- 1Key Laboratory of Tropical and Subtropical Fishery Resource Application and Cultivation, Ministry of Agriculture and Rural Affairs of the People’s Republic of China, Pearl River Fisheries Research Institute, Chinese Academy of Fishery Sciences, Guangzhou, China
- 2Key Laboratory of Aquatic Animal Immune Technology of Guangdong Province, Guangzhou, China
Paddy field carp (PF-carp) is an economically important fish cocultured with rice in traditional agricultural systems. Several distinctive strains of PF-carp have been formed through years of artificial and cross breeding. There is a concern about the status of germplasm resources among PF-carp, since little is known about the genetic sources, diversity, or differentiation. In this study we collected 17 PF-carp populations covering Daotian carp (DTL), Ru Yuan No. 1 (RY), Jinbian carp (JBL), Shaijiang carp (SJL), and Wu carp (WL) along the Pearl River basin to explore their genetic sources and diversity using concatenated sequences of the mitochondrial cytochrome b gene and the D-Loop region. According to the haplotype network analyses, 1, 9, and 57 haplotypes originated from Cyprinus carpio carpio, Cyprinus carpio haematopterus and Cyprinus carpio rubrofuscus, respectively, confirming that genetic introgression has occurred in Pearl River PF-carp populations and Cyprinus carpio carpio was the most common species for genetic origin. The results showed that RY exhibited the lowest level of nucleotide diversity (π = 0.0011) due to high-intensity breeding and was significantly differentiated from the other four strains. PF-carp strains in these remote traditional systems tended to experience artificial selection and a lack of farmer connection that gradually increased genetic differentiation among strains. Notably, three populations of JBL exhibited significant high-level differentiation, since they originated from mountainous areas hindering farmers from fry exchange. In contrast, no significant differentiation was uncovered in the WL populations, since this strain is the most popular cultured strain and has undergone artificial exchange of parents and fry in many cultured regions. This study helps us to understand the status of germplasm resources among PF-carp and to trace their genetic origin before being introduced for local cultivation.
Introduction
The common carp, Cyprinus carpio, is an economically important fish widely distributed in Eurasia (Kohlmann, 2015). It has adapted to various environments and has experienced long-term breeding to form numerous distinctive strains (Wu and Gui, 1999; Shen and Liu, 2000; Kohlmann, 2015). According to Monographs of Cyprinidae in China, four subspecies of common carp have been recorded in China, Cyprinus carpio carpio (CC), Cyprinus carpio haematopterus (CH), Cyprinus carpio rubrofuscus (CR), and Cyprinus caprpio chilia (CCH) (Wu, 1977a,b). However, Kottelat (2001) regarded CC and CR as two separate species and rejected CR as a valid species. Considering Zhou et al. (2004) demonstrated that CC, CH and CR formed three distinct clades using mitochondrial loci, we adopted the taxonomic classification in Wu (1977a,b). CC is distributed over vast areas of Central Asia and Europe, while being only found in Xinjiang in China. CH is distributed as far north as the Heilong River and as far south as the Min River. CR is distributed among the Pearl River, Ruan River, and Hainan Island, while CCH is found only in several lakes of Yunnan Province (Wu, 1977a; Yue, 2000).
In southern China, common carp is frequently co-cultured with rice and thus has been named the paddy field carp (PF-carp), which displays rapid growth, strong reproductive ability, and delicious meat with no muddy taste. This rice-fish system benefits both the agricultural environment and the economy. On the one hand, combining rice and fish farming can reduce the use of pesticides and fertilizers and ensure the health of the grain and fish (Berg, 2001; Ahmed and Garnett, 2011). On the other hand, the price of PF-carp is much higher compared to other freshwater fishes. The co-culture of rice and fish can maximize the use of paddy field resources, alleviate human conflict over land use, and ensure a bumper crop and increase farmers’ income (Frei and Becker, 2005; Gan et al., 2019).
Notably, the 1,200-year-old rice–fish system of Qintian county, Zhejiang Province, was listed as one of the “Globally Important Agricultural Heritage Systems (GIAHS)” by the Food and Agriculture Organization of the United Nations in 20051 (Xie et al., 2011). PF-carp farming in the GIAHS rice-fish system named Oujiang carp has been practiced and passed down for generations (Wang, 1997). Within the Qintian GIAHS site, rice-fish farmer households exchange fry and parental carp for their own rice–fish production, thereby contributing to a high rate of gene flow and high genetic diversity of PF-carp (Ren et al., 2018).
In addition to the Oujiang carp in Zhejiang, various strains of PF-carp are bred and cultured in China, including Daotian carp (DTL), Jiangbian carp (JBL), Ruyuan NO. 1 (RY), Shaijiang carp (SJL), and Wu carp (Wl) (Zhu et al., 2018; Gan et al., 2019; Yan et al., 2020). Researchers have carried out genetic diversity and germplasm resources analyses of DTL and JBL in the Pearl River basin using mitochondrial genes (mtDNA) and microsatellites (Gan et al., 2019; Pan et al., 2019; Cheng et al., 2021). Cheng et al. (2021) examined the genetic structure of three DTL populations from Guangxi Province based on the mtDNA D-loop region and the mitochondrial Cytochrome b gene (Cyt b) sequences, indicating that all populations were significantly differentiated (Cheng et al., 2021). Meanwhile, researchers pointed out that certain frequencies of CC and CH were found in these populations, suggesting that PF-carp may suffer introgressive hybridization from CC or CH. Similarly, Pan et al. (2019) used mitochondrial D-loop region and cytochrome c oxidase I sequences to analyze the genetic diversity and phylogenetic relationships between PF-carp (JBL, WL) and wild carp. PF-carp showed lower haplotype diversity than wild carp, and exhibited clear differentiation from wild carp (Pan et al., 2019).
These studies preliminarily explained the genetic diversity of PF-carp in the Pearl River basin, although the sampled populations were rather small and covered few strains. In order to investigate the genetic diversity and structure of PF-carp more comprehensively, we analyzed Cyt b gene sequences and the D-loop region of 17 populations covering five strains of PF-carp. Our main goals were (i) to trace the germplasm resources of PF-carp populations in the Pearl River basin, (ii) to explore the impact of artificial selection or farmer connectivity on the difference of genetic diversity and differentiation for different populations or strains, and (iii) to provide an effective scientific basis for the protection of genetic resources, artificial breeding, and rational development of the resources of PF-carp.
