- 1Department of Biology, Texas State University, San Marcos, TX, United States
- 2Department of Chemistry, Villanova University, Villanova, PA, United States
Anthropogenic environmental change, including climate change and urbanization, results in warmer temperatures in both terrestrial and aquatic habitats and changes in community assemblages including invasive species introductions, among many other alterations. Anurans are particularly susceptible to these changes because generally they have a biphasic lifecycle and rely on aquatic and terrestrial habitats for survival. Changes such as warmer water temperature can result in direct and carryover effects, after metamorphosis that decrease fitness. However, Gulf Coast toads (Incilius (Bufo) nebulifer) are expanding their range, including into anthropogenically disturbed areas. We hypothesize that I. nebulifer copes with warmer water, reduced water levels, and invasive species by altering their physiology and/or behavior. Corticosterone is the primary glucocorticoid in amphibians, and it modulates many aspects of physiology and behavior, potentially including lipid storage and hop performance, during unpredictable (stressful) events. As a true toad, I. nebulifer also produces bufadienolide toxins that aid in its antipredator defense and may have tradeoffs with corticosterone. In a fully factorial design, we measured baseline corticosterone levels in tadpoles in response to two treatments: decreased water levels and increased water temperatures. After metamorphosis, we measured the corticosterone profile and other associated responses to exposure to the predatory red imported fire ant (Solenopsis invicta; RIFA). We found that tadpoles had elevated baseline corticosterone release rates when reared in warmer water and reduced water levels. Toadlets also had elevated baseline corticosterone release rates when exposed to any combination of two of the three treatments but when exposed to all three treatments toadlets instead showed elevated magnitude of their stress response. Predator avoidance (as measured by hop performance) was reduced after exposure to RIFA. Tadpoles from warmer water developed more quickly and were smaller in mass after metamorphosis. Toadlets had reduced production of two of the three detected bufadienolides and increased energy storage (lipids) after exposure to warmer water and reduced growth after exposure to reduced water levels. We found direct and carryover effects of common anthropogenic changes in I. nebulifer that may aid in their ability to persist despite these changes.
Introduction
Worldwide an estimated 60% of all land changes from 1982 to 2016 were directly associated with human activities (Song et al., 2018; Homer et al., 2020). Climate change and urbanization are leading causes of anthropogenic habitat alterations. Among other effects, climate change increases air and water temperature and alters frequency of precipitation, and urbanization results in urban heat islands with reduced surface permeability which in turn elevates water temperatures and changes surface water dynamics (Nelson and Palmer, 2007; Kaushal et al., 2010; Brans et al., 2018). While the effects of anthropogenic change on biodiversity are varied, in general, they result in a decrease in native species richness and abundance, an increase in invasive species richness and abundance, and shifts in species evenness (Shochat et al., 2010; Alberti et al., 2020; Von Der Lippe et al., 2020). Together these changes present a new set of perturbations that species must adapt to or face extirpation (Cordier et al., 2021).
Amphibians are particularly susceptible to the effects of anthropogenic environmental change. Amphibians are reliant on more environmental factors than most vertebrates because they are ectotherms that often have a biphasic lifecycle, having terrestrial and aquatic stages. Beyond the direct effects, aquatic developmental environments of amphibians can also have carryover effects after metamorphosis (Relyea, 2001; Crespi et al., 2013; Charbonnier et al., 2018; Yagi and Green, 2018; Sinsch et al., 2020). These carryover effects can change the way amphibians respond to terrestrial stressors (Sinsch et al., 2020). For example, in amphibians that breed in ephemeral pools, early pool drying leads to early metamorphosis and reduced body fat (Morey, 1998). Reduced body fat at metamorphosis is correlated with reduced survival as an adult (Scott et al., 2007). While the effects of anthropogenic environmental change on amphibians are relatively understudied compared to the diversity of the clade (Bonnet et al., 2002; Cordier et al., 2021), one metanalysis found that in the United States most native amphibians showed a negative response to changes like urbanization at the population level (Scheffers and Paszkowski, 2012). Despite most amphibian populations declining (Allen et al., 2017), populations of the Gulf Coast toad (Incilius (Bufo) nebulifer) are increasing. I. nebulifer is native to central and eastern Texas and northeast Mexico but are documented in many new areas, with noteworthy range expansions to the north and east in the United States (Mendelson et al., 2015). These range expansions are strongly associated with disturbed habitats such as urban areas but could also be due to warmer temperatures. Studies in Louisiana on the effects of I. nebulifer range expansion on amphibian communities show the presence of I. nebulifer tadpoles decrease the fitness of native amphibian tadpoles (Vogel and Pechmann, 2010). This makes I. nebulifer not only an interesting species to study as it relates to the consequences of anthropogenic environmental change, but also as a conservation concern.
Glucocorticoid levels are frequently employed to study a vertebrate’s ability to physiologically cope with anthropogenic environmental change (Hopkins et al., 1997; Angelier and Wingfield, 2013; Burraco and Gomez-Mestre, 2016; Gabor et al., 2019; Bókony et al., 2021). In amphibians, the primary glucocorticoid is corticosterone. In general, corticosterone is a mediator for many physiological processes including development and energy allocation while helping amphibians maintain homeostasis (Sapolsky et al., 2000). Corticosterone also plays a role in the stress response. Exposure to stressors activates the hypothalamic–pituitary–interrenal (HPI) axis, which concludes in the release of corticosterone. When an amphibian encounters an unpredictable perturbation (stressor) such as a predator or an irregular weather event (e.g., storms) most often corticosterone levels increase, inducing physiological and behavioral responses that help cope with that stressor (Carr and Norris, 1990; Wingfield, 2013; Hau et al., 2016). Corticosterone responses can vary in absolute concentrations and in stress response or negative feedback rates (Eikenaar et al., 2012; Cockrem, 2013; Hau et al., 2016; Bókony et al., 2021). The corticosterone profile illustrates how corticosterone varies in response to stressors. Comprehensive studies of the complete corticosterone profile can aid in understanding how species cope with anthropogenic environmental change (Wingfield, 2013; Sinsch et al., 2020; Bókony et al., 2021; Kolonin et al., 2022).
Corticosterone has both direct and indirect effects on organisms. For example, tadpoles exposed to a predator cue have elevated corticosterone levels and move less when compared to a control group (Middlemis et al., 2013). After metamorphosis, hop performance provides a measure of predator avoidance. Similar to activity, hop performance decreases with chronic exposure to predators (Relyea and Hoverman, 2003; Zamora-Camacho and Aragón, 2019), but the potential interaction between hop performance and corticosterone levels is still inconclusive (Relyea and Edwards, 2010; Ricciardella et al., 2010; Woodley and Lacy, 2010; Bliley and Woodley, 2012; Wack et al., 2013; Fonner and Woodley, 2015; Cha et al., 2021). Changes in activity and corticosterone levels may be an outcome of and/or alter changes in lipid levels. Lipids are a more accurate measure of body condition and when combined with growth rates can indicate changes in energy allocation (Fitzpatrick, 1976; Crump, 1981; Scott et al., 2007; MacCracken and Stebbings, 2012). In sum, corticosterone has the potential to mediate changes in behavior and energy allocation and needs to be further explored in amphibians.
As a true toad (part of the family Bufonidae), I. nebulifer produces bufadienolides (Porto and Gros, 1971; Porto et al., 1972; Coloma et al., 1984; Garraffo and Gros, 1986). Bufadienolides are endogenously produced toxic compounds that cause cardiac arrhythmia or in some cases cardiac arrest when ingested (Clarke, 1997). They are produced in granular glands in the skin and are often concentrated in two conspicuous parotoid glands on the dorsal side of the head. Bufadienolides and corticosterone both can represent responses to environmental cues such as herbicide exposure, habitat quality, urbanization, and conspecific chemical cues by either increasing or decreasing in concentration (Bufadienolides: Hagman et al., 2009; Bókony et al., 2017, 2019; Corticosterone: Hopkins et al., 1997; Goff et al., 2020; Bókony et al., 2021). Thus, it is useful to determine whether one or both phenotypes change in response to chronic environmental change.
To evaluate the effects of the larval developmental environment, we exposed I. nebulifer tadpoles to low water levels and elevated water temperature in a fully factorial design. To determine if there are any carryover effects after metamorphosis, toadlets from each larval treatment were exposed to an invasive predator, the red imported fire ant (Solenopsis invicta; RIFA). RIFA is an invasive species in many parts of the world, and like most other invasive species, it is particularly effective at colonizing anthropogenically disturbed habitats. RIFA is implicated as a cause of many species’ declines including amphibians (Allen et al., 2004, 2017). In the United States, it is more aggressive and more toxic than the native ants including the native tropical fire ant (Solenopsis geminata). Native ants are an important food source for many recently metamorphosed amphibians (Smith et al., 2004), but RIFA has turned the tables and will prey on amphibians as they leave ephemeral pools (Todd et al., 2008).
We used an integrative approach to explore the effects of the larval developmental environment and post-metamorphic predator exposure on the corticosterone profile, hop performance, total lipid concentration, and measures of bufadienolide production in I. nebulifer. Previous studies have found that low water levels and elevated water temperature results in accelerated development and smaller sizes at metamorphosis in other species of tadpoles (Maciel and Juncá, 2009) and elevated baseline corticosterone levels (Narayan et al., 2012; Narayan and Hero, 2014; Gabor et al., 2018). Smaller toadlets with an altered corticosterone profile could have altered behaviors, energy allocations, and predator defenses when exposed to a predator.
We hypothesized that I. nebulifer will alter their corticosterone profile in response to factors associated with anthropogenic environmental change and, either associated with or in addition to their altered corticosterone profile, will have altered behaviors and/or physiological pathways. If I. nebulifer tadpoles perceive warmer water and decreased water level as a stressful habitat, we predicted that tadpoles from treatments with warmer water and decreased water levels will have elevated baseline corticosterone release rates. If toadlets have carryover effects from the potentially more stressful larval environments, we predict they will also have elevated baseline corticosterone release rates and an altered corticosterone profile, decreased hop performance, reduced lipid levels, and a reduction in bufadienolide production when compared to the control treatment. If toadlets exposed to potentially more stressful larval environments have altered physiology and behavior but still have a functioning HPI axis, when exposed to RIFA, their physiology and behavior will change compared to the no-predator exposure treatment.
