- Department of Biology, Portland State University, Portland, OR, United States
Green roofs are often installed atop buildings to provide ecological services such as mitigating storm water runoff and cooling air within urban heat islands. We found that green roofs in Portland, Oregon, also can support biodiversity, including a diverse assemblage of parasitoid wasps, with 20 morphospecies from 10 families present on the four roofs we surveyed. The roofs with greater plant diversity and structural complexity harbored comparatively more parasitoid morphospecies than the structurally simpler Sedum-dominated roofs. The oldest green roof supported much greater diversity than the younger roofs, including a comparably planted roof three times its size. Parasitoid wasps from the Hymenopteran families we found are high-trophic-level organisms known to feed on a variety of arthropods, including many insects commonly considered to be pests in urban areas. We suggest that green roofs have the potential to provide an often-overlooked ecological service by supporting parasitoid wasps that can act as natural biological control agents.
Introduction
A green roof is an installation that covers a building’s conventional roof with vegetation in a growing medium. These green spaces are increasing in frequency within urban boundaries (Oberndorfer et al., 2007; Braaker et al., 2014). With more than half of the human population residing in urban centers, and that percentage projected to increase (Angel et al., 2011; Seto et al., 2012), the amount of space devoted to green roofs will likely rise accordingly. Although green roofs have historically been installed for the benefits they provide to buildings and municipalities, such as storm-water management and summer cooling (Oberndorfer et al., 2007), they can potentially serve to support biodiversity in densely developed urban areas, as well.
In Europe, where green roofs have a longer history than in North America, these roofs have been shown to support some arthropod communities (Oberndorfer et al., 2007; Braaker et al., 2017; Petremand et al., 2018). Indeed, Kadas (2006) documented that some green roofs in London, UK, were home to certain invertebrate species of conservation concern, suggesting that these spaces could play a role in the conservation of species. In North America, green roofs have been found to support a diversity of beetles, although many of the taxa were not native to the region (Starry et al., 2018; Gonsalves et al., 2022). However, we are still in the early stages of understanding how factors such as habitat type and plant community structure contribute to arthropod biodiversity in urban green spaces (Madre et al., 2013; Williams et al., 2014). It is important to understand these factors, considering that arthropods perform a variety of services that benefit human society, most notably pollination and pest control (Losey and Vaughan, 2006).
As the human population has grown, the degree of landscape fragmentation and habitat loss likewise has increased (Pataki, 2015). Although habitat fragmentation may decrease biodiversity (Fahrig, 2003), urban green spaces can mitigate the impacts of land conversion and serve as potential reservoirs for species diversity (Bryant, 2006; Jones and Leather, 2012). Even small areas of habitat may be important in promoting species diversity (Riva and Fahrig, 2022). Green roofs can harbor surprising arthropod diversity (Kadas, 2006; Petremand et al., 2018; Wooster et al., 2022), although the factors that contribute to being an effective biodiversity reservoir are still under debate (Jones and Leather, 2012; Williams et al., 2014; Lepczyk et al., 2017). Green roofs may provide critical connections among urban green spaces, depending on the surrounding landscape matrix and the dispersal ability of colonizing organisms (Braaker et al., 2014).
Pest control is an important factor in the ecological condition of green spaces, particularly for fragmented regions (Burkman and Gardiner, 2014). Although chemical pesticides can initially be very effective in reducing infestations, over time the targeted pests can develop resistance. Furthermore, pesticides have been linked to many human health and environmental concerns, through occupational exposure as well as water, soil, and air contamination (Damalas and Eleftherohorinos, 2011). For all these reasons, biological control can be advantageous when compared to the hazards and expenses of chemical control (Gradish et al., 2011; van Lenteren et al., 2018). Furthermore, in some instances biological control can be more effective than the use of chemical pesticides (van Lenteren et al., 2018). Arthropod biological control agents are often predatory or parasitic insects that are natural enemies of herbivorous pests.
Parasitoid wasps (Arthropoda; Hexapoda; Hymenoptera) are biological control agents of other arthropods; they develop on or inside their host, ultimately killing the host upon emergence and becoming free-living adults (Godfray, 1994). The female deposits eggs on or within the bodies of host organisms, so that once the eggs hatch, the larvae feed upon the host. Parasitoid wasps represent a major radiation within the order Hymenoptera and include approximately 240,000 currently described parasitoid species (Bonet, 2008). The superfamily Ichneumonoidea alone contains approximately 60,000 described species, while many more species are presumed to remain undiscovered (Quicke, 2015). Many parasitoids are host-specific (Jervis and Kidd, 1986; Heimpel and Collier, 1996). Due to their developmental strategy, in which larvae kill their host upon emergence, parasitoids are considered top trophic-level predators (Godfray, 1994).
Parasitoids mitigate damage from herbivory and control the abundance of various pests, principally other insects whose populations could grow exponentially in the absence of a biocontrol agent (Hassell and Waage, 1984; Bonet, 2008). Indeed, Gomez and Zamora (1994) verified experimentally that parasitoid activity improved plant fitness through a significant increase in fecundity and survivorship. Parasitoid wasps are frequently used in agricultural settings to keep insect pest populations in check (Silveira et al., 2019), and may also act as valuable biocontrol agents against possible pests of green infrastructure in urban areas, contributing to the health of the plant community present (Fenoglio et al., 2013).
Despite the role that parasitoids can play in biological control, we do not currently know which, if any, parasitoid wasps are present on most urban green roofs. Given their particular sensitivity to urbanization (Fenoglio et al., 2013), documenting which parasitoids survive on green roofs might suggest potential target species to use for future pest control. In this study, we investigated the diversity and abundance of parasitoid wasps on four green roofs in downtown Portland, OR, USA. We also compared the distribution of parasitoid wasps among the green roofs, which varied in vegetation type, size, age, and distance to the nearest green space.
Materials and methods
Study sites
Four green roofs were selected from the urban center of Portland, Oregon (Figure 1). These locations were a component of a larger study on the biodiversity of beetles (Starry et al., 2018; Gonsalves et al., 2022) and other arthropod taxa present on these same roofs. Two of the green roofs selected for this study (EcoTrust, or ET, and Oregon College of Oriental Medicine, or OCOM) were dominated by succulent Sedum plants (Table 1). The OCOM roof also had two small areas of herbaceous ornamental plants but these comprised less than 10% of total vegetated area (Olyssa Starry, personal communication). The other two locations [Hamilton West (HW) and Central Wine Warehouse (CWW)] had been planted with a mix of herbaceous grasses and flowering plants (Table 1). Although these roofs were designed for storm-water management, they were also intended to promote urban biodiversity (Olyssa Starry, personal communication). The four green roofs were installed at different times and varied in soil depth and the amount of supplementary water they received (Table 1). All sites received partial to full sun exposure, with zones of shading created by rooftop structures (Gonsalves, 2016).
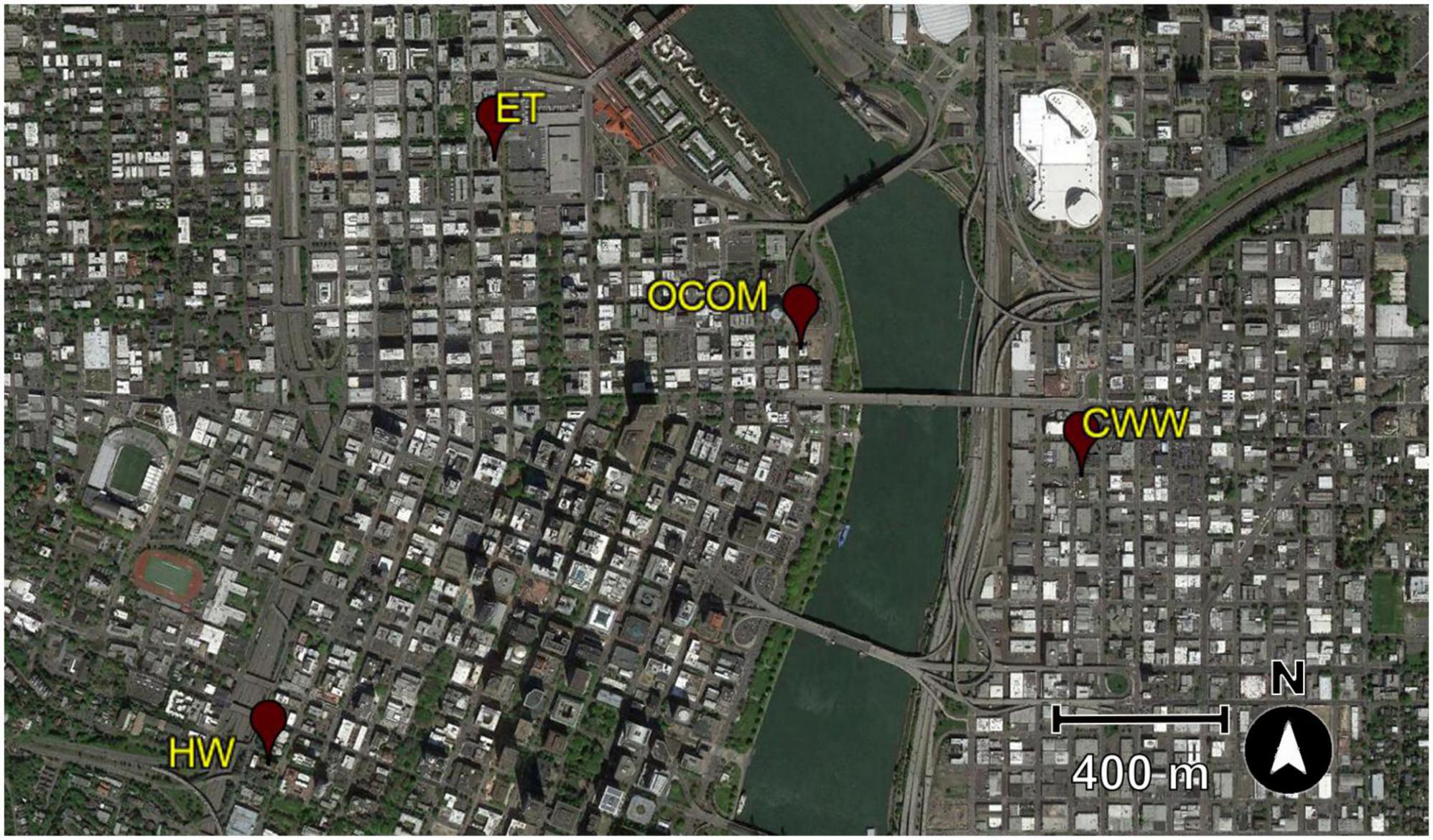
Figure 1. Google Earth image (map data 2015 © Google) of Portland, Oregon from the year of the study (2015) with the locations of the four green roof sites used in this study marked. ET, EcoTrust; OCOM, Oregon College of Oriental Medicine; CWW, Central Wine Warehouse; HW, Hamilton West.
