- 1Lushan Botanical Garden, Chinese Academy of Sciences, Jiujiang, China
- 2School of Ecology and Environment Studies, Nalanda University, Rajgir, India
- 3Departamento de Biodiversidade, Universidade Estadual Paulista (Unesp), Rio Claro, Brazil
Interpopulation variability in seed traits may drive the regeneration capacity of a species to colonize different environments. In the present study, we evaluated the variation in seed physical traits (mass / size, water imbibition, shape index) and physiological traits (germination) of five Polygonaceae species. Seeds of Polygonum lapathifolium var. salicifolium, P. lapathifolium, Reynoutria japonica, Rumex trisetifer, and R. obtusifolius were collected from two or three populations in Jiujiang, China. Physical seed traits were measured before germination tests conducted under different combinations of light and temperatures. Most species had a significant variation in seed physical and physiological traits, although populations are geographically close. Interpopulation variation in seed traits appeared to be species-specific, with the highest variation for R. japonica and lowest for R. trisetifer seeds. Germination response to temperature and light conditions also varied among species and populations, being mostly inhibited in the dark treatments. The light dependence of germination can be related to the small seed size, except for the round-seeded Rumex, depending on the temperature regime. Optimal temperature ranges mainly varied from 10/20°C to 25/35°C, with significant decreases in germination percentage at both coolest and warmest extremes. Germination requirements seem to be related to altitudinal gradients in populations of P. lapathifolium and R. japonica seeds.
Introduction
Variability in seed traits has been reported in different species, populations or individuals (see, e.g., Susko and Lovett-Doust, 2000; Lopez et al., 2008; Cervantes and Martín Gómez, 2019). Differences in seed (germination) traits among populations (i.e., within species) have been considered an effective evolutionary strategy that influence on species resilience by increasing the chances of reproduction under heterogeneous environmental conditions, thus minimizing the extinction risk (Venable and Brown, 1988). Hence, understanding interpopulation differences might help us addressing issues related to the evolution of variability patterns regarding plant regeneration from seeds. Seed physical traits (e.g., seed size/mass, shape, color, seed coat thickness, permeability, etc.), for instance, are directly or indirectly involved in regulating germination responses and many seed functions such as dispersal, type and level of dormancy, formation of the soil seed bank, seedling establishment, survival, and competitive ability (Eriksson, 1999; Larios et al., 2014; Larson and Funk, 2016; Saatkamp et al., 2019). Therefore, these traits have been related to plant fitness and species persistence across ecosystems (Atis et al., 2011; Xu et al., 2014; Bhatt et al., 2016, 2021a; Bu et al., 2016). Evolutionary history, environmental context, genetic constraints, and plasticity are responsible for variation in seed traits at both intra- and inter-species levels (Violle et al., 2012; Ge et al., 2020).
Within species, interpopulation variation is vital to distinguish the ecological and evolutionary drivers shaping regeneration traits (Moreira et al., 2012). Interpopulation variability of ecological traits generally occur either due to (i) differences in environmental conditions among the different populations (Nicotra et al., 2010; Cochrane et al., 2014) or (ii) hereditary characteristics of populations (Cheptou et al., 2008; Cochrane et al., 2015). In addition, other factors can be responsible for shaping the interpopulation variability in seed traits, including local climate, habitat characteristics, inter- and intra-species competition levels, predation, resource provisioning, and epigenetics (Kleunen et al., 2001; Tautenhahn et al., 2008; Saatkamp et al., 2019). The variability in seed germination within a species, when seeds are collected from different populations, has been linked to spatial variability due to climatic and edaphic parameters or a differentiated gene pool (Elnaggar et al., 2019; Bhatt et al., 2021b,c). Most of the studies so far focused on testing interpopulation variation regarding seed mass or size and their effect on seed germination (Xu et al., 2016; Kołodziejek, 2017; Moya et al., 2017; Bhatt et al., 2019). However, few studies investigated interpopulation variation in other physical traits, like seed shape and color and their role in regulating seed dormancy and germination (but see Zhang and Maun, 1990; Mölken et al., 2005; Atis et al., 2011; Bhatt et al., 2016).
Generally, seed germination requirements are synchronized with the population’s environmental condition to increase the possibility of seedling survival and establishment (Baskin and Baskin, 2014). Studies have shown that interpopulation variation in seed dormancy and germination is broadly correlated with abiotic factors, such as altitude, latitude, temperature, soil moisture, and habitat type (Wagmann et al., 2012; Fernández-Pascual et al., 2013; Cochrane et al., 2014; Pendleton and Pendleton, 2014). Because different populations among different localities may experience different natural selection pressure, they may lead to local adaptation over time. For instance, higher interpopulation variability in germination responses has been reported in populations living in unpredictable environmental conditions than those living in more stable (i.e., less variable) conditions (Meyer and Allen, 1999). Therefore, the same species growing in different populations can respond differently to the same environmental signals based on the selective pressures acting on the population (Oduor et al., 2016; Amini et al., 2017).
Given that interpopulation variation usually reflects the environmental adaptation of species (Kyle and Leishman, 2009), the present study focused on interpopulation variation in seed physical (mass, size, shape, color, etc.) and physiological (germination responses) traits of five Polygonaceae species. Germination at species level has been previously investigated for this family, but mostly using seeds from a single population, as reported for seeds of Polygonum lapathifolium (Timson, 1965; Staniforth and Cavers, 1979), Reynoutria japonica (Mariko et al., 1993), and Rumex obtusifolius (Hand et al., 1982; Van Assche and Vanlerberghe, 1989; Benvenuti et al., 2001). In the present study, we hypothesized that different populations of species growing in different microclimates might also differ in their seed morphology and germination strategy. To test this hypothesis, we examined the following questions (i) is there a difference in seed physical traits (size, mass, shape, and color) according to the seed population? (ii) are there any light and temperature mediated mechanisms affecting seed physiological (germination) traits under laboratory conditions? (iii) is there a different seed germination response to light and temperature among the seed populations within each study species?
Materials and methods
Seed collection
In 2020, we collected seeds of five species of Polygonaceae from two to three populations at the time of their natural dispersal to ensure that the seeds were physiologically in their mature stage (Table 1, Figure 1). We obtained permission from Jiujiang Forestry Bureau, China, for seed collection. The voucher specimens for each species were collected from each location and deposited in the herbarium of Lushan Botanical Garden, China. For each species and each population, seeds were collected from 25 to 30 randomly chosen plants to represent the genetic diversity. After collection, the seeds were cleaned and tested for germination within a week.
The seed collection areas fall under the wet subtropical monsoon climatic zone. The lower altitude of the mountain is subtropical with warm and humid climate, whereas the higher altitude is warm temperate with cool and humid climate. The mean annual temperature is 16–18°C and mean annual precipitation range between 1,000 and 1,600 mm. The average temperature of January is 4.4°C. The average temperature in July is 29.6°C, with an extreme maximum temperature of 40.2°C (Hong et al., 2013; Xie et al., 2013).
To determine the seed dispersal syndrome, we have taken into account fruit/seed morphology, the available literature, as well as our personal observations, and come to conclusion that seeds of all five species have a relatively high potential to be dispersed by more than one of these three dispersal modes (anemochory, hydrochory, and/or zoochory). These multiple dispersal vectors may facilitate seed dispersal even among fragmented populations.
