean hatching success was 84.8% for Female VI (Figure 7F; range = 32–98%; n = 10 nests).
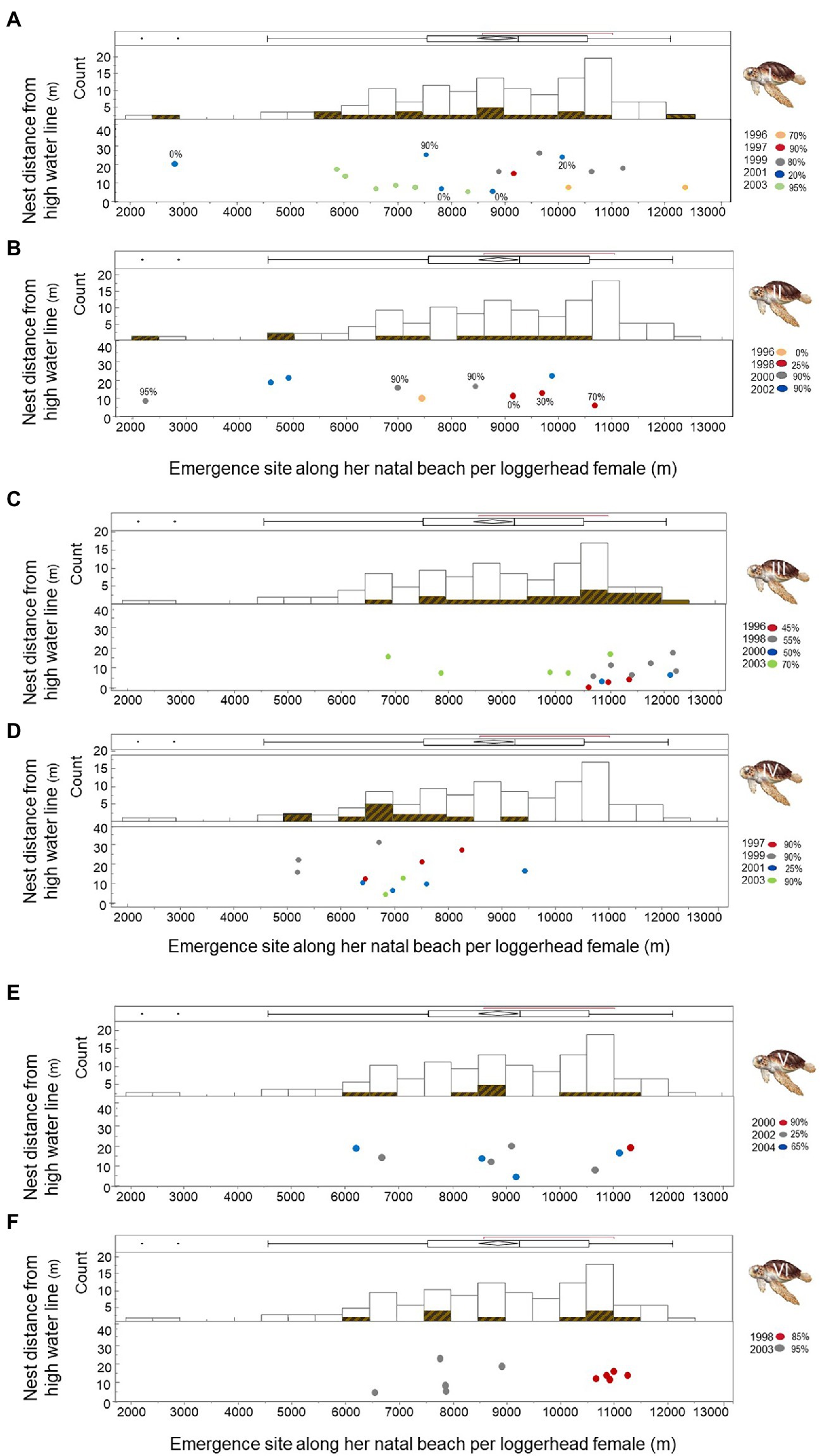
Figure 7. Nest site choice on Keewaydin Island by six loggerhead females during multiple breeding seasons from 1996 through 2004. Histograms represent the location of nest sites by each female relative to the combined nest site choices by the group of females. Dots show the years and location of nest sites for each female (A-F).
