- 1College of Animal Science (College of Bee Science), Fujian Agriculture and Forestry University, Fuzhou, Fujian, China
- 2Honeybee Research Institute, Fujian Agriculture and Forestry University, Fuzhou, Fujian, China
- 3Institute of Apicultural Research, Chinese Academy of Agricultural Sciences, Beijing, China
- 4Guangxi Key Laboratory of Translational Medicine for Treating High-Incidence Infectious Diseases With Integrative Medicine, Guangxi University of Chinese Medicine, Nanning, China
- 5College of Oceanology and Food Science, Quanzhou Normal University, Quanzhou, Fujian, China
Whether the development of honeybee broods is healthy or not determines the productivity of bee colonies. Pupation is a critical period in the development of holometabolous insects, characterized by the transition from larva to pupa, and its sensitivity to high temperature was investigated in Apis cerana worker bees. Mature larvae (ML), the first and second days of prepupa (PP1 and PP2), and the first day of pupa (P1) were exposed to 40°C for varied durations of time. The mortality, development duration, birth weight, size of the body, and appendages of eclosed Apis cerana worker bees were measured. Results showed that PP1 had the highest mortality, the lowest birth weight, and the longest development duration among the pupation stages. When exposed to 40°C for 12 h and 16 h, more than 28 and 84% of PP1 failed to complete development, respectively. Additionally, high-temperature treatment had a significant effect on the length of the proboscis, the size of the forewing, and the size of the hind leg. These findings suggest that ML and PP1 are crucial checkpoints for wing and appendage (proboscis and legs) development and provide insights into the mechanisms of honeybee brood susceptibility to high-temperature stress in the context of global warming.
1. Introduction
Honeybees are incredibly proficient at transferring pollen and provide essential pollination services for both cultivated and wild plants globally. However, their exposure to abiotic and biotic stressors has caused severe health issues in honeybees, which has received much attention in the context of global warming (Le Conte and Navajas, 2008; Rader et al., 2013; Abou-Shaara et al., 2017). Temperature is one of the abiotic factors influencing the growth and development of honeybees and other ectotherms (Sun et al., 2021).
Although honeybee colonies have adapted to a wide range of temperatures, their normal development relies on the nest temperature being regulated by the mass of adults (Kronenberg and Heller, 1982; Heinrich, 1993). The temperature of the brood area in the nest is typically maintained close to a constant (35°C), indicating that honeybee brood is sensitive to temperature fluctuations (Kleinhenz et al., 2003; Stabentheiner et al., 2010; Zhou et al., 2015). Heat waves or artificial mite-killing hyperthermia in colonies can have lethal consequences for immature honeybees, even if only exposed to high temperatures for a short period (Medina et al., 2018; Kablau et al., 2020).
Honeybee broods are susceptible to thermal stress. The capped period (last instar, prepupa, and pupa) rearing at suboptimal temperatures has been shown to have detrimental effects on adult honeybees. Studies have demonstrated that such thermal stress during the capped period can impair mushroom body development, learning and memory abilities (Tautz et al., 2003; Groh et al., 2004; Jones et al., 2005), behaviors (Becher et al., 2009), and wing morphology (Tan et al., 2005; Szentgyörgyi et al., 2018) in the resultant adult bees. Short-term heat waves of 40°C or higher have been linked to increased fluctuating asymmetry in forewing shape or abnormal venation reduced longevity and decreased age at the onset of foraging, body size and sucrose responsiveness, and male fertility in the resultant adult bees (Medina et al., 2018; Zhu et al., 2018; Kablau et al., 2020). Additionally, suboptimal brood-rearing temperatures have been found to reduce the adult bees' ability to resist external environmental pressures and disease (Flores et al., 1996; McMullan and Brown, 2005; Medrzycki et al., 2010).
The internal structures of holometabolous insects experience drastic changes during the larva-pupa transition, implying that the prepupae are more susceptible to stress than other developmental stages (Wang et al., 2016; Kablau et al., 2020). To ascertain the sensitivity of the early capped broods of indigenous Apis cerana to high temperatures that undergo metamorphosis and how heat exposure affects their developmental characteristics and morphology traits, these broods were exposed to a high temperature of 40°C for different periods of time. In this study, we answer the following questions: (1) Which stage, from mature larva to new pupa, is more sensitive to high temperature? (2) To what extent does high temperature affect the development process and morphological traits?
2. Materials and methods
2.1. Honeybee brood and high-temperature treatment
An experiment was conducted in the spring season in Fuzhou, Southeast China, with three healthy colonies of Apis cerana with strong populations. To ensure the eggs were of the same age, each queen was confined in a mesh cage to lay eggs on a new comb. After 8.5 days, the larvae were monitored until they were capped within 4 h, after which they were cut out with a comb patch and transferred into the incubators immediately (recorded as start time, 35°C ± 0.2°C, relative humidity 75 ± 5%). The control group was maintained at 35°C ± 0.2°C until eclosion. The comb patches incubated for 1 day (mature larvae, ML), 2 days (prepupa, PP1), 3 days (prepupa, PP2), and 4 days (pupa, P1) were transferred to high temperature (40°C ± 0.2°C) for 16 h before being returned to 35°C until eclosion. The survival rates were recorded to determine which stage was more vulnerable to high temperatures.
