- 1Department of Anthropology, The Pennsylvania State University, University Park, PA, United States
- 2Departamento de Ciencias Históricas y Geográficas, Universidad de Tarapacá, Arica, Chile
- 3Departamento de Antropología, Universidad de Tarapacá, Arica, Chile
- 4National Museum of Natural History, Smithsonian Institution, Washington, DC, United States
- 5Instituto de Alta Investigación, Universidad de Tarapacá, Arica, Chile
South America is a megadiverse continent that witnessed the domestication, translocation and cultivation of various plant species from seemingly contrasting ecosystems. It was the recipient and supplier of crops brought to and from Mesoamerica (such as maize and cacao, respectively), and Polynesia to where the key staple crop sweet potato was exported. Not every instance of the trans-ecological expansion of cultivated plants (both domesticated and wild), however, resulted in successful farming. Here, we review the transregional circulation and introduction of five food tropical crops originated in the tropical and humid valleys of the eastern Andes—achira, cassava, ahipa, sweet potato, and pacay—to the hyper-arid coastal valleys of the Atacama Desert of northern Chile, where they have been found in early archeological sites. By means of an evaluation of the contexts of their deposition and supported by direct radiocarbon dating, stable isotopes analyses, and starch grain analysis, we evaluate different hypotheses for explaining their introduction and adaptation to the hyper-arid soils of northern Chile, by societal groups that after the introduction of cultigens still retained a strong dependence on marine hunting, gathering and fishing ways of life based on wide variety of marine coast resources. Many of the studied plants were part of a broader package of introduced goods and technological devices and procedures, linked to food, therapeutic medicine, social and ritual purposes that transformed previous hunter-gatherer social, economic, and ideological institutions. Based on archeological data, we discuss some of the possible socio-ecological processes involved in the development of agricultural landscapes including the adoption of tropical crops originated several hundred kilometers away from the Atacama Desert during the Late Holocene.
Introduction
Archeological research into the processes of early food production, adoption of agriculture, translocation and cultivation of wild plants and subsequent socio-ecological transformations have been redefined on a global scale by a greater appreciation of the complexity of niche construction and other evolutionary processes involved and the agency that different organisms and organizations contributed to these dynamic interactions (Larson et al., 2014; Bogaard et al., 2021). In tandem, increasingly detailed studies of artifacts and ecofacts recovered from archeological sites are allowing ever more detailed information about the dynamic and multifaceted pathways associated with the morphological, genetic, and physiological changes involved in domestication and the dispersion of domesticates (Iriarte et al., 2020; Allaby et al., 2022). South America with its complex biogeography, natural and cultural history provides a myriad of comparative case studies to discuss how domestication processes developed, following varying trajectories of change and transformation (Piperno and Pearsall, 1998; Clement et al., 2010).
A particularly interesting problem is how numerous plants evolved in the humid conditions of the tropical Amazon Basin were successfully cultivated and spread to multiple environments including the hyper-aridity prevalent in much of the Pacific coast of western South America. Indeed, the ecological conditions of both regions not only affected these processes but also the conservation of the archeological evidence available to document them (Pearsall, 2008; Iriarte, 2009). Unlike the Amazon, where archaeobotanical remains are scarce and often limited to microremains (such as phytoliths, pollen, and starch grains), the Pacific coast’s extreme aridity often allows the preservation of macroremains (including desiccated fruits, seeds, wood, roots, tubers, and even whole plants), allowing a direct source of data to better investigate and solve questions related to the spread and adoption of cultigens domesticated elsewhere in the continent.
The Atacama Desert comprises the southern section of the Chilean-Peruvian coastal desert (ca. 5° S to 26° S) and extends as a longitudinal strip between the Pacific Ocean and the Andes, from Arica in the north (ca. 18° 20′ S) to Copiapó in the south (ca. 27° S) (Pinto et al., 2006). What sets the Atacama Desert apart from other deserts around the world is its extreme aridity, given by the total absence of rainfall from the coast up to ca. 2,000 m above sea level (asl) along with its particular location next to the Pacific Ocean, one of the most productive and biodiverse marine biomes of the planet. In terms of climate, coastal desert conditions predominate with abundant cloud cover, absence of frost, moderate winds, high relative humidity and high direct solar radiation throughout the year (González Vallejos et al., 2013). Increased agricultural and urban expansion has transformed the modern hydrology of the valleys of northern Chile, such as Lluta, Azapa, Chaca, and Camarones, but during Pre-Columbian times, the water available for irrigation and human consumption came from seasonal runoff from the highlands as well as surface runoff, emerging from various springs, such as those of San Miguel and Las Maytas in the Azapa valley, where many archeological sites are concentrated.
The exoreic valleys of the extreme north of Chile were inhabited by human groups since ca. 13,000 cal BP (Santoro et al., 2017). During the Middle Holocene, communities of hunters, gatherers, and fishermen, known archeologically as Chinchorro, developed a coastal way of life and human mummification practices that persisted for millennia. Starting ca. 4,000 years ago, during the Archaic/Formative transition, important changes are noted in the archeological record, including the abandonment of most mummification practices as well as the gradual adoption of new technologies such as cultivated foods and a shift in a settlement pattern from the coast toward the alluvial plains flanking the Azapa, Camarones, Chaca and other valleys (Muñoz Ovalle, 2004; Núñez and Santoro, 2011). During the Formative Period and into the Middle Horizon, increased population size and social complexity manifested in the progressive growth of the number and size of settlements and cemeteries along the coast and valleys (Uribe et al., 2020). Toward the beginning of the first millenium of our era, during the Late Intermediate Period, the archeological record shows the development of regional polities but with a shared cultural background, who intensively occupied the coast, valleys, foothills and highlands toward the east (Muñoz et al., 2016). These societies relied on a mixed agro-maritime subsistence, greater agricultural intensification with cultivation of crops on terraces and the assistance of technical irrigation, increased interregional interaction, and the development of artisanal technologies (ceramics, textiles, wood, bone, gourds, vegetable fiber, etc.) (Núñez, 1974; Rothhammer and Santoro, 2001; Valenzuela et al., 2015). While these societies were readily subjugated by the Inka and later by the Spanish, despite the transformations and the adoption of new technologies, their basic subsistence rested mainly on the exploitation of the rich Pacific Ocean maritime resources complemented by irrigation farming of a diverse range of introduced cultigens (Horta Tricallotis, 2010). Furthermore, considering the extremely good preservation conditions for plant remains offered by the Atacama Desert, its archeological record offers unique opportunities to explore processes such as the effects of novel environments on domesticated species. Therefore, in this paper we focus on five species initially domesticated in the Amazonian tropical forests, cerrado, and grasslands east of the Andes, but recovered from archeological contexts from the coast and valleys of the Atacama Desert in northern Chile.
Early food production east and west of the Andes
Recent archeological research suggests plant cultivation in the Americas began during the Early Holocene (10,700–8,200 cal BP) in regions such as Mesoamerica and the southwestern Amazon basin (Piperno and Pearsall, 1998; Clement et al., 2015; Watling et al., 2018; Iriarte et al., 2020; Lombardo et al., 2020). In the following millennia and likely driven by mobile and interconnected foraging and horticultural groups, plants such as cassava, cucurbits, maize and even cacao, progressively became disseminated throughout the Neotropics (Kistler et al., 2018; Watling et al., 2018; Zarrillo et al., 2018). While we still do not know very much about domestication processes in much of the Andean highlands, during the Middle Holocene (8,200–4,200 cal BP), increased socioeconomic engagement with camelids, wild tubers, and chenopods triggered the emergence of agropastoral communities in the central and south-central Andes (Pearsall, 1989; Lane, 2006; Bruno and Hastorf, 2016; Langlie, 2021; Miller et al., 2021). The adoption of agriculture in the Pacific coast seems to have occurred by the end of Middle Holocene and the beginning of the Late Holocene (4,200–0 cal BP), driven by semi-sedentary maritime societies, which benefited from planting cotton used for making fishing nets as well as a range of comestible cultigens (Hastorf, 1999; Dillehay, 2014; Beresford-Jones et al., 2018). These coastal agricultural communities expanded the construction of irrigation networks along with a diversification of resource utilization (Beresford-Jones et al., 2021) coupled with additional investment on significant agricultural infrastructure. Therefore, the adoption of food production in the rivers draining into the Pacific Ocean is often tied to population growth and emergent sociopolitical complexity as manifested in the construction of monumental architecture (Quilter et al., 1991; Shady Solis et al., 2001; Sandweiss et al., 2009). While the development of significant agricultural infrastructure did not occur everywhere, increased engagement with food production had major transformative effects in the entire continent by reshaping ecosystems, altering landscapes, and facilitating scalar human sedentism and demographic growth.
We should emphasize that engagement with food production and domesticated species was a heterogenous non-linear process that was shaped by various factors such as environmental conditions and population dynamics. The process of adopting agriculture in many valleys of the Peruvian central coast seems to have been facilitated by the development of agricultural infrastructure including irrigation canals opened upon extensive alluvial plains of rivers that continuously flow into the Pacific Ocean (Sotomayor Melo and Jiménez Milón, 2008; Beresford-Jones et al., 2015, 2018; Baitzel and Rivera Infante, 2019; Gayo et al., 2020).
In contrast, towards the south where runoff was seasonally constrained and perhaps involved high year-to-year variance, intensified agriculture could not occur within the intermittent river valleys. In the coast and western valleys of northern Chile, semi-sedentary maritime societies with significant social complexity persisted throughout the Holocene, and although they regularly utilized non-food inland resources, engagement with agriculture did not occur until the Late Holocene (Roberts et al., 2013; Standen et al., 2018, 2021; McRostie et al., 2019; García et al., 2020; Ugalde et al., 2021). Starting approximately 3,100 years ago, an emergence of villages, fields and cemeteries, often composed of burial mounds in valleys such as Azapa, Chaca, and Camarones, signals the advent of fully agricultural societies (Focacci and Erices, 1973; Núñez and Santoro, 2011; Muñoz Ovalle, 2012, 2017; Barba et al., 2015; Carter, 2016). During the following centuries, these sedentary communities augmented their engagement with various cultigens including maize, cotton, beans, gourds, squashes, and others. Remains of these plants has been collected thanks to the excellent preservation of organic materials in the hyper-arid ecosystem of the Atacama Desert, and although several scholars have explicitly mentioned the various cultigens found in archeological sites of the region, very few provide specific quantities or comment about the characteristics of the specimens (Vidal et al., 2004; García and Vidal, 2010; García and Uribe, 2012; Ledezma, 2014). For instance, Erices (1975) quantified the number of economically used plants in three cemeteries (Az-6, PML-4, and PML-7) excavated in the Arica coast and Azapa valley (Erices, 1975). Similarly, various reports document the presence of numerous domesticated taxa, but very few provide specific quantities from particular contexts, making any comparisons and quantitative evaluations challenging.
A recent review of plant utilization based on these and similar reports for the entire Atacama Desert concludes that a huge diversity of cultigens were present in the region in Pre-Columbian times, all of which were domesticated elsewhere (Ugalde et al., 2021). In addition, although some of the domesticates such as maize, beans, squash and even quinoa correspond to annual species propagated by seeds, an important portion of the domesticates found in the region are geophytes, which are vegetatively propagated plants that produce edible underground storage. Building on this work and employing a combination of archaeobotanical and archaeometric methods, we focus on a set of five tropical species with the goal of improving our understanding of how agriculture was adopted in the Atacama Desert. Specifically, our study species are achira (Canna indica L.), ahipa (Pachyrhizus sp. Rich. ex. DC), cassava (Manihot esculenta Crantz), sweet potato [Ipomoea batatas (L.) Lamark], and pacay (Inga feuillei DC). Because four of these species were vegetatively propagated (Denham et al., 2020), we are particularly interested in determining if these crops were indeed transplanted and cultivated, or merely transported as consumable goods by means of long-distance exchange networks. We further discuss alternative explanations to understand the socio-economic significance of these geophyte and tree crops for the societies who adopted them and their role in the transformative processes of landscape development in northern Chile during Pre-Columbian times.
The study cultigens
Achira
Achira (Canna indica L.) is a perennial herb from the Cannaceae family that grows up to 3 m tall and produces multiple fleshy stems, leaves, and multibranched eatable rhizomes (Maas-van de Kamer and Maas, 2008). Its leaves are organized alternatively, with very large leaf blades (20–60 cm long and 10–30 cm wide) and an ovate to oblong shape and entire margins (Delin and Kress, 2000). Flowers are bisexual, bright red to apricot yellow, organized in branched clusters (6–20 flowers) in a raceme of cininni (Flores et al., 2003). Fruits are ellipsoid to sub-globose capsules, surrounded by persistent sepals. When capsules are mature, they split up and release several smooth, black, large (5–8 mm long and 4–7 mm wide) and spherical to ovoid seeds with small but distinct cavities. Rhizomes are large, up to 60 cm in length, tuberous, up to 3.5 cm in diameter, and have a fibrosus adventitious root system. Rhizomes are eatable, possess a high starch content (80%), are easy to digest, and are typically cooked boiled or baked (National Research Council, 1989). Its starch can also be extracted to make flour for crackers or noodles. Starch grains are relatively large (10–100 μm), have an oval-shape, eccentric and closed hilum with a smooth surface, and diffuse lamellae (Thitipraphunkul et al., 2003; Pagán-Jiménez et al., 2015). Young shoots are occasionally consumed as a vegetable and seeds are used as beads for making jewelry. Many modern varieties of achira are planted as ornamental flowers commonly known as canna lilies.
Achira’s native distribution extends throughout the Neotropics from Mexico and the Caribbean through the Andes and southward to Brazil and Argentina at elevations between 1,000 and 2,900 m asl. The plant is currently grown through the world tropics and within a variety of environments, in areas with precipitation between 250–4,000 mm and elevations between sea level and 2,900 m asl. C. indica tolerates most types of soils with pH between 4.5 and 8, including heavy soils and tropical latosols. However, it grows better in loose, humid, well-drained and rich soils. The species is generally propagated vegetatively by farmers and stout rhizomes allow the plant to persist in the soil for several years. The domestication of achira likely occurred in the eastern Andes and disseminated quite early through the Peruvian coast (Gade, 1966; Pickersgill and Heiser, 1977). Archeological remains of achira have been recovered from sites in the Peruvian coast dating between 4,200 and 2,200 cal BP including various sites in the Casma valley and the Playa Hermosa Pampa Complex (Bird, 1948; Towle, 1961; Patterson, 1971; Ugent et al., 1984). Starch grains of Canna have been identified in grinding stones dated between 2,800–2,400 cal BP at Real Alto in Ecuador and between 4,100 and 3,200 cal BP at Antofagasta de la Sierra in Argentina (Chandler-Ezell et al., 2006; Babot, 2011). In Chile, the species has been recovered from archeological contexts dating back to the Late Archaic and Formative (Erices, 1975; Santoro, 1980; Muñoz, 1985; García et al., 2014; Agüero and Uribe, 2015; McRostie et al., 2019). In most publications referring to this cultigen archeologically as well as museum collections, the scientific name Canna edulis is used, however, a recent revision of the genus suggests this is a synonymy of C. indica (Maas-van de Kamer and Maas, 2008). Based on recent morphological and genetic revisions, C. indica forms a large complex comprising one subspecies and numerous domesticated and wild varieties widely distributed around the world (Maas-van de Kamer and Maas, 2008; IPNI, 2022).