The city of Portland experiences a temperate climate, with temperatures that allow many invertebrates to live or reproduce year-round. During this study, the average monthly air temperature during April to June of 2015 for downtown Portland ranged from 10.3 to 21.7°C with the highest temperature recorded at 33.3°C (National Oceanic and Atmospheric Administration [NOAA], 2015). A seasonal drought occurred during the sampling period. The total precipitation during the study amounted to 9.4 cm, which is roughly 7 cm lower than the average rainfall for the urban center of Portland during April, May, and June (National Oceanic and Atmospheric Administration [NOAA], 2015). Three of the study sites received some level of supplemental irrigation (Table 1), which offset the dry conditions to varying extents.
Sampling techniques
Pitfall traps are among the most widely utilized sampling methods for cursorial invertebrates and were employed in this study. Traps were established according to Woodcock (2005), with 10 pitfall traps per green roof site distributed in a grid pattern at distances of 10 m wherever possible, and with no traps being closer than 5 m from each other. Ward et al. (2001) demonstrated that there was no statistical difference in total abundance of insects collected between pitfall traps placed 5 m apart as opposed to 10 m apart.
Each pitfall trap consisted of a 125-ml plastic cup nestled inside a 5-cm diameter PVC pipe connector (Gonsalves, 2016). Cups were firmly placed into the substrate and situated level with the soil layer. A cover was constructed to minimize overflow from excessive rain events. Each trap was filled to three-fourths capacity with a 10% acetic acid solution. Vinegar was chosen due to its relatively low volatility and chosen over ethylene glycol for its non-toxic effects on vertebrates. The cups were emptied and refilled with fresh vinegar solution approximately every 2 weeks covering a period from 9 April to 25 June 2015 (except for one collection date for OCOM). The contents of all pitfall traps for each roof were pooled into a single plastic container per collection date. To avoid degradation of the specimens collected, the contents from each site were rinsed in water and filtered before being transferred to and stored in 80% ethanol. Filtration and transfer of insects to ethanol occurred within a 2-week window post-collection.
Specimen identification
Specimens were sorted according to taxonomic groups using a dissecting microscope. All parasitoid hymenopterans were handled using plastic pipettes to avoid damaging the specimens. Specimens were identified to morphospecies based upon wing venation patterns, total flagellomere number, shape of the antennae, and other key diagnostic characteristics (see Supplementary Table 1). When possible, specimens were identified to family level in collaboration with Dr. Heidi Liere (at Seattle University) and Diego Fernando Campos Moreno (at the National Pedagogical University) following the keys by Goulet and Huber (1993) and Broad (2011). Representative voucher specimens were preserved for each morphospecies and are stored in 80% ethanol at the Portland State University Museum of Natural History.
Data analysis
We constructed species accumulation curves to evaluate variation in wasp species diversity, and determine whether we had sampled sufficiently to capture the diversity present among the green roof sites. To compare the functional diversity and composition of Hymenoptera from the green roof sites, we sorted all specimens by taxonomic families and host preferences into bar charts, and then aggregated morphospecies types by roof site into pie charts. To account for the size of each location, we divided the raw number of specimens collected by the area (m2) of the site. Analyses were performed with Microsoft Excel and the R statistical environment (R Development Core Team, 2011).
In order to compare wasp biodiversity among the green roof sites, we calculated the observed species richness, as well as the effective number of species, or the number of equally abundant species that are required to create the same value of a diversity measure across sites (Hill numbers), using the iNext package (Chao et al., 2014; Hsieh et al., 2016, 2022). The importance of rare species change with the function of the parameter q, with q = 0 weighting all species equally, q = 1 weighting species by their relative abundance (exponential Shannon entropy), and q = 2 weighting rarer compounds as less important (Simpson’s inverse index). To test for the influence of roof age and height on observed species diversity, we normalized predictor values using the BBmisc package (Bischl et al., 2014) and then modeled the relationship using a Gaussian error structure.
To assess whether the surrounding landscape may have influenced the diversity of parasitoids on the green roofs, we gathered data on three measures of isolation from areas of nearby natural habitat. Using satellite imagery from GoogleEarth to provide an aerial view of landscape features around each of the four green roofs (see Figure 1), we measured: (1) distance to the nearest natural habitat (in linear meters), (2) total area (in hectares) of natural habitats within 0.5 km of each roof, and (3) total area (in hectares) of natural habitats within 1.0 km of each roof. For each of these measures, “natural habitat” was defined as a contiguous area of at least 0.50 ha that is predominantly vegetated (as opposed to paved or taken up by structures). The 0.50-ha threshold is equivalent to the size of one typical city block in downtown Portland. We then tested for correlations between these landscape measures and our measures of species diversity by running linear regressions between each landscape measure and each measure of species diversity.
Results
One-hundred and nineteen parasitoid wasps were collected from the four roofs over an 11-week period, with wasps present in samples from each of the roof sites. We found a total of 20 morphologically distinct species, including representatives from 10 families (Table 2 and Figure 2). One specimen was not identifiable to family, but represented a unique morphospecies. The highest total number of individuals (58) was found on the oldest roof (HW; Table 3). HW also had the highest value of species richness (S) and the largest number of unique morphospecies (Table 3 and Figure 3). HW was the second-smallest roof we surveyed, and when we corrected for roof size it had the greatest number of individuals per m2 (Table 3). The other three roofs in the study each contained only one unique morphospecies (Table 3). No one single morphospecies was present in all locations (Figure 3). CWW produced only 10 specimens yet held eight morphospecies, while ET hosted 34 individuals with only four morphospecies, and OCOM had 17 specimens with five morphospecies (Table 2 and Figure 3).
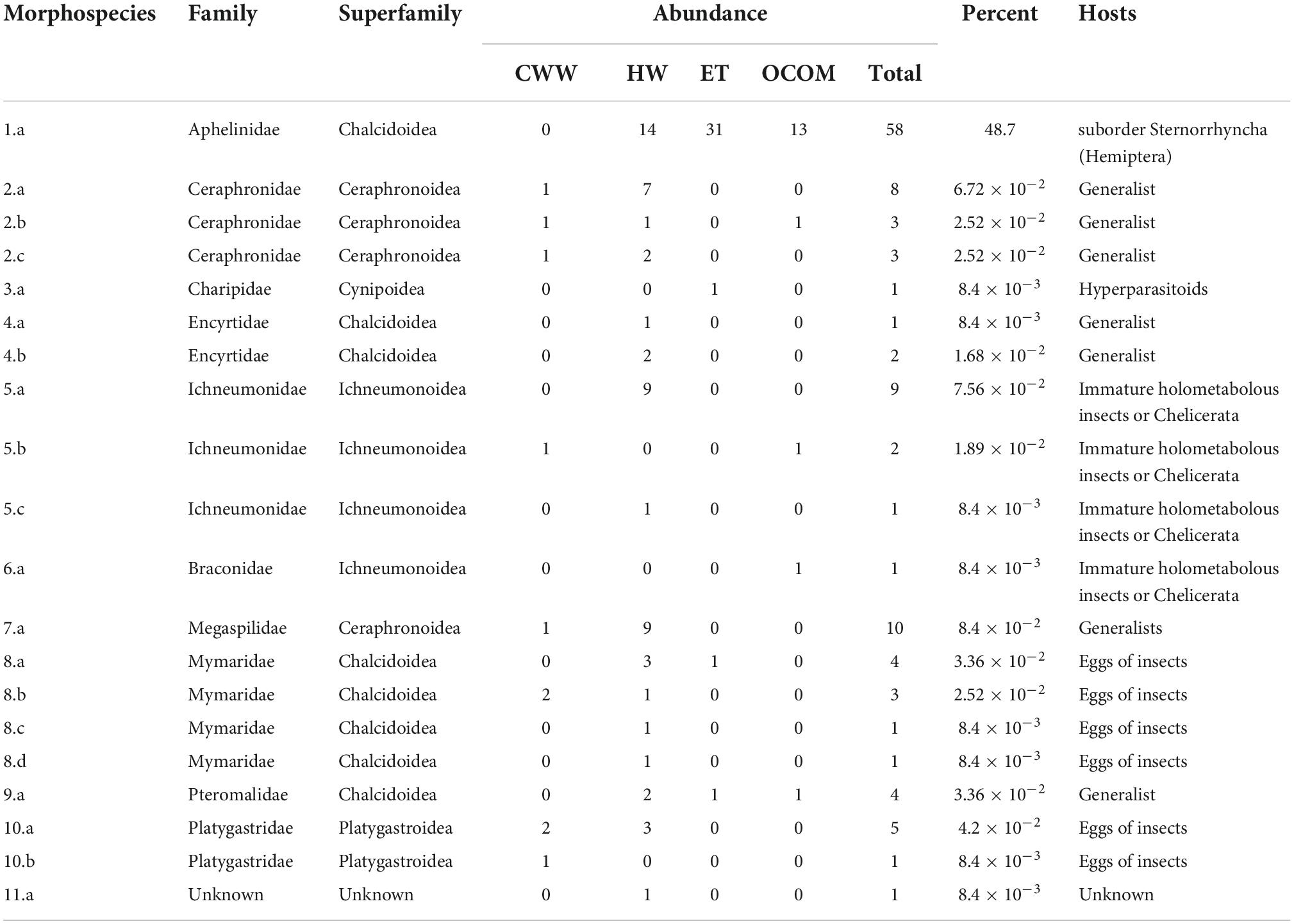
Table 2. Characterization and abundance of the morphospecies of parasitoid wasps (n = 119) present on four green roofs (CWW, Central Wine Warehouse; ET, EcoTrust; HW, Hamilton West; OCOM, Oregon College of Oriental Medicine) in the urban center of Portland, Oregon.