Materials and methods
Egg collection and tadpole husbandry
We collected about 200 eggs from four I. nebulifer egg strands (800 eggs total) in San Marcos, TX (long: −97.94285, lat: 29.90115) on 26 March 2020 (one strand) and 7 April 2020 (three strands). All eggs were at the same stage of development when collected and the site was a natural area and considered to have little anthropogenic alterations. After collection, we transported the eggs to our lab at Texas State University and we divided each egg strand among three 5.7 L polypropylene containers filled with 3 L aged tap water (12 containers total). Eggs hatched 1–2 days after collection and were free-swimming after 1 week. Once the tadpoles were free swimming, they were fed a food pellet consisting of a mixture of spirulina powder and Tetramin fish flakes in an agar base ad libitum. After the first feeding, we took 30 tadpoles from each container that were seen feeding (360 total) and mixed them in a single tank to be used for the experiment.
Heating and water level exposure: Tadpoles
We randomly divided tadpoles among 56 containers (six tadpoles per container) and each container was exposed to one of four treatments: (1) 3 L of 23°C water (i.e., low urbanization alterations), (2) 1.5 L of 23°C water (i.e., moderate urbanization alterations), (3) 3 L of 32°C water (i.e., moderate urbanization alterations), and (4) 1.5 L of 32°C water (i.e., high urbanization alterations). We used four strands to increase the genetic diversity of our sample but differences among egg strands were not the focus of this study so strand ID was not maintained. Temperatures were selected to be ecologically relevant and close to developmental thermal tolerances. The 23°C water temperature was based on the average water temperature measured at the site of collection during the time of collection (March 25, 26, 27, and April 7, 2020) which was 21.7°C. The 32°C water temperature was based on the average water temperature measurements taken toward the end of the rainy season when surface water is present but water levels are low (June 28, 29, July 5, and 6) which was 34.2°C. Developmental thermal tolerances for I. nebulifer are between a low of 18–22°C and a high of 34–38°C (Ballinger and McKinney, 1966). We chose to have two fixed water levels rather than a fixed and a reducing water level to focus on the environmental stimulus of water level rather than the stimulus of drying. We heated 32°C water treatments with 100 W submersible All-Aquarium heaters and supplied all containers with a foam float for toadlets to climb out on after metamorphosis. We maintained all containers on a 12:12 light/dark cycle and performed partial water changes bi-weekly. Containers were randomly shuffled among spaces weekly to prevent any shelf effects.
RIFA exposure: Metamorphs
Once metamorphosed, we kept toadlets individually in 500 mL deli cups with perforated lids, enough water to cover part of the bottom of the cup, and a shelter made from a cardboard egg crate. Five days post metamorphosis we fed toadlets seven wingless fruit flies (Drosophila melanogaster; determined by preliminary feeding experiments). Toadlets that ate were weighed and photographed for total length measurements and then randomly divided into two treatments: (1) RIFA exposure and (2) control groups. Two toadlets from each tank were used in the RIFA and control treatments. However, mortality reduced some tanks to one or zero toadlets (final n = 150). Toadlets were fed seven wingless fruit flies every other day for the duration of the experiment. We refreshed the water and recorded the number of flies eaten every other day. Cardboard hides were replaced bi-weekly or as needed. RIFA exposure consisted of removing the water and hide from the deli cup and introducing three fire ants for 10 min. After the 10 min exposure, we removed RIFA with a gloved hand and forceps, added clean water, replaced the hide, and fed the toadlets. RIFA were not prevented from attacking toadlets but the number of fire ants presented could not subdue the toadlets and no mortality as a direct result of the RIFA exposure was observed. The control group had the same treatment but without the introduction of RIFA (water and hide removed for 10 min and gloved hand and forceps placed in the deli cup as was done to remove RIFA). Wingless fruit flies and RIFA were sedated using CO2 for easy handling but were allowed time to recover before use. Toadlet treatments lasted 30 days from treatment assignment.
Water-borne corticosterone
After 30 days of treatment exposure, we measured water-borne corticosterone profiles: baseline, agitation, and recovery release rates following an established protocol (Gabor et al., 2013; Forsburg et al., 2019) with a minor modification for toadlets. Briefly, we placed toadlets in glass petri dishes with lids and 15 mL spring water (enough to cover the bottom 1/3 of each toadlet) for water-borne hormone collection. Separate petri dishes were used for baseline, agitation, and recovery and toadlets were transferred with a gloved hand. Toadlets were agitated by placing petri dishes on a supply cart and gently swirling and rocking the supply cart for 1 min every 3 min for 60 min. Water was collected from petri dishes into sterile falcon tubes and stored at −20°C until processing. Hormone collection was done in the same room the treatments were performed where the temperature was being maintained at 23°C.
Hormones were extracted from the water using C18 solid-phase extraction columns (SepPak Vac3 cc/500 mg; Waters, Inc., Milford, MA, United States) under vacuum pressure (following Forsburg et al., 2019). We primed the columns with 4 mL methanol and 4 mL distilled water. After extraction, we eluted columns with 4 mL methanol into borosilicate vials. The methanol was evaporated under a gentle stream of nitrogen gas while in a 37°C water bath. We re-suspended the residue in 500 μL of 5% ethanol diluted with enzyme-immunoassay (EIA) buffer (provided by Cayman Chemicals Inc., Ann Arbor, MI, United States). After resuspension, we shook samples for 2 h to fully resuspend the residue. The resuspension volume ensured that sample values were within the assay range of the EIA kits. Corticosterone release rates were measured in duplicate for all samples with an EIA kit (Cayman Chemicals Inc.) on a microplate absorbance reader (BioTek ELX800) set to 405 nm. Inter-plate coefficient of variation was 12.50% (11 plates; range: 1.01–12.03%).
Hop performance
To quantify hop performance, toadlets were placed on a flat surface covered with damp white paper towels to help maintain body moisture, provide a textured surface, and give a contrasting background for video recording. A video camera was secured to a tripod to provide an overhead view of hop performance trials. Toadlets were placed under a 118 mL cup for a 5 min acclimation period. After the acclimation period, the cup was lifted and recording of the trial started. If the toadlet did not hop, a gentle touch of the hind legs was used to encourage hopping. The first three sequential hops that were uninterrupted and in the forward direction (toadlet was not hopping sideways) were analyzed. To quantify hop performance, we measured the three hops using Kinovea1 (Puig-Diví et al., 2019). Hop distance was determined by marking the location of the urostyle at the start and end of the hop and then measuring the distance between the two locations. The longest of the three hops was used as our maximum hop performance and the average of the three hops was used for average hop performance (Moreno-Rueda et al., 2020). Both measures were interpreted as measures of predator avoidance response. Hop performance trials were performed in the same room the toadlets were housed where the temperature was maintained at 23°C. After the hop performance trials, toadlets were euthanized in an ice bath to prevent contamination of bufadienolides. After euthanasia, we weighed toadlets and photographed their dorsal side to measure snout-urostyle length (SUL) using imageJ (version 1.51w). Maximum and average hop performance were SUL corrected before analysis. Euthanized toadlets were placed in 2 mL microcentrifuge tubes with 1 mL HPLC-grade methanol and stored at room temperature for preservation and bufadienolide extraction until analysis.
Bufadienolide analysis: Liquid chromatography-mass spectrometry
After preservation in HPLC-grade methanol (utilized throughout the bufadienolide analysis), we removed toadlets and evaporated the methanol under a gentle stream of nitrogen gas while in a 37°C water bath for shipping. Samples were then reconstituted in 0.7 mL of methanol and vortexed for 45 s to solubilize the rinse. Solubilized rinses were subsequently filtered through 13 mm syringe filters (0.2 μm PTFE membrane) into clean, labeled centrifuge tubes. Another 0.7 mL of methanol was added to the syringe and filtered to remove any residual extract adhered to the membrane. We then evaporated the filtered extracts in vacuo (without heat) on a DNA SpeedVac. After drying, the extracts were resuspended in 50 μL of methanol and centrifuged. The supernatants were placed into vials for LC–MS/MS analysis.
We purchased eight bufadienolide standards (telocinobufagin, arenobufagin, cinobufotalin, cinobufagin, bufalin, marinobufagenin, resibufogenin, and gamabufotalin) from Cayman Chemicals Inc. and suspended them in methanol. Bufadienolide standards and reconstituted toadlet filtrates were then analyzed on an Exion HPLC equipped with a PDA detector and Sciex TripleTOF 5,600+ mass spectrometer in ESI positive mode with a calibrant delivery system. All samples were analyzed via reverse-phase HPLC utilizing an Agilent C18 column (4.6 × 150 mm; 3.5 μm particle size). The mobile phase consisted of two solvents: acidified water (0.1% formic acid, v/v; Solvent A) and acidified methanol (0.1% formic acid, v/v; Solvent B). We separated the extracts using the following gradient developed for the separation of the eight bufadienolides: 10% solvent B held for 2 min then stepped to 55% solvent B. The gradient was then increased from 55–65% solvent B in 5 min. Next, the gradient was increased from 65–74% solvent B over 9 min then stepped to 100% solvent B for 7 min. Finally, solvent B was stepped to initial conditions for 6 min to re-equilibrate the column. The separation was performed at a flow rate of 1.00 mL/min with the LC column oven set to 40°C. A 30 μL sample of each reconstituted toadlet rinse was injected on-column.
Within the TOFMS method developed for bufadienolide relative quantification, the curtain gas was set to 30 PSI with source gases 1 & 2 set to 60 PSI. Additionally, a source temperature of 550°C was utilized with a needle spray voltage of 5,500 V. The declustering potential and collision energy were set to 70 V and 10 V, respectively. The collision energy was kept at 10 V throughout the TOFMS scan (50–1,000 Da) to attempt to retain the molecular ion of bufadienolides for mass accuracy calculations. The mass spectrometer was calibrated every 5 samples, utilizing ESI positive calibration solution and the calibrant delivery system.
