- 1Laboratório de Ciência Aplicada à Conservação da Biodiversidade, Departamento de Zoologia, Universidade Federal de Pernambuco, Recife, Brazil
- 2Programa de Pós-Graduação em Biologia Animal, Universidade Federal de Pernambuco, Recife, Brazil
- 3Bat Lab Finland, Finnish Museum of Natural History, University of Helsinki, Helsinki, Finland
Bat caves in the Neotropical region harbor exceptional bat populations (> 100,000 individuals). These populations play a wider role in ecological interactions, are vulnerable due to their restriction to caves, and have a disproportionate conservation value. Current knowledge of bat caves in Brazil is still small. However, systematic monitoring of some bat caves in northeastern Brazil shows that they experience strong population fluctuations over short periods of time, suggesting large-scale movements between roosts and a much broader use of the landscape than previously considered. Spatio-temporal reproductive connectivity between distant populations would change our understanding of the use of roosts among bat species in Brazil, and important gaps in knowledge of long-distance bat movements in the country would be filled. Here, we used ddRADseq data to analyze the genetic structure of Pteronotus gymnonotus across nine bat caves over 700 km. Our results indicate the lack of a clear geographic structure with gene flow among all the caves analyzed, suggesting that P. gymnonotus uses a network of bat caves geographically segregated hundreds of kilometers apart. Facing strong anthropogenic impacts and an underrepresentation of caves in conservation action plans worldwide, the genetic connectivity demonstrated here confirms that bat caves are priority sites for bat and speleological conservation in Brazil and elsewhere. Moreover, our results demonstrate a warning call: the applied aspects of the environmental licensing process of the mining sector and its impact must be reviewed, not only in Brazil, but wherever this licensing process affects caves having exceptional bat populations.
Introduction
Caves are among the most used shelters by bats and are critical to the survival of several species worldwide (Furey and Racey, 2016). Although the number of bats in a cave can vary drastically, the largest aggregation of warm-blooded vertebrates is found in caves, reaching millions of individuals at a single site (Betke et al., 2008; Medellín et al., 2008). The locations that provide shelter for these large bat populations are known as bat caves and have been documented in some places across the world (Ladle et al., 2012; Furey and Racey, 2016). In the neotropics, bat caves have been documented in Mexico, Central America and the Caribbean, and in some South American countries (e.g., Corso et al., 2010; Ladle et al., 2012; Medellín et al., 2017; Otálora-Ardila et al., 2019).
In Brazil, large colonies found in bat caves are predominantly made up of Pteronotus gymnonotus (e.g., Rocha et al., 2011; Vargas-Mena et al., 2018; Otálora-Ardila et al., 2019), an insectivorous and strictly cave-dwelling species from the Mormoopidae family (de la Torre and Medellín, 2010; Pavan and Tavares, 2019). Systematic monitoring of some of these caves indicates that while some of them appear to maintain resident populations throughout the year, in others, populations may experience large fluctuations in short periods of time (Otálora-Ardila et al., 2019; Leal and Bernard, 2021). Such variations suggest the existence of a massive displacement of bats between different shelters. Thus, bats in one cave could leave the shelter and move as a group to another bat cave, leading to a far broader use of the landscape than previously considered. In fact, mark/recapture studies have proven displacements of up to 15 km between bat caves in the state of Pernambuco, Brazil (Leal and Bernard, 2021). However, could those bats be moving further away? Data for Leptonycteris yerbabuenae and Tadarida brasiliensis have indicated movements between summer bat caves in the USA and winter bat caves in Mexico of 1,000–1,600 km for the first species and >2,500 km for the second (Cole and Wilson, 2006; Wiederholt et al., 2013; Medellín et al., 2017). With such a dynamic use of roost site, some bat caves should be treated as a single management unit for both genetic, conservation, and environmental service purposes (e.g., Wiederholt et al., 2015, 2017; López-Hoffman et al., 2017a,b; Medellín et al., 2017).
Sites with large animal congregations—such as bat caves—are considered exceptional ecological and conservation locations because (1) they have a disproportionate value for the survival of the species in question, (2) species can be particularly vulnerable when present in such large congregations, and (3) because they are numerically abundant, those individuals participate in a wide range of ecological interactions (Mittermeier et al., 2003). Bat caves have all such characteristics, and their ecological and conservation importance is recognized in a few countries. For instance, in Mexico, bat caves are key sites for the conservation of nectarivorous species such as Leptonycteris curasoae and the endangered L. yerbabuenae (Arita and Santos-del-Prado, 1999; Stoner et al., 2003; Galindo et al., 2004); in Brazil, bat caves have legal protection status (Brasil, 2022) and special attention during environmental licensing; and the millions of Mexican free-tailed bats in bat caves in the USA provide an ecosystem service of pest control worth $3.42 billion/year (Betke et al., 2008; Medellín et al., 2017) justifying their economical protection.
Although research on caves and their conservation status is increasing worldwide (Medellín et al., 2017), the roadmap for the conservation of the subterranean biome highlights reliable scientific information that can help identify priority areas for cave conservation (Mammola et al., 2020; Wynne et al., 2021). This is especially true in Brazil, where caves are at high risk due to relaxation of protection laws (Bernard et al., 2021). Moreover, studies on caves—and especially bat caves—are still scarce in Brazil despite the richness of species and the ecological importance of these animals (De Oliveira et al., 2018; Silva et al., 2018; Barros et al., 2020; Delgado-Jaramillo et al., 2020). Caves are also highly susceptible to various impacts, such as mining and agricultural activities (Furey and Racey, 2016), both economically important and widespread sectors in Brazil and Latin America (Bernard et al., 2012, 2021). For instance, the protection of caves has a central role in the licensing process of mining activities in Brazil, setting such habitats under strong pressure. Furthermore, recent attempts to dismantle environmental regulations in Brazil have made matters worse, further stressing the urgent need to better understand and protect Brazilian cave heritage (Bernard et al., 2021; Ferreira et al., 2022). This is especially true for northeastern Brazil, a region that simultaneously harbors a high richness of bat species (potentially 100 species—Silva et al., 2018; Delgado-Jaramillo et al., 2020), a great potential for the occurrence of caves (Jansen et al., 2012), but where the main biome (Caatinga) has already lost ~50% of its original natural cover, and is suspected of chronic anthropogenic disturbance (Antongiovanni et al., 2020). Moreover, more than 90% of the wind turbines in operation in Brazil are installed in the Northeast, setting conflicts for biodiversity conservation (Neri et al., 2019) and bats in particular (Bernard et al., 2014; Valença and Bernard, 2015).
Although the population structure in bats is often relatively low due to their ability to fly and cross some ecological barriers impossible for other animals (Laine et al., 2013), at large geographical scales distance can be significantly correlated with genetic distance, thus the population structure can be observed (e.g., Martins et al., 2007; Hua et al., 2013; Lilley et al., 2020). In fact, phylogeographic and population studies of bats in the Neotropical region reveal several cases of genetic structure and cryptic diversity (e.g., Martins et al., 2007; Pavan and Marroig, 2016, 2017; Moras et al., 2018; Garbino et al., 2020). In this scenario, genomic data can assist in conservation studies (Dool, 2020) and shed some insights on the genetic structure and diversity of a species, as well as the large-scale movements of individuals at the landscape level (e.g., Sovic et al., 2016; Gong et al., 2019; Pinzari et al., 2020; Luna et al., 2021).
