- 1Finnish Museum of Natural History, University of Helsinki, Helsinki, Finland
- 2The Cleveland Museum of Natural History, Cleveland, OH, United States
The origin of the dung-feeding habits in dung beetles (Coleoptera: Scarabaeinae) is debatable. According to traditional views, the evolution of dung beetles (Coleoptera: Scarabaeinae) and their feeding habits are largely attributed to mammal dung. In this paper, we challenge this view and provide evidence that many dung beetle communities are actually attracted to the dung of reptiles and birds (= Sauropsida). In turn, this indicates that sauropsid dung may have played a crucial evolutionary role that was previously underestimated. We argue that it is physiologically realistic to consider that coprophagy in dung beetles could have evolved during the Cretaceous in response to the dung produced by dinosaurs. Furthermore, we demonstrate that sauropsid dung may be one of the major factors driving the emergence of insular dung beetle communities across the globe. We support our findings with amplicon-metagenomic analyses, field observations, and meta-analysis of the published literature.
1. Introduction
With over 6,000 species, dung beetles or scarabaeines (Coleoptera: Scarabaeinae) mostly feed on mammalian dung, particularly that of herbivores, and serve as primary decomposers of feces on Earth (Hanski and Cambefort, 1991; Nichols et al., 2008) directly affecting ecosystem services and processes like nutrient cycling, soil aeration, and chemical composition (deCastro Arrazola et al., 2022). The dung beetle diversification is suggested to be linked to the radiation of mammals in the Cenozoic (Scholtz et al., 2009; O'Leary et al., 2013; Gunter et al., 2016), while the origin of dung-feeding behavior is proposed to have happened early in dung beetle evolution by shifting from saprophagy to coprophagy (Hanski and Cambefort, 1991). In most cases, dung beetles are found to be associated with the feces of mammals (Stavert et al., 2014). However, a few other vertebrates whose dung is known to be consumed by dung beetles are birds, reptiles, and amphibians, but such records are rare (Halffter and Matthews, 1966; Fincher et al., 1970; Young, 1981; Kabir et al., 1990; Gill, 1991; Stavert et al., 2014).
In the present study, we demonstrate that, even though mammalian feces are undeniably important, the role of bird and reptile (= Sauropsida) dung may have been greatly undervalued in the evolution of dung beetles. In Madagascar and Mauritius, we set pitfall traps and sequenced the DNA from the dung visited in natura by dung beetles using amplicon-metagenomics to identify the dung's host species. Additionally, we performed a meta-analysis of published literature to retrieve all available records associated with sauropsid dung and to assess their overall proportion. According to our empirical findings and meta-analysis, insular dung beetles often rely on reptile and bird dung as a feeding resource, which was underappreciated previously. Whether feeding on bird and reptile dung was an ancient or a recent trait acquired by the insular communities is not clear and we discuss it below. The most likely explanation for this behavior is that insular dung beetles are generalists (Jones et al., 2012), and that they evolved this trait due to the food deficiency on the islands caused by the absence of native large mammals (Stavert et al., 2014; Ebert et al., 2019; Langton-Myers, 2022).
Our results provide further evidence that physiologically speaking, there is no restriction that the evolution of coprophagy in dung beetles could have been triggered by dinosaur dung. Based on available studies, dung beetles are estimated to have originated between 132 and 40 Mya (Ahrens et al., 2014; Gunter et al., 2016; Davis et al., 2017; McKenna et al., 2019). This period equivocally fits both scenarios regarding the origin of dung-feeding behavior, i.e., dinosaurs vs. mammals. Below, we reevaluate the scenarios in light of our findings. Overall, we provide evidence that sauropsid dung could play a crucial role in the emergence of coprophagy, and that it may be one of the major factors driving the establishment of insular dung beetle communities.
2. Methods
2.1. Pitfall traps
In February 2021, we set pitfall traps in two Mauritian localities: Mount Le Pouce Nature Reserve (−20.1986, 57.5294) and Black River Gorges National Park (−20.3755, 57.4442; −20.4415, 57.47229). In this locality, we set up 13 traps baited with chicken dung. In March 2022, we also set two traps in the Kirindy village area, Madagascar (−20.066805, 44.657255), with chicken and reptile dung, respectively (Figure 1A).
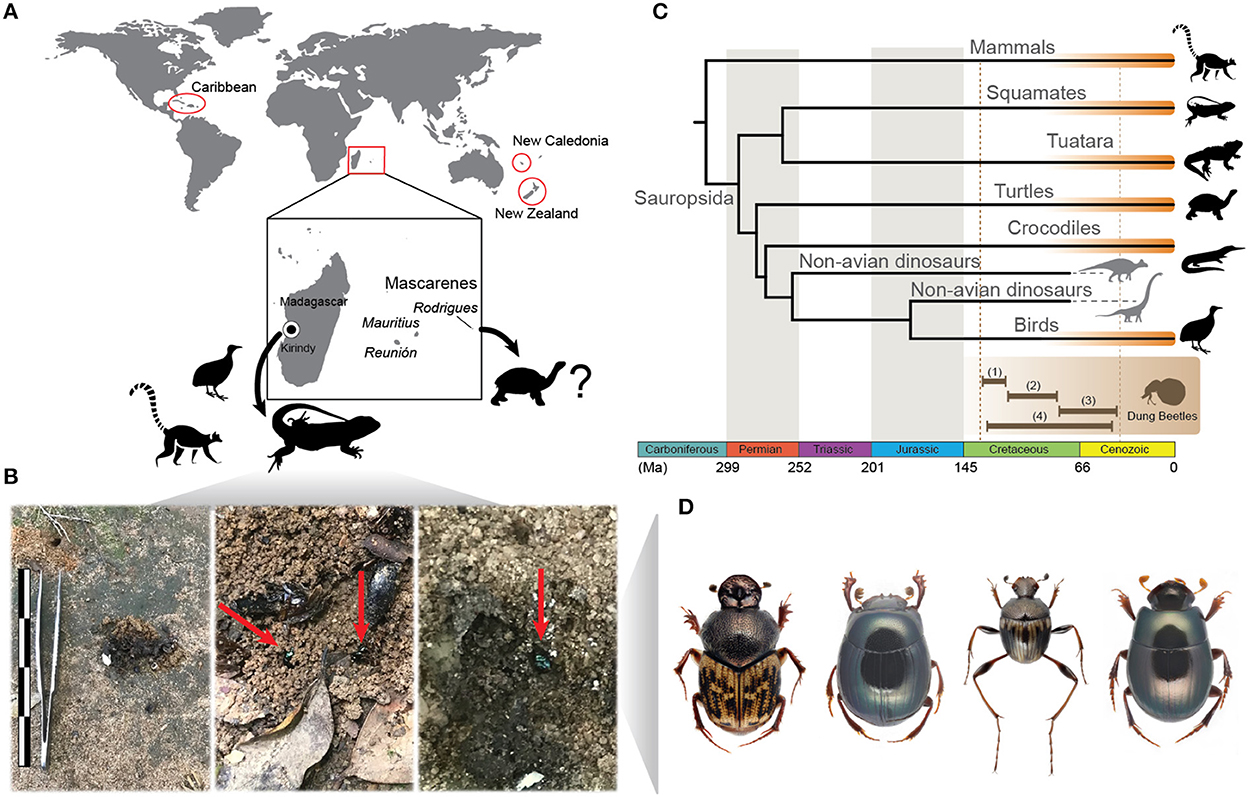
Figure 1. (A) Five island groups with authentic dung beetle communities: Madagascar, Mascarenes, New Caledonia, New Zealand, and Caribbean; in the center are the vertebrates whose dung supports dung beetle communities in Madagascar and Mascarenes; the point on Madagascar indicates Kirindy village area. (B) Photos of squamate dung visited by Helictopleurus species in Kirindy; the scale bar is 14 cm in length. (C) Phylogenetic tree illustrating which clades of sauropsids and mammals produce suitable dung for scarabaeines (the clades are highlighted in orange); the brown lines on the bottom indicate the ages for the origin of dung beetles according to: (1) Gunter et al. (2016), (2) Ahrens et al. (2014), (3) McKenna et al. (2019), and (4) Davis et al. (2017). (D) Dung beetles recorded on sauropsid dung, from left to right: Helictopleurus infimus (Madagascar), Apotolamprus metallicus (Madagascar), Nesosisyphus vicinus (Mauritius), and Nesovinsonia vinsoni (Mauritius).
