- 1Faculty of Agriculture, Saga University, Saga, Japan
- 2United Graduate School of Agricultural Sciences, Kagoshima University, Kagoshima, Japan
- 3Department of Health Chemistry, Showa Pharmaceutical University, Tokyo, Japan
- 4Yakushima Evergreen Broad-Leaved Forest Network, Kagoshima, Japan
- 5National Museum of Nature and Science, Ibaraki, Japan
Dendrobium officinale (Orchidaceae) is an endangered epiphytic orchid that has been well studied as a medicinal plant. Although previous studies have shown that various fungal isolates promote D. officinale seed germination and seedling development in vitro, mycorrhizal associations among its wild populations remain poorly understood. In this study, we identified mycorrhizal fungi associated with D. officinale (36 individuals from six sites) using Sanger sequencing and compared fungal communities among sites and habitats (lithophytic vs. epiphytic individuals). Among the obtained sequences, 76 belonged to orchid mycorrhizal fungi (OMF), among which Tulasnellaceae accounted for 45.8% and Serendipitaceae for 28.1%. The Serendipitaceae operational taxonomic unit (OTU) SE1 was the most dominant partner, accounting for 27.1% of all detected fungal sequences, followed by a Tulasnellaceae OTU, TU27, which accounted for 15.6%. The relative frequencies of Serendipitaceae and Tulasnellaceae differed greatly between lithophytic and epiphytic individuals. Serendipitaceae accounted for 47.3% of the OMF sequences among lithophytes, and Tulasnellaceae for 95.2% among epiphytes. Mycorrhizal community composition also varied among sites. We further conducted in vitro symbiotic culture from seeds with six fungal isolates. Two Serendipitaceae and two Tulasnellaceae isolates, including SE1 and TU27, significantly promoted seed germination and seedling development. These results indicate that D. officinale is mainly associated with Tulasnellaceae and Serendipitaceae as its main fungal partners, which strongly induced seed germination and seedling development in vitro, suggesting their association with D. officinale through its life cycle.
Introduction
Orchidaceae is among the largest angiosperm plant families, comprising more than 28,000 species (Christenhusz and Byng, 2016), 69% of which are epiphytic (Zotz, 2013). Orchids form symbiotic associations with mycorrhizal fungi, in which fungal hyphae penetrate living plant cells to form intracellular pelotons (Smith and Read, 2008). Orchid seeds are highly dependent on mycorrhizal fungi for carbon, nitrogen, and other nutrients during seed germination; such associations generally persist in mature plants (Rasmussen and Rasmussen, 2009). Most orchid mycorrhizal fungi (OMF) belong to a rhizoctonia aggregate, a polyphyletic group of fungi belonging to a combination of Tulasnellaceae, Serendipitaceae, and Ceratobasidiaceae (Rasmussen, 2002; Dearnaley et al., 2012). Orchid mycorrhizal associations do not always remain stable throughout the plant life cycle, with some orchids continuing their association with the same fungi and others switching partners from the seed germination to adult stages (Ventre Lespiaucq et al., 2021). Habitat type, which can be terrestrial, epiphytic, or lithophytic, also often affects mycorrhizal communities (Xing et al., 2019; Qin et al., 2020). OMF may have a significant impact on the distribution, abundance, and population dynamics of orchid species (Jacquemyn et al., 2012; McCormick et al., 2018). However, despite the rich diversity of epiphytic orchids, far fewer studies have explored OMF associations among epiphytic orchids than among terrestrial orchids.
The genus Dendrobium Swartz is among the largest genera in Orchidaceae, including approximately 1,450 species distributed in tropical and subtropical regions from India to Southeast Asia, China, Japan, and Oceania (Schuiteman, 2014). Dendrobium species have long been studied for their economic, medicinal, and ornamental value (Teixeira da Silva et al., 2015; Teoh, 2016). Mycorrhizal associations with Dendrobium species have also been investigated for the propagation of medicinal species and conservation of endangered species (Chen et al., 2021). However, most such studies have focused on symbiotic culture with fungal isolates from roots, seeds, or seedlings (Nontachaiyapoom et al., 2011; Mala et al., 2017; Maharjan et al., 2020), whereas mycorrhizal associations among wild orchid populations remain poorly understood, although a few studies have revealed in situ associations with several wild populations (Xing et al., 2013; Rammitsu et al., 2021).
Dendrobium officinale Kimura and Migo (syn. Dendrobium stricklandianum Rchb.f and Dendrobium tosaense Makino; Jin and Huang, 2015) is a component of many traditional Chinese medicines and its symbionts have been well studied (Ding et al., 2008; Jin et al., 2017; Zuo et al., 2021). This species is distributed from southern China to southern Japan, where it grows on cliffs (lithophyte) or tree trunks (epiphyte) covered with humus and moss (Zhu et al., 2009; Hou et al., 2012). Although various fungal isolates from Dendrobium species promote seed germination and seedling development in D. officinale (Guo and Xu, 1991; Wu et al., 2012; Shao et al., 2019; Wang et al., 2021), the mycorrhizal associations of its wild populations remain unclear. Mycorrhizal fungi can vary among sites and substrates (lithophytic or epiphytic individuals). D. officinale is an endangered species due to over collection; therefore, understanding the mycorrhizal associations of its wild populations is important for its conservation.
In this study, we also examined the effects of major and minor mycorrhizal fungal associations on seed germination, protocorm formation, and seedling development. We examined 36 wild D. officinale individuals (27 lithophytic and 9 epiphytic) sampled from six sites and conducted in vitro symbiotic seed germination testing using D. officinale seeds and six fungal isolates obtained from roots.
Materials and methods
Sample collection
In all, 36 D. officinale individuals were collected from six sites in Kochi and Kagoshima Prefectures in Japan (Table 1). To examine differences in mycorrhizal fungal associations between epiphytic and lithophytic individuals, we collected epiphytic root samples from seven tree species and lithophytic root samples from rocks, cement bridges, and a roof. Root samples (3–5 cm per plant) were washed with tap water, and hand-sliced sections were observed under a microscope to assess fungal colonization. Mycorrhizal root segments were cut into 1–2 cm fragments and stored in Tris-EDTA (TE) buffer at –20°C for fungal molecular identification. Sections with living hyphal coils were used for fungal isolation.
Fungal isolation
Root sections with living hyphal coils were washed with sterile distilled water (SDW) to remove bark debris from the root surface and crushed with forceps to disperse the viable hyphae coils into 100 mL SDW. Hyphal coils (pelotons) were collected using a micropipette and rinsed four times in sterile water. For culture, these pelotons with 20–40 μL SDW were dropped onto 1.5% agar medium containing 50 ppm streptomycin and tetracycline. Plates were incubated at 25 ± 1°C for 1 week. Fungal colonies that formed from single pelotons were transferred to fresh potato dextrose agar (PDA) plates for subculture. The fungal isolates obtained in this study were deposited in the Biological Resource Center of the National Institute of Technology and Evaluation (NBRC) (Table 2).
Molecular identification of mycorrhizal fungi
DNA was extracted from root samples as described previously (Rammitsu et al., 2021). Samples were crushed with forceps to disperse hyphal coils into TE buffer. We collected 100–200 coils per fragment and homogenized these with 20 μL TE buffer using a BioMasher II homogenizer (Nippi Inc., Tokyo, Japan). For fungal isolate DNA, hyphae growing on the culture medium were collected using a sterilized toothpick and suspended in 50 μL TE buffer. DNA was extracted from the suspension as described previously (Izumitsu et al., 2012). Polymerase chain reaction (PCR) amplification of the internal transcribed spacer (ITS) sequences was performed using the fungal universal primer pairs ITS1F/ITS4 (White et al., 1990; Gardes and Bruns, 1993) and ITS1F/ITS4B (Gardes and Bruns, 1993). These primer pairs failed to amplify sequences of Tulasnellaceae, which is a dominant mycorrhizal fungal family associated with orchids. Therefore, we also used the Tulasnellaceae-specific primer pairs ITS5/ITS4-Tul2 (White et al., 1990; Oja et al., 2015) and 5.8S-Tulngs/ITS4-Tul2 (Oja et al., 2015; Rammitsu et al., 2021). PCR amplification was performed using MightyAmp DNA polymerase Ver.3 (TaKaRa, Shiga, Japan) in a total volume of 10 μL, containing 1 μL sample DNA, 5 μL 2 × MightyAmp buffer, 5 pmol each primer, 0.2 μL MightyAmp DNA Polymerase Ver.3, and 1 μL 10 × Additive for High Specificity (TaKaRa).
