- 1Marine Science and Oceanography, Florida Atlantic University, Boca Raton, FL, United States
- 2Department of Biological Sciences, Florida Atlantic University, Boca Raton, FL, United States
Sea turtle nest success, defined as the number of eggs in a nest that successfully hatch and emerge, is closely linked to environmental conditions. Interacting biotic and abiotic factors influence hatching and hatchling emergence success. To date, combinations of multiple factors interacting together, which result in highly successful sea turtle nests are not well understood. Using 25 years of historic nest data and local expert experience, we identified five historically successful loggerhead (Caretta caretta) nesting beaches (hotspots) along the Florida (United States) Atlantic coast and measured nest environments along with the nest success. Principal component analysis was used to reduce 12 environmental variables so that the relative contributions of sand characteristics, nest temperatures, sand moisture, and nest location were considered. The nest environments differed among nesting beaches and were broadly segregated into two distinct climates: subtropical (hot and humid) and warm-temperate (warm and dry). We found that nests at subtropical sites, compared with warm-temperate sites, were characterized by environmental gradients in contrasting ways. Nest locations were predominantly mid-beach in subtropical sites but clustered at higher elevations and closer to the base of the dune at warm-temperate climate sites. Collectively, highly successful nest hotspots represent a mosaic of abiotic factors providing conditions that promote successful hatching and emergence. This new perspective on consistently successful loggerhead nesting beach traits demonstrate that the key traits of sea turtle nesting habitat vary with prevailing climate type and should be managed accordingly.
Introduction
Much of our understanding of sea turtle production is based on nest surveys and experiments done on nesting beaches. Focus on nesting beaches is largely due to the ease of access to nesting females, incubating eggs, and the nests; these studies contribute to sea turtle population growth and stability (Fuentes et al., 2013; Butt et al., 2016). While various sensitivity analyses highlight the importance of survival of later life stages found at sea (Crouse et al., 1987; Crowder et al., 1994), life history stages are necessarily linked (Congdon et al., 1993; Heppell, 1997). Therefore, the deterioration of nesting habitat due to coastal development, erosion, and climate change poses a prominent threat to sea turtle populations (Mazaris et al., 2009; Rizkalla and Savage, 2011; Witherington et al., 2011; Lyons et al., 2020). Assessments of nesting beaches frequently focus on the factors that lead to nest failure (National Marine Fisheries Service and U.S. Fish and Wildlife Service, 2008) while not considering the multiple factors that together define successful nests.
The distributions of successful sea turtle nests are affected by several abiotic and biotic factors acting on different spatial scales. Abiotic factors include large-scale (regional) climates (i.e., subtropical, warm-temperate) to local-scale variables such as beach morphology and sand characteristics. At regional-scales, temperature and precipitation are the main determinants driving the distribution and temporal window of suitable nesting habitats (Pike, 2007, 2013). For example, sea turtle embryonic development is thermally constrained to 24–35°C (Miller, 1985).
Whereas regional-scale factors are important drivers of nest success, their effects may be mediated locally. On local scales, the nest microclimate varies with clutch sizes, nest location, sand characteristics, temperature, and available moisture. Precipitation can mitigate the effects of extreme warm temperatures by evaporative cooling of the nest and eggs (Tezak et al., 2018; Lolavar and Wyneken, 2021). These factors may be critical because they affect the likelihood that the nest is inundated by seawater or exposed to extreme temperatures (Horrocks and Scott, 1991; Zare et al., 2012; McElroy et al., 2015), as well as gas exchange and metabolic processes of developing embryos (Ackerman, 1997). For example, the metabolic heat produced by developing embryos increases during the final third of incubation and contributes to overall clutch temperature, typically above adjacent sand temperatures at an equivalent depth (Godfrey et al., 1997; Broderick et al., 2001). Nests with larger clutches establish greater thermal flux between sand and the egg chamber as metabolic heat increases (Ackerman, 1980). Sand characteristics, particularly bulk density, reflect the percentage of air in a known volume of sand, which, in turn, affects the gas exchange processes of the nest (Ackerman, 1997). Generally, eggs laid in sands with higher bulk density may suffocate the developing embryos or, if the embryos complete development, impede the hatchlings’ emergence (McGehee, 1990; Mortimer, 1990; Ackerman, 1997). Nest location along the landward-seaward axis ultimately may determine whether eggs incubate in a suitable environment. A nest’s elevation must be high enough to prevent it from being inundated by high tides, ground water, wave runup, or eroded (Katselidis et al., 2013). However, high-elevation nests may also experience higher temperatures (Ackerman, 1997; Wood and Bjorndal, 2000; Matsuzawa et al., 2002; Zárate et al., 2013). Thus, nest success is not dependent upon a single environmental factor but rather a combination of interacting regional- and local-scale factors.
Florida’s sea turtle nesting beaches extend along much of the peninsula’s Atlantic coast and to a smaller extent along the southwest coast and panhandle. Most loggerhead turtle (Caretta caretta) nesting activity in the United States occurs in the southeastern coastal region with ∼90% of the Northwest Atlantic subpopulation nesting in Florida (Dodd, 1988; National Marine Fisheries Service and U.S. Fish and Wildlife Service, 2008; Casale and Tucker, 2017). Florida beaches provide critically important nesting habitat for loggerheads, yet that habitat is being compromised by “coastal squeeze” associated with urban development, alongside the encroachment of rising seas which further reduces available nesting habitat (Mazaris et al., 2009). Beach modifications to protect beachfront property via coastal armoring (e.g., seawalls and revetments) may impede beach access for nesting females. The installation of seawalls may also change beach morphology making the nesting areas less suitable with lower profiles and more frequent inundation (Basco, 2006; Ells and Murray, 2012). Within this context, protecting and maintaining high quality nesting habitat is imperative (National Marine Fisheries Service and U.S. Fish and Wildlife Service, 2008). To address this goal, essential first steps must include both identifying areas of high nest success and the regional- and local-scale environmental factors associated with that success.
Multiple studies assessed the role of the nest environment on sea turtle embryo mortality (e.g., Foley et al., 2006; Awong-Taylor et al., 2008; Camargo et al., 2020). However, information regarding the environment of highly successful nests is sparse, and typically restricted to just a few factors. Here we identify factors that define highly successful loggerhead nesting habitats and address how loggerhead nest success is distributed across nesting beaches along Florida’s Atlantic Coast. We noted diversity in regional- and local-scale variables most strongly correlated with successful nests and compared successful nest environments among nesting beaches. The study described here draws approaches from ecological developmental biology and beach geomorphology. To enhance clarity, we provide a glossary to the terms that may be specific to one field or the other (Supplementary Table 1). We discuss multiple biotic and abiotic factors acting simultaneously with regional- and local-scale and how they contribute to the success of loggerhead clutches.
Materials and Methods
Historical Nest Success
Five highly productive loggerhead nesting beaches were selected based on their consistent long-term sea turtle nest success and beach data (Figure 1). Red Reef Park, Boca Raton (RR), Juno Beach (JB), Hutchinson Island (HI), Archie Carr National Wildlife Refuge (AC), and Canaveral National Seashore (CC) span more than 2 degrees in latitude (Figure 1). Together, these nesting beaches span approximately 100 km of contiguous Florida loggerhead nesting habitat. The latitudinal breadth crosses the bioclimatic transition zone between subtropical and warm temperate climates (28°00′N). We designate the southeast sites (RR, JB, HI) as in the “subtropical” region and the central east coast sites (AC, CC) as in the “warm-temperate” region, based on relative geographic positions and climatic regions.
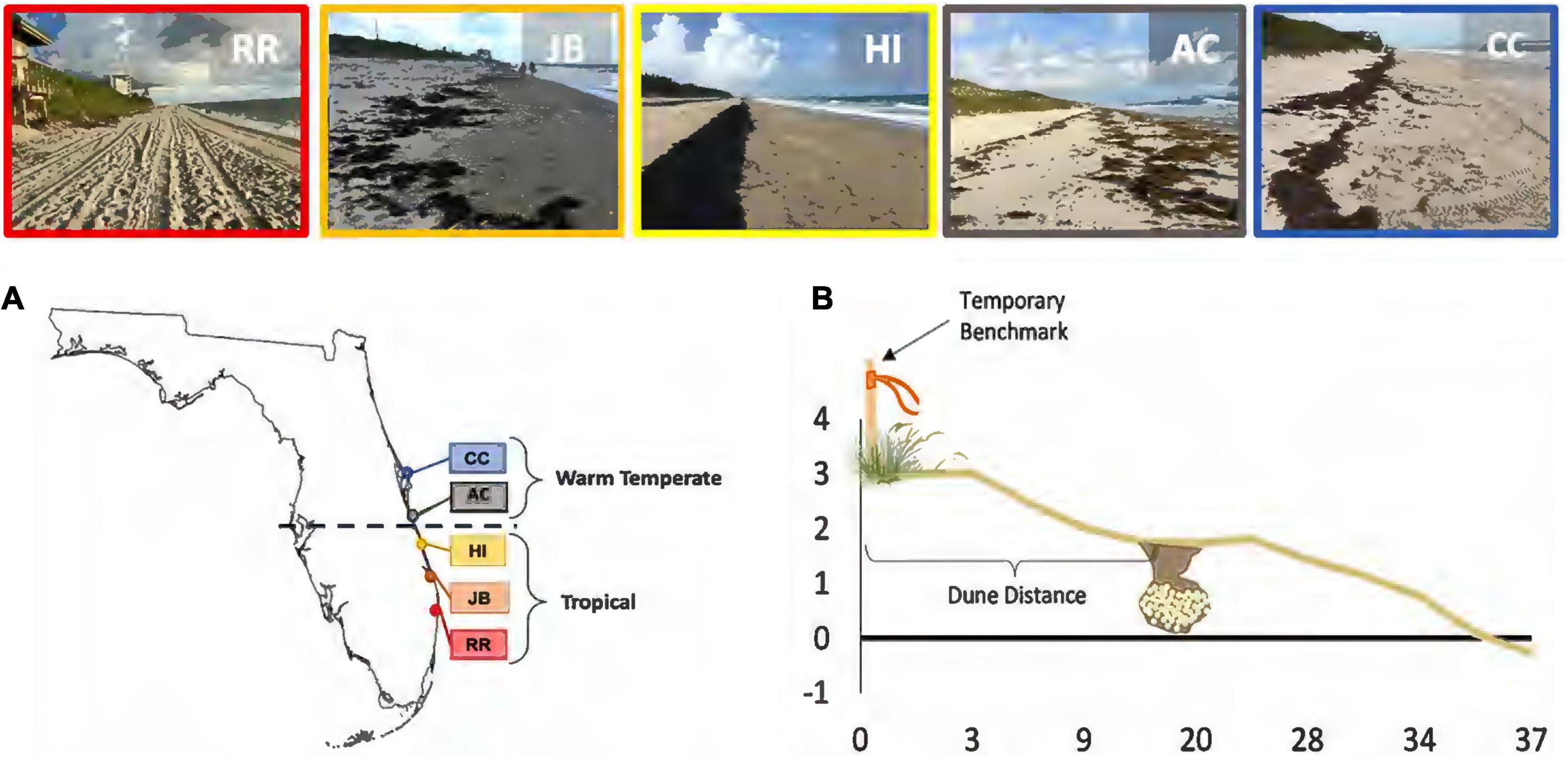
Figure 1. Site map with nesting habitat illustrated for each beach. (A) Study beaches by location relative to the bioclimatic break shown by dashed line. (B) Diagram profiling a nesting beach with a clutch located at the middle beach. The temporary benchmark was used to establish beach morphology transects. Red Reef Park, Boca Raton (RR), Juno Beach (JB), Hutchinson Island (HI), Archie Carr National Wildlife Refuge (AC), and Canaveral National Seashore (CC).
