- 1Institute for Zoology, Halle University, Halle, Germany
- 2Department of Biology, University of Oxford, Oxford, United Kingdom
- 3Department of Ecology, Evolution and Behaviour, University of Liverpool, Liverpool, United Kingdom
- 4Evolutionary Biology, Bielefeld University, Bielefeld, Germany
- 5UMR 6553, ECOBIO, Université de Rennes, Rennes, France
Reproductive ageing can occur due to the deterioration of both the soma and germline. In males, it has mostly been studied with respect to age-related changes in sperm. However, the somatic component of the ejaculate, seminal fluid, is also essential for maintaining reproductive function. Whilst we know that seminal fluid proteins (SFPs) are required for male reproductive success across diverse taxa, age-related changes in SFP quantity and composition are little understood. Additionally, only few studies have explored the reproductive ageing of the tissues that produce SFPs, and the resulting reproductive outcomes. Here we provide a systematic review of studies addressing how advancing male age affects the production and properties of seminal fluid, in particular SFPs and oxidative stress, highlighting many open questions and generating new hypotheses for further research. We additionally discuss how declines in function of different components of seminal fluid, such as SFPs and antioxidants, could contribute to age-related loss of reproductive ability. Overall, we find evidence that ageing results in increased oxidative stress in seminal fluid and a decrease in the abundance of various SFPs. These results suggest that seminal fluid contributes towards important age-related changes influencing male reproduction. Thus, it is essential to study this mostly ignored component of the ejaculate to understand male reproductive ageing, and its consequences for sexual selection and paternal age effects on offspring.
1. Introduction
Ageing is the time-dependent decline of an organism’s biological function (Monaghan et al., 2008), leading to reduced physiological abilities and ultimately death. Ageing results in numerous biological changes that include telomere shortening, accumulation of somatic mutations, loss of proteostasis, mitochondrial dysfunction, and disruption of nutrient sensing pathways (Charlesworth, 1993; Kirkwood, 2005; López-Otín et al., 2013). Organisms also tend to have a lower reproductive output at older compared to younger ages. However, the onset and rate of decline in female fertility varies considerably across taxa, depending on life-history strategies and ecologies of species (e.g., Lemaître et al., 2020b; Campos et al., 2022), making it difficult to generalise patterns of ageing across the tree of life (Jones et al., 2014).
Onset and rate of age-related biological decline and impaired reproductive function varies between males and females (Bronikowski et al., 2022). There has been a long-standing focus on females in life-history research, and studies have only recently begun to consider male reproductive ageing (e.g., Fricke and Koppik, 2019; Comizzoli and Ottinger, 2021; Archer et al., 2022). Evidence suggests that male reproductive ageing can affect male fertilizing ability (Paul and Robaire, 2013; Aich et al., 2021), influence female behavior (Dean et al., 2010; Vuarin et al., 2019), and lead to paternal effects on offspring (Daxinger and Whitelaw, 2012). Male houbara bustards (Chlamydotis undulata), for example, produce fewer progeny as they age, and sons of old fathers have greatly reduced sperm numbers (Vuarin et al., 2019, 2021). Other studies show that male ageing can lead to lower sperm quality (Gasparini et al., 2010, 2014; Velando et al., 2011; Cornwallis et al., 2014; Selvaratnam and Robaire, 2016; Monaghan and Metcalfe, 2019; Vega-Trejo et al., 2019; Turnell and Reinhardt, 2020), and quantity (Johnson et al., 2015; Sepil et al., 2020). Additionally, sperm from older males have lower success in sperm competition and fertilize fewer eggs than sperm from younger males, as seen in guppies (Poecilia reticulata, Gasparini et al., 2019), zebra fish (Danio rerio, Kanuga et al., 2011) and crickets (Acheta domesticus, Reinhardt and Siva-Jothy, 2005). Sperm ageing can also affect the quality of offspring (Gasparini et al., 2017), characterized by offspring lifespan (Xie et al., 2018; Wylde et al., 2019), telomere length (Bouwhuis et al., 2018; Noguera et al., 2018; Bauch et al., 2019), development (Preston et al., 2015), reproduction (Bouwhuis et al., 2015; Vuarin et al., 2021), and viability (Tan et al., 2013). While most studies on male ageing have focused on sperm traits, only few have tested for changes in the quality and quantity of seminal fluid with age, and its resultant fitness outcomes. Therefore, whether the reported effects of male ageing are actually driven by changes in seminal fluid rather than just sperm are yet unknown.
Ejaculated sperm are usually surrounded by a cocktail of substances collectively called the seminal fluid (Poiani, 2006; Hopkins et al., 2017). These consist of somatic cells such as immune cells; macromolecules such as carbohydrates, vitamins, minerals; hormones; and seminal fluid proteins (SFPs). Seminal fluid (SF) in most species is made in specialized accessory reproductive cells, tissues, or glands, such as the prostate, seminal vesicle, bulbourethral, and ampullary glands in humans (McGraw et al., 2015). SFPs have been shown to be especially crucial in male and female reproduction; they belong to a range of molecular classes such as antioxidants, lipases, lectins, proteases, and protease inhibitors and have been shown to have a diverse set of functions (Chapman, 2001; Avila et al., 2011; Perry et al., 2013; Ramm, 2020). For instance, SFPs facilitate normal sperm function (Wolfner, 1997), aid sperm storage and male sperm competitiveness (Fiumera et al., 2005, 2007; Goenaga et al., 2015; Patlar et al., 2020), maintain sperm viability (den Boer et al., 2008, 2009; King et al., 2011) and regulate sperm capacitation (Manjunath and Thérien, 2002). But SFPs can also act on attributes beyond sperm, for example, by affecting female reproductive behavior (Chapman et al., 2003; Liu and Kubli, 2003; Bath et al., 2017). Indeed, seminal fluid has been shown to affect female immunity modulation (Short and Lazzaro, 2010), investment in the mating partner’s male function in hermaphrodites (Nakadera et al., 2014), female egg-laying behavior (Chapman et al., 2003; Liu and Kubli, 2003), and mating plug formation to prevent female re-mating (Stockley et al., 2020).
The germline is predicted to receive higher protection from somatic ageing (Maklakov and Immler, 2016). Although seminal fluid is produced by somatic tissue it directly interacts with germ cells and thus could play an important role in facilitating interactions between somatic cells and the germline. This could have effects across the Weismann barrier (i.e., despite the germline and somatic tissue being separated early in development, changes in the soma could affect the germline or the next generation; Sciamanna et al., 2019; Bline et al., 2020). Knowing how seminal fluid changes with age and how this can influence sperm, offspring, and female physiological and behavioral responses to mating, in addition to understanding age-related changes in sperm, is essential to gain a complete picture of male reproductive ageing. Here, we first conduct a systematic review on how advancing male age influences the non-sperm components of the ejaculate (i.e., seminal fluid) across animals, and then discuss the impacts this might have on age-specific reproductive success. While the effects of male ageing on sperm have been reviewed elsewhere (e.g., Reinhardt, 2007; Pizzari et al., 2008; Monaghan and Metcalfe, 2019), to our knowledge, this is the first systematic review of how advancing male age affects seminal fluid. As studies differ greatly in their biological and methodological factors, which can modulate or confound male ageing effects, we discuss their possible influence on the conclusions that are reached.
2. Systematic review
2.1. Literature search and data collection
To understand how male age affects seminal fluid, we conducted a literature search following PRISMA eco-evo guidelines (O’Dea et al., 2021). We used a search string for abstracts, titles, and keywords “(sfp* OR seminal fluid OR seminal plasma) AND (ageing OR age OR aging OR senescence)” to identify studies which test how advancing male age affects seminal fluid, using two search engines: SCOPUS and Web of Science (WoS), on December 14th 2021, accessed through the University of Oxford server. The searches returned a total of 738 hits from WoS (year range: 1991 to 2021) and 620 from SCOPUS (year range: 1941 to 2021). After duplicate deletion, which was done using Rayyan (Ouzzani et al., 2016), we obtained a total of 970 unique papers. We then screened the abstracts of these papers using pre-defined inclusion and exclusion criteria (see below), before screening the full-texts to obtain a final list of papers from which relevant data was extracted.
To be retained for full-text screening, the paper had to be a research article (not review or meta-analysis), on any animal, and measure a seminal fluid trait for males of different ages, judged from its abstract. We excluded studies during abstract screening if they were on the wrong topic, did not compare males of different ages, did not have clear ageing data, only covered a small proportion of lifespan (e.g., only included young males), did not measure seminal fluid traits, or only measured seminal fluid during maturation of males (i.e., during juvenile or pubertal stages). The initial screening of abstracts produced a total of 94 studies whose full texts were considered in more detail.
When assessing full texts, to be included in our analysis review, a study needed to: compare males of non-overlapping age groups, compare non-sperm components of the seminal fluid (like oxidative stress enzymes, proteins, hormones, lipids, and macro- or micronutrients), report sample sizes of males in each age group and exact ages (or range of ages) to which males in each age group belonged. We excluded studies whose full texts were not available (two studies), or which were not in English (three studies). We additionally conducted a scoping search on Google Scholar to obtain additional papers which might have been missed in our systematic screening and search. This was done by using the keywords “seminal fluid protein + aging + ageing + senescence” for each of the following taxa: “bulls,” “insects,” “pigs,” “rodents,” “humans,” “birds,” “mammals,” “fish,” and searching the first five search result pages for relevant studies.
From all studies which fulfilled our inclusion criteria, we collected information on how male age affected various non-sperm characteristics of the ejaculate, as described in the paper. Additionally, we collected data on factors which could modulate the influence of seminal fluid ageing, such as: male mating history (i.e., whether males were held as virgin or not prior to testing), at which ages males were sampled, what fraction of average lifespan was covered and sampling methodology. The fraction of average lifespan covered is likely to influence whether seminal fluid ageing is detected in a study because ageing trajectories are expected to follow a non-linear pattern, with senescence being more prominent in late-adult life (e.g., Jones et al., 2014; Lemaître et al., 2020b).