Seed physical traits
A Stereo Microscope (Nikon SMZ800N) fitted with a microscope camera IMG-SC600C was used to examine the shape, dimensions (length, width, and height), and color of the seed. Fifteen seeds were examined for each species/population by ventrally attaching them to filter paper using double-sided sticky tape. Seed mass was determined at the time of collection (fresh mass) from three 100-seed replicates per species, using a Sartorius electronic balance (Sartorius Co., Göttingen, Germany).
Water imbibition
Seed permeability to water was assessed by recording the mass of three 25-seed replicates before and after moistening them in 9-cm-diameter Petri dishes containing two sheets of Whatman No. 1 filter paper. Seeds were moistened for 24 h at room temperature (22 ± 2°C) using 10 mL of distilled water. The water uptake by seeds was calculated using the formula (Water absorption (%) = [(W2 − W1)/W1] × 100), where W2 is the mass of the seeds after imbibition for a given interval and W1 is the initial seed mass (Baskin et al., 2004).
Effect of temperature and light on seed germination (physiological traits)
Seeds were surface sterilized in 0.50% sodium hypochlorite for 1 min, then washed thrice with deionized water to avoid attack by fungi. To determine the effect of temperature and light, seed germination tests were conducted in incubators (Kesheng incubators, Model- DRX-800C- LED, China) set at five different alternate temperature regimes (5/10, 10/20, 20/30, 25/35, and 35/40°C) in 24-h darkness (dark treatment) and/or 12 h light/12 h darkness (light treatment). The incubators were fitted with cool white fluorescent tubes (60 μmol photons M−2S−1). These temperature regimes were chosen to stimulate the average temperature of natural habitat (seed collection site) for different months (i.e., 5/10°C—December to February; 10/20°C—March, April, and October, November; 20/30°C—May, June and September; 25/35°C—July and August). The highest temperature regime (35/40°C) was used to investigate the ability of seeds to tolerate the high temperature during germination. Seeds were exposed to 12 h light at the highest temperature within each alternating temperature regime.
Seeds were sown in 9-cm Petri dishes containing three disks of Whatman No. 1 filter paper moistened with 10 ml of distilled water and placed in incubators. Darkness was achieved by wrapping Petri dishes in two layers of aluminum foil. Four replicates of 25 seeds each were used for each treatment. The seeds were considered to be germinated with the emergence of the radicle (≥2 mm). Germinated seeds were counted and removed daily for a 30-days period. However, seeds incubated in the dark regimes were checked only at the end of the test. At the end of the germination test, all the ungerminated seeds from the light treatment were dissected to evaluate the embryo status and viabilities of the nongerminated seeds (living and therefore white; turgid and brown and, therefore, dead) under a stereoscope.
Data analysis
The seed physical traits (including seed mass, seed dimensions such as length, width, height, and water imbibition in 24 h) met the assumptions of both the Shapiro–Wilk normality test and Levene’s test for equality of variances. Therefore, depending on the number of studied populations (2 or 3) for each species, the independent-samples t-test or one-way ANOVA were used to compare interpopulation variation in seed physical traits. The physiological traits (germination data) did not meet the assumptions of normality and equality of variances; therefore, the relationship between mean percentage of germination (dependent variable) and the value of three predictors (population, incubation temperature and light) was compared for each species independently using a generalized linear model (GLM) with a Poisson probability distribution and identity-link functions. The effects of three predictors and their interaction in the model were tested by Wald Chi-square (X2) values. All statistical analyses were performed using SPSS 16.
Results
Polygonum lapathifolium var. salicifolium
No difference was detected regarding seed mass and seed length between the two populations (Pls1 and Pls2), but the other physical traits (water imbibition, seed width, height, and shape index) differed significantly between populations (p < 0.05; Table 2). GLM revealed a significant effect of population, incubation temperature, light, and their interactions on the percentage of germination (p < 0.001; Table 3). Seeds of both populations had negligible germination (<12%) in the dark treatment (Figure 2). In the light treatment, optimum temperature regime differed for populations. Seeds of Pls1 reached maximum germination of 56% at 20/30°C, whereas seeds of Pls2 germinated at comparatively higher temperature regimes, reaching germination of 84 and 83% under 25/35°C and 35/40°C, respectively (Figure 2; raw data available at Supplementary Table S1).
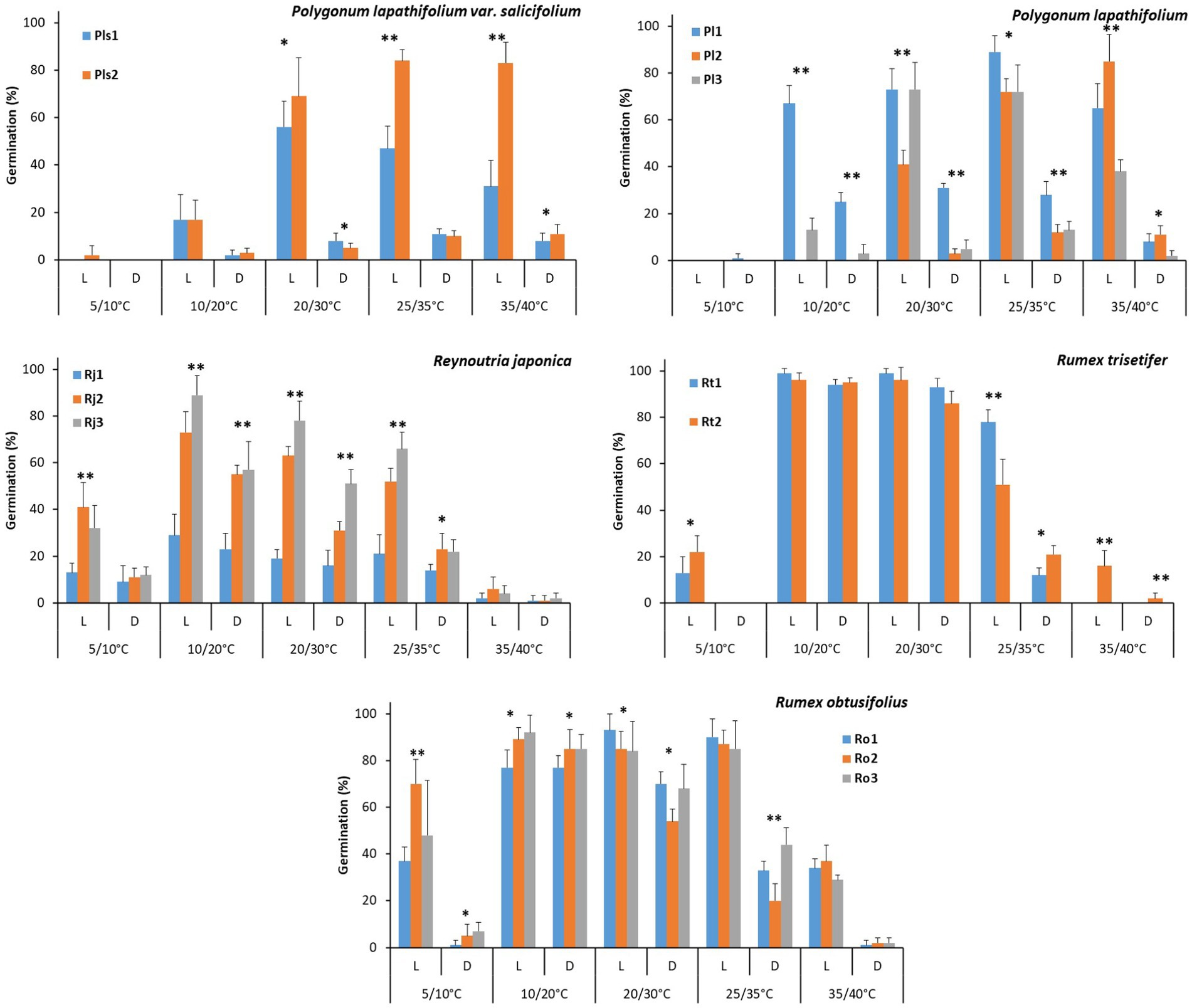
Figure 2. Influence of population, incubation temperatures, and photoperiod on mean seed germination percentage of five Polygonaceae species. Significance values of different factors are indicated in Table 2. Bars with * or ** signs indicate germination percentage differed significantly (P < 0.01 or < 0.001) between and /or among the populations at particular incubation conditions.
