- 1Department of Biological Sciences, University of Cincinnati, Cincinnati, OH, United States
- 2Department of Plant and Microbial Biology, BioTechnology Institute, University of Minnesota, St. Paul, MN, United States
- 3Department of Geography and GIS, University of Cincinnati, Cincinnati, OH, United States
- 4Archaeological Consulting Services, Ltd., Tempe, AZ, United States
- 5Department of Anthropology, University of Calgary, Calgary, AB, Canada
- 6Laboratorio de Geomática, Centro de Estudios de Desarrolló Sustentable y Aprovechamiento de la Vida Silvestre (CEDESU), Universidad Autónoma de Campeche, Campeche, Mexico
- 7Florida Museum of Natural History (FLMNH), University of Florida, Gainesville, FL, United States
- 8Department of Molecular Genetics, Biochemistry and Microbiology, University of Cincinnati, Cincinnati, OH, United States
Yaxnohcah was a major city of the ancient Maya world, especially during the Preclassic period (1000 BCE–200 CE). Data from excavations provide important insights into the interactions between the ancient inhabitants and its surrounding Neotropical forests, a topic that, as a whole, remains largely enigmatic. This study aspired to fill that void in understanding by using traditional paleoethnobotanical approaches and a powerful new technology, the analysis of environmental DNA. Our results enabled us to characterize the vegetation growing in association with the principal structures and the artificial reservoirs that provided the city’s water supply. Because the area is without access to permanent water sources, such as rivers or lakes, these reservoirs were key to the development and survival of the city. Our results indicate that although there were large areas cleared for agricultural purposes, a mosaic of mature upland and bajo forest remained throughout the Maya occupation. In addition, our studies reveal that there were incursions of pine savanna into the area that may have been a reflection of prevailing edaphic conditions, or alternatively, the result of frequent burning.
Introduction
A primary focus of this investigation addresses the unresolved question of how the ancient occupants of the Maya Lowlands were able to sustain substantial urban populations for centuries in a tropical environment with pronounced wet and dry seasons. The greatest challenge for the Yaxnohcah occupants was access to water during the dry season. This area is in a karstic plateau with a geological under-bedding of porous carbonate rock. When tropical forest covers the landscape a dense mat of tree roots, lianas, and epiphytes will soak up the rainwater and recycle it, but when the land is cleared for agriculture, whatever rainwater hits the ground will be drained off to the water table which is hundreds of meters below the surface and is essentially inaccessible. Because there are no permanent water sources, e.g., lakes or rivers, in the area, the Maya survived by creating plaster or clay-lined reservoirs to retain water from the rainy season for use during the dry season.
In this study, we are interested in the development of water and land management systems at the large urban center of Yaxnohcah in what is now southern Campeche, Mexico. We hypothesize that their landuse adaptations were sustainable over a long period of time and if not, the Maya would adjust their practices to better meet their resource needs. Yaxnohcah represents an ideal study area for testing these hypotheses because of the changing trajectories of human–environment interactions in the “Maya heartland.” The community grew into a large urban center early in the Preclassic period (1000 BCE–200 CE), endured through the Preclassic collapse that witnessed the disbanding of some of its large neighboring communities, and became a Classic period center of some prominence before its ultimate demise in the Postclassic period (Table 1). With this long arc of shifting needs and population growth as a backdrop, we will examine the agroforestry activities, agricultural practices, and water retention strategies as enacted by the ancient Maya of Yaxnohcah.
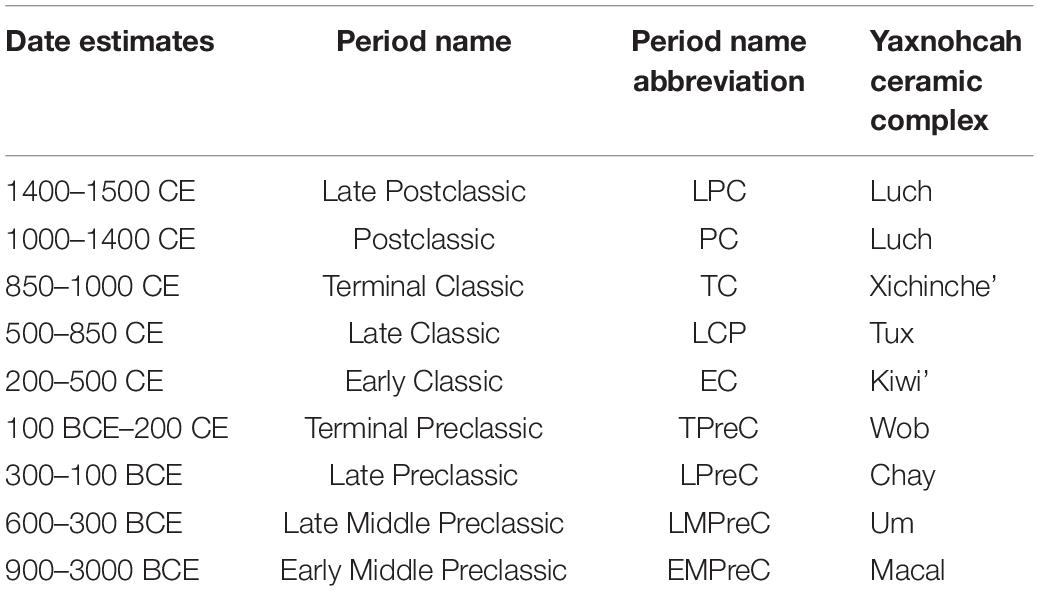
Table 1. Yaxnohcah chronology based on ceramic complexes (after Reese-Taylor, 2017, Table 15.1).
Site History
The site of Yaxnohcah is located near the center of the Elevated Interior Region (EIR) of the Yucatan Peninsula, a large physiographic zone (Figure 1) that extends from northern Guatemala to what is now the southwestern part of the state of Yucatan (Dunning et al., 2012). The EIR can be subdivided into three culturally distinct sub-regions: the Puuc Hills, the Central Karstic Uplands (CKU), and the Rio Bec zone (Reese-Taylor et al., 2016). The division between the Rio Bec zone and the CKU is based on geographic distinctions as well as specific cultural associations that link sites within each sub-region (Figure 1). Well known sites in the Rio Bec sub-region include Río Bec and Becan, plus recently identified sites such as Chactun and Tamchen (Šprajc, 2015, 2020; Šprajc et al., 2021). The CKU are dominated by the sites of Calakmul, Tikal, and El Mirador. In addition to the geographic association, the sites in the CKU also share a material culture extending back to the pre-Mamon period (prior to 550 BCE) (Reese-Taylor, 2017).
The Yucatan Peninsula is a former Cretaceous seabed that gave rise to the porous carbonate geological substrate of the region with limestone ridges and uplands that are well-drained. These uplands, although covered with tropical forest prior to Maya settlement, provided most of the agricultural land for the ancient Maya following forest removal which began at Yaxnohcah during the Early Middle Preclassic period (900–600 BCE) (Reese-Taylor, 2017). Seasonal wetlands, locally referred to as bajos, form in the low-lying karst depressions that typically are filled with a thick clay soil that forms a semi-permeable barrier to the internal drainage of water and promotes their inundation in the rainy season.
The ancient Maya polity of Yaxnohcah resides upon a broad upland ridge between the extensive Bajo Laberinto (Figure 2) and the smaller Bajo Tomatal. In its own right, Bajo Tomatal is a large upland bajo of approximately 4 km2 and has a higher elevation than Laberinto. Consequently, runoff from Tomatal regularly discharges into Laberinto during the rainy season and thereafter. Bajo Tomatal is surrounded by steep escarpments on all sides and the remains of ancient Maya settlements, many of which feature monumental architecture (Dunning et al., 2019). One of the striking features of the higher elevations of the CKU is the almost complete lack of permanent water bodies. This characteristic, combined with a precipitation pattern that is highly seasonal with months of heavy rains followed by months with almost no rain (Nations, 2006), made the year-round access to water problematic for expanding human populations. The annual rainfall in the area ranges from 1500 to 2000 mm per year (Dunning et al., 2019) with most precipitation (90%) occurring in the rainy season which generally lasts from May through November (Islebe et al., 2019). In addition to the annual hydrometeorological variability, this region has been plagued by periodic episodes of intense drought (e.g., Hodell et al., 1995, 2005; Haug et al., 2003; Wahl et al., 2007, 2014; Dunning et al., 2012, 2014; Kennett et al., 2012; Medina-Elizade and Rohling, 2012; Griffin et al., 2014; Douglas et al., 2015; Luzzadder-Beach et al., 2016; Bhattacharya et al., 2017). These episodic droughts coupled with the physiographic features and the bimodal rainfall pattern were highly influential in the formation of the natural vegetation of the area and the cultural history, as well.
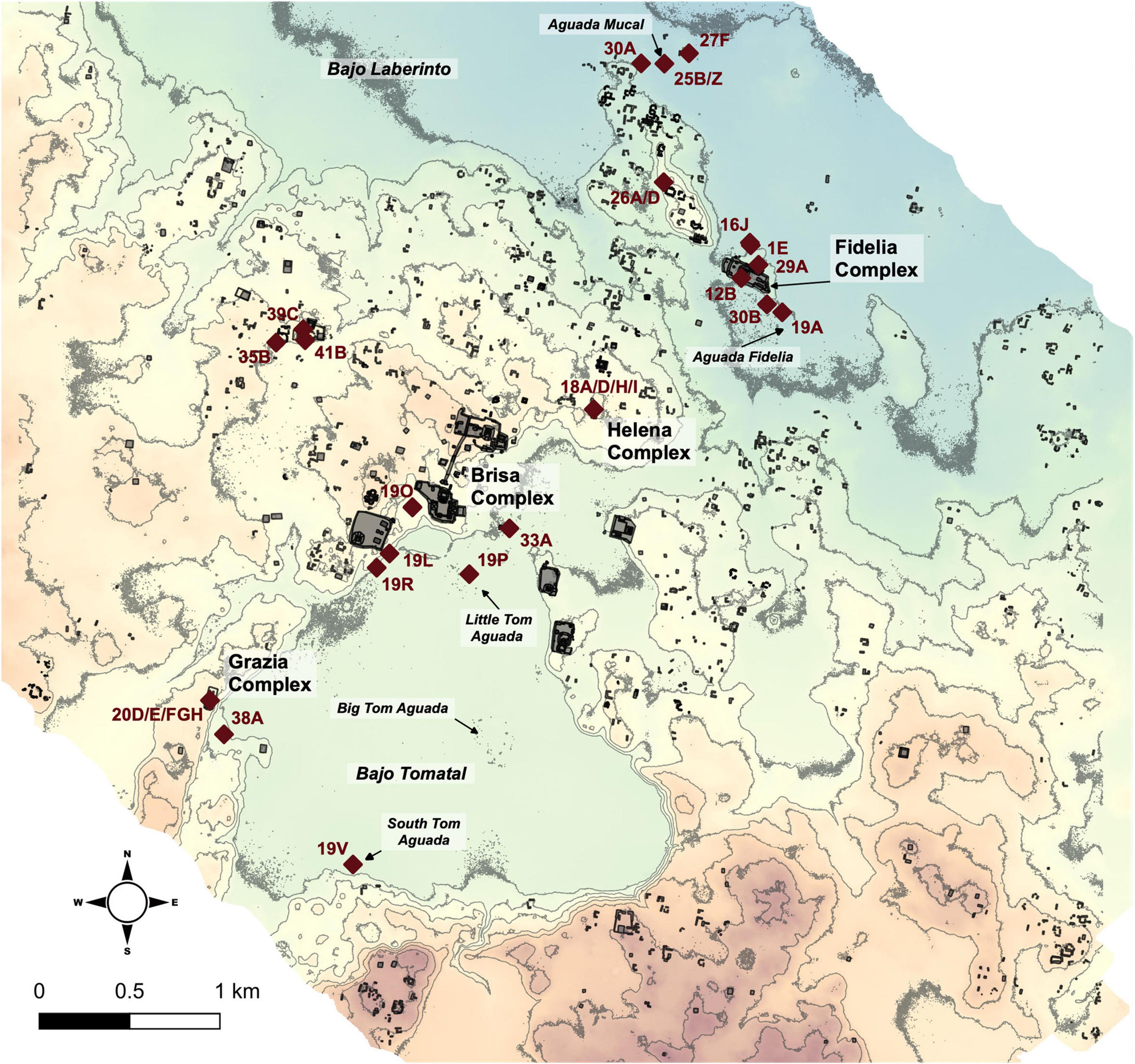
Figure 2. Map of Yaxnohcah showing the location of the structures, operations, and features mentioned in the text.
To survive the dry season in the EIR of the Maya Lowlands, the ancient Maya created reservoirs large and small (Dunning et al., 2012, 2022; Scarborough et al., 2012). Large reservoirs at Yaxnohcah ranged in capacity from about 115,000 to 1900 m3. The Maya also created hundreds of smaller tanks at Yaxnohcah, generally in close proximity to residences (Brewer, 2017, 2018; Brewer and Carr, 2021). These features were designed to retain water during the rainy seasons and store it for use by the local occupants during the dry seasons. This system of water retention during the rainy seasons worked exceptionally well for the Maya for many centuries.
The city was founded at roughly 1000 BCE, during the early Middle Preclassic period. The first civic-ceremonial complex constructed during this time period was the Brisa E-group. The Brisa reservoir was constructed shortly thereafter at 800–600 BCE (Reese-Taylor, 2017). Additional large civic complexes were built over the landscape during the ensuing Middle and Late Preclassic periods accompanied by a surge in population during the Late/Terminal Preclassic (400 BCE–200 CE). Much of the massive civic construction ceased during the Early Classic period (200–550 CE), with the significant exception of a palace attached to the Brisa E-group, which was expanded during this time (Reese-Taylor, 2017). Although cut-stone architectural features were largely absent and the scope of settlement was reduced, there is evidence for modest construction activities during the Early Classic occupation.
