- 1Evolution & Ecology Research Centre, School of Biological, Earth and Environmental Sciences, UNSW Sydney, Sydney, NSW, Australia
- 2Department of Forest Resources, University of Minnesota, St. Paul, MN, United States
- 3CSIRO Health & Biosecurity, Brisbane, QLD, Australia
- 4School of Chemistry and Molecular Biosciences, The University of Queensland, St. Lucia, QLD, Australia
- 5School of Mathematics and Statistics, UNSW Sydney, Kensington, NSW, Australia
- 6Earth and Sustainability Science Research Centre, School of Biological, Earth and Environmental Sciences, UNSW Sydney, Sydney, NSW, Australia
- 7School of Biological, Earth and Environmental Sciences, UNSW Sydney, Sydney, NSW, Australia
- 8Hawkesbury Institute for the Environment, Western Sydney University, Penrith, NSW, Australia
- 9Systems Ecology, Department of Ecological Science, Vrije Universiteit Amsterdam, Amsterdam, Netherlands
- 10Jonah Ventures, Manhattan, KS, United States
- 11Max Planck Institute for Biogeochemistry, Jena, Germany
- 12German Centre for Integrative Biodiversity Research (iDiv) Halle-Jena-Leipzig, Leipzig, Germany
- 13Institute of Agricultural and Environmental Sciences, Estonian University of Life Sciences, Tartu, Estonia
- 14Estonian Academy of Sciences, Tallinn, Estonia
- 15CSIC, Global Ecology Unit CREAF-CSIC-UAB, Bellaterra, Spain
- 16CREAF, Cerdanyola del Vallès, Spain
Australia’s distinctive biogeography means that it is sometimes considered an ecologically unique continent with biological and abiotic features that are not comparable to those observed in the rest of the world. This leaves some researchers unclear as to whether findings from Australia apply to systems elsewhere (or vice-versa), which has consequences for the development of ecological theory and the application of ecological management principles. We analyzed 594,612 observations spanning 85 variables describing global climate, soil, geochemistry, plants, animals, and ecosystem function to test if Australia is broadly different to the other continents and compare how different each continent is from the global mean. We found significant differences between Australian and global means for none of 15 climate variables, only seven of 25 geochemistry variables, three of 16 soil variables, five of 12 plant trait variables, four of 11 animal variables, and one of five ecosystem function variables. Seven of these differences remained significant when we adjusted for multiple hypothesis testing: high soil pH, high soil concentrations of sodium and strontium, a high proportion of nitrogen-fixing plants, low plant leaf nitrogen concentration, low annual production rate to birth in mammals, and low marine productivity. Our analyses reveal numerous similarities between Australia and Africa and highlight dissimilarities between continents in the northern vs. southern hemispheres. Australia ranked the most distinctive continent for 26 variables, more often than Europe (15 variables), Africa (13 variables), Asia (12 variables each), South America (11 variables) or North America (8 variables). Australia was distinctive in a range of soil conditions and plant traits, and a few bird and mammal traits, tending to sit at a more extreme end of variation for some variables related to resource availability. However, combined analyses revealed that, overall, Australia is not significantly more different to the global mean than Africa, South America, or Europe. In conclusion, while Australia does have some unique and distinctive features, this is also true for each of the other continents, and the data do not support the idea that Australia is an overall outlier in its biotic or abiotic characteristics.
1. Introduction
When early European naturalists observed the conditions, organisms, and ecosystems of Australia, they were struck by the apparent differences from those they were familiar with elsewhere. Charles Darwin wrote in 1836 “I … was reflecting on the strange character of the Animals of this country as compared to the rest of the World. An unbeliever in everything beyond his own reason, might exclaim “Surely two distinct Creators must have been [at] work…” (Darwin and Keynes, 2001, p. 402). Framed in the entrance to the Great Hall in Australia’s Parliament House is a quote from Clarke (1876), that opens with “In Australia alone is to be found the Grotesque, the Weird, the strange scribblings of nature learning to write.” Similarly, François Péron proclaimed in 1809 that Australia was “an absolute exception; as if … the animals and vegetables of this singular continent [have] peculiar laws, which differ from all the principles of our sciences and all the rules of our systems” (Péron, 1809, p. 291). Two centuries later, our understanding of biogeography has advanced significantly, and using big-data science and an increasingly global approach to scientific collaboration (Tydecks et al., 2018) we have addressed research questions at continental-or intercontinental-scales to uncover global, macro-ecological patterns. However, the idea that the species, conditions and ecosystem processes of Australia are different from elsewhere on Earth persists amongst some scientists (Clarke, 1876; Braithwaite, 1990; Greenwood et al., 2004; Hadden, 2007; Orians and Milewski, 2007; Wiens, 2016). The empirical evidence for Australia’s distinctiveness is surprisingly limited, with direct quantitative comparisons of the biological, geological and climatological features of Australia with those elsewhere in the world mostly focused on a small number of non-randomly selected sites (e.g., Dodson and Westoby, 1985; Westoby, 1988; Wiens, 1991; Kirkby et al., 2011), and no continental scale tests. Australia has not generally been identified as an outlier in global analyses (Wright et al., 2005; Moles et al., 2007, 2009), though global analyses do not usually test whether continents differ. In this paper, we provide a broad test of whether Australia, or any other continent, is quantitatively different from the other continents.
Among the six vegetated continents, all except Australia are (or were recently until the construction of a canal) connected by land to at least one other continent. This has led to a high level of zoological uniqueness (Mazel et al., 2017) and endemism in Australia at the species level. It has been estimated that of the species found in Australia, ~93% of plants, ~93% of reptiles and ~ 74–87% of mammals are found nowhere else (Ceballos and Brown, 1995; Chapman, 2009); [for comparison, other regions with relatively high levels of mammalian endemism globally are Madagascar (90%) and the Philippines (60%), and other large continental areas such as Mexico (33%) and the United States (27%) (Ceballos and Brown, 1995)]. Australia has a high diversity of Elapidae compared to other continents and is the only continent without Viperidae (see Figure 1 of Terribile et al., 2009). It also has a high diversity of Proteaceae, with almost 1,100 of the world’s ~ 1,660 species (McCarthy and Orchard, 1995; Christenhusz and Byng, 2016); and is home to four of the only five extant species of monotremes, and to around two-thirds of the world’s marsupials (Lee and Cockburn, 1985). There is also evidence that Australia has high phylogenetic and trait turnover relative to other parts of the world (Holt et al., 2018).