Male mating history could influence the ageing of seminal fluid, such that if males are kept virgins, old males would have stored seminal fluid for longer durations, thus have more degraded SFPs and higher accumulation of oxidative damage than mated old males or virgin young males. On the other hand, old virgin males would accumulate higher quantities of SFPs than younger virgin males (Koppik et al., 2018; Sepil et al., 2020). If previously mated males are tested the quantity of seminal fluid produced would depend on the timing of the last mating, number of times the male mated in succession and its rate of replenishment, given that the abundance of SFPs within accessory tissues/glands decreases significantly immediately after a mating event (Hopkins et al., 2019a; Sepil et al., 2019). Furthermore, if mating history is not controlled for, then older males would have mated more times over their life (e.g., Aich et al., 2021), and thus have undergone more rounds of SFP replenishment and thus potentially experienced a higher turnover of the glandular tissue producing the SFP than young males.
Male sampling methodology (if samples are collected longitudinally or cross-sectionally) can also have a large impact on the study outcome. Cross-sectional sampling of males makes age-dependent individual-level deterioration in ejaculate traits with advancing age harder to detect (Nussey et al., 2013), especially if low-quality males selectively disappear (Bouwhuis et al., 2009; Hämäläinen et al., 2014). This non-random age-dependent mortality could lead to biased sampling of males, where younger age classes would have higher variance and might bias estimates of averages in seminal fluid traits compared to old age classes. Thus, cross-sectional studies might underestimate male reproductive senescence, compared to longitudinal sampling measuring the same individuals at different ages.
2.2. Summary of studies from the systematic review
Overall, we obtained data from 27 papers through our systematic searches, and seven additional papers from Google Scholar (see Supplementary Table S1 for the full list of included studies). Out of these 34 studies, 14 reported how male age affected SFPs (see Table 1), although some of these studies reported changes in total protein content, while others, changes in specific SFPs only. 10 studies reported data on oxidative damage levels or anti-oxidants present in seminal fluid (henceforth collectively called “oxidative stress,” see Table 2). Apart from these two components of the seminal fluid, a smaller fraction of studies assessed the concentration of lipids or lipoproteins (four studies), minerals/vitamins content (four studies), sugar content (two studies), or hormone concentrations (four studies) in the seminal plasma/ejaculate.
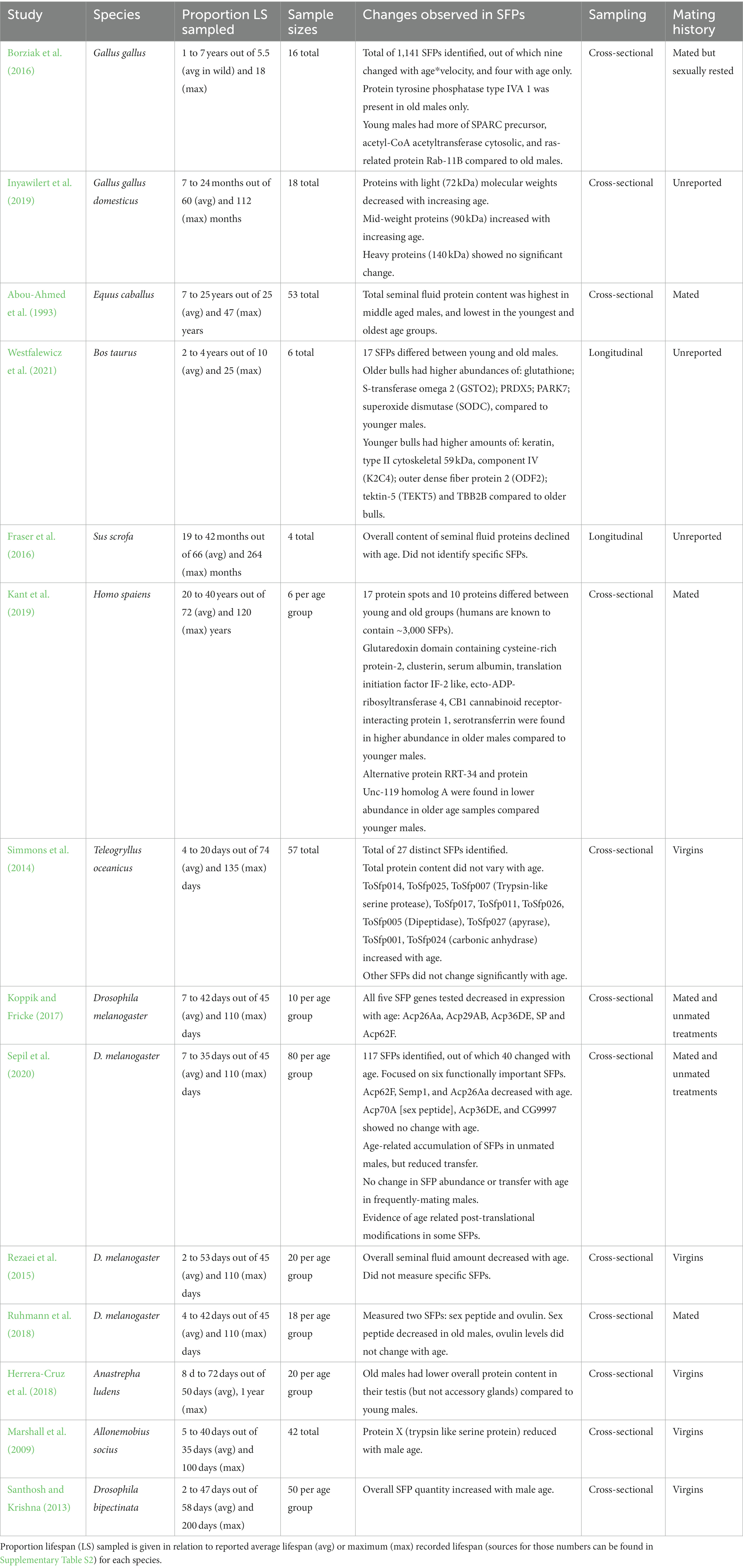
Table 1. Summary of studies testing the effect of male age on seminal fluid proteins across different taxa as found in the systematic search.
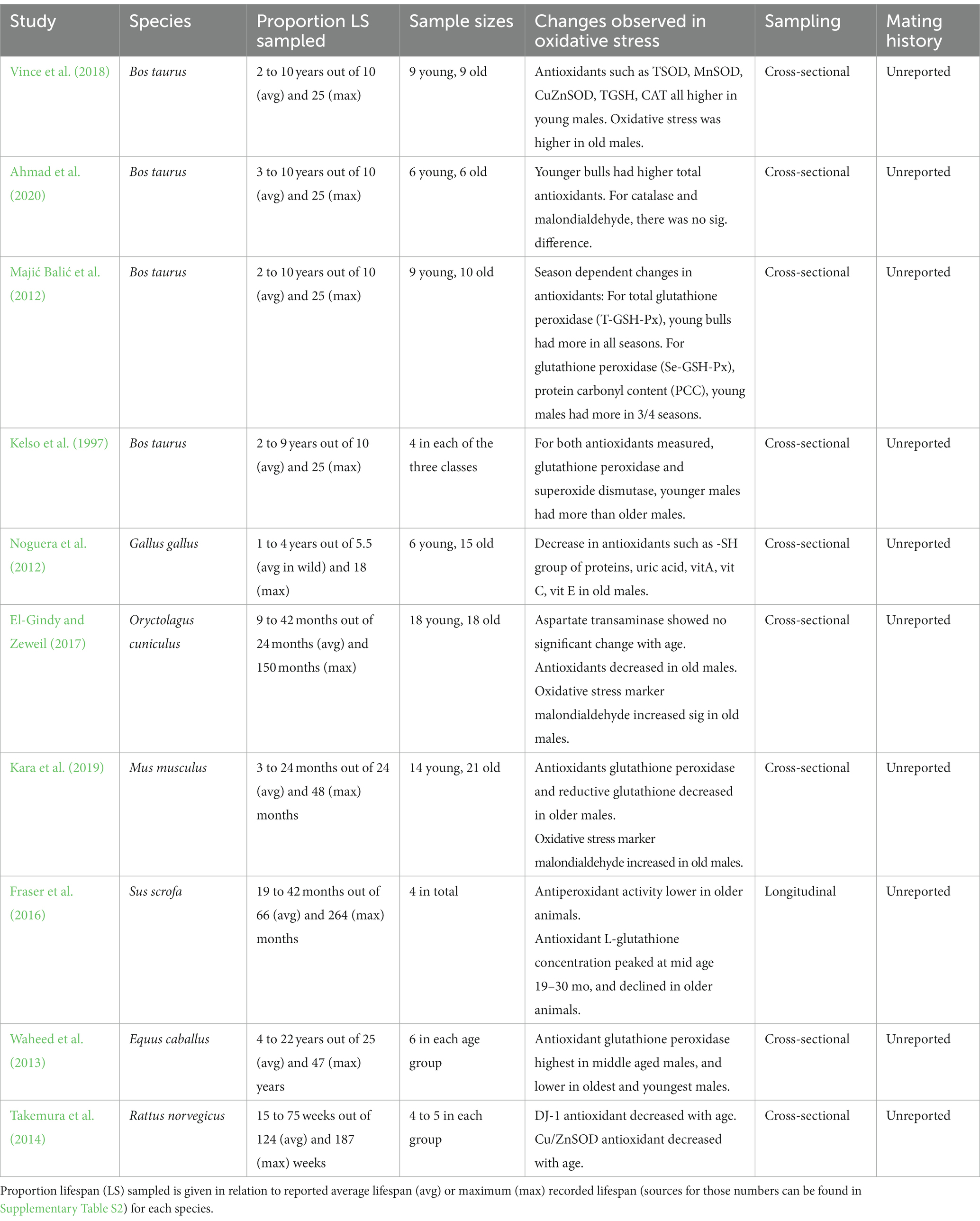
Table 2. Summary of studies found in the systematic literature search that focus on male-age dependent changes in antioxidants, oxidative stress biomarkers and reactive oxygen species in male ejaculates/seminal plasma.
The low number of studies dedicated to male age-related changes in the seminal fluid is also reflected in the limited taxonomic breadth, with a strong focus on mammals (see Figure 1). Within mammals, studies were conducted on farm animals, humans, and laboratory rodents (see Supplementary Table S1). For most studies, males were sampled up to around 80% of their average adult lifespan and 50% of their maximum adult lifespan (see Tables 1 and 2 for lifespan sampled by studies; Supplementary Table S2 for sources of lifespan measurements). Another caveat is that non-significant results might go unpublished and it is difficult to estimate this extent, though a number of studies in our set of papers report no changes with age, so we hope the bias is not strong. In the following review, we restricted our discussion to studies that tested for male age-related changes in SFPs and oxidative stress response, as these aspects of the seminal fluid were better represented compared to other ejaculatory components.
