- 1Gembloux Agro-Bio Tech, University of Liège, Gembloux, Belgium
- 2Natagora, Traverse des Muses, Namur, Belgium
- 3Natagriwal ASBL, Gembloux, Belgium
- 4Avignon Univ, Aix Marseille Univ, CNRS, IRD, Avignon, France
The restoration of hay meadows is one of the major challenges in temperate Europe, to overcome the drastic loss and degradation of these semi-natural habitats. Evaluation of restoration success requires the definition of a relevant reference ecosystem. Historical phytosociological relevés dating from the 1960s–1970s, before large scale agriculture intensification, may be used as the reference framework for such purposes. However, this reference framework may no longer be pertinent in our present-day ecological and socio-economic context. In this study, we assessed the success of three restoration techniques for lowland hay meadows, based on plant species richness and composition and functional traits, by comparing the restoration trajectories to historical and current references. The three restoration techniques, passive restoration mowing, active fresh hay transfer and active sowing of threshing material, were implemented depending on the initial level of degradation and the proximity to well-preserved meadows. The species composition of restored meadows clearly differed from historical references but converged toward current references, for both passive and active restoration techniques. Historical references are characterized by short, stress-tolerant dicotyledonous plant species with small specific leaf area, indicators of nutrient-poor environments. The gap observed between plant communities of historical and current meadows suggests that the historical data are an over-ambitious target for restoration purposes and can hardly be considered relevant to our present-day context. Therefore, creation of a new reference framework of lowland hay meadows is needed for the adequate implementation of restoration projects. Successful outcomes of active and passive techniques must encourage practitioners to adapt the choice of the restoration method depending on the local ecological context, i.e., level of degradation and proximity to well-preserved habitats.
1. Introduction
Temperate meadows are among the habitats that host the most diverse fauna and flora in temperate Western Europe (Veen et al., 2009; Bruchmann and Hobohm, 2010; Wilson et al., 2012; Habel et al., 2013). The high conservation value of these habitats is the result of centuries, even millennia, of extensive traditional management allowing the coexistence of many species in these open environments (Isselstein et al., 2005). Since the second half of the twentieth century, changes in agricultural practices were encouraged by the implementation of the European Common Agricultural Policy (CAP). Land use changes and intensification of agricultural systems have led to the decline and degradation of species-rich meadows (Stoate et al., 2009; Veen et al., 2009), and therefore to a significant loss of animal and plant biodiversity in rural landscapes (Zechmeister et al., 2003; Marini et al., 2008; Manning et al., 2015; Emmerson et al., 2016). Specifically, lowland hay meadows are assessed as being in a poor conservation state over almost all of their range in the EU, which extends from northern Portugal to southern Sweden with a high occurrence in Belgium and Germany (European Environment Agency, 2019, 2020). Hence, ecological restoration of hay meadows is a major challenge in temperate Europe.
The definition of a clear and relevant reference ecological system is a central question for the evaluation of restoration success (Holl and Cairns, 2002; Waldén and Lindborg, 2016; Prach et al., 2019). Reference information is usually either existing intact sites or historical data (White and Walker, 1997). In Europe, a large dataset of phytosociological relevés have been collected historically (1960s–70s). Those relevés, providing information on plant communities before the major effect of agriculture intensification, are often used as references for evaluating the conservation status and restoration success of ecosystems. In southern Belgium, they have been used to build the regional version of EUNIS habitat classification (Davies et al., 2004) and are considered to be the reference framework for evaluating the outcome of EU-funded restoration programs targeting ecosystem restoration (Dufrêne and Delescaille, 2005). However, this historical reference may no longer be achievable in our present-day ecological and socio-economic context. Rapid global environmental changes, mostly climate change and global eutrophication (Matson et al., 2002), may impose the reconsideration of the use of historical data as a reference for ecological restoration (Jackson and Hobbs, 2009; Higgs and Jackson, 2017). When choosing the reference data for the restoration of agriculture-dependent ecosystems, the evolution of agro-ecosystem management driven by socio-economic factors must also be taken into consideration as they greatly influence farmers’ practices (McGinlay et al., 2017). In this context, former reference communities imposing very specific species assemblages may be an over-ambitious target. Despite this question of the use of historical references for evaluating the restoration of agro-ecosystems, only a small number of studies compared historical and contemporary references for evaluating large-scale, ecological restoration projects (Barak et al., 2015).
A variety of restoration techniques, including active and passive methods, are commonly used to overcome abiotic, biotic and dispersal constraints in meadow restoration projects (Sengl et al., 2017). Hay meadows have been historically managed by mowing with low fertilization. Adaptation of key management practices (fertilization, mowing regime) is seen as a passive way to restore meadows (Jones et al., 2018). Passive restoration is recommended when the habitat is slightly degraded, has maintained a great potential for natural self-repairing and/or is integrated into a propitious landscape context, i.e., when surrounded by less impaired and traditionally managed meadows in which target species remain common (Jones et al., 2018; Prach et al., 2020). In more degraded landscape contexts and more strongly altered ecosystems, modification of management alone is insufficient for restoration. Lack of dispersal of the target species in time or space, i.e., the dispersal constraint is often identified as a major barrier (Donath et al., 2003; Hölzel et al., 2012; Pywell et al., 2012; Kiss et al., 2016). In such contexts, active restoration with assisted species dispersal combined with appropriate management practices is needed.
Plant assemblages are traditionally studied at the species taxonomic level, which allows for direct reference to a target species assemblage. Using predictions based on the taxonomic level alone may not allow the detection of functional differences, and the results obtained from a list of species in a given context cannot be extended to all contexts. Trait-based approaches are complementary to help to identify functional patterns and ecological functions independently of the studied pool of species (Lavorel and Garnier, 2002; Kahmen and Poschlod, 2004). Functional traits are useful tools to understand responses to environmental changes, such as changes in nutrient availability and disturbances (Lavorel and Garnier, 2002). As these abiotic conditions are expected to change during meadow restoration and between historical and current communities, we can also expect to see changes in functional composition (Fagan et al., 2008).
In this study, we aimed to assess the success of passive (mowing regime) and active (transfer of fresh seed-containing hay and sowing of threshing material) restoration of lowland hay meadows by confronting the restoration trajectories to historical and current references. We use an in-situ large scale experiment situation provided by the LIFE project in southern Belgium. We specifically addressed the following questions:
(1) Does the restoration of degraded meadows correspond to the recovery of the species and functional composition of historical and/or current references?
(2) Do passive and active restoration efforts, that were implemented depending on the initial level of meadow degradation, lead to equivalent restoration success?
2. Materials and methods
2.1. The restoration project
In southern Belgium, the ecological situation of lowland hay meadows is particularly unfavorable (Wibail et al., 2014; DEMNA, 2019). Several EU-funded LIFE projects have been launched to restore hundreds of hectares of lowland hay meadows using a variety of empirical techniques and offering great in situ experiments to evaluate restoration success. This study focused on the LIFE project “Prairies bocagères” (LIFE11 NAT/BE/001059; Goret et al., 2020) in which the restoration of approximately 200 hectares of lowland hay meadows was carried out in the Fagne-Famenne bioclimatic region, which is characterized by Upper Devonian shale geology and loam-rocky to clay soils. The mean annual temperature is 9.5°C and the mean annual precipitation is 976 mm (Van der Perre et al., 2015). Restoration actions were implemented in seven nature reserves with meadows presenting different levels of degradation (Figure 1). A large number of degraded meadows were included in the restoration process each year from 2013 to 2018. This chronosequence of restoration is of significant value as it allows us to analyse changes in community composition over time (Engst et al., 2017; Sullivan et al., 2020).
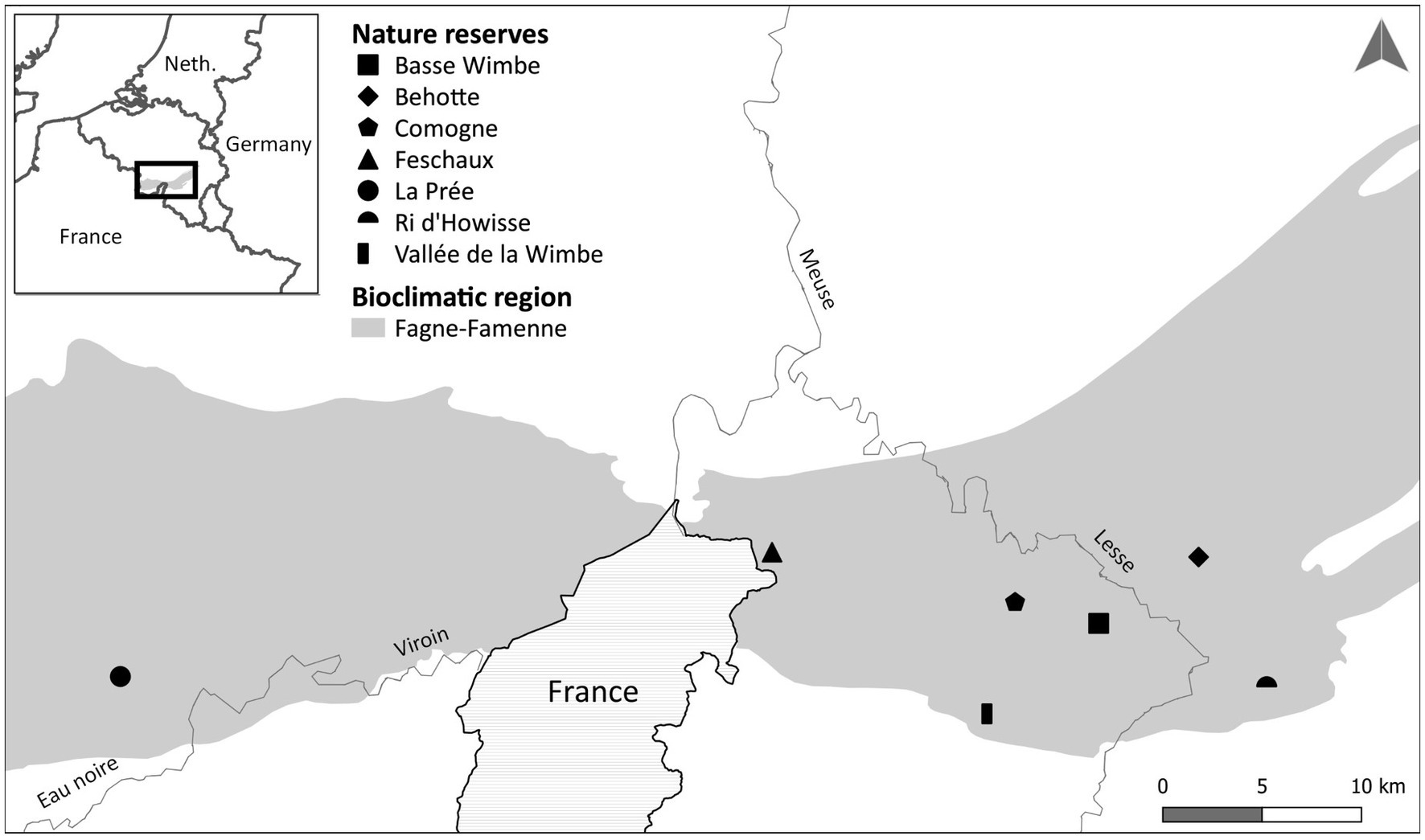
Figure 1. Location of the Fagne-Famenne region and the seven locations where meadow restoration occurred in the vicinity of reference nature reserves.