After identifying the sensitive stage, the heat time effect on its development parameters was further investigated. The honeybees in the PP1 stage, being the most sensitive, were exposed to 40°C for 8 h, 12 h, and 16 h, before it was recovered to 35°C until emergence. The newly capped broods reared at 35°C until emergence were used as the control group. A triplicate of newly capped brood patches (>22 capped brood) from three colonies per treatment or control group simultaneously were harvested to reduce the influence of factors such as heredity and nutrition on the results.
2.2. Mortality, birth weight, and development duration measurement
Upon the emergence of the capped brood, the comb was inspected every hour, and the number of eclosed bees was recorded as the birth weight. An electronic balance (±0.1 mg, Sartorius, Germany) was used to weigh each bee immediately. If the capped cells did not emerge within 15 days post-capping, they were deemed dead (which is 3 days longer than the usual eclosion period). The development duration of each honeybee since the start time was also recorded. All treatment groups and control groups were repeated with three colonies.
2.3. Morphology measurement
Dissections and mounting of the proboscis, right forewing, and the right hind leg of eclosed bees (20 bees in each colony, 3 colonies per group, except for the 20 workers analyzed in PP1 that had high mortality upon eclosion) onto slides under a stereoscope were conducted. Subsequently, six appendage characteristics, including proboscis length, forewing length, forewing width, femur length, tibia length, and metatarsus length of each bee, were measured according to the methods outlined by Ruttner (1988) and Zhu et al. (2017).
2.4. Statistical analysis
Mortality data between treated groups were examined using one-way ANOVA after the transformation of arcsin (square root) of the mortality rates (SNK, Wang et al., 2016). Additionally, a principal component analysis (PCA) was conducted to determine parameters of overall body sizes with 17 morphological traits measured on individual bees. The correlation matrix procedure was selected as the resulting principal component 1 (PC1) was found to be more closely related to body size (Poot-Báez et al., 2019). The coefficients for each PC were then used to calculate scores as individual measures of appendage sizes. The PCA was completed using the R packages FactoMineR (Lê et al., 2008). A one-way ANOVA was also used to compare the variance of the development duration, birth weight, and morphological characteristics between treatments, with a post-hoc analysis conducted using the Student–Newman-Keuls tests (SPSS 21.0). Data were represented as means ± standard deviations.
3. Results
3.1. Mortality, birth weight, and development duration
The early prepupal honeybee (PP1) was found to be the most sensitive to high-temperature stress among the test groups during the larva-pupa transition. When exposed to 16 h at 40°C, the PP1 group showed an average mortality rate of 84.94%±8.49%, significantly higher (P < 0.01, Figure 1A) than the control, ML, PP2, and P1 groups, which had < 5% mortality and no difference between them (P > 0.05, Figure 1A).
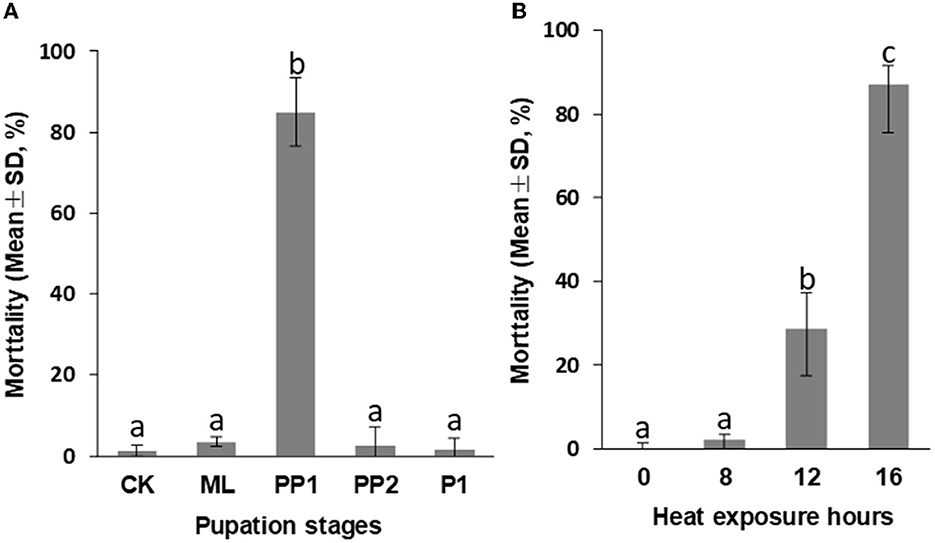
Figure 1. Mortality of honeybee broods exposed to 40°C for 16 h (A) and PP1 exposed to 40°C for 0 (CK), 8, 12, 16 h (B). CK, control group; ML, mature larva; PP1, the first day of prepupa; PP2, the second day of prepupa; P1, the first day of the pupa. The different letters in the lowercase indicate a significant difference at the P < 0.01 level.