2.2. Sampling dung for metagenomics
In March 2022 at Kirindy village area, Madagascar (−20.074773, 44.590188; −20.066805, 44.657255), we collected ~30 fecal samples, of which ~70% contained specimens of dung beetles (see Section 3, Figures 1A, B). We primarily identified them as belonging to sauropsids. For the identification of the host species, we selected six pellets for amplicon-metagenomic analyses.
2.3. DNA extraction, library preparation, and sequencing
For each sample, we performed DNA extractions using QIAGEN DNeasy PowerSoil Kit and fecal samples (up to 0.25 g) following the manufacturer's protocol. The purified DNA was quantified using Qubit fluorometer 4.0, high-sensitivity reagents, and 5 μl of DNA extract. The DNA extracts were used for amplicon-metagenomics sequencing using COI universal primers Sauron-878 (5-′GGDRCWGGWTGAACWGTWTAYCCNCC-3′; Rubbmark et al., 2018) and jgHCO2198 (5'-TAIACYTCIGGRTGICCRAARAAYCA-3′; Geller et al., 2013) and PCR profile following the protocol of Rubbmark et al. (2018). The PCR products were selected from 2% agarose gel electrophoresis and the same amount from each sample was pooled and tailed with Illumina adapters (Supplementary Table 1). Libraries were prepared using the NEBNextⓇ UltraTM II DNA Library Prep Kit, checked with Qubit (high-sensitive reagents) and real-time PCR for quantification, and Bioanalyzer for size distribution detection. The amplicon paired-end libraries (PE250) targeting an insert size of 320 bp were sequenced on an Illumina NovaSeq 6000 platform aiming for 30 K raw tags (raw reads generated from the opposite end of the same DNA fragment) per sample. Library preparation, quality control, and sequencing were performed at Novogene (Cambridge, UK).
2.4. Bioinformatics
Sequenced reads were demultiplexed based on barcode sequences for each sample using Qiime 1.7.0 (Bokulich et al., 2013). Reads with sequencing Phred-score < 30 and chimeric reads were filtered out from the downstream pipeline. Cleaned paired-end reads (barcode and primer-free sequences) were merged with FLASH 1.2.7 (Chaisson et al., 2004). The Operational Taxonomic Units (OTUs) clustering was carried out on cleaned and merged paired-end sequences (Effective Tags). To obtain community composition in each fecal sample, OTUs were assembled from sequences with ≥ 97% of similarity using Uparse 10 (Edgar, 2013). The taxonomic annotation was automatically constructed using NCBI BLAST+ 2.13 and the COI database.
From the full annotation table, we extracted and quantified reads assigned to vertebrates (aiming at host identification). We double-checked the OTUs automatically assigned by blasting the sequences manually against the NCBI database with the BLASTn algorithm.
To validate the identification of the hosts, we recovered a Maximum-Likelihood (ML) phylogeny for the COI gene with the most representative OTUs (≥94% of the Effective Tags assigned to sauropsids, see Section 3) from each sample and sequences obtained from GenBank for closely related Madagascan species. Sequences were aligned with MAFFT 7.490 (Katoh and Standley, 2013) and manually checked and trimmed with MEGA X (Kumar et al., 2018). The ML phylogeny of the host species was recovered with IQ-Tree 2.0.3 (Minh et al., 2020), 1,000 replicates of ultrafast bootstrap, and SH approximate likelihood ratio test (aLRT).
2.5. Meta-analysis of literature
The feeding records data set was assembled using our data and an extensive literature survey on dung beetles (Supplementary Table 6). In order to test the hypothesis that feeding on sauropsid dung is more likely on islands than on continents, we use a binomial test. In our null hypothesis, finding a sauropsid dung feeder is equally likely on islands and continents. Therefore, the total number of insular records should be proportional to the relative area of islands. This area was calculated by using data from Sayre et al. (2019) for the islands with an area of over one kilometer squared and by subtracting the area of Greenland (since no dung beetles occur there). The total area of the continental landmasses was calculated as a sum of the areas of Afro-Eurasia, the Americas, and Australia (dung beetles do not occur in Antarctica). We considered Australia as a continental environment, given its peculiar landmass and number of dung beetle lineages (see Section 4). The relative area of islands was thus 0.059. According to the null hypothesis, insular dung beetle records should follow the binomial distribution with a probability of 0.059. Our alternative hypothesis proposes that sauropsid dung feeders are more common on islands than on continents. The R script to perform the test is available at doi: 10.17605/OSF.IO/JH3AM.
