- 1Department of Zoology and Entomology, University of Pretoria, Hatfield, South Africa
- 2Mammal Research Institute, University of Pretoria, Hatfield, South Africa
- 3Department of Biological Sciences, University of Manitoba, Winnipeg, MB, Canada
- 4Institute of Biological and Environmental Sciences, University of Aberdeen, Aberdeen, United Kingdom
- 5School of Biological Sciences, Queen’s University Belfast, 19 Chlorine Gardens, Belfast, United Kingdom
The doubly labeled water (DLW) technique and indirect calorimetry enable measurement of an animal’s daily energy expenditure (DEE, kJ/day), resting metabolic rate (RMR, kJ/d), sustained metabolic scope (SusMS), body fat content (BF, %) as well as water turnover (WTO, ml/day), and water economy index (ml/kJ). Small mammals have been the primary focus of many of the DLW studies to date. From large multi-species analyses of the energetics and water flux of aboveground small mammals, well-defined trends have been observed. These trends mainly refer to an adaptive advantage for lower RMR, DEE, SusMS, WTO and WEI in more ariddwelling animals to increase water and energy savings under low and unpredictable resource availability. The study of the subterranean rodent family Bathyergidae (African mole-rats) has been of particular interest with regards to field metabolic rate and metabolic studies. Although a great deal of research has been conducted on the Bathyergidae, a complete overview and multi-species analysis of the energetics and water flux of this family is lacking. Consequently, we assessed DEE, RMR, SusMS, BF, WTO and WEI across several different species of bathyergids from various climatic regions, and compared these to the established patterns of energetics and water flux for aboveground rodents. There was notable variation across the Bathyergidae inhabiting areas with different aridities, often contrary to the variations observed in above-ground species. These include increased DEE and WEI in arid-dwelling bathyergid species. While the climate was not a clear factor when predicting the SusMS of a bathyergid species, rather the degree of group living was a strong driver of SusMS, with solitary species possessing the highest SusMS compared to the socially living species. We conclude that the constraints of the underground lifestyle and the consequent spectrum of social behaviors possessed by the family Bathyergidae are most likely to be more crucial to their energetics and water flux than their habitat; however other important unstudied factors may still be at play. More so, this study provides evidence that often unreported parameters, measured through use of the DLW technique (such as BF and WEI) can enable species to be identified that might be at particular risk to climate change.
Introduction
The doubly labeled water (DLW) technique has been a powerful tool for measuring free-living energy expenditure in animals since its development in 1955 (Lifson et al., 1955; Lifson and McClintock, 1966; Speakman, 1997a). This technique has enabled great strides in understanding how biotic and abiotic pressures shape the daily energy expenditure (DEE) of animals whilst undertaking natural behaviors in the field. However, many other important biological parameters can be calculated using DLW methodology, which involves isotope dilution and elimination. Such aspects include water turnover (WTO), which is the rate that water leaves the body of an animal (‘efflux’), water economic index (WEI), which is the ratio of WTO to DEE (Nagy, 2004), and body fat content (BF) which can be calculated by the body water dilution space (e.g., Scantlebury et al., 2006a). Furthermore, when DEE is combined with the measurements of resting metabolic rate (RMR), through the use of indirect calorimetry, one can determine measures of sustained metabolic scope (SusMS, DEE/RMR), which is an independent index of how hard an animal is working (Drent and Daan, 1980; Peterson et al., 1990; Hammond and Diamond, 1997). Determinations of both WEI and SusMS are useful as they enable comparisons between different species to be made (Peterson et al., 1990; Hammond and Diamond, 1997; Nagy, 2004). However, in most cases, researchers only report some of the biological parameters that can be calculated through the use of DLW and RMR measurements, which can restrict our understanding of the selection pressures that shape an animal’s energy balance (DEE, RMR, and SusMS), health (BF) as well as water balance (WTO and WEI).
In particular, small mammals, such as rodents, have been the primary focus of much of the DLW research due to their ease of capture in the wild and requirements for a lower (and less expensive) DLW dose (Speakman and Król, 2005). For several reasons, the rodent family Bathyergidae (African mole-rats) has been a group of animals of particular interest for researchers. Apart from being an exclusively subterranean rodent family, they also exhibit varying degrees of sociality ranging from solitary to social to even eusocial (Jarvis and Bennett, 1993; Faulkes et al., 1997; Bennett and Faulkes, 2000; Burland et al., 2002). Social and eusocial bathyergids exhibit cooperative breeding and a reproductive division of labor in which a single breeding female and one to three of the largest males breed within the colony (Bennett and Faulkes, 2000). The remaining colony members are reproductively suppressed, and a marked division of labor, with breeders spending approximately half as much time foraging as their non-breeding counterparts, is apparent (Scantlebury et al., 2006c; Lacey and Sherman, 2009; Oosthuizen and Bennett, 2015; Francioli et al., 2020; Houslay et al., 2020). Furthermore, they persist over a wide range of climates, elevations and substrates from both mesic and arid environments, with behavioral and physiological adaptations being suggested to correspond to the macroclimate they experience (Bennett et al., 1988; Faulkes et al., 2004; McGowan et al., 2020; Wallace et al., 2021). For example, species of African mole-rats that inhabit soft sandy substrates with a mesic climate are often solitary, possess a larger body masses and use both their teeth and forelimbs, “scratch digging”, to dig, such as those species belonging to the genus of Bathyergus (Bennett and Faulkes, 2000). In contrast, species that inhabit arid areas with harder substrates are often smaller, social, and utilize “chisel-tooth” digging to extend burrows (Bennett and Faulkes, 2000). The family also hosts a range of thermoregulatory abilities, from endothermic homeothermy (Šumbera, 2019) to heterothermy (Bennett et al., 1993; Boyles et al., 2012; Oosthuizen et al., 2021) and poikilothermy (Buffenstein and Yahav, 1991; Cheng et al., 2021) which has been observed to affect their behavior (Hart et al., 2021; Finn et al., 2022).
The subterranean niche provides a relatively thermostable environment (Bennett et al., 1988, 1993; Šumbera et al., 2004; Holtze et al., 2018; Šumbera, 2019) as well as a shelter from aboveground predators and temperature extremes (Bennett and Faulkes, 2000; Finn et al., 2020). However, burrow systems have hypoxic and hypercapnic atmospheres with poor ventilation, high humidity, and limited access to light (Kennerly, 1964; Darden, 1972; Roper et al., 2001; Ivy et al., 2020; Logan et al., 2020). In addition, excavation of tunnel systems is energetically more expensive than aboveground exploration (Vleck, 1979), with energy requirements of digging directly related to soil moisture and hardness (Lovegrove, 1989; Thomas et al., 2009, 2016). The added energy expenditure necessary for digging, in conjunction with the fact that African mole-rats obtain all their nutrient and water requirements from the storage organs of underground geophytes (Bennett et al., 1994; Bennett and Jarvis, 1995; Spinks et al., 1999; Malherbe et al., 2003), has been suggested to exacerbate the selection pressures placed upon African mole-rats to enable them to locate adequate resources for survival and reproduction (Bennett and Faulkes, 2000). The energetic cost of digging under the unique microclimate within the tunnels they inhabit has been hypothesized to lead to lower RMRs and body temperatures displayed by African mole-rats in comparison to aboveground rodent species (McNab, 1966; Shkolnik and Schmidt-Nielsen, 1976; Bennett et al., 1992, Bennett et al., 1993, 1994; McGowan et al., 2020) and a social and eusocial lifestyle in species inhabiting very arid environments [the ‘Arid-Food-Distribution-Hypothesis’ Jarvis et al. (1994); Lacey and Sherman (1997)].
Studies measuring the DEE and RMR in various species of African mole-rat (Table 1) have revealed insights into the biotic and abiotic pressures that influence the energy fluxes of these species. For example, Scantlebury et al. (2006c) revealed distinct physiological castes related to body fat and dispersal likelihood. Both Scantlebury et al. (2006c) and Zelová et al. (2011) showed that seasonal fluctuation in soil moisture due to the seasonal rainfall affects DEE and SusMS in a eusocial and solitary mole-rat species, respectively. To-date, only one study has directly compared the ecological relevance of energetic differences between two species of African mole-rat (Scantlebury et al., 2006b). This study concluded that the energy costs of sexual dimorphism are likely to be due to the maintenance of a larger body size in males, rather than sex-differences in behavior per se. This study also compared an exclusive chisel tooth digger with a forelimb (scratch digger) that also uses tooth digging and found that the latter species possessed a higher SusMS (i.e., it worked ‘harder’) than the exclusive chisel tooth digger. However, as in other studies that measured DEE and RMR, not all possible biological parameters were investigated.
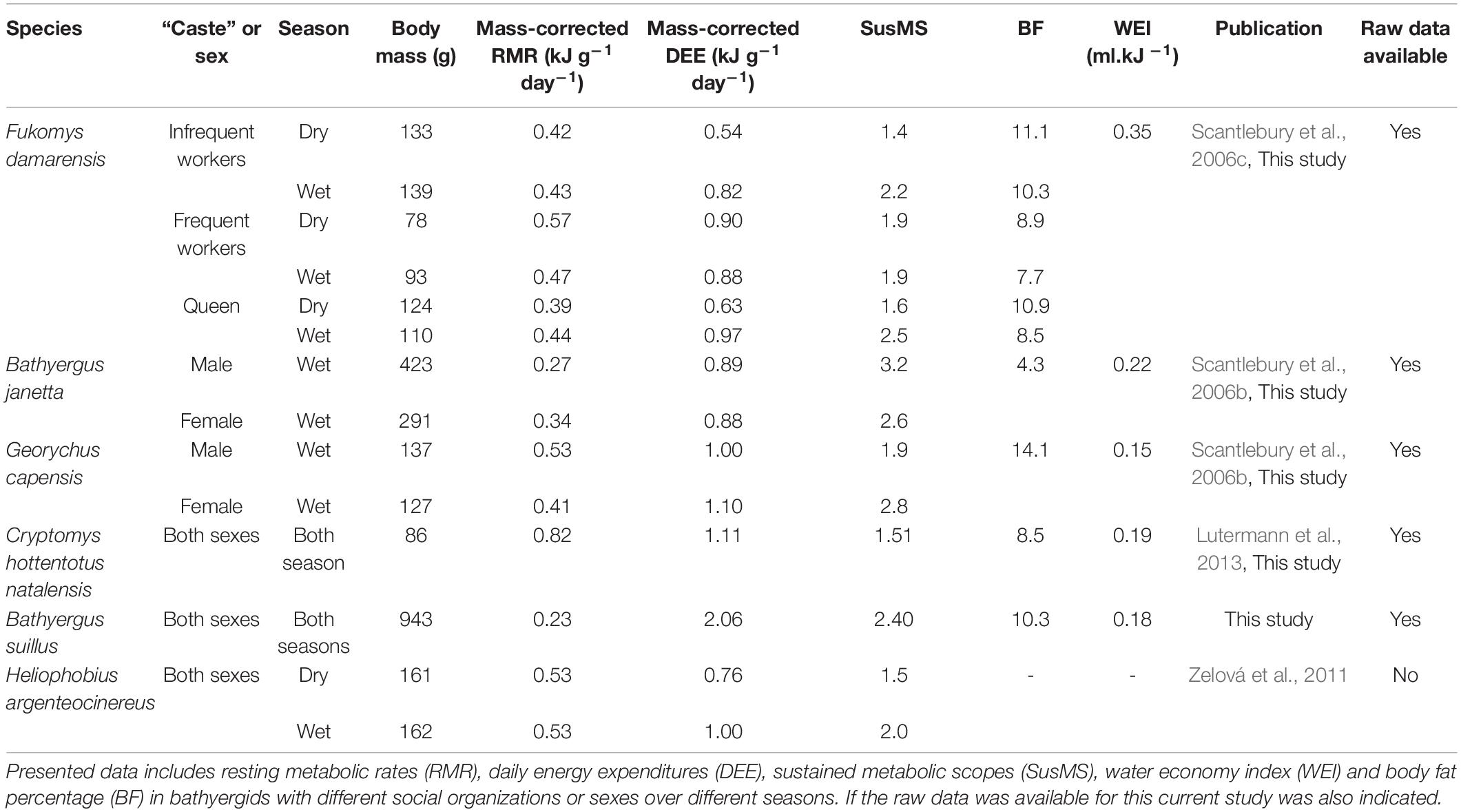
Table 1. Overview of previously published and original data that utilized the DEE methodology on various African mole-rat species.
