- Department of Biology, University of Colorado, Colorado Springs, Colorado Springs, CO, United States
Organisms compete for food in many ways, but it is often difficult to know why they use certain competition strategies over others. Bats compete for food either through aggression coupled with food-claiming signals or by actively interfering with a competitor’s sensory processing during prey pursuit (i.e., jamming). It is not known why these different behaviors are exhibited. I studied food competition between Mexican free-tailed bats (Tadarida brasiliensis) at foraging sites in Arizona and New Mexico using passive acoustic recording, insect sampling and 3-D infrared videography with or without supplemental lighting that concentrated prey. Bat activity was quantified by the number of recorded echolocation calls, while feeding behavior was indicated by feeding buzzes. Two competitive behaviors were observed—song, which was produced by bats chasing conspecifics, and sinFM calls, which jam echolocation of competitors pursuing prey. Song production was most common when few bats were present and feeding at low rates. In contrast, jamming signals were most common with many bats present and feeding at high rates. Supplemental lighting increased the numbers of bats, feeding buzzes and sinFM calls, but not song. These results indicate that bats employ different strategies—singing and chasing competitors at low bat densities but jamming competitors at high bat densities. Food claiming signals (song) may only be effective with few competitors present, whereas jamming can be effective with many bats at a foraging site. Multiple competition strategies appear to have evolved in bats that are used under different densities of competitors.
Introduction
Food competition is a fundamental ecological interaction. Individuals compete indirectly by consuming a limited resource and depleting its availability for others (Petren and Case, 1996) or they can directly prevent competitors from accessing a resource through interference competition (Amarasekare, 2002). Direct agonistic encounters between individuals can have costs that increase with the frequency of interactions. For example, frequent territorial encounters between lizards can lead to suppressed immune function (Svensson et al., 2001). Therefore, it may be predicted that the frequency of competitive behaviors changes depending on the density of competitors. However, little research has been conducted to test this hypothesis.
Bats (order Chiroptera) provide an interesting test case for understanding mechanisms of food competition. Bat flight abilities allow them to exploit a wide range of food resources (Voigt et al., 2017). Echolocation provides bats the ability to detect food items in complete darkness, but it operates over a limited range of several meters for finding most food items (Schnitzler et al., 2003). Bats emitting echolocation calls also alert nearby competitors to their presence. Insectivorous bats increase their call repetition rate to produce a feeding buzz in the final moments before attacking insect prey. Other bats eavesdrop on these feeding buzzes to find ephemeral food patches, thus setting the stage for potential interference competition between bats hunting in the same food patch (Gillam, 2007; Dechmann et al., 2009).
In addition to echolocation calls, bats emit social calls to communicate to other bats (Bohn and Gillam, 2018). Social call repertoires are varied and serve many functions, including as signals used in food competition. For example, two closely related species of Pipistrellus bats in Britain each have their own distinct social calls (Barlow and Jones, 1997b). These calls are produced more often at lower insect densities and playbacks of social calls cause a reduction of bat activity for conspecifics on foraging grounds (Barlow and Jones, 1997a). Male big brown bats (Eptesicus fuscus) also produce social calls during food competitions (Wright et al., 2014). These calls have individual-specific acoustic features, and they cause competitors to fly away from available prey in food competitions staged in a laboratory. Big brown bats that made more social calls were more successful capturing prey, indicating that they were being used by the bats for defending or claiming food items (Wright et al., 2014).
Here, I study food competition at natural foraging sites in Arizona and New Mexico, United States by Mexican free-tailed bats (Tadarida brasiliensis), which live in colonies that can exceed one million individuals (Betke et al., 2008). Bats of this species disperse from colonies across the landscape to search for ephemeral patches of insects while traveling distances of over 100 km (Best and Geluso, 2003). Mexican free-tailed bats eavesdrop on feeding buzzes of conspecifics to find food (Gillam, 2007). They also produce sinusoidal Frequency Modulated (sinFM) signals to jam the echolocation of conspecifics (Corcoran and Conner, 2014). These sinFM calls are produced at the same time and frequency as conspecific feeding buzzes and they cause other bats to miss prey items. In addition to sinFM calls, Mexican free-tailed bats have a large repertoire of social calls (Bohn et al., 2008), including song, which are used by male bats in roosts to attract mates and fend off male competitors (Bohn et al., 2009).
The objective of this study was to determine what food defense mechanisms Mexican free-tailed bats use under different conditions at foraging patches in the field. Initial observations indicated that these bats use both song and sinFM calls at food patches. My main hypothesis was that Mexican free-tailed bats use song as a food claiming defense at low bat densities but switch to jamming conspecifics when more competitors are present because it would not be possible to defend food patch from many competitors. I tested this hypothesis by analyzing continuous acoustic recordings made at two foraging sites for a total of nine nights. I used number of echolocation calls as an index of total bat activity, feeding buzzes as indicators of foraging activity and numbers of songs and sinFM calls as indicators of different competitive behaviors. I also recorded interactions at foraging sites using four calibrated infrared cameras to reconstruct interactions in 3-D to examine behavioral interactions with finer detail.
Materials and Methods
Field Locations
I made ultrasound recordings with concurrent insect surveys at two field locations between July 7-26th, 2015 (Sampling intervals were not uniform because of weather). The first field site was a large grassy area (approximately 100 m by 50 m) at the Southwestern Research Station (SWRS), Portal Arizona. This location is normally almost entirely dark with minimal human lighting for several kilometers in all directions. I experimentally added ultraviolet illumination with a single black light (BioQuip 2805 night collecting light; Rancho Dominguez, CA) mounted 2 m off the ground on a pole for two of six recording nights at this location. I recorded a total of 13 h with lights on and 21 h with the lights off at this location. The light was added to concentrate insects during part of the recording period to ensure a range of bat and insect activities during the study. While some bats may be sensitive to ultraviolet light (Gorreson et al., 2015), there is little reason to believe that the presence of ultraviolet light would affect bat competitive interactions other than by increasing insect abundance.