Ahipa
The genus Pachyrhizus ex. DC from the Fabaceae family comprises five species, two wild (Pachyrhizus panamensis and Pachyrhizus ferrugineus) and three possibly domesticated species. Jicama (Pachyrhizus erosus) was likely domesticated in Mesoamerica, while jiquima or chuin (Pachyrhizus tuberosus) and ahipa (Pachyrhizus ahipa) were domesticated in the eastern Andes of South America (Zeven and Zhukovsky, 1975). The cultivated species are relatively small climbing herbs with long and tuberous roots that can reach up to 30 cm in length and weight up to a kilogram (Cárdenas, 1989). The roots of the cultivated species are round, white inside, have a succulent texture rich in starch, protein, and iron, and can be consumed raw, cooked or as flour (Santayana et al., 2014). Starch granules of Pachyrhizus exhibit an oval and bell-shape, with straight facets, and a closed centric hilum and lamellae as well as central fissures are occasionally present (Piperno, 2006b). Currently, ahipa is cultivated mainly on the eastern slopes of the Andes, between 1,500 and 3,000 m asl, on humid climates such as the eastern mesothermal valleys and on the edge of the rainforest (National Research Council, 1989; Ørting et al., 1996). There are two described varieties of P. ahipa, one characterized by erect plants with high tuber production in southern Bolivia and northern Argentina and another with tangled plants predominantly in northwestern Bolivia (Ørting et al., 1996). Jiquima (P. tuberosus), on the other hand, is currently cultivated in the tropical lowlands from Venezuela to Bolivia. Although historical records suggest that it was widely cultivated in Brazil, Paraguay, and as far north as French Guiana and several Caribbean islands, including Cuba and Puerto Rico (Sørensen et al., 1997). P. tuberosus grows in evergreen and semi-deciduous lowland and premontane forests (up to 1,900 m asl), in humid (total rainfall between 640 and 4,100 mm) and hot (21–27°C) areas (Sørensen et al., 1997).
Recent molecular studies show strong genetic differentiation among the domesticated species (P. ahipa, P. tuberosus, and P. erosus) in the Neotropics (Santayana et al., 2014; Delêtre et al., 2017). An analysis based on chloroplast DNA and nuclear microsatellite markers found a strong molecular differentiation between cultivated P. ahipa and P. tuberosus plants and wild P. tuberosus and P. panamensis plants, suggesting a common, albeit diverse, ancestral population (Delêtre et al., 2017). Additionally, P. ahipa and P. tuberosus seem to have a common origin and therefore potentially reflect independent domestication with P. ahipa suffering a loss of genetic diversity as it was distributed away from its putative center of origin. However, the relationship between all cultivated species, including P. ahipa, is still not entirely clear, and several studies have suggested the importance of carrying out a taxonomic revision of the group (Delêtre et al., 2013, 2017). It is believed that Pachyrhizus was widely cultivated across the central Andes in the past, and plant remains have been reported from the Casma valley of Peru spanning between 1,800 BC and 1,532 AD (Ugent et al., 1986).
Cassava
Cassava (Manihot esculenta Crantz) also known as manioc and yuca, is a perennial shrub with numerous starchy tuberous roots that belongs to the Euphorbiaceae family. It has sympodial branching of variable height and ranging from plants having few slender unbranched, and tall stems (up to 5 m tall) to shorter (1.5 m tall) plants with multiple branched stems. It has simple and glabrous leaves, covered by a waxy cuticle and with a palmate blade that present variable (3–9) number of lobes (Domínguez et al., 1983; Ceballos and de la Cruz, 2012). Racemes include both male and female flowers, which are monecious, apetalus, and present a primary bract and a bracteole. Male flowers contain a single reduced stamen and form inflorescences of ten single stamen flowers, known as cyanthia (Prenner and Rudall, 2007; Perera et al., 2013). Female flowers possess a reduced pistil and a trilocular ovary protected by petaloid bracts and contain a set of trilocular ovoid or globular capsules that contain three seeds with an ovoid-ellipsoidal shape. Both female and male flowers are produced on the same inflorescence, racemes, or panicles, on the same plant, but mature at different times to deter self-pollination. Cassava can outcross but is almost exclusively vegetatively propagated by means of stem cuttings in cultivation (Ramu et al., 2017). Starch grains of cassava have a bell-shape, with rounded facet edges, centric and open or closed hilum, and a y-, wing-shaped or stellate fissure (Piperno, 2006b). Oval-shaped granules, with 5–6 pressure facets and a single pressure facet at a distal section are less common (Pagán-Jiménez et al., 2016).
Cassava is one of the most important staple crops worldwide and is a major source of calories for millions of people living in tropical regions (Howeler et al., 2013). All parts of the plant—including the starchy roots—can be lethally toxic to humans because of high cyanogenic glucoside content. “Sweet” cassava has been selected to be non-toxic and can be eaten boiled or roasted as a vegetable. Most cultivated types are “bitter” cassava, retaining the toxicity and requiring processing by extensive rinsing or boiling, and processed into starch including tapioca. Leaves are occasionally consumed after extensive boiling to detoxify, especially in the northern Amazon region, and have a high protein content (18–22%) (Buitrago, 1990). In contrast to other tropical root crops, cassava tolerates droughts (<400 mm of average annual rainfall) and can grow on acidic and low-nutrient soils as well as in terrains with high phosphorous fixation, erosion, and high aluminum content (Alves and Setter, 2000; Howeler et al., 2013). Domesticated varieties tolerate a broad range of environmental conditions, and produce higher starch yields than wild varieties (Wang et al., 2014).
The species was domesticated from M. esculenta ssp. flabellifolia in the cerrado and the lowland rainforests of the Amazon basin (Allem, 1994, 1999; Olsen and Schaal, 1999, 2001). A more recent phylogenomic study of a large assemblage of cassava varieties confirmed its wild ancestry and identified numerous instances of hybridization with other wild Manihot species, but also suggests that because of the vegetatively propagated nature of its dispersion, a spatial structure to its diffusion may be challenging to map out (Simon et al., 2022). The timing of cassava domestication is uncertain, but cultivated varieties might have reached the Andean Pacific Coast and Mesoamerica by the early Middle Holocene (Isendahl, 2011). Archeologically, Manihot phytoliths are not narrowly diagnostic to the species level for cassava (Chandler-Ezell et al., 2006), but have been recovered from anthropogenic deposits in forest islands distributed in southwestern Amazonia, suggesting cassava cultivation may have started ca. 10,000 years ago (Lombardo et al., 2020). Findings of cassava starch grains and phytoliths have also been documented in a range of Neotropical sites in the Andes and northwestern South America and actual desiccated tubers in various sites in the Peruvian coast as early as the onset of the Late Holocene, ca. 4,000 years ago, suggesting that cassava was widely cultivated during the middle Holocene (Pearsall, 2008; Piperno, 2011).
Sweet potato
Sweet potato [Ipomoea batatas (L.) Lamarck] also known as camote and batata, is an herbaceous perennial vine from the Convolvulaceae family. It produces edible tuberous roots, which are elongated, starchy, sweet tasting and exhibit a diversity of shapes, colors, and textures, depending on the variety. Stems are slender and tend to crawl on the ground, forming adventitious roots that grow a few centimeters above the ground. The plant bears alternate rounded, reniform or cordate leaves, with a palmate venation and tend to twist along the stems (Austin, 1978). Leaves are green or purple depending on the accumulation of anthocyanins along the leaf veins. Cultivars vary in their ability to flower with some producing large amounts of flowers while others none at all. The inflorescence is a biparous cyme, bearing a single or several flowers with a short pedicel (Huamán, 1992). Flowers are bisexual, funnelform, 3–5 cm long, with white to pale violet petals. Fruits are spherical capsules that contain one to four irregular seeds. Starch granules are oval and bel-shaped, with angular and several pressure facets, hilum is centric and occasionally presents a vacuole. Some bell-shaped grains exhibit an eccentric hila and lamellae and sometimes a brownish tinge (Piperno, 2006a; Horrocks et al., 2008).
Sweet potato is a staple food crop in several countries in Africa, Asia, Oceania, and the Americas. Roots are an important source of carbohydrates, vitamins A and C, fiber, iron, potassium, and protein (Woolfe, 1992). The crop is native to the Neotropics, and initial molecular analyses suggested an autopolyploid origin of I. batatas from its putative wild ancestor I. trifida (Huang and Sun, 2000; Srisuwan et al., 2006; Roullier et al., 2013). More recent genetic work suggests the existence of two fairly distinct domesticated lineages possibly evolved in northern South America (Muñoz-Rodríguez et al., 2018). There are presently dozens of sweet potatoes varieties grown across the world and developed to various environmental and soil conditions that range from organically poor and sandy to well-drained silty and organically rich soils (Zeven and Zhukovsky, 1975). Remains of sweet potato tubers and starch grains have been documented in a wide range of archeological sites of the Peruvian coast as well as in the Caribbean Basin, Ecuador, and the northern coast of Chile (Towle, 1961; Santoro, 1980; Perry, 2002; Zarrillo, 2012; Pagán-Jiménez et al., 2015). More recent research relying on starch grains suggest that sweet potatoes disseminated quite early and possibly in Pre-Columbian times throughout the Americas (Iriarte et al., 2020). Pre-Columbian sweet potato dispersal included into Polynesia (Scaglion and Cordero, 2011), where it became an essential staple (Horrocks et al., 2004; Tromp and Dudgeon, 2015; Barber and Higham, 2021). Pre-European dispersal into Polynesia was likely based on strong linguistic patterns linking Polynesian and Andean words for sweet potato [reviewed in Clarke (2009)], the presence of native South American human ancestry in pre-contact human remains from Rapa Nui (Ioannidis et al., 2020), and more recently, the direct evidence of sweet potato carbonized remains from sites such as Rano Raraku in the island (Tilburg et al., 2022).
Pacay
Pacay (Inga feuillei DC), is an evergreen, medium-sized fruit tree (up to 15–30 m high) from the Fabaceae family. The species, which is cultivated for its sweet tasting fruits and wood, presents paripinnate compound leaves with 3–4 pairs of opposite, large, dark-green, pubescent, oval to broadly ellipsoid leaflets (Pennington, 1997; Lim, 2012). The rachis is winged between each pair of leaflets and poses a nectary glad at the base of each leaflet pair. The flowers are sessile, pentamerous, fragrant, and organized in axillary spikes. Each flower possesses a short, white calix with numerous stamens (75, up to 120), fused into a tube. Male flowers occur occasionally with the hermaphrodite flowers. The fruits are pendant, indehiscent pods, laterally flattened with sulcate margins, and reach a length of 30–50 cm. The seeds are covered by a white, sweet, and edible grainy pulp. Starch grains derived from seeds are oval and elliptical with a single longitudinal fissure (Piperno and Dillehay, 2008).
Pacay is cultivated for its fruits and wood through the lowlands and highland Andean valleys of Peru, Ecuador, and Bolivia up to 1,800 m asl (Lim, 2012). It has also been introduced to Central America, Southeast Asia, and Australia. It grows better in areas with a short dry season, a mean annual precipitation between 1,500 and 1,700 mm, and a mean annual temperature around 25°C, but it can tolerate short periods of high (up to 35°C) and freezing temperatures. The species grows mostly in soils with a pH of 4–8 and tolerates high concentrations of aluminum in acid soils. Pacay presents copious root nodules that contain mycorrhiza, helping to uptake phosphates from the soil. The history of domestication of pacay is not well documented, but archeological studies have evidenced that its early consumption (∼8,000 years ago) in the Peruvian northern coast (Piperno and Dillehay, 2008). Furthermore, it has been documented that this tree crop was being cultivated in the coast of Peru by ∼4,500 cal yr BP and its pods have been portrayed on ceramics (National Research Council, 1989; Shady Solis et al., 2001; Haas et al., 2004). Remains of Inga feuillei have been also recovered from Formative sites in the northern coast of Chile (Muñoz Ovalle, 2004). Specimens from southern Peru and northern Chile are sometimes identified as the ice-cream bean (Inga edulis), but as suggested by various scholars and given the morphology of the pods and seeds, it is more likely the species represented in the archeological record is I. feuillei (Piperno and Dillehay, 2008). Pacay fruit was commonly consumed by indigenous communities of southern Peru, western Bolivia (Conlee et al., 2009), and rarely in northern Chile (Núñez, 2006).
Materials and methods
To characterize the cultivation of the selected crops from Amazonian origin in the western valleys of the Atacama Desert in Pre-Columbian times, we established their spatiotemporal range by determining their ubiquity, distribution, and antiquity in archeological sites of the Atacama Desert of northern Chile. More specifically, to determine the geographic and chronological variation of the selected plant cultigens, we relied on a database of economically utilized plants found in archeological contexts in the Atacama Desert developed as a collaborative endeavor by various scholars from northern Chile (Ugalde et al., 2021). The database was compiled from published and unpublished reports from over 235 sites assembled by various researchers including part of our research team. From this initial list that included over 130 plant taxa, we focused on instances of five species that were likely domesticated in the Amazon basin and made their way to the highlands and coast for which we calculated the inter-site ubiquity as the percentage of presence within different periods. Many of these materials are currently curated in the Museo Arqueológico San Miguel de Azapa (MASMA) at Universidad de Tarapacá in Arica. Therefore, during a second phase of research, we focused on these records by further reviewing reports and collection inventories by refining the initial taxonomic identifications based on direct observations and photographs of diagnostic morphological attributes of each available specimen and their comparisons with reference collections of modern specimens collected from the Azapa valley and the Arica farmer’s market. To further refine our identifications and chronology by means of specialized archaeometric analyses, we collected 38 samples from specimens within ten sites expanding the widest spatiotemporal variability we could and limited to either underground storage organs or seeds. At the archeological context level, we collected samples opportunistically from replicates or specimens that had fragmented portions that we could utilize without compromising their integrity. We then proceeded to conduct direct starch grain identifications, carbon and nitrogen stable isotopes, and radiocarbon dating as described below.
The identification of plant remains was further corroborated by conducting a starch grain analysis. Desiccated archeological roots, rhizomes, and seeds were soaked in distilled water for 24 h and processed following methods described by Kovárník and Beneš (2018). Dry samples were mounted in a medium of 1:1 glycerin and water and observed under a microscope with cross polarized light (Leica DM750). Morphological characteristics were collected for each sample, including shape, position of hilum, faceting, and fissuring patterns. Photographs of starch grains were taken under 400× magnification and measured using ImageJ1. We measured maximum length and maximum width of starch grains that exhibited good preservation. Given that there was high variation of starch granules preservation among samples, the number of measured starch grains varied greatly per sample, from 1 to 19 (average: 7.6 starch grains/sample).
To further verify if these plants were cultivated locally or possibly imported by down the line exchange or by means of llama caravans as well as to estimate their importance for human diets, we conducted carbon and stable isotope analysis. Selected samples were processed using an acid-base-acid (ABA) decontamination pretreatment. Approximately 20 mg of each organic sample was submersed in a 1N HCl acid wash at 70°C for 20 min followed by successive 0.1 N NaOH base washes also at 70°C for 20 min at a time until the solution was clear, after which a final acid wash was applied followed by two washes of nanopure water to rinse and neutralize the resulting organic fraction. The diluted base was used because of the delicate nature of the samples. Liquid was then extracted, and the samples were left at 40°C until completely dried. Aliquots of the resulting fractions were then packed for spectrometric analyses. Approximately ∼2.5 mg of pretreated organic material was sampled to measure carbon and nitrogen stable isotopes at the Yale Analytical and Stable Isotope Center using a Costech ECS 4010 Elemental Analyzer with a Conflo III interface and a Thermo Delta Plus mass spectrometer along with controls and comparative standards to guarantee both high measurement accuracy and precision. Samples with particularly low nitrogen peaks during the spectrometric analysis were reanalyzed for corroboration. We present isotopic values as parts per mil (‰) in delta (δ) notation in relation to the Vienna Pee Dee Belemnite (VPDB) standard for δ13C and the atmospheric nitrogen (AIR) standard for δ15N.