The habitat targeted by restoration is the Natura 2000 habitat 6,510 protected by the EU Habitats Directive 92/43/EEC (European Community, 1992 – Annex I “Lowland hay meadows”; code EUNIS E2.22; phytosociology Arrhenatherion elatioris Koch 1926). Arrhenatherion hay meadows are permanent grasslands found on mesic soils at low altitudes (<300 m in Belgium). They are first mowed in early summer (end of June to early July). Depending on the meadow productivity and the yearly weather conditions, a second mowing or low-intensity grazing can occur at the end of summer. No or low fertilization is applied. When in good conservation status, they are characterized by oligo- to mesotrophic plant species (see lists 1 and 2, Supplementary Table S1).
The LIFE project implemented three restoration techniques: passive restoration mowing, active restoration with either transfer of hay containing fresh seed or direct sowing of threshing material. The restoration technique was adapted depending on the initial degradation level and the direct vicinity of the meadow following the decision-making tool of Goret et al. (2021). The techniques used depended on the initial conservation status (CS) of the meadow to be restored (Supplementary Table S2). CS was determined using indicators of plant community integrity following the evaluation grid of the Department for Nature and Agriculture Study of the Regional Administration (Supplementary Table S1; A: very good CS; B: good to medium CS; C: degraded CS; D: not a lowland hay meadow).
A total of 42 restored parcels were selected for this study. If the CS corresponded to recent degradation (B or C for less than 5 years) or a degraded meadow (C) with an adjacent seed source (meadow with very good CS-A), the strategy was to use mowing (passive restoration), cutting and exporting biomass twice a year: once after June 15 and once after September 15. The first mowing was delayed by about a month compared to the regime applied before the restoration (May 15). During the first years of restoration, the first mowing cannot be too late, even though this is recommended to allow all species to complete their life cycle, because abiotic conditions first need to be adequate to favor forb germination and development. Mowing in mid-June helps to maximize nutrient export and to create sites where reduced competition for light allows forb seedlings to establish. Fertilization was proscribed.
If the CS was C (degraded) for more than 5 years with no adjacent grassland in CS-A or if CS was D, the restoration strategy included: stopping fertilization, soil preparation for seedling establishment (creation of bare soil strips by two harrow operations or by milling to a depth of 10 cm), assisted seed dispersal by fresh hay transfer or sowing of threshing material, and a post-restoration mowing regime similar to passive restoration. For both assisted seed dispersal methods, plant material was collected directly from a donor site in CS-A, located as close as possible (less than 10 km). Threshing material and fresh hay were always collected at the end of July, the year of restoration, in order to maximize the amount of characteristic species that had reached seed-maturity. Threshing material was collected using a small combine harvester adjusted to limit the amount of plant residues garnered. The harvest product was dried for about 48 h and then roughly sorted using an alveolar pre-sorter in order to not lose seed diversity. Through this process, less than 10% of plant debris remained in the seed mixture. In September, 25 kg per hectare was sown with a mechanical seeder in bare soil strips. For fresh hay transfer, the hay was spread on the degraded site on the same day that the donor site was mowed.
2.2. Vegetation sampling
A space-for-time substitution approach was used to characterize the restoration trajectory based on plant communities. In 2019, we chose meadows that have been restored for one to 6 years (restoration age) with an equal repartition among three age classes since restoration: 1–2 years, 3–4 years and 5–6 years (Table 1). Altogether, the restored meadows covered a total of 58.6 ha.
A complete botanical survey of each meadow was undertaken before the restoration (initial state) and once at the end of the project in 2019 (restored state). These surveys were conducted from mid-May to the end of June and were carried out by walking the entire meadow, avoiding edges. The cover of all herbaceous species encountered at the parcel scale was visually estimated using the Braun-Blanquet scale (r = one individual, + = <1%, 1 = 1–5%, 2 = 5–25%, 3 = 25–50%, 4 = 50–75%, 5 = >75%). Braun-Blanquet scale was chosen to use the same method and precision of abundance estimation as historical references. The list was considered exhaustive if no other species was found after a walk of approximately 10 min in the meadow. The nomenclature used in this study follows the flora of Lambinon and Verloove (2015).
2.3. Reference data
Community recovery trajectories were evaluated against two types of reference data representing Arrhenatherion meadows in favorable CS: (1) six meadows in nature reserves of Fagne-Famenne region that were meadows in the best conservation status in the region (as defined in Supplementary Table S1; hereafter current references). Floristic composition of the six current references was surveyed following the same protocol as described above for restored parcels and (2) 36 historical surveys recorded from 1955 to 1963, and one recorded in 1996, in the Fagne-Famenne region (hereafter historical references). Historical surveys were taken from the data used for the phytosociological description of the EUNIS typology in southern Belgium which served to define the very good conservation state of meadows (CS-A). For the historical references, one relevé per meadow was realized with sampling area varying from 50 to 200 m2.
2.4. Functional traits
Seven functional traits were retained to characterize the functional differences between reference, restored and degraded plant communities. Each trait was selected because it is expected to change with restoration according to predetermined hypotheses (Table 2).
Information on leaf attributes (leaf dry matter content LDMC and specific leaf area SLA) and canopy height of species was extracted from the LEDA database (Kleyer et al., 2008). For traits where several values per species were given, the average of the values from countries close to Belgium (Germany, Netherlands, United Kingdom, France) was calculated. If no height data was provided for a species, the maximum height given in the flora of Lambinon and Verloove (2015) was used. Raunkiaer’s biological types were taken from Lambinon and Verloove (2015). When two biological types were mentioned, the first cited was retained. Life forms (grass, legume and other dicotyledonous plant) were also included in the analysis. The N-Ellenberg’s index classifying species according to their nutrient requirements was taken from the Baseflor database (Julve, 1998). Grime’s CSR values of each survey were calculated using the electronic spreadsheet-based tool of Hunt et al. (2004) which relies on the CSR types of 1,000 European species identified by Hodgson et al. (1999). The calculation of CSR positions in this spreadsheet is based on the relative abundance of species in the survey. If a species was not present in the spreadsheet, the CSR type in the BiolFlor database (Kühn and Klotz, 2002) was used.
For the analysis of changes in plant community functional traits between the historical reference and the current system (current reference and restored meadows), we proceeded to the following calculations. (1) Nominal traits (Raunkiaer’s biological types and life forms) were transformed to as many binary traits as there were values for the trait. We then computed trait relative abundance as the proportion of total cover occupied by species sharing the trait. Only therophyte and hemicryptophyte were retained as binary traits for Raunkiaer’s biological types as other types were too sparse in this habitat and (2) For numeric attributes (LDMC, SLA, canopy height, Ellenberg index), we calculated the community weighted mean (CWM; Violle et al., 2007; Lavorel et al., 2008) taking species cover as weight. Cover values were derived from the van der Maarel coefficients using the median cover (Supplementary Table S3). CWM of LDMC was only computed for grass species (hereafter LDMCgrass) in order to avoid effects due to changes in the proportion of life forms; the different life forms have contrasting ranges of LDMC (Cruz et al., 2002; Al Haj Khaled et al., 2005). Before any further calculations, the square root transformation was applied to variables when necessary in order to improve normality. This was done for the relative abundances of legumes, hemicryptophytes and therophytes, and S and R values.
2.5. Statistical analysis
Prior to data analysis, the Braun-Blanquet scale was transformed into van der Maarel (1979; Supplementary Table S3). All analyses were conducted using R statistical software (R Core Team, 2021).
To determine whether the restoration of degraded meadows corresponds to the recovery of the species composition of historical and/or current references, we used a principal coordinate analysis (PCoA) based on Bray-Curtis dissimilarity (Legendre and Legendre, 2012). In order to visualize the global trajectory and to explore the variation in species composition between historical and current references, the PCoA was first run using the entire dataset (historical references included). Because we observed differences between historical references and other situations, we used the multipatt() function of the “indicspecies” package with the IndVal index to identify species characteristics of the historical reference, current references and restored meadows (func = “IndVal.g”; De Cáceres et al., 2010; De Cáceres, 2020). This function was used because it allows the identification of indicator species of combinations of groups.