The second location was at a mercury vapor streetlight in the parking lot of the Animas High School, Animas, New Mexico, where I recorded for a total of 14 h over three nights. Animas is a rural area with relatively little development. However, there were several streetlights in the surrounding area including at the high school and neighboring houses. Therefore, there was more human lighting at this location than at SWRS. Observations conducted over multiple years indicated that Mexican free-tailed bats commonly foraged at both of the recording areas.
Acoustic Recording and Analysis
I used an Avisoft Ultrasound Gate with CM16/CMPA microphones (Avisoft Bioacoustics, Glienicke/Nordbahn, Germany) to make field recordings. The Ultrasound Gate was set to trigger recordings any time the sound level exceeded a set threshold. Recordings continued until no more signals were detected. The sensitivity on the device was set to high to make as many recordings as possible. The device transmitted recordings in real time to a laptop therefore there was no down time between recordings. The same recording unit and settings were used for all recording nights at both locations. Each night, recordings were started one hour after sunset and continued for up to nine hours. However, thunderstorms caused me to reduce this recording period on multiple nights.
Concurrent with the acoustic recordings, an ultraviolet light insect funnel trap (Bioquip 2851) with 95% ethyl alcohol as a killing agent was used to measure insect abundance. The insect trap was set approximately 200 m away from each recording location in open habitats. This distance was selected to sample insect activity near the recording area while avoiding attracting insects directly from the recording area. However, this setup had the disadvantage of not sampling insects in the foraging patches where the study occurred. Insects were collected hourly out of the traps and labeled and stored in collecting bags. The number of insects with a wingspan > 1 cm were counted and weighed for each hourly collection. Tadarida brasiliensis consumes a wide variety of insects including moths, beetles, flies, and other groups (Lee and McCracken, 2005), therefore I did not exclude insects based on taxonomy.
All acoustic recordings were analyzed using a custom spectrogram visualization program written in MATLAB r2016a (Natick, MA). Each audio clip recorded by the ultrasound gate was classified based on the presence or absence of (1) feeding buzz, (2) song, and (3) sinFM calls. Feeding buzzes are characterized by a rapid increase in calling rate, a decrease in minimum frequency, and progressively reduced call duration (Schwartz et al., 2007; Figure 1B). To be considered a feeding buzz, I required a pulse interval shorter than 11 ms, which corresponds to the beginning of buzz 2 phase in T. brasiliensis (Schwartz et al., 2007). Tadarida brasiliensis songs are highly variable but are always made of three types of phrases: chirps, buzzes, and trills (Bohn et al., 2009). Chirps contain short, frequency-modulated “A” syllables and more complex “B” syllables, which contain individual-specific information (Bohn et al., 2008; Figure 1C). Song observed in the field clearly had these components, but an analysis was not conducted to determine whether and how they may differ from song produced in the roost. Sinusoidal frequency-modulated signals, as the name implies, have a distinct pattern of frequency oscillation (Figure 1D). They nearly always co-occur with the feeding buzz of a conspecific and can be produced as a single syllable or as a series of multiple syllables separated by short gaps (Corcoran and Conner, 2014). Finally, for each echolocation recording (Figure 1A), I measured the number of T. brasiliensis echolocation calls using automated functions in Sonobat v. 3.0 (Arcata, CA). In addition, a student examined echolocation patterns to classify all recordings as having either one bat present for most of the recording or two or more bats present.
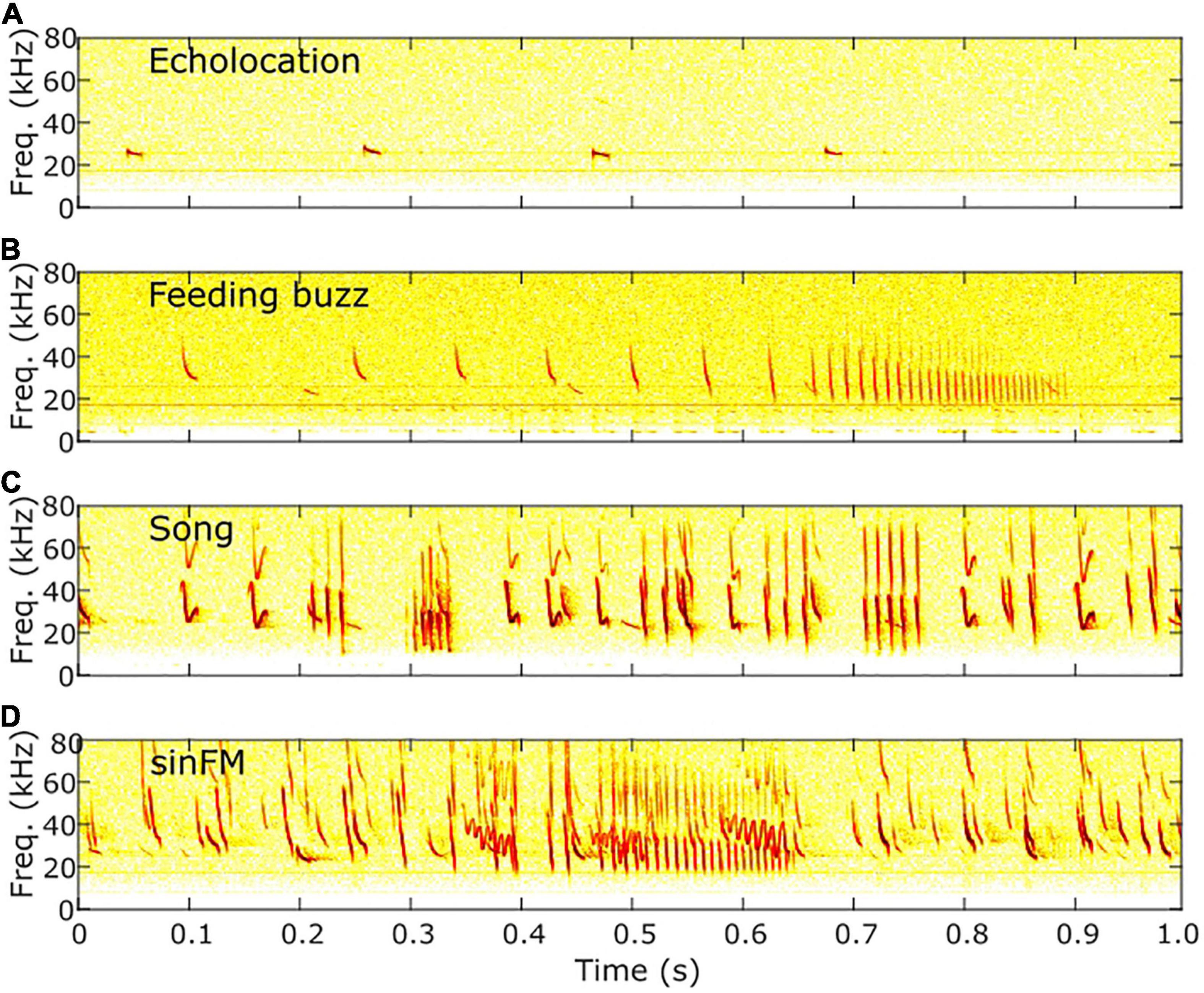