In comparison with the control group (92.38 ± 9.19 mg), ML (88.96 ± 5.87 mg), PP2 (88.72 ± 6.10 mg), and P1 (89.08 ± 7.01 mg) groups, the PP1 group (84.30 ± 4.46 mg) significantly reduced birth weight and increased development duration (P < 0.01, Figure 2). The ML, PP2, and P1 groups showed no difference in birth weight with the control group (P > 0.05), but the PP1 group decreased its birth weight significantly (P < 0.01) under the same condition. The development durations in the ML, PP1, and P1 groups, excluding PP2, increased significantly (P < 0.01, Figure 1B) compared to the control group. These results suggest that the PP1 stage is the most vulnerable to thermal stress, which delays the rate of honeybee pupation and post-stress development.
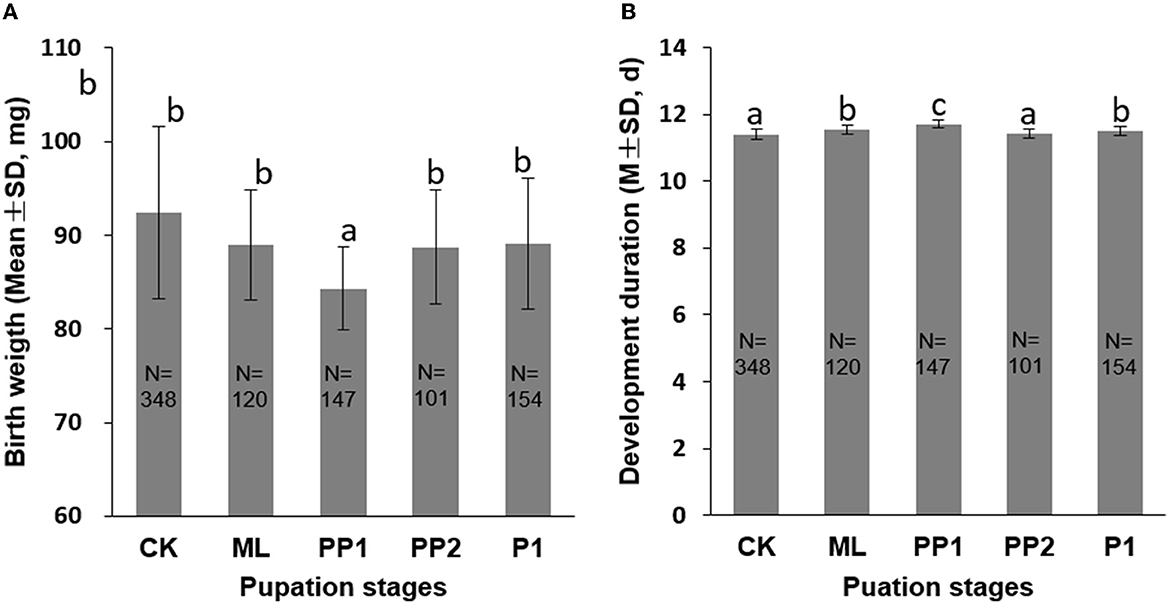
Figure 2. Birth weight (A) and development duration (B) of honeybee broods exposed to 40°C for 16 h. CK, control group; ML, mature larva; PP1, the first day of prepupa; PP2, the second day of prepupa; P1, the first day of the pupa. The different letters in the lowercase indicate a significant difference at the P < 0.01 level. The numbers in the columns indicate sample sizes.
Furthermore, we observed a significant increase in PP1 mortality rates as heat exposure time was prolonged. An increase in heat exposure from 8 h to 16 h resulted in a mortality rate increase from 2.17 to 87.08% (P < 0.01, Figure 1B), a decrease in birth weight from 95.40 mg (8 h exposure) to 84.30 mg (16 h exposure; P < 0.01, Figure 3A), and an extension of development duration from 11.48 days to 11.71 days, significantly (P < 0.01; Figure 3B). Additionally, an 8-h exposure to 40°C did not increase mortality or birth weight but did increase the development duration compared to the control group, suggesting an 8-h heat exposure may cause subtle damage to the honeybee development.
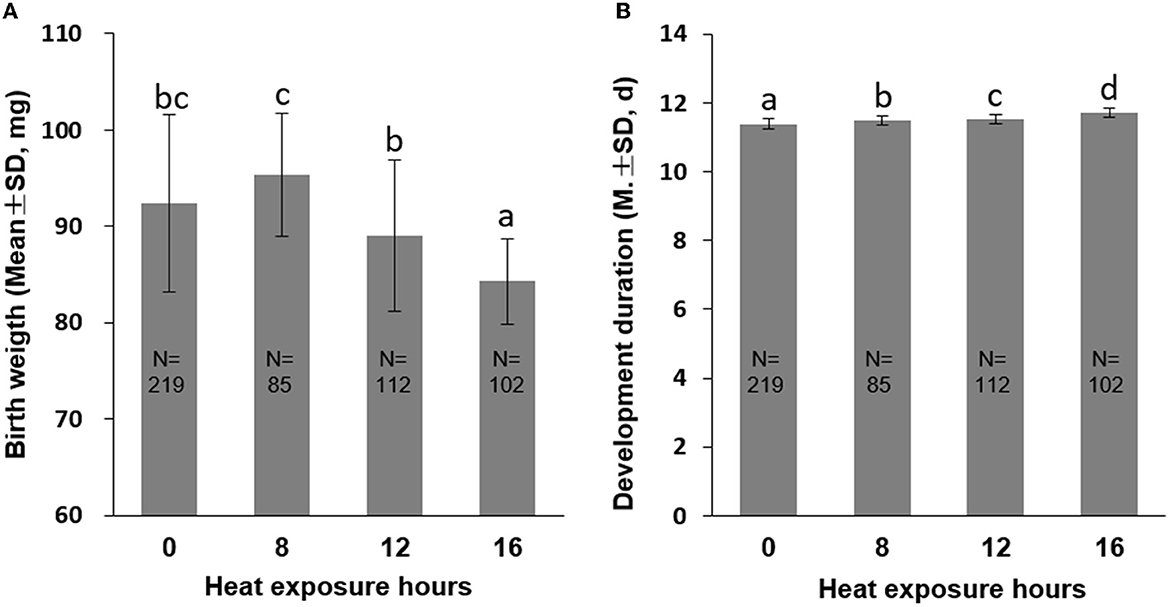
Figure 3. Birth weight (A) and development duration (B) of honeybee brood PP1 exposed to 40°C for 0 (CK), 8, 12, and 16 h. The different letters in lowercase indicate a significant difference at the P < 0.01 level.
