- 1Leibniz Institute on Aging – Fritz Lipmann Institute, Jena, Germany
- 2Leibniz Institute for Zoo and Wildlife Research, Berlin, Germany
- 3Faculty of Life Sciences, Albrecht Daniel Thaer-Institute, Humboldt University, Berlin, Germany
Naked mole-rats form colonies with a single reproductively active female surrounded by subordinate workers. Workers perform offspring care, construction and defense of the burrow system, and food supply. Such division of labor, called “cooperative breeding,” is strongly associated with the evolution of monogamous mating behavior, as seen in several mammalian lineages. This association is explained by the evolutionary theory of kin selection, according to which a subordinate adult may help to raise other’s offspring if they are in full sibling relationship. In conflict with this theory, the naked mole-rat is widely considered to be polyandrous, based on reports on multiple males contributing to a colony’s progeny. In order to resolve this contrast, we undertook an in-depth microsatellite-based kinship analysis on captive colonies. Four independent colonies comprising a total of 265 animals were genotyped using a panel of 73 newly established microsatellite markers. Our results show that each mole-rat colony contains a single monogamous breeder pair, which translates to a reproductive skew of 100% for both sexes. This finding, also in conjunction with previously published parental data, favors monogamy as the best-fitting model to describe naked mole-rat reproduction patterns. Polyandry or other polygamous reproduction models are disfavored and should be considered as exceptional. Overall, the empirical genetic data are in agreement with the kin selection theory.
Introduction
The naked mole-rat (NMR; Heterocephalus glaber) is a rodent species outstanding for its extraordinary longevity (maximum lifespan > 37 years) (Lee et al., 2020) cancer resistance (Tian et al., 2013; Seluanov et al., 2018), adaptation to an underground hypoxic and hypercapnic habitat (Smith et al., 2011; Fang et al., 2014; Park et al., 2021), lack of fur (Thigpen, 1940; Braude et al., 2001), and its cooperative social system (Jarvis, 1991). In NMR colonies a dominant reproductively active female, called “queen,” is surrounded by subordinate workers, which take care of the offspring, defend the burrow system, and dig tunnels for foraging on plant roots and tubers (Jarvis, 1991; Lacey and Sherman, 1991). Such division of labor among sexually mature adults, termed “cooperative breeding,” is also found in other mammals including wolfs, meerkats and beavers (Wilson, 1975; Sparkman et al., 2011; Lukas and Clutton-Brock, 2012). In these species, reproduction is significantly skewed toward one mating pair per colony, which means their breeding behavior tends to monogamy. It is assumed that the NMR queen sustains her reproductive monopoly by suppressive dominance behaviors, such as pushing and biting subordinates, during parade walks across the colony (Faulkes and Abbott, 1993; Van der Westhuizen et al., 2013). Thus, mole-rat workers usually refrain from reproductive activity as long the queen is fertile and dominant (Jarvis, 1991; Lacey and Sherman, 1991; Edwards et al., 2021).
Queens can maintain their breeding privilege for up to 17 years (Jarvis, 1991). However, extensive reshaping of colonies, which also affects the genetic composition, can occur by different mechanisms. An opportunity to take over a queen position is usually followed by social colony instability and violent fightings between high-ranking individuals (Lacey and Sherman, 1991; Van der Westhuizen et al., 2013). There is only a very small chance that animals successfully migrate to other foreign colonies (Braude, 2000) since NMRs have a fine smell- and vocalization-based kin recognition and do aggressively discriminate against foreign conspecifics (O’Riain and Jarvis, 1997; Buffenstein et al., 2022). New colonies may be formed by colony splitting, inter-colony fusion or as nascent colonies started by small groups descending from different colonies (Braude, 2000).
Cooperative breeding is tightly linked with a monogamous mating system, according to a thorough analysis of 14 independent cooperatively breeding mammal lineages (Lukas and Clutton-Brock, 2012). According to this analysis it appears that ancestors of cooperatively breeding mammals were all monogamous and that cooperative breeding without monogamy is unstable. Such a linkage is explained by the model of kin selection, according to which a subordinate adult may help to raise other’s young if it has a full sibling relation to the young (Bourke, 2014). The altruistic behavior of the adult individual can function as a selective trait due to the close genetic relationship to the young sibling (50%, on average), and this is assumed to balance the cost of the adults that refrain from reproduction.
However, according to the literature, the NMR is currently viewed as polyandrous, meaning that a colony’s reproduction is attributed to a single queen, but up to three breeding males (Faulkes et al., 1997; Lacey and Sherman, 1997; Buffenstein, 2005; Lukas and Clutton-Brock, 2012; Buffenstein et al., 2022). Primary evidence for this view refers to a single genetic study, on a single colony, which identified two males that contributed to colony offspring (Faulkes et al., 1997). A third male could not be excluded from paternal contribution due to limited resolution of minisatellite markers. Other studies based on behavioral observation have reinforced the classification of NMR mating behavior as polyandrous (Jarvis, 1991; Lacey and Sherman, 1991). These are, however, less conclusive since it remains unresolved whether copulation behavior leads to successful fertilization. In fact, a number of parentage studies on other species has revealed disagreements between observed mating behavior and reproduction outcome (Goossens et al., 1998; Gagneux et al., 1999; Vigilant et al., 2001).
Taken together, limited primary evidence for a small reproductive skew for males in NMR colonies leaves the question if this species poses a specific challenge to the generalized evolutionary model for cooperative breeding. In order to resolve this peculiarity, we undertook an in-depth microsatellite-based kinship analysis on four independent NMR laboratory colonies. The results indicate a high reproductive skew for both sexes, favoring monogamy as the mating model for this species, which is in agreement with the kin selection theory.