Figure 1. Example spectrograms of acoustic behaviors. (A) Search-phase echolocation calls, (B) approach and terminal phase echolocation calls (“feeding buzz”), (C) Song, and (D) sinFM calls overlapping another bat’s feeding buzz.
Three-Dimensional Video Analysis
To examine competitive interactions in detail, I recorded infrared video with four infrared cameras (Basler Ace ACA-2000) recording at 50 frames per second with pixel resolution of 1,920 × 1,080 and Navitar 12 mm C-mount lenses. Illumination was provided by four Raytec Raymax 200 illuminators. Video recordings were triggered by a human observer after events of interest (including feeding behavior and any apparent competitive interactions) using a post-trigger and recording time of 10 s. The trigger was also connected to the ultrasound gate to acquire synchronized ultrasound recordings. The microphone was placed 20 m in front of the cameras near the center of the cameras’ fields of view to maximize correspondence between acoustic and video detections.
A 3-D calibration was made by moving a wand with two infrared markers at a fixed distance through the calibration volume and using easyWand software (Theriault et al., 2014). The gravitational axis was established by tracking movement of a tennis ball thrown in the air. I used dltDV8 software to digitize bats and make 3-D tracks (Hedrick, 2008). Calibrations were shown to have good quality, with the standard deviation of wand lengths being <1% of total wand length and measured speed of gravity having <1% error.
Data Analysis
To analyze acoustic events, I binned recording periods into 1-minute intervals and determined the number of echolocation calls, feeding buzzes, songs and sinFM within each interval using the methods described above. This interval was chosen to characterize the acoustic environment near the time when bats produce song and sinFM calls. Choosing an interval of 30 s or 2 min instead of one minute did not change the results. To reduce pseudo-replication, I sub-sampled events to no more than one interval every 15 min. I compared the number of echolocation calls and feeding buzzes per minute for sampling intervals having only echolocation (no song or sinFM calls) vs. intervals with song or sinFM calls using a Kruskal Wallace test with Tukey-Kramer post hoc comparisons.
The insect data had a sampling interval of one hour. Therefore, I conducted a second analysis with counts of the four acoustic events binned into one-hour intervals corresponding with the insect sampling periods. I used generalized linear mixed models to predict the frequency of echolocation calls, feeding buzzes, song and sinFM calls using insect count, time of night (“hour”) and presence/absence of additional lighting as fixed effects and recording night and recording location as random effects. To control for false positives that could occur with numerous statistical tests, I used adjusted P-values using the Benamini-Hochberg method (Benjamini and Hochberg, 1995). Results were considered statistically significant if they had an adjusted P-value < 0.05. All statistics were conducted using MATLAB v2020b.
Results
I recorded 356,159 echolocation calls, 4,491 feeding buzzes, 336 songs and 144 sinFM calls during the nine nights of this study. During one-minute intervals including song, rates of echolocation recordings were slightly, but significantly higher than periods containing only echolocation (Figure 2A). In comparison, intervals with sinFM calls had substantially higher numbers of echolocation calls recorded, indicating the presence of many more bats (Figure 2A). Only 9% of recordings (17 of 186) made within one-minute of sinFM calls were classified manually as one bat being present during most of the recording, compared to 38% of recordings (171 of 453) made within one minute of song, a highly significant difference (Fisher’s exact test, P = 2.5e-14). This result and inspection of echolocation recordings (Supplementary Figure 1) confirms that the observed differences in echolocation rates reflected a difference in the number of individual bats present at the field site.