3. Results
3.1. Dung beetles sampled in sauropsid dung
In reptile pellets, dung beetles were observed by us conducting their normal activities and spending substantial time in the dung. The droppings in which dung beetles were found were bored and disrupted, showing signs of intense dung beetle activity commonly associated with feeding and/or food relocation behavior (see Figure 1B).
The pitfall traps set in Mauritius clearly showed a high attractiveness of native dung beetles to chicken excrement. The samplings allowed us to recollect four of the five known Scarabaeinae species endemic to the island: Nesosisyphus regnardi (Alluaud, 1898) (Mount Le Pouce), N. pygmaeus Vinson, 1946 (Mount Ory), N. vicinus Vinson, 1939 (Mount Cocotte), and Nesovinsonia vinsoni (Paulian, 1939; Mount Le Pouce). Although the majority of dung beetles were captured using chicken dung, including the only four specimens of the monotypic genus Nesovinsonia, some Nesosisyphus specimens were also collected using human excrement, and among them the only specimen of N. pygmaeus collected during the field trip. It is noteworthy that about 10 specimens of N. regnardi were collected using pitfall traps baited with the carrion of the invasive giant snail Lissachatina fulica (Bowdich, 1822). The same behavior was formerly reported for N. pygmaeus, which was observed feeding on the excrement of the endemic Mauritian snail Pachystyla bicolor (Vinson, 1951).
In Kirindy village, north of the Kirindy National Park, Madagascar, a total of 140 specimens were collected in sauropsid dung. The pitfall trap baited with chicken dung yielded four specimens and four species: Apotolamprus metallicus Montreuil, 2008; Epilissus cuprarius Fairmaire, 1899; and two unidentified Arachnodes Westwood, 1,842 species, tentatively related to A. kelifelyi Paulian, 1976 and A. pillula Paulian, 1976. Instead, reptile dung (both in pitfall and sampled by hand) yielded 136 dung beetle specimens belonging to six species as follows: Onthophagus pipitzi Ancey, 1883, Helictopleurus infimus (Fairmaire, 1901) and H. peyrierasi Paulian and Cambefort, 1991; A. metallicus; Nanos humeralis; Paulian, 1975; and an unidentified Arachnodes species possibly related to A. kelifelyi (Figure 1D). In reptile dung, H. infimus was the most abundant species, followed by A. metallicus and O. pipitzi (Figure 1D).
3.2. Metagenomics: Identifying dung hosts
We recovered 349,012 Effective Tags from 399,605 Raw Tags (reads), with an average of 58,170 (SD ± 20.9) Effective Tags per sample. This amount represents 86% (SD ± 8.9) of the raw reads with Phred-score >30 (Supplementary Table 2). In the process of constructing OTUs, summary information from different samples was collected, such as Effective Tags, low-frequency Tags, and Tags' annotation. The summary is shown in Supplementary Figure 1.
We found a relatively high alpha (Supplementary Figure 2) and beta diversity (Supplementary Figures 3, 4), with the majority of the reads belonging to microorganisms like bacteria and fungi (Supplementary Figure 2). This high diversity of microorganisms was expected since the source of the DNA was organic material (excrement) in different stages of decomposition. However, from the total number of Effective Tags generated, 40,743 were assigned to vertebrates. Of this number, 99.95% were assigned to species of reptiles (Figure 2A), while the remaining 0.05% were assigned to mammals and therefore considered to be contamination (Supplementary Tables 3, 4 and Supplementary Figure 5). Under this number and among the six fecal samples, the proportion of reads assigned to the same species of reptile ranged from 94.2 to 100%, indicating that the most representative OTUs probably represent the host species (Figure 2A). We found no traces of dung beetles (Scarabaeinae) among the sequences identified as Coleoptera (Supplementary Table 5).
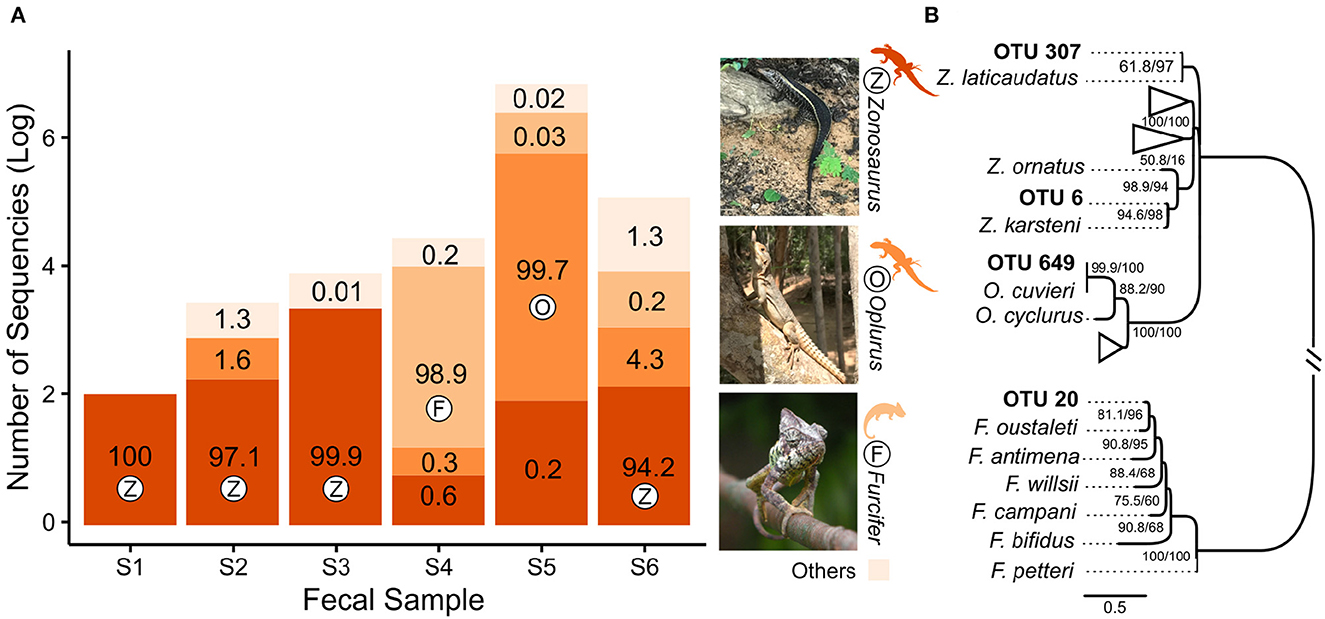
Figure 2. Results of the amplicon-metagenomic analysis. (A) The proportion of Effective Tags (log-scale) within each fecal sample (S1–S6); numbers in the bars show relative abundance (%) of reads; colors and letters (Z, F, O) represent squamate genera shown on the right; in each fecal sample, the genus with the highest proportion of Effective Tags is marked with the respective letter. (B) Maximum-Likelihood tree, based on COI region, that shows the phylogenetic position of squamate OTUs from the fecal samples; the node numbers are the ultra-fast bootstrap support and SH-aLRT values, respectively; full phylogeny is available in Supplementary Figure 6. The picture of Furcifer oustaleti (male), Lake Ravelobe, Madagascar, by Frank Vassen is licensed under CC BY 2.0. The image was slightly cropped to fit the layout.