Discussion
In our 1996–2004 study of tagged loggerhead females nesting on Keewaydin Island’s beach, hatching success per nest averaged 65.3%. Although nest site choice accounted for 19.2% of explained variation in hatching success, breeding year and breeding month accounted for 81.8% of explained variation. We hypothesized that a simple random distribution of nest sites by loggerhead females could provide significant mitigation of hatching failure. However, our study did not support our null hypothesis. The distribution of nest site choices along the beach-length and beach-width axes did not fit a uniform-random distribution. We show that loggerhead females preferentially deposited 94% of clutches in nest sites over 6,000 m of the 13,000 m beach. Moreover, females deposited 69% of clutches on narrow sections of the beach ranging from 8 to 22 m wide. On narrow section, nests were close to the vegetative line, and at the same time, close to the high water line. On wider areas of the beach, females spread clutches in open-sand sites between the vegetative line and the high water line. By “voting” with their feet, loggerhead females indicated that half of Keewaydin Island was a “good” beach area. Females also preferred to nest on narrower beaches such that their clutches had the benefit of higher elevation near the vegetative line and hatchlings had the benefit of shorter distances to the sea. Occasionally, females selected a nest site far outside the preferred beach length and beach width areas, perhaps on the chance that a high-risk site might pay off.
In short, nest site choice by the population of Keewaydin Island loggerhead females followed a “Goldilocks” distribution strategy that fell between a normal and random distribution. Within breeding seasons, nest sites were not too clustered or too dispersed.
How do females manage to prevent future nest sites from overlapping with nest sites from current or previous breeding seasons? Recent studies show that geomagnetic navigational signatures and local cues such as wind currents, tidal currents, taste, and smell are imprinted in the brains of hatchlings and might continue to be imprinted on the brains of juveniles and adults as they migrate to and from foraging and breeding areas (Papi et al., 2000; Lohmann et al., 2008a,b, 2017; Brothers and Lohmann, 2015; Kishkinev, 2015; Lohmann and Lohmann, 2019). The frontal brain of migratory sea turtles (i.e., the medial cortex) plays a key role in spatial cognition without extensive training (Striedter, 2016; Reiter et al., 2017). In vertebrates, the hippocampus and other homologous brain structures encode and consolidate spatial information into short-term and long-term memory. These findings present an exciting possibility for explaining the mechanisms by which females distribute nest sites that rarely overlap within and among breeding seasons. As they crawl up the beach, secure a nest site, excavate a pit, oviposit eggs, and then bury and camouflage the site, females might imprint the geomagnetic signature of each nest on the medial cortex and at the same time, encode and consolidate local ecological conditions of the beach in their hippocampus homolog.
Do loggerhead females choose a “good” beach or do they select “good” sites on a low-quality beach? Our study suggests that females do both. It is possible that the presence of human activity and dwellings along the first half of Keewaydin Island beach explains their preference for selecting nest sites along the more distant half of the beach (Arianoutsou, 1988; Salmon et al., 1995; Witherington, 1997; Margaritoulis, 2005; Mazaris et al., 2009; Lutcavage, 2017). The steeper slope on the narrow beach areas might explain their preference for nest sites that were closer to the dune and, at the same time, the sea (Hays et al., 1995; Wood and Bjorndal, 2000). Certainly, sand temperature and sand quality played a role in nest site choice (Garmestani et al., 2000; Matsuzawa et al., 2002) as would female size (Hatase and Omuta, 2018) and their immediate energy budget (Hays and Speakman, 1991; Hatase and Tsukamoto, 2008; Perrault and Stacy, 2018).
In conclusion, evidence accumulated over recent decades supports the hypothesis that loggerhead sea turtles use multiple sensory cues when selecting nest sites (Mazaris et al., 2006). With increasing anthropogenic challenges, including rapid climate change, our hope is that this study on nest site choice by loggerhead females nesting on Keewaydin Island encourages other long-term studies of small populations with the aim of improving the management and conservation of sea turtles.
