- 1ELKH-ELTE-MTM Integrative Ecology Research Group, Budapest, Hungary
- 2Department of Systematic Zoology and Ecology, Institute of Biology, ELTE Eötvös Loránd University, Budapest, Hungary
- 3Doctoral School of Biology, Institute of Biology, ELTE Eötvös Loránd University, Budapest, Hungary
- 4Department of Biology, Biotechnical Faculty, University of Ljubljana, Ljubljana, Slovenia
- 5Research Centre of the Slovenian Academy of Sciences and Arts, Jovan Hadži Institute of Biology, Ljubljana, Slovenia
Body size is a trait of fundamental ecological and evolutionary importance that is often different between males and females (sexual size dimorphism; SSD). The island rule predicts that small-bodied species tend to evolve larger following a release from interspecific competition and predation in insular environments. According to Rensch’s rule, male body size relative to female body size increases with increasing mean body size. This allometric body size – SSD scaling is explained by male-driven body size evolution. These ecogeographical rules are rarely tested within species, and has not been addressed in a cave–surface context, even though caves represent insular environments (small and isolated with simple communities). By analyzing six cave and nine surface populations of the widespread, primarily surface-dwelling freshwater isopod Asellus aquaticus with male-biased SSD, we tested whether cave populations evolved larger and showed higher SSD than the surface populations. We found extensive between-population variation in body size (maximum divergence being 74%) and SSD (males being 15%–50% larger than females). However, habitat type did not explain the body size and SSD variation and we could not reject isometry in the male–female body size relationship. Hence, we found no support for the island or Rensch’s rules. We conclude that local selective forces stemming from environmental factors other than island vs. mainland or the general surface vs. cave characteristics are responsible for the reported population variation.
1. Introduction
Body size correlates to various physiological and behavioral traits and it is often directly linked to fitness, underscoring the evolutionary and ecological importance of this quantitative trait (Peters, 1983; Roff, 1992; Stearns, 1992). The evolution of body size is shaped by different, often opposite forces of natural selection. For instance, fecundity selection, inter- and intrasexual selection usually favors large body size (Shine, 1989; Andersson, 1994). However, organisms do not constantly evolve larger (Stanley, 1973; Blanckenhorn, 2000). The two main selective forces acting against evolving larger are predation and interspecific competition. This is because prey-size-unlimited predators actively prefer larger prey and the increased behavioral activity needed for achieving and maintaining large body size increases general predation risk, while larger bodied competitors select against an evolutionary increase in body size (Wilson, 1975; Lomolino, 1985; Blanckenhorn, 2000; Simberloff et al., 2000; Herczeg et al., 2009). Considering the above detailed selective forces, one would expect the release from the selective pressures acting against large body size when small-bodied species colonize islands, which are typically isolated and characterized by simple communities. Such mechanism would result in gigantism of small-bodied species evolving under insular conditions. On the other hand, resource limitation combined with reduced prey-size-limited predation pressure on islands could result in the dwarfism of large-bodied species evolving under insular conditions. The evolution of gigantism of small species and dwarfism of large species on islands was observed long ago and referred as the island rule (Foster, 1964; Van Valen, 1973a,b; Lomolino, 1985), which later received further support (e.g., Lomolino, 2005; Benítez-López et al., 2021). This rule, originally proposed in an interspecific context was also supported in intraspecific population comparisons (Herczeg et al., 2009; MacColl et al., 2013; Runemark et al., 2015).
Adult body size also varies within population. A major source of this variation is sexual size dimorphism (SSD). It is widespread among animals (Fairbairn, 1997). In ectothermic vertebrates and invertebrates, typically females, while among endothermic vertebrates, males are larger (e.g., Fairbairn and Preziosi, 1994; Fairbairn, 1997; Blanckenhorn, 2005). The degree of SSD often scales allometrically with body size across species (Fairbairn, 1997). Rensch’s rule states that in species with female-biased SSD, the level of SSD will decrease (hypo-allometry), while in species with male-biased SSD, the level of SSD will increase (hyper-allometry) with increasing body size (Rensch, 1950, 1959). To emphasize that hypo- and hyper-allometry are two parts of the same continuum, the rule was simplified (with the same meaning) stating that male body size relative to female body size increases with increasing mean body size (Meiri and Liang, 2021). Rensch’s rule was supported in many taxa (mainly with male-biased SSD; e.g., Fairbairn, 1997; Székely et al., 2004; Fairbairn et al., 2007). However, in taxa with female-biased SSD, the rule has been questioned (Webb and Freckleton, 2007; Liang et al., 2022) and there are even examples of inverse Rensch’ rule (see Fairbairn, 1997). Opposite trends have been observed even between closely related taxa (Piross et al., 2019). Patterns following Rensch’s rule or the inverse of Rensch’s rule are both accommodated by Fairbairn’s (1997) correlational selection hypothesis. It states that allometric SSD patterns are resulting from directional selection on body size acting primarily on one sex (e.g., sexual selection on males or fecundity selection on females) followed by correlational selection occurring in the other. In a wider sense, if body size of one sex shows a stronger response to selection stemming from geographic or ecological factors than the other, the intraspecific level of SSD will vary (Blanckenhorn et al., 2006). Similarly to the island rule, Rensch’s rule was originally proposed for interspecific comparisons, but intraspecific tests have also started to accumulate with mixed results (Herczeg et al., 2010; Wu et al., 2014; Liao et al., 2015; Yu et al., 2022).
