- 1Yunnan Provincial Key Laboratory for Zoonosis Control and Prevention, Institute of Pathogens and Vectors, Dali University, Dali, China
- 2Jilin Provincial Key Laboratory of Animal Resource Conservation and Utilization, Northeast Normal University, Changchun, China
Condition-dependent acoustic signals that potentially reveal information about the signaler’s physical or physiological condition are common and important in the animal kingdom. Given the negative effects of parasites on the health and fitness of their hosts, it is reasonable to expect animal acoustic signals to reflect detailed information concerning parasite infection. However, despite previous studies having verified the potential of sexually selected vocalizations to provide information on parasitism based on the correlations between call acoustic properties and parasitism in some animal taxa, less is known about whether acoustic signals used in a non-sexual context also reflect parasite infection especially for highly vocal bats. We thus investigated the relationships between the acoustic properties of distress calls and echolocation pulses and the infestation intensity of gamasid mites and bat flies in Chinese horseshoe bats (Rhinolophus sinicus) to determine whether acoustic signals potentially contain information about parasite infection. We found that bats infected with more gamasid mites uttered significantly shorter echolocation pulses, suggesting that echolocation pulses may contain information on the intensity of mite infection. Additionally, bats infected with more gamasid mites emitted distress calls with narrower bandwidth, while bats with more bat flies emitted calls with longer pause duration. These results suggest that distress calls may not only reflect a signaler’s parasite infection intensity but also may provide information concerning infection with specific parasites. In short, our findings suggest that acoustic signals of bats potentially reflect detailed information about parasite infection.
Introduction
Acoustic signals that mediate various aspects of animals’ life history (e.g., resource defense, mate choice, parental care, and anti-predator behavior), have been confirmed to be honest and condition dependent (Galeotti et al., 2005; Wilkins et al., 2013; Nouri and Blumstein, 2019; Sun et al., 2021). This means that acoustic signal expression varies as a function of an individual’s physical or physiological condition, and therefore potentially contains relevant information that could be used by receivers to estimate a caller’s condition (Nouri and Blumstein, 2019). For example, young mammals and birds in poorer nutritional state always utter begging calls at higher frequencies than young in better condition to communicate their needs and thereby receive more food and parental care (Manser and Avey, 2000; Perez et al., 2016). Similarly, birds that are healthy emit harsher alarm calls than unhealthy birds to signal their ability to escape, thereby discouraging potential predators (Laiolo et al., 2004, 2007). It raises the intriguing question concerning whether animal acoustic signals being able to reflect information on some factors such as parasitism that can potentially impact the health of signalers.
Parasites are ubiquitous and can exert detrimental effects on the health and fitness of their hosts. For example, infections can deplete energy and nutrients of the host (Aalto et al., 2015), induce physiological stress in the host (Romano et al., 2015), impair the host’s anti-predator responses (Luong et al., 2011), reduce host fecundity (Rogalski and Duffy, 2020), or increase the host’s probability of morbidity and mortality (Lynsdale et al., 2017). At present, extensive research on avian mate choice has demonstrated that both the temporal (e.g., duration and syllable rate) and spectral properties (e.g., maximum frequency and bandwidth) of male songs can vary in relation to their parasite load (chewing lice, fleas, or blood parasites), suggesting that bird songs may contain potential indicators of parasitism status (Redpath et al., 2000; Garamszegi, 2005; Bischoff et al., 2009). In anurans, males with lower intestinal parasite intensity emit advertisement calls at higher rates, again supporting the potential of acoustic signals to encode parasite information (Madelaire et al., 2014). Gilman et al. (2007) found that Leucocytozoon and Plasmodium infections differentially influenced the singing behavior of male mountain white-crowned sparrows (Zonotrichia leucophrys oriantha); infection with Leucocytozoon significantly increased song consistency, while infection with Plasmodium significantly reduced song output. The authors thus suggested that songs possibly reflect information not only on whether a caller is infected but also on the type of parasite it carries.
Although previous research has verified the potential of sexually selected vocalizations to provide information on parasitism based on a significant correlation between call acoustic properties and parasite infection status or intensity, less is known about whether acoustic signals used in a non-sexual context also reflect parasite infection (Laiolo et al., 2007; Nouri and Blumstein, 2019). In addition, studies linking vocal signals to parasites have been largely restricted to birds and anurans. Little information is available for other highly vocal taxa such as bats. Bats (order Chiroptera) may be an ideal group with which to investigate whether acoustic signals can act as reliable indicators of parasitism. First, bats are one of the most diverse and widely distributed groups of mammals, and they are infected with a plethora of endoparasites and ectoparasites (Klimpel, 2014). Second, bats are nocturnal mammals that rely heavily on acoustic signals for communication in darkness (Fenton, 2003). Third, bats possess two different call types: echolocation pulses, primarily used in navigation and prey detection, and social calls, emitted for mediating social activities, including aggressive calls in antagonistic and territorial interactions (Zhao et al., 2018), distress calls against close predators (Huang et al., 2018), and contact calls facilitating individual recognition (Carter and Wilkinson, 2016). Furthermore, both echolocation pulses and social calls can encode socially relevant information about the sender such as sex, age, reproductive status, body condition, or individual identity (Voigt-Heucke et al., 2010; Chaverri et al., 2018). However, little is known concerning the potential to encode information about parasites.
In this study, we investigated the potential of two types of acoustic signals (distress calls and echolocation pulses) emitted by hand-held individuals (during distress) to reflect information on ectoparasite infection in Chinese horseshoe bats (Rhinolophus sinicus). The most common ectoparasites parasitizing bats belong to two taxa, gamasid mites (Acarina) and bat flies (Diptera) (Klimpel, 2014). As blood feeding parasites, bat ectoparasites serve as excellent vectors of various zoonoses, and thus negatively affect the behavior and health of their hosts (Klimpel, 2014). In light of this, we hypothesized that acoustic signals emitted by bats potentially contain detailed information about parasite infection. To test this hypothesis, we first quantified the intensity of infestation of gamasid mites and bat flies for each individual bat (i.e., mite load and bat-fly load) and then measured the temporal and spectral parameters of distress calls and echolocation pulses per bat. We made the following predictions. (1) If acoustic signals of R. sinicus potentially encode information on parasite infection intensity, acoustic properties of distress calls or echolocation pulses should vary based on individual parasite loads (mite load or bat-fly load). (2) If acoustic signals of R. sinicus potentially provide information on infection with particular parasites, mite loads and bat-fly loads should influence different acoustic parameters of distress calls or echolocation pulses.
Materials and Methods
Study Animals and Site
Rhinolophus sinicus is a medium-sized bat (Rhinolophidae) with a widespread geographical range in China and Vietnam (Sun, 2019). Like most horseshoe bats, R. sinicus emits echolocation signals comprising a long constant frequency and a brief frequency-modulated start and tail (FM-CF-FM; Figures 1A,B). We studied a wild population of R. sinicus severely infected by ectoparasitic mites and bat flies located at Bianfu Cave (102°57′E, 25°48′N, 1957 m) in Kunming, Yunnan Province, China. At this cave, R. sinicus occurs in groups of about 200 individuals along with four other bat species, R. pusillus, R. affinis, Myotis badius, and Hipposideros armiger.