PCR amplification was performed with the following cycling parameters: initial denaturation at 98°C for 2 min, followed by denaturation at 98°C for 10 s, annealing at 58°C for 15 s, extension at 68°C for 40 s, for a total of 35 cycles. The resulting amplicons were purified using the Fast Gene Gel/PCR Extraction Kit (Nippon Genetics, Tokyo, Japan) and sequenced using the BigDye Terminator v3.1 Cycle Sequencing Kit (Thermo Fisher Scientific Baltics, Vilnius, Lithuania) and 3,130 Genetic Analyzer (Applied Biosystems, Tokyo, Japan) according to the manufacturer’s instructions. All ITS sequences were assigned to operational taxonomic units (OTUs) defined by 97% sequence similarity. All ITS sequences were analyzed using BLAST searches (Altschul, 1997) against the GenBank sequence database to find the closest matching sequence. The full-length ITS sequences of each OTU were edited using the ATGC v7 sequence assembly software (Genetyx, Tokyo, Japan) and deposited in the DNA Data Bank of Japan under accession numbers LC597346, LC597350, and LC683198–LC683206.
Phylogenetic analysis
OTUs belonging to Tulasnellaceae, Serendipitaceae and Ceratobasidiaceae, which are known OMF, were considered putative mycorrhizal associates and subjected to phylogenetic analysis using ITS sequences. Sequences obtained from Dendrobium species in previous studies were included in the analysis (Wang et al., 2011; Shao et al., 2019; Zhang et al., 2020). The phylogenetic analysis was performed using the MEGA 11 software (Nei and Kumar, 2000; Stecher et al., 2020; Tamura et al., 2021). Maximum likelihood (ML) trees were obtained using the GTR + G + I model. Bootstrap (BS) analysis of the ML trees was performed using 1,000 replicates (Felsenstein, 1985). All positions with < 90% site coverage was eliminated, i.e., < 10% of alignment gaps, missing dates, and ambiguous bases were allowed at any position.
Symbiotic culture
Six fungal isolates from D. officinale were used (Table 2). A fungal colony of each isolate was transferred onto PDA as pre-culture and cultured in the dark at 25 ± 1°C for 7 days. Seeds were obtained from nine mature capsules from five individuals. Seeds from four to five capsules of two or three individuals were mixed and used for symbiotic culture. Prior to each use, seeds were tested using the TTC (2,3,5-triphenyl tetrazolium chloride) method to ensure high viability (> 90%) (Vujanovic et al., 2000). The collected capsules were sterilized using 75% ethanol and dried for 1 week using silica gel desiccant until they had nearly ruptured. Seeds were collected from the capsules and stored at 5°C until use. Seeds were sterilized with 1% sodium hypochlorite solution for 3 min, sown on oatmeal agar medium (OMA; 2.5 g/L oatmeal and 15 g/L agar) and maintained at 25°C for 1 week for contamination checking. After 1 week without contamination, 1 cm × 1 cm discs were cut (5–10 seeds per disc) and transplanted to new OMA media. A total of 20 seeds on two to four discs were placed on each new medium plate. Each treatment consisted of 5–15 replicates, for a total of 100–300 seeds. A 6-mm plug of fungal culture was inoculated onto the OMA medium, and the cultures were placed under a 12 h/12 h light/dark photoperiod at 25 ± 1°C. Petri dishes without fungal inoculum were prepared as a control. After 90 days of culture, the seeds were counted under a stereomicroscope. Germination and seedling growth and development were scored on a scale of 0–5 as described previously (Stewart et al., 2003; Table 3). The data were analyzed by one-way ANOVA and Turkey-Kramer test using IBM SPSS (ver. 27 IBM Corp., NY, USA).
To confirm fungal colonization, the protocorms were cleared using 10% KOH solution, washed in 2% HCl, and stained with 0.05% trypan blue in lactoglycerol, as described previously (Phillips and Hayman, 1970), with modifications. Stained protocorms were de-stained in lactoglycerol prior to microscopic observation (Nikon Eclipse 50i, Nikon, Tokyo, Japan).
Results
Molecular identification of mycorrhizal fungi
In total, 60 root samples and 34 isolates collected from 36 individuals from six sites were analyzed (Table 1). In total, 96 fungal sequences were obtained from these samples and 79.2% of the sequences were OMF, including 45.8% Tulasnellaceae, 28.1% Serendipitaceae, 3.1% Ceratobasidiaceae, and 2.1% Fusarium (Figure 1). Two or three different sequences were obtained from each of the six samples using different primer sets. The independent data sets of fungal sequences for root samples and isolates showed that both data sets consisted of Tulasnellaceae, Serendipitaceae and Ceratobasidiaceae (Supplementary Figure 1). The OMF sequences were assigned to 10 OTUs including six Tulasnellaceae, two Serendipitaceae, one Ceratobasidiaceae, and one Fusarium (Figure 2). Two Fusarium sequences obtained in this study showed high sequence similarity (99–100%) with Fusarium oxysporum according to BLAST analysis. This species formed fungal coils in D. candidum root cells (Jiang et al., 2019) and has been sampled from D. officinale seedlings (Chen et al., 2021). Therefore, we added these Fusarium sequences to the OMF OTUs as FU1.
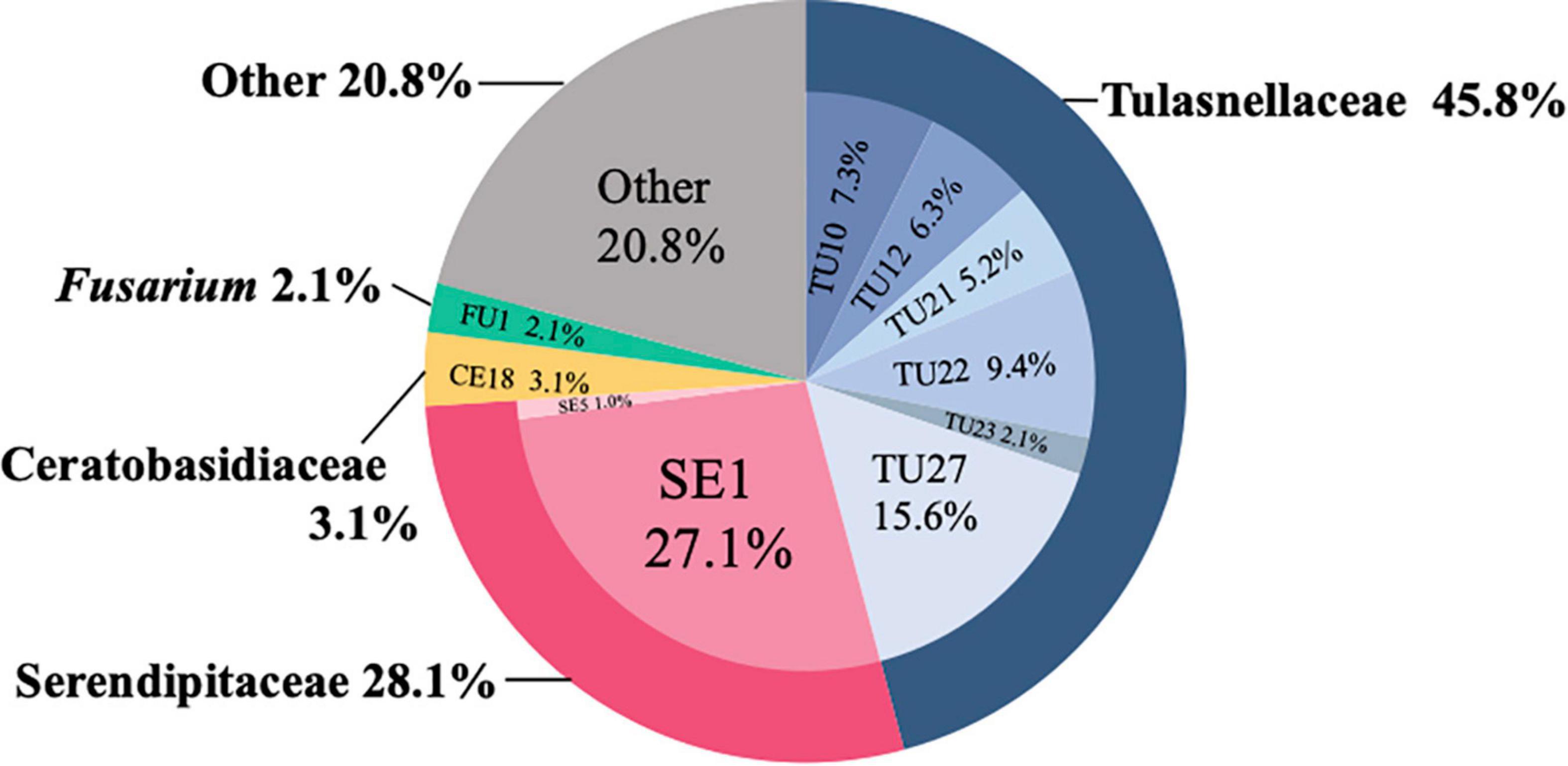
Figure 1. Frequency distribution of fungal sequences identified from Dendrobium officinale using 96 sequences. Identical sequences obtained from a single sample using different primer pairs were discarded.