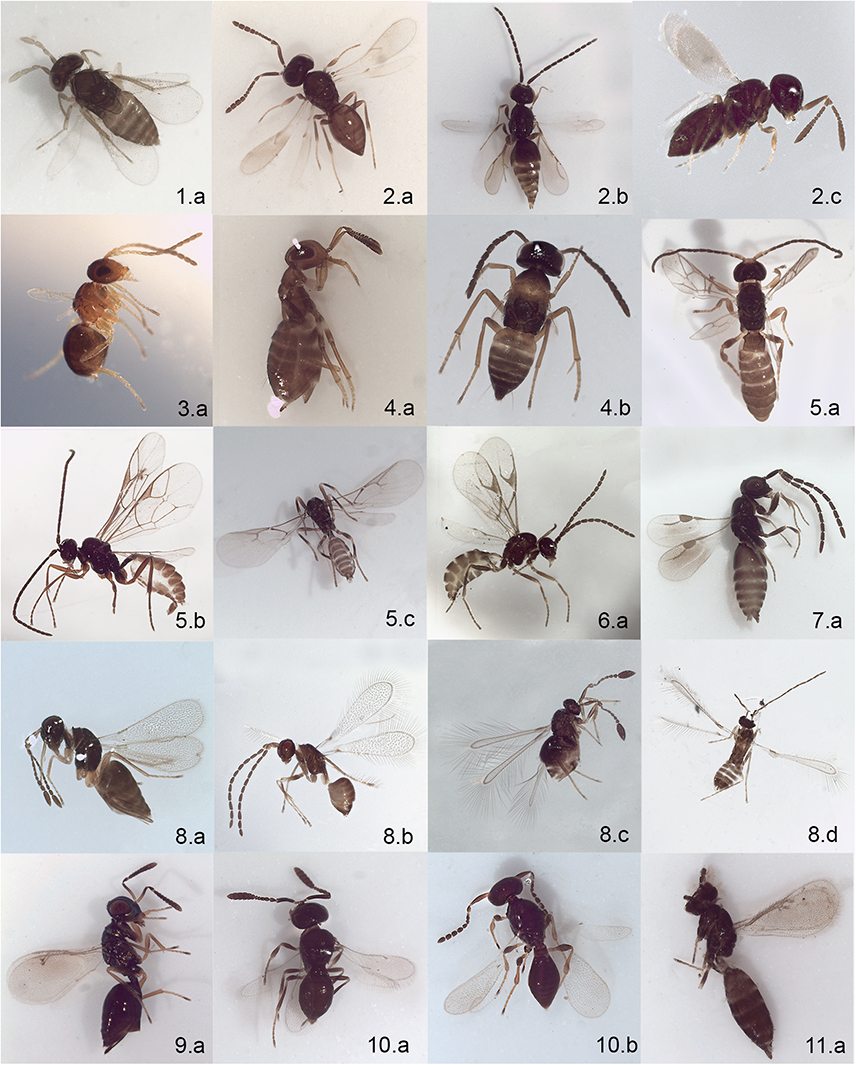
Figure 2. Morphotype catalog of parasitoid wasp (Hymenoptera) diversity as collected from pitfall traps on four green roofs across the urban core of Portland, Oregon.
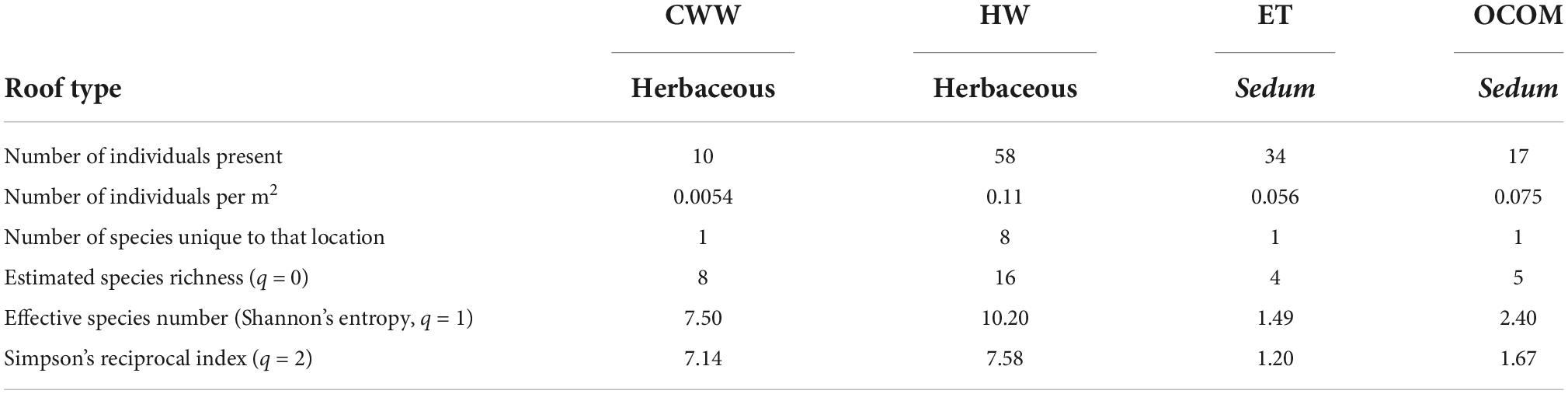
Table 3. Abundance and diversity metrics for parasitoid wasp communities present on four green roofs (CWW, Central Wine Warehouse; ET, EcoTrust; HW, Hamilton West; OCOM, Oregon College of Oriental Medicine) within Portland, Oregon.
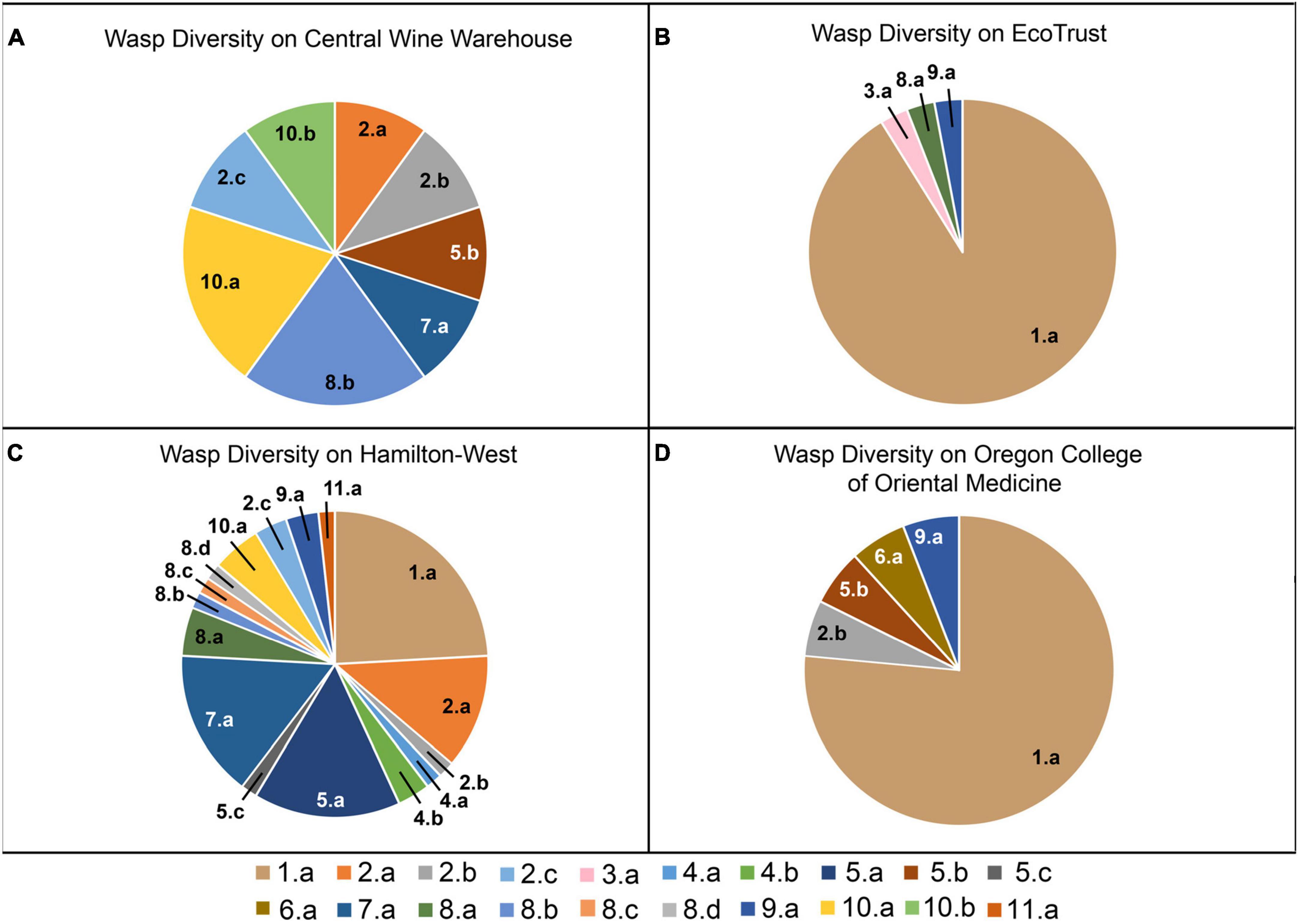
Figure 3. Composition of Hymenoptera morphospecies collected using pitfall traps during April to June of 2015 on green roof locations in Portland, Oregon: (A) CWW, (B) ET, (C) HW, and (D) OCOM.