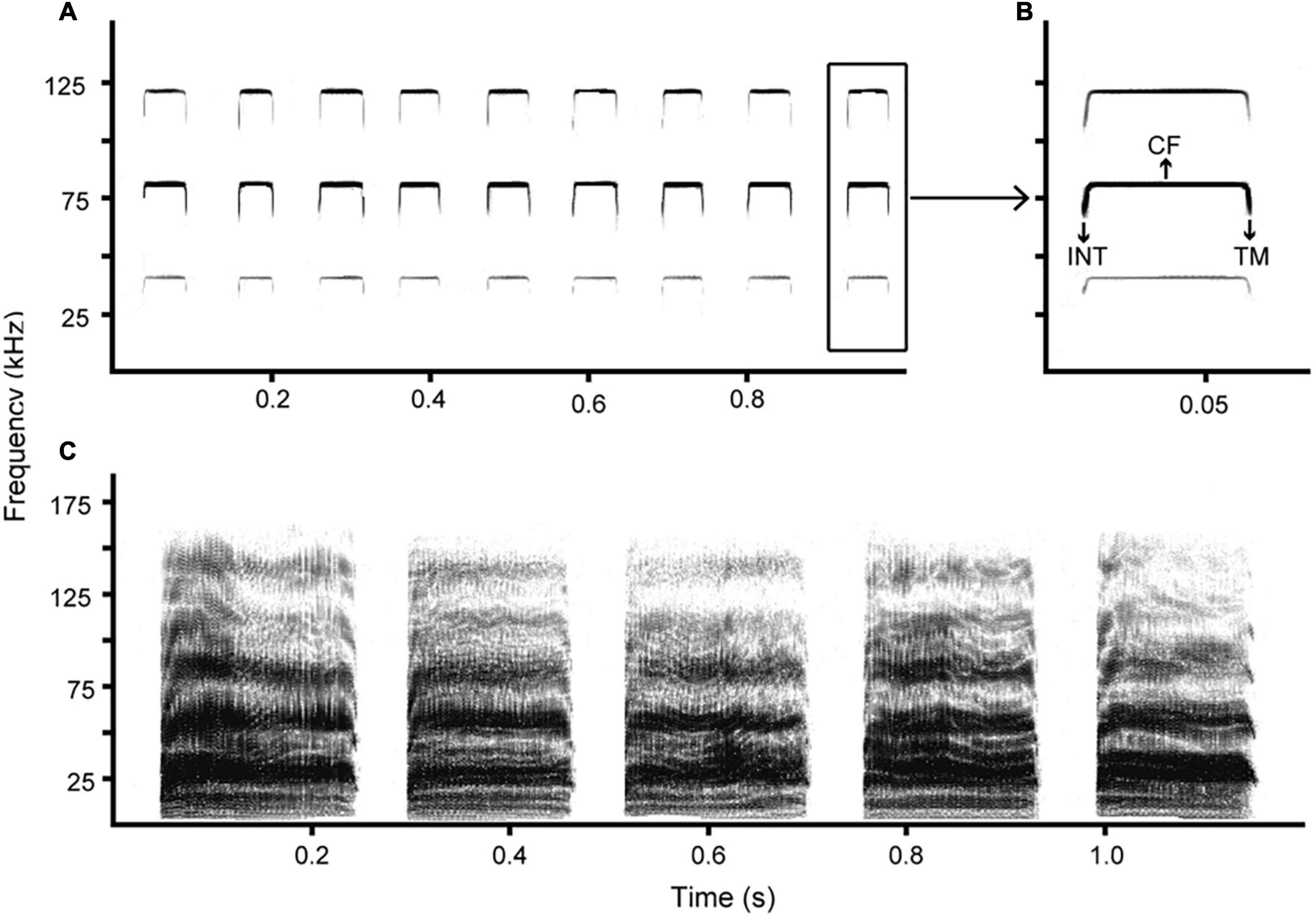
Figure 1. Spectrograms of echolocation pulses (A,B) and distress calls (C) emitted by R. sinicus. Distress call sequences of R. sinicus are composed of mainly broadband noise burst syllables (Huang et al., 2018). CF, constant frequency component; INT, initial frequency modulated component; TM, terminal frequency modulated component.
Capture of Bats
From August to September 2019 (non-pregnant and non-lactating periods) we captured R. sinicus bats using mist nets placed at the cave entrance. Captured bats were kept individually in clean cloth bags to avoid ectoparasite contamination and were transferred to a temporary laboratory near their roost. Sex was then identified visually. Age-class was determined by examining the level of epiphyseal–diaphyseal fusion (Brunet-Rossinni et al., 2009). Only adult bats were used in subsequent analyses. For ethical reasons, all juvenile bats were released immediately. Forearm length and body mass were measured using digital calipers and an electronic scale. Bats were temporarily marked by shaving hair on their head to avoid resampling the same individuals. Altogether, we captured 66 adult R. sinicus bats (41 males and 25 females).
Collection and Quantification of Bat Ectoparasites
We visually inspected the body surface of each bat (i.e., wing and tail membranes, pelage, ears, and face) for ectoparasites; the cloth bags also were examined carefully for any ectoparasites which may have left the bat; these were collected with stainless steel pointed tweezers. To standardize sampling effort and minimize effects of handling the animal, each bat was examined for 2 min. This sampling protocol has been effective for predicting total ectoparasite abundance in several other taxa (Whiteman and Parker, 2004). The collected ectoparasite samples were preserved in individually labeled vials with 70% ethanol until further processing. After each bat inspection, all instruments were cleaned with disposable paper towels to reduce the chance of cross-contamination. We followed the protocol described in Dias (1982) to clear and mount the ectoparasite specimens on glass slides before identification. All mounted specimens were finally identified as gamasid mites, bat flies, or other groups under a light microscope according to identification keys and descriptions available in published papers (Maa, 1962; Radovsky, 1967). Then, we quantified the intensity of infestation of gamasid mites and bat flies (mite load and bat-fly load) calculated as the number of gamasid mites or bat flies per bat.
Recording and Analysis of Acoustic Signals
Bats can emit distress calls and echolocation pulses when cornered, attacked or captured by a predator or researcher (Jiang et al., 2017). In this study, each individual of R. sinicus was hand-held softly for 1 min by the same researcher. Both distress calls and echolocation pulses were recorded from bats held 1 m in front of a condenser microphone (CM16/CMPA; frequency range: 10–200 kHz) of an UltrasoundGate 116 (Avisoft Bioacoustics, Glienicke, Germany) with a sampling frequency of 375 kHz and 16-bit resolution. In this way, a 1 min sound file (in WAV format) was obtained for each individual.
The acoustic analyses were carried out with Avisoft SASLab Pro (Avisoft Bioacoustics, Glienicke, Germany). Spectrograms were generated using a 512-point fast Fourier transform (FFT), a Hamming window with 75% overlap, and 100% frame size. In the present study, distress calls of R. sinicus were composed primarily of broadband noise burst syllables (Figure 1C) that were defined following the terminology described in Kanwal et al. (1994). Therefore, we measured eight parameters (two temporal parameters and six spectral parameters; Table 1) of broadband noise burst syllables with high signal-to-noise ratio, including syllable duration (ms), pause duration (ms), peak frequency (kHz), minimum frequency (kHz), maximum frequency (kHz), bandwidth (kHz), harshness (measured as the frequency range in which the signaler concentrated 50% of the call energy; kHz), and Wiener entropy (a measure of randomness). Altogether, we obtained information on distress calls from 55 adult bats (33 males and 22 females) after excluding some individuals for which we did not obtain good-quality calls.
