- 1Graduate Program in Ecology, Biological Science Institute, University of Brasilia, Brazilia, Brazil
- 2Laboratory of Bat Biology and Conservation, Department of Zoology, Biological Science Institute, University of Brasilia, Brazilia, Brazil
- 3Laboratory of Conservation Planning, Department of Zoology, Biological Science Institute, University of Brasília, Brazilia, Brazil
Ecosystem services (ES) are essential for human society worldwide. ES originate from ecological processes commonly occurring in well-preserved regions. Bats play an essential role in providing such services, primarily insect suppression, plant pollination, and seed dispersal. Human activities have affected Earth’s systems, compromising species and ecosystems and, consequently, the ES provision. Brazil is a country with many bat species but is also one of the world’s leaders in deforestation. Here we aimed to identify regions with high potential for bats ecosystem services provision, assuming that the predicted presence of the species represents the existence of the services. First, we used a Maximum Entropy algorithm to model the distribution of 128 bat species, which correspond to 71% of the Brazilian species. We classify all species into 10 different groups, which resulted from a combination of three body sizes and four predominant trophic guilds (i.e., frugivores, insectivores, nectarivores, and carnivores). The guilds were associated with services of seed dispersion, pest control, pollination, and animal control. Then, we created a 0.5 × 0.5-degree grid to represent an index of ES per guild, which is defined by the sum of the product of the bat’s size weight (1, 2, or 3) by the area occupied of each species in each cell. For comparison, the index was normalized and scaled from 0 to 1. Finally, we used a map of current land use to compare the effects of natural area suppression on the provision of ES in each cell. Our results indicate a substantial reduction in the provision of ES by bats in extensive parts of the central and eastern parts of Brazil, but changes in ES varies among biomes. While the loss of species is an important factor affecting the provision of ES in the Atlantic Forest and the Cerrado, the reduction of species occurrence is most important factor in Amazonia, Caatinga, or Pantanal regions. We suggest that degraded area restoration should be promoted in areas with high ES values and areas near cities and croplands and that a precautionary approach of promoting the conservation of high provider richness should be applied to protect the continuation of bat’s ES.
Introduction
Through land-use change processes habiat loss has been pointed to as a major driver of biodiversity loss worldwide (Foley et al., 2005; Newbold et al., 2015). The human ecological footprint is such that biologically and ecologically intact landscapes devoid of anthropogenic disturbances stand for only 23% of Earth’s terrestrial surface (Watson et al., 2016). About 40–50% of occupied areas have been converted to near homogeneous urban or agriculture-dominated landscapes (Chapin, Zavaleta et al., 2000; Barnosky et al., 2012). Such simplified landscapes support less diverse communities than those originally present in the native systems (Tscharntke et al., 2005; Schipper et al., 2008; Flynn et al., 2009). Consequently, human’s indiscriminate interventions interfere with both structure and functioning of ecosystems (Hooper et al., 2012), often resulting in less adaptive systems that are highly vulnerable to disturbances and changing environmental conditions (Folke et al., 2002).
Stability is a desirable characteristic when considering our dependence on the current state of functioning of ecosystems. Stability is often associated with resilience, which relates to a system’s reorganization capacity after disturbance without disrupting crucial ecosystem processes and characteristics (Holling, 1973). One way species diversity contributes to resilience is through mechanisms of redundancy. Redundancy relates to a situation where the same function is performed by multiple species (Walker, 1992). Thus, ecosystem processes are safeguarded against loss of function through local extinctions (Yachi and Loreau, 1999; Oliver et al., 2015). Effects of redundancy on function stability are more significant if the species of interest differ in their responses to environmental change (Mouillot et al., 2013; Oliver et al., 2015), which implies the need for diversity within the group of species sharing ecological roles. Such view benefits from the reunion of species into functional groups representative of a suite of organisms with similar functional traits related to their shared ecological role (Luck et al., 2009).
Bats form the second most speciose order (Burgin et al., 2018). Most of this diversity is concentrated in the Neotropics, where bats gather in ecologically diverse assemblages critical for ecosystems (Medellín et al., 2000; Meyer and Kalko, 2008). Their contribution results from the diversity of ecological roles performed by bat species. Through morphological and sensorial adaptations, they can explore a various of resources such as fruit, nectar, pollen, insects, small vertebrates, and even blood (Fenton, 1992). These give bats a place in almost every trophic level. While acting as seed dispersers, pollinators, and predators, bats’ roles in the ecosystem can be translated into services (Kunz et al., 2011; Aguiar et al., 2021).
Bats build on nature’s contributions to people (Díaz et al., 2018) as ecosystem services (ES) providers (Luck et al., 2009). Bats sustain plant diversity (Wang and Smith, 2002) by acting as mobile agents of seed and pollen for hundreds of species (Kunz et al., 2011), facilitating tropical forest succession and accelerating the regeneration of degraded areas such as abandoned pastures (Galindo-González et al., 2000; Muscarella and Fleming, 2007). From a more direct perspective of human welfare, bats play essential roles in agriculture by acting as biological agents of pests suppression in crucial crops, reducing plant damage, and increasing crop yield (Maine and Boyles, 2015; Taylor et al., 2017; Aguiar et al., 2021). Bats also interact mutualistically with economically essential species associated with the survival and cultural reproduction of many social groups (Chévez-Pozo and Ortiz, 1997; Aguiar and Antonini, 2008; Scanlon et al., 2014; Aziz et al., 2017).
Despite the ubiquity of bats and the relative ease of spot one flying by in disturbed and undisturbed areas, their nocturnal and volant habits make diversity relatively inconspicuous and ongoing declines considerably less evident. Nonetheless, bat communities in heterogeneous native habitats can differ substantially in abundance and composition from those found in adjacent occupied areas such as urban and agricultural landscapes (Medellín et al., 2000; Frank et al., 2017; Gonçalves et al., 2017; Jung and Threlfall, 2018). This indicates that habitat conversion, translated into the simplification of landscapes, results in simplified communities remaining to support the ecological functions bats offer for humans’ benefit. This is a worrisome scenario given that diverse communities are key to the delivery stable functions (Tilman, 1999) and services (Isbell et al., 2011; Bartomeus et al., 2013).
Bat research has a long history of studies relating form to function (Vaughan, 1959; Norberg, 1972; Barclay and Brigham, 1991; Muscarella and Fleming, 2007) and grouping bats into body size classes is a common approach. Bat body size is known to influence resource use, with larger bats being able to use a greater diversity of resources and with greater intensity (Bonaccorso, 1979; Barclay and Brigham, 1991; Fleming, 1991; Kalko et al., 1996; Wendeln et al., 2000; Houston et al., 2004; Bonaccorso et al., 2007; Esbérard and Bergallo, 2009). Although the relationship between body size and its importance on ES provision is a theoretically valid assumption (Peters, 1983) it requires more conclusive testing. Nevertheless, these aspects are expected to differentiate larger, medium, and smaller bats in terms of how each size group potentially participates in providing associated ecosystem services. Furthermore, body size is also a well-established response trait indicating vulnerability to disturbances like habitat fragmentation among bats (Schulze et al., 2000; Farneda et al., 2015).
Without proper planning, we may reduce to fragments areas with the potential to harbor great diversity and potentially sustain stable services through high provider richness (Isbell et al., 2011). The present study aims to determine for Brazil, a large-scale neotropical country, the negative impacts of habitat conversion on bat diversity and associated services, considering their functional roles and the importance of the habitat areas lost for sustaining such diversity and services. We apply a broad definition of ES that considers ecosystem functions (Isbell et al., 2011; Ceauşu et al., 2021). We employ species distribution models (SDMs) to depict the environmental suitability of sites within each species’ distribution. We use guilds as a way of assembling bats within functional groups (Simberloff and Dayan, 1991) associated with their ecological functions (ca. frugivores, nectarivores, insectivores, and carnivores) and account for the diversity of species performing similar functions (ca. “guild diversity,” see Elmqvist et al., 2003) by classifying bats within each guild according to body size. Thus, we assume traits are associated with the relative importance of individual species for ES provision (Ceauşu et al., 2021) and use body size as a best-available proxy for a large-scale assessment of bat contribution to ES.
As our working hypothesis, we expect that the loss of native areas will affect the feeding bat guilds differently, being more significant in guilds with a low level of redundancy (than in guilds with higher redundancy. Thus, we expect groups with less redundancy (lower species richness, such as carnivores and nectarivores) will be more impacted by deforestation than frugivores and insectivores. Likewise, we expect the loss of ecosystem services will follow the species’ body size, being more significant in large species than in medium and small species. Finally, understanding diversity can buffer ES provision loss through compensation mechanisms, and we test for the role of species richness and redundancy on ES persistence in the face of natural habitat suppression. We expect ES will persist in altered areas, although reduced, unless redundancy is completely lost.
Materials and Methods
Study Area
We considered the Brazilian territory in the study as its 8.5 million km2 makes it the largest country in Latin America (5th in the world), ranging from various climatic zones from the humid tropics in the north to temperate areas in the south. Those climatic differences led to the formation of the following distinct biogeographic regions: the Amazon and Atlantic Forest (rainforest), the Pantanal (floodplain), the Cerrado (Neotropical savannah), the Caatinga (tropical dry forest), and the Pampa (natural grasslands) (Figure 1).
Species Distribution Models
Species Occurrences
We gathered occurrence data throughout all of South America for the 181 bat species currently recognized to occur in Brazil (available at https://www.sbeq.net/lista-de-especies, Nogueira et al., 2018). The dataset consisted of 7,730 records describing the geographical distribution of bats within Brazil (Aguiar et al., 2020) and 28,496 records obtained from the Global Biodiversity Information Facility—GBIF1 complementing the data with a representation of bat records across South America (Supplementary Figure 1). The Brazilian dataset was assembled from museum vouchers, published literature, and fieldwork by the Laboratory of Bat Biology and Conservation at the University of Brasília, coordinated by L.M.S. Aguiar.
The GBIF collected occurrences were then subjected to an examination of taxonomic and spatial errors. We followed the taxonomy adopted by Nogueira et al. (2018), and spatial issues of spatial autocorrelation and sampling bias were assessed separately (Syfert et al., 2013). First, the Clark-Evans index (Clark and Evans, 1954) for all species showed some level of sample clustering, so a general spatial thinning was done to maintain unique records for any locality within a 10 km radius (Phillips et al., 2009). Second, now considering the distribution of point density along with the map (Fithian and Hastie, 2013; Renner et al., 2014), we performed the balanced design described at Kramer-Schadt et al. (2013), aiming to balance the representation (i.e., point density) of sampled areas and to avoid over-representation of certain aspects of the environmental space due to artifacts of sampling bias (see Supplementary Material 2 for detailed method). All analyses were conducted in R 3.4.1 (R Core Team, 2021). We used the package dismo (Hijmans et al., 2011) and rangeBuilder (Rabosky et al., 2016) for the general spatial filter and the sf (Pebesma, 2018), lwgeom (Pebesma, 2020), and dplyr (Wickham et al., 2021) packages for the balanced design. The data treatment described above resulted in a dataset of 12,058 occurrences, from which 128 species were considered to have sufficient records (10 or more observed presences) for distribution modeling (Wisz et al., 2008; Varela et al., 2014).