Materials and methods
Sample collection
A total of 524 paddy field carp (PF-carp) specimens were sampled from 17 localities across Guang Xi Province and Guang Dong Province between 2020 and 2021 (Figure 1 and Supplementary Table 1). Five morphologically different strains of PF-carp, “Daotian carp” (DTL, n = 161), “Ru Yuan No. 1” (RY, n = 65), “Jinbian carp” (JBL, n = 64), “Shaijiang carp” (SJL, n = 42), and “Wu carp” (WL, n = 192) were obtained (Figure 2). In addition, we collected both JBL and DTL at the same location of RS (Supplementary Table 1). DTL, JBL, and WL comprised five, three, and eight populations, respectively. RY specifically refers to the PF-carp that has been bred for multiple generations in Yao Autonomous County of Ruyuan. SJL is an indigenous PF-carp cultured in Shaijiang Village of Guangxi Province. A fin clip was taken from each specimen and kept in 99% ethanol. All specimens were stored at the Pearl River Fisheries Research Institute, Chinese Academic of Fishery Sciences.
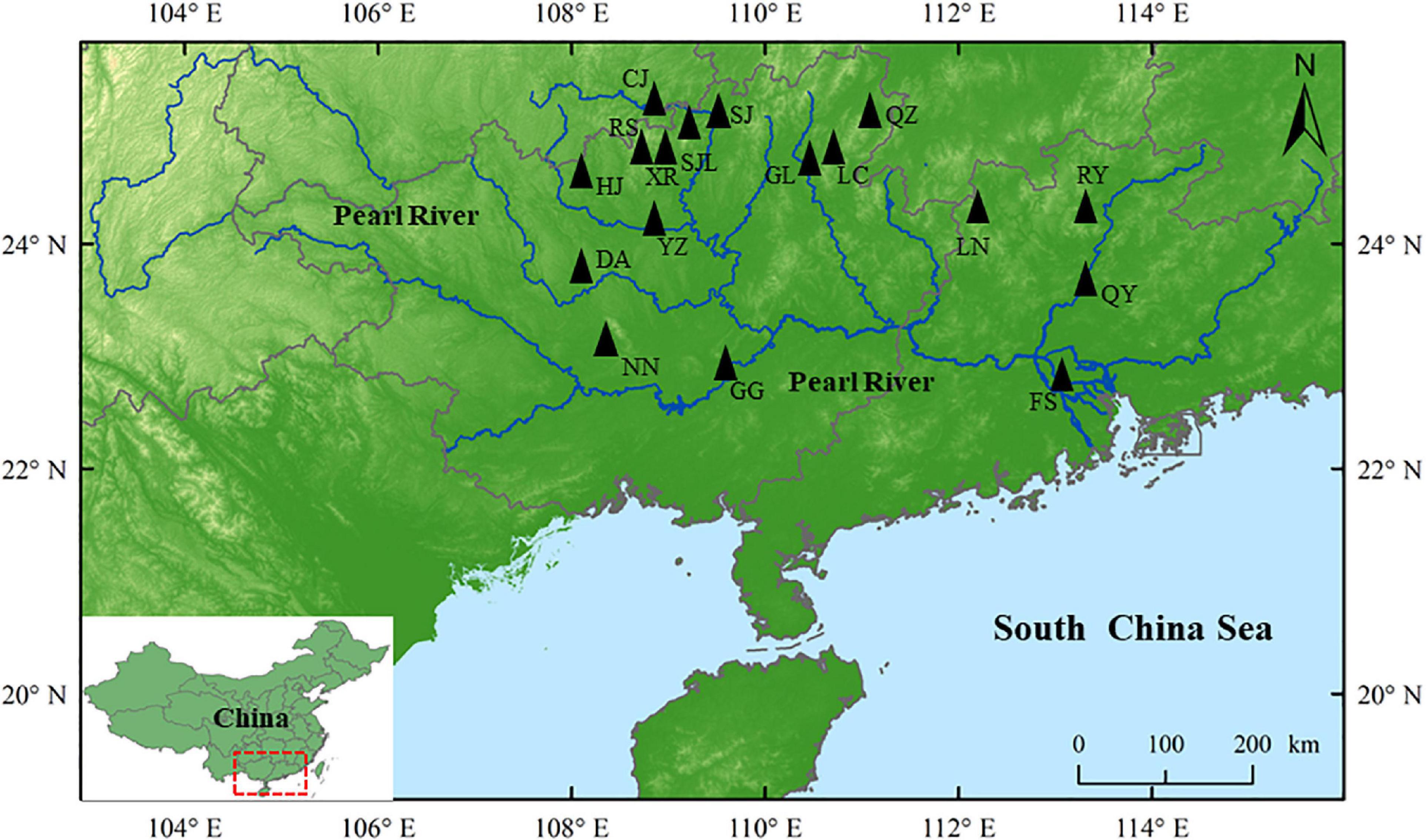
Figure 1. Map of sample sites of PF-carp specimens. Geographical distribution details and the number of samples collected per site are provided in Supplementary Table 1. CJ, Congjiang; DA, Du’an; FS, Foshan; GG, Guigang; GL, Guilin; HJ, Huanjiang; LC, Linchuan; LN, Liannan; NN, Nanning; QY, Qingyuan; QZ, Quanzhou; RS, Rongshui; RY, Ruyuan; SJ, Sanjiang; SJL, Shaijiang; XR, Xiaorong; YZ, Yizhou.