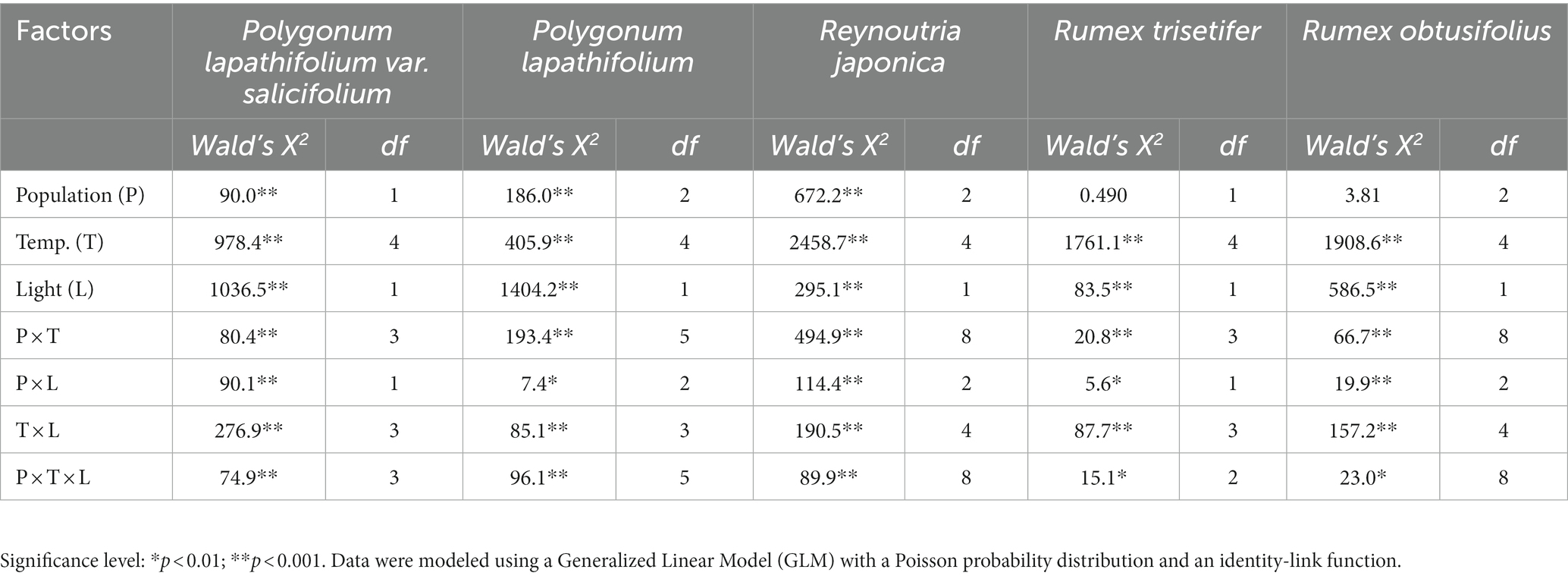
Table 3. Effect of population (P), incubation temperature (T), and photoperiod (L) on seed germination percentage of five Polygonaceae species.
Polygonum lapathifolium
There was significant interpopulation variation in seed mass, length, width, and seed shape index (p < 0.05). However, seed height and water imbibition were not significant among the populations (Table 2). Populations, incubation temperature, light, and their interactions had a significant effect on seed germination (p < 0.001; Table 3). Dark treatment significantly inhibited germination compared to light treatment in most temperature regimes (Figure 2 and Supplementary Table S1). There was also a significant variation in the optimum germination temperature among populations (Pl1, Pl2, and Pl3). Seeds from Pl1 had a wide germination range, showing germination >65% from 10/20°C to 35/40°C, reaching 89% at 25/35°C (Figure 2 and Supplementary Table S1). A narrower germination window was found for Pl2 (germination ranged from 72 to 85% at warm temperatures of 25/35° and 35/40°C) and for Pl3 (72–73% at intermediate temperatures of 20/30° and 25/35°C).
Reynoutria japonica
Except for water imbibition, all other seed physical traits differed among populations, with a prominent 2.5-fold variation in seed mass (p < 0.05; Table 2). Furthermore, there was a significant effect of populations, incubation temperatures, light, and their interactions on the percentage of seed germination (p < 0.001; Table 3). The overall germination percentage varied 2 to 3 folds among populations (Rj1, Rj2, and Rj3). Seeds from Rj1 germinated a relatively low (<30%) in all treatments, irrespective of dark and light conditions. The other two populations had maximum germination from 52 to 89% at temperature regimes from 10/20°C to 25/35°C in the light, with a decrease under the dark treatment, which varied from 52 to 22% (Figure 2 and Supplementary Table S1).
Rumex trisetifer
Unlike the other species studied, none of the seed physical traits of R. trisetifer varied significantly (p = 0.85) between the two populations (Table 2). Populations also had no effect on germination responses, but incubation temperature, light, and their interactions significantly affected germination (p < 0.001; Table 3). Seeds of both populations germinated from 86 to 99% in the temperature regimes of 10/20° and 20/30°C, both in light and dark treatments (Figure 2 and Supplementary Table S1). However, germination decreased significantly in all other tested temperature regimes (5/10°C or ≥ 25/35°C), mainly in the dark.
Rumex obtusifolius
Three physical traits (water imbibition, seed width, and seed shape index) varied significantly among the populations (p < 0.05; Table 2), but no differences were found regarding seed mass, length, and height. Similar to R. trisetifer, populations had no effect on germination responses. However, incubation temperature, light, and their interactions significantly affected germination (p < 0.001; Table 3). At 10/20°C, all populations had a similar percentage of germination compared to light/dark treatments, ranging from 77 to 92%; dark conditions tended to decrease the percentage of germination at all other temperatures (Figure 2 and Supplementary Table S1). Optimal germination conditions differed among populations (Ro1, Ro2, and Ro3) in the light: germination of Ro1 reached ≥90% at 20/30°C and 25/35°C, while germination of Ro2 and Ro3 varied from 84 to 92% in a broader range of temperature regimes of 10/20°C to 25/35°C. Even at the coolest temperature regime (5/10°C), 70% seeds of Ro2 germinated.