Data availability statement
The raw data supporting the conclusions of this article will be made available by the authors, without undue reservation.
Author contributions
DC analyzed and authored the manuscript. AW contributed the simulation and edited all versions of the manuscript including the final.
Acknowledgments
We thank David Addison and staff from The Conservancy of Southwest Florida for access to the unique data recorded since 1990 on nesting loggerhead sea turtles, their nest sites and hatching success. We also thank Janet Capron for line edits and USF St. Petersburg Campus undergraduate students Cassandra Auseklis, Brenna Madden, Serenity Poston-Hymel, Jocyvette Rivera-Radilla, Malisa Chunsawang, and Amanda Lawniczak for assistance with the graphics on individual nesting females.
Conflict of interest
The authors declare that the research was conducted in the absence of any commercial or financial relationships that could be construed as a potential conflict of interest.
Publisher’s note
All claims expressed in this article are solely those of the authors and do not necessarily represent those of their affiliated organizations, or those of the publisher, the editors and the reviewers. Any product that may be evaluated in this article, or claim that may be made by its manufacturer, is not guaranteed or endorsed by the publisher.
Supplementary material
The Supplementary material for this article can be found online at: https://www.frontiersin.org/articles/10.3389/fevo.2022.850091/full#supplementary-material
References
Ackerman, R. A. (2017). “The nest environment and the embryonic development of sea turtles,” in The Biology of Sea Turtles. eds. P. L. Lutz and J. A. Musick (Boca Raton: CRC Press), 83–106.
Arianoutsou, M. (1988). Assessing the impacts of human activities on nesting of loggerhead sea-turtles (Caretta Caretta L.) on Zakynthos island, western Greece. Environ. Conserv. 15, 327–334. doi: 10.1017/S0376892900029829
Brothers, J. R., and Lohmann, K. J. (2015). Evidence for geomagnetic imprinting and magnetic navigation in the natal homing of sea turtles. Curr. Biol. 25, 392–396. doi: 10.1016/j.cub.2014.12.035
Cassill, D. L. (2019). Extending r/K selection with a maternal risk-management model that classifies animal species into divergent natural selection categories. Sci. Rep. 9, 1–8. doi: 10.1038/s41598-019-42562-7
Cassill, D. L. (2021). Multiple maternal risk-management adaptations in the loggerhead sea turtle (Caretta caretta) mitigate clutch failure caused by catastrophic storms and predators. Sci. Rep. 11, 1–15. doi: 10.1038/s41598-021-81968-0
Esteban, N., Mortimer, J. A., and Hays, G. C. (2017). How numbers of nesting sea turtles can be overestimated by nearly a factor of two. Proc. R. Soc. B Biol. Sci. 284:20162581. doi: 10.1098/rspb.2016.2581
Garmestani, A. S., Percival, H. F., Portier, K. M., and Rice, K. G. (2000). Nest-site selection by the loggerhead sea turtle in Florida's ten Thousand Islands. J. Herpetol. 34, 504–510. doi: 10.2307/1565263
Hatase, H., and Omuta, K. (2018). Nest site selection in loggerhead sea turtles that use different foraging areas: do less fecund oceanic foragers nest at safer sites? J. Zool. 305, 232–239. doi: 10.1111/jzo.12557
Hatase, H., and Tsukamoto, K. (2008). Smaller longer, larger shorter: energy budget calculations explain intrapopulation variation in remigration intervals for loggerhead sea turtles (Caretta caretta). Can. J. Zool. 86, 595–600. doi: 10.1139/Z08-035
Hays, G. C., Mackay, A., Adams, C. R., Mortimer, J. A., Speakman, J. R., and Boerema, M. (1995). Nest site selection by sea turtles. J. Mar. Biol. Assoc. U. K. 75, 667–674. doi: 10.1017/S0025315400039084
Hays, G. C., and Speakman, J. R. (1991). Reproductive investment and optimum clutch size of loggerhead sea turtles (Caretta caretta). J. Anim. Ecol. 60, 455–462. doi: 10.2307/5290
Hughes, E. J., and Brooks, R. J. (2006). The good mother: does nest-site selection constitute parental investment in turtles? Can. J. Zool. 84, 1545–1554. doi: 10.1139/z06-148
Kishkinev, D. (2015). Sensory mechanisms of long-distance navigation in birds: a recent advance in the context of previous studies. J. Ornithol. 156, 145–161. doi: 10.1007/s10336-015-1215-4
Kraemer, J. E., and Bell, R. (1980). Rain-induced mortality of eggs and hatchlings of loggerhead sea turtles (Caretta caretta) on the Georgia coast. Herpetologica 36, 72–77.