Materials and Methods
Animals
Naked mole-rat colonies B1 and B2 were kept at the IZW in Berlin, with the approval to keep and breed NMR for research purposes by the local ethics committee of the “Landesamt für Gesundheit und Soziales,” Berlin, Germany (reference no. #ZH 156; September 23, 2008). The mole-rats were housed in an artificial burrow system inside a climatized box (2 × 1 × 1 m3 size) consisting of eight acrylic glass boxes and interconnecting tunnels. Temperature was adjusted to 25.0 ± 2.0°C, and humidity ranged from 40 to 70%. The chambers contained wood shavings for bedding and unbleached paper tissue as nesting material. Animals were fed with a mixed diet of vegetables, fruits ad libitum and cereals were provided three times per week. All newborns were marked within 12 h after birth as previously described (Jarvis, 1991; Roellig et al., 2011) and individual tissue samples were stored for later DNA extraction. For permanent marking, all pups further received a transponder microchip at an age of 3 months (7 × 1 mm size). Colony B1 consisted of 6 adult females, including the known queen, and 9 adult males when the monitoring started (September 2009). Over an observation period of 3 years the queen bore eleven litters with a total of 130 pups (Table 1 and Supplementary Figure 1). One pup could not be sampled for analysis. Colony B2 consisted of 6 adult females, including the known queen, and 5 adult males when the monitoring started (May 2010). Over a period of 1 year the queen bore four litters, comprising 50 pups, before she died in the course of rivalry fights (Table 1 and Supplementary Figure 1). Another two colonies, V1 and V2, were kept at the Vienna Zoo, Schönbrunn, Austria. Burrow systems and feeding protocols were very similar to those of colonies B1 and B2; temperature was regulated via infrared lamps, adjusting temperature to 26.0 ± 2.0°C. When colonies arrived at the Vienna Zoo, colony V1 consisted of six adult moles and colony V2 was already a breeding colony with 33 moles, including 11 pups from the last litter. After some successfully litters in V1 and V2, both colonies ceased breeding, at least for 2 years. At the time of sample collection (April 2012), colony V1 member size was 21 and V2 size was 39 animals (Table 1), and both colonies contain an unresolved mixture of adult and juvenile animals. Biopsies from the tail tips were taken from all colony members using a sterile scalpel.
Ultrasound Examination
Ultrasound investigations were executed in all four colonies to investigate the reproductive conditions of the single mole-rats using a high-frequency and high-resolution ultrasonic device (Vevo 2100, VisualSonics, Inc., Toronto, ON, Canada). Reproductive activity was documented by measuring the size and condition of the reproductive organs (e.g., size, presence of corpus luteum, placental scars, sperm production) (Garcia Montero et al., 2016). General anesthesia was induced by subjecting animals inside of a small container to 5 vol.% isoflurane at an oxygen flow rate of 1.5 l/min using a Dräger Vapor 2000 system. Once the animal was asleep, anesthesia was maintained using a small mask suitable for rodents at 1.5 to 2.0 vol.% isoflurane for a maximum of 10 min. To prevent hypothermia of the moles, all procedures were performed on a heating pad (HT 200, Minitub GmbH, Tiefenbach, Germany) operating at 37°C. When fully awake, mole-rats were returned to their respective colonies.
DNA Isolation
Genomic DNA was isolated from ca. 20 mg of tissue sample using the QIAamp DNA Mini kit (Qiagen, Hilden, Germany).
Sex Determination
Genetic sex determination based on the DDX3Y-psi gene locus was previously described for the NMR (Katsushima et al., 2010). We modified this concept to include the DDX3X gene locus as an internal reference. Degenerate PCR primers, 5’-CAG ATG GTC CAG GAG AIG CTT-3’ and 5’-FAM-CCC ATA CCT TCC ATT TTC CTA A-3’, were designed to target the intron 8 region of both gene loci while circumventing amplification of additional autosomal, retroposed DDX3 gene copies. Five ng of genomic DNA was used in reactions with Taq BioMix White (Bioline), 10 pmol primers (Metabion) in a volume of 25 μl. The cycling conditions were 2 min initial denaturation at 94°C, followed by 40 cycles of 45 s denaturation at 94°C, 50 s annealing at 56°C, 60 s extension at 72°C, and a final 30 min extension step at 72°C. Each FAM-labeled PCR product was diluted with water (typically 1:150), and 1 μl of the dilution was mixed with 10 μl optically pure formamide (Applied Biosystems) and 0.25 μl GeneScan-500 LIZ length standard (Applied Biosystems). After denaturation for 3 min at 94°C the samples were separated on POP-7 polymer on an ABI 3730xl capillary sequencer (Applied Biosystems), injecting 10 s at 1.6 kV and running at 15 kV. The electropherograms were analyzed with the GeneMapper 4.0 software (Applied Biosystems). A DDX3Y peak (291 bp) with a signal area greater than 0.1-fold that of DDX3X (237 bp) was used as an indication for male sex.
Microsatellite Markers
In total 73 novel microsatellite markers were established. This comprised three microsatellite markers developed according to a previously described enrichment protocol (Nolte et al., 2005; Leese et al., 2008), with some own modifications as described elsewhere (Molecular Ecology Resources Primer Development, Andree et al., 2010; Supplementary Table 1, label “Hetgla”). The method uses DNA from distantly related species for capturing of low complexity sequences in the species of interest. A bulk of 70 microsatellite markers was selected using the published H. glaber genome sequence (Kim et al., 2011). The genome sequence was screened for perfect sequence repetitions of unit sizes 2 and 3 bp. Microsatellites with 13–20 dinucleotide or 15–24 trinucleotide repeat units were selected as marker candidates (58,389 and 868 loci, respectively) since this length promised a balance between likely polymorphisms and a decent stutter noise during PCR amplification. PCR primers for these loci were defined using the command line version of PRIMER3 (version 2.2.3), and the primer score was used to prioritize the marker list (Supplementary Table 1, label “nmrsat”).
Microsatellite Genotyping
PCR was done using 5 ng genomic DNA, 2.5 units of Taq polymerase (Qiagen, cat. no. 201207), 10 pmol marker-specific primers (Metabion; sequences in Supplementary Table 1), one 5’-labeled with 6-carboxyfluorescein (FAM) or hexachloro-fluorescein (HEX), and 20% solution Q in a reaction volume of 25 μl. The cycling conditions were 2 min initial denaturation at 94°C, followed by 35 cycles of 45 s denaturation at 94°C, 50 s annealing at 58°C, 1 min extension at 66°C, and a final 30 min extension step at 72°C. The fluorescence-labeled PCR products were size-separated on an ABI 3730xl capillary sequencer as described for sex determination. Following automatic peak calling by GeneMapper, all electropherograms were checked for calling errors at least twice. Animals which remained orphans after a first round of kinship analysis were additionally checked for the validity of exclusive marker signals, provoking correction of two allele calls.