For the relative quantitation of the seven bufadienolides and absolute quantitation of bufalin, we prepared a serial dilution of the bufalin standard to generate a series of standard curves via LC–MS/MS. Due to structural similarities in the backbone of bufalin compared to the other bufadienolides being quantified (Figure 1), we assumed these compounds have relatively equivalent ionization allowing for their quantitation based on bufalin measurements. As a result of bufadienolide standards’ high cost and sporadic availability, we were unable to make standard curves for each bufadienolide. Bufalin standards ranging from 0.00819 μg/mL to 6.55 μg/mL were analyzed via LC–MS/MS (Supplementary Table S1) from low to high concentration with blanks between each standard to generate standard curves for bufalin. Three full bufalin standard curves were analyzed on three separate days. To account for concentration ranges in which instrument response was non-linear, a weighted linear regression was utilized. Two of the three calibration curves were split for linear equations to be generated for high and low concentrations (high spine/low spine linear equations; Supplementary Table S2). Sample concentrations calculated from the curve generated closest to when samples were analyzed to properly control for instrument variability (Supplementary Tables S3, S4). Four system suitability bufalin standards of 0.0164 μg/mL, 0.328 μg/mL, 1.64 μg/mL, and 6.55 μg/mL were analyzed prior, mid, and post sample batch acquisition to assess and account for instrument variability throughout the duration samples were analyzed.
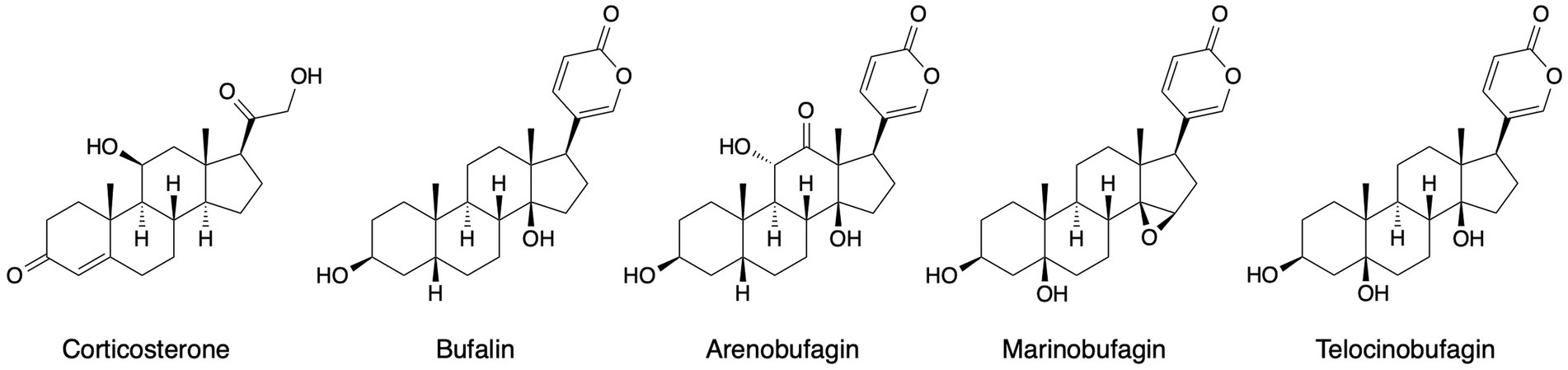
Figure 1. Chemical structures of the stress hormone corticosterone, as well as four bufadienolides: bufalin, arenobufagin, marinobufagin, and telocinobufagin.
We used PeakView™ software for the evaluation of data. The protonated exact mass of the eight bufadienolide standards was extracted from the total ion chromatogram (TIC) for peak area quantitation. The mass accuracy of the measured versus actual bufadienolide protonated exact masses were calculated using the formula:
Compounds with a mass accuracy less than 5 ppm were considered identical to bufadienolide standards. For secondary confirmation of the identification of the bufadienolide, retention time was utilized.
Lipid analysis
After removal of toadlet remains from the methanol for bufadienolide analysis, we dried the toadlets at 60°C until they were a constant weight (36–48 h). We then placed the toadlet remains in a lipid free filter paper (Whatman™ Grade 602H) envelope made by cutting the filter paper into a 4 × 6 cm rectangle. The short sides of the rectangle were pre-folded into the middle and the long sides of the rectangle were pre-folded into thirds to create guidelines for sample placement. Toadlet remains were then placed in the 2 × 2 cm center square made by the pre-folding guidelines. We repeated the folding, the short sides folded followed by the long sides, and stapled the end of the resulting 2 × 2 cm square envelope to seal it. Preliminary trials showed that staples did not change weight during the extraction process and encased remains were sufficiently retained. Because of the small size of the toadlet remains, we used the intact toadlets to prevent any loss of material that could occur if homogenized.
We labeled the filter paper envelopes using a graphite pencil and placed the filter paper envelopes into a Soxhlet extraction setup. For efficiency, we processed samples in batches with ~12 samples per setup. We used petroleum ether as our extraction solvent and heated it to 45°C. This resulted in a cycle time of about 15 min. We extracted the samples for 6 h, allowed the remaining petroleum ether to evaporate off the samples, and placed the samples in a 60°C dryer for 1 h.
We weighed the toadlet remains after initial drying (), after being placed in the filter paper envelope, and after the final drying (). We determined the total lipid content as the percent of total body weight lost during the extraction process and used the following formula:
Where is the weight of the filter paper determined by subtracting the initial dried weight from the weight of the sample in the filter paper envelope.
Growth and development
As additional measurements of energy allocation tradeoffs we measured tadpole development time, tadpole growth, and toadlet growth. Tadpole development time was measured as days from collection to complete tail reabsorption at metamorphosis. Tadpole growth was estimated from toadlet mass after metamorphosis but before first feeding. Tadpoles were too small at the start of the experiment to get an accurate starting weight (<10 mg) without excessive handling to dry tadpoles. Differences in size immediately following metamorphosis were assumed to represent differences in growth as tadpoles. Toadlet growth was measured as the difference in weight from complete tail reabsorption to weight at euthanasia. Tadpole and toadlet growth were log10 transformed.
Statistical analysis
We performed all statistics in RStudio (version 1.4.1106) using the R statistical software (version 4.0.5). All response variables were analyzed with linear mixed-effects models using the packages “lme4” and “lmerTest” with water temperature, water level and RIFA exposure as fixed effects and container as a random effect. Mass or SUL was used to control for size for corticosterone, bufadienolide, lipid, and hop performance measurements. Toad stress response was analyzed at absolute release rates (baseline, stress induced, and recovery release rates) and relative release rates (magnitude of stress response and negative feedback). Corticosterone absolute release rates were quantified as the amount of water-borne corticosterone measured over 1 h, divided by body mass (pg/g/h), and log base-10 transformed. The magnitude of stress response was quantified using the following formula (Lattin and Kelly, 2019; Bókony et al., 2021):
Similarly, negative feedback was quantified using the following formula:
A type-2 analysis-of-deviance table was used to pairwise compare the three absolute concentrations across the treatment groups using the “Anova” function in the “car” package. The significance level was corrected using the false discovery rate (FDR) method in the package “emmeans.” To parse out any three-way interactions we calculated the estimated marginal means using the “emmeans” package and plotted the results with 95% confidence intervals. Correlations between corticosterone measurements and total measured bufadieniolide amount, hop performance, and lipid levels were performed using Spearman’s rank correlation. Because of the inherent variability of these response variables, we considered a value of p threshold of 0.06 to be significant rather than the arbitrary convention of 0.05.
Results
Water-borne corticosterone
The main treatment effects significantly changed baseline tadpole corticosterone release rates. Warmer water increased corticosterone release rates and reduced water level also increased corticosterone release rates (temperature: estimate = 0.82, df = 51.9, t = 3.4, p = 0.001; water level: estimate = 0.45, df = 51.9, t = 1.9, p = 0.06). Pairwise comparisons of toadlet corticosterone release rates across all treatments showed a significant effect of sample type indicating that our hormone sampling methods were effective in detecting an active HPI axis (Table 1).
Baseline toadlet corticosterone release rate showed a significant three-way interaction with water temperature, water level, and exposure to RIFA (Table 2). Baseline corticosterone release rates are higher when exposed to RIFA and 32°C water or low water level and RIFA, but when exposed to 32°C water, low water level, and RIFA baseline corticosterone release rates are similar to other treatments although more variable (Figure 2).
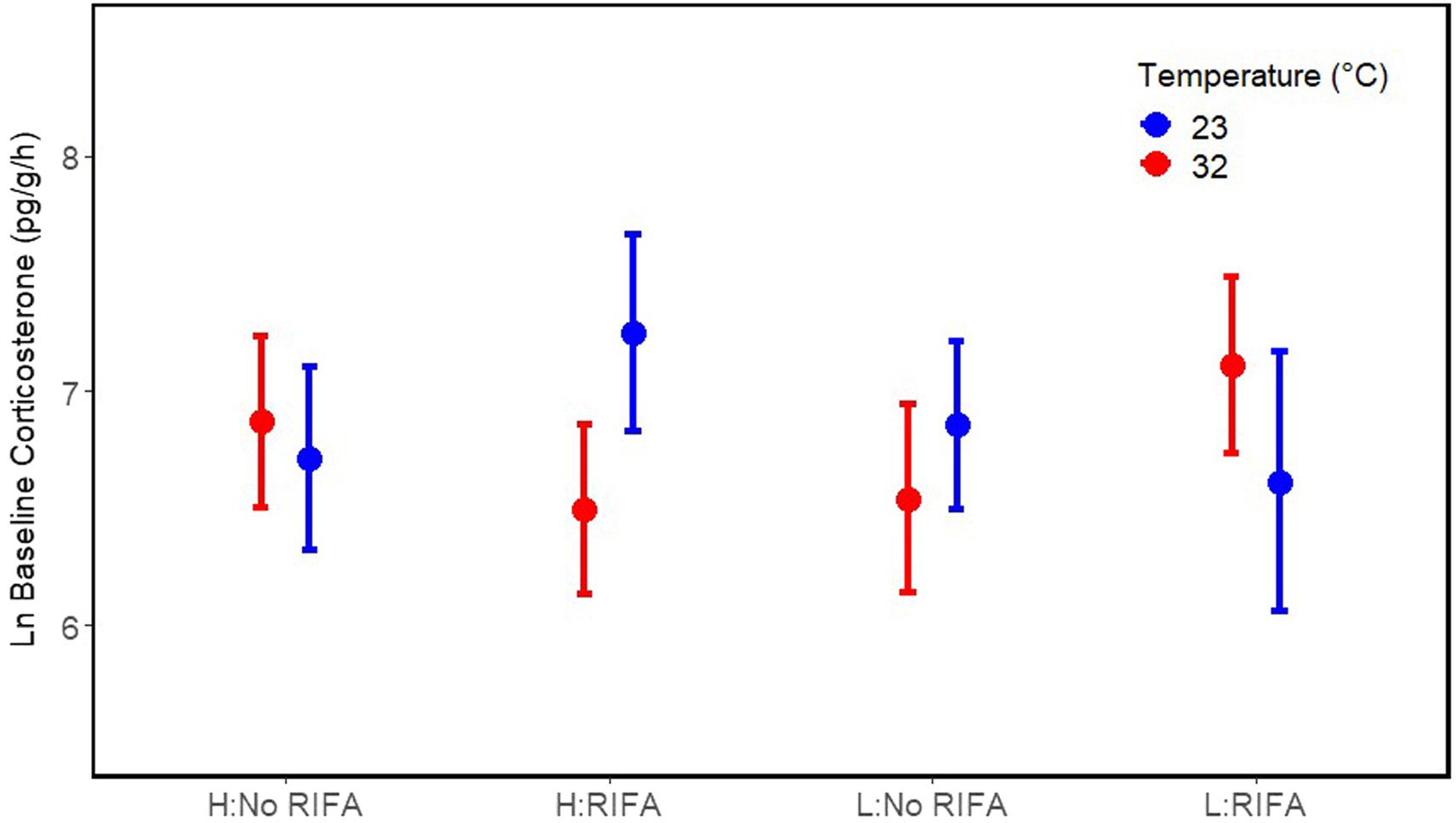
Figure 2. The estimated marginal means of Ln baseline corticosterone release rates of Incilius nebulifer toadlets by treatment. Error bars are 95% confidence intervals. “L” and “H” refer to low and high (adequate) water levels, respectively.
