- 1Marine Science and Biology, Eckerd College, St. Petersburg, FL, United States
- 2Marine Science, Eckerd College, St. Petersburg, FL, United States
Although there is growing concern about ingestion of microplastics by marine organisms, little research has been conducted on marine herbivores. This is the first study to document microplastic ingestion within the family Sirenia. Subsamples were collected from five locations in the gastrointestinal tracts (GI) of 26 dead manatees (Trichechus manatus latirostris) from Tampa Bay, Florida. During gross necropsies, macroplastic pieces were found in seven individuals (26.9%). Careful visual examination of the subsampled portions of the GI contents indicated that 19 individuals (73.1%) contained plastic particles. As five individuals had both macro and microplastic pieces, the overall frequency of occurrence of plastic ingestion was 76.9%. Due to the large volume of cellulose-rich ingested material, it was not feasible to analyze the entire gut contents, nor was it feasible to conduct chemical or enzymatic digestion; therefore, it is very likely that many microplastic pieces were not detected. Despite these technical challenges, it is clear that manatees in Tampa Bay are routinely consuming microplastics in addition to larger plastic pieces. Currently, nothing is known about the physiological effects of microplastic ingestion in sirenians, however environmental plastics could be concentrated by manatees through ingestion and the subsequent production of microplastics-laden feces.
Introduction
The amount of plastic pollution in marine systems is concerning due to the potential for severe repercussions on humans and wildlife. Around 700 marine species have been documented to have encountered marine debris and 92% of those encounters were with plastic debris (Gall and Thompson, 2015). Plastics threaten marine life through physical interactions such as entanglement, ingestion, acting as a vector for invasive organisms and diseases, and by transporting and leaching toxic substances (Gall and Thompson, 2015; Saliu et al., 2019; Bucci et al., 2020). Early concerns focused on larger pieces of plastic clearly visible to the naked eye (e.g., Laist, 1987), but increasingly the plastic pollution crisis is focusing on microplastics (defined as plastic smaller than 5 mm; Arthur et al., 2009) which are ubiquitous throughout the marine environment and can be ingested by even the smallest of marine organisms (Fibbe et al., 2023).
Marine mammals are vulnerable to entanglement and ingestion of macroplastics (e.g., Simmonds, 2012; Baulch and Perry, 2014; Butterworth, 2016). Microplastics have been found in the gastrointestinal (GI) tract or scat of a variety of cetaceans and pinnipeds (see Zantis et al., 2021 for a review). Florida manatees (Trichechus manatus latirostris) have been known to ingest a variety of macroplastics including fishing line and plastic bags (Beck and Barros, 1991; Adimey et al., 2014; Reinert et al., 2017) as have Antillean manatees (Trichechus manatus manatus; Attademo et al., 2015) and Amazonian manatees (Trichechus inunguis; Guterres-Pazin et al., 2012).
Sirenian diets differ greatly from those of other marine mammals as they forage on vegetation, therefore the routes and impact of plastic ingestion may also differ. Detection of microplastics in manatees is especially challenging due to the large quantities of cellulose-rich food they consume. Manatees have long gut passage time (6–9 days; Larkin et al., 2007), large GI tract (tract and contents weighing upwards of 100 kg; Reynolds and Rommel, 1996) and a 500 kg manatee is estimated to consume 4 to 9% of their body weight daily depending on food type and season (Bengtson, 1983; Worthy and Worthy, 2014).
Manatees routinely forage in seagrass beds (Reich and Worthy, 2006) where there is increasing evidence that microplastics are trapped and retained. Microplastic concentrations in sediments from seagrass beds were higher than in sediments from surrounding non-vegetated areas (Huang et al., 2020; Jones et al., 2020; Plee and Pomory, 2020) or higher than in the surrounding seawater (Tahir et al., 2019; Kreitsberg et al., 2021), although some study sites did not see enrichment (e.g., Cozzolino et al., 2020; Unsworth et al., 2021; Boshoff et al., 2023). In some cases, there was variability between different locations within a single study, some showing enrichment of microplastics while other nearby sites did not (Huang et al., 2021). However, all studies found relatively high levels of microplastics in the sediments of seagrass beds. Cozzolino et al. (2020) also found macroplastic (particles >5 mm) concentrations were elevated in seagrass habitats in comparison to nearby unvegetated sites. Additionally, microplastics have been found adhering to seagrass blades or their epibonent algae (Goss et al., 2018; Datu et al., 2019; Cozzolino et al., 2020; Jones et al., 2020; Seng et al., 2020; Sawalman et al., 2021). Seagrass associated benthic invertebrates (Tahir et al., 2019; Jones et al., 2020; Plee and Pomory, 2020; Sawalman et al., 2021), fish (Baalkhuyur et al., 2018; Sbrana et al., 2020; Cabansag et al., 2021) and turtles (Caron et al., 2018; Duncan et al., 2019) have also ingested microplastics.
Given the ability of seagrass beds to trap microplastics and the large volume of seagrasses that manatees consume, it is likely that manatees ingest microplastics, however this has not been previously investigated. The Florida manatee, a subspecies of the West Indian manatee, is native to the southeastern United States, mainly coastal Florida. The subspecies is listed as threatened by the US Fish and Wildlife Service1 and endangered by the IUCN.2 Continued threats (Runge et al., 2017) and several recent (Landsberg et al., 2022) and ongoing unusual mortality events3 indicate that this species is still at risk. This is the first study of microplastic ingestion by any sirenian species.