Previous studies indicated that individual loggerhead females are capable of nesting for a minimum of 25 years (National Marine Fisheries Service and U.S. Fish and Wildlife Service, 2008), therefore we analyzed 25 years of nest locations and post-emergence nest inventory data spanning 1994–2019. We obtained the study sites’ systematic historical data from data owners that follow the Florida’s Index Nesting Beach Survey and Statewide Nesting Beach Survey programs (Florida Fish and Wildlife Conservation Commission, 2016) for all but one site. The most productive nesting beach areas in AC were identified by the long-term sea turtle survey staff (C. Long, personal communication).
Surveyors verify and mark each clutch location, then record its location with either GPS coordinates or zone and physical landmarks. For nests without latitude and longitude data, we approximated locations by randomly generating a point within the spatial boundary of the zone noted by the surveyor. All historical data were evaluated for potential inconsistencies prior to analyses. We excluded data that were incomplete or manipulated in at least one of the following ways: (1) the nest was not located at the time of inventory; (2) surveyors gave the nest special experimental treatment; (3) a storm event destroyed the nest; (4) the clutch was relocated; (5) nest inventory data had gaps for certain years (i.e., loggerhead nests were not evaluated, only nest counts were recorded). The “imputeTS” package in R estimated missing data using a weighted moving average (Moritz and Bartz-Beielstein, 2017).
To reconstruct hatching and emergence success time series and to define the threshold values of “highly successful” hatching and emergence rates, we used a locally weighted scatterplot smoother (Cleveland et al., 1990). Mean monthly hatching and emergence success was modeled as a function of year. We determined the threshold values of highly successful nests as being greater than or equal to the moving average produced by the model (Table 1). To identify hotspots for highly successful nests across years, we analyzed historical data with the heatmap plugin for QGIS version 3.10 (QGIS Development Team, 2019). “Heatmap” generates a density raster through kernel density estimation from sea turtle nest positions. All 25 years of nesting data were represented as a single input layer to generate the overall trend. Nests with success rates lower than the moving average of its respective site were excluded, ensuring that only highly successful nests were plotted on the heatmap.
Nest Parameter Surveys
To characterize the nest environment at these historically successful sites, we measured several biotic and abiotic parameters in 10 nests at each of the five study sites during the 2020 nesting season (N = 50 nests). Sampling spanned June–August 2020 when most loggerhead turtle nests were incubating. Clutch temperatures were recorded using HOBO-U22 temperature dataloggers (accuracy ± 0.2°C, resolution ± 0.02°C per manufacturer’s specification and verified empirically; Onset Computer Corp., Bourne, Massachusetts, United States). The dataloggers were placed in the middle of the clutch with minimal disturbance by gently excavating the sand from the top of the clutch, removing approximately 40–50 eggs within a few hours of deposition, protecting the eggs from heat and desiccation while inserting the datalogger, then replacing the eggs in the order they were removed, followed by replacing the sand (after Lolavar and Wyneken, 2015). All dataloggers were placed within 12 h of oviposition to maintain embryonic viability while handling the eggs (Limpus et al., 1985; Rafferty et al., 2013). Nest temperatures were recorded every 15 min and the dataloggers were retrieved at nest inventory. To account for the metabolic heat produced by developing embryos, a control datalogger was buried at each site at a depth (∼45 cm) representing the approximate middle of a loggerhead egg chamber (Maloney et al., 1990; Matsuzawa et al., 2002; Lolavar and Wyneken, 2015). The control datalogger for each site was buried within 2–3 m of a study nest at the same approximate beach elevation. The sand site was selected randomly among marked nests early in the sampling period without a nearby nest. Daily mean sand or clutch temperatures and maximum temperatures were calculated for the incubation period for all nests and controls.
Depending on site-specific and nest-specific protocols, nests were excavated and inventoried either the day of first major hatchling emergence or 3 days after first emergence. If there was no sign of emergence, nests were excavated 70 days after oviposition per Florida Fish and Wildlife Conservation Commission (2016). Clutch inventory included the number of hatched eggshells, dead and live hatchlings in nest, and the number of unhatched eggs.
Hatching (HS) and emergence success (ES) were determined using the following formulas:
S is the number of empty eggshells that are > 50% intact, Ci is the total number of eggs in the nest, and R is the number of hatchlings remaining in the nest at inventory (Miller, 1999). We also recorded the depth of the top egg and bottom of the egg chamber for chamber dimensions, and landward distance from the nest to the dune vegetation line or seawall.
Sand Characteristics
To analyze the sediment surrounding the egg chamber, ∼350–500 g of subsurface sand were collected by hand from a hand-dug 45 cm hole along the periphery of each nest but not breaching the egg chamber. Replicate samples (3 replicates/nest × 10 nests × 5 sites N = 150 total samples) were collected at the beginning, middle, and end of incubation.
The field water content (θ) was determined gravimetrically by weighing a sample before and after drying in a 52°C oven for a minimum of 24 h (after Gardner, 1986).
Particle density, bulk density, and sand porosity are factors that indicate the density of solid constituents, mass of material contained within a given volume, and amount of pore space contained in a sample, respectively (Mota, 2009). To calculate particle density, 40 g of dried sand was placed in a 100 mL graduated cylinder with 50 mL of deionized water (diH2O). After stirring to displace air, the water meniscus volume was recorded. This volume, minus the 50 mL initial water volume, equals the volume of sand solids. Particle density (g. cm–3) was calculated by dividing the weight of dry solid particles by the volume of sand solid particles.
Bulk density is the mass of soil per unit volume (= bulk soil volume; Tan, 1995) and was measured as the mass of sand required to fill a graduated cylinder up to 100 mL (= cm3).
Sand porosity is defined as percentage of sand volume occupied by pore spaces and was calculated as bulk density/particle density (Tan, 1995) converted to a percentage using the following formula.
The sand salinity was measured using a handheld conductivity meter (model HI98192, accuracy: ± 0.01 mS/cm, resolution: 0.001 mS/cm, Hanna Instruments, Woonsocket, Rhode Island, United States). To measure sand salinity, 40 g of dried sand was mixed with 200 mL of diH2O. The electrical conductivity of the sand solution, in microsiemens was converted to ppt (after Bennett et al., 1995; Foley et al., 2006).
Sand grain size distribution (i.e., size and sorting) of each sample was measured using a mechanical sediment shaker (RX-29 Ro-Tap, accuracy: ± 0.5-micron, resolution: ± 0.01 micron, W.S. Tyler, Mentor, Ohio) at half-phi sieve intervals between −4 and 2 φ and quarter-phi sieve intervals between 2 and 4 φ.
Percent calcium carbonate (%CaCO3) was determined as the change in mass of a sample measured before and after mixing with 4N hydrochloric acid (HCl) and air-drying for a minimum of 24 h.
Sand color was determined using a Munsell soil color book. Color charts were placed over the sand sample under a fluorescent lamp at a fixed distance and the corresponding chroma and hue were recorded.
Beach Morphology
The spatiotemporal variations in beach morphology were characterized by time series beach profiles in zones with historically high hatching and emergence success hotspots. Temporary benchmarks (wooden stakes in the dune field for the duration of the study, Figure 1B) were established for each site using a Real-Time Kinematic Global Positioning System (RTK-GPS, accuracy ± 1.0 cm, resolution ± 0.01 cm; Precision Agriculture, Boston, Massachusetts, United States). Each benchmark allowed for subsequent beach profile surveys to locate the same transect line for temporal comparisons provided an elevation control for the entire profile transect. Beach profiles were measured in April, June, and September 2020 and plotted as cross-sectional profiles to identify morphologic features including dune toe, shoreline position (0 m elevation), dry beach (from dune toe to shoreline), and foreshore (from shoreline to the waterline). To account for cross-shore variability, slope estimates were calculated: (i) between the dune toe and shoreline at the three sampling times. Beach width was calculated as the distance between the dune toe and waterline.
Data Analyses
All statistical analyses were performed in R Studio ver. 3.5.1 (R Core Team, 2018) and MS Excel version 16.46. The Shapiro-Wilkes (for normality) and Levene’s tests (for equality of variances) were used to check sand characteristics, temperature, and beach morphology, hatching and emergence success, and clutch sizes data. A nested ANOVA tested for differences in hatching and emergence success among study locations. Region (subtropical, warm-temperate) was treated as a fixed effect, site nested within region was classified as a random effect. Due to the large number of environmental factors and the potential for high spatial autocorrelation (Griffith, 2011), a principal components analysis (PCA) was used for variables related to: (i) nest location and egg chamber dimensions, and (ii) nest microclimate (sand grain size, sorting, porosity, temperature, sand moisture, calcium carbonate content). The PCA reduced the dimensionality and collinearity of the standardized predictor variables collected in the field. The scores of the first two principal components were used as predictor variables in generalized linear mixed models (GLMM) of hatching and emergence success. Multiple models were fit using all possible combinations of PC scores and their interactions. Candidate models were compared using delta AIC scores, with a delta AIC threshold of 4 for model selection (Burnham and Anderson, 2002).