To corroborate the temporal extent of their presence in the region, we conducted direct accelerated mass spectrometry (AMS) radiocarbon dating of the processed samples. Aliquots of ∼1.7 mg were combusted, graphitized, and subsequently dated at the Pennsylvania State University AMS Radiocarbon Dating Lab (PSUAMS). Results were corrected for mass fractionation and reported in conventional radiocarbon years before present (BP). We then calibrated the results using the southern hemisphere calibration curve SHCal20 (Hogg et al., 2020) in Oxcal 4.4 (Bronk Ramsey, 2021) and report the results in calendar years before the current era (BCE) or during the current era (CE) in 95.4% (2σ) ranges. We also used Oxcal 4.4 to estimate the start and end of the utilization of each studied cultigen using Bayesian modeling phase, sum, and combine functions.
Results
Ubiquity and cultural associations
In the following sections we provide a summary of our results, evaluating if and how these cultigens were locally cultivated. Ugalde et al. (2021), document that the five studied taxa (achira, cassava, ahipa, sweet potato, and pacay) are present in 26 archeological sites from the Atacama Desert albeit in relatively low proportions (Table 1). More specifically, the records of the studied cultigens begin during the Late Archaic Period (7,500–4,000 cal BP) when achira, cassava, and sweet potatoes appear in just two sites suggesting they were relatively rare during this timeframe. During the following Formative Period (4,000–1,700 cal BP), these three taxa plus pacay become more abundant reaching ten sites but only reach 7.3% ubiquity suggesting they were still uncommon. Throughout the subsequent Middle Horizon (1,700–1,000 cal BP) and Late Intermediate Period (1,000–600 cal BP) as ahipa becomes incorporated into the assemblage these species return to a rare status or even disappear temporarily. It is during the Late Period (600–470 cal BP) of Inka dominance and also known as the Late Horizon, when some of these taxa such as sweet potatoes acquire their greatest ubiquity, which nevertheless, is lower than 15%.
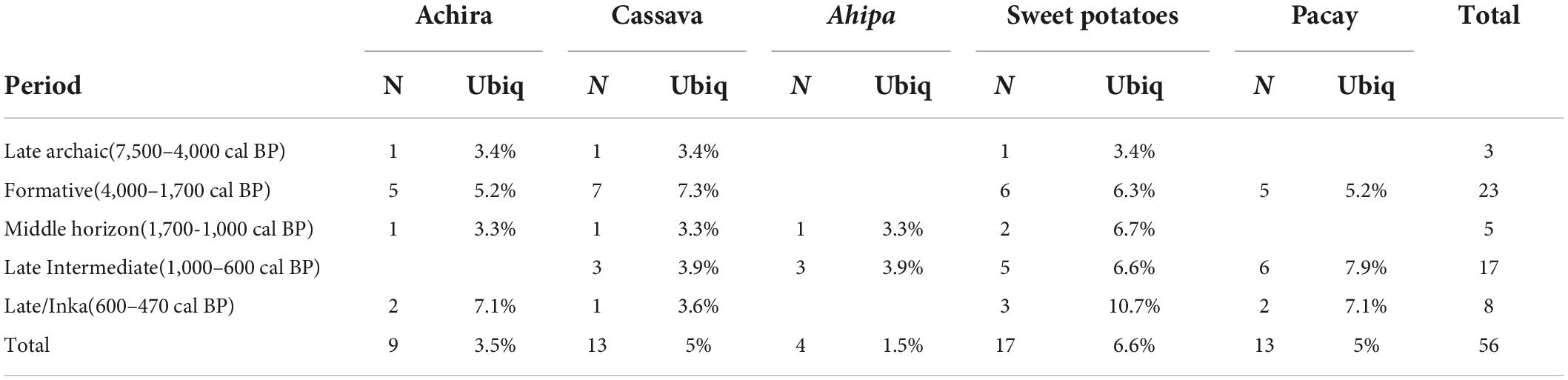
Table 1. Presence of crops in number of sites (N) from the Atacama Desert including ubiquity (Ubiq) represented as percentage of presence per chronological period based on Ugalde et al. (2021; Supplementary Table 1).
With very few exceptions such as the finding of cassava in Chaca 5 and El Vertedero Municipal de Antofagasta, most of the findings were made in sites within the Azapa valley and coast. A further look into the plant specimens themselves reveals additional insights.
Our research, focused on specimens held at the MASMA allowed us to further identify additional instances of these cultigens. This also allowed us to observe some of their physical attributes. The updated compilation incorporates revisions from previously documented sites with new records from both previously and recently studied archeological sites. As a result, combining information from the museum inventory, reports, and our personal revision of collections from this museum, we were able to document as many as 462 individual specimens from at least 15 sites located between the Azapa valley and its immediate coast (Figure 1).
Although we tried to be as thorough as possible in quantifying these findings, we recognize that our list is not exhaustive as we could not personally revise the assemblages from various other sites (Table 2). For instance, Muñoz Ovalle (1981) reports the number of structures including specific taxa at the Late Intermediate Period village Aldea Cerro Sombrero (Az-28), but it is to be determined how many individual specimens are present in each structure. Similarly, in various instances, only presence of certain taxa is reported and so we have opted to tally these as one instance per context documented. Moreover, in many sites not even basic plant identifications have been attempted. Indeed, because all of the studied sites have been relatively comparably studied, that some sites such as Az-6, Az-71, Az-28, and PLM-4 have more identified specimens, might not necessarily be the result of spatial or temporal research bias, but of differential preservation, recovery and identification. Having said this, we have tried to directly revise records from as many sites and contexts as we could. As a result, our counts show some discrepancies with a few existing reports and should be taken as a conservative estimate of how many specimens exist from the documented sites.
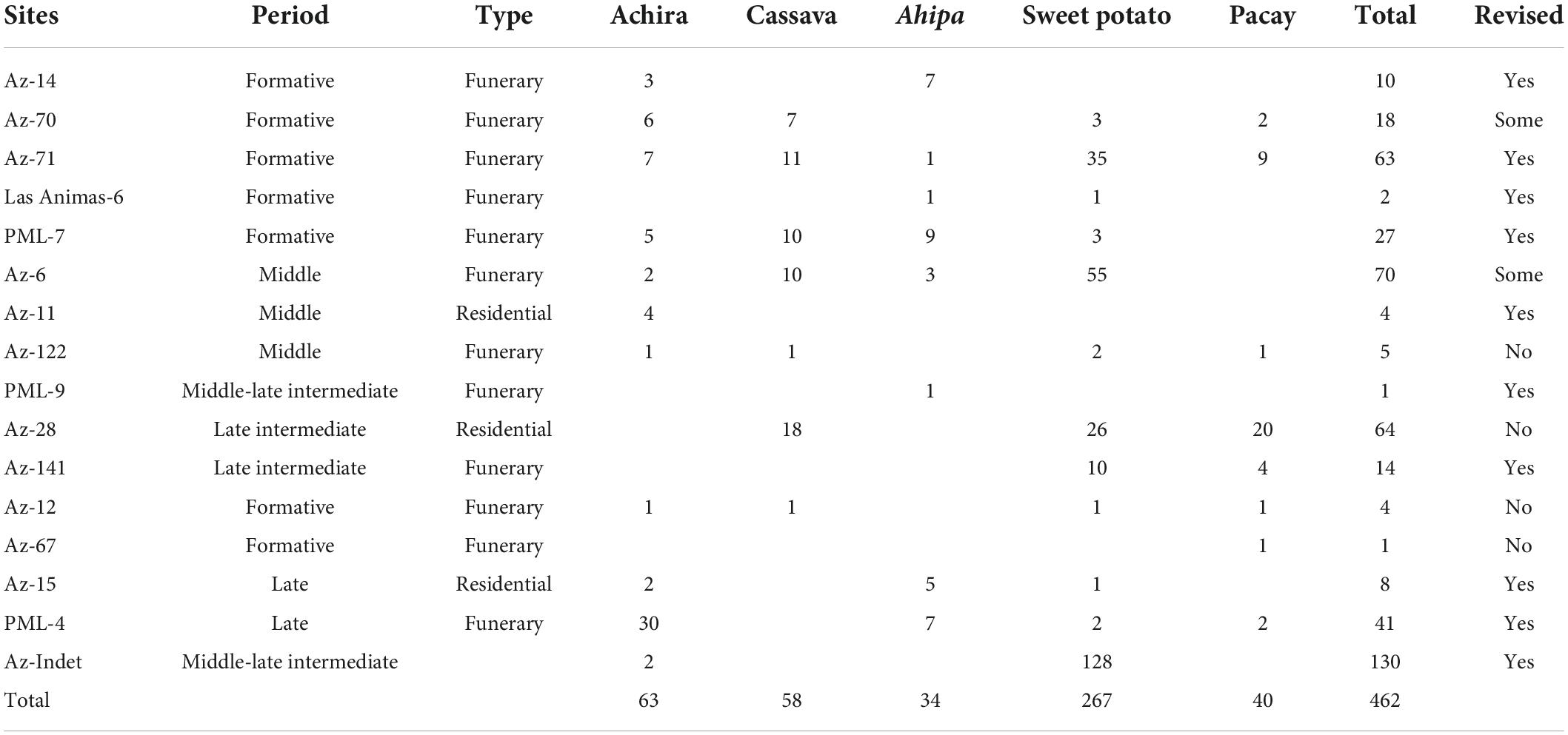
Table 2. Identified specimens in records from sites in the Azapa valley observed MASMA collections including counts of analyzed specimens.
Of the specimens we were able to observe directly, their provenience, associated cultural contexts and overall preservation were quite varied but generally, most were well preserved complete or semi-complete rhizomes, tubers, and seeds. Cultigens were found as either funerary offerings typically associated with vessels and other often complete artifacts within individual burials or as discarded food remains within middens and abandoned domestic structures in residential sites. Typically, specimens were in relatively good state of conservation and were often the result of hand collection and therefore, affected by preservation bias. In most cases, only one specimen was identified within a given burial or residential structure, but there were a few instances where several dozen specimens were also identified.
In the case of achira, most specimens were represented by rhizomes but in a few but significant instances, a few seeds caches were also observed (Figure 2). Rhizome specimens that included roots, stems and even leaves were observed (Figure 2B). These seeds are often perforated, slightly compressed in the middle and sometimes have strings passing through them suggesting they were used as beads (Figure 2A). Initially identified as seeds of mucuna (Mucuna sp.) or velvet bean, a legume native to the Chaco lowlands toward the southeast of the study region, morphological comparisons suggest these identifications (initially made half a century ago) are inaccurate. Given this finding, it is unclear if mucuna is actually present in Atacama Desert archeological sites. Interestingly enough, the small cavity that the achira seeds possess was used for facilitating their perforation and their use as beads has also been documented at other Atacama sites including Topater 1 in the Loa River (McRostie et al., 2019). Additionally, the finding of seed caches suggests the possibility achira was planted (and/or potentially imported) not just through asexual propagation but also by seed planting.
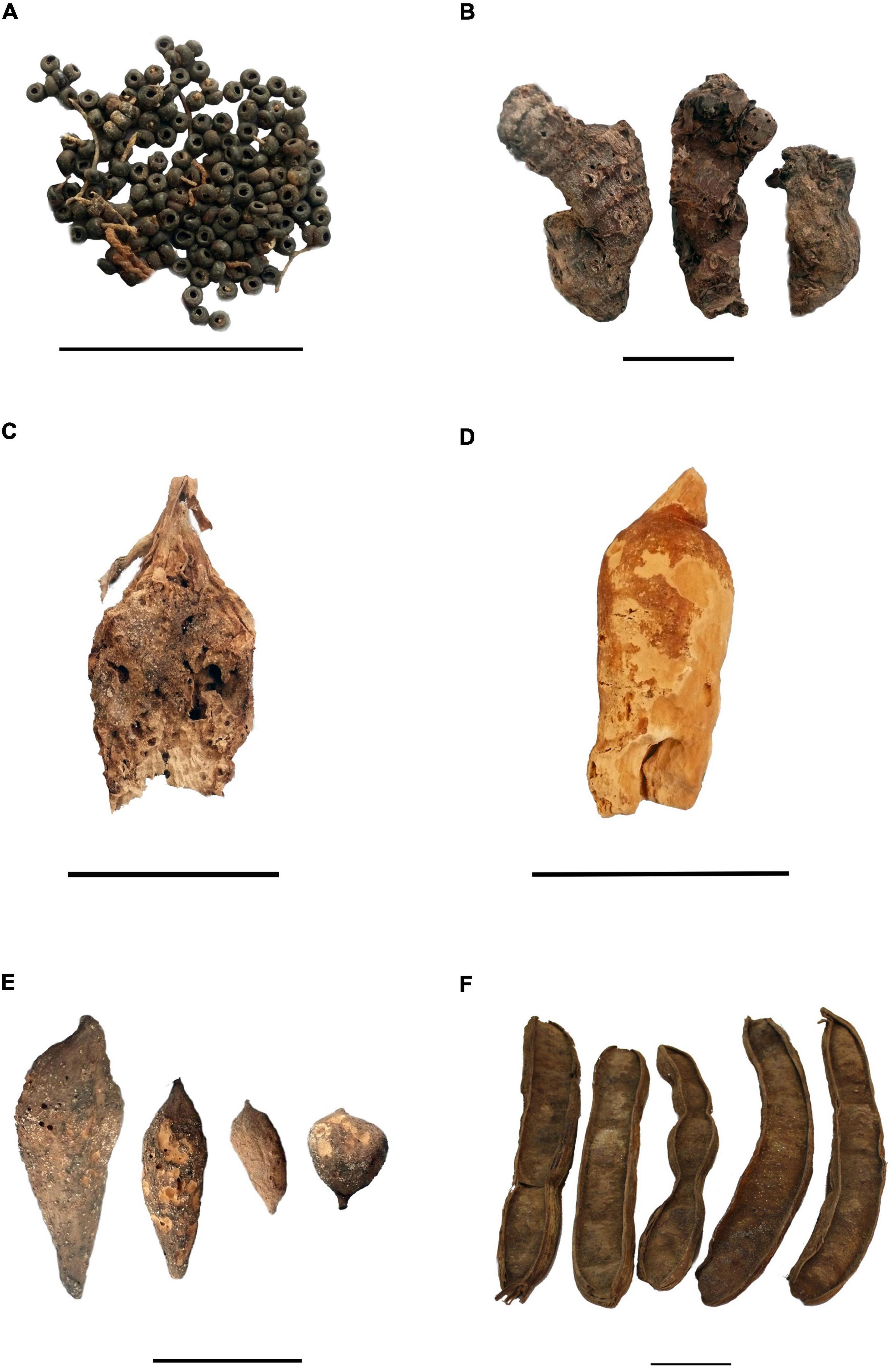
Figure 2. Archaeobotanical remains from the Atacama Desert, northern Chile: (A) Seeds and (B) rhizomes of achira (C. indica) from Az-14 (sample 27691), (C) tuberous root of ahipa (Pachyrhizus sp.) from Az-14 (sample 27690); (D) tuberous root of cassava (M. esculenta) from PLM-7 (sample 27501.1), (E) tuberous roots of sweet potato (I. batatas) from sample Az-Indet (sample 28), (F) pods of pacay (I. feuillei) from Az-71a (sample 5). Scale bars: 5 cm.