Yaxnohcah apparently became an agricultural, agroforestry, and trade outpost for Calakmul, located 21 km to the NE, during the Late Classic period (550–850 CE). Yaxnohcah’s population seems to have peaked at this time with new and extremely large courtyard groups appearing on the landscape. This expansion is clearly seen in the construction in the Fidelia Barrio located in the northeast sector of the city along the southern rim of the Laberinto Bajo. Like most other cities in the CKU, Yaxnohcah experienced a decline in population as well as civic construction at the end of the Terminal Classic period. However, evidence from various locations throughout the site, especially water storage features, suggest that a remnant Postclassic population continued to live in the region—or returned periodically to the area—to exploit the rich environment until 1550 CE.
Floristic Complexes
The modern vegetation of the area surrounding Yaxnohcah has been described as semi-deciduous tropical forest (Rzedowski, 1981; Greller, 2000; Nations, 2006) owing to the fact that some of the trees lose their leaves during the dry season. Previous studies (Miranda, 1958; Boege, 1993; Martínez et al., 2001; Geovannini Acuña, 2008) have used the terms: low semi-evergreen forest, medium semi-evergreen forest, and high evergreen or semi-evergreen forest to describe these habitats, depending mostly on the height of the canopy of each vegetation type (Reese-Taylor et al., 2016). Similarly, in our studies we divided the local area into four vegetation types: aquatic vegetation, bajo forest, transition forest, and upland forest with a stronger emphasis on the substrate rather than just the vegetation structure of each plant cover type.
Aquatic vegetation typically resides within the interior and edges of aguadas (small ponds) in the bajos. These are open areas that tend to retain water for most of the year and are dominated by sedges locally known as navajuela (Scleria bracteata Cav.) (Arellano Rodriguez et al., 2003) with a host of other herbaceous plants and small shrubs. Most aguadas within the EIR have been found to be the remains of ancient Maya reservoirs (Dunning et al., 2022).
Bajo forest has a relatively low forest canopy with dense underbrush. Soils in these seasonal wetlands are dark-colored, clayey Vertisols. Characteristic trees in this vegetation type include: chilonche (Eugenia winzerlingii Standl.), tinta (Haematoxylum campechianum L.), chechem (Metopium brownei [Jacq.] Urb.), tkansik (Hyperbaena winzerlingii Standl.), nance agrio (Byrsonima bucidifolia Standl.), tzitzilche (Gymnopodium floribundum Rolfe), naranjillo (Bonellia macrocarpa [Cav.] B.Ståhl & Källersjö); palo de gusano (Piscidia piscipula [L.] Sarg.), jobo (Spondias mombin L.), and yaya (Mosannona depressa [Baill.] Chatrou).
Transition forest has a mix of plant species found in both bajo forest and upland forest but has a lower canopy (less than 12 m) than upland forest and is not seasonally inundated as is the bajo forest. This forest type is similar to the category described by Lundell (1937) as an “Intermediate Formation.” Common tree species in this forest include: zapotillo (Pouteria reticulata [Engl.] Eyma), succoutz (Drypetes lateriflora [Sw.] Krug & Urb.), chico zapote (M. zapota [L.] P. Royen), cabbage bark (Lonchocarpus xuul Lundell), laurelillo (Nectandra coriacea (Sw.) Griseb.), pixoy (Guazuma ulmifolia Lam.), yaxnik (Vitex gaumeri Greenm.), matapalo (Ficus spp.), palo de gusano (P. piscipula), jobo (S. mombin), and the guano palm (Sabal sp.).
Upland forest has the highest canopy (up to 35 m) of all the vegetation types and is rooted in the most well-drained soils in the Yaxnohcah area. Ramón (Brosimum alicastrum Sw.), zapotillo (P. reticulata [Engl.] Eyma), wild lime (Trichilia minutiflora Standl.), succoutz (D. lateriflora), and chico zapote (M. zapota) are among the visually dominant forest trees. Other forest species include: cedro (Cedrela odorata L.), sac chaka (Dendropanax arboreus [L.] Decne. & Planch.), chacte viga (Leptolobium panamense [Benth.] Sch. Rodr.), higuera (Ficus spp.), guaya (Melicoccus oliviformis Kunth), sapotillo (Pouteria campechiana [Kunth] Baehni), katsin (Senegalia gaumeri [S.F.Blake] Britton & Rose), xate (Chamaedorea oblongata Mart.), and cordoncillo (Piper spp.). In addition to this overall forest classification, Reese-Taylor et al. (2016) have identified several microenvironments that reflect local indigenous ecological perspectives. For example, areas dominated by cordoncillo plants are referred to as “cordoncial.” Likewise, an area with abundant chico zapote is called a “zapotal” and one with numerous ramón trees is called a “ramonal.” These indigenous classifications reflect the deep history of human–environmental interaction across this landscape.
A unique feature of this study area is that the forest surrounding Yaxnohcah has largely been undisturbed by modern forest extraction activities since the Maya departed (Nations, 2006), aside from some selective harvesting of a few commercial timber species, e.g., cedro (C. odorata) and mahogany (Swietenia macrophylla King) in the 1950s (Bray and Klepeis, 2005). Accordingly, the current landscape is, in at least some ways, a reflection of the ancient Maya forest. To take advantage of this unique feature, our study included intensive, quantitative forest surveys (72 plots of 500 m2 each) guided by satellite imagery and lidar data to assess the biodiversity, species richness, and biomass of the modern forests surrounding Yaxnohcah. In conjunction with the modern forest surveys, we conducted pollen analyses on sediments from the reservoirs and buried soil surfaces of the site. These efforts were coupled with a systematic macrobotanical analysis, conducted as part of all excavations, to recover archeological plant remains. Finally, we employed improved environmental DNA extraction and sequencing procedures that provided whole new vistas of understanding of the paleoecological setting in which the Maya interacted. All of these techniques were combined to provide fresh insights into the land use activities at ancient Yaxnohcah, beginning in the Early Middle Preclassic period and extending into the Postclassic period (Table 1).
Materials and Methods
Paleoethnobotanical analysis of ancient plant remains from Yaxnohcah allows for reconstruction of the environmental conditions in which the ancient Maya lived as well as their dietary habits and other plant use practices. Thorough descriptions of the materials and methods employed in this study, both in the field and in the laboratory, have been presented in previous publications (Scarborough et al., 2012; Lentz et al., 2014, 2015, 2020, 2021; Dunning et al., 2019). Soil samples for pollen and macrobotanical analyses were collected from each discrete context (lot).
In brief, 38 soil samples slated for pollen analysis were extracted from stratigraphic columns (Pearsall, 2009) in excavation profiles from Operations 19A, 19R, and 30B and sediment cores (Operations 19C, 19V, and 25Z) and processed at the Texas A&M University Pollen Laboratory employing standard chemical extraction procedures (Pearsall, 2009). For each extraction, approximately 5 g of sediment sample was immersed in a sequence of strong reagents, e.g., hydrofluoric, hydrochloric, nitric, and phosphoric acids as well as sodium hydroxide, followed by centrifuging and rinsing with distilled water. After these steps came an acetolysis procedure which involved washes of glacial acetic acid, sulfuric acid, and acetic anhydride. All of these steps essentially dissolved away the sediment components, leaving behind mostly pollen grains with their highly durable sporopollenin exine layers. Prior to extraction, spores of Lycopodium sp. (clubmoss) were added to each sample to evaluate pollen concentration. Using a compound light microscope, 200 pollen grains were counted for each sample with a sufficient amount of pollen. These standard methodological procedures have been employed in previous studies (e.g., Scarborough et al., 2012; Lentz et al., 2014, 2021; Dunning et al., 2019) with consistent success.
Ancient, carbonized macrobotanical specimens, including carbonized seeds and charcoal were collected during excavations by project archeologists. Flotation of sediment samples was performed in an effort to systematically retrieve carbonized plant materials from measured sediment samples gathered throughout the site. Archeologists collected 2-L sediment samples from all discrete contexts (lots). A modified Ankara flotation system (Pearsall, 2009) was adapted for use at Yaxnohcah, utilizing three 55-gallon drums to re-cycle and conserve water. Each sediment sample was floated (light fraction) and filtered until no more carbonized or organic materials were visible. Any ceramics or lithics encountered were removed and set aside for further analysis. The remaining heavy fraction was collected and separated from non-plant debris in a field lab setting to retrieve any remaining carbonized plant materials.
All floated materials were analyzed at the University of Cincinnati Paleoethnobotany Laboratory. There were 141 flotation samples processed in the laboratory that had been collected over the years beginning in 2011 from a total of 12 operations and a variety of contexts. As with other macrobotanical remains, flotation materials were analyzed initially using a Wild Heerbrugg light stereomicroscope at 40× magnification. Tiny seeds and charcoal fragments were further examined using a Philips XL30 environmental scanning electron microscope housed in the Advanced Materials Characterization Center at the University of Cincinnati College of Engineering.
Field samples designated for eDNA analysis were collected from profile columns (Pearsall, 2009) using sterile, sealable containers half-filled with RNAlater (nucleic acid stabilization solution, made by Invitrogen). The sediment and soil samples from Yaxnohcah were hand carried to lab facilities in the United States. After arrival at the University of Cincinnati, samples designated for eDNA molecular genetic analysis were stored in a −80°C freezer prior to processing. Before DNA extraction, sediment samples were allowed to thaw to 4°C before insertion into tubes, under sterile conditions, with glass beads then sealed prior to homogenization in a bead-beating machine. To avoid the possibility of contamination, samples were processed in an isolated laboratory in the Department of Biological Sciences that previously had been dedicated to the extraction of DNA from bacteria, so there was no chance of contamination from vascular plant DNA, the target of our study. All standard protocols to prevent and detect the introduction of contaminants were employed (Cooper and Poinar, 2000; Fulton and Shapiro, 2019; Lentz et al., 2020, 2021). Following strict workflow procedures, the clean bench and equipment (pipettes, tips, etc.) were exposed to UV light between extractions and were cleaned with 70% ethanol and bleach after each use. The DNA obtained in our study was extracted from sediment samples using DNeasy Power Soil Kits made by Qiagen (Hamilton et al., 2013; Epp et al., 2019). To facilitate the process of sequencing the fragmented genetic material from our DNA extractions, we relied upon the services of RAPiD Genomics LLC (Gainesville, FL, United States) to design genetic probes compatible with their capture-Seq protocol to retrieve plant gene sequences for selected markers across a wide variety of plant taxa (Table 2 and Supplementary Table 1). To do this, we targeted chloroplast and nuclear gene sequences by employing a set of genetic markers, e.g., rbcL, trnL, and ITS regions, similar to those used successfully in previous ancient vegetation studies (Sønstebø et al., 2010; Jørgensen et al., 2012; Parducci et al., 2019) to construct the probes. All samples underwent equimolar pooling and were sequenced by means of an Illumina HiSeq 2 × 150 sequencing system with subsequent bioinformatics processing of all sequences obtained. Identification of retrieved sequences was achieved by comparison to existing sequences stored in the National Center for Biotechnology Information (NCBI) database, GenBank, using their BLAST searching tool. Overall, 16 extracted samples generated identifiable plant sequences (n = 697). The size range of the sequences went from 151 to 2715 bp with an average sequence length of 415 bp (Supplementary Tables 2–19). Genetic data generated by this project have been submitted to NCBI and are available under BioProject Numbers PRJNA782546 and PRJNA804249.
Results
Results were obtained through analysis of pollen, eDNA, and macrobotanical remains. Although the area of Yaxnohcah is quite extensive, in this paper we will focus on four of the site localities: the Fidelia complex, the Aguada Mucal, the Bajo Tomatal, and the Brisa complex (Figure 2).
Fidelia
The Fidelia complex is located approximately 1.8 km to the northeast of the Brisa and Alba complexes which, when combined, formed the major civic-ceremonial center of Yaxnohcah. A main feature of the Fidelia complex is a large rectangular platform that is 8 m high and measures 110 by 250 m. The platform supported eight smaller structures. To the southeast of the complex is a large reservoir (Figure 3) that likely served as the water storage tank for the Fidelia area occupants.
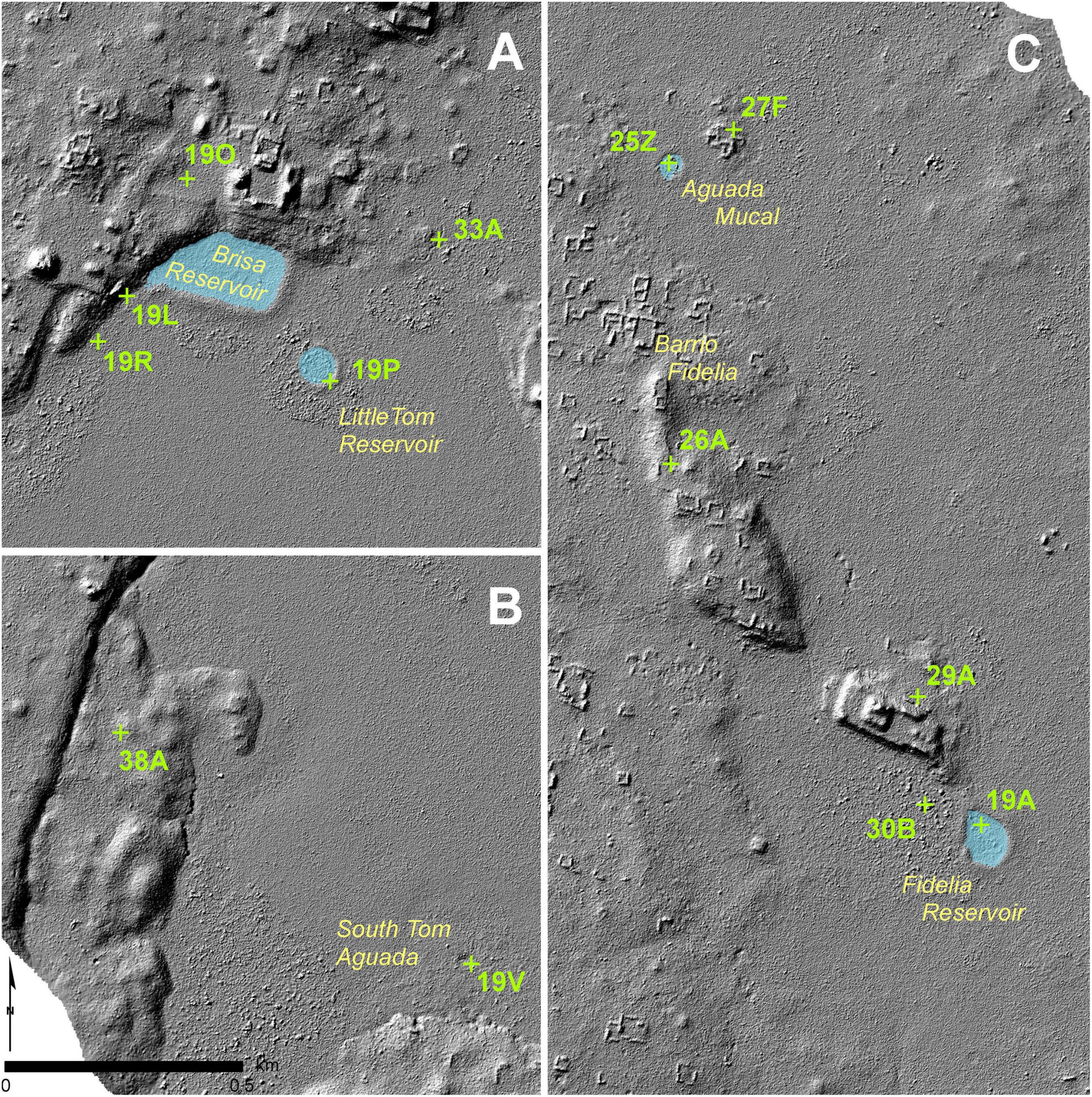
Figure 3. Close-up lidar images of: (A) the Brisa reservoir, (B) the South Tom aguada, and (C) the Fidelia reservoir.