Bioethics
This study did not involve live vertebrates; no IACUC authorizations was needed. The study adhered to guidelines in the FWC Sea Turtle Conservation Handbook (Florida Fish and Wildlife Conservation Commission, 2016).
Results
Historical Nest Success
Hatching and emergence success of 13,407 loggerhead nests from 1994 to 2019 were analyzed for RR, JB, HI, and CC nesting beaches (Table 1). Using the kernel density estimates of highly successful nests, a minimum of two hotspots were identified within each study site (Figures 2A–E). The surface area and number of the hotspots varied among the study beaches. Although overall hatching and emergence success values fluctuated from year to year, hotspot locations were relatively consistent. Data for AC were not shared for site selection; instead, staff with no less than a decade of experience conducting nest inventory at the site and working knowledge of the multidecadal nest inventory data provided landmarks for highly successful nest areas. Two hotspots within the historically productive areas of each nesting beach were selected for 2020 field measurements of nest sites and clutches.
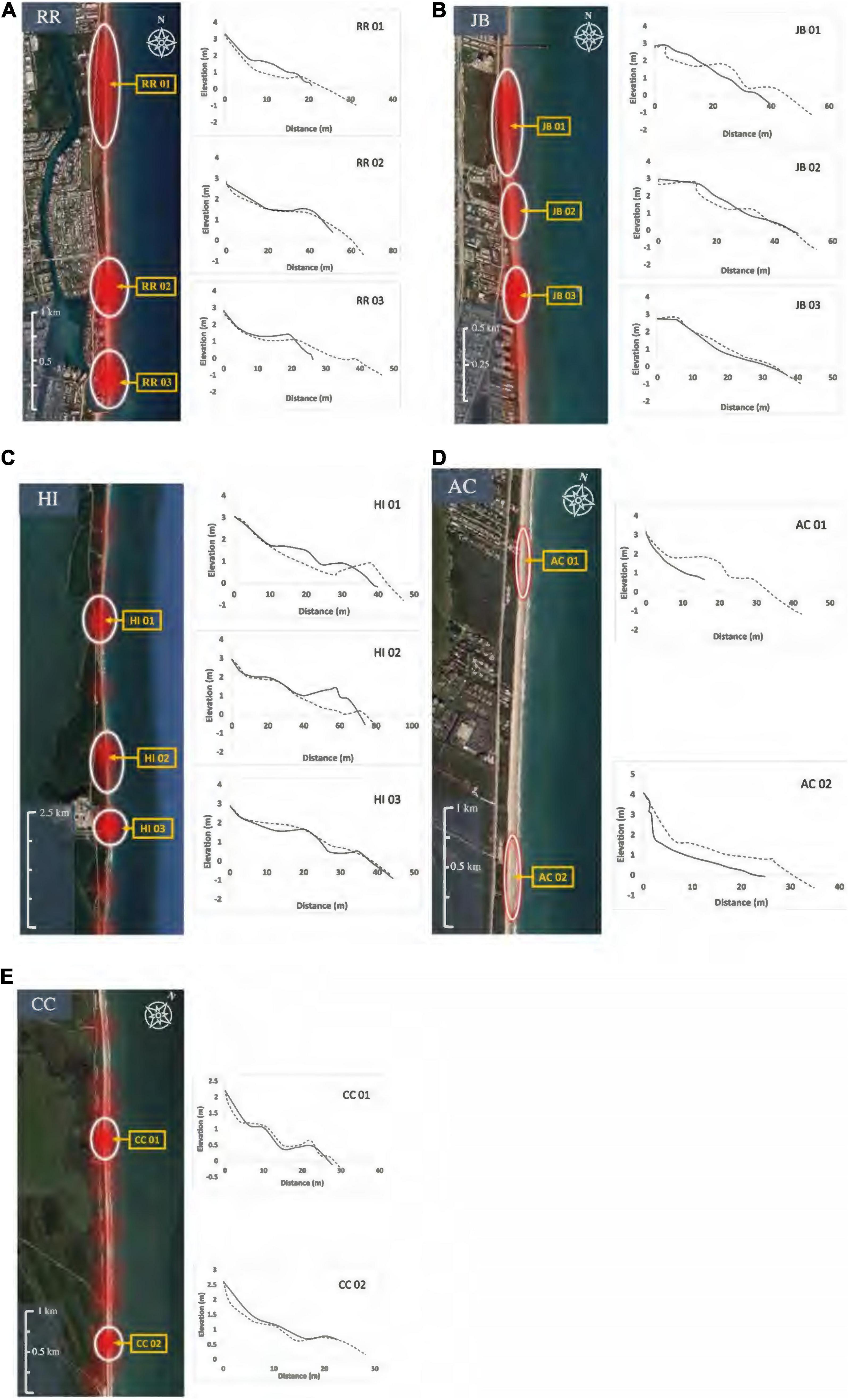
Figure 2. (A–E) Heatmaps (QGIS 3.11) for highly successful loggerhead nesting areas. Hotspots are shown for Red Reef (RR), Juno Beach (JB), Hutchinson Island (HI), and Cape Canaveral (CC). Archie Carr National Wildlife Refuge (AC) hotspots were identified qualitatively through personal communication with long-term inventory staff. Loggerhead nests and sand were examined at two hotspots for each site. Transect locations for beach morphology measures are indicated with yellow arrows. Beach profiles corresponding with each transect are displayed to the right of each heatmap (June 2020 profiles—dashed line, September 2020 profile—solid line).
2020 Nesting Season’s Sand and Clutch Characteristics
Of the 50 clutches instrumented, measured, and inventoried, all were instrumented between 3 June and 15 August 2020 and inventoried between 28 July and 14 September 2020. All study nests incubated during the time period when most loggerhead turtle nests in the study region were incubating and hatching. Two major storm events occurred during the study: Hurricane Isaias (30 July to 5 August 2020) and Hurricane Laura (16–27 August 2020). During Hurricane Isaias, two nests at JB, one at AC, and one at CC experienced wave washover and inundation. At HI, two nests were severely depredated. These 6 nests were omitted from analyses. Hatching and emergence success summary statistics for the 5 sites is in Table 2. Neither hatching success nor emergence success differed among sites (Supplementary Table 2).
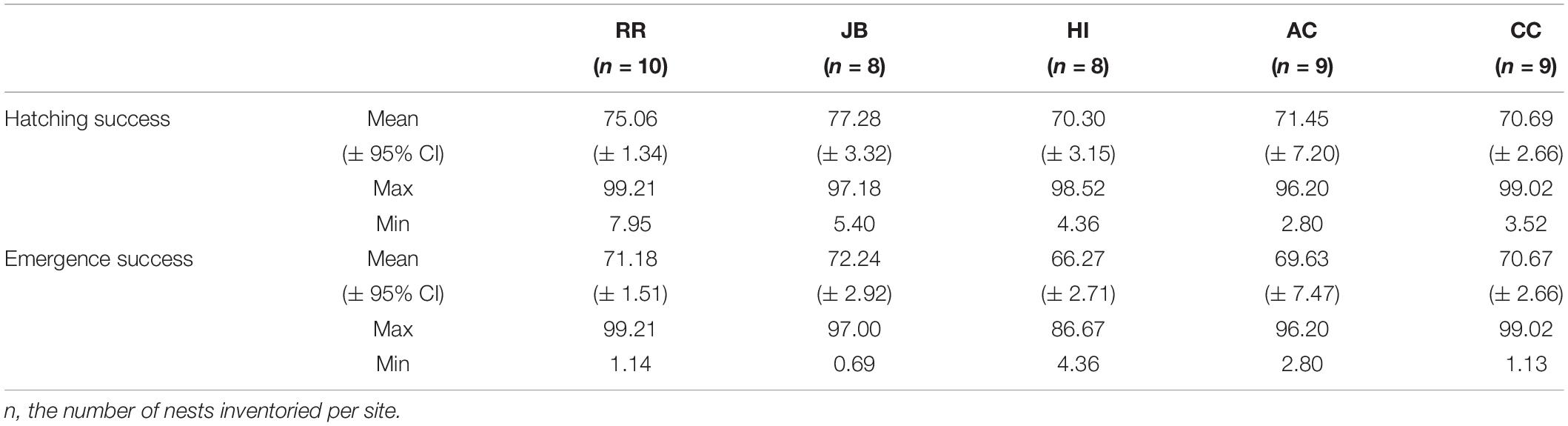
Table 2. Descriptive statistics for hatching and emergence success at each nesting beach during the 2020 study period.
Details the local nest environments (clutch temperatures, sand temperatures, sand water content), beach morphology (beach slope, beach width), clutch characteristics (clutch size, top depth, bottom depth), nest location (dune distance), and sand characteristics (size, sorting, bulk density, particle density, porosity, CaCO3 content, salinity, color) are aggregated in Supplementary Table 3. Sand color varied relatively little among sites yet subtropical sites were warmer and had slightly darker sand than the warm-temperate sites.
Climate
Climatic variation spanned the study sites; those south of the climatic break (subtropical; BR, JB, HI) are characterized by hot, humid conditions while those north (AC, CC) have a drier and warm-temperate climate (Figure 1). Of the 44 nests analyzed, 60.5% were in the subtropical climate; the remainder were in the warm-temperate climate. Throughout the 2020 study period, clutches were warmer than the adjacent sand without eggs (Table 3). Daily mean sand temperatures of warm-temperate sites rarely exceeded 32°C, while subtropical regions often ranged from 32 to 35°C (Figure 3). Of the 44 analyzed clutches, 22 met or exceeded mean daily nest temperatures above 34°C and 12 of these nests experienced temperatures above 35°C. Generally, temperature profiles of the clutches were similar to those of each site’s sand control during the first third of incubation. Some warming due to metabolic heating was evident by days 19–27 of incubation (Figure 4). During the last third of incubation when embryos are growing, metabolic heat generation is substantial (Hendrickson, 1958; Carr and Hirth, 1961) and clutch temperature surpasses the surrounding sand (Figure 4).