3.2. Wing and appendage size
Exposure to high temperatures for 16 h significantly reduced the proboscis length of eclosed bees in the ML, PP1, and PP2 groups (P < 0.01, Figure 4) compared to the CK group. The PP1 bees had the shortest proboscis length, followed by the ML and PP2 groups. However, no significant decrease was observed in the P1 group (P > 0.05; Supplementary Table 5). The forewing size decreased significantly in all treatment groups, with the ML group showing the smallest wing length and width, followed by the PP2, PP1, and P1 groups (P < 0.01, Figure 4). High-temperature stress on prepupal stages (PP1 and PP2) had the most significant impact on the hind leg size (P < 0.01, Figure 4), followed by the ML group, while there was no impact on the hind leg size of the P1 group in terms of the femur and metatarsus length (P > 0.05, Figure 4).
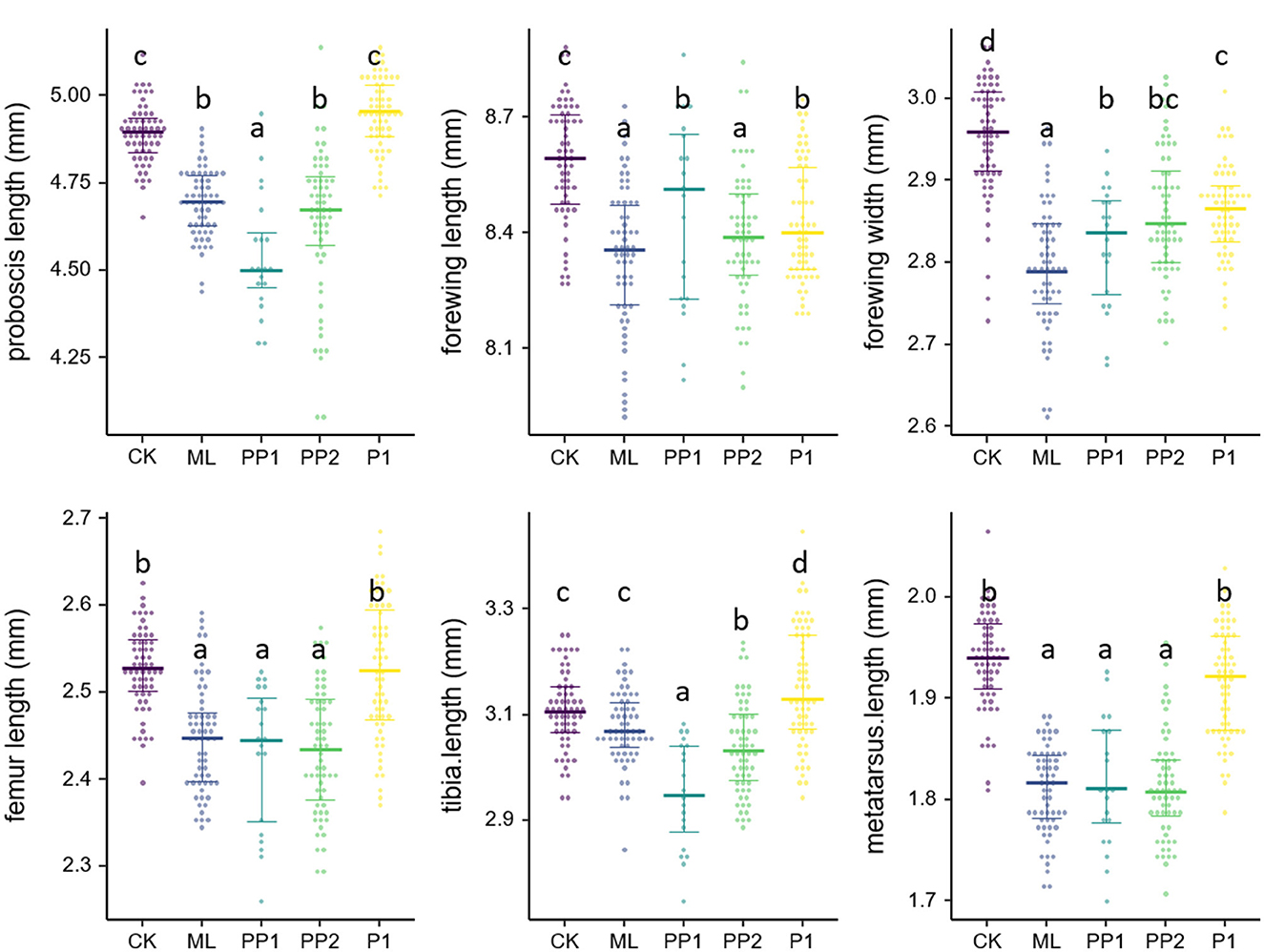
Figure 4. Wing size and appendage size of honeybee broods exposed to 40°C for 16 h. Colored dots represent experiment groups: CK, control group. ML, mature larva; PP1, the first day of prepupa; PP2, the second day of prepupa; P1, the first day of the pupa. Crossbars with whiskers in each group indicate the median value and the 25th and 75th percentiles.