Operation 30B (Soil Pit in Bajo)
Operation 30B was a 1 × 1.5 m trench dug in the floor of an inlet of the Bajo Laberinto south of the Fidelia Group. The trench revealed a Vertisol soil in which argilloturbation (clay-heaving with seasonal wetting and drying) has greatly distorted soil horizons (Dunning et al., 2019). A buried soil surface (2Abss horizon) was present at a depth varying between about 60 and 110 cm. Organic matter within this soil produced an AMS date of 410–550 CE. This soil surface thus represents a period of geomorphic stability when it was exposed and accumulated organic matter during the latter part of the Maya Early Classic period (Table 1). A sample taken from the buried soil contained ancient pollen. Common taxa in the pollen sample included open environment herbaceous plants with sedge (22.5%), low spine Asteraceae (37.5%), Cheno-Ams (mostly Amaranthus and Chenopodium species lumped together) (4%), and grasses (8%).
The eDNA sample from Operation 30B came from the same context (80 cm BS) and time period (Early Classic, 410–550 CE) as the pollen sample. The length of the 24 eDNA sequences analyzed from this sample ranged from 2715 to 153 bp. The plant groups with the largest number of sequences represented in the eDNA samples were the palm family (Arecaceae) with eight sequences and the Moraceae family with seven sequences (four from Ficus, two from Brosimum [B. alicastrum and Brosimum sp.] and one indeterminate). There were two sequences from the Apiaceae, and one sequence each from the grass family (Poaceae), the Asteraceae (Bidens sp.), the Sapotaceae (Sideroxylon tepicense [Standl.] T.D.Penn.), the Pinaceae (Pinus sp.), the Apocynaceae (Forsteronia sp.), and the mahogany family (Meliaceae). The eDNA results overlap in many areas with the pollen profile, for example the Arecaceae, Asteraceae, Moraceae, Pinaceae, and Poaceae were recovered by both methodologies. The eDNA technique did detect several families that were not detected by the pollen analysis, i.e., the Sapotaceae, Meliaceae, Apocynaceae, and the Apiaceae. The evidence of arboreal vegetation was more substantial from the eDNA results than from the pollen data because most Neotropical trees are zoophilous pollen producers and generate small amounts of large sticky pollen grains that are scarce in the ambient pollen rain. The few trees that are wind-pollinated, e.g., ramón and bullet tree (Terminalia buceras [L.] C.Wright), were not observed in the pollen sample, likely due to the overabundance of wind pollinated taxa such as the sedges, Cheno-Ams and composites. This underscores the value of using both of these techniques in tandem because the overall breadth of detection of vascular plants is greatly enhanced.
Operation 19A (Fidelia Reservoir)
The Fidelia Reservoir (Figure 3) is located adjacent to the large Preclassic Fidelia group featuring monumental architecture and likely received runoff from its platform via a ramp (Dunning et al., 2022). The reservoir lies near the edge of one of the many finger-like inlets of the sprawling Bajo Laberinto. Operation 19A was a 1 × 1.5 m trench excavated into the floor of the reservoir. Eight pollen samples were collected from the trench profile with the lowermost sample from above a bedrock and cobble floor at 150 cm in depth BS and an associated AMS radiocarbon date of 360–170 BCE. This places the creation of the reservoir sometime during the Late Preclassic period. At a depth of 135 cm, there is a clear break in the sediment strata, most likely the result of ancient Maya dredging which was carried out to maintain water capacity (Akpinar-Ferrand et al., 2012; Dunning et al., 2022). A lack of ceramic sherds above 135 cm suggests that these sediments accumulated mostly after the reservoir fell into disuse late in Yaxnohcah’s occupation and thereafter, a finding affirmed by a radiocarbon date of 993–1155 CE obtained on organic matter in sediment at a depth of 80 cm BS (below surface).
The lowest and earliest pollen sequence (Figure 4) was high in sedge (Cyperaceae) pollen. This reading indicated an extensive wetland at or before the time of the reservoir construction. Thereafter, the sedge pollen signature declined especially in relationship to disturbance taxa, possibly because the reservoir impacted the local hydrology and affected the surrounding wetland. At a depth of 140 cm, the sedge pollen signature was most abundant but afterward there was a notable increase in pollen from the Asteraceae (composite or sunflower family), Amaranthaceae, and Poaceae (grass) families. These changes were reflective of an opening up or clearance of the area to accommodate agriculture. Indeed, the observed presence of maize and cotton pollen are also indicative of those changes. Note that around 130 cm and higher in the profile, arboreal pollen became more prevalent and indicated an expansion of bajo forest, likely in those areas less well suited to agriculture. Also note that around the 140 cm depth pine (Pinus sp.) and oak (Quercus sp.) pollen grains began to appear in higher percentages. Both pine and oak are anemophilous species and the pollen of both taxa can travel long distances. The signals we observe from the pollen in the Fidelia reservoir, however, indicate that there were stands of pine and oak nearby and it is likely that a biome indicative of these pollen counts, such as a pine savanna, existed somewhere in the area. The uppermost pollen data (most recent) continue to reflect agricultural activity with abundant disturbance taxa and maize pollen. Also, the arboreal taxa remain a feature of the landscape until the uppermost levels. The radiocarbon date (cal. 993–1155 CE) at 80 cm suggests that our samples captured much of the Maya occupation of Yaxnohcah, in this sample beginning in the Late Preclassic period and ending in the Postclassic.
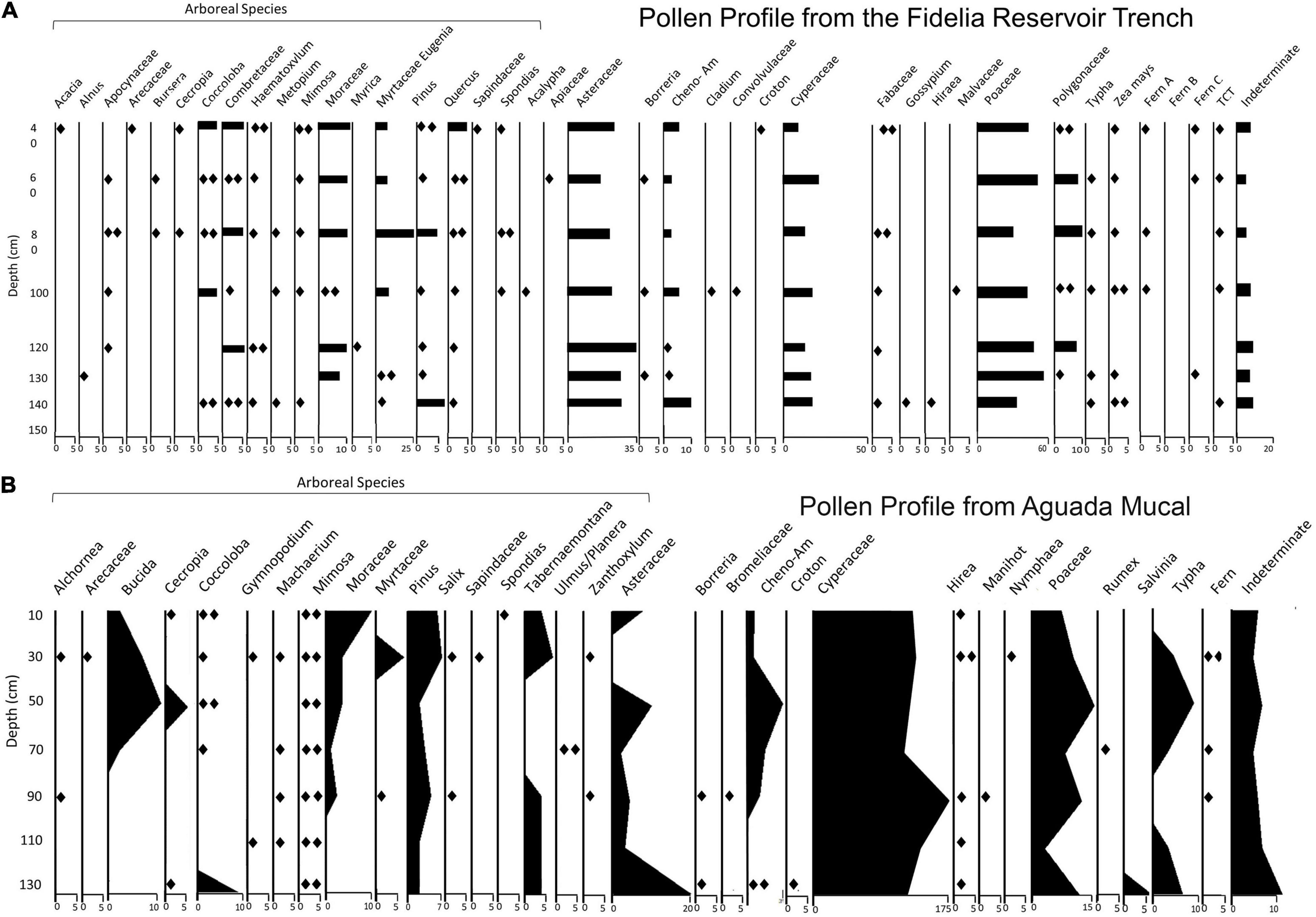
Figure 4. Pollen profiles taken from Operations 19A (A) and 25Z (B). Note that in pollen profile (A), calibrated radiocarbon dates of 360–170 BCE and 993–1155 CE were associated with the sediment from depths of 150 cm and 80 cm BS, respectively. In profile (B), organic matter from 130 cm BS produced an AMS date of 160 BCE–40 CE, and 90 cm BS produced an AMS date of 1160–1270 CE.
Operation 26A
Operation 26A was an excavation unit placed into a depression adjacent to a residential group in the “Fidelia Barrio” which is located to the northeast of the Fidelia platform. Excavations revealed a multi-household water tank that was constructed in a quarried depression.
Sample 2, Operation 26A
This sample from the A Horizon (Figure 5), which lies directly beneath the modern topsoil and humus layer at 30 cm BS. Sample 1 was from the uppermost part of the modern topsoil (humic layer or O horizon) and was not included in this analysis. The underlying cumulic A Horizon corresponds with excavation Lots 2, 3, and 10 from Operation 26A and was the source of Sample 2. These lots consisted of mostly clay sediment with some admixture of organic matter. Moreover, the context is super-positioned over a uniform and clearly defined Late Classic stratum (Figure 5). The composition of the soil indicates that it is likely the result of slumping colluvium that accumulated in the depression after the site was abandoned. In light of these indications, our assessment of this layer is that it is associated with the Postclassic period or perhaps even the early historic period, though the colluvium may have included earlier material.