Discussion
Germination responses can be difficult to predict over time and space, as they are affected by several environmental factors such as temperature, light, and soil moisture (Baskin and Baskin, 2014; Bhatt et al., 2019, 2020, 2021a). Identification of the environmental factors that are responsible for maintaining phenotypic variation in nature is essential to understand the evolutionary mechanisms of plants (Larios et al., 2014; Manzano-Piedras et al., 2014). Existence of interpopulation variability in seed traits is commonly found, and important for enhancing the ability of species to persist against climatic variability (Cochrane et al., 2015). In this study, we found a relatively high interpopulation variation in seed morphology and germination response (physical and physiological traits) of five Polygonaceae, although these species belong to the same family and were collected from geographically close locations (located approximately 4–32 km from each other). Despite the multiple seed dispersal vectors of the study species and relatively close distance between populations, it is important to note that the collected populations occur in a fragmented landscape, which may limit their gene flux from one population to others.
Intraspecific (interpopulation) trait variation could be due to environmental, genetic differences, or a combination of both genetic and environmental factors. Understanding the role of seed physical traits and their relationship with seed germination could thus be important for predicting which seed physical traits are more influential in affecting germination. However, until now, most of the studies mainly focused on the role of seed mass/size in germination responses (Wu and Du, 2007; Münzbergová and Plačková, 2010; Wu et al., 2011; Alstad et al., 2018; Chen et al., 2018). Despite differences between species, seeds of all studied species can be classified as small-sized, with a fresh mass varying from 0.45 to 4.44 mg. Small-seeded species have been recognized to be light-dependent (positively photoblastic) in different vegetation types worldwide, including temperate grasslands (Milberg et al., 2000), arid zones (Flores et al., 2011), and tropical rainforests (Aud and Ferraz, 2012). Such physical trait-germination relatedness may help to explain the germination patterns we found.
Only seeds of P. lapathifolium and R. japonica had significant interpopulation variability in seed mass and seed length. The smallest seed size found in the Rj1 population proportioned a low percentage of germination regardless of light and temperature treatments. However, other seed traits, such as seed width, seed height, and seed shape index, varied significantly among populations of most studied species. Differences in the environmental conditions among populations can be responsible for seed trait variability, as suggested to vegetative and floral plant traits (Kuppler et al., 2020). But in our study, one must take in account that seeds of the different species were collected from populations growing geographically in close to moderate distance localities (4–32 km). Therefore, we argue that spatial heterogeneity in edaphic factors (i.e., nutrients) or differences in the gene pool among populations can contribute to determining the interpopulation variability, especially in geographically distance populations. In addition to subtle contrasts in environmental gradients, different populations are highly likely to be subjected to different selection pressures under natural conditions, leading to the evolution of interpopulation variability in seed traits as an adaptive response that allows the species to survive under spatially heterogeneous landscapes (Ge et al., 2020).
The exception was found for R. trisetifer seeds, with no difference in seed traits among the studied populations. Additionally, both Rumex species had the least light-dependent germination responses, despite their small seed size and round seed shape. Round and small seeds, due to their compact structure, should be more easily incorporated into soil seed banks and therefore subjected to dark conditions or minimal light penetration in the upper layer of the soil (Benvenuti, 2007; Saatkamp et al., 2014). Funes et al. (1999) reported that compact seeds tend to persist for longer periods ungerminated in the soil. In contrast, in our case, the seeds of R. trisetifer and R. obtusifolius had the lowest shape index but high germination under both light and dark conditions, under optimal temperature. This indicates that their seeds would be promptly recruited rather form persistent seed banks. Factors other than seed physical traits may influence germination responses, such as adaptive responses and phylogenetic relatedness, regardless of population variability. Moreover, interactions of temperature and light have been pointed out as important drivers of the capacity of seeds to germinate depending on the species and specific temperature requirements (see Pons, 1992).
Seeds of all the five species were able to absorb water, indicating they do not show physical dormancy. Seeds of only two taxa (P. lapathifolium var. salicifolium and R. obtusifolius) had a significant variability in seed coat permeability (water imbibition) among populations. This variability in water imbibition among populations could be related to variation in coat hardness (thickness) due to spatial heterogeneity in habitat conditions, genetic background, and their interactions, which may be responsible for interpopulation variability in seed coat permeability as reported in other species (Jaganathan et al., 2019; Bhatt et al., 2021b). Bearing non-dormant seeds, all studied species were able to germinate immediately after dispersal, although the percentage of germination varied between or among populations and the tested temperature conditions. Fast germination could be related to the availability of sufficient precipitation throughout the year due to subtropical moist monsoonal climate in the seed collection sites (Kang et al., 2017). This contrasts with seasonal water restrictions, which are generally considered one of the main limitations for germination in most climatic conditions.
Temperature is one of the main environmental factors that regulate the germination, especially where availability of water is not a constraint for germination (Fenner and Thompson, 2005). Regarding thermal requirements, in the light, optimal germination ranges mainly varied from 10/20°C to 25/35°C, with significant decrease in germination percentage at both cooler and warmer extremes. However, some species, such as Polygonum spp., showed a marked variation in the optimal temperature requirement for germination depending on the population. Seeds of P. lapathifolium var. salicifolium from Pls2, for example, germinated equally well at the warmest tested temperatures, reaching >80% germination at 25/35°C and 35/40°C. Conversely, seeds from Pls1 attained maximum germination (56%) at a milder temperature regime (20/30°C). Seeds of P. lapathifolium also showed similar trends: The Pl2 population attained 85% germination under the warmest conditions (35/40°C), while Pl1 and Pl3 (both populations collected at elevation >1,000 m) attained maximum germination of 72–89% at temperatures 20/30°C and 25/35°C. Producing seeds with varying germination cues (temperature) may enhance fitness of these species in future climate change scenarios and reduce the risks of synchronizing recruitment in case of unfavorable conditions (Santo et al., 2015; Bhatt et al., 2020, 2021b).
An even higher interpopulation variability in maximum germination percentage was observed for R. japonica seeds—from 29% in Rj1 to 89% in Rj3, both at 10/20°C. Although Rj3 also germinated well (66%) at 25/35°C. This relatively wide temperature niche width (especially in Rj3) indicates such seeds (populations collected at elevation from 500 to 1,200 m) can better recruit when other environmental factors controlling seed germination (light &/or moisture) may be available beyond the normal window of seed germination season. Collection sites of R. japonica and P. lapathifolium populations had remarkable differences in altitude, which may be responsible for microclimatic contrasts. We argue that habitat heterogeneity and climatic variability contribute more toward determining the interpopulation variability rather than the geographic distance. Additionally, differences in germination requirements among populations of P. lapathifolium var. salicifolium could be attributed to the variation in competition, soil moisture, and mineral nutrition (Burton et al., 2000; Allison, 2002; Swain et al., 2006). Spatio-temporal heterogeneity in climatic conditions and edaphic factors among the population are reported to be the main driver for interpopulation variability (Kuppler et al., 2020), which ultimately lead to the interpopulation variability in seed morphology and germination traits (Baskin and Baskin, 2014; Hudson et al., 2015; Bhatt et al., 2021b).