After 8, 12, and 16 h of high-temperature treatment during the PP1 period, a significant decrease in proboscis length (P < 0.01), forewing width (P < 0.01), femur length (P < 0.01), tibia length (P < 0.01), metatarsus length (P < 0.01), and metatarsus width was observed (P < 0.01, see the Supplementary material). As heat exposure time prolonged, morphological characteristics were further diminished, implying that the heat impairment had a cumulative effect on the metamorphic staged brood honeybees.
The results of the PCA analysis indicated that the test groups exposed to high-temperature stress for 16 h were morphologically different from the CK group. Notably, the PP1 group, followed by the ML and PP2 groups, was grouped distinct from the CK group, indicating that PP1, among the pupation stages, was particularly susceptible to deviated high-temperature stress (Figure 5).
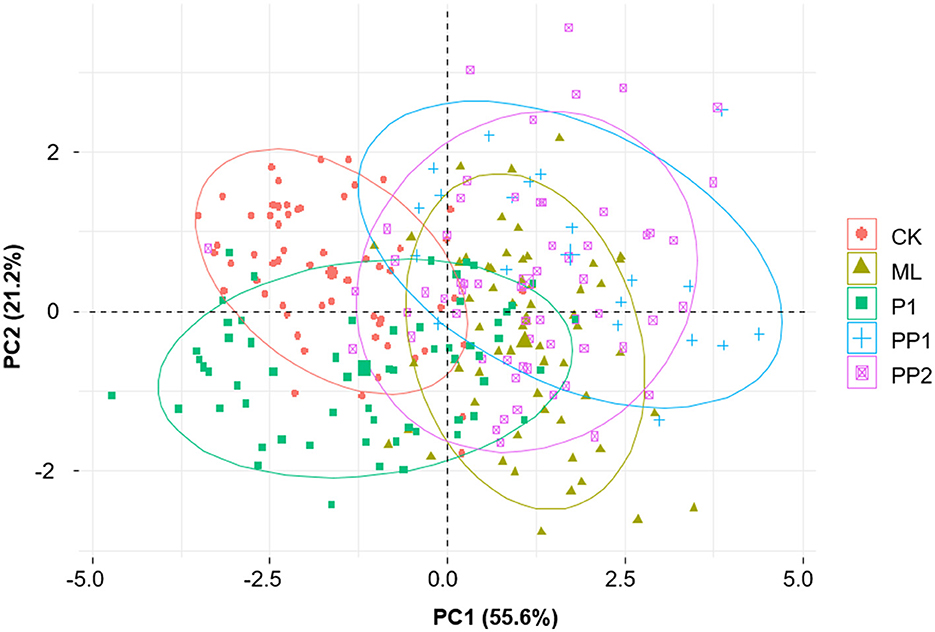
Figure 5. PCA plot for the appendage sizes in honeybee broods exposed to high temperatures for 16 h. Each dot indicates the appendage sizes of an individual bee, and the shapes of colored dots represent experiment groups: CK, control group; ML, mature larva; PP1, the first day of prepupa; PP2, the second day of prepupa; P1, the first day of the pupa. The horizontal and vertical axes represent PC1 and PC2, respectively. The numbers in the parentheses show the proportion of the variance explained by PC1 and PC2. Morphologically similar samples will be plotted close to each other (ellipse of 90% confidence interval). Raw data are available in Supplementary Table 5.
4. Discussion
The pupal stage of holometabolous insects affords them greater resistance to hostile environmental conditions, yet the pupation process from larva to the pupa is a fragile stage that is sensitive to the environment. Hironaka and Morishita (2017) proposed that holometabolous insects evolved pupal development stages to tolerate harsh environments in both winter and summer. Our study examined the effects of high temperatures on the mortality rate, development duration, and birth weight of honeybees during the transition from the cessation of feeding at the mature larval stage to the molting into pupae. It was determined that the early pupae PP1 stage was the most sensitive to high-temperature stress among the metamorphic stages, akin to our previous finding that 3-day-old capped broods were susceptible to low temperature (Wang et al., 2016). During the metamorphic period, their internal tissues and organs undergo tremendous changes (Myser, 1954). Moreover, several studies have demonstrated that pupation is particularly vulnerable to abnormal temperatures and nest spleen shaking (Jay, 1963; Bennett et al., 2015; Zhu et al., 2018). To further assess the effects of high temperatures on early capped brood development and the size of appendages (wings, legs, and proboscis) in eclosed honeybees, we examined the response to thermal stress at different stages from mature larvae to pupa. Based on the extent of the response to a high temperature between stages, our findings indicated that the early pupae PP1 stage was the most sensitive among the metamorphic stages. This stage-specific response to thermal stress can help to better understand the mechanisms underlying the thermal stress on honeybee development, as well as to assess the potential damage to honeybee development in heat, such as under global warming and heat waves (Le Conte and Navajas, 2008; Rader et al., 2013) and heat treatment in hive mite control (Kablau et al., 2020). To preserve or cultivate bee pupae effectively, it is essential to maintain an accurate and stable development temperature during this delicate period, or else the quality of the emerging bees may be impaired. Simultaneously, there could be a vulnerable stage of development for damaging insects. This study also proposes a method of controlling these insects.