Kinship Analysis
Parentage was resolved at the individual level using a Mendelian test on all possible trio scenarios (“exclusion test I”), where each scenario involved the single known mother, a candidate descendant, and one of the possible fathers. For colonies which lacked family history records, each animal was tested for a possible parental role for all other animals. Iteration over trios and Mendelian testing was implemented as in-house software. Each marker was tested for X-chromosomal linkage, indicated by strict mono-allelic genotypes in males in contrast to frequent bi-allelic genotypes in females. In parallel, kinship analysis on the individual level was done using the program CERVUS (version 3.0.7) (Kalinowski et al., 2007), in order to cross-validate the findings. A second parentage test scheme (“exclusion test II”) was used to resolve ambiguities after exclusion test I: given a parental model F-M (F = female parent, M = male parent) with colony-wide significance, an alternative parental model F-M’, F’-M, or F’–M’ (with alternative female parent F’, alternative male parent M’) is taken valid only if it has produced offspring that is genetically distinct from any offspring of F-M. The genotype intersection between offspring F-M and offspring F’-M’ reflects the lack of resolving power in exclusion test I and quantifies the beta error in exclusion test II (i.e., false maintenance of parentage presumption, H0). Beta likelihood was calculated from the parental genotypes, iterating the fractional intersection marker by marker. Since this beta calculation is based on the assumption of free marker recombination, markers with possible short-range linkage to other markers (e.g., nmrsat3763 and nmrsat3764; Supplementary Table 1) were excluded from this analysis.
Results
Microsatellite Genotyping
We established a total of 73 microsatellite markers for the genotyping of NMRs (Supplementary Table 1), of which 68 markers (93%) were polymorphic in a test panel of 6 animals from three colonies. Altogether we genotyped 27 to 37 informative markers in 265 mole-rats from four captive colonies (Table 1 and Supplementary Figure 2). The allele patterns indicated X-chromosomal linkage for three markers (nmrsat3637, nmrsat215074, nmrsat233031), which was confirmed by the downstream parental tests. In the progeny genotype data we identified eight potentially novel alleles, that is, alleles that did not exist in the respective adult subpopulations. Of those alleles, six were validated as true novel alleles through repeated measurements and Sanger sequencing. The remaining two were considered as drop-outs. From this we extrapolate a germline mutation rate of 6.6⋅10–4 per generation per marker. The frequency of unrecognized mutations is probably lower because mutations more likely give rise to novel alleles than converting to pre-existing alleles. Moreover, the validation rate for novel alleles indicates that the genotype mis-calling rate is lower than the rate of novel alleles, estimated to 4⋅10–4.
Parentage Analysis in Captive Naked Mole-Rat Colonies
Parentage analysis at the resolution of individual offspring was done by Mendelian testing on all possible trio scenarios, also known as exclusion approach (Jones et al., 2010). In this approach, termed “exclusion test I,” trios were excluded from the list of possible relations if they did not show valid Mendelian genotype patterns. In this procedure, we ignored allelic trios which involved verified spontaneous mutations (eight cases, cf. Results see section “Microsatellite Genotyping”) or calling drop-outs. As a result we obtained highly resolved parentage models for all colonies (Table 1), including colonies V1 and V2, for which no information about the family structure was available. According to the genotype results, colony V1 contained three co-founders in addition to two parents and their 16 pups. Colony V2 was explained as a composite of two parents and their 37 pups, including one animal whose relation remained uncertain. This latter could, alternatively, have descended from two progeny individuals of the universal parents and would then be their only (surviving) pup. However, in a scenario of breeder pair switch it is very unlikely that the former breeders would have survived since breeding role takeover events are typically mediated by fatal rivalry fight in the NMR (Faulkes et al., 1997; Van der Westhuizen et al., 2013). For each of the other NMR colonies in our study, B1 and B2, we knew the identities of queen, additional founder animals and the offspring (Table 1). Paternity testing of the progeny against the adult founder animals gave mostly clear answers for colonies B1 (127 of 129 pups) and B2 (49 of 50 pups), indicating single universal fathers for all offspring. But, 16 additional ambiguities in the kinship model of B1 arose if we considered that grown-up progeny may have participated in breeding activity (Table 1). This is possible because B1 was monitored over a period of 3 years, and young NMR become sexually mature at an age of 180–225 days (Jarvis, 1991; Buffenstein, 2005; Supplementary Text 1). Other colonies have been monitored for a period shorter than the sexual maturation time of early offspring (colony B2), or have been analyzed in an undirected approach, considering all animals as both, parental and offspring candidates (colonies V1, V2).
So far, finding that all resolved relationships indicate one universal father per colony, and, given that ambiguous relationships always include that same universal father, we favor the kinship model with the universal father as the most parsimonious one. This is corroborated by the fact that it does not imply mixed-sire litters. In any case, in order to quantify the relevance of ambiguous parental relationships we calculated the likelihood that a parental pair F-M produces offspring which may also be considered offspring of parental pair F-M’, or vice versa (where F is the female parent, M and M’ are alternative male parental candidates; Figure 1A). The median degree of these genotype intersections (beta) is 0.017 for colony B1 (maximum 0.036 in individual tests; Figure 1B). This small beta value indicates a minor loss of detection power for true additional parents if we ignore the ambiguity of intersection genotypes. That is, putative alternative paternal contributors (fathers M’, M”, etc.) had a very high chance to produce offspring that is genetically distinct from progeny of father M. This modified paternity test, termed “exclusion test II,” was applied to all remaining ambiguities. In colony V2, the kinship model was resolved with beta = 1.5⋅10–6, clearly indicating a single universal breeder pair for all progeny (Table 1 and Figure 1C). In colony B2, the one ambiguous paternal relation was resolved in favor of a universal father with beta = 1.3⋅10–3.
