- 1Institute of Environmental Sciences, Faculty of Biology, Jagiellonian University, Kraków, Poland
- 2Nencki Institute of Experimental Biology, Polish Academy of Sciences, Warsaw, Poland
Animal life requires hard work but the ability to endure such workload appears to be limited. Heat dissipation limit (HDL) hypothesis proposes that the capacity to dissipate the excess of body heat during hard work may limit sustained energy use. Experimental facilitations of heat loss rate via feather-clipping in free-living birds seem to support HDL hypothesis but testing of HDL through laboratory experiments under controlled conditions is not reported. We employed a two-factorial experimental design to test HDL hypothesis by manipulating the capacity to dissipate heat through exposure of captive zebra finches (Taeniopygia guttata) to a cold and warm ambient temperature (14°C and 25°C), and through manipulation of the insulating layer of feathers around the brood patch in females (clipped and unclipped). To simulate foraging costs encountered in the wild we induced foraging effort by employing a feeding system that necessitated hovering to access food, which increased energetic costs of reproduction despite ad libitum conditions in captivity. We quantified the outcome of reproductive performance at the level of both parents, females, and offspring. Thermal limitations due to warm temperature appeared at the beginning of reproduction for both parents with lower egg-laying success, smaller clutch size and lower egg mass, compared to the cold. After hatching, females with an enhanced ability to dissipate heat through feather-clipping revealed higher body mass compared to unclipped females, and these clipped females also raised heavier and bigger nestlings. Higher levels for oxidative stress in plasma of females were detected prior to reproduction in warm conditions than in the cold. However, oxidative stress biomarkers of mothers were neither affected by temperature nor by feather-clipping during the reproductive activities. We document upregulation of antioxidant capacity during reproduction that seems to prevent increased levels of oxidative stress possibly due to the cost of female body condition and offspring growth. Our study on reproduction under laboratory-controlled conditions corroborates evidence in line with the HDL hypothesis. The link between temperature-constrained sustained performance and reproductive output in terms of quality and quantity is of particular interest in light of the current climate change, and illustrates the emerging risks to avian populations.
Introduction
Energy is fundamental for life, its availability and use determines the reproductive success and survival of organisms (Carey, 1996). Along the annual cycle, animals often face challenging conditions requiring particularly high levels of energy. During reproduction for instance, adults spend an increased amount of energy for a sustained period to establish a territory, find a mate, build a nest, lay and incubate eggs, and finally raise their offspring (Drent and Daan, 1980). While it is generally accepted that the rate at which animals can expend energy is limited we lack detailed understanding of such limitations (Drent and Daan, 1980; Peterson et al., 1990; Weiner, 1992; Hammond and Diamond, 1997; Speakman and Król, 2010a). Several hypotheses suggest that energy limitations can be imposed by either external (e.g., food availability; Speakman et al., 2003) or internal physiological and morphological factors (Drent and Daan, 1980; Hammond and Diamond, 1997; West et al., 1999). For instance, the capacity to process and digest the incoming energy (“central limitation hypothesis,” Drent and Daan, 1980; Koteja, 1996; Thurber et al., 2019), to deliver nutrients to organs and tissues limits energy (“metabolic theory of ecology,” West et al., 1999), or even the working capacity of the organs themselves (“peripheral limitation hypothesis,” Hammond and Diamond, 1997), may constrain maximum and sustained energy expenditure. Lately, it has been proposed that the capacity to dissipate the excess of body heat may limit the use of energy, forwarded as the heat dissipation limit (HDL) hypothesis (Król et al., 2007; Speakman and Król, 2010b).
First observations of cold exposure as experimental manipulation that enhances the rate heat is dissipated from the body revealed that the capacity to thermoregulate in order to avoid overheating may constrain reproductive performance and thus optimization of energy use (Johnson and Speakman, 2001; Król and Speakman, 2003). When exposed to a cold ambient temperature of 8°C during lactation (i.e., the most energetic demanding phase) mice (outbred MF1, Mus musculus) increased food intake, produced more milk and raised heavier offspring compared to mice exposed to a higher ambient temperature of 21°C (Johnson and Speakman, 2001; Król et al., 2007). Avian heat production during reproduction seems less directly affected as in mammals during lactation, but chick-rearing requires an intensive physical workload for food provisioning. Zebra finches (Taeniopygia guttata) raised offspring heavier in mass and a more advanced in structural size (tarsus length at day 28 post hatching) when exposed to a cold temperature of 14°C than those, exposed to 30°C (Andrew et al., 2017) a temperature considered within the thermoneutral zone (TNZ in zebra finches varies from 30°C to 38°C; Calder, 1964). Further studies have provided support for HDL hypothesis by manipulating and enhancing heat dissipation via decrease of insulation, either through fur removal in mammals (Król et al., 2007), or feather-clipping in birds (Nilsson and Nord, 2018). Increased ability to dissipate heat via a feather-clipping in female blue tits (Cyanistes caeruleus) seemed to have relaxed energetic limitations compared to un-manipulated control birds (Nilsson and Nord, 2018); feather-clipped females could minimize body mass loss compared to controls and also raised heavier nestlings (Nilsson and Nord, 2018). Feather-clipped tree swallows (Tachycineta bicolor) raised nestlings with higher body mass and maintained higher workload and lower day-time body temperature, compared to controls (Tapper et al., 2020a). Those effects of ambient temperature and treatment with increased heat loss rate in both mammals and birds seem to be consistent with HDL hypothesis in endotherms (although see Thurber et al., 2019 for humans, Rogowitz, 1998; Yang et al., 2013), resulting in high reproductive performance and output. So far, evidence supporting HDL hypothesis in birds exists only under field conditions while laboratory studies in birds are yet to be implemented. Understanding the costs of oxidative stress associated with thermal limitations, as well as the costs associated with avoiding oxidative stress during periods of high workload, is also required.
Aerobic metabolism results in reactive oxygen species (ROS) production, and any imbalance between ROS and the antioxidant defense mechanism toward ROS may come with a consequence of oxidative stress damaging biomolecules (Sies, 1997; Finkel and Holbrook, 2000; Alonso-Alvarez et al., 2006; Rahal et al., 2014). However, organisms may employ different ways to maintain oxidative balance. The first line of protection tries to minimize ROS production within cells either through membrane composition or uncoupling of oxygen consumption and ATP production (Monaghan et al., 2009; Alan and McWilliams, 2013). Antioxidant defense system comes as a second line with antioxidants to counteract ROS and plays a significant role in protection against oxidative stress (Halliwell and Gutteridge, 1999; Garratt et al., 2011; Speakman and Selman, 2011; Yang et al., 2013; Brzęk et al., 2014). Antioxidants may occur in enzymatic and non-enzymatic form in the intracellular and extracellular environment (Nimse and Pal, 2015). Maintaining oxidative balance might be a challenge when organisms face metabolic changes due to exposure to low or high ambient temperatures or increased physical activities during reproduction. For instance, current evidence shows that breeding adults are rather confronted with a reduced amount of antioxidant capacity (Wiersma et al., 2004; Losdat et al., 2011; van de Crommenacker et al., 2012; Costantini et al., 2014a) implying perhaps that breeding adults may not be able to invest enough for self-maintenance (i.e., by self-feeding and hence increasing antioxidant capacity with exogenous antioxidants) or reflecting the antioxidant response to oxidative stress (Costantini, 2011). On the other hand, oxidative damage is not always detected due to elevated reproductive performance in both birds and mammals (Ołdakowski et al., 2012; Brzęk et al., 2014; Costantini et al., 2014b). Exposure to high ambient temperature may also cause a series of physiological costs, such as increased body temperature and metabolic rate and oxidative stress (Lin et al., 2006; Mestre-Alfaro et al., 2012; Khan et al., 2021). Given the suite of benefits of experimentally enhancing the excess of body heat during reproduction, it is reasonable to suggest simultaneous benefits for self-maintenance investing toward current reproduction. To the best of our knowledge, there are only a few studies investigating the consequences of ambient temperature in oxidative stress during reproduction (lactating Mongolian gerbils; Yang et al., 2013, see review in broiler chickens; Khan et al., 2021), and no study has been conducted until now to investigate the benefits or costs in terms of oxidative stress under experimental facilitations of the capacity to dissipate heat in endotherms during hard work.
To test HDL hypothesis, we employed two separate manipulations of the capacity to dissipate heat in captive zebra finches (Taeniopygia guttata). In a two-factorial experimental design, we manipulated the capacity to dissipate heat through exposure of zebra finches to cold and a warm ambient temperature (factor I, 14°C and 25°C respectively; established 1 month before hatching), and through manipulation of the insulating layer of feathers around the brood patch (factor II, feather-clipped versus unclipped; applied on day 6 post-hatching). Initially, we estimated the effects of ambient temperature on both parents by following egg-laying and egg production. Female reproductive performance was determined by measuring body mass and food intake during offspring provisioning. Additional maternal measurements of several oxidative stress biomarkers were implemented to increase our knowledge on the potential costs or benefits when removing the problem of heat dissipation. Reproductive output was estimated by measuring body mass of the nestlings several times along their development, tarsus (an indicator of skeletal development) and wing length (a predictor for bone development and feather growth) when nestlings reached almost a fully juvenile size (35 days old). If indeed the metabolic performance is limited by the capacity to dissipate body heat, we formulated several predictions: (a) feather-clipped females will show higher body mass and food intake than unclipped females, (b) the enhanced ability to dissipate heat will enable females to invest more in self-maintenance resulting in lower oxidative stress than the unclipped controls, and this effect will be more pronounced in warm compared to cold conditions, (c) females with increased heat loss rate (either through cold exposure or feather-clip treatment) will raise heavier and more developed nestlings than the unclipped control females.
Materials and Methods
Individuals and Temperature Set-Up
For this study, we applied two separate manipulations of the capacity to dissipate heat using captive zebra finches (Taeniopygia guttata) in a two-factorial experimental design. Temperature manipulations influence the capacity to transfer body heat since heat loss depends on the difference between the body and the ambient temperature (Speakman and Król, 2010b), and thus, before reproduction, we exposed total 82 pairs of birds to 14°C and 25°C with 39 and 43 pairs, respectively (factor I). Both temperatures are considered below the thermoneutral zone (TNZ) in zebra finches, which ranges from 30°C to 38°C (Calder, 1964; Briga and Verhulst, 2017). In the breeding season, zebra finches under natural conditions may experience temperatures ranging from 10°C to 30°C (even in some cases above 36°C) but most frequently they lay eggs at ambient temperatures of 18°C to 20°C (Griffith et al., 2017). In laboratory conditions, ambient temperature for breeding zebra finches is set from 18°C to 22°C (Rutkowska et al., 2005; Williamson et al., 2008; Arct et al., 2010; Olson et al., 2014) for high reproductive success. For this reason, we applied two ambient temperatures with a difference of at least ten degrees, one below 18°C and one above 22°C, considering them as cold and warm, respectively. We monitored egg-laying success and hatching success between the two ambient temperatures. About 82% of the 39 pairs (32 out of 39) laid eggs in the cold conditions, while about 51% of the 43 pairs (22 out of 43) laid eggs in warm conditions. From those pairs that laid eggs, a total of 26 out of 32 chicks hatched in cold conditions and 18 out of 22 in the warm (χ2 = 0.002, p = 0.95). During reproduction, and more specifically on day 6 post-hatching, we applied our second manipulation of heat dissipation via manipulation of the insulating layer of feathers around the brood patch (factor II, feather-clipped versus unclipped females). At the end, 26 out of 26 pairs successfully raised fully developed chicks until independence at the cold conditions (14 feather-clipped, 12 unclipped), while 14 pairs out of 18 raised fully developed chicks at the warm (7 feather-clipped, 7 unclipped) (χ2 = 6.35, p = 0.01).