High temperatures can have a detrimental effect on the hormone regulation system, an essential component of bee growth and development. This study revealed that exposing pupae to a temperature of 40°C for 16 h caused PP1 with marked pupal mortality and delayed development durations. This could be attributed to the disruption of hormones responsible for the pupation process, such as juvenile hormones and ecdysone. The metamorphosis and development of insects are regulated by juvenile hormones and ecdysone (Thummel, 2001). Cold temperatures were shown to increase the juvenile hormone titers in nurses and foragers (Lin et al., 2004), indicating its potential as a stress hormone. JH antagonizes ecdysone in metamorphic transition, which may inhibit the development process (Lyu et al., 2019). A rise in JH titer in response to high temperatures may delay the transition from prepupa to pupa, thus prolonging the development duration (Supplementary Table 2). Additionally, we reported that a key transcription factor FoxO, regulated by the insulin signaling pathway, has been identified as being involved in suppressing the pupation process (Yao et al., 2020). Despite the knowledge gained from current research, the physiological, and molecular mechanisms behind the thermal damage in honeybee metamorphic stages have yet to be determined. Further investigations are needed to elucidate the effects of high temperatures on the degradation of juvenile hormones, the synthesis of ecdysone, and hormone-regulated signal transmission.
Our findings indicated that thermal stress during metamorphic stages had an effect on tissue remodeling and morphogenesis during pupation, leading to a decrease in the size of the proboscis, legs, and wings in adults. Notably, the most pronounced effect was seen on forewing size during the mature larvae stage. It was consistent with our previous study that abnormal veins mainly occurred at ML under low temperatures, with the highest abnormal rate and the largest variety of types (Zhu et al., 2018). These findings indicate that the ML stage is probably a checkpoint for wing development in Apis cerana workers and other holometabolous insects (Nardi and Norby, 1987). Additionally, our data showed that the prepupal stages (PP1 and PP2) were particularly vulnerable to thermal stress with regard to the proboscis and leg development. However, the development of tergites, wax plates, and sternites was not affected by high temperatures. Collectively, the development of wings and ventral appendages (proboscis and legs), which are vulnerable to thermal stress in early prepupae, indicates that the early capped brood honeybee had a stage-specific response to thermal stress. The productivity of adult bees is closely connected to the development of honeybee appendages. Therefore, it is of great importance to explore the molecular mechanism of how high temperature influences appendage development.
5. Conclusion
Our experiments with mature larvae, first- and second-day pupae, and new pupae Apis cerana exposed to high temperatures demonstrated that heat exposure impairs honeybee development health, as well as wing, proboscis, and leg sizes. Moreover, the first-day pupae were found to be more susceptible to high-temperature stress, and mature larvae and the first-day pupae were essential stages for wing and appendage development in honeybees.
Data availability statement
The original contributions presented in the study are included in the article/Supplementary material, further inquiries can be directed to the corresponding author.
Author contributions
Conceived and designed the experiments: XX, XD, SZ, BZ, and XZhu. Performed the experiments: XX, KL, QW, HL, CZ, XZha, and XZhu. Analyzed the data: XX, CZ, MC, and XZhu. Contributed to reagents, materials, and analysis tools: QW, XX, XZhu, and BZ. Wrote the paper: XX and XZhu. All authors contributed to the article and approved the submitted version.
Funding
This study was supported by the China Agriculture Research System of MOF and MARA (CARS-44-KXJ11), Fujian Natural Science Foundation Project (2021j01079), Fujian Provincial Innovation Initiative for Undergraduates (S202110389055 and S202110389074), and Educational Research Project for Young Teachers of the Fujian Provincial Department of Education (JAT170486).
Acknowledgments
We thank Huahui Lan, Dongming Yang, Chun Zhang, Zhongxin Su, and Fan Fu for their laboratory assistance. We also thank the editor and two referees for their helpful comments on this study.
Conflict of interest
The authors declare that the research was conducted in the absence of any commercial or financial relationships that could be construed as a potential conflict of interest.
The reviewer YF declared a shared affiliation with the author XD to the handling editor at the time of the review.
Publisher's note
All claims expressed in this article are solely those of the authors and do not necessarily represent those of their affiliated organizations, or those of the publisher, the editors and the reviewers. Any product that may be evaluated in this article, or claim that may be made by its manufacturer, is not guaranteed or endorsed by the publisher.