Although a great deal of research has been conducted on the Bathyergidae family, a complete overview and multi-species analysis of the energetics and water flux of the family is still absent. Consequently, we assessed a combination of energetic parameters, namely DEE, RMR, SusMS, BF, WTO, and WEI, across several different species of bathyergid mole-rats (Tables 1, 2). We also include previously unpublished energetics data on the largest African mole-rat species, Bathyergus suillus, and data from two sympatric terrestrial aboveground rodent species for comparison (Table 2).
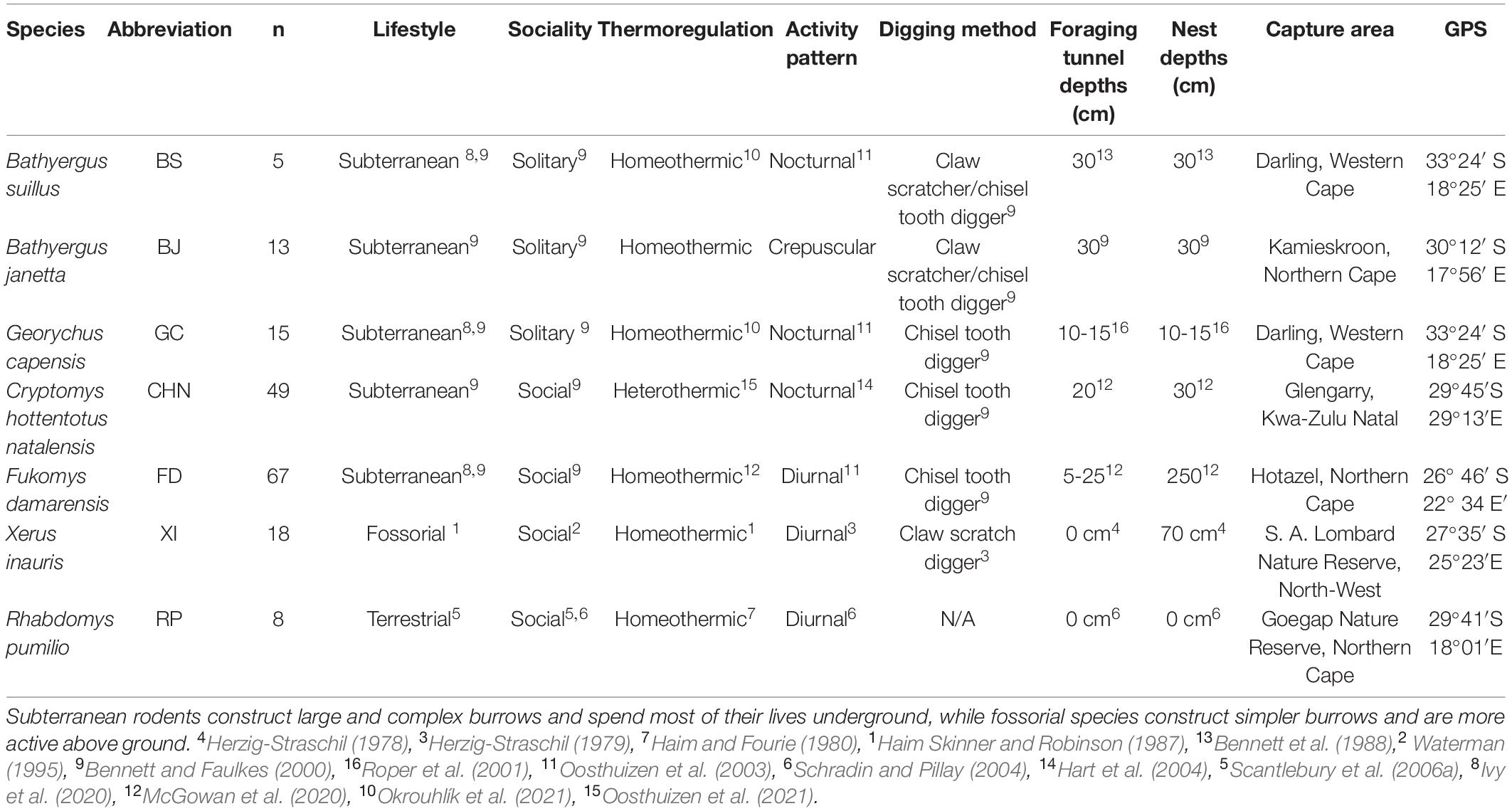
Table 2. Scientific names and life history characteristics of the five mole-rat species and two aboveground foraging rodent species used in this study.
This overview aimed to highlight various features that drive the existing variation in energetics and water flux within the family Bathyergidae. As DEE, RMR, and SusMS of certain species have been described previously; we focus on inter-specific comparisons and the previously understudied biological parameters of BF, WTO, and WEI. Furthermore, this current study also attempts to compare the well-known patterns of energetics and water flux for aboveground rodents and other small mammals (Scantlebury et al. (2002, 2008); Scantlebury and Haim, 2012) against those found in the African mole-rats. Extensive multi-species analyses of both the energetics and water flux are present for aboveground small mammals (see Nagy et al. (1999) for an overview of the energetics of terrestrial mammals and Nagy (2004) for an overview of the water flux of terrestrial mammals). At both the inter- and intra-specific levels, aboveground mammals inhabiting drier (more arid) environments display consistent differences in their energetics and water flux compared to their counterparts in mesic or wetter (less arid) habitats (Nagy et al., 1999; Nagy, 2004). These differences include lower RMR, DEE and WEI in mammals inhabiting arider environments than those inhabiting more mesic environments (Speakman, 1997a; Nagy et al., 1999; Nagy, 2004). Even though African mole-rats are exclusively subterranean and thus are buffered from some of the macroclimatic (the region they inhabit broad climate) variations, recent studies have suggested that the macroclimatic differences experienced by different mole-rat species affect their physiology (Šumbera, 2019; McGowan et al., 2020; Wallace et al., 2021). As such it can be expected that similar trends in the energetic parameters, including RMR, DEE and WEI, between the African mole-rat species may be observed as seen between small aboveground mammals. This would include lower RMR, DEE, and WEI in mole-rats inhabiting arid regions compared to those inhabiting mesic environments (Speakman, 1997a; Nagy et al., 1999; Nagy, 2004). Alternatively, the microclimatic conditions found in African mole-rat burrows (the subterranean niche) may result in contrasting energetic patterns; specifically, all African mole-rats may possess similar energetic parameters regardless of their experienced macroclimate on account of their shared buffered lifestyle and behavioral adaptations (for example, social living). Furthermore, African mole-rats would then be expected to possess lower RMR, and similar WTO and WEI than the aboveground rodents in this study due to living in a buffered microclimate. At the same time, African mole-rats would also be predicted to have higher DEE, SusMS and BF values compared to the aboveground rodents as the result of increased energy expenditure due to digging.
Materials and Methods
Novel Data Analysis
Study Site and Animal Capture
A total of 11 Cape dune mole-rats (B. suillus – ‘BS’) were captured with live Hickman traps (Hickman, 1979) on two separate occasions, August 2003 and June 2007, near the village of Darling (33°22′S 15°25′ E) in the Western Cape, South Africa. The Western Cape region has predictable winter (May-September) rainfall and moderate temperatures throughout the year (Rutherford and Westfall, 1994). All individuals were captured prior to their respective breeding season (Hart et al., 2006). Using growth curve data (D. Hart unpublished work), all but one individual was defined as an adult (body mass > 350grams). Individuals were not anaesthetized and were handled in cotton bags to minimize stress. Trapping occurred throughout the day; animals were transported to a field laboratory close by (less than 2 km) upon capture. Animals were housed in plastic containers 30 cm in diameter, 50 cm in height, with sawdust as bedding and sweet potatoes provided ad libitum as food overnight. On completing the experiments, the animals were returned to their original capture sites. All experimental procedures and animal husbandry practices were approved by the Animal Ethics Committee, University of Pretoria.
Daily Energy Expenditure and Water Turnover Measurements in Bathyergus suillus
The DEEs (kJ d–1) of 6 Cape dune mole-rats (B. suillus) were measured using the DLW technique (Lifson and McClintock, 1966; Speakman, 1997b). Upon capture, individuals were taken to a field laboratory, where their RMR values were determined (below). The following morning (c. 09:00), they were weighted (± 0.1 g Sartorius balance), and a 0.2 ml blood sample was obtained from the cephalic vein in a foot to estimate the background isotope enrichments of 2H and 18O. Blood samples were immediately heat-sealed into 4 × 50 μL glass capillaries and stored at room temperature. Thereafter, a known mass of DLW (100 g 95% APE enriched 18O water (Rotem Industries Ltd., Beer Sheva, Israel) and 50g 99.9% APE enriched 2H water (Isotec, Inc. Miamisburg OH, United States) mixed with 342 g 1H216O) was administered (IP, 0.3 g/100 g body mass). Syringes were weighed before and after administration (± 0.0001 g, Sartorius balance) to calculate the mass of DLW injected. Blood samples were taken after 2 h to estimate the initial isotope enrichments, and subsequently, the animals were released at their original capture sites. Animal recaptures (via re-setting traps) were attempted between 2 and 5 days post-injection of DLW. Traps were set at approximately 06:30 h on the intended day of recapture in an attempt to capture them before 09.00 hrs so that measurements of isotope turnover would be for whole 24 h periods (Speakman and Racey, 1988). Six out of the 11 injected individuals were recaptured. These were five adults (two males and three females) and one juvenile. Recaptured animals were weighed, and final blood samples (0.2 ml) were taken. Capillary tubes that contained blood samples were then vacuum distilled (Nagy, 1983), and water from the resulting distillate was used to produce CO2 and H2 (methods in Speakman (1997b) for CO2 and Speakman et al. (2004) for H2). The isotope ratios 18O:16O and 2H:1H were then analyzed using gas source isotope ratio mass spectrometry (Optima, Micromass IRMS and Isochrom mG, Manchester, United Kingdom), before calculation of DEE.
Resting Metabolic Rate (RMR) Measurements in Bathyergus suillus
All 11 BS were weighed (± 0.1 g Sartorius balance), and their RMR was measured upon initial capture. An open-circuit respirometry system (Depocas and Hart, 1957; Hill, 1972) was used. A metabolic chamber (6912 cm3) was immersed in a temperature-controlled water bath (Labotec) and maintained at 28–29°C (LAUDA, Konigshofen, Germany) within the thermoneutral zone of the species. Dried air was pumped into the chamber at a rate of approximately 900 ml min–1. A flow regulator that controlled airflow (F900, Applied Electrochemistry, AEI Technologies, Inc. United States) was placed upstream of the metabolism chamber. Measurements of VO2 were taken using an oxygen analyzer (S-2A Applied Electrochemistry, AEI Technologies, Inc. United States). The analyzer was calibrated to an upper value (20.95% O2) prior to the measurement of each animal and a lower value (0% O2 in N2 gas, AFROX, South Africa) every two weeks. After an initial hour in which animals were observed to settle in the respirometry chamber, measurements were taken every minute for half an hour. The mean of the lowest ten readings of oxygen consumption (mlO2h–1) was taken when animals were seen to be at rest (Bennett et al., 1992). Measurements were carried out between 11.00 and 17.00. Results were corrected to standard temperature and pressure.
Data Analysis
Sustained metabolic scope (SusMS = DEE/RMR) for each recaptured animal was calculated (n = 6) (Drent and Daan, 1980). The ratio of water efflux (ml/day) relative to energy expenditure (kJ/day), the Water Economy Index (WEI, ml/kJ), was calculated (Nagy, 2004). Body fat percentage (BF) was calculated using isotope dilution (Scantlebury et al., 2005). The juvenile BS was excluded from all statistical analyses, but the data are presented for visual comparison with the adult BS. The response variables’ normality was determined using Shapiro Wilk (S–W) tests and residual plots. Homogeneity of all dependent variables was confirmed with a Levene’s test. All variables were normally distributed. An two-sample t-test was used to compare the body mass of males and females.
Linear regression lines were fitted to determine the relationship between body mass and DEE, RMR and WTO, respectively, in adult BS. Due to the low sample size of male recaptures, only descriptive analyses were possible for DEE, SusMS, WTO, WEI and BF. The effect of sex on RMR of adult BS was investigated using a linear model (LM) with body mass as a covariate. Linear regression lines were fitted to explore the relationships between body mass and RMR in male and female adult BS. IBM SPSS 28 (IBM Corp., Armonk, NY, United States) was used for all statistical analyses and significance was assumed at p ≤ 0.05. All data are presented as mean ± SE.
