Predictors of Mammalian Diversity in the New York Metropolitan Area
- 1Department of Biology, Queens College, City University of New York, Queens, NY, United States
- 2Mianus River Gorge, Bedford, NY, United States
- 3American Museum of Natural History, New York, NY, United States
- 4Department of Natural Sciences, Mercy College, Bronx, NY, United States
Urbanization can have profound consequences for mammalian biodiversity and is thought to contribute to patterns of species richness and community composition. Large cities can be particularly challenging environments for mammals because these habitats are often impacted by anthropogenic perturbations, including high human population density, fragmented habitats, and extensive human development. In this study, we investigated mammalian species richness, Shannon–Wiener diversity, and evenness in the most densely populated region in the United States: the New York metropolitan area. Specifically, we deployed camera traps from 2015 to 2019 to investigate six drivers of mammalian diversity across 31 greenspaces: (1) human population density, (2) patch size, (3) habitat type, (4) surrounding land cover, (5) geographical barriers to dispersal, and (6) habitat heterogeneity. We found that mammal community composition is largely influenced by a multitude of anthropogenic factors. Specifically, mammal species richness was higher in greenspaces with larger patch sizes and lower in greenspaces surrounded by more development. Moreover, Shannon–Wiener diversity and evenness were higher in urban natural landscapes than human-altered landscapes. In a subset of data that only included carnivores, we found that carnivore Shannon–Wiener diversity was higher in urban natural habitats and in sites with lower human population densities. Finally, we found that geographical barriers to dispersal contributed to both patterns of mammalian diversity and patterns of carnivore diversity: mammal taxa richness, Shannon–Wiener diversity, and evenness were all significantly higher on the continent (Bronx/Westchester) than on Long Island. These results suggest that preserving urban greenspaces is important for maintaining both mammalian and carnivore biodiversity and that management of mammals in cities should concentrate on maintaining large, connected, natural greenspaces.
Introduction
As urbanization continues to spread throughout the world, its consequences, including increased human population growth, habitat fragmentation, and deforestation, are becoming increasingly important to examine and understand, especially in terms of biodiversity (McKinney, 2002; McDonald et al., 2008). Urbanization has had profound impacts on ecosystem function and is thought to be a major driver of biodiversity loss (Czech et al., 2000; Ray, 2000; Sala et al., 2000; Dobson et al., 2006; McCleery, 2010; Seto et al., 2012; Lewis et al., 2015). Mammals are sensitive to urbanization because their habitats within cities are often fragmented or replaced by human developments (Fernández and Simonetti, 2013; Łopucki and Kitowski, 2017). Carnivores are especially impacted by increased urbanization due to their large home ranges, human intolerance, limited diets, and low densities (Ordeñana et al., 2010; Stark et al., 2020). However, while urbanization is often associated with a lack of wildlife, recent studies have shown that species diversity, richness, and abundance are actually high in some urban greenspaces (Gehrt et al., 2009; Gallo et al., 2017; Matthies et al., 2017; Parsons et al., 2018). Indeed, some mammals, including coyotes (Canis latrans), raccoons (Procyon lotor), opossums (Didelphis virginiana), and foxes (e.g., Vulpes vulpes), are known as “urban adapters”, and have benefited from being in close proximity to developed areas reaping the benefits of increased food sources, decreased competition, and increased protection from large predators (McKinney, 2002; Adams and Lindsey, 2010; Gehrt et al., 2013; Lombardi et al., 2017). Understanding what factors influence patterns of mammalian community composition are important considerations in maintaining and protecting biodiversity in urban habitats. In this study, we used camera traps to investigate the impact of six drivers of mammalian diversity across greenspaces in the New York metropolitan area: (1) human population density (Magura et al., 2021), (2) patch size (Arrhenius, 1921), (3) habitat type (McDonnell and Pickett, 1990), (4) surrounding land cover (Cavia et al., 2009), (5) geographical barriers to dispersal (MacArthur and Wilson, 1967), and (6) habitat heterogeneity (Cornelis and Hermy, 2004).
At first glance it would seem human population density would negatively affect wildlife diversity, and indeed this is the case for many species and circumstances (Olifiers et al., 2005; Schipper et al., 2008; Stark et al., 2020). However, the impact of human population density on biodiversity is species-specific and scale-dependent (e.g., Prange et al., 2004; McCleery, 2010; Gehrt et al., 2013; Lombardi et al., 2017). On a global scale, as human population increases, more natural habitats are destroyed for residential, commercial, and agricultural use; mammals are forced from their habitats, and many do not survive (Lopes and Ferrari, 2000; Kasso and Bekele, 2014). Accordingly, several studies have reported a negative correlation between human population density and species richness (e.g., Gehrt and McGraw, 2007; Parsons et al., 2018; Stark et al., 2020). Another consequence of increased human population density is the occurrence of more hunting and culling in habitats once dominated by animals (Cullen et al., 2000). This is especially the case for large mammals that humans perceive as threats, such as coyotes (Nagy et al., 2017). Finally, although not as well-studied, vehicles have a major impact on mammalian diversity, in some years even greater than that of hunting (Forman and Alexander, 1998). Due to the abundance of roads located near forested areas, vehicular killings have contributed to overall mammal diversity loss in recent decades (Bashore et al., 1985; Forman and Alexander, 1998; Seiler, 2001; Shilling et al., 2021). While an inverse relationship between biodiversity and human population density has been documented in many circumstances, on a local scale, species richness and abundance of certain taxa, such as free-ranging cats (Felis catus), raccoons (P. lotor), and certain rodents, are actually higher in areas of high human population density (Luck, 2007; Gehrt et al., 2013; Lombardi et al., 2017). This may be largely attributed to the high availability of anthropogenic food sources in areas with high human population densities (Contesse et al., 2004; Prange et al., 2004; McCleery, 2010; Magura et al., 2021). Furthermore, close proximity to humans in urban landscapes has been shown to reduce predation pressures for small and medium size mammal species (Ditchkoff et al., 2006; McCleery, 2010). This is predominantly due to the displacement of large predators as a result of high human presence and activity in centrally populated areas (Muhly et al., 2011). When taking all these factors into consideration, densely populated cities typically have a net negative effect on mammalian species richness and especially pose a threat for larger mammals (Gehrt and McGraw, 2007; Parsons et al., 2018; Stark et al., 2020).
Patch size, defined as the arrangement and size of usable land area, have a major influence on patterns of mammalian diversity (Lombardi et al., 2017). In highly developed areas, increased urbanization often results in habitat fragmentation or isolation effects, which reduces the amount of available living space (Fahrig, 2003; Cavia et al., 2009; Nielsen et al., 2014) and separates larger populations into smaller subpopulations that, individually, are less viable. Some species of mammals are less likely to tolerate isolated patches of habitats that result from increased urbanization because they require more space to acquire vital resources (Etter et al., 2002; Prange et al., 2004; Moll et al., 2020). Accordingly, several studies have reported a positive association between patch size and species diversity (Saunders et al., 1991; Iida and Nakashizuka, 1995; Fahrig, 2003). Nielsen et al. (2014) found that a minimum of 10 hectares of patch size is necessary to ensure high species richness in urban greenspaces. However, some mammals, such as large carnivores, require an even greater amount of greenspace sometimes extending five square kilometers and beyond (Stark et al., 2020). Thus, mammalian diversity is largely defined by species-area effects whereby species richness is a function of patch size (MacArthur and Wilson, 1967; Hodgkison et al., 2007; Matthies et al., 2017).
The type of habitat might also affect patterns of mammalian diversity (Gallo et al., 2017). In urban areas, some greenspaces are dominated by human-altered habitats while other greenspaces are mainly comprised of urban natural habitats. Human-altered habitats include parks, which are characterized by manicured vegetation and high human activity (Nielsen et al., 2014); golf courses, which are comprised of large, semi-artificial greenspaces where human activity varies temporally (Boone et al., 2008); and cemeteries (Gallo et al., 2017). Some species can flourish in human-altered habitats (urban adapters), while other species cannot (urban avoiders) (McKinney, 2002, 2006). In contrast to human-altered habitats, urban natural habitats are typically composed of undeveloped woodlands with secondary growth forest (Vernon et al., 2014) situated within an urban or human-altered matrix. Natural areas within urban environments remain crucial in supporting mammal populations (Baker and Harris, 2007). Several studies have found that the presence of natural habitats within urban greenspaces allows for greater movement of multiple species (Radeloff et al., 2005; Gehrt et al., 2011; Bateman and Fleming, 2012; Scott et al., 2014).
The amount of developed land cover surrounding a habitat is another factor thought to be associated with mammalian diversity (Bierwagen, 2006; Cavia et al., 2009). Urban habitats often have decreased structural and functional connectivity between the highly developed cityscape and the less developed natural habitats within (LaPoint et al., 2015; Beninde et al., 2016). This reduced connectivity resulting from urbanization often leads to decreased distribution of smaller species (Zollner, 2000). Highly developed areas also have a high percent of impervious surface cover (i.e., roads, buildings, landscaping), and often have lower biodiversity than less developed areas (McKinney, 2008; Gallo et al., 2017). High impervious surface cover surrounding natural habitats like roads, buildings, and parking lots restrict home ranges and can lead to increased death by vehicle collision (Trombulak and Frissell, 2000; Seiler, 2001; Gehrt et al., 2013). These barriers to dispersal reduce gene flow and population viability relative to a connected metapopulation and can lead to decreased species richness (Bateman and Fleming, 2012). Mammals situated in habitats surrounded by highly developed land cover also experience high rates of habitat and resource disturbance (Blair and Launer, 1997) and the loss of natural vegetation to development (McKinney, 2008; McCleery, 2010). Together, these changes to habitats that encompass greenspaces place limitations on the species within the greenspace itself. Therefore, in order to maintain mammalian diversity, it is important to have patches of natural habitat and vegetation as well as increased connectivity between greenspaces and highly developed surrounding areas (Beninde et al., 2016; Gallo et al., 2017; Fidino et al., 2020).
Another factor that contributes to patterns of mammalian diversity is habitat heterogeneity. The habitat heterogeneity hypothesis proposes that the species diversity of an area will increase as the number of habitats increases (MacArthur and MacArthur, 1961). Hence, it is predicted that a greater number of habitats can offer more resources to a wider range of animals (Garden et al., 2007; Hodgkison et al., 2007; Matthies et al., 2017). In more heterogeneous habitats, there tend to be more habitat edges, creating more resources for supporting a greater number of mammals (Racey and Euler, 1982; Cramer and Willig, 2005). Accordingly, several studies of mammals have reported a positive association between habitat heterogeneity and species richness (Jepsen and Topping, 2004; Arques et al., 2014; Presley et al., 2019). Thus, urban greenspaces that have a variety of habitats are thought to be vital to mammalian diversity (Hodgkison et al., 2007).
Lastly, geographical barriers can also influence patterns of mammalian diversity. Specifically, islands that are bounded by water create barriers to dispersal for many species (Weckel et al., 2015). Hice and Schmidly (2002) found that continental regions exhibit higher species diversity than island regions mainly because they do not require the crossing of harsh waterways or busy bridges for colonization. Some mammals, like coyotes (Harrison, 1992) and raccoons (Prange et al., 2004), can cross these barriers and move between the mainland and the island, which has been observed in major cities like New York (Weckel et al., 2015; Henger et al., 2020) and San Francisco (Sacks et al., 2006). Nonetheless, the intersection of urbanization and geographical barriers to dispersal are thought to lead to reduced mammal richness and evenness among urban habitats (Ekernas and Mertes, 2006; Weckel et al., 2015).
Our goal was to determine predictors of mammalian diversity in various greenspaces across the New York metropolitan area. To do so, we deployed camera traps across 31 study sites (Figure 1 and Table 1). We hypothesized that mammal community composition is influenced by a multitude of anthropogenic factors including human population density (Magura et al., 2021), patch size (Arrhenius, 1921), habitat type (McDonnell and Pickett, 1990), surrounding land cover (Cavia et al., 2009), geographical barriers to dispersal (MacArthur and Wilson, 1967), and habitat heterogeneity (Cornelis and Hermy, 2004). Specifically, we predicted that mammalian diversity would be higher in study sites where there were lower human population densities, larger patch sizes, urban natural habitats, less developed land cover surrounding the core habitat, and more heterogenous habitats. Because bodies of water are important geographical barriers to dispersal, we also predicted that mammal diversity would be greater in the mainland (Bronx/Westchester) than in Long Island, Manhattan, and Randall’s Island. Identifying and understanding what factors influence patterns of mammalian diversity is critical to maintaining both mammalian populations and overall biodiversity.