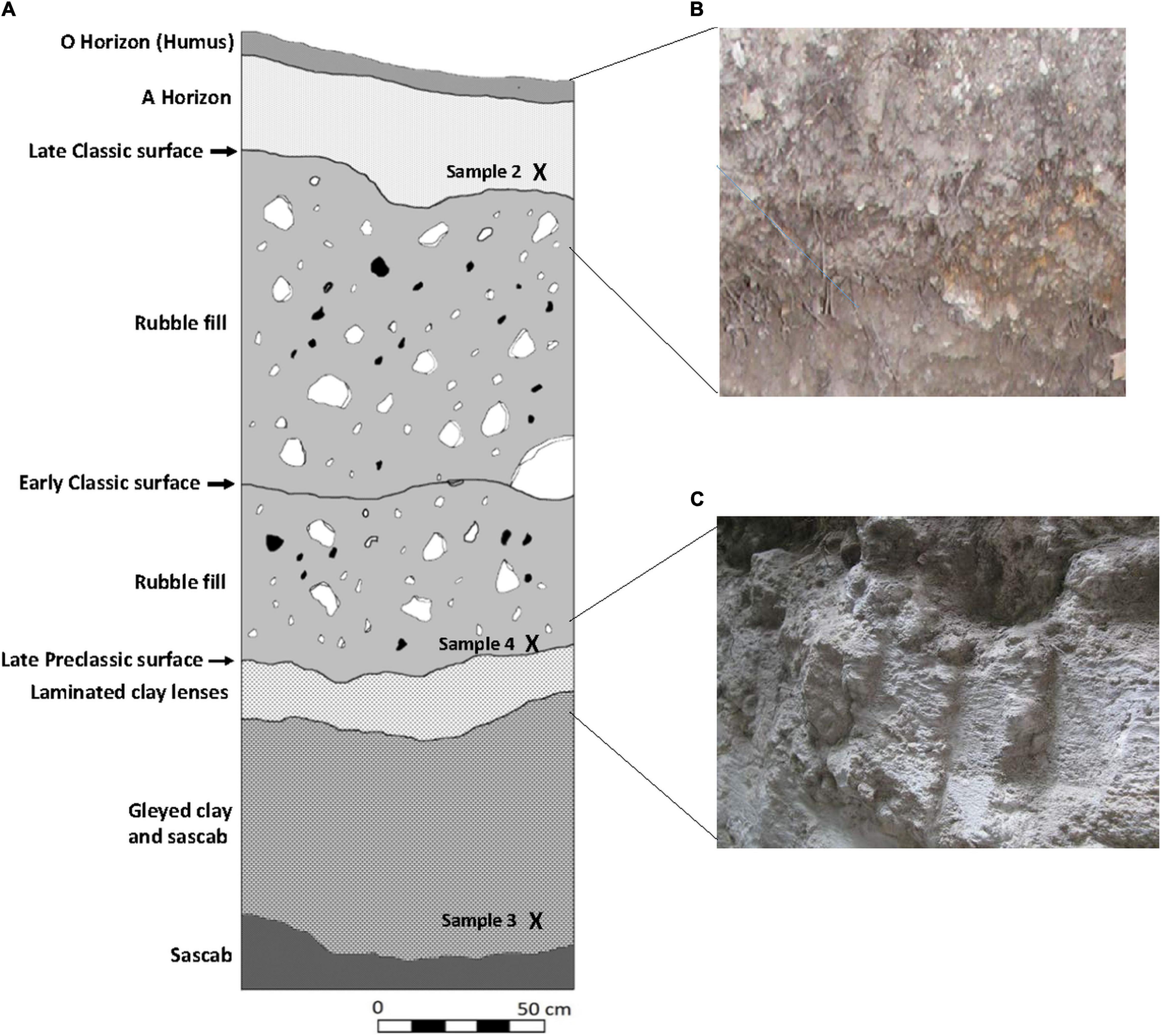
Figure 5. Profile drawing and photograph of Operation 26ABC. The “X” marks the location where the eDNA samples were collected. (A) Drawing of the entire profile, (B) photograph of the (A) horizon and (C) is a photograph of the Late Preclassic surface.
Plants identified from the eDNA analysis in this sample contained several species of trees, including B. alicastrum, Sapindus sp., Ceanothus sp., Tabernaemontana litoralis Kunth, Cascabela thevetia (L.) Lippold, D. lateriflora, Albizia sp., P. piscipula, and Cordia sp. Cordia sp. In addition there were species, likely tree species, in the Lauraceae, Annonaceae, and Arecaceae (palms). Herbaceous plants included Hybanthus sp., Physalis sp., numerous grass sequences (8) and two ferns (Hemionitis sp. and Adiantum tricholepis Fée). Finally, there was also a sequence from the Bignoniaceae, likely representing a vine species. This set of plants suggests some stage of secondary growth or perhaps transition forest that was prevalent in this locality during the Postclassic period.
Sample 3, Operation 26A
Sample 3 was retrieved from a gleyed (frequently water-logged) layer found near the bottom of the pit around 280 cm depth. The sample (#3) comes from a deposit of weathering sascab and clay lying atop pure sascab (highly chemically altered limestone). All identifiable ceramics (N = 15) were types belonging to the Late Preclassic period (400 BCE–200 CE), including Sapote Striated, Sierra Red, and Polvero Black. An AMS radiocarbon date of 50 BCE–80 CE was associated with the base of the construction fill and the laminated clay layers beneath it which agree with the ceramic correlation.
The plant remains in the eDNA record from Sample 3 were heavily dominated by trees in the Sapotaceae (13 gene sequences) with species Chrysophyllum caimito L. (3 sequences), M. zapota (2 sequences), and P. campechiana. The Sapindaceae had nine sequences with genera Matayba and Talisia represented with one sequence each. The Rutaceae had nine sequences but all were identified only to the family level. The Asteraceae was represented by eight sequences, seven of which were from Lactuca sp. The Moraceae family had three sequences and two came from the dominant forest species, B. alicastrum. The grasses (Poaceae) had three sequences identified. Three sequences of C. thevetia in the Apocynaceae were found. Other taxa represented were Lonchocarpus guatemalensis Benth. (Fabaceae), D. lateriflora (Putranjivaceae), Piper sp. (Piperaceae), Cordia sp. (Boraginaceae), and the Annonaceae each were represented by one sequence.
Sample 4, Operation 26A
This eDNA sample came from a zone of rubble fill just above a constructed “floor” within the depression above the zone with laminated (probably water-laid) clays at 195 cm BS (Lot 14). Identifiable sherds were from the Kiwi’ complex (Early Classic).
Results of the eDNA analysis of Sample 4 sediments revealed that there was a strong presence of plants in the Moraceae with six sequences, one of which was from Ficus sp. and another was from B. alicastrum. Five sequences were present from the Apocynaceae with two sequences identified to species: C. thevetia and Tabernaemontana donnell-smithii Rose. Three sequences from the Rosaceae were identified and two were recovered from the Poaceae. The Arecaceae (palm), Meliaceae (C. odorata), and the Violaceae (Hybanthus yucatanensis Millsp.) were represented by one sequence each. These results are indicative of secondary or transition forest.
Operation 29A
Operation 29A was an excavation within a chultun (subterranean chamber) located directly north of the Fidelia monumental platform. The main features of the chultun relevant to this discussion include a sascab floor (Lot 15) and a burial in the levels (Lots 20 and 22) below the floor.
Sample 39, Operation 29A
This eDNA sample came from 262 cm BD (below datum) below Floor #1 in the chultun (Figure 6) and was associated with an array of specialized artifacts that were placed just above a burial with an associated AMS radiocarbon date of 80–240 CE. The stratum contained numerous Kiwi’ (Early Classic) and Wob mode ceramics (Terminal Preclassic).
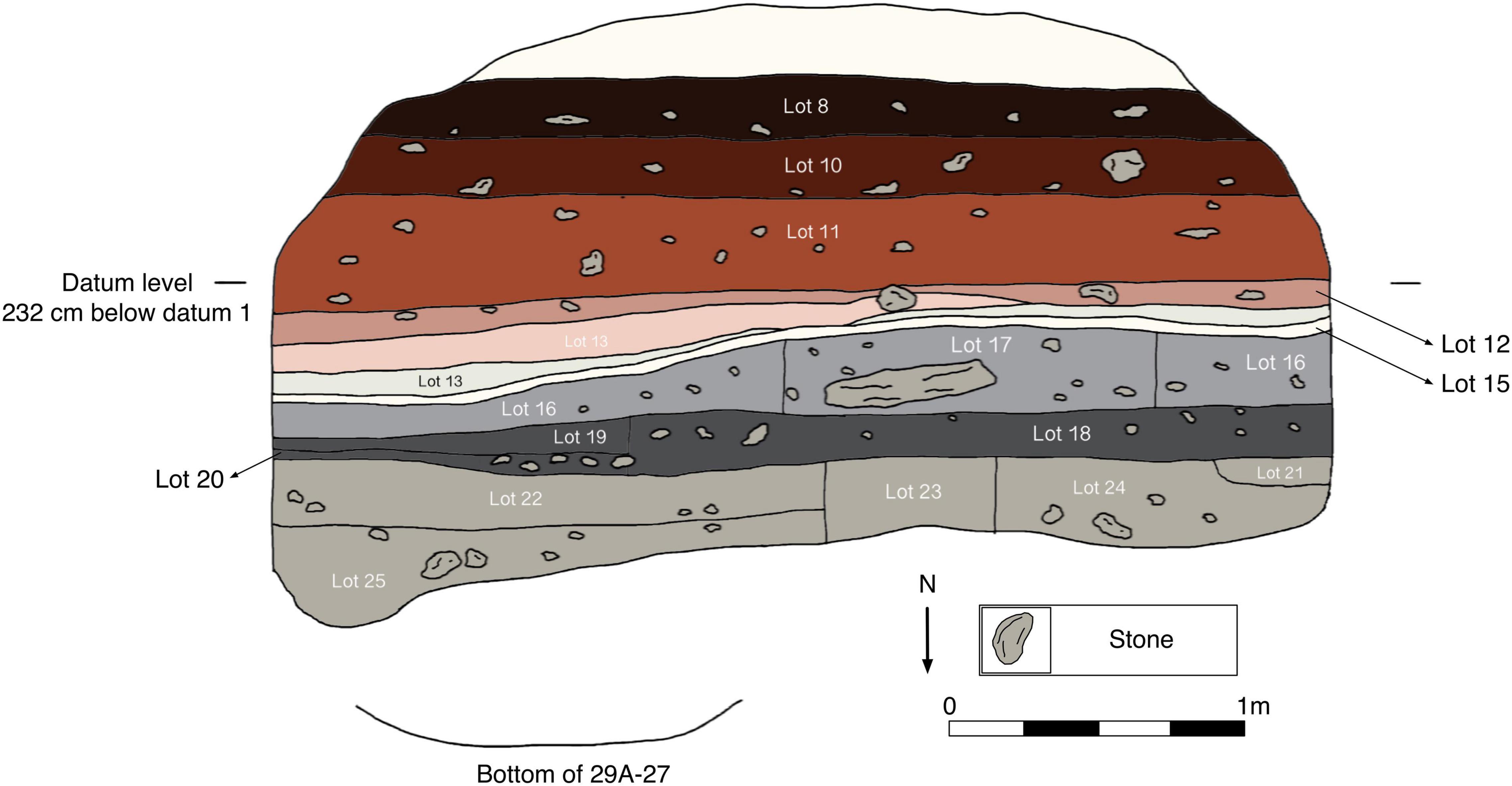
Figure 6. Profile drawing of Operation 29A (the chultun next to the Fidelia complex). Lot number 15 corresponds with Floor #1 discussed in the text.
The eDNA data from this chultun stratum were dominated by 12 sequences from the Boraginaceae family which included 2 species from the Ehretia genus (E. tinifolia L. [3], E. latifolia Loisel. [2], and Ehretia sp. [3]). Ten sequences were recovered that represented the Fabaceae (bean) family including Pithecellobium sp. (2 sequences), Senegalia sp. (2 sequences), Piptadenia sp. (1 sequence), and the remaining sequences were identified only to family. Nine sequences were recovered from the Moraceae family including two sequences from Brosimum sp., one representing Ficus sp., and the rest of the Moraceae sequences were identifiable only to the family level. Seven sequences came from the Lauraceae family including Damburneya coriacea (Sw.) Trofimov & Rohwer (one sequence), Litsea sp. (one sequence), and the rest were identified only to the family level. Two species in the Piperaceae were also identified including Piper auritum Kunth with two sequences and Piper guazacapanense Trel. & Standl. with one sequence. Drypetes sp. in the Euphorbiaceae, the Poaceae family, and the Apocynaceae family were identified with two sequences each. Other taxa identified in this sample with one sequence each included Bursera simaruba (L.) Sarg. (Burseraceae), Lactuca sp. (Asteraceae), the Anacardiaceae, Bignoniaceae, Cannabaceae, Malpighiaceae, Nyssaceae, Pteridaceae (ferns), Solanaceae, Gentianales, Liliales, and the Zingiberales.
Sample 40, Operation 29A
Sample 40 from Operation 29A came from the fill layer (230 cm BD) above Floor #1. It is highly likely that this sample was derived from Early Classic contexts and this assessment is supported by the discovery of numerous sherds from the Kiwi’ complex within this stratum. The environmental DNA results revealed the strong presence of Apocynaceae sequences (6) with one from C. thevetia and another from Tabernaemontana sp. The other four could only be identified to the family or order level. Four sequences were found from the Zingiberales, two sequences were identified from the grass family, including one from Saccharum villosum Steud., and two sequences could be assigned to the Moraceae.
Sample 41, Operation 29A
Sample 41 in Operation 29A was positioned above Floor #1 in a zone of laminated clays (252 cm BD). Kiwi’ phase ceramics (Early Classic) were associated with this level and an AMS date of 240–390 CE was recorded from within it. The Moraceae family was the most common taxon represented in the eDNA data set with six sequences, one of which could be identified to species, Maclura tinctoria (L.) D.Don ex Steud. The Boraginaceae was represented by four sequences, all of which could be identified to Ehretia sp. or E. tinifolia L. The grass family (Poaceae) was represented by two sequences and all other taxa identified, i.e., Astronium sp. (Anacardiaceae), Arecaceae, Bignonia sp. (Bignoniaceae), Celtis sp. (Cannabaceae), Sapindaceae, Rhamnaceae, and the Rutaceae were each represented by one DNA sequence.
Aguada Mucal
Operation 25Z (Aguada Mucal Core)
Operation 25Z was a core taken from saturated sediments in the floor of Aguada Mucal, a circular reservoir located in a narrow arm of the Bajo Laberinto (Figure 2). An excavation in the earthen berm surrounding the reservoir revealed that it was initially created in the Middle Preclassic period (Chmilar and Dunning, 2019). The berm was significantly elevated toward the end of the Early Classic period, probably in conjunction with a major dredging episode of sediments that had accumulated within the reservoir interior. Another excavation in the floor of the reservoir revealed a large stone-lined filtration well and evidence that at least this portion of the reservoir had been reopened in the Early Postclassic period. The Operation 25Z core reached a depth of about 133 cm BS; organic matter from 130 cm BS produced an AMS date of 160 BCE–40 CE, within the Late Preclassic period. A sharp stratigraphic break occurs at about 100 cm BS and probably reflects dredging that removed all Classic period sediment. Organic matter at a depth of 90 cm BS produced an AMS date of 1160–1270 CE, within the Early Postclassic period.