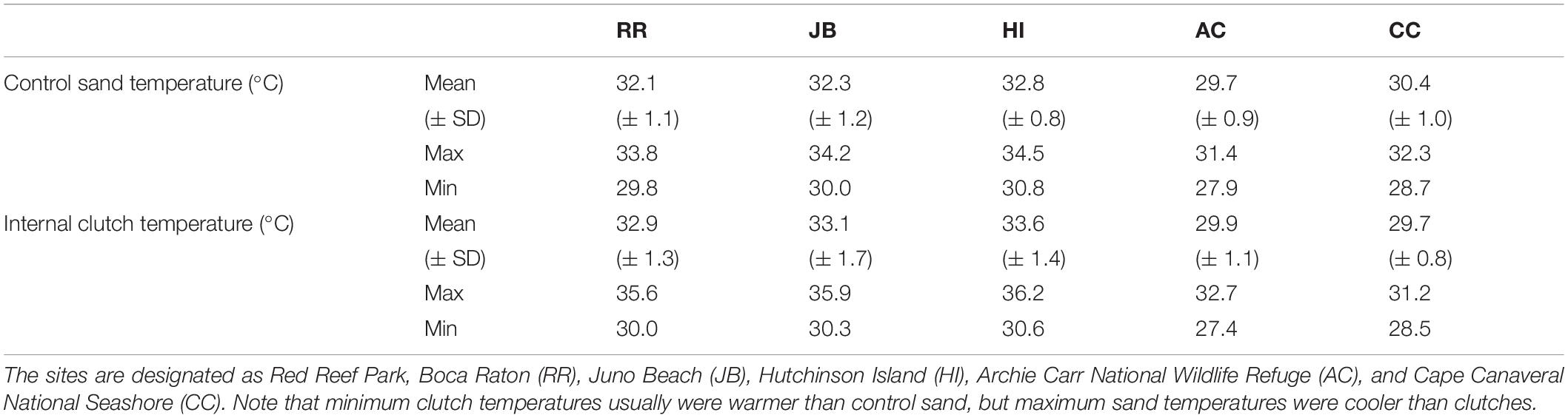
Table 3. Mean (± SD), minimum and maximum daily clutch temperatures and adjacent (control) sand temperature at 45 cm deep for the five study beaches during the 2020 nesting season.
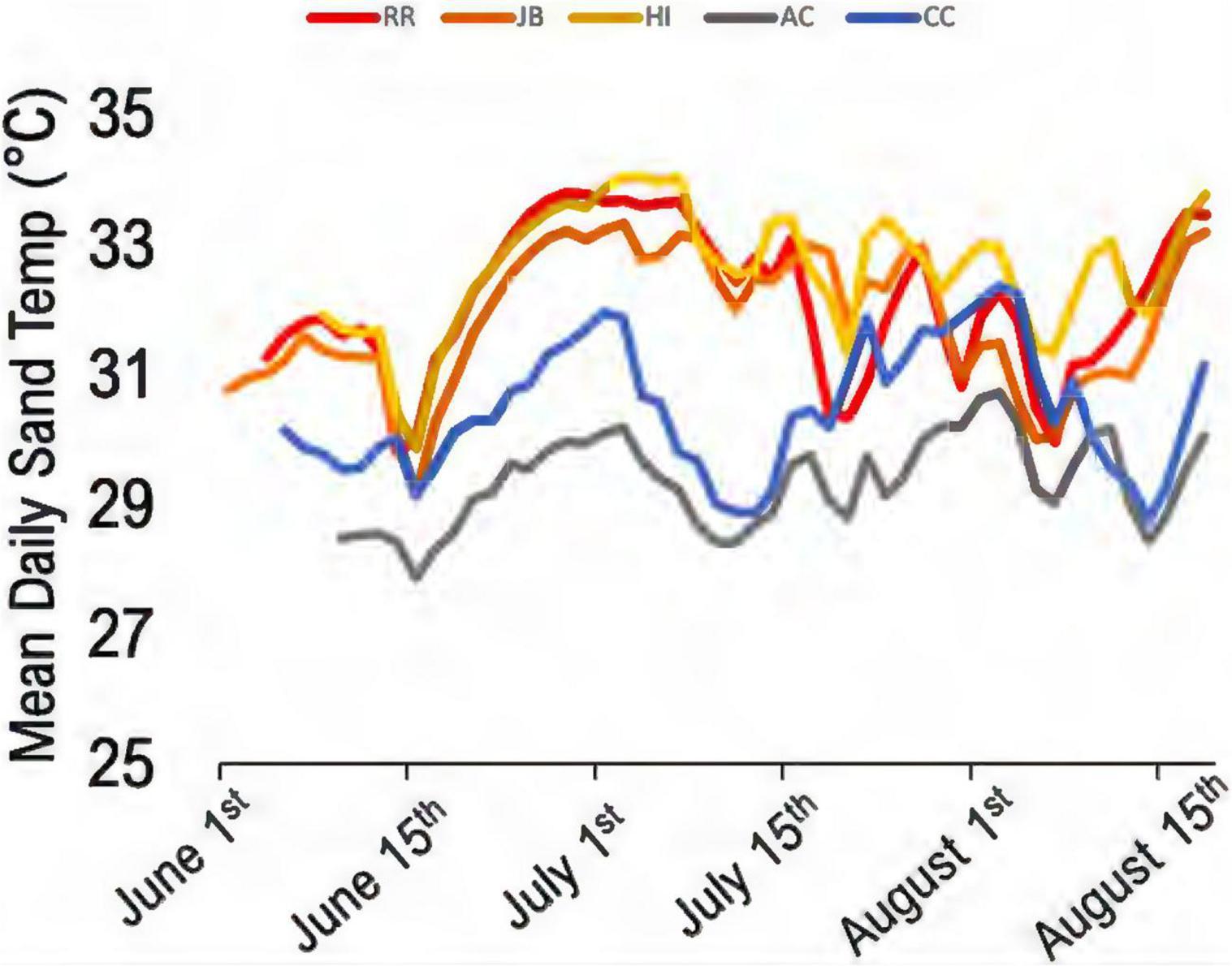
Figure 3. Mean daily sand temperature profiles from 2020 control dataloggers. Gray and blue lines are north of the bioclimatic break in the warm-temperate regions. Red, orange, and yellow lines are south of the break (subtropical region) (n = 112 days).
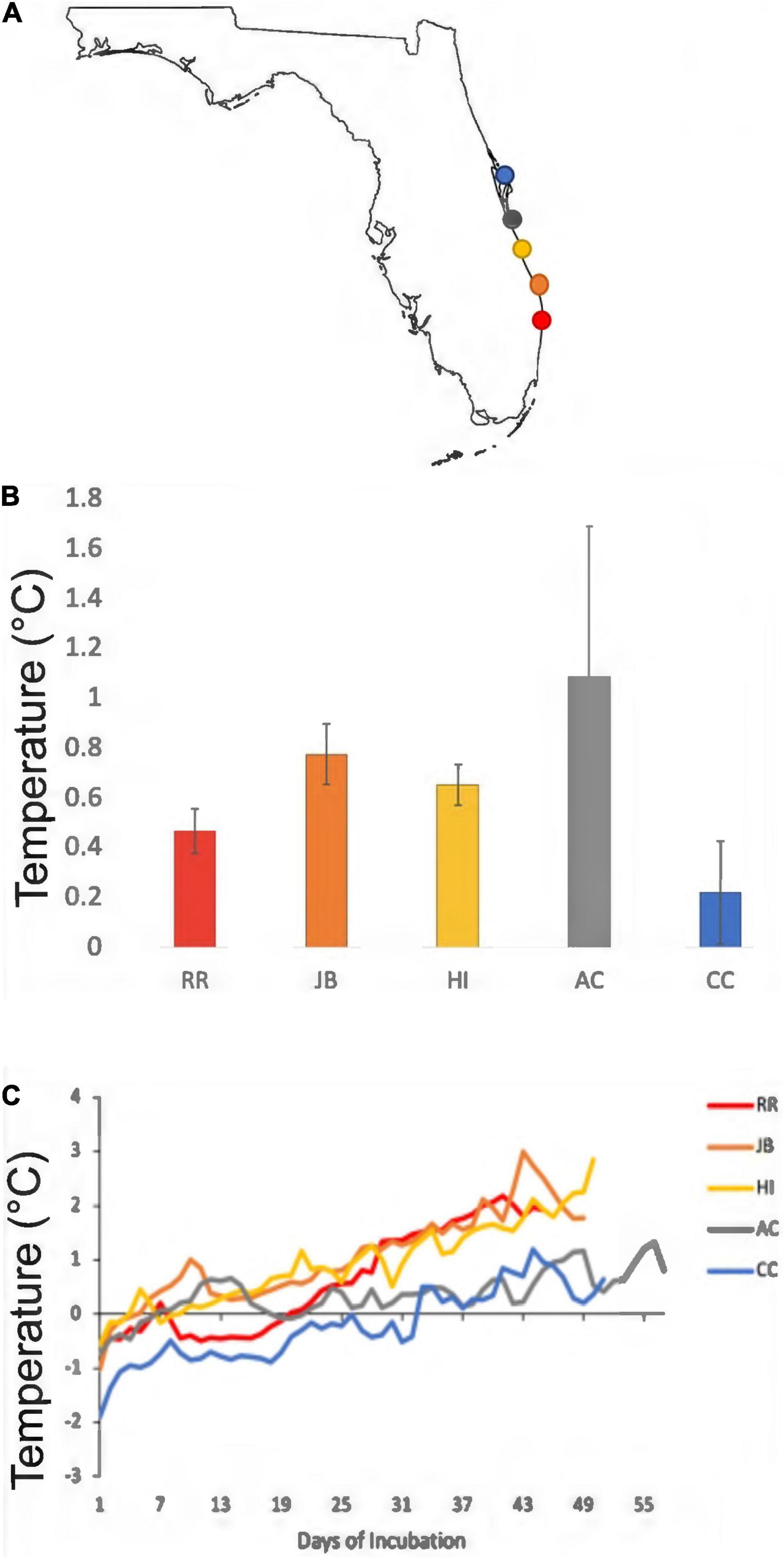
Figure 4. Mean daily differences between internal clutch temperatures and control sand temperatures from the 2020 nesting season. (A) Gray and blue colors are the warm-temperate sites. Red, orange, and yellow colors are the subtropical sites. The colors are the same in (B,C). (B) The mean temperature differentials between internal nest and control sand for each study site. Error bars represent 95% confidence intervals. (C) Differences between daily mean clutch and sand temperature throughout incubation durations (days). Note how nest temperatures exceed surrounding sand temperatures due to metabolic heating, and how metabolic heating is more pronounced in subtropical nests compared to warm-temperate nests.