Caves and other subterranean habitats are widespread in every continent, yet they have some important general characteristics. Organisms living in caves are typically faced with total darkness, limited food supply, highly stable daily, seasonal, and annual environmental conditions, and live in simple communities (Culver et al., 1995; Culver and Pipan, 2009; Romero, 2009, 2011). The latter results in low or negligible predation and interspecific competition, which jointly with the typical high isolation from surface habitats and other caves make caves insular habitats (e.g., Poulson and White, 1969; Mammola, 2019). Adaptations to cave environment have attracted considerable scientific interest ever since (Darwin, 1859). Morphological adaptations observed across independent caves and phylogenetically distant cave-adapted taxa were recognized early and referred as troglomorphism (Christiansen, 1962). This term has been since broadened to include any kind of cave-related adaptations. Classic examples for cave adaptations are loss of pigmentation, eye reduction, appendage elongation, decreased egg number/increased egg volume, increased longevity, and loss of circadian rhythm (e.g., Voituron et al., 2011; Pipan and Culver, 2012; Beale et al., 2013; Howarth and Maoldovan, 2018; Lunghi and Bilandžija, 2022). Even though cave–surface species or population pairs are rarely studied in the island–mainland framework, perhaps because the general environmental differences like the presence/absence of light seem more important than the insular characteristics of caves, some results can be interpreted from this aspect. For instance, Fišer et al. (2013) showed that females of cave-dwelling Niphargus (freshwater amphipod crustacean) species are generally larger than those of surface-dwelling species and Mojaddidi et al. (2018) showed in a common garden experiment that a cave Asellus aquaticus (freshwater isopod crustacean) population has larger hatchlings than two surface populations. These results are consistent with the predictions drawn from the island rule. However, we are not aware of an intraspecific study on the same effect with repeated sampling of cave vs. surface habitats or any studies testing predictions of the Rensch’s rule, or even simply comparing SSD between cave and surface species or population pairs.
In the present paper, we performed an intraspecific test of the predictions drawn from the island and Rensch’s rules, using cave vs. surface habitats in an island–mainland context. We studied six cave and nine surface populations of the common waterlouse, A. aquaticus, species complex. This widespread surface-dwelling freshwater isopod successfully colonized European aquatic cave habitats on several independent and relatively recent occasions (Verovnik et al., 2003, 2004, 2009). Cave-adapted populations show troglomorph characteristics, like eye reduction and depigmentation (Verovnik and Konec, 2019). Body size is heritable in this species (Thompson, 1986), and male-biased SSD has been recognized (Ridley and Thompson, 1979; Adams et al., 1985; Bertin and Cezilly, 2003). We tested two predictions drawn from the island and Rensch’s rules. First and in-line with the island rule, we predicted that A. aquaticus will evolve larger in the low predation, simple communities of caves. Second, assuming that the first prediction holds, we predicted that patterns in SSD will follow the Rensch’s rule, resulting in larger male-biased SSD in caves than in surface populations.
2. Materials and methods
2.1. Study system
The taxonomic status of distinct cave and surface A. aquaticus populations has not yet been resolved (Verovnik and Konec, 2019). Cave populations typically show troglomorphic traits and genetic isolation from each other and from adjacent surface populations (e.g., Verovnik et al., 2009; Pérez-Moreno et al., 2017). They have been described as a new species, new subspecies or simply as an isolated population (Turk-Prevorčnik and Blejec, 1998; Verovnik et al., 2004, 2009; Pérez-Moreno et al., 2017; Simčič and Sket, 2019). Similarly, some surface populations have been given a subspecies status due to differences in morphology (Sket, 1994). In the absence of comparative genetic and phenotypic data to clarify the whole situation, we treat each population used in this study as a sample from the “A. aquaticus species complex” and refer to them as A. aquaticus populations for simplicity.
The six caves sampled were not similar in their ecological characteristics. Four of them are “typical” caves, in which meteoric water and sinking rivers formed the subterranean passages (epigenic speleogenesis), whereas Movile aquifer and Molnár János Cave are hydrothermal cave systems, in which hypogean thermal water is responsible for cave formation (hypogenic speleogenesis). The temperature of a “typical” cave corresponds approximately to the average annual temperature of the respective region, in our case between 11°C and 12°C. In these caves, food is transported from the surface by sinking rivers and is of limited quantity and, more importantly, low quality (Poulson and Lavoie, 2000). The temperature of a hydrothermal cave depends on the temperature of the thermal water forming the cave and approximates 21–23°C in both Movile aquifer and Molnár János Cave. The ecosystems in the studied hydrothermal caves are highly isolated from the surface and likely rely on the endogenous production of organic matter by chemoautotrophic bacteria (Sarbu et al., 1996; Herczeg et al., 2020, 2022). It is assumed that the amount of available food is higher in hydrothermal caves than in epigenic caves (Poulson and Lavoie, 2000).
2.2. Sampling and measurements
We collected 358 adult male and 298 adult female A. aquaticus from six cave and nine surface populations spanning through Slovenia, Italy, Hungary and Romania (Table 1; Figure 1). Sampling was done with hand nets in all but the Molnár János Cave population, where cave diving was necessary. The Movile aquifer was sampled at the Dimitru Ana 9. well. According to Hasu et al., (2007) and Bloor (2010), individuals larger than 3–3.5 mm can already be considered as adults, however, we used a more conservative 4 mm threshold. Sex was determined via visual inspection of gonopod morphology under stereomicroscope.