Multi-Species Analyses
Only studies that possessed both DEE and RMR were included in the analyses. Table 1 shows the mole-rat species for which raw data were available for analysis. Additionally, raw data for outgroup rodent species were available for this study, namely, the Cape ground squirrel (Xerus inauris; Scantlebury et al., 2007) and four-striped mouse (Rhabdomys pumilio; Scantlebury et al., 2006a). Table 2 presents the general biological, ecological and behavioral information about each rodent species used in this study. IBM SPSS 28 (IBM Corp., Armonk, NY, United States) was used for all statistical analyses and significance was assumed at p ≤ 0.05. The normality of the response variables (RMR, DEE, SusMS, WTO, WEI, and BF) was determined using Shapiro Wilk tests (S–W) and residual plots. Homogeneity of all dependent variables was confirmed with a Levene’s test. All non-normally distributed dependent variables were log-transformed in an attempt to obtain a normal distribution.
Species Comparison
Linear regressions were fitted to examine the relationships between body mass with RMR, DEE and WTO, respectively, for all species combined and each species separately. We used generalized linear models (GLMs), with Gamma log-link distribution, or LMs, to examine differences in body mass, SusMS, WEI, and BF among species. Subsequently, GLMs or LMs, with body mass as a covariate, were used to examine differences in DEE, RMR, and WTO among species. Subsequent post-hoc analyses were conducted using LSD using estimated marginal means (EMMs). All data are presented as adjusted means (EMMs) ± SE.
Environmental Conditions
Climate data were gathered using the methods outlined by Wallace et al. (2021). All environmental conditions are presented in Table 3. Climate data for each site were retrieved from ERA5-Land of the European Centre for Medium-Range Weather Forecasts-the latest generation created by the Copernicus Climate Change Service (Muñoz-Sabater et al., 2021). The spatial (resolution is 0.1° by 0.1°). These data were used to calculate an annual aridity index (AI) (eq. (1)). Whereas total precipitation (tp) was directly obtained from ERA5-Land, potential evapotranspiration (PET) was calculated from the well-known Romanenko estimation (eq. (2)) (Romanenko, 1961). For eq. (2), relative humidity (RH) was calculated from ERA5-Land d2m (eq. (3)).
Each environmental condition (Table 3) was analyzed separately using a GLM, Gamma log-link distribution, and the site as the primary predictor. Subsequent post-hoc analyses were conducted using LSD using estimated marginal means (EMMs). All data are presented as mean ± SD.
Results
Novel Species Analysis - Bathyergus suillus
Male B. suillus, on average, were 165.2% heavier than female BS (t = −5.406, p = 0.001, Figure 1A). When both sexes were combined, there was a strong effect of body mass on RMR; the linear fitted regression
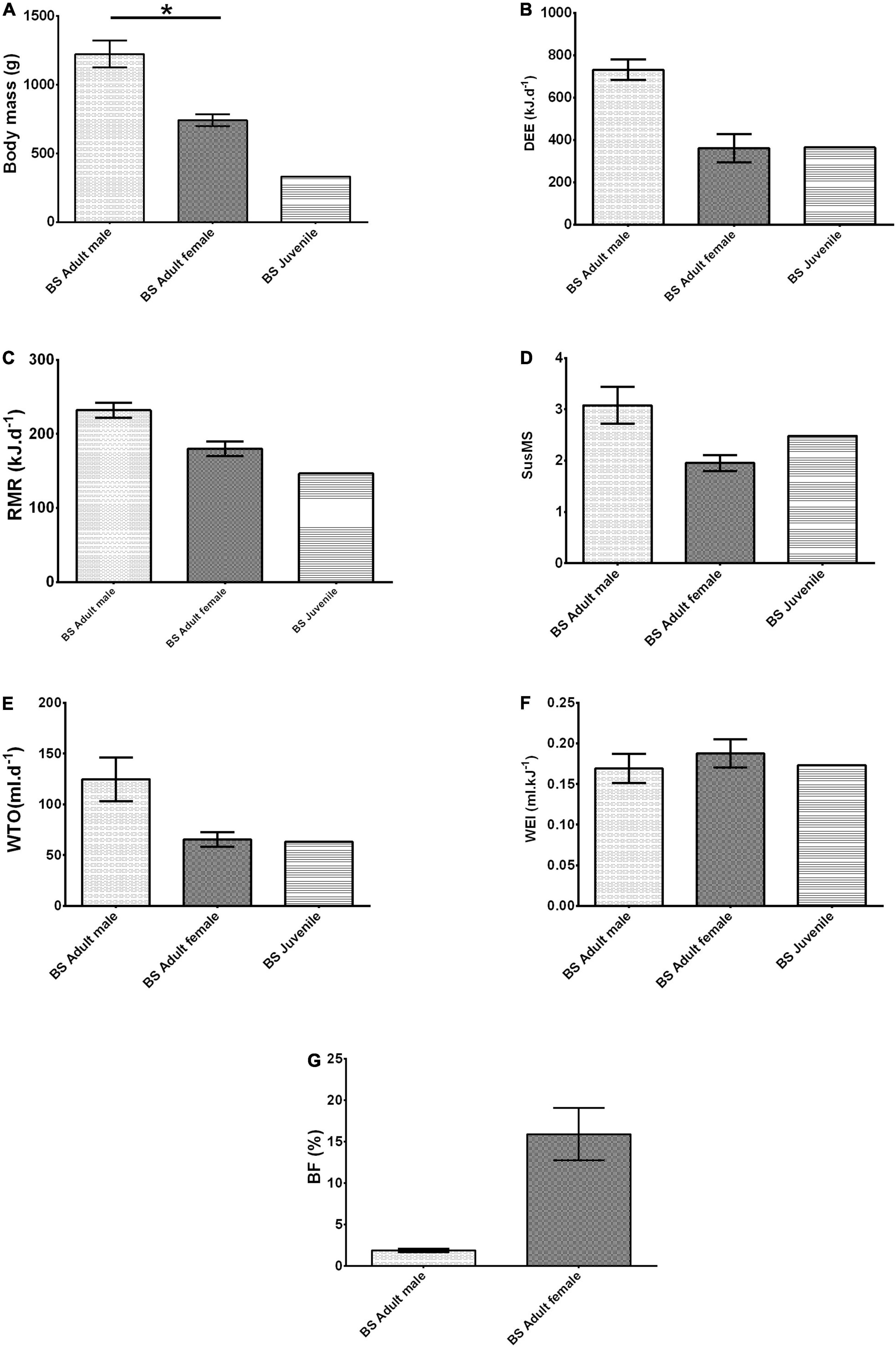
Figure 1. (A) Body mass (g), (B) daily energy expenditure (DEE- kJ.d– 1), (C) resting metabolic rate (RMR- kJ.d– 1), (D) sustained metabolic scope (SusMS), (E) water turnover (WTO- ml.d– 1), (F) water economy index (WEI- ml.kJ– 1), and (G) body fat percent (BF-%) of adult (male and female) and juvenile Bathyergus suillus (BS). Data are shown as Adjusted mean (EMMs) ± SE. An asterisk indicates significance (p ≤ 0.05).
significantly explained the variance (F = 16.8, p = 0.004, Figure 2A). Likewise, when both sexes were combined, there was a strong effect of body mass on DEE; the linear fitted regression
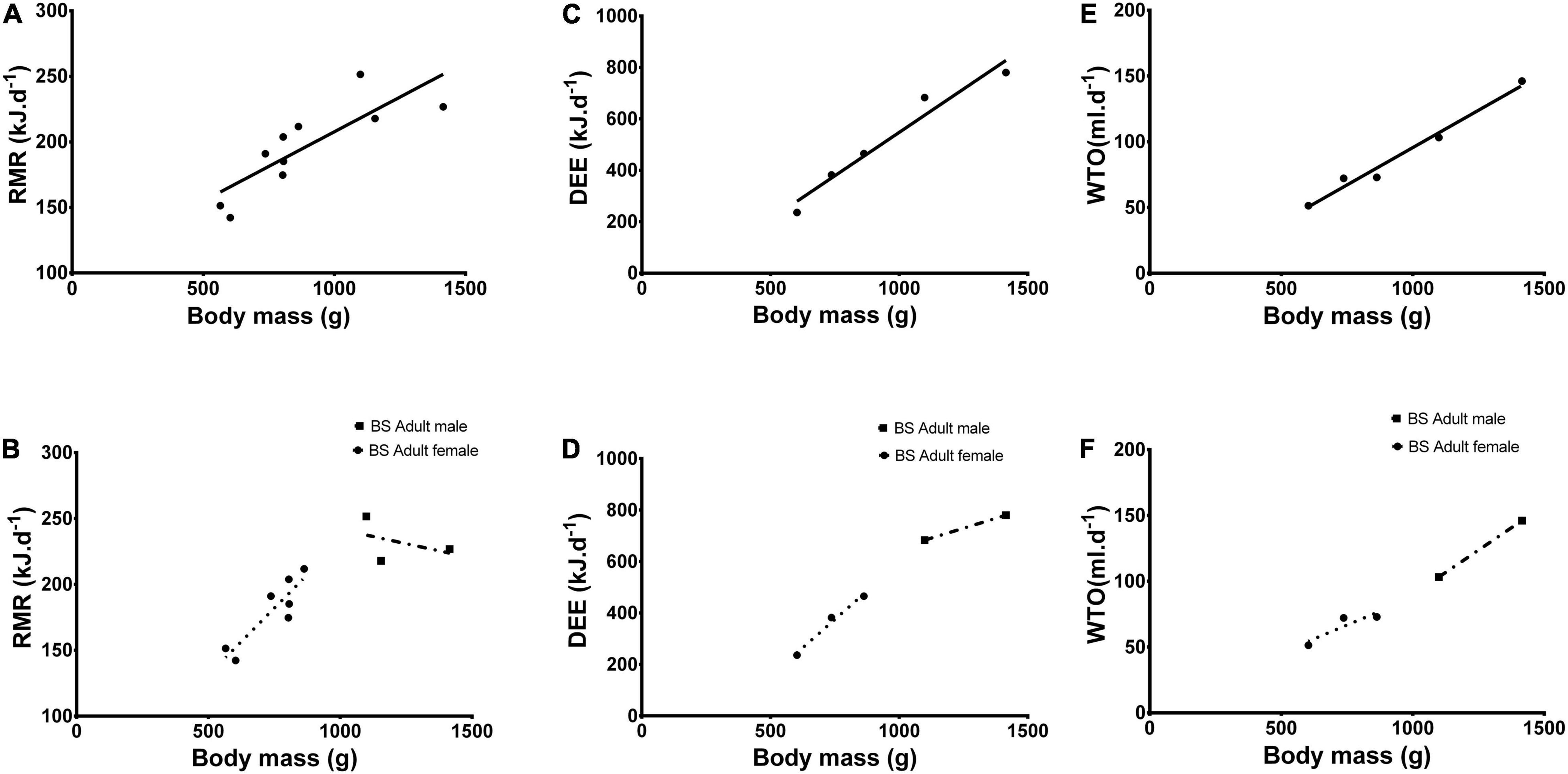
Figure 2. (A) Resting metabolic rate (RMR- kJ.d– 1), (C) daily energy expenditure (DEE- kJ.d– 1) and (E) water turn over (WTO- ml.d– 1) against body mass (g) of adult Bathyergus suillus (BS). (B) RMR, (D) DEE and (F) against male and female adult Bathyergus suillus (BS) body mass. Solid circles (•) and solid squares (■) represent female and male adult Bathyergus suillus (BS), respectively.
significantly explained the variance (F = 61.6, p = 0.004, Figure 2C).
Furthermore, when both sexes were combined, there was a strong effect of body mass on WTO; the linear fitted regression
significantly explained the variance (F = 135.2, p = 0.001, Figure 2E).
Neither sex (F = 9.78, p = 0.89, Figure 1C) nor body mass (F = 1291.1, p = 0.14) affected RMR. Interestingly, in females there was a strong effect of body mass on RMR;the linear fitted regression
significantly explained the variance (F = 18.9, p = 0.007, Figure 2B). While, body mass did not have a significant effect on RMR in males (F = 0.21, p = 0.73, Figure 2B) but did possess a negative relationship with RMR (Slope:−0.04, Figure 2B).