Here, we used genomic data to assess the genetic structure of P. gymnonotus colonies from nine bat caves along 700 km in northeastern Brazil. We hypothesized that, due to strong population fluctuations observed in some bat caves, bats use networks of caves as roosts and thus no strong population structure would be observed. If spatio-temporal reproductive connectivity between populations hundreds of kilometers apart is identified, it will fill important gaps in knowledge of long-distance bat movements in Brazil. Moreover, the legal status of those caves and their populations will have to be revised, as they have to be considered priority areas for the conservation of the biological and speleological heritage of Brazil.
Methods
Sample collection and library construction
Nine colonies of P. gymnonotus from bat caves in the Brazilian states of Ceará, Rio Grande do Norte, Pernambuco, and Sergipe were sampled in July 2019 (Figure 1, Table 1). The distance to the nearest bat cave ranged from 15 km (PEMR to PEFM) up to 196 km (RNGA to RNCU) (Table 1), and the distance from the southernmost to the northernmost cave was 700 km. Colony size estimates were made using a non-invasive thermal detection system (see Otálora-Ardila et al., 2019; Pimentel et al., 2022), with two counts per cave. Following the guidelines of the American Society of Mammalogists, the bats were captured with a hand net inside the cave and euthanized, and liver tissue samples were collected from ~20 adult individuals in each cave (Sikes and Animal Care and Use Committee of the American Society of Mammalogists, 2016). Tissue samples were stored in 1.5-ml tubes with 95% EtOH and stored at −80°C until further analysis. All specimens were deposited in the Mammal Collection of UFPE as vouchers. The project activities were conducted under the SISBIO/ICMBio permit 68992-1, registered in SisGen under the protocol A974BB7 and approved by the Commission on Ethics and Animal Use of Federal University of Pernambuco (CEUA-UFPE 114/2019).
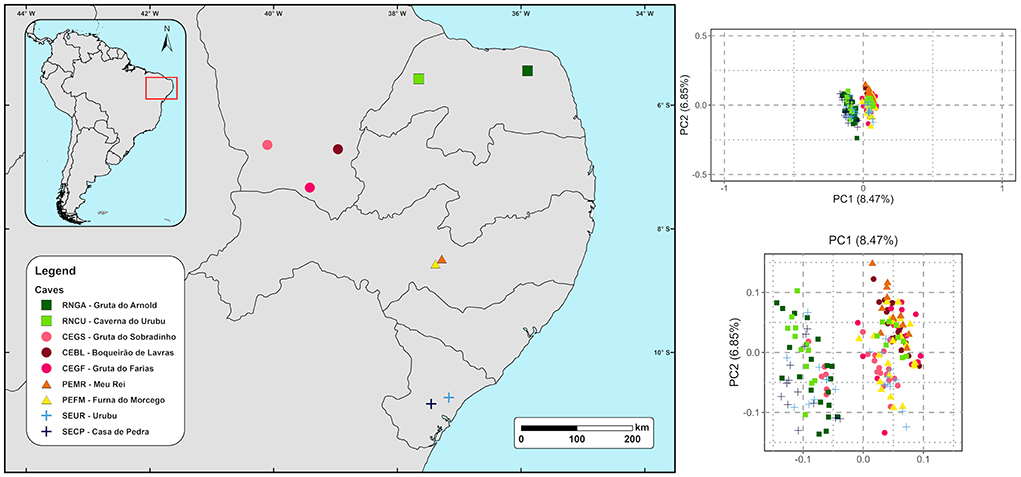
Figure 1. Bat caves in northeastern Brazil where Pteronotus gymnonotus bats were sampled (Left) and the genetic structure of the species shown by a principal component analysis (PCA) (Right). The two most important components explain 15.32% of the nucleotide variation for the species.
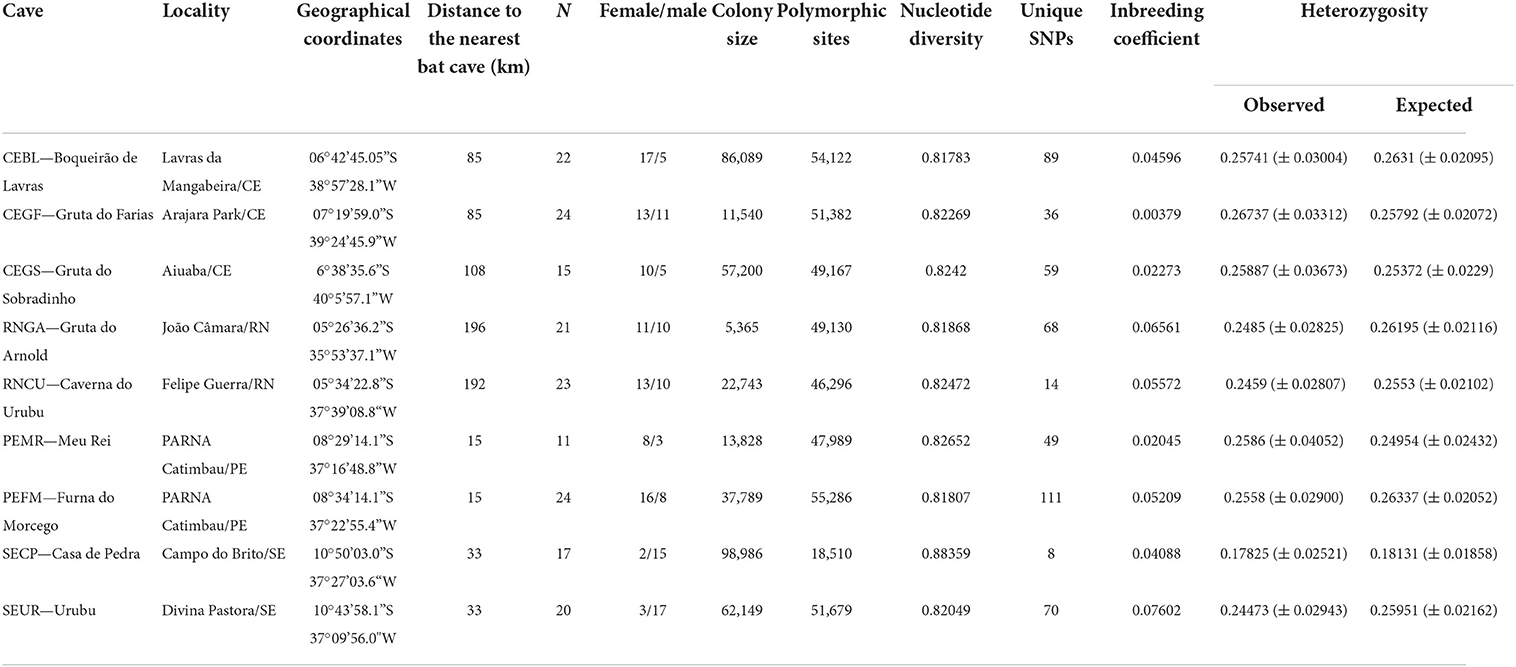
Table 1. Geographical and population data for Pteronotus gymnonotus per bat cave in northeastern Brazil, including sampling location, the number of genetic samples collected (N), and the division of samples by gender (female/male), estimated colony size, and genetic diversity information for each cave.
Genomic deoxyribonucleic acid (DNA) was extracted using the Biopur Mini Spin Plus kit (Biometrix) according to the manufacturers' protocols. All extractions were performed at Instituto Aggeu Magalhães, FIOCRUZ/Pernambuco and stored at −80°C before being sent to LUOMUS, Helsinki/Finland, for the following analyses. The amount of DNA from each sample was tested and quantified using the Thermo Scientific Nanodrop spectrophotometer, and all samples were diluted into a 12 ng/ml solution, following Lilley et al. (2020).