Seven pollen samples (Figure 4) were collected as a sequence from the Operation 25Z core. Well preserved pollen was present in all samples. Sedge pollen was the dominant pollen type throughout the sequence indicating its growth around the margins of the aguada. Open-air taxa were also well represented in the sequence with pollen from cattail, Asteraceae. Cheno-Ams, and grasses occurring in most samples. These taxa prefer cleared or disturbed areas and are usually associated with agriculture or clearing for settlement. Cultigens were poorly represented in this sequence, but a single Manihot esculenta (manioc) grain was noted in the sample from 90 cm BS dating to the Early Postclassic. Manioc pollen is large and heavy, and even this fragmentary grain would not travel any distance from its source, indicating that manioc plants were cultivated very near the aguada, possibly atop the berm. Bullet tree pollen was scarce in this sequence, occurring only in the uppermost four samples, and then in low percentages.
Also from Operation 25Z in Aguada Mucal, eDNA Sample 51, collected from 90 cm BS revealed a substantial amount of information about plants in the vicinity. As with the pollen data, the eDNA data indicated a significant signal from grasses (four sequences) which suggested an open landscape. Other taxa identified from the eDNA analysis included 11 sequences from the Boraginaceae which were mostly Ehretia sp. (6 sequences) or E. tinifolia (2) with the rest identified only to the family level. The Sapotaceae was represented by M. zapota (five sequences) Pouteria sp. (one sequence). The Lauraceae had six sequences with two identified as D. coriacea and the rest remained at the family level. Four sequences were retrieved from the Moraceae with two identified as Ficus sp. and Ficus paraensis (Miq.) Miq., a third from Brosimum sp. and another at the family level. There were three sequences from the Sapindaceae. Two sequences each were recovered from the Rutaceae family and the Piperaceae family from the genus Piper sp. Other taxa represented by only one sequence included Tanaecium tetragonolobum (Jacq.) L.G. Lohmann (Bignoniaceae), Celtis sp. (Cannabaceae), Coulteria platyloba (S.Watson) N.Zamora (Fabaceae), Alvaradoa amorphoides Liebm. (Picramnaceae), C. thevetia (Apocynaceae), Pistacia sp. (Anacardiaceae), Casearia thamnia (L.) T.Samar. & M.H.Alford (Salicaceae), Vitis sp. (Vitaceae), Hydrangea sp. (Hydrangeaceae), Nelumbo lutea Willd. (Nelumbonaceae), Malvaceae, Meliaceae, Apiales, Gentianales, and Zingiberales.
In addition to the pollen and eDNA results obtained from Aguada Mucal, several macrobotanical remains were identified from a nearby context. In a midden behind a residential complex just east of Aguada Mucal, a Zea mays kernel was found in a Late Classic period context (Operation 27F Lot 2). Lower down in that same midden, a Celtis iguanea pit was found in an Early Classic/Late Classic deposit (Operation 27F Lot 9). Other Z. mays L. kernels (2) were recovered from excavations in the Aguada Mucal berm (Operation 25A) in Late Preclassic-Late Classic deposits.
Bajo Tomatal
Operation 19R
Operation 19R was a 1 × 1.5 m trench dug into the floor of Bajo Tomatal near its northwestern edge (Dunning et al., 2019). The steep escarpment face nearby shows evidence of ancient stone quarrying. Erosion from these adjacent uplands occurred in pulses that buried two ancient soil surfaces from which pollen was recovered. Sample 1 (2Abss horizon) from 55 cm BS produced an AMS date of 160 BCE–40 CE and represents a Late Preclassic age soil surface. Sample 2 (3Abss horizon) from 120 cm BS dates to 800–550 BCE and is a Middle Preclassic soil surface.
The deeper context (Sample 66, 120 cm) was dominated by Asteraceae, Cheno-Ams, and Poaceae pollen. These are all essentially herbaceous taxa and suggest human activity (clearing) around the bajo margins. Cattail pollen is moderately common in this sample at 5.5%. These plants prefer to remain permanently wet and favor an environment with high phosphate levels. The eDNA data from 19R (Sample 66) offer similar results and provide more information about insect pollinated species. In the sample from 120 cm (Early Middle Preclassic) we also find evidence of bullet tree, Amaranthus sp. and grass. In addition, sequences of avocado (Persea americana), ramón, lechoso (Tabernaemontana littoralis), palm, Curculigo sp., C. thevetia, Dalbergia sp., Ocotea sp., and Cynanchum sp. were identified.
The Late Preclassic sample (#64) from 55 cm BS in Operation 19R, is similar in species composition to the earlier sample. There is an increase in sedge pollen in this level, and an absence of cattail pollen, possibly representing sedimentation along the margin of the bajo as indicated by the pulse of sediment out of which the 2Abss soil developed. Sedimentation of bajos within parts of the EIR was common during the Preclassic period, a phenomenon that contributed to the hydrologic drying along with general climatic drying (Dunning et al., 2006; Beach et al., 2018). Bullet tree pollen is also more common in this 55 cm sample, though the low numbers of bullet tree grains in both of these samples may reflect just a few or even a single nearby tree.
In the eDNA sample from 55 cm in Operation 19R (the Late Preclassic sample), grasses, and legumes were common and avocado (P. americana), white sapote (Casimiroa edulis), ramón, Tabernaemontana undulata Vahl, mahogany (S. macrophylla), silillon (P. campechiana), pine (Pinus sp.), Senegalia sp., Damburneya sp., Trichilia sp., and Anemopaegma sp., Fagaceae (probably Quercus sp.) were identified as well. Many of these are large trees, such as mahogany, ramón, and silillon are typically found in the upland forest.
Operation 19L (Soil Pit Behind Agricultural Terrace)
The eDNA Sample 19L (20 cm BS) is from the soil behind a presumed agricultural terrace wall. This terrace is one of a series of footslope terraces situated at the base of the steep escarpment forming the northwest margin of Bajo Tomatal (Dunning et al., 2019). The sherds from this terrace and its cumulic soil are from the Late Classic period. Although an AMS radiocarbon date of 1810–1917 CE was obtained from the sample stratum, the soil is shallow and there has been substantial root penetration and probably other bioturbation.
Data from the eDNA analysis in this sample revealed a strong signal from the Fabaceae family with 18 sequences identified including: Acacia sp. (3 sequences), Pithecellobium sp. (2 sequences), Leucaena trichandra (Zucc.) Urb, S. gaumeri (3 sequences), Senegalia sp. (2 sequences), Zapoteca portoricensis (Jacq.) H.M.Hern. (1 sequence), and the rest identified to the family level only.
The Burseraceae had 11 sequences total: 3 from Bursera simaruba, 3 from B. fagaroides (Kunth) Engl., 1 of Bursera sp., and 4 could be identified to the family level only. There were five sequences from the Lauraceae including one from D. coriacea, one from Litsea sp., one from P. americana and two that were identifiable to the family level. There were four sequences identified from the Moraceae, two were B. alicastrum and two could be identified to the family level. Two sequences from the grass family (Poaceae) were recorded. Four sequences from oak (Quercus sp.) were identified. Two species in the Malvaceae were observed: Ceiba sp. and Pseudobombax ellipticum (Kunth) Dugand. A single sequence was found for each of the following taxa: S. mombin (Anacardiaceae), M. depressa (Annonaceae), Rochefortia spinosa (Jacq.) Urb. (Boraginaceae), Neea psychotrioides Donn. Sm. (Nyctaginaceae) Drypetes sp. (Putranjivaceae), Pouteria sp. (Sapotaceae), Bignoniaceae, Cucurbitaceae, and Theaceae.
Operation 19V (South Tom Aguada Core)
Operation 19V was a sediment core taken through standing water in the floor of South Tom Aguada, a relatively small circular reservoir lying near the southern edge of Bajo Tomatal (Figure 3). The core penetrated to a depth of 108 cm, but the very saturated sediment compressed to a length of 25–26 cm during extrusion from the coring tube. Pollen samples (Figure 7) were collected from 24, 20, 16, 12, 8, and 4 cm BS. Organic sediment from 25 cm BS yielded a Late/Terminal Preclassic calibrated date of 80–250 CE. Unlike many reservoirs, the sediments within the South Tom core exhibit no clear evidence of a stratigraphic break resulting from ancient dredging. Organic matter from 15 cm produced an AMS date of 660–960 CE corresponding to the Late to Terminal Classic period. While the basal sample contained insufficient grains for analysis, all other samples contained good to near perfectly preserved pollen grains. Concentration values increased up the column reflecting a slow degradation of organic materials through time. All samples were dominated by pollen from bullet trees, which still flank the aguada. Moraceae pollen was also fairly common in the samples, likely representing ramón. Open area taxa were common throughout the sequence represented by Cheno-Ams, sedges, and grasses. These taxa are largely heliophytes, preferring cleared or disturbed areas and are often associated with clearing for agriculture or settlement. Maize was represented by single grain occurrences in the 16 and 12 cm samples. Other potentially significant taxa that represent economic species include Coccoloba sp. present in all samples and Spondias sp. present in one sample.
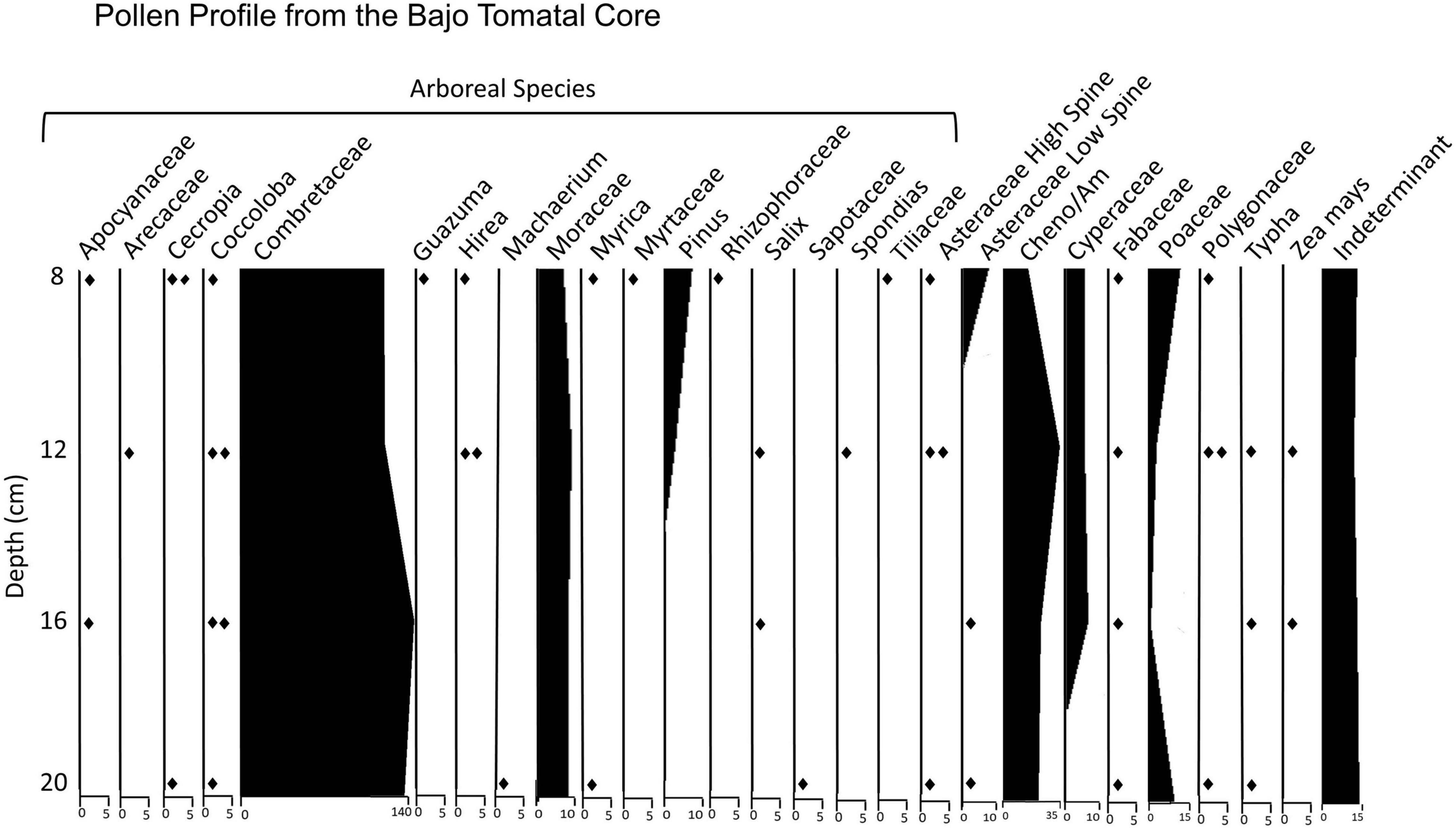
Figure 7. Pollen profile from Operation 19V, the South Tom Reservoir core. Sediment from 25 cm BS yielded a Late/Terminal Preclassic calibrated date of 80–250 CE. Organic matter from 15 cm produced an AMS date of 660–960 CE.
Interestingly, we obtained charcoal samples from our macroremain flotation sampling from Operations 19P, 33A, and 38A that were in an around the Bajo Tomatal. These charcoal samples with mostly small particles reflect episodes of burning in the bajo that began during the Late Middle Preclassic (33A, Lot 9 and 33A, Lot 12) and ended during the Postclassic period (33A, Lot 15) with numerous episodes in between.
Brisa Complex (and Adjacent Depression)
Operation 19O
This excavation took place in a depression that is located to the west of the Brisa monumental architecture complex (Figure 2) and just above and to the north of the Brisa reservoir. An eDNA sample (#72) and ceramics from this context were recovered from a paleosol that was buried by later episodes of sedimentation that eroded into the basin from the surrounding area. This sample was taken from 100 cm BS at the base of the paleosol. All ceramics collected from this level were from the Macal and Um complexes which are associated with the Early Middle Preclassic and Late Middle Preclassic periods, respectively.
Plant remains recovered from this context using the eDNA extraction protocol included two plant groups with known cultivars: two sequences of Pouteria sp. (Sapotaceae), which is the genus of the zapote fruit tree and one sequence of the Cucurbitaceae which is the squash family. Other taxa found in this sample included the vine Adenocalymma sp. (Bignoniaceae), Lactuca sp. (Asteraceae), Apocynaceae (four sequences), Asparagaceae (one sequence), Lauraceae (one sequence), Malpighiaceae (one sequence), and the Moraceae (one sequence).