Environmental Variables
We extracted environmental information from the bioclimatic variables available at the WorldClim database2 (Hijmans et al., 2005). We first considered the 19 bioclimatic current variables at 2.5 arc min resolution (ca. 5 km), all derived from monthly temperature and rainfall values. We tested the correlation between pairs of groups of variables (i.e., temperature bio1 to bio11 and precipitation bio12 to bio19) by using the Pearson’s test (Supplementary Tables 1A,B). In each group, we kept only one of the variables each variable when pairs showed values of over 70% of correlation. This resulted in the selection of eight bioclimatic variables described as annual mean temperature (BIO1), mean diurnal temperature range (BIO2), isothermality (BIO3), temperature seasonality (BIO4), the maximum temperature of the warmest month (BIO5), annual precipitation (BIO12), precipitation of the driest month (BIO14), and precipitation seasonality (BIO15), as used to model bats distribution in Aguiar et al. (2016).
Species Distribution Model—Species Distribution Model—Building
We used the MaxEnt software Version 3.4.0 (Phillips et al., 2017), a widely used presence-background algorithm. MaxEnt derives a species’ distribution by comparing the environmental conditions within the area where the species is known to occur with the conditions along with the background (i.e., area of unknown presence or absence) and then projects this environmental relation to the geographical space (Phillips et al., 2006). For model construction, we initially used the geographic extent of South America and subsequently cropped the results to the boundaries of Brazil and within the country, for each biome. As a rule, 10,000 points were randomly sampled as background, and we randomly set aside 10–20% of the data for internal model testing. The test/train ratios depended on the number of records available for each species. We used the latest MaxEnt’s complementary log log (cloglog) output, which carries the same advantages as the previous logistic output, but with greater theoretical support for its interpretations (Fithian et al., 2015; Phillips et al., 2017).
We used a regularization multiplier of 2.5, a magnitude proper for fitting less complex and more general models (Elith et al., 2010; Radosavljevic and Anderson, 2014). Finally, we assessed model accuracy with True Statistic Skill—TSS (Allouche et al., 2006), a threshold-dependent measure unaffected by prevalence and validation dataset size. It considers omission and commission errors and ranges from −1 to + 1, where values below zero indicate a performance no better than random. We established a TSS threshold value of 0.6 to determine whether a model presented satisfying predictive performance or not. Classifying bats.
The species distribution modeling results are represented in a continuous surface of values ranging from 0 to 1, showing areas where species are potentially more likely to occur due to their environmental suitability. These distribution models were reclassified to produce a discrete distribution map for each species. Our maps were then grouped into 10 categories resulting from a combination of four bat guilds (e.g., frugivores, insectivores, nectarivores, and carnivores) and three size classes (e.g., small, medium, and large). We placed omnivorous species into all guilds their feeding habitats are known to contribute to, and we excluded hematophagous species from further analysis because of their small representation in our validated models.
Classifying Bats
We classified the species within each guild according to the body size classes of small, medium, and large bats. Our approach used mean forearm length as a proxy for body size, which is an adequate classification method since forearm measures are precise and associated with bat’s body length and mass content (Meng et al., 2016; Thiagavel et al., 2017). We gathered the morphological data from published literature (Supplementary Table 2). With a kmeans clustering analysis, we identified intervals of mean forearm lengths that represented small, medium, and large categories for each guild separately. These were then attributed to the values of 1, 2, and 3, respectively.
The hierarchical classification expresses the decrease in the number of species for a potential replacement with increased body size. Further, it also expresses the broader functional range of larger species, which are less limited by morphology in performing their ecological functions (Fleming, 1991; Kalko et al., 1996; Houston et al., 2004). Thus, we attributed greater value to larger species due to their rarity and potential functional uniqueness within their guilds.
Ecosystem Services Potential Analysis
We considered all species indicated by the modeling process in each biome separately for this stage. We analyzed the potential contribution of bats to ecosystem service in each major Brazilian biomes, which were divided in a regular grid of cells with a resolution of 0.5 × 0.5 decimal degrees (Figure 1). Within each grid cell we calculated a diversity index of provider richness (hereafter Index of Potential Ecosystem Services Provision or ES index) like the one applied in Ceauşu et al., 2021. This was done by summing the area occupied by a species within the same guild multiplied by their class size (i.e., 1, 2, or 3). The grid cell values were normalized by dividing each cell value by the maximum value found among cells for each biome separately. This resulted in values ranging from 0 to 1 representative of the cell’s importance for providing of each guild’s associated services. We repeated this analysis using all bats independent of the guild in each biome and considered the scenarios with and without natural habitat suppression.
Impacts of Natural Habitat Suppression
We wanted to test how habitat conversion has affected the potential provision of ecosystem services by bat species in Brazil, identifying regions with different loss of ecosystem service provision.
For the representation of land conversion, we used the MapBiomas version 5 land cover product,3 representing the year 2020. The map is presented at a 30 m resolution and features several landcover classes. We resampled it to the same spatial resolution as our models (ca. 2.5 arc min resolution). We created a binary map of natural vegetation remnants, which included all classes of forest and non-forest natural formations as remnants. All areas occupied by pastures, agriculture, urban infrastructure, and water bodies became zero-valued. Thus, this mask was used to suppress suitable areas within the species’ potential distribution. With it, we could compare the potential for ES provision prior to and after natural area suppression. With this approach, we assume that diversity, its functions, and associated services depend on the existence of natural remnants. This means we followed the notion of “supporting systems” suggested in Kremen (2005) and Tscharntke et al. (2005), i.e., any diversity present in altered landscapes depends upon the support of the surrounding native landscape. All spatial analyses were conducted in the R software (R Core Team, 2021), using the packages raster (Hijmans, 2021) and sf (Pebesma, 2018).
Statistical Analysis
Species Contributions and Redundancy for Ecosystem Services Provision
Magnitude of Losses
We compared each biome’s loss of ecosystem services with a Kruskal-Wallis test since the distribution pattern was not normal (Shapiro test W = 0.8061, p < 0.001). Then we used the function kwAllPairsNemenyiTest from PMCMRplus package (Pohlert, 2021) to perform a non-parametric pairwise comparison between all biomes.
We used a binomial proportion test (two proportions z-test) to test our hypothesis. Proportion tests were performed between guilds to see whether we would observe a gradient of losses following the expected pattern of carnivory > nectarivory > frugivory > insectivory. Another set of tests was performed between size classes within the guild and within all bats to ascertain whether proportional losses were associated with bat’s body size in the respective order: large > medium > small. All analyses were conducted in the R software (R Core Team, 2021).
Species Contributions and Redundancy for Ecosystem Services Provision
We were interested in identifying which features of diversity work to maintain the ecosystem service provision within grids where natural habitat suppression has occurred. We applied generalized linear models (GLM) with Gaussian distribution using the ES index values after deforestation as our response variable and remnant area occupied by species plus the number of lost species of the same size class as our explanatory variables. We only consider insectivores, frugivores, and nectarivores for this stage because of their number of species. This was done separately for each guild within each biome separately.
Results
Bats Species Distribution Models and Classification
We generated 128 generated models with good predictive performance. TSS values ranged from 0.6 to 0.97, with 53 species validated with scores between 0.6 and 0.69 and 60 species validated between 0.7 and 0.97. Five species were removed from the study due to unsatisfactory model results after a visual inspection. Thus, all results presented here are based on 128 bat species, of which 76 were identified as insectivores, 36 as frugivores, 12 as nectarivores, and four as carnivores (Supplementary Table 2).
We calculated for each trophic guild three mean forearm lengths intervals (small, medium, and large) using kmeans clustering (Table 1). Overall, there were 63 small, 38 medium, and 27 large bat species. Size differences were most marked for insectivorous and frugivorous bats (Table 1) and after specialist consultation we decided to keep only small and medium classes for nectarivores and only medium and large for carnivores. Overall, most species within each guild were classified as small (Supplementary Table 2).
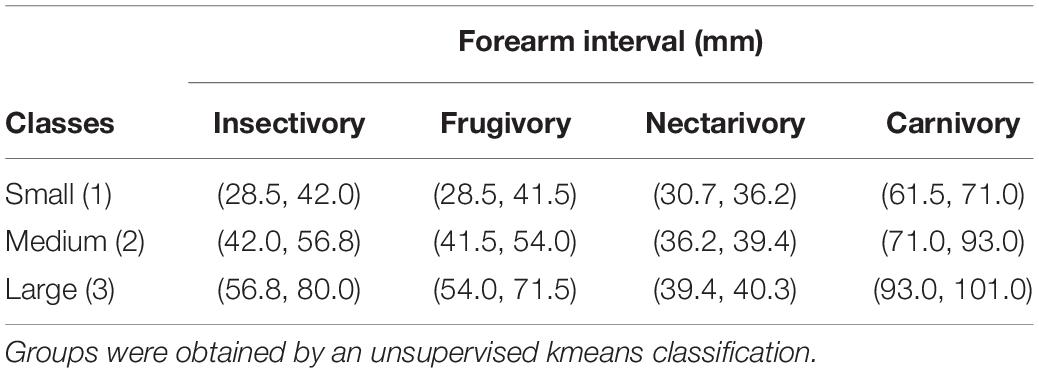
Table 1. Forearm length intervals corresponding small, medium, and big size classes for each trophic group considered.
Provision Potential of Ecosystem Services Associated to the Brazilian Bat Fauna
For Brazil and for each biome, values of the corresponding potential for the provision of ES were identified (i.e., disregarding natural area suppression). Overall, the values of the ES index varied among biomes, indicating differences in species compositions between regions (Table 2). Cerrado was the only biome that presented mean ES index values greater than the national average for all guilds (Table 2). The potential for insectivores’ associated services is predicted to be greater in the Pantanal, Caatinga, and Cerrado biomes (Table 2). Nectarivores species presented higher values for the Amazon and Caatinga, on average. Frugivory seems to be well distributed across Brazil, with the weakest potentials predicted for Caatinga and Pampa (Table 2). Finally, carnivory potential is predicted to be distributed toward Pantanal, Atlantic Forest, and Caatinga (Table 2).