Deoxyribonucleic acid was prepared for genotyping-by-sequencing using a double digestion RAD-seq method adapted from Elshire et al. (2011) and Lemopoulos et al. (2018) for the use of low-concentration samples. Therefore, 180 ng of each DNA extract was used in a reaction together with 20 U of each of the restriction enzymes PstI-HF™ and BamHI (New England Biolabs) and 2 μl of CutSmart Buffer. PstI-BamHI-digested libraries were prepared and sequenced using the Illumina Novaseq6000 over two lanes with 100 bp single-end reads by Bioname Oy. Details of the library preparation methods and sequencing runs are provided as Supplementary Information, and raw data are available from the NCBI SRA archive under the bio project PRJNA824143.
Bioinformatic processing
The resulting reads were processed according to Lilley et al. (2020), and the complete scripts and pipelines are available at https://github.com/itofs/Pgymno_radseq. Demultiplexing and quality filtering were carried out using Stacks 1.48 (Catchen et al., 2013) “process_radtags.” Trimming of barcodes, low-quality regions, and uncalled bases was performed, followed by a minimum read length filter of 30 bp. After quality control, reads were mapped against the Pteronotus mesoamericanus genome (Scheben et al., 2020), using BWA mem 0.7.17 with parameters -B 3 -O 5 -k 15 (Li and Durbin, 2009). Stacks 1.48 (Catchen et al., 2013) pipeline “ref_map.pl” was used for single nucleotide polymorphism (SNP) calls and filtering. Several stack depths were tested to optimize analyses (Catchen et al., 2013), with a value of 3 being used for a downstream analysis. The samples were assigned to nine different populations, representing the bat caves where they were collected. SNPs with a low (≤5) or high (≥125) coverage were excluded, and only biallelic SNPs were used in subsequent analyses (Lilley et al., 2020).
Data analysis
After sequencing data processing and SNP filtering, principal component analysis (PCA) was performed using Plink 1.09 (Purcell et al., 2007). The inference of individual ancestry coefficients was conducted using two approaches: the first is based on sparse non-negative matrix factorization (NMF) and least-squares optimization, implemented in the sNMF software (Frichot et al., 2014); and the second with ADMIXTURE, which is based on likelihood models with the quasi-Newton convergence acceleration method (Alexander et al., 2009). Considering each of the nine caves as a population, sNMF runs were performed for values of the number of clusters fixed at K = 1–10 and the regularization parameter (α) varying between 0 and 1,000. The cross-entropy criterion was used to select the best K. For ADMIXTURE, possible K values ranging from 1 up to 9 were evaluated, representing the bat caves analyzed, and 10 runs were performed for each K number.
Nei's pairwise FST, the expected (HE) and observed (HO) heterozygosity, nucleotide diversity (π), and inbreeding coefficient were calculated with the populations program in Stacks 1.48 (Catchen et al., 2013), using each cave as a population. FST is a standardized variance, which represents the portion of the total genetic variance that is due to differences between subpopulations (Hartl and Clark, 1997). FST values of 0 to 0.05 were considered poorly differentiated, and 0.05 to 0.15 moderately differentiated, respectively, whereas FST values > 0.15 were considered distinctly differentiated (Hartl and Clark, 1997). The latitude and longitude coordinates of the sampling locations were used to calculate the pairwise geographic distances between the caves in kilometers using the Haversine method, assuming a spherical earth, implemented in the function distm in the R package geodist v. 1.5.10. Then, isolation by distance was estimated with a Mantel test with complete permutations, using the pairwise FST as a measure of genetic distances and distances between the caves as geographic coordinates for populations and considering α = 0.05.
Results
Colony size estimates ranged from 5,365 bats at Gruta do Arnold (RNGA) to 98,986 bats at Casa de Pedra (SECP), confirming that all of those caves harbor large populations (Table 1). We analyzed samples from 177 individuals of P. gymnonotus, 84 males and 93 females (Table 1). The number of samples per cave varied from 11 for the Meu Rei cave in Pernambuco to 24 bats for the Gruta do Farias cave, Ceará, and Furna do Morcego, in Pernambuco (Table 1). In total, the ddRADseq yielded an average of 1.6 million single-end raw reads. After quality filtering and SNP calls with Stacks, we obtained 62,644 biallelic genotypes and 26,091 SNPs were retained after pruning the data sets of variants that were linked (Purcell et al., 2007).
In the PCA, the first two axes explained 15.32% of the data variation (Figure 1), and based on the two axes there is no clear geographic structure among P. gymnonotus sampled in the nine bat caves, along 700 km. Although at a fine scale PC1 generated two clusters (Figure 1, bottom panel at right), the variation between them is not very pronounced and does not represent a case of isolation by distance. In fact, cluster one covers samples from five caves, while the second cluster has samples from seven of the nine caves analyzed (Supplementary Figure S1). Additionally, individuals from the northernmost cave (RNGA) are grouping together with bats from the southernmost bat cave (SECP). Also, the individuals from the caves located at intermediate points of the sampling area (PEFM and PEMR) are clustering with bats from the north (RNCU), west (CEGS, CEBL, and CEGF), and south caves (SEUR; Supplementary Figure S1), which indicates that the genetic distance observed here for P. gymnonotus has no correlation with the geographical distance between the caves. Moreover, individuals from the RNCU, CEGS, and SEUR caves are found in both clusters, despite the geographical distance between them. Therefore, bats in these three caves are connected to bats in all of the caves analyzed. Genetic connectivity between cave populations is also observed in both sNMF and ADMIXTURE (Figure 2), where K = 1 shows the lowest cross-entropy (Figure 3). Thus, each individual bat belongs to the same population and has the contributions of a single ancestral population, K = 1.
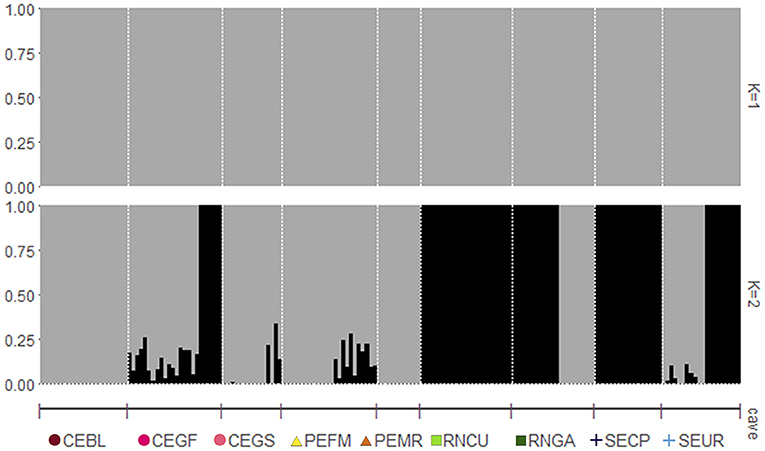
Figure 2. An ADMIXTURE analysis shows the proportion of ancestry for P. gymnonotus from nine bat caves in northeastern Brazil. Each vertical bar represents an individual, ordered by cave. Vertical bars with both colors indicate individuals of mixed ancestry. Plots represent individuals from one ancestral population (K = 1; at the top) and two ancestral populations (K = 2; at the bottom). For complete cave names, please see Table 1.
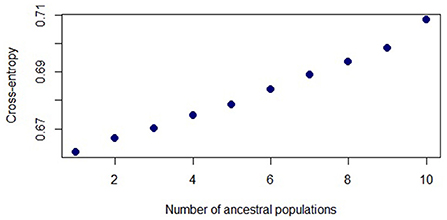
Figure 3. sNMF plot shows the values of cross-entropy for each K (number of ancestral populations) calculated for P. gymnonotus from nine bat caves in northeastern Brazil. K = 1 has the lowest cross-entropy.