Rumex species had less variation in germination percentage among the tested populations within their respective optimal ranges. Seeds of R. trisetifer germinated equally well at 10/20°C and 20/30°C (≥96%), while R. obtusifolius germinated equally well at 10/20°C, 20/30°C, and 25/35°C (89%). The relatively broad thermal germination niche indicates that seeds of R. obtusifolius can germinate throughout the year, although the germination percentage was significantly reduced during winter (December to February). Similarly, Polygonum spp. can germinate outside their normal germination window, when the temperature ranges between 20 and 35°C. These results further support that seed recruitment in our study species is widely spread throughout the growing season, and this will increase their long-term fitness by spreading the risk of germination failure. Besides this, these species have higher possibility to establish in a broad environmental condition (Carlucci et al., 2015) and will be less affected by climate change as compared to those have specific temperature requirement for germination. For example, R. trisetifer and R. japonica have a specific temperature requirement and thus their germination may be restricted to the specific time (i.e., between March to June or between September to November for R. trisetifer, and between March to April or between October to November for R. japonica).
Overall, seed germination was significantly inhibited at the lowest temperature regime (except for Ro2), although different species showed interpopulation variability in terms of germination percentage. Avoiding germination in winter (December to February), when the temperature is around 5/10°C, could be a survival strategy for these species, as the chances of seedling successful establishment would be extremely low due to cold and frost. Low-temperature conditions may induce physiological dormancy, while the cold stratification period also helps seeds to synchronize germination with a favorable season, as reported in Polygonum and Rumex spp. (Totterdell and Roberts, 1979; Bouwmeester and Karssen, 1992; Batlla and Benech-Arnold, 2005). However, at the highest temperature (35/40°C), one population of P. lapathifolium var. salicifolium (Pls2) and a population of P. lapathifolium (Pl2), both from the lowlands, germinated well (>80%). For all other species/populations, germination percentage was significantly reduced at the warmest condition. These results indicate that tolerance to high and low temperatures during the germination stage can vary between/among populations, depending on species (Cochrane, 2016). Therefore, it could be imperative to test the interpopulation variability under a wider range of temperature, in order to model regeneration behavior regarding threshold models (Bradford, 2002).
Here, we found general patterns of seed and germination traits common to all five Polygonaceae, such as small seed size and predominant light-dependent germination (except Rumex). However, we also identified particularities of the studied populations. The optimal temperature requirements for germination varied according to the population of the seeds, mostly for P. lapathifolium and R. japonica, seeds collected from comparatively longer altitudinal gradients. Furthermore, interpopulation variability among the studied species could be linked to maternal effects (Luzuriaga et al., 2006; Kagaya et al., 2011; Bhatt et al., 2019, 2021b). Heterogeneity in maternal habitat conditions where populations grow is likely to be the main factor contributing to interpopulation trait variability. Phylogenetically related species have been reported to share similar traits in a general sense (Losos, 2008). For example, some studies suggest that related species share similarities regarding seed traits, dormancy, and germination characteristics (Moles et al., 2005; Norden et al., 2009; Carta et al., 2016). Other studies argue that seed traits, dormancy, and germination characteristics are regulated by environmental cues rather than the phylogeny (Vandelook et al., 2008; Arana et al., 2016; Fang et al., 2017). Therefore, it is important to compare the physical and physiological traits of closely related species to better understand which factors (phylogeny and/or environment) are responsible for influencing regeneration strategies, aiding to improve our predictive capacity to comprehend species distribution related to trait variability in changing environments.
Conclusion
Although seeds of the Polygonaceae shared similar physical traits, generally displaying small-sized seeds and light-dependent germination patterns. We found great variation in germination requirements between and/or among populations. For instance, optimal temperature regimes may change according to seed population, mostly in altitudinal gradients, as we show for P. lapathifolium and R. japonica seeds. Seeds of Rumex spp., on the other hand, seemed to be less affected by light availability, with similar responses regardless of the collection sites. Variability in regeneration traits can be crucial to comprehending the species’ ability to colonize different areas, aiding in the identification of tolerant/sensitive populations affected by environmental changes.
Data availability statement
The original contributions presented in the study are included in the article/Supplementary material, further inquiries can be directed to the corresponding author.
Author contributions
AB conceived and designed the experiments. AB and XC performed the experiments. AB and SP analyzed the data. AB, SP, and LD wrote the manuscript. All authors approve of this submission.
Funding
AB thanks to the Talents Program of Jiangxi Province (PR China) (Grants jxsq2020104003) for funding.
Acknowledgments
AB thanks Dr. Deshui Yu and Yanli Niu for their valuable contributions during the seed collection. LD thanks the São Paulo Research Foundation (FAPESP) for the postdoctoral fellowship (grant #2022/01560-9).
Conflict of interest
The authors declare that the research was conducted in the absence of any commercial or financial relationships that could be construed as a potential conflict of interest.
Publisher’s note
All claims expressed in this article are solely those of the authors and do not necessarily represent those of their affiliated organizations, or those of the publisher, the editors and the reviewers. Any product that may be evaluated in this article, or claim that may be made by its manufacturer, is not guaranteed or endorsed by the publisher.
Supplementary material
The Supplementary material for this article can be found online at: https://www.frontiersin.org/articles/10.3389/fevo.2023.1093285/full#supplementary-material
References
Allison, V. J. (2002). Nutrients, arbuscular mycorrhizas and competition interact to influence seed production and germination success in Achillea millefolium. Funct. Ecol. 16, 742–749. doi: 10.1046/j.1365-2435.2002.00675.x
Alstad, A. O., Damschen, E. I., and Ladwig, L. M. (2018). Fire as a site preparation tool in grassland restoration: seed size effects on recruitment success. Ecol. Restor. 36, 219–225. doi: 10.3368/er.36.3.219
Amini, R., Gholami, F., and Ghanepour, S. (2017). Effects of environmental factors and burial depth on seed germination and emergence of two populations of Caucalis platycarpos. Weed Res. 57, 247–256. doi: 10.1111/wre.12259
Arana, M. V., Gonzalez-Polo, M., Martinez-Meier, A., Gallo, L. A., Benech-Arnold, R. L., Sánchez, R. A., et al. (2016). Seed dormancy responses to temperature relate to Nothofagus species distribution and determine temporal patterns of germination across altitudes in Patagonia. New Phytol. 209, 507–520. doi: 10.1111/nph.13606
Atis, I., Atak, M., Can, E., and Mavi, K. (2011). Seed coat color effects on seed quality and salt tolerance of red clover (Trifolium pratense). Int. J. Agric. Biol. 13, 363–368. doi: 10–539/AWB/2011/13–3–363–368
Aud, F. F., and Ferraz, I. D. (2012). Seed size influence on germination responses to light and temperature of seven pioneer tree species from the Central Amazon. An. Acad. Bras. Cienc. 84, 759–766. doi: 10.1590/S0001-37652012000300018
Baskin, C.C., and Baskin, J.M. (2014). Seeds: Ecology, biogeography, and evolution of dormancy and germination (2nd Edn). San Diego, CA: Academic Press.