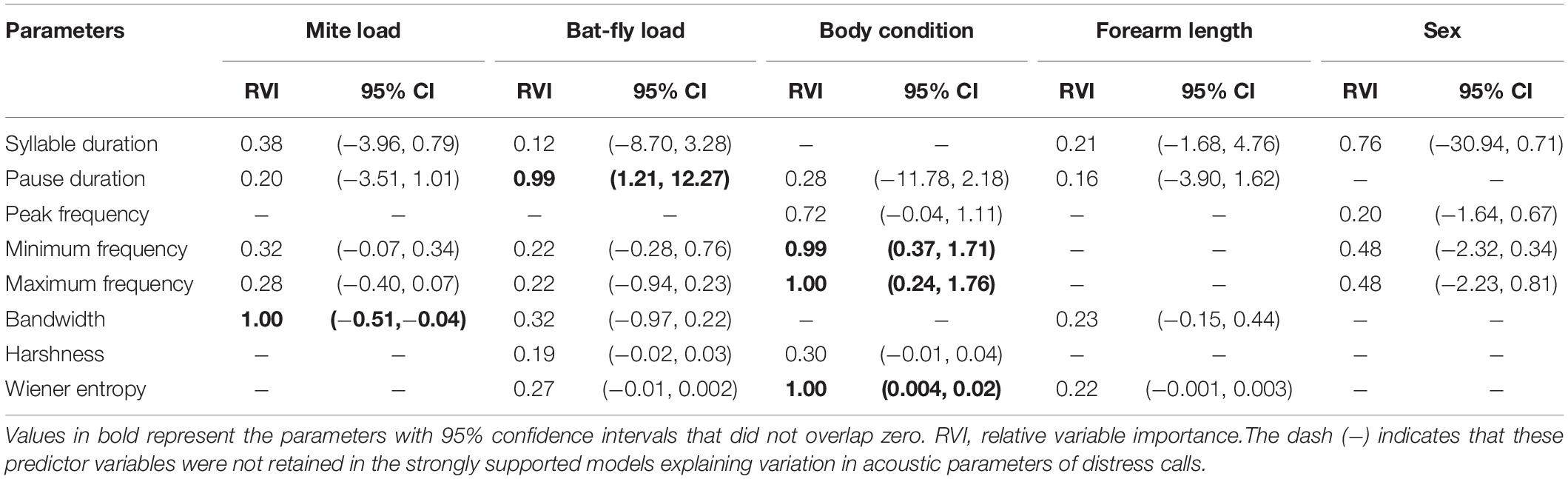
Table 1. Model-averaged parameter estimates of strongly supported linear models (ΔAICc ≤ 2) explaining the inter-individual variation of distress calls in Rhinolophus sinicus.
The acoustic analyses of echolocation pulses were restricted to the dominant second harmonic, since this contained the most energy (Figures 1A,B). We also excluded the first 10 pulses of a recording for analysis because horseshoe bats tune into their resting frequencies from lower frequencies after periods of silence (Siemers et al., 2005). We thus measured eight parameters (three temporal parameters and five spectral parameters; Table 2) of individual pulses with high signal-to-noise ratio from the remaining sequences, including pulse duration (ms), resting frequency (kHz) and six parameters of FM components. For the initial and terminal FM components (marked as INT and TM, respectively), we obtained minimum frequency (kHz), maximum frequency (kHz), and duration (the time from the start of the FM component until the start of the CF component, and the time from the end of the CF component to the end of the FM component; ms). Altogether, we obtained information on echolocation pulses from 64 adults (39 males and 25 females) after excluding some individuals for which we did not obtain good-quality pulses.
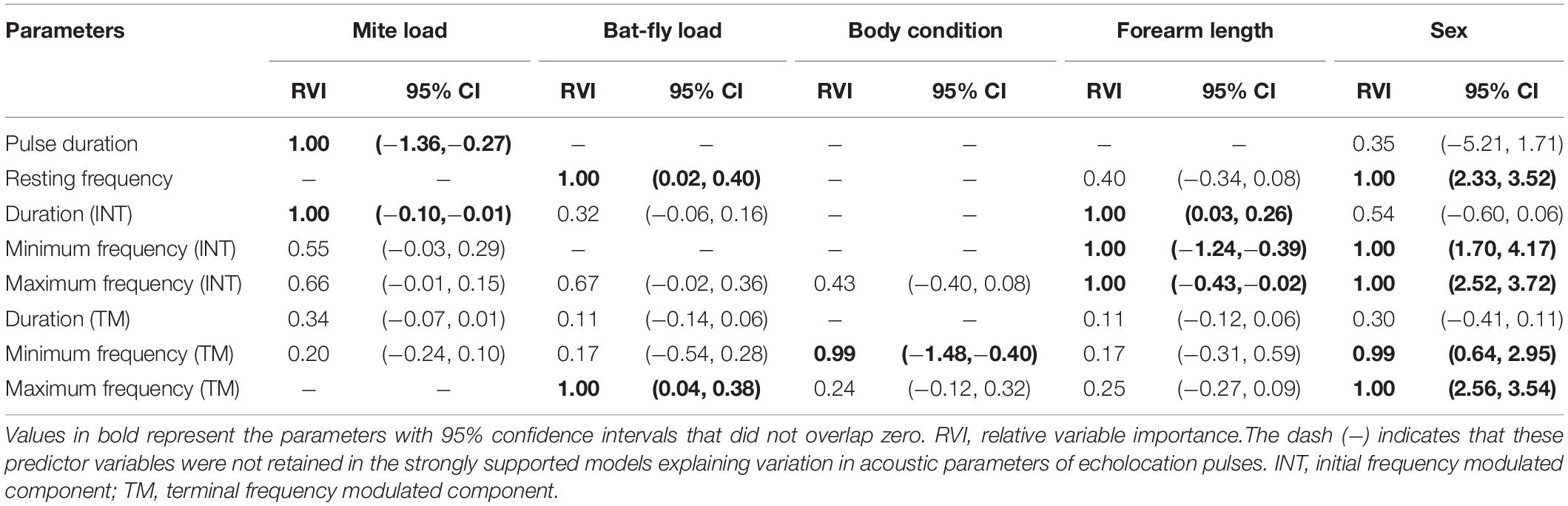
Table 2. Model-averaged parameter estimates of strongly supported linear models (ΔAICc ≤ 2) explaining the inter-individual variation of echolocation pulses in Rhinolophus sinicus.
Statistical Analysis
We fitted a generalized linear model (GLM) analysis with a Gaussian distribution to assess the effects of parasite loads and other predictor factors on call variation, separately for distress calls and echolocation pulses. The average value of each acoustic parameter per individual was assigned as the response variable. Except for harshness, all response variables were confirmed as normally distributed by Kolmogorov–Smirnov test (all P > 0.05). A log10-transformation was used for harshness to attain normality. Mite load, bat-fly load, body condition (measured via the residuals of the linear regression of log body mass on log forearm length), forearm length, and sex were assigned as predictor variables. We then conducted a model selection procedure for GLM based upon the Akaike information criterion corrected for small sample size (AICc) using the function “dredge” in the R package “MuMIn” (Barton, 2015). The competing models were ranked using differences in AICc scores (ΔAICc; Supplementary Tables 1, 2). Models with ΔAIC scores within two of the best models were considered to have strong support (Supplementary Tables 1, 2) (Jiang et al., 2019). We finally performed model averaging and obtained model-averaged parameter estimates and 95% confidence intervals (95% CIs) for all variables included in strongly supported models (Tables 1, 2) using the function “model.avg” in the R package “MuMIn” (Grueber et al., 2011; Barton, 2015). We concluded that there was evidence for the influence of a single predictor variable on call variability among individuals when the 95% CIs did not overlap with zero (Grueber et al., 2011).