Because historical references were found to be different from current references in their species and functional composition, we focused the analysis of restoration trajectories on current references. A PCoA was performed on a restricted dataset excluding the historical references but with current references, in order to better examine variation in species composition between the initial and final states of restored parcels. The latter allowed the restoration trajectory of each meadow and technique-dependent trajectories to be visualized. PCoA were performed using the “vegan” package (Oksanen et al., 2020) and the cmdscale() command in the “stats” package.
Species richness of each survey was calculated as the number of observed species. In order to evaluate whether active and passive restoration led to changes in species composition, similarity with current references was calculated for all initial states and restored states at different periods. We first calculated the average current reference by computing the mean of species abundance across the six current references, and then we computed the Bray-Curtis distance between all restored sites and this average reference. Similarity was calculated as 1 Bray-Curtis distance. To determine whether species richness and similarity significantly increase with the age of restoration, linear regressions were computed separately for the three restoration techniques. To test for significant differences in slopes and intercepts, analysis of covariance (ANCOVA) was performed using the anova_test() command in the “rstatix” package (Kassambara, 2021) with species richness or similarity as the dependent variable, restoration technique as the grouping variable and restoration age as the covariate. Post-hoc tests were carried out using the emmeans_test() command of the “rstatix” package with a Bonferroni correction for multiple comparisons. To examine whether species richness of 5–6 year old restored parcels was similar to that of current references, analysis of variance (ANOVA) followed by a Dunnett test was performed. The glht() function of the “multcomp” package (Hothorn et al., 2021) was used for the Dunnett test. To evaluate whether the species composition of restored parcels was similar to that of current references, we compared similarities between restorations and current references with similarity found within current targets. Plots were visualized using the “ggplot2” package (Wickham et al., 2020).
3. Results
3.1. Restoration success against historical targets
The first two dimensions of the PCoA performed on the entire dataset (historical references included) explained 18.22% of the existing floristic variation (12.92 and 5.30%, respectively for the first two PCoA axes; Figure 2). The third dimension explained 4.69% of the variation but did not show any meaningful pattern. The species composition of the historical references (HR) clearly differed from all other floristic surveys (the six current references (CR), the initial state of restored meadows, the final states of restored meadows whatever the age of restoration), with the historical references exclusively positioned on the negative values of the first axis and others mainly situated on the positive values. The ordination on the second axis revealed a global trajectory where the plant communities of restored meadows become more distant from their initial degraded states over time. Correlations between plant attributes and PCoA scores showed that plant communities of historical references were more stress-tolerant (S) and had a higher proportion of dicotyledonous and legume species compared to current reference and restored parcels (Figure 2). In contrast, current plant communities (reference and restoration parcels) had a higher proportion of grasses and had a more competitive (C) and eutrophic (Ellenberg N) character. They were also characterized by a higher SLA and height. Plant communities of initial degraded states in restored meadows presented a higher ruderal strategy (R) and were more tolerant of frequent disturbances, showing the effect of the intensive mowing regime on degraded meadows. Initial states of restored meadows had a higher proportion of hemicryptophytes compared to final states of restoration and current references. Restored plant communities had a higher proportion of therophytes, dicotyledonous and legume species and a higher LDMCgrass.
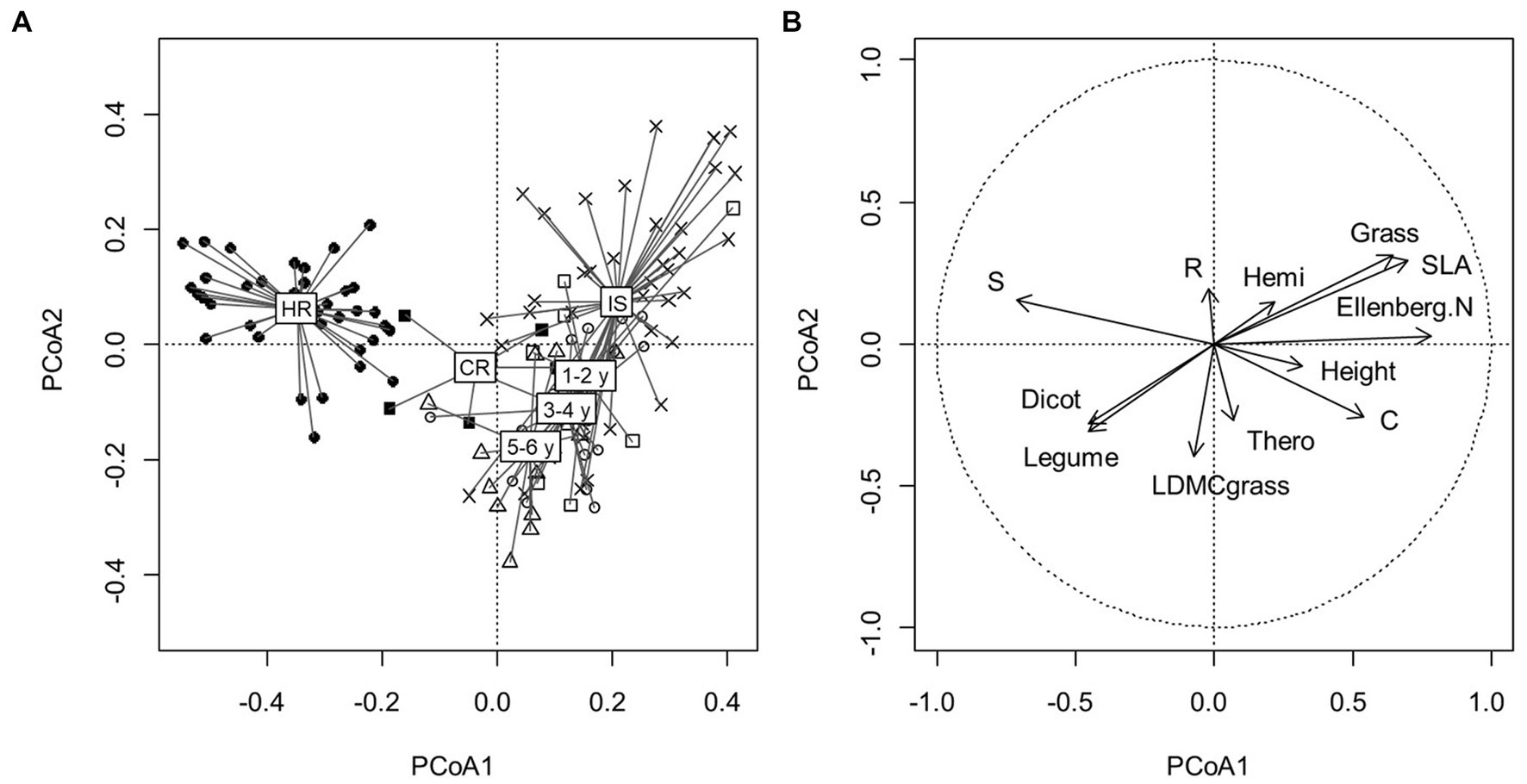
Figure 2. (A) Principal coordinate analysis (PCoA) performed on the 127 floristic surveys: × IS: initial states of restored parcels (n = 42), □ final states for 1–2-year-old restoration (n = 9), ○ final states for 3–4-year-old restoration (n = 18), △ final states for 5–6-year-old restoration (n = 15), ■ CR: current references (n = 6), ● HR: historical references (n = 37). The first two axes explained 18.22% of the floristic variation (PCoA1: 12.92%, PCoA2: 5.30%). (B) Correlation circle showing relations between PCoA scores and community plant functional traits.
We found seven significant exclusive indicator species of historical references that were not found in current references nor in restored meadows, and we identified eight other significant exclusive indicator species of historical references that were found at low frequency in current references and in restored meadows (Figure 3). Moreover, four species from the list of rare, threatened and protected spermatophyte species of the Walloon Region (Saintenoy-Simon et al., 2006) were only found in a very small number of historical references (Alchemilla filicaulis, Alchemilla glaucescens, Festuca ovina, Ophioglossum vulgatum). Inversely, nine significant exclusive indicators of current references were never found in historical references (e.g., Myosotis arvensis, Elymus repens). The complete list of all recorded species is provided in the Supplementary Table S4 as well as the value of p associated with their indicator status and their frequency in each group.