The most abundant morphospecies present was in the family Aphelinidae, and this morphospecies constituted nearly half of all the specimens collected (Table 2 and Figures 2, 3). The second-most represented family on the green roofs was Ichneumonidae, which comprised roughly 10% of the total specimens (Table 2 and Figure 4). Interestingly, there were nine specimens from the family Mymaridae, a group of especially small parasitic wasps, yet five unique morphospecies from this family across all roofs. Roughly half (∼52%) of the wasps were determined to be specialists on Hemiptera (Arthropoda; Hexapoda) as a primary host (Figure 5), followed by 21% being generalists and 15% being specialists on insects (Table 2 and Figure 5).
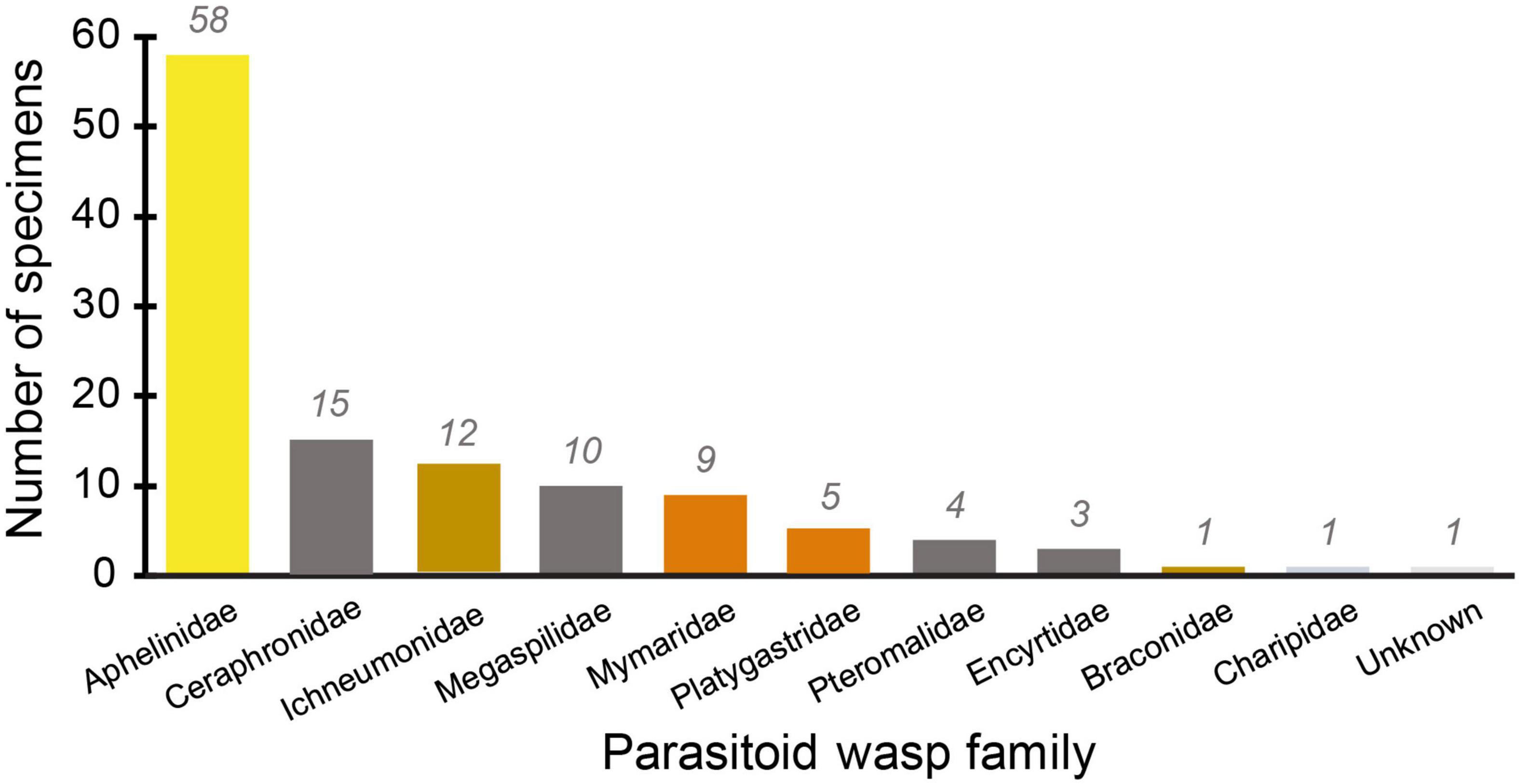
Figure 4. Abundance of individuals (N = 119) from within each family of Hymenoptera present on four green roofs.
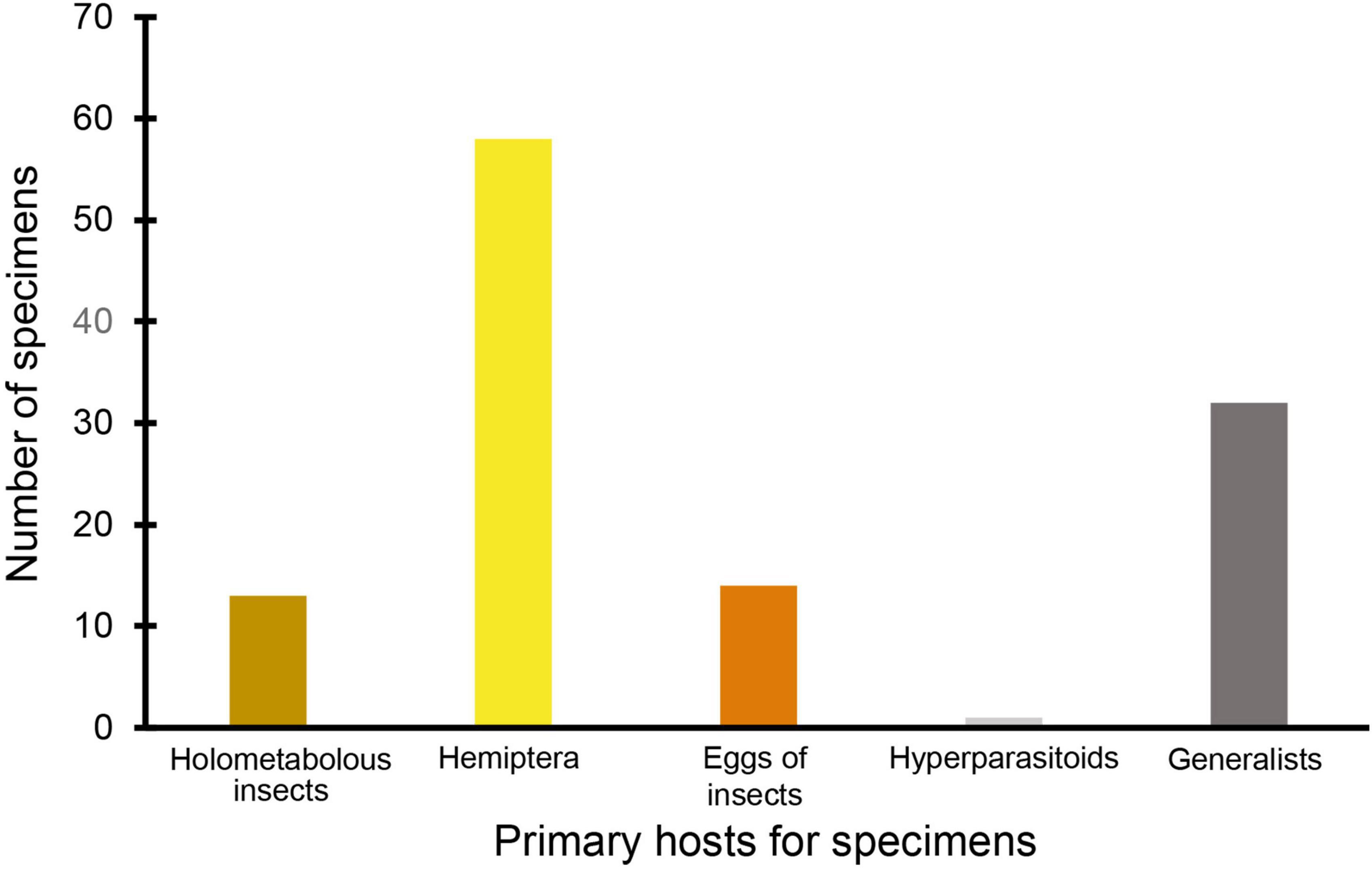
Figure 5. Total abundance of parasitoid wasps (Hymenoptera) from each functional feeding guild from all four green roofs. The family and feeding guild to which one specimen belonged could not be determined and therefore was omitted.