In each chamber, the ambient temperature was recorded with six data loggers (thermochrons, DS1921H-F5, iButtonLink, Maxim Integrated products, United States) and the humidity with one additional (hygrochron, DS1923-F5, iButtonLink, Maxim Integrated products, United States) to monitor and maintain the ambient temperature and humidity within established levels. All data loggers were synchronized at the same time to record three times when lights were on (“daytime”) and three times when lights off (“nighttime”). Temperature significantly differed between daytime and nighttime for both chambers (F1,7789 = 6.03, p < 0.001). Humidity significantly differed between daytime and nighttime (F1,940 = 46.71, p < 0.001) but it did not differ between the two chambers (F1,940 = 0.001, p = 0.97). The summary of the statistics of temperature and humidity separated for day and night for each chamber is shown in Supplementary Table 1.
Acclimation Period
Our laboratory colony of zebra finches is usually habituated in a common outdoor aviary at the Institute of Environmental Sciences, Jagiellonian University, Krakow. Thus, at the beginning of the experiment we moved the experimental birds and separated them into the two indoor climatic chambers. Birds were initially housed in individual cages (70 × 70 × 45 cm; L × W × H) with two birds of the same sex in each cage and visually separated from other birds. We let birds to acclimatize for 2 weeks at the new laboratory conditions with a photoperiod 13:11 (L:D) with lights on at 7 AM and lights off at 8 PM. After 2 weeks of acclimation, we implemented a new feeding system for every cage to increase physical activity and foraging costs for all birds (see details below). The training procedure lasted for 2 months. When all birds were trained to the new feeding system, we left them for 3 weeks at the ambient temperatures of 14°C and 25°C before the mating (see a summary of the experimental timeline in Supplementary Figure 1).
For this experiment, we implemented a feeding system for every cage comparable to the “high foraging cost feeders” of Koetsier and Verhulst (2011). Such high foraging costs feeders may increase foraging effort by increasing time for foraging and the flights for obtaining food as it was previously shown for zebra finches (Koetsier and Verhulst, 2011; Yap et al., 2017), simulating foraging costs in field conditions. Female zebra finches revealed to have increased flight muscle mass, lung mass and heart mass in response to high foraging cost feeders compared to females exposed to regular feeders (although no differences were detected in males, Yap et al., 2017). Therefore, we constructed a similar feeding system using a transparent plastic feeder and opened one hole fitted with a tube. The feeder was mounted 40 cm from the floor of the cage and initially a wooden stick of 15 cm was attached for perching. After 4 days, we gradually shortened the wooden stick size down to 7 cm. Birds continued to have access to food by perching on the wooden stick for a total of 9 days, thereafter which we removed the perch to start training the birds to fly toward the foraging hole and hover briefly to collect the seeds (see Supplementary Figure 2 and uploaded video at Supplementary Material). On the floor of the cage, below the feeder, a plastic container and a covered glass tube collected all the spilled seeds. This prevented the birds to collect any spilled seeds on the floor and the containers were emptied every second day. During the whole experiment and reproduction, all birds had unlimited access to food (a mixture of different millet species; Megan, Poland), but as outlined we did increase their physical exercise for foraging to simulate natural conditions. Birds were trained to the new high foraging cost system for 12 days. For more information of the training procedure and the hovering behavior of the birds, see Supplementary Material.
Experimental Procedure
After the training to the new feeding system and 3 additional weeks of acclimation period, we randomly mated the birds with unrelated partners and randomly separated the pairs in four different blocks. Each block was assigned for mating with 4 days of interval. On the mating day, each individual cage was equipped with an internal carton-made nest box and nest-material (wood wool and shredded toilet paper) which was replaced every day until birds stopped using it. After the mating, nest boxes were checked every morning between 10 AM and 11 AM in order to inspect the nest-building stage, the egg laying date (females lay one egg per day early in the morning) and the start of incubation. During the egg-laying period, we marked all eggs alphabetically with a pen, in the sequence they were laid, and each was weighed with an electronic balance (Kern MM 60 2N ± 0.01, Kern & Sohn GmbH, Germany). When incubation started, birds were supplied three times per week with a small spoon of a mix of chopped hard boiled eggs (with the shell), grated carrot and supplementary vitamins. Birds also had unlimited access to water and to a piece of cuttlebone for the entire period.
Adult Measurements
Just before mating, we caught all birds early in the morning to measure initial body mass using an electronic balance (±0.1 g; KERN 440-45N, Kern & Sohn, GmbH, Germany). When first eggs hatched, which we assigned as day 0, we also measured female body mass just after lights on, and repeated the body mass measurement on day 4, 6, 8, 10, 12, 16, and day 35 (see also Supplementary Figures 1, 5). At the same time that we caught each female from the cage, we also measured nestlings to follow growth rate (more details on nestling measurements see below). On day 4, we removed the male from the cage to increase the physical activity of the female, assuring that the reproductive effort is only operated from a single parent. On day 6, we applied the feather clip manipulation by trimming the feathers around the brood patch and those covering the pectoral muscles. We established two manipulation groups: feather-clipped and unclipped females, which were handled and measured in the same way but not feather-clipped (sham-manipulation). On day 12, just before lights off, we captured the females from inside the nest-box and we performed respiratory measurements to estimate thermal conductance of the females (for more details see Supplementary Material). Feather-clipped females revealed significantly higher thermal conductance, hence heat loss (up to 9.5% increase), than the unclipped ones (Supplementary Figure 4). Last, females were blood-sampled three times during the experiment: (a) just before mating (after taking initial body mass), (b) on day 13 just after respiratory measurements, and (c) at the end of the experiment, on day 35, when chicks reached full independence.
Nestlings
During the expected hatching date, we monitored the nests every day between 10 AM and 11 AM to inspect for possible newly fresh hatchlings. We distinguished day of hatching based on the presence of eggshells’ leftovers and the appearance of the hatchlings, since fresh hatchlings are reddish and wet. The true hatching date was recorded as day 0 and the true age of each hatchling was assigned accordingly. We immediately weighed each hatchling with an electronic balance (Kern MM 60 2N ± 0.01, Kern & Sohn GmbH, Germany), marked them by nail clipping their claw and returned them to the nest. Body mass of the nestlings was measured several times during mornings (from 7 AM to 10 AM) until they reached adult size to capture growth rate (day 0, 4, 6, 8, 10, 12, 16, and day 35, see also Supplementary Figure 5). On day 16, just before fledgling, we also ringed chicks with an individually numbered aluminum ring and measured tarsus and wing length. As a part of a different study, we collected blood sample from all nestlings on day 16. Nestling survival was followed up to 35 days of age, which was the last time we measured their body mass, tarsus and wing length to estimate their final adult size.
Food Intake
We estimated food intake by measuring the initial seed mass and after 48-h period the final seed mass. For the food intake we also took into account the spilled seeds in the container that were not obtained and consumed from the birds. We measured food intake over a 48-h period during the peak of food provisioning and reproductive performance on day 4 to 6, day 6 to 8, day 8 to10, and day 10 to 12.
Blood Sampling and Oxidative Stress Biomarkers
We blood-sampled females three times: (a) before mating, (b) on day 13, just after respiratory measurements, and (c) at the end of the experiment, on day 35, when chicks reached full independence. Blood sampling always took part early in the morning just after lights on. After a brachial vein puncture, we collected blood sample of 75 μl in capillaries and stored in heparinized Eppendorfs 100 μl. Blood sample was immediately centrifuged for 10 min at 3340 g to separate plasma (Centrifuge MPW-56, MPW Med. instruments). Plasma sample was stored at −80°C until further analyses which took place within 1 month after the end of the experiment. Following the protocol of Costantini and Dell’Omo (2006) for the d-ROM test, we estimated oxidative damage and the results are expressed as mmol of H2O2 equivalents. The oxy-adsorbent (OXY) test measures the total non-enzymatic antioxidant capacity (Costantini and Dell’Omo, 2006) by quantifying the ability of plasmatic antioxidants to cope with hypochlorous acid (HClO); an endogenously produced oxidant. We followed the protocol of Sudyka et al. (2016) and values are expressed as mmol of HClO neutralized. The plasma concentration of uric acid was measured by the endpoint uric acid assay kit (Diacron International, Grosseto, Italy) and we followed the protocol of Zagkle et al. (2020). All analyses for the colorimetric assays were run in duplicate using an absorbance reader (Sunrise, Tecan’s Magellan, Tecan Trading, Switzerland). In the end, we estimated oxidative stress index by calculating the ratio of the oxidative damage to the total non-enzymatic antioxidant capacity in the plasma multiplied by 1000.
Statistical Analysis
We performed all analysis using R computer software (R version 4.1.2, R Core Team, 2021). For the linear mixed effect models we used lme4 package (Bates et al., 2015) and lmerTest package (Kuznetsova et al., 2017) to calculate the degrees of freedom and p values. Post hoc comparisons were performed using Tukey method in case of two groups of means, and Sidak method for multiple groups of means. Least square means (LSM) and standard error means (±SE) were calculated using emmeans package (Lenth, 2022). All non-significant interactions (p > 0.05) were removed from the models. We checked all our models for normality assumptions and homogeneity of variance by visual inspection of the residuals and in some cases variables (i.e., clutch size, uric acid and oxidative stress index) were log-transformed to meet the assumptions of normality. All figures presented are based on raw data. The sample size in many biological experimental studies may not always reach a sufficient amount (i.e., for logistic reasons as in this study) and thus decreasing considerably the statistical power. We, therefore, present and interpret the biological effects in a language of evidence (Muff et al., 2022) and clarity (Dushoff et al., 2019) rather than in the cut-off binary decision of the p-Value < 0.05.
Egg Mass
We tested differences in egg mass by performing linear mixed effect model analysis, with egg mass the response variable and the ambient temperature as the predictor. Clutch size (the number of eggs) and the body mass of the mother were included as covariates, and the identity of the nest box was included as a random effect to control for any dependence laid on the same nest by the same mother.
Female Body Mass
We performed two-way ANOVA for testing differences in body mass in mothers at the beginning of the experiment between the ambient temperatures. Linear mixed-effect model analysis was performed for body mass in females including ambient temperature and nestling age (day 0, 4, and 6 - which was the day of feather-clip manipulation) as fixed effects and their interaction. Another linear mixed effect model was performed for analysis of female body mass with the explanatory variables of ambient temperature (factor I; cold and warm), feather-clip manipulation (factor II; feather-clipped, unclipped), the nestling age (day 8, 10, 12, 16, and 35) and their interaction. Number of chicks was included as a covariate. For both models, the identity of the female was included as a random effect.
Oxidative Status
We tested for differences in oxidative stress biomarkers (response variable) in females between the two ambient temperatures (factor I) performing one-way ANOVA when sampled just before reproduction. We sampled mothers at the nesting age of 13 and 35 days old, at the peak of food provisioning and toward the end of offspring rearing respectively. The given oxidative stress biomarker was set as a response variable and we added nestling age (categorical variable with two levels: 13 and 35), ambient temperature and the feather-clip manipulation groups as predictors and their interaction as well. The body mass of the female was included as a covariate, while the identification number of the female was a random effect to account for the repeated measures.
Food Intake
We tested for differences in food intake between the two ambient temperature groups before the feather-clip manipulation, at the nestling age of 4 to 6 days old performing ANCOVA. We included female body mass and clutch size as covariates. After feather-clip manipulation, we performed a linear mixed effect model to detect variation in food intake during the chick development over three 48 h periods; (1) from 6 to 8 days old of chicks, (2) from 8 to 10 days old, and (3) from 10 to 12 days old. As predictor variables, we added ambient temperature, feather-clip manipulation, the sampling point, and their interaction. Brood size and female body mass were included in the model as covariates.