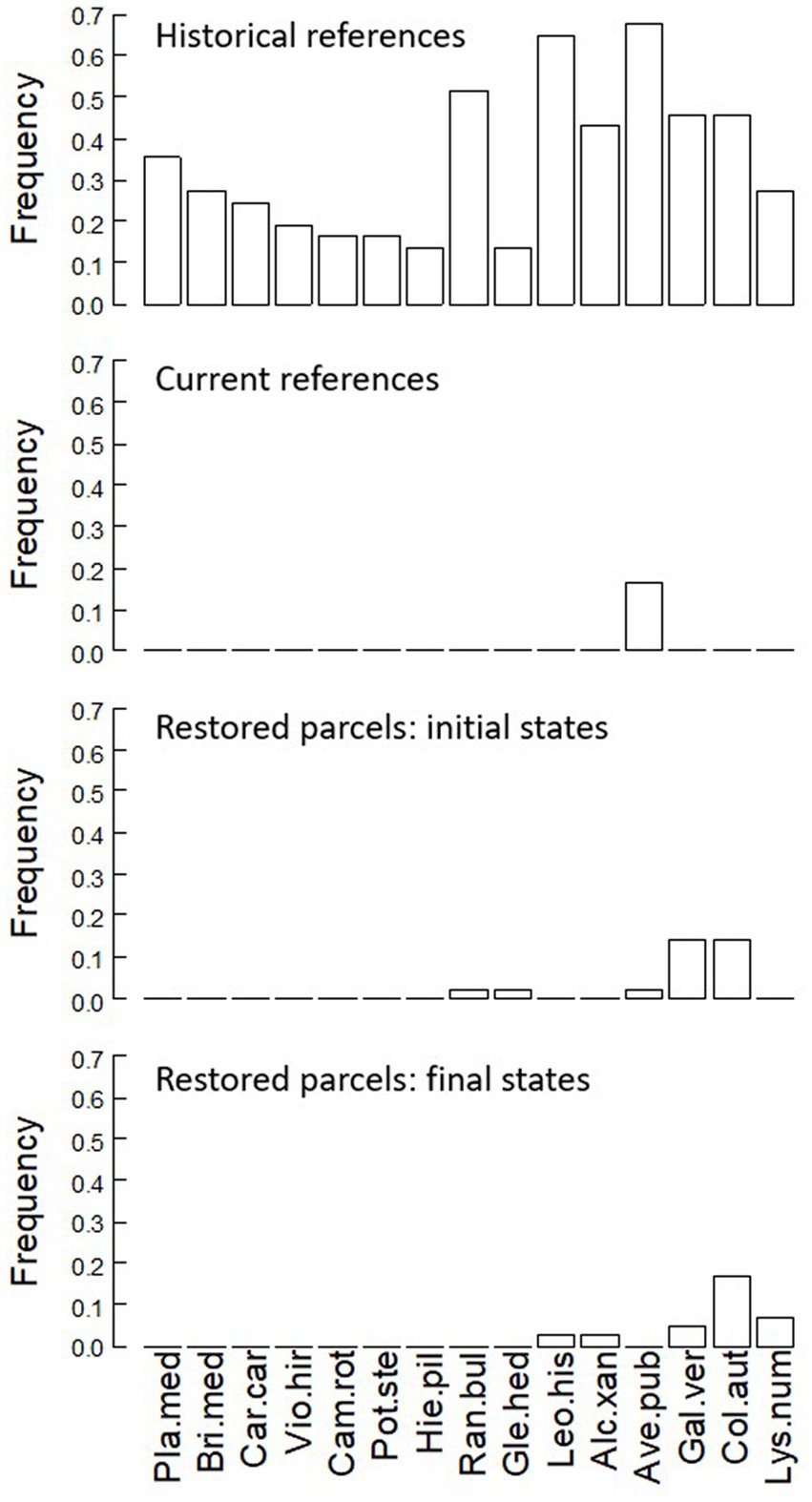
Figure 3. Frequency of significant exclusive indicator species (identified by the multipatt function) observed in historical references, current references, initial and final states of restored meadows. Species abbreviations: Pla.med, Plantago media; Bri.med, Briza media; Car.car, Carex caryophyllea; Vio.hir, Viola hirta; Pot.ste, Potentilla sterilis; Hie.pil, Hieracium pilosella; Ran.bul, Ranunculus bulbosus; Gle.hed, Glechoma hederacea; Leo.his, Leotondon hispidus; Alc.xan, Alchemilla xanthochlora; Ave.pub, Avenula pubescens; Gal.ver, Galium verum; Col.aut, Colchicum autumnale; Lys.num, Lysimachia nummularia.
3.2. Recovery of species richness and species composition against current references
Species richness significantly increased with time for the three restoration techniques (mowing: F = 13.94, p < 0.001, threshing material sowing: F = 10.49, p = 0.003, and hay transfer: F = 19.1, p < 0.001; Figure 4). The slopes of the three regression lines were not significantly different according to the ANCOVA (p = 0.972) and each restoration trajectory gained on average 2.4 species per year. However, intercepts were significantly different (p = 0.003), in line with the fact that initial states differed among the meadows restored with the different techniques. Post-hoc tests showed that initial species richness of parcels restored with fresh hay transfer was significantly lower than that of parcels restored only by mowing (p-ajd = 0.002) but was not significantly different from that of parcels restored by threshing material sowing (p-adj = 0.190). The initial species richness was not significantly different for the latter two (p-ajd = 0.207). ANOVA and Dunnett tests revealed a significantly lower final species richness of parcels restored with fresh hay transfer (5–6 years after restoration) compared to species richness of current references. Other restoration methods led to similar species richness to current references (46.7 species) with for mowing: 45.5 species, t = −0.264, p = 0.988, threshing material sowing: 36.4 species, t = −2.479, p = 0.062, and fresh hay transfer: 33.5 species, t = −3.335, p = 0.011.
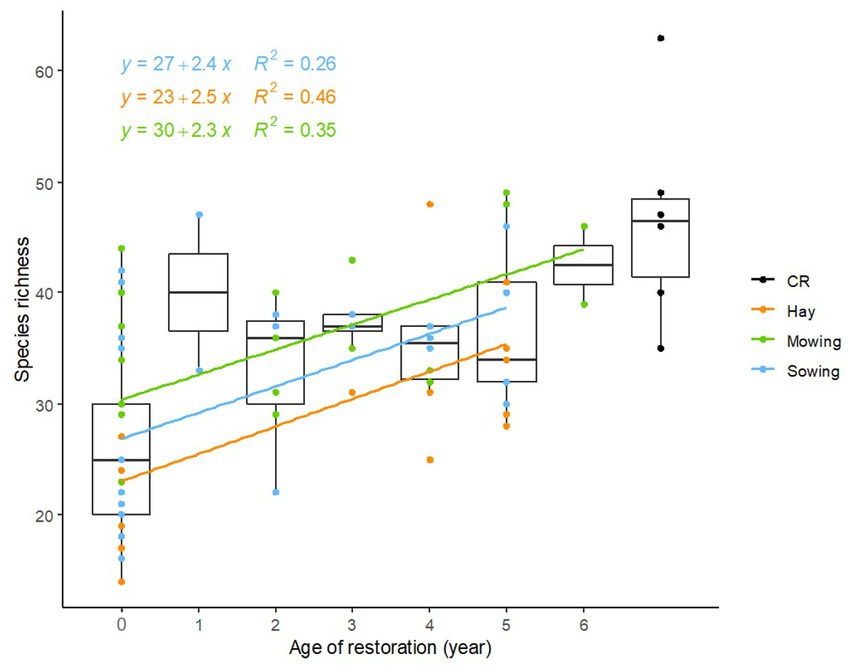
Figure 4. Box-plots of species richness variation for initial state of restored meadows (year 0), species richness of restored meadows at different restoration ages and current references (CR). Lines represent linear regression models between restored meadow species richness and time since restoration began for the three restoration methods. Hay: fresh hay transfer, Sowing: sowing of threshing material, Mowing: passive restoration by mowing regime adaptation (Mowing: F = 13.94, p < 0.001, Sowing: F = 10.49, p = 0.003, Hay: F = 19.1, p < 0.001).
Similarity of restored plant communities to the average species composition of current references significantly increased with time from restoration for the three restoration techniques (mowing: F = 12.18, p = 0.002, threshing material sowing: F = 25.86, p < 0.001, and hay transfer: F = 10.17, p = 0.004; Figure 5). The slopes and intercepts of the three regression lines were not significantly different from one another (slopes: p = 0.605, intercepts: p = 0.803). Each restoration trajectory gained on average 2.37% similarity to current references per year. Similarity between final states of restored plant communities and current references was close to the mean similarity observed among current references (mean similarity among current references: 0.52, mean similarity between final states and current references: 0.51 for mowing, 0.50 for fresh hay transfer, 0.52 for sowing; Figure 5). This result indicates restoration success since the recovery of species composition attained the level of similarity found within references.
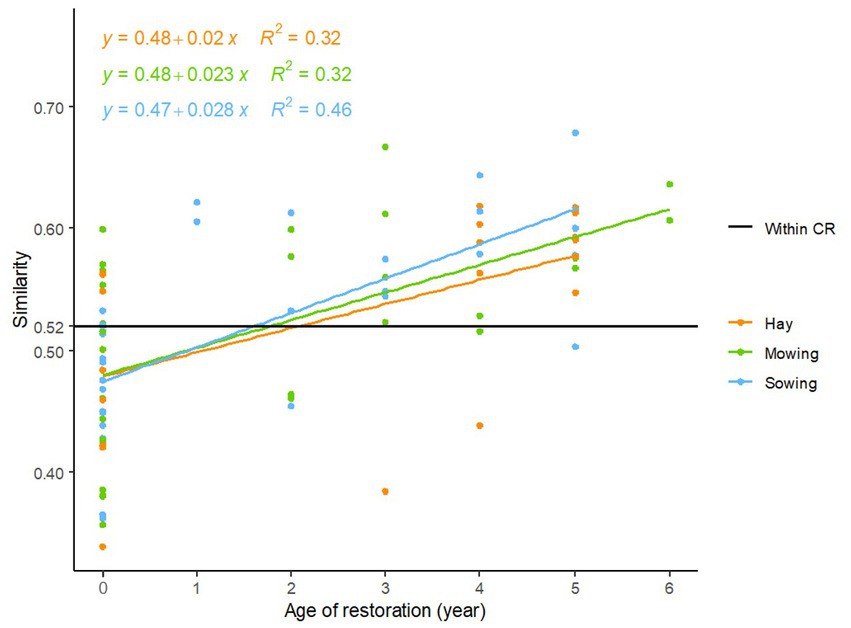
Figure 5. Linear regression models showing significant relationships between species composition similarity (1 Bray-Curtis distance) with the average species composition of current references (CR) and time since restoration began for the three restoration methods. Hay: fresh hay transfer, Sowing: sowing of threshing material, Mowing: passive restoration by mowing regime adaptation (Mowing: F = 12.18, p = 0.002, Sowing: F = 25.86, p < 0.001, Hay: F = 10.17, p = 0.004). Within CR = average similarity within current references. Slopes and intercepts of the three regression lines are not significantly different (slopes: p = 0.605, intercepts: p = 0.803).