Materials and methods
Manatee sample collection
In Florida, manatee carcasses are reported by the public to a state-managed wildlife hotline, and regional biologists respond to collect carcass information. A portion of the carcasses are transported to the Marine Mammal Pathobiology Laboratory, located in St. Petersburg, Florida and operated by Florida Fish and Wildlife Conservation Commission’s Fish and Wildlife Research Institute, where they are examined according to standardized procedures (Bonde et al., 1983). As a part of a larger project to investigate microplastics in Tampa Bay, we collected samples from the GI tract of manatee carcasses found dead in the Tampa Bay estuary. We excluded all manatees that did not have an intact external body to avoid contamination of the gut contents by external microplastics. Manatees were assigned an age class based on total length: adult >265 cm; juvenile 235–265 cm and calf <235 cm (which includes young animals capable of foraging on their own; Runge et al., 2017).
Approximately 500 mL of GI tract contents were collected from each of five gut segments: stomach, duodenum, cecum, proximal colon and distal colon (Figure 1). Samples were stored in (~700 mL) whirl packs during the necropsy and were frozen at −20°C until processed.
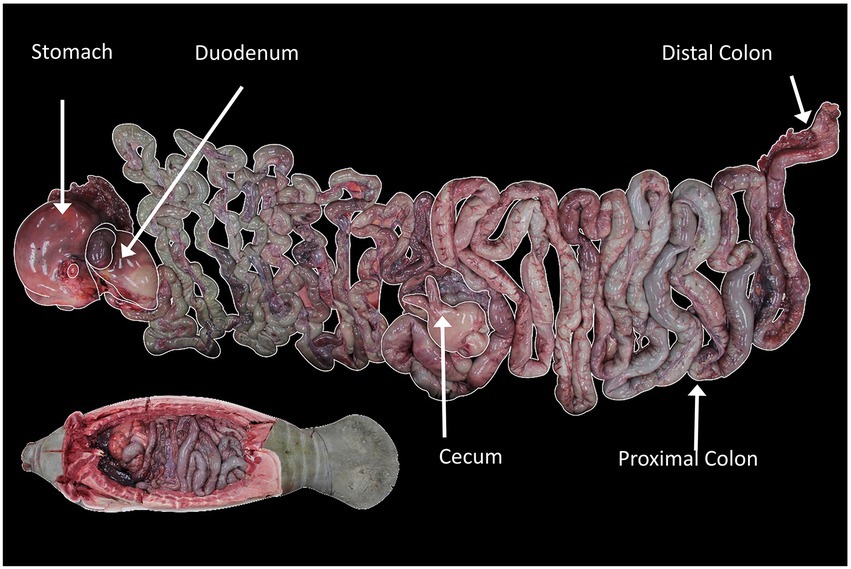
Figure 1. Location of sampling sites in the GI tract of the manatee. The lower inset image shows GI tract inside the manatee carcass in anatomical orientation, while the larger image shows the tract layed out. Image credit: Florida Fish and Wildlife Conservation Commission.
Sample processing
A wide variety of approaches have been used to separate microplastics from the surrounding gut contents. Dissection and visual observation is the simplest approach, but limits the lower size particle that can be detected and, because it typically is a slow process, leads to a higher risk of contamination during processing (Dehaut et al., 2019). Chemical digestion is often used and certain protocols have been proposed as monitoring standards, such as the use of HNO3:HClO4 in a 4:1 ratio by ICES (2015). Enzymatic digestions are time-consuming and expensive (Dehaut et al., 2019), while acidic digestions frequently degrade or alter the plastic particles (Enders et al., 2017). Bases such as KOH may be less likely to alter the plastics, but are corrosive and must be used with caution (Dehaut et al., 2019). Separating microplastics from the guts of marine grazers which consume vascular plants can be especially problematic as cellulose remains resistant to many digestion processes (Hurley et al., 2018) and can be difficult to differentiate from microplastic particles (Egea-Corbacho et al., 2022). Hence, a combination of visual separation and fluorescent staining was used in the present study.
To eliminate contamination, cotton clothing or lab coats were worn at all times, samples and reagent solutions were kept covered except for brief periods while handling. After thawing samples, wet weights were collected. Gut contents were initially diluted at a ratio of 1 l water per 100 g of gut content sample. Tap water was used as we were only looking for microplastic pieces ~1 mm or larger. Diluted samples were initially hand stirred and then placed on a stir plate and mechanically stirred for 1 h. The samples were left to settle for 24 h. The entire sample was sieved through a 212 μm sieve and then rinsed with a pressure hose to separate plastics and vegetation from sand. To prevent the degradation of plastics and to conduct the analysis in an efficient and cost effective manner, the GI contents were visually examined by systematically sweeping through the sieved GI contents using stainless steel forceps and spatulas. Potential plastic pieces were collected and removed from the sample. The remaining GI contents were placed in a drying oven at 50°C for 3–5 days and then dry weights were recorded. A similar process was used for Asian elephants (Katlam et al., 2022). All plastic pieces identified from sub-samples collected from the GI tract were missed during the gross necropsy which relied on manual palpitation or visual identification.
Plastic particle identification and imaging
Potential plastics were individually stained with Nile Red (9-diethylamino-5H-benzo[a] phenoxazine-5-one) stock solution (5 mg Nile Red powder dissolved in 1 l acetone) (adapted from Maes et al., 2017) for 30 min and then individually placed in petri dishes to dry and for storage. Dried plastics were imaged using a dissecting microscope equipped with a digital camera and Stereo Microscope Fluorescence Adapter system (NightSea, Lexington, MA). Samples were exposed to cyan light (excitation wavelength: 490–515 nm) and viewed through an orange emission filter (550 nm longpass). Plastics were categorized as micro or macroplastics, as well as fragments, sheets or fibers. Monofilament lines, typically from fishing gear, were recorded as fibers as they can break down into smaller fibers (Wright et al., 2021), but they are additionally listed as monofilament lines in Supplementary Table S1. ImageJ (Schneider et al., 2012) was used to determine the length of the longest axis and area.