We also carried out a hierarchical partitioning analysis to assess the independent contribution of each predictor variable to the inter-individual variation in acoustic parameters using the R package “hier.part” (Walsh et al., 2013), with separate analyses for distress calls and echolocation pulses. This method can effectively alleviate multicollinearity between different explanatory variables. In the hierarchical partitioning, a randomization approach was carried out to determine the significance for each variable (Nally and Walsh, 2004). Agreement as to the best predictor variables from both the multimodel inference (model averaging) and hierarchical partitioning methods indicates that it was more likely for those variables (retained by both methods) to have an important influence over the response variable (Mac Nally, 2002; Bommarco et al., 2014).
Ethics
All research was conducted according to the relevant laws for experiments involving vertebrates of the People’s Republic of China and was approved by the Animals Ethics Committee of Dali University (approval number: DLDXLL2019-810). Experimental procedures were in accordance with the ABS/ASAB guidelines for the Use of Animals in Research. Ectoparasite sampling and acoustic recording protocol also were designed to minimize the effects of handling the animals and the time spent in captivity. No bats were harmed during capture and handling. All bats were released at the site of capture immediately after data collection.
Results
Parasites
Overall, 54 of the 66 bats (81.82%) in the recorded sample were infected with at least one of the two focal parasite taxa (i.e., gamasid mites or bat flies). Of these, gamasid mites were the most prevalent, occurring in 44 (66.67%) individuals. Thirty-four individuals (51.52%) were infected with bat flies. Only one bat was infected with another parasite group (chigger mites).
Distress Calls
The multimodel inference approach showed that mite load, bat-fly load, and body condition had significant effects on acoustic parameters of distress calls (Table 1). The hierarchical partitioning approach largely supported these results (Figure 2). That is, mite load was negatively correlated with bandwidth (Figure 3A) and explained 62.07% of the acoustic parameter variation (Figure 2F). Bat-fly load was positively correlated with pause duration (Figure 3B) and explained 67.30% of the variation (Figure 2B). Additionally, body condition had a strong effect on minimum frequency, maximum frequency, and Wiener entropy, accounting for 64.21, 64.41, and 78.24% of the variation (Figures 2D,E,H and Table 1), respectively.
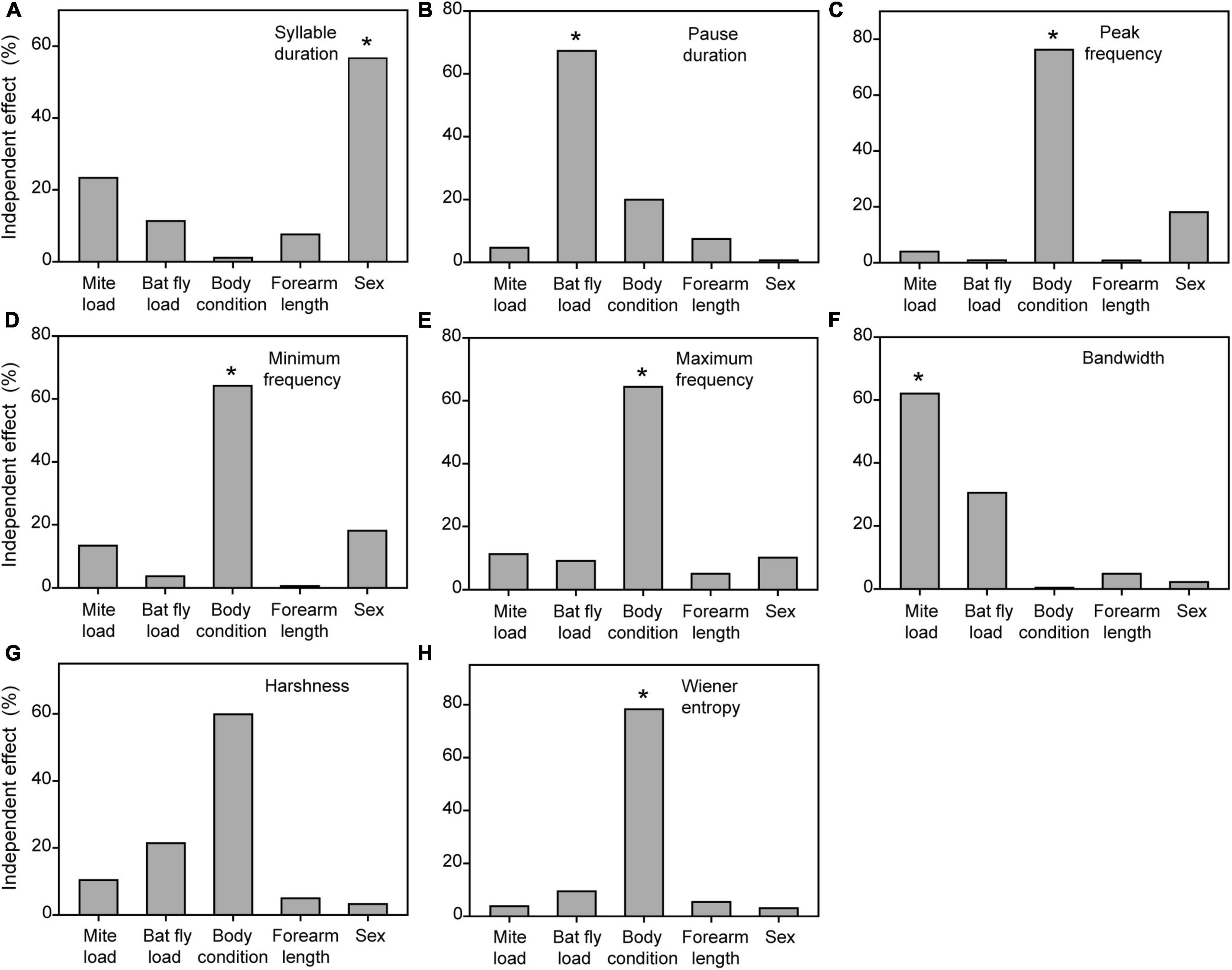
Figure 2. Independent contributions (%) of the five explanatory variables to the inter-individual variation in syllable duration (A), pause duration (B), peak frequency (C), minimum frequency (D), maximum frequency (E), bandwidth (F), harshness (G), and wiener entropy (H) of distress calls, calculated with hierarchical partitioning. Asterisks denote statistical significance (*P < 0.05).