The magnitude of the stress response showed a significant three-way interaction as well (Table 2). Low water level, 32°C water, and exposure to RIFA increased the magnitude of stress response, while the same larval treatment that was not exposed to RIFA reduced the magnitude of stress response (Figure 3). The treatments showed no significant effect on the other measures of the corticosterone profile (negative feedback efficiency, stress induced release rates or recovery release rates).
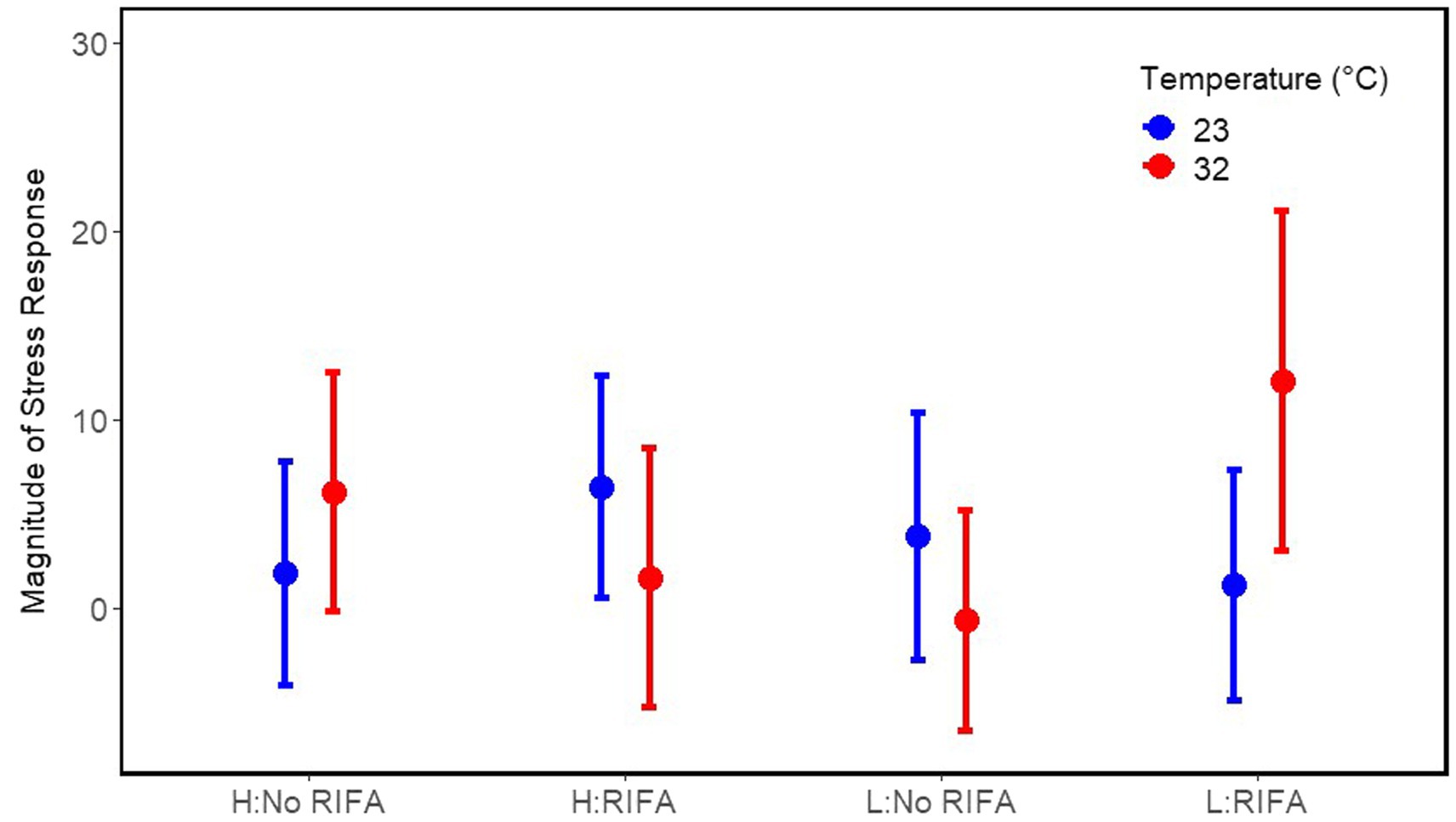
Figure 3. The estimated marginal means of the magnitude of the stress response of I. nebulifer toadlets by treatment. Error bars are 95% confidence intervals. “L” and “H” refer to low and high (adequate) water levels, respectively.
Hop performance
Maximum and average hop performance were SUL corrected to account for the different sizes of individuals. Our treatments had no effect on maximum hop performance. Still, average hop performance was reduced in toadlets exposed to RIFA (Table 3).
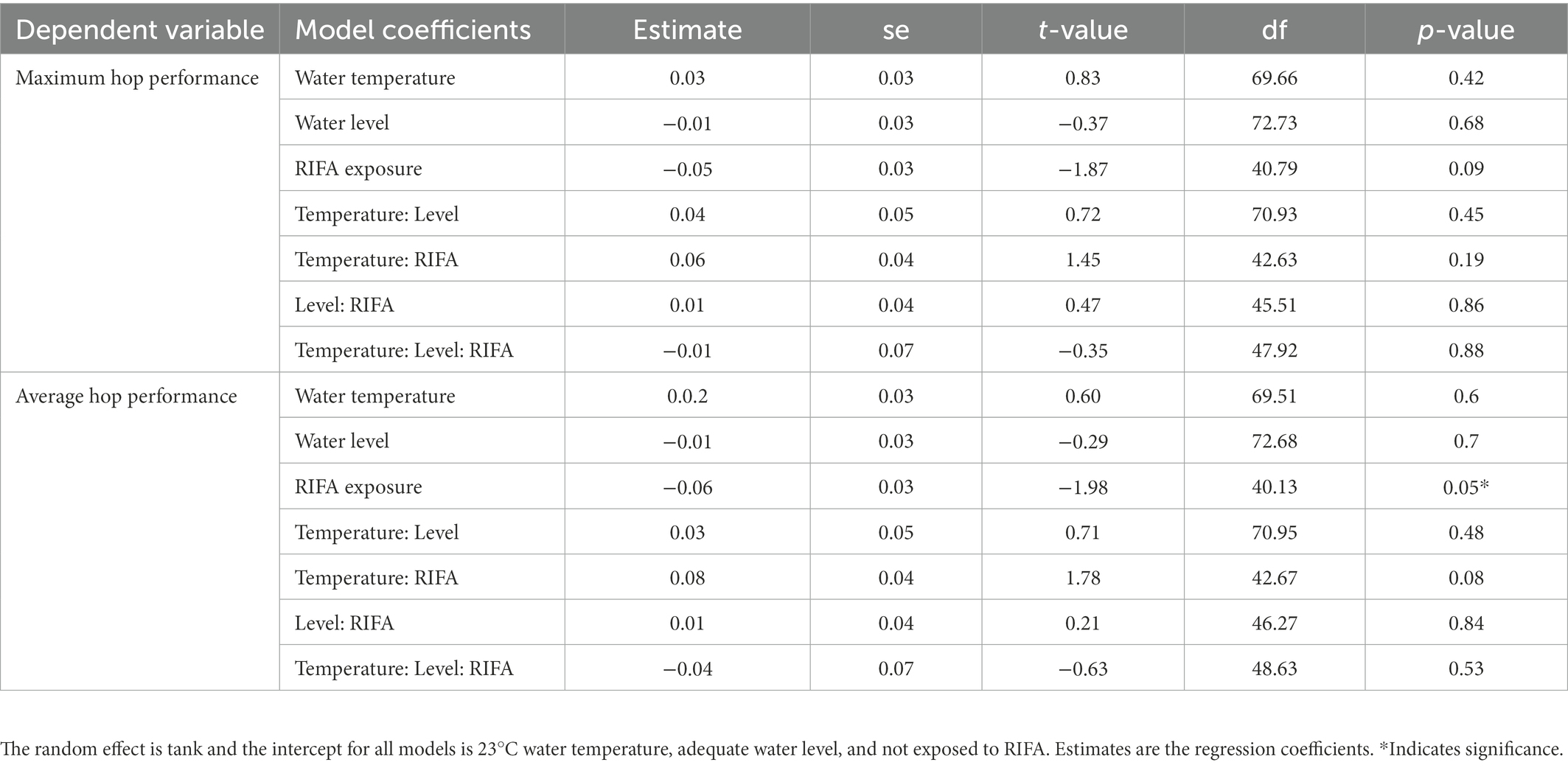
Table 3. Results of mixed-effects models for hop performance analysis for Incilius nebulifer toadlets.
