- 1Department of Biology, Wilfrid Laurier University, Waterloo, ON, Canada
- 2YukonU Research Centre, Yukon University, Whitehorse, YT, Canada
- 3Institute of Arctic Biology, University of Alaska Fairbanks, Fairbanks, AK, United States
- 4Département des Sciences du Bois et de la Forêt, Québec, QC, Canada
- 5School of Biological Sciences, Victoria University of Wellington, Wellington, New Zealand
- 6Center for Ecosystem Science and Society, Department of Biological Sciences, Northern Arizona University, Flagstaff, AZ, United States
In boreal North America, much of the landscape is covered by fire-adapted forests dominated by serotinous conifers. For these forests, reductions in fire return interval could limit reproductive success, owing to insufficient time for stands to reach reproductive maturity i.e., to initiate cone production. Improved understanding of the drivers of reproductive maturity can provide important information about the capacity of these forests to self-replace following fire. Here, we assessed the drivers of reproductive maturity in two dominant and widespread conifers, semi-serotinous black spruce and serotinous jack pine. Presence or absence of female cones were recorded in approximately 15,000 individuals within old and recently burned stands in two distinct ecozones of the Northwest Territories (NWT), Canada. Our results show that reproductive maturity was triggered by a minimum tree size threshold rather than an age threshold, with trees reaching reproductive maturity at smaller sizes where environmental conditions were more stressful. The number of reproductive trees per plot increased with stem density, basal area, and at higher latitudes (colder locations). The harsh climatic conditions present at these higher latitudes, however, limited the recruitment of jack pine at the treeline ecotone. The number of reproductive black spruce trees increased with deeper soils, whereas the number of reproductive jack pine trees increased where soils were shallower. We examined the reproductive efficiency i.e., the number of seedlings recruited per reproductive tree, linking pre-fire reproductive maturity of recently burned stands and post-fire seedling recruitment (recorded up to 4 years after the fires) and found that a reproductive jack pine can recruit on average three times more seedlings than a reproductive black spruce. We suggest that the higher reproductive efficiency of jack pine can explain the greater resilience of this species to wildfire compared with black spruce. Overall, these results help link life history characteristics, such as reproductive maturity, to variation in post-fire recruitment of dominant serotinous conifers.
Introduction
Much of the fire-prone boreal forest consists of serotinous conifers. Serotinous species possess an aerial seed bank that releases seeds in the initial years after a fire (Lamont et al., 2020), facilitating seedling recruitment and promoting stand self-replacement and resilience to fire (Ilisson and Chen, 2009; Johnstone et al., 2009a). Since the end of the last glaciation, the boreal forest has been characterized by a disturbance regime of large, frequent, stand-replacing fires (Lynch et al., 2002). In recent decades, the boreal biome has warmed at rates 3–4× faster than the global average (IPCC, 2018), which has resulted in an increase in the size, frequency, and severity of fires (Kasischke and Turetsky, 2006; Coops et al., 2018). For fire-adapted species, changes in the fire regime may limit reproductive success and alter forest composition (Johnstone et al., 2010; Buma et al., 2013; Enright et al., 2014). Shorter fire return intervals and intense canopy fires may both result in damage to or death of a large number of seeds in the cone bank, thereby reducing viable seed rain and consequently recruitment of serotinous conifers following fire (Zasada et al., 1979; Arseneault, 2001; Johnstone and Chapin, 2006; Johnstone et al., 2009a,2010).
Post-fire recruitment of serotinous conifers is affected by climatic limitations at northern latitudes. Low temperatures, short growing seasons and low stem densities reduce pollen production, seed viability and seedling survivorship (Elliott, 1979; Black and Bliss, 1980a; Viereck and Johnston, 1990; Sirois, 1992; Trant et al., 2018). While ongoing warming should reduce these climatic constraints on tree growth and reproduction at the northern range edge, leading to increased recruitment and growth of trees, contrasting responses have been reported with respect to treeline advance (Harsch et al., 2009; Brown et al., 2019; Lantz et al., 2019). This may be related to the fact that successful tree recruitment requires multiple individuals to accomplish several reproductive milestones, from the creation of reproductive bud primordia through pollination to the maturation of seeds (Brown et al., 2019).
Reproductive maturity, i.e., the initiation of cone production, is a fundamental life-history trait, closely linked to fitness in an environment (Stearns, 2000). Conifers must reach a minimum age and/or size threshold to initiate cone production, a trait that varies among species and in response to disturbance regimes and environmental gradients (e.g., stand structure or climate) (Obeso, 2002; de Jong and Klinkhamer, 2005; Climent et al., 2008; Andrus et al., 2020). Variability in reproductive age/size thresholds has been linked to differential allocation of resources to growth or reproduction. In fire-prone habitats, early reproductive maturity is crucial for self-replacement (Climent et al., 2008). However, even where a high proportion of individuals reach reproductive maturity, species-specific fitness and environmental constraints mediate their reproductive efficiency, i.e., the number of seedlings recruited per parent or reproductive tree. Understanding species-specific drivers of reproductive maturity and efficiency for the serotinous conifers dominating North American boreal forests has the potential to significantly improve the projections of tree recruitment dynamics and species-compositional shifts in response to climate warming and associated increases in fire activity.
Here we investigated the reproductive size and age thresholds, and the environmental drivers of reproductive maturity of two widespread boreal conifers, the semi-serotinous black spruce [Picea mariana (Mill) BSP] and the serotinous jack pine (Pinus banksiana Lamb.). We used a large observational study combining older (19–275 years after the last fire) and recently burned stands (less than 5 years after the last recent fire) from two geomorphologically distinct terrestrial ecozones (Ecosystem Classification Group, 2008, 2009), Taiga Plains and Taiga Shield, in the boreal forest of Northwest Territories, Canada. Our dataset represents a snapshot of reproductive maturity across a latitudinal gradient that approaches the treeline and throughout in the Taiga Shield and includes stands growing on sites with and without permafrost (perennially cryotic ground) in both ecozones. Most of the recently burned plots were sampled following a large (2.85 Mha) fire season that occurred in 2014 and impacted both ecozones (Walker et al., 2018b). By including recently burned plots in our study, we could examine the relationship between pre-fire reproductive maturity of the stands (inferred from counts of reproductive, fire-killed trees) and post-fire seedling recruitment. From these, we could estimate the reproductive efficiency, i.e., the number of seedlings recruited per reproductive tree, for both black spruce and jack pine. Specifically, we asked: (1) What drives reproductive maturity in dominant serotinous conifers, a minimum threshold of size, age, or both? (2) What are the main environmental drivers mediating higher or lower plot-level number of reproductive trees? (3) How do reproductive size and age thresholds, the number of reproductive trees, and reproductive efficiency vary by species and ecozone? These results will inform our understanding of limitations to tree regeneration and range expansion within a regime of increasing fire frequency, severity, and size.
Methods
Study Area and Target Species
The study area was located in the discontinuous permafrost zone (Zhang et al., 2008) of the Northwest Territories (NWT), Canada, and included the Taiga Plains ecozone (hereafter Plains) in the western part of the NWT, and the Taiga Shield ecozone (hereafter Shield) in the eastern part of the NWT (Supplementary Figure 1A). The Plains and the Shield ecozones differ in climate and soil conditions (Supplementary Appendix 1). The 30-year (1981–2010) climates normal for mean annual temperature and precipitation were −5.4°C (range: −7.4 to −4.5°C) and 309 mm (range: 267–397 mm), respectively, on the Plains and –3.6°C (range: −6.6 to −2.4°C) and 272 mm (range: 265–282 mm), respectively, on the Shield (Wang et al., 2016). The Plains overlie sedimentary bedrock whereas the Shield is characterized by exposed granite bedrock (Ecosystem Classification Group, 2008, 2009).
The most common and widespread tree species in both ecozones are black spruce and jack pine. Less abundant species include trembling aspen (hereafter aspen; Populus tremuloides Michx.) on the Plains and paper birch (hereafter birch; Betula papyrifera Marsh and B. neoalaskana Sargent) on the Shield. Black spruce is considered a transcontinental boreal species that forms the latitudinal treeline in northeastern and north-central Canada (Payette, 1983; Viereck and Johnston, 1990). It tolerates poor drainage, shallow permafrost soils, and active (seasonally thawed) layers as thin as 0.25 m (Ritchie, 1987). The distribution of jack pine extends from the eastern slope of the Rocky Mountains to Nova Scotia, Canada (Brandt, 2009). In contrast to black spruce, jack pine grows poorly in peatlands (Viereck and Johnston, 1990) and its range is limited by seasonal heat sums (growing degree-days above 5°C) and its intolerance of deep snow (McLeod and MacDonald, 1997). Both species re-expanded into northern Canada after the retreat of the ice sheet at 13,000 BP from southern and eastern glacial refugia (MacDonald, 2000; Jaramillo-Correa et al., 2004; Brandt, 2009). Black spruce spread rapidly and in western Canada was present from Alberta to the Northwest Territories and north of its modern range by 9,000 BP. The expansion of jack pine was slower; it reached its current northwestern limit in the Northwest Territories by 4,150 BP (McLeod and MacDonald, 1997) concurrent with the retreat of the spruce treeline southward to its current range (MacDonald, 2000).