Both indices of diversity (the effective species number or Shannon’s entropy and Simpson’s reciprocal indices) indicated that wasp biodiversity was higher on the two green roofs with a mix of herbaceous grasses and flowering plants than on the two roofs with mostly Sedum plants (Table 3). Roof age (β = −2.4 ± 4.9, t = −0.5, P = 0.7) and size (β = 0.4 ± 4.9, t = 0.09, P = 0.9) did not impact the richness of wasps (q = 0). Diversity of wasps (q = 1) was also not affected by roof age (β = −2.1 ± 3.2, t = −0.6, P = 0.6) or size (β = 1.8 ± 4.9, t = 0.5, P = 0.7). Roof age (β = −1.5 ± 2.3, t = −0.7, P = 0.6) and size (β = 2.1 ± 2.3, t = 0.9, P = 0.5) similarly did not impact Simpson’s inverse index (q = 2). Finally, the species accumulation curves suggest that additional sampling would likely maintain and strengthen existing patterns, with the herbaceous roofs hosting greater parasitoid diversity than the Sedum-dominated roofs (Figure 6 and Supplementary Figure 1).
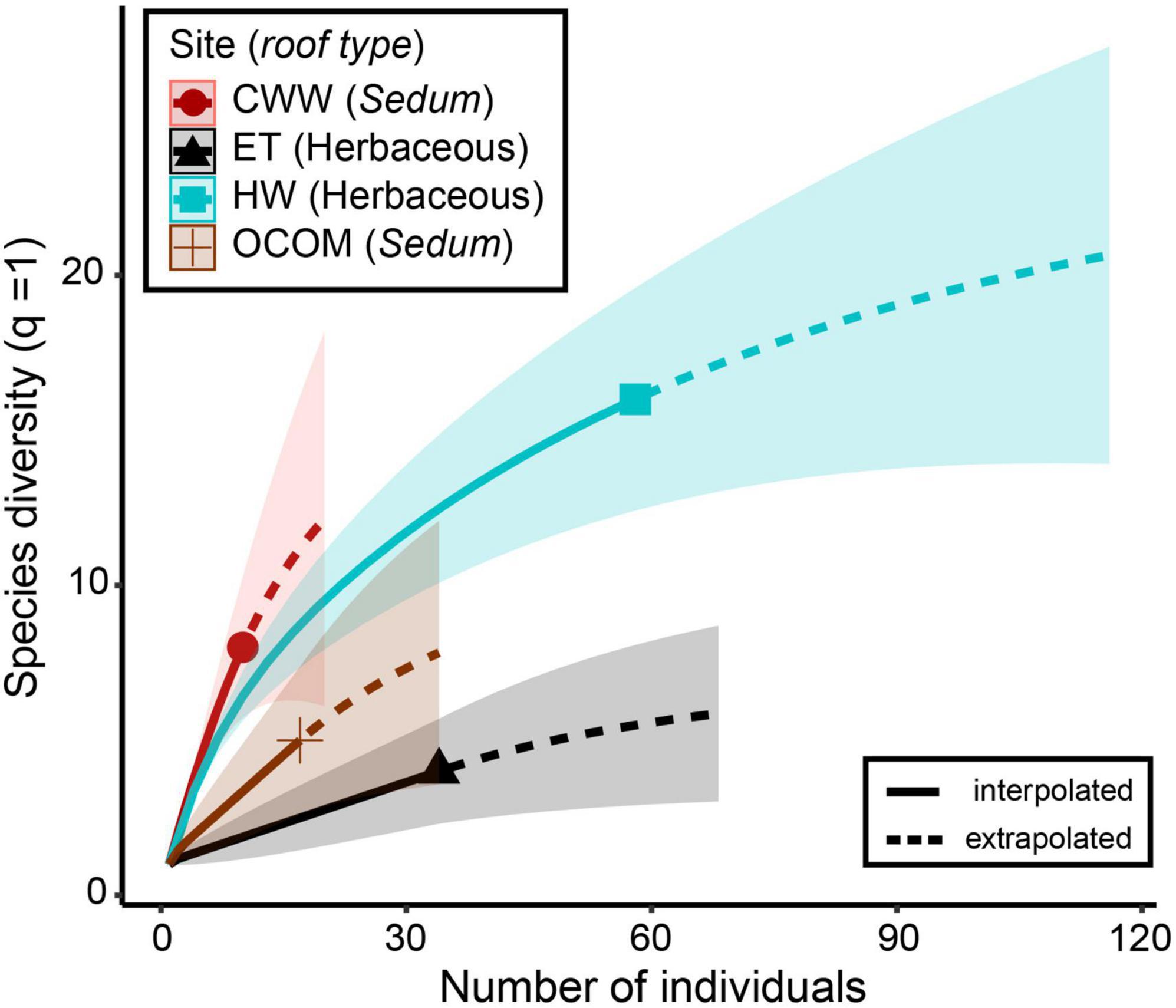
Figure 6. Sample-based species accumulation curves for Shannon diversity (q = 1) based on rarefaction (solid line segment) and extrapolation (dotted line segments) with bootstrapped 95% confidence intervals (shaded areas) for Hymenoptera specimens (N = 119) collected from April to June of 2015 for each green roof locations: Central Wine Warehouse (CWW), Hamilton West (HW), EcoTrust (ET), and Oregon College of Oriental Medicine (OCOM).
Measurements from aerial imagery of the landscape surrounding the roofs showed substantial variability among the four sites. Distance to the nearest natural habitat varied among the four roof sites from 32 to 485 m. Within a radius of 0.5 km, total summed area of natural habitat ranged from 0 to 8.84 ha (i.e., 0–11.3% of the available area). Within a radius of 1.0 km, total summed area of natural habitat ranged from 9.68 to 42.67 ha (i.e., 3.1–13.6% of the available area). However, these measures showed little apparent relationship to our species diversity values. Distance to the nearest natural habitat had no statistically significant bearing on any of the three species diversity measures. Likewise, all regression analyses were also insignificant for the measure of total area of natural habitats within 0.5 km of each roof. For total area of natural habitats within 1.0 km of each roof, this measure significantly predicted species richness (β = 0.36 ± 0.20, t = 7.71, P < 0.02), but did not significantly predict the other two measures of species diversity.
Discussion
Parasitoid wasps as biological control agents
Biological control agents include primarily predatory or parasitic insects that function as natural enemies of herbivorous pests, helping to keep populations of such pests in check. Host specialization, combined with the hyper-diverse nature of parasitoid wasps, makes them ideal agents for biological control (Strand and Obrycki, 1996). Wasps from three of the families we found on green roofs in Portland, Oregon, are commonly used in biological control: Aphelinidae, Encyrtidae, and Mymaridae (Kimberling, 2004). This suggests that Portland’s green roofs may be functioning as small-scale ecosystems with some internal capacity for pest control. Even though some of the parasitoids may exist in low numbers, the presence of such biocontrol agents could reduce the need for green roof managers to use chemical pesticides, which can have harmful unintended consequences and have been implicated in the decline of pollinators (Pimentel et al., 1992; Gill et al., 2012; Potts et al., 2016).
Parasitoids and urbanization
We documented that a diverse assemblage of parasitoid wasp taxa exists on green roofs within the urban core of downtown Portland. Although these isolated patches of habitat are located in a densely developed metropolitan center, we found them to contain a strikingly high abundance and diversity of these insects. Of the 119 specimens captured and identified over a 3-month period, there were 10 families present with 20 morphologically distinct species. In contrast, in a study of 15 green roofs in a semi-rural region of Argentina, only eight morphospecies of parasitoid wasps were found during the two peak months of arthropod activity (Dominguez et al., 2020). The Argentinian green roofs were all relatively new, with an average age of about 3 years since construction, which may have limited their biodiversity. However, in Portland, a seasonal drought occurred during our sampling period, and parasitoid wasp populations tend to decrease under drought conditions (Aslam et al., 2013; Portman et al., 2021). This is particularly relevant for CWW, which received no supplementary irrigation (Table 1). Our most biodiverse roof, HW, was only moderately irrigated, while the drought conditions were likely offset at the ET location, which had automated irrigation running regularly (Gonsalves, 2016). All told, the data available from this study and from Dominguez et al. (2020) suggest that green roofs in urban Portland support a more diverse assemblage of parasitoid wasps than green roofs in some semi-rural regions of the Americas.
Studies examining the role of biological control in urban greenspaces have shown mixed results as to how urbanization influences parasitoid assemblages. The degree of urban development did not impact the species richness of wasps collected across 15 remnant green spaces inside Sydney, Australia (Christie and Hochuli, 2009). However, in a study of 18 urban gardens across the central California coast, species richness decreased with increasing urbanization (Burks and Philpott, 2017). A recent meta-analysis on the effect of urbanization on biological control concluded that although the abundance of wasps was not greatly impacted, the effectiveness of parasitoid Hymenoptera tended to decline as urbanization increased (Korányi et al., 2022). These mixed results could be due to disparities in the degree of habitat fragmentation and complexity around the study locations, as well as differences in dispersal abilities of the biological control insects captured.