Overall, pairwise FST was low when considering each cave as a population (Table 2), with a range from 0.012 (SEUR~CEGF) to 0.029 (PEMR~SECP). As FST represents the portion of the total genetic variance that is due to differences between subpopulations (Weir, 1996), the low values observed here indicate no genetic differentiation between colonies. This also suggests the existence of gene flow between P. gymnonotus colonies, which is supported by the low values of the inbreeding coefficient in all populations analyzed, which ranged from 0.003 to 0.076 (Table 1). Furthermore, the observed (HO) and expected (HE) heterozygosity ranged from 0.178 to 0.267 and from 0.181 to 0.263, respectively. We did not find a strong correlation between genetic and geographical distance, as estimated by the Mantel test: r = 0.1372 (p = 0.01).
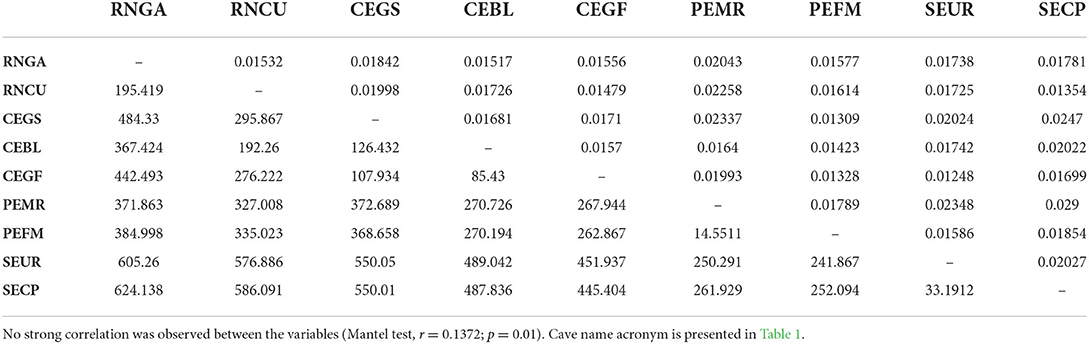
Table 2. Pairwise FST (above the diagonal) and pairwise geographical distance (below the diagonal) between subpopulations of P. gymnonotus from nine bat caves in northeastern Brazil.
Discussion
Using an individual-based approach with genome-wide markers, we conducted population genetic analyses of P. gymnonotus bats sampled from nine geographically distant caves in northeastern Brazil, and our results indicate that these bats do not present a clear geographical structure. Although some caves are separated by 700 km, the large bat populations analyzed show a poor pattern of isolation by distance and present high gene flow between bat caves. Our results help better understand the large population fluctuations reported in some bat caves (Otálora-Ardila et al., 2019) and confirmed our initial hypothesis that P. gymnonotus move between distant bat caves, using them as a network of reproductive roosts. As shown in the PCA, individuals from the SEUR, CEGS, and RNCU caves had close genetic proximity to individuals from all sampled caves, while samples from the PEMR and PEFM caves were genetically similar to samples from the six other caves. Although this did not happen in all caves, the bats sampled in one cave are similar to populations from at least four different caves, suggesting that these animals move between the analyzed roosts. Based on our findings, the current understanding of how these bats use the landscape needs to be revised, as the exchange of genetic material between bats sampled in distant caves indicates a much broader use of the landscape than previously understood.
Pteronotus gymnonotus is a relatively mobile species (Pavan and Tavares, 2019), with the broadest latitudinal range among mormoopid bats, and is found in several different habitats (Pavan and Tavares, 2019). The wide distribution range could contribute to the genetic population structure (Burland and Wilmer, 2001), as an array of factors can affect the extent of genetic partitioning among populations, including dispersal ability and geographic barriers (Burland and Wilmer, 2001). In fact, phylogeographic and population studies of bats in the Neotropical region reveal several cases of genetic structure and cryptic diversity, such as in the genera Chiroderma, Myotis, and Pteronotus (e.g., Martins et al., 2007; Pavan and Marroig, 2016, 2017; Moras et al., 2018; Garbino et al., 2020; Novaes et al., 2021). The genetic structure of bat populations in Brazil is poorly understood, but some available data indicate a strong population structure between the populations analyzed (e.g., Carstens et al., 2004; Pavan and Marroig, 2016, 2017; Moras et al., 2018; Garbino et al., 2020). However, our results indicate that P. gymnonotus occurs as a strongly connected population in northeastern Brazil, with a poor correlation between geographic and genetic distances.
Estimates of the inbreeding coefficient and genetic differentiation of P. gymnonotus presented here suggest strong gene flow between caves on a broad geographic scale. Thus, together with population fluctuations (Otálora-Ardila et al., 2019) and movements previously observed for Pteronotus in some of the caves that contribute to our study (Leal and Bernard, 2021), these results suggest a very dynamic use of roosts. These observed fluctuations had no correlation with the amount of rainfall or the environmental conditions around the caves (Otálora-Ardila et al., 2019) and nursery colonies have been observed in some, but not all, of the bat caves analyzed (Otálora-Ardila et al., 2019; Barros et al., 2020; Leal and Bernard, 2021; Pimentel, 2021). Therefore, the lack of correlation between genetic and geographic distances, along with the existence of gene flow between the colonies observed here, suggests that reproduction-related displacements can be a driver for better understanding extreme population fluctuations already observed.
Movements associated with sexual segregation are known to occur in bat species in the family Mormoopidae, with female philopatry and male-biased dispersal (Pavan et al., 2019). Similar patterns are also observed in other species that form bat caves, such as T. brasiliensis (Llaven-Macías et al., 2021) and L. curasoae (Galindo et al., 2004). These species can be found in bat caves in Mexico, and their movements between roosts are also related to reproductive periods (Galindo et al., 2004; Llaven-Macías et al., 2021). The free-tailed bat (T. brasiliensis) forms the largest colonies ever reported for mammals, up to ~2 million individuals (Betke et al., 2008), and uses a roost network along the southwestern USA and the south of Mexico (Llaven-Macías et al., 2021). The species migration hypothesis proposes that northward migration is mostly undertaken by female individuals, while males only migrate short distances with mating occurring in transitional roosts (Llaven-Macías et al., 2021). Migrations between the southern USA and Mexico were also evidenced by genetic analysis, proving that the bats in these caves are genetically similar to each other (Morales et al., 2016). Our study adds to the evidence that long-distance reproductive-related movements are also observed for bat species in the Neotropics, and that bat caves in northeastern Brazil may be considered a network of reproductive roosts used by P. gymnonotus bats.
Conservation implications
Our results suggest that bat caves in northeastern Brazil are priority areas for national bat conservation. In a scenario of strong anthropogenic impacts, maintaining gene flow between bat populations in these bat caves is essential, as studies indicate losses in species and genetic diversity of bat assemblages and populations from fragmented habitats compared to those occurring in continuous habitats (Meyer et al., 2009; Struebig et al., 2011; Ripperger et al., 2013; Lino et al., 2019, 2021). These bat caves are essential for the preservation of Pteronotus bats, as the species of this genus are mostly cave-dwelling (de la Torre and Medellín, 2010; Pavan and Tavares, 2019) and the large colonies they form are essential to control both environmental and body temperature, being a very specialized expression of eco-evolutionary adaptation (de la Torre and Medellín, 2010).