Regarding cassava, ahipa, and sweet potatoes, these were typically identified mostly as complete tubers and sometimes as fragmented storage underground organs (Figures 2C–E). In a few instances, coloring and fragmentation hints the plant was cooked prior to deposition, but in most cases, the specimens seem raw and often include attached roots and rootlets. Pacay were typically recorded as complete or fragmented pods, usually bundled in groups of more than one pod (Figure 2F). The morphology of these pods further suggests that the represented species is pacay (I. feuillei) and not the ice-cream bean (I. edulis) as sometimes this species is referred to in the archeological literature. Pacay branches and fragmented portions of roots have been recovered from the layers than structure Formative Period mounds in sites such as Az-12, Az-70, and Az-122 (Ledezma, 2014). It has been also reported for Caserones village in the Tarapacá basin in the core of the Atacama Desert (Núñez, 1982; Núñez and Briones, 2017, 2022). There were also a few contexts in which more than one of the studied species was documented, but we did not note a clear pattern of covariance.
Starch grain identification
Starch grains were recovered from 17 samples from a total of 24 processed samples (Supplementary Table 1). In all cases, starch grains verified morphological identifications at least to the genus level with the exception of two samples (Az-15/54.1 and Az-6/12497.1), which were too degraded to confirm the taxa identification based on morphological characters. We found starch grains characteristic of Canna in five samples as they are large (maximum length: 22.9 ± 4.3 μm, maximum width: 16.4 ± 4.5 μm), ovate with an eccentric hilum, and diffuse lamellae (Figure 3A). Five samples included starch grains with a combination of attributes characteristic of Pachyrhizus (Figure 3B). The starch grains are oval, relatively small (maximum length: 9.85 ± 2.3 μm, maximum width: 9.8 ± 2.5 μm), with 3–4 acutely angled pressure facets, closed hilum, and some of them exhibit central simple fissures. In seven samples, we recovered starch grains characteristic of Ipomoea (Figure 3C). The starch grains are 10 ± 4.3 μm long and 10.5 ± 5.1 μm wide. Furthermore, they exhibit an oval and sub-triangular shape with up to 5 pressure facets, a centric hilum and occasionally a visible vacuole. The identification of these samples as I. batatas matched mostly the identification of the samples based on morphological characters. It must be noted that starch grains from Ipomoea and Pachyrhizus show similar morphological characteristics, but Ipomoea grains are slightly larger and often feature a central vacuole.
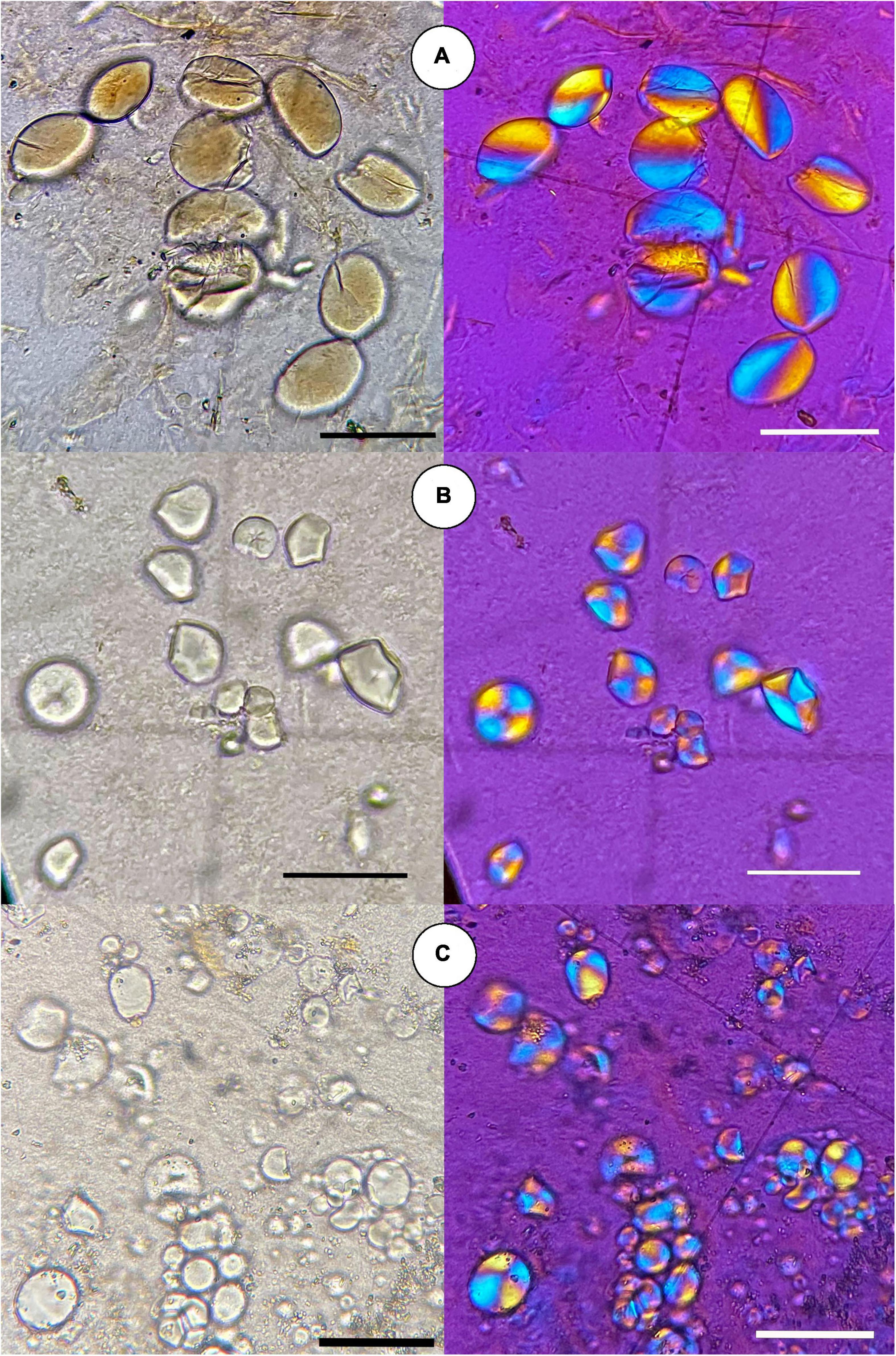
Figure 3. Starch grains recovered from ancient macrobotanical remains from the Atacama Desert, northern Chile (each starch image is shown in DIC and polarized light): (A) achira (C. indica) grain from rhizomes from Az-11 (sample 001); (B) ahipa (Pachyrhizus sp.) grain from tuberous roots from PLM-7 (sample 43501); (C) sweet potato (I. batatas) grain from tuberous roots from Az-71b (sample 27026). Scale bars: 10 μm.
Direct radiocarbon dating
The chronology of the sites where the studied cultigens were found ranges between the Late Archaic and the Late Periods, but many of these sites have multiple components as a result of continuous use over extended periods of time as well as reutilization after abandonment, making some chronological associations potentially unreliable. Therefore, we proceeded to directly radiocarbon date a diverse sample of desiccated specimens. In total, we conducted 37 new direct radiocarbon dates from ten sites, which we used to reconstruct the sequence of use of the studied plants in the Azapa valley (Table 3). These direct dates allow a preliminary assessment of the chronology of adoption and use of these crops, which hopefully will be enhanced and refined by future additional sampling.
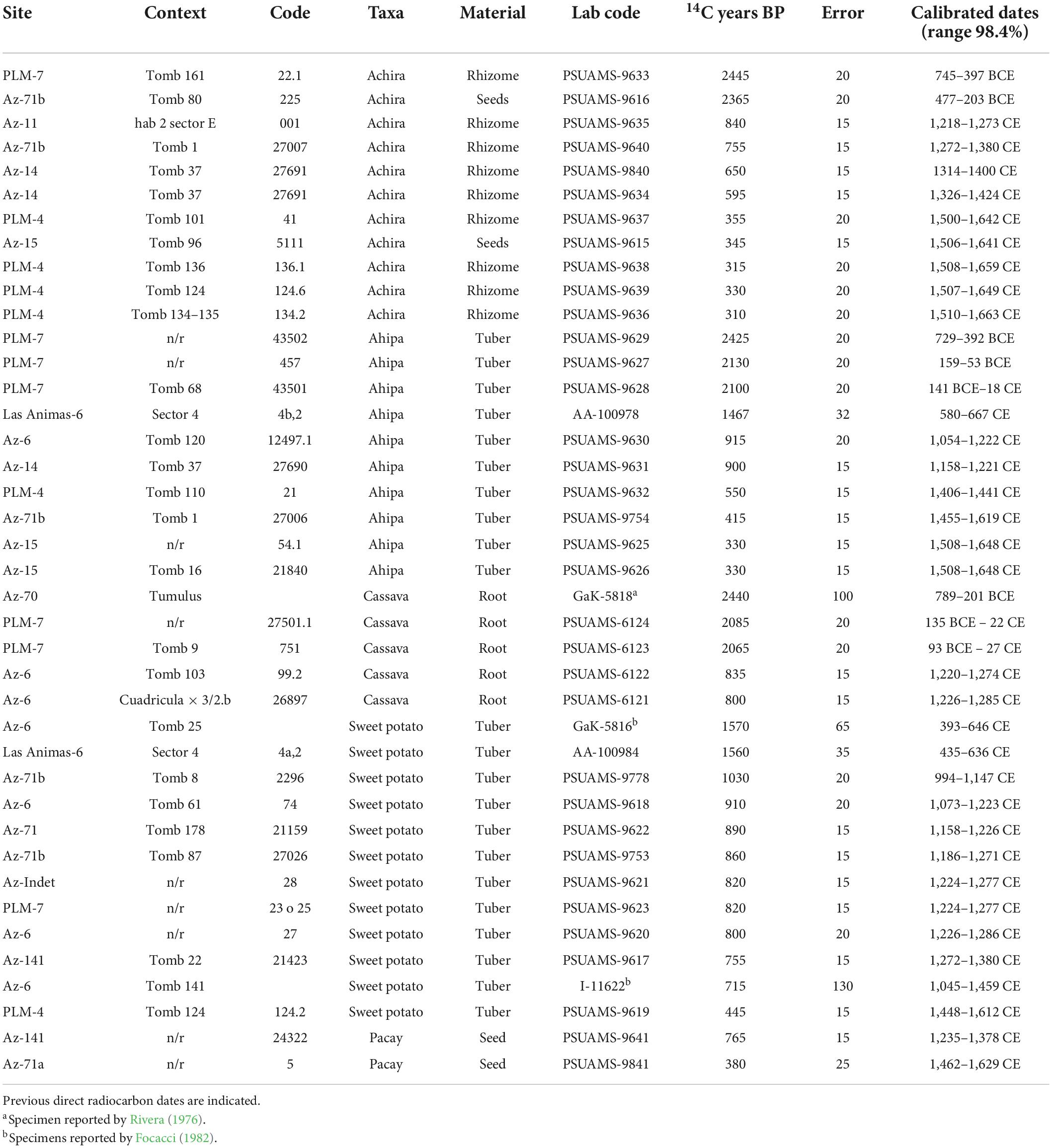
Table 3. Results of direct accelerated mass spectrometry radiocarbon dating including calibrations carried out using Oxcal 4.4 (Bronk Ramsey, 2021) and the southern hemisphere calibration curve (Hogg et al., 2020).
Eleven samples correspond to achira specimens from six sites and included both rhizome but also seed specimens. Two of these samples are replicates from the same specimen (Az-14/27691) and produced an otherwise convergent date supported by a chi-square test that did not find a statistically significant difference between them [χ2-Test: df = 1, T = 6.722 (5% 3.8)]. The oldest documented specimen dates to approximately 745–397 BCE and the most recent to 1,510–1,663 CE, but most likely dates to the beginning of the Spanish colonial period. A Bayesian model including all eleven dates and combining the replicates largely confirms these ranges albeit providing slightly larger potential temporal boundaries allowing for some uncertainty (Figure 4).
Ten samples of ahipa specimens from seven sites suggest the use of this cultigen dates between 729–392 BCE (based on a sample from PLM-7) and 1,508–1,648 CE, again likely converging toward the end of the pre-Hispanic period as verified by two different samples from the Az-15 site. A Bayesian model also supports this sequence with radiocarbon samples relatively evenly sampled throughout the sequence.
Four samples from two sites allow to date the use of cassava between 135–22 BCE and 1,226–1,285 CE. This sequence could be further extended deeper in time, considering the sample reported from Az-70 by Rivera (1976), whose recalibrated date resulted in 789–201 BCE. This sample, however, was dated with pre-AMS procedures.
Ten samples of sweet potatoes from six sites allow dating the use of this cultigen between 435–636 CE and 1,448–1,612 CE. In addition, two samples reported by Focacci (1982) from Az-6 and recalibrated provide further support for this temporal range. A Bayesian model incorporating all 12 direct sweet potato dates verifies this sequence. Interestingly, despite the important sample size, most of the samples, date between 1,100 and 1,300 CE, suggesting that sweet potatoes might have been adopted later than initially thought and/or that within these two centuries cultivating sweet potatoes was especially significant.
Finally, two pacay samples from two sites were directly radiocarbon dated between 1,235–1,378 CE and 1,462–1,629 CE, most likely dating toward the end of the pre-Hispanic Period.
Carbon and nitrogen stable isotopes
We run a total 29 specimens for carbon and nitrogen stable isotopes (Table 4). In addition, we reanalyzed eight samples because initial results showed low nitrogen peaks and percentages. Although samples with low nitrogen peaks produced relatively high C:N atomic ratios, we did not find significant differences between the resulting δ15N values at both the inter and intraspecific levels. Given the consistency of the data, we include all samples containing duplicates in the results presented below.
The δ13C results combining all samples show a relatively narrow range of values (between −21.8 and −28‰), verifying that as expected all specimens behave like C3 plants (Figure 5). This might not be surprising as with the exception of cassava, which has a crassulacean acid metabolism (CAM), all species have C3 photosynthetic pathways. In contrast, the δ15N results exhibit very high and continuous variability ranging between 1.2 and 31‰ and crosscutting some categorical (taxonomic, temporal, and contextual) diversity. For instance, achira and sweet potatoes, which included the largest sample sizes, also showed wide δ15N variability. In contrast, notwithstanding that most ahipa specimens had relatively low nitrogen values and most pacay samples had relatively high nitrogen values, both measured cassava specimens produced drastically different results. Similarly, although some of the specimens with the lowest δ15N values originate from Formative Period sites, the observed values do not seem to follow a temporal gradient nor directly correspond with site groupings. Therefore, it is possible that sample size could affect the observed range of variation. More importantly, given that we would expect all of these plants to have relatively low nitrogen values, that many of the analyzed specimens possessed relatively high δ15N values requires a specific explanation, which we discuss below.