Sampling Design and Stand-Level Forest Compositional Attributes
Between 2015 and 2018, we established 552 plots nested within 233 sites. 324 of these plots had not experienced a fire in at least 19 years (hereafter “old burned plots”) and the rest (228) burned between 2011 and 2014 (hereafter “recently burned plots”). Details about the sample design are provided in Walker et al. (2018a,b), Day et al. (2020). Briefly, sites were selected using a stratified random sampling design (Walker et al., 2018a). At each site we established a plot consisting of a 60 m2 belt transect at that location and at least one, but often two additional plots corresponding to different soil moisture classes within 100–500 m from the random plot. Soil moisture was classified by drainage, as dry (xeric or subxeric), moist (mesic-subxeric or mesic), or wet (mesic-subhygric or subhygric) following Johnstone et al. (2008). Soil organic layer (SOL) and residual SOL thickness were measured at 10 or 20 locations per plot for old and recently burned plots, respectively. Depth of burn was calculated 1 year after the fire in the burned plots by measuring the adventitious root height and the associated offset for black spruce sites, or residual SOL subtracted from average organic depth from old burned plots for jack pine dominated sites (Walker et al., 2018a). Pre-fire SOL values in the recently burned plots were reconstructed by summing the depth of burn, the residual SOL thickness, and a small offset value (Walker et al., 2018a). Graduated steel rods (2 m in length) pushed into the soil were used to determine the presence or absence of end-of-season ground ice in the plots, indicating ice-rich permafrost. For all sites, the depth to an impermeable layer was measured to reflect the rooting zone conditions.
To determine forest structure, we identified to species, counted, and measured the diameter at breast height (DBH; 1.3 m) of all woody stems reaching 1.3 m within the 60 m2 plots; this included fire-killed trees in the recently burned stands. We then calculated black spruce and jack pine stem density (trees⋅ha–1) and basal area (m2⋅ha–1) for each plot. As a measure of species dominance, we calculated the percentage of black spruce and jack pine trees per plot dividing the species’ stem count by the total number of trees per plot. For the recently burned plots we calculated pre-fire values of black spruce and jack pine stem density, basal area and the percentage of black spruce and jack pine trees from the counts and measurements of fire-killed trees. Plot age, a proxy of time since last fire, was estimated by tree ring counts from basal cookies and cores collected just above the root collar for five trees from the dominant size class of each dominant tree species, including fire-killed trees in the burned stands (Walker et al., 2018a).
Reproductive Maturity and Reproductive Efficiency
We define reproductive maturity as the initiation of cone production. We classed trees as reproductively mature if they bore at least one female cone (hereafter reproductive trees), and as non-reproductive otherwise. Thus, we scored the presence or absence of female cones within the crowns of all black spruce and jack pine trees in each belt transect (14,851 trees in total), including fire-killed trees in the recently burned plots. We also summed the number of reproductive black spruce and jack pine trees per plot and calculated plot-level density of reproductive trees (trees⋅ha–1). Black spruce and jack pine, as is the case for other serotinous conifers, accumulate closed cones in the canopy as a fire-adaptive trait (Lamont et al., 2020). Although black spruce are considered semi-serotinous and open their cones gradually in the absence of fire (Viereck and Johnston, 1990). Following reproductive maturity, conifers typically retain the cones, even those emptied of seeds, for long periods and thus it is unlikely that we would score a stem as juvenile a few years after it created its first cone (Alfaro-Sánchez et al., 2015).
We define reproductive efficiency as the number of seedlings recruited per reproductive tree. To measure the reproductive efficiency of black spruce and jack pine, we divided plot-level density of black spruce and jack pine seedlings established after fire, by plot-level density of reproductive black spruce and jack pine trees, respectively. Tree seedlings were identified and counted in five 1 × 1 m quadrats established at 6 m intervals along the eastern side of the transect in each of the recently burned plots. We then estimated plot-level density of black spruce and jack pine seedlings (seedlings⋅ha–1) by scaling the summed quadrat counts. Tree seedling counts were conducted between 2 and 4 years after the fires, a timeframe that has been shown to reflect future canopy cover (Lavoie and Sirois, 1998; Johnstone et al., 2004, 2020). Plots with no black spruce or jack pine both before and after the fire were excluded from this analysis. Reproductive efficiency of black spruce and jack pine was calculated for 203 and 129 plots, respectively.
Climatic Conditions
To characterize the climatic conditions at the sites, we retrieved 30-year gridded climate data (1985–2015) from the ClimateNA v5.60 software package (Wang et al., 2016). Selected variables included spring values of growing degree-days above 5°C (sum of daily temperatures above 5°C accumulated during the months of April-May, DD5), average spring monthly values of minimum temperature (Tmin, °C), and average spring-summer (April–August) monthly values of Hargreaves’ climatic moisture deficit (CMD, mm).
Spring DD5 was highly correlated with spring Tmin (r = 0.95, p < 0.001) and spring-summer CMD (r = 0.75, p < 0.001). We selected spring DD5 as a proxy for growing season length in our plot-level reproductive models because of its relevance to the reproductive potential, i.e., productivity of cones, seeds and viable seeds of boreal conifers (Elliott, 1979; Sirois, 1992, 2000; Trant et al., 2018; Brown et al., 2019). We also assessed temporal trends in spring DD5, spring Tmin and spring-summer CMD, by fitting linear regression models for each site between the individual climate variable and year (from 2000 to 2015; significance levels were α = 0.05).
Statistical Analyses
All statistical analyses and graphics were performed using R version 3.6.2 (R Core Team, 2019). We modeled the probability of presence of cones on the 10,862 individual living trees from the 324 old burned plots as a function of DBH or plot age, using generalized linear mixed models (GLMM) with a binomial response and logit link, using the R package glmmTMB (Brooks et al., 2017). The models were run separately for each predictor (DBH or plot age), for the two tree species and ecozones. Random effects for plot and site were introduced in the models to account for the hierarchical structure of the data, i.e., trees within plots that are nested within sites. We used the fitted models to estimate the size (DBH) and age thresholds at reproductive maturity for each species within each ecozone. For these models we only used living trees from the old burned plots because fire severity increased the reproductive thresholds of fire-killed trees in the recently burned plots (data not shown).
To determine the drivers of reproductive maturity we modeled the number of reproductive black spruce and jack pine trees per plot, from the 552 recently and old burned plots, using GLMMs with a negative binomial response using glmmTMB. The models were run by species and ecozone. Predictors included a factor for type of plot (recently burned or old burned plots), tree basal area, plot age (transformed into a categorical variable with three levels: less than 50 years; 50–100 years and older than 100 years), presence or absence of ground ice, SOL thickness, and DD5, with a random effect for site. Including the factor type of plot allowed us to determine if there were significant differences in the number of reproductive trees among types, controlling for the other predictors. The model for jack pine on the Shield did not include the factor for ground ice because jack pine was rarely present on sites underlain by permafrost. To account for possible nonlinearities in the reproductive response, we compared alternative full-saturated models including linear, quadratic, or spline adjustments (with up to three degrees of freedom) for each continuous predictor and selected the adjustment with the lowest Akaike Information Criterion (AIC) score (the best supported model corrected for parsimony; Crawley, 2013). Next, the selected saturated model was reduced by dropping uninformative covariates (according to AIC) using the dredge function from the R package MuMIn (Barton, 2022). Model fits were checked visually to ensure that they conformed to model assumptions. We calculated marginal r (i.e., the proportion of variance explained by fixed effects) and conditional r (i.e., the proportion of variance explained by both fixed and random effects) with the sjPlot R package (Lüdecke, 2021).
In the recently burned plots, we examined differences in density of reproductive trees, density of seedlings established after fire, and reproductive efficiency among the combined species-ecozone groups using the non-parametric Kruskal-Wallis rank sum tests to account for non-normality and heteroscedasticity.
Results
Differences in Reproductive Maturity Between Species and Ecozones
Despite the lack of young plots in our sampling design (98% of the plots were > 30 years old), only 40% of black spruce and 55% of jack pine trees were reproductive on the Plains. Higher numbers of reproductive trees were present on the Shield (64% of black spruce trees and 91% of jack pine trees; Supplementary Table 2A). Both species increased in probability of cone presence with tree DBH and plot age (Figure 1 and Supplementary Figure 4). However, DBH was a much better predictor of reproductive maturity than plot age for both species and ecozones (Marginal R2 between 0.47 and 0.56 for the DBH models compared with marginal R2< 0.1 for the age models, Supplementary Table 2B). Reproductive maturity showed high plot and site variability for both species and ecozones (Supplementary Tables 2B,C). Both species reached reproductive maturity (probability of cone presence > 0.5) at smaller sizes (DBH) on the Shield than the Plains. On the Plains, black spruce and jack pine reached reproductive maturity at similar sizes (3.5 cm) whereas on the Shield, jack pine reached reproductive maturity at smaller sizes than black spruce (effectively 0.1 vs. 1.4 cm DBH, respectively, Figure 1 and Supplementary Table 2B). Black spruce trees on the Plains reached reproductive maturity 138 years after the last fire whereas for black spruce on the Shield, plot age was not a significant driver of reproductive maturity. In both ecozones, Jack pine trees reached reproductive maturity within the first 20 years after post-fire regeneration, although our models cannot determine the exact age (Supplementary Figure 4 and Supplementary Table 2C). For both species, density of reproductive trees was greater on the Shield. Within ecozones, densities of reproductive black spruce trees were higher than those of jack pine (Supplementary Figures 1C,D, Figures 2, 4 and Supplementary Table 1; Figure 3 and Supplementary Table 4 -Only for old burned plots-).