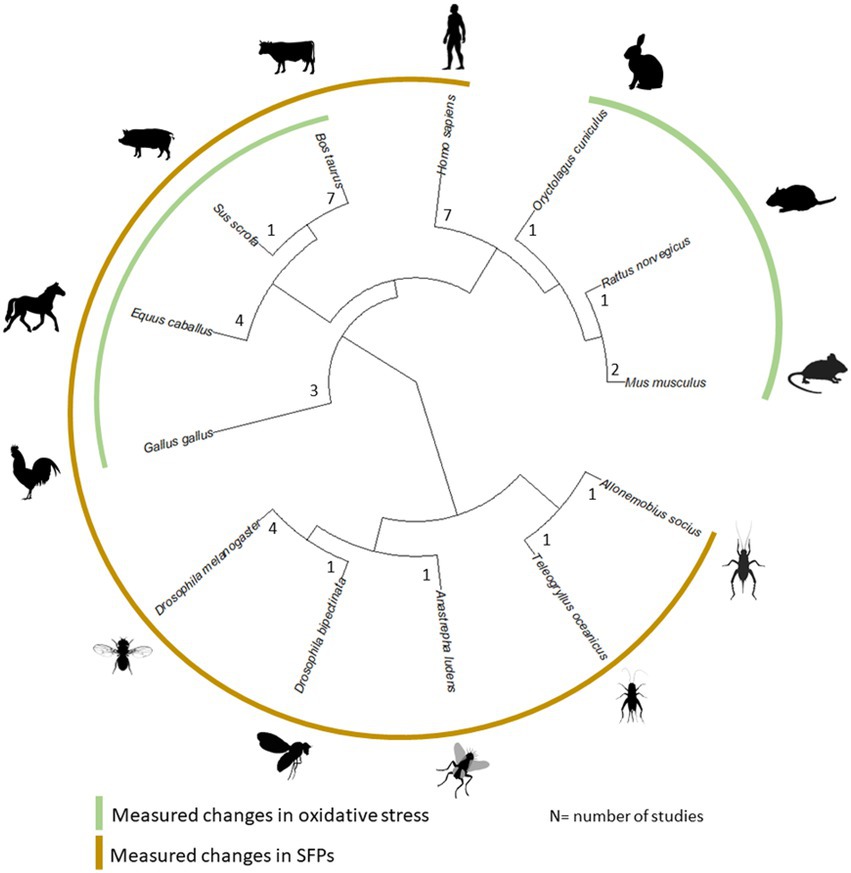
Figure 1. Phylogenetic distribution of all species in our review across 34 studies along with the number of studies on each. Species which had data reported for age-dependent changes in SFPs and oxidative stress are marked. Images to generate the figure were taken from the Open Tree of Life.
2.2.1. Age-dependent changes in SFPs
Many studies that measured accessory tissue/gland protein content found an overall decline in SFPs with male ageing (Rezaei et al., 2015; Fraser et al., 2016; Koppik et al., 2018; see also Table 1). However, this pattern becomes less clear when considering studies that quantified individual SFPs or overall compositional changes. Here, some SFPs increased (Santhosh and Krishna, 2013; Simmons et al., 2014; Borziak et al., 2016; Inyawilert et al., 2019; Kant et al., 2019; Westfalewicz et al., 2021), while others decreased in abundance (Marshall et al., 2009; Rezaei et al., 2015; Koppik and Fricke, 2017; Herrera-Cruz et al., 2018; Ruhmann et al., 2018; Sepil et al., 2020; Westfalewicz et al., 2021) with male age. Furthermore, in studies which analyzed the full proteome of the seminal fluid only a small proportion of SFPs changed with age (e.g., Sepil et al., 2020).
Methodologies differed widely between studies, ranging from estimating changes in overall SFP content to reporting individual protein changes. Generally, studies which tended to report increases in SFPs with age (e.g., in Homo sapiens, Bos taurus, and Teleogryllus oceanicus) sampled <50% of the average lifespan of the species (e.g., Simmons et al., 2014; Kant et al., 2019; Westfalewicz et al., 2021). Hence, extending sampling to cover the entire average lifespan is crucial, especially when ageing trajectories are expected to follow a non-linear pattern, with senescence being more prominent in late-adult life.
Most studies on non-human mammals did not report male mating history (virgin or mated) prior to testing. In farm animal studies, older males are likely to have been mated as part of a breeding program, although this was not always explicitly stated. For studies on insects, males were primarily kept as virgins prior to testing. It is known that in D. melanogaster, age-related changes in SFPs depend on male mating history (Koppik and Fricke, 2017; Koppik et al., 2018; Sepil et al., 2020). Old unmated males transfer a lower abundance of SFPs in a first mating relative to young males, despite having a higher abundance of SFPs in storage, whereas old frequently mated males show no change in either transfer or storage (Sepil et al., 2020). Thus, mating history has the potential to influence the results reported in studies which do not control for it. We suggest future studies should adopt a fully factorial design to test for effects of mating history on seminal fluid ageing and use young and old males both as virgin and mated males, and ideally control for mating number.
In most studies, samples were acquired from the male directly (e.g., via dissection or masturbation), but whether this correctly represents what would be transferred to females in a natural ejaculate is uncertain, especially when males have the potential for strategic ejaculation (Wedell et al., 2002). Moreover, the vast majority of studies were cross-sectional. It would be ideal to conduct longitudinal studies in species where males do not need to be sacrificed to extract their ejaculate and a large cohort of males can be followed across their lifetime.
2.2.2. Age-dependent changes in oxidative stress responses
Overall, the enzymes involved in protecting against oxidative damage decreased significantly in the seminal fluid with advancing male age (see Table 2). The three studies that measured both enzyme abundance and oxidative stress in the seminal fluid found oxidative stress markers increased in older males. Specifically, all studies which measured antioxidant content in the seminal fluid (e.g., TSOD, MnSOD, CuZnSOD, TGSH, CAT) consistently reported a decline in older males compared to younger or middle-aged males. Additionally, an oxidative stress marker was found in higher quantities in older male seminal fluid compared to younger males in two studies (El-Gindy and Zeweil, 2017; Kara et al., 2019). Notably, all these oxidative stress studies used mammals, so we cannot judge whether this is a pattern also seen in other animal groups. None of these studies reported the mating history of the males, and only one study sampled males longitudinally (Fraser et al., 2016).
3. Discussion
Here, we systematically reviewed how the non-sperm components of the ejaculate (i.e., seminal fluid) changed with male age. Sperm ageing has been a major focus of previous studies, while seminal fluid has not been studied as extensively. This is highlighted by the limited number of studies and taxa found in our systematic review, with the majority of studies either probing at age-related changes in SFPs or oxidative stress. Below, we discuss how the age-dependent changes in seminal fluid components found in our systematic review might influence male reproductive ageing, suggest some hypotheses, and discuss why the omission of seminal fluid and its associated somatic tissue is an important oversight in evolutionary and ecological research.
3.1. Seminal fluid protein ageing
We found some heterogeneity between studies in age-related SFP changes. This could be due to studies reporting quantitative changes in a set of proteins only (rather than all the proteins), or due to specific proteins responding differently to age based on their function or tissue-of-origin (Borziak et al., 2016; Sepil et al., 2020). Proteomics techniques that quantify the whole ejaculate are needed to better elucidate these biological patterns and with the advance of molecular approaches and particularly proteomics, this will become ever more feasible for a range of taxa.
Confounding factors could also explain some of the inconsistencies observed between studies. For instance, male mating history could have a large influence on SFP quantity changes as explained in the methods section above. Another caveat in comparing studies is that males are not always sampled up to old age and so an important fraction of the ageing trajectory is missed. This could be a serious bias, potentially compounded by there being stronger selection to maintain functionality earlier in life, meaning realised phenotypes may in part represent compensatory adaptive responses to the onset of seminal fluid ageing. Disentangling age-related changes from compensatory responses would require sampling beyond the 50% average lifespan into older ages, when the latter responses are expected to wane as the strength of selection declines.
While studies in our review rarely directly discuss the functional importance of the changes in observed SFPs, below, we suggest testable hypotheses for how male seminal fluid ageing might have functional consequences. Overall, studies in our review show changes in specific SFP abundances which are known to influence male fertilization success as well as a variety of female responses. For instance, older male D. melanogaster are less able to delay female remating and stimulate egg laying compared to younger males (Koppik and Fricke, 2017; Ruhmann et al., 2018; Sepil et al., 2020). Similarly in Aedes aegypti mosquitoes, older males are less able to prevent female remating (Agudelo et al., 2021). These responses are largely mediated by SFPs in D. melanogaster (Chapman et al., 2003) and the expression of functionally important SFPs declines with age (Koppik and Fricke, 2017). Therefore, it is possible that the decline in SFPs are driving the changes in female post-mating behavior. For instance, Sepil et al. (2020) found a significant age-related increase in SFP abundances in the accessory glands of unmated males, but no change in SFP abundances in the accessory glands of frequently-mated males. Yet, the authors also found that female egg laying behavior and remating affinity changed as a function of male age following matings with spermless males, hence the seminal fluid alone does contribute to the decline in reproductive function with male age. SFP transfer data can partially explain these findings. While there is no age-related decline in SFP abundances in the accessory glands, old unmated males transferred a lower quantity of SFPs to females compared to younger unmated males. There was no age-related change in the quantity of SFPs transferred to females for frequently-mated males, so it is likely that changes in SFP quality rather than quantity explain the decline in reproductive function with age in this group of males.
Apart from affecting male ability to induce female post-mating responses, age-related changes in seminal fluid might also affect sperm traits. For example, in the jungle fowl Gallus gallus, age-related changes in proteins which affect sperm velocity were detected (Borziak et al., 2016). Thus, the decreased ability of older males to gain paternity under sperm competition and fertilize eggs may be driven by changes in SFPs rather than changes in sperm per se.