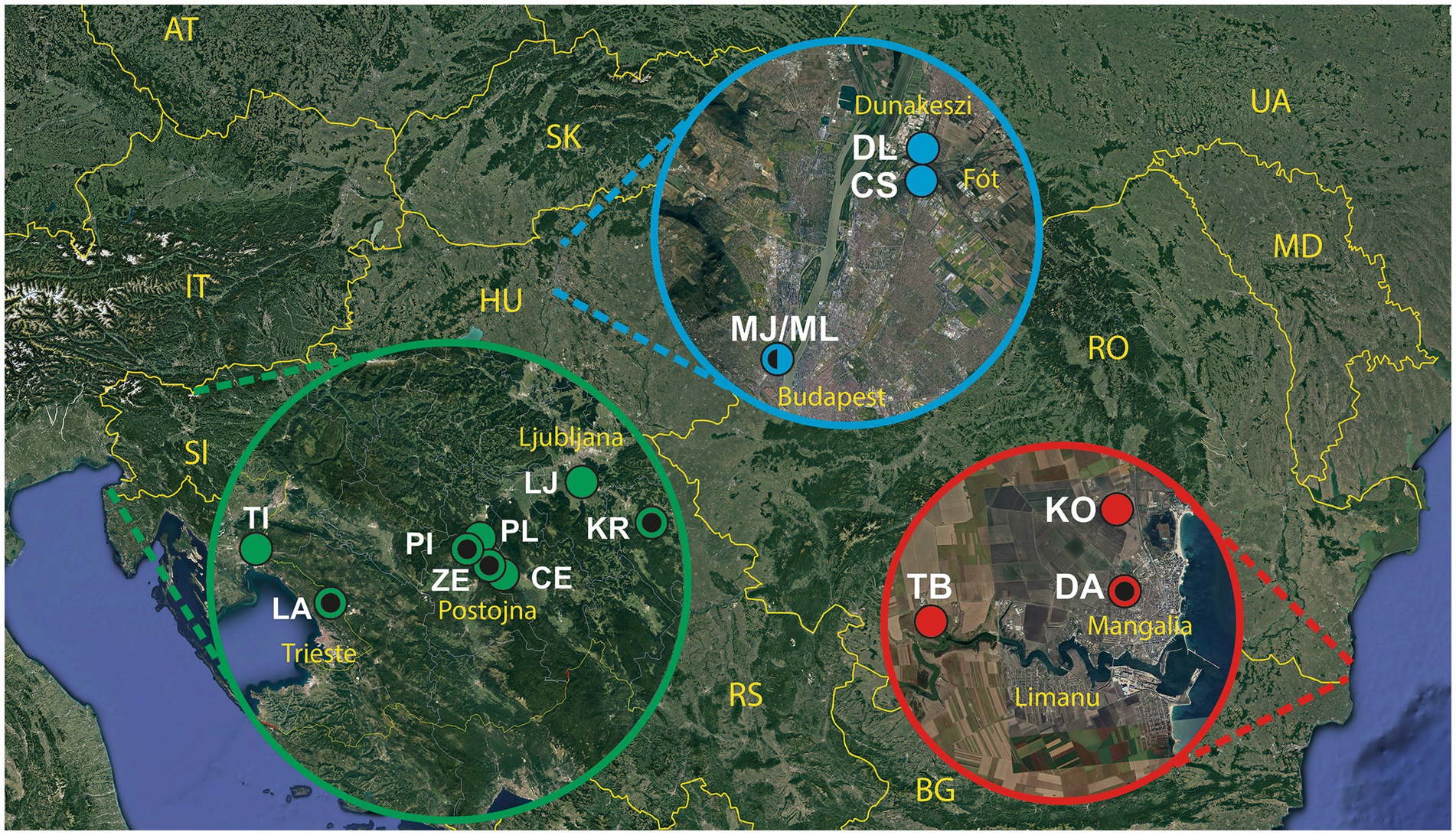
Figure 1. Map showing the geographical locations of sample sites. Black dots in the middle of colored circles denotes cave populations. For the population abbreviations, see Table 1. Note that MJ (cave) and ML (surface) populations are very close to each other, hence, they are represented by the same circle.
Collected individuals were conserved in RNAlater (Thermo Fisher Scientific Inc., Waltham, Massachusetts, United States). For measuring body size, digital images were taken with a Canon 600D (Canon Inc. Tokyo, Japan) camera in a standardized setup and a millimeter scale bar for reference. We used body length (distance between the end of the pleotelson to the apical line of the head) as a proxy for body size and measured it from the digital images with the TpsUtil 1.74 and TpsDig2 2.30 softwares.1 We performed two independent body length measurements for each individual and the average value of the two measurements was used in the analysis.
2.3. Statistical analyses
Asellus aquaticus undergo indeterminate growth (e.g., Lafuente et al., 2021) and thus using mean body size to compare populations can be biased by variation in the relative contribution of different age groups in the sample. Therefore, we analyzed the five largest males and females from every population to correct for the potential bias (e.g., Stamps and Andrews, 1992; Kratochwíl and Frynta, 2002). We note that analyzing the complete dataset revealed qualitatively similar results (data not shown). We also note that our approach could not control for age effects directly, since the age of the sampled individuals was unknown. Hence, there is a possibility that in the low predation cave habitats the largest individuals were older, than the largest conspecifics in surface habitats.
For testing habitat-dependent body size and SSD trends, we ran a Linear Mixed Model (LMM) with body length as the dependent variable, habitat, sex and their interaction as fixed effects and population nested in habitat as a random effect. We checked model residual distribution via Q–Q plots. This model revealed no significant habitat effect, but a highly significant population effect (see Results). Therefore, to compare populations directly, we also ran a Linear Model (LM) with body length as the dependent variable and population, sex and their interaction as fixed effects. We judged differences between groups based on 95% confidence intervals. This is a conservative approach, because according to Payton et al. (2003), the lack of overlap between 83% and 84% confidence intervals is analogous to p < 0.05.
We note that Rensch’s rule could be present in our sample irrespective of the validity of island rule. With other words, an allometric SSD–body size relationship could be present in the absence of habitat-dependent body size or SSD patterns (i.e., the outcome of the previous models). For testing Rensch’s rule directly (see Herczeg et al., 2010), we calculated the mean size for each sex in every population and then regressed log10 male body length against log10 female body length. This allowed us to test whether the slope differed from zero (i.e., the size of the sexes is not independent) and from one (i.e., there is a departure from isometry, supporting Rensch’s rule or its inverse). Because in such case neither variable is fixed, or with other words, both variables are measured with error, ordinary least squares (Model I) regression would be statistically incorrect and thus major axis (Model II) regression is recommended (Fairbairn, 1997). However, this view was challenged recently, and the parallel usage of Model I and II regressions were recommended (Meiri and Liang, 2021; Liang et al., 2022).