Baskin, J. M., Davis, B. H., Baskin, C. C., Gleason, S. M., and Cordell, S. (2004). Physical dormancy in seeds of Dodonaea viscosa (Sapindales, Sapindaceae) from Hawaii. Seed Sci. Res. 14, 81–90. doi: 10.1079/SSR2003157
Batlla, D., and Benech-Arnold, R. L. (2005). Changes in the light sensitivity of buried Polygonum aviculare seeds in relation to cold-induced dormancy loss: development of a predictive model. New Phytol. 165, 445–452. doi: 10.1111/j.1469-8137.2004.01262.x
Benvenuti, S. (2007). Natural weed seed burial: effect of soil texture, rain and seed characteristics. Seed Sci. Res. 17, 211–219. doi: 10.1017/S0960258507782752
Benvenuti, S., Macchia, M., and Miele, S. (2001). Light, temperature and burial depth effects on Rumex obtusifolius seed germination and emergence. Weed Res. 41, 177–186. doi: 10.1046/j.1365-3180.2001.00230.x
Bhatt, A., Bhat, N. R., al-Nasser, A., Carón, M. M., and Santo, A. (2019). Inter-population variabilities in seed mass and germination of Panicum turgidum and Pennisetum divisum on the desert of Kuwait. J. Arid. Land 12, 144–153. doi: 10.1007/s40333-019-0017-6
Bhatt, A., Carón, M. M., Gallacher, D. J., and Souza-Filho, P. R. D. M. (2021c). Storage duration, light, temperature, and salinity exposure influence germination of the glycophyte Rhanterium epapposum. Botany 99, 261–267. doi: 10.1139/cjb-2020-0153
Bhatt, A., Carón, M. M., Souza-Filho, P. R. D. M., and Gallacher, D. J. (2021b). Maternal source affects seed germination of a rare Arabian desert species (Astragalus sieberi). Botany 99, 293–301. doi: 10.1139/cjb-2020-0144
Bhatt, A., Gairola, S., and El-Keblawy, A. (2016). Seed colour affects light and temperature requirements during germination in two lotus species (Fabaceae) of the Arabian subtropical deserts. Rev. Biol. Trop. 64, 483–492. doi: 10.15517/rbt.v64i2.18575
Bhatt, A., Gallacher, D. J., and Souza-Filho, P. R. (2021a). Germination strategies of annual and short-lived perennial species in the Arabian Desert. J. Arid. Land 12, 1071–1082. doi: 10.1007/s40333-020-0023-8
Bhatt, A., Silva, W., Gallacher, D. J., and Pompelli, M. F. (2020). Germination of Cenchrus ciliaris, Pennisetum divisum, and Panicum turgidum is seasonally dependent. Botany 98, 449–458. doi: 10.1139/cjb-2019-0194
Bouwmeester, H. J., and Karssen, C. M. (1992). The dual role of temperature in the regulation of the seasonal changes in dormancy and germination of seeds of Polygonum persicaria L. Oecologia 90, 88–94. doi: 10.1007/BF00317813
Bradford, K. J. (2002). Applications of hydrothermal time to quantifying and modeling seed germination and dormancy. Weed Sci. 50, 248–260. doi: 10.1614/0043-1745(2002)050[0248:AOHTTQ]2.0.CO;2
Bu, H. Y., Wang, X. J., Zhou, X. H., Qi, W., Liu, K., Ge, W. J., et al. (2016). The ecological and evolutionary significance of seed shape and volume for the germination of 383 species on the eastern Qinghai-Tibet plateau Hai-Yan. Folia Geobot. 51, 333–341. doi: 10.1007/s12224-016-9271-y
Burton, M. G., Lauer, M. J., and Mcdonald, M. B. (2000). Calcium effects on soybean seed production, elemental concentration, and seed quality. Crop Sci. 40, 476–482. doi: 10.2135/cropsci2000.402476x
Carlucci, M. B., Debastiani, V. J., Pillar, V. D., and Duarte, L. D. (2015). Between-and within-species trait variability and the assembly of sapling communities in forest patches. J. Veg. Sci. 26, 21–31. doi: 10.1111/jvs.12223
Carta, A., Hanson, S., and Müller, J. V. (2016). Plant regeneration from seeds responds to phylogenetic relatedness and local adaptation in Mediterranean Romulea (Iridaceae) species. Ecol. Evol. 6, 4166–4178. doi: 10.1002/ece3.2150
Cervantes, E., and Martín Gómez, J. J. (2019). Seed shape description and quantification by comparison with geometric models. Horticulturae 5:60. doi: 10.3390/horticulturae5030060
Chen, K., Burgess, K. S., Yang, X. Y., Luo, Y. H., Gao, L. M., and Li, D. Z. (2018). Functional trade-offs and the phylogenetic dispersion of seed traits in a biodiversity hotspot of the mountains of Southwest China. Eco Evol. 8, 2218–2230. doi: 10.1002/ece3.3805
Cheptou, P. O., Carrue, O., Rouifed, S., and Cantarel, A. (2008). Rapid evolution of seed dispersal in an urban environment in the weed Crepis sancta. Proc. Natl. Acad. Sci. U. S. A. 105, 3796–3799. doi: 10.1073/pnas.0708446105
Cochrane, A. (2016). Can sensitivity to temperature during germination help predict global warming vulnerability? Seed Sci. Res. 26, 14–29. doi: 10.1017/S0960258515000355
Cochrane, A., Hoyle, G. L., Yates, C. J., Wood, J., and Nicotra, A. B. (2014). Predicting the impact of increasing temperatures on seed germination among populations of Western Australian Banksia (Proteaceae). Seed Sci. Res. 24, 195–205. doi: 10.1017/S096025851400018X
Cochrane, A., Yates, C. J., Hoyle, G. L., and Nicotra, A. B. (2015). Will among-population variation in seed traits improve the chance of species persistence under climate change? Glob. Ecol. Biogeogr. 24, 12–24. doi: 10.1111/geb.12234
Elnaggar, A., El-Keblawy, A., Mosa, K. A., and Navarro, T. (2019). Adaptive drought tolerance during germination of Salsola drummondii seeds from saline and non-saline habitats of the arid Arabian deserts. Botany 97, 123–133. doi: 10.1139/cjb-2018-0174
Eriksson, O. (1999). Seed size variation and its effect on germination and seedling performance in the clonal herb Convallaria majalis. Acta Oecol. 20, 61–66. doi: 10.1016/S1146-609X(99)80016-2
Fang, X. W., Zhang, J. J., and Xu, D. H. (2017). Seed germination of Caragana species from different regions is strongly driven by environmental cues and not phylogenetic signals. Sci. Rep. 7, 1–11. doi: 10.1038/s41598-017-11294-x
Fernández-Pascual, E., Jiménez-Alfaro, B., Caujapé-Castells, J., Jaén-Molina, R., and Díaz, T. E. (2013). A local dormancy cline is related to the seed maturation environment, population genetic composition and climate. Ann. Bot. 112, 937–945. doi: 10.1093/aob/mct154
Flores, J., Jurado, E., Chapa-Vargas, L., Ceroni-Stuva, A., Dávila-Aranda, P., Galíndez, G., et al. (2011). Seeds photoblastism and its relationship with some plant traits in 136 cacti taxa. Environ. Exp. Bot. 71:79–88. doi: 10.1016/j.envexpbot.2010.10.025
Funes, G., Basconcelo, S., Dı´az, S., and Cabido, M.. (1999). Seed size and shape are good predictors of seed persistence in soil in temperate mountain grasslands of Argentina. Seed Sci Res 9:341–345. doi: 10.1017/S0960258599000355
Ge, W., Bu, H., Wang, X., Martinez, S. A., and Du, G. (2020). Inter-and intra-specific difference in the effect of elevation and seed mass on germinability of eight Allium species. Global Ecology and Conservation 22, e01016. doi: 10.1016/j.gecco.2020.e01016