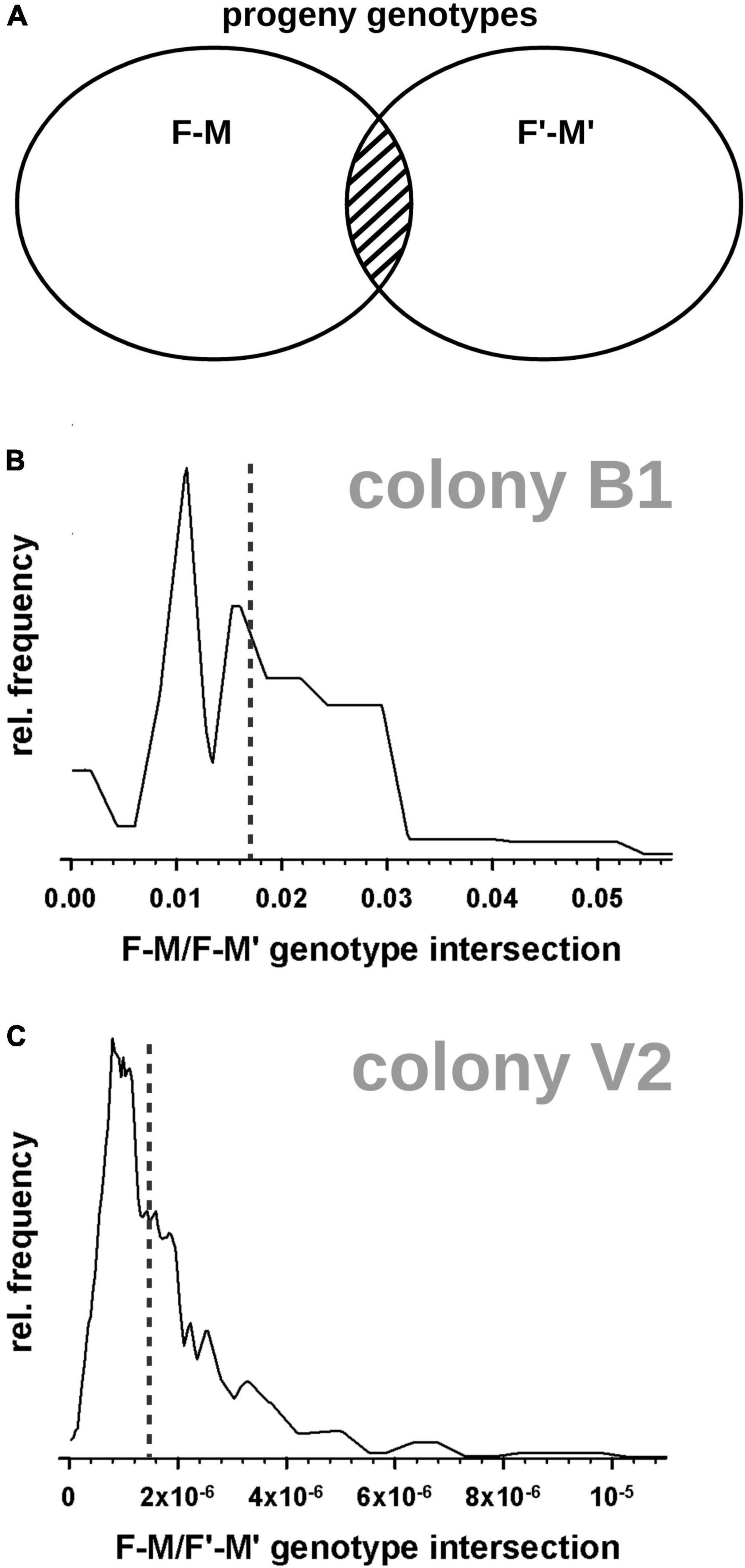
Figure 1. Probabilistic model for ambiguous parental relationships underlying the exclusion test II. A known female parent (F or F’) and a candidate male parent (M or M’) were evaluated for possible progeny genotypes they may generate. (A) Two alternative parent pairs (F-M and F’-M’) produce progeny genotypes (circles), which may intersect (striped region). (B,C) Histograms showing the full distribution of the individually calculated progeny genotype intersections, for alternative parental animals (F’ or M’ in panel A) compared to the universally consistent breeding animals in a colony (F-M in panel A). The size of the intersection (x-axis) is scaled as fraction of genotype space F-M. (B) Alternative breeding males in colony B1; median intersection 0.017 (dotted vertical line). (C) Alternative breeding pairs (F’-M’) in colony V2; median intersection 0.0000015 (dotted vertical line).
As a confirmation, the genotype-based attribution of breeders matched the results of the ultrasound-based examination. Queens identified in the Vienna colonies showed placental scars, indicative of former breeding activity, the identified breeding males were among those that had increased testis sizes (two in V1, three in V2).
Opportunities for Reproductive (Re)binding
Having observed the exclusive breeding activity of only a single male NMR per monitored colony we wondered: is it possible that the NMR actually was polyandrous (or any kind of polygamous) biologically, but there is any factor in the colony structures or settings that urged the analyzed animals into strictly bound reproductive partnerships? In other words, is there sufficient competition for reproductive partnering within our colony scenarios justifying model conclusions?
Detailed records of the age composition for two of the analyzed colonies, B1 and B2, helped to resolve this issue (Supplementary Figure 1). A total of 15 litters have been recorded in these colonies, while at the siring time points, between 4 and 9 adult founder males were present (average 5.3; Supplementary Figure 1A). We consider these the least fraction of male competitors for breeding activity. In addition, at an age of 180–225 days offspring is considered to reach sexual competence, according to literature and consistent with our own observations (Jarvis, 1991; Buffenstein, 2005; Supplementary Text 1). This is relevant for colony B1 which gave rise to 25 newborn males that reached the age of sexual maturation before the siring of the last litter of that colony (Supplementary Figure 1B). Since an incest barrier does not exist in the NMR (Reeve et al., 1990), young males may sire their solitary mothers upon reaching their age of sexual maturation, according to literature and our own observations (Clarke and Faulkes, 1999; Buffenstein, 2005; Supplementary Text 1). Thus, these grown-up males should also be considered as breeding competitors (on average, 9.5 per siring event in colony B1; Supplementary Figure 1A). In summary, the colony scenarios analyzed here contained multiple competing mating partners, both adult and newly matured, giving realistic chance for alternative mating patterns (such as polygamy, polyandry) to occur in our experimental setting. Thus, the obtained results are considered conclusive in the sense that the lacking genetic manifestation of alternative mating patterns can be attributed to the behavioral level.
Discussion
The microsatellite data reported here for four captive NMR colonies reveal a single breeding pair per colony, which translates to a maximum reproductive skew of 100%. The only previous genetic study on intra-colony kinship has reported two males participating in colony reproduction (Faulkes et al., 1997). While not explicitly stated, we assume that Faulkes et al. (1997) have studied only this one colony in relevant detail. Pre-selection for high heterogeneity, as the authors state, may have favored a multi-parental result, albeit such potential bias cannot be estimated adequately. Taking together all these genetic results for the NMR, the average reproductive skew of females is 100%, that of males is 90% (one colony 50%, four others 100%). Operationally, these values let us categorize the NMR mating system as sexually monogamous, clearly distinguished from skews of about 50% which would indicate continuous competition for reproduction success. We point out, however, that monogamy in the NMR should be understood as serial monogamy, since upon death of one breeder, the surviving partner likely rebinds to a breeding successor (Lacey and Sherman, 1991; Faulkes et al., 1997; Van der Westhuizen et al., 2013) (and own observation). In addition, the high reproductive skews for the NMR fall well into the range of other cooperatively breeding species (females 88–100%, median 100%; males 76–100%, median 88%) (Lukas and Clutton-Brock, 2012), which answers the introductory question if the NMR may be an outlier to the striking evolutionary correlation between cooperative breeding and monogamous reproduction – no, given the novel results, it is in agreement.