All analyses were done in the R 4.0.3 statistical environment (R Core Team, 2020). We used the lme4 (Bates et al., 2015), lmerTest (Kuznetsova et al., 2017), emmeans (Lenth, 2020), and lmodel2 (Legendre, 2018) packages.
3. Results
The LMM revealed a significant sex effect, but no habitat or habitat × sex effects (habitat: F1,13 = 2.17, p = 0.16; sex: F1,133 = 468.59, p < 0.001; habitat × sex: F1,133 = 0.32, p = 0.57). SSD was male-biased (least squares means and 95% confidence intervals in mm; male: 8.95 [8.27–9.63]; female: 6.81 [6.13–7.49]). The lack of habitat effect rejected the island rule, because there was no sign of larger size in the insular cave environment than in surface habitats. The lack of habitat × sex interaction revealed no systematic difference in SSD between cave and surface habitats. The random effect of population nested in habitat was highly significant (likelihood ratio test: χ2 = 171.14, df = 1, p < 0.001), indicating high between-population variation in body size.
Our LM revealed significant population, sex and population × sex effects (population: F14,120 = 70.61, p < 0.001; sex: F1,120 = 784.18, p < 0.001; population × sex: F14,120 = 6.89, p < 0.001). Between-population variation in body size was large, reaching 74% difference between the smallest and largest population (Molnár János Cave vs. Movile aquifer; Figure 2). Male-biased SSD was present in every population, showing large variation from 15% (Molnár János Cave) to 50% (Movile aquifer; Figure 2).
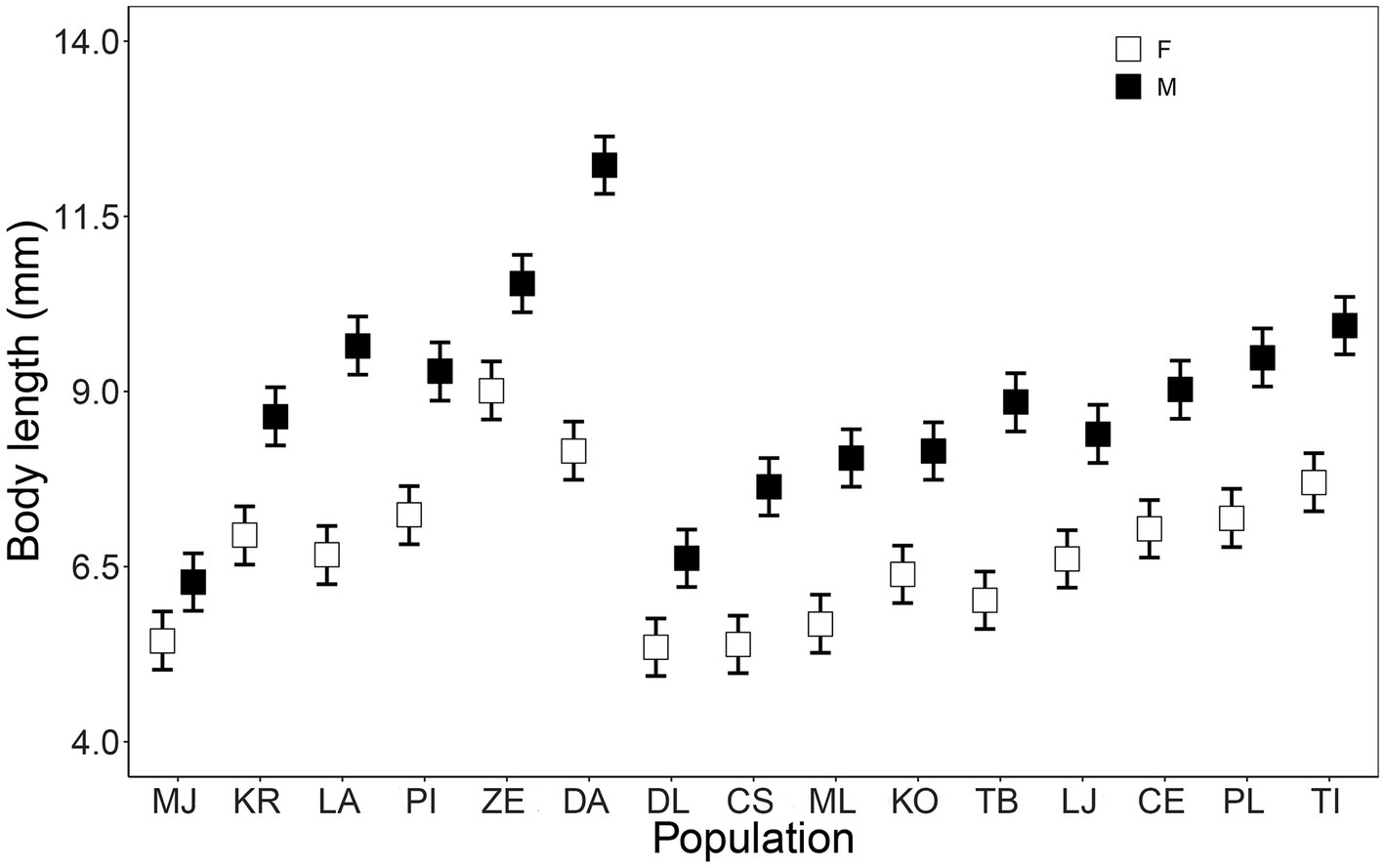
Figure 2. Body length variation of female (F) and male (M) Asellus aquaticus from the cave (MJ, KR, LA, PI, ZE, DA) and surface (DL, CS, ML, KO, TB, LJ, CE, PL, TI) populations. Estimated marginal means and 95% confidence intervals are shown. The population codes are explained in Table 1.