Hand, D. J., Craig, G., Takaki, M., and Kendrick, R. E. (1982). Interaction of light and temperature on seed germination of Rumex obtusifolius L. Planta 156, 457–460. doi: 10.1007/BF00393317
Hong, H., Gu, Y., Yin, K., Wang, C., and Li, Z. (2013). Clay record of climate change since the mid-P leistocene in J iujiang, South China. Boreas 42, 173–183. doi: 10.1111/j.1502-3885.2012.00276.x
Hudson, A. R., Ayre, D. J., and Ooi, M. K. (2015). Physical dormancy in a changing climate. Seed Sci. Res. 25, 66–81. doi: 10.1017/S0960258514000403
Jaganathan, G. K., Li, J., Biddick, M., Han, K., Song, D., Yang, Y., et al. (2019). Mechanisms underpinning the onset of seed coat impermeability and dormancy-break in Astragalus adsurgens. Sci. Rep. 9, 1–10. doi: 10.1038/s41598-019-46158-z
Kagaya, M., Tani, T., and Kachi, N. (2011). Maternal and paternal effects on the germination time of non-dormant seeds of a monocarpic perennial species, Aster kantoensis (Compositae). Plant Species Biol. 26, 66–72. doi: 10.1111/j.1442-1984.2010.00303.x
Kang, H., Seely, B., Wang, G., Cai, Y., Innes, J., Zheng, D., et al. (2017). Simulating the impact of climate change on the growth of Chinese fir plantations in Fujian province, China. N Z J For Sci. 47, 1–14. doi: 10.1186/s40490-017-0102-6
Kleunen, M. V., Fischer, M., and Schmid, B. (2001). Effects of intraspecific competition on size variation and reproductive allocation in a clonal plant. Oikos 94, 515–524. doi: 10.1034/j.1600-0706.2001.940313.x
Kołodziejek, J. (2017). Effect of seed position and soil nutrients on seed mass, germination and seedling growth in Peucedanum oreoselinum (Apiaceae). Sci. Rep. 7, 1–11. doi: 10.1038/s41598-017-02035-1
Kuppler, J., Albert, C. H., Ames, G. M., Armbruster, W. S., Boenisch, G., Boucher, F. C., et al. (2020). Global gradients in intraspecific variation in vegetative and floral traits are partially associated with climate and species richness. Glob. Ecol. Biogeogr. 29, 992–1007. doi: 10.1111/geb.13077
Kyle, G., and Leishman, M. R. (2009). Functional trait differences between extant exotic, native and extinct native plants in the Hunter River, NSW: a potential tool in riparian rehabilitation. River Res App. 25, 892–903. doi: 10.1002/rra.1192
Larios, E., Búrquez, A., Becerra, J. X., and Venable, D. (2014). Natural selection on seed size through the life cycle of a desert annual plant. Ecology 95, 3213–3220. doi: 10.1890/13-1965.1
Larson, J. E., and Funk, J. L. (2016). Regeneration: an overlooked aspect of trait-based plant community assembly models. J. Ecol. 104, 1284–1298. doi: 10.1111/1365-2745.12613
Lopez, M. G., Wulff, A. F., Poggio, L., and Xifreda, C. C. (2008). South African fireweed Senecio madagascariensis (Asteraceae) in Argentina: relevance of chromosome studies to its systematics. Bot. J. Linn. Soc. 158, 613–620. doi: 10.1111/j.1095-8339.2008.00865.x
Losos, J. B. (2008). Phylogenetic niche conservatism, phylogenetic signal and the relationship between phylogenetic relatedness and ecological similarity among species. Ecol. Lett. 11, 995–1003. doi: 10.1111/j.1461-0248.2008.01229.x
Luzuriaga, A. L., Escudero, A., and Perez-Garcia, F. (2006). Environmental maternal effects on seed morphology and germination in Sinapis arvensis (Cruciferae). Weed Res. 46, 163–174. doi: 10.1111/j.1365-3180.2006.00496.x
Manzano-Piedras, E., Marcer, A., Alonso-Blanco, C., and Pico, F. X. (2014). Deciphering the adjustment between environment and life history in annuals: lessons from a geographically-explicit approach in Arabidopsis thaliana. PLoS One 9:e87836. doi: 10.1371/journal.pone.0087836
Mariko, S., Koizumi, H., Suzuki, J. I., and Furukawa, A. (1993). Altitudinal variations in germination and growth responses of Reynoutria japonica populations on Mt Fuji to a controlled thermal environment. Ecol. Res. 8, 27–34. doi: 10.1007/BF02348604
Meyer, S. E., and Allen, P. S. (1999). Ecological genetics of seed germination regulation in Bromus tectorum L. II. Reaction norms in response to a water stress gradient imposed during seed maturation. Oecologia 120, 35–43. doi: 10.1007/s004420050830
Milberg, P., Andersson, L., and Thompson, K. (2000). Large-seeded spices are less dependent on light for germination than small-seeded ones. Seed Sci. Res. 10, 99–104. doi: 10.1017/S0960258500000118
Moles, A. T., Ackerly, D. D., Webb, C. O., Tweddle, J. C., and Dickie, J. B. (2005). A brief history of seed size. Science 307, 576–580. doi: 10.1126/science.1104863
Mölken, T., Jorritsma-Wienk, L. D., Hoek, P. H., and Kroon, W. H. (2005). Only seed size matters for germination in different populations of the dimorphic Tragopogonp ratensiss subsp. pratensis (Asteraceae). Am. J. Bot. 92, 432–437. doi: 10.3732/ajb.92.3.432
Moreira, B., Tavsanoglu, Ç., and Pausas, J. G. (2012). Local versus regional intraspecific variability in regeneration traits. Oecologia 168, 671–677. doi: 10.1007/s00442-011-2127-5
Moya, R. S., Meza, S. E., Díaz, C. M., Ariza, A. C., Calderón, S. D., and Peña-Rojas, K. (2017). Variability in seed germination and seedling growth at the intra-and inter-provenance levels of Nothofagus glauca (Lophozonia glauca), an endemic species of Central Chile. N. Z. J. For. Sci. 47, 1–9. doi: 10.1186/s40490-017-0091-5
Münzbergová, Z., and Plačková, I. (2010). Seed mass and population characteristics interact to determine performance of Scorzonera hispanica under common garden conditions. Flora 205, 552–559. doi: 10.1016/j.flora.2010.04.001
Nicotra, A. B., Atkin, O. K., Bonser, S. P., Davidson, A. M., Finnegan, E. J., Mathesius, U., et al. (2010). Plant phenotypic plasticity in a changing climate. Trends Plant Sci. 15, 684–692. doi: 10.1016/j.tplants.2010.09.008
Norden, N., Daws, M. I., Antoine, C., Gonzalez, M. A., Garwood, N. C., and Chave, J. (2009). The relationship between seed mass and mean time to germination for 1037 tree species across five tropical forests. Funct. Ecol. 23, 203–210. doi: 10.1111/j.1365-2435.2008.01477.x
Oduor, A. M., Leimu, R., and Kleunen, M. V. (2016). Invasive plant species are locally adapted just as frequently and at least as strongly as native plant species. J. Ecol. 104, 957–968. doi: 10.1111/1365-2745.12578
Pendleton, R. L., and Pendleton, B. K. (2014). Germination patterns of a suite of semiarid grassland forbs from Central New Mexico. Native Plants J. 15, 17–28. doi: 10.3368/npj.15.1.17
Pons, T. L. (1992). “Seed responses to light” in Seeds the ecology of regeneration in plant communities. ed. M. Fenner (Wallingford, United Kingdom: CAB International), 259–284.