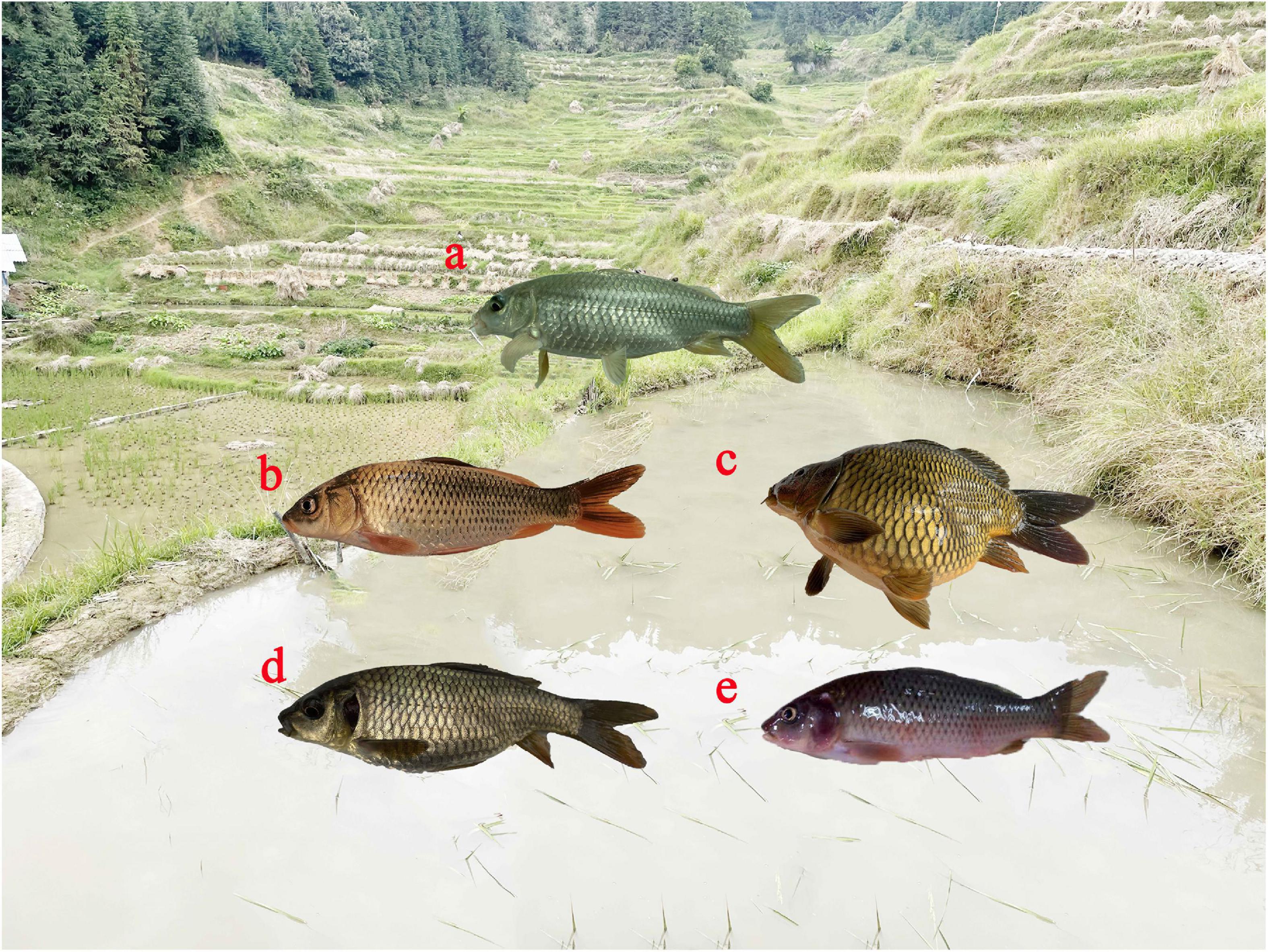
Figure 2. Morphology of five PF-carp strains of (a) Daotian carp, (b) Jinbian carp, (c) Ruyuan No. 1, (d) Shaijiang carp, and (e) Wu carp.
DNA extraction, sequencing, and data processing
Genomic DNA was extracted using a Genomic DNA Extraction Kit according to the manufacturer’s instructions (Axygen, United States). DNA samples were kept at −20°C for further PCR amplification. The primers were designed for the Cyt b gene (Forward primer: 5′-GACTTGAAAAACCACCGTTG-3′, Reverse primer: 5′- CTCCGATCTCCGGATTACAAGAC-3′) and D-loop region (Forward primer: 5′-CATCTTAGCATCTTCAGTG-3′, Reverse primer: 5′- TCACCCCTGGCTCCCAAAGC-3′) based on the Cyprinus carpio mitochondrial genome sequence (GenBank: X61010.1). The amplification program was as follows: an initial denaturation at 94°C for 3 min and then 30 cycles of denaturation at 94°C for 1 min, annealing at 60°C for 1 min, and extension at 72°C for 1 min followed by final extension at 72°C for 5 min. The amplified products were checked and purified using 1.0% low-melting agarose gel electrophoresis and sequenced on 3,730 xl DNA Analyzer (Applied Biosystems, United States). The Cyt b gene and D-loop region were sequenced bi-directionally and unidirectionally, respectively. Cyt b contigs were assembled using SEQMAN (Swindell and Plasterer, 1997). All sequences were aligned, checked, and trimmed using MEGA X (Kumar et al., 2018). The Cyt b sequences and D-loop sequences were concatenated to form combined sequences.
Haplotype network analyses
PopART 1.7.2 was used to reconstruct phylogenetic relationships among haplotypes via a median-joining network method (Leigh and Bryant, 2015). To characterize the genetic resources of different strains and populations, Cyt b and D-loop sequences of CR (n = 10), CH (n = 6), and CC (n = 4) were downloaded from Zhou et al. (2004) to construct a haplotype network diagram. Due to no corresponding Cyt b and D-loop sequences published for CCH and the fact that most previous studies considered that the three abovementioned subspecies were sources for PF-carp selection breeding, our study did not include CCH.
Evaluation of genetic diversity and differentiation
The genetic diversity indices of the number of haplotypes (H), haplotype diversity (Hd) (Shohdy and West, 2020), private haplotype (ph) and nucleotide diversity (π) were assessed with Dansp 6.0 (Rozas et al., 2017) for 17 populations and five strains. Genetic differentiation among and within the five strains was estimated from the fixation index (FST) using Arlequin 3.5 (Excoffier and Lischer, 2010). Analysis of molecular variance (AMOVA) in Arlequin 3.5 was implemented to partition total genetic variation among and within strains and populations. Both FST calculation and AMOVA were based on the pairwise difference between haplotypes estimated according to a non-parametric permutation procedure (1,000 permutations/analysis). In order to assess the possible occurrence of an isolation-by-distance pattern, a Mantel test was carried out in Arlequin 3.5. Geographic distances among localities were estimated using Google Earth pro.
Results
Data information
For all the individuals analyzed (n = 524), we obtained sequences of 948 bp of the Cyt b gene and 708 bp of the D-loop after alignment and trimming. Sixty variable positions (48 parsimony-informative) and 41 variable positions (31 parsimony-informative) were found in the Cyt b and D-loop, respectively. A total of 66 haplotypes were obtained from the concatenated sequences (1,656 bp).