Nestling Development
(a) To test differences in body mass, tarsus, and wing length (response variables) between the two ambient temperatures (factor I) until the feather-clip manipulation that took place on day 6 we performed linear mixed effect model including interaction between ambient temperature and experiment day. Brood size was also included as a covariate to control for any differences between small and large broods and the identity of the female and the identity of the chicks were set as random effects. (b) We performed linear models over the nestling age since the relationship appeared to be linear (contrary to the asymptomatic growth slope) to test differences in body mass, wing, and tarsus length in nestlings (response variable) between the two ambient temperatures (factor I) and the feather-clip manipulation groups (factor II). For this model we included a third factor of the experimental day (factor III; levels: day 8, 10, 12, 16, and 35) and the interaction between the three factors (factor I × factor II × factor III). The brood size was included as a covariate to control for any differences in nestling development between small and large broods. The identity of the chicks was included as a random effect to account for the repeated measurements during the development nested in the identity of the mother to control for any dependence in the nestling development from the same mothers.
Results
Biparental Traits
A total of 82 pairs were exposed to either cold conditions of 14°C (n = 39) or warm conditions of 25°C (n = 43). Birds were more successful in laying eggs at the cold compared to warm conditions; about 82% of the 39 pairs (32 out of 39) in the cold and about 51% of the 43 pairs (22 out of 43) in the warm laid eggs (χ2 = 8.67, p = 0.003; Figure 1A). From those pairs that laid eggs, a total of 26 out 32 chicks hatched in the cold, and 18 out of 22 in the warm. There was no evidence that ambient temperature influenced hatching success (χ2 = 0.002, p = 0.95). When chicks hatched (day 0), there was moderate evidence in clutch size (after log-transformation) between the two ambient conditions (F1,52 = 5.7, p = 0.02; one-way ANOVA, Figure 1B); females in the warm laid significantly smaller clutches than the females in the cold. Variation in egg mass (ANCOVA) was explained by the ambient temperature (F1,49.5 = 5.7, p = 0.02; Figure 1C) and female body mass (t = 2.52, F1,46.7 = 6.3, p = 0.01), while there was no evidence that the clutch size had an effect on egg mass (t = 0.91, F1,37.9 = 0.8, p = 0.36).
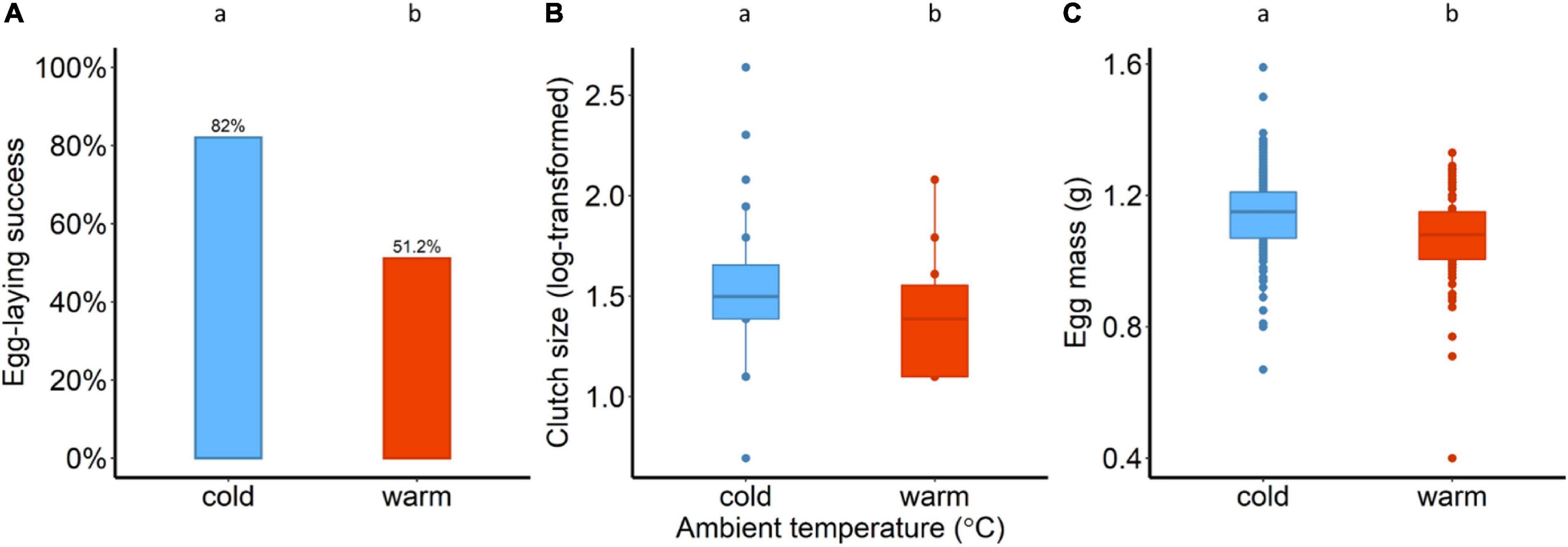
Figure 1. (A) Egg-laying success in percentage (χ2 = 8.67, df = 1, p = 0.003), (B) clutch size (after log-transformation) and (C) egg mass (g) for the two ambient conditions (14°C and 25°C). Different lower-case letters indicate statistical significance.
Female Traits
Body Mass
Initial body mass measured before mating did not differ between the two ambient temperatures (F1,81 = 2.28, p = 0.13; one-way ANOVA). Body mass decreased continuously from the day that chicks hatched until day 6 (F2,78.6 = 297.8, p < 0.001), but no difference was found between the two ambient temperatures (F1,45.5 = 2.72, p = 0.11). Interaction between ambient temperature and nestling age was found to be statistically unclear. We applied the feather-clip manipulation when chicks were 6 days old and there was no evidence that the body mass of the females differ between the experimental groups (F1,38 = 2.02, p = 0.16). Linear mixed effect model analysis revealed that there was strong evidence that nestling age was associated with female body mass (F4,157,0 = 44.4, p < 0.001); body mass decreased significantly for all females during nestling–rearing period. We found weak evidence for an interactive effect between feather-clip manipulation and ambient temperature (F1,36,9 = 3.7, p = 0.06). Unclipped females in the warm temperature revealed the lowest body mass (11.9 ± 0.4; LSM ± SE) compared to the feather-clipped females in the warm (13.2 ± 0.4; LSM ± SE), unclipped (13 ± 0.3; LSM ± SE) and feather-clipped (12.9 ± 0.3; LSM ± SE) in the cold (model results in Supplementary Table 2 and post hoc analysis in Supplementary Table 3). We also performed ANCOVA for female body mass (g) on day 16 and found moderate evidence for an interactive effect between ambient temperature and feather-clip manipulation (F1,37 = 4.26, p = 0.04). Unclipped mothers in the warm temperature revealed the lowest body mass in comparison to the other three groups (Figure 2A).
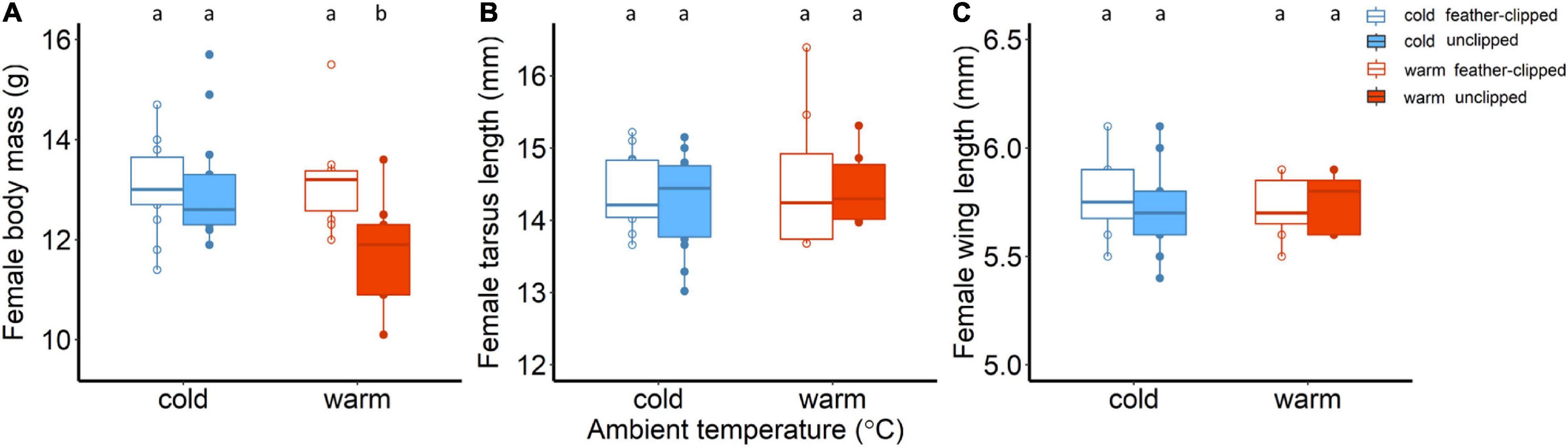
Figure 2. (A) Body mass (g), (B) tarsus length (mm) and (C) wing length (mm) of the females. Body mass of the females is shown at nestling age of 16 days old. Blue color stands for the cold conditions while red for the warm conditions. Filled symbols and boxplots represent unclipped mothers (control) while the open symbols and boxplots the feather-clipped mothers. Boxplots show the median, the range and the inter-quartiles, and the symbols indicate individual values. Different lower-case letters indicate statistical significance.
Oxidative – Antioxidant Status
Effect of Temperature Before Reproduction
There was no evidence for an influence of ambient temperature on uric acid when birds were sampled in the morning, just before mating (F1,72 = 0.61, p = 0.43; Figure 3A). Similarly, no evidence of ambient temperature in non-enzymatic antioxidant capacity (F1,69 = 0.25, p = 0.61; Figure 3B). We found moderate evidence in oxidative damage, measured as d-ROM, between the two groups of ambient temperature (F1,73 = 5.36, p = 0.02); females in the warm conditions revealed higher oxidative damage (LSM ± SE: 1.75 ± 0.07) than females in the cold conditions (LSM ± SE: 1.49 ± 0.07) (Figure 3C). Differences between the two ambient temperature in oxidative stress index (after log-transformation), calculated as the ratio of the oxidative damage to the total non-enzymatic antioxidant capacity multiplied by 1000 (Costantini et al., 2007), were not statistically clear (F1,68 = 2.46, p = 0.12; Figure 3D).