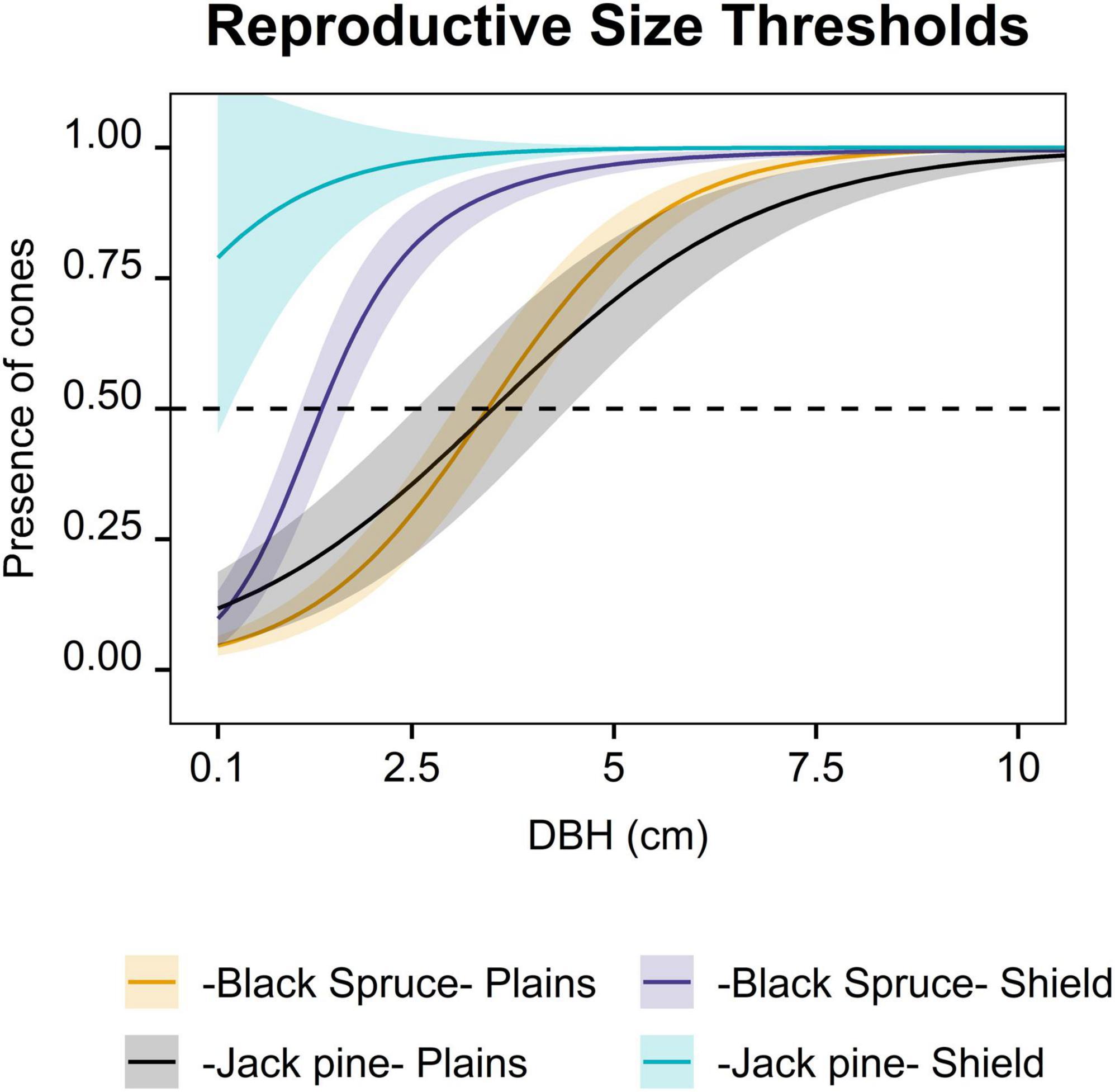
Figure 1. Probability of cone presence as a function of diameter at breast height (DBH; 1.3 m) (reproductive size thresholds) for individual black spruce and jack pine trees on the Taiga Plains (Plains) and the Taiga Shield (Shield) in the Northwest Territories, Canada. Transparent ribbons indicate 95% confidence intervals. The horizontal dashed lines indicate the reproductive size thresholds (probability of cone presence on a tree > 0.5). Full model outputs are in Supplementary Table 2B.
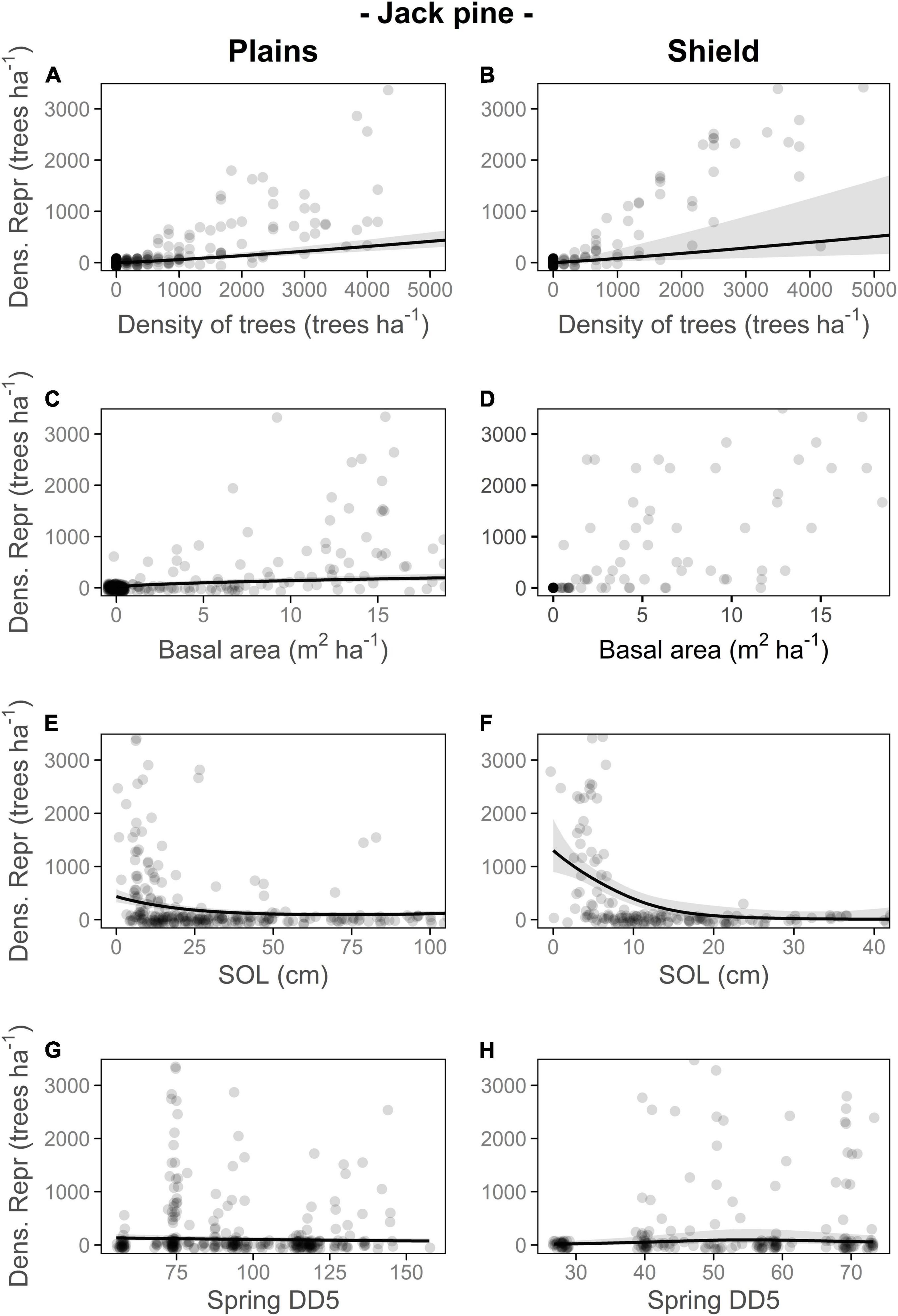
Figure 2. Responses of number of reproductive jack pine trees per 60 m2 plot (converted to density of reproductive trees jack pine trees (Dens. Repr.; trees⋅ha–1) for visual interpretation) to density of jack pine trees (trees trees⋅ha–1; A,B), jack pine basal area (m2⋅ha–1; C,D), soil organic layer thickness (SOL; centimeters; E,F) and spring growing degree days (Spring DD5; G,H) on the Plains and Shield. Different colors for the lines and the circles in panel (B) are used to differentiate between age classes; in all other panels, the plot age category was not significant. For each ecozone, relationships are plotted if included in the top model. For each relationship shown, other terms in the model are held constant at their medians. Transparent ribbons indicate 95% confidence intervals. Full GLMMs outputs are in Supplementary Table 4.