The Model I and II regressions revealed a significant relationship between male and female body size across populations (R2 = 0.77; p (for both regressions) < 0.001). However, the slope did not differ from one (Model I: β = 0.96; 95% confidence interval [CI] = 0.65–1.27; Model II: β = 1.1; CI = 0.79–1.54), indicating no violation of isometry (Figure 3). With other words, the level of SSD was unrelated to size, and thus our data reject the Rensch’s (or inverse Rensch’s) rule in our system.
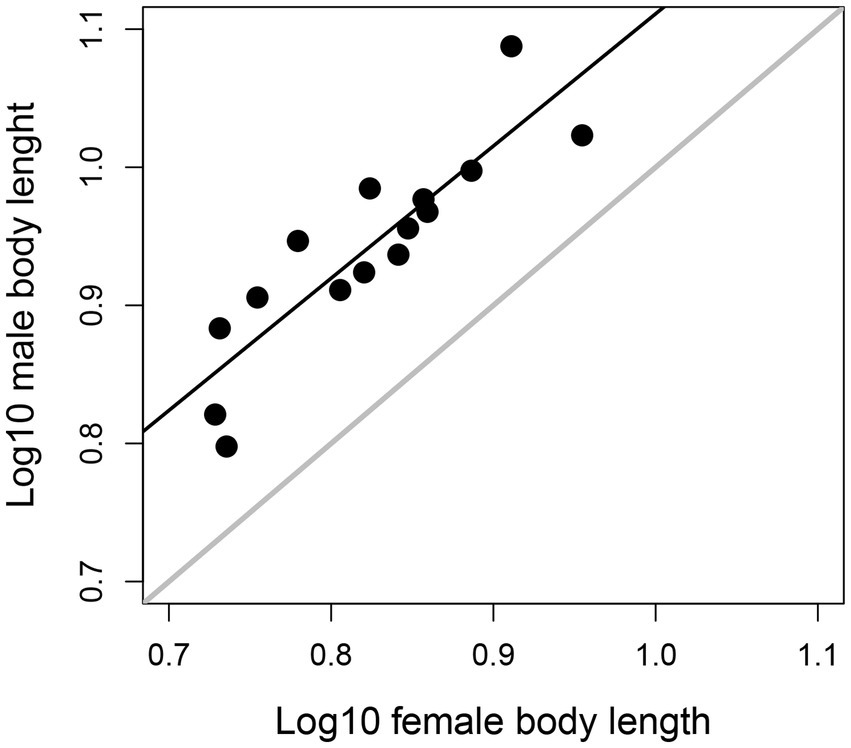
Figure 3. Lack of deviation from isometry in male-biased sexual size dimorphism in Asellus aquaticus. Ordinary Least Squares (Model I) regression line (β = 0.96) and its 95% confidence interval (shaded area) are shown. The thick gray line depicts isometry (β = 1) without sexual size dimorphism.
4. Discussion
The most salient finding of our study is that there is high variation in body size and the level of SSD across the studied 15 A. aquaticus populations. However, habitat type (cave vs. surface) did not explain a significant proportion of the variation and the female–male body size relationship across populations did not diverge from isometry. Therefore, we found no support for the island and Rensch’s rules in our study system.
We expected an increase in body size in cave habitats based on the predictions of the island rule (e.g., Lomolino, 1985, 2005; Benítez-López et al., 2021). According to this rule, the relaxation of predation and interspecific competition in insular environments (McNab, 1994; Lomolino, 2005) would allow for an evolutionary increase in body size due to its advantages in fecundity, and inter- and intrasexual selection in small-bodied species (Clutton-Brock et al., 1982; Andersson, 1994). Our data rejected this expectation. While the two populations with the largest body size were indeed from caves, the population with the smallest body size was also a cave population and the rest of the cave populations were within the (large) range covered by surface populations. The lack of habitat effect is definitely not because the cave populations had no time to adapt. The estimated divergence times between cave and surface populations exceeds 60,000 years (Verovnik et al., 2003, 2004; Konec et al., 2015; Pérez-Moreno et al., 2017) and the typical troglomorphic adaptations, like eye degeneration and depigmentation, are observed in all cave populations studied (Verovnik et al., 2009; Pérez-Moreno et al., 2017; Balázs et al., 2021). Adaptive variation in body size can be the result of various environmental factors other than predation and interspecific competition. For instance, resource levels, prey size, and intraspecific competition can be important sources of selection on body size (e.g., Case, 1978; Forsman, 1991; Clegg and Owens, 2002; Wu et al., 2006; Herczeg et al., 2012), and even random events might be responsible for it (Wasserzug et al., 1979; Biddick and Burns, 2021). Obviously, there are other characteristics of caves that are not related to their insularity, such as the absence of light or scarcity of food (Culver and Pipan, 2009; Romero, 2009). For instance, food scarcity, especially in caves that rely on exogenous organic material, might have led to smaller body size in caves (Fišer, 2019). It is conceivable that this factor counterbalanced the above detailed selective forces stemming from the caves’ insular characteristics and thus constrained body size evolution. Sexual or fecundity selection also acts differently between cave and surface A. aquaticus populations (Balázs et al., 2021), but their effects on body size might be different from that predicted by the island rule. For instance, the generally lower population densities in caves (Mammola et al., 2021) might relax selection for traits used in male–male aggression (e.g., body size) and target traits aiding mate finding (e.g., sensory systems; Bertin and Cézilly, 2005; Thiel and Duffy, 2007; Elipot et al., 2013; Balázs et al., 2021). At any rate, body size is not a conservative trait in the studied A. aquaticus species complex: body size divergence reached up to 74% in cave (Molnár János Cave vs. Movile aquifer, the two hydrothermal cave systems) and 47% in surface populations (Dunakeszi Marsh vs. Timavo Spring). It seems that variation among cave populations is considerably larger than among surface populations, suggesting that divergent selective forces acting on body size are stronger in caves, which are intuitively more similar to each other than surface environments. Speculating about cave colonizations might also invoke founder effects, bottlenecks and subsequent genetic drift as an evolutionary driver. Therefore, understanding the striking body size variation in A. aquaticus, especially among its cave populations, warrants future studies.