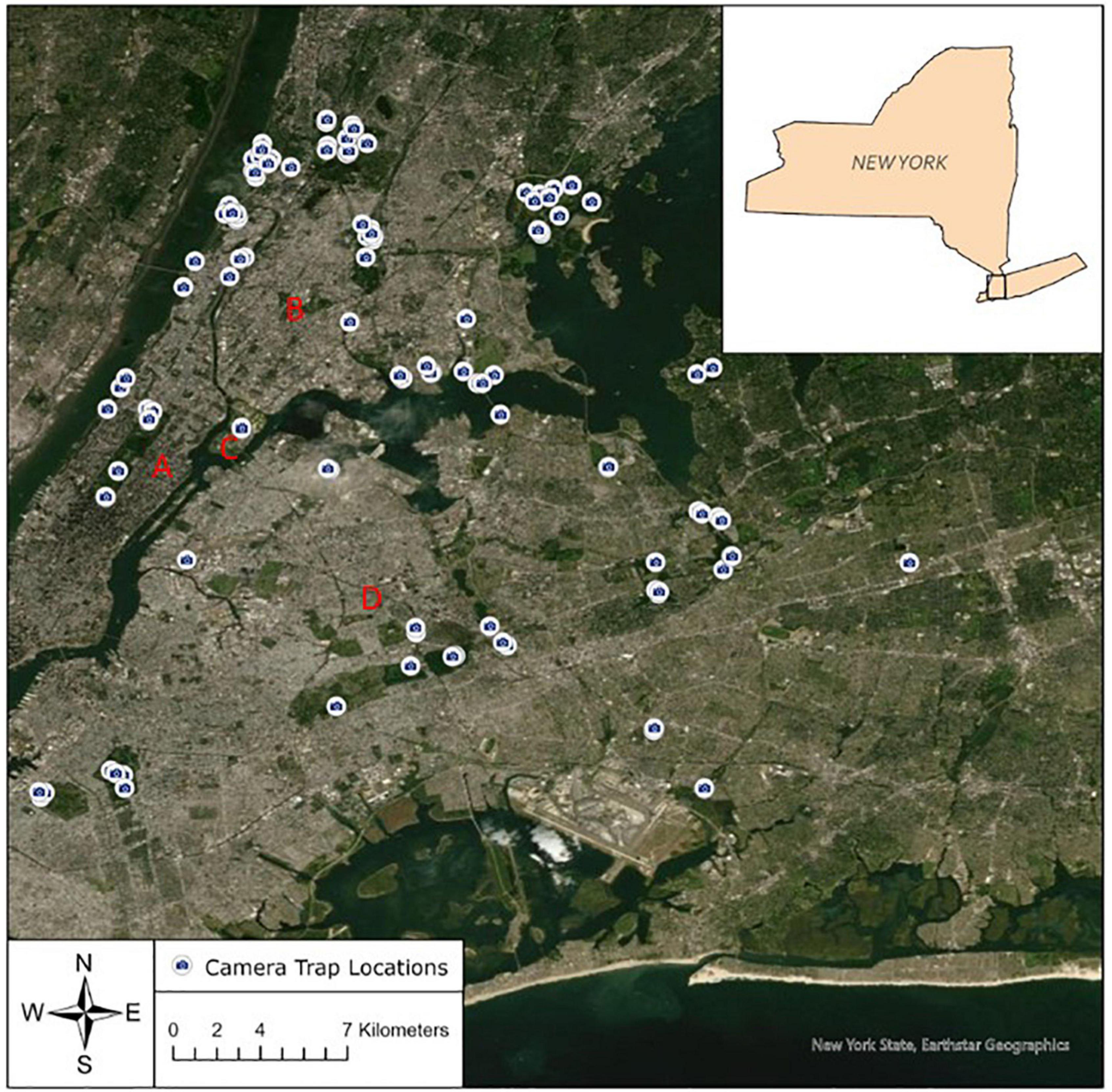
Figure 1. Map of camera locations across 31 greenspaces in which mammals were surveyed in the New York metropolitan area, 2015–2019. A = Manhattan; B = Mainland (Bronx/Westchester); C = Randall’s Island; D = Long Island.
Materials and Methods
Field Sites
This research focused on mammalian diversity across various greenspaces in the New York metropolitan Area. Our study sites included 31 greenspaces throughout four geographical regions: (1) Long Island; (2) mainland (Bronx and Westchester); (3) Manhattan; and (4) Randall’s Island (Supplementary Table 1). Of the 31 sites, 16 were located in Long Island, nine in the mainland, five in Manhattan, and one in Randall’s Island. Within each of these regions, there were various types of greenspaces. Of the 16 sites on Long Island, one was a baseball field, one was a golf course, one was a ranch/farm, two were cemeteries, and the remaining eleven sites were urban parks. Of the nine mainland sites, all contained natural vegetation with one site also containing multiple recreational and tourist facilities. In Manhattan, four of the five sites were parks consisting mainly of natural vegetation, while the fifth site was a more developed urban park containing a mixture of natural vegetation and human-altered habitats. The Randall’s Island site was comprised of a combination of open areas, natural vegetation, and human made recreational facilities. Each of the greenspaces contained one or more cameras to monitor wildlife and predict patterns of mammalian diversity.
Camera Surveys
Camera traps were deployed across 31 sites in the New York metropolitan area from December 2015 to June 2019 following the guidelines described in Nagy et al. (2016). Except for small rodents, which we classified at the family level (family Muridae), we identified all mammals to the species level using photographs captured by each camera trap. Throughout the five-year course of this study, three types of Reconyx cameras were used: RC55, PC800, and HC500 motion- and heat-activated camera traps (Reconyx, Inc., Holmen, WI, United States). Each of these cameras had 0.2 s trigger speed, 1080p resolution, and comparable infrared flash ranges (RC55 and HC500: 15 m, PC800: 21 m). There were two models of the RC55 used, with the older one having a 5.0 × 7.6 cm red/infrared flash primarily for night pictures, and the newer one with a single LED bulb for a “semi-covert” infrared flash. Using ArcGIS 9 and ArcGIS10 (ESRI, Redlands, CA, United States), random locations were selected for camera deployment in the different greenspaces with at least 500 m distance between each camera. Exact survey dates varied year-to-year but generally cameras were deployed either year-round or more commonly twice a year, first in early January through mid-April and again June through August. These twice-annual deployments allowed for the detection of seasonally-active, hibernating mammals (e.g., chipmunk: Tamias striatus; groundhogs: Marmota monax) and the detection of new coyote breeding sites in the winter—i.e., when occupancy across the landscape is highest as dispersers establish new territories and pairs form (Nagy et al., 2016). At each location, cameras were deployed in a substantial patch of forest or undeveloped area. Because we had a limited number of camera traps, in cases in which a study area consistently surveyed the same mammals, we shifted camera traps to new locations within a greenspace to increase coverage. Many of the cameras required relocation due to theft, legal ramifications, or necessity for a better-suited placement. Each of these relocations were at least within ∼50 m of the originally planned locations. The actual number of cameras per greenspace varied, however, the density of cameras in most cases was at least one camera per 0.5 km2 in each of the greenspaces (Table 1). Across the five years of the study, the cameras were typically placed in the same general areas unless there were natural disasters or theft that previously influenced the camera placement.
Predictors of Mammalian Diversity
To gain better insight into what predicts mammalian diversity across an urban landscape, we modeled three response variables: (1) richness, defined as the number of different mammal taxa present in a given greenspace or region (Dorji et al., 2014); (2) evenness, defined as the abundance equality of mammal taxa in a specific greenspace or region (Dorji et al., 2014); and (3) Shannon–Wiener diversity, defined as the proportional abundance of each mammal taxon from the total sample of individuals within the given surveyed community (Ekernas and Mertes, 2006). We conducted two analyses, one that included all mammal taxa identified in our camera trap surveys, and another that excluded humans and domestic dogs. In cases in which both analyses yielded the same best supported model, we report the results including all mammal taxa. In cases in which the best supported model differed between analyses, we report two sets of results, one including all mammal taxa and another excluding humans and domestic dogs. We included free-ranging cats in both analyses because these can include feral cats as well as free-roaming cats possibly subsidized by human foods (Elizondo and Loss, 2016).
For our analysis of interest—predictors of mammalian diversity in the greenspaces of the New York metropolitan area—we modeled six predictor variables (Table 2): (1) human population density; (2) patch area; (3) habitat heterogeneity; (4) type of habitat; (5) percent developed land cover; and (6) region. We also included cameras per square kilometer in our models to control for variation in camera deployment. The number of cameras deployed at each site ranged from one to sixteen with a mean of 4.45 cameras per site (SD ± 4.01). Sampling effort ranged from 50 camera trap days to 2,279 camera trap days (SD ± 478).
1. Human population density. To measure human population density, we used United States Zip Code data1 to ascertain the number of individuals per square mile in the zip code in which the study site was located and then we converted these data to the number of individuals per square kilometer. In cases in which study sites were situated in more than one zip code, we calculated the mean human population density of the zip codes surrounding the study site.
2. Patch size. We calculated patch size using the ‘‘measure distance’’ tool on Google Maps.2
3. Habitat type. We classified study sites into two main categories based on habitat type. If a greenspace was comprised of greater than 50% secondary growth forest, we classified this habitat as urban natural (Gallo et al., 2017). On the other hand, if a greenspace was comprised of greater than 50% manicured lawns, athletic fields, playgrounds, and golf courses, we classified this habitat as human-altered (Gallo et al., 2017).
4. Percent developed land cover. ArcGIS Pro 2.6 and the National Land Cover Database3 were used to calculate percent developed land cover at each of the 31 greenspaces. We constructed buffers of 500 m to assess whether developed land cover in the immediate habitat impacted mammalian diversity (e.g., Gallo et al., 2017) and buffers of 1,000 m to assess whether land use surrounding a greenspace impacted mammalian diversity (e.g., Gomes et al., 2011). In cases in which the best supported model yielded the same results regardless of spatial scale, we report and discuss the model results based on land cover within 500 m (Gallo et al., 2017). Following Callaghan et al. (2019); Stark et al. (2020), and Goldstein et al. (2022), we combined the percent land cover of three different NLCD classes (“developed, low intensity”; “developed, medium intensity”; and “developed, high intensity”) into a superclass called “developed land cover.” Developed land cover included areas with a mixture of both constructed materials and vegetation, and where impervious surface cover accounted for at least 20 percent of the land cover.
5. Habitat heterogeneity. To calculate habitat heterogeneity, we counted the number of different habitat types based on 15 categories provided by the National Land Cover Database (Supplementary Table 2). We calculated habitat heterogeneity at two spatial scales: buffers of 500 and 1,000 m surrounding each of the 31 greenspaces. As with percent developed land cover, in cases in which the best supported model yielded the same results regardless of spatial scale, we report and discuss the model results based on land cover within 500 m (Gallo et al., 2017).
6. Region. We classified region into four categories: (1) Long Island; (2) Manhattan; (3) mainland (Bronx and Westchester); and (4) Randall’s Island.
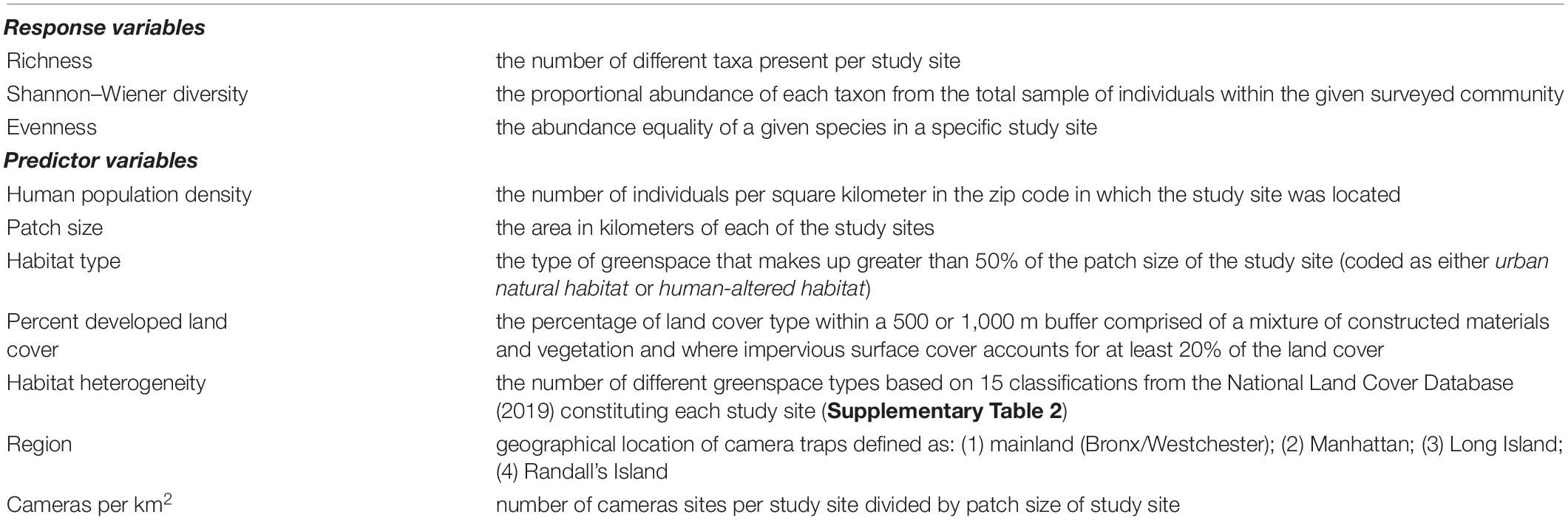
Table 2. Response and predictor variables for mammalian diversity among 31 greenspaces in the New York metropolitan area.