Furthermore, bat caves are very important from an ecological point of view due to a wide range of ecological interactions in which large Pteronotus aggregations participate (Mittermeier et al., 2003; Furey and Racey, 2016; Pimentel et al., 2022). For instance, recent studies show that energy input into bat caves is strongly related to their presence in the caves, with guano deposition on the ground in some bat caves reaching 738 g/m2/96 h (Pimentel et al., 2022). Thus, the presence of bats is essential for the maintenance of the cave ecosystem as part of the cave biota is totally dependent on guano deposition (Furey and Racey, 2016), in a way that entire communities can disappear without guano input into the caves (Trajano, 1995; Gilbert and Deharveng, 2002; Ferreira, 2004). In addition, caves are hotspots of subterranean invertebrate diversity (Myers et al., 2000; Gilbert and Deharveng, 2002; Deharveng and Bedos, 2012; Furey and Racey, 2016) and bat caves are identified as hotspots for fungal diversity (Cunha et al., 2020; Pereira et al., 2022). Along with P. gymnonotus, other bat species can also be found in the analyzed bat caves, including endangered species such as Natalus macrourus and Furipterus horrens (e.g., Rocha et al., 2011; Feijó and Rocha, 2017; Delgado-Jaramillo et al., 2018; Vargas-Mena et al., 2018, 2020; Otálora-Ardila et al., 2019; Leal and Bernard, 2021). Therefore, in addition to their relevance as sites holding thousands of individuals, bat caves can also act as umbrella sites for bat conservation.
The finding of genetically connected populations in bat caves 700 km distance apart also has legal implications in Brazil. Currently, Brazil's national legislation on the environmental licensing process of mining activities (Burland and Wilmer, 2001; Brasil, 2022) establishes that caves within those areas have to pass a classification process and only those with “maximum relevance” will be under strict protection. Bat caves are identified as caves of maximum relevance (Brasil, 2022). Moreover, in the licensing process, mining companies should identify how far a cave can influence its surroundings (Brasil, 2017) However, this is rarely done and most of the companies adopt a 250-m buffer surrounding the cave. Thousands of caves are found within mineral exploration areas in Brazil, and this concept of an area “under the influence” of a cave is crucial, as mining activities must stop at its limits. However, bats have remarkable mobility and the 250 m established by law is not sufficient to represent such an area of influence (e.g., Bernard and Fenton, 2003; Delgado-Jaramillo et al., 2018; Otálora-Ardila et al., 2019; Leal and Bernard, 2021). The lack of genetic structure and the existence of gene flow between colonies in bat caves 700 km apart, as we observed, emphasizes that the 250-m buffer around the caves adopted by Brazilian mining companies is an underestimated measure and clearly insufficient to maintain all ecological interactions those animals have with the caves and their surroundings. Therefore, our data provides a warning call that the current practices adopted in the licensing of mining activities in Brazil must be reviewed in the face of the new existing evidence.
Data availability statement
The datasets presented in this study can be found in the NCBI SRA repository, under SRA BioProject PRJNA824143, https://www.ncbi.nlm.nih.gov/bioproject/PRJNA824143, and the complete scripts for the analyses performed are available in the GitHub repository, https://github.com/itofs/Pgymno_radseq.
Ethics statement
The animal study was reviewed and approved by Comissão de Ética no Uso de Animais (CEUA) Universidade Federal de Pernambuco (UFPE).
Author contributions
FI contributed to conception and design, data collection, analysis, and interpretation, and manuscript drafting. TL contributed to data analysis and interpretation, funding acquisition, and manuscript drafting. VT contributed to data analysis and interpretation and manuscript drafting. EB contributed to conception and design, funding acquisition, data analysis and interpretation, and manuscript drafting and revision for publication. All authors contributed to the article and approved the submitted version.
Funding
The analysis presented here was made possible thanks to the financial resources received via speleological environmental compensation from Gerdau S.A. to Centro Nacional de Pesquisa e Conservação de Cavernas CECAV/ICMBio (Termo de Compromisso de Compensação Espeleológica n°3/2018).
Acknowledgments
The authors thank Eder Barbier, Edson Leal, Frederico Hintze, Gabriel Ghedin, Jennifer Barros, and Narjara Pimentel for their assistance during the fieldwork. We also thank Clebio Rocha, Diego Bento, Inara Leal, José Iatagan, Juan Carlos, Marcelo Tabarelli, Patrício Rocha, Paulo Maier, and Uilson Campos for making the fieldwork possible. We thank Gabriel Wallau, Luisa Silva, and Lais Ceschini for allowing and assisting DNA extractions, and Veronika Laine for valuable insights and help with the data analyses. The analyses presented here were made possible thanks to the financial resources received via speleological environmental compensation from Gerdau S.A. to Centro Nacional de Pesquisa e Conservação de Cavernas CECAV/ICMBio (Termo de Compromisso de Compensação Espeleológica n° 3/2018). We thank Nilmara Soares, from ADESITA, for the financial management of these resources. We thank Bioname Oy and the Finnish Functional Genomics Center supported by the University of Turku, Åbo Akademi University, and Biocenter Finland for allowing us to sequence the samples. This research is part of the PhD thesis of F. Ito at Programa de Pós-Graduação em Biologia Animal PPGBA/UFPE, and we thank Coordenação de Aperfeiçoamento de Pessoal de Nível Superior CAPES and Programa Institucional de Internacionalização—PRINT initiative for the grant received. EB has a fellowship grant from Conselho Nacional de Desenvolvimento Científico e Tecnológico - CNPq.
Conflict of interest
The authors declare that the research was conducted in the absence of any commercial or financial relationships that could be construed as a potential conflict of interest.
Publisher's note
All claims expressed in this article are solely those of the authors and do not necessarily represent those of their affiliated organizations, or those of the publisher, the editors and the reviewers. Any product that may be evaluated in this article, or claim that may be made by its manufacturer, is not guaranteed or endorsed by the publisher.
Supplementary material
The Supplementary Material for this article can be found online at: https://www.frontiersin.org/articles/10.3389/fevo.2022.934633/full#supplementary-material
References
Alexander, D. H., Novembre, J., and Lange, K. (2009). Fast model-based estimation of ancestry in unrelated individuals. Genome Res. 19, 1655–1664. doi: 10.1101/gr.094052.109
Antongiovanni, M., Venticinque, E. M., Matsumoto, M., and Fonseca, C. R. (2020). Chronic anthropogenic disturbance on Caatinga dry forest fragments. J. Appl. Ecol. 57, 2064–2074. doi: 10.1111/1365-2664.13686
Arita, H. T., and Santos-del-Prado, K. (1999). Conservation biology of nectar-feeding bats in Mexico. J. Mammal. 80, 31–41. doi: 10.2307/1383205
Barros, J. S., Bernard, E., and Ferreira, R. L. (2020). Ecological preferences of neotropical cave bats in roost site selection and their implications for conservation. Basic Appl. Ecol. 45, 31–41. doi: 10.1016/j.baae.2020.03.007
Bernard, E., Aguiar, L. M., Brito, D., Cruz-Neto, A. P., Gregorin, R., Machado, R. B., et al. (2012). Uma análise de horizontes sobre a conservação de morcegos no Brasil. Mamíferos Brasil Genét. Sist. Ecol. Conservação 2, 19–35.
Bernard, E., and Fenton, M. B. (2003). Bat mobility and roosts in a fragmented landscape in central Amazonia, Brazil. Biotropica 35, 262–277. doi: 10.1111/j.1744-7429.2003.tb00285.x
Bernard, E., Ferreira, R. L., Cruz, F. W., and Piló, L. (2021). In the International Year of Caves and Karst, Brazilian caves are at high risk (Commentary). Mongabay – News and Inspiration from Nature's Frontline. Available online at: https://news.mongabay.com/2021/05/in-the-international-year-of-caves-and-karst-brazilian-caves-are-at-high-risk-commentary/ (accessed September 23, 2022).