A similar trend was seen in DEE (Figure 1B) as in RMR, with increased DEE in adult male B. suillus compared to adult female B. suillus. Likewise, adult male B. suillus possess a higher SusMS than adult female B. suillus (Figure 1D). Similar values of WEI occurred for both male and female adult B. suillus (Figure 1F), while higher WTO was observed in adult male B. suillus compared to adult female B. suillus (Figure 1E). In addition, both male and female adult B. suillus DEE and WTO showed a positive relationship with body mass (Figures 2D,F). Contrastingly, BF of adult female B. suillus was higher than adult male B. suillus (Figure 1G).
Multi-Species Analysis
Species Comparison
Body Mass
All species possessed significantly different body masses from each other (Figure 3A and Supplementary Tables 1, 2).
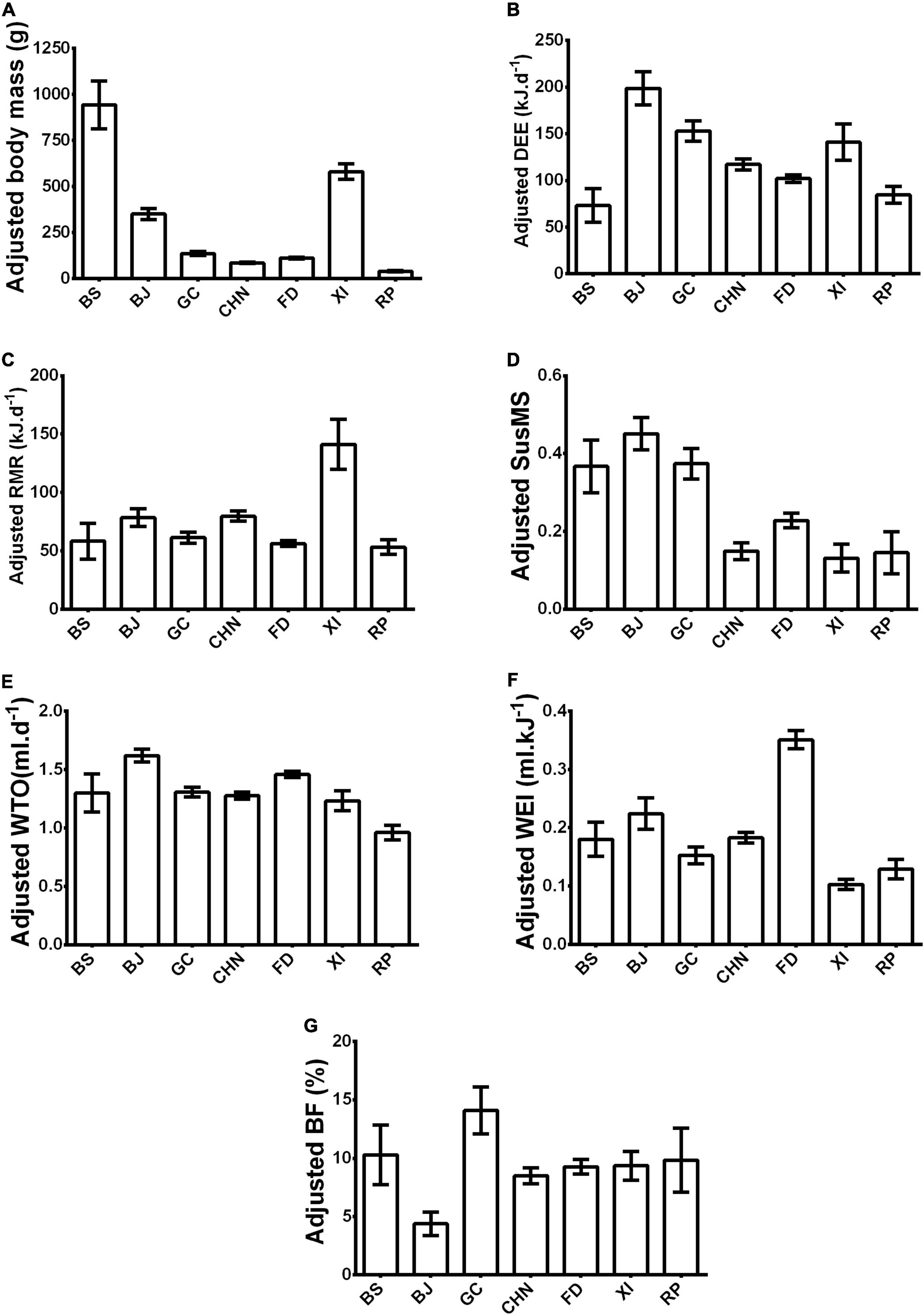
Figure 3. (A) Body mass (g), (B) daily energy expenditure (DEE- kJ.d– 1), (C) resting metabolic rate (RMR- kJ.d– 1), (D) sustained metabolic scope (SusMS), (E) water turnover (WTO- ml.d– 1), (F) water economy index (WEI- ml.kJ– 1), and (G) body fat percent (BF- %) of all seven species of rodent featured in this study. BS - Bathyergus suillus; BJ: Bathyergus janetta; GC - Georychus capensis; CHN - Cryptomys hottentotus natalensis; FD - Fukomys damarensis; XI - Xerus inauris; RP - Rhabdomys pumilio. Data are shown as adjusted mean(EMMs) ± SE.
Daily Energy Expenditure
Both species and body mass significantly affected DEE (Supplementary Table 1). When all species were combined, there was a strong effect of body mass on DEE; the linear fitted regression
significantly explained the variance (F = 1222,0, p < 0.0001, Figure 4A).
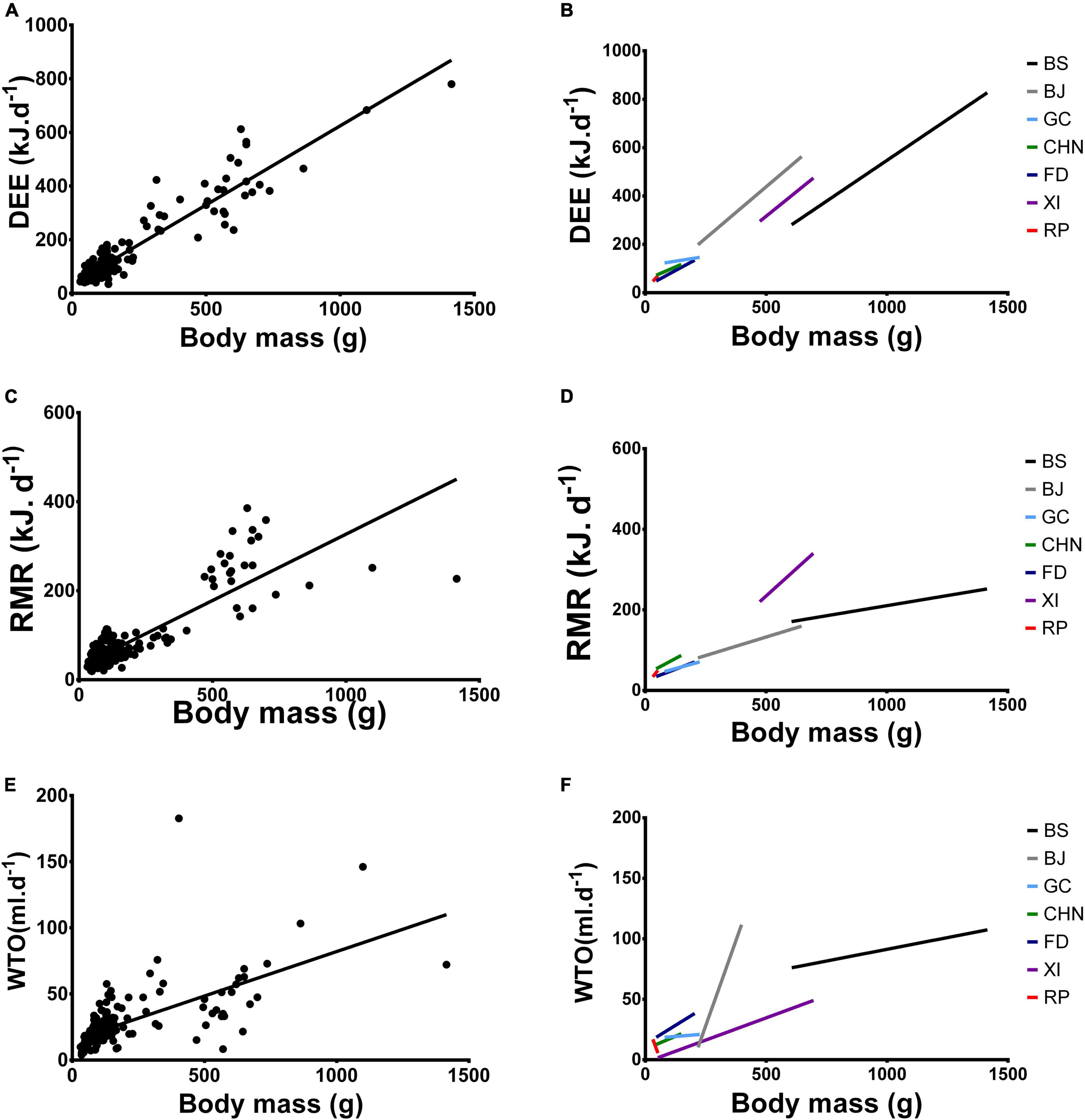
Figure 4. Daily energy expenditure (DEE- kJ.d– 1) against body mass (g) of (A) all seven species of rodent combined and (B) all seven species of rodent separated. Resting metabolic rate (RMR- kJ.d– 1) against body mass (C) all seven species of rodent combined and (E) all seven species of rodent separated. Water turnover (WTO- ml.d– 1) against body mass (D) all seven species of rodent combined and (F) all seven species of rodent separated. BS - Bathyergus suillus; BJ: Bathyergus janetta; GC - Georychus capensis; CHN - Cryptomys hottentotus natalensis; FD - Fukomys damarensis; XI - Xerus inauris; RP - Rhabdomys pumilio.
All species, but G. capensis, had DEE values that were significantly affected by body mass (Supplementary materialTable 3 and Figure 4B).
Daily energy expenditure differed significantly among species (Supplementary material, Table 1). Bathyergus janetta had significantly higher DEE than all other mole-rat species and R. pumilio and X. inauris (Figure 3B and Supplementary Table 4). While B. suillus had significantly lower DEE than all mole-rat species, F. damarensis had a similar DEE to B. suillus (Figure 3B and Supplementary Table 4). Furthermore, B. suillus possessed a similar DEE to R. pumilio, but a lower DEE than X. inauris (Figure 3B and Supplementary Table 4). Georychus capensis possessed the second-highest DEE for the mole-rats species and a similar DEE to X. inauris but a lower DEE than R. pumilio (Figure 3B and Supplementary Table 4). Cryptomys h. natalensis and F. damarensis possessed similar DEE to one another (Figure 3B and Supplementary Table 4). Additionally, C. h. natalensis and F. damarensis possessed similar DEE to X. inauris and R. pumilio (Figure 3B and Supplementary Table 4). Lastly, X. inauris a higher DEE than R. pumilio (Figure 3B and Supplementary Table 4).
Resting Metabolic Rate
Both species and body mass significantly affected RMR (Supplementary Table 1). When all species were combined, there was a strong effect of body mass on RMR; the linear fitted regression
significantly explained the variance (F = 431.9, p < 0.0001, Figure 4C).
Body mass significantly affected all species’ RMR (Supplementary Table 3 and Figure 4D).
The RMR differed significantly among species (Supplementary Table 1). For example, Xerus inauris possessed significantly higher RMR values than all mole-rat species and R. pumilio (Figure 3C and Supplementary Table 4). Contrastingly, R. pumilio possessed a similar RMR to all mole-rat species (Figure 3C and Supplementary Table 4). Similarly, B. suillus possessed similar RMR to all other mole-rats species (Figure 3C and Supplementary Table 4). Likewise, B. janetta possessed a similar RMR to all other mole-rats species, but F. damarensis possessed a lower RMR to B. janetta (Figure 3C and Supplementary Table 4). Fukomys damarensis also possessed a lower RMR than C. h. natalensis but a similar RMR to G. capensis (Figure 3C and Supplementary Table 4). Georychus capensis possessed a lower RMR than C. h. natalensis (Figure 3C and Supplementary Table 4).