Statistical analysis
To investigate if the concentration of microplastics varied between the different sections of the GI tract, we calculated the number of plastic particles per gram of both wet and dry weight of material examined for each sampled location in each manatee. A Shapiro–Wilk test was used to test for normality in the distribution of the data and a nonparametric Kruskal-Wallis test was used to compare the distribution of the plastic particles between the different segments of the GI tract. All data analysis was performed in JMP (SAS Institute, NC, United States).
Results
Between December 2017 and March 2020, 26 manatee carcasses recovered from Tampa Bay were sampled for plastics (see Supplementary Table S1 for details on each individual). There were 16 adults, 7 juveniles and 3 calves; 12 females and 14 males; all of the calves had GI tracts filled with vegetation. During gross necropsy, macroplastics were detected in 26.9% of the individuals (7/26). Most carcasses (81%; 21/26) had full GI tracts, with large quantities of vegetation present throughout the tract. While cause of death varied for these manatees, plastic ingestion was not a direct cause of death, although GI lesions of one (MNW18003) were consistent with having previously passed a foreign body.
A total of 12 pieces of macroplastic were found during gross necropsy ( = 0.46, S.E. = 0.19 per individual); 48 plastic pieces were found in the selected sub-samples ( = 1.86 S.E. = 0.33 per individual); for a total of 60 plastic pieces =2.31, S.E. = 0.41 per individual). Plastic pieces ranged in linear measurement from 0.02 mm to 14.9 mm, including eight plastic fibers which were larger than 5 mm, which is the generally accepted definition of microplastics. Fibers were the most common type of microplastics identified in manatees (Table 1; Figure 2), including 11 pieces which were clearly visible as monofilament fishing line. Five of the eight larger plastic fibers were monofilament fishing line. Fragments were typically small particles with an irregular oval shape, while sheets were relatively uncommon, but did include small portions of plastic bags.
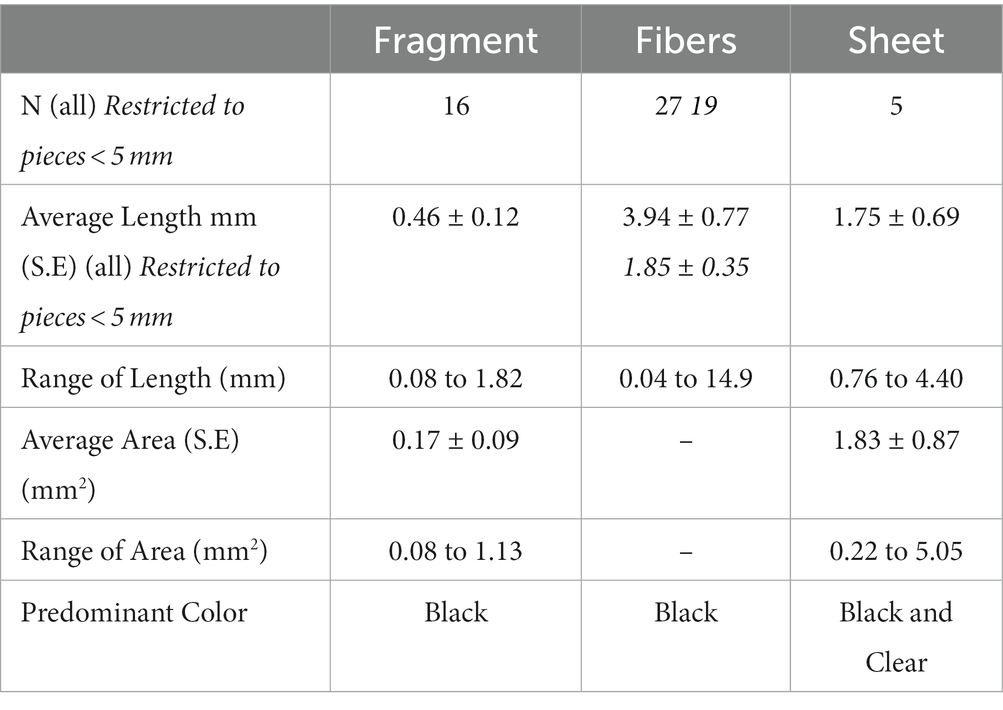
Table 1. Summary of plastic pieces collected from sub-sampled GI tract from 26 Florida manatees collected in Tampa Bay, Florida.
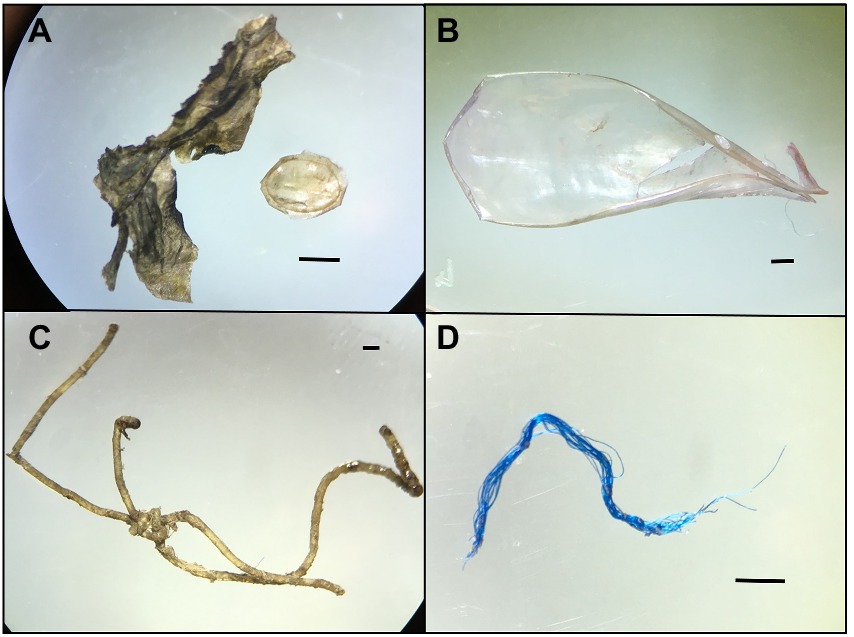
Figure 2. Microscopic images of representative plastics of different shapes collected from the GI tract of manatees (A) Fragment, two different particles, (B) Sheet, (C) Monofilament Line Fiber and (D) Fiber. Scale bar = 1 mm.