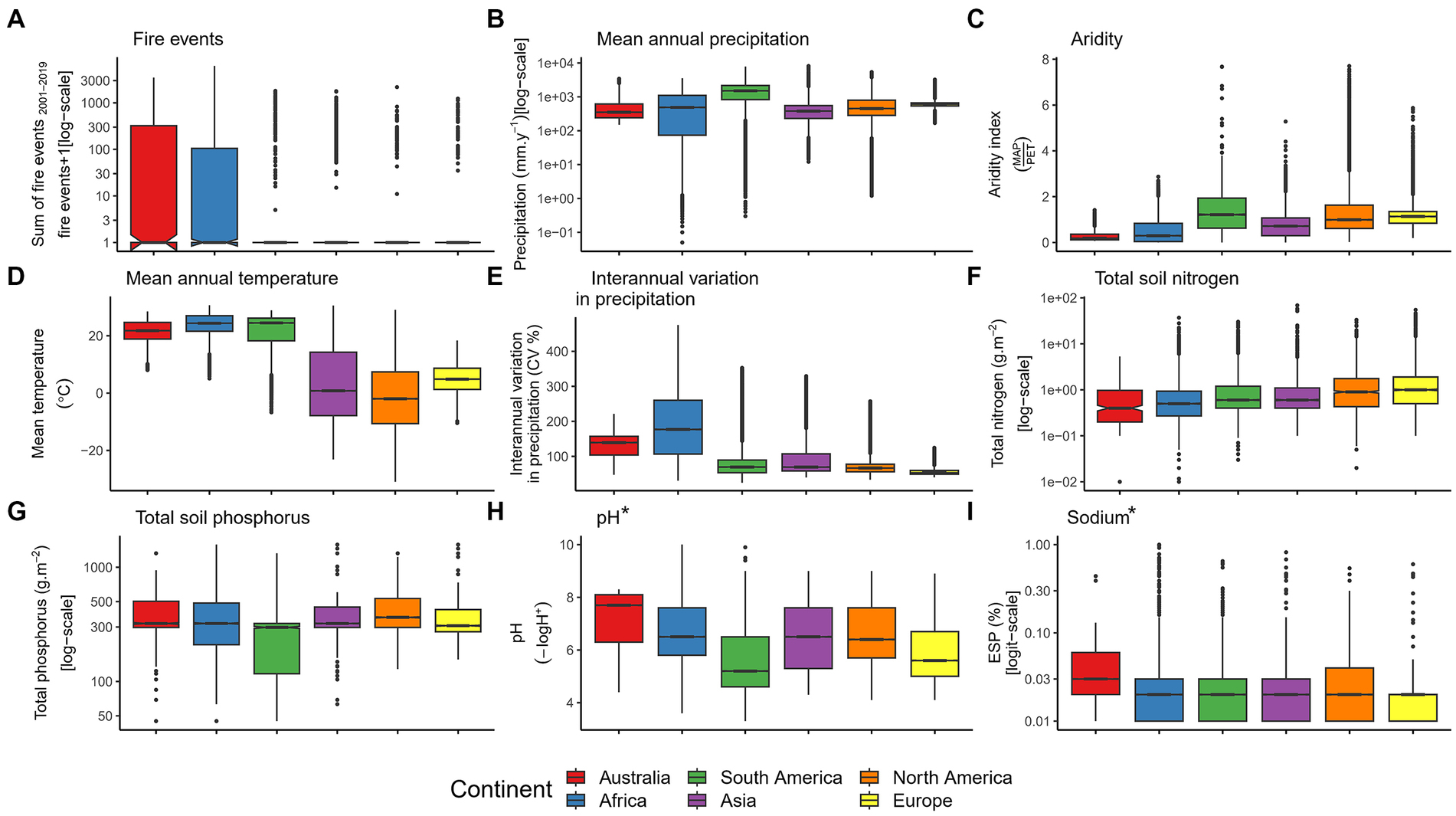
Figure 1. Comparison of a set of abiotic characteristics of Australia and other continents including (A) fire events, (B) mean annual precipitation, (C) aridity, (D) mean annual temperature, (E) interannual variation in precipitation, (F) total soil nitrogen, (G) total soil phosphorus, (H) pH, and (I) sodium. The boxes represent the 25th, 50th, and 75th percentiles. Whiskers represent the lowest and highest value still within the 1.5 interquartile range (IQR) of the lower or upper quartile; points represent outliers beyond 1.5 IQR. Asterisks indicate significant terms (p < 0.05) after False Discovery Rate (FDR) correction for multiple testing.
Differences between Australia and the rest of the world have been attributed to geographic isolation, a relatively flat and nutrient-poor landscape, a hot climate with low and unpredictable rainfall, a long history of human-caused fires, and a lack of recent glaciation (Mabbutt, 1988; Squires, 1988; Braithwaite, 1990; Milewski and Diamond, 2000; Orians and Milewski, 2007; Peel et al., 2007; Kooyman et al., 2016). However, comparative evidence for differences among continents in these environmental variables is currently lacking. For example, the assertion that Australia is a particularly hot and dry continent (Peel et al., 2007) has not been quantitatively tested. Australia has been fairly tectonically stable for 10 million to 100 million years, and its soils have low levels of soil phosphorous, having been exposed to prolonged weathering and re-working over this substantial time frame (Pillans, 2007; Kooyman et al., 2016). However, phosphorus limitation is a globally broad phenomenon (Du et al., 2020). Australian soils have on average lower phosphorus content than US soil (Wild, 1958) and lower nitrogen content than a collection of international soils (Kirkby et al., 2011), but these studies are confounded by the use of different methods to quantify soil fertility on different continents. Thus, there is surprisingly little unconfounded empirical evidence for Australia having unusually low nutrient soils in a global context.
The environmental conditions of a region are important drivers of species and community evolution. For example, the low fertility of Australian soils is central to many explanations for Australia’s unique ecosystems (Braithwaite, 1990; Orians and Milewski, 2007; Kooyman et al., 2016). Ancient, nutrient-poor soils are an explanation for low productivity of Australian ecosystems and are thought to have favored plants with conservative nutrient-use strategies and low nutrient content (Milewski and Diamond, 2000; Burness et al., 2001; Orians and Milewski, 2007; Hopper, 2009; Kooyman et al., 2016). Many authors highlight the distinctiveness of Australia’s hard-leaved (sclerophyllous), fire-adapted plants (Beadle, 1966; Braithwaite, 1990; Orians and Milewski, 2007; Bradstock et al., 2012). Reduced and less-predictable resource availability is also considered to have exerted selective pressure on characteristics of Australian fauna such as body size, growth and metabolic rates, sociality and reproductive output, and may contribute to Australia’s absence of large carnivorous mammals and food chains with relatively few apex predators compared to other continents (Orians and Milewski, 2007 and references therein; Lee and Cockburn, 1985; Braithwaite, 1990; Milewski and Diamond, 2000; Burness et al., 2001; Ritchie and Johnson, 2009; Jetz and Rubenstein, 2011). Low nutrient concentrations and unique oceanographic patterns underpin globally low annual yields in Australian marine wild fisheries (Savage, 2015; FAO, 2016). Scaling up of expected differences in characteristics of climate, soil and the biota has been predicted to result in significant differences in key ecological processes such as productivity, decomposition and herbivory between Australia and the other continents (Braithwaite, 1990; Coley and Barone, 1996; Cornwell et al., 2008; Reich, 2012). All of these pieces of evidence point to how Australia may differ from other continents. However, empirical evidence that Australia is globally unusual is scarce.
We asked if Australia’s environment is distinct or more different to other continents, in three ways. First, we tested if Australia is significantly different from the global mean of each variable. Next, we sought to determine the most distinctive continent in terms of data distribution for each of the variables, to establish if Australia, or indeed any other continent, is distinctive from the rest of the world more often. Quantifying how Australia and the other continents compare in individual variables capturing information about environmental conditions, life history traits and ecosystem processes, is a valuable place to begin. However, these factors are highly interdependent. Thus, our final goal was to determine the overall similarity between continents by comparing distances from global means across all of our variables together.
2. Materials and methods
We compiled global datasets spanning 85 features of climate (marine and terrestrial), soil chemistry and soil edaphic attributes, plant and animal (birds and mammals) life history traits, and indicators of ecosystem function (see full list Supporting information – Supplementary Table S1). The datasets included were the largest, most complete datasets available at the time. While datasets differ in coverage and resolution depending on their source (for instance, climate, soil edaphology and productivity datasets have the highest global coverage, while marine herbivory and decomposition have the lowest global coverage), they were chosen based on having comparable or synthesized data across continents. Overall, our study encompasses 594,612 observations (see Supplementary Tables S1, S2 for a list of variables in each category; all data sources and their details are listed in the Supplementary materials under the “Data” subheading).
We assigned continent of origin to each observation in our datasets. The analyses include land masses >1,000,000 km2, and marine continental areas no more than 200 km away from these land masses. The databases used for our comparison can be divided into two groups: spatially explicit databases, where every record has an assigned latitude and longitude, and non-spatial databases, where records are assigned to a continent.
We began by individually comparing the means of each variable across continents. For each variable in both types of dataset, we ran linear mixed effect models (Harrison et al., 2018) with a fixed effect for Australian-ness (whether or not the observations belong to the Australian continent) and a random effect for continent. For the spatially explicit data, we chose the best spatial correlation structure (i.e., spherical, exponential, gaussian, linear, rational) for each variable using Akaike information criterion (AIC) values. Then we used the selected correlation structure for each observation in each continent to account for the effect of spatial autocorrelation between observations. For both the spatially explicit and non-spatial datasets, we calculated p-values for the univariate models using likelihood ratio statistics. We used the False Discovery Rate (FDR) test to control for multiple testing, however, p-values reported in the main text are not corrected for multiple testing. Supplementary Tables S1, S2 list all dependent variables, whether they are spatially explicit or not, units, sample size, as well as χ2, p-values, and FDR corrected p-values.
Then, for each variable, we identified which continent was the most distinctive overall in the distribution of data to determine if Australia, or indeed any other continent, was distinctive more often than other continents. To do this we adapted the KSI test used by Cornwell et al. (2014). Given a distribution of values among continents, the KSI measures the distinctiveness of all possible groups, in our case continents. The test compares the frequency distribution of values for continents from the distribution for all other continents using a nonparametric two-sample Kolmogorov–Smirnov test. This test asks how likely is it that the groups in each comparison came from the same distribution, using the Kolmogorov–Smirnov significance test to rank the distinctiveness of different continents. This involves calculating the difference between each continent and that of other continents for each variable, based on the maximum difference in their cumulative distribution. Then, ranking the continents in the likelihood that their distribution comes from the same underlying distribution to that of all other continents. Thus, the method is designed to identify continents that significantly alter the distribution of values observed in a given variable. Without these continents, the distribution of a variable across continents would be very different. The Kolmogorov–Smirnov test can distinguish not only differences in mean values but also changes in variance and skewness of distributions among comparison groups.