Bufadienolide relative quantification via liquid chromatography–mass spectrometry
The limit of detection (LOD) and limit of quantitation (LOQ) were calculated for the three bufalin standard curves (Supplementary Tables S4, S5). Most samples contained bufadienolides above the limit of quantitation for their respective calibration curves, but all calculated bufadienolides concentrations were above the limit of detection (Supplementary Table S3). Of the eight bufadienolide compounds we analyzed for in the reconstituted toadlet filtrates via LC–MS/MS, only three bufadienolides (arenobufagin, marinobufagin, and telecinobufagin) were detected (Supplementary Table S3). Since no bufadienolides without a standard were included in our analysis, we were unable to conclude if alternative bufadienolides were present in our samples.
We found a significant interaction between the temperature treatment and the RIFA exposure treatment on arenobufagin concentration. Toadlets reared in 32°C water had reduced concentrations of arenobufagin when not exposed to RIFA but the concentration found in toadlets across temperature treatments did not significantly differ when exposed to RIFA (Figure 4). We found a marginal effect of the temperature treatment on marinobufagin concentration as well with the 32°C water treatment again reducing the concentration of marinobufagin. We did not find any significant effect of our treatments on telecinobufagin concentration, the total amount of bufadienolides, or bufadienolide diversity (Table 4).
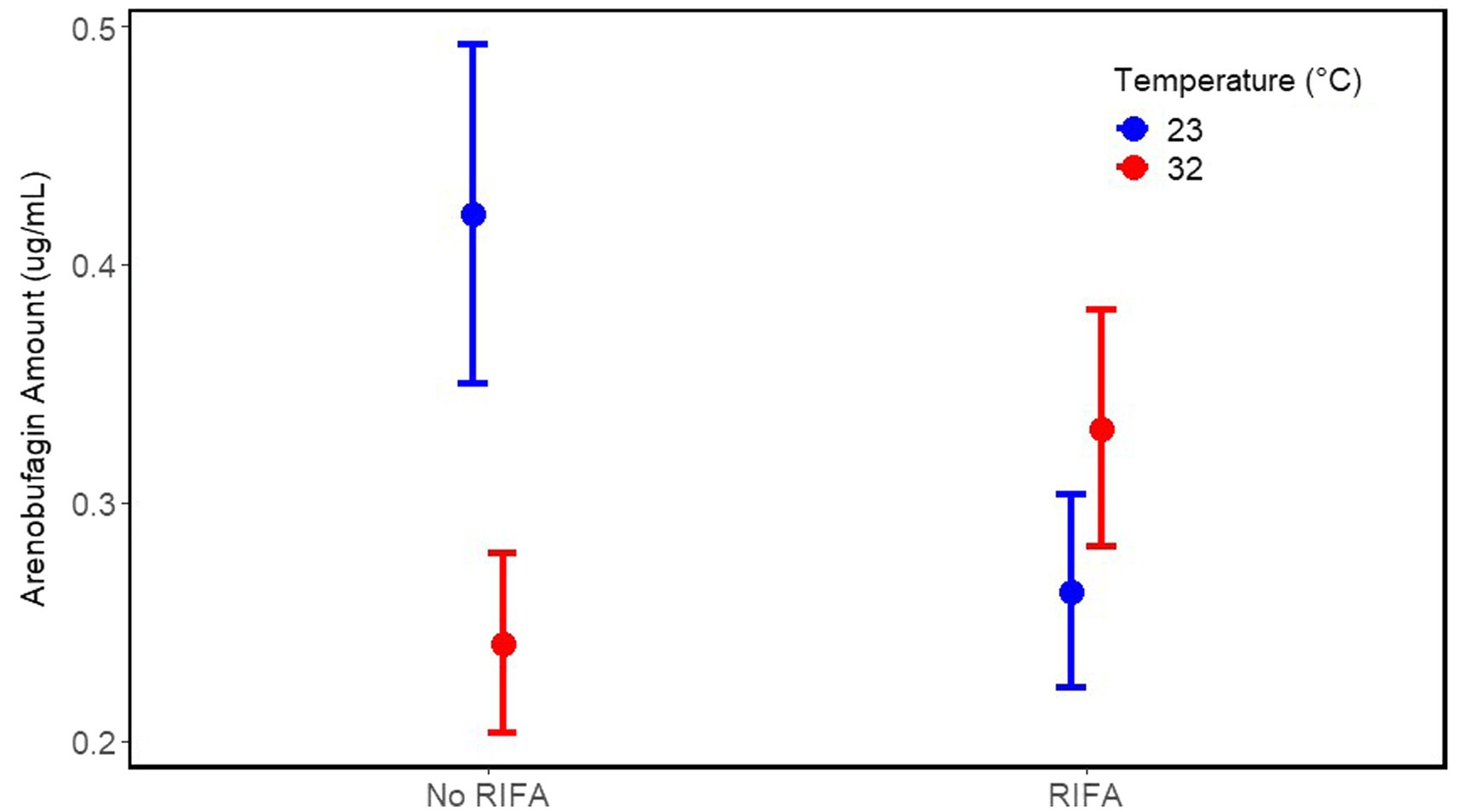
Figure 4. Estimated marginal means of the concentration of Arenobufagin in I. nebulifer toadlets in response to RIFA exposure and water temperature. Error bars are 95% confidence intervals.
Lipids
We found a significant interaction between water level and RIFA exposure. Low water levels were associated with reduced lipid levels regardless of RIFA exposure but those toadlets that were reared in low water levels and not exposed to RIFA had slightly increased lipid levels and those toadlets that were reared in sufficient water levels and not exposed to RIFA had decreased lipid levels. We also found an effect of temperature where 32°C water increased lipid levels (Table 5).
Growth and development
Tadpoles from 32°C water also developed quicker and were smaller in mass after metamorphosis than tadpoles from 23°C water. However, toadlets from reduced water level treatments as tadpoles had slower growth rates (Table 6).
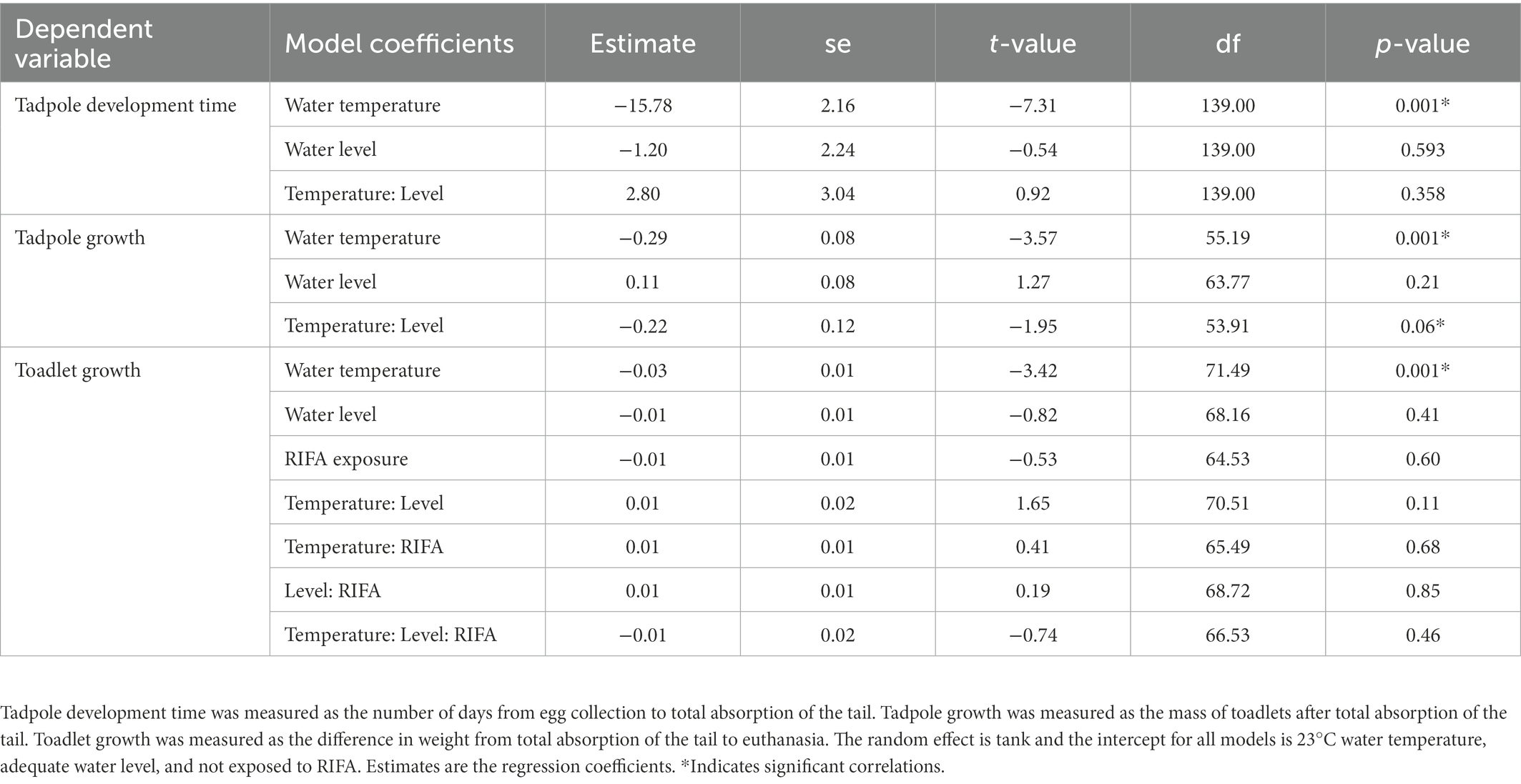
Table 6. Results of mixed-effects models for tadpole development time, tadpole growth, and toadlet growth analyzes for I. nebulifer.
Correlations with corticosterone
We found that total measured bufadienolides were negatively correlated with magnitude of stress response and average (and marginally maximum) hop performance was positively correlated with baseline corticosterone levels. Interestingly, lipid levels were not correlated with any measure of corticosterone (Table 7).