Discussion
The data presented from our three sources of information, i.e., pollen, eDNA, and macrobotanical results, provide useful insights into the land use practices of the ancient Maya of Yaxnohcah. Information gleaned from these data sources are summarized in Table 3 and will be discussed in this section as they relate to the impact of human activities on the local vegetation, reservoir construction, agricultural practices, and indications of ceremonial activities.
Anthropogenic Environmental Impacts
In general, our data indicate that portions of the forests surrounding Yaxnohcah were cleared for agricultural purposes, but patches of the upland and bajo forests, although somewhat fragmented, remained intact. We see evidence for this from the Fidelia Reservoir pollen sequence and from the South Tom Aguada sequences. In the former, the bajo forest pollen signal remains robust through time even after the wetlands were reduced in the Postclassic period. Despite a substantial clearance taking place as indicated by a strong disturbance signal from heliophilic species in the later phases of the pollen series, the percentages of bullet tree and ramón pollen, two forest dominants, remain relatively constant through time. Curiously, the Bajo Tomatal pollen sample tells us of some infilling of the bajo during the Maya occupation, suggesting that there may have been an influx of sediment from the upland areas, perhaps as a result of erosion from deforested upland areas that were under cultivation. This would have been a problem for the Yaxnohcah farmers because the fertile upland soils are not excessively deep. As a possible blessing in disguise, however, this kind of erosion scenario could have stimulated greater cultivation of the bajo fringes as seen elsewhere in the Maya Lowlands (Dunning et al., 2015, 2019).
An unanticipated aspect of the pollen, macrobotanical, and eDNA data is that they all indicated the presence of pine and oak in the Yaxnohcah area during Precolumbian times. Neither pine nor oak was observed in our modern forest transects or in the vegetation surveys of other local researchers (e.g., Folan et al., 1999), but it now seems likely that these plants grew there in the past. Our earliest pollen sample (Operation 19A) reveals that pine and oak pollen appear in Late Preclassic contexts. Similarly, the earliest appearance of the pine in the eDNA samples is in the Terminal Preclassic period (Supplementary Table 1). Likewise, the earliest pine macroremains we have for Yaxnohcah came from Terminal Preclassic contexts (Operation 20D, Lot 20). Information from all of these data sets suggest that the pine savanna appeared after the first Maya colonizers arrived in the Yaxnohcah area and this biome was present at least until the Postclassic period (as seen in the macrobotanical samples from Operation 35B). It is possible that the pine and oak pollen could have blown in from afar, but it is far less likely that the leaves (probably the source of most of the DNA that we have been analyzing) would have blown in from any great distance. Thus, the evidence strongly indicates that, in the past, there was a pine savanna, probably with some oaks, somewhere in the area around Yaxnohcah.
Oaks are fire-resistant trees that often form associations with pine. There are several species of oak that are native to Campeche (Quercus corrugata Hook., Q. cortesii Liebm., Q. eliptica Nee, Q. insignis M. Martens & Galeotti, and Q. oleoides Schltdl. & Cham.), but the latter, Q. oleoides, is most likely the source of the pollen and eDNA evidence because it is the only oak of the five that is adapted to lowland areas (Standley and Steyermark, 1952; Pennington and Sarukhan, 2005; Parker, 2008; Royal Botanic Gardens, Kew, 2021).
The most likely location for pine savannas would have been in the interior areas of the huge Bajo Laberinto. Soils within parts of this bajo are both gypsic and acidic (Gunn et al., 2002), edaphic conditions that would favor pine colonization and which are similar to soils documented in pine savannas in the Bajo de Santa Fe, northeast of Tikal (Laws, 1961; Dunning et al., 2015, 2019). Other likely places where the pine savannas could have thrived could have been on the faces of the escarpments surrounding Bajo Tomatal or in frequently burned areas along the margins of the bajos.
Elsewhere, pine savannas have been documented in southern Quintana Roo, northern Guatemala, and northern Belize (Perry, 1991) so seed stocks of pine are not far from the area today and may have been even closer in the past. Many of the tropical pine stands are believed to have developed as a result of repeated burning without giving the tropical forest trees enough time to re-seed themselves after a burn (Perry, 1991). As we have numerous archeological charcoal samples from throughout our excavations, we posit that this is what happened in the Yaxnohcah area during Preclassic times.
Pine is a widely used product among many contemporary Maya groups. The Tzeltal and Tzotzil of central Chiapas use pine for furniture, domestic utensils, houses, and fences (Berlin et al., 1974; Breedlove and Laughlin, 2000). The Kekchi and Mopan of central Belize also use the wood to construct houses (Thompson, 1930). It is widely used as a fuel (Wisdom, 1940; Oakes, 1951; Breedlove and Hopkins, 1971; Barrera Marin et al., 1976; Atran and Ucan Ek’, 1999; Breedlove and Laughlin, 2000). Because it is easier to carry after it is carbonized, pinewood can be converted to charcoal near the source of the wood then carried by carboneros to markets in 30–40 kg loads (Hehr, 1967). Similarly, pine charcoal was found in two archeological contexts in the Sakjol marketplace of Yaxnohcah (Operation 35B) suggesting that the ancient Maya of Yaxnohcah also may have used pine charcoal as a commodity for exchange.
Beyond the everyday applications, Maya groups utilize pine as components of rituals, often combined with copal incense (Wisdom, 1940; Vogt, 1969; Berlin et al., 1974; Tedlock, 1982; Deal, 1988; McGee, 1990; Atran and Ucan Ek’, 1999; Breedlove and Laughlin, 2000; McAnany, 2010). Furthermore, paleoethnobotanical observations provide a deep history of pine use by the ancient Maya. Pine has been observed in a diversity of domestic contexts, especially middens and platform fill (Miksicek, 1983, 1991; Lentz, 1989, 1991, 1999; Lentz et al., 1996, 1997, 2005; Wiesen and Lentz, 1999; Dickau and Lentz, 2001; Morehart, 2001, 2003; Morehart et al., 2005). Pine has been reported from caves, which were communication portals with the Maya gods of the underworld (Morehart, 2001; Morehart et al., 2003), in caches at Caracol (Chase and Chase, 1998, p. 317), in tombs at Copan (Lentz, 1991), and ritual offerings at the site of La Milpa (Hammond, 2000).
It may be that the Maya created the pine savannas inadvertently as a result of repeated burning for agricultural purposes. Our macrobotanical remains indicate that there was repeated burning in the bajos throughout the Maya occupation. Alternatively, it is also possible that the Maya deliberately created the pine savannas as a source of valuable fuel. This would have been a productive and utilitarian form of agroforestry among the Maya who apparently endured for close to a millennium at Yaxnohcah. Parenthetically, the population expansion of nearby Calakmul during the Late Classic period would have created a ready fuel market for the local carboneros.
Reservoirs of Yaxnohcah
Excavations at the Aguada Mucal indicate that the reservoir was created by dredging from the center during the Early Middle Preclassic period and using the dredged material (mostly clay) to create a wall or berm around the center. This had the effect of lowering the floor of the reservoir and raising the berm to impound a larger volume of water. At some point during the Early Postclassic period this reservoir was re-opened by dredging out accumulated sediment from a stone-lined filtration well in the center of the reservoir and the immediate area surrounding it. The cultivation of manioc resumed in this area at the same time and this activity could have been enhanced during dry periods by the presence of a year-round water source.
The South Tom Aguada sequence reflects a typical profile from an aguada located near agricultural fields and a nearby settlement. This reservoir may have served a double function: both as a source of drinking water and a source for pot irrigation if needed. Similarly, the Fidelia Reservoir could have served both functions, as well, as it was conveniently located near a settlement and an agricultural area.
The multi-household water tank of Operation 26A in the Fidelia Barrio was an unusual find. It was originally a quarry that was converted into a neighborhood or household reservoir. Such household reservoirs are very common across the Yaxnohcah urban landscape, though the quarries out of which many of these features were constructed are seldom as deep (Brewer, 2018; Brewer and Carr, 2021).
Agricultural Adaptations
In terms of agricultural activity at Yaxnohcah, we have solid evidence, from both the pollen and macrobotanical sources, that they were growing maize in a broad number of contexts. Other crops in evidence at Yaxnohcah include chili peppers (Capsicum annuum), squash (Cucurbita sp.), manioc (M. esculenta), and cotton (Gossypium hirsutum). Also, a number of domesticated or semi-domesticated fruit trees were found, including Acrocomia aculeata, Chrysophyllum cainito, Ehretia tinifolia, Persea americana, and Spondias purpurea. In addition to the cultivated plants there were numerous wild food plants identified, i.e., Amaranthus sp., Chenopodium sp., S. mombin, Mosannona depressa, Chamaedorea sp., Lactuca sp., Celtis iguanaea, Brosimum alicastrum, Nelumbo lutea, Coccoloba spp., Casimiroa edulis, Manilkara zapota, Pouteria sp., and Vitis sp. Many other plants used for food, construction, medicine, fuel, and other purposes can be found in Table 2.
Several different techniques for the practice of agriculture were observed at Yaxnohcah. One of these was the use of footslope terraces as seen in Operation 19L on the northwest side of Bajo Tomatal. The stone-faced terraces, just to the south of the Brisa civic/ceremonial complex, were extremely effective at collecting the colluvium that washed off of the adjacent escarpments. Evidence of avocados, hog plums, zapotes, white sapotes, and cucurbits were found on these terraces, suggesting that they were used for the cultivation of orchards as well as field crops.
Another area that appears to have served as an agricultural garden was the depression just to the southwest of the Brisa Complex (Operation 19O) in what was probably upland forest at one time. This was one of the earliest agricultural areas identified by the project (Early Middle Preclassic period). Among the plant remains in this context were sequences from Pouteria sp. (the genus of the zapote tree) and plants from the Cucurbitaceae. Here again, the evidence suggests it was a mixed-use garden with potential orchard trees and field crops. The large number of economically useful trees from Operation 26A in the Fidelio Barrio, e.g., C. cainito, M. zapota, B. alicastrum, Talisia sp., Lonchocarpus guatemalensis, and Cascabela thevetia indicate that this was an anthropogenically managed forest something like a zapotal or more likely a home garden or orchard. Like the Brisa depression garden, the Fidelio Barrio orchard was positioned on land that was once upland forest, the habitat with the most fertile soils in the region.
Bajo margins also seemed to have been contexts favored by the Maya of Yaxnohcah for agricultural purposes as seen in the area around the South Tom Aguada. Economic taxa found there included maize, wild grapes, and hogplums. The excavations near the Fidelia Aguada (Operation 19A) revealed that the Maya were growing maize and cotton on the bajo fringes during the Late Preclassic period. Similarly, bajo margins were employed for the cultivation of manioc on the lands adjacent to the Aguada Mucal in the Postclassic period.
Ceremonial Activities
The eDNA Sample 39 from Operation 29A, located just north of the Fidelia structural complex, came from a chultun, an example of underground features that are often associated with Maya ceremonial activities. This sample was of great interest because it was located beneath a floor (Floor #1) and was associated with a large number of artifacts including numerous Early Classic ceramics, obsidian objects, a bi-valve seashell and figurines that were placed above a deteriorated burial. The largest number of DNA sequences found in this sample (8) came from the genus Ehretia. Also, there was a sequence from the genus Ficus. The bark of both of these genera are believed to have been sources of fibers for making paper by the ancient Maya (Martínez del Campo Lanz, 2018). The cluster of artifacts and human remains provide a strong indication that this was some kind of a ceremonial cache and the Ehretia and Ficus sequences may well represent the remains of some kind of paper or bark cloth. Paper was involved in Maya ceremonies in many ways. In bloodletting rituals the practitioner would pierce a body part and then allow the blood to fall on paper. The bloodied paper was then burned as a sacrificial offering to the deities (Miller and Taube, 1993). Priests often dressed in paper vestments and used the material in many ways for ceremonial apparel such as headbands and the elaborate headdresses depicted on the altars of Tikal (Miller and Taube, 1993). The codices, or ancient books of the Maya, were made of bark paper and were highly prized objects, suitable as grave offerings for a deceased ruler, scribe, or priest. A bark paper codex was found as a grave offering in an elite Maya burial at Uaxactun in Guatemala and at several other Maya sites, as well (Carter and Dobereiner, 2016). Ethnographically, Maya healers have been observed placing healing ritual bundles beneath floors (Faust and López, 2006) and the Maya practice of placing caches in both non-domestic and domestic ceremonial contexts is believed to be of great antiquity (McAnany, 2010).
Likewise, Sample 41 from Operation 29A offers some equally intriguing plant remains. This sample comes from just above Floor #1 in the chultun and because of the associated Kiwi’ phase ceramics, is also an Early Classic deposition. As with Sample 39, Ehretia has the largest number of DNA sequences in Sample 41 along with five from the Moraceae (the fig family). In addition, this context also has a sequence from Maclura tinctoria, a well-known source of dye or ink (Balick et al., 2000). Thus, the juxtaposition of plants that are sources of bark paper and a plant that is the source of ink reinforces the suggestion that these finds, located in ceremonial contexts, represent the remains of ancient bark paper used as ritual paraphernalia, dyed bark cloth garments, or possibly as Maya books or codices.
Conclusion and Outlook
The data from our research revealed that there were stands of upland and scrub swamp (or bajo) forest during the Maya occupation, although they were substantially reduced in size and distribution from what is currently observable. Agriculture using cotton, manioc, squash, and maize was common near the reservoirs in the bajos and likely in the uplands, as well. Also, a rich diversity of tree crops was observed. In terms of land management, it appears likely that the Maya of Yaxnohcah converted at least some of their habitat to pine savanna. Whether this was intentional or a by-product of swidden activities is unknown, but it appears that they maintained, possibly employing agroforestry techniques, and exploited this biome for over a millennium, from the Late Preclassic to the Postclassic time periods. All of these observations are indications that the Maya modified their Neotropical landscape, but nevertheless managed their fields and forests prudently enough to sustain themselves over the many centuries they occupied Yaxnohcah.