Sustained Metabolic Scope
Sustained metabolic scope differed significantly among species (Supplementary Table 1). All solitary mole-rat species, namely B. suillus, B. janetta and G. capensis, possessed similar SusMS values (Figure 3D and Supplementary Table 2). All solitary mole-rat species had SusMS values that were significantly higher than the social mole-rat species, namely C. h. natalensis and F. damarensis, and the two aboveground rodent species (Figure 3D and Supplementary Table 2). However, the social mole-rat species were found to be significantly different from one another, with F. damarensis possessing a higher SusMS than C. h. natalensis (Figure 3D and Supplementary Table 2). Likewise, F. damarensis possessed significantly higher SusMS than X. inauris, but similar SusMS to R. pumilio (Figure 3D and Supplementary Table 2). In contrast, C. h. natalensis shared a similar SusMS to both X. inauris and R. pumilio (Figure 3D and Supplementary Table 2).
Water Turn Over
Both species and body mass significantly affected WTO (Supplementary Table 1). When all species were combined, there was a strong effect of body mass on WTO; the linear fitted regression
significantly explained the variance (F = 124.3, p < 0.0001, Figure 4E).
All solitary mole-rat species, namely B. suillus, B. janetta and G. capensis, had WTO values that were not significantly related to body mass (Supplementary Table and Figure 4F). Similarly, R. pumilio WTO was unaffected by body mass (Supplementary Table 5 and Figure 4F). The social mole-rat species, namely C. h. natalensis and F. damarensis, and X. inauris WTO were significantly affected by body mass (Supplementary Table 5 and Figure 4F).
Water turnover differed significantly among species (Supplementary Table 1). Bathyergus janetta possessed a significantly higher WTO in comparison to all other African mole-rat species and the two aboveground rodent species (Figure 3E and Supplementary Table 6). Fukomys damarensis possessed the second-highest WTO, with F. damarensis possessing significantly higher WTO in comparison to the two aboveground rodent species and all other African mole-rats species but B. suillus (Figure 3E and Supplementary Table 6). Additionally, G. capensis, C. h. natalensis and B. suillus possessed similar WTO species (Figure 3E and Supplementary Table 6). At the same time, R. pumilio possessed significantly lower WTO values in comparison to all species except B. suillus species (Figure 3E and Supplementary Table 6). Lastly, X. inauris possessed similar WTO values to C. h. natalensis and G. capensis species (Figure 3E and Supplementary Table 6).
Water Economy Index
Water economy index differed significantly among species (Supplementary materialTable 1). Fukomys damarensis possessed the highest WEI of all species (Figure 3F and Supplementary Table 6). Alternatively, X. inauris possessed the lowest WEI of all species, besides R. pumilio, which possessed a similar WEI (Figure 3F and Supplementary Table 6). Bathyergus janetta possessed higher WEI than G. capensis and R. pumilio, but similar WEI to B. suillus and C. h. natalensis (Figure 3F and Supplementary Table 6). Georychus capensis, C. h. natalensis and B. suillus possessed similar WEI (Figure 3F and Supplementary Table 6). Both G. capensis and B. suillus possessed similar WEI to R. pumilio; however, C. h. natalensis possessed a higher WEI than R. pumilio (Figure 3F and Supplementary Table 4).
Body Fat Percent
The terrestrial rodent species, R. pumilio, possessed similar BF to all other species (Supplementary Tables 1, 7 and Figure 4E). Bathyergus janetta possessed a significantly lower BF when compared to the other African mole-rat species and X. inauris (Supplementary Table 7 and Figure 4E). The solitary B. suillus possessed similar BF to the other African mole-rat species and X. inauris (Supplementary Table 7 and Figure 4E). In contrast, G. capensis possessed higher BF than the social African mole-rat species and X. inauris (Supplementary Table 7 and Figure 4E). Cryptomys h. natalensis and F. damarensis possessed similar BF to one another and X. inauris (Supplementary Table 7 and Figure 4E).
Environmental Conditions
Environmental conditions are presented in Table 3. The sites exhibited significant differences in environmental conditions (χ2≥ 32.2, p ≤ 0.0001). The locations of Darling and Glengarry share similar aridity indices (‘AIs’) (Table 3 and Supplementary Table 8). Individuals from both Darling and Glengarry possessed significantly higher AIs than mole-rats from all other sites (Table 3 and Supplementary Table 8). Individuals from Goegap possessed a similar AI to Hotazel and Kamieskroon, but a lower AI to S.A. Lombard Nature Reserve (Table 3 and Supplementary Table 8). Hotazel and Kamieskroon possessed similar AIs, but both these sites had a lower AI than S. A. Lombard Nature Reserve (Table 3 and Supplementary Tables 7, 8). Air temperature (Tair) was similar between Darling, Goegap and Kamieskroon but different at all other sites (Table 3 and Supplementary Table 9). Soil temperatures (Ts1, Ts2 and Ts3) showed a similar pattern with S. A. Lombard Nature Reserve, Goegap and Kamieskroon being similar, while all other sites were significantly different from one another (Table 3 and Supplementary Tables 9, 10). Darling and Goegap possessed similar total precipitations (tp), while, Goegap and Kamieskroon possessed similar tps (Table 3 and Supplementary Table 11). All other sites possessed tps which were significantly different from one another (Table 3 and Supplementary Table 11). Soil moistures (Ms1, Ms2 and Ms3) were significantly different among all sites, respectively, apart from S. A. Lombard Nature Reserve and Kamieskroon, which were found to have similar Ms1, and Kamieskroon and Goegap had similar Ms3 (Table 3 and Supplementary Tables 11, 12).
Discussion
Daily Energy Expenditure
Speakman (1997a) suggested that field metabolic rate (FMR), the DEE of animals in the wild or “field”, is affected by environmental conditions. For example ambient temperature affects DEE with the trend of animals inhabiting a cooler climate (a characteristic of a mesic environment) possessing a higher FMR to animals that persist in hotter climates (a characteristic of an arid environment). A similar pattern found in other desert-dwelling vertebrates (see Nagy et al. (1999) for review). However, a contrasting pattern was observed within the African mole-rat family, particularly in the solitary genus Bathyergus. Bathyergus janetta, which inhabits an arid and hot region of South Africa, possessed a greater DEE than its mesic-dwelling cousin, B. suillus. Indeed, B. janetta possessed the highest DEE compared to other arid dwelling mole-rat species, including F. damarensis, and arid dwelling aboveground species, namely X. inauris and R. pumilio. African mole-rats need to forage for geophytes through the energetically expensive method of digging (Vleck, 1979), which becomes energetically more expensive the drier the soils become (Lovegrove, 1989). It would, therefore, be expected that the arid-dwelling species, such as B. janetta and F. damarensis, would possess the highest DEE. Our results suggest that B. janetta follows this expected trend, but the social F. damarensis does not, as F. damarensis appears to possess similar DEE to the mesic dwelling solitary B. suillus and the social C. h. natalensis. As proposed by the aridity food distribution hypothesis (Jarvis et al., 1994), the evolution of social living may negate or ameliorate the need for increased physiological adaptations to an arid environment (Scantlebury et al., 2006a,2008). In species that form large groups, such as F. damarensis, animals will not have to expend as much energy daily, even in arid environments, as the increased group size allows for the efficient locating and retrieval of food. Group-living allows for such substantial reduction of DEE in F. damarensis that their DEE is similar to aboveground rodent species, both X. inauris and R. pumilio, who do not need to dig to forage and thus should possess a lower DEE. The benefit of group living is absent in the arid dwelling (solitary) B. janetta, in an increased DEE to allow them to locate and retrieve the supply of sufficient resources. By comparison, even though B. suillus lives solitarily, it exists in much softer soils and with access to a more abundant food resources, resulting in less energy being spent daily foraging (Bennett and Faulkes, 2000).
Both Speakman (1997a) and Nagy et al. (1999) revealed a strong correlations between body mass and DEE. As expected, in this study, body mass played a significant role in predicting an animal’s DEE, with larger animals expending more energy per day when performing their natural behaviors compared to smaller animals. Interestingly, G. capensis was the only species not to show a significant relationship with body mass. Unlike G. capensis, both B. janetta (Scantlebury et al., 2006b) and B. suillus (this study) exhibit sexual dimorphism in body mass that leads to sexual dimorphism in DEE, lending added support to the body-maintenance hypothesis for sexual dimorphism (see Key and Ross (1999)). The lack of sexual dimorphism in G. capensis may be the leading cause for the absence of association between body mass and DEE, as even though equal numbers of males and females were captured, they were all adults (Bennett et al., 1991) and thus, all individuals were of similar mass resulting in no effect of body mass on DEE (Scantlebury et al., 2006b). We would expect that if smaller G. capensis were captured and compared with larger individuals, an effect of body mass would be seen.
Resting Metabolic Rate
It has long been thought that with increasing aridity (decreasing A.I.), there is an adaptive advantage for a lower RMR (Haim, 1987; Haim Skinner and Robinson, 1987; Tieleman et al., 2003; Williams et al., 2004; Van Sant et al., 2012; Klüg-Baerwald and Brigham, 2017), as the adaptive value of reduced metabolic rates in more arid dwelling animals is thought to be related to increased water and energy savings under low and unpredictable resource availability (Lovegrove, 2000; Tieleman et al., 2003). Along this line of thinking, the arid dwelling mole-rat species (F. damarensis, B. janetta) are predicted to possess lower RMR than the mesic dwelling species (G. capensis; B. suillus, C. h. natalensis). Alternatively, African mole-rat are known to possess low RMRs in response to inhabiting an environment where they are exposed to hypercapnic and hypoxic conditions, whereby their low RMRs allows an equilibrium to be established between the partial pressures of oxygen and carbon dioxide in their blood and tissues, and in their external environment (Ar et al., 1977; Lovegrove, 1986; Ivy et al., 2020; Wallace et al., 2021). If this hypothesis were true, we would expect the aboveground foraging species, namely X. inauris and R. pumilio, and African mole-rat species with poor hypoxia tolerance, indicating that they are not routinely exposed to hypoxia in their burrow system, namely B. suillus (see Logan et al. (2020) for review), to possess the highest RMR. Nevertheless, both these predictions were not supported in this study. In this study, most mole-rat species possessed similar RMRs to one another. While the less hypoxic-tolerant African mole-rat species, B. suillus, possessed a similar RMR to both other solitary (G. capensis) and social (F. damarensis) mole-rat species. Therefore, other explanations, such as physiological and behavioral thermoregulatory strategies (Mota-Rojas et al., 2021), may be driving the pattern of RMR seen within these rodent species (see Speakman (1999) for review).
As with DEE, body mass seems to be the primary factor determining RMR in the current set of rodents, rather than the behavior or the micro- or macro-habitat of the animal. This conclusion is clearly evident by the strong relationship between RMR and body mass overall and within species.
Sustained Metabolic Scope
It has long been hypothesized that vertebrates that inhabit arid regions would work less (SusMS) than those inhabitating mesic regions to reduce energy expenditure and increase water and energy savings under low and unpredictable resource availability (Tieleman et al., 2003). However, as with DEE, the African mole-rat family does not follow this trend. Even though beneficial in some circumstances, an exclusively subterranean lifestyle imposes extremely high energetic demands on the animals inhabiting these ecotopes (Vleck, 1979). These energetic digging demands become greater as soils become drier and thus harder (Lovegrove, 1989). These demands are one of the fundamental suggested pressures that have resulted in some African mole-rat species evolving group-living that leads to them sharing the workload amongst group members; this sharing is especially important in more arid environments as posited by the aridity food distribution hypothesis (Jarvis et al., 1994; Lacey and Sherman, 1997).
The current analysis suggests that the aridity food distribution hypothesis proposes selective pressures for group living in African mole-rats (Jarvis et al., 1994; Lacey and Sherman, 1997). Regardless of environmental conditions and digging method, all solitary mole-rat species possessed equally high SusMS compared to individuals of a colony-forming subterranean species (C. h. natalensis; F. damarensis) and fossorial and terrestrial group-living species (X. inauris; R. pumilio). This finding suggests that if an animal is solely responsible for underground foraging and burrow construction, whether the animal is digging in an arid (B. janetta) or mesic (G. capensis; B. suillus) environment, or with teeth (G. capensis), or a combination of teeth and forelimbs (B. suillus; B. janetta), they will be working harder than animals that live in groups in similar environments and/or use similar digging methods. For example, F. damarensis and B. janetta inhabit similar climatic conditions (see Table 3), but F. damarensis lives in large groups and thus possesses a lower SusMS.