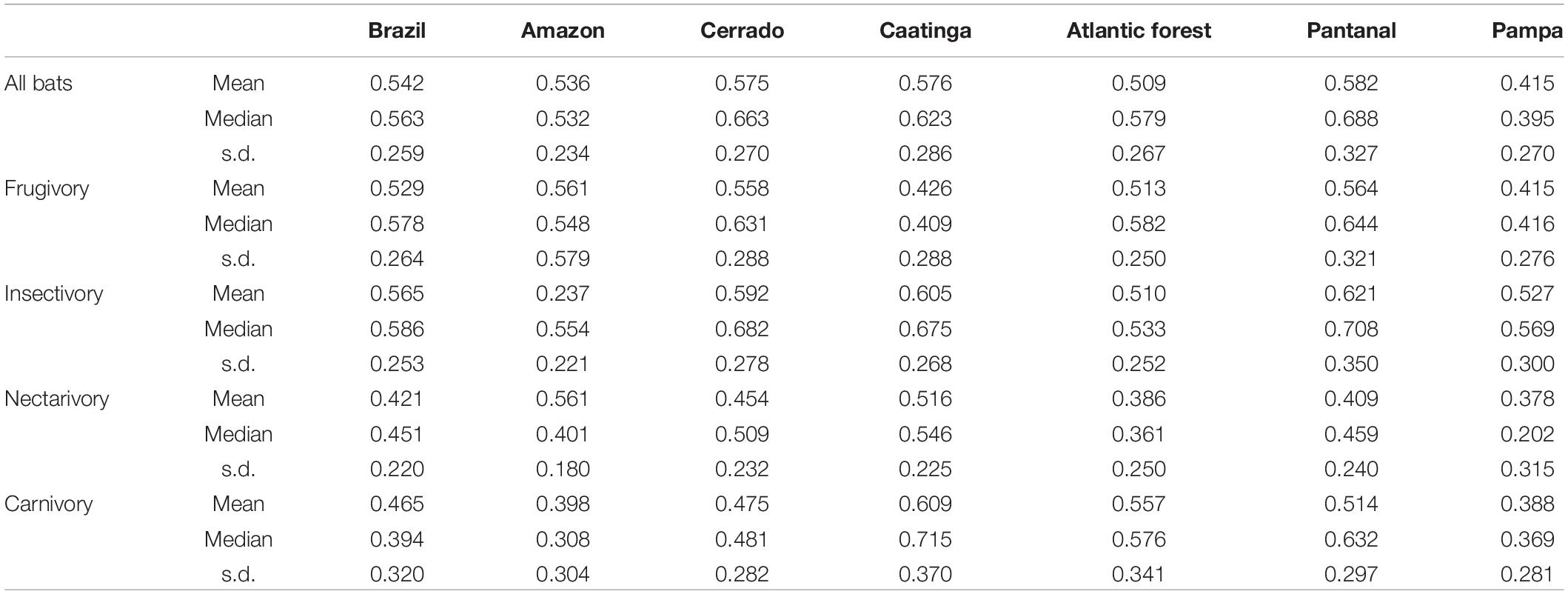
Table 2. Provision values (mean, median, and standard deviation) of ecosystem services associated with bat’s feeding guilds in Brazil per biome.
Knowing the potential provision scores for a country or region is not the only way of assessing a place’s likelihood of providing or sustaining specific ecosystem services. A complementary approach is to see how these values are distributed in space. The Cerrado, for instance, has 60.99% of its original cells, i.e., without considering area suppression, presenting ES index values greater than the national average. The other biomes have 55.52% (Caatinga), 54.44% (Pantanal), 52.83% (Atlantic Forest), 50.81% (Amazon), and 44.44% (Pampa) (Table 3).
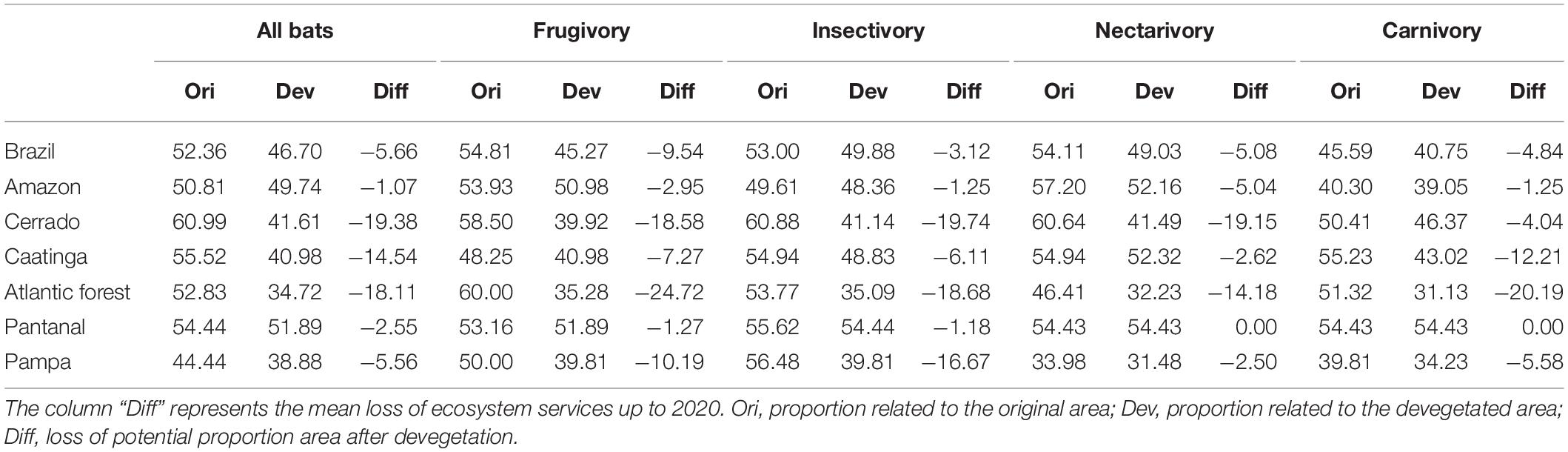
Table 3. Mean value of the ecosystem services index provide by bats considering the original (column ORI) and remaining native (column Dev) in Brazil and biomes.
Ecosystem Services Provisioning Potential Losses
With the historical occupation of Brazil, biomes’ ES provisioning potential eroded. The Atlantic Forest biome is an extraordinary case, with a total loss of more than 70% of its original area (ca.1.1 million km2). Another striking case is the one of Cerrado, which had lost more than 1 million km2 of its native area. Considering a threshold of 50% of remaining native areas, the percentage of cells below such limit is 74.53% for Atlantic Forest, 59.39% for Pampa, 55.65% for Cerrado, 27.84% for Pantanal, 27.62% for Caatinga, and 16.05% for Amazon.
The land conversion process observed up to 2020 has impacted the provision of ES differently in each biome and, on average, the Cerrado, Atlantic Forest, and Caatinga, were the biomes with the highest losses (Table 3 and Figure 2—Mapa de perda de ES). Most of central-southern Cerrado, central Atlantic Forest, and eastern Caatinga had heavily lost the provision potential of ES. When comparing the absolute loss of ES potential, i.e., the values resulting from the difference of the original provision by the remaining provision after natural area suppression, Atlantic Forest is the biome with the highest loss, followed by Cerrado, Caatinga, and Pampa, and Amazonia (Kruskal-Wallis chi2 = 685.81, p < 0.001) (Figure 3). The proportion of cells with expressive loss of ES is 62.26% for Atlantic Forest, 50.77% for Cerrado, 44.44% for Pampa, 33.72% for Caatinga, 24.05% for Pantanal, and 16.31% for Amazonia. Considering the cells with ES index equals or greater than the national mean, only 28.3% of essential areas for ecosystem services provision stand in Brazil (Figure 4). This proportion changes among the biomes, being 7.9% for Atlantic Forest, 16.7% for Pampa, 19.6% for Cerrado, 24.5% for Caatinga, 39.8% for Amazonia, and 50.1% for Pantanal.
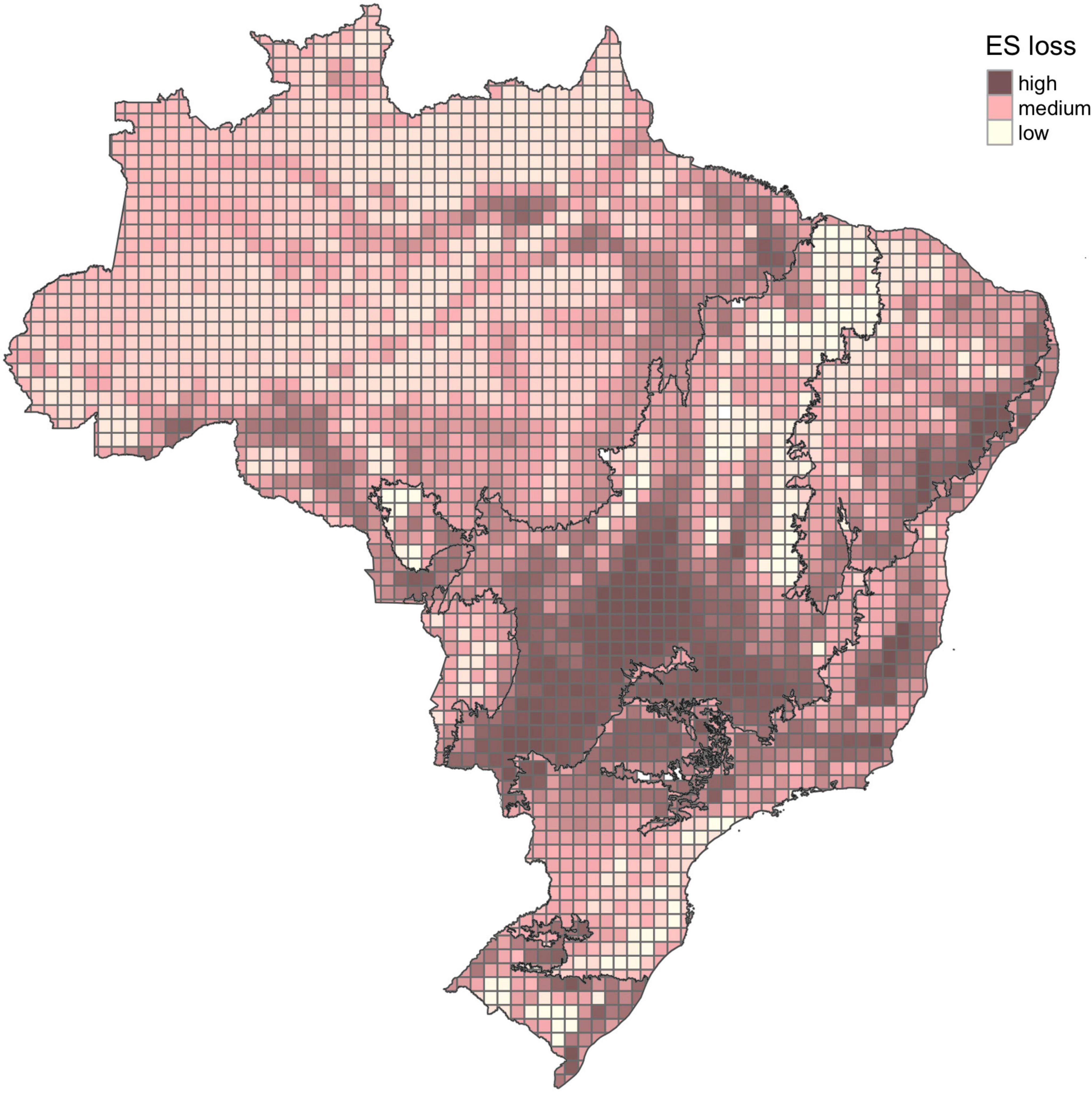
Figure 2. Loss of ecosystem services (ES) potentially provided by bats in Brazil. Classes (high, medium, low) considering the land use for 2020.