Discussion
We tested the ability of I. nebulifer raised in the lab from eggs to cope with factors commonly associated with anthropogenic environmental change. We found that tadpoles may cope with elevated water temperatures and reduced water levels with elevated baseline corticosterone release rates. After metamorphosis and exposure to RIFA, all toadlets were still able to mount a stress response and recover indicating an active HPI axis in all treatment groups (Wingfield, 2013). Toadlet baseline corticosterone release rates were elevated when exposed to RIFA and one of the larval treatments (moderate anthropogenic alterations: either 32°C water or reduced water level) but when exposed to all three treatments (high anthropogenic alterations) baseline corticosterone release rates were not elevated. However, this treatment group had a larger magnitude of stress response. At moderate urbanization alterations, I. nebulifer may cope by increasing baseline corticosterone release rates, but at high urbanization alterations, I. nebulifer may cope by increasing their magnitude of stress response. In comparison, in a field study Bókony et al. (2021) found that in urbanized ponds, common toad (Bufo bufo) tadpoles had higher baseline corticosterone release rates, a stronger stress response, and a more efficient negative feedback compared to tadpoles in natural and agricultural ponds. Interestingly unlike B. bufo, I. nebulifer toadlets did not alter their negative feedback across treatments. Unlike I. nebulifer, B. bufo struggles to persist in urban environments and is declining in many areas across its range primarily due to habitat alteration and disturbance. While not a perfect comparison, from these two results it might be more beneficial in warmer habitats to have a prolonged stress response particularly when it comes to exposure to biotic stressors like an invasive predator.
Bufadienolide concentration was primarily affected by temperature. Of the toxins measured, arenobufagin decreased with 32°C water and so did marinobufagin, indicating a possible tradeoff between baseline corticosterone release rates (which increased) and toxin production. While baseline corticosterone release rates were not significantly correlated with total measured bufadienolides, the magnitude of the stress response was negatively correlated with total measured bufadienolides giving further support to this tradeoff. However, when exposed to RIFA there was no longer a difference between water temperature treatments in arenobufagin levels. This demonstrates that I. nebulifer recognizes RIFA as a potential predator and can respond with adjustments to bufadienolide concentration. We found surprisingly little bufadienolide compound diversity within individuals, only observing three bufadienolide compounds. However, we did take a conservative approach to the bufadienolide analysis by not including toxins for which we did not have standards. We made an effort to compensate for this by including many toxin standards in our analysis (8 standards in our analysis compared to the average of 3 for most other published studies; Porto and Gros, 1971; Benard and Fordyce, 2003; Hagman et al., 2009; Hayes et al., 2009; Bókony et al., 2017, 2019). That said, because the environmental significance of arenobufagin and marinobufagin in the defense against I. nebulifer predators has not been evaluated, we can make no inferences on the changes in toxicity of I. nebulifer with changes in arenobufagin and marinobufagin concentrations. Follow up studies directly exploring palatability of isolated toxins or individuals raised in differing environments could shed more light on the ecological importance of arenobufagin and marinobufagin. While we did see some correlations and potential tradeoffs in the production of corticosterone and bufadienolides, further studies are needed to understand the link between bufadienolide production and corticosterone. Bufadienolides and corticosterone are both cholesterol-based steroids that respond to both biotic and abiotic environmental stimuli, so they have the potential to share at least some parts of their biochemical pathway. Chemical structures of corticosterone, as well as four common bufadienolides are shown in Figure 1. The bufadienolide family displays a characteristic fifth ring, a dienolide, in the top right of the common steroid structure that is not present in the corticosterone structure. However, the response times to environmental stimuli differ so it is more likely that the relationship is due to resource (energetic or building block) limitations.
Hop performance provides insight on physiological and behavioral avoidance response. Hop performance is highly dependent on size (Beck and Congdon, 2000; Bredeweg et al., 2019; Zamora-Camacho and Aragón, 2019; Moreno-Rueda et al., 2020; Lima-Santos et al., 2021). Larger anurans will have longer hind limbs and more robust muscles allowing them to hop further. However, when size is controlled for, the behavioral aspects of hop performance can be more evident (Putnam and Bennett, 1981; Kowalski et al., 2018; Bredeweg et al., 2019). We considered size corrected maximum and average hop performance as predator avoidance behavior with the understood caveat that muscular motor unit size and coordination have not been accounted for. We found no effect of our treatments on maximum hop performance, but we did find that average hop performance decreased with RIFA exposure. Compared to other anurans, toads are not as active in predator avoidance via hop performance, and in fact are known for being quite clumsy while hopping (Rand, 1952; Putnam and Bennett, 1981; Kowalski et al., 2018). It is likely that toadlets reduce hop performance and rely more on crypsis or toxicity to avoid predation rather than fleeing. We also found that maximum and average hop performance was positively correlated with baseline corticosterone release rates confirming corticosterone’s role in modulating behavior in I. nebulifer.
Total lipid concentration is an estimate of body condition and under a standardized diet can be interpreted as energetic trade-offs among growth, somatic maintenance, and energy storage. During the tadpole stage individuals were fed ad libitum, but during the toadlet stage we standardized the number of flies to the average number of flies consumed by toadlets of a similar age (as determined by preliminary studies). Interpreting our total lipid concentration results in this context, toadlets invested less in energy storage when water levels were low. We also found that toadlets from low water levels grew slower, but tadpole water level did not influence development time. This indicates that either water level alters foraging behavior, or somatic maintenance after development in a low water level environment, is more energetically costly. Toadlets from warmer water invested more in energy storage presumably due to selection favoring increased foraging behavior in habitats of higher productivity such as the warmer water (Fitzpatrick, 1976; Schultz and Conover, 1997; Morey, 1998; Álvarez and Nicieza, 2002). However, tadpoles from warmer water developed faster and had a lower mass after metamorphosis. The tradeoff of investing in energy storage and development at the expense of growth could be the result of two different selection pressures. While warmer water has higher productivity, 32°C is approaching developmental thermal limits and selection could be favoring individuals that are able to develop quickly to escape unfavorable environments (Ballinger and McKinney, 1966). Toadlets exposed to RIFA also had elevated investment of energy storage. Inicilius nebulifer’s initial response to disturbance is crypsis rather than fleeing. The increase in investment of energy storage by toadlets exposed to RIFA might be a result of decreased movement such as that observed with the reduced average hop performance after RIFA exposure or the reduction in baseline corticosterone release rates. Interestingly, lipid concentrations showed no correlation with any measure of the corticosterone profile suggesting that energetic tradeoffs are not mediated by corticosterone levels.
Taken as a whole, we found that I. nebulifer copes with anthropogenic environmental changes with elevated baseline corticosterone release rates or elevated magnitude of the stress response. Bufadienolide production decreases with anthropogenic environmental changes, presumably due to a tradeoff with corticosterone production and supported by a negative correlation between total measured bufadienolides and the magnitude of the stress response, but further testing is needed. Predator avoidance (e.g., hop performance) was decreased in response to predator (RIFA) exposure possibly due to the primary behavioral response to disturbance by toads being cryptic rather than fleeing and this behavior is potentially mediated by baseline corticosterone levels. We also found that toadlets adjust their energy allocation based on the stressor experienced but we found no support that this is mediated by corticosterone. Qualitatively, these findings suggest that I. nebulifer adaptively responds to disturbances associated with anthropogenic change and suggests that I. nebulifer are using urban areas as dispersal pathways (Milko, 2012; Mendelson et al., 2015). The higher baseline corticosterone release rates and stronger stress response with the lack of a more efficient negative feedback demonstrate that a prolonged stress response might be adaptive in warmer environments such as anthropogenically modified areas for I. nebulifer. Future research comparing populations from varying levels of anthropogenic alterations in a common garden experiment could test if the responses we observed are indeed adaptive to anthropogenic environmental change.
Ethics statement
The animal study was reviewed and approved by Texas State University Institutional Animal Care and Use Committee.
Data availability statement
The raw data supporting the conclusions of this article will be made available by the authors, without undue reservation.
Author contributions
DM and CG designed the experiment, analyzed the data, and wrote the manuscript. DM carried out the experiment and collected the data. KM, LB, and AW collected and analyzed the bufadienolide data and made significant edits to the manuscript. All authors contributed to the article and approved the submitted version.
Funding
This research was partially funded by a Texas State University graduate fellowship to DM. We acknowledge a Major Research Instrumentation grant from the National Science Foundation (CHE-2018399 to KM).
Acknowledgments
We thank the GASP lab for help with tadpole maintenance and insightful comments on the manuscript. Specifically, we thank Emily Blumstein for help with behavior and Grayson Overmann for help with tadpole measurements. We also thank Mark Olsen (Villanova University) for his suggestions and insights related to the quantitative LC–MS/MS analysis of bufadienolides.
Conflict of interest
The authors declare that the research was conducted in the absence of any commercial or financial relationships that could be construed as a potential conflict of interest.
Publisher’s note
All claims expressed in this article are solely those of the authors and do not necessarily represent those of their affiliated organizations, or those of the publisher, the editors and the reviewers. Any product that may be evaluated in this article, or claim that may be made by its manufacturer, is not guaranteed or endorsed by the publisher.