3.3. Restoration trajectories for restoration methods
The first two axes of the ordination performed on the restricted dataset excluding the historical references explained 16.55% of the floristic variation (PCoA1: 9.93%, PCoA2: 6.62%; Figure 6). In order to visualize the parcels’ individual trajectories, each initial state was linked to its final state. Most of the individual trajectories converged towards current references, even when species composition was initially far from that of current references. In some cases, final states reached current references (Figure 6A). The initial states of the restored meadows covered a wide range of floristic variation, which is in accordance with the different initial conservation status for meadows restored with passive (mowing) and active (hay transfer and sowing) restoration techniques. However, most of them differed from the other surveys in their position on the positive values of the first axis (Figure 6A).
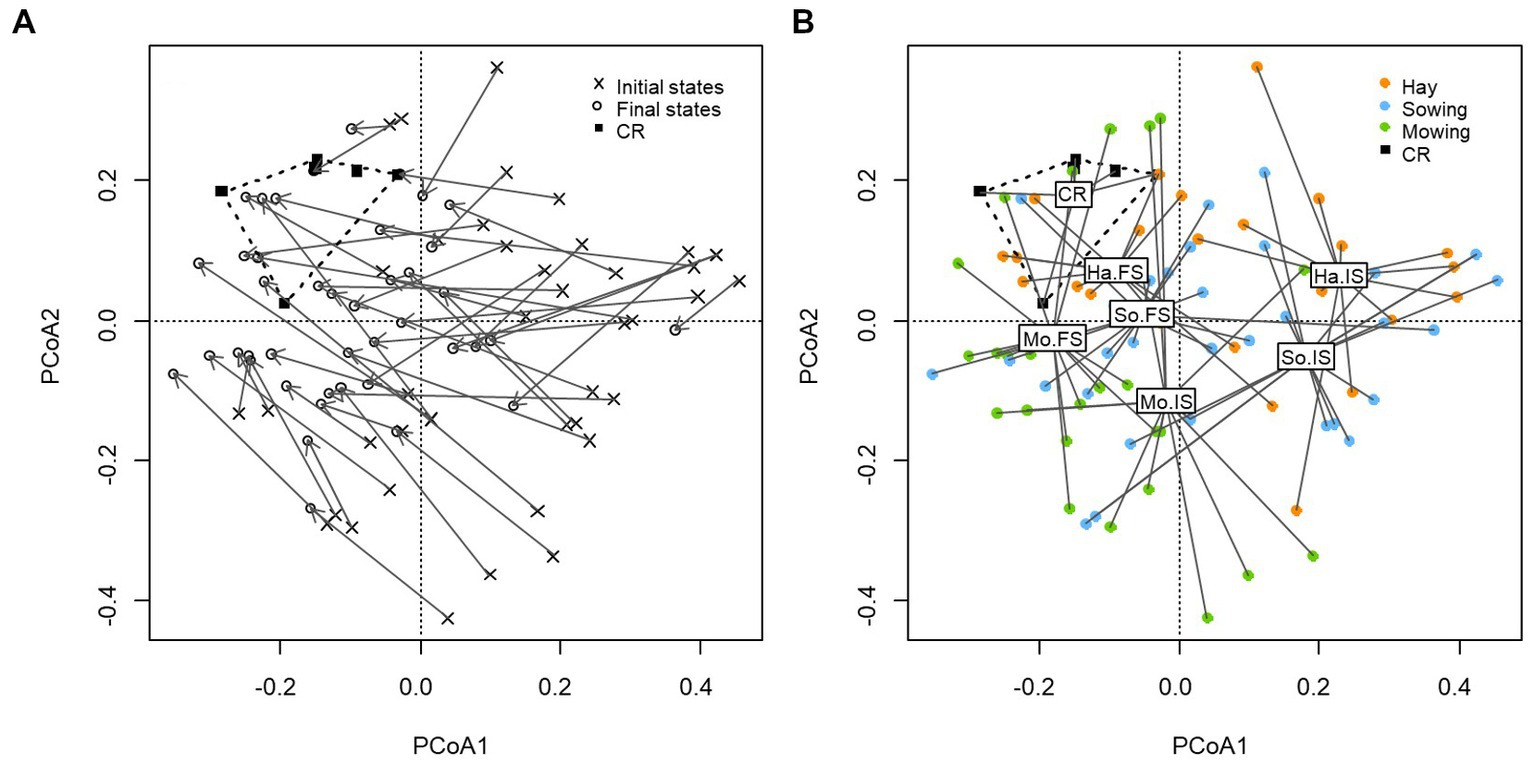
Figure 6. Principal coordinate analysis (PCoA) performed on species composition for restored meadows and current references. The first two axes explained 16.55% of the floristic variation (PCoA1: 9.93%, PCoA2: 6.62%). (A) Arrows link the initial state and final state of each restored meadow to show individual ecological trajectories. Initial states (n = 42), final states (n = 42), current target references (n = 6). (B) PCoA with dissociation of the initial and final states of the three restoration techniques to display technique-dependent ecological trajectories. Mo.IS: initial states of mowing (n = 14), Mo.FS: final states of mowing (n = 14), Ha. IS: initial state of fresh hay transfer (n = 12), Ha.FS: final state of fresh hay transfer (n = 12), So.IS: initial state of sowing (n = 16), So.FS: final state of sowing (n = 16), current reference targets (n = 6).
In order to examine technique-dependent ecological trajectories, this PCoA was then presented with a dissociation of the initial and final states of the three restoration techniques (Figure 6B). This representation allowed clear differences to be observed between the initial states (IS) of communities restored by the different restoration techniques. Initial states of meadows restored by transfer of fresh seed-containing hay (Ha) and by threshing material sowing (So) were clearly more distant from current references than those of meadows restored by restoration mowing (Mo). This representation also showed that, regardless of the restoration technique, the final states (FS) of plant communities converged in the direction of current references.
4. Discussion
Our results highlight a strong floristic difference between historical references, based on historical phytosociological data (1960s–70s) of lowland meadows, and current references based on existing meadows with the best conservation status in the studied region, toward which the final states of restoration converged. Historical meadows differed from current references and restored meadows by being mostly short, stress-tolerant dicotyledonous plant communities with small SLA. Such a functional composition indicates a prevailing resource conservation strategy in historical references compared to restored meadows and current references. This was confirmed by the detection of indicator species of historical references that are known to grow in low nutrient environments. In contrast, restored meadows and current references were characterized by a more important contribution of tall, long-lived and competitive graminoids with high SLA, and a set of indicator species that preferentially develop in more nutrient-rich environments (e.g., Elymus repens, Geranium pyrenaicum, Lapsana communis). Those results provide relevant information about community changes between the historical and contemporary states of ecosystems (Barak et al., 2015; Higgs and Jackson, 2017) and show that significant changes occurred over a time span of ca. 65 years (between 1955 and 2020). This pattern is in accordance with overall plant community changes induced by anthropogenic drivers (Timmermann et al., 2015), specifically nitrogen deposition induced by anthropogenic activities in the 20th century, which is recognized as one of the major threats to plant biodiversity in European herbaceous ecosystems (Stevens et al., 2004; Bobbink et al., 2010; Stevens et al., 2018).
The gap observed between historical and current meadows with very good conservation status suggests that the historical data based on phytosociological surveys dating from ~60 years ago, and being the basis for the European habitat typology in Belgium, may be an over-ambitious target for restoration purposes in the current global environmental and socio-economic context. As often mentioned in the ecological restoration literature, the desire to reach, at all costs, a static reference state of the past in the current context of rapid and unprecedented global changes is most likely to be in vain (Harris et al., 2006; Choi et al., 2008; Allison, 2017). Achievable and sustainable outcomes cannot be reached with a target that may satisfy a nostalgic need but is inconsistent with the future (Harris et al., 2006). The expected future reduction in nitrogen deposition is unlikely to induce significant species recovery (Payne et al., 2017). In addition to historical global eutrophication which has already impacted grassland ecosystems in the temperate regions, climate change and increasingly frequent climate extremes are expected to cause long-term shifts in plant community composition (Gornish and Tylianakis, 2013; Shi et al., 2015). Also, societal expectations may prevent the return to the historical reference because it no longer matches a socially and economically acceptable goal for agriculture-dependent meadows (Choi, 2007; McNellie et al., 2020). For these reasons, history should not serve as a target but rather as a guide for restoration (Harris et al., 2006; Higgs et al., 2014). Achieving a specific taxon-based assemblage is no longer absolutely sought, but rather a set of functions, ecological processes and ecosystem services that restore ecosystem health (SER, 2004; Choi et al., 2008) and allow biodiversity restoration on a larger scale than that of the phytosociological survey, i.e., at the landscape or ecosystem scale (Choi, 2007). In the same line, the historical targets included plant community composition described by surveys following the Zurich-Montpellier method, i.e., a non-random and subjective sampling method where the most typical and representative sampling points of the studied phytosociological association were used (Becking, 1957). They are therefore the most biologically valuable representation of the Arrhenatherion association at the time, which has probably accentuated the contrast observed with current floristic communities. In addition, the number of current references was limited in this study because of the restricted availability of such meadows in current European temperate agricultural landscapes. Thus, there is an important need to create a well-defined reference framework of lowland hay meadows for the different essential steps of a restoration project; identification of objectives, monitoring and assessment. Future restoration evaluations of lowland hay meadows in temperate Europe will need adapted reference frameworks that would be more consistent with the current regional ecological and socio-economic context. To build a better reference framework, future research should improve the representativeness of Arrhenatherion meadows under conservation management by increasing their number and extending sampling to meadows outside nature reserves, i.e., meadows under an agri-environment scheme and Natura 2000 sites. It will also be a great opportunity to include baseline values of multiple ecosystem services (Wortley et al., 2013).