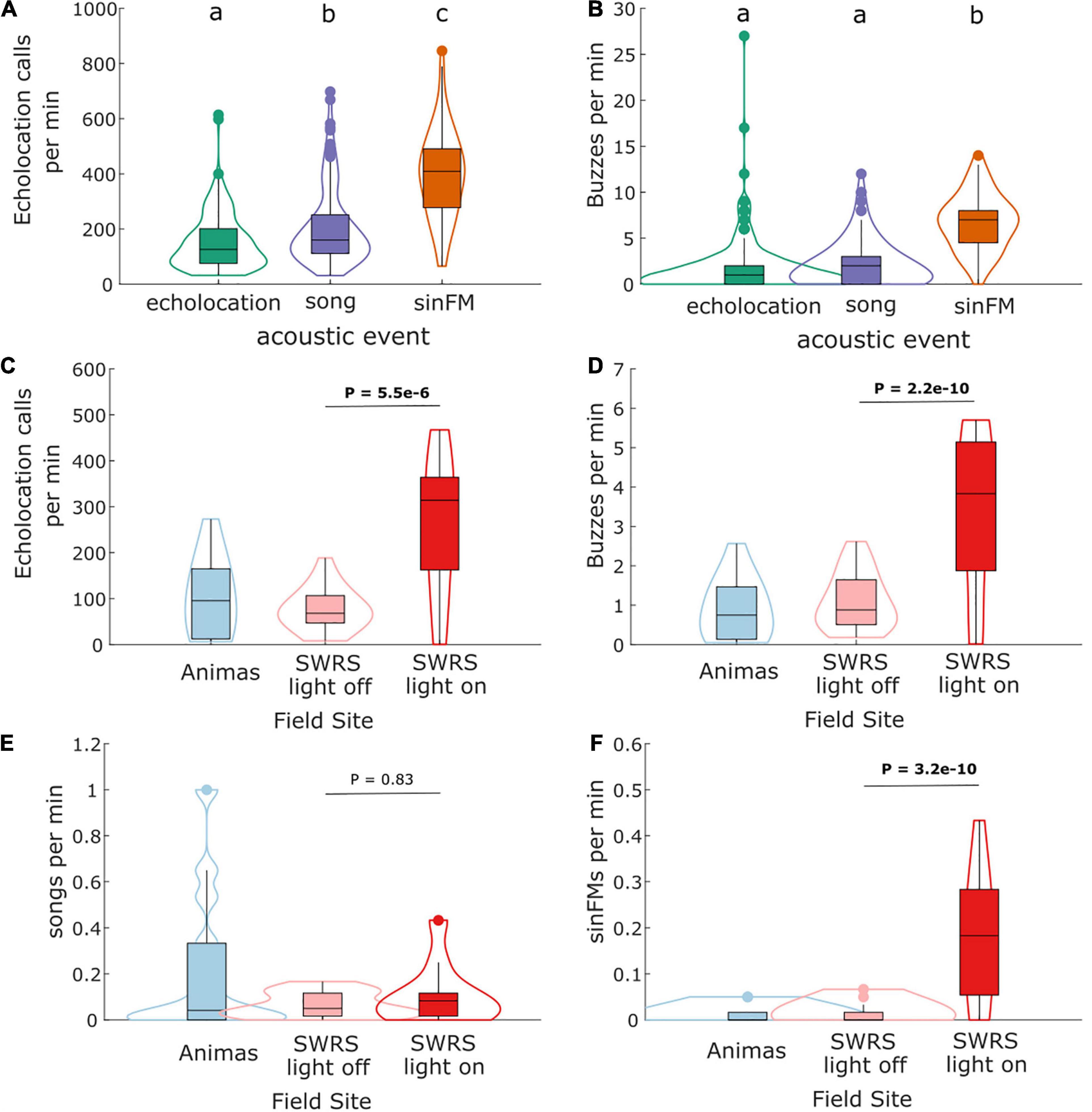
Figure 2. Conditions of song and sinFM production. Comparison of echolocation (A) and feeding buzz (B) prevalence during 1-min intervals with only echolocation (N = 230) vs. periods with song (N = 119) or sinFM calls (N = 68). Data are visualized as violin plots showing probability density functions and box plots showing 10th, 25th, 50th, 75th and 90th percentiles plus outliers. Letters above plots show groupings found to be significantly different using a Kruskal-Wallace test with Tukey-Kramer post hoc comparisons. Rates of echolocation (C), feeding buzzes (D), songs (E) and sinFMs (F) at the two field sites with or without supplemental lighting. Note that adding light to the SWRS site increased echolocation recordings, feeding buzzes and sinFMs, but not songs. See Results and Table 1 for statistics.