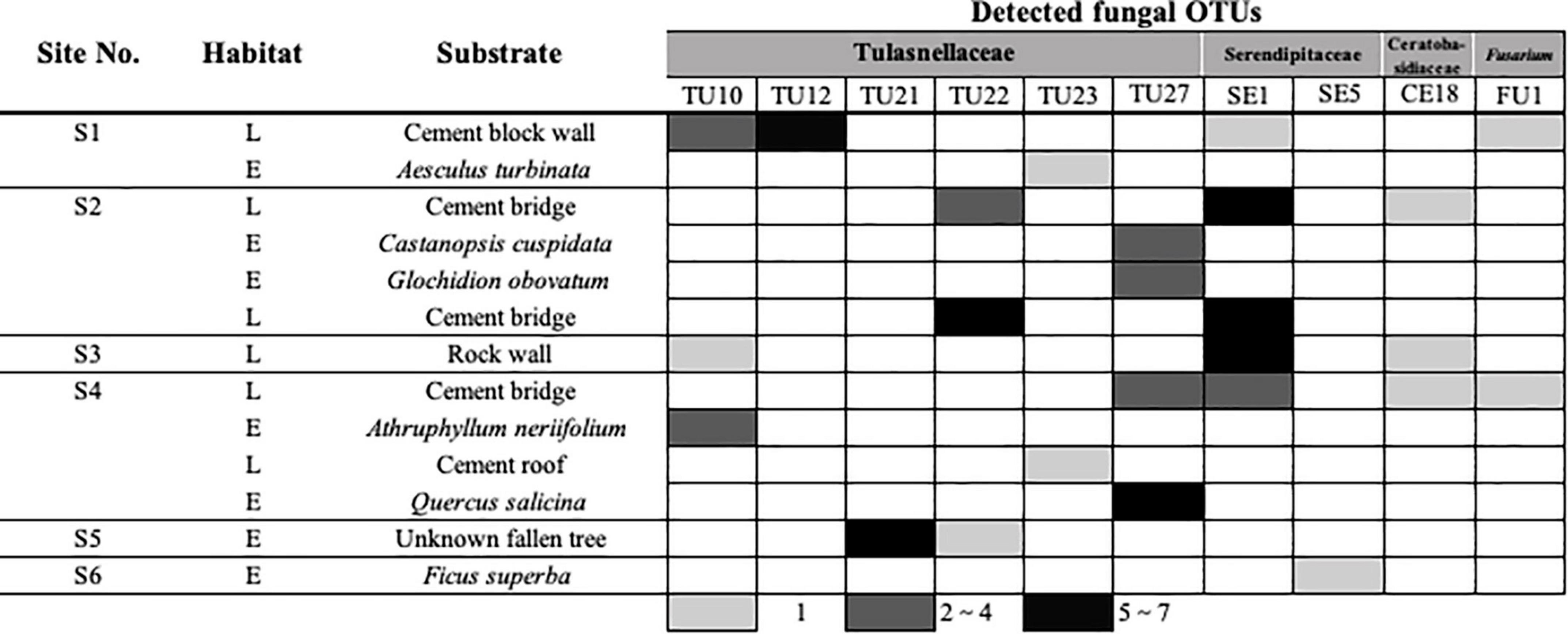
Figure 2. Binary matrix showing the relationship between the sampling sites, substrates, and detected fungal operational taxonomic units (OTUs). The abundance of detected OTUs is indicated as a gradient from white to black. L indicates lithophytic and E indicates epiphytic habitats.
Mycorrhizal fungi were compared among sites and substrates (Figure 2). We collected D. officinale samples from six different sites and 11 substrates. The dominant mycorrhizal fungi varied among both sites and substrates, even within the same site. SE1 was the most frequently detected OTU, occurring in 26 samples from four sites and accounting for 27.1% of all detected fungal OTUs (Figure 1). The second most frequently detected OTU was TU27, which was found in 15 samples from two sites, accounting for 15.6%. TU22 was detected in 9 samples from two sites (9.4%), and TU10 in 7 samples from three sites (7.3%).
The relative frequencies of Serendipitaceae and Tulasnellaceae differed greatly between lithophytic and epiphytic individuals (Figure 3). Serendipitaceae accounted for 47.3% of the total in lithophytes (Figure 3A) and only 4.8% in epiphytes (Figure 3B). By contrast, Tulasnellaceae accounted for 43.6% in lithophytes and 95.2% in epiphytes. Among the 10 detected OTUs, four (TU10, TU22, TU23, and TU27) were present in both substrates, whereas four (TU12, SE1, CE18, and FU1) and two (TU21 and SE5) OTUs were unique to lithophytes and epiphytes, respectively (Figure 3C). Serendipitaceae found in lithophytes consisted of only a single OTU, SE1, which was unique to lithophytes and accounted for approximately half of the total frequency (Figure 3A). TU27 was dominant in epiphytes, accounting for 52.4% of the total frequency, whereas it accounted for only 7.3% in lithophytes (Figures 3A,B).