While it can be difficult to pinpoint the precise changes responsible, it is becoming increasingly possible to manipulate the expression of individual SFPs to better understand how particular SFPs affect female post-mating behavior and sperm competition. For instance, using a combination of proteomics and RNAi, Marshall et al. (2009) identified a single accessory gland-derived ejaculate protein in the ground cricket Allonemobius socius that influences female egg-laying and declines in expression with male age. Hence, this protein is a prime candidate to explain the waning ability of males to induce female egg laying as the male ages. However, the link between seminal fluid expression and female responses is not necessarily straightforward. RNAi knockdown studies in other taxa have demonstrated that suppressing the expression of individual SFPs can have both positive and negative impacts on fitness-relevant traits such as female fecundity (Xu et al., 2013; Weber et al., 2019), though this may in part reflect the difficulty of measuring fitness components under realistic conditions. A further limitation of many such knockdown or knockout studies is that they tend (often of necessity) to consider only one or a few seminal fluid proteins, whereas in reality the seminal fluid proteome is a highly integrated unit whose individual components co-vary in their expression (Mohorianu et al., 2018; Patlar et al., 2019).
3.2. Effects of male age on seminal fluid quantity versus quality
In addition to changes in the abundance of individual proteins or changes to the composition of the seminal proteome, ageing can potentially impact seminal fluid through alterations to protein quality. A loss of protein homeostasis – proteostasis – is a well-known feature of ageing, characterized by a failure of chaperones, stress-response factors, and protein degradation machinery to respond to stress and prevent protein misfolding (Labbadia and Morimoto, 2014). The role of failing proteostasis in loss of SFP quality in ageing males is currently unclear but has the potential to impact ejaculate function.
Work in D. melanogaster suggests that factors other than SFP quantity may be responsible for the decline in seminal fluid-mediated functions with male age (Sepil et al., 2020). Aged males, that are known to have compromised fertility and reduced seminal fluid function, still appear capable of levels of seminal proteome production and transfer that are similar to young males (Sepil et al., 2020). However, several proteins in aged males show evidence of qualitative changes via mass shifts on Western blots (Sepil et al., 2020). While it remains to be investigated how widespread age-related changes in SFP quality are and what the functional consequences are, it nonetheless raises the possibility that a decline in SFP functionality with age is primarily related to proteostasis loss, rather than diminishing amounts of SFPs.
3.3. Ageing of seminal fluid producing reproductive tissues
While our systematic review showed general age-related declines in SFPs, how the somatic tissues which produce SFPs are affected by ageing across taxa still remains unclear. Generally, the size of prostates/accessory glands tends to increase as males grow older (Jin et al., 1996; Atalan et al., 1999; Rezaei et al., 2015; Reyes-Hernández and Pérez-Staples, 2017), but shrinkage with age was also reported in few studies (Mazeed and Mohanny, 2010; Santhosh and Krishna, 2013). However, the overall size of the organ does not necessarily predict protein content, as found in A. ludens (Herrera-Cruz et al., 2018) and D. bipectinata (Santhosh and Krishna, 2013). In humans, the increase in prostate size is known as benign prostatic hyperplasia (Berges and Oelke, 2011; Zhang et al., 2013), however prostate size varies among ethnic groups and so does the rate of change with age (i.e., Bolivian Tsimane, Trumble et al., 2015) or the occurrence of enlarged prostates in older males (Mubenga et al., 2020). Some theory predicts that the enlargement of the prostate is a side-effect of cellular hyperfunction that causes ageing of this tissue (Blagosklonny, 2021). The hyperfunction theory of ageing proposes that suboptimal nutrient-sensing molecular signaling in late-life causes ageing via excessive biosynthesis, as opposed to energy-tradeoffs (Lind et al., 2019).
3.4. Impact of male age on oxidative stress
The studies we reviewed consistently found that antioxidant quantity in the seminal fluid decreases with increasing male age, while oxidative stress markers tend to increase in the seminal fluid as males age. Reactive oxygen species (ROS) are unstable, free radical compounds and are required for vital cellular processes (Finkel and Holbrook, 2000; Hajam et al., 2022), but can also be deleterious to cells. For instance ROS play a role in sperm activation and changing sperm motility, e.g., in humans (Aitken et al., 2022) with the potential to influence male reproduction (Mannucci et al., 2022). However, work in D. melanogaster shows that while older males have higher metabolic rates in their sperm, ROS production is actually lower in these sperm (Turnell and Reinhardt, 2020).
Antioxidants, on the other hand, play a key role in stabilizing free radicals generated as part of cellular processes (Hood et al., 2019), and an imbalance between antioxidants and ROS causes oxidative stress. Oxidative stress has been shown to influence sperm homeostasis and can cause sperm DNA damage thus affecting male fertility (Mannucci et al., 2022) and has been shown to be key in regulating various intracellular pathways related to sperm, and activation of various sperm transcription factors (Aitken and Baker, 2006; Sabeti et al., 2016; Aitken, 2017). Our review suggests that older males have lower antioxidant levels but higher oxidative stress markers in their seminal fluid, and thus may have higher oxidative stress than young males. The decline in antioxidants might indicate a tradeoff where ageing males cannot maintain optimal antioxidant levels if these are energetically costly.
The mechanisms for why older males have higher oxidative stress could be several. For instance, ROS from sperm could “leak” into seminal fluid or somatic cells which produce SF could accumulate more ROS damage over time in old versus young males. This increase in oxidative stress in older males could have severe hypothesized functional consequences, such as higher oxidative damage to sperm, or the fertilized egg, and reduced sperm performance, which can be tested by future studies. More studies are needed to disentangle the origin/cause of age-dependent changes in ROS production in seminal fluid, the consequences of scavenging by SF antioxidants, and the overall effects on sperm, male and female reproduction.
3.5. Factors that could influence seminal fluid ageing rates
Studies identified in our systematic literature review included only a few factors such as proportional lifespan sampled, male mating history and sampling of males to explain differences in seminal fluid ageing.
Besides these, other factors could be predicted to influence SF ageing. For instance, evidence for reproductive ageing has been shown to be stronger in laboratory and captive animals compared to wild ones (Nussey et al., 2013; Zajitschek et al., 2020; Kappeler et al., 2022). Additionally, domestic animals, which were used in a majority of studies found in our systematic review, are kept in semi-controlled conditions and are killed off prior to reaching a senescent age (i.e., post their “prime”). Thus, evidence for reproductive senescence in the seminal fluid may be weaker in domestic animals, although in our review, we found evidence for seminal fluid senescence in both lab and domestic animals.
Other abiotic and biotic factors could influence seminal fluid ageing, such as a male’s social environment. Both sperm and seminal fluid are highly plastic in their expression (reviewed in Perry et al., 2013; and Ramm, 2020). Males are known to invest more in seminal fluid production under more competitive environments, such as under high sperm competition (Hopkins et al., 2019b), possibly at the cost of reduced later-life investment in reproduction (Lemaître et al., 2020a). The costs of ejaculate plasticity have been discussed before (see, e.g., Ramm, 2020), but to our knowledge have not been tested, and whether these costs differ for old versus young males can be investigated in the future. Knowledge of costs could be one factor predicting ageing trajectories of seminal fluid. If seminal fluid production is costly and its continued production causes damage, then strong selection on early reproduction might be favoured and we would expect rapid ageing as a consequence. However, if seminal fluid production is cheap then factors such a sperm competition, male dominance and/ or female preferences might have more scope to shape ageing patterns. For example if old males are socially dominant and preferred by females they might face little competition and selection on seminal fluid is relaxed and thus ageing might arise. Conversely, if older males are more likely to experience sperm competition or female ejaculate rejection then there might be relatively high selection for seminal fluid competency late in life. Additionally, sperm production patterns, i.e., continuously versus one bout early in life, and whether sperm is the limiting factor might be important too, because supply and demand needs to be balanced between these different components through reproductive lifespan. To test these ideas knowledge of ejaculate ageing across a broad range of taxa with different reproductive patterns is necessary.
Mating systems can also influence ageing of seminal fluid. Ageing effects are expected to be more pronounced in polyandrous species where males are likely to invest more in their ejaculates (Veltsos et al., 2022), due to facing a higher risk of sperm competition. However, the influence of sperm competition on male reproductive senescence likely depends on the life-history of a species. For example, in some species, males may preferentially invest resources in producing more SFPs early in life, and suffer faster rates of reproductive senescence later in life (see also Lemaître et al., 2020a). Older males in such species are often inferior in both pre- and postcopulatory competition (Johnson and Gemmell, 2012; Gasparini et al., 2019) and are discriminated against by females (Velando et al., 2011; Rezaei et al., 2015). Alternatively, species with increased levels of sperm competition may evolve increased investment in SF (Immler et al., 2011; Lüpold et al., 2020), which may reduce the rate of senescence in these ejaculate traits (Delbarco-Trillo et al., 2018).
Abiotic factors such as nutrition could also impact the trajectory of ageing of the seminal fluid. Studies on male rats showed that both over- and undernutrition during pregnancy seem to lead to premature male reproductive ageing (reviewed in Zambrano et al., 2021). This is because, at least in mammals, the early stages of development have an overall impact on health and quality of life during adulthood (developmental programming) with endocrine disruptors and maternal nutrition impacting developmental programming.
3.6. Male ageing effects on offspring fitness via seminal fluid
The impact of male age is not limited to his own and his mates’ reproductive success, but potentially extends to offspring fitness as well. Males are known to influence the fitness of their offspring through mechanisms other than the transmission of DNA (Curley et al., 2011; Crean and Bonduriansky, 2014). Advanced paternal age has been shown to shorten offspring lifespan, exacerbate ageing-related pathology and to alter offspring social behavior (Kong et al., 2012; Brenman-Suttner et al., 2018; Xie et al., 2018). Classically, these impacts were believed to be due to the accumulation of de novo mutations in ageing germ cells. However, recent work suggests that non-genetic mechanisms, such as changes in methylation patterns or small non-coding RNA populations, are more likely to drive the intergenerational effects of ageing (Xie et al., 2018). Importantly, it was recently suggested that seminal fluid might be an under-appreciated mediator of paternal effects (Simmons, 2011; Watkins et al., 2018; Evans et al., 2019; Simmons and Lovegrove, 2019, 2020; Kekäläinen et al., 2020), yet this has not yet been tested in a paternal ageing context.