Behavioral studies make an important contribution to detect unsolved research questions like, e.g., reproductive interaction, in the NMR. However, important for the present study, we substantially doubt that observational data can elucidate the sexual mating system in a reliable manner. Behavioral studies equate the “mating males” as “reproductive males,” although breeding NMR males cannot be distinguished by morphological characteristics (e.g., body size, large external testes or protuberant penises) (Jarvis, 1991; Braude, 2000) as in other related mole-rat species (e.g., Fukomys) (Faulkes and Bennett, 2021). Some authors defined “copulation” as contact between the genitalia of the male and female with pelvic thrusting by the male just before and during copulation (Lacey and Sherman, 1991). However, despite its potential relevance for the precise identification of breeding males, most authors identify male breeder solely based on mating-related behaviors, such as mounting and ano-genital nuzzling (Jarvis, 1991; Lacey and Sherman, 1991; Goldman et al., 2006; Van der Westhuizen et al., 2013), which is even less reliable. It has been postulated that ano-genital nuzzling is frequently practiced by breeding NMRs in order to strengthen their bonding and to obtain chemical cues about the reproductive condition from each other (Faulkes and Abbott, 1991; Goldman et al., 2006). However, ano-genital nuzzling also occurs with and among non-breeding animals of both sexes (Jarvis, 1991; Ciszek, 2000; Goldman et al., 2006), confirmed by personal observations on colonies B1 and B2. “Mounting” is described as a behavior position in which the breeding male attempts to bring his genitals into contact with those of the queen, although with the difference of no pelvic thrusting (Lacey and Sherman, 1991). Thus, numerous of mounting attempts occur but do not result in true copulations (Lacey and Sherman, 1991). Like ano-genital nuzzling, mounting also occurs with non-breeder males and was sometimes observed in colonies B1 and B2 when the queen was physically handicapped (e.g., otitis, suspected spinal disc herniation, or during late pregnancy). In sum, it is impossible to be certain which male is reproductive unless mating is actually witnessed (Jarvis, 1991), and this raises the concern that male contributions in NMR breeding could have been overestimated in the past, compared to our genetic evidence.
To date, no parentage studies have been undertaken and published from wild NMR colonies, presumably as a consequence of their special biology. NMR colonies typically contain 70–80 individuals, and sometimes up to 300 individuals in the wild (Brett, 1991), which significantly increases the number of possible mating pairs in kinship analysis and challenges marker depth for kinship resolution. Further, NMR colonies live in huge, subterranean burrows up to 2–3 km tunnel length (Brett, 1986). The breeding females, their mate(s) and newborn pups, representing the most important individuals for genetic paternity analysis, are usually trapped as the last members of a colony (Brett, 1991; Braude, 2000). In addition, as an incest avoidance mechanism appears to be absent in the NMR (Jarvis et al., 1994), colonies exhibit a high level of inbreeding and low levels of genetic variation within colonies (Reeve et al., 1990; Faulkes et al., 1997; Chau et al., 2018). These low levels of genetic variability and the lack of sufficient polymorphic markers previously made exclusion approaches in paternity analysis complicated to impossible (Lacey and Sherman, 1991). Addressing these challenges in the present study, we constructed a rich panel of novel microsatellite markers, which significantly extends the marker resource for the NMR (Ingram et al., 2014, 2015; Chau et al., 2018).
As the analytical strategy we chose a classical exclusion approach for several reasons: first, while alternative, probabilistic, methods rank paternity models by assigning posterior probabilities, an exclusion approach aims at the full resolution of alternative models. Second, probability priors in a probabilistic approach, namely probabilities of somatic mutations and typing errors, are usually difficult to estimate, while these may have a substantial influence on the ranking result. Third, a basic assumption of most probabilistic methods, the free flow of genetic alleles through the population, is certainly violated in NMR colony scenarios since the genotype patterns are dominated by kinship relations. The chosen exclusion approach has the advantage to cope with varying levels of relatedness. In the worst case, i.e., a high degree of relatedness, this approach requires a larger number of markers to become conclusive, but the analytical framework will not be thrown into question (Jones et al., 2010). Vulnerability of the exclusion approach against typing errors was counteracted here by a strict regime of genotype calling. Effectively, the rate of confident somatic mutations was found quite low (6.6 × 10–4 per offspring and marker), and the validation procedure for these mutations helped to narrow down estimates for typing uncertainties to the same frequency level.
Except the paternity study of Faulkes et al. (1997) no further parentage or extra-colony paternity investigations in NMR have been published. Another group, however, reported that in five wild colonies 5–8 microsatellite alleles were detected at one or more loci, which strongly suggests that these wild colonies contained individuals from more than a single breeding pair and inter-colony exchange of genetic material may occur (Ingram et al., 2015). Evidence of multiple queens in NMR colonies has been reported both in captivity and in the wild (Jarvis, 1991). These queens may simultaneously breed for some years, but the scenario usually is of shorter duration and typically leads to serious fightings, mortalities and low pup survival (Jarvis, 1991; Smith and Buffenstein, 2021). In established colonies, however, and multiple queens are a quite rare event in captive and wild NMR colonies (Braude, 1991; Jarvis, 1991). Further, based on long-term studies of 16 wild colonies of marked NMR, it has been observed that nascent colonies arise either via pairing of single mole-rats from different natal colonies or from small groups of males and females from different or same natal colonies (Braude, 2000). Inter-colony invasions, colony splitting and kidnapping have been observed among wild and captive NMR colonies, too (Lacey and Sherman, 2007; Braude et al., 2021) (S. Braude, personal communication). Independent studies on captive (O’Riain et al., 1996; Clarke and Faulkes, 1999; Ciszek, 2000) and wild NMR populations (Braude, 2000) demonstrated that outbreeding with unrelated mates is preferred in this species. Some authors therefore suggested that dispersing mole-rats would attempt to join established colonies (O’Riain et al., 1996). Although this may contribute to colony gene flow, it was demonstrated by a long-term recapture study that successful dispersal of individuals is rare and most of the nascent colonies did not appear to persist for more than 1 year (Braude, 2000). Laboratory and field studies further showed that NMR recognize colony members and aggressively discriminate against foreign conspecifics, which often results in the death of the mole-rat(s) (Lacey and Sherman, 1991; O’Riain and Jarvis, 1997) (and own observation). However, of note is one documented case in which a dispersing male successfully immigrated into an established colony and took over the breeding male position (Braude, 2000).