Saatkamp, A., Cochrane, A., Commander, L., Guja, L. K., Jimenez-Alfaro, B., Larson, J., et al. (2019). A research agenda for seed-trait functional ecology. New Phytol. 221, 1764–1775. doi: 10.1111/nph.15502
Saatkamp, A., Poschlod, P., and Venable, D. L. (2014). “The functional role of soil seed banks in natural communities” in Seeds – The ecology of regeneration in plant communities. ed. R. S. Gallagher (Wallingford, UK: CABI), 263–294.
Santo, A., Mattana, E., Grillo, O., and Bacchetta, G. (2015). Morpho-colorimetric analysis and seed germination of Brassica insularis Moris (Brassicaceae) populations. Plant Biol. 17, 335–343. doi: 10.1111/plb.12236
Staniforth, R. J., and Cavers, P. B. (1979). Field and laboratory germination responses of achenes of Polygonum lapathifolium, P. pensylvanicum, and P. persicaria. Can. J. Bot. 57, 877–885. doi: 10.1139/b79-108
Susko, D. J., and Lovett-Doust, L. (2000). Patterns of seed mass variation and their effects on seedling traits in Alliaria petiolata (Brassicaceae). Am. J. Bot. 87, 56–66. doi: 10.2307/2656685
Swain, A. J., Hughes, Z. S., Cook, S. K., and Moss, S. R. (2006). Quantifying the dormancy of Alopecurus myosuroides seeds produced by plants exposed to different soil moisture and temperature regimes. Weed Res. 46, 470–479. doi: 10.1111/j.1365-3180.2006.00532.x
Tautenhahn, S., Heilmeier, H., Go, L., Klotz, S., and Wirth, C. (2008). On the biogeography of seed mass in Germany e distribution patterns and environmental correlates. Europe 31, 457–468. doi: 10.1111/j.0906-7590.2008.05439.x
Timson, J. (1965). Germination in Polygonum. New Phytol. 64, 179–186. doi: 10.1111/j.1469-8137.1965.tb05388.x
Totterdell, S., and Roberts, E. H. (1979). Effects of low temperatures on the loss of innate dormancy and the development of induced dormancy in seeds of Rumex obtusifolius L. and Rumex crispus L. Plant Cell Environ. 2, 131–137. doi: 10.1111/j.1365-3040.1979.tb00783.x
Van Assche, J. A., and Vanlerberghe, K. A. (1989). The role of temperature on the dormancy cycle of seeds of Rumex obtusifolius L. Funct. Ecol. 3, 107–115. doi: 10.2307/2389681
Vandelook, F., Van de Moer, D., and Van Assche, J. A. (2008). Environmental signals for seed germination reflect habitat adaptations in four temperate Caryophyllaceae. Funct. Ecol. 22, 470–478. doi: 10.1111/j.1365-2435.2008.01385.x
Venable, D. L., and Brown, J. S. (1988). The selective interactions of dispersal, dormancy, and seed size as adaptations for reducing risk in variable environments. Am. Nat. 131, 360–384. doi: 10.1086/284795
Violle, C., Enquist, B. J., McGill, B. J., Jiang, L. I. N., Albert, C. H., Hulshof, C., et al. (2012). The return of the variance: intraspecific variability in community ecology. Trends Ecol. Evol. 27, 244–252. doi: 10.1016/j.tree.2011.11.014
Wagmann, K., Hautekèete, N. C., Piquot, Y., Meunier, C., Schmitt, S. E., and Van Dijk, H. (2012). Seed dormancy distribution: explanatory ecological factors. Ann. Bot. 110, 1205–1219. doi: 10.1093/aob/mcs194
Wu, G., and Du, G. (2007). Germination is related to seed mass in grasses (Poaceae) of the eastern Qinghai-Tibetan plateau, China. Nord J. Bot. 25, 361–365. doi: 10.1111/j.0107-055X.2007.00179.x
Wu, G. L., Li, W., and Du, G. Z. (2011). Relationship between germination and seed size in alpine shrubs in Tibetan plateau. Pak. J. Bot. 43, 2793–2796.
Xie, G., Li, L., Li, X., Zheng, Y., Wang, W., and He, J. (2013). Landscape materials diversity analysis of environmental engineering of world famous scenic resort Lushan Mountain in eastern China. IERI Proc. 5, 189–194. doi: 10.1016/j.ieri.2013.11.091
Xu, Y., Cai, N., He, B., Zhang, R., Zhao, W., Mao, J., et al. (2016). Germination and early seedling growth of Pinus densata mast. Provenances. J. For. Res. 27, 283–294. doi: 10.1007/s11676-015-0186-x
Xu, J., Li, W. L., Zhang, C. H., Liu, W., and Du, G. Z. (2014). Variation in seed germination of 134 common species on the eastern Tibetan plateau: phylogenetic, life history and environmental correlates. PLoS One 9:e98601. doi: 10.1371/journal.pone.0098601
Keywords: intraspecific variation, Polygonum, population ecology, regeneration, Rumex, seed traits
Citation: Bhatt A, Phartyal SS, Daibes LF and Chen X (2023) Interpopulation variation in seed traits of five Polygonaceae. Front. Ecol. Evol. 11:1093285. doi: 10.3389/fevo.2023.1093285
Edited by:
Mohammed Latif Khan, Dr. Hari Singh Gour University, IndiaReviewed by:
Spyros Tsiftsis, International Hellenic University, GreeceRaoudha Abdellaoui, Institut des Régions Arides, Tunisia
Copyright © 2023 Bhatt, Phartyal, Daibes and Chen. This is an open-access article distributed under the terms of the Creative Commons Attribution License (CC BY). The use, distribution or reproduction in other forums is permitted, provided the original author(s) and the copyright owner(s) are credited and that the original publication in this journal is cited, in accordance with accepted academic practice. No use, distribution or reproduction is permitted which does not comply with these terms.
*Correspondence: Arvind Bhatt, ZHJiaGF0dDc5QGdtYWlsLmNvbQ==