4. Conclusion
Despite the low number of studies found, our review is crucial in highlighting the gaps in our knowledge of seminal fluid ageing. Our review generates hypotheses on how ageing of seminal fluid could affect male and female fitness, and makes predictions for how various biological and methodological factors could modulate the effects of seminal fluid ageing. It further shows that ageing impacts the level of oxidative stress in the seminal fluid, and to some extent the abundance of SFPs in the ejaculate. We highlight how the age-dependent changes observed in the seminal fluid profile can affect male fitness. Additionally, we find that male ageing can alter expression or abundance of specific SFPs that regulate female post-mating behavior (Koppik and Fricke, 2017; Sepil et al., 2020), oviposition rate (Marshall et al., 2009), male sperm competition (Ruhmann et al., 2018; Sepil et al., 2020), response to oxidative stress (Kant et al., 2019; Westfalewicz et al., 2021), immune and antimicrobial function (Borziak et al., 2016), and sperm velocity (Borziak et al., 2016). Most research to date has been done on mammals and insects, specifically on species important for animal husbandry or biomedicine. Hence, broadening the taxonomic spread of future studies in general, and the inclusion of species with different mating systems in particular should be a priority.
We highlight how understanding reproductive ageing of sperm, but also of the seminal fluid and the tissues producing them can provide a better picture of male reproductive ageing. Any future research agenda must therefore include a more focused assessment of the downstream consequences of seminal fluid ageing on fitness-related traits, encompassing impacts on fertility, sperm competitive ability and effects on the resulting offspring. Future work should ideally study the non-sperm ejaculate components as a whole, together with changes in sperm as this will be key to advance our understanding of male reproductive ageing.
Author contributions
All authors listed have made a substantial, direct, and intellectual contribution to the work and approved it for publication.
Funding
SR’s research on seminal fluids is supported by the Deutsche Forschungsgemeinschaft (DFG, project no. 448589387), while CF is supported by the Heisenberg-Programm (DFG, FR 2973/11-1) and IL by DFG grant (FR 2973/10-1 to CF). IS was supported by a Biotechnology and Biological Sciences Research Council (BBSRC) Fellowship (grant no. BB/T008881/1), a Royal Society Dorothy Hodgkin Fellowship (grant no. DHF\R1\211084), and a Wellcome Institutional Strategic Support Fund, University of Oxford (grant no. BRR00060). SW is supported by the BBSRC (grant no. BB/V015249/1) and ME received support from the MRC (project no. 2600902). Open access fees were covered by the publication fund at the University Library MLU Halle-Wittenberg.
Conflict of interest
The authors declare that the research was conducted in the absence of any commercial or financial relationships that could be construed as a potential conflict of interest.
Publisher’s note
All claims expressed in this article are solely those of the authors and do not necessarily represent those of their affiliated organizations, or those of the publisher, the editors and the reviewers. Any product that may be evaluated in this article, or claim that may be made by its manufacturer, is not guaranteed or endorsed by the publisher.
Supplementary material
The Supplementary material for this article can be found online at: https://www.frontiersin.org/articles/10.3389/fevo.2023.1066022/full#supplementary-material
References
Abou-Ahmed, M. M., El-Belely, M. S., Ismail, S. T., El-Baghdady, Y. R. M., and Hemeida, N. A. (1993). Influence of age and season on certain biochemical constituents of seminal plasma of Arabian horses. Anim. Reprod. Sci. 32, 237–244.
Agudelo, J., Alfonso-Parra, C., and Avila, F. W. (2021). Male age influences re-mating incidence and sperm use in females of the dengue vector Aedes aegypti. Front. Physiol. 12:691221. doi: 10.3389/fphys.2021.691221
Ahmad, I., Ahmad, I., Ahmad, N., Qureshi, Z., Jamil, H., and Rahman, Z. (2020). Determination of physiological biomarkers in the semen and peripheral blood of sahiwal bulls of two age groups. Pak. J. Agric. Sci. 57, 263–268. doi: 10.21162/PAKJAS/20.7470
Aich, U., Head, M. L., Fox, R. J., and Jennions, M. D. (2021). Male age alone predicts paternity success under sperm competition when effects of age and past mating effort are experimentally separated. Proc. R. Soc. B Biol. Sci. 288:20210979. doi: 10.1098/rspb.2021.0979
Aitken, R. J. (2017). Reactive oxygen species as mediators of sperm capacitation and pathological damage. Mol. Reprod. Dev. 84, 1039–1052. doi: 10.1002/mrd.22871
Aitken, R. J., and Baker, M. A. (2006). Oxidative stress, sperm survival and fertility control. Mol. Cell. Endocrinol. 250, 66–69. doi: 10.1016/j.mce.2005.12.026
Aitken, R. J., Drevet, J. R., Moazamian, A., and Gharagozloo, P. (2022). Male infertility and oxidative stress: a focus on the underlying mechanisms. Antioxidants 11:306. doi: 10.3390/antiox11020306
Archer, C. R., Paniw, M., Vega-Trejo, R., and Sepil, I. (2022). A sex skew in life-history research: the problem of missing males. Proc. R. Soc. B Biol. Sci. 289:20221117. doi: 10.1098/rspb.2022.1117
Atalan, G., Holt, P. E., and Barr, F. J. (1999). Ultrasonographic estimation of prostate size in normal dogs and relationship to bodyweight and age. J. Small Anim. Pract. 40, 119–122. doi: 10.1111/j.1748-5827.1999.tb03052.x
Avila, F. W., Sirot, L. K., LaFlamme, B. A., Rubinstein, C. D., and Wolfner, M. F. (2011). Insect seminal fluid proteins: identification and function. Annu. Rev. Entomol. 56, 21–40. doi: 10.1146/annurev-ento-120709-144823
Bath, E., Bowden, S., Peters, C., Reddy, A., Tobias, J. A., Easton-Calabria, E., et al. (2017). Sperm and sex peptide stimulate aggression in female Drosophila. Nat. Ecol. Evol. 1:0154. doi: 10.1038/s41559-017-0154
Bauch, C., Boonekamp, J. J., Korsten, P., Mulder, E., and Verhulst, S. (2019). Epigenetic inheritance of telomere length in wild birds. PLoS Genet. 15:e1007827. doi: 10.1371/journal.pgen.1007827
Berges, R., and Oelke, M. (2011). Age-stratified normal values for prostate volume, PSA, maximum urinary flow rate, IPSS, and other LUTS/BPH indicators in the German male community-dwelling population aged 50 years or older. World J. Urol. 29, 171–178. doi: 10.1007/s00345-010-0638-z
Blagosklonny, M. V. (2021). The hyperfunction theory of aging: three common misconceptions. Onco. Targets. Ther. 8, 103–107. doi: 10.18632/oncoscience.545
Bline, A. P., Le Goff, A., and Allard, P. (2020). What is lost in the Weismann barrier? J. Dev. Biol. 8:35. doi: 10.3390/jdb8040035
Borziak, K., Álvarez-Fernández, A., Karr, T. L., Pizzari, T., and Dorus, S. (2016). The seminal fluid proteome of the polyandrous red junglefowl offers insights into the molecular basis of fertility, reproductive ageing and domestication. Sci. Rep. 6:35864. doi: 10.1038/srep35864
Bouwhuis, S., Sheldon, B. C., Verhulst, S., and Charmantier, A. (2009). Great tits growing old: selective disappearance and the partitioning of senescence to stages within the breeding cycle. Proc. R. Soc. B 276, 2769–2777. doi: 10.1098/rspb.2009.0457
Bouwhuis, S., Vedder, O., and Becker, P. H. (2015). Sex-specific pathways of parental age effects on offspring lifetime reproductive success in a long-lived seabird: sex-specific parental effects on offspring fitness. Evolution 69, 1760–1771. doi: 10.1111/evo.12692
Bouwhuis, S., Verhulst, S., Bauch, C., and Vedder, O. (2018). Reduced telomere length in offspring of old fathers in a long-lived seabird. Biol. Lett. 14:20180213. doi: 10.1098/rsbl.2018.0213