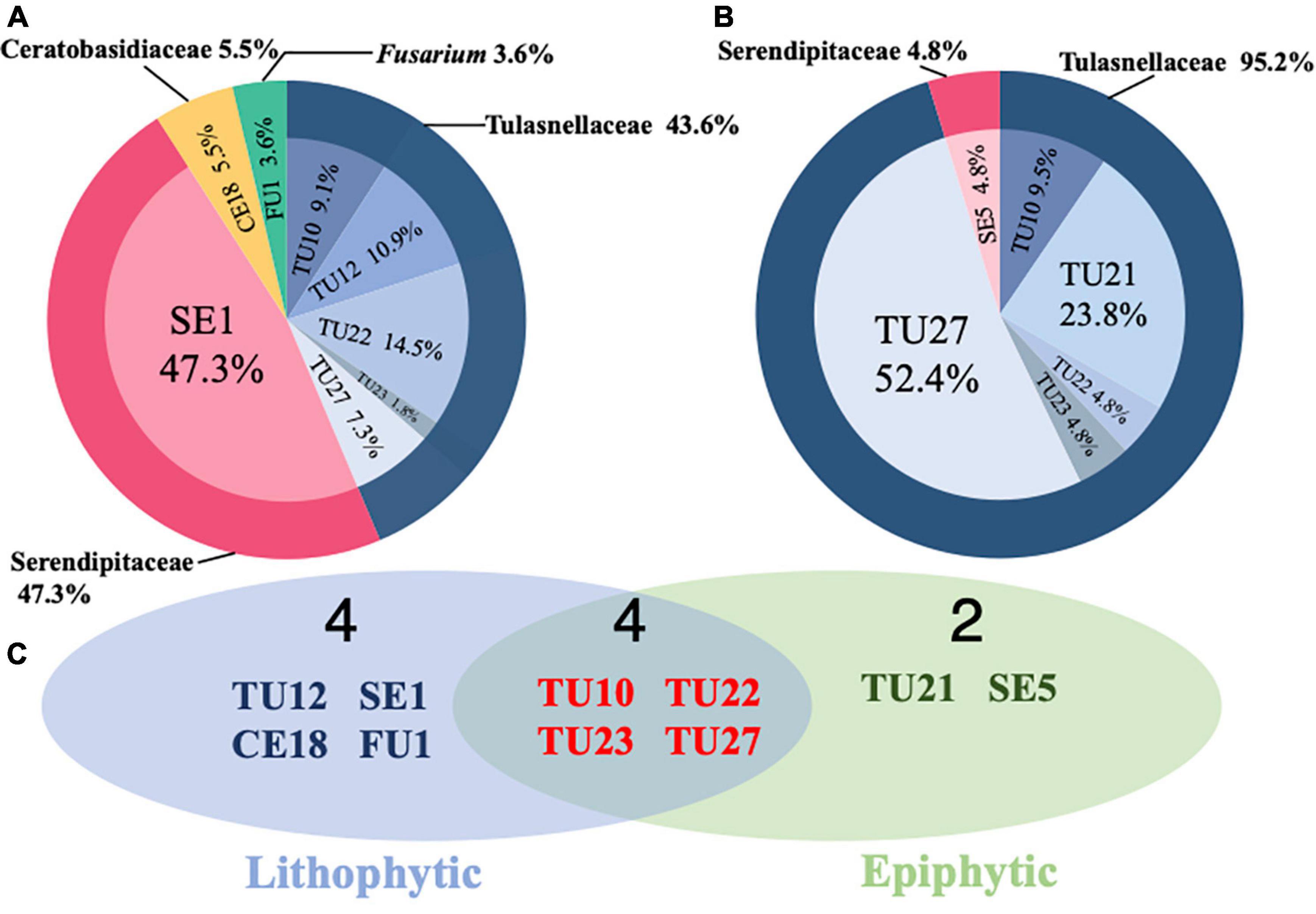
Figure 3. Comparison of orchid mycorrhizal fungi (OMF) associating with lithophytic and epiphytic Dendrobium officinale individuals. Frequency distribution of OMF sequences detected from (A) lithophytic and (B) epiphytic individuals. (C) Venn diagrams showing the numbers of OMF OTUs.
Phylogenetic analysis
Phylogenetic analysis of Serendipitaceae was conducted using two Serendipitaceae OTUs obtained in this study and 33 sequences obtained from the GenBank database (Figure 4). The most dominant mycorrhizal fungus, SE1, formed a monophyletic clade of Thanatephorus sp. SSCDO-8 (MH348617: 97.2% sequence similarity) from D. officinale (as syn. D. catenatum in Zhu et al., 2009), with BS = 99%. SE5 was closely related to Sebacinales sp. from D. officinale (MN173026) and Sebacinales sp. SSCDO-6 from D. officinale (MH348615), sharing 96.9 and 97.3% ITS sequence similarity, respectively.
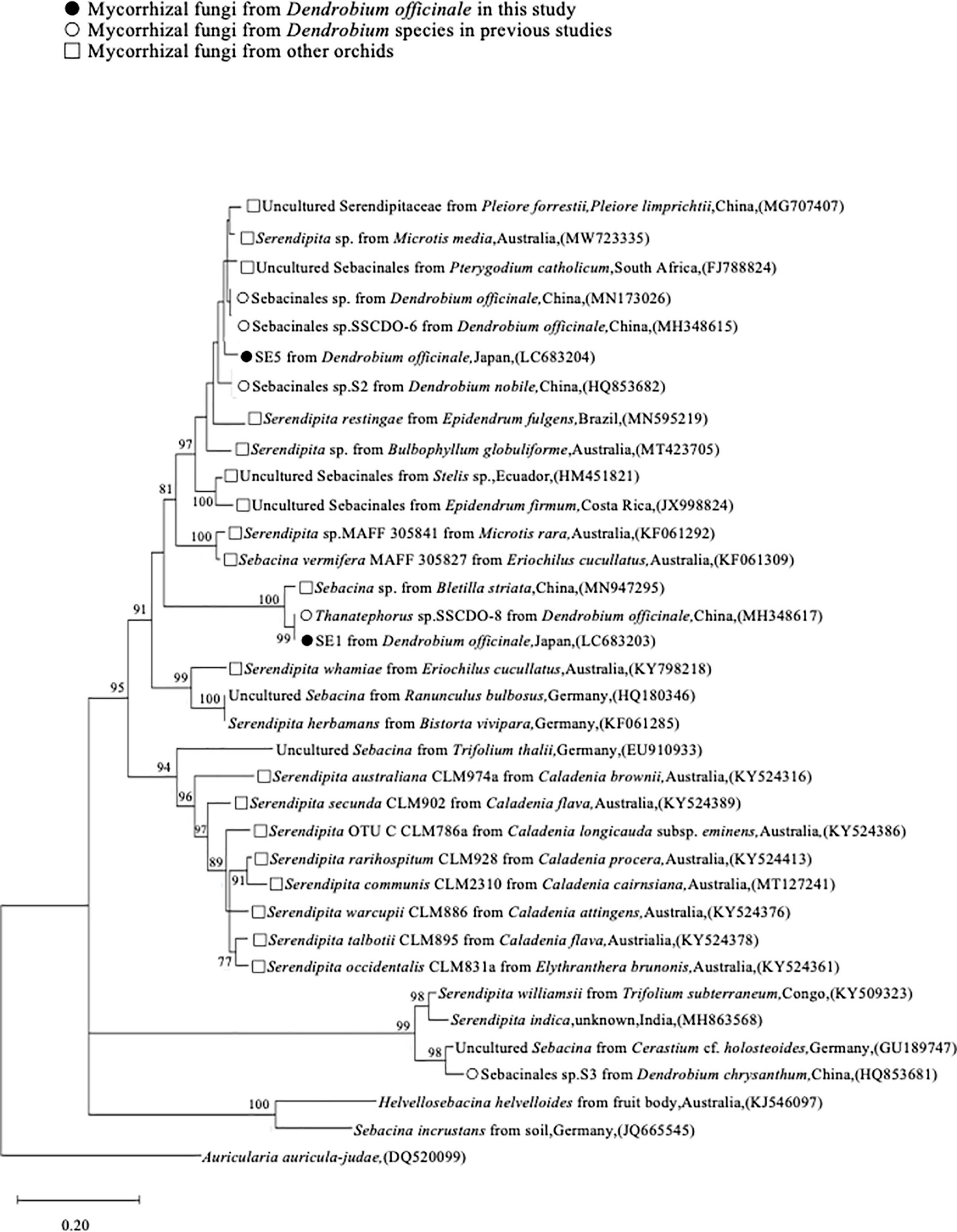
Figure 4. Maximum likelihood tree for Serendipitaceae internal transcribed spacer (ITS) sequences, including those of two OTUs from this study. Symbols indicate the origin of each sequence. Only bootstrap values ≥ 70% are shown. The tree is drawn to scale, with branch lengths reflecting the number of substitutions per site. Auricularia auricula-judae was used as an outgroup taxon. The 35 assembled sequences were aligned, and the final dataset included 382 bp sequences.
The ITS sequences of 6 Tulasnellaceae OTUs obtained in this study and 44 obtained from the GenBank database were used to generate the phylogenetic tree (Figure 5). The second dominant mycorrhizal fungus, TU27, formed a monophyletic clade with four Tulasnellaceae sequences from D. officinale (MH348611, MH348612, MH348613, and MH348616), sharing 97.8–98.0% ITS sequence similarity, with BS = 98%. The TU22 sequence was closely related to the three mycorrhizal fungal sequences from D. officinale (MN545849, MN545657, and MN545858), sharing 96.7–98.2% similarity. TU12 formed a monophyletic clade with two Tulasnella sequences from D. officinale (EF393629 and MN544859) with BS = 99% and shared 97.0–98.8% similarity. TU10 was clustered with mycorrhizal fungi from epiphytic orchid, Ascocentrum himalaicum (JQ713573), with BS = 97%, and closely related to TU27 (BS = 82%). TU23 was clustered with mycorrhizal fungi isolated from other epiphytic species (LC597355, LC568587, OL374168) with BS = 98%. TU21 was closely related to epiphytic species, Liparis viridiflora (KP053821), BS = 98%, and distantly related to the other Tulasnellaceae OTUs.