Both passive and active restoration efforts led to convergence in species richness, species composition and functional traits towards current targets, despite different pre-restoration states. Species richness increased at the same rate through time (2.4 species per year) in restored meadows whatever the restoration technique, and meadows restored with passive and sowing methods reached species richness similar to current references. The final species richness of meadows restored by hay transfer did not reach that of current references because of lower richness in initial states. Thus, having started with a lower number of species, meadows restored by fresh hay transfer may need more time to reach current reference species richness. All techniques showed successful restoration of species composition. Also, despite current and restored meadow flora indicating a richer nutrient context than historical meadows, restoration led to changes in functional composition. Observed patterns of changes are complex and may suggest that functional composition of restored meadow is not yet stabilized after 6 years. In contrast to pre-restoration communities dominated by mow-tolerant, long-lived grass with high SLA, restored communities showed higher contributions of short-lived dicotyledonous species, indicating fast resource-acquisition strategy, and grasses with higher LDMC, indicating a more conservative resource-acquisition strategy. These results demonstrate that adapting the restoration technique depending on the initial degradation state and the direct vicinity of the meadow is relevant. Our study highlights the efficiency of soil preparation and transfer of seed-containing plant material for restoring more impaired meadow sites. This is consistent with the outcomes of several studies that have tested the effectiveness of species introduction to restore lowland hay meadows or alluvial meadows (Edwards et al., 2007; Schmiede et al., 2012; Baasch et al., 2016; Harvolk-Schöning et al., 2020). Success of passive recovery also attests to the efficiency of management extensification as a means of restoring slightly degraded meadows with target species possibly remaining in the seed bank. This is congruent with the results of previous studies that reported a positive effect on species richness after cessation of fertilization and implementation of extensive management through mowing or grazing (Pallett et al., 2016; Van Vooren et al., 2018). In slightly altered landscapes that provide seed sources, stopping disturbance alone is a good tool to proceed successfully towards valuable meadow habitats and achieve restoration goals (Ruprecht, 2006). Postponing mowing from spring to summer was shown to be effective in promoting plant and invertebrate diversity in European meadows (Humbert et al., 2012). Similarly, a twice-a-year defoliation frequency was shown to be efficient in enhancing plant and insect richness and increasing export of potassium in meadow ecosystems (Uchida and Ushimaru, 2014; Piqueray et al., 2019). Before any restoration intervention, restoration practitioners should explore the local ecological conditions in order to decide which type of restoration action is most likely to succeed (Prach et al., 2020; Goret et al., 2021). Passive restoration should be favoured in mildly impaired sites, with low environmental stress and intermediate productivity, located in more natural and well-preserved landscapes (Prach et al., 2020). Financial and practical factors must also be considered. Passive recovery naturally requires lower costs than seed transfer, while fresh hay spreading additionally imposes organizational constraints. Fresh hay has to be transferred immediately to the receptor site after cutting, as storage would compromise seed viability due to rapid fermentation, and the large volume of fresh hay to be transferred requires proximity between donor and receptor sites, but compared to threshing, green haymaking is less time-consuming, requires only standard machinery (Blakesley and Buckley, 2016) and produces a more efficient seed harvest yield (Scotton and Ševčíková, 2017). The hay layer on the receptor site can also favor seedling establishment (Loydi et al., 2013).
Data availability statement
The raw data supporting the conclusions of this article will be made available by the authors, without undue reservation.
Author contributions
MD, TG, JP, and GM contributed to the conception and design of the study. MD and TG carried out the field work. MD, AF, JP, and GM designed and performed the statistical analysis. MD and GM wrote the first draft of the manuscript. All authors contributed to the article and approved the submitted version.
Funding
The study was supported by the Fund for Research Training in Industry and Agriculture (PhD Grant – FRIA, F.R.S.–FNRS) of the Walloon Region.
Acknowledgments
The authors would like to thank the team of the LIFE project “Prairies bocagères” and Natagora for making this work possible and making the data available.
Conflict of interest
The authors declare that the research was conducted in the absence of any commercial or financial relationships that could be construed as a potential conflict of interest.
Publisher’s note
All claims expressed in this article are solely those of the authors and do not necessarily represent those of their affiliated organizations, or those of the publisher, the editors and the reviewers. Any product that may be evaluated in this article, or claim that may be made by its manufacturer, is not guaranteed or endorsed by the publisher.
Supplementary material
The Supplementary material for this article can be found online at: https://www.frontiersin.org/articles/10.3389/fevo.2023.1136206/full#supplementary-material
References
Al Haj Khaled, R., Duru, M., Theau, J. P., Plantureux, S., and Cruz, P. (2005). Variation in leaf traits through seasons and N-availability levels and its consequences for ranking grassland species. J. Veg. Sci. 16, 391–398. doi: 10.1111/j.1654-1103.2005.tb02378.x
Allison, S. K. (2017). “Ecological restoration and environmental change” in Routledge Handbook of Ecological and Environmental Restoration. eds. S. K. Allison and S. D. Murphy (England: Routledge), 522–236.
Baasch, A., Engst, K., Schmiede, R., May, K., and Tischew, S. (2016). Enhancing success in grassland restoration by adding regionally propagated target species. Ecol. Eng. 94, 583–591. doi: 10.1016/j.ecoleng.2016.06.062
Barak, R. S., Hipp, A. L., Cavender-Bares, J., Pearse, W. D., Hotchkiss, S. C., Lynch, E. A., et al. (2015). Taking the long view: integrating recorded, archeological, Paleoecological, and evolutionary data into ecological restoration. Int. J. Plant Sci. 177, 90–102. doi: 10.1086/683394
Becking, W. R. (1957). The Zürich-Montpellier school of phytosociology. Bot. Rev. 23, 411–488. doi: 10.1007/BF02872328
Blakesley, D., and Buckley, P. (2016). Grassland Restoration and Management. London, Pelagic Publishing Ltd.
Bobbink, R., Hicks, K., Galloway, J., Spranger, T., Alkemade, R., Ashmore, M., et al. (2010). Global assessment of nitrogen deposition effects on terrestrial plant diversity: a synthesis. Ecol. Appl. 20, 30–59. doi: 10.1890/08-1140.1
Bruchmann, I., and Hobohm, C. (2010). Halting the loss of biodiversity: endemic vascular plants in grasslands of Europe. Grassl. Sci. Europe 15, 776–778.
Choi, Y. D. (2007). Restoration ecology to the future: a call for new paradigm. Restor. Ecol. 15, 351–353. doi: 10.1111/j.1526-100X.2007.00224.x
Choi, Y. D., Temperton, V. M., Allen, E. B., Grootjans, A. P., Halassy, M., Hobbs, R. J., et al. (2008). Ecological restoration for future sustainability in a changing environment. Écoscience 15, 53–64. doi: 10.2980/1195-6860(2008)15[53:ERFFSI]2.0.CO;2
Cruz, P., Duru, M., Therond, O., Theau, J. P., Ducourtieux, C., Jouany, C., et al. (2002). Une nouvelle approche pour caractériser les prairies naturelles et leur valeur d’usage. Fourrages 172, 335–354.
Davies, C. E., Moss, D., and Hill, M. O. (2004). Eunis Habitat Classification Revised 2004 (p. 310). Tilburg. European Environment Agency. European Topic Centre on Nature Protection and Biodiversity.
De Cáceres, M., Legendre, P., and Moretti, M. (2010). Improving indicator species analysis by combining groups of sites. Oikos 119, 1674–1684. doi: 10.1111/j.1600-0706.2010.18334.x
DEMNA. (2019). Evaluation des états de conservation des habitats et des espèces d’intérêt communautaire en Wallonie (période 2013–2018) | Natura 2000 | La biodiversité en Wallonie. Available at: http://biodiversite.wallonie.be/fr/evaluation-des-etats-de-conservation-des-habitats-et-des-especes-d-interet-communautaire-en-wallonie-periode-2013-2018.html?IDD=6292&IDC=5803
Donath, T., Hölzel, N., and Otte, A. (2003). The impact of site conditions and seed dispersal on restoration success in alluvial meadows. Appl. Veg. Sci. 6, 13–22. doi: 10.1111/j.1654-109X.2003.tb00560.x
Dufrêne, M., and Delescaille, L. –M.. (2005). La typologie WalEUNIS des biotopes wallons, version 1.0. Available at: http://biodiversite.wallonie.be/fr/la-typologie-waleunis-version-1-0.html?IDD=962&IDC=811
Edwards, A. R., Mortimer, S. R., Lawson, C. S., Westbury, D. B., Harris, S. J., Woodcock, B. A., et al. (2007). Hay strewing, brush harvesting of seed and soil disturbance as tools for the enhancement of botanical diversity in grasslands. Biol. Conserv. 134, 372–382. doi: 10.1016/j.biocon.2006.08.025
Emmerson, M., Morales, M. B., Oñate, J. J., Batáry, P., Berendse, F., Liira, J., et al. (2016). “Chapter two—how agricultural intensification affects biodiversity and ecosystem services” in Advances in Ecological Research. eds. A. J. Dumbrell, R. L. Kordas, and G. Woodward, vol. 55 (Cambridge, MA: Academic Press), 43–97.