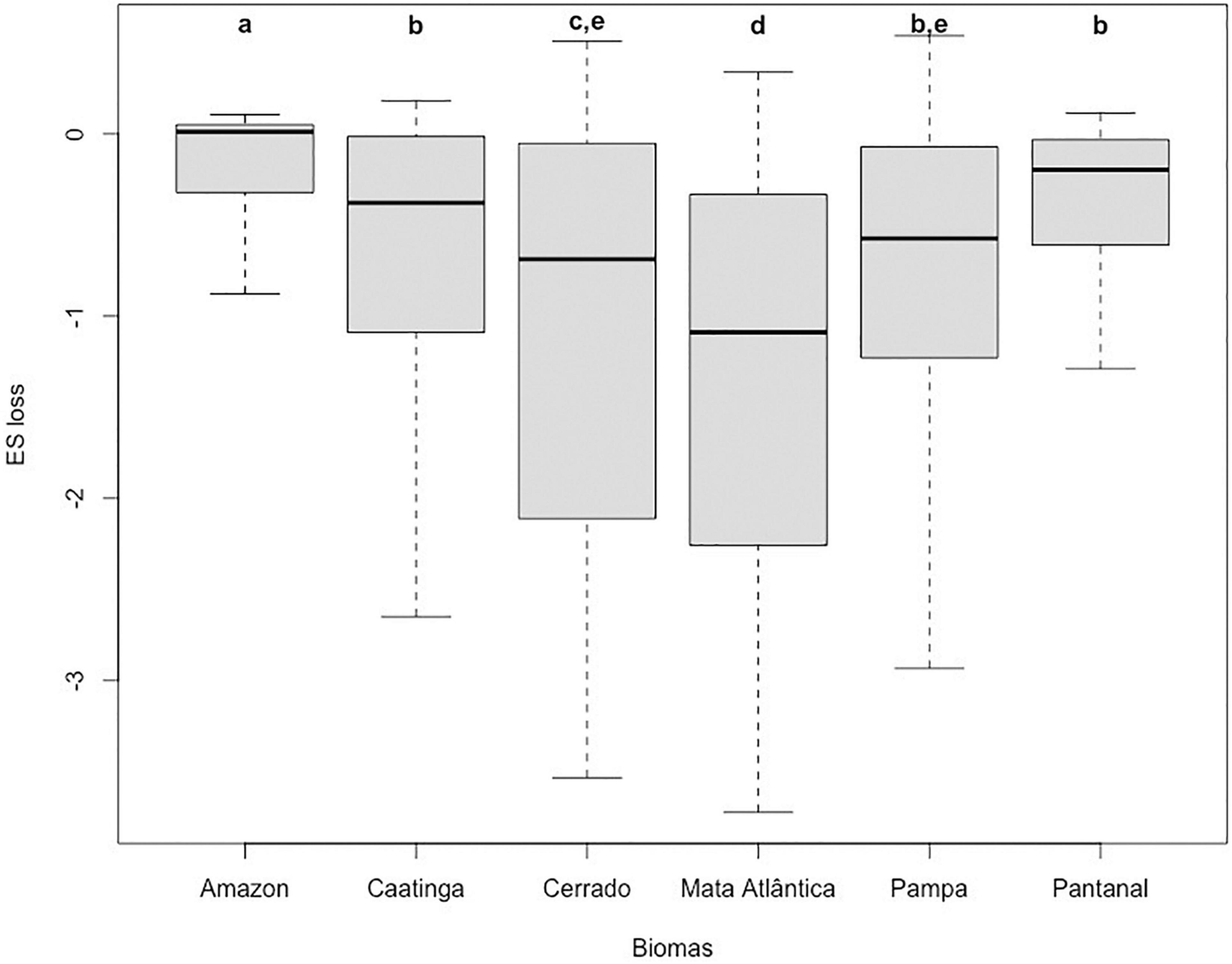
Figure 3. Loss of ecosystem services (ES) per biome and between biome differences. Significance is related to the post hoc pair-wise comparison test (p < 0.05).
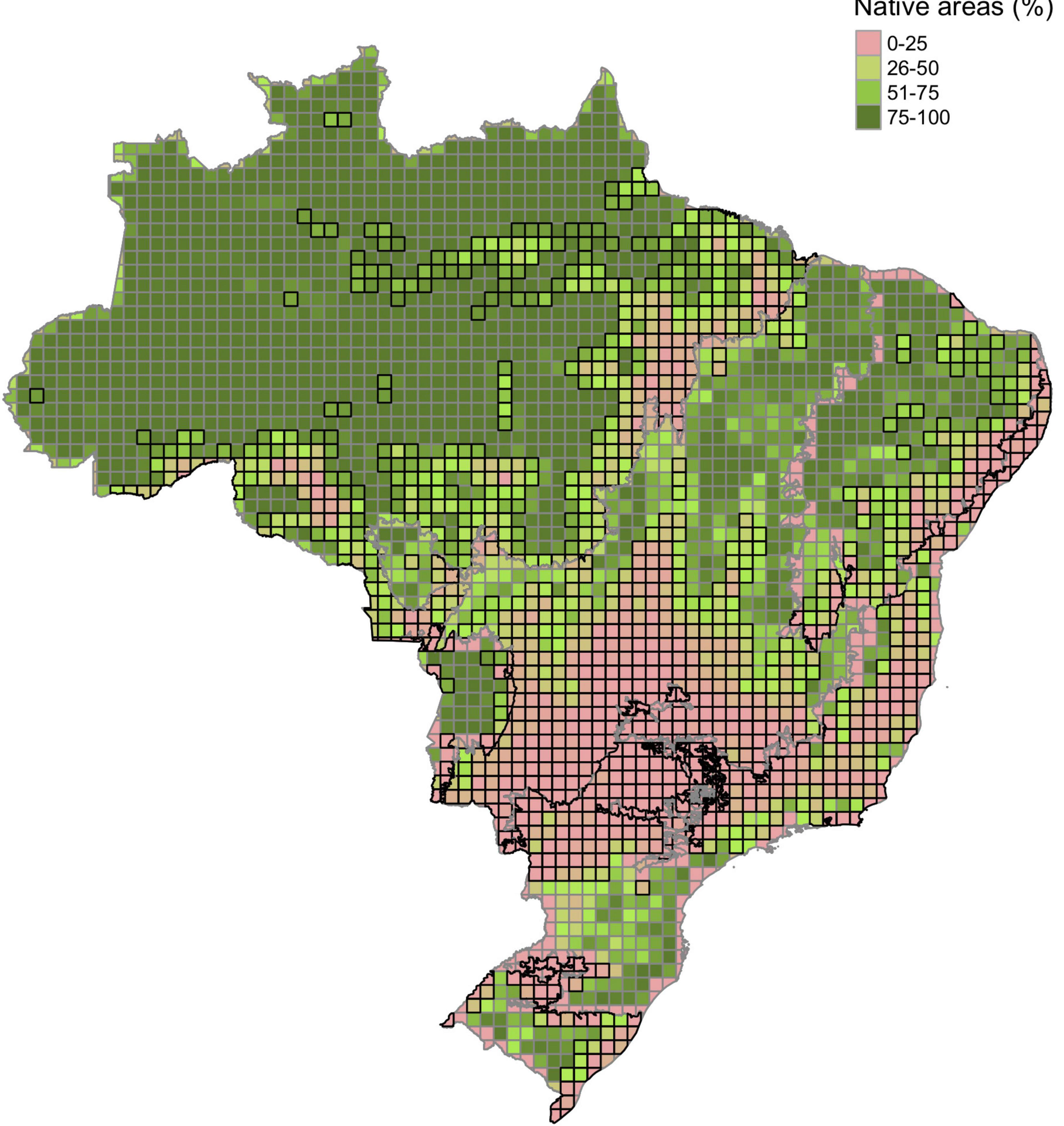
Figure 4. Natural vegetation remaining areas and the cells with loss of Ecosystem Service (ES) greater than the average of the biome. Ecosystem services include all guilds (carnivores, frugivores, insectivores, and nectarivores).
Guild’s Responses to Land Conversion
The negative impacts of natural area conversion in Brazil varied among guilds and regionally. The general pattern for Brazil is a decrescent and significant impact of devegetation on insectivores, frugivores, carnivores, and nectarivores (Kruskal-Wallis chi2 = 391.49, p < 0.001) (Table 4). However, the pattern of impacts on different guilds varied per biome. The frugivores were more impacted in the Amazon, while insectivores and frugivores were more impacted in the Cerrado and Pantanal. In Atlantic Forest, there was no statistical difference between carnivores, frugivores, and insectivores, but these three categories were more impacted than nectarivores (Table 4). The Pampa was the unique biome where the devegetation impact affected equally all guild categories (Table 4).
Responses of Different Body Size Classes to Land Conversion
Considering all feeding guilds, the loss of ES provided by large species was significantly higher than the other two size categories (Yemeni test, p < 0.05), but there was no statistical difference between medium and small size species (Yemeni test, p > 0.05) (Table 5). This result partially corroborated our working hypothesis and was regionally observed in Amazon and Cerrado. We observed, as expected, the loss of bat ecosystem services in the Atlantic Forest, higher in large species than in medium and smaller-size species (Table 5). Furthermore, we did not detect a significant loss of size groups ES for the Pantanal and Pampa biomes (Table 5).