Group living, however, does not mitigate all pressures placed upon subterranean animals by their environment. Group living mole-rat species that inhabit arid areas, namely F. damarensis, with drier soils still possess a higher SusMS than those group-living species living in mesic conditions, namely C. h. natalensis. Lovegrove (1989) observed that animals digging in moist soils use less energy than those digging in drier soils. As expected, the outgroup species, X. inauris and R. pumilio, that foraged aboveground (Table 3) possessed some of the lowest SusMS as predicted by Vleck (1979). Interestingly, C. h. natalensis, under the added benefits of group living and inhabiting a mesic environment, possessed equally low SusMS to the above ground-foragers, indicating that group living in a mesic environment could overcome the energetic demands of a subterranean lifestyle. The aridity food distribution hypothesis is often contested as several species of the genus Fukomys and Cryptomys species are found in mesic conditions (Bennett and Faulkes, 2000). These mesic conditions are predicted to be a driving force in selecting a solitary lifestyle, yet, some species still persist as group-forming mole-rat species. Using the patterns investigated in this current study, we hypothesize that the ancestors of mesic-dwelling mole-rat species may have been arid-dwelling social species that moved into a mesic environment and since there is an energetic benefit to remaining as group-forming species, as indicated by the similar SusMS to above ground-foragers, they retained a social lifestyle.
Water Turnover and Water Economy Index
Surprisingly, all studies using DLW techniques on African mole-rats have not investigated WTO or WEI. As a family, the African mole-rats occupy a range of environmental conditions, from hyper humid to hyper-arid, and as eutherian mammals, one would expect a trend in WEI ratios as seen in Nagy (2004). Nagy (2004) observed that eutherian mammals that occupy arid regions require less water per day (WTO) to achieve a water balance compared to their mesic dwelling relatives. Once Nagy (2004) adjusted for their metabolic rates (i.e., the WEI ratio), which is often lower in arid dwelling mammals (Nagy et al., 1999), arid-dwelling eutherians had significantly lower WEI compared to non-arid dwelling species. One might interpret this finding as arid-dwelling mammals using less water to perform their daily behaviors, which is expected as arid environments are water restricted. Reduced metabolism and physiological (lower evaporation, increased water retaining abilities of kidneys) or behavioral (less time spent actively above ground, being relatively more nocturnal) adaptations may be responsible for the reduction in WEI ratios of Nagy’s (2004) above ground eutherian mammals.
African mole-rats do not share this pattern as the more arid dwelling species possessed higher WEI than the more mesic dwelling species. A more surprising fact is that African mole-rats do not drink freestanding water but obtain all water requirements from underground geophytes, which become more dispersed in arid regions (Bennett et al., 1994; Bennett and Jarvis, 1995; Spinks et al., 1999; Malherbe et al., 2003). African mole-rats need to forage for geophytes through the energetically expensive method of digging (Vleck, 1979), which becomes energetically more expensive the drier the soils become (Lovegrove, 1989). It would, therefore, be expected that arid-dwelling species, such as F. damarensis, would require a lower WEI to survive their water restricted environment as water is less available and therefore they would need to conserve water the best they could. Yet, in this study, we see that F. damarensis possesses the highest WEI ratio of all species tested. The decreases in water availability and aridity seem to drive the WEI ratio higher in subterranean mole-rat species.
Several reasons for this surprising reversal in pattern could be at play. Physiological differences between arid-dwelling small mammals and mesic-dwelling small mammals that allow arid-dwelling small mammals to survive and thrive in an arid environment, such as lower RMR, body temperature and rates of evaporative water loss (Haim, 1987; Haim Skinner and Robinson, 1987; Tieleman et al., 2003; Williams et al., 2004; Van Sant et al., 2012; Klüg-Baerwald and Brigham, 2017), have been lost in African mole-rats species due to the shared constraints of their microclimate (burrow system) (Wallace et al., 2021). This hypothesis is supported by similar high water turnover rates in the arid dwelling subterranean species of Spalax ehrenbergi and Thomomys bottae (Gettinger, 1984; Yahav et al., 1989).
Furthermore, as proposed by the aridity food distribution hypothesis, the evolution of social living may negate the need for physiological adaptations to an arid environment (Jarvis et al., 1994). In species that form large groups, such as F. damarensis, animals can increase WEI as water is not a limited resource as the increased group size allows for the efficient locating and retrieval of food and water (behavioral osmoregulation). To date, no comprehensive multi-species study has been conducted on African mole-rats osmoregulatory abilities; however, anecdotal evidence has pointed to no differences in urine concentrating abilities of kidneys in different mole-rats species from different environments, again pointing toward behavioral osmoregulation to allow for the survival of social mole-rats in arid environments (M. van Dyk and D.W. Hart unpublish data).
However, this evidence begs the question of how B. janetta, possessing the second-highest WEI value, persists in their arid environment, while possessing a similar WEI to their mesic-dwelling cousin B. suillus, is not group living. On a recent research field trip (2021), a noticeable reduction in the B. janetta population in the areas of succulent Karoo (Kamieskroon, Garies and Groenrivier) was observed by one of the authors who have been working on B. janetta for the last 30 years (N.C. Bennett per comm). It is believed that due to the effects of climate change, which has seen the areas of Kamieskroon and Garies regions in the Northern Cape area receive below-average rainfall and increased temperatures resulting in desertification (increased aridity) (see Supplementary Figure 1), in combination with poor water retention abilities of solitary African mole-rats species, this has driven this population decline. Even though lacking behavioral osmoregulatory benefits of social living, the solitary African mole-rats that inhabit mesic areas (G. capensis; B. suillus) still have fewer water demands placed on them as food is more abundant and equally dispersed in the softer and wetter soils of their environment. At the same time, C. h. natalensis possesses similar WEI to the solitary mole-rat species due to living in a hyper-mesic environment (Colantoni et al., 2015).
Percent Body Fat, Body Fat Composition
Body fat composition (BF) of an animal can be linked to its resource availability and amount of work (DEE and SuSMS) it does. Thus, animals which inhabitat arid regions are often expected to possess lower body conditions, including lower BF. Once again, the African mole-rat family does not fit that trend. Only one study (before the current study) on African mole-rats using the DLW technique has reported BF. Scantlebury et al. (2006c), using BF, discovered distinct physiological castes in a social mammal, F. damarensis, which solidified the concept that F. damarensis could be classed as a eusocial organism with similar traits to bees and termites. Scantlebury et al. (2006c) showed that infrequent workers in an F. damarensis colony also possess higher BF than frequent workers (particularly in the dry season), which suggested that infrequent workers constitute a physiologically distinct dispersing caste who contribute less to cooperative behaviors in the colony and focus more on building up their body reserves in preparation for dispersal and reproduction when environmental conditions are suitable.
In the current study, all social mole-rat species (C. h. natalensis; F. damarensis) and aboveground foragers (R. pumilio; X. inauris) possessed similar BF to one another. It should be noted that within this current study, all physiological castes, namely breeding individuals, and infrequent and frequent workers, were combined. The Cape mole-rat, G. capensis possessed the highest BF of all African mole-rat investigated a surprising find considering G. capensis is a solitary species and needs to forage (indicted by their high SusMS and DEE) and thermoregulation alone which should result in a lower BF. Surprisingly, this finding also includes the solitary B. suillus. Georychus capensis and B. suillus occur sympatrically, but B. suillus lives in much softer, easier to dig sandy soils while G. capensis inhabit a harder clay soil in vlei areas (Bennett and Faulkes, 2000). This should lead to the hypothesis that B. suillus should possess a higher BF than G. capensis, mainly because this species shows a significantly lower DEE than G. capensis as digging in softer sands is less energetically demanding (Lovegrove, 1989; this study). However, a possible lack of sexual dimorphism in G. capensis (Scantlebury et al., 2006b) and an apparent sexual dimorphism in B. suillus (this study) may cause the differences in BF between species. Bathyergus suillus males show indications of increased DEE and SusMS, suggesting that males are far more active than females resulting in less stored fat in males. This sex bias is possibly due to the larger male B. suillus body size, which increases energy expenditure while constructing larger tunnels and nest complexes. Furthermore, males have been documented to search for female tunnel systems and dig into them, searching for a mate (Hart et al., 2006; Thomas et al., 2009); this searching would require more activity and energy expenditure in the males, while the females are less active and store fat in preparation for offspring care.
A finding of great concern is that the solitary arid-dwelling B. janetta possessed the lowest BF of all species tested in this study, indicating low resource reserves and possibly poor body condition. The WEI already indicated that the possible decline of the B. janetta population observed in 2021 is possibly due to desertification due to climate change and overgrazing by domestic animals on land in their distributional range (Supplementary Figure 1). The BF adds to this claim, as in 2006, when these data were collected, to 2021 there has been a drastic increase in aridity (a decrease in AI) (see Supplementary Figure 1). If B. janetta already showed reduced body fat reserves in 2006, possibly due to being a large, solitary subterranean mole-rat in an arid environment with dispersed food sources. Then as the area becomes more arid and food becomes scarcer due to geophytes drying out as well as the ground becoming harder, B. janetta may no longer obtain enough food to maintain a healthy body condition, which could drive local extinction of this species.
Conclusion
This current study attempted to provide an overview of the energetic and water flux (including previously unreported energetic parameters) in the African mole-rats (see Table 1). Furthermore, we attempted to test well established energetic and water flux trends for aboveground small mammals and rodents against trends observed within the African mole-rat family. We conclude that the unique underground lifestyle and spectrum of social behaviors possessed by the family Bathyergidae are most likely more crucial to their energetics and water flux than their habitat; however other important unstudied factors may still be at play as well. Researchers often under report all the energetic parameters that can be measured using (DLW) technique and indirect calorimetry, namely WTO, WEI, and BF. These measures are vitally important in understanding how well an animal is suited for their current and future predicted environmental conditions they experience. Therefore, we urge researchers who use the DLW technique and open-circuit respirometry to report all parameters; as with this knowledge available in the literature, even greater strides in our understanding of animal energetics and species protection can be made. These parameters can be used as important indicators of which species may be particularly vulnerable to local extinction, like B. janetta, due to the changing climate.
Data Availability Statement
The datasets presented in this article are not readily available because rights to this raw data are held by the institute and further permission is needed to release such data. Requests to access the datasets should be directed to DH, u10022725@tuks.co.za.
Ethics Statement
The animal study was reviewed and approved by the Animal Ethics Committee, University of Pretoria.
Author Contributions
DH, NB, and DS conceived and designed the manuscript. DH analyzed the data and wrote the initial draft. DS, MO, JW, and CH performed experiments and sample analysis. All authors contributed to the final and revised manuscript.
Funding
We thank all the funding agencies that made this research possible, namely, the SARChI Chair of Mammal Behavioral Ecology and Physiology (GUN 64756), National Research Foundation RSA (Grants 2053801 and 2053514), the British Ecological Society (SEPG), and the National Science Foundation, United States no. 0130600.
Conflict of Interest
The authors declare that the research was conducted in the absence of any commercial or financial relationships that could be construed as a potential conflict of interest.
Publisher’s Note
All claims expressed in this article are solely those of the authors and do not necessarily represent those of their affiliated organizations, or those of the publisher, the editors and the reviewers. Any product that may be evaluated in this article, or claim that may be made by its manufacturer, is not guaranteed or endorsed by the publisher.
Acknowledgments
We would like to thank the Duckitt Family (Darling) and the Stone Family (Kamieskroon) for allowing us to conduct research on their farms Waylands and Kardou, respectively. We want to thank the Northern Cape Department of Agriculture, Land Reform and Environment, and Goegap Nature Reserve staff for their assistance. Furthermore, we would like to thank Glengarry golf estate for access to their property for animal capture and KwaZulu-Natal Nature Conservation Service. We would like to thank all the field assistants that contributed to this large data set.
Supplementary Material
The Supplementary Material for this article can be found online at: https://www.frontiersin.org/articles/10.3389/fevo.2022.867350/full#supplementary-material
References
Ar, A., Arieli, R., and Shkolnik, A. (1977). Blood-gas properties and function in the fossorial mole rat under normal and hypoxic-hypercapnic atmospheric conditions. Respir. Physiol. 30, 201–218. doi: 10.1016/0034-5687(77)90031-7
Bennett, N. C., Aguilar, G. H., Jarvis, J. U. M., and Faulkes, C. G. (1994). Thermoregulation in three species of Afrotropical subterranean mole-rats (Rodentia: Bathyergidae) from Zambia and Angola and scaling within the genus Cryptomys. Oecologia 97, 222–227. doi: 10.1007/BF00323153
Bennett, N. C., Clarke, B. C., and Jarvis, J. U. M. (1992). A comparison of metabolic acclimation in two species of social mole-rats (Rodentia, Bathyergidae) in southern Africa. J. Arid Environ. 23, 189–198. doi: 10.1016/s0140-1963(18)30530-5
Bennett, N. C., and Faulkes, C. G. (2000). African Mole-Rats: Ecology and Eusociality. Cambridge: Cambridge University Press.