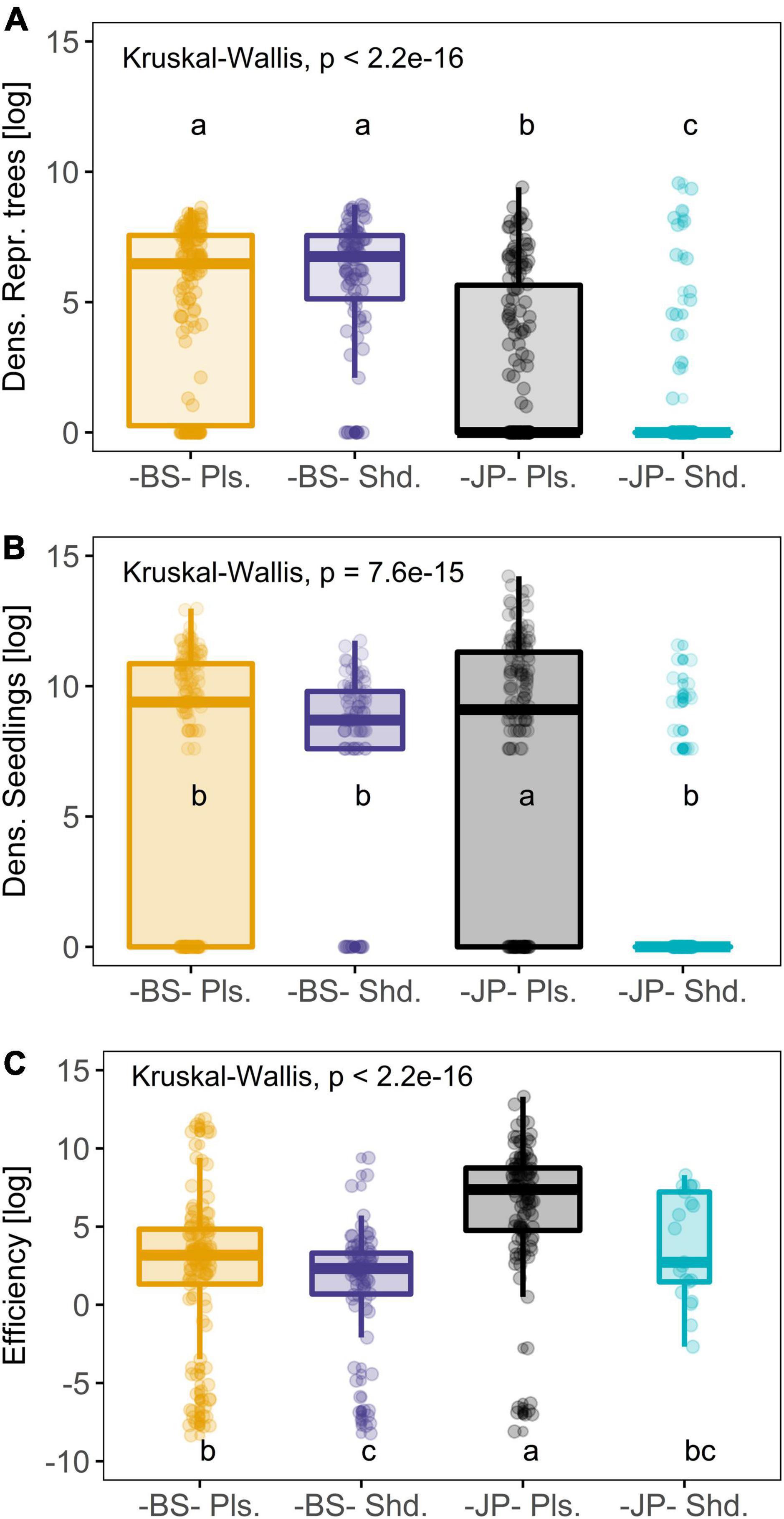
Figure 3. Density of reproductive trees in old burned plots (Dens. Repr. trees; A), density of seedlings (Dens. Seedlings; B) and reproductive efficiency (Efficiency; C) values for black spruce (-BS-) and jack pine (-JP-) trees in each ecozone (Pls.: Plains; Shd.: Shield). Values in insets correspond to Kruskal-Wallis analyses between the combined species-ecozone groups. Small letters indicate significant differences obtained in Kruskal-Wallis analyses. All variables are log-transformed (Supplementary Table 4 for raw mean and standard error values).
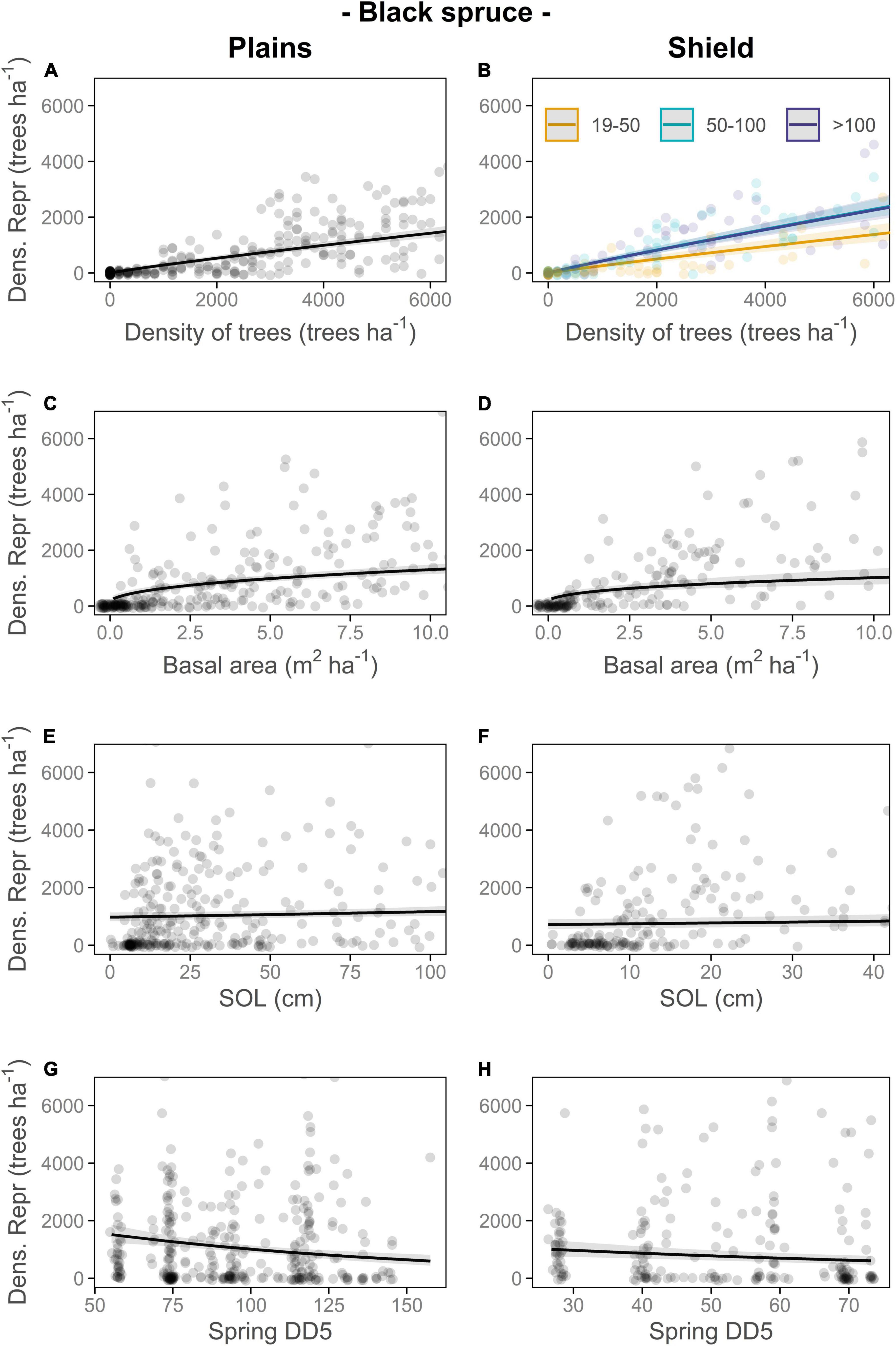
Figure 4. Responses of number of reproductive black spruce trees per 60 m2 plot (converted to density of reproductive trees black spruce trees (Dens. Repr.; trees⋅ha–1) for visual interpretation) to density of black spruce trees (trees trees⋅ha–1; A,B), black spruce basal area (m2⋅ha–1; C,D), soil organic layer thickness (SOL; centimeters; E,F) and spring growing degree days (Spring DD5; G,H) on the Plains and Shield. Different colors for the lines and the circles in panel (B) are used to differentiate between age classes; in all other panels, the plot age category was not statistically significant. For each relationship shown, other terms in the model are held constant at their medians. Transparent ribbons indicate 95% confidence intervals. Full GLMMs outputs are in Supplementary Table 4.