Various selective forces can result in SSD, for instance, sexual selection acting on males, fecundity selection acting on females or divergent ecological selection acting on both sexes (e.g., Shine, 1989; Andersson, 1994). If the relationship between SSD and body size was allometric (as predicted by Rensch’s rule or the inverse of Rensch’s rule), size of one sex shows greater variation than size of the other sex (Fairbairn and Preziosi, 1994; Fairbairn, 1997). The correlational selection framework (i.e., establishing which sex shows higher body size variation; Fairbairn, 1997) allows researchers to see that body size divergence in a certain system is a result of selection acting mainly on males or females. In our study, we detected large variation in male-biased SSD (from 15% to 50% difference), but found no allometric SSD–body size relationship. Here, the conclusion drawn from the correlational selection hypothesis would be that there is no general environmental trend resulting in selection acting mainly on one sex’s body size, but rather locally variable selective forces acting on males, females, or both sexes are responsible for the pattern. This is an attractive explanation, especially considering that even such an extreme environmental difference as the one seen between cave and surface habitats did not explain a significant proportion of variation in SSD. Since body size is a trait that might contribute to fitness in various ways in both sexes and be constrained by a variety of factors (resource levels, competition and predation), environmental variation within cave or surface habitats might cause the lack of clear habitat divergence in SSD, in contrary to certain morphological traits with marked habitat-dependent sexual dimorphism (Balázs et al., 2021). All we can say is that the male-biased SSD in our system is a sign that sexual selection on male body size is a stronger force than fecundity selection on female body size. This seems plausible when considering the importance of precopulatory mate guarding in male reproductive success (Ridley and Thompson, 1979; Adams et al., 1985; Bertin et al., 2002). It is interesting to note that in the Molnár János Cave population (with which we have been working intensively in the last years both in the field and in the laboratory: Herczeg et al., 2020, 2022; Balázs et al., 2021; Horváth et al., 2021, 2023; Berisha et al., 2022), which is the smallest in size and has the lowest SSD, precopulatory mate guarding was never observed (personal observation).
We must note that the body size range utilized in the present study is narrower (but not by magnitudes—note that we use body length here, while many studies use body mass) compared to the ranges in interspecific studies on Rensch’s rule (e.g., Colwell, 2000; Kratochwíl and Frynta, 2002; Székely et al., 2004; Webb and Freckleton, 2007). On the contrary, similar ranges in intraspecific comparisons were sufficient to detect significant allometry in other species (e.g., Herczeg et al., 2010). Further, the sample size from some populations might not guarantee that even by using only the largest individuals from the population sample we could avoid biased sampling of different age groups, especially that the samples could not been collected at the same time of the year. However, we are working with the studied populations for more than a decade now and based on our experience, the sample representation is adequate. Hence, we think that the conclusion regarding locally variable selective forces being responsible for the body size and SSD variation in the studied A. aquaticus system is plausible. One potential problem is that even though cave communities are indeed simpler than surface communities in the typical A. aquaticus habitats, some of the studied caves can be considered harboring diverse communities by subterranean standards. For example, the Postojna-Planina Cave System harbors 50+ aquatic species including predators, and can be considered as a hotspot for subterranean biodiversity (Zagmajster et al., 2021). The Movile Cave, which is one window of access to the Movile aquifer, harbors 20+ aquatic species, also including aquatic predators (Brad et al., 2021). However, hand-dug wells (including our sampling site), providing further windows of access to the Movile aquifer in and around the town Mangalia, are predator-free and harbor lower diversity (Serban Sarbu, pers. comm.). It is possible that in an intraspecific context, only insular environments with extremely simple communities will induce body size evolution in line with the island rule. For instance, in the case of the nine-spined stickleback, Pungitius pungitius, a single larger-bodied competitor species could inhibit the evolution of gigantism in insular environments (Herczeg et al., 2009).
We note that two of the studied cave populations were not typical in the sense that they were not cold-water epigenic caves relying on organic material of exogenous origin. The Molnár János Cave in Hungary and the Movile aquifer in Romania are both hypogenic hydrothermal cave systems with no exogenous organic material being present, with communities relying on endogenous bacteria of chemoautotroph origin (proven for Movile aquifer: Sarbu et al., 1996; proposed for Molnár János Cave: Herczeg et al., 2020, 2022). Despite this fact, A. aquaticus from these two caves showed the highest body size and SSD divergence among all possible population pairs (Movile aquifer > Molnár János Cave for both body size and SSD). We can only speculate about the environmental factors that led to this divergence. The water temperature is similar in the caves (between 21°C and 23°C) and both caves are characterized by stable and low flow velocity. Water chemistry is intuitively harsher in Movile aquifer with extremely low oxygen and high sulfur (Sarbu et al., 1996; Brad et al., 2021). Still, the Movile aquifer population had the largest body size among all populations tested. The endogenous bacterial mats in Molnár János Cave are likely to represent low quality food source (Herczeg et al., 2020, 2022), which might explain that this population have the smallest body size of all studied populations, but the extremely large body size in Movile aquifer definitely requires further studies.