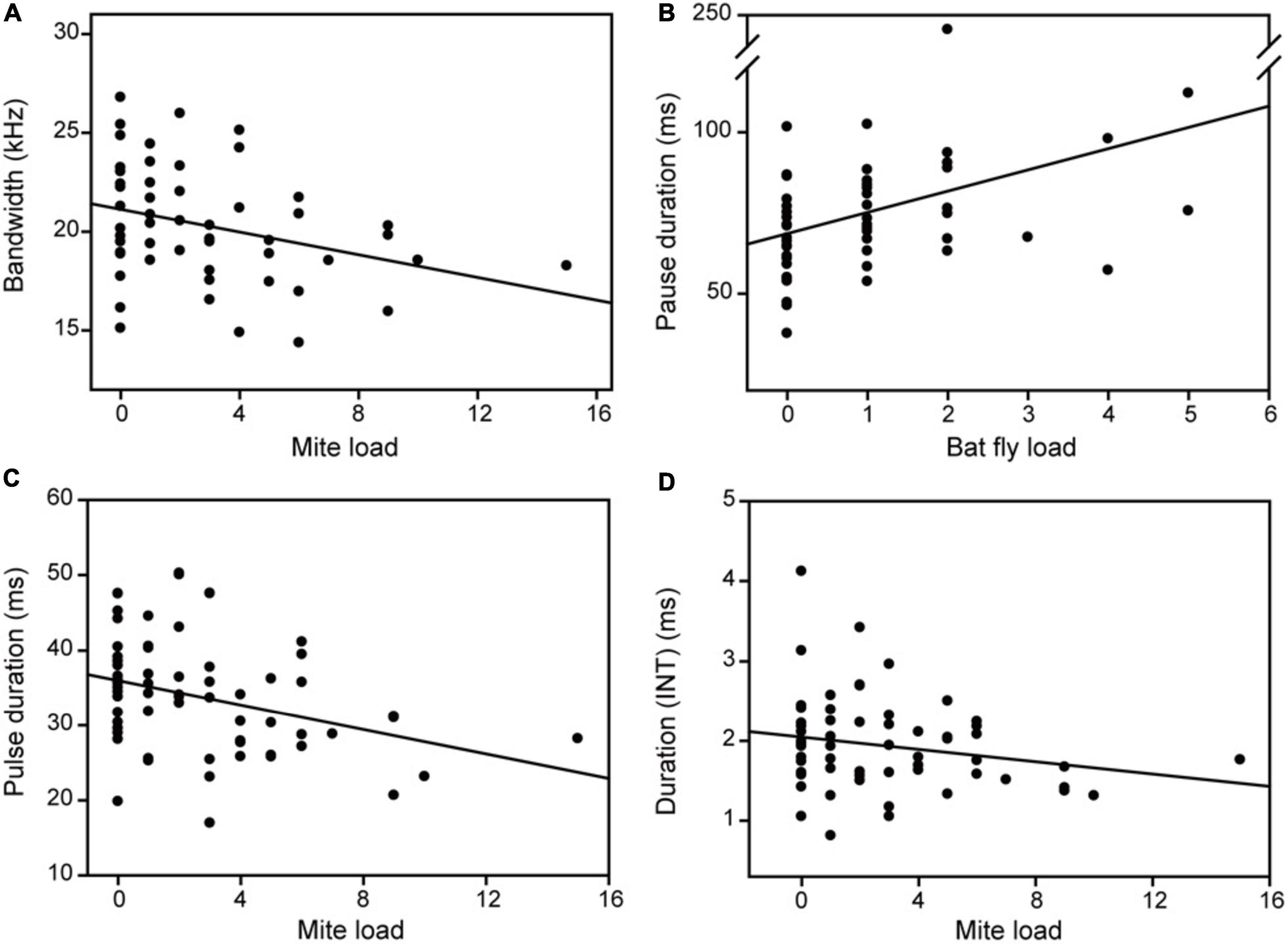
Figure 3. The relationship between parasite load and acoustic parameters of distress calls (A,B) and echolocation pulses (C,D). INT, initial frequency modulated component.
Echolocation Pulses
Both the multimodel inference and the hierarchical partitioning procedures indicated that mite load, body condition, forearm length, and sex were significantly associated with individual variation in acoustic parameters of echolocation pulses (Figure 4 and Table 2). Although bat-fly load was also suggested to explain the acoustic parameter variation by the multimodel inference approach (Table 2), the hierarchical partitioning approach did not support this result (Figure 4). Specifically, mite load was negatively related to pulse duration and duration (INT) (Figure 3) and contributed 76.32 and 36.28% of the observed variation (Figures 4A,C), respectively. In addition, body condition was related to minimum frequency (TM) and contributed 48.25% of the variation (Figure 4G and Table 2). Forearm length was correlated with duration (INT), minimum frequency (INT), and maximum frequency (INT) and explained 9.12–50.28% of the variation (Figures 4C–E and Table 2). Finally, sex had marked effects on all five spectral parameters [i.e., resting frequency, minimum frequency (INT), maximum frequency (INT), minimum frequency (TM) and maximum frequency (TM)] and explained 35.85–83.12% of the variation of these parameters (Figure 4 and Table 2).
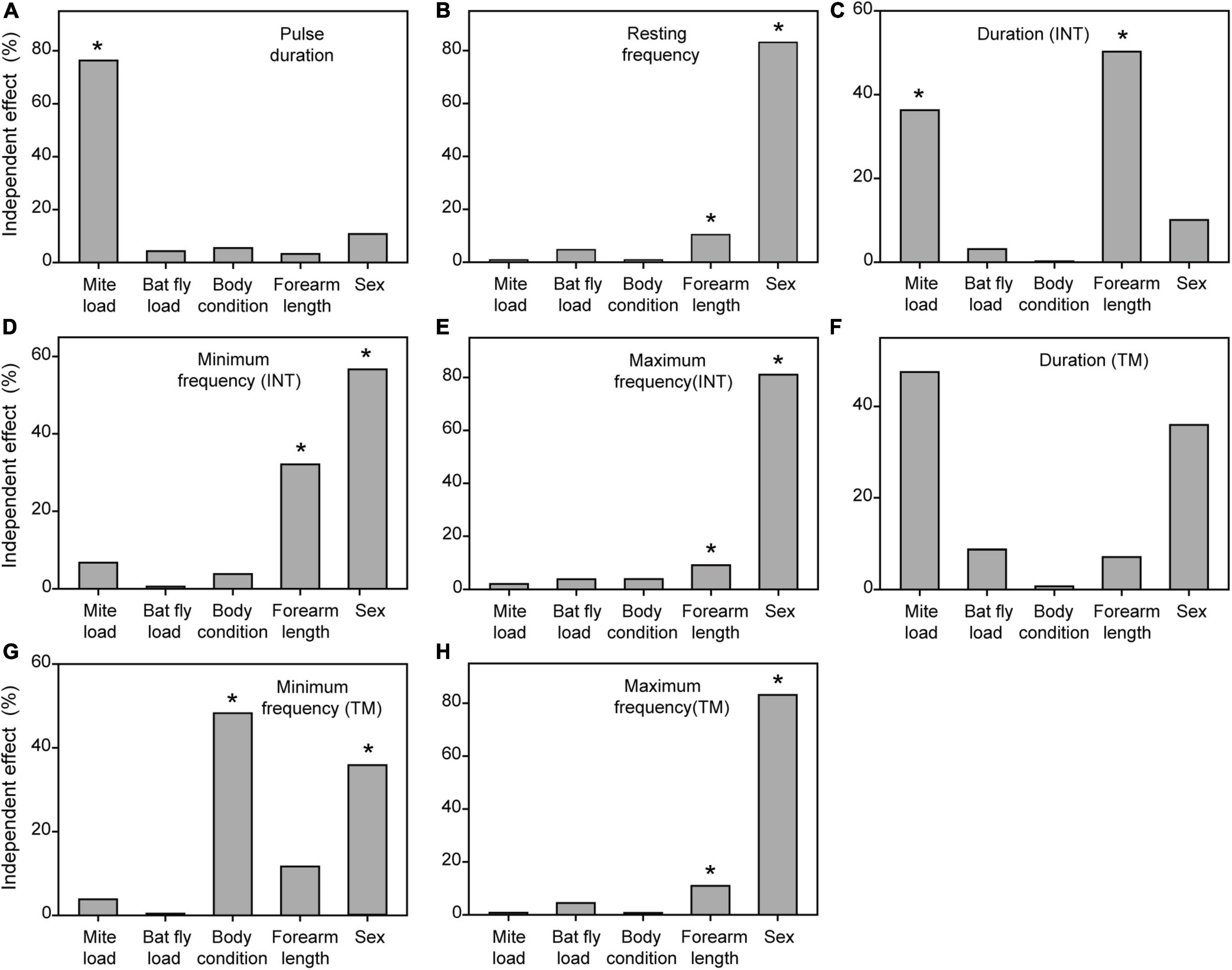
Figure 4. Independent contributions (%) of the five explanatory variables to the inter-individual variation in pulse duration (A), resting frequency (B), duration (INT) (C), minimum frequency (INT) (D), maximum frequency (INT) (E), duration (TM) (F), minimum frequency (TM) (G), and maximum frequency (H) of echolocation pulses, calculated with hierarchical partitioning. Asterisks denote statistical significance (*P < 0.05). INT, initial frequency modulated component. TM, terminal frequency modulated component.