We were able to subsample from all five locations in the GI tract for 24 individuals, however individual MNW18009 did not have any ingesta in either the stomach or duodenum, and individual MNW19038 did not have any digesta in the cecum. Plastic pieces were detected in the sub-sampled portion of the GI tract of 73.1% of the individuals (19/26) and when combined with the macroplastics found during gross necropsies, 76.9% (20/26 individuals) contained at least 1 piece of plastic in their GI tract. In carcasses with both types of plastics, the macro- and microplastics came from different items for most individuals (5/6; see Supplementary Table S1). Ingestion of plastics varied between the sexes (10/14–71% males and 10/12–83% females had ingested plastic) and age classes (12/16–75% adults, 457–71% juveniles and 3/3–100% calves had ingested plastic); however, small sample sizes prevented further analysis. Plastic was found in the GI tracts of manatees that had been feeding recently (16/22) as well as those with relatively empty GI tracts (3/4 individuals).
Microplastic pieces were found throughout the GI tract; 6 in the stomach (13%), 5 in the duodenum (10%), 8 in the cecum (19%), 10 in the proximal colon (25%) and 16 in the distal colon (33%). Concentrations of plastics per gram of GI subsample examined varied between the different sections (Table 2). The concentrations of the particles were not normally distributed (Shapiro-Wilks W = 0.433, p < 0.0001 wet; W = 0.363, p < 0.0001) and the distribution of particles between the different segments of the GI tract were not significantly different from each other (χ = 0.4371, df = 4, p = 0.9793 wet; χ = 1.9237, df = 4, p = 0.7498 dry).
Discussion
Overall it is clear that manatees in Tampa Bay routinely ingested plastic during our study period, as 76.5% of individuals sampled contained plastic in their GI tract. Although ingestion of plastic is rarely the leading cause of death in manatees, it can be a contributing factor in some mortalities (Beck and Barros, 1991; Reinert et al., 2017). While this is the first study to investigate microplastic ingestion by manatees, necropsies routinely examine the gastrointestinal tract for marine debris, much of which is plastic. Beck and Barros (1991) found 14.4% of manatees examined between 1978 and 1986 had ingested debris. Between 1993 and 2012, 9.7% of all manatee carcasses (598/6561) contained marine debris, however in just the last five years of the study (2008–2012) 19.7% of the carcasses contained marine debris (Reinert et al., 2017). While our study only examined 26 animals, 26.9% of the carcasses examined contained marine debris, all of which was plastic. Differences in detection rate during gross necropsy, for example increased awareness by prosectors, may also explain part of the increase over the years.
As all the manatees in this study were collected in Tampa Bay, it is possible that manatees in our samples were exposed to higher levels of plastic contamination as the Gulf of Mexico has been recorded to have some of the highest microplastic concentrations reported (Shruti et al., 2021). However, the previous study that included manatees from across Florida did not suggest that Tampa Bay was a hot spot for debris ingestion by manatees (Reinert et al., 2017) nor were concentrations of microplastics in Tampa Bay particularly high (McEachern et al., 2019).
In addition to the seven manatees which contained macroplastic detected during gross necropsies, our study documented an additional 13 manatees containing plastics in their GI tract; and six manatees contained plastics identified by both methods. This suggests that gross necropsy procedures do not identify all plastic pieces ingested by manatees, previous rates of plastic ingestion by manatees underrepresent the high proportion of individuals with plastic in their GI tract and manatees may be routinely ingesting many different plastics. Careful examination of the plastic pieces found in these six manatees, suggests that most of these manatees had ingested several different plastic items. For example, during the necropsy of manatee MNW18132 the Marine Mammal Pathology lab identified a piece of clear string in the colon, while we identified part of a black plastic bag in the distal colon. In contrast, MNW20012 had a large piece of clear monofilament line in its distal colon (found during the necropsy) as well as a smaller piece of monofilament line which could have broken down from the larger piece. At this point, it is not possible to determine if manatee digestive processes including grinding in the mouth are contributing to the further breakdown of plastics, as has been suggested in other organisms (Pérez-Guevara et al., 2021).
Monofilament fishing line, including recreational fishing gear, was the most common macroplastic ingested by manatees (Beck and Barros, 1991; Reinert et al., 2017) and entanglement and ingestion of fishing gear including monofilament line were the most common form of fisheries interactions in manatees (Adimey et al., 2014). Monofilament fishing line was also the most common type of plastic found during necropsies (9/12 pieces; 75%) and during the microplastic detection process (11/48 pieces; 23%). Thus, fishing gear continues to pose a threat to manatees in Florida.
Plastic pieces >1 mm may have been present in at least some of the six individuals in our study in which we did not detect plastic, as we only sub-sampled a small portion of the entire GI contents. Examining a greater proportion of the GI tract would more accurately reflect ingestion rates; however, this process is very labor intensive. Better methods to digest cellulose would be helpful. Hurley et al. (2018) and Egea-Corbacho et al. (2022) have some suggestions but these methods are likely still too complicated, toxic and expensive to deal with the large quantities of cellulose-rich food manatees ingest.