Taken together, even though we found large population variation in body size and SSD, we found no support for the island rule, habitat-dependent SSD or Rensch’s rule in our system of six cave and nine surface A. aquaticus populations. We conclude that the marked general environmental differences between cave and surface habitats, like lack of light or differences in food sources and food availability, might override differences stemming from the island–mainland characteristics. Further, local selective forces unexplained by the major environmental divergence between cave and surface habitats govern variation in both body size and SSD, without one of the sexes being the driver of body size evolution in the study system. For a better understanding of body size evolution in cave and surface A. aquaticus, common garden experiments are warranted to separate the genetic and environmental contributions to body size variation.
Data availability statement
The original contributions presented in the study are included in the article/Supplementary material, further inquiries can be directed to the corresponding author.
Author contributions
GH, SK-F, and CF contributed to the conception and design of the study. GB, AB, and ŽF organized the database. GH performed the statistical analysis and wrote the first draft of the manuscript. All authors contributed to the article and approved the submitted version.
Funding
This study was financially supported by Hungarian National Research, Development and Innovation Fund (#SNN-125627) and Slovenian Research Agency (N1-0069, P1-0236, and P1-0184). GB was supported by the ÚNKP-20-4 New National Excellence Program of the Ministry of Innovation and Technology from the source of the National Research, Development and Innovation Office.
Acknowledgments
We thank Grega Benko, Špela Borko, Gregor Bračko, Teo Delić, Franc Kljun, Ester Premate, Maja Zagmajster, and Valerjia Zakšek who helped to collect animals in Slovenia, Edi Mauri and Andrea Colla who helped to collect animals in Italy, as well as Serban Sarbu who helped to collect animals in Romania. The sample collections were done under the following permits: Slovenia, Agencija RS za okolje, document no. 35602-46/2016-4; 35602-41/2021-5; Regione Autonoma Friuli Venezia Giulia (Italia), Direzione Centrale Infrastrutture e Territorio, PROT. TERINF-GEN-2017 n. 0019455/P; DINPI/5927-0/2020 (Hungary).
Conflict of interest
The authors declare that the research was conducted in the absence of any commercial or financial relationships that could be construed as a potential conflict of interest.
Publisher’s note
All claims expressed in this article are solely those of the authors and do not necessarily represent those of their affiliated organizations, or those of the publisher, the editors and the reviewers. Any product that may be evaluated in this article, or claim that may be made by its manufacturer, is not guaranteed or endorsed by the publisher.
Supplementary material
The Supplementary material for this article can be found online at: https://www.frontiersin.org/articles/10.3389/fevo.2023.1155261/full#supplementary-material
Footnotes
References
Adams, J., Greenwood, P., Richard, P., and Tania, Y. (1985). Loading constraints and sexual size dimorphism in Asellus aquaticus. Behaviour 92, 277–287.
Balázs, G., Biró, A., Fišer, Ž., Fišer, C., and Herczeg, G. (2021). Parallel morphological evolution and habitat-dependent sexual dimorphism in cave vs. surface populations of the Asellus aquaticus species complex. Ecol. Evol. 11, 15389–15403. doi: 10.1002/ece3.8233
Bates, D., Mächler, M., Bolker, B., and Walker, S. (2015). Fitting linear mixed-effects models using lme4. J. Stat. Softw. 67, 1–48. doi: 10.18637/jss.v067.i01
Beale, A., Guibal, C., Tamai, T. K., Klotz, L., Cowen, S., Peyric, E., et al. (2013). Circadian rythms in Maxican blind cavefish Astyanax mexicanus in the lab and in the field. Nat. Commun. 4:2769. doi: 10.1038/ncomms3769
Benítez-López, A., Santini, L., Gallego-Zamorano, J., Milá, B., Walkden, P., Huijbregts, M. A. J., et al. (2021). The island rule explains consistent patterns of body size evolution in terrestrial vertebrates. Nat. Ecol Evol 5, 768–786. doi: 10.1038/s41559-021-01426-y
Berisha, H., Horváth, G., Fišer, Ž., Balázs, G., Fišer, C., and Herczeg, G. (2022). Sex-dependent increase of movement activity in the freshwater isopod Asellus aquaticus following adaptation to a predator-free cave habitat. Curr. Zool. doi: 10.1093/cz/zoac063
Bertin, A., and Cezilly, F. (2003). Sexual selection, antennae length and the mating avantage of large males in Asellus aquaticus. J. Evol. Biol. 16, 698–707. doi: 10.1046/j.1420-9101.2003.00565.x
Bertin, A., and Cézilly, F. (2005). Density-dependent influence of male characters on mate-locating efficiency and pairing success in the waterlouse Asellus aquaticus: an experimental study. J. Zool. 265, 333–338. doi: 10.1017/S0952836905006400
Bertin, A., David, B., Cézilly, F., and Alibert, P. (2002). Quantification of sexual dimorphism in Asellus aquaticus (Crustacea: Isopoda) using outline approaches. Biol. J. Linn. Soc. 77, 523–533. doi: 10.1046/j.1095-8312.2002.00125.x
Biddick, M., and Burns, K. C. (2021). A simple null model predicts the island rule. Ecol. Lett. 24, 1646–1654. doi: 10.1111/ele.13781
Blanckenhorn, W. U. (2000). The evolution of body size: what keeps organisms small? Q. Rev. Biol. 75, 385–407. doi: 10.1086/393620
Blanckenhorn, W. U. (2005). Behavioural causes and consequences of sexual size dimorphism. Ethology 111, 977–1016. doi: 10.1111/j.1439-0310.2005.01147.x
Blanckenhorn, W. U., Stillwell, R. C., Young, K. A., Fox, C. V., and Ashton, K. G. (2006). When Rensch meets Bergmann: does sexual size dimorphism change systematically with latitude? Evolution 60, 2004–2011. doi: 10.1111/j.0014-3820.2006.tb01838.x
Bloor, M. C. (2010). Animal standardisation for mixed species ecotoxicological studies: establishing a laboratory breeding programme for Gammarus pulex and Asellus aquaticus. Zool. Baetica 21, 179–190.