Environmental Constraints for Reproductive Maturity
Metrics of stand productivity were generally positively associated with the number of reproductive trees in a stand, with some differences among species and ecozones (Figures 2, 4 and Supplementary Table 3). The number of reproductive black spruce trees per plot increased with tree density and basal area of black spruce, plot age class, and SOL thickness (Figure 4 and Supplementary Table 3A). The number of reproductive jack pine trees increased with tree density and basal area of jack pine (only on the Plains) and decreased with SOL thickness, particularly on the Shield (Figure 2 and Supplementary Table 3B). The number of reproductive black spruce trees in both ecozones and jack pine trees on the Plains, decreased in locations with mild climate conditions (higher spring DD5, Supplementary Appendix 1, Figures 2G, 4G,H, Supplementary Figure 1 and Supplementary Table 3), whereas for jack pine on the Shield, our results showed an optimum number of spring DD5 (close to 55), that maximized the number of reproductive trees per plot (Figure 2H, Supplementary Figure 1 and Supplementary Table 3). Neither the presence/absence of ground ice nor the type of plot sampled (recent vs. old burns) was selected in the most parsimonious models.
Differences in Reproductive Efficiency Between Species and Ecozones
Kruskal-Wallis tests revealed significant differences in the density of seedlings, reproductive trees and reproductive efficiency among the combined species-ecozone groups in the recently burned plots (Figure 3 and Supplementary Table 4). For both species, reproductive efficiency was significantly higher on the Plains than the Shield. A reproductive black spruce tree can recruit on average 23 times more seedlings on the Plains compared with the Shield. Similarly, a reproductive jack pine tree can recruit on average 24 times more seedlings on the Plains compared with the Shield (Figure 3 and Supplementary Table 4). We also detected that in both ecozones, a reproductive jack pine can recruit on average three times more seedlings than a reproductive black spruce. The highest reproductive efficiency among the species-ecozone groups was seen in jack pine on the Plains.
Discussion
Boreal forests are characterized by even-aged stands because most fires are stand-replacing. Although high synchrony in reproductive maturity is expected in these even-aged stands, trees initiate cone production when they reach a minimum reproductive size threshold instead of an age threshold. The older the trees, the higher probability of reaching reproductive maturity, but size is the determining factor triggering it. In addition, reproductive maturity and reproductive efficiency vary markedly among tree species but are also constrained by environmental variation. As such, even when tree species exhibited presence of cones prior fire, viable seed production necessary for post-fire regeneration success is not guaranteed (Trant et al., 2018). Our results showed that the reproductive efficiency of jack pine was three times greater than that of black spruce, which explained the higher post-fire resilience of jack pine compared with black spruce in this region (Baltzer et al., 2021).
Although reproductive potential, i.e., productivity of cones, seeds and viable seeds, is known to increase as individual trees and forest stands age, the transition to reproductive maturity is often related to tree size rather than age (de Jong and Klinkhamer, 2005; Thomas, 2011; Viglas et al., 2013; Davi et al., 2016; Andrus et al., 2020). In accordance with these studies, our results confirmed that reproductive maturity is reached when trees attain a size that allows them to allocate resources to reproduction. Heterogeneity in tree growth and resources that can be allocated to cone production can explain the variability in the age that corresponds to the reproductive size thresholds (Sala et al., 2012). Plot age, a proxy of time since last fire, is the most common variable use in forest simulation models to predict outcomes of post-fire regeneration under a changing fire regime (Johnstone et al., 2010; Hart et al., 2019; Whitman et al., 2019; Baltzer et al., 2021). According to earlier studies, jack pine reaches reproductive maturity between 5 and 10 years after post-fire establishment, compared with black spruce which reached reproductive maturity 20 years after establishment (McLeod and MacDonald, 1997). We demonstrated that a high percentage of the sampled trees was not reproductive at the time of sampling, despite all our study plots being older than 19 years. Factors affecting tree growth at the levels of individuals or stands can markedly delay reproductive maturity. Thus, predicting regeneration failure based on the time since last fire (Johnstone et al., 2010; Hart et al., 2019; Whitman et al., 2019; Baltzer et al., 2021), can be misleading. Incorporation of direct or indirect measures of tree size could improve predictions of post-fire successional dynamics and lead to better estimation of the probabilities of changes in successional trajectories or losses of resilience in these boreal forests.
Jack pine reached reproductive maturity at smaller sizes than black spruce, which corresponds with findings from other parts of the boreal forest (McLeod and MacDonald, 1997; Greene et al., 2004). Our results showed that jack pine and black spruce reached reproductive maturity at smaller sizes on the Shield compared with the Plains ecozone. Low genetic differentiation in both species has been reported between ecozones due to range expansion after the last deglaciation (McLeod and MacDonald, 1997; MacDonald, 2000; Jaramillo-Correa et al., 2004; Godbout et al., 2005; Brandt, 2009). This suggests that differences in environmental conditions are likely driving observed variation in reproductive maturity. Smaller reproductive size thresholds on the Shield may be triggered by several confounding factors that are difficult to disentangle but are associated with more stressful environmental conditions such as shorter growing seasons, colder temperatures, and shallower soils (Climent et al., 2008; Santos-del-Blanco et al., 2013; Alfaro-Sánchez et al., 2015), that slow down the growth of the trees. The lower tree densities reported on the Shield could also contribute to the precocious presence of cones, as trees experiencing less competition can reach an optimal size for reproduction earlier (Roff, 1993; Andrus et al., 2020).
A higher density of reproductive trees increases the likelihood of self-replacement of stands after fire because of the concomitant increase in the pollen production resulting in more viable seeds to support post-fire regeneration (Smith et al., 1990). Our results showed that the number of reproductive trees increases proportionally with plot age, stem density, and basal area and varied with other environmental variables such as climatic conditions and SOL thickness. Numerous studies have reported a positive relationship between cone production and basal area, i.e., large and older trees tend to be the most productive ones (Greene and Johnson, 1994; Viglas et al., 2013; Alfaro-Sánchez et al., 2015; Briand et al., 2015; Davi et al., 2016). This is probably the reason why stands of these two species with higher basal area have shown enhanced resilience to disturbances, i.e., lower probability of compositional shifts following fire (Walker et al., 2017; Baltzer et al., 2021). In contrast, short fire return intervals could limit cone (and seed) production due to the lack of large reproductive trees. This can expose the stands to immaturity risk as seed production may still be below levels required for self replacement (Black and Bliss, 1980b; Enright et al., 1998, 2014; Greene and Johnson, 1999; Johnstone et al., 2009b; Splawinski et al., 2019a).
In the boreal biome, one of the most limiting factors for sexual reproduction and seed development is the number of growing degree-days (Sirois, 2000; Roland et al., 2014; Brown et al., 2019), usually calculated as number of accumulated degrees Celsius above 5°C (DD5). Consequently, we were surprised to find that mild climatic conditions (longer growing seasons) were associated with a reduction in reproductive black spruce trees in both ecozones and reproductive jack pines on the Plains. Plants growing under better growing conditions tend to take longer to produce cones (Roff, 1993). This could explain the lower allocation to reproduction in sites with mild climatic conditions compared with harsh climatic conditions. However, other confounding effects such as species dominance and post-glacial patterns of colonization make it difficult to identify a single factor explaining differences in reproductive maturity across the climatic gradient. Greater dominance of black spruce and jack pine trees toward northern latitudes will facilitate female cone fertilization (Smith et al., 1990; Kelly et al., 2001), which is reflected in the greater number of reproductive trees at these cold high latitudes. It might also be a compensatory mechanism to overcome the lower seed viability present at higher latitudes, although more research is needed in this topic (but see Trant et al., 2018). Greater allocation to reproduction is expected in colonizing or expanding populations (Obeso, 2002). Black spruce and jack pine spread relatively recently in the study area after the retreat of the ice sheet 13,000 BP (Brandt, 2009). Therefore, we cannot rule out that both species, but particularly jack pine, are still undergoing a postglacial colonization process. However, limiting factors of the current range of jack pine, which reaches its northwestern limit in the NWT, are DD5 and dominance of peat soils (Supplementary Appendix 1, McLeod and MacDonald, 1997). For jack pine on the Shield, our models showed an optimal number of spring DD5 (∼55) that maximized the number of reproductive trees per plot. This hump-shaped relationship between spring DD5 and reproductive maturity corroborates that harsh climatic conditions (growing seasons with less than ∼55 spring DD5) limit the range expansion of the species toward the treeline (Lantz et al., 2019). Our results also showed that those sites located in the northern part of the Shield, characterized by very short growing seasons, have not registered any significant increases in spring DD5 or temperature in the last 15 years (Supplementary Appendix 1 and Supplementary Figures 1F, 2B,D). Therefore, we do not expect an expansion of the range of jack pine at the most northerly sites of the study area, located near the treeline on the Shield, in the foreseeable future. Our models also showed a divergent response to SOL thickness between black spruce and jack pine in the number of reproductive trees at the plot level. Black spruce is well-adapted to the cool, nutrient-poor, organic soils characteristic of the boreal biome (Ritchie, 1987); however, thick SOLs or limited access to the mineral layer may reduce their productivity (Van Bogaert et al., 2015). The GLMMs showed a modest negative effect of reduction in SOL thickness on the number of reproductive black spruce trees, which corroborates the observed lack of resilience of black spruce stands in response to reduction in SOL thickness (Johnstone et al., 2010; Walker et al., 2017). In contrast, jack pine responded positively to thin organic soils, as has been shown in previous studies. As such, the GLMMs reported greater number of reproductive jack pine trees in sites with relatively shallower SOLs, a pattern that has also been detected for jack pine germination (Chrosciewicz, 1974; Hart et al., 2019; Baltzer et al., 2021). Our findings also revealed that the presence of ground ice was not a limiting driver for reproductive maturity in either species on the Plains or in black spruce on the Shield.