Finally, we sought to quantify the extent to which Australia differs from other continents in major abiotic and biotic characteristics overall. To do this, we calculated z-scores for each variable for each continent to determine how different each continent is to the global mean for our different variables. z-scores are calculated as , to make binary and continuous variables comparable (Gelman, 2008). We standardized the z-scores for each variable relative to the global mean and variance for that particular variable. That is, we z-transformed each variable relative to the global mean and variance for that variable, and compared the number of standard deviations that the combined z-scores of all variables within a category is away from the mean. To explore if Australia tends to be more different overall from the other continents, we applied non-parametric Kruskal-Wallis test to the absolute value of these z-scores. We then applied a post-hoc Dunn’s multiple comparison non-parametric test to identify the patterns of similarity and differences between all of the continents. If Australia is more distinct overall (i.e., being more different to the global means of the variables) compared to other continents, we would expect to see a significant difference between continents and that differences in pair-wise comparisons with Australia are driving this. To visually compare how similar continents are among each other we calculated the correlation values for the z-scores among each continent pair for the characteristics within variable category. We only plotted correlations with r ≥ 0.45 as these represent strong correlations (Cohen, 1988), and thus substantial associations between continents.
Australia is not just a continent but also occupies its own biogeographic realm, whereas some other continents have multiple biogeographic realms (Holt et al., 2013). Important differences between Australia and the rest of the world may be masked by comparing continents by inflating the variance or dispersion features of other continents and thus limit the detection of differences with Australia. Thus, we have also applied the above analyses using biogeographic realm as the unit of comparison to compare the Australian biogeographic realm to the other biogeographic realms. Results using biogeographic realm as the unit of comparison were broadly consistent with those comparing continents (see Supplementary materials for full results).
All analyses were run in R 3.3.2 (R Core Team, 2016). We fitted the univariate models using the nlme (Pinheiro et al., 2014) and lme4 (Bates et al., 2014) libraries. All models were checked to meet the constant variance (homoscedasticity) and normality assumptions of linear models. Code for data analyses is available at https://bitbucket.org/habacucfm/is_australia_weird. See supporting information for complete details of analyses.
3. Results
3.1. Are Australian means for each variable significantly different to global means?
Although it is often stated that Australia is an unusually hot continent with low, variable rainfall (e.g., Orians and Milewski, 2007; Peel et al., 2007), we did not find evidence for greater mean temperature (p = 0.437), greater interannual variation of precipitation (p = 0.668) or higher aridity (p = 0.404) in Australia compared to the global mean. Our analyses showed no significant difference between Australia and other continents in any of the 15 individual terrestrial or marine climate features explored (all p > 0.079; Supplementary Table S1 and Supplementary Figures S2, S3).
Overall, Australia was significantly different from the other continents in seven of 25 characteristics related to geochemistry (Supplementary Figures S4, S5 and Supplementary Table S1); only soil sodium and strontium concentration remained significant after correction for multiple testing (Supplementary Table S1). Despite nutrient-poor Australian soils being central to many explanations for Australia’s unique ecosystems (Braithwaite, 1990; Orians and Milewski, 2007; Kooyman et al., 2016), the Australian continent did not significantly differ from those of the rest of the world in total phosphorus (p = 0.097; Figure 1G), secondary phosphorus (p= 0.29; Supplementary Figure S4B), organic phosphorus (p= 0.156; Supplementary Figure S4C), labile inorganic phosphorus (p= 0.597; Supplementary Figure S4D), or total soil nitrogen (p= 0.103; Supplementary Figure S4E). Australian soils and geologic parent materials do differ from those of other continents in several micronutrients and elemental concentrations, with Australian parent lithologies showing lower concentrations of potassium (p= 0.030), magnesium (p = 0.028), manganese (p = 0.010), zinc (p= 0.044), calcium (p = 0.007), sodium (p < 0.0001) and strontium (p= 0.003) compared to other major land masses. Across 16 soil edaphic characteristics, three showed significant differences between Australia and other continents. Specifically, Australian soils are higher in total exchangeable bases (p = 0.021) and bulk density (p = 0.031), and are significantly more alkaline (p = 0.004; Figure 1H). However, after correction for multiple testing, only one of 16 soil variables (pH) remained significant (Supplementary Figures S5–S6 and Supplementary Table S1).
The phenotypes of Australian plants were expected to reflect resource-conservative ecological strategies (Beadle, 1966; Braithwaite, 1990; Cunningham et al., 1999; Orians and Milewski, 2007). Consistent with this prediction, we found that Australian plants have leaves that are on average more than twice as thick/dense (lower specific leaf area, p = 0.012; Figure 2A), with mass-based concentrations of phosphorus that are on average 27% lower (p = 0.013; Australian mean of 0.38 mg g−1 vs. mean of other continents of 0.52 mg g−1; Figure 2B) and mass based concentrations of nitrogen that are 31% lower (p = 0.001; Australian mean of 0.88 mg g−1 vs. mean of other continents of 1.28 mg g−1; Supplementary Figure S7D) compared to plants from other continents. Australia also has about twice as many plant species with nitrogen fixing capacity (p = 0.002; Figure 2C), and a lower proportion of species with a C3 photosynthetic pathway (p = 0.041; Supplementary Figure S7G) compared to other continents. We found no evidence of differences between Australia and other continents in the other plant characteristics, including crucial traits such as plant height, leaf size, seed mass and phenology (Supplementary Table S2 and Supplementary Figure S7). Overall, five of 12 traits of Australian plants differed from the global mean; after correction for multiple testing, two of 12 (leaf Nmass and nitrogen fixing capacity) remained significantly different from other continents (Supplementary Table S2).
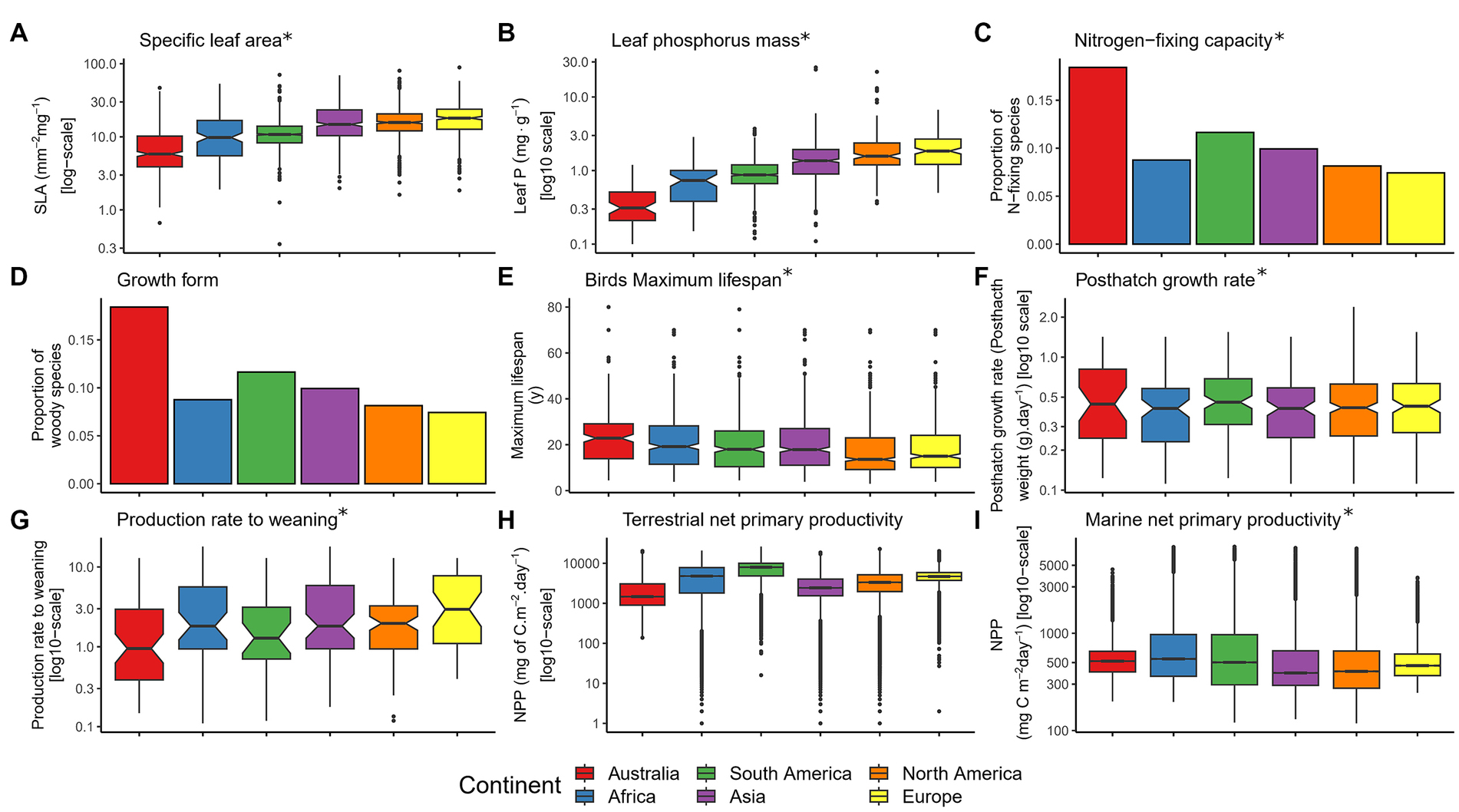
Figure 2. Comparison of a set of biotic characteristics of Australia and other continents including (A) specific leaf area, (B) leaf phosphorus mass, (C) nitrogen-fixing capacity, (D) growth form, (E) birds maximum lifespan, (F) posthatch growth rate, (G) production rate to weaning, (H) terrestrial net primary productivity, and (I) marine net primary productivity. The boxes represent the 25th, 50th, and 75th percentiles. Whiskers represent the lowest and highest value still within the 1.5 interquartile range (IQR) of the lower or upper quartile; points represent outliers beyond 1.5 IQR. Asterisks indicate significant terms (p < 0.05) after False Discovery Rate (FDR) correction for multiple testing.