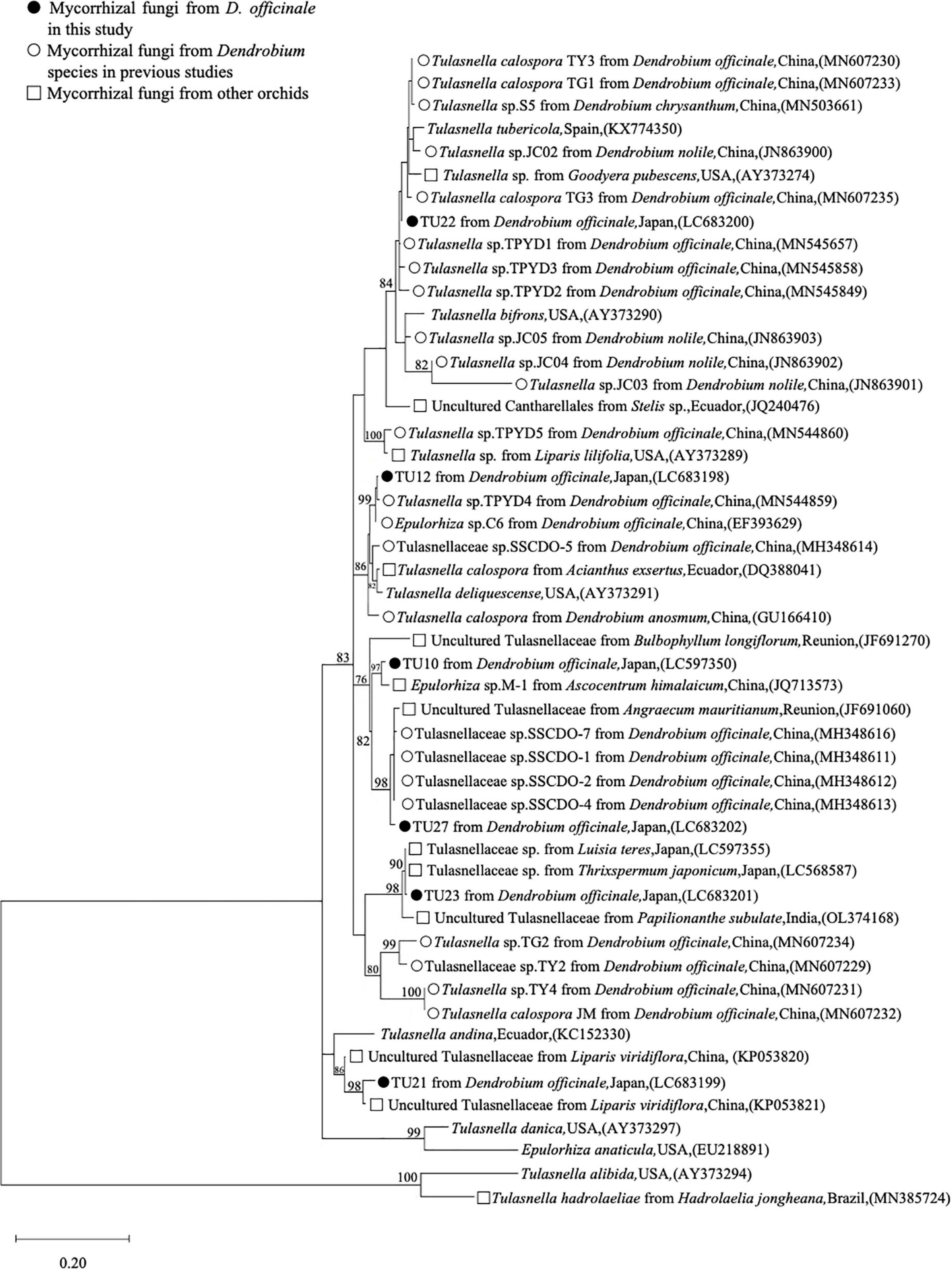
Figure 5. Maximum likelihood tree for Tulasnellaceae ITS sequences, including six OTUs from this study. Symbols indicate the origin of each sequence. Only bootstrap values ≥ 70% are shown. The tree is drawn to scale, with branch lengths reflecting the number of substitutions per site. Tulasnella alibida and Tulasnella hadrolaeliae were used as outgroup taxa. The 50 assembled sequences were aligned, and the final dataset included 442 bp sequences.
Phylogenetic analysis of Ceratobasidiaceae was conducted using one Ceratobasidiaceae OTU obtained in this study and 34 sequences obtained from the GenBank database (Supplementary Figure 2). The CE18 formed a monophyletic group with other Genbank sequences divided from mycobionts of epiphytic orchids containing D. officinale (JX545227), Aranda (AJ318429), Liparis (LC278371), terrestrial orchid of Dactylorhiza (EF536969) and three sequences from plant pathogens, Rhizoctonia sp. AG-G (JF519837, KC825348), Ceratobasidium sp. AG-G (DQ102402), sharing 99.5–100% ITS sequence similarity, with BS = 96%.
Symbiotic culture
Seeds from D. officinale were cultured symbiotically with six OTU isolates including three Tulasnellaceae, two Serendipitaceae, and one Ceratobasidiaceae (Table 2). After 3 months of culture, all isolates except for CE18 promoted seed germination to different degrees (Table 4). Seeds inoculated with TU22, TU27, SE1, and SE5 developed at stage 5, and TU22 and SE1 showed higher development rates than the other isolates. TU10 also promoted seed germination, with seeds developing at stage 4. Seeds cultured with CE18 became swollen and did not develop past stage 2. All six isolates formed intracellular hyphal coils in protocorm cells (Supplementary Figure 3).
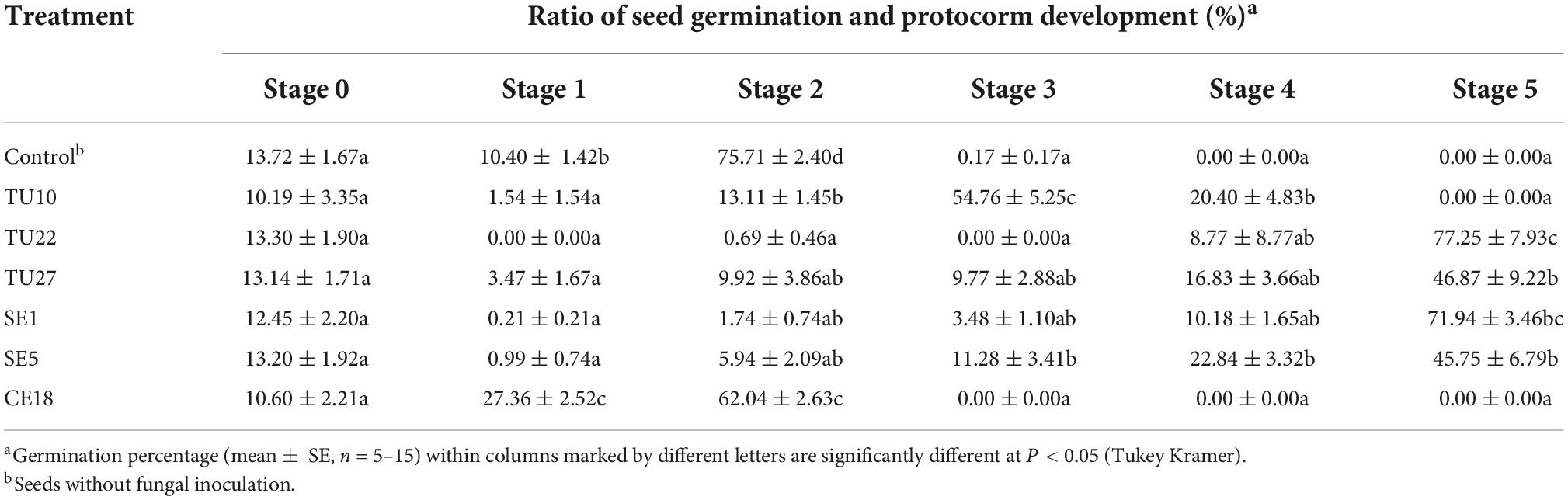
Table 4. Effects of fungal isolates on Dendrobium officinale seed germination and protocorm development after 3 months of culture.