Discussion
The results of this study indicate that acoustic signals of R. sinicus are parasite-condition dependent. First, several acoustic parameters of both distress calls (pause duration and bandwidth) and echolocation pulses [pulse duration and duration (INT)] were significantly associated with parasite loads (mite load or bat-fly load), results that were in accord with the first prediction. Second, mite load and bat-fly load had a significant influence on pause duration and bandwidth of distress calls, respectively, in accord with the second prediction. Together, these results support the hypothesis that acoustic signals of bats potentially contain detailed information about parasite infections.
Our study shows that acoustic signals of R. sinicus appear to serve as reliable indicators of a caller’s parasite infection intensity. Specifically, bats infected with more gamasid mites emitted distress calls with narrower bandwidth and echolocation pulses with shorter duration (manifested as shorter pulse duration and shorter duration of initial frequency-modulated component), and bats with more bat flies emitted distress calls with longer pause duration (mean lower syllable rate). In fact, similar results have been found in previous studies. Redpath et al. (2000) found that male tawny owls (Strix aluco) suffering from higher parasite burdens emitted songs with a narrower bandwidth compared to individuals with fewer parasites. Both in barn swallows (Hirundo rustica) and sedge warblers (Acrocephalus schoenobaenus), the song duration and rate of male individuals markedly decreased as the intensity of parasitic infection increased (Møller, 1991; Buchanan et al., 1999; Garamszegi et al., 2005). These changes in acoustic signals may result from the parasite-stimulated immune response. More specifically, the immune response is an effective defense against parasites but is also expensive to maintain, such that heavily parasitized individuals may be forced to draw a large portion of energy away from vocalizations and toward immune response, and thus may not have sufficient energy to enable them to produce costly calls (e.g., calls with longer duration, higher call rate, or wider bandwidth) (Redpath et al., 2000; Garamszegi et al., 2005; Nouri and Blumstein, 2019). Alternatively, previous research has shown that parasites can induce a variety of effects in some brain regions involved in vocalization (Gilman et al., 2007; Adamo, 2013), and it is possible that parasite infections impact the ability of an infected individual’s brain to produce “proper” calls.
In this study, we found that mite loads were negatively correlated with bandwidth, while bat-fly loads were positively correlated with pause duration, results that supported the potential of distress calls of R. sinicus to encode information concerning infection with either gamasid mites or bat flies. These results echo the findings of Gilman et al. (2007) that male mountain white-crowned sparrows infected with Leucocytozoon significantly increased song consistency, while males infected with Plasmodium significantly reduced song output, suggesting that bird songs may contain information on infection with specific parasites. A possible explanation accounting for the different influence of each parasite to acoustic signals is that different parasites have different life cycles, utilize different host tissues, and even differ in infectivity and virulence; these factors could impact brain function through different pathways and activate different parts of the immune system and thereby differentially affect the behavioral traits of their hosts (Wedekind, 1992; Gilman et al., 2007; Nouri and Blumstein, 2019). Indeed, previous studies have indicated that there are significant differences between gamasid mites and bat flies in life cycle and pathogenicity (Holz et al., 2018). However, further study investigating whether gamasid mites or bat flies can differently impact brain function or stimulate different immune responses remains to be conducted.
It is worth noting that echolocation pulses varied only in relation to mite load but not bat-fly load, suggesting that echolocation pulses are less likely to contain information on infection with either gamasid mites or bat flies as in distress calls. Several possible reasons can be suggested to explain this result. First, compared with social calls, echolocation pulses have a simpler acoustic structure, and thus a restricted acoustic space available for communication (Finger et al., 2017). Hence, although echolocation pulses may provide sufficient specificity to encode information about some simple traits, they may have limitations for encoding detailed information (Finger et al., 2017; Chaverri et al., 2018). Second, prior work has shown that echolocation pulses and social calls are controlled by different midbrain structures (Fenzl and Schuller, 2007; Liu et al., 2013). Therefore, it is possible that bat flies impact the midbrain structures that control social calls but do not affect the structures that control echolocation pulses. Finally, we do not rule out the possibility that bat-fly loads may have significant influence on the parameters that we did not measure.
Our study found that distress calls of R. sinicus varied not only as a function of parasitism but also with respect to body condition, and these results correspond with previous findings that distress calls can be condition dependent (Laiolo et al., 2004). Condition-dependent acoustic signals have been shown to play a major role in mediating various aspects of animals’ life histories through the transmitting of useful information (Luo et al., 2017; Nouri and Blumstein, 2019; Kelly et al., 2021). In a distress context, the information contained in distress calls may be profitable for both signalers and receivers, as suggested by previous studies (Laiolo et al., 2004, 2007; Nouri and Blumstein, 2019). That is, healthy callers may emit distress calls to signal their health and infection status and thus their ability to bear the costs of anti-predator defense, thereby possibly discouraging close predators. In contrast, predators might benefit from these calls by focusing on a more vulnerable prey in order to avoid wasting energy on pursuing prey with good anti-predator ability. Furthermore, vulnerable callers might conceivably solicit help from nearby conspecifics by signaling their vulnerability.
The ultrasonic echolocation pulses of R. sinicus extend well beyond the hearing range of most animal taxa (Luo et al., 2019). Therefore, it is reasonable to believe that echolocation pulses are less likely to mediate the information transfer between bats and their predators, as in distress calls. Echolocation pulses with high frequencies are not a good choice for attracting conspecifics to attack or scare away close predators, because these pulses will attenuate quickly as they travel through air (Luo et al., 2019). It is possible that bats give echolocation pulses just to scan their immediate environment in stressful situations. In contrast, parasite-condition dependent echolocation pulses might play an important role in other biological tasks. For example, a previous study found that female horseshoe bats were preferentially attracted to male echolocation pulses that indicated better body condition, a result that provided indirect support for the potential role of echolocation in mating (Puechmaille et al., 2014). If this is the case, female R. sinicus would assess the parasite infection status of males based on echolocation pulses and then select parasite-free or -resistant males in order to avoid the direct infection of parasites and obtain resistance genes for their offspring. Additionally, our study also found that echolocation pulses potentially provide information about body condition, forearm length and sex, factors that have been demonstrated to be important in the mate selection of bats (Puechmaille et al., 2014; Ward et al., 2014).
Conclusion
Our results suggest that both distress calls and echolocation pulses of R. sinicus potentially encode information about parasite infection intensity, and distress calls also reflect information on infection with specific parasites. However, further work is still needed to obtain more convincing evidence that acoustic signals provide detailed information on parasite infection which could be used by receivers to assess a caller’s condition in bats. First, similar studies in more bat species and individuals should be conducted. Second, in addition to ectoparasites, whether endoparasites (e.g., blood parasites, intestinal parasites) also have significant influence on the acoustic signals of bats need be investigated. Third, all parasite individuals should be identified to species level as far as possible, and then both the individual effects of a specific parasite species and the interactive impact of different parasite species on bat acoustic signals should be analyzed. Fourth, whether the effect of parasite infection on bat acoustic signals is different between different sexes should be investigated. Finally, future playback experiments are required to determine whether and how potential receivers respond to the acoustic signals from parasite-infected bat individuals.
Data Availability Statement
The original contributions presented in this study are included in the article/Supplementary Material, further inquiries can be directed to the corresponding authors.