Bernard, E., Paese, A., Machado, R. B., and Aguiar, L. M. S. (2014). Blown in the wind: bats and wind farms in Brazil. Nat. Conserv. 12, 106–111. doi: 10.1016/j.ncon.2014.08.005
Betke, M., Hirsh, D. E., Makris, N. C., McCracken, G. F., Procopio, M., Hristov, N. I., et al. (2008). Thermal imaging reveals significantly smaller Brazilian free-tailed bat colonies than previously estimated. J. Mammal. 89, 18–24. doi: 10.1644/07-MAMM-A-011.1
Brasil (2017). Ministério do Meio Ambiente. Instrução Normativa n° 02 de 30 de agosto de 2017. Define a metodologia para classificação do grau de relevância das cavidades naturais subterrâneas. Brasília, DF: Diário Oficial da República Federativa do Brasil.
Brasil (2022). Ministério do Meio Ambiente. Decreto n° 10.935 de 12 de Janeiro de 2022. Dispõe sobre a proteção das cavidades naturais subterrâneas existentes no território nacional. Brasília, DF: Diário Oficial da República Federativa do Brasil.
Burland, T. M., and Wilmer, J. W. (2001). Seeing in the dark: molecular approaches to the study of bat populations. Biol. Rev. 76, 389–409. doi: 10.1017/S1464793101005747
Carstens, B. C., Sullivan, J., Davalos, L. M., Larsen, P. A., and Pedersen, S. C. (2004). Exploring population genetic structure in three species of Lesser Antillean bats. Mol. Ecol. 13, 2557–2566. doi: 10.1111/j.1365-294X.2004.02250.x
Catchen, J., Hohenlohe, P. A., Bassham, S., Amores, A., and Cresko, W. A. (2013). Stacks: an analysis tool set for population genomics. Mol. Ecol. 22, 3124–3140. doi: 10.1111/mec.12354
Cole, F. R., and Wilson, D. E. (2006). Leptonycteris yerbabuenae. Mamm. Species 797, 1–7. doi: 10.1644/797.1
Corso, A. E., Woolley, J. B., and Lacher, T. E. (2010). Using digital photography and image analysis software to estimate the emergence of bats at Tou Santi Cave, Dominica, West Indies. Caribbean J. Sci. 46, 169–175. doi: 10.18475/cjos.v46i2.a5
Cunha, A. O., Bezerra, J. D., Oliveira, T. G., Barbier, E., Bernard, E., Machado, A. R., et al. (2020). Living in the dark: bat caves as hotspots of fungal diversity. PLoS ONE 15, e0243494. doi: 10.1371/journal.pone.0243494
de la Torre, J. A., and Medellín, R. A. (2010). Pteronotus personatus (Chiroptera: Mormoopidae). Mamm. Species 42, 244–250. doi: 10.1644/869.1
De Oliveira, H. F. M., Oprea, M., and Dias, R. I. (2018). Distributional patterns and ecological determinants of bat occurrence inside caves: a broad scale meta-analysis. Diversity 10, 49. doi: 10.3390/d10030049
Deharveng, L., and Bedos, A. (2012). “Diversity patterns in the tropics,” in Encyclopedia of Caves (Cambridge, MA: Academic Press), 238–250. doi: 10.1016/B978-0-12-383832-2.00032-3
Delgado-Jaramillo, M., Aguiar, L. M., Machado, R. B., and Bernard, E. (2020). Assessing the distribution of a species-rich group in a continental-sized megadiverse country: bats in Brazil. Divers. Distrib. 26, 632–643. doi: 10.1111/ddi.13043
Delgado-Jaramillo, M., Barbier, E., and Bernard, E. (2018). New records, potential distribution, and conservation of the Near Threatened cave bat Natalus macrourus in Brazil. Oryx 52, 579–586. doi: 10.1017/S0030605316001186
Dool, S. E. (2020). “Conservation genetic studies in bats,” in Conservation Genetics in Mammals (Cham: Springer), 29–62. doi: 10.1007/978-3-030-33334-8_3
Elshire, R. J., Glaubitz, J. C., Sun, Q., Poland, J. A., Kawamoto, K., Buckler, E. S., et al. (2011). A robust, simple genotyping-by-sequencing (GBS) approach for high diversity species. PLoS ONE 6, e19379. doi: 10.1371/journal.pone.0019379
Feijó, A., and Rocha, P. A. (2017). Morcegos da Estação Ecológica Aiuaba, Ceará, nordeste do Brasil: uma unidade de proteção integral na Caatinga. Mastozool. Neotropical 24, 333–351.
Ferreira, R. L. (2004). A medida da complexidade ecológica e suas aplicações na conservação e manejo de ecossistemas subterrâneos (Master's thesis). Universidade Federal de Minas Gerais, Belo Horizonte, Minas Gerais, Brazil.
Ferreira, R. L., Bernard, E., da Cruz Júnior, F. W., Piló, L. B., Calux, A., Souza-Silva, M., et al. (2022). Brazilian cave heritage under siege. Science 375, 1238–1239. doi: 10.1126/science.abo1973
Frichot, E., Mathieu, F., Trouillon, T., Bouchard, G., and François, O. (2014). Fast and efficient estimation of individual ancestry coefficients. Genetics 196, 973–983. doi: 10.1534/genetics.113.160572
Furey, N. M., and Racey, P. A. (2016). “Conservation ecology of cave bats,” in Bats in the Anthropocene: Conservation of Bats in a Changing World (Cham: Springer), 463–500. doi: 10.1007/978-3-319-25220-9_15
Galindo, G. C., Sánchez, Q. A., Quijano, R. H., and Herrera, M. L. G. (2004). Population dynamics of a resident colony of Leptonycteris curasoae (Chiroptera: Phyllostomidae) in central Mexico. Biotropica 36, 382–391. doi: 10.1111/j.1744-7429.2004.tb00331.x
Garbino, G. S., Lim, B. K., and Tavares, V. D. C. (2020). Systematics of big-eyed bats, genus Chiroderma Peters, 1860 (Chiroptera: Phyllostomidae). Zootaxa 4846, 1–93. doi: 10.11646/zootaxa.4846.1.1
Gilbert, J., and Deharveng, L. (2002). Subterranean ecosystems: a truncated functional biodiversity. Bioscience 52, 473–481. doi: 10.1641/0006-3568(2002)052[0473:SEATFB]2.0.CO;2
Gong, X., Davenport, E. R., Wang, D., and Clark, A. G. (2019). Lack of spatial and temporal genetic structure of Japanese eel (Anguilla japonica) populations. Conserv. Genet. 20, 467–475. doi: 10.1007/s10592-019-01146-8
Hartl, D. L., and Clark, A. G. (1997). Principles of Population Genetics, Vol. 116. Sunderland: Sinauer Associates.