Bennett, N. C., and Jarvis, J. U. M. (1995). Coefficients of digestibility and nutritional values of geophytes and tubers eaten by southern African mole-rats (Family, Bathyergidae). J. Zool. 236, 189–198. doi: 10.1111/j.1469-7998.1995.tb04487.x
Bennett, N. C., Jarvis, J. U. M., Aguilar, G. H., and McDaid, E. J. (1991). Growth and development in six species of African mole-rats (Rodentia: Bathyergidae). J. Zool. 225, 13–26. doi: 10.1111/j.1469-7998.1991.tb03798.x
Bennett, N. C., Jarvis, J. U. M., and Davies, K. C. (1988). Daily and seasonal temperatures in the burrows of African rodent moles. Afr. Zool. 23, 189–195. doi: 10.1080/02541858.1988.11448101
Bennett, N. C., Taylor, P. J., and Aguilar, G. H. (1993). Thermoregulation and metabolic acclimation in the Natal mole-rat (Cryptomys hottentotus natalensis). Z. Saugetierkd. 58, 362–367.
Boyles, J. G., Verburgt, L., Mckechnie, A. E., and Bennett, N. C. (2012). Heterothermy in two mole-rat species subjected to interacting thermoregulatory challenges. J. Exp. Zool. Part A Ecol. Genet. Physiol. 317 A, 73–82. doi: 10.1002/jez.723
Buffenstein, R., and Yahav, S. (1991). Is the naked mole-rat Hererocephalus glaber an endothermic yet poikilothermic mammal? J. Therm. Biol. 16, 227–232. doi: 10.1016/0306-4565(91)90030-6
Burland, T. M., Bennett, N. C., Jarvis, J. U. M., and Faulkes, C. G. (2002). Eusociality in African mole-rats: new insights from patterns of genetic relatedness in the Damaraland mole-rat (Cryptomys damarensis). Proc. Biol. Sci. 269, 1025–1030. doi: 10.1098/rspb.2002.1978
Cheng, H., Sebaa, R., Malholtra, N., Lacoste, B., El Hankouri, Z., Kirby, A., et al. (2021). Naked mole-rat brown fat thermogenesis is diminished during hypoxia through a rapid decrease in UCP1. Nat. Commun. 12:6801. doi: 10.1038/s41467-021-27170-2
Colantoni, A., Delfanti, L., Cossio, F., Baciotti, B., Salvati, L., Perini, L., et al. (2015). Soil aridity under climate change and implications for agriculture in Italy. Appl. Math. Sci. 9, 2467–2475. doi: 10.12988/ams.2015.52112
Darden, T. R. (1972). Respiratory adaptations of a fossorial mammal, the pocket gopher (Thomomys bottae). J. Comp. Physiol. 78, 121–137. doi: 10.1007/BF00693609
Depocas, F., and Hart, J. S. (1957). Use of the Pauling oxygen analyser for measurement of oxygen consumption of animals in open-circuit systems and in a short-lag, closed-circuit apparatus. J. Appl. Physiol. 10, 388–392. doi: 10.1152/jappl.1957.10.3.388
Drent, R. H., and Daan, S. (1980). The prudent parent: energetic adjustments in avian breeding 1. Ardea 55, 225–252. doi: 10.5253/arde.v68.p225
Faulkes, C. G., Bennett, N. C., Bruford, M. W., O’Brien, H. P., Aguilar, G. H., and Jarvis, J. U. M. (1997). Ecological constraints drive social evolution in the African mole-rats. Proc. Biol. Sci. 264, 1619–1627. doi: 10.1098/rspb.1997.0226
Faulkes, C. G., Verheyen, E., Verheyen, W., Jarvis, J. U. M., and Bennett, N. C. (2004). Phylogeographical patterns of genetic divergence and speciation in African mole-rats (Family: Bathyergidae). Mol. Ecol. 13, 613–629. doi: 10.1046/j.1365-294X.2004.02099.x
Finn, K., Voigt, C., van Jaarsveld, B., Hart, D. W., and Jorna, J. (2020). PSEUDASPIS CANA (Mole Snake). Herpetol. Rev. 51, 626–627.
Finn, K. T., Janse van Vuuren, A. K., Hart, D. W., Suess, T., Zottl, M., and Bennett, N. C. (2022). Seasonal changes in locomotor activity patterns of wild social Natal mole-rats (Cryptomys hottentotus natalensis). Front. Ecol. Evol. 10:819393. doi: 10.3389/fevo.2022.819393
Francioli, Y., Thorley, J., Finn, K., Clutton-Brock, T., and Zöttl, M. (2020). Breeders are less active foragers than non-breeders in wild Damaraland mole-rats. Biol. Lett. 16:20200475. doi: 10.1098/rsbl.2020.0475
Gettinger, R. D. (1984). Energy and water metabolism of free-ranging pocket gophers, Thomomys bottae. Ecology 65, 740–751. doi: 10.2307/1938046
Haim, A. (1987). Thermoregulation and metabolism of Wagner’s gerbil (Gerbillus dasyurus): a rock dwelling rodent adapted to arid and mesic environments. J. Therm. Biol. 12, 45–48. doi: 10.1016/0306-4565(87)90022-2
Haim, A., and Fourie, F. (1980). Heat production in nocturnal (Praomys natalensis) and diurnal (Rhabdomys pumilio) South African murids. Afr. Zool. 15, 91–94. doi: 10.1080/02541858.1980.11447692
Haim Skinner, J. D., and Robinson, T. J. A. (1987). Bioenergetics, thermoregulation and urine analysis of squirrels of the genus Xerus from an arid environment. Afr. Zool. 22, 45–49. doi: 10.1080/02541858.1987.11448019
Hammond, K. A., and Diamond, J. (1997). Maximal sustained energy budgets in humans and animals. Nature 386, 457–462. doi: 10.1038/386457a0
Hart, D. W., van Jaarsveld, B., Lasch, K. G., Grenfell, K. L., Oosthuizen, M. K., and Bennett, N. C. (2021). Ambient temperature as a strong Zeitgeber of circadian rhythms in response to temperature sensitivity and poor heat dissipation abilities in subterranean African mole-rats. J. Biol. Rhythms 36, 461–469. doi: 10.1177/07487304211034287
Hart, L., Bennett, N. C., Malpaux, B., Chimimba, C. T., and Oosthuizen, M. K. (2004). The chronobiology of the Natal mole-rat, Cryptomys hottentotus natalensis. Physiol. Behav. 82, 563–569. doi: 10.1016/j.physbeh.2004.05.008
Hart, L., O’Riain, M. J., Jarvis, J. U. M., and Bennett, N. C. (2006). Is the cape dune mole-rat, Bathyergus suillus (rodentia: Bathyergidae), a seasonal or aseasonal breeder? J. Mammal. 87, 1078–1085. doi: 10.1644/05-mamm-a-411r2.1
Herzig-Straschil, B. (1978). On the biology of Xerus inauris (Zimmermann, 1780)(Rodentia, Sciuridae). Z. Säugetierkd. 43, 262–278.
Herzig-Straschil, B. (1979). Xerus inauris (Rodentia, Sciuridae)-an inhabitant of the arid regions of Southern Africa. Folia Zool. 28, 119–124.
Hickman, G. C. (1979). A Live-trap and trapping technique for fossorial mammals. S. Afr. J. Zool. 9–12. doi: 10.1080/02541858.1979.11447641
Hill, R. W. (1972). Determination of oxygen consumption by use of the paramagnetic oxygen analyser. J. Appl. Physiol. 33, 261–263. doi: 10.1152/jappl.1972.33.2.261
Holtze, S., Braude, S., Lemma, A., Koch, R., Morhart, M., Szafranski, K., et al. (2018). The microenvironment of naked mole-rat burrows in East Africa. Afr. J. Ecol. 56, 279–289. doi: 10.1111/aje.12448
Houslay, T. M., Vullioud, P., Zöttl, M., and Clutton-Brock, T. H. (2020). Benefits of cooperation in captive Damaraland mole-rats. Behav. Ecol. 31, 711–718. doi: 10.1093/beheco/araa015
Ivy, C. M., Sprenger, R. J., Bennett, N. C., van Jaarsveld, B., Hart, D. W., Kirby, A. M., et al. (2020). The hypoxia tolerance of 8 related African mole-rat species rivals that of naked mole-rats, despite divergent ventilatory and metabolic strategies in severe hypoxia. Acta Physiol. 228:e13436. doi: 10.1111/apha.13436
Jarvis, J. U. M., and Bennett, N. C. (1993). Eusociality has evolved independently in two genera of bathyergid mole-rats—but occurs in no other subterranean mammal. Behav. Ecol. Sociobiol. 33, 253–260. doi: 10.1007/bf02027122
Jarvis, J. U. M., O’Riain, M. J., Bennett, N. C., and Sherman, P. W. (1994). Mammalian eusociality - a family affair. Trends Ecol. Evol. 9, 47–51. doi: 10.1016/0169-5347(94)90267-4
Kennerly, T. E. (1964). Microenvironmental conditions of pocket gopher burrow. Tex. J. Sci. 16, 395–441.
Key, C., and Ross, C. (1999). Sex differences in energy expenditure in non–human primates. Proc. R. Soc. Lond. Ser. B Biol. Sci. 266, 2479–2485. doi: 10.1098/rspb.1999.0949
Klüg-Baerwald, B. J., and Brigham, R. M. (2017). Hung out to dry? Intraspecific variation in water loss in a hibernating bat. Oecologia 183, 977–985. doi: 10.1007/s00442-017-3837-0
Lacey, E. A., and Sherman, P. W. (1997). “Cooperative breeding in naked mole-rats: implications for vertebrate and invertebrate sociality,” in Cooperative Breeding in Mammals, eds N. G. Solomon and J. A. French (Cambridge: Cambridge University Press), 267–301.
Lacey, E. A., and Sherman, P. W. (2009). “Cooperative breeding in naked mole-rats: implications for vertebrate and invertebrate sociality,” in Cooperative Breeding in Mammals, eds N. G. Solomon and J. A. French (Cambridge: Cambridge University Press), 267–301. doi: 10.1017/cbo9780511574634.011
Lifson, N., Gordon, G. B., and McClintock, R. (1955). Measurement of total carbon dioxide production by means of D2O18. J. Appl. Physiol. 7, 704–710. doi: 10.1152/jappl.1955.7.6.704
Lifson, N., and McClintock, R. (1966). Theory of use of the turnover rates of body water for measuring energy and material balance. J. Theor. Biol. 12, 46–74. doi: 10.1016/0022-5193(66)90185-8
Logan, S. M., Szereszewski, K. E., Bennet, N. C., Hart, D. W., van Jaarsveld, B., Pamenter, M. E., et al. (2020). The brains of six African mole rat species show divergent responses to hypoxia. J. Exp. Biol. 223:jeb215905. doi: 10.1242/jeb.215905
Lovegrove, B. G. (1986). The metabolism of social subterranean rodents: adaptation to aridity. Oecologia 69, 551–555. doi: 10.1007/BF00410361
Lovegrove, B. G. (1989). The cost of burrowing by the social mole rats (Bathyergidae) Cryptomys damarensis and Heterocephalus glaber: the role of soil moisture. Physiol. Zool. 62, 449–469. doi: 10.1086/physzool.62.2.30156179
Lovegrove, B. G. (2000). The zoogeography of mammalian basal metabolic rate. Am. Nat. 156, 201–219. doi: 10.1086/303383
Lutermann, H., Bennett, N. C., Speakman, J. R., and Scantlebury, M. (2013). Energetic benefits of sociality offset the costs of parasitism in a cooperative mammal. PLoS One 8:e57969. doi: 10.1371/journal.pone.0057969
Malherbe, G. P., Bennett, N. C., and Schoeman, A. S. (2003). Foraging in the subterranean social highveld mole-rat (Cryptomys hottentotus pretoriae): an investigation into mass-dependent geophyte use and foraging patterns. J. Zool. 260, 219–225. doi: 10.1017/S0952836903003698
McGowan, N. E., Scantlebury, D. M., Bennett, N. C., Maule, A. G., and Marks, N. J. (2020). Thermoregulatory differences in African mole-rat species from disparate habitats: responses and limitations. J. Therm. Biol. 88:102495. doi: 10.1016/j.jtherbio.2019.102495
McNab, B. K. (1966). The metabolism of fossorial rodents: a study of convergence. Ecology 47, 712–733. doi: 10.2307/1934259
Mota-Rojas, D., Titto, C. G., Orihuela, A., Martínez-Burnes, J., Gómez-Prado, J., Torres-Bernal, F., et al. (2021). Physiological and behavioral mechanisms of thermoregulation in mammals. Animals 11:1733. doi: 10.3390/ani11061733
Muñoz-Sabater, J., Dutra, E., Agustí-Panareda, A., Albergel, C., Arduini, G., Balsamo, G., et al. (2021). ERA5-Land: a state-of-the-art global reanalysis dataset for land applications. Earth Syst. Sci. Data 13, 4349–4383. doi: 10.5194/essd-13-4349-2021
Nagy, K. A. (1983). The Doubly Labeled Water (3HH18O) Method: A Guide to its Use. Publ 12-1417. Los Angeles, CA: UCLA.