Life history strategies of Australian animals were also expected to indicate resource-conservative ecological strategies (Braithwaite, 1990; Jetz and Rubenstein, 2011). Australian birds live for an average of ~21 years, which is significantly longer than birds from other continents, which live, on average, for ~16 years (p = 0.023; Figure 2E). The post-hatch growth rate of Australian birds is also 26% slower than that of birds elsewhere (0.14 g per day in Australia, compared to the mean of other continents of 0.19 g per day; p = 0.013; Figure 2F). Australian mammals birth (p = 0.0001; Supplementary Figure S8D) and wean (p = 0.009; Figure 2E) significantly less mass of offspring in relation to their body size compared to animals from other continents. Overall, four of 11 bird and mammal traits showed a significant difference between Australia and the rest of the world (Supplementary Table S2 and Supplementary Figure S8), with the annual production rate to birth of mammals being the only of 11 animal traits remaining globally unusual in Australia after correction for multiple testing (Supplementary Table S2).
Scaling up of expected differences in characteristics of climate, soil and the biota was predicted to result in significant differences in key ecological processes between Australia and the other continents (Braithwaite, 1990; Coley and Barone, 1996; Cornwell et al., 2008; Reich, 2012). Both before and after correction for multiple testing, Australia stood out from the other continents in only one of six indicators of ecosystem function: marine primary productivity is 38% lower in Australia than in other continents [on average 398.65 C mg m−2 day−1 in Australia vs. 646.33 C mg m−2 day−1 elsewhere; p = 0.0001 (p = 0.003 after FDR), Figure 2I]. No significant differences were found in terrestrial productivity [gross primary productivity (GPP) and net primary productivity (NPP)], marine herbivory, terrestrial decomposition or frequency of fire events (Supplementary Figure S9).
Overall, Australia was significantly different from the other continents in 20 of 85 variables related to climate, soils, biota and ecosystem processes (Supplementary Tables S1, S2), which reduced to only seven of 85 variables once we applied corrections for the large number of tests applied (marine net primary productivity, annual production rate to birth in mammals, plant N fixing capacity, nitrogen concentration per unit leaf mass, soil pH, and concentrations of sodium and strontium in soil; Supplementary Tables S1, S2).
3.2. Is Australia distinctive from global data distributions more often than the other continents?
Globally Australia was the most distinctive continent most often across 85 variables, ranking as the most distinct from the rest of the globe in 26 variables (Supplementary Tables S3, S4). The next most distinctive continent was Europe (ranked most distinctive for 15 variables), followed by Africa (most distinctive for 13 variables), Asia (12 variables), South America (11 variables), and North America (8 variables).
Australia’s distinctiveness was mainly related to soil conditions and geochemistry and functional traits of plants, but also a few bird and mammal traits (Supplementary Table S3). Australian soils were distinctive in a range of attributes which relate to nutrient availability, including cation exchange capacity, pH, particle size characteristics (sand, clay, and gravel content), carbon content and sodicity. Australian plants were the most distinctive in traits related to the leaf economic spectrum (LES), nitrogen fixing capacity, phenology and photosynthetic pathway. Australian animals were distinctive in age to maturity; birds were also distinctive in annual production rate to hatching, and post-hatch growth rate, and mammals in annual production rate to birth and weaning. Meanwhile, the distinctiveness of Europe was related to its geochemistry and marine climate, diurnal temperature range, marine herbivory, plant life form, and mammalian basal metabolic rate. Africa was mainly distinctive in terrestrial climate and soil phosphorous, but African mammal lifespan and the body mass of African birds also contribute. In theory, the distinctiveness of continents identified by the KSI test could come through shifts in the mean, variance, kurtosis or skewness of the distributions. In practice, many changes came through a shift in the mean. The distinctiveness of 10 variables is likely due to a shift in the means, as suggested by the “Australianess” tests above (Supplementary Tables S1, S2). Distinctiveness of specific leaf area (Figure 2A), leaf N mass (Supplementary Figure S7D), proportion of species with a C3 photosynthetic pathway (Supplementary Figure S7G), post hatch growth rate of birds (Supplementary Figure S8I), the annual production rates to weaning (Supplementary Figure S8D) and to birth in mammals (Supplementary Figure S8E), as well as strontium concentration (Supplementary Figure S5O) all reflect lower mean values in Australia compared to other continents. Meanwhile, Australia’s distinctiveness in proportion of species with Nitrogen fixing capacity (Supplementary Figure S7J), soil total exchangeable bases (Supplementary Figure S6L) and soil bulk density (Supplementary Figure S6E) reflect higher means in Australia compared to other continents. However, in the cases of photosynthetic pathway, nitrogen fixing capacity and phenology, the lower kurtosis of these traits for Australia was also often in contrast to the higher kurtosis of other continents. All animal traits for which Australia was the most distinctive was due to a shift in means, except for annual production rate to hatching, where the difference seems to come through the lower kurtosis of this trait in Australia compared to other continents (more infrequent outliers). Kurtosis may reflect niche breadth (Fraser, 1977; Wool, 1980). The lower kurtosis of these animal and plant traits in Australia suggests higher coexistence of functionally contrasting species in this continent, because lower kurtosis tends to indicate a more even distribution of species with different trait values (Enquist et al., 2015; Gross et al., 2017).
The distinctiveness of ecosystem function was spread among Africa, Asia, South and North America and Europe. Asia was the most distinctive continent in both terrestrial and marine NPP, Europe was the most distinctive in the impact of marine grazers, North America in fire frequency, and South America in decomposition rate. Meanwhile, distinctiveness in geochemistry was mainly concentrated in Europe and Asia. In terms of soil P, Africa was the most distinct continent in soil total and organic P, North America in secondary phosphate and South America in labile inorganic P. Europe was the most distinct continent in soil N.
3.3. How do the continents differ from each other in major abiotic and biotic characteristics overall?
Analyses of absolute z-scores of all variables across all continents revealed that there are significant differences between continents in how far they are from global means of variables (p < 0.0001; Figure 3 and Supplementary Figure S17). Post-hoc testing revealed that out of the 15 possible pair-wise combinations of continents, six pairings are significantly different from each other (Supplementary Table S6). Three groupings emerged between the continents, with Australia, Africa, South America and Europe forming one grouping; South America, North America and Europe forming another grouping; and Asia and North America forming a third (Figure 3). That is, Australia is not significantly different to Africa, South America and Europe in deviation from the global means across 85 variables.