Brenman-Suttner, D. B., Long, S. Q., Kamesan, V., de Belle, J. N., Yost, R. T., Kanippayoor, R. L., et al. (2018). Progeny of old parents have increased social space in Drosophila melanogaster. Sci. Rep. 8:3673. doi: 10.1038/s41598-018-21731-0
Bronikowski, A. M., Meisel, R. P., Biga, P. R., Walters, J. R., Mank, J. E., Larschan, E., et al. (2022). Sex-specific aging in animals: perspective and future directions. Aging Cell 21:e13542. doi: 10.1111/acel.13542
Campos, F. A., Altmann, J., Cords, M., Fedigan, L. M., Lawler, R., Lonsdorf, E. V., et al. (2022). Female reproductive aging in seven primate species: patterns and consequences. Proc. Natl. Acad. Sci. 119:e2117669119. doi: 10.1073/pnas.2117669119
Chapman, T. (2001). Seminal fluid-mediated fitness traits in Drosophila. Heredity 87, 511–521. doi: 10.1046/j.1365-2540.2001.00961.x
Chapman, T., Bangham, J., Vinti, G., Seifried, B., Lung, O., Wolfner, M. F., et al. (2003). The sex peptide of Drosophila melanogaster: female post-mating responses analyzed by using RNA interference. Proc. Natl. Acad. Sci. U. S. A. 100, 9923–9928. doi: 10.1073/pnas.1631635100
Charlesworth, B. (1993). Evolutionary mechanisms of senescence. Genetica 91, 11–19. doi: 10.1007/BF01435984
Comizzoli, P., and Ottinger, M. A. (2021). Understanding reproductive aging in wildlife to improve animal conservation and human reproductive health. Front. Cell Dev. Biol. 9, 1–8. doi: 10.3389/fcell.2021.680471
Cornwallis, C. K., Dean, R., and Pizzari, T. (2014). Sex-specific patterns of aging in sexual ornaments and gametes. Am. Nat. 184, E66–E78. doi: 10.1086/677385
Crean, A. J., and Bonduriansky, R. (2014). What is a paternal effect? Trends Ecol. Evol. 29, 554–559. doi: 10.1016/j.tree.2014.07.009
Curley, J. P., Mashoodh, R., and Champagne, F. A. (2011). Epigenetics and the origins of paternal effects. Horm. Behav. 59, 306–314. doi: 10.1016/j.yhbeh.2010.06.018
Daxinger, L., and Whitelaw, E. (2012). Understanding transgenerational epigenetic inheritance via the gametes in mammals. Nat. Rev. Genet. 13, 153–162. doi: 10.1038/nrg3188
Dean, R., Cornwallis, C. K., Løvlie, H., Worley, K., Richardson, D. S., and Pizzari, T. (2010). Male reproductive senescence causes potential for sexual conflict over mating. Curr. Biol. 20, 1192–1196. doi: 10.1016/j.cub.2010.04.059
Delbarco-Trillo, J., Tourmente, M., Varea-Sánchez,, and Roldan, E. R. S. (2018). Is male reproductive senescence minimized in Mus species with high levels of sperm competition? Biol. J. Linn. Soc. 123, 463–470. doi: 10.1093/biolinnean/blx146
den Boer, S. P. A., Boomsma, J. J., and Baer, B. (2008). Seminal fluid enhances sperm viability in the leafcutter ant Atta colombica. Behav. Ecol. Sociobiol. 62, 1843–1849. doi: 10.1007/s00265-008-0613-5
den Boer, S. P. A., Boomsma, J. J., and Baer, B. (2009). Honey bee males and queens use glandular secretions to enhance sperm viability before and after storage. J. Insect Physiol. 55, 538–543. doi: 10.1016/j.jinsphys.2009.01.012
El-Gindy, Y. M., and Zeweil, H. S. (2017). Effects of parsley supplementation on the seminal quality, blood lipid profile and oxidant status of young and old male rabbits. World Rabbit Sci. 25:215. doi: 10.4995/wrs.2017.6532
Evans, J. P., Wilson, A. J., Pilastro, A., and Garcia-Gonzalez, F. (2019). Ejaculate-mediated paternal effects: evidence, mechanisms and evolutionary implications. Reproduction 157, R109–R126. doi: 10.1530/REP-18-0524
Finkel, T., and Holbrook, N. J. (2000). Oxidants, oxidative stress and the biology of ageing. Nature 408, 239–247. doi: 10.1038/35041687
Fiumera, A. C., Dumont, B. L., and Clark, A. G. (2005). Sperm competitive ability in Drosophila melanogaster associated with variation in male reproductive proteins. Genetics 169, 243–257. doi: 10.1534/genetics.104.032870
Fiumera, A. C., Dumont, B. L., and Clark, A. G. (2007). Associations between sperm competition and natural variation in male reproductive genes on the third chromosome of Drosophila melanogaster. Genetics 176, 1245–1260. doi: 10.1534/genetics.106.064915
Fraser, L., Strzeżek, J., Filipowicz, K., Mogielnicka-Brzozowska, M., and Zasiadczyk, L. (2016). Age and seasonal-dependent variations in the biochemical composition of boar semen. Theriogenology 86, 806–816. doi: 10.1016/j.theriogenology.2016.02.035
Fricke, C., and Koppik, M. (2019). Male reproductive ageing: a tale of the whole ejaculate. Reproduction 158, R219–R229. doi: 10.1530/REP-18-0579
Gasparini, C., Devigili, A., and Pilastro, A. (2019). Sexual selection and ageing: interplay between pre- and post-copulatory traits senescence in the guppy. Proc. R. Soc. B Biol. Sci. 286:20182873. doi: 10.1098/rspb.2018.2873
Gasparini, C., Dosselli, R., and Evans, J. P. (2017). Sperm storage by males causes changes in sperm phenotype and influences the reproductive fitness of males and their sons. Evol. Lett. 1, 16–25. doi: 10.1002/evl3.2
Gasparini, C., Kelley, J. L., and Evans, J. P. (2014). Male sperm storage compromises sperm motility in guppies. Biol. Lett. 10:20140681. doi: 10.1098/rsbl.2014.0681
Gasparini, C., Marino, I. A. M., Boschetto, C., and Pilastro, A. (2010). Effect of male age on sperm traits and sperm competition success in the guppy (Poecilia reticulata). J. Evol. Biol. 23, 124–135. doi: 10.1111/j.1420-9101.2009.01889.x
Goenaga, J., Yamane, T., Rönn, J., and Arnqvist, G. (2015). Within-species divergence in the seminal fluid proteome and its effect on male and female reproduction in a beetle. BMC Evol. Biol. 15:266. doi: 10.1186/s12862-015-0547-2
Hajam, Y. A., Rani, R., Ganie, S. Y., Sheikh, T. A., Javaid, D., Qadri, S. S., et al. (2022). Oxidative stress in human pathology and aging: molecular mechanisms and perspectives. Cells 11:552. doi: 10.3390/cells11030552
Hämäläinen, A., Dammhahn, M., Aujard, F., Eberle, M., Hardy, I., Kappeler, P., et al. (2014). Senescence or selective disappearance? Age trajectories of body mass in wild and captive populations of a small-bodied primate. Proc. R. Soc. B. 281:20140830. doi: 10.1098/rspb.2014.0830
Herrera-Cruz, M., Abraham, S., Nuñez-Beverido, N., Flores-Estévez, N., Reyes-Hernández, M., Alvarado, M., et al. (2018). Male age and strain affect ejaculate quality in the Mexican fruit fly: age and strain affect Mexican fly ejaculates. Insect Sci. 25, 703–711. doi: 10.1111/1744-7917.12446
Hood, W. R., Williams, A. S., and Hill, G. E. (2019). An Ecologist’s guide to mitochondrial DNA mutations and senescence. Integr. Comp. Biol. 59, 970–982. doi: 10.1093/icb/icz097
Hopkins, B. R., Sepil, I., Bonham, S., Miller, T., Charles, P. D., Fischer, R., et al. (2019a). BMP signaling inhibition in Drosophila secondary cells remodels the seminal proteome and self and rival ejaculate functions. Proc. Natl. Acad. Sci. U. S. A. 116, 24719–24728. doi: 10.1073/pnas.1914491116
Hopkins, B. R., Sepil, I., Thézénas, M.-L., Craig, J. F., Miller, T., Charles, P. D., et al. (2019b). Divergent allocation of sperm and the seminal proteome along a competition gradient in Drosophila melanogaster. Proc. Natl. Acad. Sci. 116, 17925–17933. doi: 10.1073/pnas.1906149116
Hopkins, B. R., Sepil, I., and Wigby, S. (2017). Seminal fluid. Curr. Biol. 27, R404–R405. doi: 10.1016/j.cub.2017.03.063
Immler, S., Pitnick, S., Parker, G. A., and Birkhead, T. R. (2011). Resolving variation in the reproductive tradeoff between sperm size and number. PNAS 108, 5325–5330. doi: 10.1073/pnas.100905910
Inyawilert, W., Rungruangsak, J., Chanthi, S., Liao, Y., Phinyo, M., Tang, P., et al. (2019). Age-related difference changes semen quality and seminal plasma protein patterns of Thai native rooster. Int. J. Agric. Technol. 15, 287–296.
Jin, B., Turner, L., Crawford, B., Birrell, A., and Handelsman, D. J. (1996). The development of the baboon prostate: ultrasound methodology, modelling, and natural history. J. Androl. 17, 342–352.