Studies on microplastic ingestion by fish suggest that sample sizes below 10 are unreliable for frequency of observation of microplastic ingestion, especially when relying only on visual detection of plastic particles from gut contents (Markic et al., 2020). This suggests that our sample of 26 individuals, while still small, likely resulted in useful representation of the frequency of observation of microplastic ingestion by manatees in Tampa Bay, however our sample size prevented detailed analysis of other factors such as age, sex or cause of death. A review of microplastic ingestion by marine fishes indicated that about one-third of all individual fish (regardless of species) had ingested plastic, and on average, each fish contained about two plastic pieces (Markic et al., 2020). In our study, a much greater proportion of individuals contained plastic in their GI tract and although we estimated 2.3 plastic pieces per manatee, this is likely an underestimate as we only sub-sampled a small portion of the GI tract. If we had been able to use chemical digestion, filtration and microscopic identification as suggested by Savoca et al. (2021), we would likely have identified many more plastic pieces.
While it is challenging to calculate plastic ingestion rates for manatees, we can calculate a rough ingestion rate based on plastic pieces found in manatee stomachs as this region represents the least processed material. A 500 kg manatee consumes approximately 35 kg of vegetation per day (assuming manatees consume 7% of their body weight per day; Bengtson, 1983; Worthy and Worthy, 2014). Therefore, if we scale the average concentration of 5 plastic pieces/kg wet material examined (Table 2) up to 35 kg of ingested material, it suggests that manatees may be ingesting upwards of 175 microplastic pieces per day. The physiological implications of this ingestion rate are currently unknown for manatees; however, work on several species of sea turtles indicated that greater amounts of plastic ingestion lead to higher risks of mortality (Wilcox et al., 2018).
Plastic ingestion by marine herbivores has not been well studied, and of the herbivores studied, most are fish grazing on algae. Marine herbivorous fish likely ingest plastic particles adhered to vegetation (Cardozo-Ferreira et al., 2021) and have lower rates of plastic ingestion than fish with other foraging strategies (Savoca et al., 2021). Benthic foragers also have relatively high rates of plastic ingestion (Savoca et al., 2021). Manatees in Tampa Bay predominately forage on seagrass, but also often contain sand, shells and benthic invertebrates in their GI tract (Reich and Worthy, 2006) and thus their plastic ingestion rates may be more similar to benthic foragers than marine herbivores.
While there was no clear indication of a concentration of microplastic pieces through the GI tract (Table 2), manatees concentrate forage materials between the stomach and fecal matter which suggests that manatees have the potential to concentrate environmental plastic in their feces. Microplastics in fecal matter is an emerging field of study, with examination of concentrations as well as the potential ecological implications of microplastic-laden feces (Pérez-Guevara et al., 2021). Fecal matter is clearly a vector of microplastics throughout the environment and the role of coprophagy in further transfer of microplastics in the food web deserves further attention (see Pérez-Guevara et al., 2021 for a review).
Conclusion
While it is challenging to identify microplastic pieces from the GI tracts of large herbivores such as manatees, it is clear from this study that manatees are ingesting microplastic in Tampa Bay and likely at fairly high rates. It is likely that all sirenian species are exposed to environmental microplastics which can be ingested while foraging. Further work is needed to assess the potential physiological impacts of microplastic consumption by sirenians as well as the role marine herbivores may play in concentrating environmental plastics into feces for trophic transfer up the food web.
Data availability statement
The original contributions presented in the study are included in the article/Supplementary material, further inquiries can be directed to the corresponding authors.
Ethics statement
Ethical review and approval was not required for the animal study because this research involved diseased animals, not killed for this study. Gross necropsies were conducted by the Florida Fish and Wildlife, Marine Mammal Pathobiology Laboratory and were conducted by authorized personnel in accordance with section 6 of the Endangered Species Act [implementing regulations 50 CFR 17.21(c) and 50 CFR 17.31(b)] and section 109(h) of the Marine Mammal Protection Act (implementing regulation 50 CFR 18.22). The authors were only involved in analysis of the gut content material provided by the Marine Mammal Pathobiology Laboratory.
Author contributions
SG and ANSS directed the research and oversaw the project. SG developed the laboratory methods and drafted the manuscript. All authors contributed to the article and approved the submitted version.
Funding
This work was funded through two grants to SG and ANSS from the Tampa Bay Environmental Restoration Fund and additional grant from the Eckerd College Natural Sciences Summer Research Program.
Acknowledgments
The authors would like to thank Martine de Wit for sample collection and feedback on an early version of the manuscript as well as everyone else at the Florida Fish and Wildlife Marine Mammal Pathobiology Lab for their assistance with the manatee necropsies and procuring our samples. Marine Mammal Protection Act and Endangered Species Permits were not required as materials from within the GI tract are not considered to be a part of the animal. Eckerd College students Sabrina Sorace, Shane Field and Shannon Day were instrumental in setting up the protocols to detect microplastics and for their efforts in identifying microplastic particles in manatee guts.
Conflict of interest
The authors declare that the research was conducted in the absence of any commercial or financial relationships that could be construed as a potential conflict of interest.
Publisher’s note
All claims expressed in this article are solely those of the authors and do not necessarily represent those of their affiliated organizations, or those of the publisher, the editors and the reviewers. Any product that may be evaluated in this article, or claim that may be made by its manufacturer, is not guaranteed or endorsed by the publisher.