Engst, K., Baasch, A., and Bruelheide, H. (2017). Predicting the establishment success of introduced target species in grassland restoration by functional traits. Ecol. Evol. 7, 7442–7453. doi: 10.1002/ece3.3268
European Environment Agency. (2019). Natura 2000 Network Viewer. Available at: https://natura2000.eea.europa.eu/#
European Environment Agency. (2020). State of nature in the EU – results from reporting under the nature directives 2013–2018 (publication no. 10/2020; 146. EEA Report). Available at: https://www.eea.europa.eu/publications/state-of-nature-in-the-eu-2020
Fagan, K. C., Pywell, R. F., Bullock, J. M., and Marrs, R. H. (2008). Do restored calcareous grasslands on former arable fields resemble ancient targets? The effect of time, methods and environment on outcomes. J. Appl. Ecol. 45, 1293–1303. doi: 10.1111/j.1365-2664.2008.01492.x
Goret, T., Janssens, X., and Godefroid, S. (2021). A decision-making tool for restoring lowland grasslands in Europe. J. Nat. Conserv. 63:126046. doi: 10.1016/j.jnc.2021.126046
Goret, T., Lighezzolo, P., Kints, O., Cavelier, D., André, A., Sevrin, D., et al. (2020). The “Bocage meadows” LIFE project. Assessment of 8 years of actions in favor of the biodiversity of the meadows of Fagne-Famenne (Belgium). LAYMAN’S REPORT LIFE+11 NAT/BE/001059 (p. 20). Natagora. Available at: https://www.lifeprairiesbocageres.eu/fileadmin/Life/Prairies_bocageres/laymans_report_FINAL_EN_basseQ.pdf
Gornish, E. S., and Tylianakis, J. M. (2013). Community shifts under climate change: mechanisms at multiple scales. Am. J. Bot. 100, 1422–1434. doi: 10.3732/ajb.1300046
Habel, J. C., Dengler, J., Janišová, M., Török, P., Wellstein, C., and Wiezik, M. (2013). European grassland ecosystems: threatened hotspots of biodiversity. Biodivers. Conserv. 22, 2131–2138. doi: 10.1007/s10531-013-0537-x
Harris, J. A., Hobbs, R. J., Higgs, E., and Aronson, J. (2006). Ecological restoration and global climate change. Restor. Ecol. 14, 170–176. doi: 10.1111/j.1526-100X.2006.00136.x
Harvolk-Schöning, S., Michalska-Hejduk, D., Harnisch, M., Otte, A., and Donath, T. W. (2020). Floodplain meadow restoration revisited: long-term success of large scale application of diaspore transfer with plant material in restoration practice. Biol. Conserv. 241:108322. doi: 10.1016/j.biocon.2019.108322
Hautier, Y., Niklaus, P. A., and Hector, A. (2009). Competition for light causes plant biodiversity loss after eutrophication. Science 324, 636–638. doi: 10.1126/science.1169640
Higgs, E., Falk, D. A., Guerrini, A., Hall, M., Harris, J., Hobbs, R. J., et al. (2014). The changing role of history in restoration ecology. Front. Ecol. Environ. 12, 499–506. doi: 10.1890/110267
Higgs, E., and Jackson, S. T. (2017). “The role of history in restoration ecology,” in Routledge Handbook of Ecological and Environmental Restoration. eds. S. K. Allison and S. D. Murphy (London: Routledge), 522–236.
Hodgson, J. G., Wilson, P. J., Hunt, R., Grime, J. P., and Thompson, K. (1999). Allocating C-S-R plant functional types: a soft approach to a hard problem. Oikos 85, 282–294. doi: 10.2307/3546494
Holl, K. D., and Cairns, J. R. (2002). “Monitoring and appraisal” in Handbook of Ecological Restoration. Volume 1. Principles of Restoration. eds. M. R. Perrow and A. J. Davy (Waldén: Cambridge University Press), 409–432.
Hölzel, N., Buisson, E., and Dutoit, T. (2012). Species introduction—a major topic in vegetation restoration. Appl. Veg. Sci. 15, 161–165. doi: 10.1111/j.1654-109X.2012.01189.x
Hothorn, T., Bretz, F., Westfall, P., Heiberger, R. M., Schuetzenmeister, A., and Scheibe, S. (2021). Package ‘multcomp’. Simultaneous inference in general parametric models (1.4–16) [computer software]. Available at: https://CRAN.R-project.org/package=multcomp
Humbert, J.-Y., Pellet, J., Buri, P., and Arlettaz, R. (2012). Does delaying the first mowing date benefit biodiversity in meadowland? Environ. Evid. 1:9. doi: 10.1186/2047-2382-1-9
Hunt, R., Hodgson, J. G., Thompson, K., Bungener, P., Dunnett, N., and Askew, A. (2004). A new practical tool for deriving a functional signature for herbaceous vegetation. Appl. Veg. Sci. 7, 163–170. doi: 10.1111/j.1654-109X.2004.tb00607.x
Isselstein, J., Jeangros, B., and Pavlu, V. (2005). Agronomic aspects of biodiversity targeted management of temperate grasslands in Europe—a review. Agron. Res. 3, 139–151.
Jackson, S. T., and Hobbs, R. J. (2009). Ecological restoration in the light of ecological history. Science 325, 567–569. doi: 10.1126/science.1172977
Jones, H. P., Jones, P. C., Barbier, E. B., Blackburn, R. C., Rey Benayas, J. M., Holl, K. D., et al. (2018). Restoration and repair of Earth’s damaged ecosystems. Proc. R. Soc. B 285:20172577. doi: 10.1098/rspb.2017.2577
Julve, P. (1998). Baseflor. Index botanique, écologique et chorologique de la flore de France. Version: 22/08/2017. Available at: Http://philippe.julve.pagesperso-orange.fr/catminat.htm
Kahmen, S., and Poschlod, P. (2004). Plant functional trait responses to grassland succession over 25 years. J. Veg. Sci. 15, 21–32. doi: 10.1111/j.1654-1103.2004.tb02233.x
Kassambara, A. (2021). Package ‘rstatix’. Pipe-Friendly framework for basic statistical tests (0.7.0) [computer software]. Available at: https://CRAN.R-project.org/package=rstatix
Kiss, R., Valkó, O., Tóthmérész, B., and Török, P. (2016). Seed bank research in central-European grasslands—an overview. In J. Murphy (Ed.), Seed Banks: Types, Roles and Research, J. Murphy, ed., pp. 1–34). Nova Science Publisher, Hauppauge.
Kleyer, M., Bekker, R. M., Knevel, I. C., Bakker, J. P., Thompson, K., Sonnenschein, M., et al. (2008). The LEDA Traitbase: a database of life-history traits of the northwest European flora. J. Ecol. 96, 1266–1274. doi: 10.1111/j.1365-2745.2008.01430.x
Kühn, I., and Klotz, S. (2002). “Systematik, Taxonomie und Nomenklatur,” in BIOLFLOR – Eine Datenbank mit biologisch-ökologischen Merkmalen zur Flora von Deutschland (Schriftenreihe für Vegetationskunde). eds. S. Klotz, I. Kühn, and W. Durka Available at: http://www.ufz.de/biolflor/taxonomie/taxonomie.jsp?action=filter&ID_Familie=84&ID_Gattung=225&ID_Taxonomie=865
Lambinon, J., and Verloove, F. (2015). Nouvelle Flore de la Belgique, du Grand-Duché de Luxembourg, du Nord de la France et des régions voisines (6e éd) Jardin botanique Meise, Meise.
Lavorel, S., Díaz, S., Cornelissen, J. H. C., Garnier, E., Harrison, S. P., McIntyre, S., et al. (2007). “Plant functional types: are we getting any closer to the holy grail?” in Terrestrial ecosystems in a changing world. eds. J. G. Canadell, D. E. Pataki, and L. F. Pitelka (Berlin: Springer), 149–164.
Lavorel, S., and Garnier, E. (2002). Predicting changes in community composition and ecosystem functioning from plant traits: revisiting the holy grail. Funct. Ecol. 16, 545–556. doi: 10.1046/j.1365-2435.2002.00664.x
Lavorel, S., Grigulis, K., McIntyre, S., Williams, N. S. G., Garden, D., Dorrough, J., et al. (2008). Assessing functional diversity in the field – methodology matters! Funct. Ecol. 22, 134–147. doi: 10.1111/j.1365-2435.2007.01339.x
Loydi, A., Eckstein, R. L., Otte, A., and Donath, T. W. (2013). Effects of litter on seedling establishment in natural and semi-natural grasslands: a meta-analysis. J. Ecol. 101, 454–464. doi: 10.1111/1365-2745.12033
Manning, P., Gossner, M. M., Bossdorf, O., Allan, E., Zhang, Y. Y., Prati, D., et al. (2015). Grassland management intensification weakens the associations among the diversities of multiple plant and animal taxa. Ecology 96, 1492–1501. doi: 10.1890/14-1307.1
Marini, L., Fontana, P., Scotton, M., and Klimek, S. (2008). Vascular plant and Orthoptera diversity in relation to grassland management and landscape composition in the European Alps. J. Appl. Ecol. 45, 361–370. doi: 10.1111/j.1365-2664.2007.01402.x
Matson, P., Lohse, K. A., and Hall, S. J. (2002). The globalization of nitrogen deposition: consequences for terrestrial ecosystems. Ambio 31, 113–119. doi: 10.1579/0044-7447-31.2.113
McGinlay, J., Gowing, D. J. G., and Budds, J. (2017). The threat of abandonment in socio-ecological landscapes: farmers’ motivations and perspectives on high nature value grassland conservation. Environ. Sci. Pol. 69, 39–49. doi: 10.1016/j.envsci.2016.12.007
McNellie, M. J., Oliver, I., Dorrough, J., Ferrier, S., Newell, G., and Gibbons, P. (2020). Reference state and benchmark concepts for better biodiversity conservation in contemporary ecosystems. Glob. Chang. Biol. 26, 6702–6714. doi: 10.1111/gcb.15383
Oksanen, J., Blanchet, F. G., Friendly, M., Kindt, R., Legendre, P., McGlinn, D., et al. (2020). Package ‘vegan’. Community ecology package (2.5–7) [computer software]. Available at: https://CRAN.R-project.org/package=vegan
Pallett, D. W., Pescott, O. L., and Schäfer, S. M. (2016). Changes in plant species richness and productivity in response to decreased nitrogen inputs in grassland in southern England. Ecol. Indic. 68, 73–81. doi: 10.1016/j.ecolind.2015.12.024
Payne, R. J., Dise, N. B., Field, C. D., Dore, A. J., Caporn, S. J., and Stevens, C. J. (2017). Nitrogen deposition and plant biodiversity: past, present, and future. Front. Ecol. Environ. 15, 431–436. doi: 10.1002/fee.1528
Piqueray, J., Gilliaux, V., Decruyenaere, V., Cornelis, J.-T., Uyttenbroeck, R., and Mahy, G. (2019). Management of Grassland-like Wildflower Strips Sown on nutrient-rich arable soils: the role of grass density and mowing regime. Environ. Manag. 63, 647–657. doi: 10.1007/s00267-019-01153-y
Prach, K., Durigan, G., Fennessy, S., Overbeck, G. E., Torezan, J. M., and Murphy, S. D. (2019). A primer on choosing goals and indicators to evaluate ecological restoration success. Restor. Ecol. 27, 917–923. doi: 10.1111/rec.13011
Prach, K., Šebelíková, L., Řehounková, K., and del Moral, R. (2020). Possibilities and limitations of passive restoration of heavily disturbed sites. Landsc. Res. 45, 247–253. doi: 10.1080/01426397.2019.1593335
Pywell, R. F., Woodcock, B., Tallowin, J. B., Mortimer, S. R., and Bullock, J. M. (2012). Restoring species-rich grassland: principles and techniques. Asp. Appl. Biol. 115, 11–21.