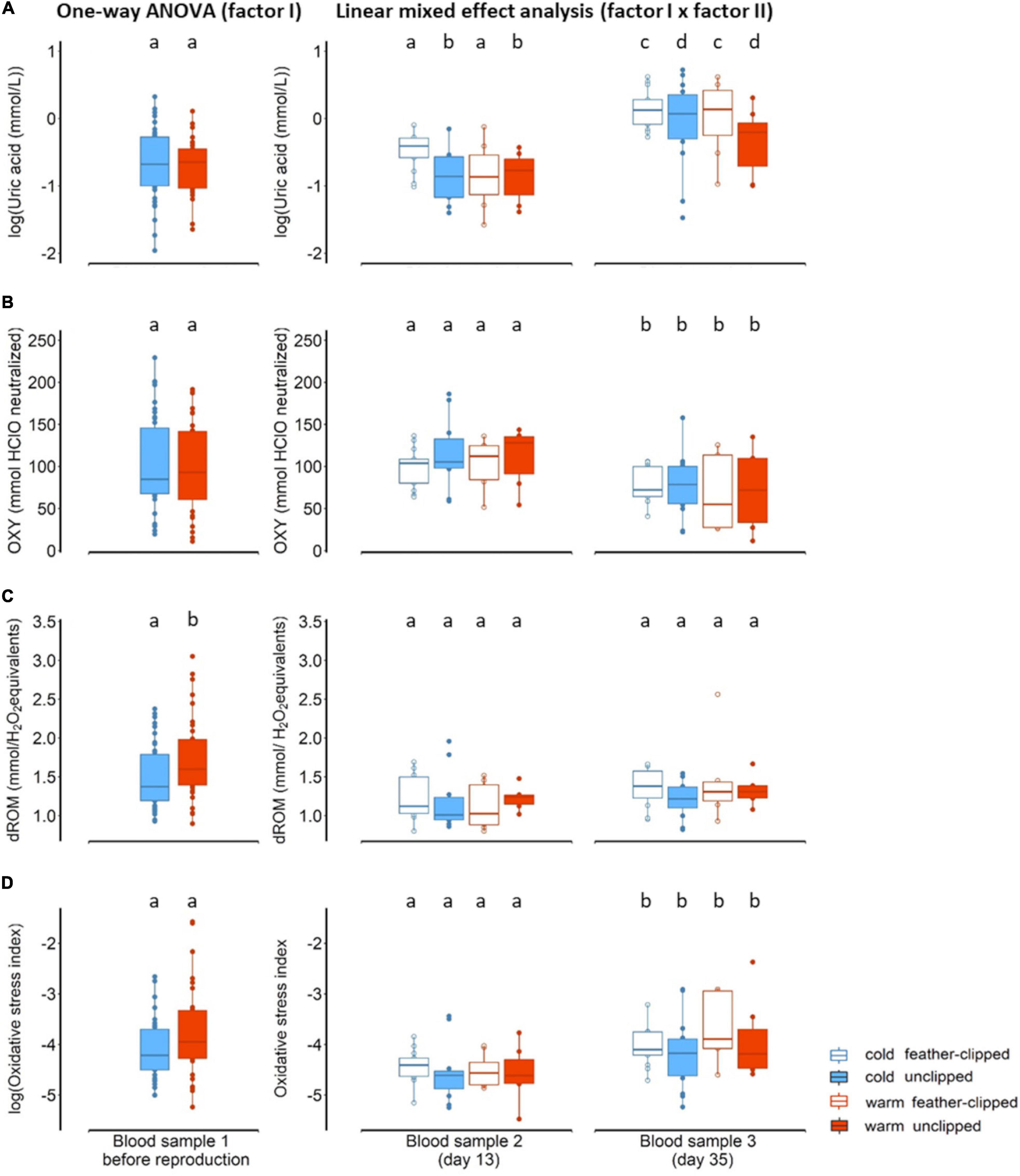
Figure 3. (A) Uric acid, log-transformed (mmol/L), (B) OXY (mmol HClO neutralized), a metric for non-enzymatic antioxidant capacity, (C) dROM (mmol/H2O2 equivalents), a metric of oxidative damage and (D) oxidative stress index, calculated as the ratio of the oxidative damage to the total non-enzymatic antioxidant capacity multiplied by 1000, in plasma concentration of the mother zebra finches (Taeniopygia guttata). At the left panel biomarkers are shown when sampled before reproduction and tested for differences using one-way ANOVA (factor I: ambient temperature) and at the right panel during offspring rearing period at nestling age of 13 and 35 days old (blood sample 2 and 3 respectively) tested with linear mixed effect model (factor I, factor II and blood sampling as a third factor). Blue color stands for the cold conditions while red for the warm conditions. Filled symbols and boxplots represent unclipped mothers (control) while the open symbols and boxplots the feather-clipped mothers. Boxplots show the median, the range and the inter-quartiles, and the symbols indicate individual values. Different lower-case letters indicate statistical significance.
Effect of Temperature and Feather-Clipping During Offspring Rearing Period
We found no evidence for an interactive effect between sample point, feather-clipping and ambient temperature in any of the oxidative stress biomarkers and thus removed these from the models. There was a statistical clear difference in uric acid (after log-transformation) between the two sampling points (F1,38.6 = 65.5, p < 0.001); uric acid in females was higher at the end of the offspring rearing period (LSM ± SE: −0.05 ± 0.07, on day 35) compared to the peak of offspring rearing period (LSM ± SE: −0.76 ± 0.07, on day 13). While ambient temperature did not explain any variation in uric acid (F1,38.2 = 2.9, p = 0.09), there was moderate evidence that feather-clip manipulation had an effect in uric acid (F1,39.0 = 4.6, p = 0.03). Feather-clipped females regardless of the ambient temperature revealed higher uric acid (LSM ± SE: −0.28 ± 0.08) than the un-manipulated (LSM ± SE: −0.53 ± 0.08) females (Figure 3A). Non-enzymatic antioxidant capacity also differed significantly between the two sampling points (F1,36.5 = 20.23, p < 0.001); non-enzymatic antioxidant capacity was significantly lower (LSM ± SE: 74.7 ± 5.5) in females toward the end of reproduction in comparison to the peak of offspring rearing period (LSM ± SE: 106.6 ± 5.4) (Figure 3B). However, neither ambient temperature (p > 0.05) nor feather-clip manipulation (p > 0.05) explained variation in antioxidant capacity. The effect of ambient temperature on oxidative damage was statistically unclear (F1,36.5 = 0.17, p = 0.67) and similarly the effect of feather-clip manipulation (F1,35.9 = 1.1, p = 0.29). There was weak evidence that oxidative damage differed between the two sampling points (F1,40.1 = 3.1, p = 0.08); oxidative damage was slightly higher at the end of offspring provisioning period (LSM ± SE: 1.25 ± 0.05) on day 35 than during the peak of offspring provisioning period (LSM ± SE; 1.13 ± 0.05) on day 13 (Figure 3C). Oxidative stress index (after log transformation) did not differ between ambient temperature groups (F1,37.39 = 0.87, p = 0.35) and feather-clip manipulation groups (F1,36.58 = 1.34, p = 0.25) but differed between the sampling points (F1,36.9 = 21.91, p < 0.001); oxidative stress index was higher toward the end of offspring-rearing period (LSM ± SE: −4.0 ± 0.09) compared to the peak of offspring-rearing period (LSM ± SE: −4.55 ± 0.09; Figure 3D).
Maternal Trait
We found statically clear differences in food intake (within 48-h period) between the two ambient temperature groups (F1,32 = 15.41, p < 0.001; Figure 4); food intake in mothers raising hatchlings 4 to 6 days old at the warm temperature was lower (9.4 ± 1.4 g; LSM ± SE) than food intake in mothers raising hatchlings from 4 to 6 days old at the cold temperature (16.1 ± 0.9; LSM ± SE). After the implementation of the feather-clip manipulation, there were statistically clear differences in food intake between the two ambient temperatures (F1,31.5 = 25.59, p < 0.001) and the three sample points (F1,84.5 = 46.59, p < 0.001). However, there was no evidence that feather-clip manipulation had an effect in food intake from the mothers (F1,31.5 = 0.01, p = 0.91). There was no evidence for a three-way interaction between factor I, factor II and sample point (p = 0.44). Brood size explained most of the variation in food intake (F1,34 = 20.5, p < 0.001). Food intake of the females increased along the development of the chicks, and it was lower for the females breeding in the warm conditions compared to the higher food intake of the females breeding in the cold conditions (Figure 4).
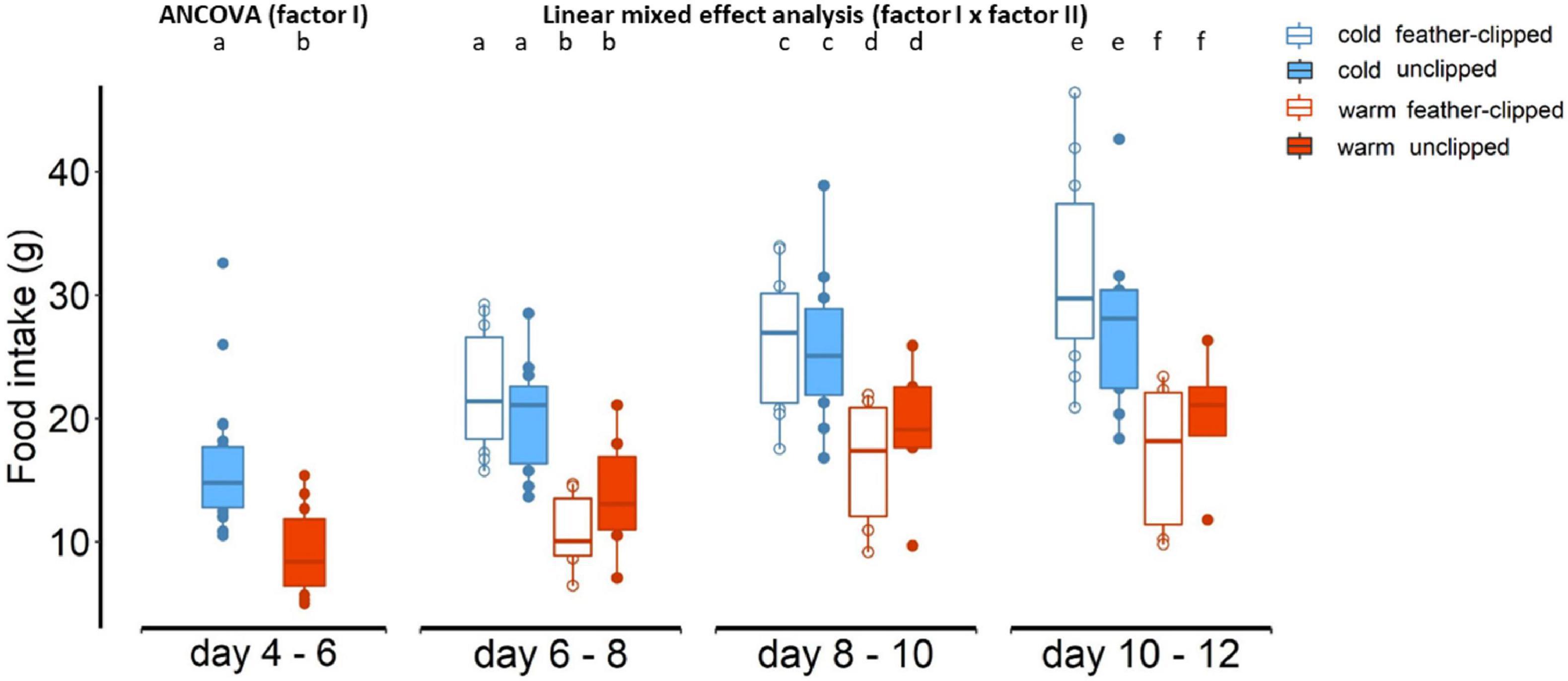
Figure 4. Food intake within 48-h period of the mothers between cold and warm conditions and after the feather-clip manipulation at day 6 food intake between feather-clipped and unclipped mothers raising chicks at the warm (red color) and cold (blue color) ambient temperature. Different lower-case letters indicate statistical significance.
Offspring Traits
There is no evidence that body mass of the hatchlings differed between the ambient temperature conditions (F1,47.2 = 0.27, p = 0.6) from when eggs hatched (day 0) until the day of the feather-clip manipulation (day 6). The interaction between ambient temperature and nestling age was statistically unclear (F1,271.3 = 0.74, p = 0.47). There was strong evidence that body mass of the nestlings differed with the nestling age (F1,271.3 = 491.54, p < 0.001) and there was moderate evidence that the brood size had an effect on body mass (F1,55.37 = 4.29, p = 0.04).
After the feather clip manipulation (day 6), we found moderate evidence for an interaction between ambient temperature (factor I), feather-clip manipulation (factor II) and the nestling age (factor III); factor I × factor II × factor III (F4,465 = 3.64, p = 0.01, see Supplementary Figure 5B). At the age of 35 days old, nestlings raised from unclipped females at warm ambient temperature had lower body mass compared to feather clipped females at the warm temperature compare to all three other groups, while no differences were detected during the nestling development (Figure 5A and Supplementary Table 4). Chick mass depended also on the brood size (F1,39.8 = 5.8, p = 0.02; Supplementary Table 5).