Discussion
In this study, 10 OTUs were detected as OMF in D. officinale samples collected from six sites; eight were included in Tulasnellaceae and Serendipitaceae, accounting for 73.9% of all detected fungal sequences (Figures 1, 2). This implies that these fungal families are the most dominant fungal partners of D. officinale. Most previous studies of D. officinale sampled from southern China have also found Tulasnellaceae and/or Serendipitaceae in roots or protocorms germinated in situ (Wang et al., 2011; Wu et al., 2012; Shao et al., 2019). These results imply that independent of its distribution range, D. officinale has mycorrhizal associations mainly with Tulasnellaceae and Serendipitaceae fungi. Phylogenetic analysis showed that three of the six Tulasnellaceae OTUs and two Serendipitaceae OTUs showed greater than 97% sequence similarity to mycorrhizal fungi associated with D. officinale from China (Figures 4, 5). These OTUs include the most frequent OTUs detected in this study, SE1 and TU27 (Figure 1). Although D. officinale is associated with a wide range of basidiomycetous mycorrhizal partners, its main fungal partners may be widely shared among D. officinale populations. In Dendrobium okinawense, 11 mature plants from four sites were predominantly associated with a single Tulasnellaceae OTU (Rammitsu et al., 2021). Such high specificity is also found in Dendrobium fimbriatum, which was associated with only two OTUs in 15 root samples from two sites (Xing et al., 2013). Mycorrhizal specificity may vary among Dendrobium species (Xing et al., 2017), and D. officinale appears to have lower specificity than its congeners.
Orchid mycorrhizal communities of D. officinale varied among sites in this study (Figure 2). Xing et al. (2013) also found that D. officinale from two sites had distinct OMF communities in Guangxi Province, China. Such community differences among sites have also been recorded in terrestrial orchids (Jacquemyn et al., 2012; Kohout et al., 2013; Oja et al., 2015). There is some evidence that soil chemical characteristics such as phosphorus, zinc, and organic matter (Kaur et al., 2021) and nitrogen, phosphorus, and water content (Han et al., 2016), impact OMF communities in orchid roots and soils. These differences in substrate chemical and physical characteristics may vary among sites, resulting in corresponding OMF community differences.
Mycorrhizal community composition differed between lithophytic and epiphytic individuals in this study (Figure 3). The dominant mycorrhizal fungus among lithophytes was a Serendipitaceae OTU, SE1, whereas that of epiphytes was a Tulasnellaceae OTU, TU27. Distinct OMF communities between lithophytic and epiphytic individuals were also recorded for the orchid Coelogyne viscosa (Xing et al., 2015). Among lithophytic and epiphytic individuals of Coelogyne corymbosa, Serendipitaceae fungi contributed a relatively large portion of the OTU communities specific to lithophytic orchids (Qin et al., 2020). Yokoya et al. (2021) surveyed 11 growing Cynorkis orchid species within lithophytic and terrestrial habitats and found that Serendipitaceae OTUs were frequently found in species inhabiting granite/rock, whereas Tulasnellaceae OTUs were found in both habitat types; they also reported that most Serendipitaceae OTUs were found in the habitat with higher phosphorus and nitrogen content, which may indicate that Serendipitaceae prefers soil conditions with high phosphorus and nitrogen levels. These differences in nutrient conditions may contribute to OMF community differences between lithophytic and epiphytic individuals in D. officinale.
Germination of D. officinale seeds was promoted by five of the six OMF used in this study (Table 4). Although all six OMF formed coiled fungal hyphae within the protocorm cells according to histological observation (Supplementary Figure 3), the ability to promote seed germination varied greatly among OMF (Table 4). All OMF, except CE18, exhibited germination-promoting effects, and seedlings with TU22, TU27, SE1, and SE5 were able to reach stage 5. Phylogenetic analysis showed that the sequences of these four OTUs shared ≥ 97% sequence similarity with fungal isolates obtained in previous studies of D. officinale (Figures 4, 5). Tulasnellaceae sp. SSCDO-7, which is closely related to TU27 (Figure 5), strongly promotes seed germination in D. officinale (Shao et al., 2019). Tulasnellaceae sp. TPYD1, TPYD2, and TPYD3, which share 97–98% sequence similarity with TU22, promote the growth of D. officinale seedlings produced in vitro (Chen et al., 2021). Serendipitaceae isolates SSCDO-8 and SSCDO-6, which are closely related to SE1 and SE5, respectively, also induce D. officinale seed germination and seedling growth (Shao et al., 2019). Our molecular analysis showed that SE1, TU27, and TU22 were the most frequent fungal OTUs in adult individuals (Figure 1), and these fungi promoted seed germination and protocorm development (Table 4). These results suggest that the main fungal partners at the adult stage can induce seed germination and support seedling development in D. officinale.
Seedlings with TU10 developed at stage 4 after 3 months of culture (Table 4) and continued growth, reaching stage 5 after 6 months (data not shown). This fungus induced seed germination, but with slower seedling growth than other effective fungal isolates. By contrast, seedlings with CE18 reached stage 2 after 2 months and showed no further growth, despite our detection of coiled fungal hyphae in protocorm cells (Supplementary Figure 3). Hence, this fungal strain appears not to contribute to seed germination in D. officinale. Ceratobasidiaceae fungi are considered important partners of other orchid genera such as Goodyera (Shefferson et al., 2010), Tolumnia (Otero et al., 2004), and Pterostylis (Bougoure et al., 2005; Bonnardeaux et al., 2007). Phylogenetic analysis showed that CE18 was closely related to OMF from epiphytic and terrestrial orchids (Supplementary Figure 2). However, it has rarely been sampled from D. officinale roots. Because all root samples bearing the Ceratobasidiaceae sequence were accompanied by Tulasnellaceae or Serendipitaceae sequences in this study, Ceratobasidiaceae may not a main fungal partner for D. officinale.
Conclusion
In conclusion, our results demonstrate that D. officinale mainly forms OMF with Tulasnellaceae and Serendipitaceae as its main fungal partners, such as SE1 and TU27. These fungal partners induced D. officinale seed germination and seedling development in vitro, suggesting that they are its main fungal partners throughout its life cycle. The in situ seed baiting technique, which was proposed as an effective and simple technique for obtaining seed germination-enhancing fungi in situ (Rasmussen and Whigham, 1993), will contribute to a more comprehensive understanding of the mycorrhizal associations of D. officinale throughout its life cycle. Our results show that the OMF community differed between lithophytic and epiphytic individuals, suggesting that mycorrhizal specificity may vary by habitat type. Our findings contribute to understanding of mycorrhizal associations among wild Dendrobium species, the conservation of endangered Dendrobium species, and the industrial production of medicinal Dendrobium species.
Data availability statement
Sequence data have been deposited in DNA Data Bank of Japan (DDBJ) under accession numbers LC597346, LC597350, and LC683198–LC683206.
Author contributions
YO-T and TY involved in the study conception and design. KT contributed to the field survey. LZ and KR performed the sampling, experiments, data collection, and analysis. LZ and YO-T wrote the manuscript. All authors commented on previous versions of the manuscript, read, and approved the final manuscript.
Funding
This research was funded by JSPS KAKENHI (grant nos. 21K06306 to YO-T, and 18H02500 to TY) and Research Grant from Yakushima Environmental and Cultural Foundation to KR.
Acknowledgments
We are very grateful to T. Hashimoto, A. Maeda, T. Saito, and T. Tetsuka for sampling and K. Watanabe for DNA analysis. The DNA sequencing analyses were made using a Genetic Analyzer at Analytical Research Center for Experimental Sciences, Saga University.