Supplementary material
The Supplementary material for this article can be found online at: https://www.frontiersin.org/articles/10.3389/fevo.2023.1143310/full#supplementary-material
Footnotes
1. ^https://www.federalregister.gov/documents/2017/04/05/2017-06657/endangered-and-threatened-wildlife-and-plants-reclassification-of-the-west-indian-manatee-from
2. ^https://www.iucnredlist.org/species/22106/9359881
3. ^https://mission.cmaquarium.org/app/uploads/2021/05/Sirenews-73-April2021-update.pdf
References
Adimey, N. M., Hudak, C. A., Powell, J. R., Bassos-Hull, K., Foley, A., Farmer, N. A., et al. (2014). Fishery gear interactions from stranded bottlenose dolphins, Florida manatees and sea turtles in Florida, USA. Mar. Poll. Bull. 81, 103–115. doi: 10.1016/j.marpolbul.2014.02.008
Arthur, C., Baker, J., and Bamford, H. E. (2009). Proceedings of the International Research Workshop on the Occurrence, Effects and Fate of Microplastic Marine Debris. University of Washington Tacoma, Tacoma, WA, Unites States.
Attademo, F. L. N., Balensiefer, D. C., da Bôaviagem Freire, A. C., de Sousa, G. P., da Cunha, F. A. G. C., and de Oliveira Luna, F. (2015). Debris ingestion by the Antillean manatee (Trichechus manatus manatus). Mar. Poll. Bull. 101, 284–287. doi: 10.1016/j.marpolbul.2015.09.040
Baalkhuyur, F. M., Dohaish, E. J. A. B., Elhalwagy, M. E., Alikunhi, N. M., AlSuwailem, A. M., Røstad, A., et al. (2018). Microplastic in the gastrointestinal tract of fishes along the Saudi Arabian Red Sea coast. Mar. Poll. Bull. 131, 407–415. doi: 10.1016/j.marpolbul.2018.04.040
Baulch, S., and Perry, C. (2014). Evaluating the impacts of marine debris on cetaceans. Mar. Poll. Bull. 80, 210–221. doi: 10.1016/j.marpolbul.2013.12.050
Beck, C. A., and Barros, N. B. (1991). The impact of debris on the Florida manatee. Mar. Pollut. Bull. 22, 508–510. doi: 10.1016/0025-326X(91)90406-I
Bengtson, J. L. (1983). Estimating food consumption of free-ranging manatees in Florida. J. Wildlife Manage. 47, 1186–1192. doi: 10.2307/3808190
Bonde, R. K., O’Shea, T. J., and Beck, C. A. (1983). Manual of Procedures for the Salvage and Necropsy of Carcasses of the West Indian Manatee (Trichechus manatus). Springfield, VA: National Technical Information Service.
Boshoff, B. J., Robinson, T. B., and von der Heyden, S. (2023). The role of seagrass meadows in the accumulation of microplastics: insights from a south African estuary. Mar. Poll. Bull. 186:114403. doi: 10.1016/j.marpolbul.2022.114403
Bucci, K., Tulio, M., and Rochman, C. M. (2020). What is known and unknown about the effects of plastic pollution: A meta-analysis and systematic review. Ecol. Appl. 30:e02044. doi: 10.1002/eap.2044
Butterworth, A. (2016). A review of the welfare impact on pinnipeds of plastic marine debris. Front. Mar. Sci. 3:00149. doi: 10.3389/fmars.2016.00149
Cabansag, J. B. P., Olimberio, R. B., and Villanobos, Z. M. T. (2021). Microplastics in some fish species and their environs in eastern Visayas. Philippines. Mar. Poll. Bull. 167:112312. doi: 10.1016/j.marpolbul.2021.112312
Cardozo-Ferreira, G. C., Calazans, T. L., Benevides, L. J., Luiz, O. J., Ferreira, C. E., and Joyeux, J. C. (2021). Ecological traits influencing anthropogenic debris ingestion by herbivorous reef fishes. Front. Mar. Sci. 8:717435. doi: 10.3389/fmars.2021.717435
Caron, A. G., Thomas, C. R., Berry, K. L., Motti, C. A., Ariel, E., and Brodie, J. E. (2018). Validation of an optimised protocol for quantification of microplastics in heterogenous samples: a case study using green turtle chyme. MethodsX 5, 812–823. doi: 10.1016/j.mex.2018.07.009
Cozzolino, L., Nicastro, K. R., Zardi, G. I., and Carmen, B. (2020). Species-specific plastic accumulation in the sediment and canopy of coastal vegetated habitats. Sci. Total Environ. 723:138018. doi: 10.1016/j.scitotenv.2020.138018
Datu, S. S., Supriadi, S., and Tahir, A. (2019). Microplastic in Cymodocea rotundata seagrass blades. IJEAB. 4, 1758–1761. doi: 10.22161/ijeab.46.21
Dehaut, A., Hermabessiere, L., and Duflos, G. (2019). Current frontiers and recommendations for the study of microplastics in seafood. TRAC 116, 346–359. doi: 10.1016/j.trac.2018.11.011
Duncan, E. M., Broderick, A. C., Fuller, W. J., Galloway, T. S., Godfrey, M. H., Hamann, M., et al. (2019). Microplastic ingestion ubiquitous in marine turtles. Glob. Chang. Biol. 25, 744–752. doi: 10.1111/gcb.14519
Egea-Corbacho, A., Martín-García, A. P., Franco, A. A., Albendín, G., Arellano, J. M., Rodríguez, R., et al. (2022). A method to remove cellulose from rich organic samples to analyse microplastics. J. Clean. Prod. 334:130248. doi: 10.1016/j.jclepro.2021.130248
Enders, K., Lenz, R., Beer, S., and Stedmon, C. A. (2017). Extraction of microplastic from biota: recommended acidic digestion destroys common plastic polymers. ICES J. Mar. Sci. 74, 326–331. doi: 10.1093/icesjms/fsw173
Fibbe, M. C., Carroll, D., Gowans, S., and Siuda, A. N. S. (2023). Ingestion of microplastics by copepods in Tampa Bay Estuary, FL. Front. Ecol. Evol. 11:1143377. doi: 10.3389/fevo.2023.1143377
Gall, S. C., and Thompson, R. C. (2015). The impact of debris on marine life. Mar. Poll. Bull. 92, 170–179. doi: 10.1016/j.marpolbul.2014.12.041