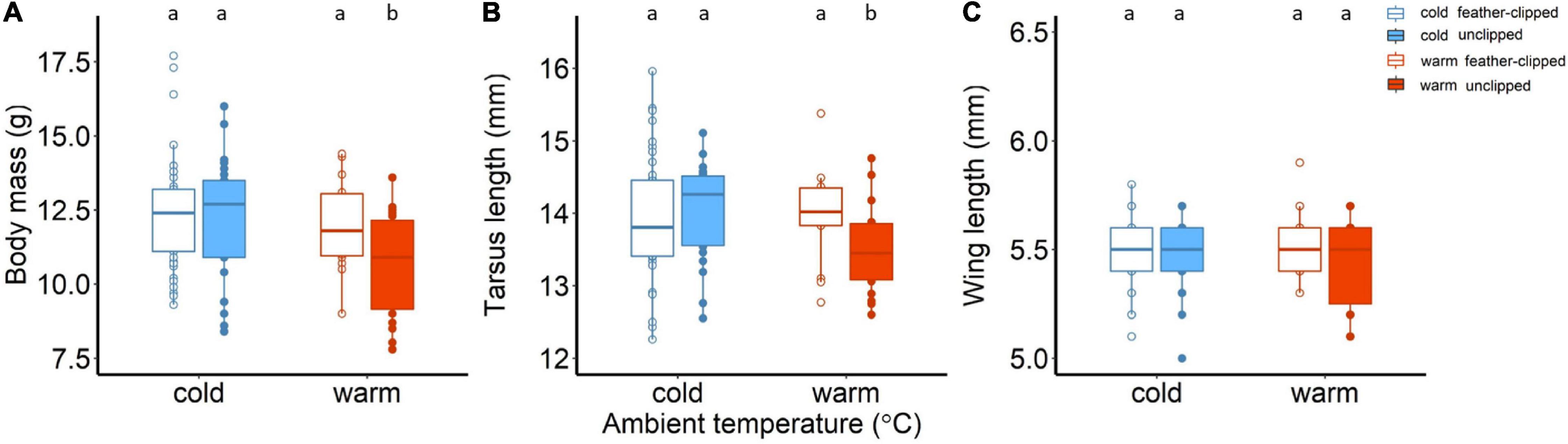
Figure 5. (A) Body mass (g), (B) tarsus length (mm), and (C) wing length (mm) of the nestlings raised in cold (14°C, blue color) and warm conditions (25°C, red color) on day 35. Filled symbols and boxplots represent nestlings raised by the unclipped mothers (control) while the open symbols and boxplots nestlings raised by the feather-clipped mothers. Boxplots show the median, the range and the inter-quartiles, and the symbols indicate individual values. Different lower-case letters indicate statistical significance.
For the tarsus length of the chicks, we detected statistically clear interaction between ambient temperature and feather-clip manipulation (factor I × factor II, F1,115.5 = 9.07, p = 0.003). Chicks raised from the unclipped females at warm conditions had smaller tarsus length (mm) in comparison to the other three groups; warm feather-clipped, cold unclipped and cold feather-clipped (Figure 5B). Tarsus length was positively related to nestling age (tarsus length increased with increasing age from 16 to 35 days old; F1,102.97 = 88.96, p < 0.001), while brood size on day 35 did not explain any variation of tarsus length (F1,117.06 = 9.07, p = 0.76). On the other hand, wing length was not affected by either of the two manipulations (factor I and factor II) but significantly related to nestling age; wing length was higher with increasing age (from 16 to 35 days old; F1,114.59 = 2233.67, p < 0.001).
Discussion
First signs of reproductive limitations in line with the heat dissipation limit hypothesis (HDL, Speakman and Król, 2010b) appeared on the level of reproductive performance of both parents, in that breeding pairs at warm temperature were less successful in producing a clutch compared to the breeding pairs at the cold (Figure 1A). Egg production in terms of egg number and mass was significantly lower under warm compared to cold ambient temperature (Figures 1B,C), which is consistent with previous findings revealing decreased egg size and mass (Williams and Cooch, 1996; Schaper and Visser, 2013) with increasing ambient temperatures (but see Griffith et al., 2020). In addition, breeding zebra finches showed decreased egg mass and clutch size when exposed to a high foraging cost feeder similar to the one we implemented (Yap et al., 2021). Here, we suggest that reduced clutch size and egg mass in the warm conditions resulted from a combination of both warm temperature and elevated foraging costs. Physical exercise increases heat production and thus combined with warm ambient temperature during reproduction resulted in lower reproductive output suggesting that the capacity of heat dissipation may indeed be important. By feather-clipping females around the brood patch and the pectoral muscles, we enhanced heat dissipation capacity up to 0.03 W (9.5%) compared to the unclipped females (for further details see Supplementary Material). Results on maternal reproductive performance clearly support HDL hypothesis with unclipped females revealing lower body mass maintenance compared to the females with increased heat loss rate. Warm ambient temperature combined with enhanced physical activity appeared to negatively affect oxidative damage in females prior to reproduction, whereas oxidative stress biomarkers were unaffected by both treatments, temperature, and feather-clip manipulation, during and toward the end of the reproductive activities. Even though food intake did not differ significantly between the clipped and unclipped groups, the variation might be masked by the food intake obtained by the mother and lack of direct measurements of food passed on to the nestlings. Offspring development was affected indirectly by our maternal manipulations of heat dissipation capacity, with smaller size and lighter mass of nestlings raised by the unclipped – and thus constrained in heat dissipation – mothers compared to the clipped mothers, corroborate evidence on HDL hypothesis. Our two-factorial experimental design indicates that warm ambient temperature limits energy expenditure during highly intensive activities like reproduction and that experimentally releasing the heat burden through feather clipping does relax the thermal limitations. Heat dissipation capacity appears as limitation of sustained performance.
Heat Dissipation Limitations in Breeding Females
All mothers gradually decreased their body mass during nestling rearing period (Supplementary Figure 5A), but un-manipulated mothers in the warm revealed lower body mass when nestlings reached 16 days old compared to the other three groups of mothers eased of heat constrains (Figure 2A). This suggests that unclipped mothers in the warm were limited by the capacity to heat dissipate. The differences in body mass cannot be explained from the food intake, since food intake, even though dependent on ambient temperature, was independent of the feather-clip manipulation (Figure 4). Body mass loss during reproduction has been previously observed in birds and it has been formulated as a consequence of either a consumption of the energy reserves (“cost of reproduction” hypothesis) or an adaptation to lower the flight costs of adults during nestling rearing (“mass-adjustment” hypothesis) (Hillström, 1995). In this study, birds had unlimited access to food during a 13 h-period of daylight and thus the hypothesis that birds were not able to replenish their energy stores may not be supported by the “cost of reproduction” hypothesis. Maternal feather-clip manipulation in blue tits (Cyanistes caeruleus) enhanced heat loss by 0.09 W and revealed lower body mass loss in feather-clipped females compared to the unclipped ones when nestlings were at 14 days old (Nord and Nilsson, 2019). This is in line to the results of our present study, even though the difference in heat loss is smaller (0.03 W). Higher energy expenditure induced by an experimentally enlarged brood size in great tits (Parus major) was negatively related to body mass (Nadav, 1984), and Nord and Nilsson (2019) suggested that unclipped females may have lost more body mass due to the higher energetic costs of panting to dissipate heat compared to the feather-clipped ones. Panting is a known mechanism in passerines for heat dissipation (McKechnie and Wolf, 2019) and more likely to take place during high ambient temperatures (Oswald et al., 2021), and during singing increasing heat loss (Pessato et al., 2020). Panting requires a high amount of water expenditure causing changes in blood chemistry (Calder and Schmidt-Nielsen, 1966; Schmidt-Nielsen, 1972), and perhaps unclipped mothers in the warm in our study may have used more energy to dissipate heat via panting (or via singing) compared to the clipped mothers in the warm (and both experimental groups in the cold) explaining the body mass difference. We did observe that birds in the climatic chamber with warm temperature were more likely to sing than birds in the cold climatic chamber (pers. observations), but such observations require further experimental testing. Also, body temperature rises during exercise (Ward et al., 1999; Guillemette et al., 2016) and this rise becomes more pronounced with increasing ambient temperatures (Tucker and Noakes, 2009; Tapper et al., 2020a). When body temperature reaches lethal levels as shown in tree-swallows during offspring provisioning period (i.e., 15% of all observations exceeded 43°C; Tapper et al., 2020a), organisms have evolved different mechanisms to avoid any irreversible physiological damage (i.e., pacing strategy in human athletes; see review in Tucker and Noakes, 2009). Eider ducks (Somateria mollissima) also stop their flights when body temperature rises (Guillemette et al., 2016). Lowering the pace may explain high body mass loss in unclipped birds at the warm temperature. Unclipped females breeding in warm conditions, hence with heat dissipation limitations, may have lost body mass as a strategy to lower metabolic heat production. For instance in humans, runners with lower body mass revealed an advantage of decreased heat production and thus were able to run faster than heavy runners under the hot ambient temperature of 35°C (Marino et al., 2000). In summary, the body mass changes of mothers in response to two manipulations provide strong experimental support that the capacity to dissipate heat may indeed represent a limit during sustained parental workload.
Enhancing the Ability to Heat Dissipate and Oxidative Stress
Since metabolic rate is affected by ambient temperature (Scholander et al., 1950), production of reactive oxygen species (ROS) is likely to be also affected by environmental temperature (Selman et al., 2000; Blagojević, 2007; Monaghan et al., 2009). In this study, after 3 weeks of acclimation to the climatic conditions, zebra finches were sampled just before reproduction and revealed higher levels of oxidative damage at the warm ambient temperature than the cold temperature (Figure 3C). Acute exposure in high ambient temperatures and/or simultaneous physical activity may induce oxidative stress (Lin et al., 2006; Mestre-Alfaro et al., 2012). Exercise produces heat and in combination with warm ambient temperature likely caused a surplus of body heat; this may explain the higher levels of oxidative damage compared to the cold we found before the reproductive event. However, oxidative damage and non-enzymatic antioxidant capacity during chick-rearing period appeared unaffected by both manipulations, such as ambient temperature and feather clipping. Mongolian gerbils (Meriones ungulicatus) exposed to 10°C, 21°C, and 30°C during reproduction also did not reveal differences in various markers of oxidative stress (Yang et al., 2013), similar outcome to this study. High levels of oxidative damage were detected in zebra finches when exposed in high temperatures (38°C and 42°C) during their adulthood, but this effect did not appear in birds that experienced heat-exposure in their early life (Costantini et al., 2012). Previous experience or duration (acute or chronic) of the exposure to the temperature may thus explain the fact that we detected differences in oxidative damage at the early stage of the experiment but not later during the reproductive events. The oxidative damage that birds experienced at the beginning, through increased physical activity at warm ambient temperatures, may have driven changes in the line of the antioxidant defense mechanisms, such as the non-enzymatic antioxidant capacity and uric acid (Ji, 1999; Stier et al., 2019).
Non-enzymatic antioxidant capacity of females in this study was lower toward the end of nestling-rearing period compared to the peak regardless of ambient temperature and feather-clipping (Figure 3B), confirming that the antioxidant capacity can be up-regulated for the reproductive activities (Costantini et al., 2014a). During intensive physical activities such as reproduction, organisms use antioxidants for maintaining oxidative balance. Ad libitum access to food in this study gave the opportunity of the mothers to upregulate their antioxidant defenses (Speakman and Garratt, 2014), thus avoiding the risk of further oxidative stress. An opposite pattern in uric acid was detected with higher uric acid toward the end of reproduction. In birds, uric acid has been suggested to act as endogenously produced antioxidant when oxidative stress is encountered (Tsahar et al., 2006; Alan and McWilliams, 2013). For example, uric acid was found to be positively related with allantoin (its’ oxidative product) in white-crowned sparrows during intensive exercise (Tsahar et al., 2006). High levels of uric acid were observed in godwits prior to migratory flight, which perhaps was caused from the breakdown of proteins that originate from body tissue (Gutiérrez et al., 2019). Here, when the up-regulated antioxidant capacity was reduced, then the uric acid took over toward the end of the reproduction and most of the variation was explained mainly by the feather-clip treatment regardless of the ambient temperature (Figure 3A). Feather-clipped females due to higher energetic investment to raise large and heavier offspring (higher reproductive output) entailed catabolism of proteins possibly due to lower investment for self-maintenance, and uric acid activated to diminish any further physiological costs. In conclusion, both oxidative stress biomarkers, non-enzymatic antioxidant capacity and uric acid, suggest that mothers during offspring-provisioning period did encounter oxidative stress and thus drove changes for the upregulation of antioxidant capacity and uric acid to protect for further costs. However, we should be cautious when interpreting the data of oxidative stress since this study is based on a limited number of assays only in plasma, without measuring enzymatic antioxidants or ROS production and is known that different assays or tissues may bring different results (Speakman and Garratt, 2014). Feather-clip manipulation influenced uric acid, suggesting that females eased from thermal limitations may increase energetic investment toward current reproduction, but this may also come with a cost of encountering oxidative stress, perhaps with lower future reproduction.