Some other traits have been used to infer monogamy in laboratory studies (Dewsbury, 1981), and these may serve as indicators to corroborate monogamy as a characteristic of the NMR mating system. To begin with, NMR breeding pairs are known to form stable, long-term partnerships for many years (Jarvis, 1991; Lacey and Sherman, 1991), and cooperative care for offspring was already highlighted as a social trait. In addition, subordinate individuals are physiologically suppressed by the queen and offspring exhibit a delayed onset in the sexual maturation (Jarvis, 1991; Buffenstein, 2005). In contrast to other related social mole-rat species, e.g. Fukomys, the NMR is sexually monomorphic and exhibits a balanced sex ratio on the colony level (Burda, 1990; Jarvis, 1991; Chau et al., 2018), which is prototypic for monogamous mating systems. Another, more specific characteristic is the condition of the male genitals in this species. The testes of both, breeding male and subordinates, are relatively small and intra-abdominal with only a little storage capacity for spermatozoa, a small baculum without a dorsal groove, no spines on the glans penis for vaginal anchoring (van der Horst et al., 2021), and no forming of copulatory plugs altogether indicate a low risk of sperm competition in this species (Seney et al., 2009). Fighting and aggression between NMR males is generally very rare and particularly do not occur during the queen’s estrus (Jarvis, 1991; Lacey and Sherman, 1991; Clarke and Faulkes, 1998). This is in contrast to other social mole-rats, e.g., the Zambian mole-rat, where copulation often takes place irrespective of the females’ reproductive cycle (Burda, 1990). In the NMR mating is found to be a rare event (Goldman et al., 2006) (and own observation) and the breeding male copulates almost during the entire estrus (2–24 h) of the queen.
To summarize, the genetic paternity results from this study together with a number of specific biological traits of the NMR consistently support the model that the preferred sexual mating system in this cooperatively breeding species is monogamy. In order to refine this model, and to resolve the underlying mechanisms for general and exceptional behavior, we would like to encourage further kinship studies on NMR colonies, particularly in the wild.
Data Availability Statement
The original contributions presented in the study are included in the article/Supplementary Material, further inquiries can be directed to the corresponding author.
Ethics Statement
The animal study was reviewed and approved by the Landesamt für Gesundheit und Soziales Berlin, Germany.
Author Contributions
KS, AL, MP, and TH designed the experiment. KS, MW, SH, IB, and DL performed the experiments, acquired, and assembled the data. KS and MW drafted the manuscript. All authors contributed to data analysis and input or discussion.
Funding
This work was supported by the Leibniz Association (SAW-2012-FLI-2).
Conflict of Interest
The authors declare that the research was conducted in the absence of any commercial or financial relationships that could be construed as a potential conflict of interest.
Publisher’s Note
All claims expressed in this article are solely those of the authors and do not necessarily represent those of their affiliated organizations, or those of the publisher, the editors and the reviewers. Any product that may be evaluated in this article, or claim that may be made by its manufacturer, is not guaranteed or endorsed by the publisher.
Acknowledgments
We thank Ivonne Görlich and Jette Ziep for expert technical assistance, Anton Weissenbacher (Vienna Zoo, Schönbrunn) for access to the two naked mole-rat colonies, as well as valuable comments, Manfred Kayser (Erasmus University Rotterdam, Rotterdam, Netherlands) for helpful discussions. We also thank two referees for their useful comments.
Supplementary Material
The Supplementary Material for this article can be found online at: https://www.frontiersin.org/articles/10.3389/fevo.2022.855688/full#supplementary-material
Supplementary Data File 1 | Genotyping data of colony B1, Cervus format.
Supplementary Data File 2 | Genotyping data of colony B2, Cervus format.
Supplementary Data File 3 | Genotyping data of colony V1, Cervus format.
Supplementary Data File 4 | Genotyping data of colony V2, Cervus format.
Abbreviations
NMR, naked mole-rat.
References
Bourke, A. F. (2014). Hamilton’s rule and the causes of social evolution. Philos. Trans. R. Soc. Lond. B. Biol. Sci. 369:20130362. doi: 10.1098/rstb.2013.0362
Braude, S. (1991). The Behaviour and Demographics of the Naked Mole-Rat. Ann Arbor: University of Michigan.
Braude, S. (2000). Dispersal and new colony formation in wild naked mole-rats: evidence against inbreeding as the system of mating. Behav. Ecol. 11, 7–12. doi: 10.1093/beheco/11.1.7
Braude, S., Ciszek, D., Berg, N. E., and Shefferly, N. (2001). The ontogeny and distribution of countershading in colonies of the naked mole-rat (Heterocephalus glaber). J. Zool. 253, 351–357. doi: 10.1017/s0952836901000322
Braude, S., Hess, J., and Ingram, C. (2021). Inter-colony invasion between wild naked mole-rat colonies. J. Zool. 313, 37–42. doi: 10.1111/jzo.12834
Brett, R. A. (1986). The Ecology and Behaviour of the Naked Mole-Rat, Heterocephalus Glaber Ruppell (Rodenti: Bathyergidae). London: University College London.
Brett, R. A. (1991). “The population structure of naked mole-rat colonies,” in The Biology of the Naked mole-Rat, eds P. W. Sherman, J. U. M. Jarvis, and R. D. Alexander (Princeton NJ: Princeton University Press), 97–136. doi: 10.1515/9781400887132-007
Buffenstein, R. (2005). The naked mole-rat: a new long-living model for human aging research. J. Gerontol. A Biol. Sci. Med. Sci. 60, 1369–1377. doi: 10.1093/gerona/60.11.1369
Buffenstein, R., Amoroso, V., Andziak, B., Avdieiev, S., Azpurua, J., Barker, A. J., et al. (2022). The naked truth: a comprehensive clarification and classification of current ‘myths’ in naked mole-rat biology. Biol. Rev. 97, 115–140. doi: 10.1111/brv.12791
Burda, H. (1990). Constraints of pregnancy and evolution of sociality in mole-rats With special reference to reproductive and social patterns in Cryptomys hottentotus (Bathyergidae, Rodentia)1. J. Zool. Syst. Evol. Res. 28, 26–39. doi: 10.1111/j.1439-0469.1990.tb00362.x
Chau, L. M., Groh, A. M., Anderson, E. C., Alcala, M. O., Mendelson, J. R. III, Slade, S. B., et al. (2018). Genetic diversity and sex ratio of naked mole rat. Heterocephalus glaber, zoo populations. Zoo. Biol. 37, 171–182. doi: 10.1002/zoo.21417
Ciszek, D. (2000). New colony formation in the “highly inbred” eusocial naked mole-rat: outbreeding is preferred. Behav. Ecol. 11, 1–6. doi: 10.1093/beheco/11.1.1
Clarke, F. M., and Faulkes, C. G. (1998). Hormonal and behavioural correlates of male dominance and reproductive status in captive colonies of the naked mole-rat, Heterocephalus glaber. Proc. Biol. Sci. 265, 1391–1399. doi: 10.1098/rspb.1998.0447
Clarke, F. M., and Faulkes, C. G. (1999). Kin discrimination and female mate choice in the naked mole-rat Heterocephalus glaber. Proc. Biol. Sci. 266, 1995–2002. doi: 10.1098/rspb.1999.0877
Dewsbury, D. A. (1981). An exercise in the prediction of monogamy in the field from laboratory data on 42 species of muroid rodents. Biologist 63, 138–162.