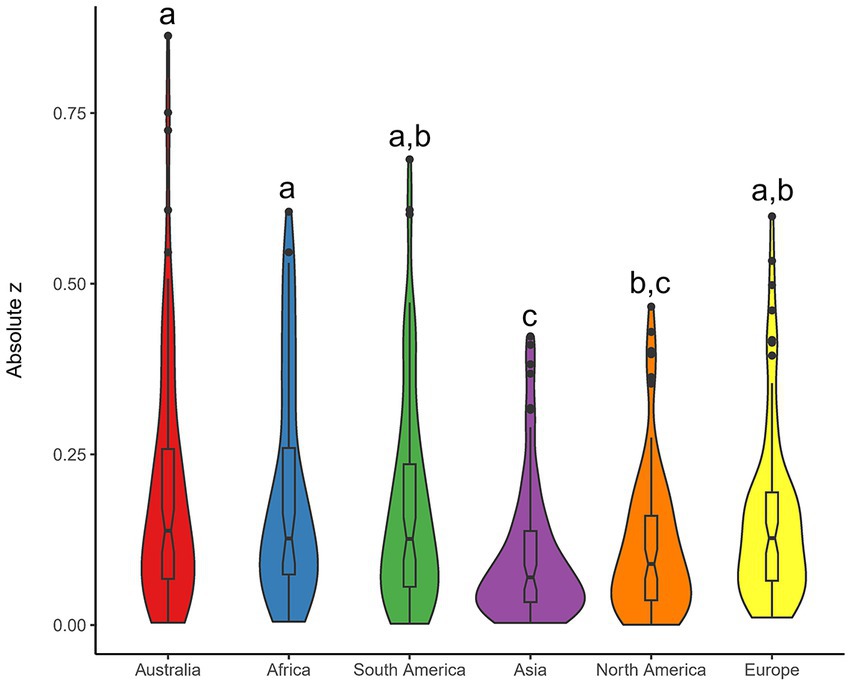
Figure 3. Differences among continents in how they deviate from the global means across 85 abiotic and biotic variables. Violin plots of absolute deviation from the mean value (absolute z-scores) for each variable. Non-parametric Kruskal-Wallis test shows that there are significant differences between continents in how different they are from the global means. Letters denote significant differences between pairs of continents from Dunn’s test multiple comparison. The boxes represent the 25th, 50th, and 75th percentiles. Whiskers represent the lowest and highest value still within the 1.5 interquartile range of the lower or upper quartile. Points show outlier values.
Pair-wise correlations of z-scores among each pair of continents show strong correlations between the plant traits found in Australia, South America and Africa, and between the terrestrial climates of Australia and Africa. This analysis also reveals that Europe and North America are the most correlated to each other, that they have fewer strong correlated to Asia and South America, and have no strong correlations with Africa and Australia (Figure 4).
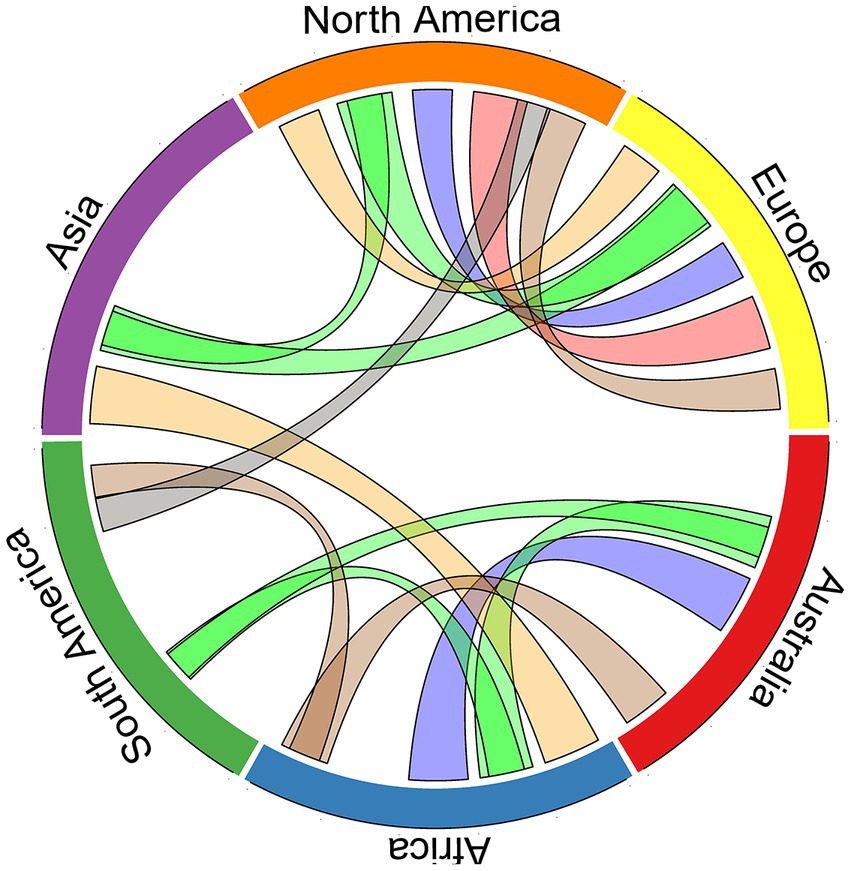
Figure 4. Correlation between continents for soil geochemistry (n = 100; gray colored connections), soil edaphic characteristics (n = 96; brown colored connections), climate (n = 90; blue colored connections), plant characteristics (n = 66; green colored connections), animal characteristics (n = 66; red colored connections), and characteristics of ecosystem function (n = 36; yellow colored connections). Linkages denote correlations >0.45 for the z-scores among each continent pair for the above trait categories. For instance, a green connection between two continents denotes that the traits of plants on the two continents are rather similar, while a blue connection indicates similarity between the terrestrial climate of the two continents.
4. Discussion
Our study has brought together an unprecedented breadth of data, spanning climate, soil, plants, birds, mammals and ecosystem processes, and we have shown that there are some differences in the biotic and abiotic environment between Australia and the rest of the world. Australia is distinct in more variables that the other continents. However, most of these differences are not driven by significant differences in means but in skewness or spread of data distributions. However, overall, our data (e.g., Figure 4) demonstrate that Australia is not an outlier to the world’s other continents but fits well within the span of global environmental variation. Rather, our results provide further indication that differences between continents are more pronounced between the hemispheres. That is, Australian researchers and land managers should consider broad ecological findings from elsewhere in the world as likely to be relevant for the Australian context rather than focusing primarily on work done in Australia. Conversely, when interesting new findings arise from research done on Australian plants and animals, researchers elsewhere in the world should consider them as potentially relevant for their context rather than assuming that they are quirks of a globally unusual ecosystem.
The infertility of Australian soils underpins much of our understanding of how Australian ecosystems differ from the rest of the world (Braithwaite, 1990; Orians and Milewski, 2007; Kooyman et al., 2016). Surprisingly, we did not find evidence that Australian soils have unusually low nitrogen or phosphorus content (Figures 1F,G and Supplementary Tables S1, S3). However, our other results are broadly consistent with the known abundance of ancient and weathered soils in the arid interior of the country, where infertile soils tend to have high pH, pedogenic carbonates are relatively common, and sodic soils are ubiquitous and diverse (Isbell, 1996; Rengasamy, 2002; de Caritat et al., 2011; Eldridge et al., 2018). High total exchangeable bases in Australia are likely driven by the predominance of arid areas with alkaline soils, as it is related to both moisture and pH (see Figure 2B in Huston, 2012). Such conditions will make soil nutrients such as phosphorus, nitrogen, and magnesium less available to plants (James et al., 2005; Huston, 2012; Singh et al., 2013). While our data do support the overarching hypothesis that low and variable resource availability is important in Australian ecosystems, our analyses demonstrated that Australia is not unique in this way. Generally low and unpredictable resource availability and infertile soils are characteristics that Australia shares with Africa (Supplementary Figure S4; Bationo et al., 2006; He et al., 2021), as are higher frequency of fire events (Supplementary Figure S9), and aridity (Figure 1).
It is perhaps unsurprising that overall similarities in plant characteristics (Figure 4) are seen within the continents that formed ancient continental mass Laurasia (which included the northern hemisphere continents of North America, Europe and Asia) and within those that were Gondwana (the great southern land mass that included what is now Africa, South America and Australia); these groupings may reveal underlying differences in the biota stemming from long, separated evolutionary histories within these two land masses (Raymond, 1987; Lamont and He, 2012). The higher proportion of evergreen species (Supplementary Figure S7) relative to other continents, among numerous other similarities which could have arisen through their shared Gondwanan history (Milewski and Bond, 1982; Cowling and Lamont, 1998; Bond and Keeley, 2005). However, the distinctiveness of some Australian plant traits globally (e.g., lower specific leaf area and leaf N) will also be related to the known functional distinctiveness of plant clades with large radiations in Australia, including Proteaceae (Cornwell et al., 2014). It is also likely that some of the differences in plant traits between continents are related to the presence of different biomes. Exploring the extent to which the climate, flora and fauna within particular biomes vary between continents would be a worthwhile direction for future research (e.g., Gross et al., 2017).