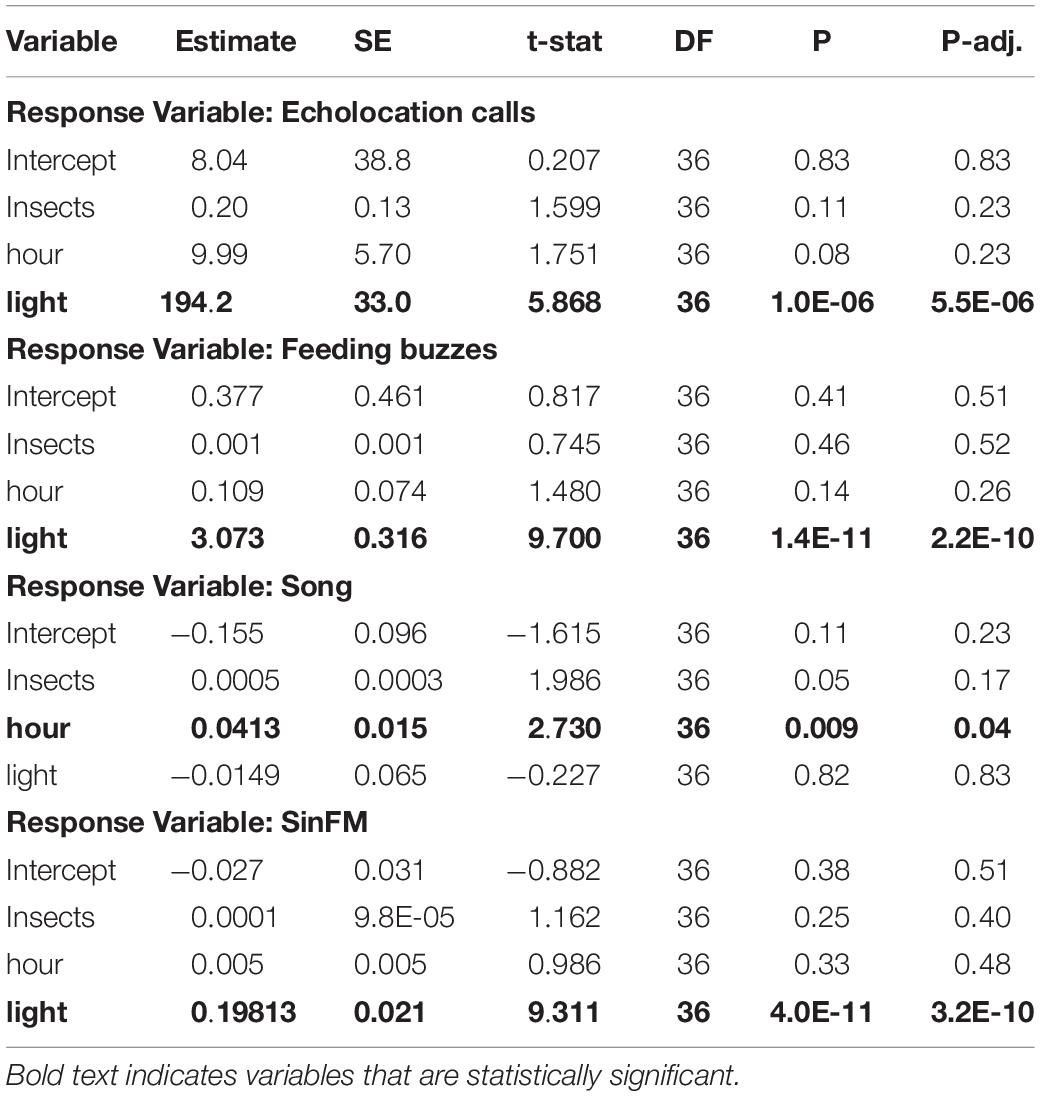
Table 1. Effects of environmental variables on acoustic activity of bats as determined by generalized linear mixed effects models.
Feeding buzz rates were substantially higher during 1-minute intervals containing sinFM calls, but not intervals containing song, compared to baseline feeding buzz activity (Figure 2B). This demonstrates that bats make song with relatively few bats present that are feeding at low rates. In contrast, bats produce sinFM calls most often when many bats that are present and feeding at high rates.
Insect abundance had no detectable effect on echolocation rates, feeding buzzes, song or sinFM call production (Table 1). However, the addition of a light at the SWRS field location caused a dramatic increase in the number of insects present (Personal observation). It should be noted that the method used was not able to document the increase of insects within the foraging patch caused by supplemental lighting. Supplemental lighting at SWRS also led to a significant increase in echolocation recordings, feeding buzzes and sinFM calls, but not song (Figure 2C-F and Table 1). This further demonstrates that sinFM calls, but not song, are produced when many bats are feeding at high rates in a food patch. Bats produced more song later in the night, but echolocation, feeding buzzes and sinFM production did not vary with time of night (Table 1).
I documented nine cases of bats chasing conspecifics on video (Supplementary Video 1). Seven of these nine events included a bat producing song, but none involved a bat producing sinFM calls, a statistically significant difference (binomial probability test; P = 0.0078). Three-dimensional reconstructions of these events show a bat flying closely behind another bat and following the leading bat’s repeated turning maneuvers (Figure 3). The trailing bat’s flight path shows wider turns and a longer path length, which is indicative of the trailing bat flying at a higher speed than the leading bat. These are all indicators of chasing behavior rather than following behavior (Shelton et al., 2014).
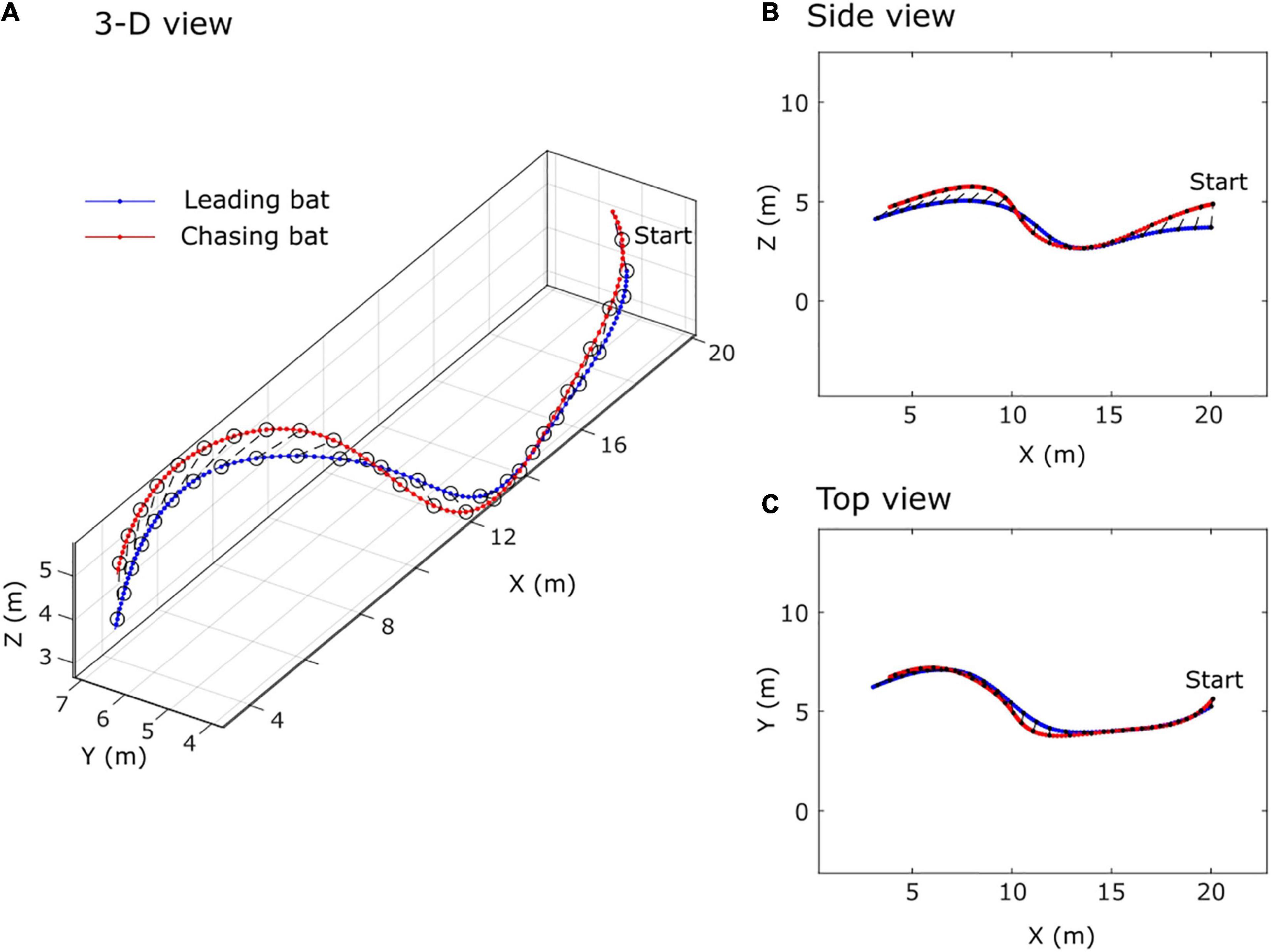
Figure 3. Example chasing sequence observed during song production. (A) 3-D perspective, (B) profile or side view, (C) top view. Note that the red bat remains slightly behind the blue bat throughout the sequence and makes slightly wider turns, which are indicative of chasing behavior.
Discussion
Mexican free-tailed bats exhibited two distinct behaviors for competing with conspecifics at foraging sites. When the density of conspecifics and feeding events were low (as indicated by echolocation recordings and feeding buzzes), bats produced song and chased conspecifics (Figures 2A,B, 3 and Supplementary Video 1). In contrast, bats produced sinFM calls to jam competitors (Corcoran and Conner, 2014) when numerous bats were present and feeding at high rates.