Edwards, P. D., Arguelles, D. A., Mastromonaco, G. F., and Holmes, M. M. (2021). Queen Pregnancy Increases Group Estradiol Levels in Cooperatively Breeding Naked Mole-Rats. Integr. Comp. Biol. 61, 1841–1851. doi: 10.1093/icb/icab106
Fang, X., Seim, I., Huang, Z., Gerashchenko, M. V., Xiong, Z., Turanov, A. A., et al. (2014). Adaptations to a subterranean environment and longevity revealed by the analysis of mole rat genomes. Cell Rep. 8, 1354–1364. doi: 10.1016/j.celrep.2014.07.030
Faulkes, C. G., and Abbott, D. H. (1991). Social control of reproduction in breeding and non-breeding male naked mole-rats (Heterocephalus glaber). J. Reprod. Fert. 93, 427–435. doi: 10.1530/jrf.0.0930427
Faulkes, C. G., and Abbott, D. H. (1993). Evidence that primer pheromones do not cause social suppression of reproduction in male and female naked mole-rats (Heterocephalus glaber). J. Reprod. Fertil. 99, 225–230. doi: 10.1530/jrf.0.0990225
Faulkes, C. G., Abbott, D. H., O’Brien, H. P., Lau, L., Roy, M. R., Wayne, R. K., et al. (1997). Micro- and macrogeographical genetic structure of colonies of naked mole-rats Heterocephalus glaber. Mol. Ecol. 6, 615–628. doi: 10.1046/j.1365-294x.1997.00227.x
Faulkes, C. G., and Bennett, N. C. (2021). Social Evolution in African Mole-Rats - A Comparative Overview. Adv. Exp. Med. Biol. 1319, 1–33. doi: 10.1007/978-3-030-65943-1_1
Gagneux, P., Boesch, C., and Woodruff, D. S. (1999). Female reproductive strategies, paternity and community structure in wild West African chimpanzees. Anim. Behav. 57, 19–32. doi: 10.1006/anbe.1998.0972
Garcia Montero, A., Vole, C., Burda, H., Malkemper, E. P., Holtze, S., Morhart, M., et al. (2016). Non-breeding eusocial mole-rats produce viable sperm-spermiogram and functional testicular morphology of Fukomys anselli. PLoS One 11:e0150112. doi: 10.1371/journal.pone.0150112
Goldman, S. L., Forger, N. G., and Goldman, B. D. (2006). Influence of gonadal sex hormones on behavioral components of the reproductive hierarchy in naked mole-rats. Horm. Behav. 50, 77–84. doi: 10.1016/j.yhbeh.2006.01.013
Goossens, B., Graziani, L., Waits, L. P., Farand, E., Magnolon, S., Coulon, J., et al. (1998). Extra-pair paternity in the monogamous Alpine marmot revealed by nuclear DNA microsatellite analysis. Behav. Ecol. Sociobiol. 43, 281–288. doi: 10.1007/s002650050492
Ingram, C. M., Troendle, N. J., Gill, C. A., Braude, S., and Honeycutt, R. L. (2015). Challenging the inbreeding hypothesis in a eusocial mammal: population genetics of the naked mole-rat, Heterocephalus glaber. Mol. Ecol. 24, 4848–4865. doi: 10.1111/mec.13358
Ingram, C. M., Troendle, N. J., Gill, C. A., and Honeycutt, R. L. (2014). Development of 12 new microsatellite markers for the naked mole-rat. Heterocephalus glaber. Conserv. Gen. Resour. 6, 589–591. doi: 10.1007/s12686-014-0147-2
Jarvis, J. U., O’Riain, M. J., Bennett, N. C., and Sherman, P. W. (1994). Mammalian eusociality: a family affair. Trends Ecol. Evol. 9, 47–51. doi: 10.1016/0169-5347(94)90267-4
Jarvis, J. U. M. (1991). “Reproduction of naked mole-rats,” in The Biology of the Naked Mole-Rat, eds P. W. Sherman, J. U. M. Jarvis, and R. D. Alexander (Princeton, NJ: Princeton University Press), 384–425. doi: 10.1007/978-3-030-65943-1_3
Jones, A. G., Small, C. M., Paczolt, K. A., and Ratterman, N. L. (2010). A practical guide to methods of parentage analysis. Mol. Ecol. Resour. 10, 6–30. doi: 10.1111/j.1755-0998.2009.02778.x
Kalinowski, S. T., Taper, M. L., and Marshall, T. C. (2007). Revising how the computer program CERVUS accommodates genotyping error increases success in paternity assignment. Mol. Ecol. 16, 1099–1106. doi: 10.1111/j.1365-294X.2007.03089.x
Katsushima, K., Nishida, C., Yosida, S., Kato, M., Okanoya, K., and Matsuda, Y. (2010). A multiplex PCR assay for molecular sexing of the naked mole-rat (Heterocephalus glaber). Mol. Ecol. Resour. 10, 222–224. doi: 10.1111/j.1755-0998.2009.02742.x
Kim, E. B., Fang, X., Fushan, A. A., Huang, Z., Lobanov, A. V., Han, L., et al. (2011). Genome sequencing reveals insights into physiology and longevity of the naked mole rat. Nature 479, 223–227. doi: 10.1038/nature10533
Lacey, E., and Sherman, P. (1991). “Social organization of naked mole rat colonies: evidence for divisions of labor,” in The Biology of the Naked Mole-Rat, eds P. W. Sherman, J. U. M. Jarvis, and R. D. Alexander (Princeton, NJ: Princeton University Press), 275–336. doi: 10.1515/9781400887132-013
Lacey, E., and Sherman, P. (2007). “Cooperative breeding in naked mole-rats: Implications for vertebrate and invertebrate sociality,” in Cooperative Breeding in Mammals, eds N. G. Solomon and J. A. French (Cambridge, UK: Cambridge University Press), 267–301. doi: 10.1017/cbo9780511574634.011
Lacey, E. A., and Sherman, P. W. (1997). Cooperative breeding in naked mole-rats: implications for vertebrate and invertebrate sociality. doi: 10.1017/cbo9780511574634.011
Lee, B. P., Smith, M., Buffenstein, R., and Harries, L. W. (2020). Negligible senescence in naked mole rats may be a consequence of well-maintained splicing regulation. Geroscience 42, 633–651. doi: 10.1007/s11357-019-00150-7
Leese, F., Mayer, C., and Held, C. (2008). Isolation of microsatellites from unknown genomes using known genomes as enrichment templates. Limnol. Oceanogr. 6, 412–426. doi: 10.4319/lom.2008.6.412