Clear differences between the hemispheres are evident in several traits, including the number of frost days and mean annual temperature (Figure 1), soil salinity (Supplementary Figure S6), and proportions of evergreen and woody plant species (Supplementary Figure S7). Southern hemisphere continents tend to be more different from the global means, with nine of the 10 highest absolute z-score values found on Southern hemisphere continents, and that Asia and North America are much closer to the global means across the 85 biotic and abiotic variables than the other continents (Figure 3). In general, we found far fewer connections between than within northern and southern hemisphere continents (Figure 4). These findings lend further support to the long-standing notion that there are greater differences between the continents of northern and the southern hemispheres than within them (e.g., in productivity – Box, 2002; in latitudinal gradients of biodiversity – Chown et al., 2004; in metabolic rates – Watson et al., 2014; in species turnover – Leslie et al., 2012; in arid landscapes – Morton et al., 2011). The hemispheric differences we demonstrate are likely due to differences in the history of glaciation (de Caritat et al., 2012), and dissimilarities in climate (Figure 1 and Supplementary Figures S2, S3; e.g., more extreme winter temperatures of northern latitudes, more variable and less predictable rainfall patterns in southern latitudes; Chown et al., 2004) driven by the unequal distribution of land masses, and the influence of ancient supercontinents Laurasia and Gondwana. The differences between continents of the two hemispheres may explain the common perception of Australia being unusual – partly because many of Australia’s colonists and early scientific explorers drew their heuristic baselines from Europe, and partly because much of the existing ecological data comes from Europe and North America (e.g., Cornwell et al., 2018), which are very similar to each other (Figure 4). For instance, Australia and Africa do sit at the lower end of the spectrum for soil nitrogen and phosphorus (Supplementary Figure S4), compared to the higher levels of both elements in European and North American soils due to the relatively recent glaciation history of those continents (de Caritat et al., 2012). Australia also sits at the opposite end of the global spectrum to Europe and North America for several variables, such as soil total nitrogen and pH, mammal annual production rate to weaning, sunshine percent, mean sea surface temperature and a range of plant characteristics (e.g., Figures 1, 2 and Supplementary Figure S3).
We have shown that overall, Australia should be considered as well within global environmental variation in a macro-ecological, geological and climatological sense. While our analyses show that Australia has several features in which it is significantly different to global means, and is the continent that was distinctive most often across our variables, Australia does not appear to be a global outlier overall. Instead, we have shown that Australia tends to be more similar to the other southern hemisphere continents than it is to northern hemisphere continents, sharing characteristics such as highly weathered and infertile soils, hot and dry climate, and frequent fire, and resource conservative plants with Africa. The data also show that, while Asia is the closest to the global mean across the range of characteristics considered, and Europe and North America are very similar to each other, all the continents have characteristics in which they are different or distinctive. For instance, Europe has a low proportion of woody species (Figure 2), a low sea surface temperature (Supplementary Figure S3) and diurnal temperature range (Supplementary Figure S2), mammals with a high production rate to weaning (Figure 2), and is distinctive in soil total nitrogen (Supplementary Table S3); Africa has unusually small birds (Supplementary Figure S8 and Supplementary Table S3) and is distinctive in numerous attributes of terrestrial climate (Supplementary Table S3); North America has gravelly soil (Supplementary Figure S6), low and distinctive seed mass (Supplementary Figure S7 and Supplementary Table S3) and short lived birds (Supplementary Figure S8 and Supplementary Table S3); Asia has a high concentration of silicate in seawater (Supplementary Figure S2) and low marine NPP (Supplementary Figure S9 and Supplementary Table S3); and South America has acidic soils with low phosphorus (Figure 2 and Supplementary Figure S4), plants with large leaves (Supplementary Figure S7 and Supplementary Table S3), and high NPP and decomposition (Figure 2, Supplementary Figure S9, and Supplementary Table S3). Perhaps finding that each continent is unique in its own way should not be surprising, given that each has different geological histories, climates, and latitudinal range spans, and through historical contingency have accumulated different assemblages of plants and animals. Thus, our findings are consistent with the wise words of Dr. Seuss, “From there to here and here to there, funny things are everywhere” (Seuss, 1960, p. 9).
Data availability statement
Publicly available datasets were analyzed in this study. Code for the analyses can be found at: https://bitbucket.org/habacucfm/is_australia_weird.
Author contributions
HF-M and AM designed the original project. HF-M, RD, and AM wrote the manuscript. WC, GP, JA, and HF-M carried out analyses. WC, SN, SL, JC, SB, LS, AC, DE, MG, RB, AV, AP, DC, GC, and AS were major contributors through development of initial ideas, datasets curation, and writing. JA, PR, JHC, JMC, FH, JK, ÜN, and JP contributed data sets, discussions, and revised drafts. All authors contributed to manuscript revision, read, and approved the submitted version.
Funding
AM was supported by funding from the Australian Research Council (DP140102861). This study was funded by the U.S. National Science Foundation (NSF) Long-Term Ecological Research program (DEB-1234162) at the Cedar Creek LTER site. ÜN was supported by the Estonian Ministry of Science and Education (institutional grant IUT-8-3) and European Commission through European Regional Development Fund (Center of Excellence EcolChange). JP was supported by the European Research Council Synergy grant ERC-2013-SyG-610028 IMBALANCE-P. The study has been supported by the TRY initiative on plant traits (http://www.try-db.org). TRY is currently supported by DIVERSITAS/Future Earth and the German Centre for Integrative Biodiversity Research (iDiv) Halle-Jena-Leipzig.
Acknowledgments
We thank the Evolution & Ecology Research Centre for supporting HF-M and funding the working group. We thank the two reviewers for their helpful comments that improved this manuscript. The TRY initiative and database is hosted, developed and maintained by JK and G. Boenisch (Max Planck Institute for Biogeochemistry, Jena, Germany).
Conflict of interest
The authors declare that the research was conducted in the absence of any commercial or financial relationships that could be construed as a potential conflict of interest.
Publisher’s note
All claims expressed in this article are solely those of the authors and do not necessarily represent those of their affiliated organizations, or those of the publisher, the editors and the reviewers. Any product that may be evaluated in this article, or claim that may be made by its manufacturer, is not guaranteed or endorsed by the publisher.
Supplementary material
The Supplementary material for this article can be found online at: https://www.frontiersin.org/articles/10.3389/fevo.2023.1073842/full#supplementary-material
References
Bates, D., Maechler, M., Bolker, B., Walker, S., Christensen, R. H. B., Singmann, H. K., et al. (2014). Package lme 4. Vienna: R Foundation for Statistical Computing.
Bationo, S., Hartemink, A. E., Lungo, O., Naimi, M., Okoth, P., Smaling, E. M. A., et al. (2006). "African soils: their productivity and profitability of fertilizer use." In African Fertilizer Summit. Abuja, Nigeria.
Beadle, N. C. W. (1966). Soil phosphate and its role in molding segments of the Australian flora and vegetation, with special reference to xeromorphy and sclerophylly. Ecology 47, 992–1007. doi: 10.2307/1935647
Bond, W. J., and Keeley, J. E. (2005). Fire as a global “herbivore”: the ecology and evolution of flammable ecosystems. Trends Ecol. Evol. 20, 387–394. doi: 10.1016/j.tree.2005.04.025
Box, E. O. (2002). Vegetation analogs and differences in the northern and southern hemispheres: a global comparison. Plant Ecol. 163, 139–154. doi: 10.1023/A:1020901722992
Bradstock, R. A., Williams, R. J., and Gill, A. M.. (2012). Flammable Australia: fire regimes, biodiversity and ecosystems in a changing world. Collingwood, Melbourne: CSIRO Publishing.