Statistical Analyses
All statistical analyses were completed using the R Project for Statistical Computing.4 To calculate measures of biodiversity, we used the R package vegan.5 First, we created a species accumulation curve of the 31 sampling sites to ensure adequate sampling effort (Supplementary Figure 1). Second, we calculated alpha diversity (mammal taxa richness) of each study site and gamma diversity (total mammal taxa richness) across all study sites. Third, we used capture frequencies of each mammalian taxon as a proxy for relative abundance (Pardo et al., 2018; Knowlton et al., 2019). To calculate relative abundance, we used the following calculation: number of independent photographs ÷ sampling effort × 100. Sampling effort was calculated as the sum of the number of active camera days. Independent photographs were calculated by counting the maximum number of individuals from the same taxon in the same photograph during hourly intervals. Only detections of the same taxon taken at durations greater than 60 minutes were considered as independent (Cusack et al., 2015; Lewis et al., 2015; Stark et al., 2020). Lastly, we calculated Shannon–Wiener diversity and evenness using the diversity function in vegan.
We performed multiple linear regressions to test for predictors of mammal taxa richness, Shannon–Wiener diversity, and evenness. Because carnivores might be more sensitive to the impacts of urbanization (Woodroffe, 2000; Crooks, 2002; Šálek et al., 2014), we also performed multiple linear regressions to detect patterns of species richness, Shannon–Wiener diversity, and evenness with a subset of the data that only included carnivores. For these analyses, we classified carnivores as a functional feeding group (Stark et al., 2020). Therefore, carnivores included all species from the order Carnivora as well as the Virginia opossum (D. virginiana), a marsupial that behaves functionally as a carnivore (Stark et al., 2020).
To perform all multiple linear regression models, we used the stats package in R. All predictor variables and response variables are described in Table 2. Because measures of richness incorporated count data, we modeled these response variables using the glm function with Poisson error distribution. Because Shannon diversity indices are strictly positive and continuous, we modeled Shannon diversity using the glm function with Gamma error distribution. Finally, we modeled evenness using the lm function and tested for model assumptions using the gvlma package (Peña and Slate, 2006). We found no violations of any of the model assumptions of linear models.
For all analyses, we performed multiple comparison tests of all possible parameter combinations using the MuMIn package,6 and we used Akaike information criterion (AIC) to select the best fitting models (Burnham and Anderson, 2004). In cases in which more than one model yielded an AICc difference of <2, we performed model averaging using the summed weight method (Burnham and Anderson, 2004; Grueber et al., 2011). The model-averaged coefficients were calculated using conditional R2 (Nakagawa and Schielzeth, 2013).
Lastly, to detect for multicollinearity, we calculated generalized variance inflation factors (GVIFs) for model predictor variables using the package car (Fox and Weisberg, 2011). We found no evidence of problematic multicollinearity as all GVIFs were <2.5 (Fox, 2015).
Results
Mammalian Taxa Richness, Diversity, and Evenness
Across the 31 study sites that were sampled, we observed a total of 15 mammal taxa including 8 carnivore species (Table 3 and Supplementary Table 3). A species accumulation curve indicated that there was adequate sampling effort (Soberón and Llorente, 1993; Supplementary Figure 1). Measures of diversity (mean ± SD) varied across study sites. Across all mammals, taxa richness ranged from five taxa at both Starlight Park and Willow Lake to a maximum of 13 taxa at Riverdale Park (8.23 ± 2.43; Table 4). Shannon–Wiener diversity ranged from 0.703 at Ridgewood Highland to 1.788 at Van Cortlandt Park (1.26 ± 0.289; Table 4). Evenness ranged from 0.392 at Ridgewood Highland to 0.856 at Railroad Park (0.61 ± 0.12; Table 4). The three most common mammal taxa were free-ranging cats (F. catus), gray squirrels (Sciurus carolinensis), and raccoons (P. lotor); these were found at all 31 study sites. The most common carnivores were free-ranging cats (100% of study sites), raccoons (100% of study sites), and opossums (D. virginiana; 87% of study sites) (Supplementary Table 3). The three rarest taxa were mink (Mustela vison; 3% of study sites; n = 1 study site), groundhogs (M. monax; 19% of study sites; n = 6 study sites), and white-tailed deer (Odocoileus virginianus; 19% of study sites; n = 6 study sites). Carnivore species richness ranged from three species at Highbridge Park, Ridgewood Park, and Starlight Park to seven species at Inwood Hill Park, Pelham Bay Park, and Riverdale Park (4.58 ± 2.12). Carnivore Shannon–Wiener diversity ranged from 0.14 at Central Park to 1.32 at Ferry Point Park (0.71 ± 0.26). Carnivore evenness ranged from 0.13 at Central Park to 0.81 at Railroad Park (0.47 ± 0.02). The parks with the lowest diversity were mainly comprised of free-ranging cats, gray squirrels, humans, raccoons, small rodents (family Muridae), and opossums (Supplementary Table 3).
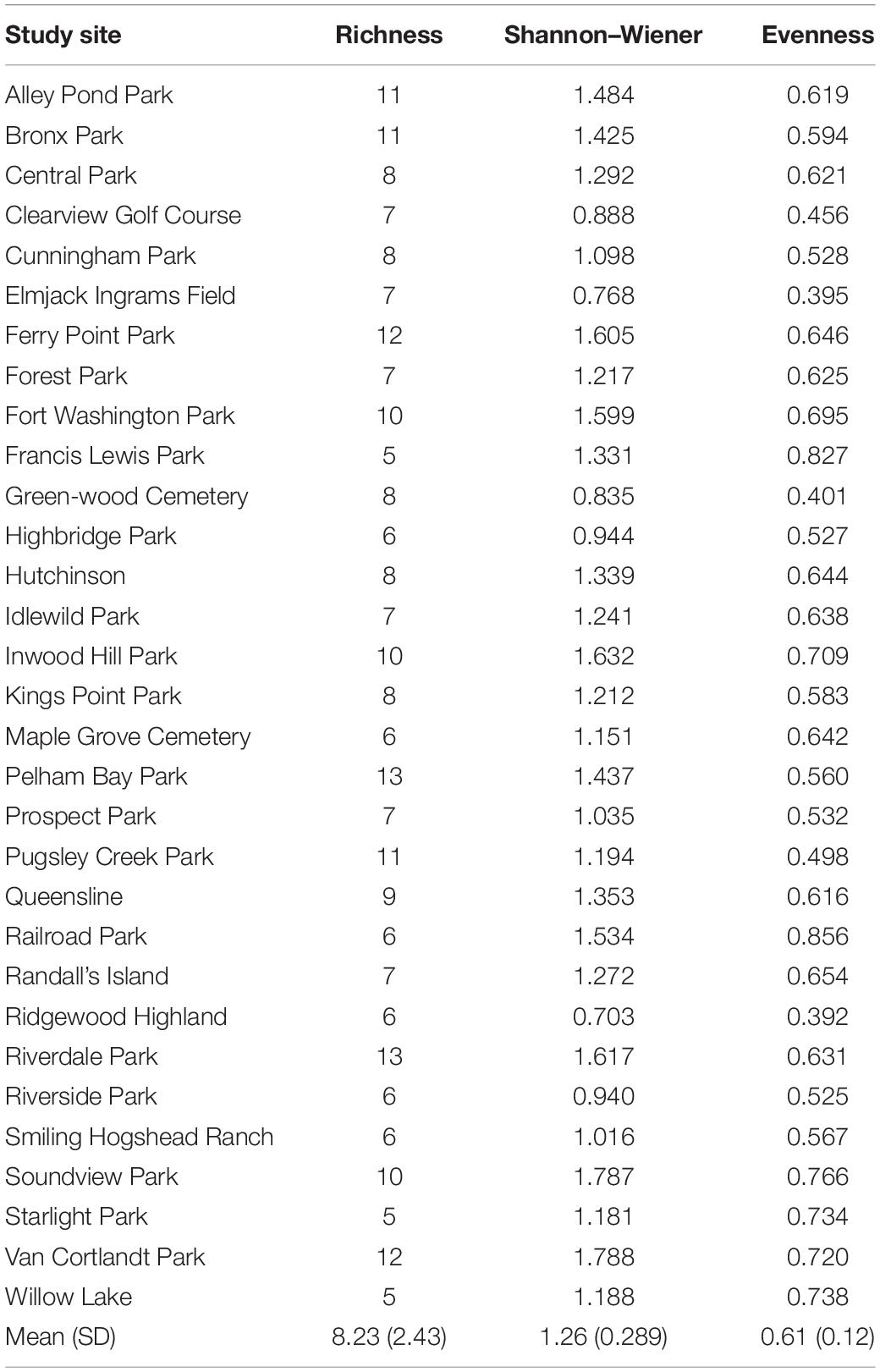
Table 4. Average mammal taxa richness, Shannon Wiener diversity, and evenness for each of the 31 study sites.
Predictors of Mammalian Taxa Richness
Based on model averages, we found that patch size and region were significant predictors of mammalian taxa richness at a scale of 500 m. Specifically, study sites with larger patch sizes harbored significantly more mammal taxa than study sites with smaller patch sizes (β = 0.109; 95% CI: 1.594–2.578; p = 0.046; Figure 2A and Table 5). Moreover, mammal taxa richness was lower in Long Island than on the mainland (Bronx and Westchester) (β = –0.408; 95% CI: –6.938 to –1.213; p = 0.005; Figure 2B and Table 5). However, at a scale of 1000 m, the effect of patch size and region on mammalian species richness was masked by the percent developed land cover surrounding urban greenspaces. Specifically, greenspaces surrounded by more developed land cover harbored significantly lower mammalian taxa richness than greenspaces surrounded by less developed land cover (β = –0.006; 95% CI: –0.012 to –0.0003; p = 0.038; Figure 2C and Table 5).
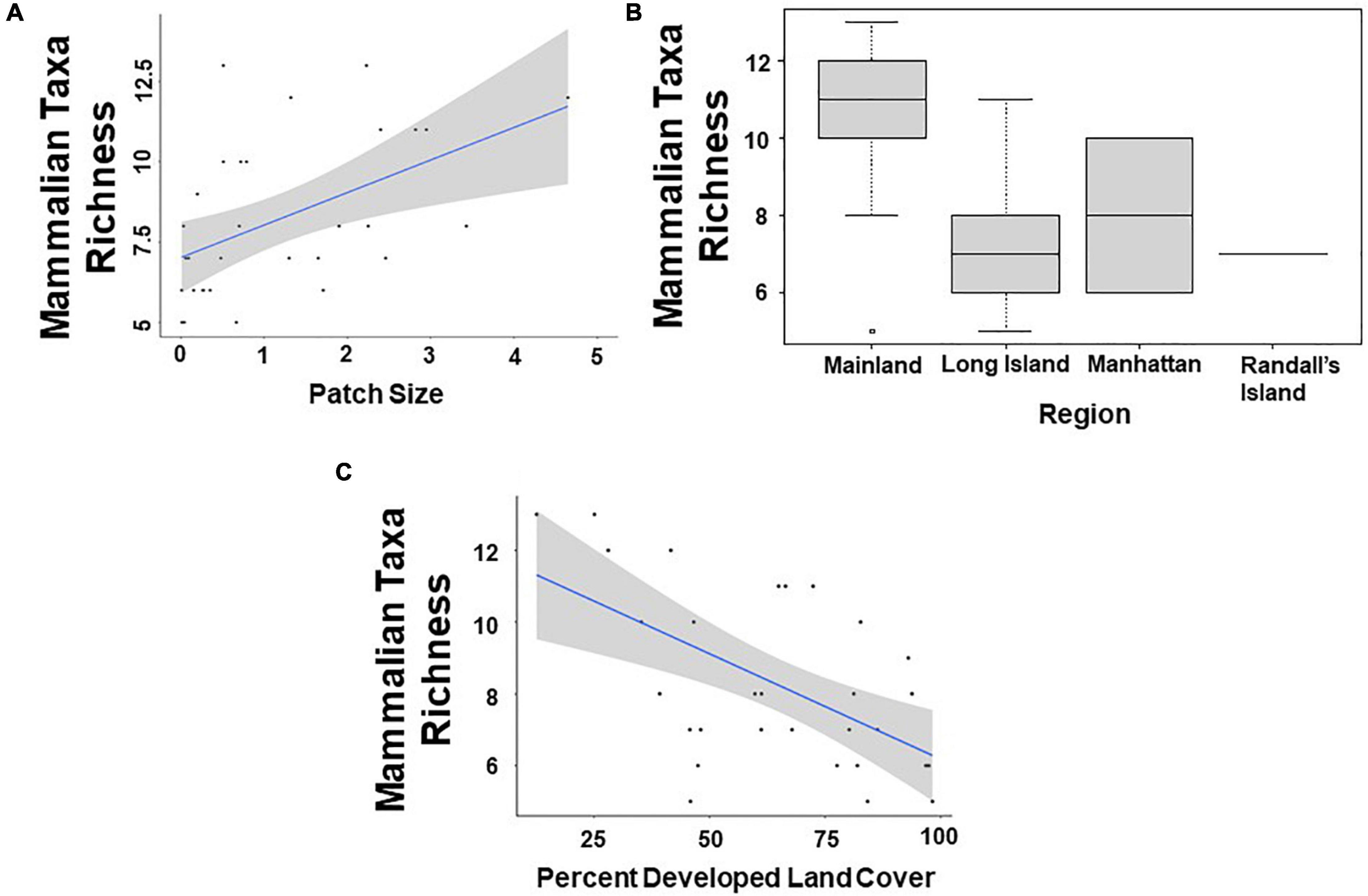
Figure 2. Patterns among mammalian taxa richness and (A) patch size (km2); (B) region; and (C) percent developed land cover (1,000 m scale) surrounding the 31 study sites.