Seed production, seed viability, and seedbed conditions are also critical for tree establishment (Black and Bliss, 1980a). As a result, similar densities of reproductive trees can lead to different seedling establishment densities following fire depending on site conditions. For example, reproductive efficiency of black spruce was 23 times greater on the Plains than on the Shield, i.e., a reproductive black spruce tree can recruit 23 times more seedlings on the Plains than on the Shield. These results demonstrate the importance of site suitability and geomorphological history on species’ reproductive traits, and suggests that advancing our understanding of the environmental constraints on seed viability and seedling establishment is essential. In line with previous studies, our results showed that jack pine was more efficient in post-fire recruitment processes, when compared with black spruce (Baltzer et al., 2021). This is because of the greater number of reproductive trees present in jack pine stands compared with black spruce ones (Greene et al., 2004). Furthermore, jack pine cones are usually described as completely serotinous cones, i.e., they are retained in the tree crown and remain closed until fire. Although the level of serotiny in this species vary with factors such as fire severity, fire regime and time since last fire (Gauthier et al., 1996). In contrast, the semi-serotinous cones of black spruce open gradually over time even in the absence of fire. Thus, post-fire seed rain may be greater in jack pine, increasing its reproductive efficiency. In addition, black spruce seed viability seems to be more affected by fire than that of jack pine. Cones from serotinous species play an important role in insulating seeds against heat of the flaming front (Lamont et al., 2020 and references therein). Black spruce has the smallest cones among serotinous conifers, and their seeds are three times smaller than those of jack pine. As such, black spruce cones and seeds will be more affected during the fire passage than those of jack pine and more sensitive to increases in fire intensity (Splawinski et al., 2019b).
Conclusion
Our study contributes to a broader understanding of the drivers of reproductive maturity for black spruce and jack pine in the fire-prone boreal biome and its relationship with the efficiency of seedling recruitment. As demonstrated in this study, a higher density of reproductive trees does not always translate into greater post-fire regeneration success per unit area. Differences in the reproductive efficiency of species, life-history characteristics, disturbance severity (how much of the pre-fire cone bank is consumed), geomorphological, and environmental variability also play a role in shaping the post-fire resilience of boreal tree species. For instance, the reproductive efficiency of jack pine is three times greater than that of black spruce, and ecozones with harsh climatic and environmental conditions showed lower post-fire seedling recruitment, despite trees attained an optimal size for reproduction earlier. These mechanisms can explain the frequent loss of resilience and regeneration failure in black spruce, compared with the high resilience and widespread regeneration success of jack pine reported in recent decades across North America (Baltzer et al., 2021). Predicted changes in climate and fire activity in the boreal region, but also in other fire-prone habitats, can jeopardized the resilience of non-serotinous, or semi-serotinous species, in favor of more reproductively efficient and resilient species such as serotinous species.
Data Availability Statement
Part of the forest compositional attributes is currently available from https://doi.org/10.3334/ORNLDAAC/156 (Walker et al., 2018c), and the remainder is available from https://doi.org/10.5061/dryad.76hdr7sth (Baltzer et al., 2020). Reproductive attributes are available from https://doi.org/10.5061/dryad.00000005x (Alfaro-Sánchez et al., 2022).
Author Contributions
ND, XW, JB, and JJ collected the data. JB, JJ, SC, and RA-S conceived the study. RA-S performed the data analysis and drafted the manuscript. All authors contributed to the manuscript edits.
Funding
This article is part of Project 170 of the Government of the Northwest Territories (GNWT) Department of Environment and Natural Resources Cumulative Impacts Monitoring Program (awarded to JB, JJ, and SC). Additional funding was provided by Natural Science and Engineering Research Council (NSERC: Changing Cold Regions Network), Northern Scientific Training Program, NSERC Discovery to JJ, NSERC Discovery Accelerator Funding to JB, CFREF Global Water Futures funding for Northern Water Futures to JB, a National Science Foundation DEB RAPID grant (grant #1542150), and NASA Arctic Boreal and Vulnerability Experiment (ABoVE) Legacy Carbon grant (grant # NNX15AT71A) to MM. ND was supported by an NSERC Postdoctoral Fellowship and Rutherford Postdoctoral Fellowship from the Royal Society of New Zealand. In kind support was provided by the Bonanza Creek LTER program. Canadian field assistants were supported by the Northern Science Training Program. The Polar Continental Shelf Program provided helicopter support. Dedicated research time for JB was supported by the Canada Research Chairs program.
Conflict of Interest
The authors declare that the research was conducted in the absence of any commercial or financial relationships that could be construed as a potential conflict of interest.
Publisher’s Note
All claims expressed in this article are solely those of the authors and do not necessarily represent those of their affiliated organizations, or those of the publisher, the editors and the reviewers. Any product that may be evaluated in this article, or claim that may be made by its manufacturer, is not guaranteed or endorsed by the publisher.
Acknowledgments
We thank numerous students and technicians for field and lab assistance. We thank the GNWT Aurora Research Institute (Research License 15879), the Ka’a’gee Tu First Nation, the Tłįchǫ Government, and the Wek’éezhìi Renewable Resources Board for their support of this research. The Wilfrid Laurier University—GNWT Partnership Agreement was instrumental in providing logistical support and laboratory space. Thanks to David Greene who provided helpful comments on earlier manuscript versions.
Supplementary Material
The Supplementary Material for this article can be found online at: https://www.frontiersin.org/articles/10.3389/fevo.2022.869130/full#supplementary-material
References
Alfaro Sánchez, R., Johnstone, J. F., Cumming, S. G., Day, N. J., Mack, M. C., Walker, X. J., et al. (2022). Reproductive Maturity in Boreal Trees, Northwest Territories, Canada. Dryad [Dataset]. doi: 10.5061/dryad.00000005x
Alfaro-Sánchez, R., Camarero, J. J., López-Serrano, F. R., Sánchez-Salguero, R., Moya, D., and Heras, J. D. L. (2015). Positive coupling between growth and reproduction in young post-fire Aleppo pines depends on climate and site conditions. Int. J. Wildland Fire 24:507. doi: 10.1071/WF14045
Andrus, R. A., Harvey, B. J., Hoffman, A., and Veblen, T. T. (2020). Reproductive maturity and cone abundance vary with tree size and stand basal area for two widely distributed conifers. Ecosphere 11:e03092. doi: 10.1002/ecs2.3092
Arseneault, D. (2001). Impact of fire behavior on postfire forest development in a homogeneous boreal landscape. Can. J. For. Res. 31, 1367–1374. doi: 10.1139/x01-065
Baltzer, J. L., Day, N. J., Walker, X. J., Greene, D., Mack, M. C., Alexander, H. D., et al. (2021). Increasing fire and the decline of fire adapted black spruce in the boreal forest. Proc. Natl. Acad. Sci. 118:e2024872118. doi: 10.1073/pnas.2024872118
Baltzer, J. L., Day, N. J., White, A. L., Reid, K., Degré-Timmons, G. É, Cumming, S. G., et al. (2020). Vascular Plant Community Data for Northwest Territories, Canada. Chapel Hill, NC: Dryad. doi: 10.5061/dryad.76hdr7sth
Barton, K. (2022). MuMIn: Multi-Model Inference. R Package Version 1.46.0. Available online at: https://CRAN.R-project.org/package=MuMIn
Black, R. A., and Bliss, L. C. (1980a). Reproductive ecology of Picea mariana (Mill.) BSP., at tree line near Inuvik, Northwest Territories, Canada. Ecol. Monogr. 50, 331–354.
Black, R. A., and Bliss, L. C. (1980b). Reproductive ecology of Picea mariana (Mill.) BSP., at tree line near Inuvik, Northwest Territories, Canada. Ecol. Monogr. 50, 331–354.