Supplementary material
The Supplementary Material for this article can be found online at: https://www.frontiersin.org/articles/10.3389/fevo.2023.1144216/full#supplementary-material
References
Abou-Shaara, H. F., Owayss, A. A., Ibrahim, Y. Y., and Basuny, N. K. (2017). A review of impacts of temperature and relative humidity on various activities of honey bees. Insectes Sociaux 64, 455–463. doi: 10.1007/s00040-017-0573-8
Becher, M. A., Scharpenberg, H., and Moritz, R. F. A. (2009). Pupal developmental temperature and behavioral specialization of honeybee workers (Apis mellifera L.). J. Compar. Physiol. A 195, 673–679. doi: 10.1007/s00359-009-0442-7
Bennett, M. M., Cook, K. M., Rinehart, J. P., Yocum, G. D., Kemp, W. P., and Greenlee, K. J. (2015). Exposure to suboptimal temperatures during metamorphosis reveals a critical developmental window in the solitary bee, Megachile rotundata. Physiol. Biochem. Zool. 88, 508–520. doi: 10.1086/682024
Flores, J., M; Ruiz, J., A; Ruz, J. M., Puerta, F., Bustos, M., Padilla, F., et al. (1996). Effect of temperature and humidity of sealed brood on chalkbrood development under controlled conditions. Apidologie 27, 185–192. doi: 10.1051/apido:19960401
Groh, C., Tautz, J., and Rossler, W. (2004). Synaptic organization in the adult honey bee brain is influenced by brood-temperature control during pupal development. PNAS 101, 4268–4273. doi: 10.1073/pnas.0400773101
Heinrich, B. (1993). The Hot-Blood Insects: Strategies and Mechanisms of Thermoregulation. Springer, Berlin. doi: 10.4159/harvard.9780674418516
Hironaka, K., and Morishita, Y. (2017). Adaptive significance of critical weight for metamorphosis in holometabolous insects. J. Theor. Biol. 417, 68–83. doi: 10.1016/j.jtbi.2017.01.014
Jay, S. C. (1963). The development of honeybees in their cells. J. Apic. Res. 2, 117–134. doi: 10.1080/00218839.1963.11100072
Jones, J. C., Helliwell, P., Beekman, M., Maleska, R., and Oldroyd, B. P. (2005). The effects of rearing temperature on developmental stability and learning and memory in the honey bee, Apis mellifera. J. Compar. Physiol. 191, 1121–1129. doi: 10.1007/s00359-005-0035-z
Kablau, A., Berg, S., Härtel, S., and Scheiner, R. (2020). Hyperthermia treatment can kill immature and adult Varroa destructor mites without reducing drone fertility. Apidologie 51, 307–315. doi: 10.1007/s13592-019-00715-7
Kleinhenz, M., Bujok, B., Fuchs, S., and Tautz, J. (2003). Hot bees in empty brood nest cells: heating from within. J. Exper. Biol. 206, 4217–4231. doi: 10.1242/jeb.00680
Kronenberg, F., and Heller, H. C. (1982). Colonial thermoregulation in honey bees (Apis mellifera). J. Compar. Physiol. 148, 65–76. doi: 10.1007/BF00688889
Le Conte, Y., and Navajas, M. (2008). Climate change: impact on honey bee populations and diseases. Revue Scientif. Techn. 27, 499–510. doi: 10.20506/rst.27.2.1819
Lê, S., Josse, J., and Husson, F. (2008). FactoMineR: An R package for multivariate analysis. J. Stat. Softw. 25, 1–18. doi: 10.18637/jss.v025.i01
Lin, H., Dusset, C., and Huang, Z. Y. (2004). Short-term changes in juvenile hormone titres in honey bee workers due to stress. Apidologie 35, 319–328. doi: 10.1051/apido:2004018
Lyu, Z., Li, Z., Jie, C., Wang, C., Chen, J., and Lin, T. (2019). Suppression of gene juvenile hormone diol kinase delays pupation in Heortia vitessoides Moore. Insects 10, 278. doi: 10.3390/insects10090278
McMullan, J. B., and Brown, M. J. (2005). Brood pupation temperature affects the susceptibility of honeybees (Apis mellifera) to infestation by tracheal mites (Acarapis woodi). Apidologie 36, 97–105. doi: 10.1051/apido:2004073
Medina, R. G., Paxton, R. J, De Luna, E, Fleites-Ayil, F. A., Medina Medina, L. A., and Quezada-Euan, J. J. G. (2018). Developmental stability, age at onset of foraging and longevity of Africanized honey bees (Apis mellifera L.) under heat stress (Hymenoptera: Apidae). J. Thermal Biol. 74, 214–225. doi: 10.1016/j.jtherbio.2018.04.003
Medrzycki, P., Sgolastra, F., Bortolotti, L., Bogo, G., Tosi, S., Padovani, E., et al. (2010). Influence of brood rearing temperature on honey bee development and susceptibility to poisoning by pesticides. J. Apic. Res. 49, 52–59. doi: 10.3896/IBRA.1.49.1.07
Myser, W. C. (1954). The larval and pupal development of the honey bee Apis mellifera Linnaeus. Ann. Entomol. Soc. Am. 47, 683–711. doi: 10.1093/aesa/47.4.683