Lohmann, K. J., and Lohmann, C. M. (2019). There and back again: natal homing by magnetic navigation in sea turtles and salmon. J. Exp. Biol. 222:jeb184077. doi: 10.1242/jeb.184077
Lohmann, K. J., Lohmann, C. M., and Endres, C. S. (2008b). The sensory ecology of ocean navigation. J. Exp. Biol. 211, 1719–1728. doi: 10.1242/jeb.015792
Lohmann, K. J., Putman, N. F., and Lohmann, C. M. (2008a). Geomagnetic imprinting: a unifying hypothesis of long-distance natal homing in salmon and sea turtles. Proc. Natl. Acad. Sci. 105, 19096–19101. doi: 10.1073/pnas.0801859105
Lohmann, K. J., Witherington, B. E., Lohmann, C. M., and Salmon, M. (2017). “Orientation, navigation, and natal beach homing in sea turtles,” in The Biology of Sea Turtles. eds. P. L. Lutz and J. A. Musick (Boca Raton: CRC Press), 108–135.
Lutcavage, M. E. (2017). “Human impacts on sea turtle survival,” in The Biology of Sea Turtles. eds. P. L. Lutz and J. A. Musick (Boca Raton: CRC press), 387–409.
Margaritoulis, D. (2005). Nesting activity and reproductive output of loggerhead sea turtles, Caretta caretta, over 19 seasons (1984–2002) at Laganas Bay, Zakynthos, Greece: the largest rookery in the Mediterranean. Chelonian Conserv. Biol. 4, 916–929.
Matsuzawa, Y., Sato, K., Sakamoto, W., and Bjorndal, K. (2002). Seasonal fluctuations in sand temperature: effects on the incubation period and mortality of loggerhead sea turtle (Caretta caretta) pre-emergent hatchlings in Minabe, Japan. Mar. Biol. eds. P. L. Lutz and J. A. Musick Boca Raton, 140, 639–646. doi: 10.1007/s00227-001-0724-2
Mazaris, A. D., Matsinos, Y. G., and Margaritoulis, D. (2006). Nest site selection of loggerhead sea turtles: the case of the island of Zakynthos, W Greece. J. Exp. Mar. Biol. Ecol. 336, 157–162. doi: 10.1016/j.jembe.2006.04.015
Mazaris, A. D., Matsinos, G., and Pantis, J. D. (2009). Evaluating the impacts of coastal squeeze on sea turtle nesting. Ocean Coast. Manag. 52, 139–145. doi: 10.1016/j.ocecoaman.2008.10.005
Miller, J. D., Limpus, C. J., and Godfrey, M. H. (2003). “Nest site selection, oviposition, eggs, development, hatching and emergence of loggerhead turtles,” in Loggerhead Turtles. eds. B. Alan and W. Blair, 125–143.