Haplotype network analysis
A haplotype network can reveal evolutionary relationships and frequency distributions of haplotypes among populations. Haplotype networks were obtained by the statistical parsimony procedure for all strains (Figure 3) and for three strains (WL, JBL, and SJL) containing more than two populations (Figure 4). Frequencies and distributions of each haplotype among the studied populations are presented in Supplementary Table 2. Overall, 1/66, 8/66, and 57/66 haplotypes originated from CC, CH, and CR, respectively (Figure 3). The genetic sources of DTL and SJL were derived from CC, CH, and CR, while the genetic resources of JBL, RY, and WL derived from CH and CR (Figure 3). According to the haplotype network of DTL (Figure 4A), CJ shared haplotypes with the other four populations, while Guigang (GG) and Liannan (LN) only shared haplotypes with Congjiang (CJ). In addition, Huanjiang (HJ) and Rongshui (RS) shared haplotypes. It was worth noting that only one shared haplotype was detected within three populations of JBL (Figure 4B). The pattern of shared haplotypes among eight populations within WL strain was frequently observed (Figure 4C).
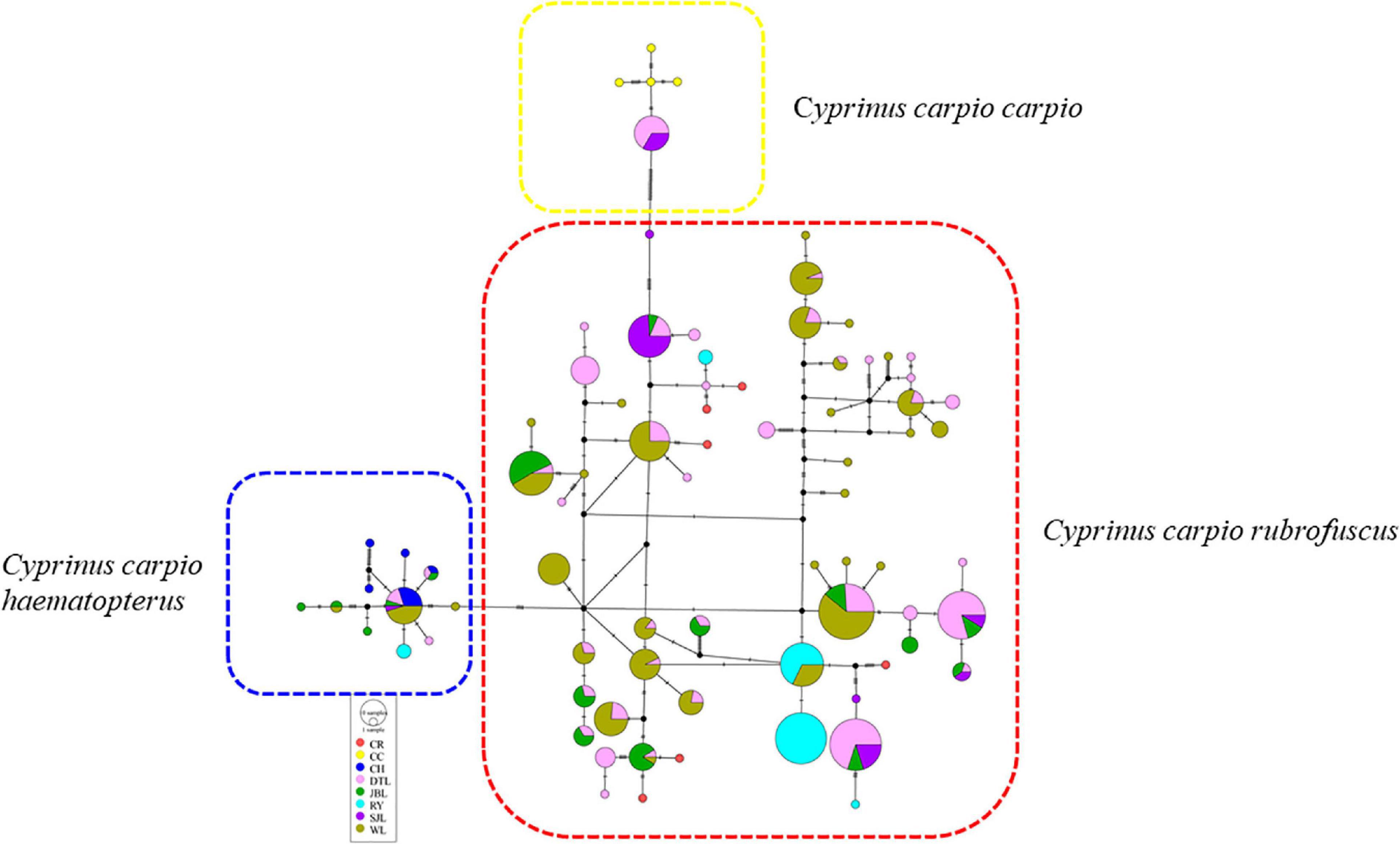
Figure 3. Haplotype network of five strains and three subspecies, including Cyprinus carpio carpio (CC), Cyprinus carpio haematopterus (CH) and Cyprinus carpio rubrofuscus (CR). Cycle size is roughly proportional to the haplotype frequency. DTL, Daotian carp; RY, Ru Yuan No. 1; JBL, Jinbian carp; SJL, Shaijiang carp; WL, Wu carp.