Singing and jamming within food patches have different contexts and apparent aims. Singing and chasing conspecifics appears to be an effort to deter conspecifics and force them to leave the food patch. The most direct evidence for this was the observations of bats producing song while chasing conspecifics in the food patch (Figure 3 and Supplementary Video 1). This is consistent with previous research conducted in a laboratory showing that big brown bats use food claiming signals to deter competitors from foraging (Wright et al., 2014). Mexican free-tailed bats also produce song when defending mates in the roost (Bohn et al., 2009). Therefore, it appears that song is used for both defending food and mates in this species. Song was produced more often later in the night (Table 1), which could result from bats having more time to establish foraging areas to defend from competitors.
In contrast to singing, jamming does not appear to be aimed at deterring conspecifics as much as directly preventing them from sensing and capturing individual prey items. SinFM calls are produced only during the last moments of prey capture, when a conspecific is trying to intercept the prey (Corcoran and Conner, 2014). Acoustic 3-D reconstructions from earlier research (Corcoran and Conner, 2014) showed that after jamming a conspecific, the jamming bat would often attempt to capture the prey item instead of chasing the other bat. The bat that was jammed would often turn the tables and try to jam its competitor. This sometimes went back and forth several times until one bat gave up and the other had a chance to capture the prey without interference (Corcoran and Conner, 2014). SinFM calls are very similar to the “herding” calls used by dominant males forcefully pushing females into a cluster in his territory (Bohn et al., 2008). However, aggressive behavior was not associated with sinFM calls being used in the field, therefore this similar acoustic signal appears to have very different function when used in food competition versus in a roost with potential mates. In summary, singing appears to be an effort to chase conspecifics out of a food patch and deter them through aggression, whereas jamming directly prevents conspecifics from acquiring a specific food item by interfering with its sensory system.
Why do Mexican free-tailed bats have multiple strategies for competing with conspecifics? At low densities of competitors, the benefits of potentially removing a competitor from a food patch may outweigh the costs. With fewer competitors present, the remaining bat can hunt insects without a competitor present that can distract attention (Cvikel et al., 2015) and capture food items. Song conveys information on the identity of the individual (Bohn et al., 2008), so it may be possible for individuals to establish dominance over others that they encounter frequently at foraging sites. When many competitors are present, food claiming via song might not be effective or energetically beneficial because the bats would spend too much time and energy chasing competitors instead of pursuing prey. Increased costs of territorial behavior with higher competitor density is known to occur, for example, in the form of reduced immune responsiveness in some lizards (Svensson et al., 2001). When more competitors are present, Mexican free-tailed bats appear to compete directly for individual prey items, with sonar jamming being a key strategy for preventing competitors from depleting the immediate food resource.