Sand moisture content differed between climates (X2 = 3.23, p = 0.001); subtropical nests had a higher average sand-water content (0.04 ± 0.004 θ) than warm-temperate nests (0.03 ± 0.0009 θ).
Beach Morphology
The nesting beaches differed significantly in the physical beach characteristics. Warm-temperate sites were significantly narrower (Mann-Whitney U = 9.96, n = 12, P = 0.008) and had steeper beach slopes (Table 4) than the more southern subtropical sites. Overall beach slope was generally steeper in warm-temperate beaches than in subtropical beaches. The steepest slope was observed at AC along both hotspot transects during the late nesting season. In the warm-temperate region, beach slopes steepened June–September and slopes were more stable in the subtropical region (Table 4).
Egg Chamber Dimensions, Locations, and Clutch Sizes
Egg chamber dimensions differed between the regions. The mean nest depth of HI (top: 30.87 ± 8.11 cm, bottom: 52 ± 4.78 cm) was significantly shallower than JB (top: 44.35 ± 9.62 cm, bottom: 63.07 ± 4.28 cm) and CC (top: 46.89 ± 7.44 cm, bottom: 59.57 ± 11.2 cm; Kruskal-Wallis: 12.08, P = 0.02). Clutch size also varied among sites (Kruskal-Wallis: 13.55, P = 0.008). AC clutches (89.28 ± 10.59 eggs) were significantly smaller than RR (119.5 ± 33.58 eggs) and JB (117.1 ± 21.63). The distance between the nests and the dune toe was significantly greater at the subtropical sites (Mann-Whitney U = 10.36, n = 43, P = 0.015) so the nests were often in the middle of the open beach. In contrast, warm-temperate site nests tended to be clustered near the dune toe.
Environments of Highly Successful Nests
The first two principal components explained 96.2% of the variation in the loggerhead nest physical characteristics. The first component, PC1 (80.7% variance), represented nest microclimate, as it described an axis of variation in temperature, sand water content (moisture), bulk density, porosity, and CaCO3 content. PC2 (15.5% variance), represented egg chamber characteristics, egg chamber depth, and clutch size. The biplots (Figures 5A–C) indicated the spread of successful nest characteristics into four quadrants. Nests ordered in quadrant I are large clutches, placed in loosely packed, moist sand with high carbonate content, and high clutch temperatures (negative PC1 coefficients and positive PC2 coefficients). Quadrant II nests have large clutches, placed deeply in densely packed sand, with low carbonate content, low moisture, and lower clutch temperatures (positive coefficients on PCs 1 and 2). Quadrant III describes small, shallowly placed clutches in loosely packed sand with high carbonate content and warm temperatures (negative coefficients on PCs 1 and 2). Quadrant IV nests are small, shallowly placed clutches in densely packed sand, with low carbonate content, low sand moisture, and lower nest temperatures (positive PC1 coefficients and negative PC2 coefficients). The nests grouped into two distinct environmental regimes with minimal overlap: the subtropical sites (RR, JB, and HI) in quadrants I and III, and the warm-temperate sites (AC and CC) in quadrants II and IV.
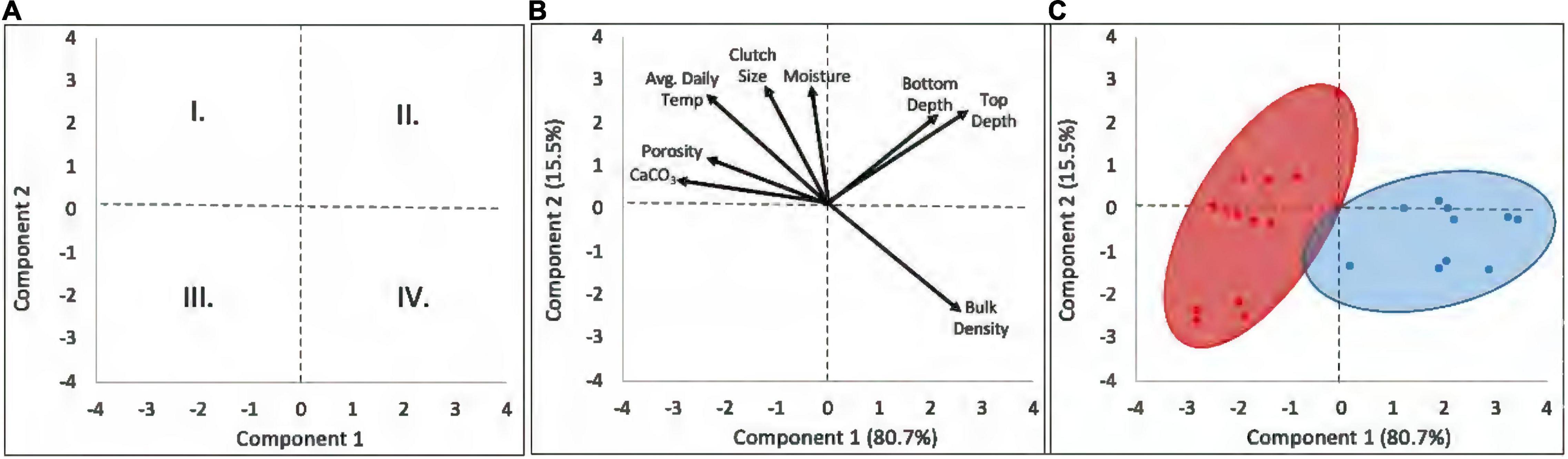
Figure 5. The biplots indicated the spread of successful nest characteristics into four quadrants. (A) Quadrant I represents large clutches, placed in loosely packed, moist sand with high carbonate content, and high clutch temperatures (negative PC1 coefficients and positive PC2 coefficients). Quadrant II represents large clutches, placed deeply, in densely packed sand, low carbonate content, low moisture, and lower clutch temperatures (positive coefficients on PCs 1 and 2). Quadrant III describes small clutches, located shallowly, loosely packed sand, high carbonate content, warm temperatures (negative coefficients on PCs 1 and 2). Quadrant IV represents small clutches, placed shallowly, densely packed sand, low carbonate content, low sand moisture, and lower nest temperatures (positive PC1 coefficients and negative PC2 coefficients). (B,C) Note that the subtropical sites (red) were clustered in quadrants I and III (negative PC1), and the warm-temperate sites (blue) clustered in quadrants II and IV (positive PC1). See text for further discussion.
The model that explained the greatest variation in hatching and emergence success was a PC1 and PC2 interaction term (Supplementary Tables 3, 4). The interaction between the principal components in the model indicates that the effects of nest microclimate and egg chamber dimensions are interdependent. At lower values of PC1, the higher values of PC2 (meaning deep, large clutches closer to the waterline) have a more important effect on hatching and emergence success.
Discussion
Together, the interactions between nest microclimate and egg chamber characteristics highlight the diversity of environments in which loggerheads can successfully nest. The connections between sand and egg chamber characteristics are illustrated in the PCA analysis. Highly successful subtropical nests showed evidence that nest microclimate is strongly associated with success in this region. Key interactions were high calcium carbonate content, low bulk density along with high porosity (that allowed for high moisture to percolate through and still provide for robust gas exchange). The large variation in subtropical egg chamber characteristics supports previous evidence suggesting that nest depth is less likely to influence clutch temperatures in nesting areas that are closer to the equator (Van De Merwe et al., 2006). The highly successful warm-temperate nests were shallow with smaller clutches (e.g., restricted to negative PC2 values) which may reflect a different set of incubation compromises. Temperature differentials between the nest and surrounding sand differed between the warm-temperate sites, suggesting that warm-temperate nests not only warm differently, but the embryos of this subpopulation may differ on physiological and developmental levels.
For sea turtles, population recruitment occurs over a large spatial scope. Consequently, understanding the factors that lead to successful hatching and emergence can enable us to better direct conservation efforts (Mazor et al., 2013). Through a reconstructed time-series of loggerhead hatching and emergence success spanning 25 years (roughly a generation), we identified nesting beach sites that were consistently productive along Florida’s east coast. Average hatching and emergence success in 2020 were considered as high relative to historical moving averages. Despite high variability in incubation environments among sites and climatic regions, hatching and emergence success were similar indicating that loggerhead eggs are resilient to diverse beach, sand, and nest environmental conditions (thermal, moisture, and porosity/potential for gas exchange) across time and space. The study spanned a subpopulation within the loggerhead turtle peninsular Florida Recovery Unit.
While hatching and emergence success can be highly variable on both spatial and temporal scales (Brost et al., 2015), distinct hotspots of high success were identified at each of the five nesting beaches and the hotspot locations remained relatively stable across the 25 years of historical loggerhead nesting, indicating that nests are consistently more successful in certain locations in any given year. The apparent consistency of hotspot locations aligns with previous studies on loggerhead nesting in Florida (Weishampel et al., 2003), which showed that nest distributions were non-random across east-central beach sites. However, here we identified patch distributions of nests on multiple beaches. These patch distribution patterns suggest that the hotspots identified in this study have characteristics that are important in loggerhead nest site selection, yet the generalities differed between the management units and between the bioclimatic regions. Although this study did not manipulate specific environmental factors and did not track the nesting behavior of individual turtles, the approach illustrates the suites of environmental factors that together were associated with high nest success. Because hatching and emergence success are necessarily linked, the study results indicate that highly variable environments can allow for both complete development and the hatchlings’ competence to escape the nest chamber.