Goss, H., Jaskiel, J., and Rotjan, R. (2018). Thalassia testudinum as a potential vector for incorporating microplastics into benthic marine food webs. Mar. Poll. Bull. 135, 1085–1089. doi: 10.1016/j.marpolbul.2018.08.024
Guterres-Pazin, M. G., Rosas, F. C. W., and Marmontel, M. (2012). Short Note: Ingestion of Invertebrates, Seeds, and Plastic by the Amazonian Manatee (Trichechus inunguis) (Mammalia, Sirenia). Aqua. Mammal. 38, 322–324. doi: 10.1578/AM.38.3.2012.322
Huang, Y., Xiao, X., Effiong, K., Xu, C., Su, Z., Hu, J., et al. (2021). New insights into the microplastic enrichment in the blue carbon ecosystem: evidence from seagrass meadows and mangrove forests in coastal South China Sea. Environ. Sci. Technol. 55, 4804–4812. doi: 10.1021/acs.est.0c07289
Huang, Y., Xiao, X., Xu, C., Perianen, Y. D., Hu, J., and Holmer, M. (2020). Seagrass beds acting as a trap of microplastics-emerging hotspot in the coastal region? Environ. Pollut. 257:113450. doi: 10.1016/j.envpol.2019.113450
Hurley, R. R., Lusher, A. L., Olsen, M., and Nizzetto, L. (2018). Validation of a method for extracting microplastics from complex, organic-rich, environmental matrices. Ea. Environ. Sci. Technol. 52, 7409–7417. doi: 10.1021/acs.est.8b01517
ICES (2015). “ICES special request advice Northeast Atlantic and Arctic Ocean” in OSPAR request on development of a common monitoring protocol for plastic particles in fish stomachs and selected shellfish on the basis of existing fish disease surveys. ICES Advice 2015, Book 1 (June), 1–6.
Jones, K. L., Hartl, M. G., Bell, M. C., and Capper, A. (2020). Microplastic accumulation in a Zostera marina L. bed at Deerness sound, Orkney, Scotland. Mar. Poll. Bull. :152:110883. doi: 10.1016/j.marpolbul.2020.110883
Katlam, G., Prasad, S., Pande, A., and Ramchiary, N. (2022). Plastic ingestion in Asian elephants in the forested landscapes of Uttarakhand. India. J. Nat. Conserv. 68:126196. doi: 10.1016/j.jnc.2022.126196
Kreitsberg, R., Raudna-Kristoffersen, M., Heinlaan, M., Ward, R., Visnapuu, M., Kisand, V., et al. (2021). Seagrass beds reveal high abundance of microplastic in sediments: a case study in the Baltic Sea. Mar. Poll. Bull. 168:112417. doi: 10.1016/j.marpolbul.2021.112417
Laist, D. W. (1987). Overview of the biological effects of lost and discarded plastic debris in the marine environment. Mar. Poll. Bull. 18, 319–326. doi: 10.1016/S0025-326X(87)80019-X
Landsberg, J. H., Tabuchi, M., Rotstein, D. S., Subramaniam, K., Rodrigues, T., Waltzek, T. B., et al. (2022). Novel lethal Clostridial infection in Florida manatees (Trichechus manatus latirostris): cause of the 2013 unusual mortality event in the Indian River lagoon. Front. Mar. Sci. 9:195. doi: 10.3389/fmars.2022.841857
Larkin, I. L., Fowler, V. F., and Reep, R. L. (2007). Digesta passage rates in the Florida manatee (Trichechus manatus latirostris). Zoo Biol. 26, 503–515. doi: 10.1002/zoo.20150
Maes, T., Jessop, R., Wellner, N., Haupt, K., and Mayes, A. G. (2017). A rapid-screening approach to detect and quantify microplastics based on fluorescent tagging with Nile red. Sci. Rep. 7, 1–10. doi: 10.1038/srep44501
Markic, A., Gaertner, J. C., Gaertner-Mazouni, N., and Koelmans, A. A. (2020). Plastic ingestion by marine fish in the wild. Crit. Rev. Environ. Sci. Technol. 50, 657–697. doi: 10.1080/10643389.2019.1631990
McEachern, K., Alegria, H., Kalagher, A. L., Hansen, C., Morrison, S., and Hastings, D. (2019). Microplastics in Tampa Bay, Florida: abundance and variability in estuarine waters and sediments. Mar. Poll. Bull. 148, 97–106. doi: 10.1016/j.marpolbul.2019.07.068
Pérez-Guevara, F., Kutralam-Muniasamy, G., and Shruti, V. C. (2021). Critical review on microplastics in fecal matter: research progress, analytical methods and future outlook. Sci. Total Environ. 778:146395. doi: 10.1016/j.scitotenv.2021.146395
Plee, T. A., and Pomory, C. M. (2020). Microplastics in sandy environments in the Florida keys and the panhandle of Florida, and the ingestion by sea cucumbers (Echinodermata: Holothuroidea) and sand dollars (Echinodermata: Echinoidea). Mar. Poll. Bull. 158:111437. doi: 10.1016/j.marpolbul.2020.111437
Reich, K. J., and Worthy, G. A. (2006). An isotopic assessment of the feeding habits of free-ranging manatees. Mar. Ecol. Prog. 322, 303–309. doi: 10.3354/meps322303
Reinert, T. R., Spellman, A. C., and Bassett, B. L. (2017). Entanglement in and ingestion of fishing gear and other marine debris by Florida manatees, 1993 to 2012. Endanger. Species Res. 32, 415–427. doi: 10.3354/esr00816
Reynolds, J. E. III, and Rommel, S. A. (1996). Structure and function of the gastrointestinal tract of the Florida manatee, Trichechus manatus latirostris. Anat. Rec. 245, 539–558. doi: 10.1002/(SICI)1097-0185(199607)245:3<539::AID-AR11>3.0.CO;2-Q
Runge, M. C., Sanders-Reed, C. A., Langtimm, C. A., Hostetler, J. A., Martin, J., Deutsch, C. J., et al. (2017). Status and Threats Analysis for the Florida Manatee (Trichechus manatus latirostris) 2016. Scientific Investigation Report 2017–5030. Reston: VA. United States Geological Survey.