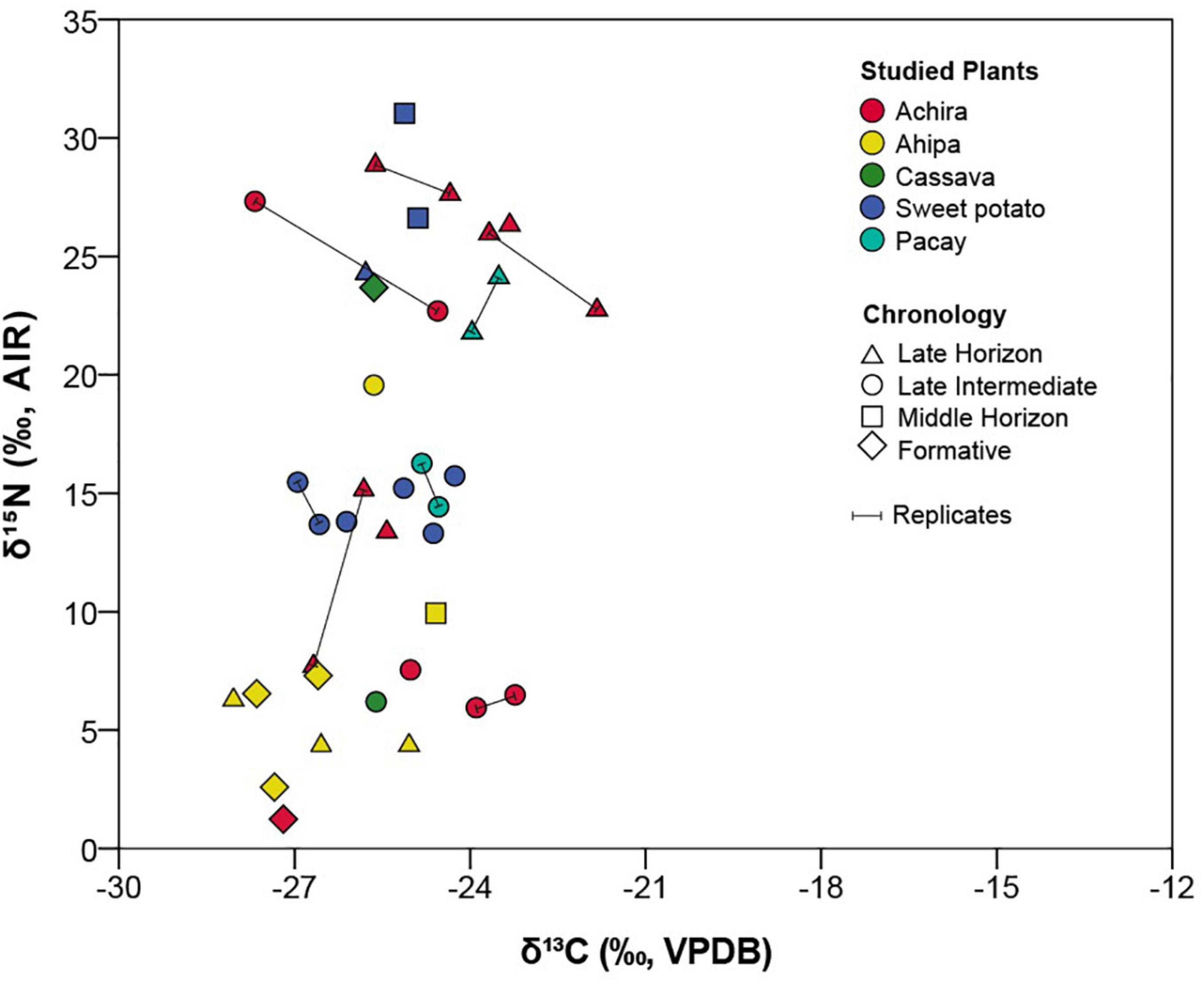
Figure 5. Bivariate plot including the carbon and nitrogen stable isotopes results organized by study plants and chronology.
Discussion
Cultivation of tropical crops in the Atacama Desert
The Atacama Desert of northern Chile is a unique region for the study of agricultural adoption processes. Here between the second and first millenium before the present era, societies primarily focused on the exploitation of marine resources from the highly productive Pacific coast began to adopt the cultivation of different complex of introduced domesticated plants throughout the Desert (García et al., 2020; Bessega et al., 2021; Ugalde et al., 2021). The evidence of these processes is constituted by uniquely preserved archaeobotanical materials including dried tissues of different anatomical parts such as tubers, roots, fruits, and others, thanks to prevailing hyper-arid conditions rarely present in other regions. This phytological material can be studied morphologically as well as through direct archaeometric techniques. Based on these analyses and a detailed review of recovered archaeobotanical specimens from archeological sites in northern Chile, it seems likely that the geophyte crops achira, cassava, sweet potatoes and ahipa, which likely followed a tuber pathway of domestication (Denham et al., 2020), were all introduced into the western Andean valleys of northern Chile nearly three millennia ago, and successfully cultivated there for the following centuries. Paleoecological evidence suggests that the initial adoption of agriculture was initially favored by wet climate conditions ca. 3,000–2,500 cal BP and correlated to population growth. However, as population became larger and climatic conditions dryer, it mobilized local populations to intensify and increase their reliance on other crops like algarrobo and maize.
The swift advent of these cultigens observed during the Formative Period in the Azapa valley mirrors what occurs with many other cultivated and wild plants when agriculture became a key subsistence strategy than in previous periods in the region and could be a consequence of increased dietary breadth and expanding agricultural populations. Although the results from the direct radiocarbon dating verifies that many of these cultigens were available during the first millenium BCE, many of the directly radiocarbon dated specimens are younger and correspond to periods when populations in the valley were at their highest levels (Gayo et al., 2015). The main function of these plants was to supply calories as carbohydrates stored in their rhizomes and tubers. A secondary but perhaps equally important role might have been tied to the capacity of these plants to store water and provide hydration, a limiting resource throughout the Atacama Desert, and therefore a particular palliative for travelers in this time of increasing population, mobility, and socioeconomic complexity.
The documented low abundance of these cultigens over time could be a result of relatively small volumes of planting possibly in rather constrained orchards or home gardens. While these plants do not require huge labor investment to plant, they do need some level of care to prevent them from spoiling. Given that these taxa are relatively easily to reproduce asexually, it is possible they were successfully propagated after an initial introduction of relatively minor populations. However, it is also plausible that additional crossbreeding with imported strains from the eastern Andes were incorporated into the region over time, particularly in relation to increased social and economic interaction during the Middle and Late Intermediate Periods (Núñez et al., 2005; Santoro et al., 2017).
At the inter-site level, the ubiquity of the studied cultigens is relatively low but consistent across time (Ugalde et al., 2021). Part of the reason why these crops, and particularly the geophytes, seem relatively rare is related to their taxonomic identifiability given that to accurately document them it is often necessary to recover complete or semi-complete well-preserved specimens and/or conduct specialized microscopic identification analysis, few of which have been conducted in the region. The database used to estimate the percentage of presence included sites not just from western valleys but also from the central depression and altiplano highlands, where not only these (originally tropical) crops were unlikely cultivated, but also where preservation conditions are substantially harsher particularly for uncharred secondary tissues including parenchyma from storage underground organs. If these crops became adapted to arid conditions, they could have been grown with the assistance of irrigation and other management techniques whereas if they were imported, we could expect to find some of these plants in intermediate nodes. In fact, imported goods from the eastern lowlands have been found in campsites both in highland rock shelters as well as llama caravan sites within the intermediate depression and nearby highlands (Briones et al., 2005; De Souza Herreros et al., 2017).
Regarding tree crops, during the first millenium CE, pacay seems to have been introduced, attractive for its sweet fruits. The introduction of pacay, however, was not as early, widespread, and successful as those of other leguminous trees such as those of the Prosopis genus, and particularly, the tamarugo (Prosopis tamarugo). Presently the tamarugo is such an iconic plant in the core of northern Chile Atacama Desert (Martínez, 1998), excluding the western valleys, that until recent archaeobotanical research verified its Pre-Columbian introduction, most scholars and local people assumed it was a native plant (McRostie et al., 2017). Similarly, algarrobo was introduced and widely dispersed in the interior of the Atacama Desert by the end of the first millenium AD, and chañar (Geoffraea decorticans) (McRostie et al., 2017), and P. lucuma (Ugalde et al., 2021) even later.
Of course, in addition to the plants that this study focuses on, other crops of Amazonian origin were also found in the same contexts, comprising peanuts, chili peppers, squashes, and coca. Future archaeometric and genomic research could share more light on the nature of their dispersion. For instance, it is still to be determined if there are specific patterns regarding the association of certain cultigens with specific kinds of individuals classified by gender, status, location, chronology, etc. Similarly, it is also to be explored if these co-varied with other specific cultigens, but incidental observations suggest significant variation in terms of burial composition over time. Indeed, our revision of the northern Chile collections reveals the existence of much more instances than that provided in the literature review. Similarly, for the Sihuas valley in southern Peru, Biwer et al. (2022) recently report remains of a long list of species from the Middle Horizon (600–1000 CE) site Quilcapampa, including achira and pacay seeds, which might have been locally grown. Valdez and Bettcher (2021) also report that in the Inka tambo of Acari in association with three earth ovens, tuber specimens of sweet potatoes were found as well as the remains of achira leaves. These ovens are similar to the ethnographically documented pachamanca cooking method practiced by contemporary local people of southern Peru who also use achira leaves to wrap and cap the oven, a practice that given the Acari evidence, could very likely originate in Pre-Columbian times.
Niche construction and the domestication of the desert
Plant, animal, and landscape domestication are complex and interrelated processes operating at multiple spatiotemporal scales. Because many of the studied plants had δ15N values much higher than those expected given their photosynthetic pathways, a specific biogeochemical transformation would explain this increase. Experimental research has demonstrated that fertilization using bird guano and camelid dung can significantly increase nitrogen isotopic values in plant tissues (Szpak et al., 2012). Fertilizing crops with marine bird guano is a well-documented practice in many Andean coastal valleys during late Pre-Columbian and Colonial times (Hidalgo et al., 2019) and significantly high δ15N values have been reported in human, camelids, maize, macaws, and other animal and plant remains in sites such as Azapa 8, Camarones 8, Pica 8, and many more spread out throughout the Atacama Desert (King et al., 2018; Alfonso-Durruty et al., 2019; Capriles et al., 2021; Santana-Sagredo et al., 2021). Therefore, our isotopic results seem to verify the hypothesis that food crops were not only cultivated locally but also fertilized using bird guano (Szpak, 2014; Santana-Sagredo et al., 2021). This implies that people were likely gardening these plants by supplying not only water through irrigation but other important nutrients to facilitate their growth.
Manuring using bird guano has been typically associated with Late Intermediate Period sites, but as our results show, this practice likely extended to the very origins of the adoption of agriculture in the Azapa valley. Nevertheless, the range of variation suggests that both fertilized and unfertilized cultivates coexisted in the valley for some time or even that some of the cultigens might have been imported from elsewhere (Díaz et al., 2019). In tandem, the ubiquity results suggest that over time, vegetatively propagated field crops became slightly less important and even extirpated altogether as other potentially more productive cultigens such as maize became more prevalent in the region (Vidal Elgueta et al., 2019).
The location of both the residential and funerary sites where these specimens were identified within the Azapa valley is also significant. The proliferation of sites and cemeteries in the Azapa valley beginning during the Formative Period is not just associated with fertile locations but also in relation to specific springs and wetlands (Muñoz, 1985; Ledezma, 2014; Muñoz et al., 2016). The total absence of rainfall should have been determinant for the introduction agriculture, which was initially organized under rather egalitarian social organization. Moreover, to enhance agricultural productivity an increasingly built infrastructure was manifested in irrigation networks. Other land social investments like interments with several individual offerings and landscape transformation through construction of extensive areas with ceremonial burial mounds, some of which are over 5 m high and 30–50 m in diameter, would have required significant labor coordination, but also signaled territorial ownership and control over key water resources and planting farming land with crops and trees (Romero et al., 2004). The burial mounds associated with the Formative Period, and which often incorporate layers of branches and wood from agricultural fields also feature plants themselves including edible portions of our study species within their conforming layers (Muñoz, 1985; Muñoz et al., 2016). It is possible that the building of these mounds was related to the processes of preparing soils. In this regard, we could hypothesize that the incorporation of the plants seems to have also featured soil development which was technically very different but not ontologically distinct from the development of dark earths in Amazonia, a hypothesis we hope to explore in future research.
Similarly, increasingly managed agricultural plots likely featured soil development through techniques such as leveling surfaces, clearing ground from rocks and other debris, and irrigation to encourage plant growth. In this regard we interpret the addition of marine bird guano (and potentially camelid dung) manure for fertilizer was likely carried out not just as an agricultural input but likely as a way to achieve lasting sustainability. This might have required scheduled fertilization along with other processes such as tilling, burning, plowing, weeding, planting multiple crops, and others that we still not fully understand. Therefore, agricultural fields were likely more similar to orchards and gardens than to single crop intensification plots such as those engineered for maize by the Inka in the neighboring Lluta valley during the Late Period. Presently, the Azapa and Lluta valleys complement more than contrast in terms of their agricultural potential with the former emphasizing olives and tomatoes and the latter maize and onions, and it is almost certain this structured dyad became structured Pre-Columbian times (Álvarez Miranda, 2014). This would explain the recurrent association of various plants as a complex (García and Uribe, 2012) including many geophyte crops and fruit trees such as our studied species.
The contexts where the studied cultigens were identified include domestic spaces and middens, but most originated from burials often as direct offerings within individual mortuary bundles. The association of these plants and burials could be related to the belief of facilitating food for the dead in their transit to the afterlife and this association has a complex dynamics in the Andes. That most of the studied plants also constitute storage underground organs provides an additional connection to the underworld as explored by various researchers particularly focused on the role of jiquima in southern Peru (Yacovleff, 1933). More importantly, the role of ancestors in facilitating fertility as social reproduction is well documented ethnographically (Girault, 1987; Arnold and Hastorf, 2008). For example, Harris (1983) emphasizes the importance of food, fertility, and agriculture in the mortuary rites practiced by the Laymi of highland Bolivia and especially their agricultural cycle which includes specific ceremonies for the dead connected to both planting and harvesting festivals. Analogous meaningful associations contributed to structuring the socio-environmental landscape of the Atacama Desert over the last millennia (Villagrán and Castro, 1997; Villagrán et al., 1998a,b, 1999).
Conclusion
In this study, we evaluated if the existing archeological evidence of a selection of four geophyte crops and one fruit tree that were likely domesticated in the South American tropics, east of the Andes, were cultivated in the western Andean valleys of northern Chile. Our initial hypothesis was that these taxonomically identified tropical cultigens were introduced into the western Andean valleys of the Atacama Desert along with other cultigens starting during the Formative Period if not earlier and peaking during the Inka expansion of the Andes. To refine the chronology of the appearance and use of these crops, we relied on direct radiocarbon dating of plant remains. To evaluate if these plants were farmed here, we used information about their ubiquity, starch grain analysis as well as carbon and nitrogen stable isotopes.
A general review of site collections and inventories suggests that all five study plants were cultivated in the region. Substantially more specimens were noted than those initially compiled from published reports and while their geographic and temporal range was significant, a strong focus was noted in the Azapa valley and the adjacent coast of Arica. The chronological and isotopic results further suggest that although the studied species were likely more ubiquitous than previously anticipated as a result of their successful adoption and planting in the studied region, they were never abundant. Nevertheless, it is likely that additional phytoliths, starch grains, and molecular studies of sediments and tools, will possibly increase the evidence of these cultigens and their frequency of consumption in the region.
High levels of nitrogen values suggest many of these crops were cultivated by means of manure fertilizing and that these practices originated in the Formative Period if not earlier. Unlike typical agricultural intensification strategies, we see the use of manuring as a diversification technique and, at the same time, a risk-reduction strategy, employed by early agriculturalists to enhance soil fertility in the long-term, which is effectively a form of niche construction. We envision manuring and other early agricultural activities, not just as inputs like in modern agriculture but more as a process of developing soils for the long term. While very different at the practical level, these processes were likely ontologically not very distinct than the long-term construction of Amazonian dark soils and the domestication of the Amazonian landscape. We can effectively see how introducing and caring for new cultigens involved various practices such as improving the soil, channeling water-flows, and protecting these from erosion, diseases, and other vulnerabilities. That these ideas are comparable to what is inferred in the Amazon is also likely not the result of cultural convergence but of the dissemination of the domesticated cultigens themselves, which was fundamentally a dynamic and highly sophisticated yet little understood socio-ecological process.