The phylogeny inferred using the COI gene, OTUs, and sequences obtained from GenBank (Figure 2B and Supplementary Figure 6) validated the OTU assignment and allowed the identification of the hosts at the species level. The phylogeny indicated four host species belonging to three families, respectively: fecal samples S1, S2, and S6 clustered with Zonosaurus laticaudatus (Grandidier, 1869) and sample S3 with Z. karsteni (Grandidier, 1869; Iguanidae); sample S4 with Furcifer oustaleti (Mocquard, 1894; Chamaleonidae); and sample S5 with Oplurus cuvieri Gray, 1831 (Opluridae; Figure 2B and Supplementary Figure 6). The distribution maps available on the IUCN Red List of Threatened Species (iucnredlist.org) and iNaturalist (inaturalist.org) databases confirm that all species potentially occur at Kirindy National Park and surrounding areas.
3.3. Meta-analysis of literature
Our survey found 80 records reporting the attraction of scarabaeine dung beetles to sauropsid dung (Supplementary Table 6). Sixteen records were insular (Madagascar, Mauritius, New Zealand, Puerto Rico), while the other 64 were continental. The continental records cover the Americas, Australia, Asia, and Africa. Dung from all major Sauropsida clades (Squamates, Tuataras, Turtles, Crocodiles, and Birds) was found to attract dung beetles (Figure 1C).
The one-sided binomial test rejects the null hypothesis that finding a sauropsid dung feeder is equally likely on islands and continents (p-value = 0.00001). Contrary to that, our findings support the alternative hypothesis that sauropsid dung feeders are 3.4 times more likely to be found on islands.
4. Discussion
4.1. Insular dung beetles communities
According to our binomial test of the feeding records published since 1894 to the present, it is 3.4 times more likely to find dung beetles feeding on sauropsid dung on islands than on the continents. This binomial model is based on the simple assumption that the number of insular records is proportional to the relative size of the islands. However, the binomial model did not take into account other parameters, such as environmental heterogeneity, which may affect species diversity. Our simple assumption was used for two reasons: (i) it is quite robust to the inclusion of more (even realistic) parameters, and (ii) the inclusion of such parameters is currently infeasible due to a lack of relevant ecological data. For example, the continents have greater environmental heterogeneity than islands, which is why they harbor a greater diversity of species (MacArthur and Wilson, 2001; Kier et al., 2009). Consequently, correcting for environmental heterogeneity in our statistical model would only further reinforce the rejection of the null hypothesis (i.e., resulting in a smaller p-value) and provide stronger support for our conclusions. Also, in the binomial test, we treated Australia (45 records for sauropsid dung) as a continent and not as an island. Given its unique biogeographical history and dung beetle community, Australia may be well considered to be a big island. As a consequence, treating it as an island would again provide substantially stronger support for our binomial test that insular dung beetles tend to be associated with sauropsid dung. Even without this treatment, the binomial test still supports our conclusion. Therefore, our simple binomial model is robust to changes in data and model specifications.
There are five island groups (Figure 1A) that harbor authentic scarabaeine communities with endemic genera: Madagascar, Mascarenes (Mauritius, Réunion, Rodrigues), New Caledonia, New Zealand and Caribbean (Davis et al., 2002). Our findings suggest that almost all of them possess a significant proportion of scarabaeines associated with sauropsid dung. With the use of COI amplicon-metagenomics, we were able to identify three lizard and one chameleon species whose dung was consumed by at least five different species of dung beetles in Madagascar (Figure 1 and Supplementary Table 6). In addition, five other scarabaeine species were recorded by us in earlier studies as visitors and potential feeders of sauropsid dung in Madagascar and Mauritius (Supplementary Table 6). The widespread consumption of sauropsid dung has also been documented in New Zealand (Stavert et al., 2014), and hypothesized to have existed in the Caribbean, where only a single record has been found so far (Matthews, 1965; Halffter and Matthews, 1966). A lack of research into the feeding behavior of scarabaeines from New Caledonia precludes any conclusions regarding their diet.
The recent discovery of a large number of Scarabaeinae subfossil remains from the Mascarene archipelago indicates that these islands used to harbor a significant diversity of dung beetles, which deserves special attention (Nick Porch personal communication, see also Rijsdijk et al., 2009). The Mascarene archipelago consists of three main volcanic islands: Mauritius, Réunion, and Rodrigues. The latter is a small island located ~1,500 km east of Madagascar. Extant endemic dung beetles occur only on Mauritius: one species of Nesovinsonia Martínez and Pereira, 1958, and four species of Nesosisyphus. One extinct endemic species from the genus Epactoides was recently found on Réunion (Rossini et al., 2021). This indicates that the scarabaeine diversity on Mascarenes was greater in the past than it is today. Due to the lack of native mammals on these islands, other than bats, it is plausible that such a diversity of dung beetles was established and maintained by the dung of giant tortoises and/or dodos, which were prevalent on the islands prior to human arrival (Rijsdijk et al., 2009; Galetti et al., 2018). We discuss below why we rule out alternate feeding options in this paper.