Questions on dispersal and establishment
Data is lacking on how most parasitoids arrive and become established atop green roofs. At our Portland sites, we found multiple wasps that specialize in parasitizing the eggs of their hosts, despite the small size and presumed limited dispersal ability of these Hymenopterans. For example, four morphospecies of wasps in the family Mymaridae were found on three of the four green roofs in our survey. All species of Mymaridae are parasitoids of the eggs of other insects, and could be important general biological control agents. They are small, rarely more than 1.5 mm (Goulet and Huber, 1993), and it has been suggested that such parasitoids would be unlikely to colonize urban green roofs due to their small size (Quispe and Fenoglio, 2015). However, Corbett and Rosenheim (1996) demonstrated that one species of Mymarid wasp managed to disperse up to 24.5 m/day, including traveling upwind. Vergnes et al. (2017) suggested that passive aerial dispersal of arthropods could be a driving force for biodiversity of urban green roofs, as they found diverse families of insects caught in aerial traps on buildings in the urban core of Paris, France. While data suggest that parasitoid wasps can colonize roofs, further research is needed to determine when Mymarid wasps can be expected to occur on green roofs and what conditions might facilitate their arrival.
Although it is possible that the parasitoid taxa we collected were initially brought in along with green roof plantings or with soil amendments, we think that is unlikely, as we would have expected to see more similar results across the four study sites had that been the case. Instead, we found that wasp diversity across sites was highly variable and was greatest on a roof that harbored a complex plant community, the HW roof (Table 2). The HW roof was planted much longer ago than the other three roofs sampled (16 years ago, versus seven or fewer years), and the diversity of parasitoids present may mirror the diversity of their host taxa on these roofs. Indeed, in a study of the beetle diversity on green roofs in Portland, this same roof was found to be more similar to the (highly diverse) ground sites that were sampled than to other comparably planted green roofs (Gonsalves et al., 2022). We suggest that this particular roof may be comparable to a later successional stage of an ecosystem, having accumulated through time an increasingly diverse assemblage of fauna.
If wasps establish on green roofs naturally by airborne dispersal from surrounding regions, then we might expect to find that roofs closer to areas of natural habitat, or those with greater amounts of natural habitat nearby, would show greater species diversity. In classic island biogeography theory, islands closer to source populations are expected to accumulate larger numbers of species. Our analyses of measures of natural habitat in the landscape surrounding the roof sites showed only the slightest suggestion of such a “distance effect” expected from theory. Of nine regression tests, only one showed a significant relationship: species richness was positively correlated with the total area of natural habitat within 1.0 km of each roof. The HW roof, which had the greatest species richness (as well as the greatest number of wasps), also had by far the most natural habitat nearby, as its location toward the edge of the downtown urban core placed it in closer proximity to residential neighborhoods with large amounts of tree cover.
Proximity to natural areas of vegetated habitat could well play an important role in shaping the diversity on green roofs. However, given our small sample size of four roofs, the highly complex nature of the urban environment, and the difficulty of knowledgably identifying productive source habitat for dispersing wasps, one should perhaps not be surprised that a stronger distance effect was not detected. Indeed, few green-roof studies have shown an island biogeographic effect, and controlling for the many variables present among different roofs and the small number of roofs sampled in each study creates challenges for drawing strong conclusions about whether green roofs follow the standard predictions of island biogeography theory (Blank et al., 2017).
Differences among green roof sites
We found the species richness of parasitoid wasps to be higher on the herbaceous roofs than on the Sedum-dominated roofs. Each herbaceous roof (CWW and HW) ranked substantially higher than the Sedum-dominated roofs (ET and OCOM) in terms of diversity as shown by both the effective species number and Simpson’s reciprocal index (Table 3). The species accumulation curves suggest that even with continued sampling effort, the two Sedum-dominated roofs would continue to produce fewer species than the two herbaceous roofs (Figure 6). A major difference between these two types of roofs is that the herbaceous roofs feature a more complex vegetative structure. Although it is difficult to draw strong conclusions given the limited number of roofs used in this study, our results suggest that a structurally diverse plant community can better support a diverse parasitoid wasp community. Each green roof provides site-specific habitat, which can directly affect the abundance and diversity of the invertebrates found there (Brenneisen, 2006). And indeed, Madre et al. (2013) and Gonsalves et al. (2022) showed with green roofs that a higher species richness for several arthropod taxa was correlated with greater structural complexity.
The two roof types in our study also differed greatly in abundance of the different types of parasitoids, with some being substantially more prevalent than others (Table 2). Interestingly, the two roofs planted primarily with Sedum plants were dominated by wasps in the family Aphelinidae (91% of ET and 76% of OCOM). The majority of aphelinids are parasitoids of the suborder Sternorrhyncha (Hemiptera), particularly the Aphidoidea (aphids), Aleyrodoidea (whiteflies), and Coccoidea (scale insects) (Goulet and Huber, 1993). Rand and Tscharntke (2007) demonstrated that parasitoids that are aphid specialists are limited by local host availability, in contrast to generalist natural enemy groups, which are less reliant on local host densities. Sedum-dominated roofs are generally a monoculture and are therefore more vulnerable to herbivore pest outbreaks than are the biodiverse plantings of an herbaceous roof. While both roof types had wasps from the generalist families of Pteromalidae and Ceraphronidae, the herbaceous roof types contained wasps from four additional generalist families (Table 2).
Roof age and size
We found that the oldest roof (HW) supported the highest number of species overall and the highest number of species unique to a location, despite the fact that it was the second-smallest roof in area vegetated (Table 1 and Figure 3). This is somewhat surprising in terms of expectations from island biogeography theory, as larger land masses (or in this case, larger green roofs) would be predicted to harbor more biodiversity. On the other hand, older roofs have had more time to accumulate biodiversity, leading to the expectation that diversity should rise with roof age, which is consistent with our findings. If we compare our two roofs planted with herbaceous plants and grasses, similar patterns are revealed. The CWW roof is more than three times larger than the HW roof, but less than half its age (7 years for CWW as opposed to 16 years for HW). In terms of parasitoid abundance, CWW held only about one-sixth as many individuals, and its species richness was less than half that of HW. Thus, our results appear to suggest that in this system, age may play a more influential role than area in contributing to species diversity.
Our finding of increased diversity with increased age of the green roof is mirrored by some previous studies of taxa on green roofs, but differs from other studies. Some predatory arthropod taxa, including parasitoid Hymenoptera, have been shown to increase in diversity with roof age for young roofs less than 4 years of age (Kyrö et al., 2022). Also in support of the idea that biodiversity can correlate with age, a study on green roofs in Argentina found that roof age was correlated with abundance of insect predators, which included parasitoid wasps (Fabian et al., 2021).
In contrast, a study of beetles on green roofs in Switzerland found that the age of the roof was negatively correlated with beetle diversity (Kyrö et al., 2018). The beetles that were collected were primarily herbivorous habitat generalists adapted to dry conditions and the authors suggested that these are species adapted to early successional habitats (Kyrö et al., 2018). However, in a study designed to assess whether green roofs undergo successional changes, it was found that there was not a general pattern of plant community succession, but instead, that other biotic and abiotic factors influenced species richness and diversity (Ksiazek-Mikenas et al., 2018). Still, that study did find that arthropod diversity generally increased with age of the roof, although those results were not statistically significant (Ksiazek-Mikenas et al., 2018).
While our results suggest that plant community structure and the age of urban green roofs may each affect parasitoid diversity, firm conclusions await further study. Future research with more roofs and longer monitoring periods could potentially speak to which type of roof vegetation best supports more varied wasp communities. Our species accumulation data suggest that longer monitoring at our sites would have resulted in additional taxa continuing to be found, particularly for the CWW site (Figure 6). Indeed, Gonsalves (2016) was still recovering beetles from pitfall traps at all of these green roof sites into the month of September, illustrating that other insects survive on green roofs for much of the year. Testing explicitly for the influence of roof age on biodiversity patterns in future studies would be useful, but could be difficult due to the fact that the number of green roofs more than a decade old is limited.
Caveats
The use of different sampling methods could potentially influence results obtained. A reliance on sampling by pitfall traps has the potential to bias the collection of parasitoid wasp specimens toward those taxa with limited flight ability or those that attach to cursorial invertebrates. As this study did not include a secondary trapping method, it is likely that we under-sampled the overall presence of parasitoids at our study sites. This makes the diversity that we did find especially notable, as adult parasitoid wasps can fly and a majority of their hosts would have been on the plants instead of in the soil. Indeed, for these reasons it seems likely that a substantially greater number and diversity of parasitoid wasps was in fact present on the green roofs we sampled. Future research could expand the sampling reach by using Malaise traps, which have been suggested as an optimal technique to understand the relationship between parasitoid abundance or diversity and habitat characteristics (Fraser et al., 2008).
An additional and important caveat to this study is that we analyzed data for only one field season. It is known that Hymenopteran communities typically experience species turnover from year to year in habitats in which they normally reside (e.g., Christie and Hochuli, 2009). As a result, we might expect that data from subsequent years from these sites could yield somewhat differing results.