Nestling Development Constraints
Maternal treatment (i.e., feather-clipping) not only affected their own body mass over the course of the reproductive event and possibly oxidative stress but also nestling development and the interactive effect was only detected at the nestling age of 35 days old (Figure 5B and Supplementary Figure 5B). At the nestling age of 35 days zebra finches reach almost a fully developed juvenile size and move toward independence from their parents (Zann, 1996). Nestling zebra finches produced in 30°C ambient temperature were smaller in body mass and tarsus length than those in 18°C; however, these differences were only visible at the age of 28 days, but not at the age of 12 days (Andrew et al., 2017). This outcome is similar with our findings, in which reduced offspring mass and size were observed at the warm temperature raised by unclipped females on day 35, when nestlings reached nearly fully developed juvenile size, and no differences were observed in the early stages of development. Parental care and offspring development are closely coupled (Ricklefs, 1984; Zann, 1996) and it is unclear if the effect during the last stage of development is due to cumulative effects over the development of the offspring or due to the difficulty to quantify the effects of maternal care at the early stages of nestling development. For instance, the early stage of development is characterized by intensive nestling signals, such as begging calls, that may modify and influence parental care and the level of food provisioning (Leonard and Horn, 2001). Begging calls take part in young zebra finches up to 14-16 days old and after fledgling young ones are less active and beg just before feeding bout (Zann, 1996). Even though mothers were constrained by the heat dissipation capacity, they were rather modulating maternal care due to nestling signals, hence masking any effects at the early stage. Perhaps, any effects of thermal constrains during sustained parental workload are more likely to shape offspring development at the later stage of development.
Any conditions experienced during a specific biological stage very often impact the performance of the individuals at the subsequent biological stage (Blomberg et al., 2014). When zebra finches bred in two ambient temperatures of 18°C and 30°C, nestlings raised in the hot temperature were lighter and smaller at 28 days than those raised in the cold but the effect of body mass was removed when they reached 90 days old (complete juvenile size) and only the effect in tarsus remained (Andrew et al., 2017), a partial “catch-up.” Here, nestlings raised by the unclipped mothers in the warm differed significantly in body mass and tarsus length compared to the other three groups, while wing length remained unchanged (Figures 5B,C). We did not follow their post-juvenile development (as in the study of Andrew et al., 2017) and we do not know whether nestlings were able to catch up with increasing their body mass. Tarsus length represents skeletal size in birds and may act as a “thermal window” which may serve for heat dissipation (Speakman and Ward, 1998). For instance, Allen’s rule suggest that small morphological traits should rather reflect an adaptive response to cold climates for heat conservation and large in warm climates for higher heat loss. Reduced body size is associated with warm climates, while increased with cold ones following Bergmann’s rule (Ashton, 2002; Cunningham et al., 2013; Kruuk et al., 2015; Andrew et al., 2017; Oswald et al., 2021). However, here we found that nestlings raised by the feather-clipped females in the warm were larger than those raised by unclipped, suggesting that the mechanistic link between the climate experienced during development and the body size is likely driven by the physiological constrains of parental care and food provisioning. Wing length represents both skeletal size and feather growth, which depends on other factors like for example nutritional supply (Senar and Pascual, 1997; Oswald and Arnold, 2012; Andrew et al., 2017). Feathers require necessary nutrients for growth (Hill and Montgomerie, 1994) and nestlings in this study even though we observed differences in body mass and tarsus length, wing length was unaffected that may be explained by the similar amount of nutrients obtained through the seeds. In addition, tarsus size of the nestlings at this stage (35 days old) was more developed close to adult size (Figures 2B, 5B) while wings were not fully developed (see differences in female and offspring wing length; Figures 2C, 5C). Perhaps any effects on wing length could be detected later when wings are fully developed close to adult size. Low mass and small structural size in nestlings implies lower survival probability (Magrath, 1991; Schwagmeyer and Mock, 2008; Ronget et al., 2018) and Tapper et al. (2020b) show evidence that nestlings with lower mass and size raised from unclipped mothers were less successful to fledge than those raised from the feather-clipped mothers. The observed effects on nestling size and mass provide strong evidence that HDL plays a pivotal role in reproductive success and thus fitness of the organisms.
We predicted that feather clipping compared to unclipped treatment would remove the thermal limitations on reproductive performance at both ambient temperatures, but we can confirm this prediction only for the high and not for the low ambient temperature. Clearly, the difference between clipped and unclipped birds for the high ambient temperature is in agreement with HDL hypothesis, but the effect of clipping at the low ambient temperature may rather hint to opposite thermal constrains. Cold ambient temperature in combination with the feather-clip manipulation may have enhanced heat dissipation so much that birds now face an additional energetic burden for thermoregulation, namely to remain normothermic and not to cool out to much (Sadowska et al., 2019). Possibly, the activity levels modulate the impact of the feather-clipping treatment and females in the cold must allocate energy to remain warm when they are not active. In high ambient temperature clipping would improve the thermoregulation of the active birds, reducing their costs of thermoregulation. The effects of clipping on the energy balance of active birds in cold temperature and of inactive birds in high temperature should be intermediate. While we observe food intake difference only between warm and cold treatment and not between the feather manipulations, we do observe body mass effects on females and offspring and structural size effects on offspring only in the difference between clipped and unclipped females at the warm ambient temperature and not in the cold. Non-enzymatic antioxidant capacity decreased for all females during nestling-rearing period (Figure 3B), uric acid increased, but this was more pronounced for the feather-clipped females, both in warm and cold temperature, than the control (Figure 3A). These results may suggest the upregulation of the antioxidant defense system could neutralize the adverse effects of oxidative stress (Alan and McWilliams, 2013; Gutiérrez et al., 2019) but the mechanism may differ between the ambient temperatures. The association between reproduction and oxidative stress is rather complex and may also act as a constrain. Experimentally induced oxidative stress in canaries (Serinus canaria) delayed the hatching date and affected the clutch size (Costantini et al., 2016), suggesting that indeed oxidative stress may act as a constrain during reproduction. On the other hand, even if females were able to increase energy toward reproductive output avoiding oxidative stress, it may not necessarily be an advantage of the nestlings to gain more mass or develop larger beyond the observed levels (Blanckenhorn, 2000). Thus, it would be interesting to experimentally enhance energetic demands through an enlarged brood size and investigate whether breeding animals released of thermal constraints may raise energy expenditure. To conclude, this study corroborates HDL hypothesis, but it also indicates that alternative limitations are likely occurring during reproduction and these may even entail a differential view of opposing thermal limitations between active and non-active phases.
Conclusion
Our study under controlled laboratory conditions provides now comprehensive support for the HDL hypothesis and adds up to the current evidence accumulated for free-living birds that also support HDL hypothesis (Nilsson and Nord, 2018; Nord and Nilsson, 2019; Tapper et al., 2020a,b). We consider the simulation of high foraging costs of free-living birds despite the typical ad libitum access to food in the laboratory as important requirement for meaningful testing of HDL hypothesis in captive species. Under the scenario of contemporary climate change, which is characterized by continuously rising ambient temperatures and extreme weather events such as heat waves (Ummenhofer and Meehl, 2017), thermal limitations can be expected to impose even bigger and strenuous challenges for organisms in general, and during reproduction in particular. Higher-than-normal temperatures may induce oxidative stress which also seems to be the driver for changes in the line of antioxidant defense, but further research is required to understand potential trade-offs between management of oxidative stress under thermal limitations and body condition. Oxidative stress avoidance during reproduction might be the key to understand the allocation of resources to either current or future reproduction. Any challenges due to high temperatures during reproductive events may suppress both current and future reproduction (see also Andreasson et al., 2020), and consequently setting limits to species distribution. Our research under controlled conditions provides clear understanding on thermal limitations to influence reproductive output in terms of quantity and quality. Such detailed understanding of what limits species distribution and fitness may enable us to tailor conservation approaches that are particularly needed under the current global change scenario.
Data Availability Statement
The original contributions presented in the study are included in the article/Supplementary Material, further inquiries can be directed to the corresponding author/s.
Ethics Statement
The animal study was reviewed and approved (Decision no 143/2017 and 241/2018).
Author Contributions
EZ, ES, and UB conceived the experiment. EZ performed the experiment and carried out all measurements with the help of PM-V. EZ analyzed the data and wrote the first draft. ES analyzed respiratory measurements presented at the Supplementary Material. All authors contributed significantly to the final version of the manuscript.
Funding
This project was funded by the National Science Center in Poland (grant 2016/22/E/NZ8/00416 to ES) and the Jagiellonian University (N18/DBS/000003).
Conflict of Interest
The authors declare that the research was conducted in the absence of any commercial or financial relationships that could be construed as a potential conflict of interest.
Publisher’s Note
All claims expressed in this article are solely those of the authors and do not necessarily represent those of their affiliated organizations, or those of the publisher, the editors and the reviewers. Any product that may be evaluated in this article, or claim that may be made by its manufacturer, is not guaranteed or endorsed by the publisher.
Acknowledgments
We want to acknowledge Sylwester Kunysz for the technical assistance during the experiment and our colleagues Amadeusz Bryła and Kyle Coughlan for providing valuable feedback on the manuscript. Our gratitude to the Guest Editor DS and reviewers for their constructive feedback improving the quality of the manuscript.
Supplementary Material
The Supplementary Material for this article can be found online at: https://www.frontiersin.org/articles/10.3389/fevo.2022.866182/full#supplementary-material
References
Alan, R. R., and McWilliams, S. R. (2013). Oxidative stress, circulating antioxidants, and dietary preferences in songbirds. Comp. Biochem. Physiol. B Biochem. Mol. Biol. 164, 185–193. doi: 10.1016/j.cbpb.2012.12.005
Alonso-Alvarez, C., Bertrand, S., Devevey, G., Prost, J., Faivre, B., Chastel, O., et al. (2006). An experimental manipulation of life-history trajectories and resistance to oxidative stress. Evolution 60, 1913–1924. doi: 10.1554/05-644.1
Andreasson, F., Nilsson, J. Å., and Nord, A. (2020). Avian Reproduction in a Warming World. Front. Ecol. Evol 8:576331. doi: 10.3389/fevo.2020.576331
Andrew, S. C., Hurley, L. L., Mariette, M. M., and Griffith, S. C. (2017). Higher temperatures during development reduce body size in the zebra finch in the laboratory and in the wild. J. Evol. Biol. 30, 2156–2164. doi: 10.1111/jeb.13181
Arct, A., Rutkowska, J., Martyka, R., Drobniak, S. M., and Cichon, M. (2010). Kin recognition and adjustment of reproductive effort in zebra finches. Biol. Lett. 6, 762–764. doi: 10.1098/rsbl.2010.0417
Ashton, K. G. (2002). Patterns of within-species body size variation of birds: strong evidence for Bergmann’s rule. Glob. Ecol. Biogeogr. 11, 505–523. doi: 10.1046/j.1466-822X.2002.00313.x
Bates, D., Mächler, M., Bolker, B. M., and Walker, S. C. (2015). Fitting linear mixed-effects models using lme4. J. Stat. Softw. 67, 1–48. doi: 10.18637/jss.v067.i01
Blagojević, D. P. (2007). Antioxidant systems in supporting environmental and programmed adaptations to low temperatures. Cryo Letters 28, 137–150.