Most species of dung beetles that have been recorded on islands are generalists since they have been observed to consume other food sources as well (Jones et al., 2012; Ebert et al., 2019; Langton-Myers, 2022). In our literature review, we found only two Nearctic species, Copris gopheri Hubbard, 1894 and Onthophagus polyphemi Hubbard, 1894, that seem to feed exclusively on the dung of the Gopher tortoise (Gopherus polyphemus; Hubbard, 1894; Young and Goff, 1939; Howden and Cartwright, 1963). These facts indicate that dung beetle communities on islands are strongly associated with sauropsid dung, but that they are not solely dependent on it. The authentic insular communities emerged as a result of colonization events from neighboring landmasses. For example, the endemic Madagascan lineages of dung beetles originated from Africa ~ 38–48 Mya (Miraldo et al., 2011; Rossini et al., 2022). The endemic clades found in New Caledonia and New Zealand dispersed from Australia at 50–55 Mya (Gunter et al., 2016). According to this evidence, it is reasonable to assume that the first colonizers evolved the generalist diet, which included feeding on sauropsid dung, as a way to circumvent food shortages caused by the lack of large mammals on the islands (Cupello et al., 2020). Nevertheless, in order to reconstruct ancestral diets and more accurate colonization times, a conclusion of this nature requires further investigation in a phylogenetic framework.
Dung beetles evolved adaptations to many diets other than dung, including carrion, fungi, and detritus (reviewed in Scholtz et al., 2009). Hence, it is important to discuss why we rule out a pivotal role for at least some of those adaptations in maintaining insular communities. For example, islands have large populations of bats that produce large amounts of guano. Do insular scarabaeines feed on guano? In total, we found only nine records (Supplementary Table 6) reporting an association/attraction of dung beetles to bat guano, and they all belong to continental environments. Thus, despite the abundance of bats on islands, there is no evidence that local dung beetles have ever fed on bat dung.
As regards other sources such as plant debris, carrion, and fungi, it is difficult to quantify their role on islands compared to continents based on available data. For example, many investigations report that dung beetles in New Zealand are saprophagous (Ecroyd, 1996; Hodge et al., 2010; Jones et al., 2012). Because of the lack of rigorous data, it is challenging to estimate the proportion and distribution of saprophagy in continental scarabaeines, which precludes any comparative analysis. Therefore, in assessing the different diets, we rely primarily on the present results due to their statistical and empirical support, and these results indicate an increased proportion of sauropsid dung feeders on islands. Yet, we must acknowledge that further research on diet distribution is needed, which may well reveal that sauropsid dung, saprophagy, and carrion-feeding, are all fundamental to the existence of the insular dung beetles.
4.2. Dung beetles and dinosaurs
Currently, the only direct evidence of an association between dung beetles and dinosaurs comes from the coprolite of a Cretaceous dinosaur. The burrows in this coprolite have been attributed to dung beetle activity (Chin and Gill, 1996), but there is no evidence to support such a conclusion (Tarasov et al., 2016). Mesozoic fossils and ichnofossils either identified as Scarabaeinae or ascribed to the activity of dung beetles are still questionable (Tarasov et al., 2016). The unreliability of fossil data led to invoke different scenarios to reconstruct the evolutionary history of Scarabaeinae (e.g., ages based on different rates of molecular divergence; origins by vicariance vs. dispersal; likelihood of association with dinosaur vs. mammal dung in the K-Pg boundary; Mckenna et al., 2015; Gunter et al., 2016; Davis et al., 2017). As a consequence, the origin of scarabaeine dung beetles is still unclear (Tarasov et al., 2016), and timing estimates suggest their emergence between 132 and 40 Mya (Ahrens et al., 2014; Gunter et al., 2016; Davis et al., 2017; McKenna et al., 2019). Considering that these estimates overlap the K-Pg boundary, it is also possible that dinosaur dung could have triggered the evolution of coprophagy in dung beetles if they coexisted.
The evolutionary history of dung beetles in association with Mesozoic dinosaurs and/or mammals has always been debated (Jeannel, 1942; Halffter, 1972; Cambefort, 1991). Davis et al. (2002) used biogeographical patterns of higher vs. lower-level classification of Scarabaeinae (older tribe/genus versus younger species level spatial separations) to demonstrate that older lineages are the byproducts of ancient vicariant events (Gondwana fragmentation), and later dispersal. However, molecular systematic approaches have challenged this view, showing extensive paraphyly across “older” scarabaeine groups, and suggesting more reliable ages of origin, dispersal, and radiation of dung beetles in the Cenozoic, together with the burst of the ancestor of modern mammals (Scholtz et al., 2009). Latter papers included an expansion of an earlier discussion concerning dinosaur physiology (i.e., high or low metabolic rate; combination or separation of urinary and fecal metabolites), rate of dung production as well as the suitability of dinosaur feces to dung beetles in relation to size, consistency, and composition (see Arillo and Ortuño, 2008; Scholtz et al., 2009; Davis et al., 2017).
More recently, Gunter et al. (2016) combined molecular data with fossil calibration in Scarabaeinae, suggesting that dung beetles may have originated in the Mesozoic in association with a highly diversified dinosaur and early mammal fauna. Also, this study estimates that the coprophagy in Scarabaeinae beetles originated in the Upper Cretaceous, a timeline that corresponds to the diverse, large sauropod fauna of that time, as well as the rise of the angiosperms. Gunter et al. (2016) hypothesized that a shift in dinosaur diet that included incidental ingestion of the more nutritious and less fibrous angiosperm foliage, provided a palatable dung source with appropriate particle size and moisture content that allowed for the key innovation of dung feeding. Further justification for the importance of sauropsids to the evolution of dung beetles was based on generalized traits of extant dung beetles, that is, very few extant scarabaeines feed on small, dry dung pellets of insectivorous mammals that would have been ecologically similar to the Upper Cretaceous mammalian fauna (Gunter et al., 2016). However, in the absence of fossils from this time period, such hypotheses remain speculative. Future phylogenetic studies with refined ages and/or new fossil evidence are necessary to disentangle the still doubtful relationship between dinosaurs and dung beetles.