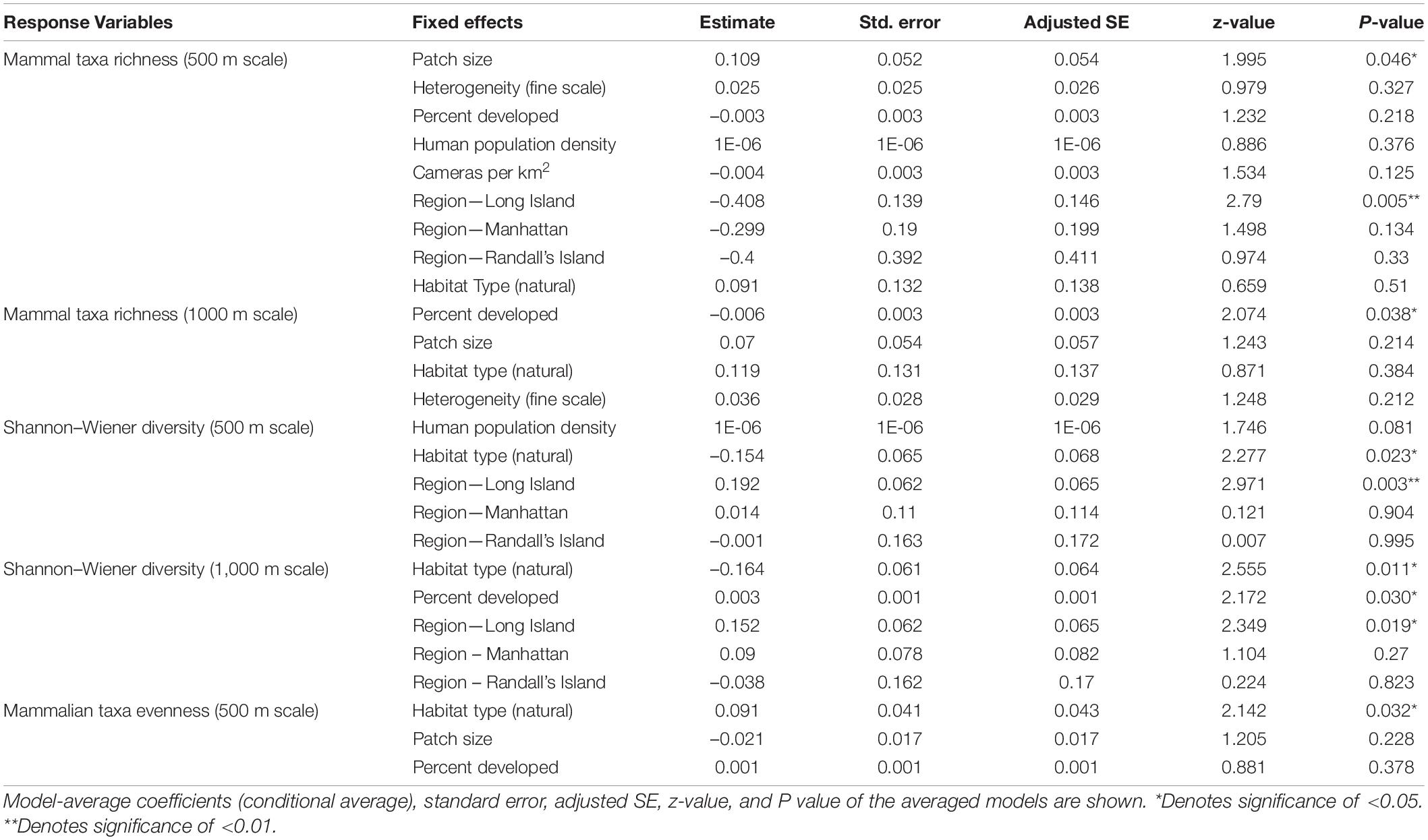
Table 5. Best supported models for each response variable for mammalian diversity based on averaging of parameter estimates.
Predictors of Mammalian Shannon–Wiener Diversity
Across all mammal taxa, there were two model parameters that best predicted Shannon–Wiener Diversity at the scale of 500 m: habitat type and region. Specifically, Shannon–Wiener diversity was significantly higher in greenspaces where the dominant habitat type was an urban natural habitat rather than a human altered habitat (β = –0.154; 95% CI: –2.860 to –2.138; p = 0.023; Figure 3A and Table 5) and on the mainland (Bronx and Westchester) than Long Island (β = 0.192; 95% CI: 6.546 to 3.193; p = 0.003; Figure 3B and Table 5). When we excluded humans and domestic dogs from our analysis, at a scale of 500 m, one additional model parameter best predicted Shannon–Wiener diversity: human population density. Specifically, Shannon–Wiener diversity was significantly higher in greenspaces with lower human population densities than in greenspaces with higher human population densities (β = 0.000002; 95% CI: 0.0000002–0.000005; p = 0.035). At a scale of 1000 m, greenspaces surrounded by less developed land cover exhibited higher Shannon–Wiener diversity indices than greenspaces surrounded by more developed land cover (β = 0.003; 95% CI: 0.0003–0.005; p = 0.030; Figure 3C and Table 5).
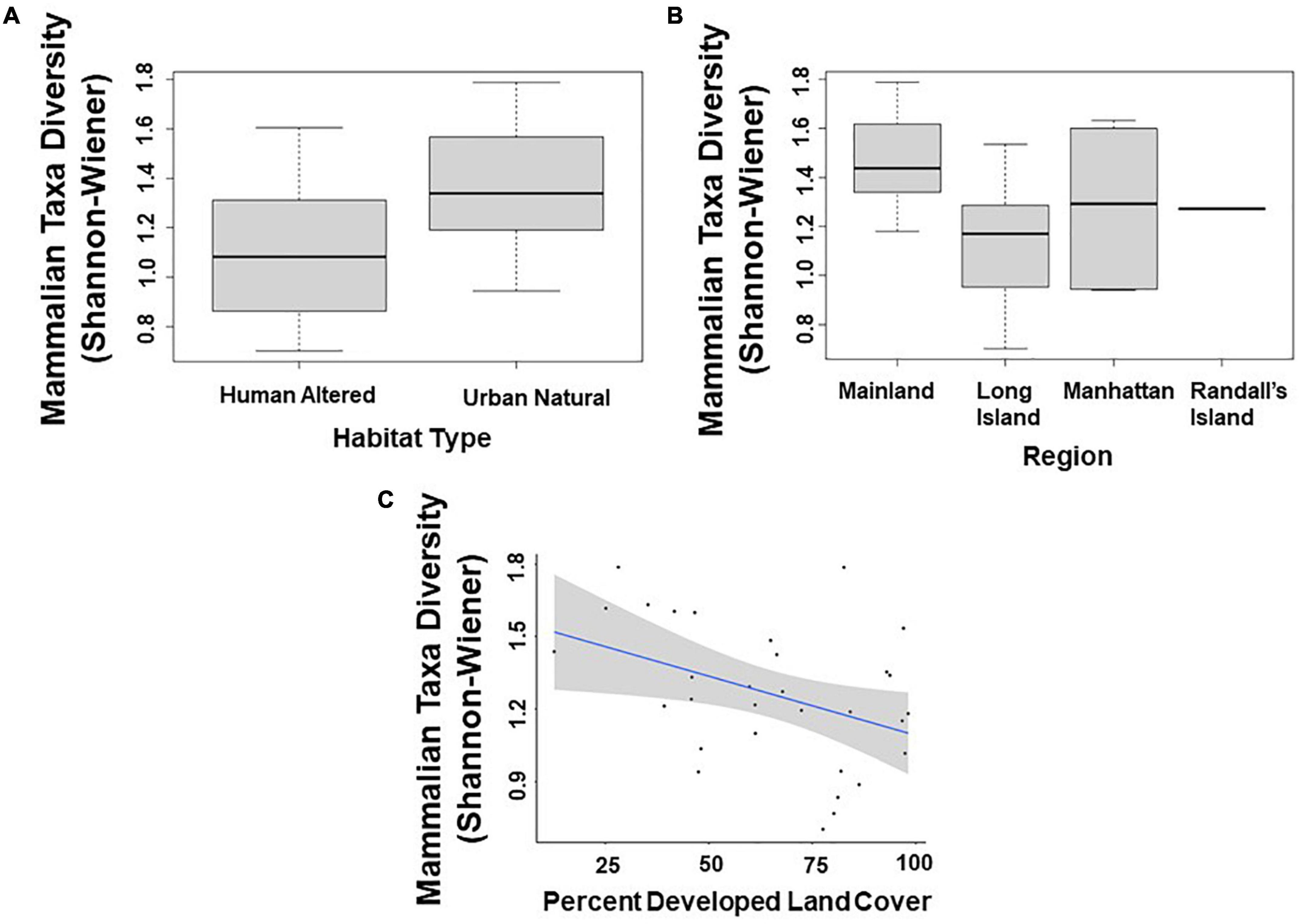
Figure 3. Patterns among mammalian taxa diversity (Shannon–Wiener index) and (A) type of habitat; (B) region; and (C) percent developed land cover (1,000 m scale) across the 31 study sites. Points and whiskers on the plot represent the mean and standard error.
Predictors of Mammalian Taxa Evenness
Across all mammal taxa, the model parameter that best predicted evenness across the 31 study sites was habitat type. Specifically, evenness was significantly higher in study sites where the dominant habitat type was an urban natural habitat than study sites where the dominant habitat type was a human-altered habitat (β = 0.091; 95% CI: 0.008–0.175; p = 0.032; Figure 4 and Table 5). However, habitat type was not a significant predictor of evenness when we excluded domestic dogs and humans from the analysis.
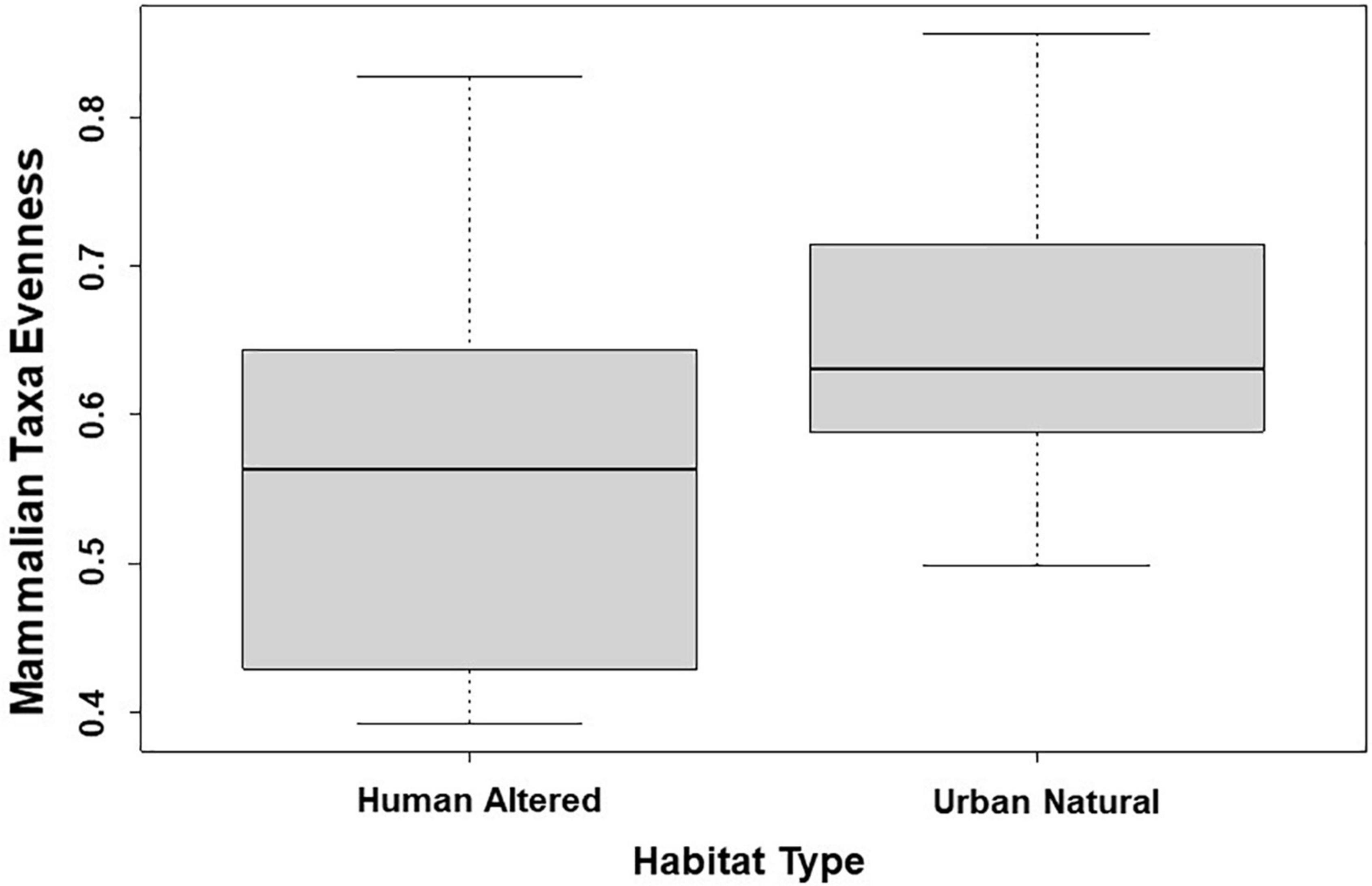
Figure 4. Patterns among mammalian taxa evenness and type of habitat across 31 study sites. Points and whiskers on the plot represent the mean and standard error.