Conflict of interest
The authors declare that the research was conducted in the absence of any commercial or financial relationships that could be construed as a potential conflict of interest.
Publisher’s note
All claims expressed in this article are solely those of the authors and do not necessarily represent those of their affiliated organizations, or those of the publisher, the editors and the reviewers. Any product that may be evaluated in this article, or claim that may be made by its manufacturer, is not guaranteed or endorsed by the publisher.
Supplementary material
The Supplementary Material for this article can be found online at: https://www.frontiersin.org/articles/10.3389/fevo.2022.994641/full#supplementary-material
References
Altschul, S. (1997). Gapped BLAST and PSI-BLAST: A new generation of protein database search programs. Nucleic Acids Res. 25, 3389–3402. doi: 10.1093/nar/25.17.3389
Bonnardeaux, Y., Brundrett, M., Batty, A., Dixon, K., Koch, J., and Sivasithamparam, K. (2007). Diversity of mycorrhizal fungi of terrestrial orchids: Compatibility webs, brief encounters, lasting relationships and alien invasions. Mycol. Res. 111, 51–61. doi: 10.1016/j.mycres.2006.11.006
Bougoure, J. J., Bougoure, D. S., Cairney, J. W. G., and Dearnaley, J. D. W. (2005). ITS-RFLP and sequence analysis of endophytes from Acianthus, Caladenia and Pterostylis (Orchidaceae) in southeastern Queensland. Mycol. Res. 109, 452–460. doi: 10.1017/S095375620500225X
Chen, D.-Y., Wang, X.-J., Li, T.-Q., Li, N.-Q., and Gao, J.-Y. (2021). In situ seedling baiting to isolate plant growth-promoting fungi from Dendrobium officinale, an over-collected medicinal orchid in China. Glob. Ecol. Conserv. 28:e01659. doi: 10.1016/j.gecco.2021.e01659
Christenhusz, M. J. M., and Byng, J. W. (2016). The number of known plants species in the world and its annual increase. Phytotaxa 261, 201–217. doi: 10.11646/phytotaxa.261.3.1
Dearnaley, J. D. W., Martos, F., and Selosse, M. A. (2012). Orchid mycorrhizas: Molecular ecology, physiology, evolution and conservation aspects. Fungal Assoc. 9, 207–230. doi: 10.1007/978-3-642-30826-0_12
Ding, G., Zhang, D., Ding, X., Zhou, Q., Zhang, W., and Li, X. (2008). Genetic variation and conservation of the endangered Chinese endemic herb Dendrobium officinale based on SRAP analysis. Plant Syst. Evol. 276, 149–156. doi: 10.1007/s00606-008-0068-1
Felsenstein, J. (1985). Confidence limits on phylogenies: An approach using the bootstrap. Evolution 39, 783–791. doi: 10.1111/j.1558-5646.1985.tb00420.x
Gardes, M., and Bruns, T. D. (1993). ITS primers with enhanced specificity for basidiomycetes - application to the identification of mycorrhizae and rusts. Mol. Ecol. 2, 113–118. doi: 10.1111/j.1365-294X.1993.tb00
Guo, S. X., and Xu, J. T. (1991). Studies on the effects of fungi on the course of seed germination of Dendrobium lohohens and Dendrobium candidum. Acta Acad. Med. Sin. 13, 46–49.
Han, J. Y., Xiao, H., and Gao, J. (2016). Seasonal dynamics of mycorrhizal fungi in Paphiopedilum spicerianum (Rchb. f) Pfitzer — A critically endangered orchid from China. Glob. Ecol. Conserv. 6, 327–338. doi: 10.1016/j.gecco.2016.03.011
Hou, B., Tian, M., Luo, J., Ji, Y., Xue, Q., and Ding, X. (2012). Genetic diversity assessment and ex situ conservation strategy of the endangered Dendrobium officinale (Orchidaceae) using new trinucleotide microsatellite markers. Plant Syst. Evol. 298, 1483–1491. doi: 10.1007/s00606-012-0651-3
Izumitsu, K., Hatoh, K., Sumita, T., Kitade, Y., Morita, A., Tanaka, C., et al. (2012). Rapid and simple preparation of mushroom DNA directly from colonies and fruiting bodies for PCR. Mycoscience 53, 396–401.
Jacquemyn, H., Brys, R., Lievens, B., and Wiegand, T. (2012). Spatial variation in below-ground seed germination and divergent mycorrhizal associations correlate with spatial segregation of three co-occurring orchid species. J. Ecol. 100, 1328–1337. doi: 10.1111/j.1365-2745.2012.01998.x
Jiang, J., Zhang, K., Cheng, S., Nie, Q., Zhou, S. X., Chen, Q., et al. (2019). Fusarium oxysporum KB-3 from Bletilla striata: An orchid mycorrhizal fungus. Mycorrhiza 29, 531–540. doi: 10.1007/s00572-019-00904-3
Jin, X., and Huang, L. (2015). Proposal to conserve the name Dendrobium officinale against D. stricklandianum, D. tosaense, and D. pere-fauriei (Orchidaceae). Taxon 64, 385–386. doi: 10.12705/642.19
Jin, Z., Li, D., Liu, T., Zhang, Z., Su, C., Wang, Y., et al. (2017). Cultural endophytic fungi associated with Dendrobium officinale: Identification, diversity estimation and their antimicrobial potential. Curr. Sci. 16, 90–97.
Kaur, J., Phillips, C., and Sharma, J. (2021). Host population size is linked to orchid mycorrhizal fungal communities in roots and soil, which are shaped by microenvironment. Mycorrhiza 31, 17–30. doi: 10.1007/s00572-020-00993-5
Kohout, P., Tìšitelová, T., Roy, M., Vohník, M., and Jersáková, J. (2013). A diverse fungal community associated with Pseudorchis albida (Orchidaceae) roots. Fungal Ecol. 6, 50–64. doi: 10.1016/j.funeco.2012.08.005
Maharjan, S., Thakuri, L. S., Thapa, B. B., Pradhan, S., Pant, K. K., Joshi, G. P., et al. (2020). In vitro propagation of the endangered orchid Dendrobium chryseum Rolfe from protocorms culture. Nepal J. Sci. Technol. 19, 39–47. doi: 10.3126/njst.v19i1.29737
Mala, B., Kuegkong, K., Sa-ngiaemsri, N., and Nontachaiyapoom, S. (2017). Effect of germination media on in vitro symbiotic seed germination of three Dendrobium orchids. S. Afr. J. Bot. 112, 521–526. doi: 10.1016/j.sajb.2017.05.008
McCormick, M. K., Whigham, D. F., and Canchani-Viruet, A. (2018). Mycorrhizal fungi affect orchid distribution and population dynamics. New Phytol. 219, 1207–1215. doi: 10.1111/nph.15223
Nei, M., and Kumar, S. (2000). Molecular evolution and phylogenetics. New York, NY: Oxford University Press.