A wide range of domesticated plants were imported and transposed into valleys that drain in the Pacific Ocean of northern Chile during the Late Holocene, starting ca. 3,100 years ago. The ecological conditions where these plants evolved and were domesticated were sharply different than those prevailing in the western valleys, yet Pre-Columbian communities successfully transported and transplanted these crops. This could have been initially part of a symbolic system of consumption of the exotic (Foster, 1982), whereby food became a privileged arena for the production and management of identity/otherness (Aguilera Bornand, 2018). The evidence collected here plus a review of the chronology of findings of similar plant species in other archeological sites of northern Chile suggests that people intentionally incorporated many of these plants to their livelihoods that resulted in major transformation of the Atacama Desert ecosystems, which were otherwise depleted during the long drought phases that characterized the Middle Holocene. The abundance and relative ubiquity of these findings suggests that plants with edible underground storage organs such as ahipa, achira, sweet potatoes, and cassava as well as fruit trees such as pacay, were successfully grown in combination with increasingly complex soil management practices, and irrigation, all of which contributed to the environmental niches that were still in experimentation and construction by the AD 16th century, when the European invasion disrupted these trajectories but also triggered new ones.
In modern times, there are still a few pacay trees in the Azapa valley and canna lilies are planted on some gardens for ornamental purposes but cassava, sweet potatoes nor ahipa are cultivated at all. Indeed, as other Old World economically important plants such as grapes, olives, and onions became increasingly produced in the region, changes in agricultural practices gave pace to other cuisine and food consumption practices.
Data availability statement
The original contributions presented in this study are included in the article/Supplementary material, further inquiries can be directed to the corresponding author.
Author contributions
JC designed, conducted research, and wrote the manuscript with the help of all authors. MG conducted research including plant identifications and contributed to writing the manuscript. DV and AD conducted research and contributed to writing the manuscript. LK contributed to writing the manuscript. FR designed the research and contributed to writing the manuscript. CS designed, conducted research, and contributed to writing the manuscript.
Funding
This investigation was funded by the Chilean FONDECYT Projects # 1150031, 1151046, 1201687, and 1201786, FONDECYT Postdoctoral 3210151, ANID UPWELL Millennium Project NCN19_153, Universidad de Tarapacá, UTA Mayor 3758-22, the National Science Foundation BCS 2015924, and The Pennsylvania State University.
Acknowledgments
We would like to thank to our colleagues and particularly Virginia McRostie, Paula Ugalde, Victoria Castro, Eugenia Gayo, Francisca Santana-Sagredo, Bárbara Cases, Luis Briones, Tom Dillehay, Claudio Latorre, Maria Bruno, Christine Hastorf, and Jacqueline Correa for their support and productive discussions. The excellent personnel at MASMA at Universidad de Tarapacá in Arica also merits acknowledgment and especially Ivan Muñoz, Mariela Santos, Teresa Cañipa, Anita Flores, and Vivien Standen. We also thank the Chilean Consejo Nacional de Monumentos Nacionales for authorizing our research export permit (CMN ORD N° 4251, 29-October-2018). We would like to especially thank Karina Mancilla, Laurie Eccles, and Brendan Culleton for their help during analysis. Finally, we would like to thank Xinyi Liu and reviewers for helping to improve the content and clarity of our manuscript.
Conflict of interest
The authors declare that the research was conducted in the absence of any commercial or financial relationships that could be construed as a potential conflict of interest.
Publisher’s note
All claims expressed in this article are solely those of the authors and do not necessarily represent those of their affiliated organizations, or those of the publisher, the editors and the reviewers. Any product that may be evaluated in this article, or claim that may be made by its manufacturer, is not guaranteed or endorsed by the publisher.
Supplementary material
The Supplementary Material for this article can be found online at: https://www.frontiersin.org/articles/10.3389/fevo.2022.993630/full#supplementary-material
Supplementary Table 1 | Results of starch grain analysis including specimen provenience, measurements, and description of observed starch grains.
Footnotes
References
Agüero, C., and Uribe, M. (2015). “Tombs and tumuli on the coast and Pampa of Tarapacá: Explaining the formative period in Northern Chile (South-Central Andes),” in Funerary practices and models in the Ancient Andes: The return of the living dead, eds P. Eeckhout and L. S. Owens (Cambridge: Cambridge University Press), 55–68. doi: 10.1017/CBO9781107444928.006
Aguilera Bornand, I. M. (2018). Comida étnica, exotismo y otredad en Chile. El caso del restaurante “Peumayén Ancestral Food”. Chungara 50, 673–680. doi: 10.4067/S0717-73562018005001401
Alfonso-Durruty, M. P., Gayo, E. M., Standen, V., Castro, V., Latorre, C., Santoro, C. M., et al. (2019). Dietary diversity in the Atacama desert during the Late intermediate period of northern Chile. Quat. Sci. Rev. 214, 54–67. doi: 10.1016/j.quascirev.2019.04.022
Allaby, R. G., Stevens, C. J., Kistler, L., and Fuller, D. Q. (2022). “Genetic revelations of a new paradigm of plant domestication as a landscape level process,” in Plant breeding reviews, ed. I. Goldman (Oxford: John Wiley & Sons), 321–343.
Allem, A. C. (1994). The origin of Manihot esculenta Crantz (Euphorbiaceae). Genet. Resour. Crop Evol. 41, 133–150. doi: 10.1007/BF00051630
Allem, A. C. (1999). The closest wild relatives of cassava (Manihot esculenta Crantz). Euphytica 107, 123–133. doi: 10.1023/A:1026422229054
Álvarez Miranda, L. (2014). Etnopercepción andina: Valles dulces y valles salados en la vertiente occidental de los Andes. Dialogo Andino 44, 5–14. doi: 10.4067/S0719-26812014000200002
Alves, A. A. C., and Setter, T. L. (2000). Response of cassava to water deficit: Leaf area growth and abscisic acid. Crop Sci. 40, 131–137. doi: 10.2135/cropsci2000.401131x
Arnold, D. Y., and Hastorf, C. A. (2008). Heads of state: Icons, power, and politics in the ancient and modern Andes. Walnut Creek, CA: Left Coast Press.
Austin, D. F. (1978). The Ipomoea batatas complex-I. Taxonomy. Bull. Torrey Bot. Club 105, 114–129. doi: 10.2307/2484429
Babot, M. D. P. (2011). Cazadores-recolectores de los andes centro-sur y procesamiento vegetal: Una discusión desde la puna meridional argentina (ca. 7.000-3.200 años AP). Chungará 43, 413–432. doi: 10.4067/S0717-73562011000300006
Baitzel, S. I., and Rivera Infante, A. F. (2019). Presencia humana, patrones de asentamientos prehispánicos y complementariedad ecológica en las lomas del Valle de Sama, Tacna, Perú. Chungará 51, 381–402.
Barba, L., Muñoz, I., Ortiz, A., and Blancas, J. (2015). El uso de técnicas geofísicas para determinar sistemas constructivos y materiales presentes en los Túmulos del período Formativo en el Valle de Azapa, Arica, Chile. Chungara 47, 131–156. doi: 10.4067/S0717-73562015005000008
Barber, I. G., and Higham, T. F. G. (2021). Archaeological science meets Māori knowledge to model pre-Columbian sweet potato (Ipomoea batatas) dispersal to Polynesia’s southernmost habitable margins. PLoS One 16:e0247643. doi: 10.1371/journal.pone.0247643
Beresford-Jones, D., Pullen, A., Chauca, G., Cadwallader, L., García, M., Salvatierra, I., et al. (2018). Refining the Maritime Foundations of Andean civilization: How plant fiber technology drove social complexity during the Preceramic period. J. Archaeol. Method Theory 25, 393–425. doi: 10.1007/s10816-017-9341-3
Beresford-Jones, D., Pullen, A. G., Whaley, O. Q., Moat, J., Chauca, G., Cadwallader, L., et al. (2015). Re-evaluating the resource potential of lomas fog oasis environments for Preceramic hunter–gatherers under past ENSO modes on the south coast of Peru. Quat. Sci. Rev. 129, 196–215. doi: 10.1016/j.quascirev.2015.10.025
Beresford-Jones, D. G., Pomeroy, E., Alday, C., Benfer, R., Quilter, J., O’Connell, T. C., et al. (2021). Diet and lifestyle in the first villages of the Middle Preceramic: Insights from stable isotope and osteological analyses of human remains from Paloma, Chilca I, La Yerba III, and Morro I. Lat. Am. Antiq. 32, 741–759. doi: 10.1017/laq.2021.24
Bessega, C., Pometti, C., Fortunato, R., Greene, F., Santoro, C. M., and Mcrostie, V. (2021). Genetic studies of various Prosopis species (Leguminosae, Section Algarobia) co-occurring in oases of the Atacama desert (northern Chile). Ecol. Evol. 11, 2375–2390. doi: 10.1002/ece3.7212
Bird, J. B. (1948). Preceramic cultures in Chicama and Viru. Mem. Soc. Am. Archaeol. 4, 21–28. doi: 10.1017/S0081130000000320
Biwer, M. E., Álvarez, W. Y., Bautista, S. L., and Jennings, J. (2022). Hallucinogens, alcohol and shifting leadership strategies in the ancient Peruvian Andes. Antiquity 96, 1–17. doi: 10.15184/aqy.2021.177
Bogaard, A., Allaby, R., Arbuckle, B. S., Bendrey, R., Crowley, S., Cucchi, T., et al. (2021). Reconsidering domestication from a process archaeology perspective. World Archaeol. 53, 56–77. doi: 10.1080/00438243.2021.1954990
Briones, L., Núñez, L., and Standen, V. G. (2005). Geoglifos y tráfico prehispánico de caravanas de llamas en el desierto de atacama (norte de Chile). Chungara 37, 195–223. doi: 10.4067/S0717-73562005000200007
Bronk Ramsey, C. (2021). OxCal program, version 4.4.4. Oxford: Oxford Radiocarbon Accelerator Unit, University of Oxford.
Bruno, M. C., and Hastorf, C. A. (2016). “Gifts from the camelids: Archaeobotanical insights into camelid pastoralism through the study of dung,” in The archaeology of Andean pastoralism, eds J. M. Capriles and N. Tripcevich (Albuquerque, NM: University of New Mexico Press), 55–65.
Buitrago, J. A. (1990). La yuca en la alimentación animal. Cali, CO: Centro Internacional de Agricultura Tropical.
Capriles, J. M., Santoro, C. M., George, R. J., Flores Bedregal, E., Kennett, D. J., Kistler, L., et al. (2021). Pre-Columbian transregional captive rearing of Amazonian parrots in the Atacama desert. Proc. Natl. Acad. Sci. U.S.A. 118:e2020020118. doi: 10.1073/pnas.2020020118
Cárdenas, M. (1989). Manual de plantas económicas de Bolivia. Cochabamba: Editorial Los Amigos del Libro.
Carter, C. P. (2016). The economy of prehistoric Northern Chile: Case study Caleta Vitor. Ph.D. dissertation. Canberra, ACT: The Australian National University.
Ceballos, H., and de la Cruz, G. (2012). “Cassava taxonomy and morphology,” in Cassava in the third millennium: Modern production, processing, use, and marketing systems, eds B. Ospina Patiño and H. Ceballos (Cali: Centro Internacional de Agricultura Tropical), 15–28.
Chandler-Ezell, K., Pearsall, D. M., and Zeidler, J. A. (2006). Root and tuber phytoliths and starch grains document manioc (Manihot esculenta) arrowroot (Maranta arundinacea) and llerén (Calathea sp.) at the real alto site Ecuador. Econ. Bot. 60, 103–120. doi: 10.1663/0013-0001(2006)60[103:RATPAS]2.0.CO;2
Clarke, A. C. (2009). Origins and dispersal of the sweet potato and bottle gourd in oceania: Implications for prehistoric human mobility. Palmerston North: Massey University.
Clement, C. R., De Cristo-Araújo, M., Coppens D’Eeckenbrugge, G., Alves Pereira, A., and Picanço-Rodrigues, D. (2010). Origin and domestication of native Amazonian crops. Diversity 2, 72–106. doi: 10.3390/d2010072
Clement, C. R., Denevan, W. M., Heckenberger, M. J., Junqueira, A. B., Neves, E. G., Teixeira, W. G., et al. (2015). The domestication of Amazonia before European conquest. Proc. R. Soc. B Biol. Sci. 282:20150813. doi: 10.1098/rspb.2015.0813
Conlee, C. A., Buzon, M. R., Gutierrez, A. N., Simonetti, A., and Creaser, R. A. (2009). Identifying foreigners versus locals in a burial population from Nasca, Peru: An investigation using strontium isotope analysis. J. Archaeol. Sci. 36, 2755–2764. doi: 10.1016/j.jas.2009.08.016
De Souza Herreros, P., Méndez-Quiros Aranda, P., Catalán Contreras, D. G., Carrasco González, C. A., and Baeza De La Fuente, V. E. (2017). Aleros ceremoniales del período Formativo en las tierras altas del Desierto de Atacama (región de Tarapacá, norte de Chile). Ñawpa Pacha 37, 63–86. doi: 10.1080/00776297.2017.1333280
Delêtre, M., Soengas, B., Utge, J., Lambourdière, J., and Sørensen, M. (2013). Microsatellite markers for the yam bean Pachyrhizus (Fabaceae). Appl. Plant Sci. 1:1200551. doi: 10.3732/apps.1200551
Delêtre, M., Soengas, B., Vidaurre, P. J., Meneses, R. I., Delgado Vásquez, O., Oré Balbín, I., et al. (2017). Ecotypic differentiation under farmers’ selection: Molecular insights into the domestication of Pachyrhizus Rich. ex DC. (Fabaceae) in the Peruvian Andes. Evol. Appl. 10, 498–513. doi: 10.1111/eva.12472
Delin, W., and Kress, W. J. (2000). “Cannaceae,” in Flora of China, eds Z. Y. Wu, P. H. Raven, and D. Y. Hong (St. Louis, MO: Missouri Botanical Garden), 378.
Denham, T., Barton, H., Castillo, C., Crowther, A., Dotte-Sarout, E., Florin, S. A., et al. (2020). The domestication syndrome in vegetatively propagated field crops. Ann. Bot. 125, 581–597. doi: 10.1093/aob/mcz212
Díaz, F. P., Latorre, C., Carrasco-Puga, G., Wood, J. R., Wilmshurst, J. M., Soto, D. C., et al. (2019). Multiscale climate change impacts on plant diversity in the Atacama desert. Glob. Change Biol. 25, 1733–1745. doi: 10.1111/gcb.14583
Dillehay, T. D. (2014). From foraging to farming in the Andes: New perspectives on food production and social organization. Cambridge: Cambridge University Press.
Domínguez, C. E., Ceballos, L. F., and Fuentes, C. (1983). “Morfología de la planta de yuca,” in Yuca: Investigación, producción y utilización, ed. C. E. Domínguez (Cali: United Nations Development Programme), 29–49.
Erices, S. (1975). Evidencias de vegetales en tres cementerios prehispánicos, Arica-Chile. Chungara 5, 65–71.
Flores, H. E., Walker, T. S., Guimarães, R. L., Pal Bais, H., and Vivanco, J. M. (2003). Andean root and tuber crops: Underground rainbows. HortScience 38, 161–167. doi: 10.21273/HORTSCI.38.2.161
Focacci, G. (1982). Nuevos fechados para la época del Tiahuanaco en la arqueología del norte de Chile. Chungara 8, 63–77.
Focacci, G., and Erices, S. (1973). “Excavaciones en túmulos de San Miguel de Azapa,” in Actas del IV Congreso de Arqueología Chilena (Santiago: Universidad de Chile), 47–62.