Johnson, S. L., Dunleavy, J., Gemmell, N. J., and Nakagawa, S. (2015). Consistent age-dependent declines in human semen quality: a systematic review and meta-analysis. Ageing Res. Rev. 19, 22–33. doi: 10.1016/j.arr.2014.10.007
Johnson, S. L., and Gemmell, N. J. (2012). Are old males still good males and can females tell the difference?: do hidden advantages of mating with old males off-set costs related to fertility, or are we missing something else. BioEssays 34, 609–619. doi: 10.1002/bies.201100157
Jones, O. R., Scheuerlein, A., Salguero-Gómez, R., Camarda, C. G., Schaible, R., Casper, B. B., et al. (2014). Diversity of ageing across the tree of life. Nature 505, 169–173. doi: 10.1038/nature12789
Kant, K., Tomar, A. K., Singh, S., and Yadav, S. (2019). Ageing associated proteomic variations in seminal plasma of Indian men. J. Proteins Proteomics 10, 83–89. doi: 10.1007/s42485-019-00013-x
Kanuga, M. K., Benner, M. J., Doble, J. A., Wilson-Leedy, J. G., Robison, B. D., and Ingermann, R. L. (2011). Effect of aging on male reproduction in zebrafish (Danio rerio). J. Exp. Zool. Part Ecol. Genet. Physiol. 315A, 156–161. doi: 10.1002/jez.661
Kappeler, P. M., Pethig, L., Prox, L., and Fichtel, C. (2022). Reproductive senescence in two lemur lineages. Front. Ecol. Evol. 10:894344. doi: 10.3389/fevo.2022.894344
Kara, H., Orem, A., Yulug, E., Yucesan, F. B., Kerimoglu, G., Yaman, S. O., et al. (2019). Hazelnut consumption improves testicular antioxidant function and semen quality in young and old male rats. Food Chem. 294, 1–8. doi: 10.1016/j.foodchem.2019.04.087
Kekäläinen, J., Jokiniemi, A., Janhunen, M., and Huuskonen, H. (2020). Offspring phenotype is shaped by the nonsperm fraction of semen. J. Evol. Biol. 33, 584–594. doi: 10.1111/jeb.13592
Kelso, K. A., Redpath, A., Noble, R. C., and Speake, B. K. (1997). Lipid and antioxidant changes in spermatozoa and seminal plasma throughout the reproductive period of bulls. Reproduction 109, 1–6. doi: 10.1530/jrf.0.1090001
King, M., Eubel, H., Millar, A. H., and Baer, B. (2011). Proteins within the seminal fluid are crucial to keep sperm viable in the honeybee Apis mellifera. J. Insect Physiol. 57, 409–414. doi: 10.1016/j.jinsphys.2010.12.011
Kirkwood, T. B. L. (2005). Understanding the odd science of aging. Cells 120, 437–447. doi: 10.1016/j.cell.2005.01.027
Kong, A., Frigge, M. L., Masson, G., Besenbacher, S., Sulem, P., Magnusson, G., et al. (2012). Rate of de novo mutations and the importance of father’s age to disease risk. Nature 488, 471–475. doi: 10.1038/nature11396
Koppik, M., and Fricke, C. (2017). Gene expression changes in male accessory glands during ageing are accompanied by reproductive decline in Drosophila melanogaster. Mol. Ecol. 26, 6704–6716. doi: 10.1111/mec.14384
Koppik, M., Ruhmann, H., and Fricke, C. (2018). The effect of mating history on male reproductive ageing in Drosophila melanogaster. J. Insect Physiol. 111, 16–24. doi: 10.1016/j.jinsphys.2018.10.003
Labbadia, J., and Morimoto, R. I. (2014). Proteostasis and longevity: when does aging really begin? F1000Prime Rep. 6. doi: 10.12703/P6-07
Lemaître, J. F., Gaillard, J. M., and Ramm, S. A. (2020a). The hidden ageing costs of sperm competition. Ecol. Lett. 23, 1573–1588. doi: 10.1111/ele.13593
Lemaître, J.-F., Ronget, V., and Gaillard, J.-M. (2020b). Female reproductive senescence across mammals: a high diversity of patterns modulated by life history and mating traits. Mech. Ageing Dev. 192:111377. doi: 10.1016/j.mad.2020.111377
Lind, M. I., Ravindran, S., Sekajova, Z., Carlsson, H., Hinas, A., and Maklakov, A. A. (2019). Experimentally reduced insulin/IGF-1 signaling in adulthood extends lifespan of parents and improves Darwinian fitness of their offspring. Evol. Lett. 3, 207–216. doi: 10.1002/evl3.108
Liu, H., and Kubli, E. (2003). Sex-peptide is the molecular basis of the sperm effect in Drosophila melanogaster. Proc. Natl. Acad. Sci. U. S. A. 100, 9929–9933. doi: 10.1073/pnas.1631700100
López-Otín, C., Blasco, M. A., Partridge, L., Serrano, M., and Kroemer, G. (2013). The hallmarks of aging. Cells 153, 1194–1217. doi: 10.1016/j.cell.2013.05.039
Lüpold, S., de Boer, R. A., Evans, J. P., Tomkins, J. L., and Fitzpatrick, J. L. (2013). How sperm competition shapes the evolution of testes and sperm: a meta-analysis. Phil. Trans. R. Soc. B 375:20200064. doi: 10.1098/rstb.2020.0064
Majić Balić, I., Milinković-Tur, S., Samardžija, M., and Vince, S. (2012). Effect of age and environmental factors on semen quality, glutathione peroxidase activity and oxidative parameters in simmental bulls. Theriogenology 78, 423–431. doi: 10.1016/j.theriogenology.2012.02.022
Maklakov, A. A., and Immler, S. (2016). The expensive germline and the evolution of ageing. Curr. Biol. 26, R577–R586. doi: 10.1016/j.cub.2016.04.012
Manjunath, P., and Thérien, I. (2002). Role of seminal plasma phospholipid-binding proteins in sperm membrane lipid modification that occurs during capacitation. J. Reprod. Immunol. 53, 109–119. doi: 10.1016/S0165-0378(01)00098-5
Mannucci, A., Argento, F. R., Fini, E., Coccia, M. E., Taddei, N., Becatti, M., et al. (2022). The impact of oxidative stress in male infertility. Front. Mol. Biosci. 8:799294. doi: 10.3389/fmolb.2021.799294
Marshall, J. L., Huestis, D. L., Hiromasa, Y., Wheeler, S., Oppert, C., Marshall, S. A., et al. (2009). Identification, RNAi knockdown, and functional analysis of an ejaculate protein that mediates a Postmating, prezygotic phenotype in a cricket. PLoS One 4:e7537. doi: 10.1371/journal.pone.0007537
Mazeed, A. M., and Mohanny, K. M. (2010). Some reproductive characteristics of honeybee drones in relation to their ages: reproductive characters of honeybee drones. Entomol. Res. 40, 245–250. doi: 10.1111/j.1748-5967.2010.00297.x
McGraw, L. A., Suarez, S. S., and Wolfner, M. F. (2015). On a matter of seminal importance: insights & perspectives. BioEssays 37, 142–147. doi: 10.1002/bies.201400117
Mohorianu, I., Fowler, E. K., Dalmay, T., and Chapman, T. (2018). Control of seminal fluid protein expression via regulatory hubs in Drosophila melanogaster. Proc. R. Soc. B Biol. Sci. 285:20181681. doi: 10.1098/rspb.2018.1681
Monaghan, P., Charmantier, A., Nussey, D. H., and Ricklefs, R. E. (2008). The evolutionary ecology of senescence. Funct. Ecol. 22, 371–378. doi: 10.1111/j.1365-2435.2008.01418.x
Monaghan, P., and Metcalfe, N. B. (2019). The deteriorating soma and the indispensable germline: gamete senescence and offspring fitness. Proc. R. Soc. B Biol. Sci. 286:20192187. doi: 10.1098/rspb.2019.2187
Mubenga, L. E., Hermans, M. P., Chimanuka, D., Muhindo, L., Bwenge, E., and Tombal, B. (2020). Prostate volume and its relationship with anthropometric variables among different ethnic groups of South-Kivu. DR Congo. Afr. J. Urol. 26:32. doi: 10.1186/s12301-020-00040-x
Nakadera, Y., Swart, E. M., Hoffer, J. N. A., den Boon, O., Ellers, J., and Koene, J. M. (2014). Receipt of seminal fluid proteins causes reduction of male Investment in a Simultaneous Hermaphrodite. Curr. Biol. 24, 859–862. doi: 10.1016/j.cub.2014.02.052
Noguera, J. C., Dean, R., Isaksson, C., Velando, A., and Pizzari, T. (2012). Age-specific oxidative status and the expression of pre- and postcopulatory sexually selected traits in male red junglefowl, Gallus gallus. Ecol. Evol. 2, 2155–2167. doi: 10.1002/ece3.300
Noguera, J. C., Metcalfe, N. B., and Monaghan, P. (2018). Experimental demonstration that offspring fathered by old males have shorter telomeres and reduced lifespans. Proc. R. Soc. B Biol. Sci. 285:20180268. doi: 10.1098/rspb.2018.0268
Nussey, D. H., Froy, H., Lemaitre, J.-F., Gaillard, J.-M., and Austad, S. N. (2013). Senescence in natural populations of animals: widespread evidence and its implications for bio-gerontology. Ageing Res. Rev. 12, 214–225. doi: 10.1016/j.arr.2012.07.004
O’Dea, R. E., Lagisz, M., Jennions, M. D., Koricheva, J., Noble, D. W. A., Parker, T. H., et al. (2021). Preferred reporting items for systematic reviews and meta-analyses in ecology and evolutionary biology: a PRISMA extension. Biol. Rev. 96, 1695–1722. doi: 10.1111/brv.12721
Ouzzani, M., Hammady, H., Fedorowicz, Z., and Elmagarmid, A. (2016). Rayyan—a web and mobile app for systematic reviews. Syst. Rev. 5:210. doi: 10.1186/s13643-016-0384-4
Patlar, B., Weber, M., and Ramm, S. A. (2019). Genetic and environmental variation in transcriptional expression of seminal fluid proteins. Heredity 122, 595–611. doi: 10.1038/s41437-018-0160-4
Patlar, B., Weber, M., Temizyürek, T., and Ramm, S. A. (2020). Seminal fluid-mediated manipulation of post-mating behavior in a simultaneous hermaphrodite. Curr. Biol. 30, 143–149.e4. doi: 10.1016/j.cub.2019.11.018
Paul, C., and Robaire, B. (2013). Ageing of the male germ line. Nat. Rev. Urol. 10, 227–234. doi: 10.1038/nrurol.2013.18
Perry, J. C., Sirot, L., and Wigby, S. (2013). The seminal symphony: how to compose an ejaculate. Trends Ecol. Evol. 28, 414–422. doi: 10.1016/j.tree.2013.03.005
Pizzari, T., Dean, R., Pacey, A., Moore, H., and Bonsall, M. B. (2008). The evolutionary ecology of pre- and post-meiotic sperm senescence. Trends Ecol. Evol. 23, 131–140. doi: 10.1016/j.tree.2007.12.003
Poiani, A. (2006). Complexity of seminal fluid: a review. Behav. Ecol. Sociobiol. 60, 289–310. doi: 10.1007/s00265-006-0178-0
Preston, B. T., Saint Jalme, M., Hingrat, Y., Lacroix, F., and Sorci, G. (2015). The sperm of aging male bustards retards their offspring’s development. Nat. Commun. 6:6146. doi: 10.1038/ncomms7146
Ramm, S. A. (2020). Seminal fluid and accessory male investment in sperm competition. Philos. Trans. R. Soc. B Biol. Sci 375:20200068. doi: 10.1098/rstb.2020.0068
Reinhardt, K. (2007). Evolutionary consequences of sperm cell aging. Q. Rev. Biol. 82, 375–393. doi: 10.1086/522811