Conclusion
This study documents that green roofs in a highly disturbed downtown urban center can support ecosystems complex enough to include a diversity of organisms at high trophic levels (in this case, parasitoid wasps). The fact that parasitoids are present in these small habitat patches in the urban core of Portland indicates that their hosts are also present. Therefore, our data allow us to infer that a wide array of invertebrates are likely utilizing the green roofs. This is important, as biodiversity is considered critical to ecosystem processes and ecosystem function, as well as to strengthening the resilience of ecosystems (Cardinale et al., 2012). Future research will be required to address the many questions that naturally arise from a survey such as ours, including questions on the role of host-specificity in green roof colonization by parasitoids, the roles of native versus non-native species, and the impacts of urbanization on parasitoid and host assemblages.
Still, by establishing that green roofs in the urban core of Portland support a diverse community of parasitoid wasps, we can help inform our understanding of the ecological services provided by green infrastructure. Wasps in the families we documented can be important in the biological control of pest insects. The fact that small areas of vegetated roofs can harbor diverse assemblages of biological control agents suggests that green roofs could play a role in providing connectivity with parks, greenways, and urban gardens, allowing parasitoids to move among these areas. If green roofs can act as source areas for biocontrol agents, this could help reduce demand for the use of chemical pesticides in urban centers, alleviating the negative impacts of these chemicals on human health and the environment. We recommend that future green roof installations should consider their utility more broadly in terms of the ecological services they can provide, and should aim to support more arthropod biological control agents. Planting green roofs with more diverse herbaceous plants, instead of Sedum-dominated roofs, may well be a straightforward way to promote biodiversity in urban landscapes. As such, this study suggests that managing elements of green infrastructure in human-dominated landscapes to enhance biodiversity can play a significant role in enhancing ecological services that contribute to human quality of life in urban areas.
Data availability statement
The original contributions presented in this study are included in the article/Supplementary material, further inquiries can be directed to the corresponding author.
Author contributions
AD and SM conceived and designed the study, planned the data analyses, and contributed to subsequent revisions. AD conducted the lab work and performed all the statistical analyses. AD wrote the first draft of the manuscript. Both authors contributed to the article and approved the submitted version.
Acknowledgments
We would gratefully like to acknowledge the funding and support of the Ronald E. McNair Scholars Program at Portland State University (PSU). The study would not have been possible without the participation of Olyssa Starry at PSU, who pioneered the study of green roof invertebrates in Portland. We would like to thank Heidi Liere and Diego Fernando Campos Moreno, for their invaluable contribution to insect identification. We are also grateful to all the members of the Masta Lab at PSU, particularly to Jessica Szabo for her mentorship. Furthermore, Adrienne Godschalx was also instrumental to the completion of this work.
Conflict of interest
The authors declare that the research was conducted in the absence of any commercial or financial relationships that could be construed as a potential conflict of interest.
Publisher’s note
All claims expressed in this article are solely those of the authors and do not necessarily represent those of their affiliated organizations, or those of the publisher, the editors and the reviewers. Any product that may be evaluated in this article, or claim that may be made by its manufacturer, is not guaranteed or endorsed by the publisher.
Supplementary material
The Supplementary Material for this article can be found online at: https://www.frontiersin.org/articles/10.3389/fevo.2022.983401/full#supplementary-material
References
Angel, S., Parent, J., Civco, D. L., Blei, A., and Potere, D. (2011). The dimensions of global urban expansion: Estimates and projections for all countries, 2000-2050. Prog. Plan. 75, 53–107. doi: 10.1016/j.progress.2011.04.001
Aslam, T. J., Johnson, S. N., and Karley, A. J. (2013). Plant-mediated effects of drought on aphid population structure and parasitoid attack. J. Appl. Entomol. 137, 136–145. doi: 10.1111/j.1439-0418.2012.01747.x
Bischl, B., Lang, M., Bossek, J., Horn, D., Richter, J., and Surmann, D. (2014). BBmisc: Miscellaneous helper functions for B. Bischl. R package version 1.13. Available online at: https://CRAN.R-project.org/package=BBmisc
Blank, L., Vasl, A., Schindler, B. Y., Kadas, G. J., and Blaustein, L. (2017). Horizontal and vertical Island biogeography of arthropods on green roofs: A review. Urban Ecosyst. 20, 911–917.
Bonet, A. (2008). “Parasitoid wasps, natural enemies of insects,” in Tropical biology and conservation management – volume 7: Phytopathology and entomology, eds K. Del Claro, P. S. Oliveira, and V. Rico-Gray (Abu Dhabi: EOLSS), 185–207.
Braaker, S., Ghazoul, J., Obrist, M. K., and Moretti, M. (2014). Habitat connectivity shapes urban arthropod communities: The key role of green roofs. Ecology 95, 1010–1021. doi: 10.1890/13-0705.1
Braaker, S., Obrist, M. K., Ghazoul, J., and Moretti, M. (2017). Habitat connectivity and local conditions shape taxonomic and functional diversity of arthropods on green roofs. J. Anim. Ecol. 86, 521–531. doi: 10.1111/1365-2656.12648
Brenneisen, S. (2006). Space for urban wildlife: Designing green roofs as habitats in Switzerland. Urban Habit. 4, 27–36.
Broad, G. (2011). Identification key to the subfamilies of Ichneumonidae (Hymenoptera). London: The Natural History Museum.
Bryant, M. M. (2006). Urban landscape conservation and the role of ecological greenways at local and metropolitan scales. Landsc. Urban Plan. 76, 23–44. doi: 10.1016/j.landurbplan.2004.09.029
Burkman, C. E., and Gardiner, M. M. (2014). Urban greenspace composition and landscape context influence natural enemy community composition and function. Biol. Control 75, 58–67. doi: 10.1016/j.biocontrol.2014.02.015
Burks, J. M., and Philpott, S. M. (2017). Local and landscape drivers of parasitoid abundance, richness, and composition in urban gardens. Environ. Entomol. 46, 201–209. doi: 10.1093/ee/nvw175
Cardinale, B. J., Duffy, J. E., Gonzalez, A., Hooper, D. U., Perrings, C., Venail, P., et al. (2012). Biodiversity loss and its impact on humanity. Nature 486, 59–67. doi: 10.1038/nature11148
Chao, A., Gotelli, N. J., Hsieh, T. C., Sander, E. L., Ma, K. H., Colwell, R. K., et al. (2014). Rarefaction and extrapolation with Hill numbers: A framework for sampling and estimation in species diversity studies. Ecol. Monogr. 84, 45–67.
Christie, F. J., and Hochuli, D. F. (2009). Responses of wasp communities to urbanization: Effects on community resilience and species diversity. J. Insect Conserv. 13, 213–221. doi: 10.1007/s10841-008-9146-5
Corbett, A., and Rosenheim, J. A. (1996). Quantifying movement of a minute parasitoid, Anagrus epos (Hymenoptera: Mymaridae), using fluorescent dust marking and recapture. Biol. Control 6, 35–44. doi: 10.1006/bcon.1996.0005
Damalas, C. A., and Eleftherohorinos, I. G. (2011). Pesticide exposure, safety issues, and risk assessment indicators. Int. J. Environ. Res. Public Health 8, 1402–1419. doi: 10.3390/ijerph8051402
Dominguez, M. V. S., Gonzalez, E., Fabian, D., Salvo, A., and Fenoglio, M. S. (2020). Arthropod diversity and ecological processes on green roofs in a semi-rural area of Argentina: Similarity to neighbor ground habitats and landscape effects. Landsc. Urban Plan. 199:103816. doi: 10.1016/j.landurbplan.2020.103816
Fabian, D., Gonzalez, E., Dominguez, M. V. S., Salvo, A., and Fenoglio, M. S. (2021). Towards the design of biodiverse green roofs in Argentina: Assessing key elements for different functional groups of arthropods. Urban For. Urban Green. 61:127107. doi: 10.1016/j.ufug.2021.127107
Fahrig, L. (2003). Effects of habitat fragmentation on biodiversity. Annu. Rev. Ecol. Evol. Syst. 34, 487–515. doi: 10.1146/annurev.ecolsys.34.011802.132419
Fenoglio, M. S., Videla, M., Salvo, A., and Valladares, G. (2013). Beneficial insects in urban environments: Parasitism rates increase in large and less isolated plant patches via enhanced parasitoid species richness. Biol. Conserv. 164, 82–89. doi: 10.1016/j.biocon.2013.05.002
Fraser, S. E. M., Dytham, C., and Mayhew, P. J. (2008). The effectiveness and optimal use of malaise traps for monitoring parasitoid wasps. Insect Conserv. Divers. 1, 22–31. doi: 10.1111/j.1752-4598.2007.00003.x
Gill, R. J., Ramos-Rodriguez, O., and Raine, N. E. (2012). Combined pesticide exposure severely affects individual- and colony-level traits in bees. Nature 491, 105–108. doi: 10.1038/nature11585
Godfray, C. (1994). Parasitoids: Behavioral and evolutionary ecology. Princeton, NJ: Princeton University Press.
Gomez, J. M., and Zamora, R. (1994). Top-down effects in a tritrophic system – parasitoids enhance plant fitness. Ecology 75, 1023–1030. doi: 10.2307/1939426
Gonsalves, S. (2016). Green roofs and urban biodiversity: Their role as invertebrate habitat and the effect of design on beetle community. M.Sc. thesis. Portland, OR: Portland State University.
Gonsalves, S., Starry, O., Szallies, A., and Brenneisen, S. (2022). The effect of urban green roof design on beetle biodiversity. Urban Ecosyst. 25, 205–219. doi: 10.1007/s11252-021-01155-x
Goulet H, Huber JT. (1993). Hymenoptera of the world: An identification guide to families. Ottawa, ON: Agriculture Canada.