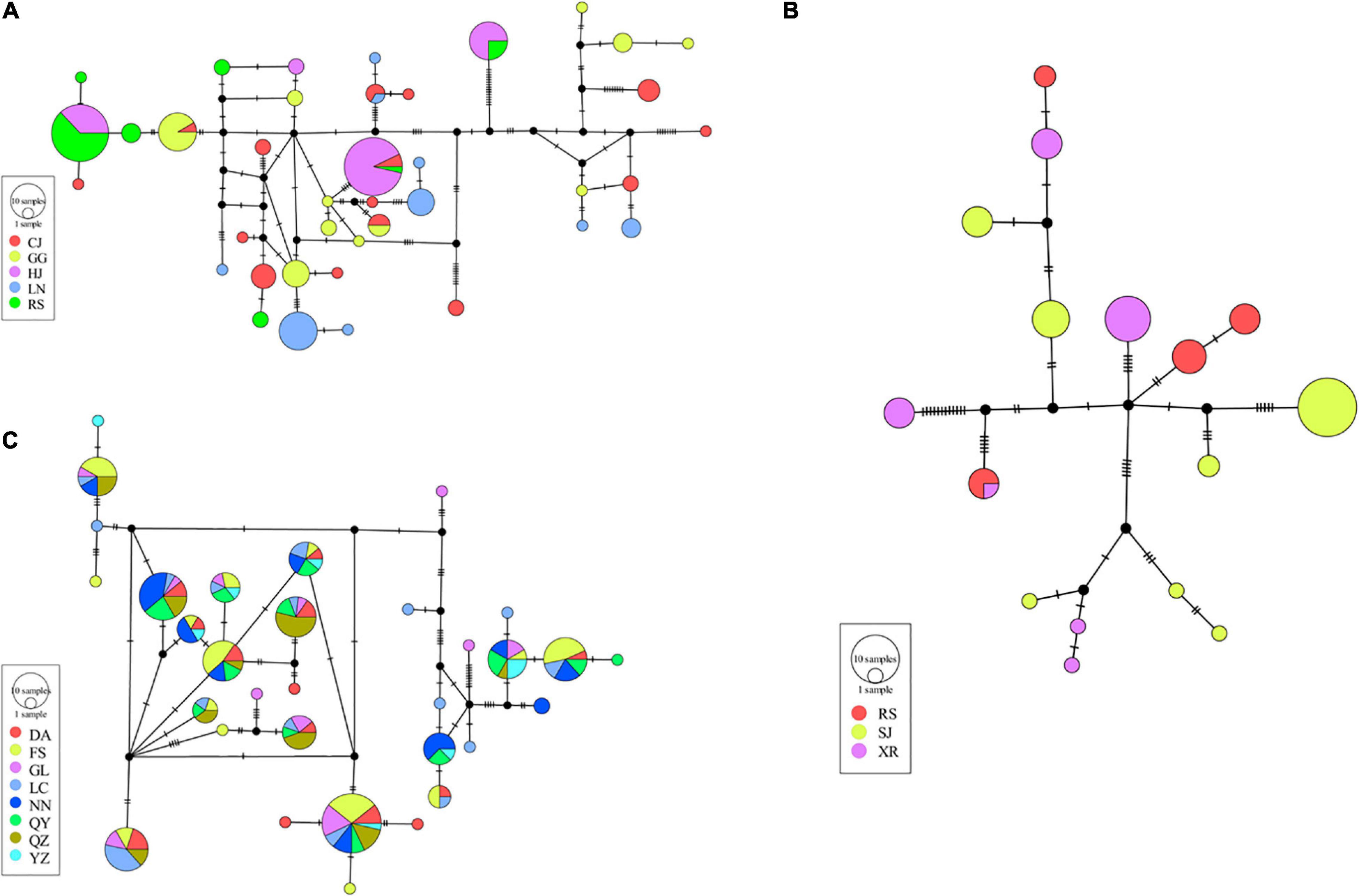
Figure 4. Haplotype network of PF-carp. Panels (A–C) represents Daotian carp (DTL), Jinbian carp (JBL), and Wu carp (WL), respectively. Cycle size is roughly proportional to the haplotype frequency. Location abbreviations refer to Figure 1 and Supplementary Table 1.
Genetic diversity of PF-carp
The indices of genetic diversity H, Hd, and π are summarized in Table 1. A total of 66 haplotypes were obtained from the 524 individual sequences, with 39, 16, 5, 8, and 32 haplotypes from the DTL, JBL, RY, SJL, and WL strains, respectively. There was a high overall haplotype genetic diversity (Hd = 0.959) while a relatively low nucleotide diversity (π = 0.0061) was observed in all strains. Hd of each population ranged from 0.559 (RY) to 0.953 (Du’an; DA), while π ranged from 0.0011 (RY) to 0.0079 (CJ). Meanwhile, the WL strain showed the highest number of unique haplotypes (ph = 16).
Inter-strain differentiation
In the pairwise comparisons of different strains, significant values of FST occurred in all pairs (Table 2), ranging from 0.051 to 0.365. It was noteworthy that RY exhibited high levels of differentiation from DTL, JBL, SJL, and WL (FST = 0.171, 0.248, 0.365, and 0.212, respectively). SJL showed moderate levels of differentiation from JBL and WL (FST = 0.148, 0.149, respectively). According to the results of the AMOVA, the majority (89.13%) of the genetic variation was attributed to variation within strains. Moderate levels of differentiation were detected among all the strains (FST = 0.109, P < 0.001, Table 3).
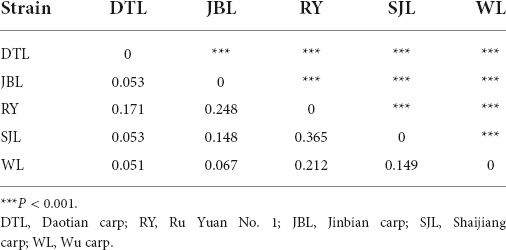
Table 2. Pairwise FST (below diagonal) and significance probability estimates (above diagonal) among strains.
Intra-strain differentiation
To further explore the differentiation among populations within strains, average pairwise FST was calculated for DTL, JBL, and WL, strains that contained more than two populations. The FST values of DTL ranged from 0.072 to 0.359. Interestingly, a significantly high degree of differentiation was observed between RS and all other populations (Supplementary Table 3). Similarly, HJ also displayed a high level of differentiation from other populations except for a moderate level with CJ. Analysis of molecular variance showed that 76.63% of the genetic variation existed within populations (Table 4).
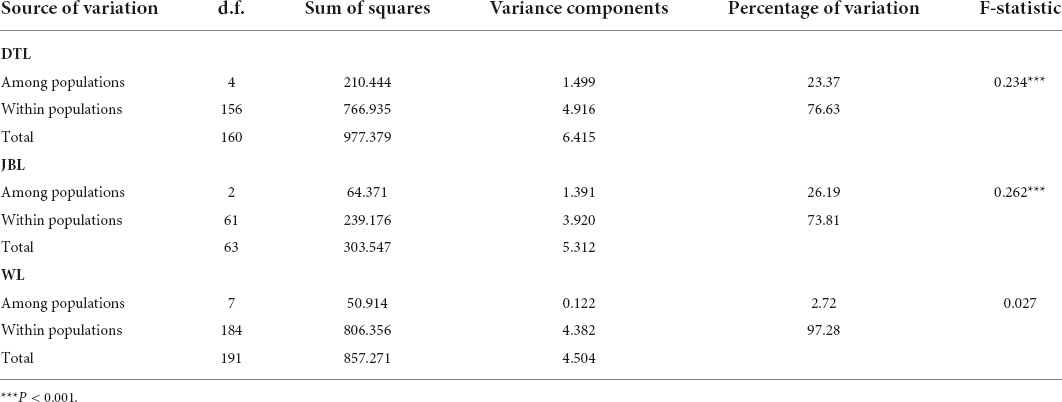
Table 4. Analysis of molecular variance (AMOVA) of Daotian carp (DTL), Jinbian carp (JBL), and Wu carp (WL).