This study allows for informed inferences about the characteristics of highly successful nests. In particular, success was not constrained within a narrow window of environmental conditions. There were variations in sand characteristics, nest locations, and egg chamber dimensions within and across climatic regions. This environmental variation is not surprising, as nesting beaches are dynamic environments that may change in width and slope within and among seasons. Individual nesting turtles spread their reproductive effort (as multiple clutches) over time and space within seasons and across years, via multi-year remigration intervals (Carthy et al., 2003; Schroeder et al., 2003). These life history traits, along with the wide latitudinal breadth of loggerhead nesting assemblages, suggest that the species has evolved to successfully nest under diverse combinations of conditions. Previous studies documented how critical tolerance limits nest success and can vary among sea turtle species, incubation scenarios, and populations (Drake and Spotila, 2002; Howard et al., 2014; Montero et al., 2018; Gatto et al., 2021).
The value of this evaluation of highly productive nesting sites identifies important locations used by southeastern management unit loggerheads (RR, JB, HI) and AC and CC provide similar information for the central eastern management unit. At a regional scale, there is a bioclimatic break at 28°N latitude (Troast et al., 2020). This climatic boundary is located between the HI and AC sites and is defined by large-scale temperature and precipitation regimes. Superimposed upon this bioclimatic separation are subtle but identifiable genetic differences. Shamblin et al. (2011) found genetic partitioning of the Peninsular Florida Recovery Unit into six regions that are treated as management units. Two of the management units: Southeast and Central East Shamblin et al., 2011; Ceriani et al., 2019). Perhaps each MU has functional adaptations to these bioclimatic regions as well.
The incubation temperatures at the different sites were among the most notable environmental differences. Nests in subtropical sites generally were 2–3°C warmer than at warm temperate sites (Figure 4). For loggerhead sea turtles in the southeastern United States, embryonic mortality tends to increase at incubation temperatures that reach 34–35°C (McGehee, 1979; Matsuzawa et al., 2002). McGehee (1979) worked with the Central East MU at a site located between the AC and CC and reported that loggerhead nests incubating at 32°C had a hatching success of 71%, while those incubating at 35°C had no surviving embryos. Several nests from the Southeast MU (which is in the subtropical regions) in our study experienced temperatures exceeding this 33–35°C range, with 33% of all nests warming beyond 35°C at some point during incubation. Differences between sand temperatures may be attributed to sand color, with dark beaches being warmer than light beaches (Hays et al., 2001; Laloë et al., 2014). Despite the differences in maximum temperatures, there were no significant differences in hatching and emergence success between warm-temperate and subtropical sites, implying that other factors, in concert, mediate the potential detrimental effects of high temperatures on embryos. Our analyses of nest traits alongside the other physical characteristics of the nest chamber, sand, and beach traits suggest that inclusion of multiple abiotic interactions more accurately characterizes suitable nesting habitat than individual ranges of “optimal” conditions.
Previous studies established clutch temperature, sand moisture, and sand physical characteristic thresholds beyond which embryonic development begins to deteriorate (Miller, 1985; Ackerman, 1997; Foley et al., 2006; Howard et al., 2014). However, the effects of these three variable types are interdependent along Florida’s dynamic Atlantic coast. For example, high sand moisture was associated with lower nest temperatures; previous research identified that moisture mitigates high temperature through evaporative cooling of the nest substrate (Lolavar and Wyneken, 2017, 2020) or eggs (Tezak et al., 2018). RR, JB, and HI nests had higher levels of sand moisture than those in the warm temperate region. To the extent that moisture mitigates the deleterious effects of high incubation temperatures, hatching and emergence success from these nests that were few degrees warmer than published lethal limits for a cheloniid that nests on the same beaches (Chelonia mydas; Laloë et al., 2014). Nevertheless, the difference in water content in this study has two important limitations: (1) the sand moisture measured represent snapshots in time of how nest hydration varied throughout incubation, and (2) while water content provides an index of moisture availability to eggs, sand water potential, which indicates the tendency of water to move, was not measured. Despite these limitations, the greater sand moisture content found within the subtropical region suggests that nests incubating at or above upper thermal tolerance limits necessitate more moist conditions to survive.
The observed regional differences in egg chamber characteristics may reflect sand characteristics. Nest excavation and egg chamber integrity are highly dependent on sand compaction (such as porosity and bulk density) and water content (Magron, 2000). When a nesting female digs in dry, loose sand, lack of grain cohesiveness makes it difficult to shape an egg chamber (Milton and Lutz, 2003). Carthy (1996) found that loggerhead nests in highly compacted sand differed in egg chamber depths. These two characteristics, sand water content and compaction, are fundamental in preserving suitable nesting habitats.
Our results also illustrate how nest microclimate interacts with nest location. Those nests located within wide and flat beaches had the highest hatching and emergence success in the mid-beach zone and closer to the waterline than those on more narrow, steep beaches with nests clustered near the base of the dune at higher elevations. Differences in sea turtle nest distributions associated with differences in beach morphology have been documented in other studies (Fish et al., 2005; Cuevas et al., 2010; Fujisaki et al., 2018), suggesting that nest distributions are structured, in part, by the nesting turtle’s responses to the beach morphology and potentially its physical limitations. For example, previous studies suggest that nest location represents a trade-off between inundation risk low on the beach (Mrosovsky, 1983; Hays and Speakman, 1993) and desiccation risk high on the beach (Witherington et al., 2009), such that predicted cross-shore clutch distributions are bell-shaped (Schoeman et al., 2014). Bladow and Briggs (2017) found significantly higher hatching success rates in nests at RR were within the upper limit of wave runup than nests closer to the dune. However, other cues seemingly unrelated to nest moisture and elevation can cause nesting turtles to select sites and cluster nests (e.g., horizon brightness, Price et al., 2018; and horizon elevation, Salmon et al., 1995). The mechanisms that explain the clustering and dispersion of nests across a continuum of beach types have not been fully explored and likely are multifactorial.
When multiple environmental factors were considered simultaneously, a pattern of resilience in loggerhead sea turtles became apparent. However, caution must be used in applying a principal components approach to environment and nest success data. While there is a clear pattern that suggests resilience in loggerhead sea turtles, the effects of any one incubation environment on hatching and emergence success were not directly measured but are inferred across the study nests. Studies which assess nest environments under controlled conditions or via split clutch manipulation experiments (Ratterman and Ackerman, 1989; Bodensteiner et al., 2015) are useful in decoupling the individual effects of environmental factors. Additionally, the major axis of variation extracted from the PCA should explain a substantial proportion of variation in the data. In our study PC1 and PC2 explained 96.5% of the total environmental variation. By collapsing multiple environmental factors into principal components, we assessed the breadth of factors in which loggerhead nests can successfully incubate. This result is informative even in highly dynamic environments of nesting beaches, but not specific to any cluster of nests.
Together, the results of this study indicate that Northwest Atlantic loggerhead clutches are resilient and successfully productive under a variety of incubation environments. What makes a highly successful beach is not one or two things. The eggs laid both in warm-temperate and subtropical climates successfully developed and hatchlings emerged without significant differences in survival. Nests in both bioclimatic zones differed in location, temperature, moisture levels, and clutch dimensions as well as the subtle genetic differences of the turtles nesting in these two regions. This examination of highly successful nesting beaches identified that loggerheads may be resilient to variable nest environments within the parameters examined in this study. Furthermore, because of the persistence of highly successful nest hotspots, there is potential for a simple and effective method for identifying high-priority conservation areas. What is clear is that the maintenance of these highly successful nest hotspots will be important for the recovery of imperiled loggerhead sea turtles and the management of essential habitats.
Data Availability Statement
The original data contributions presented in the study are included in the article and Supplementary Material. Inquiries about historic data can be directed to the corresponding author or https://myfwc.com/research/wildlife/sea-turtles/nesting/beach-survey-totals/.
Author Contributions
JG designed the study, collected the data, did the analyses, and wrote the initial manuscript. JW conceived of the project, helped design the study, and edited the manuscript. Both authors contributed to the article and approved the submitted version.
Funding
This study was supported by the Florida Atlantic University Graduate Research Scholarship to JG, National Save the Sea Turtle Foundation funding to JW, and personal funds.
Conflict of Interest
The authors declare that the research was conducted in the absence of any commercial or financial relationships that could be construed as a potential conflict of interest.
Publisher’s Note
All claims expressed in this article are solely those of the authors and do not necessarily represent those of their affiliated organizations, or those of the publisher, the editors and the reviewers. Any product that may be evaluated in this article, or claim that may be made by its manufacturer, is not guaranteed or endorsed by the publisher.
Acknowledgments
We are grateful to E. Turla, I. Serra, S. Hirsch, H. Wolverton, G. Carvahal, and S. Trail who reviewed earlier drafts of this manuscript and provided critical discussion. Sea turtle research staff and permit holders at Gumbo Limbo Nature Center, particularly K. Rusenko and D. Anderson, Loggerhead Marinelife Center, particularly S. Hirsch and J. Perrault, InWater Research Group (M. Bresette and C. Mott), Archie Carr National Wildlife Refuge and the UCF Sea Turtle Research Group (K. Mansfield, E. Seney, and C. Long), and Canaveral National Seashore (K. Kniefl) for sharing their historical data, insights, and providing logistical support throughout the project. T. Briggs generously shared equipment, lab space, and expertise. S. Milton provided essential discussion during the study.
Supplementary Material
The Supplementary Material for this article can be found online at: https://www.frontiersin.org/articles/10.3389/fevo.2022.853835/full#supplementary-material
References
Ackerman, R. A. (1980). Physiological and ecological aspects of gas exchange by sea turtle eggs. Am. Zool. 20, 575–583.
Ackerman, R. A. (1997). “The nest environment and the embryonic development of sea turtles,” in The Biology of Sea Turtles, eds P. L. Lutz and J. A. Musick (Boca Raton, FL: CRC Press), 83–106.