It should be noted that this study was not able to track individuals over time. Therefore, observed differences in competitive behavior may result from individuals switching competitive behaviors under different contexts. Alternatively, different individuals could specialize on different behaviors that are used more often under different competition densities. For example, dominant males could preferentially use song to establish food defense territories where competition is kept at a low density, whereas less dominant males and females may preferentially use sinFM calls at food patches with higher densities of competitors. Additional research is needed to differentiate between these possibilities.
Contrary to my prediction, measured insect densities did not correlate with differences of any acoustic behaviors (Table 1). The method used—placing insect traps 200 m away from foraging sites—was designed to assay insect abundance in the vicinity of the foraging areas without removing potential prey from foraging patches. However, this method was unable to measure the increases of insect activity caused by the addition of supplemental lighting. Observations at the field site indicated that supplemental lighting had a dramatic effect on insect abundance. Supplemental lighting also increased bat echolocation recordings, feeding buzzes and sinFM calls (Figures 2C–F). Therefore, insect abundance within the food patch appears to be an important factor for bat competitive behavior and moreso than insect activity in the surrounding area. Additional research is needed to confirm this finding and determine how the combination of overall insect abundance and insect abundance within a food patch influences bat behavior. This could be achieved through a combination of insect traps and imaging of the night sky (Ruczyński et al., 2019).
This study provides novel detail into the natural competitive behaviors of bats. Mexican free-tailed bats may be under particularly high selective pressure from food competition. Flight is energetically costly and Mexican free-tailed bats fly long distances in pursuit of prey (Best and Geluso, 2003) while competing with as many as one million individuals that share a colony (Betke et al., 2008). It may be more energetically favorable for individuals of this species to stay in a food patch and compete for prey via song or jamming rather than to depart in search of unoccupied food patches.
In addition to being competitors, other bats can improve efficiency of prey search. Bats can hear one another’s calls at much longer distances than they can detect prey with echolocation (Dechmann et al., 2009). Therefore, groups of bats can function as a distributed array of sensors in the environment (Egert-Berg et al., 2018). This can lead to a situation where individuals simultaneously experience costs and benefits to social foraging. This may mean that there is an optimal intermediate foraging density for insectivorous bats (Cvikel et al., 2015). The behaviors of singing and jamming documented here are therefore only part of a broader set of social interactions that likely occur in this species and more broadly within bats.
Food competition is one of the most common ecological interactions that organisms encounter. This study demonstrates that the density of competitors is a key factor in determining what competitive strategies will be effective. This research also highlights how animal sensory and locomotor abilities are important for determining the effectiveness of competition strategies.
Data Availability Statement
The original contributions presented in the study are publicly available. This data can be found here: https://osf.io/nzqtv/?view_only=1befa9e597634c12a9bfa526878a86c2.
Ethics Statement
The animal study was reviewed and approved by Wake Forest University IACUC.
Author Contributions
AC designed and conducted the experiments, conducted the data analysis and wrote the manuscript.
Funding
Funding was provided by the National Science Foundation (IOS-1257248) and the University of Colorado, Colorado Springs.
Conflict of Interest
The author declares that the research was conducted in the absence of any commercial or financial relationships that could be construed as a potential conflict of interest.
Publisher’s Note
All claims expressed in this article are solely those of the authors and do not necessarily represent those of their affiliated organizations, or those of the publisher, the editors and the reviewers. Any product that may be evaluated in this article, or claim that may be made by its manufacturer, is not guaranteed or endorsed by the publisher.
Acknowledgments
I am grateful to Orion Goodman, who assisted with data collection in the field. The staff of the Southwestern Research Station was very helpful in facilitating field research. Shin Tamura assisted with many hours of audio analysis. William Conner provided logistical support and guidance for the project. I thank five reviewers for their comments that improved the manuscript.
Supplementary Material
The Supplementary Material for this article can be found online at: https://www.frontiersin.org/articles/10.3389/fevo.2022.877579/full#supplementary-material
References
Amarasekare, P. (2002). Interference competition and species coexistence. Proc.R. Soc. London. 269, 2541–2550. doi: 10.1098/RSPB.2002.2181
Barlow, K. E., and Jones, G. (1997a). Function of pipistrelle social calls: field data and a playback experiment. Animal Behav. 1996, 991–999. doi: 10.1006/anbe.1996.0398
Barlow, K. E., and Jones, G. (1997b). Differences in songflight calls and social calls between two phonic types of the vespertilionid bat Pipistrellus pipistrellus. J. Zool. 241, 315–324. doi: 10.1111/J.1469-7998.1997.TB01962.X