Hua, P., Zhang, L., Guo, T., Flanders, J., and Zhang, S. (2013). Dispersal, mating events and fine-scale genetic structure in the lesser flat-headed bats. PLoS ONE 8, e54428. doi: 10.1371/journal.pone.0054428
Jansen, D. C., Cavalcanti, L. F., and Lamblém, H. S. (2012). Mapa de Potencialidade de Ocorrência de Cavernas no Brasil, na escala 1:2.500.000. Rev. Brasil. Espeleol. 2, 42–57. Available online at: https://revistaeletronica.icmbio.gov.br/RBEsp/article/view/255
Ladle, R. J., Firmino, J. V., Malhado, A. C., and Rodríguez-Durán, A. (2012). Unexplored diversity and conservation potential of Neotropical hot caves. Conserv. Biol. 26, 978–982. doi: 10.1111/j.1523-1739.2012.01936.x
Laine, V. N., Lilley, T. M., Norrdahl, K., and Primmer, C. R. (2013). Population genetics of Daubenton's bat (Myotis daubentonii) in the Archipelago Sea, SW Finland. Annales Zoologici Fennici. 50, 303–315. Available online at: https://www.jstor.org/stable/43923480
Leal, E. S. B., and Bernard, E. (2021). Mobility of bats between caves: ecological aspects and implications for conservation and environmental licensing activities in Brazil. Stud. Neotrop. Fauna Environ. 1–11. doi: 10.1080/01650521.2021.1964910
Lemopoulos, A., Uusi-Heikkilä, S., Vasemägi, A., Huusko, A., Kokko, H., and Vainikka, A. (2018). Genome-wide divergence patterns support fine-scaled genetic structuring associated with migration tendency in brown trout. Can. J. Fish. Aquat. Sci. 75, 1680–1692. doi: 10.1139/cjfas-2017-0014
Li, H., and Durbin, R. (2009). Fast and accurate short read alignment with Burrows–Wheeler transform. Bioinformatics 25, 1754–1760. doi: 10.1093/bioinformatics/btp324
Lilley, T. M., Sävilammi, T., Ossa, G., Blomberg, A. S., Vasemägi, A., Yung, V., et al. (2020). Population connectivity predicts vulnerability to white-nose syndrome in the Chilean myotis (Myotis chiloensis), a genomics approach. G3 10, 2117–2126. doi: 10.1534/g3.119.401009
Lino, A., Ferreira, E., Fonseca, C., Fischer, E., and Ramos Pereira, M. J. (2021). Species–genetic diversity correlation in phyllostomid bats of the Bodoquena plateau, Brazil. Biodivers. Conserv. 30, 403–429. doi: 10.1007/s10531-020-02097-0
Lino, A., Fonseca, C., Rojas, D., Fischer, E., and Pereira, M. J. R. (2019). A meta-analysis of the effects of habitat loss and fragmentation on genetic diversity in mammals. Mamm. Biol. 94, 69–76. doi: 10.1016/j.mambio.2018.09.006
Llaven-Macías, V., Ruíz-Montoya, L., López-González, C., Rico, Y., and Naranjo, E. J. (2021). Monthly fluctuation of colony size and composition of the free-tailed bat Tadarida brasiliensis in the southernmost roost of Mexico. Mammal Res. 66, 339–348. doi: 10.1007/s13364-021-00559-6
López-Hoffman, L., Chester, C. C., and Merideth, R. (2017a). Conserving transborder migratory bats, preserving nature's benefits to humans: the lesson from North America's bird conservation treaties. Bioscience 67, 321–322. doi: 10.1093/biosci/biw181
López-Hoffman, L., Chester, C. C., Semmens, D. J., Thogmartin, W. E., Rodríguez-McGoffin, M. S., Merideth, R., et al. (2017b). Ecosystem services from transborder migratory species: implications for conservation governance. Annu. Rev. Environ. Resour. 42, 509–539. doi: 10.1146/annurev-environ-110615-090119
Luna, L. W., Ribas, C. C., and Aleixo, A. (2021). Genomic differentiation with gene flow in a widespread Amazonian floodplain-specialist bird species. J. Biogeogr. 49, 1670–1682. doi: 10.1111/jbi.14257
Mammola, S., Amorim, I. R., Bichuette, M. E., Borges, P. A., Cheeptham, N., Cooper, S. J., et al. (2020). Fundamental research questions in subterranean biology. Biol. Rev. 95, 1855–1872. doi: 10.1111/brv.12642
Martins, F. M., Ditchfield, A. D., Meyer, D., and Morgante, J. S. (2007). Mitochondrial DNA phylogeography reveals marked population structure in the common vampire bat, Desmodus rotundus (Phyllostomidae). J. Zool. Syst. Evol. Res. 45, 372–378. doi: 10.1111/j.1439-0469.2007.00419.x
Medellín, R. A., Arita, H. T., and Sánchez, O. (2008). Identificación de los murciélagos de México: Clave de campo, 2nd Edn. Instituto de Ecología, Universidad Nacional Autónoma de México, Ciudad de México, México.
Medellín, R. A., Wiederholt, R., and Lopez-Hoffman, L. (2017). Conservation relevance of bat caves for biodiversity and ecosystem services. Biol. Conserv. 211, 45–50. doi: 10.1016/j.biocon.2017.01.012
Meyer, C. F., Kalko, E. K., and Kerth, G. (2009). Small-scale fragmentation effects on local genetic diversity in two Phyllostomid bats with different dispersal abilities in Panama. Biotropica 41, 95–102. doi: 10.1111/j.1744-7429.2008.00443.x
Mittermeier, R. A., Gil, P. R., and Konstant, W. K. (2003). Wildlife spectacles. CEMEX-Agrupación Sierra Madre-Conservation International. Mexico, 324.
Morales, A., Villalobos, F., Velazco, P. M., Simmons, N. B., and Piñero, D. (2016). Environmental niche drives genetic and morphometric structure in a widespread bat. J. Biogeogr. 43, 1057–1068. doi: 10.1111/jbi.12666
Moras, L. M., Gregorin, R., Sattler, T., and da Tavares, V. C. (2018). Uncovering the diversity of dog-faced bats of the genus Cynomops (Chiroptera: Molossidae), with the redescription of C. milleri and the description of two new species. Mamm. Biol. 89, 37–51. doi: 10.1016/j.mambio.2017.12.005
Myers, N., Mittermeier, R. A., Mittermeier, C. G., Da Fonseca, G. A., and Kent, J. (2000). Biodiversity hotspots for conservation priorities. Nature 403, 853–858. doi: 10.1038/35002501
Neri, M., Jameli, D., Bernard, E., and Melo, F. P. L. (2019). Green versus green? Adverting potential conflicts between wind power generation and biodiversity conservation in Brazil. Perspect. Ecol. Conserv. 17, 131–135. doi: 10.1016/j.pecon.2019.08.004
Novaes, R. L. M., Cláudio, V. C., Carrión-Bonilla, C., Abreu, E. F., Wilson, D. E., Maldonado, J. E., et al. (2021). Variation in the Myotis keaysi complex (Chiroptera, Vespertilionidae), with description of a new species from Ecuador. J. Mammal. 103, 540–559. doi: 10.1093/jmammal/gyab139
Otálora-Ardila, A., Torres, J. M., Barbier, E., Pimentel, N. T., Barbosa Leal, E. S., and Bernard, E. (2019). Thermally-assisted monitoring of bat abundance in an exceptional cave in Brazil's Caatinga drylands. Acta Chiropterol. 21, 411–423. doi: 10.3161/15081109ACC2019.21.2.016
Pavan, A. C., and Marroig, G. (2016). Integrating multiple evidences in taxonomy: species diversity and phylogeny of mustached bats (Mormoopidae: Pteronotus). Mol. Phylogenet. Evol. 103, 184–198. doi: 10.1016/j.ympev.2016.07.011
Pavan, A. C., and Marroig, G. (2017). Timing and patterns of diversification in the Neotropical bat genus Pteronotus (Mormoopidae). Mol. Phylogenet. Evol. 108, 61–69. doi: 10.1016/j.ympev.2017.01.017
Pavan, A. C., and Tavares, V. (2019). Pteronotus gymnonotus (Chiroptera: Mormoopidae). Mammal. Species 52, 40–48.