Recent research from Amazonia (Pueyo et al., 2010; Boulton et al., 2022) has reported increasingly rapid degradation of rainforest habitats, some of it due to widespread burning, thus reducing the environmental resilience of the entire region and its ability to offer critical ecosystem services at a time when they are needed most. If our study offers any consolation to the observed dilemma, it is that tropical forests do harbor great resilience as seen in the modern return of robust tropical deciduous forest to the lands around Yaxnohcah after some of the landscape had been converted to pine savanna and remained that way for over a thousand years.
A fundamentally important aspect of this research effort is that we have demonstrated how pollen, macrobotanical studies and eDNA analysis can be integrated to provide a more thorough evaluation of environmental conditions and plant use practices of the past. The eDNA data are an excellent complement to the pollen data because pollen can reflect plant growth over a broad range of territory as windborne pollen can travel great distances. The eDNA data tend to reflect the leaves that fall into reservoirs and other contexts and thereby provide information about plants in the immediate area. Also, the eDNA data collection can detect plants that have different pollination syndromes and do not favor anemophilous or zoophilous pollen producers. The eDNA technique helped us to identify plants that were involved in ceremonial activities at the site, thereby enriching our analyses. In short, the techniques of pollen, macrobotanical and eDNA analysis are complementary and can be used together as powerful tools for paleoenvironmental analysis.
Data Availability Statement
The datasets presented in this study can be found in online repositories. The names of the repository/repositories and accession number(s) can be found below: NCBI (accession: PRJNA782546 and PRJNA804249).
Author Contributions
DL, TH, ND, JJ, KR-T, AA, ET, and AW designed the research protocols for this project. DL, TH, ND, KR-T, SM, AG, and MV accomplished the data analysis and data interpretation, and helped to wrote the manuscript. All authors contributed to the manuscript and approved the submitted version.
Funding
The United States National Science Foundation [Grants BCS-1632392 (ND and DL); BCS-1642547 (DL, ND, and TH)], the Social Sciences and Humanities Research Council of Canada Grants 430-2017-00190 and 892-2019-3070 (KR-T), a University of Calgary URGC Seed Grant (KR-T), the Universidad Autónoma de Campeche (AA), and the University of Cincinnati Intellectual Property Fund (AW) provided financial support for this research.
Conflict of Interest
JJ is employed by Archaeological Consulting Services, Ltd.
The remaining authors declare that the research was conducted in the absence of any commercial or financial relationships that could be construed as a potential conflict of interest.
Publisher’s Note
All claims expressed in this article are solely those of the authors and do not necessarily represent those of their affiliated organizations, or those of the publisher, the editors and the reviewers. Any product that may be evaluated in this article, or claim that may be made by its manufacturer, is not guaranteed or endorsed by the publisher.
Acknowledgments
We thank Dr. Annette Rowe for advice and access to her Lab Equipment. Dr. Stephen Houston was especially helpful in offering suggestions about the utility of the potential bark paper find. Site reports and other basic data from the Yaxnohcah project are available online through the following link: https://www.mesoweb.com/resources/informes/Yaxnohcah2016.html.
Supplementary Material
The Supplementary Material for this article can be found online at: https://www.frontiersin.org/articles/10.3389/fevo.2022.868033/full#supplementary-material
References
Akpinar-Ferrand, E., Dunning, N. P., Lentz, D. L., and Jones, J. G. (2012). Use of aguadas as water management sources in two southern Maya Lowland sites. Anc. Mesoam. 23, 85–101. doi: 10.1017/s0956536112000065
Arellano Rodriguez, J. A., Flores Guido, J. S., Tun Garrido, J., and Cruz Bojorquez, M. M. (2003). Etnoflora Yucatanense: Nomenclatura, Forma de Vida, Uso, Manejo y Distribución de las Especies Vegetales de la Península de Yucatán Facultad de Medicina Veterinaria y Zootecnia. Mérida: Universidad Autónoma de Yucatán, 20.
Atran, S., and Ucan Ek’, E. (1999). “Classification of useful plants by the northern Peten Maya (Itzaj),” in Reconstructing Ancient Maya Diet, C. D. White (Salt Lake City: University of Utah Press), 19–59.
Balick, M. J., Nee, M. H., and Atha, D. E. (2000). Checklist of the Vascular Plants of Belize. New York, NY: New York Botanical Garden Press.
Barrera Marin, A., Vazquez, A. B., and Lopez Franco, R. M. (1976). Nomenclatura etnobotanica Maya: Una Interpretacion Taxonomica. Mexico City: Instituto Nacional de Antropologia e Historia.
Beach, T. Luzzadder-Beach, S., Cook, D., Krause, S., Doyle, C., Eshleman, S., et al. (2018). Stability and instability on Maya Lowlands tropical hillslope soils. Geomorphology 305, 185–208. doi: 10.1016/j.geomorph.2017.07.027
Berlin, B., Breedlove, D. E., and Raven, P. H. (1974). Principles of Tzeltal Plant Classification: an Introduction to the Botanical Ethnography of a Mayan-Speaking People of Highland Chiapas. New York, NY: Academic.
Bhattacharya, T., Chiang, J. C. H., and Cheng, W. (2017). Ocean-atmosphere dynamics linked to 800–1050 CE dry interval in Mesoamerica. Quat. Sci. Rev. 169,, 263–277. doi: 10.1016/j.quascirev.2017.06.005
Boege, E. (1993). El desarrollo sustentable y la reserva de la biosfera de calakmul, Campeche, México. Bol. Antropol. Am. 28, 99–132.
Boulton, C. A., Lenton, T. M., and Boers, N. (2022). Pronounced loss of Amazon rainforest resilience since the early 2000s. Nat. Clim. Change 12, 271–278. doi: 10.1038/s41558-022-01287-8
Bray, D., and Klepeis, P. (2005). Deforestation, forest transitions, and institutions for sustainability in Southeastern Mexico, 1900-2000. Environ. Hist. 11, 195–223. doi: 10.3197/096734005774434584
Breedlove, D. E., and Hopkins, N. A. (1971). A study of Chuj (Mayan) plants with notes on their uses II. Wasmann J. Biol. 29, 107–128.
Breedlove, D. E., and Laughlin, R. M. (2000). The Flowering of Man: a Tzotzil Botany of Zinacantan, ed. Abridged. Washington, DC: Smithsonian Institution Press.
Brewer, J. L. (2017). A Landscape Archaeology Approach to Understanding Household Water Management Practices of the Ancient Lowland Maya. Doctoral dissertation. Cincinnati, OH: University of Cincinnati.
Brewer, J. L. (2018). Householders as water managers: a comparison of domestic-scale water management practices from two central Maya Lowlands sites. Anc. Mesoam. 29, 197–217. doi: 10.1017/s0956536117000244
Brewer, J. and Carr, C. (2021). Household quarry-reservoirs at the ancient maya site of Yaxnohcah, Mexico. Lat. Am. Antiq. 16, 1–9. doi: 10.1017/laq.2021.95
Carter, N. P., and Dobereiner, J. (2016). Multispectral imaging of an early classic Maya codex fragment from Uaxactun, Guatemala. Antiquity 90, 711–725. doi: 10.15184/aqy.2016.90f
Chase, D. Z., and Chase, A. F. (1998). “The architectural contexts of caches, burials, and other ritual activities for the Classic period Maya (as reflected at Caracol, Belize),” in Function and Meaning in Classic Maya Architecture, ed. S. D. Houston (Washington, DC: Dumbarton Oaks), 299–332.
Chmilar, J. A., and Dunning, N. (2019). “Excavaciones en la aguada mucal: operación 25,” in Proyecto Arqueológico Yaxnohcah, Informe de las Temporadas de Investigaciones 2017-18. Report Submitted to the Consejo de Arqueología, eds V. A. Vázquez López, K. Reese-Taylor, and A. A. Hernández (Mexico: Instituto Nacional de Antropología e Historia).
Cooper, A., and Poinar, H. (2000). Ancient DNA: do it right or not at all. Science 289:1139. doi: 10.1126/science.289.5482.1139b
Deal, M. (1988). “Recognition of ritual pottery in residential units: an ethnoarchaeological model of the Maya family altar tradition,” in Ethnoarchaeology Among the Highland Maya of Chiapas, Papers of the New World Archaeological Foundation no. 26, eds T. A. Lee and B. Hayden (Provo: Brigham Young University), 61–90.
Dickau, R., and Lentz, D. (2001). “Diets under duress: paleoethnobotanical evidence from dye Late Classic Maya of Aguateca,” in Paper Presented of the 66th Annual Meeting for the Society for American Archaeology, New Orleans, LA.
Douglas, P. M. J., Pagani, M., Canuto, M. A., Brenner, M., Hodell, D. A., Eglinton, T. I., et al. (2015). Drought, agricultural adaptation, and sociopolitical collapse in the Maya Lowlands. Proc. Natl. Acad. Sci. U.S.A. 112, 5607–5612. doi: 10.1073/pnas.1419133112
Dunning, N. P., Wahl, D., Beach, T. P., Jones, J. G., Luzzadder-Beach, S., and McCane, C. (2014). “The end of the beginning: Drought, environmental change, and the Preclassic to Classic transition in the east-central Yucatan Peninsula.” in The Great Maya Droughts in Cultural Context, ed. G. Iannone (Boulder, CO: University of Colorado Press), 107–129.
Dunning, N., and Beach, T. Luzzadder-Beach, S. (2006). “Environmental variability among bajos in the southern Maya Lowlands and its implications for ancient Maya civilization and archaeology,” in Pre-Columbian Water Management, eds L. Lucero and Fash, B. (Tempe, AZ: University of Arizona), 111–133.
Dunning, N. P., Beach, T., and Luzzadder-Beach, S. (2012). Kax and kol: collapse and resilience in Lowland Maya civilization. Proc. Natl. Acad. Sci. U.S.A. 106, 3652–3657. doi: 10.1073/pnas.1114838109
Dunning, N. P., Brewer, J. C., Carr, A., Anaya Hernandez, T., Beach, J., Chmilar, L., et al. (2022). “Harvesting ha: ancient water collection and storage in the elevated interior region of the Maya Lowlands,” in Sustainability and Water Management in the Maya World and Beyond, eds J. Larmon, L. Lucero, and F. Valdez. (Boulder, CO: University Press of Colorado), 13–52. doi: 10.2307/j.ctvx070r2.8
Dunning, N. P., Griffin, R., Jones, J. G., Terry, R., Larsen, Z., Carr, C. (2015). “Life on the edge: Tikal in a bajo landscape,” in Tikal: Paleoecology of an Ancient Maya City, eds Lentz, D. L., Dunning, N. P., and Scarborough, V. L. (Cambridge: Cambridge University Press), 95–123.
Dunning, N. P., Hernández, A. A., Beach, T., Carr, C., Griffin, R., Jones, J. G., et al. (2019). Margin for error: anthropogenic geomorphology of Bajo edges in the Maya Lowlands. Geomorphology 333, 127–145. doi: 10.1016/j.geomorph.2018.09.002
Epp, L., Zimmermann, H., and Leichsenring, K. (2019). “Sampling and extraction of ancient DNA from sediments.” in Ancient DNA: Methods and Protocols, eds B. Shapiro, A. Barlow, P. Heintzman, M. Hofreiter, J. Paijmans, A. Soares et al. (Singapore: Springer), 31–44. doi: 10.1007/978-1-4939-9176-1_5
Faust, B. B., and López, J. H. (2006). “Maya healing ceremonies of Don Pedro Ucan Itza.” in Chocolate in Mesoamerica, ed. C. L. McNeil (Gainesville, FL: University of Florida Press), 408–427. doi: 10.5744/florida/9780813029535.003.0020
Folan, W. J., Sánchez González, M. C., and García Ortega, J. M. (1999). Naturaleza y Cultura en Calakmul, Campeche. (Campeche: Universidad Autónoma de Campeche).
Fulton, T. L., and Shapiro, B. (2019). “Setting up an ancient DNA laboratory.” in Ancient DNA, eds B. Shapiro, A. Barlow, P. Heintzman, M. Hofreiter, J. Paijmans, and A. Soares (Totowa, NJ: Humana Press), 1–14. doi: 10.1007/978-1-61779-516-9_1
Geovannini Acuña, H. (2008). Rain Harvesting in the Rainforest. (London: British Archaeological Reports).
Greller, A. M. (2000). “Vegetation in the floristic regions of North and Central America.” in Imperfect Balance, ed. D. L. Lentz (New York, NY: Columbia University Press), 39–87. doi: 10.1073/pnas.1714977115
Griffin, R., Oglesby, R., Sever, T., and Nair, U. (2014). “Agricultural landscapes, deforestation, and drought severity.” in The Great Maya Droughts in Cultural Context, ed. G. Iannone (Boulder, CO: University of Colorado Press), 71–86.
Gunn, J. D., Matheny, R. T., and Folan, W. J. (2002). Climate-change studies in the Maya area: a diachronic analysis. Anc. Mesoam. 13, 79–84. doi: 10.1017/s0956536102131105
Hamilton, T. L., Peters, J. W., Skidmore, M. L., and Boyd, E. S. (2013). Molecular evidence for an active endogenous microbiome beneath glacial ice. ISME J. 7, 1402–1412. doi: 10.1038/ismej.2013.31
Hammond, N. (2000). “The Maya lowlands: pioneer farmers to merchant princes.” in The Cambridge History of the Native Peoples of the Americas: Mesoamerica, Volume II, Part 1, eds R. Adams, and M. Macleod (Cambridge: Cambridge University Press), 197–249. doi: 10.1017/chol9780521351652.006
Haug, G. H., Gunther, D., Peterson, L. C., Sigman, D. M., Hughen, K. A., and Aeschlimann, B. (2003). Climate and the collapse of Maya civilization. Nature 299, 1731–1735. doi: 10.1126/science.1080444
Hehr, J. G. (1967). Charcoal: Its Multifarious Effect in a Rural Guatemalan Community. MA thesis. Kalamazoo, MI: Western Michigan University.