Brandt, J. P. (2009). The extent of the North American boreal zone. Environ. Rev. 17, 101–161. doi: 10.1139/A09-004
Briand, C. H., Schwilk, D. W., Gauthier, S., and Bergeron, Y. (2015). Does fire regime influence life history traits of jack pine in the southern boreal forest of Québec, Canada? Plant Ecol. 216, 157–164. doi: 10.1007/s11258-014-0424-x
Brooks, M. E., Kristensen, K., van Benthem, K. J., Magnusson, A., Berg, C. W., Nielsen, A., et al. (2017). glmmTMB balances speed and flexibility among packages for zero-inflated generalized linear mixed modeling. R J. 9, 378–400.
Brown, C. D., Dufour-Tremblay, G., Jameson, R. G., Mamet, S. D., Trant, A. J., Walker, X. J., et al. (2019). Reproduction as a bottleneck to treeline advance across the circumarctic forest tundra ecotone. Ecography 42, 137–147. doi: 10.1111/ecog.03733
Buma, B., Brown, C. D., Donato, D. C., Fontaine, J. B., and Johnstone, J. F. (2013). The impacts of changing disturbance regimes on serotinous plant populations and communities. BioScience 63, 866–876. doi: 10.1525/bio.2013.63.11.5
Chrosciewicz, Z. (1974). Evaluation of fire-produced seedbeds for jack pine regeneration in central Ontario. Can. J. For. Res. 4, 455–457. doi: 10.1139/x74-067
Climent, J., Prada, M. A., Calama, R., Chambel, M. R., de Ron, D. S., and Alía, R. (2008). To grow or to seed: ecotypic variation in reproductive allocation and cone production by young female Aleppo pine (Pinus halepensis, Pinaceae). Am. J. Bot. 95, 833–842. doi: 10.3732/ajb.2007354
Coops, N. C., Hermosilla, T., Wulder, M. A., White, J. C., and Bolton, D. K. (2018). A thirty year, fine-scale, characterization of area burned in Canadian forests shows evidence of regionally increasing trends in the last decade. PLoS One 13:e0197218. doi: 10.1371/journal.pone.0197218
Davi, H., Cailleret, M., Restoux, G., Amm, A., Pichot, C., and Fady, B. (2016). Disentangling the factors driving tree reproduction. Ecosphere 7:e01389. doi: 10.1002/ecs2.1389.
Day, N. J., White, A. L., Johnstone, J. F., Degré-Timmons, G. É, Cumming, S. G., Mack, M. C., et al. (2020). Fire characteristics and environmental conditions shape plant communities via regeneration strategy. Ecography 43, 1464–1474. doi: 10.1111/ecog.05211
de Jong, T., and Klinkhamer, P. (2005). Evolutionary Ecology of Plant Reproductive Strategies. Cambridge, MA: Cambridge University Press.
Ecosystem Classification Group (2008). Ecological Regions of the Northwest Territories -Taiga Shield. Department of Environment and Natural Resources. Yellowknife, NT: Government of the Northwest Territories, viii+146.
Ecosystem Classification Group 2007 (2009). Ecological Regions of the Northwest Territories - Taiga Plains. Department of Environment and Natural Resources. Yellowknife, NT: Government of the Northwest Territories, viii+173.
Elliott, D. L. (1979). The current regenerative capacity of the northern canadian trees, Keewatin, N.W.T., Canada: some preliminary observations. Arct. Alp. Res. 11, 243–251. doi: 10.2307/1550648
Enright, N. J., Fontaine, J. B., Lamont, B. B., Miller, B. P., and Westcott, V. C. (2014). Resistance and resilience to changing climate and fire regime depend on plant functional traits. J. Ecol. 102, 1572–1581. doi: 10.1111/1365-2745.12306
Enright, N. J., Marsula, R., Lamont, B. B., and Wissel, C. (1998). The ecological significance of canopy seed storage in fire-prone environments: a model for non-sprouting shrubs. J. Ecol. 86, 946–959. doi: 10.1046/j.1365-2745.1998.00312.x
Gauthier, S., Bergeron, Y., and Simon, J.-P. (1996). Effects of fire regime on the serotiny level of jack pine. J. Ecol. 84:539. doi: 10.2307/2261476
Godbout, J., Jaramillo-Correa, J. P., Beaulieu, J., and Bousquet, J. (2005). A mitochondrial DNA minisatellite reveals the postglacial history of jack pine (Pinus banksiana), a broad-range North American conifer. Mol. Ecol. 14, 3497–3512. doi: 10.1111/j.1365-294X.2005.02674.x
Greene, D. F., and Johnson, E. A. (1994). Estimating the mean annual seed production of trees. Ecology 75, 642–647. doi: 10.2307/1941722
Greene, D. F., and Johnson, E. A. (1999). Modelling recruitment of Populus tremuloides, Pinus banksiana, and Picea mariana following fire in the mixedwood boreal forest. Can. J. For. Res. 29, 462–473.
Greene, D. F., Noël, J., Bergeron, Y., Rousseau, M., and Gauthier, S. (2004). Recruitment of Picea mariana, Pinus banksiana, and Populus tremuloides across a burn severity gradient following wildfire in the southern boreal forest of Quebec. Can. J. For. Res. 34, 1845–1857. doi: 10.1139/x04-059
Harsch, M. A., Hulme, P. E., McGlone, M. S., and Duncan, R. P. (2009). Are treelines advancing? A global meta-analysis of treeline response to climate warming. Ecol. Lett. 12, 1040–1049. doi: 10.1111/j.1461-0248.2009.01355.x
Hart, S. J., Henkelman, J., McLoughlin, P. D., Nielsen, S. E., Truchon, A., and Johnstone, J. F. (2019). Examining forest resilience to changing fire frequency in a fire-prone region of boreal forest. Glob. Change Biol. 25, 869–884. doi: 10.1111/gcb.14550
Ilisson, T., and Chen, H. Y. H. (2009). The direct regeneration hypothesis in northern forests. J. Veg. Sci. 20, 735–744. doi: 10.1111/j.1654-1103.2009.01066.x
Jaramillo-Correa, J. P., Beaulieu, J., and Bousquet, J. (2004). Variation in mitochondrial DNA reveals multiple distant glacial refugia in black spruce (Picea mariana), a transcontinental North American conifer. Mol. Ecol. 13, 2735–2747. doi: 10.1111/j.1365-294X.2004.02258.x
Johnstone, J. F., and Chapin, F. S. (2006). Effects of soil burn severity on post-fire tree recruitment in boreal forest. Ecosystems 9, 14–31. doi: 10.1007/s10021-004-0042-x
Johnstone, J. F., Boby, L., Tissier, E., Mack, M., Verbyla, D., and Walker, X. (2009a). Postfire seed rain of black spruce, a semiserotinous conifer, in forests of interior Alaska. Can. J. For. Res. 39, 1575–1588.
Johnstone, J. F., Boby, L., Tissier, E., Mack, M., Verbyla, D., and Walker, X. (2009b). Postfire seed rain of black spruce, a semiserotinous conifer, in forests of interior Alaska. Can. J. For. Res. 39, 1575–1588.
Johnstone, J. F., Celis, G., Chapin, F. S., Hollingsworth, T. N., Jean, M., and Mack, M. C. (2020). Factors shaping alternate successional trajectories in burned black spruce forests of Alaska. Ecosphere 11:e03129. doi: 10.1002/ecs2.3129
Johnstone, J. F., Hollingsworth, T. N., and Chapin, F. S. (2008). A key for Predicting Postfire Successional Trajectories in Black Spruce Stands of Interior Alaska. Portland, OR: Department of Agriculture, Forest Service, Pacific Northwest Research Station. doi: 10.2737/PNW-GTR-767
Johnstone, J. F., Hollingsworth, T. N., Chapin, F. S., and Mack, M. C. (2010). Changes in fire regime break the legacy lock on successional trajectories in Alaskan boreal forest. Glob. Change Biol. 16, 1281–1295. doi: 10.1111/j.1365-2486.2009.02051.x
Johnstone, J. F., Iii, F. S. C., Foote, J., Kemmett, S., Price, K., and Viereck, L. (2004). Decadal observations of tree regeneration following fire in boreal forests. Can. J. For. Res. 34:8.