It is important to stress that the earlier hypotheses in favor of an ancient association between dung beetles and mammals were primarily based on the fact that most extant dung beetles do not feed on reptile excrement. The purpose of this paper was to demonstrate the opposite: at least some dung beetle communities do exhibit a certain attraction to the feces of sauropsids. We found that all major clades of extant sauropsids, including squamates, tuataras, turtles, crocodiles, and birds, produce suitable excrement for dung beetles (Figure 1C). Therefore, it is logical to assume that past scarabaeines were physiologically capable of consuming dinosaur dung, just as extant scarabaeines consume that of living sauropsids.
5. Conclusion
Despite being primarily based on observational data, and in the lack of a more comprehensive study of the relative attraction between reptile and mammal dung, this study sheds light on the importance of considering atypical dung beetle communities, such as those from islands, to clarify the evolution of dung beetles. While mammals have undoubtedly played a key role in the diversification of dung beetles, the contribution of sauropsids has been overlooked. The extent of how widespread feeding on reptile dung or bird droppings is in extant dung beetles is still unknown, which can potentially result in a bias in studies conducted with alternative resources of non-mammalian dung-feeding scarabaeines from areas that lack a diverse fauna of mammals. For example, Australian native dung beetles are widely reported as generalists (Matthews, 1974; Gunter et al., 2019), and as such multiple bait types are commonly used for specimen collection. Regardless, this literature review identified reports of sauropsid dung feeding in almost all biogeographic realms where dung beetles occur, with the exception of Indomalayan, including countries with diverse mammal faunas. On the basis of this manuscript, we recommend that future studies on diet of dung beetles also consider sauropsid dung as a resource. For example, the use of non-mammalian dung options in feeding ecology studies, the use of broad vertebrate primers, or database screening beyond Mammalia in molecular gut content analyses will provide more reliable positive and negative association data that will help to disentangle the extent of generalist feeding behavior in dung beetles. As a consequence, we expect that generalist feeding ecology is more widespread than currently reported, being essential to the understanding of the evolutionary history of dung beetles, as well as more precise to predict the impact of vertebrate decline on them.
Data availability statement
The data presented in the study are deposited in the NCBI Sequence Read Archive (SRA) database, accession number PRJNA889602, and OSF, access link https://osf.io/jh3am/.
Author contributions
ST and MR collected the samples. ST and FLop designed the study. Data analyses were performed by FLop with the contribution of ST, MR, and NG. Morphological identification of the dung beetles was performed by MR with the support of GM and FLos. The initial manuscript draft was written by FLop, MR, NG, and ST. All authors edited, contributed to the interpretation of results, and approved the final version of the manuscript.
Funding
This work was supported by the Academy of Finland grant: 331631, and 3-year grant from the University of Helsinki, awarded to ST.
Acknowledgments
We are very thankful to the Malagasy Institut pour la Conservation des Ecosystèmes Tropicaux (MICET) for the support in the acquisition of permits and logistic support during the fieldwork; The Mauritian National Parks and Conservation Service (NPCS) for providing permits to collect in Mauritius; Vincent Florens (University of Mauritius) and Owen Griffiths (La Vanille Nature Park, Mauritius) for the logistic support and continuous help during our 2021 expedition to Mauritius; Philippe Moretto (Toulon, France) for sharing his extensive bibliography on scarab beetles. In addition, we are grateful to Dmitry Zastrozhnov for giving us a hint about the area of the landmasses. We also thank the reviewers for the suggestions that helped to improve the final version of the manuscript. All people in the Tarasov Lab for their general suggestions and discussions. We are also thankful to the staff of the DNA Lab of the Finnish Museum of Natural History for the wet-lab support.
Conflict of interest
The authors declare that the research was conducted in the absence of any commercial or financial relationships that could be construed as a potential conflict of interest.
Publisher's note
All claims expressed in this article are solely those of the authors and do not necessarily represent those of their affiliated organizations, or those of the publisher, the editors and the reviewers. Any product that may be evaluated in this article, or claim that may be made by its manufacturer, is not guaranteed or endorsed by the publisher.
Supplementary material
The Supplementary Material for this article can be found online at: https://www.frontiersin.org/articles/10.3389/fevo.2023.1132729/full#supplementary-material
References
Ahrens, D., Schwarzer, J., and Vogler, A. P. (2014). The evolution of scarab beetles tracks the sequential rise of angiosperms and mammals. Proc. R. Soc. B Biol. Sci. 281:20141470. doi: 10.1098/rspb.2014.1470
Arillo, A., and Ortuño, V. M. (2008). Did dinosaurs have any relation with dung-beetles?(the origin of coprophagy). J. Nat. Hist. 42, 1405–1408. doi: 10.1080/00222930802105130
Bokulich, N. A., Subramanian, S., Faith, J. J., Gevers, D., Gordon, J. I., Knight, R., et al. (2013). Quality-filtering vastly improves diversity estimates from illumina amplicon sequencing. Nat. Methods 10, 57–59. doi: 10.1038/nmeth.2276
Cambefort, Y. (1991). “Dung beetles in tropical savannas,” in Dung Beetle Ecology, eds I. Hanski and Y. Cambefort (Princeton, NJ: Princeton University Press), 156–178. doi: 10.1515/9781400862092.156
Chaisson, M., Pevzner, P., and Tang, H. (2004). Fragment assembly with short reads. Bioinformatics 20, 2067–2074. doi: 10.1093/bioinformatics/bth205
Chin, K., and Gill, B. D. (1996). Dinosaurs, dung beetles, and conifers: participants in a Cretaceous food web. Palaios 280–285. doi: 10.2307/3515235
Cupello, M., Ribeiro-Costa, C. S., and Vaz-de Mello, F. Z. (2020). Systematics of the enigmatic South American Streblopus Van Lansberge, 1874 dung beetles and their transatlantic origin: a case study on the role of dispersal events in the biogeographical history of the Scarabaeinae (Coleoptera: Scarabaeidae). Eur. J. Taxon. doi: 10.5852/ejt.2020.603
Davis, A. L., Scholtz, C. H., and Philips, T. K. (2002). Historical biogeography of Scarabaeine dung beetles. J. Biogeogr. 29, 1217–1256. doi: 10.1046/j.1365-2699.2002.00776.x
Davis, A. L., Scholtz, C. H., and Sole, C. L. (2017). Biogeographical and co-evolutionary origins of scarabaeine dung beetles: mesozoic vicariance versus Cenozoic dispersal and dinosaur versus mammal dung. Biol. J. Linnean Soc. 120, 258–273. doi: 10.1111/bij.12893
deCastro Arrazola, I., Andrew, N. R., Berg, M. P., Curtsdotter, A., Lumaret, J.-P., Menéndez, R., et al. (2022). A trait-based framework for dung beetle functional ecology. J. Anim. Ecol. 92, 44–65. doi: 10.1111/1365-2656.13829
Ebert, K. M., Monteith, G. B., Menéndez, R., and Merritt, D. J. (2019). Bait preferences of Australian dung beetles (Coleoptera: Scarabaeidae) in tropical and subtropical Queensland forests. Austral. Entomol. 58, 772–782. doi: 10.1111/aen.12396
Ecroyd, C. E. (1996). The ecology of Dactylanthus taylorii and threats to its survival. N. Z. J. Ecol. 20, 81–100.