Nagy, K. A. (2004). “Water economy of free-living desert animals,” in International Congress Series, eds S. Morris and A. Vosloo (Amsterdam: Elsevier), 1275, 291–297. doi: 10.1016/j.ics.2004.08.054
Nagy, K. A., Girard, I. A., and Brown, T. K. (1999). Energetics of free-ranging mammals, reptiles, and birds. Annu. Rev. Nutr. 19, 247–277. doi: 10.1146/annurev.nutr.19.1.247
Okrouhlík, J., Šumbera, R., Gardner, B., Schoemann, K., Lövy, M., and Bennett, N. C. (2021). Are southern African solitary mole-rats homeothermic or heterothermic under natural field conditions? J. Therm. Biol. 95:102810. doi: 10.1016/j.jtherbio.2020.102810
Oosthuizen, M. K., and Bennett, N. C. (2015). The effect of ambient temperature on locomotor activity patterns in reproductive and non-reproductive female Damaraland mole-rats. J. Zool. 297, 1–8. doi: 10.1111/jzo.12254
Oosthuizen, M. K., Cooper, H. M., and Bennett, N. C. (2003). Circadian rhythms of locomotor activity in solitary and social species of African mole-rats (family: Bathyergidae). J. Biol. Rhythms 18, 481–490. doi: 10.1177/0748730403259109
Oosthuizen, M. K., Robb, G., Harrison, A., Froneman, A., Joubert, K., and Bennett, N. C. (2021). Flexibility in body temperature rhythms of free-living natal mole-rats (Cryptomys hottentotus natalensis). J. Therm. Biol. 99:102973. doi: 10.1016/j.jtherbio.2021.102973
Peterson, C. C., Nagy, K. A., and Diamond, J. (1990). Sustained metabolic scope. Proc. Natl. Acad. Sci. U.S.A. 87, 2324–2328. doi: 10.1073/pnas.87.6.2324
Romanenko, V. A. (1961). Computation of the autumn soil moisture using a universal relationship for a large area. Proc. Ukr. Hydrometeorol. Res. Inst. 3, 12–25.
Roper, T. J., Bennett, N. C., Conradt, L., and Molteno, A. J. (2001). Environmental conditions in burrows of two species of African mole-rat, Georhychus capensis and Cryptomys damarensis. J. Zool. 254, 101–107. doi: 10.1017/S0952836901000590
Rutherford, M. C., and Westfall, R. H. (1994). Biomes of Southern Africa: An Objective Categorisation. Claremont, CA: National Botanical Institute.
Scantlebury, M., Afik, D., Shanas, U., and Haim, A. (2002). Comparative non-shivering thermogenesis in adjacent populations of the common spiny mouse (Acomys cahirinus) from opposite slopes: the effects of increasing salinity. J. Comp. Physiol. B 172, 1–5. doi: 10.1007/s003600100220
Scantlebury, M., Bennett, N. C., Speakman, J. R., Pillay, N., and Schradin, C. (2006a). Huddling in groups leads to daily energy savings in free-living African Four-Striped Grass Mice, Rhabdomys pumilio. Funct. Ecol. 20, 166–173. doi: 10.1111/j.1365-2435.2006.01074.x
Scantlebury, M., Speakman, J. R., Oosthuizen, M. K., Roper, T. J., and Bennett, N. C. (2006c). Energetics reveals physiologically distinct castes in a eusocial mammal. Nature 440, 795–797. doi: 10.1038/nature04578
Scantlebury, M., Speakman, J. R., and Bennett, N. C. (2006b). The energy costs of sexual dimorphism in mole-rats are morphological not behavioural. Proc. Biol. Sci. 273, 57–63. doi: 10.1098/rspb.2005.3280
Scantlebury, M., and Haim, A. (2012). Environmental challenges and physiological solutions: comparative energetic daily rhythms of field mice populations from different ecosystems. PLoS One 7:51247. doi: 10.1371/journal.pone.0051247
Scantlebury, M., Oosthuizen, M. K., Speakman, J. R., Jackson, C. R., and Bennett, N. C. (2005). Seasonal energetics of the Hottentot golden mole at 1500 m altitude. Physiol. Behav. 84, 739–745. doi: 10.1016/j.physbeh.2005.02.022
Scantlebury, M., Waterman, J. M., and Bennett, N. C. (2008). Alternative reproductive tactics in male Cape ground squirrels Xerus inauris. Physiol. Behav. 94, 359–367. doi: 10.1016/j.physbeh.2008.02.003
Scantlebury, M., Waterman, J. M., Hillegass, M., Speakman, J. R., and Bennett, N. C. (2007). Energetic costs of parasitism in the Cape ground squirrel Xerus inauris. Proc. Biol. Sci. 274, 2169–2177. doi: 10.1098/rspb.2007.0690
Schradin, C., and Pillay, N. (2004). The striped mouse (Rhabdomys pumilio) from the succulent karoo, South Africa: a territorial group-living solitary forager with communal breeding and helpers at the nest. J. Comp. Psychol. 118, 37–47. doi: 10.1037/0735-7036.118.1.37
Shkolnik, A., and Schmidt-Nielsen, K. (1976). Temperature Regulation in Hedgehogs from Temperate and Desert Environments. Physiol. Zool. 49, 56–64. doi: 10.1086/physzool.49.1.30155677
Speakman, J. (1997a). Factors influencing the daily energy expenditure of small mammals. Proc. Nutr. Soc. 56, 1119–1136. doi: 10.1079/pns19970115
Speakman, J. R. (1999). The cost of living: field metabolic rates of small mammals. Adv. Ecol. Res. 30, 177–297. doi: 10.1016/s0065-2504(08)60019-7
Speakman, J. R., and Król, E. (2005). Comparison of different approaches for the calculation of energy expenditure using doubly labeled water in a small mammal. Physiol. Biochem. Zool. 78, 650–667. doi: 10.1086/430234
Speakman, J. R., Król, E., and Johnson, M. S. (2004). The functional significance of individual variation in basal metabolic rate. Physiol. Biochem. Zool. 77, 900–915. doi: 10.1086/427059
Speakman, J. R., and Racey, P. A. (1988). Consequences of non steady-state CO 2production for accuracy of the doubly labelled water technique: The importance of recapture interval. Comp. Biochem. Physiol. A Physiol. 90, 337–340. doi: 10.1016/0300-9629(88)91125-5
Spinks, A. C., Branch, T. A., Croeser, S., Bennett, N. C., and Jarvis, J. U. M. (1999). Foraging in wild and captive colonies of the common mole-rat Cryptomys hottentotus hottentotus (Rodentia: Bathyergidae). J. Zool. 249, 143–152. doi: 10.1017/S0952836999010031
Šumbera, R. (2019). Thermal biology of a strictly subterranean mammalian family, the African mole-rats (Bathyergidae, Rodentia) - a review. J. Therm. Biol. 79, 166–189. doi: 10.1016/j.jtherbio.2018.11.003
Šumbera, R., Chitaukali, W. N., Elichová, M., Kubová, J., and Burda, H. (2004). Microclimatic stability in burrows of an Afrotropical solitary bathyergid rodent, the silvery mole-rat (Heliophobius argenteocinereus). J. Zool. 263, 409–416. doi: 10.1017/S095283690400545X
Thomas, H. G., Bateman, P. W., Le Comber, S. C., Bennett, N. C., Elwood, R. W., and Scantlebury, M. (2009). Burrow architecture and digging activity in the Cape dune mole rat. J. Zool. 279, 277–284. doi: 10.1111/j.1469-7998.2009.00616.x
Thomas, H. G., Swanepoel, D., and Bennett, N. C. (2016). Burrow architecture of the Damaraland mole-rat (Fukomys damarensis) from South Africa. Afr. Zool. 51, 29–36. doi: 10.1080/15627020.2015.1128355
Tieleman, B. I., Williams, J. B., and Bloomer, P. (2003). Adaptation of metabolism and evaporative water loss along an aridity gradient. Proc. Biol. Sci. 270, 207–214. doi: 10.1098/rspb.2002.2205
Van Sant, M. J., Oufiero, C. E., Muñoz-Garcia, A., Hammond, K. A., and Williams, J. B. (2012). A Phylogenetic approach to total evaporative water loss in mammals. Physiol. Biochem. Zool. 85, 526–532. doi: 10.1086/667579
Vleck, D. (1979). The Energy Cost of Burrowing by the Pocket Gopher Thomomys bottae. Physiol. Zool. 52, 122–136. doi: 10.1086/physzool.52.2.30152558
Wallace, K. M. E., van Jaarsveld, B., Bennett, N. C., and Hart, D. W. (2021). The joint effect of micro- and macro-climate on the thermoregulation of two African mole-rat (Bathyergidae) sub-species, Cryptomys hottentotus mahali and C. hottentotus pretoriae. J. Therm. Biol. 99:103025. doi: 10.1016/j.jtherbio.2021.103025
Waterman, J. M. (1995). The social organisation of the Cape ground squirrel (Xerus inauris; Rodentia: Sciuridae). Ethology 101, 130–147. doi: 10.1111/j.1439-0310.1995.tb00352.x
Williams, J. B., Muñoz-Garcia, A., Ostrowski, S., and Tieleman, B. I. (2004). A phylogenetic analysis of basal metabolism, total evaporative water loss, and life-history among foxes from desert and mesic regions. J. Comp. Physiol. B 174, 29–39. doi: 10.1007/s00360-003-0386-0
Yahav, S., Simson, S., and Nevo, E. (1989). Total body water and adaptive water turnover rate in four chromosomal species of subterranean mole rats of the Spalax ehrenbergi superspecies in Israel. J. Zool. 218, 461–469. doi: 10.1111/j.1469-7998.1989.tb02557.x
Zelová, J., Šumbera, R., Okrouhlík, J., Šklíba, J., Lövy, M., and Burda, H. (2011). A seasonal difference of daily energy expenditure in a free-living subterranean rodent, the silvery mole-rat (Heliophobius argenteocinereus; Bathyergidae). Comp. Biochem. Physiol. A Mol. Integr. Physiol. 158, 17–21. doi: 10.1016/j.cbpa.2010.07.026
Keywords: daily energy expenditure (DEE), resting metabolic rate (RMR), sustained metabolic scope (SusMS), water turnover (WTO), water economy index (WEI), body fat (BF), African mole-rats, aridity
Citation: Hart DW, Bennett NC, Oosthuizen MK, Waterman JM, Hambly C and Scantlebury DM (2022) Energetics and Water Flux in the Subterranean Rodent Family Bathyergidae. Front. Ecol. Evol. 10:867350. doi: 10.3389/fevo.2022.867350
Received: 01 February 2022; Accepted: 19 April 2022;
Published: 02 June 2022.
Edited by:
Todd Jason McWhorter, University of Adelaide, AustraliaReviewed by:
Hynek Burda, University of Duisburg-Essen, GermanyMichał S. Wojciechowski, Nicolaus Copernicus University in Toruń, Poland
Copyright © 2022 Hart, Bennett, Oosthuizen, Waterman, Hambly and Scantlebury. This is an open-access article distributed under the terms of the Creative Commons Attribution License (CC BY). The use, distribution or reproduction in other forums is permitted, provided the original author(s) and the copyright owner(s) are credited and that the original publication in this journal is cited, in accordance with accepted academic practice. No use, distribution or reproduction is permitted which does not comply with these terms.
*Correspondence: Daniel W. Hart, u10022725@tuks.co.za; David M. Scantlebury, m.scantlebury@qub.ac.uk