A particularly high level of differentiation was detected between populations of the JBL strain, including Sanjiang (SJ), Rongshuijinbian (RSJ), and Xiaorong (XR) (ranging from 0.217 to 0.282) (Supplementary Table 4). According to the results of AMOVA, the 73.81% of the genetic variation was attributed to variation within populations (Table 4).
With respect to the WL strain, the differentiation level was relatively low among eight populations, varying from −0.042 to 0.176 (Supplementary Table 5). FST values were significant for 7 of 28 combinations of pairs, within which a moderate level of differentiation was only observed in three pairs. The AMOVA results also showed a relatively high percentage of variation within populations (97.28%) (Table 4).
The Mantel test revealed no significant correlation between geographic distance and FST for the three strains, (DTL: P = 0.345, JBL strain: P = 0.188, and WL: P = 0.738).
Discussion
Germplasm resources of PF-carp
It has been suggested that common carp primarily consists of three subspecies in European and Asian populations: CC, CH, and CR, an exception being CCH in lakes of the Yunnan–Guizhou plateau of China (Wu, 1977a; Zhou et al., 2003; Wang et al., 2010). In accordance with Cheng et al. (2021), the haplotype network in our study indicated that the majority haplotype (57/66) originated from CR, and thus this strain was deemed as the main matrilineal resource of the PF-carp. Moreover, certain frequencies of the mitochondrial haplotypes of CC (1/66) or CH (8/66) were also detected in these PF-carp. Considering that CC is distributed over vast areas of central Asia, Europe, and Xinjiang in China, one explanation for this observation is introgression. During its domestication, common carp has been intentionally introduced into different geographical locations, generating hybridization and genetic introgression during breeding (Wang et al., 2010; Dong et al., 2015). Moreover, it has been demonstrated that not only hybridization but also introgression was detected between European and Asian carp by mtDNA and allozyme markers (Kohlmann et al., 2003).
Effects of artificial selection on low genetic diversity of RY
In this study, we used one mitochondrial gene and the mitochondrial control region to estimate the genetic diversity and differentiation among and within strains. Notably, RY showed the lowest level of haplotype diversity (Hd = 0.559) and nucleotide diversity (π = 0.0011) while displaying a high degree of differentiation from the other four strains (Table 2). This result was consistent with our previous study that had revealed that the genetic diversity of selectively bred populations of RY was lower than in two landrace populations (Zhu et al., 2018). “RY NO. 1” is the first new variety of PF-carp approved in China, a strain that has been artificially selected for five generations based on desirable traits of rapid growth, a round body, and weak jumping ability. Researchers have revealed that consecutive breeding for many generations has led to decreased genetic diversity in golden mandarin fish (Siniperca scherzeri Steindachner) as revealed by microsatellite markers (Luo et al., 2015). Likewise, artificial selection is likely to have imposed distinctive signatures on Atlantic salmon (Salmo salar) characterized by reduction in genetic diversity and high genetic differentiation from other populations (Lopez et al., 2019). The effects of artificial selection practices on loss of genetic diversity have been reflected in other aquaculture species such as the Pacific abalone (Haliotis discus hannai) (Chen et al., 2017), the eastern oyster (Crassostrea virginica Gmelin) (Yu and Guo, 2004) and clam (Meretrix petechialis). Long-term mass selection increases the levels of inbreeding and co-ancestry, thereby decreasing the pool of genetic diversity (Rodriguez-Ramilo et al., 2015). Therefore, it is reasonable to believe that genetic diversity of RY has been eroded by continuous intense selection practices.
Genetic differentiation and diversity of PF-carp cultured under traditional rich-fish systems
Researchers have pointed out that vicariance and geographic isolation contribute to genetic differentiation. In our case, it was noteworthy that the three populations of the JBL strain (SJ, RSJ, and XR) exhibited significantly high differentiation. Similarly, both HJ and RS were significantly differentiated from other populations of the DTL strain (Supplementary Table 3). The common point shared by these five populations was that they all originated from remote mountainous areas that hindered genetic exchange with other populations and thus intensified differentiation.
In this study, indigenous PF-carp in these regions are managed in traditional rice-fish co-culture systems, where farmers maintain small numbers of parental carp and produce fry for themselves. Unlike crop seeds that can be stored and transmitted from a long distance (Pautasso et al., 2013), parental PF-carp used in rice-fish co-culture systems have to be continuously cultivated by farmers. Notably, farmer connectivity (parental carp exchange and fry transmission) is indispensable to promote gene flow and maintain a high level of genetic diversity (Ren et al., 2018). Considering inbreeding, artificial selection or lack of farmer connection would gradually degrade the genetic diversity (Kincaid, 1983; Ren et al., 2018), and thus it was no wonder that the nucleotide diversity values of RS (π = 0.0042), RSJ (π = 0.0035) and SJ (π = 0.0042) were relatively low compared to other populations.
By contrast, the populations of the WL strain exhibited a low degree of differentiation (Supplementary Table 5). The WL strain is the most widely farmed strain in southwestern China, and parental carp and fry are frequently exchanged. In traditional farming systems, seed exchange can help maintain genetic diversity (Deletre et al., 2011; Labeyrie et al., 2016; Ren et al., 2018). Farmers dynamically manage agricultural biodiversity by continually collecting, testing, and selecting seeds with unusual and interesting traits through exchanging germplasm with other farmers (Deletre et al., 2011).
Implications for management and conservation
Research has revealed how the genetic diversity of crops and livestock can be maintained in traditional agricultural systems (Jarvis et al., 2008; Deletre et al., 2011; Labeyrie et al., 2016). For example, farmers are typically exchanging seed material with neighbors, relatives, and distant farmers, moving crop genetic diversity across farming units.
Moreover, farming practices including different cultivation methods and the use of multiple varieties shaped by culture, economics, and religion have been demonstrated to help conserve genetic diversity (Berthouly et al., 2009; Boettcher and Hoffmann, 2011). According to Ren’s model, the genetic diversity (He) of PF-carp is positively related to the number of connected rice-fish farming farmer households and the frequency of exchange among these households (Ren et al., 2018). In this study, nucleotide diversity of RS, RSJ, and SJ was relatively low due to the lack of farmer connectivity. Therefore, it is necessary to encourage local farmers to share parental carp and fry to avoid genetic degradation and to maintain a dependable supply of genetically diverse fish. As for the geographically specific strains such as JBL and SJL, attention should be paid to preserve the native nucleotide diversity. On one hand, farmers should mind the intensity of selection and enhance communication with other RF-farmer households. On the other, farmers should watch out for introgression of exotic species. For example, we observed that CC genetic resources have invaded PF-carp in China, and this serves as a warning to trace the genetic origin of introduced common carp and to preserve native germplasm resources.