Blanckenhorn, W. U. (2000). The Evolution of Body Size: What Keeps Organisms Small? Q. Rev. Biol. 75, 385–407. doi: 10.1086/393620
Blomberg, E. J., Sedinger, J. S., Gibson, D., Coates, P. S., and Casazza, M. L. (2014). Carryover effects and climatic conditions influence the postfledging survival of greater sage-grouse. Ecol. Evol. 4, 4488–4499. doi: 10.1002/ece3.1139
Briga, M., and Verhulst, S. (2017). Individual variation in metabolic reaction norms over ambient temperature causes low correlation between basal and standard metabolic rate. J. Exp. Biol. 220, 3280–3289. doi: 10.1242/jeb.160069
Brzęk, P., Ksiązek, A., OLdakowski, L., and Konarzewski, M. (2014). High basal metabolic rate does not elevate oxidative stress during reproduction in laboratory mice. J. Exp. Biol. 217, 1504–1509. doi: 10.1242/jeb.100073
Calder, W. A. (1964). Gaseous metabolism and water relations of the zebra finch, Taeniopygia castanotis. Physiol. Zool. 37, 400–413. doi: 10.1086/physzool.37.4.30152758
Calder, W. A., and Schmidt-Nielsen, K. (1966). Evaporative cooling and respiratory alkalosis in the pigeon. Proc. Natl. Acad. Sci. U.S.A. 55, 750–756. doi: 10.1073/pnas.55.4.750
Carey, C. (1996). Avian Energetics and Nutritional Ecology. Boston, MA: Springer Science & Business Media.
Costantini, D. (2011). On the measurement of circulating antioxidant capacity and the nightmare of uric acid. Methods Ecol. Evol. 2, 321–325. doi: 10.1111/j.2041-210X.2010.00080.x
Costantini, D., Bonisoli-Alquati, A., Rubolini, D., Caprioli, M., Ambrosini, R., Romano, M., et al. (2014a). Nestling rearing is antioxidant demanding in female barn swallows (Hirundo rustica). Naturwissenschaften 101, 541–548. doi: 10.1007/s00114-014-1190-2
Costantini, D., Casasole, G., and Eens, M. (2014b). Does reproduction protect against oxidative stress? J. Exp. Biol. 217, 4237–4243. doi: 10.1242/jeb.114116
Costantini, D., Cardinale, M., and Carere, C. (2007). Oxidative damage and anti-oxidant capacity in two migratory bird species at a stop-over site. Comp. Biochem. Physiol. C Toxicol. Pharmacol. 144, 363–371. doi: 10.1016/j.cbpc.2006.11.005
Costantini, D., Casasole, G., AbdElgawad, H., Asard, H., and Eens, M. (2016). Experimental evidence that oxidative stress influences reproductive decisions. Funct. Ecol. 30, 1169–1174. doi: 10.1111/1365-2435.12608
Costantini, D., and Dell’Omo, G. (2006). Environmental and genetic components of oxidative stress in wild kestrel nestlings (Falco tinnunculus). J. Comp. Physiol. B Biochem. Syst. Environ. Physiol. 176, 575–579. doi: 10.1007/s00360-006-0080-0
Costantini, D., Monaghan, P., and Metcalfe, N. B. (2012). Early life experience primes resistance to oxidative stress. J. Exp. Biol. 215, 2820–2826. doi: 10.1242/jeb.072231
Cunningham, S. J., Martin, R. O., Hojem, C. L., and Hockey, P. A. R. (2013). Temperatures in excess of critical thresholds threaten nestling growth and survival in a rapidly-warming arid savanna: a study of common Fiscals. PLoS One 8:e74613. doi: 10.1371/journal.pone.0074613
Dushoff, J., Kain, M. P., and Bolker, B. M. (2019). I can see clearly now: reinterpreting statistical significance. Methods Ecol. Evol. 10, 756–759. doi: 10.1111/2041-210X.13159
Finkel, T., and Holbrook, N. J. (2000). Oxidants, oxidative stress and the biology of ageing. Nature 408, 239–247. doi: 10.1038/35041687
Garratt, M., Vasilaki, A., Stockley, P., McArdle, F., Jackson, M., and Hurst, J. L. (2011). Is oxidative stress a physiological cost of reproduction? An experimental test in house mice. Proc. R. Soc. B Biol. Sci. 278, 1098–1106. doi: 10.1098/rspb.2010.1818
Griffith, S. C., Andrew, S. C., McCowan, L. S. C., Hurley, L. L., Englert Duursma, D., Buchanan, K. L., et al. (2020). Egg size is unrelated to ambient temperature in the opportunistically breeding zebra finch. J. Avian Biol. 51, 1–14. doi: 10.1111/jav.02356
Griffith, S. C., Crino, O. L., and Andrew, S. C. (2017). Commentary: a bird in the house: the challenge of being ecologically relevant in captivity. Front. Ecol. Evol. 5:21. doi: 10.3389/fevo.2017.00021
Guillemette, M., Woakes, A. J., Larochelle, J., Polymeropoulos, E. T., Granbois, J. M., Butler, P. J., et al. (2016). Does hyperthermia constrain flight duration in a short-distance migrant? Philos. Trans. R. Soc. B Biol. Sci. 371:20150386. doi: 10.1098/rstb.2015.0386
Gutiérrez, J. S., Sabat, P., Castañeda, L. E., Contreras, C., Navarrete, L., Peña-Villalobos, I., et al. (2019). Oxidative status and metabolic profile in a long-lived bird preparing for extreme endurance migration. Sci. Rep. 9:17616. doi: 10.1038/s41598-019-54057-6
Halliwell, B., and Gutteridge, J. M. C. (1999). Free Radicals in Biology and Medicine. Oxford: Oxford University Press.
Hammond, K. A., and Diamond, J. (1997). Maximal sustained energt budgets in humans and animals. Nature 386, 457–462. doi: 10.1038/386457a0
Hill, G. E., and Montgomerie, R. (1994). Plumage colour signals nutritional condition in the house finch. Proc. R. Soc. B Biol. Sci. 258, 47–52. doi: 10.1098/rspb.1994.0140
Hillström, L. (1995). Body mass reduction during reproduction in the pied flycatcher Ficedula hypoleuca: Physiological stress or adaptation for lowered costs of locomotion? Funct. Ecol. 9, 807–817. doi: 10.2307/2389978
Ji, L. L. (1999). Antioxidants and oxidative stress in exercise. Proc. Soc. Exp. Biol. Med. 222, 283–292. doi: 10.1046/j.1525-1373.1999.d01-145.x
Johnson, M. S., and Speakman, J. R. (2001). Limits to sustained energy intake V. EFFECT OF COLD-EXPOSURE DURING LACTATION IN MUS MUSCULUS M. J. Exp. Biol. 204, 1967–1977. doi: 10.1242/jeb.204.11.1967
Khan, R. U., Naz, S., Ullah, H., Ullah, Q., Laudadio, V., and Qudratullah, et al. (2021). Physiological dynamics in broiler chickens under heat stress and possible mitigation strategies. Anim. Biotechnol. [Epub ahead of print]. doi: 10.1080/10495398.2021.1972005
Koetsier, E., and Verhulst, S. (2011). A simple technique to manipulate foraging costs in seed-eating birds. J. Exp. Biol. 214, 1225–1229. doi: 10.1242/jeb.050336
Koteja, P. (1996). Limits to the energy budget in a rodent, Peromyscus maniculatus: the central limitation hypothesis. Phsyiol. Zool. 69, 981–993. doi: 10.1086/physzool.69.5.30164242
Król, E., Murphy, M., and Speakman, J. R. (2007). Limits to sustained energy intake. X. Effects of fur removal on reproductive performance in laboratory mice. J. Exp. Biol. 210, 4233–4243. doi: 10.1242/jeb.009779
Król, E., and Speakman, J. R. (2003). Limits to sustained energy intake VI. Energetics of lactation in laboratory mice at thermoneutrality. J. Exp. Biol. 206, 4255–4266. doi: 10.1242/jeb.00674
Kruuk, L. E. B., Osmond, H. L., and Cockburn, A. (2015). Contrasting effects of climate on juvenile body size in a Southern Hemisphere passerine bird. Glob. Chang. Biol. 21, 2929–2941. doi: 10.1111/gcb.12926
Kuznetsova, A., Brockhoff, P. B., and Christensen, R. H. B. (2017). lmerTest Package: tests in linear mixed effects models. J. Stat. Softw. 82, 1–26. doi: 10.18637/jss.v082.i13
Lenth, R. V (2022). Emmeans: estimated marginal Means, aka least-squares means. R package version 1.7.2., Available at: https://CRAN.R-project.org/package=emmeans
Leonard, M. L., and Horn, A. G. (2001). Begging calls and parental feeding decisions in tree swallows (Tachycineta bicolor). Behav. Ecol. Sociobiol. 49, 170–175. doi: 10.1007/s002650000290
Lin, H., Decuypere, E., and Buyse, J. (2006). Acute heat stress induces oxidative stress in broiler chickens. Comp. Biochem. Physiol. A Mol. Integr. Physiol. 144, 11–17. doi: 10.1016/j.cbpa.2006.01.032
Losdat, S., Helfenstein, F., Gaude, B., and Richner, H. (2011). Reproductive effort transiently reduces antioxidant capacity in a wild bird. Behav. Ecol. 22, 1218–1226. doi: 10.1093/beheco/arr116
Magrath, R. D. (1991). Nestling weight and juvenile survival in the blackbird, Turdus merula. J. Anim. Ecol. 60, 335–351. doi: 10.2307/5464
Marino, F. E., Mbambo, Z., Kortekaas, E., Wilson, G., Lambert, M. I., Noakes, T. D., et al. (2000). Advantages of smaller body mass during distance running in warm, humid environments. Pflugers Arch. Eur. J. Physiol. 441, 359–367. doi: 10.1007/s004240000432
McKechnie, A. E., and Wolf, B. O. (2019). The physiology of heat tolerance in small endotherms. Physiology 34, 302–313. doi: 10.1152/physiol.00011.2019
Mestre-Alfaro, A., Ferrer, M. D., Banquells, M., Riera, J., Drobnic, F., Sureda, A., et al. (2012). Body temperature modulates the antioxidant and acute immune responses to exercise. Free Radic. Res. 46, 799–808. doi: 10.3109/10715762.2012.680193
Monaghan, P., Metcalfe, N. B., and Torres, R. (2009). Oxidative stress as a mediator of life history trade-offs: mechanisms, measurements and interpretation. Ecol. Lett. 12, 75–92. doi: 10.1111/j.1461-0248.2008.01258.x
Muff, S., Nilsen, E. B., O’Hara, R. B., and Nater, C. R. (2022). Rewriting results sections in the language of evidence. Trends Ecol. Evol. 37, 203–210. doi: 10.1016/j.tree.2021.10.009
Nadav, N. (1984). The Consequences of Brood Size for Breeding Blue Tits I. Adult survival, weight change and the cost of reproduction. J. Anim. Ecol. 53, 479–496. doi: 10.2307/4529
Nilsson, J. Å., and Nord, A. (2018). Testing the heat dissipation limit theory in a breeding passerine. Proc. R. Soc. B Biol. Sci. 285:20180652. doi: 10.1098/rspb.2018.0652
Nimse, S. B., and Pal, D. (2015). Free radicals, natural antioxidants, and their reaction mechanisms. RSC Adv. 5, 27986–28006. doi: 10.1039/c4ra13315c
Nord, A., and Nilsson, J. Å. (2019). Heat dissipation rate constrains reproductive investment in a wild bird. Funct. Ecol. 33, 250–259. doi: 10.1111/1365-2435.13243
Ołdakowski, Ł., Piotrowska, Z., Chrząścik, K. M., Sadowska, E. T., Koteja, P., and Taylor, J. R. E. (2012). Is reproduction costly? No increase of oxidative damage in breeding bank voles. J. Exp. Biol. 215, 1799–1805. doi: 10.1242/jeb.068452
Olson, C. R., Wirthlin, M., Lovell, P. V., and Mello, C. V. (2014). Proper care, husbandry, and breeding guidelines for the zebra finchm, Taeniopygia guttata. Cold Spring Harb. Protoc. 2014, 1243–1248. doi: 10.1101/pdb.prot084780
Oswald, K. N., Smit, B., Lee, A. T. K., Peng, C. L., Brock, C., and Cunningham, S. J. (2021). Higher temperatures are associated with reduced nestling body condition in a range-restricted mountain bird. J. Avian Biol. 52, 1–10. doi: 10.1111/jav.02756
Oswald, S. A., and Arnold, J. M. (2012). Direct impacts of climatic warming on heat stress in endothermic species: seabirds as bioindicators of changing thermoregulatory constraints. Integr. Zool. 7, 121–136. doi: 10.1111/j.1749-4877.2012.00287.x
Pessato, A., McKechnie, A. E., Buchanan, K. L., and Mariette, M. M. (2020). Vocal panting: a novel thermoregulatory mechanism for enhancing heat tolerance in a desert-adapted bird. Sci. Rep. 10:18914. doi: 10.1038/s41598-020-75909-6
Peterson, C. C., Nagy, K. A., and Diamond, J. (1990). Sustained metabolic scope. Proc. Natl. Acad. Sci. U.S.A. 87, 2324–2328. doi: 10.1073/pnas.87.6.2324
Rahal, A., Kumar, A., Singh, V., Yadav, B., Tiwari, R., Chakraborty, S., et al. (2014). Oxidative stress, prooxidants, and antioxidants: the interplay. Biomed Res. Int. 2014:761264. doi: 10.1155/2014/761264
R Core Team (2021). R: A Language and Environment for Statistical Computing. Vienna: R Foundation for Statistical Computing.