Considering each feeding guild separately, we observed different responses for each biome. For carnivores, the loss of ES per size group was not significantly different for Brazil, Amazon, Pantanal, and Pampa. We observed the expected trend for Caatinga and Atlantic Forest (Table 5). For the Cerrado, we observed the expected trend for all groups except for carnivores and nectarivores (Table 5). For Brazil, Cerrado, and Atlantic Forest, we observed the loss of ES per size group according to expectations for frugivores. The expected trend was observed only for insectivores in the Atlantic Forest and for most other biomes, we found only a partial corroboration. Finally, nectarivores were the only group with a consistent response but presented the opposite pattern than expected (i.e., small species being more impacted than medium-size species).
Species Richness, Redundancy, and Ecosystem Service Maintenance
Our results indicated that reducing area of occurrence and loss of species impacted the provision of ES by bats in Brazil. However, such changes vary among biomes (Table 6). For Atlantic Forest and Cerrado, both factors significantly reduced the ES index values for multiple groups. For instance, for frugivores and nectarivores, the reduction of species richness and occurrence area had significant relationship with the loss of ES in Cerrado (Medium frugivores species loss: t = −4.808, p < 0.001; Medium frugivores occurrence area reduction: t = 7.674, p < 0.001) (Table 6). A similar pattern was found for the Atlantic Forest, where loss of species and occurrence area reduction were relevant, but only for small nectarivores (Small nectarivores species loss: t = 2.494, p < 0.05; Small nectarivores area reduction: t = 7.121, p < 0.05). For the other bat groups only one of the factors significantly reduced the ES index values. For example, small insectivores associated services were affected only by the loss of species, whereas medium insectivores, only by the reduction of occurrence area (Small insectivores: t = −3.535, p < 0.05; Medium insectivores: t = 7.572, p < 0.05). For the biomes of Amazon, Caatinga, and Pampa only the reduction of occurrence area was significantly associated with the loss of ES index values for all guilds and sizes (Table 6).
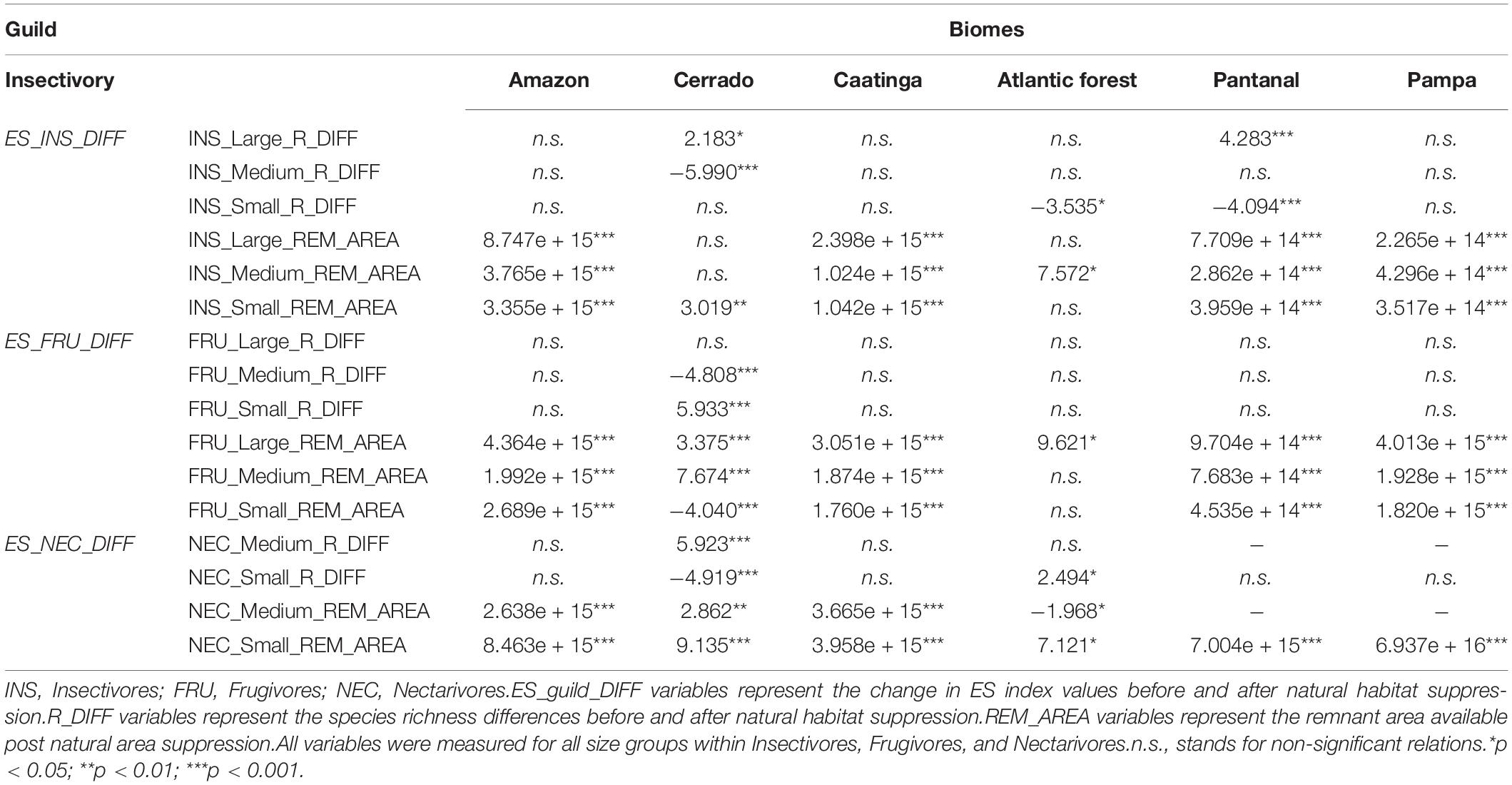
Table 6. Results of the GLMs testing the effects of occurrence area reduction and of species loss on the ES index values after the incorporation of natural area suppression for the most speciose guilds.
Discussion
Our results reflect distributional patterns of ES provisioning potential and overall functional impacts of natural area suppression on regional bat assemblages across Brazil. We observed that ES index values vary between biomes, indicating regional differences in the composition of species available for service provision. Furthermore, guilds are differently impacted by land conversion with no apparent pattern. This indicates that different functional groups should be the focus of conservation actions in different regions. However, we did find a general relationship between the magnitude of loss and species body size. Except for nectarivores, in all other guilds and guilds combined, larger bats were generally more impacted by natural habitat conversion than the other size classes. Thus, larger bats have lost a greater extension of environmentally adequate area within their distribution and are disappearing at a faster rate than their smaller counterparts. By our assumptions, these species hold greater ES provisioning importance, and such pattern of losses should come with greater ecological impact. This general pattern of downsizing and its functional consequences have been documented for other taxonomical groups (Dirzo et al., 2014; Galetti et al., 2015; Donoso et al., 2020).
Generally, biomes held an above average potential for ES provisioning for about half of their territories. This means that about half of each biome is represented by essential areas for ES provision due to their predicted high provider richness (Isbell et al., 2011). Consequently, these regions should be safeguarded from further degradation if we aim to keep historical levels of ES potential in our biomes. However, the natural area suppression culminated in expressive loss of ES index values for large extensions across biomes. Considering a habitat threshold of 50% remaining area, biomes such as Atlantic Forest, Cerrado and Pampa have over half of their territories below this limit. This is a worrisome scenario since bat communities were shown to be sensitive to habitat loss, presenting a higher-than-average habitat threshold before abundances and diversity start to accentually drop (Muylaert et al., 2016). This means that these extensive areas likely hold impoverished communities despite their potentials to hold above area average diversity and provide above average levels of ES.
How Are We Losing Services?
ES provisioning potential is being lost across Brazil through two main processes. First, by guild occurrence area reduction where land conversion shrinks the available suitable area for species within a guild. This habitat area reduction likely culminates in population declines, negative impacting ES provision numerically (Kremen, 2005; Watling et al., 2020). Second, by the loss of species. Some species may lose all their previously available area to land conversion and be considered locally extinct. This reduces the number of provider species present and adds yet another layer of ES vulnerability, the loss of redundancy within guilds (Elmqvist et al., 2003). One can imagine that ES can be sustained in low provider richness situations if the populations of the few species present are healthy enough to maintain a stable provision (Kremen, 2005). However, habitat loss affects both species population and communities’ composition, likely affecting ES provision and stability synergically (Oliver et al., 2015).
Both processes (i.e., occurrence area reduction and species loss) were not always relevant for all groups within all biomes. Meaning that some groups’ associated services were more impacted by area reduction, species loss or both. This is to be expected since guilds are differently distributed across biomes and biomes have different histories of occupation (Lapola et al., 2014). For example, for biomes where anthropogenic occupation is advanced (e.g., Cerrado and Mata Atlantica), both processes seem relevant, although not for all size groups within guilds. This means that beyond occurrence area reduction, species are being lost and this is also significantly impacting the ES potential of the region. Consequently, these regions are experiencing a strong enough loss of provider richness that outweighs the compensational effects of the remaining species within the assemblage. On the other hand, biomes where anthropogenic occupation is not yet as drastic or follows different spatial patterns (e.g., Amazon and Caatinga), reduction of occurrence area is the main driver of ES index values differences. Thus, area reduction is driving the observed differences in ES values, meaning that the functional impact of species losses is being buffered by the functional effect of remaining species. In the scenario, ES should be better safeguarded through mechanisms of redundancy. Are we losing ecosystem services?
Studies on bat’s provision of ecosystem services have mainly focused on the aggregate function (i.e., the service resulting from the ensemble of providers present, Kremen, 2005). For example, a common practice for the studies on pest suppression is exclosure experiments (Maas et al., 2016). While these studies offer essential insights on local diversity impacts on crop yield and quality (Williams-Guillén et al., 2008; Maas et al., 2013; Maine and Boyles, 2015), little light is shed on the dynamics of provision. Even with the aggregate service measure at hand (e.g., number of fruit sets, plant damage), questions of how much diversity is needed for the desired outcome or which species/traits are associated with the effectiveness of service delivery remain unanswered. As such, these results offer evidence for service existence while making good cases for conservation of bat diversity, accounting for the importance of maintaining multiple species contributions to a service (Elmqvist et al., 2003; Aguiar et al., 2021). In other words, the importance of maintaining high provider richness (Isbell et al., 2011).
Thus, conservation of guild diversity is an essential precautionary approach to ecosystem services persistence in front of uncertainties in the dynamics of provision since it aims to secure function stability (Walker, 1992). As we assumed, function stability implies redundancy, which implies replacement among species (Luck et al., 2009). Through our classification, the potential for redundancy (i.e., the number of species for replacement) decreases as body size increases. This is a pattern observed on mutualistic networks (Zamora, 2000). It means that the local loss of larger species with relatively greater functional importance should come with greater functional consequences due to reduced stability and the trait-limited possibility of compensatory responses from other species. Consequently, this may result in rapid function loss (Kremen, 2005). As reported, we observed such general pattern, but our results cannot ascertain that this is happening because we are not actually measuring ES provision, we calculated a proxy theoretically associated with the potential for ES provision.