Data availability statement
The datasets presented in this study can be found in online repositories. The names of the repository/repositories and accession number(s) can be found below: https://www.ncbi.nlm.nih.gov/genbank/, OM933729—OM934252; https://www.ncbi.nlm.nih.gov/genbank/, OM934253—OM934776.
Ethics statement
The animal study was reviewed and approved by animal care and use committee of Pearl River Fisheries Research Institute, Chinese Academy of Fishery Sciences.
Author contributions
ZZ and DM conceived this work and designed the methodology. ZZ, JF, HS, and YL collected and analyzed the data. ZZ, DM, and HZ created the original draft. DM supervised the analysis and writing process. All authors contributed to the article and approved the submitted version.
Funding
This work was supported by the National Natural Science Foundation of China (32072971), the Science and Technology Program of Guangzhou, China (202002030370), Guangdong Provincial Special Fund for Modern Agriculture Industry Technology Innovation Teams (2019KJ150), and the Guangdong Provincial Special Fund For Technology (200901096270243).
Conflict of interest
The authors declare that the research was conducted in the absence of any commercial or financial relationships that could be construed as a potential conflict of interest.
Publisher’s note
All claims expressed in this article are solely those of the authors and do not necessarily represent those of their affiliated organizations, or those of the publisher, the editors and the reviewers. Any product that may be evaluated in this article, or claim that may be made by its manufacturer, is not guaranteed or endorsed by the publisher.
Supplementary material
The Supplementary Material for this article can be found online at: https://www.frontiersin.org/articles/10.3389/fevo.2022.896609/full#supplementary-material
Footnotes
References
Ahmed, N., and Garnett, S. T. (2011). Integrated rice-fish farming in Bangladesh: meeting the challenges of food security. Food Secur. 3, 81–92. doi: 10.1007/s12571-011-0113-8
Berg, H. (2001). Pesticide use in rice and rice–fish farms in the Mekong Delta, Vietnam. Crop Prot. 20, 897–905. doi: 10.1016/s0261-2194(01)00039-4
Berthouly, C., Do Ngoc, D., Thevenon, S., Bouchel, D., Nhu Van, T., Danes, C., et al. (2009). How does farmer connectivity influence livestock genetic structure? A case-study in a Vietnamese goat population. Mol. Ecol. 18, 3980–3991. doi: 10.1111/j.1365-294X.2009.04342.x
Boettcher, P. J., and Hoffmann, I. (2011). Protecting indigenous livestock diversity. Science 334, 1058–1058. doi: 10.1126/science.334.6059.1058-a
Chen, N., Luo, X., Lu, C., Ke, C., and You, W. (2017). Effects of artificial selection practices on loss of genetic diversity in the Pacific abalone, Haliotis discus hannai. Aquac. Res. 48, 4923–4933. doi: 10.1111/are.13311
Cheng, L., He, P., Wei, B., Lu, C., Wu, T., and Peng, J. (2021). Genetic structure of three populations of rice flower carp (Cyprinus carpio) in Guangxi ZhuangAutonomous Region based on mitochondrial d-loop region and cyt b gene. Acta Hydrobiol. Sin. 45, 54–59. doi: 10.7541/2021.2020.041
Deletre, M., McKey, D. B., and Hodkinson, T. R. (2011). Marriage exchanges, seed exchanges, and the dynamics of manioc diversity. Proc. Natl. Acad. Sci. U.S.A. 108, 18249–18254. doi: 10.1073/pnas.1106259108
Dong, C., Xu, J., Wang, B., Feng, J., Jeney, Z., Sun, X., et al. (2015). Phylogeny and Evolution of Multiple Common Carp (Cyprinus carpio L.) Populations Clarified by Phylogenetic Analysis Based on Complete Mitochondrial Genomes. Mar. Biotechnol. 17, 565–575. doi: 10.1007/s10126-015-9639-7
Excoffier, L., and Lischer, H. E. (2010). Arlequin suite ver 3.5: a new series of programs to perform population genetics analyses under Linux and Windows. Mol. Ecol. Resour. 10, 564–567. doi: 10.1111/j.1755-0998.2010.02847.x
Frei, M., and Becker, K. (2005). Integrated rice-fish culture: coupled production saves resources. Nat. Resour. Forum 29, 135–143. doi: 10.1111/j.1477-8947.2005.00122.x
Gan, W., Zhang, S., Wei, L., Huang, J., Mo, J., Gan, X., et al. (2019). Genetic Structure Analysis of Local Variety of Common Carp Cyprinus carpio var. Jinbian Farmed in Paddy Fields in Guangxi Region. Fish. Sci. 38, 636–646. doi: 10.16378/j.cnki.1003-1111.2019.05.008
Jarvis, D. I., Brown, A. H., Cuong, P. H., Collado-Panduro, L., Latournerie-Moreno, L., Gyawali, S., et al. (2008). A global perspective of the richness and evenness of traditional crop-variety diversity maintained by farming communities. Proc. Natl. Acad. Sci. U.S.A. 105, 5326–5331. doi: 10.1073/pnas.0800607105
Kincaid, H. L. (1983). Inbreeding in fish populations used for aquaculture. Aquaculture 33, 215–227. doi: 10.1016/0044-8486(83)90402-7Get
Kohlmann, K. (2015). The Natural History of Common Carp and Common Carp Genetics. Boca Raton: CRC Press, 3–26.