Predictors of Carnivore Diversity
In analyses that specifically focused on carnivores, there were no significant predictors of carnivore species richness. Carnivore Shannon–Wiener diversity was higher in greenspaces with lower human population densities than in greenspaces with higher human population densities (β = 0.00001; 95% CI: 3.3 e-06–1.6 e-05; p = 0.007; Figure 5A and Table 6). Carnivore Shannon–Wiener diversity was also significantly higher on the mainland (Bronx and Westchester) than Long Island (β = 0.777; 95% CI: 3.438–1.225; p = 0.002; Figure 5B and Table 6). Further, Shannon–Wiener diversity of carnivores was higher in greenspaces where the dominant habitat type was urban natural rather than human altered (β = –0.528; 95% CI: –1.065 to –5.737; p = 0.049; Figure 5C and Table 6). Finally, the best model parameter for predicting carnivore evenness was region. Specifically, evenness was significantly lower in Long Island than on the mainland (β = –0.203; 95% CI: –3.411 to –6.5678; p = 0.004; Figure 6 and Table 7).
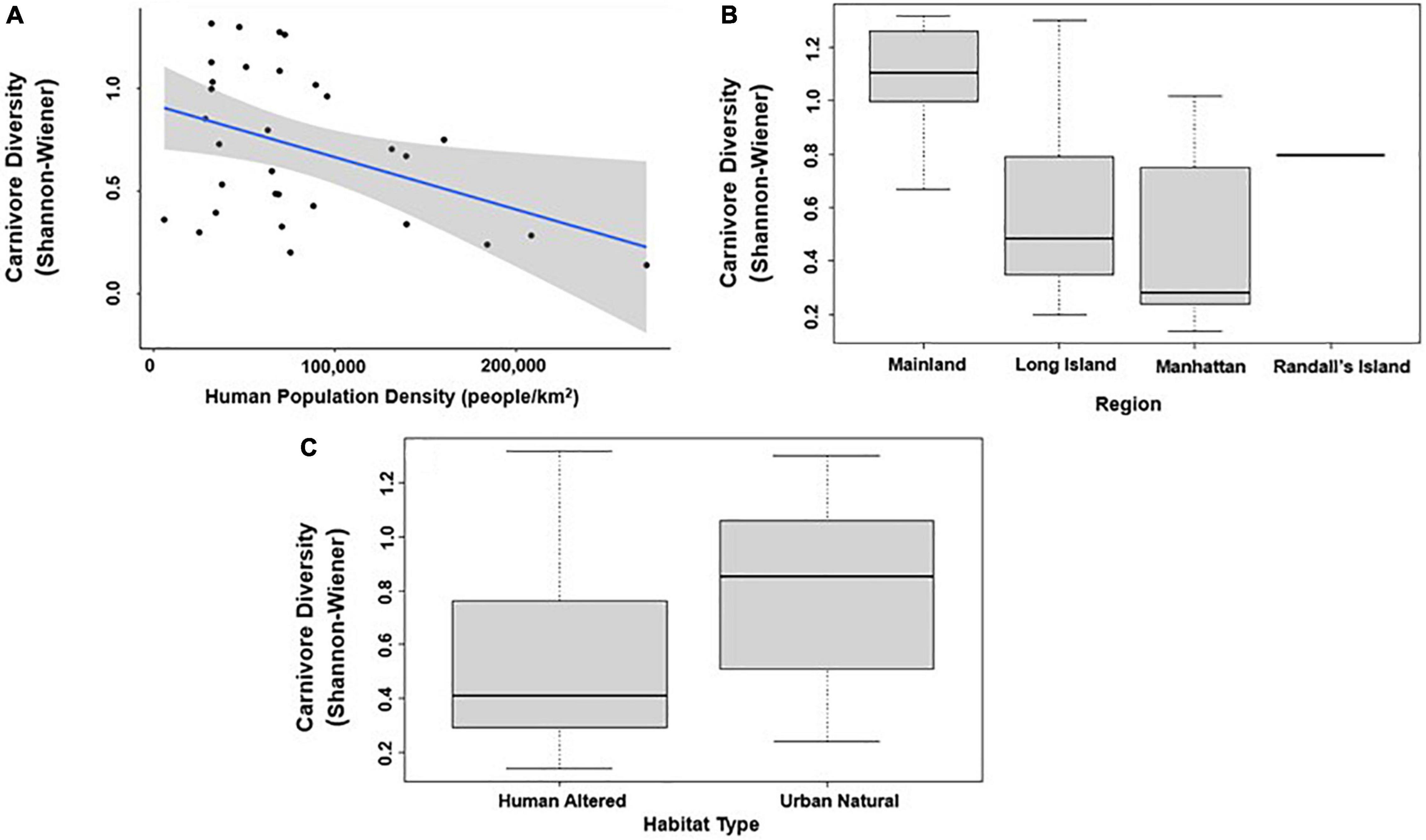
Figure 5. Patterns among Carnivore taxa diversity (Shannon–Wiener index) and (A) human population density; (B) region; and (C) habitat type across the 31 study sites. Points and whiskers on the plot represent the mean and standard error.

Table 6. Best supported model for Carnivore Shannon–Wiener diversity (500 m scale) based on averaging of parameter estimates.
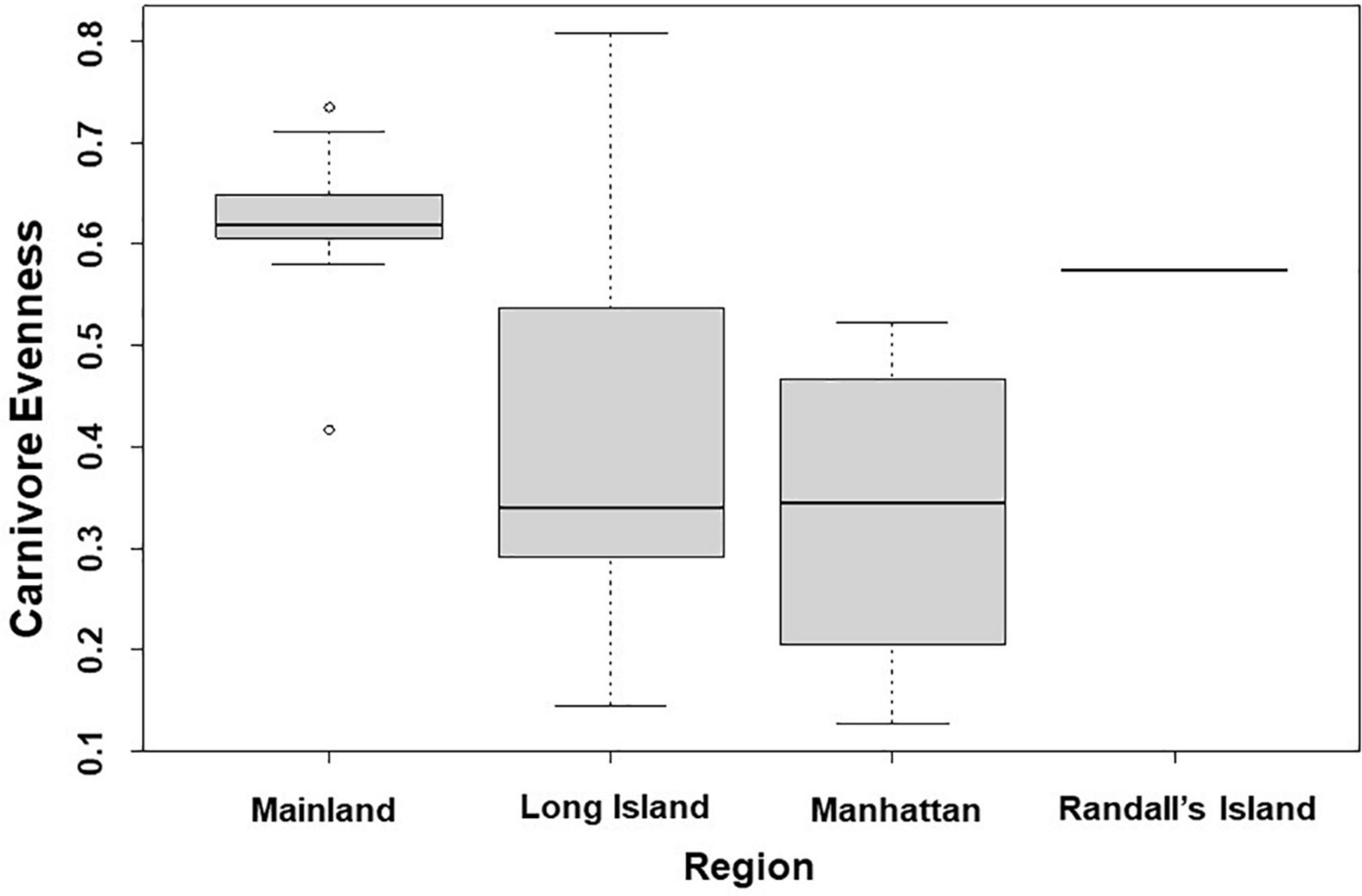
Figure 6. Patterns among Carnivore evenness and region across the 31 study sites. Points and whiskers on the plot represent the mean and standard error.
Discussion
In this investigation of predictors of mammalian diversity in the New York metropolitan area, we found ample support for the hypothesis that mammalian diversity is associated with anthropogenic factors and geographical barriers. Consistent with our predictions, we found that mammal taxa richness was higher in greenspaces with larger patch sizes and less developed land cover suggesting that species richness is a function of habitat area (Arrhenius, 1921). Moreover, mammal taxa richness and Shannon–Wiener diversity were greater on the continent (Bronx/Westchester) than Long Island suggesting that there are barriers to dispersal that limit the movement of species between regions (Weckel et al., 2015). Further, both Shannon–Wiener diversity and evenness were higher in urban natural habitats than human-altered habitats. Measures of carnivore diversity were also associated with anthropogenic factors and geographical barriers. Among carnivores, Shannon–Wiener diversity was significantly lower in greenspaces with higher human population densities and in human-altered habitats. Further, both Shannon–Wiener diversity and evenness were significantly higher among carnivores on the mainland (Bronx/Westchester) than in Long Island. Collectively, these results provide insights into the drivers of mammalian community composition and are helpful for drawing inferences on what factors contribute to mammalian diversity in urban environments.
Throughout the greenspaces of the New York metropolitan area, some mammalian taxa were more common than others. Three species were identified in all 31 study sites: free-ranging cats, gray squirrels, and raccoons. In addition to these three species, the greenspaces that exhibited low taxa diversity also tended to harbor small rodents (family Muridae) and opossums. The five taxa found at these low diversity sites—free-ranging cats, gray squirrels, raccoons, small rodents (family Muridae), and opossums—are known as urban adapters (McKinney, 2002; Adams and Lindsey, 2010; Gehrt et al., 2013; Lombardi et al., 2017). This is largely because these mammals have the capacity to exploit both urban greenspaces while also supplementing their diets with anthropogenic food sources (Cove et al., 2018; Guiry and Buckley, 2018; Nicholson and Cove, 2022). One of these urban adapters, free-ranging cats, is associated with several negative impacts, including the local extinction of native wildlife and the spread of certain pathogens (Gehrt et al., 2013).
In contrast to these animals, three mammalian species were rare among the greenspaces of the New York metropolitan area: minks, groundhogs, and white-tailed deer. Minks were identified in only one of the 31 greenspaces. This is probably because minks require wetland habitats and tend to reside in freshwater streams and rivers (Allen, 1984). Most of our study sites were not situated in wetland habitats, which might explain why the only mink identified was located in a greenspace adjacent to the Hudson River. Previous studies indicate that minks are typically found in sparsely populated rural areas and only occasionally in urban settings (Silva-Rodríguez et al., 2020). White-tailed deer were identified in six of the 31 greenspaces surveyed in this study. Five of these greenspaces were located on the mainland; one was located on Long Island. These findings suggest that the roads and waterways that separate the mainland from the islands make it particularly challenging for large-bodied mammals such as deer to disperse to highly urbanized islands with high human population densities (Michael, 1965; Long et al., 2010). Like deer, groundhogs were also identified in six of the 31 greenspaces surveyed in this study. Surveys indicate that both white-tailed deer and groundhogs are more likely to reside in rural and suburban habitats; however, both of these species have increasingly become more abundant in urban greenspaces in recent years (Gaughan and Destefano, 2005; Lehrer and Schooley, 2010). These findings suggest that minks, groundhogs, and white-tailed deer are urban avoiders (McKinney, 2002), but still have the capacity to exploit some urban habitats. Our findings suggest that urban greenspaces can be refuges for many mammalian taxa, especially urban adapters; however, in some cases, even urban avoiders can make use of certain urban greenspaces.