Supplementary material
The Supplementary material for this article can be found online at: https://www.frontiersin.org/articles/10.3389/fevo.2023.1112982/full#supplementary-material
Footnotes
1. ^Kinovea.org
References
Alberti, M., Palkovacs, E. P., Roches, S. D., De Meester, L., Brans, K. I., Govaert, L., et al. (2020). The complexity of urban eco-evolutionary dynamics. Bioscience 70, 772–793. doi: 10.1093/biosci/biaa079
Allen, C. R., Birge, H. E., Slater, J., and Wiggers, E. (2017). The invasive ant, Solenopsis Invicta, reduces Herpetofauna richness and abundance. Biol. Invasions 19, 713–722. doi: 10.1007/s10530-016-1343-7
Allen, C. R., Epperson, D. M., and Garmestani, A. S. (2004). Red imported fire ant impacts on wildlife: a decade of research. Am. Midl. Nat. 152, 88–103. doi: 10.1674/0003-0031(2004)152[0088:RIFAIO]2.0.CO;2
Álvarez, D., and Nicieza, A. G. (2002). Effects of induced variation in anuran larval development on Postmetamorphic energy reserves and locomotion. Oecologia 131, 186–195. doi: 10.1007/s00442-002-0876-x
Angelier, F., and Wingfield, J. C. (2013). Importance of the glucocorticoid stress response in a changing world: theory, hypotheses and perspectives. Gen. Comp. Endocrinol. 190, 118–128. doi: 10.1016/j.ygcen.2013.05.022
Ballinger, R. E., and McKinney, C. O. (1966). Developmental temperature tolerance of certain anuran species. J. Exp. Zool. 161, 21–28. doi: 10.1002/jez.1401610104
Beck, C. W., and Congdon, J. D. (2000). Effects of age and size at metamorphosis on performance and metabolic rates of southern toad, Bufo Terrestris, Metamorphs. Funct. Ecol. 14, 32–38. doi: 10.1046/j.1365-2435.2000.00386.x
Benard, M. F., and Fordyce, J. A. (2003). Are induced Defences costly? Consquences of predator-induced defenses in Western toads, Bufo Boreas. Ecology 84, 68–78. doi: 10.1890/0012-9658(2003)084[0068:AIDCCO]2.0.CO;2
Bliley, J. M., and Woodley, S. K. (2012). The effects of repeated handling and corticosterone treatment on behavior in an amphibian (Ocoee salamander: Desmognathus Ocoee). Physiol. Behav. 105, 1132–1139. doi: 10.1016/j.physbeh.2011.12.009
Bókony, V., Mikó, Z., Móricz, Á. M., Krüzselyi, D., and Hettyey, A. (2017). Chronic exposure to a glyphosate-based herbicide makes toad larvae more toxic. Proc. R. Soc. B Biol. Sci. 284:20170493. doi: 10.1098/rspb.2017.0493
Bókony, V., Ujhegyi, N., Hamow, K. Á., Bosch, J., Thumsová, B., Vörös, J., et al. (2021). Stressed tadpoles mount more efficient glucocorticoid negative feedback in anthropogenic habitats due to phenotypic plasticity. Sci. Total Environ. 753:141896. doi: 10.1016/j.scitotenv.(2020).141896
Bókony, V., Üveges, B., Verebélyi, V., Ujhegyi, N., and Móricz, Á. M. (2019). Toads phenotypically adjust their chemical Defences to anthropogenic habitat change. Sci. Rep. 9:3163. doi: 10.1038/s41598-019-39587-3
Bonnet, X., Shine, R., and Lourdais, O. (2002). Taxonomic chauvinism. Trends Ecol. Evol. 17, 1–3. doi: 10.1016/S0169-5347(01)02381-3
Brans, K. I., Engelen, J. M. T., Souffreau, C., and De Meester, L. (2018). Urban hot-tubs: Local urbanization has profound effects on average and extreme temperatures in ponds. Landsc. Urban. Plan. 176, 22–29. doi: 10.1016/j.landurbplan.2018.03.013
Bredeweg, E. M., Morzillo, A. T., Thurman, L. L., and Garcia, T. S. (2019). The integrative effects of behavior and morphology on amphibian movement. Ecol. Evol. 9, 1278–1288. doi: 10.1002/ece3.4837
Burraco, P., and Gomez-Mestre, I. (2016). Physiological stress responses in amphibian larvae to multiple stressors reveal marked anthropogenic effects even below lethal levels. Physiol. Biochem. Zool. 89, 462–472. doi: 10.1086/688737
Carr, J. A., and Norris, D. O. (1990). Immunohistochemical localization of Corticotropin-releasing factor-like and arginine Vasotocin-like immunoreactivities in the brain and pituitary of the American bullfrog (Rana-Catesbeiana) during development and metamorphosis. Gen. Comp. Endocrinol. 78, 180–188. doi: 10.1016/0016-6480(90)90004-6
Cha, E. S., Uhrin, M. T., McClelland, S. J., and Woodley, S. K. (2021). Brain plasticity in response to short-term exposure to corticosterone in larval amphibians. Can. J. Zool. 99, 839–844. doi: 10.1139/cjz-2021-0031
Charbonnier, J., Pearlmutter, J., Vonesh, J., Gabor, C., Forsburg, Z., and Grayson, K. (2018). Cross-life stage effects of aquatic larval density and terrestrial moisture on growth and corticosterone in the spotted salamander. Diversity 10:68. doi: 10.3390/d10030068
Clarke, B. T. (1997). The natural history of amphibian skin secretions, their normal functioning and potential medical applications. Biol. Rev. 72, 365–379. doi: 10.1111/j.1469-185X.1997.tb00018.x
Cockrem, J. F. (2013). Individual variation in glucocorticoid stress responses in animals. Gen. Comp. Endocrinol. 181, 45–58. doi: 10.1016/j.ygcen.2012.11.025
Coloma, A. S., Garraffo, H. M., Pignataro, O. P., and Charreau, E. H. (1984). Biosynthesis of Bufadienolides in toads. V. the origin of the cholesterol used by toad Parotoid glands for biosynthesis of Bufadienolides. Steroids 44, 11–22. doi: 10.1016/S0039-128X(84)80012-4
Cordier, J. M., Aguilar, R., Lescano, J. N., Leynaud, G. C., Bonino, A., Miloch, D., et al. (2021). A global assessment of amphibian and reptile responses to land-use changes. Biol. Conserv. 253:108863. doi: 10.1016/j.biocon.2020.108863
Crespi, E. J., Williams, T. D., Jessop, T. S., and Delehanty, B. (2013). Life history and the ecology of stress: how do glucocorticoid hormones influence life-history variation in animals? Funct. Ecol. 27, 93–106. doi: 10.1111/1365-2435.12009
Crump, M. L. (1981). Energy accumulation and amphibian metamorphosis. Oecologia 49, 167–169. doi: 10.1007/BF00349184
Eikenaar, C., Husak, J., Escallón, C., and Moore, I. T. (2012). Variation in testosterone and corticosterone in amphibians and reptiles: relationships with latitude, elevation, and breeding season length. Am. Nat. 180, 642–654. doi: 10.1086/667891
Fitzpatrick, L. C. (1976). Life history patterns of storage and utilization of lipids for energy in amphibians. Am. Zool. 16, 725–732. doi: 10.1093/icb/16.4.725
Fonner, C. W., and Woodley, S. K. (2015). Testing the predation stress hypothesis: behavioural and hormonal responses to predator cues in Allegheny Mountain dusky salamanders. Behaviour 152, 797–819. doi: 10.1163/1568539x-00003254
Forsburg, Z. R., Goff, C. B., Perkins, H. R., Robicheaux, J. A., Almond, G. F., and Gabor, C. R. (2019). Validation of water-borne cortisol and corticosterone in tadpoles: recovery rate from an acute stressor, repeatability, and evaluating rearing methods. Gen. Comp. Endocrinol. 281, 145–152. doi: 10.1016/j.ygcen.2019.06.007
Gabor, C. R., Davis, D. R., Kim, D. S., Zabierek, K. C., and Bendik, N. F. (2018). Urbanization is associated with elevated corticosterone in Jollyville plateau salamanders. Ecol. Indic. 85, 229–235. doi: 10.1016/j.ecolind.2017.10.047
Gabor, C. R., Fisher, M. C., and Bosch, J. (2013). A non-invasive stress assay shows that tadpole populations infected with Batrachochytrium dendrobatidis have elevated corticosterone levels. PLoS One 8:e56054. doi: 10.1371/journal.pone.0056054
Gabor, C. R., Perkins, H. R., Heitmann, A. T., Forsburg, Z. R., and Aspbury, A. S. (2019). Roundup™ with corticosterone functions as an Infodisruptor to antipredator response in tadpoles. Front. Ecol. Evol. 7:114. doi: 10.3389/fevo.2019.00114
Garraffo, H. M., and Gros, E. G. (1986). Biosynthesis of Bufadienolides in toads. VI. Experiments with [1, 2-3H] cholesterol,[21-14C] Coprostanol, and 5β-[21-14C] Pregnanolone in the toad Bufo Arenarum. Steroids 48, 251–257. doi: 10.1016/0039-128X(86)90008-5
Goff, C. B., Susan, C. W., David, R., and Gabor, C. R. (2020). Changes in physiology and microbial diversity in larval ornate chorus frogs are associated with habitat quality. Conserv. Physiol. 8:coaa047. doi: 10.1093/conphys/coaa047
Hagman, M., Hayes, R. A., Capon, R. J., and Shine, R. (2009). Alarm cues experienced by cane toad tadpoles affect post-metamorphic morphology and chemical defences. Funct. Ecol. 23, 126–132. doi: 10.1111/j.1365-2435.2008.01470.x
Hau, M., Casagrande, S., Ouyang, J. Q., and Baugh, A. T. (2016). Glucocorticoid-mediated phenotypes in vertebrates. Adv. Study Behav. 48, 41–115. doi: 10.1016/bs.asb.2016.01.002
Hayes, R., Andrew, M. R., Crossland, M. H., Capon, R. J., and Shine, R. (2009). Ontogenetic variation in the chemical defenses of cane toads (Bufo Marinus): toxin profiles and effects on predators. J. Chem. Ecol. 35, 391–399. doi: 10.1007/s10886-009-9608-6
Homer, C., Dewitz, J., Jin, S., Xian, G., Costello, C., Danielson, P., et al. (2020). Conterminous United States land cover change patterns 2001–2016 from the 2016 National Land Cover Database. ISPRS J. Photogramm. Remote Sens. 162, 184–199. doi: 10.1016/j.isprsjprs.2020.02.019
Hopkins, W. A., Mendonça, M. T., and Congdon, J. D. (1997). Increased circulating levels of testosterone and corticosterone in southern Toads, Bufo Terrestris, Exposed to coal combustion waste. Gen. Comp. Endocrinol. 108, 237–246. doi: 10.1006/gcen.1997.6969
Kaushal, S. S., Likens, G. E., Jaworski, N. A., Pace, M. L., Sides, A. M., Seekell, D., et al. (2010). Rising stream and river temperatures in the United States. Front. Ecol. Environ. 8, 461–466. doi: 10.1890/090037
Kolonin, A. M., Bókony, V., Bonner, T. H., Zúñiga-Vega, J. J., Aspbury, A. S., Guzman, A., et al. (2022). Coping with urban habitats via glucocorticoid regulation: physiology, behavior, and life history in stream fishes. Integr. Comp. Biol. 62, 90–103. doi: 10.1093/icb/icac002
Kowalski, K., Sawościanik, O., and Rychlik, L. (2018). Do Bufonids employ different anti-predator behaviors than Ranids? Comparison among three European anurans. Copeia 106, 120–129. doi: 10.1643/CE-16-567
Lattin, C. R., and Kelly, T. R. (2019). Glucocorticoid negative feedback as a potential mediator of trade-offs between reproduction and survival. Gen. Comp. Endocrinol., October:113301. doi: 10.1016/j.ygcen.2019.113301
Lima-Santos, J., Carvalho, J. E., and Brasileiro, C. A. (2021). How sexual differences affect locomotor performance and metabolism of the sword snake: an integrated view from energetics. J. Zool. 315, 149–155. doi: 10.1111/jzo.12908
MacCracken, J. G., and Stebbings, J. L. (2012). Test of a body condition index with amphibians. J. Herpetol. 46, 346–350. doi: 10.1670/10-292
Maciel, T. A., and Juncá, F. A. (2009). Effects of temperature and volume of water on the growth and development of tadpoles of Pleurodema Diplolister and Rhinella Granulosa (Amphibia: Anura). Zoologia (Curitiba) 26, 413–418. doi: 10.1590/S1984-46702009000300005
Mendelson, J. R., Kinsey, C. T., and Murphy, J. B. (2015). A review of the biology and literature of the Gulf coast toad (Incilius nebulifer), native to Mexico and the United States. Zootaxa 3974, 517–537. doi: 10.11646/zootaxa.3974.4.4
Middlemis, M. J., Werner, E. E., and Denver, R. J. (2013). Stress hormones mediate predator-induced phenotypic plasticity in amphibian tadpoles. Proc. R. Soc. B Biol. Sci. 280:20123075. doi: 10.1098/rspb.2012.3075
Milko, L. V. (2012). Integrating museum and GIS data to identify changes in species distributions driven by a disturbance-induced invasion. Copeia 2012, 307–320. doi: 10.1643/CE-10-159
Moreno-Rueda, G., Requena-Blanco, A., Zamora-Camacho, F. J., Comas, M., and Pascual, G. (2020). Morphological determinants of jumping performance in the Iberian Green frog. Curr. Zool. 66, 417–424. doi: 10.1093/cz/zoz062
Morey, S. R. (1998). “Pool duration influences age and body mass at metamorphosis in the Western spadefoot toad: implications for vernal Pool conservation.” C. W. Witham, E. T. Bauder, D. Belk, W. R. Ferren Jr., and R. Ornduff (Eds.), Ecology, Conservation, and Management of Vernal Pool Ecosystems, California Native Plant Society: Sacramento, CA: California Native Plant Society, 86–91.