Nontachaiyapoom, S., Sasirat, S., and Manoch, L. (2011). Symbiotic seed germination of Grammatophyllum speciosum Blume and Dendrobium draconis Rchb. f., native orchids of Thailand. Sci. Hortic. 130, 303–308. doi: 10.1016/j.scienta.2011.06.040
Oja, J., Kohout, P., Tedersoo, L., Kull, T., and Kõljalg, U. (2015). Temporal patterns of orchid mycorrhizal fungi in meadows and forests as revealed by 454 pyrosequencing. New Phytol. 205, 1608–1618. doi: 10.1111/nph.13223
Otero, J. T., Ackerman, J. D., and Bayman, P. (2004). Differences in mycorrhizal preferences between two tropical orchids. Mol. Ecol. 13, 2393–2404. doi: 10.1111/j.1365-294X.2004.02223.x
Phillips, J. M., and Hayman, D. S. (1970). Improved procedures for clearing roots and staining parasitic and vesicular-arbuscular mycorrhizal fungi for rapid assessment of infection. Trans. Br. Mycol. Soc. 55, 18–29. doi: 10.1016/S0007-1536(70)80110-3
Qin, J., Zhang, W., Zhang, S. B., and Wang, J. H. (2020). Similar mycorrhizal fungal communities associated with epiphytic and lithophytic orchids of Coelogyne corymbosa. Plant Diversity 42, 362–369. doi: 10.1016/j.pld.2020.07.005
Rammitsu, K., Abe, S., Abe, T., Kotaka, N., Kudaka, M., Kudaka, N., et al. (2021). The endangered epiphytic orchid Dendrobium okinawense has a highly specific mycorrhizal association with a single Tulasnellaceae fungus. J. For. Res. 26, 215–221. doi: 10.1080/13416979.2021.1876587
Rasmussen, H. N. (2002). Recent developments in the study of orchid mycorrhiza. Plant Soil 244, 149–163. doi: 10.1023/A:1020246715436
Rasmussen, H. N., and Rasmussen, F. N. (2009). Orchid mycorrhiza: Implications of a mycophagous life style. Oikos 118, 334–345. doi: 10.1111/j.1600-0706.2008.17116.x
Rasmussen, H. N., and Whigham, D. F. (1993). Seed ecology of dust seeds in situ: A new study technique and its application in terrestrial orchids. Am. J. Bot. 80, 1374–1378. doi: 10.1002/j.1537-2197.1993.tb15381.x
Schuiteman, A. (2014). Genera orchidacearum: Orchidoideae, Vol. 6, eds A. M. Pridgeon, P. J. Cribb, and M. W. Chase (Oxford: Oxford University Press), 51–73.
Shao, S. C., Xi, H. P., and Mohandass, D. (2019). Symbiotic mycorrhizal fungi isolated via ex situ seed baiting induce seed germination of Dendrobium catenatum lindl. (Orchidaceae). Appl. Ecol. Environ. Res. 17, 9753–9771. doi: 10.15666/aeer/1704_97539771
Shefferson, R. P., Cowden, C. C., McCormick, M. K., Yukawa, T., Ogura-Tsujita, Y., and Hashimoto, T. (2010). Evolution of host breadth in broad interactions: Mycorrhizal specificity in East Asian and North American rattlesnake plantains (Goodyera spp.) and their fungal hosts. Mol. Ecol. 19, 3008–3017. doi: 10.1111/j.1365-294X.2010.04693.x
Smith, S. E., and Read, D. J. (2008). Mycorrhizal symbiosis, 3rd Edn. San Diego, CA: Academic Press.
Stecher, G., Tamura, K., and Kumar, S. (2020). Molecular evolutionary genetics analysis (MEGA) for macOS. Mol. Biol. Evol. 37, 1237–1239. doi: 10.1093/molbev/msz312
Stewart, S. L., Zettler, L. W., Minso, J., and Brown, P. M. (2003). Symbiotic germination and reintroduction of Spiranthes brevilabris Lindley, an endangered orchid native to Florida. Selbyana 24, 64–70.
Tamura, K., Stecher, G., and Kumar, S. (2021). MEGA11: Molecular evolutionary genetics analysis version 11. Mol. Biol. Evol. 38, 3022–3027. doi: 10.1093/molbev/msab120
Teixeira da Silva, J. A., Tsavkelova, E. A., Ng, T. B., Parthibhan, S., Dobránszki, J., Cardoso, J. C., et al. (2015). Asymbiotic in vitro seed propagation of Dendrobium. Plant Cell Rep. 34, 1685–1706. doi: 10.1007/s00299-015-1829-2
Ventre Lespiaucq, A., Jacquemyn, H., Rasmussen, H. N., and Méndez, M. (2021). Temporal turnover in mycorrhizal interactions: A proof of concept with orchids. New Phytol. 230, 1690–1699. doi: 10.1111/nph.17291
Vujanovic, V., St-Arnaud, M., Barabé, D., and Thibeault, G. (2000). Viability testing of orchid seed and the promotion of colouration and germination. Ann. Bot. 86, 79–86. doi: 10.1006/anbo.2000.1162
Wang, H., Fang, H., Wang, Y., Duan, L., and Guo, S. (2011). In situ seed baiting techniques in Dendrobium officinale Kimura et Migo and Dendrobium nobile Lindl.: The endangered Chinese endemic Dendrobium (Orchidaceae). World J. Microbiol. Biotechnol. 27, 2051–2059. doi: 10.1007/s11274-011-0667-9
Wang, X. J., Wu, Y. H., Ming, X. J., Wang, G., and Gao, J. Y. (2021). Isolating ecological-specific fungi and creating fungus-seed bags for epiphytic orchid conservation. Glob. Ecol. Conserv. 28:e01714. doi: 10.1016/j.gecco.2021.e01714
White, T. J., Bruns, T., Lee, S. J. W. T., and Taylor, J. (1990). Amplification and direct sequencing of fungal ribosomal RNA genes for phylogenetics. PCR Protoc. 18, 315–322.
Wu, H. F., Song, X. Q., and Liu, H. X. (2012). Ex-situ symbiotic seed germination of Dendrobium catenatum. Acta Ecol. Sin. 32, 2491–2497.
Xing, X., Gai, X., Liu, Q., Hart, M. M., and Guo, S. (2015). Mycorrhizal fungal diversity and community composition in a lithophytic and epiphytic orchid. Mycorrhiza 25, 289–296. doi: 10.1007/s00572-014-0612-5
Xing, X., Jacquemyn, H., Gai, X., Gao, Y., Liu, Q., Zhao, Z., et al. (2019). The impact of life form on the architecture of orchid mycorrhizal networks in tropical forest. Oikos 128, 1254–1264. doi: 10.1111/oik.06363
Xing, X., Ma, X., Deng, Z., Chen, J., Wu, F., and Guo, S. (2013). Specificity and preference of mycorrhizal associations in two species of the genus Dendrobium (Orchidaceae). Mycorrhiza 23, 317–324. doi: 10.1007/s00572-012-0473-8
Xing, X., Ma, X., Men, J., Chen, Y., and Guo, S. (2017). Phylogenetic constrains on mycorrhizal specificity in eight Dendrobium (Orchidaceae) species. Sci. China Life Sci. 60, 536–544. doi: 10.1007/s11427-017-9020-1
Yokoya, K., Jacob, A. S., Zettler, L. W., Kendon, J. P., Menon, M., Bell, J., et al. (2021). Fungal diversity of selected habitat specific Cynorkis species (Orchidaceae) in the Central Highlands of Madagascar. Microorganisms 9:792. doi: 10.3390/microorganisms
Zhang, Y., Li, Y. Y., Chen, X. M., Guo, S. X., and Lee, Y. I. (2020). Effect of different mycobionts on symbiotic germination and seedling growth of Dendrobium officinale, an important medicinal orchid. Bot. Stud. 61:2. doi: 10.1186/s40529-019-0278-6
Zotz, G. (2013). The systematic distribution of vascular epiphytes - a critical update. Bot. J. Linn. Soc. 171, 453–481. doi: 10.1111/boj.12010
Keywords: lithophytes, orchid, Serendipitaceae, Tulasnellaceae, wild populations, epiphytes
Citation: Zhang L, Rammitsu K, Tetsuka K, Yukawa T and Ogura-Tsujita Y (2022) Dominant Dendrobium officinale mycorrhizal partners vary among habitats and strongly induce seed germination in vitro. Front. Ecol. Evol. 10:994641. doi: 10.3389/fevo.2022.994641
Received: 15 July 2022; Accepted: 21 September 2022;
Published: 06 October 2022.
Edited by:
Dennis Whigham, Smithsonian Institution, United StatesReviewed by:
Peter Zale, Longwood Gardens, United StatesStefania Cevallos, Universidad Técnica Particular de Loja, Ecuador
Copyright © 2022 Zhang, Rammitsu, Tetsuka, Yukawa and Ogura-Tsujita. This is an open-access article distributed under the terms of the Creative Commons Attribution License (CC BY). The use, distribution or reproduction in other forums is permitted, provided the original author(s) and the copyright owner(s) are credited and that the original publication in this journal is cited, in accordance with accepted academic practice. No use, distribution or reproduction is permitted which does not comply with these terms.
*Correspondence: Yuki Ogura-Tsujita, eXRzdWppdGFAY2Muc2FnYS11LmFjLmpw