Pavan, A. C., Wilson, D. E., and Mittermeier, R. A. (2019). “Family mormoopidae (ghost-faced bats, naked-backed bats and mustached bats),” in Handbook of the Mammals of the World, eds D. E. Wilson and R. A. Mittermeier (Barcelona: Lynx Edicions). 424–443.
Pereira, M. L., Carvalho, J. L., Lima, J., Barbier, E., Bernard, E., Bezerra, J. D., et al. (2022). Richness of Cladosporium in a tropical bat cave with the description of two new species. Mycol. Prog. 21, 345–357. doi: 10.1007/s11557-021-01760-2
Pimentel, N. T. (2021). A contribuição dos morcegos no input de energia na forma de guano para bat caves no semiárido nordestino (Master's thesis). Universidade Federal de Pernambuco, Recife, Pernambuco, Brazil.
Pimentel, N. T., da Rocha, P. A., Pedroso, M. A., and Bernard, E. (2022). Estimates of insect consumption and guano input in bat caves in Brazil. Mammal Res. 67, 355–366. doi: 10.1007/s13364-022-00629-3
Pinzari, C. A., Kang, L., Michalak, P., Jermiin, L. S., Price, D. K., and Bonaccorso, F. J. (2020). Analysis of genomic sequence data reveals the origin and evolutionary separation of Hawaiian hoary bat populations. Genome Biol. Evol. 12, 1504–1514. doi: 10.1093/gbe/evaa137
Purcell, S., Neale, B., Todd-Brown, K., Thomas, L., Ferreira, M. A., Bender, D., et al. (2007). PLINK: a tool set for whole-genome association and population-based linkage analyses. Am. J. Hum. Genet. 81, 559–575. doi: 10.1086/519795
Ripperger, S. P., Tschapka, M., Kalko, E. K., Rodriguez-Herrera, B., and Mayer, F. (2013). Life in a mosaic landscape: anthropogenic habitat fragmentation affects genetic population structure in a frugivorous bat species. Conserv. Genet. 14, 925–934. doi: 10.1007/s10592-012-0434-y
Rocha, P. A., Feijó, J. A., Mikalauskas, J. S., and Ferrari, S. F. (2011). First records of mormoopid bats (Chiroptera, Mormoopidae) from the Brazilian Atlantic Forest. Mammalia 75, 295–299. doi: 10.1515/mamm.2011.029
Scheben, A., Kramer, M., Goodwin, S., Oppenheim, S., Becker, D. J., Schatz, M. C., et al. (2020). Long-read sequencing reveals rapid evolution of immunity-and cancer-related genes in bats. BioRxiv [Preprint]. doi: 10.1101/2020.09.09.290502
Sikes, R. S., and Animal Care and Use Committee of the American Society of Mammalogists (2016). 2016 Guidelines of the American Society of Mammalogists for the use of wild mammals in research and education. J. Mammal. 97, 663–688. doi: 10.1093/jmammal/gyw078
Silva, U. B. T., Delgado-Jaramillo, M., de Souza Aguiar, L. M., and Bernard, E. (2018). Species richness, geographic distribution, pressures, and threats to bats in the Caatinga drylands of Brazil. Biol. Conserv. 221, 312–322. doi: 10.1016/j.biocon.2018.03.028
Sovic, M. G., Carstens, B. C., and Gibbs, H. L. (2016). Genetic diversity in migratory bats: results from RADseq data for three tree bat species at an Ohio windfarm. Peer J. 4, e1647. doi: 10.7717/peerj.1647
Stoner, K. E., O-Salazar, K. A., R-Fernández, R. C., and Quesada, M. (2003). Population dynamics, reproduction, and diet of the lesser long-nosed bat (Leptonycteris curasoae) in Jalisco, Mexico: implications for conservation. Biodivers. Conserv. 12, 357–373. doi: 10.1023/A:1021963819751
Struebig, M. J., Kingston, T., Petit, E. J., Le Comber, S. C., Zubaid, A., Mohd-Adnan, A., et al. (2011). Parallel declines in species and genetic diversity in tropical forest fragments. Ecol. Lett. 14, 582–590. doi: 10.1111/j.1461-0248.2011.01623.x
Valença, R. B., and Bernard, E. (2015). Another blown in the wind: bats and the licensing of wind farms in Brazil. Nat. Conserv. 13, 117–122. doi: 10.1016/j.ncon.2015.09.001
Vargas-Mena, J. C., Cordero-Schmidt, E., de Medeiros Bento, D., Rodriguez-Herrera, B., Medellín, R. A., and Venticinque, E. M. (2018). Diversity of cave bats in the Brazilian tropical dry forest of Rio Grande do Norte state. Mastozool. Neotrop. 25, 199–212. doi: 10.31687/saremMN.18.25.1.0.16
Vargas-Mena, J. C., Cordero-Schmidt, E., Rodriguez-Herrera, B., Medellín, R. A., Bento, D. D. M., and Venticinque, E. M. (2020). Inside or out? Cave size and landscape effects on cave-roosting bat assemblages in Brazilian Caatinga caves. J. Mammal. 101, 464–475. doi: 10.1093/jmammal/gyz206
Weir, B. S. (1996). Genetic data analysis IImethods for discrete population genetic data. Sinauer Assoc. Sunderl. 66, 150–156.
Wiederholt, R., Bagstad, K. J., McCracken, G. F., Diffendorfer, J. E., Loomis, J. B., Semmens, D. J., et al. (2017). Improving spatio-temporal benefit transfers for pest control by generalist predators in cotton in the southwestern US. Int. J. Biodiv. Sci. Ecosyst. Serv. Manag. 13, 27–39. doi: 10.1080/21513732.2016.1240712
Wiederholt, R., López-Hoffman, L., Cline, J., Medellín, R. A., Cryan, P., Russell, A., et al. (2013). Moving across the border: modeling migratory bat populations. Ecosphere 4, 1–16. doi: 10.1890/ES13-00023.1
Wiederholt, R., López-Hoffman, L., Svancara, C., McCracken, G., Thogmartin, W., Diffendorfer, J. E., et al. (2015). Optimizing conservation strategies for Mexican free-tailed bats: a population viability and ecosystem services approach. Biodivers. Conserv. 24, 63–82. doi: 10.1007/s10531-014-0790-7
Keywords: Chiroptera (bats), ddRADseq, population structure, roost network, karstic habitats
Citation: Ito F, Lilley T, Twort VG and Bernard E (2022) High genetic connectivity among large populations of Pteronotus gymnonotus in bat caves in Brazil and its implications for conservation. Front. Ecol. Evol. 10:934633. doi: 10.3389/fevo.2022.934633
Received: 02 May 2022; Accepted: 08 August 2022;
Published: 05 October 2022.
Edited by:
Thales Renato Ochotorena De Freitas, Federal University of Rio Grande do Sul, BrazilReviewed by:
Gabriela Paula Fernández, Universidad Nacional del Noroeste de la Provincia de Buenos Aires (UNNOBA), ArgentinaAdriana Gava, Federal University of Rio Grande, Brazil
Copyright © 2022 Ito, Lilley, Twort and Bernard. This is an open-access article distributed under the terms of the Creative Commons Attribution License (CC BY). The use, distribution or reproduction in other forums is permitted, provided the original author(s) and the copyright owner(s) are credited and that the original publication in this journal is cited, in accordance with accepted academic practice. No use, distribution or reproduction is permitted which does not comply with these terms.
*Correspondence: Enrico Bernard, ZW5yaWNvLmJlcm5hcmRAdWZwZS5icg==
†ORCID: Enrico Bernard orcid.org/0000-0002-2304-1978