Furthermore, in the case of redundancy, the very own capacity of the bat population to respond in a compensatory way should be experimentally investigated. Neotropical bat communities are not consistently structured by competitive interactions (Stevens and Willig, 2000), and bat species are long-lived organisms with low fecundity (Findley, 1993). Thus, bats are likely not responsive to population fluctuations and not capable of the rapid growth such compensatory responses require. Instead, due to their high mobility, function stability might depend on a rescue effect from surrounding populations, which suggests the importance of biodiversity conservation on a landscape scale and the preservation of diversity sources (Tscharntke et al., 2005; Farneda et al., 2018). We argue that areas with above average ES index values should be considered diversity sources for regional ES provision and, as such, should be of conservational focus and restoration actions.
Despite the uncertain role of redundancy in bat function stability, guild diversity can enhance aggregate function through mechanisms of species complementarity (Gaston and Spicer, 2004; Williams-Guillén et al., 2008; Aguiar et al., 2021). Bats exploring similar resources separate their uses in space and time (i.e., niche partitioning) (Arlettaz et al., 1997; Schnitzler and Kalko, 2001; Lopez and Vaughan, 2007; Amaral et al., 2016). Consequently, species performing similar functions at different spatial-temporal scales provide stability to ecosystem services provision (Peterson et al., 1998). This reiterates the importance of conserving the natural levels of diversity of regional bat assemblages. However, in the finer scale of ecological function, species likely differ in their potential contribution to ecosystem services provision, and body size, as we assumed, could be a trait dictating this in bats, as it is on other vertebrates (Luck et al., 2012). If such is proven, it is also a well-established response trait indicating vulnerability to disturbances like habitat fragmentation (Schulze et al., 2000; Farneda et al., 2015) and greater attention directed toward those species.
Identifying potential key provider species and their contributions to aggregate function and the stability of associated ES is crucial and urgent. The current trend of rapid destruction of native habitat may be leading to the disruption of ecosystem functioning and service provision with unknown consequences. The true state of the functional contribution of bat species and assemblages across Brazil to ecosystem service provision is yet to be described. As we stated, our results reflect general patterns, and any attempt to describe the effective distribution of ES provision by bats would be speculative. Many assumptions remain to be tested for our understanding of the dynamic ES provided by bats to be consolidated. For now, we may be losing the benefits provided by these populations without even acknowledging their existence. Although Brazil is one of the countries with the most incredible diversity of bat species (181), this diversity could not be completely represented in our study for a couple of reasons. First, there are not enough records of good enough quality for all species to be modeled. In our study, seventy-eight species (42.6%) could not be modeled due to the lack of records. Secondly, Brazil is large country where only a few areas were well surveyed, remaining many areas with very few surveys. This causes an imbalance on the representation of the environmental space occupied by large ranged species that resulted in badly fit models even for species that had sufficient occurrence records (e.g., Desmodus rotundus, Carollia perspicillata, etc.).
If, on the one hand, the species that we modeled were sufficient to produce a scenario of the potential provision of ecosystem services in Brazil and its biomes, on the other, we detected that there is still a large gap to be filled concerning the occurrence of species in Brazil, as already pointed out by Bernard et al. (2011) and Aguiar et al. (2020). The search for records must be done optimally since the inclusion of new records in little-known regions can substantially alter the distribution modeling results (Aguiar et al., 2015). Furthermore, the study on bat’s contribution should be done at the scale of the service, but country scale descriptions of species distribution must also continue in parallel. This way we can continue to improve our methods for large-scale descriptions of ecosystem service provision and better incorporate this aspect in future conservation plans.
Concluding Remarks
Our results showed how the potential for ecosystem services provision associated with each bat guild is differentially distributed across Brazil, how each biogeographical region holds different species assemblages of providers, and how habitat conversion is affecting these groups. Our study is limited to descriptions and presents the many pros and cons of the SDM method (See Delgado-Jaramillo et al., 2020). Many questions about the role of bats in the provision of ecosystem services need to be further addressed. How much diversity is needed for the desired outcome of an ecosystem service? What is the relative role of abundance and species richness in ecosystem service provision? Which species are the key ecosystem service providers? Is aggregate function in high diversity areas different from those found in less diverse areas? How land use affects aggregate function? The answer to those questions will deepen our understanding of the underlying role of bat species as ecosystem services providers in Brazil and how our interventions on the landscape have been affecting such contributions. Nevertheless, we believe our results can substantiate public policies for the identification of degraded areas where redundancy is not yet completely lost and historical levels of ecosystem service provision can be regained by restoration. We suggest that degraded area restoration should be promoted in areas with high ES values and areas near cities and croplands and that a precautionary approach of promoting the conservation of high provider richness should be applied to protect the continuation of bat’s ES.
Data Availability Statement
Publicly available datasets were analyzed in this study. This data can be found here: Species data available at: doi:10.5061/dryad.c59zw3r5.
Author Contributions
LA and LB contributed to conceptualization of the study. LA organized the database. RM and LB performed the analysis. LB wrote the first draft of the manuscript. All authors contributed to manuscript revision, read, and approved the submitted version.
Funding
LB was supported by the CNPq M.Sc scholarship (Grant # 135848/2017-2). LA (Process # 304989/2019-3) and RM (Process # 304221/2019-8) research was also funded by research productivity grants awarded by CNPq.
Conflict of Interest
The authors declare that the research was conducted in the absence of any commercial or financial relationships that could be construed as a potential conflict of interest.
Publisher’s Note
All claims expressed in this article are solely those of the authors and do not necessarily represent those of their affiliated organizations, or those of the publisher, the editors and the reviewers. Any product that may be evaluated in this article, or claim that may be made by its manufacturer, is not guaranteed or endorsed by the publisher.
Acknowledgments
We thank the teams of the Laboratory of Bat Biology and Conservation and the Laboratory of Conservation Planning for the data and support during the development of this research. We also thank the two reviewers that help us improve the manuscript. And we thank CNPq for the funding that allowed this research to be developed and published.
Supplementary Material
The Supplementary Material for this article can be found online at: https://www.frontiersin.org/articles/10.3389/fevo.2022.852177/full#supplementary-material
Footnotes
References
Aguiar, L. M. S., and Antonini, Y. (2008). Diet of two sympatric insectivores’ bats (Chiroptera: Vespertilionidae). Rev. Bras. Zool. 25, 28–31.
Aguiar, L. M. S., Bernard, E., Ribeiro, V., Machado, R. B., and Jones, G. (2016). Should I stay or should I go? Climate change effects on the future of Neotropical savannah bats. Glob. Ecol. Conserv. 5, 22–33. doi: 10.1016/j.gecco.2015.11.011
Aguiar, L. M. S., Bueno-Rocha, I. D., Oliveira, G., Pires, E. S., Vasconcelos, S., Nunes, G. L., et al. (2021). Going out for dinner – the consumption of agriculture pests by bats in urban areas. PLoS One 16:e0258066. doi: 10.1371/journal.pone.0258066
Aguiar, L. M. S., da Rosa, R. O. L., Jones, G., and Machado, R. B. (2015). Effect of chronological addition of records to species distribution maps: The case of Tonatia saurophila maresi (Chiroptera, Phyllostomidae) in South America. Aust. Ecol. 40, 836–844.
Aguiar, L. M. S., Ramos Pereira, M. J., Zortea, M., and Machado, R. B. (2020). Data from: where are the bats? An environmental complementarity analysis in a megadiverse country. Dryad Digit. Reposit. *vol&page, doi: 10.5061/dryad.c59zw3r52
Allouche, O., Tsoar, A., and Kadmon, R. (2006). Assessing the accuracy of species distribution models: prevalence, kappa and the true skill statistic (TSS). J. Appl. Ecol. 43, 1223–1232. doi: 10.1111/j.1365-2664.2006.01214.x
Amaral, T. S., Macário, L. M., and Aguiar, L. M. S. (2016). Testing the Coexistence of Artibeus lituratus and A. planirostris in a neotropical savanna. Acta Chiropterol. 18, 441–449. doi: 10.3161/15081109acc2016.18.2.011
Arlettaz, R., Perrin, N., and Hausser, J. (1997). Trophic resource partitioning and competition between the two sibling bat species myotis myotis and Myotis blythii. J. Anim. Ecol. 66, 897–911.
Aziz, S. A., Clements, G. R., McConkey, K. R., Sritongchuay, T., Pathil, S., Abu Yazid, M. N. H., et al. (2017). Pollination by the locally endangered island flying fox (Pteropus hypomelanus) enhances fruit production of the economically important durian (Durio zibethinus). Ecol. Evol. 7, 8670–8684. doi: 10.1002/ece3.3213
Barclay, R. M. R., and Brigham, R. M. (1991). Prey detection, dietary niche breadth, and body size in bats: why are aerial insectivorous bats so small? Am. Nat. 137, 693–703.
Barnosky, A. D., Hadly, E. A., Bascompte, J., Berlow, E. L., Brown, J. H., Fortelius, M., et al. (2012). Approaching a state shift in Earth’s biosphere. Nature 486, 52–58. doi: 10.1038/nature11018
Bartomeus, I., Park, M. G., Gibbs, J., Danforth, B. N., Lakso, A. N., and Winfree, R. (2013). Biodiversity ensures plant-pollinator phenological synchrony against climate change. Ecol. Lett. 16, 1331–1338. doi: 10.1111/ele.12170
Bernard, E., Aguiar, L. M. S., and Machado, R. B. (2011). Discovering the Brazilian bat fauna: a task for two centuries? Mammal Rev. 41, 23–39. doi: 10.1111/j.1365-2907.2010.00164.x
Bonaccorso, F. J. (1979). Foraging and reproductive ecology in a panamanian bat community. Bull. Florida Mus., Biol. Sci. 24, 359–408.
Bonaccorso, F. J., Winkelmann, J. R., Shin, D., Agrawal, C. I., Aslami, N., Bonney, C., et al. (2007). Evidence for exploitative competition: comparative foraging behavior and roosting ecology of short-tailed fruit bats (Phyllostomidae). Biotropica 39, 249–256.