Edgar, R. C. (2013). Uparse: highly accurate OTU sequences from microbial amplicon reads. Nat. Methods 10, 996–998. doi: 10.1038/nmeth.2604
Fincher, G. T., Stewart, T. B., and Davis, R. (1970). Attraction of coprophagous beetles to feces of various animals. J. Parasitol. 56, 378–383. doi: 10.2307/3277680
Galetti, M., Moleón, M., Jordano, P., Pires, M. M., Guimaraes, P. R. Jr, Pape, T., et al. (2018). Ecological and evolutionary legacy of megafauna extinctions. Biol. Rev. 93, 845–862. doi: 10.1111/brv.12374
Geller, J., Meyer, C., Parker, M., and Hawk, H. (2013). Redesign of PCR primers for mitochondrial cytochrome c oxidase subunit I for marine invertebrates and application in all-taxa biotic surveys. Mol. Ecol. Resour. 13, 851–861. doi: 10.1111/1755-0998.12138
Gill, B. D. (1991). “Dung beetles in tropical American forests,” in Dung Beetle Ecology, eds I. Hanski and Y. Cambefort (Princeton, NJ: Princeton University Press), 211–229. doi: 10.1515/9781400862092.211
Gunter, N. L., Lemann, C., and Weir, T. A. (2019). “Scarabaeidae: scarabaeinae latreille, 1802,” in Australian Beetles Vol. 2, Archostemata, Myxophaga, Adephaga, Polyphaga, eds A. Slipinski and J. F. Larence (Clayton, VIC: CSIRO Pubmishing), 443–466.
Gunter, N. L., Weir, T. A., Slipinksi, A., Bocak, L., and Cameron, S. L. (2016). If dung beetles (Scarabaeidae: Scarabaeinae) arose in association with dinosaurs, did they also suffer a mass co-extinction at the K-Pg boundary? PLoS ONE 11:e0153570. doi: 10.1371/journal.pone.0153570
Halffter, G. (1972). “Element anciens de l'entomofaune néotropical: ses implications biogéographiques. Biogéographie et liaisons intercontinentales au cours du Mésozoique,” in 17th International Zoological Congress (Monte Carlo), 1–40.
Halffter, G., and Matthews, E. G. (1966). The Natural History of Dung Beetles of the Subfamily Scarabaeinae (Coleoptera, Scarabaeidae). Mexico: Folia Entomologica Mexicana.
Hanski, I., and Cambefort, Y. (1991). Dung Beetle Ecology, Vol. 1195. Princeton, NJ: Princeton University Press. doi: 10.1515/9781400862092
Hodge, S., Marshall, S. A., Oliver, H., Berry, J., Marris, J., and Andrew, I. (2010). A preliminary survey of the insects collected using mushroom baits in native and exotic New Zealand woodlands. N. Z. Entomol. 33, 43–54. doi: 10.1080/00779962.2010.9722191
Howden, H. F., and Cartwright, O. L. (1963). Scarab Beetles of the Genus Onthophagus Latreille North of Mexico (Coleoptera: Scarabaeidae). Washington, DC: Proceedings of the United States National Museum. doi: 10.5479/si.00963801.114-3467.1
Hubbard, H. G. (1894). The Insect Guests of the Florida Land Tortoise. Washington, DC: US Government Printing Office.
Jeannel, R. (1942). La genèse des Faunes Terrestres. 'Eléments de Biogéographie. Presses Universitaires de France.
Jones, A. G., Forgie, S. A., Scott, D. J., and Beggs, J. R. (2012). Generalist dung attraction response in a New Zealand dung beetle that evolved with an absence of mammalian herbivores. Ecol. Entomol. 37, 124–133. doi: 10.1111/j.1365-2311.2012.01344.x
Kabir, S., Kabir, A., and Majumder, M. (1990). “Relative abundance and species composition of some dung beetles (Coleoptera: Scarabaeinae) in Bangladesh,” in Medical and Veterinary Entomology, 439–443. doi: 10.1111/j.1365-2915.1990.tb00463.x
Katoh, K., and Standley, D. M. (2013). Mafft multiple sequence alignment software version 7: improvements in performance and usability. Mol. Biol. Evol. 30, 772–780. doi: 10.1093/molbev/mst010
Kier, G., Kreft, H., Lee, T. M., Jetz, W., Ibisch, P. L., Nowicki, C., et al. (2009). A global assessment of endemism and species richness across island and mainland regions. Proc. Natl. Acad. Sci. U.S.A. 106, 9322–9327. doi: 10.1073/pnas.0810306106
Kumar, S., Stecher, G., Li, M., Knyaz, C., and Tamura, K. (2018). Mega X: Molecular evolutionary genetics analysis across computing platforms. Mol. Biol. Evol. 35:1547. doi: 10.1093/molbev/msy096
Langton-Myers, S. S. (2022). An endemic dung beetle species feeding on kauri snail carrion in Northland, New Zealand. Austr. Ecol. 47, 127–130. doi: 10.1111/aec.13125
MacArthur, R. H., and Wilson, E. O. (2001). The Theory of Island Biogeography, Vol. 1. Princeton, NJ: Princeton University Press. doi: 10.1515/9781400881376
Matthews, E. (1974). A revision of the scarabaeine dung beetles of Australia. II. Tribe Scarabaeini. Austr. J. Zool. Supplem. Ser. 22, 1–211. doi: 10.1071/AJZS024
Matthews, E. G. (1965). The taxonomy, geographical distribution, and feeding habits of the canthonines of Puerto Rico (Soleoptera: Scarabaeidae). Trans. Am. Entomol. Soc. 91, 431–465. doi: 10.1001/archderm.1965.01600110017006
McKenna, D. D., Shin, S., Ahrens, D., Balke, M., Beza-Beza, C., Clarke, D. J., et al. (2019). The evolution and genomic basis of beetle diversity. Proc. Natl. Acad. Sci. U.S.A. 116, 24729–24737. doi: 10.1073/pnas.1909655116