Mortimer, J. A. (1990). The influence of beach sand characteristics on the nesting behavior and clutch survival of green turtles (Chelonia mydas). Copeia 1990, 802–817. doi: 10.2307/1446446
Papi, F., Luschi, P., Akesson, S., Capogrossi, S., and Hays, G. C. (2000). Open-sea migration of magnetically disturbed sea turtles. J. Exp. Biol. 203, 3435–3443. doi: 10.1242/jeb.203.22.3435
Papi, F., Luschi, P., Crosio, E., and Hughes, G. R. (1997). Satellite tracking experiments on the navigational ability and migratory behaviour of the loggerhead turtle Caretta caretta. Mar. Biol. 129, 215–220. doi: 10.1007/s002270050162
Perrault, J. R., and Stacy, N. I. (2018). Note on the unique physiologic state of loggerhead sea turtles (Caretta caretta) during nesting season as evidenced by a suite of health variables. Mar. Biol. 165, 1–6. doi: 10.1007/s00227-018-3331-1
Pfaller, J. B., Limpus, C. J., and Bjorndal, K. A. (2009). Nest‐site selection in individual loggerhead turtles and consequences for doomed‐egg relocation. Conserv. Biol. 23, 72–80.
Reiter, S., Liaw, H. P., Yamawaki, T. M., Naumann, R. K., and Laurent, G. (2017). On the value of reptilian brains to map the evolution of the hippocampal formation. Brain Behav. Evol. 90, 41–52. doi: 10.1159/000478693
Salmon, M., Reiners, R., Lavin, C., and Wyneken, J. (1995). Behavior of loggerhead sea turtles on an urban beach. I. Correlates of nest placement. J. Herpetol. 29, 560–567. doi: 10.2307/1564739
Serafini, T. Z., Lopez, G. G., and da Rocha, P. L. B. (2009). Nest site selection and hatching success of hawksbill and loggerhead sea turtles (Testudines, Cheloniidae) at Arembepe Beach, northeastern Brazil. Phyllomedusa. J. Herpetol. 8, 03–17. doi: 10.11606/issn.2316-9079.v8i1p03-17
Stewart, K. R., and Wyneken, J. (2004). Predation risk to loggerhead hatchlings at a high-density nesting beach in Southeast Florida. Bull. Mar. Sci. 74, 325–335.
Striedter, G. F. (2016). Evolution of the hippocampus in reptiles and birds. J. Comp. Neurol. 524, 496–517. doi: 10.1002/cne.23803
Tucker, A. D. (2010). Nest site fidelity and clutch frequency of loggerhead turtles are better elucidated by satellite telemetry than by nocturnal tagging efforts: implications for stock estimation. J. Exp. Mar. Biol. Ecol. 383, 48–55. doi: 10.1016/j.jembe.2009.11.009
van Houtan, K. S., and Bass, O. L. (2007). Stormy oceans are associated with declines in sea turtle hatching. Curr. Biol. 17, R590–R591. doi: 10.1016/j.cub.2007.06.021
Whitmore, C. P., and Dutton, P. H. (1985). Infertility, embryonic mortality and nest-site selection in leatherback and green sea turtles in Suriname. Biol. Conserv. 34, 251–272. doi: 10.1016/0006-3207(85)90095-3
Witherington, B. E. (1997). “The problem of photopollution for sea turtles and other nocturnal animals,” in Behavioral Approaches to Conservation in the Wild. eds. J. R. Clemmons and R. Buchholz (Cambridge University Press), 303–328.
Keywords: behavioral plasticity, spatial navigation, maternal investment, Caretta caretta, parental care, lifetime replacement fitness, climate change
Citation: Cassill DL and Watkins A (2022) Nest-site choice by loggerhead sea turtles as a risk-management adaptation to offset hatching failure by unpredictable storms and predators. Front. Ecol. Evol. 10:850091. doi: 10.3389/fevo.2022.850091
Edited by:
Jeanine M. Refsnider, University of Toledo, United StatesReviewed by:
Graeme Clive Hays, Deakin University, AustraliaMatthew Ware, Florida State University, United States
Copyright © 2022 Cassill and Watkins. This is an open-access article distributed under the terms of the Creative Commons Attribution License (CC BY). The use, distribution or reproduction in other forums is permitted, provided the original author(s) and the copyright owner(s) are credited and that the original publication in this journal is cited, in accordance with accepted academic practice. No use, distribution or reproduction is permitted which does not comply with these terms.
*Correspondence: Deby L. Cassill, Y2Fzc2lsbEB1c2YuZWR1