Gradish, A. E., Scott-Dupree, C. D., Shipp, L., Harris, C. R., and Ferguson, G. (2011). Effect of reduced risk pesticides on greenhouse vegetable arthropod biological control agents. Pest Manag. Sci. 67, 82–86. doi: 10.1002/ps.2036
Hassell, M. P., and Waage, J. K. (1984). Host-parasitoid population interactions. Annu. Rev. Entomol. 29, 89–114. doi: 10.1146/annurev.en.29.010184.000513
Heimpel, G. E., and Collier, T. R. (1996). The evolution of host-feeding behaviour in insect parasitoids. Biol. Rev. 71, 373–400. doi: 10.1111/j.1469-185X.1996.tb01279.x
Hsieh, T. C., Ma, K. H., and Chao, A. (2016). iNEXT: An R package for rarefaction and extrapolation of species diversity (Hill numbers). Methods Ecol. Evol. 7, 1451–1456.
Hsieh, T. C., Ma, K. H., and Chao, A. (2022). iNEXT: Interpolation and extrapolation for species diversity. R package version 3.0.0. Available online at: http://chao.stat.nthu.edu.tw/wordpress/software_download/
Jervis, M. A., and Kidd, N. A. C. (1986). Host-feeding strategies in hymenopteran parasitoids. Biol. Rev. 61, 395–434. doi: 10.1111/j.1469-185X.1986.tb00660.x
Jones, E. L., and Leather, S. R. (2012). Invertebrates in urban areas: A review. Eur. J. Entomol. 109, 463–478. doi: 10.14411/eje.2012.060
Kimberling, D. N. (2004). Lessons from history: Predicting successes and risks of intentional introductions for arthropod biological control. Biol. Invasions 6, 301–318. doi: 10.1023/B:BINV.0000034599.09281.58
Korányi, D., Egerer, M., Rusch, A., Szabó, B., and Batáry, P. (2022). Urbanization hampers biological control of insect pests: A global meta-analysis. Sci. Total Environ. 834:155396. doi: 10.1016/j.scitotenv.2022.155396
Ksiazek-Mikenas, K., Herrmann, J., Menke, S. B., and Köhler, M. (2018). If you build it, will they come? Plant and arthropod diversity on urban green roofs over time. Urban Nat. 1, 52–72.
Kyrö, K., Brenneisen, S., Kotze, D. J., Szallies, A., Gerner, M., and Lehvävirta, S. (2018). Local habitat characteristics have a stronger effect than the surrounding urban landscape on beetle communities on green roofs. Urban For. Urban Green. 29, 122–130. doi: 10.1016/j.ufug.2017.11.009
Kyrö, K., Kankaanpää, T., Vesterinen, E. J., Lehvävirta, S., and Kotze, D. J. (2022). Arthropod communities on young vegetated roofs are more similar to each other than to communities at ground level. Front. Ecol. Evol. 10:785448. doi: 10.3389/fevo.2022.785448
Lepczyk, C. A., Aronson, M. F. J., Evans, K. L., Goddard, M. A., Lerman, S. B., and Macivor, J. S. (2017). Biodiversity in the city: Fundamental questions for understanding the ecology of urban green spaces for biodiversity conservation. BioScience 67, 799–807. doi: 10.1093/biosci/bix079
Losey, J. E., and Vaughan, M. (2006). The economic value of ecological services provided by insects. BioScience 56, 311–323.
Madre, F., Vergnes, A., Machon, N., and Clergeau, P. (2013). A comparison of 3 types of green roof as habitats for arthropods. Ecol. Eng. 57, 109–117. doi: 10.1016/j.ecoleng.2013.04.029
National Oceanic and Atmospheric Administration [NOAA] (2015). Daily summaries station details, National weather service. Available online at: https://www.ncdc.noaa.gov/cdo-web/datasets/GHCND/stations/GHCND:USW00024229/detail (accessed November 21, 2022).
Oberndorfer, E., Lundholm, J., Bass, B., Coffman, R. R., Doshi, H., Dunnett, N., et al. (2007). Green roofs as urban ecosystems: Ecological structures, functions, and services. BioScience 57, 823–833. doi: 10.1641/b571005
Pataki, D. E. (2015). Grand challenges in urban ecology. Front. Ecol. Evol. 3:57. doi: 10.3389/fevo.2015.00057
Petremand, G., Chittaro, Y., Braaker, S., Brenneisen, S., Gerner, M., Obrist, M. K., et al. (2018). Ground beetle (Coleoptera: Carabidae) communities on green roofs in Switzerland: Synthesis and perspectives. Urban Ecosyst. 21, 119–132. doi: 10.1007/s11252-017-0697-7
Pimentel, D., Acquay, H., Biltonen, M., Rice, P., Silva, M., Nelson, J., et al. (1992). Environmental and economic costs of pesticide use. BioScience 42, 750–760. doi: 10.2307/1311994
Portman, S. L., Santa Cruz, K. E., and Moran, P. J. (2021). Host plant water deficit stress impairs reproduction and development of the galling fly (Parafreutreta regalis), a biological control agent of cape-ivy (Delairea odorata). Biol. Control 156:104555. doi: 10.1016/j.biocontrol.2021.104555
Potts, S. G., Imperatriz-Fonseca, V., Ngo, H. T., Aizen, M. A., Biesmeijer, J. C., Breeze, T. D., et al. (2016). Safeguarding pollinators and their values to human well-being. Nature 540, 220–229. doi: 10.1038/nature20588
Quicke, D. L. J. (2015). The braconid and ichneumonid parasitoid wasps: Biology, systematics, evolution and ecology. Hoboken, NJ: Wiley-Blackwell.
Quispe, I., and Fenoglio, M. S. (2015). Host-parasitoid interactions on urban roofs: An experimental evaluation to determine plant patch colonisation and resource exploitation. Insect Conserv. Divers. 8, 474–483. doi: 10.1111/icad.12127
Rand, T. A., and Tscharntke, T. (2007). Contrasting effects of natural habitat loss on generalist and specialist aphid natural enemies. Oikos 116, 1353–1362. doi: 10.1111/j.2007.0030-1299.15871.x
R Development Core Team (2011). R: A language and environment for statistical computing. Vienna: R Foundation for Statistical Computing.
Riva, F., and Fahrig, L. (2022). The disproportionately high value of small patches for biodiversity conservation. Conserv. Lett. 15:e1288. doi: 10.1111/conl.12881
Seto, K. C., Guneralp, B., and Hutyra, L. R. (2012). Global forecasts of urban expansion to 2030 and direct impacts on biodiversity and carbon pools. Proc. Natl. Acad. Sci. U.S.A. 109, 16083–16088. doi: 10.1073/pnas.1211658109
Silveira, L. C. P., Souza, I. L., Tomazella, V. B., and Mendez, H. A. G. (2019). “Parasitoid insects,” in Natural enemies of insect pests neotropical agroecosystems: Biological control and functional biodiversity, eds B. Souza, L. L. Vázquez, and R. C. Marucci (Cham: Springer International Publishing), 97–109.
Starry, O., Gonsalves, S., Ksiazek-Mikenas, K., MacIvor, J. S., Gardner, M., Szallies, A., et al. (2018). A global comparison of beetle community composition on green roofs and the potential for homogenization. Urban Nat. Spec. Issue 2018, 1–15.
Strand, M. R., and Obrycki, J. J. (1996). Host specificity of insect parasitoids and predators. BioScience 46, 422–429. doi: 10.2307/1312876
van Lenteren, J. C., Bolckmans, K., Kohl, J., Ravensberg, W. J., and Urbaneja, A. (2018). Biological control using invertebrates and microorganisms: Plenty of new opportunities. Biocontrol 63, 39–59. doi: 10.1007/s10526-017-9801-4
Vergnes, A., Le Saux, E., and Clergeau, P. (2017). Preliminary data on low aerial plankton in a large city center, Paris. Urban For. Urban Green. 22, 36–40. doi: 10.1016/j.ufug.2017.01.012
Ward, D. F., New, T. R., and Yen, A. L. (2001). Effects of pitfall trap spacing on the abundance, richness and composition of invertebrate catches. J. Insect Conserv. 5, 47–53. doi: 10.1023/A:1011317423622
Williams, N. S. G., Lundholm, J., and MacIvor, J. S. (2014). Do green roofs help urban biodiversity conservation? J. Appl. Ecol. 51, 1643–1649. doi: 10.1111/1365-2664.12333
Woodcock, B. A. (2005). “Pitfall trapping in ecological studies,” in Insect sampling in forest ecosystems, ed. S. R. Leather (New York, NY: Wiley), 37–57.
Keywords: green roof, urban ecology, biocontrol, Hymenoptera, urban biodiversity
Citation: Diethelm AC and Masta SE (2022) Urban green roofs can support a diversity of parasitoid wasps. Front. Ecol. Evol. 10:983401. doi: 10.3389/fevo.2022.983401
Received: 01 July 2022; Accepted: 11 November 2022;
Published: 06 December 2022.
Edited by:
Olivia Sanllorente, University of Granada, SpainReviewed by:
Ek Del-Val, National Autonomous University of Mexico, MexicoLuís Cláudio Áterno Silveira, Universidade Federal de Lavras, Brazil
Copyright © 2022 Diethelm and Masta. This is an open-access article distributed under the terms of the Creative Commons Attribution License (CC BY). The use, distribution or reproduction in other forums is permitted, provided the original author(s) and the copyright owner(s) are credited and that the original publication in this journal is cited, in accordance with accepted academic practice. No use, distribution or reproduction is permitted which does not comply with these terms.
*Correspondence: Susan E. Masta, smasta@pdx.edu