Hodell, D. A., Brenner, M., and Curtis, J. H. (2005). Terminal classic drought in the Northern Maya lowlands inferred from multiple sediment cores in Lake Chichancanab (Mexico). Quat. Sci. Rev. 24, 1413–1427. doi: 10.1016/j.quascirev.2004.10.013
Hodell, D. A., Curtis, J. H., and Brenner, M. (1995). Possible role of climate in the collapse of Classic Maya Civilization. Science 375, 391–394. doi: 10.1038/375391a0
Islebe, G. A., Carrillo-Bastos, A., Aragón-Moreno, A. A., Valdez-Hernández, M., Torrescano-Valle, N., and Cabanillas-Terán, N. (2019). “Holocene paleoecology and paleoclimatology of south and Southeastern Mexico: a palynological and geospatial approach,” in The Holocene and Anthropocene Environmental History of Mexico, eds N. Torrescano-Valle, G. A. Islebe, and P. D. Roy (Cham: Springer Nature Switzerland AG), 195–207. doi: 10.1007/978-3-030-31719-5_10
Jørgensen, T., Kjaer, K. H., Haile, J., Rasmussen, M., Boessenkool, S., Andersen, K., et al. (2012). Islands in the ice: detecting past vegetation on Greenlandic nunataks using historical records and sedimentary ancient DNA meta-barcoding. Mol. Ecol. 21, 1980–1988. doi: 10.1111/j.1365-294X.2011.05278.x
Kennett, D. J., Breienbach, S. F. M., Aquino, V. V., Asmerom, Y., Awe, J., Baldini, J. U., et al. (2012). Development and disintegration of Maya political systems in response to climate change. Science 338, 788–791. doi: 10.1126/science.1226299
Laws, W. D. (1961). Investigations of swamp soils from the Tintal and Pinal associations of Peten, Guatemala. Wrightia 2, 127–132.
Lentz, D. L. (1989). “Botanical remains from the El Cajon area: insights into a prehistoric dietary pattern,” in Archaeological Research in the El Cajon Region, 1: Prehistoric Cultural Ecology, Memoirs in Latin American Archaeology, No. 1, eds K. Hirth, G. L. Pinto, and G. Hasemann (Pittsburgh, PA: University of Pittsburgh), 187–206.
Lentz, D. L. (1991). Maya diets of the rich and poor: paleoethnobotanical evidence from Copan. Lat. Am. Antiq. 2, 269–287. doi: 10.2307/972172
Lentz, D. L. (1999). “Plant resources of the ancient Maya: the paleoethnobotanical evidence,” in Reconstructing Ancient Maya Diet, ed C. D. White (Salt Lake City: University of Utah Press), 3–18.
Lentz, D. L., Beaudry-Corbett, M. P., De Aguilar, M. L. R., and Kaplan, L. (1996). Foodstuffs, forest, fields and shelter: a paleoethnobotanical analysis of vessel contents from the Ceren site, El Salvador. Lat. Am. Antiq. 7, 247–262. doi: 10.2307/971577
Lentz, D. L., Dunning, N. P., Scarborough, V. L., Magee, K. S., Thompson, K. M., Weaver, E.,, et al. (2014). Forests, fields, and the edge of sustainability at the ancient Maya city of Tikal. Proc. Natl. Acad. Sci. U.S.A. 111, 18513–18518. doi: 10.1073/pnas.1408631111
Lentz, D. L., Hamilton, T. L., Dunning, N. P., Tepe, E. J., Scarborough, V. L., Meyers, S. A., et al. (2021). Environmental DNA reveals arboreal cityscapes at the ancient Maya Center of Tikal. Sci. Rep. 11:12725. doi: 10.1038/s41598-021-91620-6
Lentz, D. L., Magee, K., Weaver, E., Jones, J. G., Tankersley, K. B., Hood, A., et al. (2015). “Agroforestry and agricultural practices of the ancient Maya at Tikal,” in Tikal: Paleoecology of an Ancient Maya City, eds D. L. Lentz, N. P. Dunning, and V. L. Scarborough (Cambridge: Cambridge University Press), 152–185. doi: 10.1017/cbo9781139227209.009
Lentz, D. L., Ramirez, C. R., and Grimson, B. W. (1997). Formative period subsistence and forest product extraction at the Yarumela site, Honduras. Anc. Mesoam. 8, 63–74. doi: 10.1017/s0956536100001577
Lentz, D. L., Yaeger, J., Robin, C., and Ashmore, W. (2005). Pine, prestige and politics of the Late Classic Maya at Xunantunich, Belize. Antiquity 79, 573–585. doi: 10.1017/s0003598x00114516
Lentz, D. L., Hamilton, T. L., Dunning, N. P., Scarborough, V. L., Luxton, T. P., Vonderheide, A., et al. (2020). Molecular genetic and geochemical assays reveal severe contamination of drinking water reservoirs at the ancient Maya city of Tikal. Sci. Rep. 10:10316. doi: 10.1038/s41598-020-67044-z
Lundell, C. L. (1937). The Vegetation of Petén. Volume I. Carnegie Institution of Washington Publication 478. (Washington, DC: Carnegie Institution).
Luzzadder-Beach, S., Beach, T., Hutson, S., and Krause, S. (2016). Sky-earth, lake-sea: climate and water in Maya research. Antiquity 90, 426–442. doi: 10.15184/aqy.2016.38
Martínez, E., Sousa, S. M., and Ramos Álvarez, C. H. (2001). Listados Florísticos de México. XXII. Región de Calakmul Campeche. Mexico City: Instituto de Biología Universidad Nacional Autónoma de México.
Martínez del Campo Lanz, S. (2018). El Códice Maya de México, Antes Grolier. (Mexico City: Instituto Nacional de Antropología e Historia).
McAnany, P. A. (2010). Ancestral Maya Economies in Archaeological Perspectives. Cambridge: Cambridge University Press.
Medina-Elizade, M., and Rohling, E. J. (2012). Collapse of Classic Maya Civilization related to modest reduction in precipitation. Science 335, 956–959. doi: 10.1126/science.1216629
Miller, M., and Taube, K. (1993). The Gods and Symbols of Ancient Mexico and the Maya: An Illustrated Dictionary of Mesoamerican Religion. London: Thames and Hudson.
Miksicek, C. H. (1983). “Macrofloral remains in the Pulltrouser area: settlements and fields,” in Pulltrouser Swamp: Ancient Maya Habitat, Agriculture and Settlement in Northern Belize, eds B. L. Turner and P. D. Harrison (Austin, TX: University of Texas Press), 94–104. doi: 10.7560/750678-008
Miksicek, C. H. (1991). “The economy and ecology of Cuello,” in Cuello: An Early Maya Community in Belize, ed N. Hammond (Cambridge: Cambridge University Press), 70–97.
Miranda, F. (1958). “Estudios acerca de la vegetación,” in Los Recursos Naturales del Sureste y su Aprovechamiento, Vol. 2, ed. E. Beltrán (Mexico City: Instituto Mexicano de Recursos Naturales Renovables), 215–271.
Morehart, C. T. (2001). Preliminary Analysis of Paleoethnobotanical Samples from Pook’s Hill, Cayo District Belize. MA thesis. Tallahassee, FL: Department of Anthropology, Florida State University.
Morehart, C. T. (2003). “Paleoethnobotany at Avila,” in Belize Postclassic Project 2002: Investigation of the Shore Settlements of Progresso Lagoon and San Estevan, eds J. M. Ferguson, M. H. Oland, and M. Masson (Albany, NY: Occasional Publication No. 9. Institute of Mesoamerican Studies, University at Albany-SUNY), 126-151.
Morehart, C. T., Prufer, K. M., and Lentz, D. L. (2003). “Wood of the Gods: the ritual use of pine in ancient Maya society,” in Paper Presented at the 68th Annual Meetings of the Society for American Archaeology, Milwaukee, WS.
Morehart, C. T., Lentz, D. L., and Prufer, K. M. (2005). Wood of the Gods: the ritual use of pine (Pinus spp.) by the ancient Lowland Maya. Lat. Am. Antiq. 16, 255–274.
Oakes, M. (1951). The Two Crosses of Todos Santos: Survivals of Mayan Religious Ritual. New York, NY: Pantheon.
Parducci, L., Nota, K., and Wood, J. (2019). “Reconstructing past vegetation communities using ancient DNA from lake sediments,” in Paleogenomics, eds C. Lindqvist and O. P. Rajora (Singapore: Springer), 163–187. doi: 10.1007/13836_2018_38
Pearsall, D. M. (2009). Paleoethnobotany: A Handbook of Procedures, 2nd Edn. New York, NY: Routledge.
Pennington, T. D., and Sarukhan, J. (2005). Arboles Tropicales de México. Mexico City: Universidad Nacional Autonoma de México.
Pueyo, S., Lima de Alencastro Graca, P. M., Imbrozio Barbosa, R., Cots, R., Cardona, E., and Fearnside, P. M. (2010). Testing for criticality in ecosystem dynamics: the case of Amazonian rainforest and savanna fire. Ecol. Lett. 13, 793–802. doi: 10.1111/j.1461-0248.2010.01497.x
Reese-Taylor, K. (2017). “Founding landscapes in the central karstic uplands.” in Early Maya E-Groups, Solar Calendars, and the Role of Astronomy in the Rise of Lowlands Maya Urbanism, eds D. A. Freidel, A. Chase, and A. Dowd (Gainesville, FL: University Press of Florida), 480–513. doi: 10.2307/j.ctvx072vd.22
Reese-Taylor, K., Hernández, A., Flora Esquivel, A., Monteleone, K., Uriarte, A., Carr, C., et al. (2016). Boots on the Ground at Yaxnohcah. Adv. Archaeol. Pract. 4, 314–338. doi: 10.7183/2326-3768.4.3.314
Royal Botanic Gardens, Kew (2021). Oaks. Available Online at: http://plantsoftheworldonline.org/ [accessed November 21, 2021].
Scarborough, V. L., Dunning, N. P., Tankersley, K. B., Carr, C., Weaver, E., Grazioso, L., et al. (2012). Water and sustainable land use at the ancient tropical city of Tikal, Guatemala. Proc. Natl. Acad. Sci. U.S.A. 109, 12408–12413. doi: 10.1073/pnas.1202881109
Sønstebø, J. H., Gielly, L., Brysting, A. K., Elven, R., Edwards, M., Haile, J., et al. (2010). Using next-generation sequencing for molecular reconstruction of past Arctic vegetation and climate. Mol. Ecol. Resour. 10, 1009–1018. doi: 10.1111/j.1755-0998.2010.02855.x
Šprajc, I. (2015). Exploraciones Arqueologicas en Chactún, Campeche, Mexico. Prostor, kraj, cas 7. Ljubljana: Zalozba ZRC. 612547806 doi: 10.3986/9789
Šprajc, I. (2020). Paisaje Arqueologico y Dinamica Cultural en el Area de Chactún, Campeche (2016–2018): Informe de la Temporada 2018. Mexico City: Instituto Nacional de Antropología e Historia.
Šprajc, I., Dunning, N. P., Stajdohar, J., Hernández Gómez, Q., Chato López, I., Marsetiè, A., et al. (2021). Ancient Maya water management, agriculture, and society in the area of Chactún, Campeche, Mexico. J. Anthropol. Archaeol. 61:101261. doi: 10.1016/j.jaa.2020.101261
Standley, P. C., and Steyermark, J. A. (1952). Flora of Guatemala, Vol. 24, Part III. Chicago, IL: Chicago Natural History Museum.
Thompson, J. E. S. (1930). Ethnology of the Mayas of southern and central British Honduras. Field Mus. Nat. Hist. Anthropol. Ser. 17, 23–213.
Vogt, E. Z. (1969). Zinacantan: a Maya Community in the Highlands of Chiapas. Cambridge: Belknap Press of Harvard University.
Wahl, D., Byrne, R., and Anderson, L. (2014). An 8700-year paleoclimate reconstruction from the Southern Maya Lowlands. Quat. Sci. Rev. 103, 19–25. doi: 10.1016/j.quascirev.2014.08.004
Wahl, D., Schreiner, T., Byrne, R., and Hansen, R. (2007). A paleoecological record from a Late Classic Maya reservoir in the north Petén. Lat. Am. Antiq. 18, 212–222. doi: 10.2307/25063105
Wiesen, A., and Lentz, D. L. (1999). “Preclassic floral remains at Cahal Pech and Pacbitun, Belize,” in Belize Valley Preclassic Maya Project: Report on the 1996 and 1997 Field Seasons, Occasional Papers No. 12, ed. P. F. Healy (Peterborough: Trent University), 53–67.
Keywords: agroforestry, environmental DNA, lidar, pine savanna, reservoirs, Neotropical forest, Mesoamerica
Citation: Lentz DL, Hamilton TL, Dunning NP, Jones JG, Reese-Taylor K, Anaya Hernández A, Walker DS, Tepe EJ, Carr C, Brewer JL, Ruhl T, Meyers SA, Vazquez M, Golden A and Weiss AA (2022) Paleoecological Studies at the Ancient Maya Center of Yaxnohcah Using Analyses of Pollen, Environmental DNA, and Plant Macroremains. Front. Ecol. Evol. 10:868033. doi: 10.3389/fevo.2022.868033
Received: 02 February 2022; Accepted: 22 April 2022;
Published: 02 June 2022.
Edited by:
Priyadarsi D. Roy, National Autonomous University of Mexico, MexicoReviewed by:
Samantha Krause, Texas State University, United StatesCatalina González, University of Los Andes, Colombia, Colombia
Copyright © 2022 Lentz, Hamilton, Dunning, Jones, Reese-Taylor, Anaya Hernández, Walker, Tepe, Carr, Brewer, Ruhl, Meyers, Vazquez, Golden and Weiss. This is an open-access article distributed under the terms of the Creative Commons Attribution License (CC BY). The use, distribution or reproduction in other forums is permitted, provided the original author(s) and the copyright owner(s) are credited and that the original publication in this journal is cited, in accordance with accepted academic practice. No use, distribution or reproduction is permitted which does not comply with these terms.
*Correspondence: David L. Lentz, bGVudHpkbEB1Y21haWwudWMuZWR1; Alison A. Weiss, d2Vpc3NhYUB1Y21haWwudWMuZWR1