Saliu, F., Montano, S., Leoni, B., Lasagni, M., and Galli, P. (2019). Microplastics as a threat to coral reef environments: detection of phthalate esters in neuston and scleractinian corals from the Faafu atoll, Maldives. Mar. Poll. Bull. 142, 234–241. doi: 10.1016/j.marpolbul.2019.03.043
Savoca, M. S., McInturf, A. G., and Hazen, E. L. (2021). Plastic ingestion by marine fish is widespread and increasing. Glob. Chang. Biol. 27, 2188–2199. doi: 10.1111/gcb.15533
Sawalman, R., Werorilangi, S., Ukkas, M., Mashoreng, S., Yasir, I., and Tahir, A. (2021). Microplastic abundance in sea urchins (Diadema setosum) from seagrass beds of Barranglompo Island, Makassar, Indonesia. IOP Conf. Ser. Earth Environ. Sci. 763:012057. doi: 10.1088/1755-1315/763/1/012057
Sbrana, A., Valente, T., Scacco, U., Bianchi, J., Silvestri, C., Palazzo, L., et al. (2020). Spatial variability and influence of biological parameters on microplastic ingestion by Boops boops (L.) along the Italian coasts (Western Mediterranean Sea). Environ. Pollut. 263:114429. doi: 10.1016/j.envpol.2020.114429
Schneider, C. A., Rasband, W. S., and Eliceiri, K. W. (2012). NIH image to ImageJ: 25 years of image analysis. Nat. Methods 9, 671–675. doi: 10.1038/nmeth.2089
Seng, N., Lai, S., Fong, J., Saleh, M. F., Cheng, C., Cheok, Z. Y., et al. (2020). Early evidence of microplastics on seagrass and macroalgae. Mar. Freshw. Res. 71, 922–928. doi: 10.1071/MF19177
Shruti, V. C., Pérez-Guevara, F., and Kutralam-Muniasamy, G. (2021). The current state of microplastic pollution in the world’s largest gulf and its future directions. Environ. Pollut. 291:118142. doi: 10.1016/j.envpol.2021.118142
Simmonds, M. P. (2012). Cetaceans and marine debris: the great unknown. J. Mar. Biol. 2012, 1–8. doi: 10.1155/2012/684279
Tahir, A., Samawi, M. F., Sari, K., Hidayat, R., Nimzet, R., Wicaksono, E. A., et al. (2019). Studies on microplastic contamination in seagrass beds at Spermonde archipelago of Makassar Strait, Indonesia. JPCS 1341:022008. doi: 10.1088/1742-6596/1341/2/022008
Unsworth, R. K., Higgs, A., Walter, B., Cullen-Unsworth, L. C., Inman, I., and Jones, B. L. (2021). Canopy accumulation: are seagrass meadows a sink of microplastics? Oceans 2, 162–178. doi: 10.3390/oceans2010010
Wilcox, C., Puckridge, M., Schuyler, Q. A., Townsend, K., and Hardesty, B. D. (2018). A quantitative analysis linking sea turtle mortality and plastic debris ingestion. Sci. Rep. 8, 1–11. doi: 10.1038/s41598-018-30038-z
Worthy, G. A., and Worthy, T. A. (2014). Digestive efficiencies of ex situ and in situ west Indian manatees (Trichechus manatus latirostris). Physiol. Biochem. Zool. 87, 77–91. doi: 10.1086/673545
Wright, L. S., Napper, I. E., and Thompson, R. C. (2021). Potential microplastic release from beached fishing gear in Great Britain’s region of highest fishing litter density. Mar. Poll. Bull. 173:113115. doi: 10.1016/j.marpolbul.2021.113115
Keywords: manatee, microplastic, seagrass, estuary, ingestion
Citation: Gowans S and Siuda ANS (2023) Microplastics in large marine herbivores: Florida manatees (Trichechus manatus latirostris) in Tampa Bay. Front. Ecol. Evol. 11:1143310. doi: 10.3389/fevo.2023.1143310
Edited by:
Michael C. Murrell, University of West Florida, United StatesReviewed by:
Francesco Tiralongo, University of Catania, ItalyElizabeth E. Hieb, Dauphin Island Sea Lab, United States
Copyright © 2023 Gowans and Siuda. This is an open-access article distributed under the terms of the Creative Commons Attribution License (CC BY). The use, distribution or reproduction in other forums is permitted, provided the original author(s) and the copyright owner(s) are credited and that the original publication in this journal is cited, in accordance with accepted academic practice. No use, distribution or reproduction is permitted which does not comply with these terms.
*Correspondence: Shannon Gowans, Z293YW5zc0BlY2tlcmQuZWR1; Amy N. S. Siuda, c2l1ZGFhbkBlY2tlcmQuZWR1