Awong-Taylor, J., Craven, K. S., Griffiths, L., Bass, C., and Muscarella, M. (2008). Comparison of biochemical and molecular methods for the identification of bacterial isolates associated with failed loggerhead sea turtle eggs. J. Appl. Microbiol. 104, 1244–1251. doi: 10.1111/j.1365-2672.2007.03650.x
Basco, D. (2006). Seawall impacts on adjacent beaches: separating fact from fiction. J. Coast. Res. 39, 741–744.
Bennett, D. L., George, R., and Ryder, A. (1995). Soil Salinity Assessment Using the EM38: Field Operating Instructions and Data Interpretation. Report 4/95. Perth, WA: Department of Agriculture and Food, Western Australia.
Bladow, R. A., and Briggs, T. R. (2017). Identifying potential natural and human influences on loggerhead sea turtle nesting, hatching, and emergence success. Shore Beach 85, 3–11.
Bodensteiner, B. L., Mitchell, T. S., Strickland, J. T., and Janzen, F. J. (2015). Hydric conditions during incubation influence phenotypes of neonatal reptiles in the field. Funct. Ecol. 29, 710–717.
Broderick, A. C., Godley, B. J., and Hays, G. C. (2001). Metabolic heating and the prediction of sex ratios for green turtles (Chelonia mydas). Physiol. Biochem. Zool. 74, 161–170. doi: 10.1086/319661
Brost, B., Witherington, B., Meylan, A., Leone, E., Ehrhart, L., and Bagley, D. (2015). Sea turtle hatchling production from Florida (USA) beaches, 2002-2012, with recommendations for analyzing hatching success. Endanger. Species Res. 27, 53–68.
Burnham, K. P., and Anderson, D. R. (2002). Model Selection and Inference: A Practical Information-Theoretic Approach, 2nd Edn. (New York, NY: Springer-Verlag), 70–71.
Butt, N., Whiting, S., and Dethmers, K. (2016). Identifying future sea turtle conservation areas under climate change. Biol. Conserv. 204, 189–196.
Camargo, A. J. C., Gutiérrez, Y. Á, Véliz, J. J., and Tortosa, F. S. (2020). Nesting failure of sea turtles in Ecuador-causes of the loss of sea turtle nests: the role of the tide. J. Coast. Conserv. 24, 1–10.
Carr, A., and Hirth, H. (1961). Social facilitation in green turtle siblings. Anim. Behav. 9, 68–70. doi: 10.1016/0003-3472(61)90051-3
Carthy, R. R. (1996). The Role of the Eggshell and Nest Chamber in Loggerhead Turtle (Caretta caretta) Egg Incubation. Ph.D. thesis. Gainesville, FL: University of Florida.
Carthy, R. R., Foley, A. M., and Matsuzawa, Y. (2003). “Incubation environments of loggerhead turtle nests: effects on hatching success and hatchling characteristics,” in Loggerhead Sea Turtles, eds A. B. Bolten and B. E. Witherington (Washington, DC: Smithsonian Books), 144–154. doi: 10.1002/ecy.1603
Casale, P., and Tucker, A. D. (2017). Caretta caretta (amended version of 2015 assessment). IUCN Red List Threat. Species 2017:e.T3897A11 9333622.
Ceriani, S. A., Casale, P., Brost, M., Leone, E. H., and Witherington, B. E. (2019). Conservation implications of sea turtle nesting trends: elusive recovery of a globally important loggerhead population. Ecosphere 10: e02936.
Cleveland, R. B., Cleveland, W. S., McRae, J. E., and Terpenning, I. (1990). STL: a seasonal-trend decomposition. J. Off. Stat. 6, 3–73.
Congdon, J. D., Dunham, A. E., and van Loben Sels, R. C. (1993). Delayed sexual maturity and demographics of Blanding’s turtles (Emydoidea blandingii): implications for conservation and management of long-lived organisms. Conserv. Biol. 7, 826–833. doi: 10.1046/j.1523-1739.1993.740826.x
Crouse, D. T., Crowder, L. B., and Caswell, H. (1987). A stage-based population model for loggerhead sea turtles and implications for conservation. Ecology 68, 1412–1423. doi: 10.2307/1939225
Crowder, L. B., Crouse, D. T., Heppell, S. S., and Martin, T. H. (1994). Predicting the impact of turtle excluder devices on loggerhead sea turtle populations. Ecol. Appl. 4, 437–445. doi: 10.2307/1941948
Cuevas, E., de los Ángeles Liceaga-Correa, M., and Mariño-Tapia, I. (2010). Influence of beach slope and width on hawksbill (Eretmochelys imbricata) and green turtle (Chelonia mydas) nesting activity in El Cuyo, Yucatán, Mexico. Chelonian Conserv. Biol. 9, 262–267.
Dodd, C. K. Jr. (1988). Synopsis of the biological data on the loggerhead sea turtle Caretta caretta (Linnaeus 1758). US Fish Wildl. Serv. Biol. Rep. 88, 1–110.
Drake, D. L., and Spotila, J. R. (2002). Thermal tolerances and the timing of sea turtle hatchling emergence. J. Thermal Biol. 27, 71–81.
Ells, K., and Murray, A. B. (2012). Long-term, non-local coastline responses to local shoreline stabilization. Geophys. Res. Lett. 39:L19401.
Fish, M. R., Cote, I. M., Gill, J. A., Jones, A. P., Renshoff, S., and Watkinson, A. R. (2005). Predicting the impact of sea-level rise on Caribbean Sea turtle nesting habitat. Conserv. Biol. 19, 482–491. doi: 10.1111/j.1523-1739.2005.00146.x
Florida Fish and Wildlife Conservation Commission (2016). Florida Fish and Wildlife Conservation Commission FWC Marine Turtle Conservation Handbook. Available online at: https://myfwc.com/media/3133/fwc-mtconservationhandbook.pdf (accessed October 23, 2021).
Foley, A. M., Peck, S. A., and Harman, G. R. (2006). Effects of sand characteristics and inundation on the hatching success of loggerhead sea turtle (Caretta caretta) clutches on low-relief mangrove islands in southwest Florida. Chelonian Conserv. Biol. 5, 32–41.
Fuentes, M. M., Pike, D. A., Dimatteo, A., and Wallace, B. P. (2013). Resilience of marine turtle regional management units to climate change. Glob. Change Biol. 19, 1399–1406. doi: 10.1111/gcb.12138
Fujisaki, I., Lamont, M., and Carthy, R. (2018). Temporal shift of sea turtle nest sites in an eroding barrier island beach. Ocean Coast. Manag. 155, 24–29.
Gardner, W. H. (1986). “Water content,” in Methods of Soil Analysis: Part 1 Physical and Mineralogical Methods, Vol. 5, ed. A. Klute (Madison, WI: American Society of Agronomy, Soil Science Society of America), 493–544.
Gatto, C. R., Matthews, B., and Reina, R. D. (2021). Role of incubation environment in determining thermal tolerance of sea turtle hatchlings. Endanger. Species Res. 44, 397–408. doi: 10.1098/rspb.2014.1135
Godfrey, M. H., Barreto, R., and Mrosovsky, N. (1997). Metabolically-generated heat of developing eggs and its potential effect on sex ratio of sea turtle hatchlings. J. Herpetol. 31, 616–619.
Griffith, D. A. (2011). Visualizing analytical spatial autocorrelation components latent in spatial interaction data: an eigenvector spatial filter approach. Comput. Environ. Urban. Syst. 35, 140–149.
Hays, G. C., Ashworth, J. S., Barnsley, M. J., Broderick, A. C., Emery, D. R., Godley, B. J., et al. (2001). The importance of sand albedo for the thermal conditions on sea turtle nesting beaches. Oikos 93, 87–94. doi: 10.1034/j.1600-0706.2001.930109.x
Hays, G. C., and Speakman, J. R. (1993). Nest placement by loggerhead turtles, Caretta caretta. Anim. Behav. 45, 47–53.
Hendrickson, J. R. (1958). The green sea turtle, Chelonia mydas (Linn.) in Malaya and Sarawak. Proc. Zool. Soc. Lond. 130, 455–535. doi: 10.1111/j.1096-3642.1958.tb00583.x
Horrocks, J. A., and Scott, N. M. (1991). Nest site location and nest success in the hawksbill turtle Eretmochelys imbricata in Barbados, West Indies. Mar. Ecol. Prog. Ser. 69, 1–8.
Howard, R., Bell, I., and Pike, D. A. (2014). Thermal tolerances of sea turtle embryos: current understanding and future directions. Endanger. Species Res. 26, 75–86.
Katselidis, K. A., Schofield, G., Stamou, G., Dimopoulos, P. A., and Pantis, J. D. (2013). Evidence based management to regulate the impact of tourism at a key sea turtle rookery. Oryx 47, 584–594. doi: 10.1017/S0030605312000385
Laloë, J. O., Cozens, J., Renom, B., Taxonera, A., and Hays, G. C. (2014). Effects of rising temperature on the viability of an important sea turtle rookery. Nat. Clim. Change 4, 513–518.
Limpus, C. J., Reed, P., and Miller, J. D. (1985). “Temperature dependent sex determination in Queensland turtles: intraspecific variation in Caretta caretta,” in ‘Biology of Australian Frogs and Reptiles’, eds G. Grigg, R. Shine, and H. Ehmann (Sydney, NSW: Surrey Beatty), 305–310.
Lolavar, A., and Wyneken, J. (2015). Effect of rainfall on loggerhead turtle nest temperatures, sand temperatures and hatchling sex. Endanger. Species Res. 28, 235–247.
Lolavar, A., and Wyneken, J. (2017). Experimental assessment of the effects of moisture on loggerhead sea turtle hatchling sex ratios. Zoology 123, 64–70. doi: 10.1016/j.zool.2017.06.007
Lolavar, A., and Wyneken, J. (2020). The impact of sand moisture on the temperature-sex ratio responses of developing loggerhead (Caretta caretta) sea turtles. Zoology 138:125739. doi: 10.1016/j.zool.2019.125739
Lolavar, A., and Wyneken, J. (2021). Effects of supplemental watering on loggerhead (Caretta caretta) nests and hatchlings. J. Exp. Mar. Biol. Ecol. 534:151476. doi: 10.1016/j.jembe.2020.151476
Lyons, M. P., Von Holle, B., Caffrey, M. A., and Weishampel, J. F. (2020). Quantifying the impacts of future sea level rise on nesting sea turtles in the southeastern United States. Ecol. Appl. 30:e02100. doi: 10.1002/eap.2100
Magron, J. P. R. (2000). Impact of Upland Sand Beach Nourishment on the Physical Environment and Marine Turtles Nesting at Sebastian Inlet, Florida. Doctoral dissertation. Melbourne, FL: Florida Institute of Technology.