Mckenna, D. D., Wild, A. L., Kanda, K., Bellamy, C. L., Beutel, R. G., Caterino, M. S., et al. (2015). The beetle tree of life reveals that Coleoptera survived end-Permian mass extinction to diversify during the Cretaceous terrestrial revolution. Syst. Entomol. 40, 835–880. doi: 10.1111/syen.12132
Minh, B. Q., Schmidt, H. A., Chernomor, O., Schrempf, D., Woodhams, M. D., Von Haeseler, A., et al. (2020). IQ-TREE 2: new models and efficient methods for phylogenetic inference in the genomic era. Mol. Biol. Evol. 37, 1530–1534. doi: 10.1093/molbev/msaa015
Miraldo, A., Wirta, H., and Hanski, I. (2011). Origin and diversification of dung beetles in Madagascar. Insects 2, 112–127. doi: 10.3390/insects2020112
Nichols, E., Spector, S., Louzada, J., Larsen, T., Amezquita, S., Favila, M., et al. (2008). Ecological functions and ecosystem services provided by Scarabaeinae dung beetles. Biol. Conserv. 141, 1461–1474. doi: 10.1016/j.biocon.2008.04.011
O'Leary, M. A., Bloch, J. I., Flynn, J. J., Gaudin, T. J., Giallombardo, A., Giannini, N. P., et al. (2013). The placental mammal ancestor and the post-K-Pg radiation of placentals. Science 339, 662–667. doi: 10.1126/science.1229237
Rijsdijk, K. F., Hume, J. P., Bunnik, F., Florens, F. V., Baider, C., Shapiro, B., et al. (2009). Mid-Holocene vertebrate bone concentration-lagerstätte on oceanic island Mauritius provides a window into the ecosystem of the dodo (Raphus cucullatus). Quarter. Sci. Rev. 28, 14–24. doi: 10.1016/j.quascirev.2008.09.018
Rossini, M., Grebennikov, V., Merrien, T., Miraldo, A., Viljanen, H., and Tarasov, S. (2022). Paleogene forest fragmentation and out-of-Africa dispersal explain radiation of the Paleotropical dung beetle tribe Epactoidini trib. nov. (Coleoptera: Scarabaeinae). Syst. Entomol. 47, 655–667. doi: 10.1111/syen.12564
Rossini, M., Vaz-de Mello, F. Z., Montreuil, O., Porch, N., and Tarasov, S. (2021). Extinct before discovered? Epactoides giganteus sp. nov. (Coleoptera, Scarabaeidae, Scarabaeinae), the first native dung beetle to réunion island. ZooKeys 1061:75. doi: 10.3897/zookeys.1061.70130
Rubbmark, R. O., Sint, D., Horngacher, N., and Traugott, M. (2018). A broadly applicable COI primer pair and an efficient single-tube amplicon library preparation protocol for metabarcoding. Ecol. Evol. 8, 12335–12350. doi: 10.1002/ece3.4520
Sayre, R., Noble, S., Hamann, S., Smith, R., Wright, D., Breyer, S., et al. (2019). A new 30 meter resolution global shoreline vector and associated global islands database for the development of standardized ecological coastal units. J. Oper. Oceanogr. 12(Suppl. 2), S47–96S56. doi: 10.1080/1755876X.2018.1529714
Scholtz, C. H., Davis, A. L. V., and Kryger, U. (2009). Evolutionary Biology and Conservation of Dung Beetles. Pensoft Sofia-Moscow.
Stavert, J., Gaskett, A., Scott, D., and Beggs, J. (2014). Dung beetles in an avian-dominated island ecosystem: feeding and trophic ecology. Oecologia 176, 259–271. doi: 10.1007/s00442-014-3001-z
Tarasov, S., Vaz-de Mello, F. Z., Krell, F.-T., and Dimitrov, D. (2016). A review and phylogeny of Scarabaeine dung beetle fossils (Coleoptera: Scarabaeidae: Scarabaeinae), with the description of two Canthochilum species from Dominican amber. PeerJ 4:e1988. doi: 10.7717/peerj.1988
Vinson, J. (1951). “Le cas des Sisyphes Mauriciens (Insectes, Coléoptères),” in Proceedings of the Royal Society of Arts and Science of Mauritius, Vol. 1 (Royal Society of Arts and Sciences of Mauritius), 105–122.
Keywords: Madagascar, Mascarenes, metabarcoding, sauropsids, excrement, insects
Citation: Lopes F, Rossini M, Losacco F, Montanaro G, Gunter N and Tarasov S (2023) Metagenomics reveals that dung beetles (Coleoptera: Scarabaeinae) broadly feed on reptile dung. Did they also feed on that of dinosaurs? Front. Ecol. Evol. 11:1132729. doi: 10.3389/fevo.2023.1132729
Received: 27 December 2022; Accepted: 15 February 2023;
Published: 10 March 2023.
Edited by:
Pedro Giovâni Da Silva, Federal University of Minas Gerais, BrazilReviewed by:
Liang Lü, Hebei Normal University, ChinaAdrian Davis, University of Pretoria, South Africa
Clarke Scholtz, University of Pretoria, South Africa
Copyright © 2023 Lopes, Rossini, Losacco, Montanaro, Gunter and Tarasov. This is an open-access article distributed under the terms of the Creative Commons Attribution License (CC BY). The use, distribution or reproduction in other forums is permitted, provided the original author(s) and the copyright owner(s) are credited and that the original publication in this journal is cited, in accordance with accepted academic practice. No use, distribution or reproduction is permitted which does not comply with these terms.
*Correspondence: Fernando Lopes, ZmVybmFuZG8udmllaXJhbG9wZXNAaGVsc2lua2kuZmk=