R Core Team. (2021). R: A language and environment for statistical computing. Available at: https://www.r-project.org/
Ruprecht, E. (2006). Successfully recovered grassland: a promising example from Romanian old-fields. Restor. Ecol. 14, 473–480. doi: 10.1111/j.1526-100X.2006.00155.x
Saintenoy-Simon, J., Barbier, Y., Delescaille, L.-M., Dufrêne, M., Gathoye, J.-L., and Verté, P. (2006). Première liste des espèces rares, menacées et protégées de la Région Wallonne (Ptéridophytes et Spermatophytes). Version 1 (7/3/2006). Available at: http://observatoire.biodiversite.wallonie.be/especes/flore/LR2010/
Schmiede, R., Otte, A., and Donath, T. W. (2012). Enhancing plant biodiversity in species-poor grassland through plant material transfer – the impact of sward disturbance. Appl. Veg. Sci. 15, 290–298. doi: 10.1111/j.1654-109X.2011.01168.x
Scotton, M., and Ševčíková, M. (2017). Efficiency of mechanical seed harvesting for grassland restoration. Agric. Ecosyst. Environ. 247, 195–204. doi: 10.1016/j.agee.2017.06.040
Sengl, P., Magnes, M., Weitenthaler, K., Wagner, V., Erdős, L., and Berg, C. (2017). Restoration of lowland meadows in Austria: a comparison of five techniques. Basic Appl. Ecol. 24, 19–29. doi: 10.1016/j.baae.2017.08.004
SER. (2004). The SER International Primer on Ecological Restoration. Society for Ecological Restoration International. Available at: www.ser.org
Shi, Z., Sherry, R., Xu, X., Hararuk, O., Souza, L., Jiang, L., et al. (2015). Evidence for long-term shift in plant community composition under decadal experimental warming. J. Ecol. 103, 1131–1140. doi: 10.1111/1365-2745.12449
Stevens, C. J., David, T. I., and Storkey, J. (2018). Atmospheric nitrogen deposition in terrestrial ecosystems: its impact on plant communities and consequences across trophic levels. Funct. Ecol. 32, 1757–1769. doi: 10.1111/1365-2435.13063
Stevens, C. J., Dise, N. B., Mountford, J. O., and Gowing, D. J. (2004). Impact of nitrogen deposition on the species richness of grasslands. Science 303, 1876–1879. doi: 10.1126/science.1094678
Stoate, C., Báldi, A., Beja, P., Boatman, N. D., Herzon, I., van Doorn, A., et al. (2009). Ecological impacts of early 21st century agricultural change in Europe – a review. J. Environ. Manag. 91, 22–46. doi: 10.1016/j.jenvman.2009.07.005
Sullivan, E., Hall, N., and Ashton, P. (2020). Restoration of upland hay meadows over an 11-year chronosequence: an evaluation of the success of green hay transfer. Restor. Ecol. 28, 127–137. doi: 10.1111/rec.13063
Timmermann, A., Damgaard, C., Strandberg, M. T., and Svenning, J.-C. (2015). Pervasive early 21st-century vegetation changes across Danish semi-natural ecosystems: more losers than winners and a shift towards competitive, tall-growing species. J. Appl. Ecol. 52, 21–30. doi: 10.1111/1365-2664.12374
Uchida, K., and Ushimaru, A. (2014). Biodiversity declines due to abandonment and intensification of agricultural lands: patterns and mechanisms. Ecol. Monogr. 84, 637–658. doi: 10.1890/13-2170.1
van der Maarel, E. (1979). Transformation of cover-abundance values in phytosociology and its effects on community similarity. Vegetatio 39, 97–114. doi: 10.1007/BF00052021
Van der Perre, R., Bythell, S., Bogaert, P., Claessens, H., Ridremon, F., Tricot, C., et al. (2015). La carte bioclimatique de Wallonie: Un nouveau découpage écologique du territoire pour le choix des essences forestières. Forêt. Nature 135, 47–58.
Van Vooren, L., Reubens, B., Broekx, S., Reheul, D., and Verheyen, K. (2018). Assessing the impact of grassland management extensification in temperate areas on multiple ecosystem services and biodiversity. Agric. Ecosyst. Environ. 267, 201–212. doi: 10.1016/j.agee.2018.08.016
Veen, P., Jefferson, R., de Smidt, J., and van der Straaten, J.. (2009). Grasslands in Europe: Of high nature value, BRILL. Leiden.
Violle, C., Navas, M.-L., Vile, D., Kazakou, E., Fortunel, C., Hummel, I., et al. (2007). Let the concept of trait be functional! Oikos 116, 882–892. doi: 10.1111/j.0030-1299.2007.15559.x
Waldén, E., and Lindborg, R. (2016). Long term positive effect of grassland restoration on plant diversity—success or not? PLoS One 11:e0155836. doi: 10.1371/journal.pone.0155836
Walker, K. J., Stevens, P. A., Stevens, D. P., Mountford, J. O., Manchester, S. J., and Pywell, R. F. (2004). The restoration and re-creation of species-rich lowland grassland on land formerly managed for intensive agriculture in the UK. Biol. Conserv. 119, 1–18. doi: 10.1016/j.biocon.2003.10.020
White, P. S., and Walker, J. L. (1997). Approximating nature’s variation: selecting and using reference information in restoration ecology. Restor. Ecol. 5, 338–349. doi: 10.1046/j.1526-100X.1997.00547.x
Wibail, L., Goffart, P., Smits, Q., Delescaille, L.-M., Couvreur, J.-M., Keulen, C., et al. (2014). Évaluation de l’état de conservation des habitats et espèces Natura 2000 en Wallonie. Résultats du Rapportage Article 17 au titre de la Directive 92/43/CEE pour la période 2007–2012 (p. 277). Available at: http://biodiversite.wallonie.be/servlet/Repository/docsynthese-rapp2013-finale-26-06-2014.pdf?ID=31436&saveFile=true
Wickham, H., Chang, W., Henry, L., Pedersen, T. L., Takahashi, K., Wilke, C., et al. (2020). Package ‘ggplot2’. Create elegant data Visualisations using the grammar of graphics (3.3.3) [computer software]. Available at: https://CRAN.R-project.org/package=ggplot2
Wilson, J. B., Peet, R. K., Dengler, J., and Pärtel, M. (2012). Plant species richness: the world records. J. Veg. Sci. 23, 796–802. doi: 10.1111/j.1654-1103.2012.01400.x
Wortley, L., Hero, J. M., and Howes, M. (2013). Evaluating ecological restoration success: a review of the literature. Restor. Ecol. 21, 537–543. doi: 10.1111/rec.12028
Keywords: grassland, restoration success, diaspore transfer, restoration reference, plant community, hay transfer, mowing
Citation: Dellicour M, Goret T, Piqueray J, Fayolle A, Bindelle J and Mahy G (2023) Success of passive and active restoration of lowland hay meadows with regard to current and historical references. Front. Ecol. Evol. 11:1136206. doi: 10.3389/fevo.2023.1136206
Edited by:
Zoltán Bátori, University of Szeged, HungaryReviewed by:
Karel Prach, University of South Bohemia in České Budějovice, CzechiaLászló Erdős, Hungarian Academy of Science, Hungary
Copyright © 2023 Dellicour, Goret, Piqueray, Fayolle, Bindelle and Mahy. This is an open-access article distributed under the terms of the Creative Commons Attribution License (CC BY). The use, distribution or reproduction in other forums is permitted, provided the original author(s) and the copyright owner(s) are credited and that the original publication in this journal is cited, in accordance with accepted academic practice. No use, distribution or reproduction is permitted which does not comply with these terms.
*Correspondence: Grégory Mahy, Zy5tYWh5QHVsaWVnZS5iZQ==