We found support for the idea that mammalian diversity is characterized by species-area effects. In support of this idea, patch size was positively correlated with species richness. This result is consistent with other studies that have also reported higher mammal taxa richness in greenspaces with relatively larger patch sizes (Yates et al., 1997; Hodgkison et al., 2007; Nielsen et al., 2014). These results also lend support to the species-area hypothesis, the idea that species richness is a function of the amount of available habitat (Arrhenius, 1921; Turner et al., 2005). In the case of the current study, we find evidence of the species-area effect in an urban context.
Habitat type was a significant predictor of two measures of biodiversity: Shannon–Wiener diversity and evenness. First, we found that Shannon–Wiener diversity was higher in study sites where the dominant habitat was an urban natural habitat rather than a human-altered habitat. Although some mammals may thrive in human-altered habitats, like golf courses and cemeteries (Gallo et al., 2017; Wurth et al., 2020), most mammals tend to have higher persistence in urban natural areas, or habitats that consist of large patches of secondary growth forest and shrubs (Atwood et al., 2004; Baker and Harris, 2007). It has also been found that manicured lawns and artificial nocturnal illumination, as seen in parks, golf courses, and cemeteries, may limit dispersal (Mahan and O’Connell, 2005; Fitzgibbon et al., 2007). Second, we also found a positive correlation between habitat type and evenness. Specifically, we found that evenness was higher in study sites where more than fifty percent of the patch area was secondary growth forest. These findings are consistent with previous research that suggest that diversity in urban natural habitats is higher than in human-altered habitats (Parsons et al., 2018). Taken together, these two findings—higher Shannon–Wiener diversity and higher taxa evenness among mammals in urban natural habitats—suggest that it is important for park managers to maintain at least 50% natural area within urban parks to support mammalian diversity.
Consistent with our predictions, we found that greenspaces surrounded by more developed land cover exhibited lower mammal taxa richness than greenspaces surrounded by less developed land cover. This result was only significant at a scale of 1000 meters, but at this scale, it masked the effects of any of the other predictor variables. This finding appears to contradict the urban refugia hypothesis (Stark et al., 2020), which suggests that urban greenspaces in highly developed areas are oases for biodiversity. More specifically, our results differ from the findings of a recent study in the New York metropolitan area that found support for the urban refugia hypothesis: Stark et al. (2020) found that the relative abundance of mammalian carnivores was higher in greenspaces with higher surrounding human development. In our analysis of mammalian carnivores, we found no significant relationship between human development and carnivore species richness. However, the Stark et al. (2020) study was conducted in nature preserves outside of New York City and in less populous areas than the current study. Specifically, the mean (± SD) human population density for the Stark et al. (2020) study was 7,305 (± 11,222) humans per square kilometer while the mean human population density in the current study was 81,451 (± 60,515) humans per square kilometer. Perhaps in highly urbanized, densely populated areas, a minimum amount of greenspace is required for urban greenspaces to function as refugia for mammals, especially carnivores. Another factor that might explain our result is that in highly developed areas, buildings, roads, and other anthropogenic structures reduce connectivity between habitats (LaPoint et al., 2015; Beninde et al., 2016). Obstruction in connectivity can also lead to resource depletion, habitat disturbance, and ultimately reduced species richness (Blair and Launer, 1997; Fidino et al., 2020). Additionally, our result might also be attributed to higher mortality rates caused by humans, including vehicle collisions (Trombulak and Frissell, 2000; Seiler, 2001; Collins and Kays, 2011) and poisoning (Brooks et al., 2020). Overall, our results suggest that highly developed areas pose a particularly daunting challenge for maintaining high levels of mammalian diversity.
Urban greenspaces located on the mainland (Bronx and Westchester) harbored greater mammal taxa richness and Shannon–Wiener diversity than urban greenspaces located on urban islands (Long Island, Manhattan, Randall’s Island). These findings suggest that geographical barriers to dispersal might impact patterns of mammalian diversity. In support of this idea, previous studies have documented multiple barriers to dispersal between the mainland (Bronx and Westchester) and the other three regions (Long Island, Manhattan, and Randall’s Island) (Weckel et al., 2015; Nagy et al., 2016). Additionally, roads and waterways, as well as other barriers, play a large role in limiting dispersal (Oxley et al., 1974; Merriam et al., 1989; Baker and Harris, 2007). For example, coyotes have not established strong breeding populations yet in Long Island mainly because of bridges and rivers that separate the island from the Bronx and Westchester (Nagy et al., 2017). Our results lend support to the hypothesis that islands, even those in close proximity to the continent, can serve as barriers to dispersal.
The New York metropolitan area harbors several carnivore species that vary in their abundance and distribution. We found that the greenspaces surveyed in this study supported one or more of the following carnivores: coyotes (C. latrans), free-ranging cats (F. catus), domestic dogs (Canis familiaris), minks (M. vison), opossums (D. virginiana), raccoons (P. lotor), red foxes (V. vulpes), and striped skunks (Mephitis mephitis). Because of their low densities, need for large habitat area, and conflict with humans, many large carnivores once native to this region, including black bears (Ursus americanus), bobcats (Lynx rufus), fishers (Pekania pennanti), and long-tailed weasels (Neogale franata) were not observed in any of the greenspaces surveyed in this study, although these mammals persist in nature preserves outside of New York City (Spinola et al., 2008; Stark et al., 2020; Nagy, unpublished data). In the present study, we found that Shannon–Wiener diversity among carnivores was higher in urban natural habitats and in greenspaces with lower human population densities. In support of these findings, previous studies have found that many carnivores tend to avoid human interaction, preferring less developed urban habitats with lower human population densities (Grinder and Krausman, 2001; George and Crooks, 2006; Gehrt et al., 2009). We also found that carnivore evenness was significantly higher in the mainland than in sites located in Long Island and Manhattan. This finding suggests that there might be differences in the habitat characteristics between the mainland sites (Bronx and Westchester) and the island sites (Manhattan and Long Island) including differences in floral diversity (Ekernas and Mertes, 2006), resource availability (Matthies et al., 2017), the historical range of various species (e.g., Rooney, 2001; Hody and Kays, 2018), and management strategies (McPhearson et al., 2016). Furthermore, the asymmetric distribution of carnivore communities on the islands might also be explained by barriers to dispersal between the mainland and island sites. Although large mammals, like carnivores, are more adept at crossing bridges and waterways than smaller mammals, these barriers still hinder and reduce the likelihood of movement between regions (Weckel et al., 2015; Henger et al., 2020). Collectively, these findings suggest that carnivores are particularly sensitive to urbanization and barriers to dispersal such as bridges and large bodies of water.
Because we did not trap or tag species and relied solely on camera data, one limitation of this study was that we were unable to identify individuals. Therefore, the abundance indices that we calculated are rough estimates and may not be representative of actual mammal abundances. In future studies, the use of mark-recapture or identification techniques to assess individual sightings would ensure a more accurate representation of abundance (Ekernas and Mertes, 2006; Lewis et al., 2015; Lombardi et al., 2017). The current study surveyed sixteen sites on Long Island, thirteen of which were located in Queens, two in Brooklyn, and one in Nassau County. Since the majority of the Long Island study sites were located in Queens, the results may not be representative of mammal diversity for all of the island. For future studies, it would be beneficial to have a more even distribution of study sites across the island to allow for more accurate comparison of regions.
Conclusion and Future Directions
Our findings suggest that anthropogenic variables, such as percent developed land cover, patch size, and habitat type, influence patterns of mammalian diversity, and that it is crucial to understand and study the consequences of increasing urbanization on mammalian communities. There were some mammals in the current study that appeared to flourish across all urban greenspaces (i.e., urban exploiters) and were less sensitive to urbanization, apparently because they can take better advantage of anthropogenic food resources. On a more nuanced level, our findings suggest that there are some habitat features that are more suitable for mammals that are sensitive to urbanization (i.e., urban avoiders), including large patch sizes and high proportions of secondary growth forest. Hence, while urbanization is generally detrimental to biodiversity, we found that there were greenspaces in the New York metropolitan area where mammalian diversity was high. This suggests that there is a potential to make cities more suitable habitats for many mammalian species and that a barren ecology is not inevitable. The current study provides correlative evidence that some features of urbanization are associated with patterns of mammalian biodiversity. Our findings might be helpful in terms of management and conservation efforts within similarly large urban centers. Based on our results, we recommend that planners and managers of cities focus their efforts on maintaining large, connected, natural greenspaces, especially when surrounded by highly developed areas with high human population densities. Urban ecological research would benefit from longitudinal studies conducted across multiple large cities that can document changes in diversity, abundance, and evenness of mammals.
Data Availability Statement
The original contributions presented in this study are included in the article/Supplementary Material, further inquiries can be directed to the corresponding author.
Ethics Statement
Ethical review and approval was not required for the animal study because this was an observational study using photographs.
Author Contributions
AB, CN, MW, DL, and BH designed the study. CN and MW collected the data. AB and BH analyzed the data. AB, DL, and BH wrote the manuscript. All authors contributed to the article and approved the submitted version.
Conflict of Interest
The authors declare that the research was conducted in the absence of any commercial or financial relationships that could be construed as a potential conflict of interest.
Publisher’s Note
All claims expressed in this article are solely those of the authors and do not necessarily represent those of their affiliated organizations, or those of the publisher, the editors and the reviewers. Any product that may be evaluated in this article, or claim that may be made by its manufacturer, is not guaranteed or endorsed by the publisher.
Acknowledgments
We thank Larissa Swedell and John Waldman for providing commentary on previous drafts of this manuscript. We also thank Amanda Goldstein for providing help in constructing maps and calculating percent land cover for this study.
Supplementary Material
The Supplementary Material for this article can be found online at: https://www.frontiersin.org/articles/10.3389/fevo.2022.903211/full#supplementary-material
Footnotes
- ^ https://www.unitedstateszipcodes.org/
- ^ https://www.google.com/maps
- ^ https://www.mrlc.gov/data/legends/national-land-cover-database-2019-nlcd2019-legend
- ^ https://www.R-project.org/
- ^ http://vegan.r-forge.r-project.org/
- ^ https://r-forge.r-project.org/projects/mumin/
References
Allen, A. W. (1984). Habitat Suitability Index Models: Mink. FWS/OBS-82/10.61 Revised. Washington, DC: U.S. Fish and Wildlife Service, 19.
Arques, J., Belda, A., Peiró, V., Seva, E., and Martínez-Pérez, J. E. (2014). Main ecological gradients and landscape matrix affect wild rabbit Oryctolagus cuniculus (Linnaeus, 1758) (Mammalia: Leporidae) abundance in a coastal region (South-East Spain). Ital. J. Zool. 81, 440–450. doi: 10.1080/11250003.2014.9226
Atwood, T. C., Weeks, H. P., and Gehring, T. M. (2004). Spatial ecology of coyotes along a suburban-to-rural gradient. J. Wildlife Manage. 68, 1000–1009.