Kasischke, E. S., and Turetsky, M. R. (2006). Recent changes in the fire regime across the North American boreal region—Spatial and temporal patterns of burning across Canada and Alaska. Geophys. Res. Lett. 33:L09703. doi: 10.1029/2006GL025677
Kelly, D., Hart, D. E., and Allen, R. B. (2001). Evaluating the wind pollination benefits of mast seeding. Ecology 82, 117–126. doi: 10.1890/0012-96582001082[0117:ETWPBO]2.0.CO;2
Lamont, B. B., Pausas, J. G., He, T., Witkowski, E. T. F., and Hanley, M. E. (2020). Fire as a selective agent for both serotiny and nonserotiny over space and time. Crit. Rev. Plant Sci. 39, 140–172. doi: 10.1080/07352689.2020.1768465
Lantz, T. C., Moffat, N. D., Fraser, R. H., and Walker, X. (2019). Reproductive limitation mediates the response of white spruce (Picea glauca) to climate warming across the forest–tundra ecotone. Arct. Sci. 5, 167–184. doi: 10.1139/as-2018-0012
Lavoie, L., and Sirois, L. (1998). Vegetation changes caused by recent fires in the northern boreal forest of eastern Canada. J. Veg. Sci. 9:483. doi: 10.2307/3237263
Lüdecke, D. (2021). sjPlot: Data Visualization for Statistics in Social Science. R Package Version 2.8.10. Available online at: https://CRAN.R-project.org/package=sjPlot
Lynch, J. A., Clark, J. S., Bigelow, N. H., Edwards, M. E., and Finney, B. P. (2002). Geographic and temporal variations in fire history in boreal ecosystems of Alaska. J. Geophys. Res. 108:8152. doi: 10.1029/2001JD000332
MacDonald, G. M. (2000). “Postglacial vegetation and climate,” in The Physical Environment of the Mackenzie Valley, Northwest Territories: a Base Line for the Assessment of Environmental Change, eds L. D. Dyke and G. R. Brooks (Ottawa, ON: Geological Survey of Canada Bulletin), 57–63.
IPCC (2018). “Global warming of 1.5°C,” in An IPCC Special Report on the Impacts of Global Warming of 1.5°C Above Pre-industrial Levels and Related Global Greenhouse gas Emission Pathways, in the Context of Strengthening the Global Response to the Threat of Climate Change, Sustainable Development, and Efforts to Eradicate Poverty, eds V. Masson-Delmotte, P. Zhai, H.-O. Pörtner, D. Roberts, J. Skea, P. R. Shukla, et al. (Geneva: Intergovernmental Panel on Climate Change).
McLeod, T., and MacDonald, G. M. (1997). Postglacial range expansion and population growth of Picea mariana, Picea glauca and Pinus banksiana in the western interior of Canada. J. Biogeogr. 24, 865–881. doi: 10.1046/j.1365-2699.1997.00151.x
Obeso, J. R. (2002). The costs of reproduction in plants. New Phytol. 155, 321–348. doi: 10.1046/j.1469-8137.2002.00477.x
Payette, S. (1983). The forest tundra and present tree lines of the northern Québec-Labrador Peninsula. Nordicana 47, 3–23.
R Core Team (2019). R: A Language and Environment for Statistical Computing. Vienna: R Foundation for Statistical Computing.
Roland, C. A., Schmidt, J. H., and Johnstone, J. F. (2014). Climate sensitivity of reproduction in a mast-seeding boreal conifer across its distributional range from lowland to treeline forests. Oecologia 174, 665–677. doi: 10.1007/s00442-013-2821-6
Sala, A., Woodruff, D. R., and Meinzer, F. C. (2012). Carbon dynamics in trees: feast or famine? Tree Physiol. 32, 764–775. doi: 10.1093/treephys/tpr143
Santos-del-Blanco, L., Bonser, S. P., Valladares, F., Chambel, M. R., and Climent, J. (2013). Plasticity in reproduction and growth among 52 range-wide populations of a mediterranean conifer: adaptive responses to environmental stress. J. Evol. Biol. 26, 1912–1924. doi: 10.1111/jeb.12187
Sirois, L. (1992). “The transition between boreal forest and tundra,” in A Systems Analysis of the Global Boreal Forest, eds H. H. Shugart, R. Leemans, and G. B. E. Bonan (Cambridge: Cambridge University Press), 196–215. doi: 10.1017/CBO9780511565489.009
Sirois, L. (2000). Spatiotemporal variation in black spruce cone and seed crops along a boreal forest – tree line transect. Can. J. For. Res. 30:10.
Smith, C. C., Hamrick, J. L., and Kramer, C. L. (1990). The advantage of mast years for wind pollination. Am. Nat. 136, 154–166. doi: 10.1016/0169-5347(94)90310-7
Splawinski, T. B., Greene, D. F., Michaletz, S. T., Gauthier, S., Houle, D., and Bergeron, Y. (2019a). Position of cones within cone clusters determines seed survival in black spruce during wildfire. Can. J. For. Res. 49, 121–127. doi: 10.1139/cjfr-2018-0209
Splawinski, T. B., Greene, D. F., Michaletz, S. T., Gauthier, S., Houle, D., and Bergeron, Y. (2019b). Position of cones within cone clusters determines seed survival in black spruce during wildfire. Can. J. For. Res. 49:8.
Stearns, S. C. (2000). Life history evolution: successes, limitations, and prospects. Naturwissenschaften 87, 476–486. doi: 10.1007/s001140050763
Thomas, S. C. (2011). “Age-related changes in tree growth and functional biology: the role of reproduction,” in Size- and Age-Related Changes in Tree Structure and Function Tree Physiology, eds F. C. Meinzer, B. Lachenbruch, and T. E. Dawson (Dordrecht: Springer), 33–64. doi: 10.1007/978-94-007-1242-3_2
Trant, A. J., Jameson, R. G., and Hermanutz, L. (2018). Variation in reproductive potential across a multi-species treeline. Arct. Antarct. Alp. Res. 50:e1524191. doi: 10.1080/15230430.2018.1524191
Van Bogaert, R., Gauthier, S., Drobyshev, I., Jayen, K., Greene, D. F., and Bergeron, Y. (2015). Prolonged absence of disturbance associated with increased environmental stress may lead to reduced seedbank size in Picea mariana in boreal eastern North America. Ecosystems 18, 1135–1150.
Viereck, L. A., and Johnston, W. F. (1990). Silvics of North America Vol. 1, Conifers. Washington, DC: United States Department of Agriculture, 654,675.
Viglas, J. N., Brown, C. D., and Johnstone, J. F. (2013). Age and size effects on seed productivity of northern black spruce. Can. J. For. Res. 43, 534–543. doi: 10.1139/cjfr-2013-0022
Walker, X. J., Rogers, B. M., Baltzer, J. L., Cumming, S. G., Day, N. J., Goetz, S. J., et al. (2018b). Cross-scale controls on carbon emissions from boreal forest megafires. Glob. Change Biol. 24, 4251–4265. doi: 10.1111/gcb.14287
Walker, X. J., Baltzer, J. L., Cumming, S. G., Day, N. J., Johnstone, J. F., Rogers, B. M., et al. (2018a). Soil organic layer combustion in boreal black spruce and jack pine stands of the Northwest Territories, Canada. Int. J. Wildland Fire 27:125. doi: 10.1071/WF17095
Walker, X. J., Rogers, B. M., Baltzer, J. L., Cummings, S. R., Day, N. J., Goetz, S. J., et al. (2018c). ABoVE: Wildfire Carbon Emissions and Burned Plot Characteristics, NWT, CA, 2014-2016. Oak Ridge, TN: ORNL DAAC.
Walker, X. J., Mack, M. C., and Johnstone, J. F. (2017). Predicting ecosystem resilience to fire from tree ring analysis in black spruce forests. Ecosystems 20, 1137–1150. doi: 10.1007/s10021-016-0097-5
Wang, T., Hamann, A., Spittlehouse, D., and Carroll, C. (2016). Locally downscaled and spatially customizable climate data for historical and future periods for north America. PLoS One 11:e0156720. doi: 10.1371/journal.pone.0156720
Whitman, E., Parisien, M.-A., Thompson, D. K., and Flannigan, M. D. (2019). Short-interval wildfire and drought overwhelm boreal forest resilience. Sci. Rep. 9:18796. doi: 10.1038/s41598-019-55036-7
Zasada, J. C., Viereck, L. A., and Foote, M. J. (1979). “Quantity and quality of dispersed seed,” in Ecological effects of the Wickersham Dome fire near Fairbanks, Alaska, eds L. Viereck and C. T. Dyrness (Portland: USDA For. Servo Gen. Tech. Rep. PNW-90), 45–49.
Keywords: post-fire regeneration, serotinous species, boreal biome, reproductive size threshold, reproductive age threshold, species-compositional shifts, cone presence
Citation: Alfaro-Sánchez R, Johnstone JF, Cumming SG, Day NJ, Mack MC, Walker XJ and Baltzer JL (2022) What Drives Reproductive Maturity and Efficiency in Serotinous Boreal Conifers? Front. Ecol. Evol. 10:869130. doi: 10.3389/fevo.2022.869130
Received: 03 February 2022; Accepted: 23 June 2022;
Published: 22 July 2022.
Edited by:
Teresa Valor, Universitat de Lleida, SpainReviewed by:
Chris Briand, Salisbury University, United StatesByron Lamont, Curtin University, Australia
Copyright © 2022 Alfaro-Sánchez, Johnstone, Cumming, Day, Mack, Walker and Baltzer. This is an open-access article distributed under the terms of the Creative Commons Attribution License (CC BY). The use, distribution or reproduction in other forums is permitted, provided the original author(s) and the copyright owner(s) are credited and that the original publication in this journal is cited, in accordance with accepted academic practice. No use, distribution or reproduction is permitted which does not comply with these terms.
*Correspondence: Raquel Alfaro-Sánchez, ci5hbGZhcm9zYW5jaGV6QGdtYWlsLmNvbQ==