Ricklefs, R. E. (1984). The optimization of growth rate in Altricial Birds. Ecology 65, 1602–1616. doi: 10.1242/jeb.114108
Rogowitz, G. L. (1998). Limits to milk flow and energy allocation during lactation of the hispid cotton rat (Sigmodon hispidus). Physiol. Zool. 71, 312–320. doi: 10.1086/515923
Ronget, V., Gaillard, J. M., Coulson, T., Garratt, M., Gueyffier, F., Lega, J. C., et al. (2018). Causes and consequences of variation in offspring body mass: meta-analyses in birds and mammals. Biol. Rev. 93, 1–27. doi: 10.1111/brv.12329
Rutkowska, J., Cichoń, M., Puerta, M., and Gil, D. (2005). Negative effects of elevated testosterone on female fecundity in zebra finches. Horm. Behav. 47, 585–591. doi: 10.1016/j.yhbeh.2004.12.006
Sadowska, J., Gêbczynski, A. K., Lewoc, M., and Konarzewski, M. (2019). Not that hot after all: no limits to heat dissipation in lactating mice selected for high or low BMR. J. Exp. Biol. 222:jeb204669. doi: 10.1242/jeb.204669
Schaper, S. V., and Visser, M. E. (2013). Great tits provided with ad libitum food lay larger eggs when exposed to colder temperatures. J. Avian Biol. 44, 245–254. doi: 10.1111/j.1600-048X.2012.00129.x
Scholander, P. F., Hock, R., Walters, V., and Irving, L. (1950). Adaptation to cold in arctic and tropical mammals and birds in relation to body temperature, insulation, and basal metabolic rate. Source Biol. Bull. 99, 259–271. doi: 10.2307/1538742
Schwagmeyer, P. L., and Mock, D. W. (2008). Parental provisioning and offspring fitness: size matters. Anim. Behav. 75, 291–298. doi: 10.1016/j.anbehav.2007.05.023
Selman, C., McLaren, J. S., Himanka, M. J., and Speakman, J. R. (2000). Effect of long-term cold exposure on antioxidant enzyme activities in a small mammal. Free Radic. Biol. Med. 28, 1279–1285. doi: 10.1016/S0891-5849(00)00263-X
Senar, J. C., and Pascual, J. (1997). Keel and tarsus length may provide a good predictor of avian body size. Ardea 85, 269–274.
Sies, H. (1997). Physiological society symposium: impaired endothelial and smooth muscle cell function in oxidative stress. Exp. Physiol. 82, 269–376.
Speakman, J. R., Ergon, T., Cavanagh, R., Reid, K., Scantlebury, D. M., and Lambin, X. (2003). Resting and daily energy expenditures of free-living field voles are positively correlated but reflect extrinsic rather than intrinsic effects. Proc. Natl. Acad. Sci. U.S.A. 100, 14057–14062. doi: 10.1073/pnas.2235671100
Speakman, J. R., and Garratt, M. (2014). Oxidative stress as a cost of reproduction: beyond the simplistic trade-off model. Bioessays 36, 93–106. doi: 10.1002/bies.201300108
Speakman, J. R., and Król, E. (2010a). Maximal heat dissipation capacity and hyperthermia risk: neglected key factors in the ecology of endotherms. J. Anim. Ecol. 79, 726–746. doi: 10.1111/j.1365-2656.2010.01689.x
Speakman, J. R., and Król, E. (2010b). The heat dissipation limit theory and evolution of life histories in endotherms-time to dispose of the disposable soma theory? Integr. Comp. Biol. 50, 793–807. doi: 10.1093/icb/icq049
Speakman, J. R., and Ward, S. (1998). Infrared thermography: principles and applications. Zoology 101, 224–232.
Speakman, J. R., and Selman, C. (2011). The free-radical damage theory: accumulating evidence against a simple link of oxidative stress to ageing and lifespan. BioEssays 33, 255–259. doi: 10.1002/bies.201000132
Stier, A., Schull, Q., Bize, P., Lefol, E., Haussmann, M., Roussel, D., et al. (2019). Oxidative stress and mitochondrial responses to stress exposure suggest that king penguins are naturally equipped to resist stress. Sci. Rep. 9:8545. doi: 10.1038/s41598-019-44990-x
Sudyka, J., Casasole, G., Rutkowska, J., and Cichoń, M. (2016). Elevated reproduction does not affect telomere dynamics and oxidative stress. Behav. Ecol. Sociobiol. 70, 2223–2233. doi: 10.1007/s00265-016-2226-8
Tapper, S., Nocera, J. J., and Burness, G. (2020a). Experimental evidence that hyperthermia limits offspring provisioning in a temperate-breeding bird: hyperthermia influences parental care. R. Soc. Open Sci. 7:201589. doi: 10.1098/rsos.201589
Tapper, S., Nocera, J. J., and Burness, G. (2020b). Heat dissipation capacity influences reproductive performance in an aerial insectivore. J. Exp. Biol. 223:jeb222232. doi: 10.1242/jeb.222232
Thurber, C., Dugas, L. R., Ocobock, C., Carlson, B., Speakman, J. R., and Pontzer, H. (2019). Extreme events reveal an alimentary limit on sustained maximal human energy expenditure. Sci. Adv. 5:eaaw0341. doi: 10.1126/sciadv.aaw0341
Tsahar, E., Arad, Z., Izhaki, I., and Guglielmo, C. G. (2006). The relationship between uric acid and its oxidative product allantoin: a potential indicator for the evaluation of oxidative stress in birds. J. Comp. Physiol. B Biochem. Syst. Environ. Physiol. 176, 653–661. doi: 10.1007/s00360-006-0088-5
Tucker, R., and Noakes, T. D. (2009). The physiological regulation of pacing strategy during exercise: a critical review. Br. J. Sports Med. 43:e1. doi: 10.1136/bjsm.2009.057562
Ummenhofer, C. C., and Meehl, G. A. (2017). Extreme weather and climate events with ecological relevance: a review. Philos. Trans. R. Soc. B Biol. Sci. 372:20160135. doi: 10.1098/rstb.2016.0135
van de Crommenacker, J., Richardson, D. S., Koltz, A. M., Hutchings, K., and Komdeur, J. (2012). Parasitic infection and oxidative status are associated and vary with breeding activity in the Seychelles warbler. Proc. R. Soc. B Biol. Sci. 279, 1466–1476. doi: 10.1098/rspb.2011.1865
Ward, S., Rayner, J. M. V., Möller, U., Jackson, D. M., Nachtigall, W., and Speakman, J. R. (1999). Heat transfer from starlings Sturnus vulgaris during flight. J. Exp. Biol. 202, 1589–1602. doi: 10.1242/jeb.202.12.1589
Weiner, J. (1992). Physiological limits to sustainable energy budgets in birds and mammals. Trends Ecol. Evol. 7, 384–388. doi: 10.1016/0169-5347(92)90009-Z
West, G. B., Brown, J. H., and Enquist, B. J. (1999). The fourth dimension of life: fractal geometry and allometric scaling of organisms. Science 284, 1677–1679. doi: 10.1126/science.284.5420.1677
Wiersma, P., Selman, C., Speakman, J. R., and Verhulst, S. (2004). Birds sacrifice oxidative protection for reproduction. Proc. R. Soc. B Biol. Sci. 271, 360–363. doi: 10.1098/rsbl.2004.0171
Williams, T. D., and Cooch, E. G. (1996). Egg Size, Temperature and Laying Sequence: Why do Snow Geese Lay Big Eggs When it’s cold? Funct. Ecol. 10, 112–118. doi: 10.2307/2390269
Williamson, K., Gilbert, L., Rutstein, A. N., Pariser, E. C., and Graves, J. A. (2008). Within-year differences in reproductive investment in laboratory zebra finches (Taeniopygia guttata), an opportunistically breeding bird. Naturwissenschaften 95, 1143–1148. doi: 10.1007/s00114-008-0436-2
Yang, D. B., Xu, Y. C., Wang, D. H., and Speakman, J. R. (2013). Effects of reproduction on immuno-suppression and oxidative damage, and hence support or otherwise for their roles as mechanisms underpinning life history trade-offs, are tissue and assay dependent. J. Exp. Biol. 216, 4242–4250. doi: 10.1242/jeb.092049
Yap, K. N., Powers, D. R., Vermette, M. L., Tsai, O. H. I., and Williams, T. D. (2021). Physiological adjustments to high foraging effort negatively affect fecundity but not final reproductive output in captive zebra finches. J. Exp. Biol. 224:jeb235820. doi: 10.1242/JEB.235820
Yap, K. N., Kim, O. R., Harris, K. C., and Williams, T. D. (2017). Physiological effects of increased foraging effort in a small passerine. J. Exp. Biol. 220, 4282–4291. doi: 10.1242/jeb.160812
Zagkle, E., Grosiak, M., Bauchinger, U., and Sadowska, E. T. (2020). Rest-phase hypothermia reveals a link between aging and oxidative stress: a novel hypothesis. Front. Physiol. 11:575060. doi: 10.3389/fphys.2020.575060
Keywords: heat dissipation limit, reproduction, oxidative stress, sustained energy, nestling provisioning, laboratory avian model, temperature manipulation
Citation: Zagkle E, Martinez-Vidal PA, Bauchinger U and Sadowska ET (2022) Manipulation of Heat Dissipation Capacity Affects Avian Reproductive Performance and Output. Front. Ecol. Evol. 10:866182. doi: 10.3389/fevo.2022.866182
Received: 30 January 2022; Accepted: 21 March 2022;
Published: 14 April 2022.
Edited by:
David L. Swanson, University of South Dakota, United StatesReviewed by:
Simon Tapper, Ontario Ministry of Natural Resources and Forestry, CanadaYufeng Zhang, University of Memphis, United States
Copyright © 2022 Zagkle, Martinez-Vidal, Bauchinger and Sadowska. This is an open-access article distributed under the terms of the Creative Commons Attribution License (CC BY). The use, distribution or reproduction in other forums is permitted, provided the original author(s) and the copyright owner(s) are credited and that the original publication in this journal is cited, in accordance with accepted academic practice. No use, distribution or reproduction is permitted which does not comply with these terms.
*Correspondence: Elisavet Zagkle, ZWxpc2F2ZXQuemFna2xlQGRvY3RvcmFsLnVqLmVkdS5wbA==