Ethics Statement
The animal study was reviewed and approved by the Animals Ethics Committee of Dali University (approval number: DLDXLL2019-810).
Author Contributions
XH and TJ conceived and designed the experiments, and contributed to reagents, materials, and analysis tools. BF and YW performed the experiments and analyzed the data. BF, YW, and XH wrote the manuscript. All authors contributed to the article and approved the submitted version.
Funding
This research was supported by the National Natural Science Foundation of China (Grant Nos. 32001096, 31872680, and 31922050), Yunnan Provincial Department of Science and Technology (202001AT070025), and Major Science and Technique Programs in Yunnan Province (Grant No. 202102AA310055).
Conflict of Interest
The authors declare that the research was conducted in the absence of any commercial or financial relationships that could be construed as a potential conflict of interest.
Publisher’s Note
All claims expressed in this article are solely those of the authors and do not necessarily represent those of their affiliated organizations, or those of the publisher, the editors and the reviewers. Any product that may be evaluated in this article, or claim that may be made by its manufacturer, is not guaranteed or endorsed by the publisher.
Supplementary Material
The Supplementary Material for this article can be found online at: https://www.frontiersin.org/articles/10.3389/fevo.2022.908209/full#supplementary-material
References
Aalto, S. L., Decaestecker, E., and Pulkkinen, K. (2015). A three-way perspective of stoichiometric changes on host-parasite interactions. Trends Parasitol. 31, 333–340. doi: 10.1016/j.pt.2015.04.005
Adamo, S. A. (2013). Parasites: evolution’s neurobiologists. J. Exp. Biol. 216, 3–10. doi: 10.1242/jeb.073601
Barton, K. (2015). MuMIn: Multi-Model Inference. R Package Version 1134. Available Online at: http.CRANR-project.org/package=MuMIn. CRANR-project. org/package= MuMIn (accessed December, 2021).
Bischoff, L. L., Tschirren, B., and Richner, H. (2009). Long-term effects of early parasite exposure on song duration and singing strategy in great tits. Behav. Ecol. 20, 265–270. doi: 10.1093/beheco/arp012
Bommarco, R., Lindborg, R., Marini, L., and Öckinger, E. (2014). Extinction debt for plants and flower-visiting insects in landscapes with contrasting land use history. Divers. Distrib. 20, 591–599. doi: 10.1111/ddi.12187
Brunet-Rossinni, A., Wilkinson, G., Kunz, T., and Parsons, S. (2009). “Methods for age estimation and the study of senescence in bats,” in Ecological and Behavioral Methods for the Study of Bats, ed. T. H. Kunz (Baltimore, MD: The John Hopkins University Press), 315–325.
Buchanan, K. L., Catchpole, C., Lewis, J., and Lodge, A. (1999). Song as an indicator of parasitism in the sedge warbler. Anim. Behav. 57, 307–314. doi: 10.1006/anbe.1998.0969
Carter, G. G., and Wilkinson, G. S. (2016). Common vampire bat contact calls attract past food-sharing partners. Anim. Behav. 116, 45–51. doi: 10.1016/j.anbehav.2016.03.005
Chaverri, G., Ancillotto, L., and Russo, D. (2018). Social communication in bats. Biol. Rev. 93, 1938–1954. doi: 10.1111/brv.12427
Dias, D. (1982). Contribuicao para o conhecimento dos ectoparasitas de quir opteros de Portugal (Acarina: Macronyssidae e Spinturnicidae). An. Inst. Hig. Med. Trop. 8, 3–14.
Fenton, M. B. (2003). Eavesdropping on the echolocation and social calls of bats. Mamm. Rev. 33, 193–204. doi: 10.1046/j.1365-2907.2003.00019.x
Fenzl, T., and Schuller, G. (2007). Dissimilarities in the vocal control over communication and echolocation calls in bats. Behav. Brain Res. 182, 173–179. doi: 10.1016/j.bbr.2006.12.021
Finger, N. M., Bastian, A., and Jacobs, D. S. (2017). To seek or speak? Dual function of an acoustic signal limits its versatility in communication. Anim. Behav. 127, 135–152. doi: 10.1016/j.anbehav.2017.03.005
Galeotti, P., Sacchi, R., Fasola, M., Rosa, D. P., Marchesi, M., and Ballasina, D. (2005). Courtship displays and mounting calls are honest, condition-dependent signals that influence mounting success in Hermann’s tortoises. Can. J. Zool. 83, 1306–1313. doi: 10.1139/z05-130
Garamszegi, L. Z. (2005). Bird song and parasites. Behav. Ecol. Sociobiol. 59, 167–180. doi: 10.1007/s00265-005-0041-8
Garamszegi, L. Z., Heylen, D., Møller, A. P., Eens, M., and de Lope, F. (2005). Age-dependent health status and song characteristics in the barn swallow. Behav. Ecol. 16, 580–591. doi: 10.1093/beheco/ari029
Gilman, S., Blumstein, D. T., and Foufopoulos, J. (2007). The effect of hemosporidian infections on white-crowned sparrow singing behavior. Ethology 113, 437–445. doi: 10.1111/j.1439-0310.2006.01341.x
Grueber, C. E., Nakagawa, S., Laws, R. J., and Jamieson, I. G. (2011). Multimodel inference in ecology and evolution: challenges and solutions. J. Evol. Biol. 24, 699–711. doi: 10.1111/j.1420-9101.2010.02210.x
Holz, P. H., Lumsden, L. F., and Hufschmid, J. (2018). Ectoparasites are unlikely to be a primary cause of population declines of bent-winged bats in south-eastern Australia. Int. J. Parasitol. Parasites 7, 423–428. doi: 10.1016/j.ijppaw.2018.10.006
Huang, X., Metzner, W., Zhang, K., Wang, Y., Luo, B., Sun, C., et al. (2018). Acoustic similarity elicits responses to heterospecific distress calls in bats (Mammalia: Chiroptera). Anim. Behav. 146, 143–154. doi: 10.1016/j.anbehav.2018.10.018
Jiang, T., Huang, X., Hui, W., and Jiang, F. (2017). Size and quality information in acoustic signals of Rhinolophus ferrumequinum in distress situations. Physiol. Behav. 173, 252–257. doi: 10.1016/j.physbeh.2017.02.025
Jiang, T., Wang, J., Wu, H., Csorba, G., Puechmaille, S. J., Benda, P., et al. (2019). The patterns and possible causes of global geographical variation in the body size of the greater horseshoe bat (Rhinolophus ferrumequinum). J. Biogeogr. 46, 2363–2377. doi: 10.1111/jbi.13658
Kanwal, J. S., Matsumura, S., Ohlemiller, K., and Suga, N. (1994). Analysis of acoustic elements and syntax in communication sounds emitted by mustached bats. J. Acoust. Soc. Am. 96, 1229–1254. doi: 10.1121/1.410273
Kelly, P. W., Pfennig, D. W., and Pfennig, K. S. (2021). A condition-dependent male sexual signal predicts adaptive predator-induced plasticity in offspring. Behav. Ecol. Sociobiol. 75:28. doi: 10.1007/s00265-021-02968-8
Laiolo, P., Serrano, D., Tella, J. L., Carrete, M., Lopez, G., and Navarro, C. (2007). Distress calls reflect poxvirus infection in lesser short-toed lark Calandrella rufescens. Behav. Ecol. 18, 507–512. doi: 10.1093/beheco/arm008
Laiolo, P., Tella, J. L., Carrete, M., Serrano, D., and López, G. (2004). Distress calls may honestly signal bird quality to predators. Proc. Royal Soc. B 271, 513–515. doi: 10.1098/rsbl.2004.0239
Liu, Y., Feng, J., and Metzner, W. (2013). Different auditory feedback control for echolocation and communication in horseshoe bats. PLoS One 8:e62710. doi: 10.1371/journal.pone.0062710
Luo, B., Leiser-Miller, L., Santana, S. E., Zhang, L., Liu, T., Xiao, Y., et al. (2019). Echolocation call divergence in bats: a comparative analysis. Behav. Ecol. Sociobiol. 73:154. doi: 10.1007/s00265-019-2766-9
Luo, B., Lu, G., Chen, K., Guo, D., Huang, X., Liu, Y., et al. (2017). Social calls honestly signal female competitive ability in Asian particoloured bats. Anim. Behav. 127, 101–108. doi: 10.1016/j.anbehav.2017.03.012
Luong, L. T., Hudson, P. J., and Braithwaite, V. A. (2011). Parasite-induced changes in the anti-predator behavior of a cricket intermediate host. Ethology 117, 1019–1026. doi: 10.1111/j.1439-0310.2011.01951.x
Lynsdale, C. L., Mumby, H. S., Hayward, A. D., Mar, K. U., and Lummaa, V. (2017). Parasite-associated mortality in a long-lived mammal: variation with host age, sex, and reproduction. Ecol. Evol. 7, 10904–10915. doi: 10.1002/ece3.3559
Maa, T. (1962). Records and descriptions of Nycteribiidae and Streblidae (Diptera). Pac. Insects 4, 417–436.