Braithwaite, R. W. (1990). Australia's unique biota: implications for ecological processes. J. Biogeogr. 17, 347–354. doi: 10.2307/2845364
Burness, G. P., Diamond, J., and Flannery, T. (2001). Dinosaurs, dragons, and dwarfs: the evolution of maximal body size. Proc. Natl. Acad. Sci. 98, 14518–14523. doi: 10.1073/pnas.251548698
Ceballos, G., and Brown, J. H. (1995). Global patterns of mammalian diversity, endemism, and endangerment. Conserv. Biol. 9, 559–568. doi: 10.1046/j.1523-1739.1995.09030559.x
Chapman, Arthur D. (2009). Numbers of living species in Australia and the world. Toowoomba, Australia: Australian Biodiversity Information Services.
Chown, S. L., Sinclair, B. J., Leinaas, H. P., and Gaston, K. J. (2004). Hemispheric asymmetries in biodiversity—a serious matter for ecology. PLoS Biol. 2:e406. doi: 10.1371/journal.pbio.0020406
Christenhusz, M. J. M., and Byng, J. W. (2016). The number of known plants species in the world and its annual increase. Phytotaxa 261, 201–217. doi: 10.11646/phytotaxa.261.3.1
Clarke, M. (1876). “Preface,” in Sea spray and smoke drift. Ed. A. Lindsay (Melbourne: Clarson, Messina).
Coley, P. D., and Barone, J. A. (1996). Herbivory and plant defenses in tropical forests. Annu. Rev. Ecol. Syst. 27, 305–335. doi: 10.1146/annurev.ecolsys.27.1.305
Cornwell, W. K., Cornelissen, J. H. C., Amatangelo, K., Dorrepaal, E., Eviner, V. T., Godoy, O., et al. (2008). Plant species traits are the predominant control on litter decomposition rates within biomes worldwide. Ecol. Lett. 11, 1065–1071. doi: 10.1111/j.1461-0248.2008.01219.x
Cornwell, W. K., Pearse, W. D., Dalrymple, R. L., and Zanne, A. E. (2018). What we (don’t) know about global plant diversity. bioRxiv. doi: 10.1111/ecog.04481
Cornwell, W. K., Westoby, M., Falster, D. S., FitzJohn, R. G., O'Meara, B. C., Pennell, M. W., et al. (2014). Functional distinctiveness of major plant lineages. J. Ecol. 102, 345–356. doi: 10.1111/1365-2745.12208
Cowling, R. M., and Lamont, B. B. (1998). On the nature of Gondwanan species flocks: diversity of Proteaceae in Mediterranean South-Western Australia and South Africa. Aust. J. Bot. 46, 335–355. doi: 10.1071/BT97040
Cunningham, S. A., Summerhayes, B., and Westoby, M. (1999). Evolutionary divergences in leaf structure and chemistry, comparing rainfall and soil nutrient gradients. Ecol. Monogr. 69, 569–588. doi: 10.1890/0012-9615(1999)069[0569:EDILSA]2.0.CO;2
Darwin, Charles, and Keynes, Richard Darwin. (2001). Charles Darwin's beagle diary. Cambridge: Cambridge University Press.
de Caritat, P., Cooper, M., and Wilford, J. (2011). The pH of Australian soils: field results from a national survey. Soil Research 49, 173–182. doi: 10.1071/SR10121
de Caritat, P., and Reimann, C., NGSA Project Team, GEMAS Project Team (2012). Comparing results from two continental geochemical surveys to world soil composition and deriving predicted empirical global soil (PEGS2) reference values. Earth Planet. Sci. Lett. 319–320, 269–276. doi: 10.1016/j.epsl.2011.12.033
Dodson, J. R., and Westoby, M. (1985). “Are Australian ecosystems different? In proceedings of the ecological Society of Australia” in Dodson and Mark Westoby. ed. R. John (University of New South Wales, Sydney, Australia: Ecological Society of Australia; Distributed by Blackwell Scientific)
Du, E., Terrer, C., Pellegrini, A. F. A., Ahlström, A., van Lissa, C. J., Zhao, X., et al. (2020). Global patterns of terrestrial nitrogen and phosphorus limitation. Nat. Geosci. 13, 221–226. doi: 10.1038/s41561-019-0530-4
Eldridge, D. J., Maestre, F. T., Koen, T. B., and Delgado-Baquerizo, M. (2018). Australian dryland soils are acidic and nutrient-depleted, and have unique microbial communities compared with other drylands. J Biogeogr 45, 2803–2814. doi: 10.1111/jbi.13456
Enquist, B. J., Norberg, J., Bonser, S. P., Violle, C., Webb, C. T., Henderson, A., et al. (2015). “Chapter nine - scaling from traits to ecosystems: developing a general trait driver theory via integrating trait-based and metabolic scaling theories” in Advances in ecological research. eds. S. Pawar, G. Woodward, and A. I. Dell (Academic Press).
FAO (2016). The state of world fisheries and aquaculture 2016. Contributing to food security and nutrition for all. In, 200. Rome: FAO. pp.200.
Fraser, A. (1977). The use of canalized characters to specify the limits of ecological niches. Am. Nat. 111, 196–198. doi: 10.1086/283152
Gelman, A. (2008). Scaling regression inputs by dividing by two standard deviations. Stat. Med. 27, 2865–2873. doi: 10.1002/sim.3107
Greenwood, D. R., Wilf, P., Wing, S. L., and Christophel, D. C. (2004). Paleotemperature estimation using leaf-margin analysis: is Australia different? PALAIOS 19, 129–142. doi: 10.1669/0883-1351(2004)019<0129:PEULAI>2.0.CO;2
Gross, N., Le Bagousse-Pinguet, Y., Liancourt, P., Berdugo, M., Gotelli, N. J., and Maestre, F. T. (2017). Functional trait diversity maximizes ecosystem multifunctionality. Nat. Ecol. Evol. 1:0132. doi: 10.1038/s41559-017-0132
Hadden, M. (2007). “Fisheries and their management” in Marine ecology. eds. S. D. Connell and B. M. Gillanders (South Melbourne: Oxford University Press)
Harrison, X. A., Donaldson, L., Correa-Cano, M. E., Evans, J., Fisher, D. N., Goodwin, C. E. D., et al. (2018). A brief introduction to mixed effects modelling and multi-model inference in ecology. PeerJ 6:e4794. doi: 10.7717/peerj.4794
He, X., Augusto, L., Goll, D. S., Ringeval, B., Wang, Y., Helfenstein, J., et al. (2021). Global patterns and drivers of soil total phosphorus concentration. Earth Syst. Sci. Data 13, 5831–5846. doi: 10.5194/essd-13-5831-2021
Holt, B. G., Costa, G. C., Penone, C., Lessard, J.-P., Brooks, T. M., Davidson, A. D., et al. (2018). Environmental variation is a major predictor of global trait turnover in mammals. J. Biogeogr. 45, 225–237. doi: 10.1111/jbi.13091
Holt, B. G., Lessard, J.-P., Borregaard, M. K., Fritz, S. A., Araújo, M. B., Dimitrov, D., et al. (2013). An update of Wallace’s zoogeographic regions of the world. Science 339, 74–78. doi: 10.1126/science.1228282
Hopper, S. D. (2009). OCBIL theory: towards an integrated understanding of the evolution, ecology and conservation of biodiversity on old, climatically buffered, infertile landscapes. Plant Soil 322, 49–86. doi: 10.1007/s11104-009-0068-0
Huston, M. A. (2012). Precipitation, soils, NPP, and biodiversity: resurrection of Albrecht’s curve. Ecol. Monogr. 82, 277–296. doi: 10.1890/11-1927.1
Isbell, Raymond Frederick. (1996). The Australian soil classification. (Melbourne: CSIRO Australia).
James, J. J., Tiller, R. L., and Richards, J. H. (2005). Multiple resources limit plant growth and function in a saline-alkaline desert community. J. Ecol. 93, 113–126. doi: 10.1111/j.0022-0477.2004.00948.x
Jetz, W., and Rubenstein, D. R. (2011). Environmental uncertainty and the global biogeography of cooperative breeding in birds. Curr. Biol. 21, 72–78. doi: 10.1016/j.cub.2010.11.075
Kirkby, C. A., Kirkegaard, J. A., Richardson, A. E., Wade, L. J., Blanchard, C., and Batten, G. (2011). Stable soil organic matter: a comparison of C:N:P:S ratios in Australian and other world soils. Geoderma 163, 197–208. doi: 10.1016/j.geoderma.2011.04.010
Kooyman, R. M., Laffan, S. W., and Westoby, M. (2016). The incidence of low phosphorus soils in Australia. Plant Soil 412, 143–150.