Lukas, D., and Clutton-Brock, T. (2012). Cooperative breeding and monogamy in mammalian societies. Proc. Biol. Sci. 279, 2151–2156. doi: 10.1098/rspb.2011.2468
Molecular Ecology Resources Primer Development, Andree, K., Axtner, J. A. N., Bagley, M. J., et al. (2010). Permanent Genetic Resources added to Molecular Ecology Resources Database 1 April 2010 – 31 May 2010. Mol. Ecol. Resour. 10, 1098–1105. doi: 10.1111/j.1755-0998.2010.02898.x
Nolte, A. W., Stemshorn, K. C., and Tautz, D. (2005). Direct cloning of microsatellite loci from Cottus gobio through a simplified enrichment procedure. Mol. Ecol. Notes 5, 628–636. doi: 10.1111/j.1471-8286.2005.01026.x
O’Riain, M. J., Jarvis, J. U., and Faulkes, C. G. (1996). A dispersive morph in the naked mole-rat. Nature 380, 619–621. doi: 10.1038/380619a0
O’Riain, M. J., and Jarvis, J. U. M. (1997). Colony member recognition and xenophobia in the naked mole-rat. Anim. Behav. 53, 487–498. doi: 10.1006/anbe.1996.0299
Park, T. J., Smith, E. S. J., Reznick, J., Bennett, N. C., Applegate, D. T., Larson, J., et al. (2021). African Naked Mole-Rats Demonstrate Extreme Tolerance to Hypoxia and Hypercapnia. Adv. Exp. Med. Biol. 1319, 255–269. doi: 10.1007/978-3-030-65943-1_9
Reeve, H. K., Westneat, D. F., Noon, W. A., Sherman, P. W., and Aquadro, C. F. (1990). DNA “fingerprinting” reveals high levels of inbreeding in colonies of the eusocial naked mole-rat. Proc. Natl. Acad. Sci. U.S.A 87, 2496–2500. doi: 10.1073/pnas.87.7.2496
Roellig, K., Drews, B., Goeritz, F., and Hildebrandt, T. B. (2011). The long gestation of the small naked mole-rat (Heterocephalus glaber Ruppell, 1842) studied with ultrasound biomicroscopy and 3D-ultrasonography. PLoS One 6:e17744. doi: 10.1371/journal.pone.0017744
Seluanov, A., Gladyshev, V. N., Vijg, J., and Gorbunova, V. (2018). Mechanisms of cancer resistance in long-lived mammals. Nat. Rev. Cancer 18, 433–441. doi: 10.1038/s41568-018-0004-9
Seney, M. L., Kelly, D. A., Goldman, B. D., Sumbera, R., and Forger, N. G. (2009). Social structure predicts genital morphology in African mole-rats. PLoS One 4:e7477. doi: 10.1371/journal.pone.0007477
Smith, E. S., Omerbasic, D., Lechner, S. G., Anirudhan, G., Lapatsina, L., and Lewin, G. R. (2011). The molecular basis of acid insensitivity in the African naked mole-rat. Science 334, 1557–1560. doi: 10.1126/science.1213760
Smith, M., and Buffenstein, R. (2021). Managed Care of Naked Mole-Rats. Adv. Exp. Med. Biol. 1319, 381–407. doi: 10.1007/978-3-030-65943-1_16
Sparkman, A. M., Adams, J., Beyer, A., Steury, T. D., Waits, L., and Murray, D. L. (2011). Helper effects on pup lifetime fitness in the cooperatively breeding red wolf (Canis rufus). Proc. Roy. Soc. Lond. B 278, 1381–1389. doi: 10.1098/rspb.2010.1921
Thigpen, L. W. (1940). Histology of the skin of a normally hairless rodent. J. Mammal. 21, 449–456. doi: 10.2307/1374885
Tian, X., Azpurua, J., Hine, C., Vaidya, A., Myakishev-Rempel, M., Ablaeva, J., et al. (2013). High-molecular-mass hyaluronan mediates the cancer resistance of the naked mole rat. Nature 499, 346–349. doi: 10.1038/nature12234
van der Horst, G., Kotze, S., O’Riain, M. J., Muller, N., and Maree, L. (2021). A possible highway system for the rapid delivery of sperm from the testis to the penis in the naked mole-rat. Heterocephalus glaber. J. Morphol. 282, 1478–1498. doi: 10.1002/jmor.21399
Van der Westhuizen, L. A., Jarvis, J. U. M., and Bennett, N. C. (2013). A case of natural queen succession in a captive colony of naked mole-rats. Heterocephalus glaber. Afr. Zool. 48, 56–63. doi: 10.3377/004.048.0119
Vigilant, L., Hofreiter, M., Siedel, H., and Boesch, C. (2001). Paternity and relatedness in wild chimpanzee communities. Proc. Natl. Acad. Sci. U.S.A. 98, 12890–12895. doi: 10.1073/pnas.231320498
Keywords: naked mole-rat, cooperative breeding, monogamy, polyandry, kinship analysis, microsatellite marker
Citation: Szafranski K, Wetzel M, Holtze S, Büntjen I, Lieckfeldt D, Ludwig A, Huse K, Platzer M and Hildebrandt T (2022) The Mating Pattern of Captive Naked Mole-Rats Is Best Described by a Monogamy Model. Front. Ecol. Evol. 10:855688. doi: 10.3389/fevo.2022.855688
Received: 15 January 2022; Accepted: 07 April 2022;
Published: 11 May 2022.
Edited by:
Ann Valerie Hedrick, University of California, Davis, United StatesReviewed by:
Melissa Holmes, University of Toronto Mississauga, CanadaDustin J. Penn, University of Veterinary Medicine, Vienna, Austria
Copyright © 2022 Szafranski, Wetzel, Holtze, Büntjen, Lieckfeldt, Ludwig, Huse, Platzer and Hildebrandt. This is an open-access article distributed under the terms of the Creative Commons Attribution License (CC BY). The use, distribution or reproduction in other forums is permitted, provided the original author(s) and the copyright owner(s) are credited and that the original publication in this journal is cited, in accordance with accepted academic practice. No use, distribution or reproduction is permitted which does not comply with these terms.
*Correspondence: Karol Szafranski, a2Fyb2wuc3phZnJhbnNraUBsZWlibml6LWZsaS5kZQ==
†These authors have contributed equally to this work