Kohlmann, K., Gross, R., Murakaeva, A., and Kersten, P. (2003). Genetic variability and structure of common carp (Cyprinus carpio) populations throughout the distribution range inferred from allozyme, microsatellite and mitochondrial DNA markers. Aquat. Living Resour. 16, 421–431. doi: 10.1016/S0990-7440(03)00082-2
Kumar, S., Stecher, G., Li, M., Knyaz, C., and Tamura, K. (2018). MEGA X: molecular evolutionary genetics analysis across computing platforms. Mol. Biol. Evol. 35:1547. doi: 10.1093/molbev/msy096
Labeyrie, V., Thomas, M., Muthamia, Z. K., and Leclerc, C. (2016). Seed exchange networks, ethnicity, and sorghum diversity. Proc. Natl. Acad. Sci. U.S.A. 113, 98–103. doi: 10.1073/pnas.1513238112
Leigh, J. W., and Bryant, D. (2015). popart: full-feature software for haplotype network construction. Methods Ecol. Evol. 6, 1110–1116. doi: 10.1111/2041-210X.12410
Lopez, M. E., Linderoth, T., Norris, A., Lhorente, J. P., Neira, R., and Yanez, J. M. (2019). Multiple Selection Signatures in Farmed Atlantic Salmon Adapted to Different Environments Across Hemispheres. Front. Genet. 10:901. doi: 10.3389/fgene.2019.00901
Luo, X. N., Yang, M., Liang, X. F., Jin, K., Lv, L. Y., Tian, C. X., et al. (2015). Genetic diversity and genetic structure of consecutive breeding generations of golden mandarin fish (Siniperca scherzeri Steindachner) using microsatellite markers. Genet. Mol. Res. 14, 11348–11355. doi: 10.4238/2015.September.25.1
Pan, X., Zhou, K., Chen, Z., Du, X., Huang, Y., Tan, J., et al. (2019). Genetic diversity and phylogenetic relationship among two populations of Procypris merus and wild carp based on mtDNA D-loop region and COI gene sequences. Freshw. Fish. 49, 33–41. doi: 10.13721/j.cnki.dsyy.2019.06.006
Pautasso, M., Aistara, G., Barnaud, A., Caillon, S., Clouvel, P., Coomes, O. T., et al. (2013). Seed exchange networks for agrobiodiversity conservation. A review. Agron. Sustain. Dev. 33, 151–175. doi: 10.1007/s13593-012-0089-6
Ren, W., Hu, L., Guo, L., Zhang, J., Tang, L., Zhang, E., et al. (2018). Preservation of the genetic diversity of a local common carp in the agricultural heritage rice-fish system. Proc. Natl. Acad. Sci. U.S.A. 115:E546–E554. doi: 10.1073/pnas.1709582115
Rodriguez-Ramilo, S. T., Garcia-Cortes, L. A., and de Cara, M. A. (2015). Artificial selection with traditional or genomic relationships: consequences in coancestry and genetic diversity. Front. Genet. 6:127. doi: 10.3389/fgene.2015.00127
Rozas, J., Ferrer-Mata, A., Sánchez-DelBarrio, J. C., Guirao-Rico, S., Librado, P., Ramos-Onsins, S. E., et al. (2017). DnaSP 6: DNA sequence polymorphism analysis of large data sets. Mol. Biol. Evol. 34, 3299–3302. doi: 10.1093/molbev/msx248
Shen, J., and Liu, M. (2000). Carp Breeding Research. Harbin: Heilongjiang Science and Technology Press.
Shohdy, K. S., and West, H. J. (2020). Circulating Tumor DNA Testing-Liquid Biopsy of a Cancer. JAMA Oncol. 6:792. doi: 10.1001/jamaoncol.2020.0346
Swindell, S. R., and Plasterer, T. N. (1997). Seqman. in Sequence Data Analysis Guidebook. Totowa: Springer, 75–89.
Wang, C., Liu, H., Liu, Z., Wang, J., Zou, J., and Li, X. (2010). Mitochondrial genetic diversity and gene flow of common carp from main river drainages in China. Freshw. Biol. 55, 1905–1915. doi: 10.1111/j.1365-2427.2010.02424.x
Wu, Q., and Gui, J. (1999). Fish Genetic and Breeding Engineering. Shanghai: Shanghai Science and Technology Press.
Xie, J., Hu, L., Tang, J., Wu, X., Li, N., Yuan, Y., et al. (2011). Ecological mechanisms underlying the sustainability of the agricultural heritage rice–fish coculture system. Proc. Natl. Acad. Sci. U.S.A. 108:E1381–E1387. doi: 10.1073/pnas.1111043108
Yan, X., Wei, L., Huang, J., Wang, J., Yang, Z., Gan, B., et al. (2020). Comparative skin transcriptome between common carp and the variety Jinbian carp (Cyprinus carpio v. jinbian). Aquac. Res. 51, 187–196. doi: 10.1111/are.14363
Yu, Z., and Guo, X. (2004). Genetic analysis of selected strains of eastern oyster (Crassostrea virginica Gmelin) using AFLP and microsatellite markers. Mar. Biotechnol. 6, 575–586. doi: 10.1007/s10126-004-3600-5
Zhou, J., Wu, Q., Wang, Z., and Ye, Y. (2004). Molecular phylogeny of three subspecies of common carp Cyprinus carpio, based on sequence analysis of cytochrome b and control region of mtDNA. J. Zoolog. Syst. Evol. Res. 42, 266–269. doi: 10.1111/j.1439-0469.2004.00266.x
Zhou, J., Wu, Q., Ye, Y., and Tong, J. (2003). Genetic divergence between Cyprinus carpio carpio and Cyprinus carpio haematopterus as assessed by mitochondrial DNA analysis, with emphasis on origin of European domestic carp. Genetica 119, 93–97. doi: 10.1023/a:1024421001015
Keywords: paddy field carp, genetic diversity, germplasm resource, farmer connectivity, introgression
Citation: Zhong Z, Fan J, Su H, Li Y, Ma D and Zhu H (2022) Genetic sources and diversity of the paddy field carp in the Pearl River basin inferred from two mitochondrial loci. Front. Ecol. Evol. 10:896609. doi: 10.3389/fevo.2022.896609
Received: 15 March 2022; Accepted: 30 June 2022;
Published: 28 July 2022.
Edited by:
Mikolaj Adamek, University of Veterinary Medicine Hannover, GermanyReviewed by:
Chengchi Fang, Institute of Hydrobiology (CAS), ChinaJingliang Kang, The University of Hong Kong, Hong Kong SAR, China
Wenjing Tao, Southwest University, China
Copyright © 2022 Zhong, Fan, Su, Li, Ma and Zhu. This is an open-access article distributed under the terms of the Creative Commons Attribution License (CC BY). The use, distribution or reproduction in other forums is permitted, provided the original author(s) and the copyright owner(s) are credited and that the original publication in this journal is cited, in accordance with accepted academic practice. No use, distribution or reproduction is permitted which does not comply with these terms.
*Correspondence: Dongmei Ma, bWRtQHByZnJpLmFjLmNu; Huaping Zhu, emhocGluZzIwMDBAMTYzLmNvbQ==