Reinhardt, K., and Siva-Jothy, M. T. (2005). An advantage for young sperm in the house cricket Acheta domesticus. Am. Nat. 165, 718–723. doi: 10.1086/430010
Reyes-Hernández, M., and Pérez-Staples, D. (2017). Mating senescence and male reproductive organ size in the Mexican fruit fly: age effects on mating in Mexican fruit fly. Physiol. Entomol. 42, 26–35. doi: 10.1111/phen.12160
Rezaei, A., Krishna, M. S., and Santhosh, H. T. (2015). Male age affects female mate preference, quantity of accessory gland proteins, and sperm traits and female fitness in D. melanogaster. Zool. Sci. 32, 16–24. doi: 10.2108/zs140121
Ruhmann, H., Koppik, M., Wolfner, M. F., and Fricke, C. (2018). The impact of ageing on male reproductive success in Drosophila melanogaster. Exp. Gerontol. 103, 1–10. doi: 10.1016/j.exger.2017.12.013
Sabeti, P., Pourmasumi, S., Rahiminia, T., Akyash, F., and Talebi, A. R. (2016). Etiologies of sperm oxidative stress. Int. J. Reprod. Biomed. 14, 231–240. doi: 10.29252/ijrm.14.4.231
Santhosh, H. T., and Krishna, M. S. (2013). Relationship between male age, accessory gland, sperm transferred, and fitness traits in Drosophila bipectinata. J. Insect Sci. 13, 1–14. doi: 10.1673/031.013.15901
Sciamanna, I., Serafino, A., Shapiro, J. A., and Spadafora, C. (2019). The active role of spermatozoa in transgenerational inheritance. Proc. R. Soc. B Biol. Sci. 286:20191263. doi: 10.1098/rspb.2019.1263
Selvaratnam, J., and Robaire, B. (2016). Overexpression of catalase in mice reduces age-related oxidative stress and maintains sperm production. Exp. Gerontol. 84, 12–20. doi: 10.1016/j.exger.2016.08.012
Sepil, I., Hopkins, B. R., Dean, R., Bath, E., Friedman, S., Swanson, B., et al. (2020). Male reproductive aging arises via multifaceted mating-dependent sperm and seminal proteome declines, but is postponable in Drosophila. Proc. Natl. Acad. Sci. 117, 17094–17103. doi: 10.1073/pnas.2009053117
Sepil, I., Hopkins, B. R., Dean, R., Thezenas, M. L., Charles, P. D., Konietzny, R., et al. (2019). Quantitative proteomics identification of seminal fluid proteins in male Drosophila melanogaster. MCP 18, S46–S58. doi: 10.1074/mcp.RA118.000831
Short, S. M., and Lazzaro, B. P. (2010). Female and male genetic contributins to post-mating immune defence in female Drosophila melanogaster. Proc. R. Soc. Lond. B 277, 3649–3657. doi: 10.1098/rspb.2010.0937
Simmons, L. W. (2011). Allocation of maternal- and ejaculate-derived proteins to reproduction in female crickets, Teleogryllus oceanicus: allocation of maternal- and ejaculate-derived proteins. J. Evol. Biol. 24, 132–138. doi: 10.1111/j.1420-9101.2010.02158.x
Simmons, L. W., Beveridge, M., Li, L., Tan, Y., and Millar, A. H. (2014). Ontogenetic changes in seminal fluid gene expression and the protein composition of cricket seminal fluid. Evol. Dev. 16, 101–109. doi: 10.1111/ede.12068
Simmons, L. W., and Lovegrove, M. (2019). Nongenetic paternal effects via seminal fluid. Evol. Lett. 3, 403–411. doi: 10.1002/evl3.124
Simmons, L. W., and Lovegrove, M. (2020). Can paternal effects via seminal fluid contribute to the evolution of polyandry? Biol. Lett. 16:20200680. doi: 10.1098/rsbl.2020.0680
Stockley, P., Franco, C., Claydon, A. J., Davidson, A., Hammond, D. E., Brownridge, P. J., et al. (2020). Revealing mechanisms of mating plug function under sexual selection. Proc. Natl. Acad. Sci. 117, 27465–27473. doi: 10.1073/pnas.1920526117
Takemura, S., Ichikawa, H., Naito, Y., Takagi, T., Yoshikawa, T., and Minamiyama, Y. (2014). S-allyl cysteine ameliorates the quality of sperm and provides protection from age-related sperm dysfunction and oxidative stress in rats. J. Clin. Biochem. Nutr. 55, 155–161. doi: 10.3164/jcbn.14-39
Tan, C. K. W., Pizzari, T., and Wigby, S. (2013). Parental age, gametic age, and inbreeding interact to modulate offspring viability in Drosophila melanogaster: brief communication. Evolution 67, 3043–3051. doi: 10.1111/evo.12131
Trumble, B. C., Stieglitz, J., Rodriguez, D. E., Linares, E. C., Kaplan, H. S., and Gurven, M. D. (2015). Challenging the inevitability of prostate enlargement: low levels of benign prostatic hyperplasia among tsimane forager-horticulturalists. J. Gerontol. A Biol. Sci. Med. Sci. 70, 1262–1268. doi: 10.1093/gerona/glv051
Turnell, B. R., and Reinhardt, K. (2020). Metablic rate and oxygen radical levels increase but radical generation rate decreases with male age in Drosophila melanogaster sperm. J. Gerontol. Biol. Sci. 75:glaa078. doi: 10.1093/gerona/glaa078
Vega-Trejo, R., Fox, R. J., Iglesias-Carrasco, M., Head, M. L., and Jennions, M. D. (2019). The effects of male age, sperm age and mating history on ejaculate senescence. Funct. Ecol. 33, 1267–1279. doi: 10.1111/1365-2435.13305
Velando, A., Noguera, J. C., Drummond, H., and Torres, R. (2011). Senescent males carry premutagenic lesions in sperm. J. Evol. Biol. 24, 693–697. doi: 10.1111/j.1420-9101.2010.02201.x
Veltsos, P., Porcelli, D., Fang, Y., Cossins, A. R., Ritchie, M. G., and Snook, R. R. (2022). Experimental sexual selection reveals rapid evolutionary divergence in sex-specific transcriptomes and their interactions following mating. Mol. Ecol. 31, 3374–3388. doi: 10.1111/mec.16473
Vince, S., Zaja, I. Z., Samardzija, M., Balic, I. M., Vilic, M., Duricic, D., et al. (2018). Age-related differences of semen quality, seminal plasma, and spermatozoa antioxidative and oxidative stress variables in bulls during cold and warm periods of the year. Animal 12, 559–568. doi: 10.1017/S1751731117001811
Vuarin, P., Bouchard, A., Lesobre, L., Levêque, G., Chalah, T., Jalme, M. S., et al. (2019). Post-copulatory sexual selection allows females to alleviate the fitness costs incurred when mating with senescing males. Proc. Biol. Sci. 286:20191675. doi: 10.1098/rspb.2019.1675
Vuarin, P., Lesobre, L., Levêque, G., Saint Jalme, M., Lacroix, F., Hingrat, Y., et al. (2021). Paternal age negatively affects sperm production of the progeny. Ecol. Lett. 24, 719–727. doi: 10.1111/ele.13696
Waheed, M. M., El-Bahr, S. M., and Al-haider, A. K. (2013). Influence of seminal plasma antioxidants and osteopontin on fertility of the Arabian horse. J. Equine Vet. Sci. 33, 705–709. doi: 10.1016/j.jevs.2012.11.006
Watkins, A. J., Dias, I., Tsuro, H., Allen, D., Emes, R. D., Moreton, J., et al. (2018). Paternal diet programs offspring health through sperm- and seminal plasma-specific pathways in mice. Proc. Natl. Acad. Sci. 115, 10064–10069. doi: 10.1073/pnas.1806333115
Weber, M., Giannakara, A., and Ramm, S. A. (2019). Seminal fluid-mediated fitness effects in the simultaneously hermaphroditic flatworm Macrostomum lignano. Ecol. Evol. 9, 13889–13901. doi: 10.1002/ece3.5825
Wedell, N., Gage, M. J. G., and Parker, G. A. (2002). Sperm competition, male prudence and sperm-limited females. Trends Ecol. Evol. 17, 313–320. doi: 10.1016/S0169-5347(02)02533-8
Westfalewicz, B., Słowińska, M., Judycka, S., Ciereszko, A., and Dietrich, M. A. (2021). Comparative proteomic analysis of young and adult bull (Bos taurus) cryopreserved semen. Animals 11:2013. doi: 10.3390/ani11072013
Wolfner, M. F. (1997). Tokens of love: functions and regulation of Drosophila male accessory gland products. Insect Biochem. Mol. Biol. 27, 179–192. doi: 10.1016/S0965-1748(96)00084-7
Wylde, Z., Spagopoulou, F., Hooper, A. K., Maklakov, A. A., and Bonduriansky, R. (2019). Parental breeding age effects on descendants’ longevity interact over 2 generations in matrilines and patrilines. PLoS Biol. 17:e3000556. doi: 10.1371/journal.pbio.3000556
Xie, K., Ryan, D. P., Pearson, B. L., Henzel, K. S., Neff, F., Vidal, R. O., et al. (2018). Epigenetic alterations in longevity regulators, reduced life span, and exacerbated aging-related pathology in old father offspring mice. Proc. Natl. Acad. Sci. 115, E2348–E2357. doi: 10.1073/pnas.1707337115
Xu, J., Baulding, J., and Palli, S. R. (2013). Proteomics of Tribolium castaneum seminal fluid proteins: identification of an angiotensin-converting enzyme as a key player in regulation of reproduction. J. Proteome 78, 83–93. doi: 10.1016/j.jprot.2012.11.011
Zajitschek, F., Zajitschek, S., and Bonduriansky, R. (2020). Senescence in wild insects: key questions and challenges. Funct. Ecol. 34, 26–37. doi: 10.1111/1365-2435.13399
Zambrano, E., Nathanielsz, P. W., and Rodríguez-González, G. L. (2021). Developmental programming and ageing of male reproductive function. Eur. J. Clin. Investig. 51:e13637. doi: 10.1111/eci.13637
Keywords: senescence, reproduction, ejaculates, seminal fluid, germline, oxidative damage, seminal fluid proteins
Citation: Fricke C, Sanghvi K, Emery M, Lindenbaum I, Wigby S, Ramm SA and Sepil I (2023) Timeless or tainted? The effects of male ageing on seminal fluid. Front. Ecol. Evol. 11:1066022. doi: 10.3389/fevo.2023.1066022
Edited by:
Jean-Francois Lemaitre, UMR5558 Biométrie et Biologie Evolutive (LBBE), FranceReviewed by:
Matt Dean, University of Southern California, United StatesGeoff A. Parker, University of Liverpool, United Kingdom
Copyright © 2023 Fricke, Sanghvi, Emery, Lindenbaum, Wigby, Ramm and Sepil. This is an open-access article distributed under the terms of the Creative Commons Attribution License (CC BY). The use, distribution or reproduction in other forums is permitted, provided the original author(s) and the copyright owner(s) are credited and that the original publication in this journal is cited, in accordance with accepted academic practice. No use, distribution or reproduction is permitted which does not comply with these terms.
*Correspondence: Claudia Fricke, ✉ Y2xhdWRpYS5mcmlja2VAem9vbG9naWUudW5pLWhhbGxlLmRl
†These authors have contributed equally to this work and share first authorship
‡These authors have contributed equally to this work and share senior authorship
§ORCID: Krish Sanghvi http://orcid.org/0000-0002-5125-2983