Burgin, C. J., Colella, J. P., Kahn, P. L., and Upham, N. S. (2018). How many species of mammals are there? J. Mammal. 99, 1–14. doi: 10.1093/jmammal/gyx147
Ceauşu, S., Apaza-Quevedo, A., Schmid, M., Martín-López, B., Cortés-Avizanda, A., Maes, J., et al. (2021). Ecosystem service mapping needs to capture more effectively the biodiversity important for service supply. Ecosyst. Serv 48:101259. doi: 10.1016/j.ecoser.2021.101259
Chapin, F. S., Zavaleta, E. S., Eviner, V. T., Naylor, R. L., Vitousek, P. M., Reynolds, H. L., et al. (2000). Consequences of changing biodiversity. Nature 405, 234–242. doi: 10.1038/35012241
Chévez-Pozo, O. V., and Ortiz, M. A. (1997). O Pequi (Caryocar brasiliense): viabilidade socioeconômica do cerrado no norte de minas gerais. Cad. Adm. Rural. 9, 203–218.
Clark, P. J., and Evans, F. C. (1954). Distance to nearest neighbour as a measure of spatial relationships in populations. Ecology 35, 445–453. doi: 10.2307/1931034
Delgado-Jaramillo, M., Aguiar, L. M. S., Machado, R. B., and Bernard, E. (2020). Assessing the distribution of a species-rich group in a continental-sized megadiverse country: bats in Brazil. Divers. Distrib. 26, 632–643. doi: 10.1111/ddi.13043
Díaz, S., Pascual, U., Stenseke, M., Martín-López, B., Watson, R. T., Molnár, Z., et al. (2018). Assessing nature’s contributions to people. Science 359, 270–272. doi: 10.1126/science.Aap8826
Dirzo, R., Young, H. S., Galetti, M., Ceballos, G., Isaac, N. J., and Collen, B. (2014). Defaunation in the anthropocene. Science 345, 401–406. doi: 10.1126/science.1251817
Donoso, I., Sorensen, M. C., Blendinger, P. G., Kissling, W. D., Neuschulz, E. L., Mueller, T., et al. (2020). Downsizing of animal communities triggers stronger functional than structural decay in seed-dispersal networks. Nat. Commun. 11:1582. doi: 10.1038/s41467-020-15438-y
Elith, J., Kearney, M., and Phillips, S. (2010). The art of modelling range-shifting species. Methods Ecol. Evol. 1, 330–342. doi: 10.1111/j.2041-210X.2010.00036.x
Elmqvist, T., Folke, C., Nystrom, M., Peterson, G., Bengtsson, J., Walker, B., et al. (2003). Response diversity, ecosystem change, and resilience. Front. Ecol. Environ. 1:488–494. doi: 10.2307/3868116
Esbérard, C., and Bergallo, H. (2009). Do bigger bats need more time to forage? Braz. J. Biol. 68, 819–822. doi: 10.1590/s1519-6984200800040001
Farneda, F. Z., Rocha, R., López-Baucells, A., Groenenberg, M., Silva, I., Palmeirim, J. M., et al. (2015). Trait-related responses to habitat fragmentation in Amazonian bats. J. Appl. Ecol. 52, 1381–1391. doi: 10.1111/1365-2664.12490
Farneda, F. Z., Rocha, R., López-Baucells, A., Sampaio, E. M., Palmeirim, J. M., Bobrowiec, P. E., et al. (2018). Functional recovery of amazonian bat assemblages following secondary forest succession. Biol. Conserv. 218, 192–199. doi: 10.1016/j.biocon.2017.12.036
Fithian, W., Elith, J., Hastie, T., and Keith, D. A. (2015). Bias correction in species distribution models: pooling survey and collection data for multiple species. Methods Ecol. Evol. 6, 424–438. doi: 10.1111/2041-210X.12242
Fithian, W., and Hastie, T. (2013). Finite-sample equivalence in statistical models for presence-only data. Ann. Appl. Stat. 7, 1917–1939. doi: 10.1214/13-AOAS667
Fleming, T. H. (1991). The relationship between body size, diet, and habitat use in frugivorous bats, genus carollia (Phyllostomidae). J. Mammal. 72, 493–501. doi: 10.2307/1382132
Flynn, D. F. B., Gogol-Prokurat, M., Nogeire, T., Molinari, N., Richers, B. T., Lin, B. B., et al. (2009). Loss of functional diversity under land use intensification across multiple taxa. Ecol. Lett. 12, 22–33. doi: 10.1111/j.1461-0248.2008.01255.x
Foley, J. A., DeFries, R., Asner, G. P., Barford, C., Bonan, G., Carpenter, S. R., et al. (2005). Global consequences of land use. Science 309, 570–574. doi: 10.1126/science.1111772
Folke, C., Carpenter, S., Elmqvist, T., Gunderson, L., Holling, C. S., and Walker, B. (2002). Resilience and sustainable development: building adaptive capacity in a world of transformations. Ambio 31, 437–440. doi: 10.1579/0044-7447-31.5.437
Frank, H. K., Frishkoff, L. O., Mendenhall, C. D., Gretchen, C. D., and Hadly, E. A. (2017). Phylogeny, traits, and biodiversity of a neotropical bat assemblage: close relatives show similar responses to local deforestation. Am. Nat. 190, 200–212. doi: 10.1086/692534
Galetti, M., Bovendorp, R. S., and Guevara, R. (2015). Defaunation of large mammals leads to an increase in seed predation in the Atlantic forests. Glob. Evol. 3, 824–830. doi: 10.1016/j.gecco.2015.04.008
Galindo-González, J., Guevara, S., and Sosa, V. J. (2000). Bat- and bird-generated seed rains at isolated trees in pastures in a tropical rainforest. Conserv. Biol. 14, 1693–1703. doi: 10.1111/j.1523-1739.2000.99072.x
Gaston, K. J., and Spicer, J. I. (2004). Biodiversity. An Introduction. Oxford: Blackwell Publishing.
Gonçalves, F., Fischer, E., and Dirzo, R. (2017). Forest conversion to cattle ranching differentially affects taxonomic and functional groups of neotropical bats. Biol. Conserv. 210, 343–348. doi: 10.1016/j.biocon.2017.04.021
Hijmans, R. J. (2021). raster: Geographic Data Analysis and Modeling. R Packg. Version 3.4-13. Available online at: https://CRAN.R-project.org/package=raster.
Hijmans, R. J., Cameron, S. E., Parra, J. L., Jones, P. G., and Jarvis, A. (2005). Very high-resolution interpolated climate surfaces for global land areas. Int. J. Climatol. 25, 1965–1978. doi: 10.1002/joc.1276
Hijmans, R. J., Phillips, S., Leathwick, J., and Elith, J. (2011). dismo: Species Distribution Modeling. R Packg. Version 1.3-5. Available online at: http://cran.r-project.org/web/packages/dismo/index.html
Holling, C. S. (1973). Resilience and stability of ecological systems. Annu. Rev. Ecol. Syst. 4, 1–23.
Hooper, D. U., Adair, E. C., Cardinale, B. J., Byrnes, J. E. K., Hungate, B. A., Matulich, K. L., et al. (2012). A global synthesis reveals biodiversity loss as a major driver of ecosystem change. Nature 486, 105–108. doi: 10.1038/nature11118
Houston, R. D., Boonman, A. M., and Jones, G. (2004). Echolocation in Bats and Dolphins. Chicago: University of Chicago Press.
Isbell, F., Calcagno, V., Hector, A., Connolly, J., Harpole, W. S., Reich, P. B., et al. (2011). High plant diversity is needed to maintain ecosystem services. Nature 477, 199–202. doi: 10.1038/nature10282
Jung, K., and Threlfall, C. G. (2018). Trait-dependent tolerance of bats to urbanization: a global meta-analysis. Proc. R. Soc. B Biol. Sci. 285:20181222. doi: 10.1098/rspb.2018.1222
Kalko, E. K. V., Herre, E. A., and Handley, C. O. (1996). Relation of fig fruit characteristics to fruit-eating bats in the new and old-world tropics. J. Biogeogr. 23, 565–576.
Kramer-Schadt, S., Lindenborn, J., Reinfelder, V., Stillfried, M., Schr, B., Heckmann, I., et al. (2013). The importance of correcting for sampling bias in MaxEnt species distribution models. Divers. Distrib. 19, 1366–1379. doi: 10.1111/ddi.12096
Kremen, C. (2005). Managing ecosystem services: what do we need to know about their ecology? Ecol. Lett. 8, 468–479. doi: 10.1111/j.1461-0248.2005.00751.x
Kunz, T. H., de Torrez, E. B., Bauer, D., Lobova, T., and Fleming, T. H. (2011). Ecosystem services provided by bats. Ann. N. Y. Acad. Sci. 1223, 1–38. doi: 10.1111/j.1749-6632.2011.06004.x
Lapola, D. M., Martinelli, L. A., Peres, C. A., Ometto, J. P. H. B., Ferreira, M. E., Nobre, C. A., et al. (2014). Pervasive transition of the Brazilian land-use system. Nat. Clim. Change 4, 27–35. doi: 10.1038/nclimate2056
Lopez, J. E., and Vaughan, C. (2007). Food niche overlap among neotropical frugivorous bats in costa rica. Rev. Biol. Trop. 55, 301–313.
Luck, G. W., Harrington, R., Harrison, P. A., Kremen, C., Pam, M., Bugter, R., et al. (2009). Quantifying the contribution of organisms to the provision of ecosystem services. Biosci. J. 59, 223–232. doi: 10.1025/bio.2009.59.3.7
Luck, G. W., Lavorel, S., McIntyre, S., and Lumb, K. (2012). Improving the application of vertebrate trait-based frameworks to the study of ecosystem services. J. Anim. Ecol. 81, 1065–1076. doi: 10.1111/j.1365-2656.2012.01974.x
Maas, B., Clough, Y., and Tscharntke, T. (2013). Bats and birds increase crop yield in tropical agroforestry landscapes. Ecol. Lett. 16, 1480–1487. doi: 10.1111/ele.12194
Maas, B., Karp, D. S., Bumrungsri, S., Darras, K., Gonthier, D., Huang, J. C. C., et al. (2016). Bird and bat predation services in tropical forests and agroforestry landscapes. Biol. Rev 91, 1081–1101. doi: 10.1111/brv.1221
Maine, J. J., and Boyles, J. G. (2015). Bats initiate vital agroecological interactions in corn. Proc. Natl. Acad. Sci. U.S.A. 112, 12438–12443. doi: 10.1073/pnas.1505413112
Medellín, R. A., Equihua, M., and Amin, M. A. (2000). Bat diversity and abundance as indicators of disturbance in neotropical rainforests. Conserv. Biol. 14, 1666–1675. doi: 10.1111/j.1523-1739.2000.99068.x
Meng, F., Zhu, L., Huand, W., Irwin, D. M., and Zhang, S. (2016). Bats: body mass index, forearm mass index, blood glucose levels and SLC2A2 genes for diabetes. Sci. Rep. 6:29960. doi: 10.1038/srep29960
Meyer, C. F. J., and Kalko, E. K. V. (2008). Bat assemblages on neotropical land-bridge islands: nested subsets and null model analyses of species co-occurrence patterns. Divers. Distrib. 14, 644–654. doi: 10.1111/j.1472-4642.2007.00462.x
Mouillot, D., Graham, N. A. J., Villéger, S., Manson, N. W. H., and Bellwood, D. R. (2013). A functional approach reveals community response to disturbances. Trends Ecol. Evol. 28, 167–177. doi: 10.1016/j.tree.2012.10.004
Muscarella, R., and Fleming, T. H. (2007). The role of frugivorous bats in tropical forest succession. Biol. Rev. 82, 573–590. doi: 10.1111/j.1469-185X.2007.00026.x
Muylaert, R. L., Stevens, R. D., and Ribeiro, M. C. (2016). Threshold effect of habitat loss on bat richness in cerrado-forest landscapes. Ecol. Appl. 26, 1854–1867. doi: 10.1890/15-1757.1
Newbold, T., Hudson, L. N., Hill, S. L. L., Contu, S., Lysenko, I., Senior, R. A., et al. (2015). Global effects of land use on local terrestrial biodiversity. Nature 520, 45–50. doi: 10.1038/nature14324
Nogueira, M. R., Lima, I. P., Garbino, G. S. T., Moratelli, R., Tavares, V. C., Gregorin, R., et al. (2018). Updated Checklist of Brazilian Bats: Version 2018.1. Comitê da Lista de Morcegos do Brasil—CLMB. Sociedade Brasileira para o Estudo de Quirópteros (Sbeq). Available online at: http://www.sbeq.net/updatelist
Norberg, U. M. (1972). Bat wing stuctures important for aerodinamics and rigidity (Mammalia, Chiroptera). J. Morphol. 73, 45–61.