Baker, P. J., and Harris, S. (2007). Urban mammals: what does the future hold? An analysis of the factors affecting patterns of use of residential gardens in Great Britain. Mammal. Rev. 37, 297–315. doi: 10.1111/j.1365-2907.2007.00102.x
Bashore, T., Tzilkowski, W., and Bellis, E. (1985). Analysis of deer-vehicle collision sites in Pennsylvania. J. Wildlife Manage. 49, 769–774. doi: 10.2307/3801709
Bateman, P., and Fleming, P. (2012). Big city life: carnivores in urban environments. J. Zool. 287, 1–23. doi: 10.1111/j.1469-7998.2011.00887.x
Beninde, J., Feldmeier, S., Werner, M., Peroverde, D., Schulte, U., Hochkirch, A., et al. (2016). Cityscape genetics: structural vs. functional connectivity of an urban lizard population. Mol. Ecol. 25, 4984–5000. doi: 10.1111/mec.13810
Bierwagen, B. G. (2006). Connectivity in urbanizing landscapes: the importance of habitat configuration, urban area size, and dispersal. Urban Ecosyst. 10, 29–42. doi: 10.1007/s11252-006-0011-6
Blair, R. B., and Launer, A. E. (1997). Butterfly diversity and human land use: species assemblages along an urban gradient. Biol. Conserv. 80, 113–125. doi: 10.1016/S0006-3207(96)00056-0
Boone, M. D., Semlitsch, R. D., and Mosby, C. (2008). Suitability of golf course ponds for amphibian metamorphosis when bullfrogs are removed. Conserv. Biol. 22, 172–179. doi: 10.1111/j.1523-1739.2007.00817.x
Brooks, J., Kays, R., and Hare, B. (2020). Coyotes living near cities are bolder: implications for dog evolution and human-wildlife conflict. Behaviour 157, 289–313. doi: 10.1163/1568539x-bja10002
Burnham, K. P., and Anderson, D. R. (2004). Multimodel inference: understanding AIC and BIC in model selection. Sociol. Method Res. 33, 261–304. doi: 10.1177/0049124104268644
Callaghan, C. T., Bino, G., Major, R. E., Martin, J. M., Lyons, M. B., and Kingsford, R. T. (2019). Heterogeneous urban green areas are bird diversity hotspots: insights using continental-scale citizen science data. Landsc. Ecol. 34, 1231–1246. doi: 10.1007/s10980-019-00851-6
Cavia, R., Cueto, G. R., and Suárez, O. V. (2009). Changes in rodent communities according to the landscape structure in an urban ecosystem. Landsc. Urban Plan. 90, 11–19. doi: 10.1016/j.landurbplan.2008.10.017
Collins, C., and Kays, R. (2011). Causes of mortality in North American populations of large and medium-sized mammals. Anim. Conserv. 14, 474–483. doi: 10.1111/j.1469-1795.2011.00458.x
Contesse, P., Hegglin, D., Gloor, S., Bontadina, F., and Deplazes, P. (2004). The diet of urban foxes (Vulpes vulpes) and the availability of anthropogenic food in the city of Zurich, Switzerland. Mamm. Biol. 69, 81–95. doi: 10.1078/1616-5047-00123
Cornelis, J., and Hermy, M. (2004). Biodiversity relationships in urban and suburban parks in Flanders. Landsc. Urban Plan. 69, 385–401. doi: 10.1016/j.landurbplan.2003.10.038
Cove, M. V., Gardner, B., Simons, T. R., Kays, R., and O’Connell, A. F. (2018). Free-ranging domestic cats (Felis catus) on public lands: estimating density, activity, and diet in the Florida keys. Biol. Invasions 20, 333–344. doi: 10.1007/s10530-017-1534-x
Cramer, M., and Willig, M. (2005). Habitat heterogeneity, species diversity and null models. Oikos 108, 209–218. doi: 10.1111/j.0030-1299.2005.12944.x
Crooks, K. R. (2002). Relative sensitivities of mammalian carnivores to habitat fragmentation. Conserv. Biol. 16, 488–502. doi: 10.1046/j.1523-1739.2002.00386.x
Cullen, L., Bodmer, R. E., and Valladares-Padua, C. (2000). Effects of hunting in habitat fragments of the Atlantic forests, Brazil. Biol. Conserv. 95, 49–56. doi: 10.1016/s0006-3207(00)00011-2
Cusack, J. J., Dickman, A. J., Rowcliffe, J. M., Carbone, C., Macdonald, D. W., and Coulson, T. (2015). Random versus game trail-based camera trap placement strategy for monitoring terrestrial mammal communities. PLoS One 10:e0126373. doi: 10.1371/journal.pone.01263
Czech, B., Krausman, P. R., and Devers, P. K. (2000). Economic associations among causes of species endangerment in the United States. Bioscience 50, 593–601.
Ditchkoff, S. S., Saalfeld, S. T., and Gibson, C. J. (2006). Animal behavior in urban ecosystems: modifications due to human-induced stress. Urban Ecosyst. 9, 5–12. doi: 10.1007/s11252-006-3262-3
Dobson, A., Lodge, D., Alder, J., Cumming, G. S., Keymer, J., McGlade, J., et al. (2006). Habitat loss, trophic collapse, and the decline of ecosystem services. Ecology 87, 1915–1924. doi: 10.1890/0012-9658(2006)87[1915:hltcat]2.0.co;2
Dorji, T., Moe, R. S., Klein, J. A., and Totland, O. (2014). Plant species richness, evenness, and composition along environmental gradients in an alpine meadow grazing ecosystem in Central Tibet, China. Arct. Antarct. Alp. Res. 46, 308–326. doi: 10.1657/1938-4246-46.2.308
Ekernas, L. S., and Mertes, K. J. (2006). The Influence of Urbanization, Patch Size, and Habitat Type on Small Mammal Communities in the New York Metropolitan Region. New York, NY: WildMetro.
Elizondo, E. C., and Loss, S. R. (2016). Using trail cameras to estimate free-ranging domestic cat abundance in urban areas. Wildlife Biol. 22, 246–252. doi: 10.2981/wlb.00237
Etter, D. R., Hollis, K. M., Deelen, T. R. V., Ludwig, D. R., Chelsvig, J. E., Anchor, C. L., et al. (2002). Survival and movements of white-tailed deer in suburban Chicago, Illinois. J. Wildlife Manage. 66, 500–510. doi: 10.2307/3803183
Fahrig, L. (2003). Effects of habitat fragmentation on biodiversity. Annu. Rev. Ecol. Evol. Syst. 34, 487–515. doi: 10.1146/annurev.ecolsys.34.011802.132419
Fernández, I. C., and Simonetti, J. A. (2013). Small mammal assemblages in fragmented shrublands of urban areas of Central Chile. Urban Ecosyst. 16, 377–387. doi: 10.1007/s11252-012-0272-1
Fidino, M., Gallo, T., Lehrer, E. W., Murray, M. H., Kay, C., Sander, H. A., et al. (2020). Landscape-scale differences among cities alter common species’ responses to urbanization. Ecol. Appl. 31:2. doi: 10.1002/eap.2253
Fitzgibbon, S. I., Putland, D. A., and Goldizen, A. W. (2007). The importance of functional connectivity in the conservation of a ground-dwelling mammals in an urban Australian landscape. Landsc. Ecol. 22, 1513–1525. doi: 10.1007/s10980-007-9139-x
Forman, R., and Alexander, L. (1998). Roads and their major ecological effects. Annu. Rev. Ecol. Evol. Syst. 29, 207–231. doi: 10.1146/annurev.ecolsys.29.1.207
Gallo, T., Fidino, M., Lehrer, E. W., and Magle, S. B. (2017). Mammal diversity and metacommunity dynamics in urban green spaces: implications for urban wildlife conservation. Ecol. Appl. 27, 2330–2341. doi: 10.1002/eap.1611
Garden, J. G., McAlpine, C. A., Possingham, H. P., and Jones, D. N. (2007). Habitat structure is more important than vegetation composition for local-level management of native terrestrial reptile and small mammal species living in urban remnants: a case study from Brisbane, Australia. Austral. Ecol. 32, 669–685. doi: 10.1111/j.1442-9993.2007.01750.x
Gaughan, C. R., and Destefano, S. (2005). Collaboration for community-based wildlife management. Urban Ecosyst. 8, 191–202. doi: 10.1007/s11252-005-3265-5
Gehrt, S. D., Anchor, C., and White, L. (2009). Home range and landscape use of coyotes in a metropolitan landscape: conflict or coexistence? J. Mammal. 90, 1045–1057. doi: 10.1644/08-mamm-a-277.1
Gehrt, S. D., and McGraw, M. (2007). “Ecology of coyotes in urban landscapes,” in Proceedings of the 12th Wildlife Damage Management Conference, eds D. L. Nolte, W. M. Arjo, and D. H. Stalman (Lincoln: University of Nebraska), 303–311.
Gehrt, S. D., Brown, J., and Anchor, C. (2011). Is the urban coyote a misanthropic synanthrope? The case from Chicago. CATE 4:1. doi: 10.15365/CATE.4132011
Gehrt, S. D., Wilson, E. C., Brown, J. L., and Anchor, C. (2013). Population ecology of free-roaming cats and interference competition by coyotes in urban parks. PLoS One 8:9. doi: 10.1371/journal.pone.00757
George, S. L., and Crooks, K. R. (2006). Recreation and large mammal activity in an urban nature reserve. Biol. Conserv. 133, 107–117. doi: 10.1016/j.biocon.2006.05.0
Goldstein, A. J., Lahti, D. C., and Habig, B. (2022). Avian diversity and land use along the Bronx river. Urban Nat. 50, 1–20.
Gomes, V., Ribeiro, R., and Carretero, M. A. (2011). Effects of urban habitat fragmentation on common small mammals: species versus communities. Biodivers. Conserv. 20, 3577–3590.
Grinder, M. I., and Krausman, P. R. (2001). Home range, habitat use, and nocturnal activity of coyotes in an urban environment. J. Wildlife Manage. 65:887. doi: 10.2307/3803038
Grueber, C. E., Nakagawa, S., Laws, R. J., and Jamieson, I. G. (2011). Multimodel inference in ecology and evolution: challenges and solutions. J. Evol. Biol. 24, 699–711. doi: 10.1111/j.1420-9101.2010.02210.x
Guiry, E., and Buckley, M. (2018). Urban rats have less variable, higher protein diets. Proc. R. Soc. B. 285:20181441. doi: 10.1098/rspb.2018.1441
Harrison, D. J. (1992). Dispersal characteristics of juvenile coyotes in Maine. J. Wildlife Manage. 56:128. doi: 10.2307/3808800
Henger, C. S., Herrera, G. A., Nagy, C. M., Weckel, M. E., Gormezano, L. J., Wultsch, C., et al. (2020). Genetic diversity and relatedness of a recently established population of eastern coyotes (Canis latrans) in New York City. Urban Ecosyst. 23, 319–330. doi: 10.1007/s11252-019-00918-x
Hice, C. L., and Schmidly, D. J. (2002). The mammals of coastal Texas: a comparison between mainland and barrier island faunas. Southwest. Nat. 47, 244–256. doi: 10.2307/3672912
Hodgkison, S., Hero, J., and Warnken, J. (2007). The efficacy of small-scale conservation efforts, as assessed on Australian golf courses. Biol. Conserv. 136, 576–586. doi: 10.1016/j.biocon.2006.11.0
Hody, J. W., and Kays, R. (2018). Mapping the expansion of coyotes (Canis latrans) across North and Central America. Zookeys 759, 81–97. doi: 10.3897/zookeys.759.15149
Iida, S., and Nakashizuka, T. (1995). Forest fragmentation and its effect on species diversity in sub-urban coppice forests in Japan. For. Ecol. Manage. 73, 197–210. doi: 10.1016/0378-1127(94)03484-e
Jepsen, J. U., and Topping, C. J. (2004). Modelling roe deer (Capreolus capreolus) in a gradient of forest fragmentation: behavioural plasticity and choice of cover. Can. J. Zool. 82, 1528–1541. doi: 10.1139/z04-131
Kasso, M., and Bekele, A. (2014). Threats to mammals on fragmented habitats around Asella Town, Central Ethiopia. Int. J. Biodivers. 2014, 1–7. doi: 10.1155/2014/903898
Knowlton, J. L., Mata Zayas, E. E., Ripley, A. J., Valenzuela-Cordova, B., and Collado-Torres, R. (2019). Mammal diversity in oil palm plantations and forest fragments in a highly modified landscape in Southern Mexico. Front. For. Glob. Change 2:67. doi: 10.3389/ffgc.2019.00067
LaPoint, S., Balkenhol, N., Hale, J., Sadler, J., and van der Ree, R. (2015). Ecological connectivity research in urban areas. Funct. Ecol. 29, 868–878. doi: 10.1111/1365-2435.12489
Lehrer, E. W., and Schooley, R. L. (2010). Space use of woodchucks across an urbanization gradient within an agricultural landscape. J. Mammal. 91, 1342–1349. doi: 10.1644/09-MAMM-A-254.1
Lewis, J. S., Logan, K. A., Alldredge, M. W., Bailey, L. L., VandeWoude, S., and Crooks, K. R. (2015). The effects of urbanization on population density, occupancy, and detection probability of wild felids. Ecol. Appl. 25, 1880–1895. doi: 10.1890/14-1664.1
Lombardi, J. V., Comer, C. E., Scognamillo, D. G., and Conway, W. C. (2017). Coyote, fox, and bobcat response to anthropogenic and natural landscape features in a small urban area. Urban Ecosyst. 20, 1239–1248. doi: 10.1007/s11252-017-0676-z
Long, E. S., Diefenbach, D. R., Wallingford, B. D., and Rosenberry, C. S. (2010). Influence of roads, rivers, and mountains on natal dispersal of white-tailed deer. J. Wildlife Manage. 74, 1242–1249. doi: 10.1111/j.1937-2817.2010.tb01244.x
Lopes, M., and Ferrari, S. (2000). Effects of human colonization on the abundance and diversity of mammals in Eastern Brazilian Amazonia. Conserv. Biol. 14, 1658–1665. doi: 10.1111/j.1523-1739.2000.98402.x
Łopucki, R., and Kitowski, I. (2017). How small cities affect the biodiversity of ground-dwelling mammals and the relevance of this knowledge in planning urban land expansion in terms of urban wildlife. Urban Ecosyst. 20, 933–943. doi: 10.1007/s11252-016-0637-y
Luck, G. W. (2007). A review of the relationships between human population density and biodiversity. Biol. Rev. 82, 607–645. doi: 10.1111/j.1469-185x.2007.00028.x
MacArthur, R., and MacArthur, J. (1961). On bird species diversity. Ecology 42, 594–598. doi: 10.2307/1932254
MacArthur, R., and Wilson, E. O. (1967). The Theory of Island Biogeography. Princeton, NJ: Princeton University Press.