Narayan, E. J., Cockrem, J. F., and Hero, J.-M. (2012). Effects of temperature on urinary corticosterone metabolite responses to short-term capture and handling stress in the cane toad (Rhinella marina). Gen. Comp. Endocrinol. 178, 301–305. doi: 10.1016/j.ygcen.2012.06.014
Narayan, E. J., and Hero, J.-M. (2014). Acute thermal stressor increases glucocorticoid response but minimizes testosterone and locomotor performance in the cane toad (Rhinella marina). PLoS One 9:e92090. doi: 10.1371/journal.pone.0092090
Nelson, K. C., and Palmer, M. A. (2007). Stream temperature surges under urbanization and climate change: data, models, and responses. JAWRA J. Am. Water Resour. Assoc. 43, 440–452. doi: 10.1111/j.1752-1688.2007.00034.x
Porto, A. M., Baralle, F. E., and Gros, E. G. (1972). Biosynthesis of Bufadienolides in toads. J. Steroid Biochem. 3, 11–17. doi: 10.1016/0022-4731(72)90006-4
Porto, A. M., and Gros, E. G. (1971). Biosynthesis of the Bufadienolide Marinobufagin in toads Bufo Paracnemis from Cholesterol-20-14 C. Experientia 27:506. doi: 10.1007/BF02147562
Puig-Diví, A., Escalona-Marfil, C., Padullés-Riu, J. M., Busquets, A., Padullés-Chando, X., and Marcos-Ruiz, D. (2019). Validity and reliability of the Kinovea program in obtaining angles and distances using coordinates in 4 perspectives. PLoS ONE 14:e0216448. doi: 10.1371/journal.pone.0216448
Putnam, R. W., and Bennett, A. F. (1981). Thermal dependence of behavioural performance of anuran amphibians. Anim. Behav. 29, 502–509. doi: 10.1016/S0003-3472(81)80111-X
Rand, A. S. (1952). Jumping ability of certain anurans, with notes on endurance. Copeia 1952:15. doi: 10.2307/1437615
Relyea, R. A. (2001). The lasting effects of adaptive plasticity: predator-induced tadpoles become long-legged frogs. Ecology 82, 1947–1955. doi: 10.1890/0012-9658(2001)082[1947:TLEOAP]2.0.CO;2
Relyea, R. A., and Edwards, K. (2010). What doesn’t kill you makes you sluggish: how sublethal pesticides Alter predator–prey interactions. Copeia 2010, 558–567. doi: 10.1643/CE-09-027
Relyea, R. A., and Hoverman, J. T. (2003). The impact of larval predators and competitors on the morphology and fitness of juvenile Treefrogs. Oecologia 134, 596–604. doi: 10.1007/s00442-002-1161-8
Ricciardella, L. F., Bliley, J. M., Feth, C. C., and Woodley, S. K. (2010). Acute stressors increase plasma corticosterone and decrease locomotor activity in a terrestrial salamander (Desmognathus ochrophaeus). Physiol. Behav. 101, 81–86. doi: 10.1016/j.physbeh.2010.04.022
Sapolsky, R. M., Romero, M. L., and Munck, A. U. (2000). How do glucocorticoids influence stress responses? Integrating permissive, suppressive, stimulatory, and preparative actions. Endocr. Rev. 21, 55–89. doi: 10.1210/er.21.1.55
Scheffers, B. R., and Paszkowski, C. A. (2012). The effects of urbanization on north American amphibian species: identifying new directions for urban conservation. Urban Ecosyst. 15, 133–147. doi: 10.1007/s11252-011-0199-y
Schultz, E. T., and Conover, D. O. (1997). Latitudinal differences in somatic energy storage: adaptive responses to seasonality in an estuarine fish (Atherinidae: Menidia menidia). Oecologia 109, 516–529. doi: 10.1007/s004420050112
Scott, D. E., Casey, E. D., Donovan, M. F., and Lynch, T. K. (2007). Amphibian lipid levels at metamorphosis correlate to post-metamorphic terrestrial survival. Oecologia 153, 521–532. doi: 10.1007/s00442-007-0755-6
Shochat, E., Lerman, S. B., Anderies, J. M., Warren, P. S., Faeth, S. H., and Nilon, C. H. (2010). Invasion, competition, and biodiversity loss in urban ecosystems. Bioscience 60, 199–208. doi: 10.1525/bio.2010.60.3.6
Sinsch, U., Leus, F., Sonntag, M., and Hantzschmann, A. M. (2020). Carry-over effects of the larval environment on the post-metamorphic performance of Bombina variegata (Amphibia, Anura). Herpetol. J. 30, 126–134. doi: 10.33256/hj30.3.126134
Smith, L. M., Gray, M. J., and Quarles, A. (2004). Diets of newly metamorphosed amphibians in West Texas playas. Southwest. Nat. 49, 257–263. doi: 10.1894/0038-4909(2004)049<0257:DONMAI>2.0.CO;2
Song, X.-P., Hansen, M. C., Stehman, S. V., Potapov, P. V., Tyukavina, A., Vermote, E. F., et al. (2018). Global land change from 1982 to 2016. Nature 560, 639–643. doi: 10.1038/s41586-018-0411-9
Todd, B. D., Rothermel, B. B., Reed, R. N., Luhring, T. M., Schlatter, K., Trenkamp, L., et al. (2008). Habitat alteration increases invasive fire ant abundance to the detriment of amphibians and reptiles. Biol. Invasions 10, 539–546. doi: 10.1007/s10530-007-9150-9
Vogel, L. S., and Pechmann, J. H. K. (2010). Response of Fowler’s toad (Anaxyrus Fowleri) to competition and Hydroperiod in the presence of the invasive coastal plain toad (Incilius nebulifer). J. Herpetol. 44, 382–389. doi: 10.1670/08-300.1
Von Der Lippe, M., Buchholz, S., Hiller, A., Seitz, B., and Kowarik, I. (2020). CityScapeLab Berlin: a research platform for untangling urbanization effects on biodiversity. Sustainability 12:2565. doi: 10.3390/su12062565
Wack, C. L., Ratay, M. K., and Woodley, S. K. (2013). Effects of corticosterone on Locomotory activity in red-legged salamanders. Herpetologica 69, 118–126. doi: 10.1655/HERPETOLOGICA-D-12-00040R2
Wingfield, J. C. (2013). The comparative biology of environmental stress: behavioural endocrinology and variation in ability to cope with novel, changing environments. Anim. Behav. 85, 1127–1133. doi: 10.1016/j.anbehav.2013.02.018
Woodley, S. K., and Lacy, E. L. (2010). An acute stressor alters steroid hormone levels and activity but not sexual behavior in male and female Ocoee salamanders (Desmognathus ocoee). Horm. Behav. 58, 427–432. doi: 10.1016/j.yhbeh.2010.05.011
Yagi, K. T., and Green, D. M. (2018). Post-metamorphic carry-over effects in a complex life history: behavior and growth at two life stages in an amphibian, Anaxyrus fowleri. Copeia 106, 77–85. doi: 10.1643/CE-17-593
Keywords: amphibian, behavior, bufadienolide, Bufonidae, corticosterone, physiology
Citation: Monroe DJ, Barny LA, Wu A, Minbiole KPC and Gabor CR (2023) An integrated physiological perspective on anthropogenic stressors in the Gulf coast toad (Incilius nebulifer). Front. Ecol. Evol. 11:1112982. doi: 10.3389/fevo.2023.1112982
Edited by:
Melissa Lin Grunst, Université de la Rochelle, FranceReviewed by:
Fernando Ribeiro Gomes, University of São Paulo, BrazilLéa Lorrain-Soligon, UMR7372 Centre d'études biologiques de Chizé (CEBC), France
Copyright © 2023 Monroe, Barny, Wu, Minbiole and Gabor. This is an open-access article distributed under the terms of the Creative Commons Attribution License (CC BY). The use, distribution or reproduction in other forums is permitted, provided the original author(s) and the copyright owner(s) are credited and that the original publication in this journal is cited, in accordance with accepted academic practice. No use, distribution or reproduction is permitted which does not comply with these terms.
*Correspondence: Caitlin R. Gabor, ✉ gabor@txstate.edu