Nardi, J. B., and Norby, S. W. (1987). Evocation of venation pattern in the wing of a moth, Manduca sexta. J. Morphol. 193, 53–62. doi: 10.1002/jmor.1051930106
Poot-Báez, V., Medina-Hernández, R., Medina-Peralta, S., José Javier, G., and Quezada-Euán. (2019). Intranidal temperature and body size of Africanized honey bees under heatwaves (Hymenoptera: Apidae). Apidologie. 51, 382–390. doi: 10.1007/s13592-019-00725-5
Rader, R., Reilly, J., Bartomeus, I., and Winfree, R. (2013). Native bees buffer the negative impact of climate warming on honey bee pollination of watermelon crops. Global Change Biol. 19, 3103–3110. doi: 10.1111/gcb.12264
Ruttner, F. (1988). Biogeography and Taxonomy of Honeybee. Berlin: Springer-verlag. 66–78. doi: 10.1007/978-3-642-72649-1
Stabentheiner, A., Kovac, H., and Brodschneider, R. (2010). Honeybee colony thermoregulation regulatory mechanisms and contribution of individuals in dependence on age, location and thermal stress. PLoS ONE 5, e8967. doi: 10.1371/journal.pone.0008967
Sun, B., J; Ma, L., Wang, Y., Mi, C. R., Buckley, L. B., Levy, O., et al. (2021). Latitudinal embryonic thermal tolerance and plasticity shape the vulnerability of oviparous species to climate change. Ecol. Monogr. 93, e01468. doi: 10.1002/ecm.1468
Szentgyörgyi, H., Czekońska, K., and Tofilski, A. (2018). Honey bees are larger and live longer after developing at low temperature. J. Thermal Biol. 78, 219–226. doi: 10.1016/j.jtherbio.2018.09.007
Tan, K., Bock, F., Fuchs, S., Streit, S., Brockmann, A., and Tautz, J. (2005). Effects of brood temperature on honey bee Apis mellifera wing morphology. Acta Zool. Sin. 51. 768–771.
Tautz, J., Maier, S., Groh, C., Rossler, W., and Brockmann, A. (2003). Behavioral performance in adult honey bees is influenced by the temperature experienced during their pupal development. PNAS 100, 7343–7347. doi: 10.1073/pnas.1232346100
Thummel, C. S. (2001). Molecular mechanisms of developmental timing in C. elegans and Drosophila. Develop. Cell 1, 453–465. doi: 10.1016/S1534-5807(01)00060-0
Wang, Q., Xu, X. J., Zhu, X. J., Chen, L., Zhou, S. J., Huang, Z. Y., et al. (2016). Low-temperature stress during capped brood stage increases pupal mortality, misorientation and adult mortality in honey bees. PLoS ONE 11, e0154547. doi: 10.1371/journal.pone.0154547
Yao, D., Zhou, S. J., Zhou, B. F., Xu, X. J., and Zhu, X. J. (2020). Trend analysis of differentially expressed genes in prepupae of Apis mellifera ligustica under low temperature stress. Acta Entomol. Sinica 63, 688–696. doi: 10.16380/j.kcxb.2020.06.004
Zhou, L., Zhu, X. J., Xu, X. J., Zhou, S. J., Fu, Z. M., Wen, X. Z., et al. (2015). Nest temperature distribution and dynamics of Apis cerana cerana during spring thriving stage at ambient temperature 20°C. J. Fujian Agric. Forestry Univ. 44, 198–202.
Zhu, X. J., Xu, X. J., Zhou, S. J., Wang, Q., Chen, L., Hao, Z. B., et al. (2018). Low temperature exposure (20°C) during the sealed brood stage induces abnormal venation of honey bee wings. J. Apic. Res. 57, 458–465. doi: 10.1080/00218839.2017.1412575
Keywords: high-temperature stress, pupation, honey bee, appendage, development
Citation: Xu X, Du X, Zhou S, Zhou B, Lai K, Wang Q, Li H, Zhu C, Xu H, Zhang X, Cao M and Zhu X (2023) Impacts of high temperature during early capped brood on pupal development and the size of appendages in adult workers Apis cerana. Front. Ecol. Evol. 11:1144216. doi: 10.3389/fevo.2023.1144216
Received: 13 January 2023; Accepted: 27 February 2023;
Published: 24 March 2023.
Edited by:
Shudong Luo, Institute of Apiculture Research (CAAS), ChinaReviewed by:
Yu Fang, Chinese Academy of Agricultural Sciences (CAAS), ChinaSampat Ghosh, Andong National University, Republic of Korea
Shunhua Yang, Yunnan Agricultural University, China
Copyright © 2023 Xu, Du, Zhou, Zhou, Lai, Wang, Li, Zhu, Xu, Zhang, Cao and Zhu. This is an open-access article distributed under the terms of the Creative Commons Attribution License (CC BY). The use, distribution or reproduction in other forums is permitted, provided the original author(s) and the copyright owner(s) are credited and that the original publication in this journal is cited, in accordance with accepted academic practice. No use, distribution or reproduction is permitted which does not comply with these terms.
*Correspondence: Xiangjie Zhu, eGlhbmdqaWVfemh1QDEyNi5jb20=
†These authors have contributed equally to this work