Foster, S. W. (1982). The exotic as a symbolic system. Dialect. Anthropol. 7, 21–30. doi: 10.1007/BF00247064
Gade, D. W. (1966). Achira, the edible canna, its cultivation and use in the Peruvian Andes. Econ. Bot. 20, 407–415. doi: 10.1007/BF02904063
García, M., Santoro, C. M., Mcrostie, V., Mendez-Quiros, P., Salas-Egaña, C., Carter, C., et al. (2020). Pre-European plant consumption and cultural changes in the Coastal Lluta Valley, Atacama desert, Northern Chile (Ca. 5140–390 Cal Yr BP). Econ. Bot. 74, 445–463. doi: 10.1007/s12231-020-09513-0
García, M., and Uribe, M. (2012). Contextos de uso de las plantas vinculadas al Complejo Pica Tarapacá, Andes Centro-Sur: Arqueobotánica y agricultura en el período Intermedio Tardío (ca. 1250-1450 DC). Estud. Atacameños 44, 107–122. doi: 10.4067/S0718-10432012000200006
García, M., and Vidal, A. (2010). “Distribución y contexto de uso de las plantas arqueológicas del asentamiento Camiña-1 (1.250–1.450 años DC), región de Tarapacá,” in Actas del XVII Congreso Nacional de Arqueología Chilena (Valdivia: Ediciones Kultrún, Sociedad Chilena de Arqueología, Universidad Austral de Chile), 1225–1236.
García, M., Vidal, A., Mandakovic, V., Maldonado, A., Peña, M. P., and Belmonte, E. (2014). Alimentos, tecnologías vegetales y paleoambiente en las aldeas formativas de la pampa del Tamarugal, Tarapacá (ca. 900 aC-800 dC). Estud. Atacameños 47, 33–58. doi: 10.4067/S0718-10432014000100004
Gayo, E. M., Latorre, C., and Santoro, C. M. (2015). Timing of occupation and regional settlement patterns revealed by time-series analyses of an archaeological radiocarbon database for the South-Central Andes (16°–25°S). Quat. Inter. 356, 4–14. doi: 10.1016/j.quaint.2014.09.076
Gayo, E. M., Martens, T., Stuart-Williams, H., Fenner, J., Santoro, C. M., Carter, C., et al. (2020). Procurement of camelid fiber in the hyperarid Atacama desert coast: Insights from stable isotopes. Quat. Int. 548, 71–83. doi: 10.1016/j.quaint.2019.12.008
González Vallejos, F., Riquelme Garcés, A., Contreras Luque, P., and Mazuela, P. (2013). Antecedentes generales para la sustentabilidad de la producción hortícola en el valle de Azapa, Arica, Chile. Idesia 31, 119–123. doi: 10.4067/S0718-34292013000400016
Haas, J., Creamer, W., and Ruiz, A. (2004). Dating the late Archaic occupation of the Norte Chico region in Peru. Nature 432, 1020–1023. doi: 10.1038/nature03146
Hastorf, C. A. (1999). “Cultural implications of crop introductions in Andean prehistory,” in The emergence of agriculture: Appetites for change, eds C. Gosden and J. G. Hather (London: Routledge), 106–133. doi: 10.4324/9781003060765-6
Hidalgo, J., Cisternas, P., and Aguilar, J. (2019). Cambios políticos, sociales y económicos en relación a la pesca y extracción del guano en la costa de Arica y Tarapacá: Siglos XVI a inicios del XIX. Estud. Atacameños 61, 275–298. doi: 10.4067/S0718-10432019005000502
Hogg, A. G., Heaton, T. J., Hua, Q., Palmer, J. G., Turney, C. S. M., Southon, J., et al. (2020). SHCal20 Southern Hemisphere calibration, 0–55,000 years cal BP. Radiocarbon 62, 759–778. doi: 10.1017/RDC.2020.59
Horrocks, M., Irwin, G., Jones, M., and Sutton, D. (2004). Starch grains and xylem cells of sweet potato (Ipomoea batatas) and bracken (Pteridium esculentum) in archaeological deposits from northern New Zealand. J. Archaeol. Sci. 31, 251–258. doi: 10.1016/S0305-4403(03)00018-9
Horrocks, M., Smith, I. W. G., Nichol, S. L., Shane, P. A., and Jackman, G. (2008). Field survey, sedimentology and plant microfossil analysis of sediment cores from possible cultivation sites at Tolaga Bay, eastern North Island, New Zealand. J. R. Soc. N. Z. 38, 131–147. doi: 10.1080/03014220809510551
Horta Tricallotis, H. (2010). El Señorío Arica y los Reinos Altiplánicos: Complementariedad ecológica y multietnicidad durante los siglos pre-conquista en el Norte de Chile (1000-1540 d.C.). Ph.D. thesis. Santiago: Universidad de Chile.
Howeler, R., Lutaladio, N., and Thomas, G. (2013). Save and grow: Cassava. A guide to sustainable production intensification. Rome: Food and Agriculture Organization.
Huamán, Z. (1992). Botánica sistemática y morfología de la planta de batata o camote. Lima: International Potato Center.
Huang, J. C., and Sun, M. (2000). Genetic diversity and relationships of sweetpotato and its wild relatives in Ipomoea series Batatas (Convolvulaceae) as revealed by inter-simple sequence repeat (ISSR) and restriction analysis of chloroplast DNA. Theor. Appl. Genet. 100, 1050–1060. doi: 10.1007/s001220051386
Ioannidis, A. G., Blanco-Portillo, J., Sandoval, K., Hagelberg, E., Miquel-Poblete, J. F., Moreno-Mayar, J. V., et al. (2020). Native American gene flow into Polynesia predating Easter Island settlement. Nature 583, 572–577. doi: 10.1038/s41586-020-2487-2
Iriarte, J. (2009). Narrowing the gap: Exploring the diversity of early food-production economies in the Americas. Curr. Anthropol. 50, 677–680. doi: 10.1086/605493
Iriarte, J., Elliott, S., Maezumi, S. Y., Alves, D., Gonda, R., Robinson, M., et al. (2020). The origins of Amazonian landscapes: Plant cultivation, domestication and the spread of food production in tropical South America. Quat. Sci. Rev. 248:106582. doi: 10.1016/j.quascirev.2020.106582
Isendahl, C. (2011). The domestication and early spread of manioc (Manihot esculenta Crantz): A brief synthesis. Lat. Am. Antiq. 22, 452–468. doi: 10.7183/1045-6635.22.4.452
King, C. L., Arriaza, B. T., Standen, V. G., Millard, A. R., Gröcke, D. R., Muñoz, I., et al. (2018). Estudio isotópico del consumo de recursos marítimos y terrestres en la prehistoria del desierto de Atacama. Chungara 50, 369–396. doi: 10.4067/S0717-73562018005000802
Kistler, L., Maezumi, S. Y., Gregorio De Souza, J., Przelomska, N. A., Malaquias Costa, F., Smith, O., et al. (2018). Multiproxy evidence highlights a complex evolutionary legacy of maize in South America. Science 362, 1309–1313. doi: 10.1126/science.aav0207
Kovárník, J., and Beneš, J. (2018). Microscopic analysis of starch grains and its applications in the archaeology of the Stone Age. Interdiscip. Archaeol. 9, 83–93. doi: 10.24916/iansa.2018.1.6
Lane, K. (2006). Through the looking glass: Re-assessing the role of agro-pastoralism in the north-central Andean highlands. World Archaeol. 38, 493–510. doi: 10.1080/00438240600813806
Langlie, B. S. (2021). “Origins of food production in the high Andes,” in Oxford research encyclopedia of anthropology, ed. M. S. Aldenderfer (Oxford: Oxford University Press). doi: 10.1093/acrefore/9780190854584.013.442
Larson, G., Piperno, D. R., Allaby, R. G., Purugganan, M. D., Andersson, L., Arroyo-Kalin, M., et al. (2014). Current perspectives and the future of domestication studies. Proc. Natl. Acad. Sci. U.S.A. 111, 6139–6146. doi: 10.1073/pnas.1323964111
Ledezma, M. (2014). “Área de captación de recursos vegetales para la construcción del túmulo 1-sitio Az-67, valle de Azapa, Arica-Chile,” in Mil Años de Historia de los Constructores de Túmulos de los Valles Desérticos de Arica: Paisaje, Monumentos y Memoria, eds I. Muñoz Ovalle and M. S. Fernández Murillo (Arica: Ediciones Universidad de Tarapacá), 213–226.
Lim, T. K. (2012). “Inga edulis,” in Edible medicinal and non-medicinal plants: Volume 2, fruits, ed. T. K. Lim (Dordrecht: Springer Netherlands), 715–719. doi: 10.1007/978-94-007-1764-0_80
Lombardo, U., Iriarte, J., Hilbert, L., Ruiz-Pérez, J., Capriles, J. M., and Veit, H. (2020). Early Holocene crop cultivation and landscape modification in Amazonia. Nature 581, 190–193. doi: 10.1038/s41586-020-2162-7
Maas-van de Kamer, H., and Maas, P. J. M. (2008). The Cannaceae of the world. Blumea Biodivers. Evol. Biogeogr. Plants 53, 247–318. doi: 10.3767/000651908X607945
Martínez, J. L. (1998). Pueblos del Chañar y el Algarrobo, los Atacama en el siglo XVI. Santiago: Universidad de Chile.
McRostie, V., Gallardo, F., Vidal, A., and Croxatto, S. (2019). Evidencias arqueobotánicas tempranas en el Oasis de Calama, el cementerio Topater 1 (Formativo medio, 500 a. C.-100 d. C., Desierto de Atacama, norte de Chile). Darwiniana 7, 16–38. doi: 10.14522/darwiniana.2019.71.824
McRostie, V. B., Gayo, E. M., Santoro, C. M., De Pol-Holz, R., and Latorre, C. (2017). The pre-Columbian introduction and dispersal of Algarrobo (Prosopis, Section Algarobia) in the Atacama desert of northern Chile. PLoS One 12:e0181759. doi: 10.1371/journal.pone.0181759
Miller, M. J., Kendall, I., Capriles, J. M., Bruno, M. C., Evershed, R. P., and Hastorf, C. A. (2021). Quinoa, potatoes, and llamas fueled emergent social complexity in the Lake Titicaca Basin of the Andes. Proc. Natl. Acad. Sci. U.S.A. 118:e2113395118. doi: 10.1073/pnas.2113395118
Muñoz, I. (1985). Tempranos cultivos de plantas en poblaciones prehispánicas del valle de Azapa (Arica, Chile). Idesia 9, 57–62.
Muñoz, I., Agüero, C., and Valenzuela, D. (2016). “Poblaciones prehispánicas de los valles occidentales del norte de Chile: Desde el período Formativo al Intermedio Tardío (ca. 1.000 a.c. – 1.400 d.C.),” in Prehistoria en Chile desde sus Primeros Habitantes hasta los Incas, eds F. Falabella, L. Sanhueza, C. Aldunate, and J. Hidalgo (Santiago: Editorial Universitaria, Sociedad Chilena de Arqueología), 181–237.
Muñoz Ovalle, I. (1981). La aldea de cerro sombrero en el periodo del desarrollo regional de Arica. Chungara 7, 105–141.
Muñoz Ovalle, I. (2004). El período Formativo en los valles del norte de Chile y sur de Perú: Nuevas evidencias y comentarios. Chungara 36, 213–225. doi: 10.4067/S0717-73562004000300024
Muñoz Ovalle, I. (2012). Agua y monumentalidad en el valle de Azapa: Indicadores del uso del espacio en las poblaciones Alto Ramírez, Periodo Formativo, Norte de Chile. Chungará 44, 571–591. doi: 10.4067/S0717-73562012000400003
Muñoz Ovalle, I. (2017). Espacios fúnebres, prácticas mortuorias y cronología en el cementerio Azapa-115: Aproximaciones en torno a la estructura social de los agricultores prehispánicos del período medio. Estud. Atacameños 54, 5–36. doi: 10.4067/S0718-10432016005000027
Muñoz-Rodríguez, P., Carruthers, T., Wood, J. R. I., Williams, B. R. M., Weitemier, K., Kronmiller, B., et al. (2018). Reconciling conflicting phylogenies in the origin of sweet potato and dispersal to Polynesia. Curr. Biol. 28, 1246–1256.e12. doi: 10.1016/j.cub.2018.03.020
National Research Council (1989). Lost crops of the Incas: Little-known plants of the Andes with promise for worldwide cultivation. Washington, DC: National Academies Press.
Núñez, L. (1982). Temprana emergencia de sedentarismo en el desierto chileno: Proyecto Caserones. Chungara 9, 80–122.
Núñez, L. (2006). Asentamientos formativos complejos en el centro-sur andino: Cuando la periferia se constituye en núcleo. Bol. Arqueol. PUCP 10, 321–356.
Núñez, L., and Briones, L. (2017). Tráfico e interacción entre el oasis de Pica y la costa arreica en el desierto tarapaqueño (norte de Chile). Estud. Atacameños 56, 133–161. doi: 10.4067/S0718-10432017000300006
Núñez, L., and Briones, L. (2022). “Intersite locations of prehistoric caravan traffic in the core of the Atacama desert, northern Chile,” in Caravans in socio-cultural perspective: Past and present, eds P. B. Clarkson and C. M. Santoro (London: Routledge), 212–235. doi: 10.4324/9781003179276-13
Núñez, L., Grosjean, M., and Cartajena, I. (2005). Ocupaciones humanas y paleoambientes en la Puna de Atacama. San Pedro de Atacama: Universidad Católica del Norte, Taraxacum.
Núñez, L., and Santoro, C. M. (2011). El tránsito Arcaico-Formativo en la circumpuna y valles occidentales del centro sur andino: Hacia los cambios “Neolíticos”. Chungara 43, 487–530. doi: 10.4067/S0717-73562011000300010
Olsen, K. M., and Schaal, B. A. (1999). Evidence on the origin of cassava: Phylogeography of Manihot esculenta. Proc. Natl. Acad. Sci. U.S.A. 96, 5586–5591. doi: 10.1073/pnas.96.10.5586
Olsen, K. M., and Schaal, B. A. (2001). Microsatellite variation in cassava (Manihot esculenta, Euphorbiaceae) and its wild relatives: Further evidence for a southern Amazonian origin of domestication. Am. J. Bot. 88, 131–142. doi: 10.2307/2657133
Ørting, B., Grüneberg, W. J., and Sørensen, M. (1996). Ahipa (Pachyrhizus ahipa (Wedd.) Parodi) in Bolivia. Genet. Resour. Crop Evol. 43, 435–446.
Pagán-Jiménez, J. R., Guachamín-Tello, A. M., Romero-Bastidas, M. E., and Constantine-Castro, A. R. (2016). Late ninth millennium B.P. use of Zea mays L. at Cubilán area, highland Ecuador, revealed by ancient starches. Quat. Int. 404, 137–155. doi: 10.1016/j.quaint.2015.08.025
Pagán-Jiménez, J. R., Rodríguez-Ramos, R., Reid, B. A., Van Den Bel, M., and Hofman, C. L. (2015). Early dispersals of maize and other food plants into the Southern Caribbean and Northeastern South America. Quat. Sci. Rev. 123, 231–246. doi: 10.1016/j.quascirev.2015.07.005
Pearsall, D. (1989). “Adaptation of prehistoric hunter-gatherers to the high Andes: The changing role of plant resources,” in Foraging and farming: The evolution of plant exploitation, eds D. R. Harris and G. C. Hillman (London: Routledge), 318–334.