Lamont, B. B., and He, T. (2012). Fire-adapted Gondwanan angiosperm floras evolved in the cretaceous. BMC Evol. Biol. 12:223. doi: 10.1186/1471-2148-12-223
Lee, A. K., and Cockburn, A. (1985). Evolutionary ecology of marsupials. (Cambridge: Cambridge University Press).
Leslie, A. B., Beaulieu, J. M., Rai, H. S., Crane, P. R., Donoghue, M. J., and Mathews, S. (2012). Hemisphere-scale differences in conifer evolutionary dynamics. Proc. Natl. Acad. Sci. U. S. A. 109, 16217–16221. doi: 10.1073/pnas.1213621109
Mazel, F., Wüest, R. O., Lessard, J.-P., Renaud, J., Ficetola, G. F., Lavergne, S., et al. (2017). Global patterns of β-diversity along the phylogenetic time-scale: the role of climate and plate tectonics. Glob. Ecol. Biogeogr. 26, 1211–1221. doi: 10.1111/geb.12632
McCarthy, P, and Orchard, AE. (1995). Flora of Australia. Volume 16–Elaeagnaceae, Proteaceae 1. Australian Biological Resources Study and CSIRO Publishing: Melbourne.
Milewski, A. V., and Bond, W. J. (1982). “Convergence of myrmecochory in mediterranean Australia and South Africa” in Ant-plant interactions in Australia. ed. R. C. Buckley (Dordrecht: Springer Netherlands).
Milewski, A. V., and Diamond, R. E. (2000). Why are very large herbivores absent from Australia? A new theory of micronutrients. J. Biogeogr. 27, 957–978. doi: 10.1046/j.1365-2699.2000.00436.x
Moles, A. T., Ackerly, D. D., Tweddle, J. C., Dickie, J. B., Smith, R., Leishman, M. R., et al. (2007). Global patterns in seed size. Glob. Ecol. Biogeogr. 16, 109–116. doi: 10.1111/j.1466-8238.2006.00259.x
Moles, A. T., Warton, D. I., Warman, L., Swenson, N. G., Laffan, S. W., Zanne, A. E., et al. (2009). Global patterns in plant height. J. Ecol. 97, 923–932. doi: 10.1111/j.1365-2745.2009.01526.x
Morton, S. R., Stafford Smith, D. M., Dickman, C. R., Dunkerley, D. L., Friedel, M. H., McAllister, R. R. J., et al. (2011). A fresh framework for the ecology of arid Australia. J. Arid Environ. 75, 313–329. doi: 10.1016/j.jaridenv.2010.11.001
Orians, G. H., and Milewski, A. V. (2007). Ecology of Australia: the effects of nutrient-poor soils and intense fires. Biol. Rev. 82, 393–423. doi: 10.1111/j.1469-185X.2007.00017.x
Peel, M. C., Finlayson, B. L., and McMahon, T. A. (2007). Updated world map of the Köppen-Geiger climate classification. Hydrol. Earth Syst. Sci. 4, 1633–1644. doi: 10.5194/hess-11-1633-2007
Péron, M. F. (1809). A voyage of discovery to the southern hemisphere: Performed by order of the emperor Napoleon, during the years 1801, 1802, 1803, and 1804 [translated from the French]. London: Richard Phillips.
Pillans, B. (2007). Pre-quaternary landscape inheritance in Australia. J. Quat. Sci. 22, 439–447. doi: 10.1002/jqs.1131
Pinheiro, J., Bates, D., DebRoy, S., and Sarkar, D., R Core Team (2014). nlme: linear and nonlinear mixed effects models. R package version 3.1-117. Available at: http://cran.r-project.org/web/packages/nlme/index.html.
R Core Team (2016). R: A language and environment for statistical computing [Internet]. Vienna, Austria: R Foundation for Statistical Computing.
Raymond, A. (1987). Paleogeographic distribution of early Devonian plant traits. PALAIOS 2, 113–132. doi: 10.2307/3514640
Reich, P. B. (2012). Key canopy traits drive forest productivity. Proc. Biol. Sci. 279, 2128–2134. doi: 10.1098/rspb.2011.2270
Rengasamy, P. (2002). Transient salinity and subsoil constraints to dryland farming in Australian sodic soils: an overview. Aust. J. Exp. Agric. 42, 351–361. doi: 10.1071/EA01111
Ritchie, E. G., and Johnson, C. N. (2009). Predator interactions, mesopredator release and biodiversity conservation. Ecol. Lett. 12, 982–998. doi: 10.1111/j.1461-0248.2009.01347.x
Savage, J. (2015). Australian fisheries and aquaculture statistics 2015, Fisheries Research and Development Corporation project 2016-246. ABARES: Canberra.
Singh, K., Singh, B., and Singh, R. R. (2013). Effect of land rehabilitation on physicochemical and microbial properties of a sodic soil. Catena 109, 49–57. doi: 10.1016/j.catena.2013.05.006
Squires, V. R. (1988). Landscape: a southern hemisphere perspective. Earth Sci. Rev. 25, 481–484. doi: 10.1016/0012-8252(88)90014-1
Terribile, L. C., Olalla-Tárraga, M. Á., Morales-Castilla, I., Rueda, M., Vidanes, R. M., Rodríguez, M. Á., et al. (2009). Global richness patterns of venomous snakes reveal contrasting influences of ecology and history in two different clades. Oecologia 159, 617–626. doi: 10.1007/s00442-008-1244-2
Tydecks, L., Jeschke, J. M., Wolf, M., Singer, G., and Tockner, K. (2018). Spatial and topical imbalances in biodiversity research. PLoS One 13:e0199327. doi: 10.1371/journal.pone.0199327
Watson, S.-A., Morley, S. A., Bates, A. E., Clark, M. S., Day, R. W., Lamare, M., et al. (2014). Low global sensitivity of metabolic rate to temperature in calcified marine invertebrates. Oecologia 174, 45–54. doi: 10.1007/s00442-013-2767-8
Westoby, M. (1988). Comparing Australian ecosystems to those elsewhere. Bioscience 38, 549–556. doi: 10.2307/1310763
Wiens, J. A. (1991). Ecological similarity of Shrub-Desert Avifaunas of Australia and North America. Ecology 72, 479–495. doi: 10.2307/2937189
Wiens, J. A. (2016). Ecological challenges and conservation conundrums: Essays and reflections for a changing world. (John Wiley & Sons: West Sussex).
Wild, A. (1958). The phosphate content of Australian soils. Crop Pasture Sci. 9, 193–204. doi: 10.1071/AR9580193
Wool, D. (1980). On ecological inference from kurtosis and skewness of morphological characters. Popul. Ecol. 22, 263–272. doi: 10.1007/BF02530850
Keywords: continent, global comparison, biological, geological, climatological, macroecology
Citation: Flores-Moreno H, Dalrymple RL, Cornwell WK, Popovic G, Nakagawa S, Atkinson J, Cooke J, Laffan SW, Bonser SP, Schwanz LE, Crean AJ, Eldridge DJ, Garratt M, Brooks RC, Vergés A, Poore AGB, Cohen DR, Clark GF, Sen Gupta A, Reich PB, Cornelissen JHC, Craine JM, Hemmings FA, Kattge J, Niinemets &, Peñuelas J and Moles AT (2023) Is Australia weird? A cross-continental comparison of biological, geological and climatological features. Front. Ecol. Evol. 11:1073842. doi: 10.3389/fevo.2023.1073842
Edited by:
Matteo Marcantonio, Université catholique de Louvain, BelgiumReviewed by:
Patrice de Caritat, Geoscience Australia, AustraliaGreg R. Guerin, University of Adelaide, Australia
Copyright © 2023 Flores-Moreno, Dalrymple, Cornwell, Popovic, Nakagawa, Atkinson, Cooke, Laffan, Bonser, Schwanz, Crean, Eldridge, Garratt, Brooks, Vergés, Poore, Cohen, Clark, Sen Gupta, Reich, Cornelissen, Craine, Hemmings, Kattge, Niinemets, Peñuelas and Moles. This is an open-access article distributed under the terms of the Creative Commons Attribution License (CC BY). The use, distribution or reproduction in other forums is permitted, provided the original author(s) and the copyright owner(s) are credited and that the original publication in this journal is cited, in accordance with accepted academic practice. No use, distribution or reproduction is permitted which does not comply with these terms.
*Correspondence: Joe Atkinson, ai5hdGtpbnNvbkB1bnN3LmVkdS5hdQ==
†Present address
Julia Cooke and Alex Sen Gupta, School of Environment, Earth and Ecosystem Sciences, The Open University, Walton Hall, Buckinghamshire, United Kingdom