Benjamini, Y., and Hochberg, Y. (1995). Controlling the false discovery rate: A practical and powerful approach to multiple testing. J. R. Stat. Soc. 57, 289–300. doi: 10.1111/J.2517-6161.1995.TB02031.X
Best, T. L., and Geluso, K. N. (2003). Summer foraging range of mexican free-tailed bats (Tadarida brasiliensis mexicana) from Calsbad Cavern. New Mexcio. Southwest. Nat. 48, 590–596. doi: 10.1894/0038-4909(2003)048<0590:sfromf>2.0.co;2
Betke, M., Hirsh, D. E., Makris, N. C., McCracken, G. F., Procopio, M., Hristov, N. I., et al. (2008). Thermal imaging reveals significantly smaller Brazilian free-tailed bat colonies than previously estimated. J.Mammal. 89, 18–24. doi: 10.1644/07-MAMM-A-011.1
Bohn, K. M., and Gillam, E. H. (2018). In-flight social calls: a primer for biologists and managers studying echolocation. Can. J. Zool. 96, 787–800. doi: 10.1139/CJZ-2017-0188
Bohn, K. M., Schmidt-French, B., Ma, S. T., and Pollak, G. D. (2008). Syllable acoustics, temporal patterns, and call composition vary with behavioral context in Mexican free-tailed bats. J. Acoust. Soc. Am. 124, 1838–1848. doi: 10.1121/1.2953314
Bohn, K. M., Schmidt-French, B., Schwartz, C., Smotherman, M., and Pollak, G. D. (2009). Versatility and stereotypy of free-tailed bat songs. PloS One 4:e6746. doi: 10.1371/journal.pone.0006746
Corcoran, A. J., and Conner, W. E. (2014). Bats jamming bats: Food competition through sonar interference. Science 346, 745–747. doi: 10.1126/science.1259512
Cvikel, N., Egert Berg, K., Levin, E., Hurme, E., Borissov, I., Boonman, A., et al. (2015). Bats aggregate to improve prey search but might be impaired when their density becomes too high. Curr. Biol. 25, 206–211. doi: 10.1016/J.CUB.2014.11.010
Dechmann, D. K. N., Heucke, S. L., Giuggioli, L., Safi, K., Voigt, C. C., and Wikelski, M. (2009). Experimental evidence for group hunting via eavesdropping in echolocating bats. Proc.R. Soc. B 276, 2721–2728. doi: 10.1098/RSPB.2009.0473
Egert-Berg, K., Hurme, E. R., Greif, S., Goldstein, A., Harten, L., and Herrera, M. L. G. (2018). Resource ephemerality drives social foraging in bats. Curr. Biol. 28, 3667.e–3673.e. doi: 10.1016/j.cub.2018.09.064
Gillam, E. H. (2007). Eavesdropping by bats on the feeding buzzes of conspecifics. Can. J. Zool. 85, 795–801. doi: 10.1139/Z07-060
Gorreson, P. M., Cryan, P., Dalton, D. C., Wolf, S., and Bonaccorso, F. J. (2015). Ultraviolet vision may be widespread in bats. Acta Chiropterol. 17, 193–186. doi: 10.3161/15081109acc2015.17.1.017
Hedrick, T. L. (2008). Software techniques for two- and three-dimensional kinematic measurements of biological and biomimetic systems. Bioinspir. Biomim. 3:6. doi: 10.1088/1748-3182/3/3/034001
Lee, Y.-F., and McCracken, G. F. (2005). Dietary variation of Brazilian free-tailed bats links to migratory populations of pest insects. J.Mammal. 86, 67–76. https://academic.oup.com/jmammal/article/86/1/67/2373681. doi: 10.1644/1545-1542(2005)086<0067:dvobfb>2.0.co;2
Petren, K., and Case, T. J. (1996). An experimental demonstration of exploitation competition in an ongoing invasion. Ecology 77, 118–132. doi: 10.2307/2265661
Ruczyński, I., Hałat, Z., Zegarek, M., Borowik, T., and Dechmann, D. K. N. (2019). Camera transects as a method to monitor high temporal and spatial ephemerality of flying nocturnal insects. Methods Ecol. Evol. 11, 294–302. doi: 10.1111/2041-210x.13339
Schnitzler, H.-U., Moss, C. F., and Denzinger, A. (2003). From spatial orientation to food acquisition in echolocating bats. Trends Ecol. Evol. 18, 386–394. doi: 10.1016/S0169-5347(03)00185-X
Schwartz, C., Tressler, J., Keller, H., Vanzant, M., Ezell, S., and Smotherman, M. (2007). The tiny difference between foraging and communication buzzes uttered by the Mexican free-tailed bat, Tadarida brasiliensis. J. Comp. Physiol. A 193, 853–863. doi: 10.1007/s00359-007-0237-7
Shelton, R. M., Jackson, B. E., and Hedrick, T. E. (2014). The mechanics and behavior of cliff swallows during tandem flights. J. Exper. Biol. 217, 2717–2725. doi: 10.1242/jeb.101329
Svensson, E., Sinervo, B., and Comendant, T. (2001). Density-dependent competition and selection on immune function in genetic lizard morphs. Proc.Natl. Acad. Sci. 98, 12561–12565. doi: 10.1073/pnas.211071298
Theriault, D. H., Fuller, N. W., Jackson, B. E., Bluhm, E., Evangelista, D., Wu, Z., et al. (2014). A protocol and calibration method for accurate multi-camera field videography. J. Exper. Biol. 217, 1843–1848 doi: 10.1242/jeb.100529
Voigt, C. C., Frick, W. F., Holderied, M. W., Holland, R., and Kerth, G. (2017). Principles and patterns of bat movements: from aerodynamics to ecology. Q. Rev. Biol. 92, 267–287. doi: 10.1086/693847
Keywords: echolocation, exploitation competition, interference competition, social behavior, social calls, sonar
Citation: Corcoran AJ (2022) Sing or Jam? Density-Dependent Food Competition Strategies in Mexican Free-Tailed Bats (Tadarida brasiliensis). Front. Ecol. Evol. 10:877579. doi: 10.3389/fevo.2022.877579
Received: 16 February 2022; Accepted: 29 March 2022;
Published: 25 April 2022.
Edited by:
Han Li, University of North Carolina at Greensboro, United StatesReviewed by:
Erin Gillam, North Dakota State University, United StatesRolf Mueller, Virginia Tech, United States
M. Jerome Beetz, Julius Maximilian University of Würzburg, Germany
Michael Smotherman, Texas A&M University, United States
Laura Kloepper, University of New Hampshire, United States
Copyright © 2022 Corcoran. This is an open-access article distributed under the terms of the Creative Commons Attribution License (CC BY). The use, distribution or reproduction in other forums is permitted, provided the original author(s) and the copyright owner(s) are credited and that the original publication in this journal is cited, in accordance with accepted academic practice. No use, distribution or reproduction is permitted which does not comply with these terms.
*Correspondence: Aaron J. Corcoran, YWNvcmNvcmFAdWNjcy5lZHU=