Magura, T., Kiss, E., and Lövei, G. L. (2021). No consistent diversity patterns in terrestrial mammal assemblages along rural-urban forest gradients. Basic Appl. Ecol. 52, 38–45. doi: 10.1016/j.baae.2021.02.004
Mahan, C. G., and O’Connell, T. (2005). Small mammal use of suburban and urban parks in Central Pennsylvania. Northeast. Nat. 12, 307–314.
Matthies, S. A., Rüter, S., Schaarschmidt, F., and Prasse, R. (2017). Determinants of species richness within and across taxonomic groups in urban green spaces. Urban Ecosyst. 20, 897–909. doi: 10.1007/s11252-017-0642-9
McCleery, R. A. (2010). “Urban mammals,” in Urban Ecosystem Ecology Madison, WI: American Society of Agronomy, 87–102.
McDonald, R. I., Kareiva, P., and Forman, R. T. T. (2008). The implications of current and future urbanization for global protected areas and biodiversity conservation. Biol. Conserv. 141, 1695–1703. doi: 10.1016/j.biocon.2008.04.0
McDonnell, M. J., and Pickett, S. T. A. (1990). Ecosystem structure and function along urban-rural gradients: an unexploited opportunity for ecology. Ecology 71, 1232–1237. doi: 10.2307/1938259
McKinney, M. L. (2006). Urbanization as a major cause of biotic homogenization. Biol. Conserv. 127, 247–260. doi: 10.1016/j.biocon.2005.09.0
McKinney, M. L. (2008). Effects of urbanization on species richness: a review of plants and animals. Urban Ecosyst. 11, 161–176. doi: 10.1007/s11252-007-0045-4
McPhearson, T., Pickett, S. T., Grimm, N. B., Niemelä, J., Alberti, M., Elmqvist, T., et al. (2016). Advancing urban ecology toward a science of cities. Bioscience 66, 198–212. doi: 10.1093/biosci/biw002
Merriam, G., Kozakiewicz, M., Tsuchiya, E., and Hawley, K. (1989). Barriers as boundaries for metapopulations and demes of Peromyscus leucopus in farm landscapes. Landsc. Ecol. 2, 227–235. doi: 10.1007/bf00125093
Michael, E. D. (1965). Movements of white-tailed deer on the Welder Wildlife Refuge. J. Wildlife Manage. 29:44. doi: 10.2307/3798630
Moll, R. J., Cepek, J. D., Lorch, P. D., Dennis, P. M., Robison, T., and Montgomery, R. A. (2020). At what spatial scale(s) do mammals respond to urbanization? Ecography 43, 171–183. doi: 10.1111/ecog.04762
Muhly, T. B., Semeniuk, C., Massolo, A., Hickman, L., and Musiani, M. (2011). Human activity helps prey win the predator-prey space race. PLoS One 6:e17050. doi: 10.1371/journal.pone.00170
Nagy, C. M., Koestner, C., Clemente, S., and Weckel, M. (2016). Occupancy and breeding status of coyotes in New York City parks, 2011 to 2014. Urban Nat. 9, 1–16.
Nagy, C. M., Weckel, M., Monzón, J., Duncan, N., and Rosenthal, M. R. (2017). Initial colonization of Long Island, New York by the eastern coyote, Canis latrans (Carnivora, Canidae), including first record of breeding. Check List 13, 901–907. doi: 10.15560/13.6.901
Nakagawa, S., and Schielzeth, H. (2013). A general and simple method for obtaining R2 from generalized linear mixed-effects models. Methods Ecol. Evol. 4, 133–142. doi: 10.1111/j.2041-210x.2012.00261.x
Nicholson, M., and Cove, M. V. (2022). Stable isotopes point to anthropogenic subsidies in northern raccoons at the urban-wild interface. Food Webs 31:e00233. doi: 10.1016/j.fooweb.2022.e00233
Nielsen, A. B., van den Bosch, M., Maruthaveeran, S., and van den Bosch, C. K. (2014). Species richness in urban parks and its drivers: a review of empirical evidence. Urban Ecosyst. 17, 305–327. doi: 10.1007/s11252-013-0316-1
Olifiers, N., Gentile, R., and Fiszon, J. T. (2005). Relation between small-mammal species composition and anthropic variables in the Brazilian Atlantic Forest. Braz. J. Biol. 65, 495–501. doi: 10.1590/S1519-69842005000300015
Ordeñana, M. A., Crooks, K. R., Boydston, E. E., Fisher, R. N., Lyren, L. M., Siudyla, S., et al. (2010). Effects of urbanization on carnivore species distribution and richness. J. Mammal. 91, 1322–1331. doi: 10.1644/09-MAMM-A-312.1
Oxley, D. J., Fenton, M. B., and Carmody, G. R. (1974). The effects of roads on populations of small mammals. J. Appl. Ecol. 11, 51–59. doi: 10.2307/2402004
Pardo, L. E., Campbell, M. J., Edwards, W., Clements, G. R., and Laurance, W. F. (2018). Terrestrial mammal responses to oil palm dominated landscapes in Colombia. PLoS One 13:5. doi: 10.1371/journal.pone.01975
Parsons, A. W., Forrester, T., Baker-Whatton, M. C., McShea, W. J., Rota, C. T., Schuttler, S. G., et al. (2018). Mammal communities are larger and more diverse in moderately developed areas. eLife 7:e38012. doi: 10.7554/eLife.38012
Peña, E., and Slate, E. (2006). Global validation of linear model assumptions. J. Am. Stat. Assoc. 101:341. doi: 10.1198/016214505000000637
Prange, S., Gehrt, S. D., Wiggers, E. P., and O’Shea, T. J. (2004). Influences of anthropogenic resources on raccoon (Procyon lotor) movements and spatial distribution. J. Mammal. 85, 483–490. doi: 10.1644/bos-121
Presley, S., Cisneros, L. M., Klingbeil, B. T., and Willig, M. (2019). Landscape ecology of mammals. J. Mammal. 100, 1044–1068. doi: 10.1093/JMAMMAL/GYY169
Racey, G. D., and Euler, D. L. (1982). Small mammal and habitat response to shoreline cottage development in central Ontario. Can. J. Zool. 60, 865–880. doi: 10.1139/z82-119
Radeloff, V. C., Hammer, R. B., Stewart, S. I., Fried, J. S., Holcomb, S. S., and McKeefry, J. F. (2005). The wildland-urban interface in the United States. Ecol. Appl. 15, 793–1110. doi: 10.1890/04-1413
Ray, J. C. (2000). Mesocarnivores of northeastern North America: status and conservation issues. Wildlife Conserv. Soc. Work. Paper 15, 1–82.
Rooney, T. P. (2001). Deer impacts on forest ecosystems: a North American perspective. Forestry 74, 201–208. doi: 10.1093/forestry/74.3.201
Sacks, B. N., Ernest, H. B., and Boydston, E. E. (2006). San Francisco’s golden gate: a bridge between historically distinct coyote (Canis latrans) populations. West. North Am. Nat. 66, 263–264.
Sala, O. E., Chapin, F. S. III, Armesto, J. J., Berlow, E., Bloomfield, J., Dirzo, R., et al. (2000). Global biodiversity scenarios for the year 2100. Science 287, 1770–1774. doi: 10.1126/science.287.5459.1770
Šálek, M., Drahníková, L., and Tkadlec, E. (2014). Changes in home range sizes and population densities of carnivore species along the natural to urban habitat gradient. Mammal. Rev. 45:1. doi: 10.1111/mam.12027
Saunders, D., Hobbs, R., and Margules, C. (1991). Biological consequences of ecosystem fragmentation: a review. Conserv. Biol. 5, 18–32. doi: 10.1016/0006-3207(92)90725-3
Schipper, J., Chanson, J. S., Chiozza, F., Cox, N. A., Hoffman, M., Katariya, V., et al. (2008). The status of the world’s land and marine mammals: diversity, threat, and knowledge. Science 322, 225–230. doi: 10.1126/science.1165115
Scott, D. M., Berg, M. J., Tolhurst, B. A., Chauvenet, A. L. M., Smith, G. C., Neaves, K., et al. (2014). Changes in the distribution of red foxes (Vulpes vulpes) in urban areas in Great Britain: findings and limitations of a media-driven nationwide survey. PLoS One 9:6. doi: 10.1371/journal.pone.00990
Seiler, A. (2001). Ecological Effects of Roads. A Review. Introductory Research Essay No. 9. Uppsala: Department of Conservation Biology.
Seto, K. C., Güneralp, B., and Hutyra, L. R. (2012). Global forecasts of urban expansion to 2030 and direct impacts on biodiversity and carbon pools. Proc. Natl. Acad. Sci. U.S.A. 109, 16083–16088. doi: 10.1073/pnas.1211658109
Shilling, F., Nguyen, T., Saleh, M., Kyaw, M. K., Tapia, K., Trujillo, G., et al. (2021). A reprieve from US wildlife mortality on roads during the COVID-19 pandemic. Biol. Conserv. 256:109013. doi: 10.1016/j.biocon.2021.109013
Silva-Rodríguez, E. A., Gálvez, N., Swan, G. J. F., Cusack, J. J., and Moreira-Arce, D. (2020). Urban wildlife in times of COVID-19: what can we infer from carnivore sightings in urban areas? Sci. Total Environ. 765:142713. doi: 10.1016/j.scitotenv.2020.1
Soberón, M. J., and Llorente, B. J. (1993). The use of species accumulation functions for the prediction of species richness. Conserv. Biol. 7, 480–488. doi: 10.1046/j.1523-1739.1993.07030480.x
Spinola, R. M., Serfass, T. L., and Brooks, R. P. (2008). Survival and post-release movements of river otters translocated to Western New York. Northeast. Nat. 15, 13–24.
Stark, J. R., Aiello-Lammens, M., and Grigione, M. M. (2020). The effects of urbanization on carnivores in the New York metropolitan area. Urban Ecosyst. 23, 215–225. doi: 10.1007/s11252-019-00923-0
Trombulak, S. C., and Frissell, C. A. (2000). Review of ecological effects of roads on terrestrial and aquatic communities. Conserv. Biol. 14, 18–30. doi: 10.1046/j.1523-1739.2000.99084.x
Turner, W., Tjørve, E., and Hillebrand, H. (2005). Scale-dependence in species-area relationships. Ecography 28, 721–730. doi: 10.1111/j.2005.0906-7590.04273.x
Vernon, M. E., Magle, S. B., Lehrer, E. W., and Bramble, J. E. (2014). Invasive European buckthorn (Rhamnus cathartica L.) association with mammalian species distribution in natural areas of the Chicagoland region, USA. Nat. Area J. 34, 134–143. doi: 10.3375/043.034.0203
Weckel, M., Bogan, D. A., Burke, R. L., Nagy, C., Siemer, W. F., Green, T., et al. (2015). Coyotes go “bridge and tunnel”: a narrow opportunity to study the socio-ecological impacts of coyote range expansion on Long Island, NY pre-and post-arrival. CATE 8:5.
Woodroffe, R. (2000). Predators and people: using human densities to interpret declines of large carnivores. Anim. Conserv. 3, 165–173. doi: 10.1111/j.1469-1795.2000.tb00241.x
Wurth, A. M., Ellington, E. H., and Gehrt, S. D. (2020). Golf courses as potential habitat for urban coyotes. Wildlife Soc. B. 44, 333–341. doi: 10.1002/wsb.1081
Yates, M. D., Loeb, S. C., and Guynn, D. C. Jr. (1997). “The effect of habitat patch size on small mammal populations,” in Proceedings of the Annual Conference, Vol. 51, (Laurel, MS: Southeastern Association of Fish and Wildlife Agencies), 501–510.
Keywords: urban ecology, mammals, habitat heterogeneity, patch size, biodiversity, human population density, New York metropolitan area, land cover
Citation: Bradfield AA, Nagy CM, Weckel M, Lahti DC and Habig B (2022) Predictors of Mammalian Diversity in the New York Metropolitan Area. Front. Ecol. Evol. 10:903211. doi: 10.3389/fevo.2022.903211
Received: 24 March 2022; Accepted: 27 May 2022;
Published: 06 July 2022.
Edited by:
Juliano André Bogoni, University of São Paulo, BrazilReviewed by:
Marcelo Magioli, Institute for the Conservation of Neotropical Carnivores, Pró-Carnívoros, BrazilRobert A. Montgomery, University of Oxford, United Kingdom
Copyright © 2022 Bradfield, Nagy, Weckel, Lahti and Habig. This is an open-access article distributed under the terms of the Creative Commons Attribution License (CC BY). The use, distribution or reproduction in other forums is permitted, provided the original author(s) and the copyright owner(s) are credited and that the original publication in this journal is cited, in accordance with accepted academic practice. No use, distribution or reproduction is permitted which does not comply with these terms.
*Correspondence: Bobby Habig, heybobby99@gmail.com