Oliver, T. H., Heard, M. S., Isaac, N. J. B., Roy, D. B., Procter, D., Eigenbrod, F., et al. (2015). Biodiversity and resilience of ecosystem functions. Trends Ecol. Evol. 30, 673–684. doi: 10.1016/j.tree.2015.08.009
Pebesma, E. (2018). Simple features for R: standardized support for spatial vector data. R. J. 10, 439–446. doi: 10.32614/RJ-2018-009
Pebesma, E. (2020). lwgeom: Bindings to Selected ‘liblwgeom’ Functions for Simple Features. R Packg. Version 0.2-5. Available online at: https://CRAN.R-project.org/package=lwgeom.
Peterson, G., Allen, C. R. A., and Holling, C. S. (1998). Ecological resilience. Biodiversity, and scale. Ecosystems 1, 6–18. doi: 10.3917/presa.005.0785
Phillips, S. J., Anderson, R. P., Dudík, M., Schapire, R. E., and Blair, M. E. (2017). Opening the black box: an open-source release of maxent. Ecography 40, 887–893. doi: 10.1111/ecog.03049
Phillips, S. J., Anderson, R. P., and Schapire, R. E. (2006). Maximum entropy modeling of species geographic distributions. Ecol. Modell. 190, 231–259. doi: 10.1016/j.ecolmodel.2005.03.026
Phillips, S. J., Dudık, M., Elith, J., Graham, C. H., Lehmann, A., Leathwick, J., et al. (2009). Sample selection bias and presence-only distribution models: implications for background and pseudo absence data. Ecol. App. 19, 181–197. doi: 10.1890/07-2153.1
Pohlert, T. (2021). PMCMRplus: Calculate Pairwise. Multiple Comparisons of Mean Rank Sums Extended. R Packg. Version 1.9.3. Available online at: https://CRAN.R-project.org/package=PMCMRplus.
R Core Team (2021). R: A Language and Environment for Statistical Computing. Vienna: R Foundation for Statistical Computing. Available online at: https://www.R-project.org/
Rabosky, A. R. D., Cox, C. L., Rabosky, D. L., Title, P. O., Holmes, I. A., Feldman, A., et al. (2016). Coral snakes predict the evolution of mimicry across New World snakes. Nat. Commun. 7:11484. doi: 10.1038/ncomms11484
Radosavljevic, A., and Anderson, R. P. (2014). Making better Maxent models of species distributions: complexity, overfitting and evaluation. J. Biogeogr. 41, 629–643. doi: 10.1111/jbi.12227
Renner, I. W., Baddeley, A., Elith, J., Fithian, W., Hastie, T., Phillips, S., et al. (2014). Point process models for presence-only analysis. Methods Ecol. Evol. 6, 366–379. doi: 10.1111/2041-210X.12352
Scanlon, A. T., Petit, S., Tuiwawa, M., and Naikatini, A. (2014). High similarity between a bat-serviced plant assemblage and that used by humans. Biol. Conserv. 174, 111–119. doi: 10.1016/j.biocon.2014.03.023
Schipper, J., Chanson, J. S., Chiozza, F., Cox, N. A., Hoffmann, M., Katariya, V., et al. (2008). The status of the world land and marine mammals. Science 322, 225–230. doi: 10.1126/science.1165115
Schnitzler, H. U., and Kalko, E. K. V. (2001). Echolocation by insect-eating bats. Am. Inst. Biol. Sci. 51, 557–569.
Schulze, M. D., Seavy, N. E., and Whitacre, D. F. (2000). A Comparison of the phyllostomid bat assemblages in undisturbed neotropical forest and in forest fragments of a slash-and-burn farming mosaic in petén, guatemala. Biotropica 32, 174–184. doi: 10.1111/j.1744-7429.2000.tb00459.x
Simberloff, D., and Dayan, T. (1991). The guild concept and the structure of ecological communities. Annu. Rev. Ecol. Syst. 22, 115–143.
Stevens, R. D., and Willig, M. R. (2000). Density compensation in new world bat communities. Oikos 89, 367–377.
Syfert, M. M., Smith, M. J., Coomes, D. A., Meagher, T. R., and Roberts, D. L. (2013). The effects of sampling bias and model complexity on the predictive performance of maxent species distribution models. PLoS One. 8:e55158. doi: 10.1371/journal.pone.0055158
Taylor, P. J., Grass, I., Alberts, A. J., Joubert, E., and Tscharntke, T. (2017). Economic value of bat predation services – a review and new estimates from macadamia orchards. Ecosyst. Serv. 30, 372–381. doi: 10.1016/j.ecoser.2017.11.015
Thiagavel, J., Santana, S. E., and Ratcliffe, J. M. (2017). Body size predicts echolocation call peak frequency better than gape height in vespertilionid bats. Sci. Rep. 7, 1–6. doi: 10.1038/s41598-017-00959-2
Tilman, D. (1999). Global environmental impacts of agricultural expansion: the need for sustainable and efficient practices. Proc. Natl. Acad. Sci. U.S.A. 96, 5995–6000. doi: 10.1073/pnas.96.11.5995
Tscharntke, T., Klein, A. M., Kruess, A., Steffan-Dewenter, I., and Thies, C. (2005). Landscape perspectives on agricultural intensification and biodiversity - ecosystem service management. Ecol. Lett. 8, 857–874. doi: 10.1111/j.1461-0248.2005.00782.x
Varela, S., Anderson, R. P., García-Valdés, R., and Fernández-González, F. (2014). Environmental filters reduce the effects of sampling bias and improve predictions of ecological niche models. Ecography 37, 1084–1091. doi: 10.1111/j.1600-0587.2013.00441.x
Vaughan, T. A. (1959). Functional morphology of three bats: Eumops, Myotis, Macrotus. Mus. Nat. Hist. 12, 1–153.
Walker, B. H. (1992). Biodiversity and ecological redundancy. Biol. Conserv. 6, 18–23. doi: 10.1016/0006-3207(93)90661-j
Wang, B. C., and Smith, T. B. (2002). Closing the seed dispersal loop. Trends Ecol. Evol. 17, 379–386. doi: 10.1016/S0169-5347(02)02541-7
Watling, J. I., Arroyo-Rodríguez, V., Pfeifer, M., Baeten, L., Banks-Leite, C., Cisneros, L. M., et al. (2020). Support for the habitat amount hypothesis from global synthesis of species density studies. Ecol. Lett. 23, 674–681. doi: 10.1111/ele.13471
Watson, J. E. M., Shanahan, D. F., Di Marco, M., Allan, J., Laurance, W. F., Sanderson, E. W., et al. (2016). Catastrophic declines in wilderness areas undermine global environment targets. Current 26, 2929–2934. doi: 10.1016/j.cub.2016.08.049
Wendeln, M. C., Runkle, J. R., and Kalko, E. K. V. (2000). Nutritional values of 14 fig species and bat feeding preferences in panama. Biotropica. 32, 489–501.
Wickham, H., Romain, F., Henry, L., and Müller, K. (2021). dplyr: A Grammar of Data Manipulation. R Packg. Version 1.0.7. Available online at: https://dplyr.tidyverse.org/.
Williams-Guillén, K., Perfecto, I., and Vandermeer, J. (2008). Bats limit insects in a neotropical agroforestry system. Science 320, 70. doi: 10.1126/science.1152944
Wisz, M. S., Hijmans, R. J., Li, J., Peterson, A. T., Graham, C. H., and Guisan, A. (2008). Effects of sample size on the performance of species distribution models. Divers. Distrib. 14, 763–773. doi: 10.1111/j.1472-4642.2008.00482.x
Yachi, S., and Loreau, M. (1999). Biodiversity and ecosystem productivity in a fluctuating environment: the insurance hypothesis. Proc. Natl. Acad. Sci. U.S.A. 96, 1463–1468. doi: 10.1073/pnas.96.4.1463
Keywords: Chiroptera, feeding guilds, functional diversity, habitat loss, species distribution models
Citation: Brasileiro LAM, Machado RB and Aguiar LMS (2022) Ecosystems Services Provided by Bats Are at Risk in Brazil. Front. Ecol. Evol. 10:852177. doi: 10.3389/fevo.2022.852177
Received: 10 January 2022; Accepted: 30 May 2022;
Published: 06 September 2022.
Edited by:
Juliano André Bogoni, University of São Paulo, BrazilReviewed by:
Carlos Ivan Espinosa, Universidad Técnica Particular de Loja, EcuadorPedro Giovâni Da Silva, Federal University of Minas Gerais, Brazil
Copyright © 2022 Brasileiro, Machado and Aguiar. This is an open-access article distributed under the terms of the Creative Commons Attribution License (CC BY). The use, distribution or reproduction in other forums is permitted, provided the original author(s) and the copyright owner(s) are credited and that the original publication in this journal is cited, in accordance with accepted academic practice. No use, distribution or reproduction is permitted which does not comply with these terms.
*Correspondence: Lais A. M. Brasileiro, bGFpc2Jpb3VmY0BnbWFpbC5jb20=
†These authors have contributed equally to this work and share first authorship