Maloney, J. E., Darian-Smith, C., Takahashi, Y., and Limpus, C. J. (1990). The environment for development of the embryonic loggerhead turtle (Caretta caretta) in Queensland. Copeia 1990, 378–387.
Matsuzawa, Y., Sato, K., Sakamoto, W., and Bjorndal, K. (2002). Seasonal fluctuations in sand temperature: effects on the incubation period and mortality of loggerhead sea turtle (Caretta caretta) pre-emergent hatchlings in Minabe, Japan. Mar. Biol. 140, 639–646.
Mazaris, A. D., Matsinos, G., and Pantis, J. D. (2009). Evaluating the impacts of coastal squeeze on sea turtle nesting. Ocean Coast. Manag. 52, 139–145. doi: 10.1111/eva.13277
Mazor, T., Levin, N., Possingham, H. P., Levy, Y., Rocchini, D., Richardson, A. J., et al. (2013). Can satellite-based night lights be used for conservation? The case of nesting sea turtles in the Mediterranean. Biol. Conserv. 159, 63–72. doi: 10.1016/j.biocon.2012.11.004
McElroy, M. L., Dodd, M. G., and Castleberry, S. B. (2015). Effects of common loggerhead sea turtle nest management methods on hatching and emergence success at Sapelo Island, Georgia, USA. Chelonian Conserv. Biol. 14, 49–55.
McGehee, M. A. (1979). Factors Affecting the Hatching Success of Loggerhead Sea Turtle Eggs (Caretta caretta). Unpublished Master’s thesis. Orlando, FL: University of Central Florida.
McGehee, M. A. (1990). Effects of moisture on eggs and hatchlings of loggerhead sea turtles (Caretta caretta). Herpetologica 46, 251–258.
Miller, J. D. (1985). “Embryology of marine turtles,” in Biology of the Reptilia, eds C. Gans, F. Billett, and P. F. A. Maderson (New York, NY: John Wiley & Sons), 269–328.
Miller, J. D. (1999). “Determining clutch size and hatching success,” in Research and Management Techniques for the Conservation of Sea Turtles. Publication No. 4, eds K. L. Eckert, K. A. Bjorndal, F. A. Abreu-Grobois, and M. Donnelly (Blanchard, PA: IUCN/SSC Marine Turtle Specialist Group), 124–129.
Milton, S. L., and Lutz, P. L. (2003). “Physiological and genetic responses to environmental stress,” in The Biology of Sea Turtles, Vol. II, eds P. L. Lutz, J. A. Musick, and J. Wyneken (Boca Raton, FL: CRC Press), 163–197. doi: 10.1201/9781420040807.ch6
Montero, N., Ceriani, S. A., Graham, K., and Fuentes, M. M. (2018). Influences of the local climate on loggerhead hatchling production in North Florida: implications from climate change. Front. Mar. Sci. 5:262. doi: 10.3389/fmars.2018.00262
Moritz, S., and Bartz-Beielstein, T. (2017). imputeTS: time series missing value imputation in R. R J. 9:207.
Mortimer, J. A. (1990). The influence of beach sand characteristics on the nesting behavior and clutch survival of green turtles (Chelonia mydas). Copeia 1990, 802–817.
Mota, M. J. (2009). Beach Restoration in Florida: Effects on Sea Turtle Nesting and Hatchling Physiology. Unpublished Doctoral dissertation. Gainesville, FL: University of Florida.
Mrosovsky, N. (1983). Ecology and nest-site selection of leatherback turtles Dermochelys coriacea. Biol. Conserv. 26, 47–56.
National Marine Fisheries Service and U.S. Fish and Wildlife Service (2008). Recovery Plan for the Northwest Atlantic Population of the Loggerhead Sea Turtle (Caretta caretta), Second Revision. Silver Spring, MD: National Marine Fisheries Service.
Pike, D. A. (2007). Sea turtle species vary in their susceptibility to tropical cyclones. Oecologia 153, 471–478. doi: 10.1007/s00442-007-0732-0
Pike, D. A. (2013). Climate influences the global distribution of sea turtle nesting. Glob. Ecol. Biogeogr. 22, 555–566.
Price, J. T., Drye, B., Domangue, R. J., and Paladino, F. V. (2018). Exploring the role of artificial lighting in loggerhead turtle (Caretta caretta) nest-site selection and hatchling disorientation. Herpetol. Conserv. Biol. 13, 415–422.
QGIS Development Team (2019). QGIS Geographic Information System. Open Source Geospatial Foundation Project. Available online at: http://qgis.osgeo.org (accessed April 30, 2021).
R Core Team (2018). R: A Language and Environment for Statistical Computing. Vienna: R Foundation for Statistical Computing.
Rafferty, A. R., Evans, R. G., Scheelings, T. F., and Reina, R. D. (2013). Limited oxygen availability in utero may constrain the evolution of live birth in reptiles. Am. Nat. 181, 245–253. doi: 10.1086/668827
Ratterman, R. J., and Ackerman, R. A. (1989). The water exchange and hydric microclimate of painted turtle (Chrysemys picta) eggs incubating in field nests. Physiol. Zool. 62, 1059–1079. doi: 10.1086/physzool.62.5.30156196
Rizkalla, C. E., and Savage, A. (2011). Impact of seawalls on loggerhead sea turtle (Caretta caretta) nesting and hatching success. J. Coast. Res. 27, 166–173. doi: 10.2112/jcoastres-d-10-00081.1
Salmon, M., Reiners, R., Lavin, C., and Wyneken, J. (1995). Behavior of Loggerhead Sea turtles on an urban beach. I. Correlates of nest placement. J. Herpetol. 29, 560–567.
Schoeman, D. S., Schlacher, T. A., and Defeo, O. (2014). Climate-change impacts on sandy-beach biota: crossing a line in the sand. Glob. Change Biol. 20, 2383–2392. doi: 10.1111/gcb.12505
Schroeder, B. A., Foley, A. M., and Bagley, D. A. (2003). “Nesting patterns, reproductive migrations, and adult foraging areas of loggerhead turtles,” in Loggerhead Sea Turtles, eds A. B. Bolten and B. E. Witherington (Washington, DC: Smithsonian Institution Press), 114–124.
Shamblin, B. M., Dodd, M. G., Bagley, D. A., Ehrhart, L. M., Tucker, A. D., Johnson, C., et al. (2011). Genetic structure of the southeastern United States loggerhead turtle nesting aggregation: evidence of additional structure within the peninsular Florida recovery unit. Mar. Biol. 158, 571–587. doi: 10.1007/s00227-010-1582-6
Tezak, B. M., Sifuentes-Romero, I., and Wyneken, J. (2018). A new approach for measuring temperature inside turtle eggs. J. Exp. Biol. 221:jeb188698. doi: 10.1242/jeb.188698
Troast, B., Paperno, R., and Cook, G. S. (2020). Multidecadal shifts in fish community diversity across a dynamic biogeographic transition zone. Divers. Distrib. 26, 93–107.
Van De Merwe, J., Ibrahim, K., and Whittier, J. (2006). Effects of nest depth, shading, and metabolic heating on nest temperatures in sea turtle hatcheries. Chelonian Conserv. Biol. 5, 210–215. doi: 10.2744/1071-8443(2006)5[210:EONDSA]2.0.CO;2
Weishampel, J. F., Bagley, D. A., Ehrhart, L. M., and Rodenbeck, B. L. (2003). Spatiotemporal patterns of annual sea turtle nesting behaviors along an East Central Florida beach. Biol. Conserv. 110, 295–303. doi: 10.1016/S0006-3207(02)00232-X
Witherington, B., Hirama, S., and Mosier, A. (2011). Barriers to sea turtle nesting on Florida (United States) beaches: linear extent and changes following storms. J. Coast. Res. 27, 450–458. doi: 10.2112/JCOASTRES-D-09-00146.1
Witherington, B., Kubilis, P., Brost, B., and Meylan, A. (2009). Decreasing annual nest counts in a globally important loggerhead sea turtle population. Ecol. Appl. 19, 30–54. doi: 10.1890/08-0434.1
Wood, D. W., and Bjorndal, K. A. (2000). Relation of temperature, moisture, salinity, and slope to nest site selection in loggerhead sea turtles. Copeia 2000:119.
Zárate, P., Bjorndal, K. A., Parra, M., Dutton, P. H., Seminoff, J. A., and Bolten, A. B. (2013). Hatching and emergence success in green turtle Chelonia mydas nests in the Galápagos Islands. Aquatic Biology 19, 217–229.
Keywords: sea turtle, nesting beach, loggerhead, incubation, nest success and survival, turtle eggs, nest, clutch temperature
Citation: Gravelle J and Wyneken J (2022) Resilient Eggs: Highly Successful Loggerhead Sea Turtle Nesting Sites Vary in Their Characteristics. Front. Ecol. Evol. 10:853835. doi: 10.3389/fevo.2022.853835
Received: 13 January 2022; Accepted: 24 February 2022;
Published: 05 April 2022.
Edited by:
Jeanine M. Refsnider, University of Toledo, United StatesReviewed by:
Deby Cassill, University of South Florida St. Petersburg, United StatesGail Schofield, Queen Mary University of London, United Kingdom
Matthew Ware, Florida State University, United States
Copyright © 2022 Gravelle and Wyneken. This is an open-access article distributed under the terms of the Creative Commons Attribution License (CC BY). The use, distribution or reproduction in other forums is permitted, provided the original author(s) and the copyright owner(s) are credited and that the original publication in this journal is cited, in accordance with accepted academic practice. No use, distribution or reproduction is permitted which does not comply with these terms.
*Correspondence: Jeanette Wyneken, and5bmVrZW5AZmF1LmVkdQ==