Pearsall, D. M. (2008). “Plant domestication and the shift to agriculture in the Andes,” in Handbook of South American archaeology, eds H. Silverman and W. H. Isbell (New York, NY: Springer), 105–120. doi: 10.1007/978-0-387-74907-5_7
Perera, P. I. P., Quintero, M., Dedicova, B., Kularatne, J. D. J. S., and Ceballos, H. (2013). Comparative morphology, biology and histology of reproductive development in three lines of Manihot esculenta Crantz (Euphorbiaceae: Crotonoideae). AoB Plants 5:pls046. doi: 10.1093/aobpla/pls046
Perry, L. (2002). Starch granule size and the domestication of manioc (Manihot esculenta) and sweet potato (Ipomoea batatas). Econ. Bot. 56, 335–349. doi: 10.1663/0013-0001(2002)056[0335:SGSATD]2.0.CO;2
Pickersgill, B., and Heiser, C. B. (1977). “Origins and distribution of plants domesticated in the New World tropics,” in Origins of agriculture, ed. C. A. Reed (The Hague: Mouton), 803–835. doi: 10.1515/9783110813487.803
Pinto, R., Barría, I., and Marquet, P. A. (2006). Geographical distribution of Tillandsia lomas in the Atacama desert, northern Chile. J. Arid Environ. 65, 543–552. doi: 10.1016/j.jaridenv.2005.08.015
Piperno, D. R. (2006b). Phytoliths: A comprehensive guide for archaeologists and paleoecologists. Lanham, MD: AltaMira Press.
Piperno, D. R. (2006a). “Identifying manioc (Manihot esculenta Crantz) and other crops in pre-Columbian tropical America through starch grain analysis: A case study from central Panama,” in Documenting domestication: New genetic and archaeological paradigms, eds M. A. Zeder, D. Bradley, E. Emshwiller, and B. D. Smith (Berkeley, CA: University of California Press), 46–67. doi: 10.1525/9780520932425-008
Piperno, D. R. (2011). The origins of plant cultivation and domestication in the New World tropics: Patterns, process, and new developments. Curr. Anthropol. 52, S453–S470. doi: 10.1086/659998
Piperno, D. R., and Dillehay, T. D. (2008). Starch grains on human teeth reveal early broad crop diet in northern Peru. Proc. Natl. Acad. Sci. U.S.A. 105, 19622–19627. doi: 10.1073/pnas.0808752105
Piperno, D. R., and Pearsall, D. M. (1998). The origins of agriculture in the Lowland Neotropics. San Diego, CA: Academic Press.
Prenner, G., and Rudall, P. J. (2007). Comparative ontogeny of the cyathium in Euphorbia (Euphorbiaceae) and its allies: Exploring the organ–flower–inflorescence boundary. Am. J. Bot. 94, 1612–1629. doi: 10.3732/ajb.94.10.1612
Quilter, J., Ojeda, B., Pearsall, D. M., Sandweiss, D. H., Jones, J. G., and Wing, E. S. (1991). Subsistence economy of El Paraíso, an early Peruvian site. Science 251, 277–283. doi: 10.1126/science.251.4991.277
Ramu, P., Esuma, W., Kawuki, R., Rabbi, I. Y., Egesi, C., Bredeson, J. V., et al. (2017). Cassava haplotype map highlights fixation of deleterious mutations during clonal propagation. Nat. Genet. 49, 959–963. doi: 10.1038/ng.3845
Rivera, M. A. (1976). “Nuevos aportes sobre el desarrollo cultural altiplanico del extremo norte de Chile durante el Periodo Intermedio Temprano,” in Proceedings of the 42nd international congress of Americanists, Paris, 31–38.
Roberts, A., Pate, F. D., Petruzzelli, B., Carter, C., Westaway, M. C., Santoro, C. M., et al. (2013). Retention of hunter-gatherer economies among maritime foragers from Caleta Vitor, northern Chile, during the late Holocene: Evidence from stable carbon and nitrogen isotopic analysis of skeletal remains. J. Archaeol. Sci. 40, 2360–2372. doi: 10.1016/j.jas.2013.01.009
Romero, Á., Santoro, C. M., Valenzuela, D., Chacama, J., Rosello, E., and Piacenza, L. (2004). Túmulos, idelogía y paisaje de la fase alto ramírez del valle de azapa. Chungara 36, 261–272. doi: 10.4067/S0717-73562004000300028
Rothhammer, F., and Santoro, C. M. (2001). Cultural development in the Azapa Valley in the far north of Chile, and its connection with population displacement in the highlands. Lat. Am. Antiq. 12, 59–66. doi: 10.2307/971757
Roullier, C., Duputié, A., Wennekes, P., Benoit, L., Fernández Bringas, V. M., Rossel, G., et al. (2013). Disentangling the origins of cultivated sweet potato (Ipomoea batatas (L.) Lam.). PLoS One 8:e62707. doi: 10.1371/journal.pone.0062707
Sandweiss, D. H., Solís, R. S., Moseley, M. E., Keefer, D. K., and Ortloff, C. R. (2009). Environmental change and economic development in coastal Peru between 5,800 and 3,600 years ago. Proc. Natl. Acad. Sci. U.S.A. 106, 1359–1363. doi: 10.1073/pnas.0812645106
Santana-Sagredo, F., Schulting, R. J., Méndez-Quiros, P., Vidal-Elgueta, A., Uribe, M., Loyola, R., et al. (2021). ‘White gold’ guano fertilizer drove agricultural intensification in the Atacama desert from ad 1000. Nat. Plants 7, 152–158. doi: 10.1038/s41477-020-00835-4
Santayana, M., Rossel, G., Núñez, J., Sørensen, M., Delêtre, M., Robles, R., et al. (2014). Molecular characterization of cultivated species of the genus Pachyrhizus Rich. ex DC. by AFLP markers: Calling for more data. Trop. Plant Biol. 7, 121–132. doi: 10.1007/s12042-014-9143-7
Santoro, C. (1980). Fase Azapa transición del arcaico al desarrollo agrario inicial en los valles bajos de Arica. Chungara 6, 46–56.
Santoro, C. M., Capriles, J. M., Gayo, E. M., De Porras, M. E., Maldonado, A., Standen, V. G., et al. (2017). Continuities and discontinuities in the socio-environmental systems of the Atacama desert during the last 13,000years. J. Anthropol. Archaeol. 46, 28–39. doi: 10.1016/j.jaa.2016.08.006
Scaglion, R., and Cordero, M.-A. (2011). “Did ancient Polynesians reach the new world? Evaluating evidence from the Ecuadorian Gulf of Guayaquil,” in Polynesians in America: Pre-Columbian contacts with the New World, eds T. L. Jones, A. A. Storey, E. Matisoo-Smith, and J. M. Ramírez-Aliaga (Lanham, MA: AltaMira Press), 171–193.
Shady Solis, R., Haas, J., and Creamer, W. (2001). Dating Caral, a Preceramic Site in the Supe Valley on the central coast of Peru. Science 292, 723–726. doi: 10.1126/science.1059519
Simon, M. F., Mendoza Flores, J. M., Liu, H.-L., Lacerda Lopes Martins, M., Drovetski, S. V., Przelomska, N. A. S., et al. (2022). Phylogenomic analysis points to a South American origin of Manihot and illuminates the primary gene pool of cassava. New Phytol. 233, 534–545. doi: 10.1111/nph.17743
Sørensen, M., Gruneberg, W. J., Orting, B., Herman, M., and Heller, J. (1997). “Andean roots and tubers: Ahipa, arracacha, maca and yacon,” in Promoting the conservation and use of underutilized and neglected crops, eds M. Hermann and J. Heller (Rome: International Plant Genetic Resources Institute).
Sotomayor Melo, D. A., and Jiménez Milón, P. (2008). Condiciones meteorológicas y dinámica vegetal del ecosistema costero lomas de Atiquipa (Caravelí-Arequipa) en el sur del Perú. Ecol. Apl. 7, 1–8. doi: 10.21704/rea.v7i1-2.353
Srisuwan, S., Sihachakr, D., and Siljak-Yakovlev, S. (2006). The origin and evolution of sweet potato (Ipomoea batatas Lam.) and its wild relatives through the cytogenetic approaches. Plant Sci. 171, 424–433. doi: 10.1016/j.plantsci.2006.05.007
Standen, V. G., Santoro, C. M., Arriaza, B., and Coleman, D. (2018). Hunting, gathering, and fishing on the coast of the Atacama desert: Chinchorro population mobility patterns inferred from strontium isotopes. Geoarchaeology 33, 162–176. doi: 10.1002/gea.21594
Standen, V. G., Santoro, C. M., Arriaza, B., Verano, J., Monsalve, S., Coleman, D., et al. (2021). Violence among the first horticulturists in the Atacama desert (1000 BCE–600 CE). J. Anthropol. Archaeol. 63:101324. doi: 10.1016/j.jaa.2021.101324
Szpak, P. (2014). Complexities of nitrogen isotope biogeochemistry in plant-soil systems: Implications for the study of ancient agricultural and animal management practices. Front. Plant Sci. 5:288. doi: 10.3389/fpls.2014.00288
Szpak, P., Millaire, J.-F., White, C. D., and Longstaffe, F. J. (2012). Influence of seabird guano and camelid dung fertilization on the nitrogen isotopic composition of field-grown maize (Zea mays). J. Archaeol. Sci. 39, 3721–3740. doi: 10.1016/j.jas.2012.06.035
Thitipraphunkul, K., Uttapap, D., Piyachomkwan, K., and Takeda, Y. (2003). A comparative study of edible canna (Canna edulis) starch from different cultivars. Part I. Chemical composition and physicochemical properties. Carbohydr. Polym. 53, 317–324. doi: 10.1016/S0144-8617(03)00081-X
Tilburg, J. A. V., Huebert, J. M., Sherwood, S. C., and Barrier, C. R. (2022). “Pre-European contact sweet potato (Ipomoea batatas) at Rapa Nui: Macrobotanical evidence from recent excavations in Rano Raraku Quarry, Rapa Nui,” in The prehistory of Rapa Nui (Easter Island): Towards an integrative interdisciplinary framework, eds V. Rull and C. Stevenson (Cham: Springer), 85–108. doi: 10.1007/978-3-030-91127-0_5
Tromp, M., and Dudgeon, J. V. (2015). Differentiating dietary and non-dietary microfossils extracted from human dental calculus: The importance of sweet potato to ancient diet on Rapa Nui. J. Archaeol. Sci. 54, 54–63. doi: 10.1016/j.jas.2014.11.024
Ugalde, P. C., Mcrostie, V., Gayo, E. M., García, M., Latorre, C., and Santoro, C. M. (2021). 13,000 years of sociocultural plant use in the Atacama desert of northern Chile. Veg. Hist. Archaeobot. 30, 213–230. doi: 10.1007/s00334-020-00783-1
Ugent, D., Pozorski, S., and Pozorski, T. (1984). New evidence for ancient cultivation of Canna edulis in Peru. Econ. Bot. 38, 417–432. doi: 10.1007/BF02859081
Ugent, D., Pozorski, S., and Pozorski, T. (1986). Archaeological manioc (Manihot) from coastal Peru. Econ. Bot. 40, 78–102. doi: 10.1007/BF02858949
Uribe, M., Angelo, D., Capriles, J., Castro, V., De Porras, M. E., García, M., et al. (2020). El Formativo en Tarapacá (3000-1000 aP): Arqueología, naturaleza y cultura en la Pampa del Tamarugal, Desierto de Atacama, norte de Chile. Lat. Am. Antiq. 31, 81–102. doi: 10.1017/laq.2019.92
Valdez, L. M., and Bettcher, K. J. (2021). Pachamanka: Inka earthen ovens from Tambo Viejo, Peru. Lat. Am. Antiq. 32, 858–864. doi: 10.1017/laq.2021.43
Valenzuela, D., Santoro, C. M., Capriles, J. M., Quinteros, M. J., Peredo, R., Gayo, E. M., et al. (2015). Consumption of animals beyond diet in the Atacama desert, northern Chile (13,000-410 BP): Comparing rock art motifs and archaeofaunal records. J. Anthropol. Archaeol. 40, 250–265. doi: 10.1016/j.jaa.2015.09.004
Vidal, A., García, M., and Vega, G. (2004). Trabajando con plantas en la localidad arqueológica de Pisagua. Bol. Soc. Chil. Arqueol. 37, 49–59.
Vidal Elgueta, A., Hinojosa, L. F., Pérez, M. F., Peralta, G., and Rodríguez, M. U. (2019). Genetic and phenotypic diversity in 2000 years old maize (Zea mays L.) samples from the Tarapacá region, Atacama desert, Chile. PLoS One 14:e0210369. doi: 10.1371/journal.pone.0210369
Villagrán, C., and Castro, V. (1997). Etnobotánica y manejo ganadero de las vegas, bofedales y quebradas en el Loa superior, Andes de Antofagasta, Segunda Región, Chile. Chungará 29, 275–304.
Villagrán, C., Castro, V., Sánchez, G., Hinojosa, F., and Latorre, C. (1999). La tradición altiplánica: Estudio etnobotánico en los Andes de Iquique, Primera Región, Chile. Chungará 31, 81–186.
Villagrán, C., Castro, V., Sánchez, G., and Colamar, D. J. (1998a). Etnobotánica y percepción del paisaje en Caspana (Provincia de El Loa, Región de Antofagasta, Chile): ?Una cuña atacameña en el Loa Superior? Estud. Atacamenos 16, 107–170. doi: 10.22199/S07181043.1998.0016.00003
Villagrán, C., Castro, V., Sánchez, G., Romo, M., Latorre, C., and Hinojosa, L. F. (1998b). La tradición surandina del desierto: Etnobotánica del área del Salar de Atacama (Provincia de El Loa, Región de Antofagasta, Chile). Estud. Atacamenos 16, 7–105. doi: 10.22199/S07181043.1998.0016.00002
Wang, W., Feng, B., Xiao, J., Xia, Z., Zhou, X., Li, P., et al. (2014). Cassava genome from a wild ancestor to cultivated varieties. Nat. Commun. 5:5110. doi: 10.1038/ncomms6110
Watling, J., Shock, M. P., Mongeló, G. Z., Almeida, F. O., Kater, T., De Oliveira, P. E., et al. (2018). Direct archaeological evidence for Southwestern Amazonia as an early plant domestication and food production centre. PLoS One 13:e0199868. doi: 10.1371/journal.pone.0199868
Woolfe, J. A. (1992). Sweet potato: An untapped food resource. Cambridge: Cambridge University Press.
Zarrillo, S. (2012). Human adaptation. Food production and cultural interaction during the formative period in highland Ecuador. Ph.D. dissertation. Calgary, AB: University of Calgary.
Zarrillo, S., Gaikwad, N., Lanaud, C., Powis, T., Viot, C., Lesur, I., et al. (2018). The use and domestication of Theobroma cacao during the mid-Holocene in the upper Amazon. Nat. Ecol. Evol. 2, 1879–1888. doi: 10.1038/s41559-018-0697-x
Keywords: Andes, archeology, plant domestication, cultivation, tubers, archaeobotany, starch grain analysis
Citation: Capriles JM, García M, Valenzuela D, Domic AI, Kistler L, Rothhammer F and Santoro CM (2022) Pre-Columbian cultivation of vegetatively propagated and fruit tree tropical crops in the Atacama Desert. Front. Ecol. Evol. 10:993630. doi: 10.3389/fevo.2022.993630
Received: 13 July 2022; Accepted: 09 September 2022;
Published: 29 September 2022.
Edited by:
Xinyi Liu, Washington University in St. Louis, United StatesReviewed by:
Gayle Fritz, Washington University in St. Louis, United StatesAnna Maria Mercuri, University of Modena and Reggio Emilia, Italy
Hongen Jiang, University of Chinese Academy of Sciences, China
Copyright © 2022 Capriles, García, Valenzuela, Domic, Kistler, Rothhammer and Santoro. This is an open-access article distributed under the terms of the Creative Commons Attribution License (CC BY). The use, distribution or reproduction in other forums is permitted, provided the original author(s) and the copyright owner(s) are credited and that the original publication in this journal is cited, in accordance with accepted academic practice. No use, distribution or reproduction is permitted which does not comply with these terms.
*Correspondence: José M. Capriles, anVjNTU1QHBzdS5lZHU=