Mac Nally, R. (2002). Multiple regression and inference in ecology and conservation biology: further comments on identifying important predictor variables. Biodivers. Conserv. 11, 1397–1401. doi: 10.1023/A:1016250716679
Madelaire, C., Da Silva, R., and Gomes, F. (2014). Calling behavior and parasite intensity in treefrogs, Hypsiboas prasinus. J. Herpetol. 47, 450–455. doi: 10.1670/11-315
Manser, M. B., and Avey, G. (2000). The effect of pup vocalisations on food allocation in a cooperative mammal, the meerkat (Suricata suricatta). Behav. Ecol. Sociobiol. 48, 429–437. doi: 10.1007/s002650000248
Møller, A. P. (1991). Parasite load reduces song output in a passerine bird. Anim. Behav. 41, 723–730. doi: 10.1016/S0003-3472(05)80909-1
Nally, R., and Walsh, C. J. (2004). Hierarchical partitioning public-domain software. Biodivers. Conserv. 13, 659–660. doi: 10.1023/B:BIOC.0000009515.11717.0b
Nouri, K., and Blumstein, D. T. (2019). Parasites are associated with noisy alarm calls. Front. Ecol. Evol. 7:28. doi: 10.3389/fevo.2019.00028
Perez, E. C., Mariette, M. M., Cochard, P., Soulage, C. O., Griffith, S. C., and Vignal, C. (2016). Corticosterone triggers high-pitched nestlings’ begging calls and affects parental behavior in the wild zebra finch. Behav. Ecol. 27, 1665–1675. doi: 10.1093/beheco/arw069
Puechmaille, S. J., Borissov, I. M., Zsebok, S., Allegrini, B., Hizem, M., Kuenzel, S., et al. (2014). Female mate choice can drive the evolution of high frequency echolocation in bats: a case study with Rhinolophus mehelyi. PLoS One 9:e103452. doi: 10.1371/journal.pone.0103452
Radovsky, F. J. (1967). The Macronyssidae and Laelapidae (Acarina: Mesostigmata) Parasitic on Bats. Berkeley & Los Angeles: University of California Press.
Redpath, S. M., Appleby, B. M., and Petty, S. J. (2000). Do male hoots betray parasite loads in tawny owls? J. Avian Biol. 31, 457–462. doi: 10.1034/j.1600-048X.2000.310404.x
Rogalski, M. A., and Duffy, M. A. (2020). Local adaptation of a parasite to solar radiation impacts disease transmission potential, spore yield, and host fecundity. Evolution 74, 1856–1864. doi: 10.1111/evo.14064
Romano, M. C., Jiménez, P., Miranda, C., and Valdez, R. A. (2015). Parasites and steroid hormones: corticosteroid and sex steroid synthesis, their role in the parasite physiology and development. Front. Neurosci. 9:224. doi: 10.3389/fnins.2015.00224
Siemers, B. M., Beedholm, K., Dietz, C., Dietz, I., and Ivanova, T. (2005). Is species identity, sex, age or individual quality conveyed by echolocation call frequency in European horseshoe bats? Acta Chiropt. 7, 259–274. doi: 10.3161/150811005775162579
Sun, C., Zhang, C., Lucas, J. R., Lin, A., Feng, J., and Jiang, T. (2021). Territorial calls of the bat Hipposideros armiger may encode multiple types of information: body mass, dominance rank and individual identity. Anim. Cogn. 24, 689–702. doi: 10.1007/s10071-020-01455-3
Sun, K. (2019). Rhinolophus sinicus. The IUCN Red List of Threatened Species: e.T41529A22005184. Available Online at: https://dx.doi.org/10.2305/IUCN.UK.2019-3.RLTS.T41529A22005184.en. (accessed Mar 30, 2022).
Voigt-Heucke, S. L., Taborsky, M., and Dechmann, D. K. (2010). A dual function of echolocation: bats use echolocation calls to identify familiar and unfamiliar individuals. Anim. Behav. 80, 59–67. doi: 10.1016/j.anbehav.2010.03.025
Ward, H. L., Ransome, R. D., Jones, G., and Rossiter, S. J. (2014). Determinants and patterns of reproductive success in the greater horseshoe bat during a population recovery. PLoS One 9:e87199. doi: 10.1371/journal.pone.0087199
Wedekind, C. (1992). Detailed information about parasites revealed by sexual ornamentation. Proc. Royal Soc.B 247, 169–174. doi: 10.1098/rspb.1992.0024
Whiteman, N. K., and Parker, P. G. (2004). Effects of host sociality on ectoparasite population biology. J. Parasitol. 90, 939–947. doi: 10.1645/ge-310r
Wilkins, M. R., Seddon, N., and Safran, R. J. (2013). Evolutionary divergence in acoustic signals: causes and consequences. Trends Ecol. Evol. 28, 156–166. doi: 10.1016/j.tree.2012.10.002
Keywords: Rhinolophus sinicus, echolocation pulses, distress calls, gamasid mites, bat flies
Citation: Fan B, Wang Y, Huang X, Zhang X, Yang J and Jiang T (2022) The Potential to Encode Detailed Information About Parasites in the Acoustic Signals of Chinese Horseshoe Bats (Rhinolophus sinicus). Front. Ecol. Evol. 10:908209. doi: 10.3389/fevo.2022.908209
Received: 30 March 2022; Accepted: 20 June 2022;
Published: 13 July 2022.
Edited by:
Han Li, University of Nebraska Omaha, United StatesReviewed by:
David Martín-Gálvez, Complutense University of Madrid, SpainGregory Mutumi, University of California, Merced, United States
Copyright © 2022 Fan, Wang, Huang, Zhang, Yang and Jiang. This is an open-access article distributed under the terms of the Creative Commons Attribution License (CC BY). The use, distribution or reproduction in other forums is permitted, provided the original author(s) and the copyright owner(s) are credited and that the original publication in this journal is cited, in accordance with accepted academic practice. No use, distribution or reproduction is permitted which does not comply with these terms.
*Correspondence: Xiaobin Huang, aHVhbmd4YjYzM0BuZW51LmVkdS5jbg==; Tinglei Jiang, amlhbmd0bDczMEBuZW51LmVkdS5jbg==
†These authors have contributed equally to this work and share first authorship