Brad, T., Iepure, S., and Sarbu, S. M. (2021). The chemoautotrophically based Movile cave groundwater ecosystem, a hotspot of subterranean biodiversity. Diversity 13:128. doi: 10.3390/d13030128
Case, T. J. (1978). A general explanation of insular body size trends in terrestrial vertebrates. Ecology 59, 1–18. doi: 10.2307/1936628
Christiansen, K. (1962). Proposition pour la classification des animaux cavernicoles. Spelunca 2, 75–78.
Clegg, S. M., and Owens, I. P. F. (2002). The ‘island rule’ in birds: medium body size and its ecological implications. Proc. Roy. Soc. Lond. B 269, 1359–1365. doi: 10.1098/rspb.2002.2024
Clutton-Brock, T. H., Guiness, F. E., and Albon, S. D. (1982). Red deer: Behaviour and ecology of two sexes. Chicago: University of Chicago Press.
Colwell, R. K. (2000). Rensch’s rule crosses the line: convergent allometry of sexual size dimorphismin hummingbirds and flower mites. Am. Nat. 156, 495–510. doi: 10.1086/303406
Culver, D. C., Kane, T. C., and Fong, D. W. (1995). Adaptation and natural selection in caves, the evolution of Gammarus minus. London: Harvard University Press.
Culver, D. C., and Pipan, T. (2009). Biology of caves and other subterranean habitats. Oxford: Oxford University Press.
Darwin, C. (1859). On the origin of species by means of natural selection, or the preservation of favoured races in the struggle for life, 1st. London: John Murray.
Elipot, Y., Hinaux, H., Callebert, J., and Rétaux, S. (2013). Evolutionary shift from fighting to foraging in blind cavefish through changes in the serotonin network. Curr. Biol. 23, 1–10. doi: 10.1016/j.cub.2012.10.044
Fairbairn, D. J. (1997). Allometry for sexual size dimorphism: patterns and process in the coevolution of body size in males and females. Annu. Rev. Ecol. Syst. 28, 659–687. doi: 10.1146/annurev.ecolsys.28.1.659
Fairbairn, D. J., Blanckenhorn, W., and Székely, T. (2007). Sex, size and gender roles–evolutionary studies of sexual dimorphism. Oxford: Oxford University Press.
Fairbairn, D. J., and Preziosi, R. F. (1994). Sexual selection and the evolution of allometry for sexual size dimorphism in the water strider, Aquarius remigis. Am. Nat. 144, 101–118. doi: 10.1086/285663
Fišer, Ž. (2019). “Adaptation to low food” in Encyclopedia of caves. eds. W. B. White, D. C. Culver, and T. Pipan. 3rd ed (London: Academic Press)
Fišer, C., Zagmajster, M., and Zakšek, V. (2013). Coevolution of life history traits and morphology in female subterranean amphipods. Oikos 122, 770–778. doi: 10.1111/j.1600-0706.2012.20644.x
Forsman, A. (1991). Variation in sexual size dimorphism and maximum body size among adder populations: effects of prey size. J. Anim. Ecol. 60, 253–267. doi: 10.2307/5458
Hasu, T., Holmes, J. C., and Valtonen, E. T. (2007). Isopod (Asellus aquaticus) size and Acanthocepalan (Acanthocephalus licii) infections. J. Parasitol. 93, 450–457. doi: 10.1645/GE-1032R.1
Herczeg, G., Gonda, A., Kuparinen, A., and Merilä, J. (2012). Contrasting growth strategies of pond versus marine populations of nine-spined stickleback (Pungigius pungitius): a combined effect of predation and competition? Evol. Ecol. 26, 109–122. doi: 10.1007/s10682-011-9491-9
Herczeg, G., Gonda, A., and Merilä, J. (2009). Evolution of gigantism in nine-spined sticklebacks. Evolution 63, 3190–3200. doi: 10.1111/j.1558-5646.2009.00781.x
Herczeg, G., Gonda, A., and Merilä, J. (2010). Rensch’s rule inverted—female-driven gigantism in nine-spined stickleback (Pungitius pungitius). J. Anim. Ecol. 79, 581–588. doi: 10.1111/j.1365-2656.2010.01665.x
Herczeg, G., Hafenscher, V. P., Balázs, G., Fišer, Z., Kralj-Fišer, S., and Fišer, C. (2020). Is foraging innovation lost following colonisation of a less variable environment? A case study in surface- vs. cave-dwelling Asellus aquaticus. Ecol. Evol. 10, 5323–5331. doi: 10.1002/ece3.6276
Herczeg, G., Nyitrai, V., Balázs, G., and Horváth, G. (2022). Food preference and food type innovation of surface- vs. cave-dwelling waterlouse (Asellus aquaticus) after 60000 years of isolation. Behav. Ecol. Sociobiol. 76:1. doi: 10.1007/s00265-021-03109-x
Horváth, G., Kerekes, K., Nyitrai, V., Balázs, G., Berisha, H., and Herczeg, G. (2023). Exploratory behaviour divergence between surface populations, cave colonists and a cave population in the water louse, Asellus aquaticus. Behav. Ecol. Sociobiol. 77:15. doi: 10.1007/s00265-022-03288-1
Horváth, G., Sztruhala, S. S., Balázs, G., and Herczeg, G. (2021). Population divergence in aggregation and sheltering behaviour in surface- vs. cave-adapted Asellus aquaticus (Crustacea: Isopoda). Biol. J. Linn. Soc. 134, 667–678. doi: 10.1093/biolinnean/blab093
Howarth, F. G., and Moldovan, O. T. (2018). “The ecological classification of cave animals and their adaptations” in Cave ecology. eds. O. T. Moldovan, Ľ. Kováč, and S. Halse (Cham: Springer), 23–37.
Konec, M., Prevočnik, S., Sarbu, S. M., Verovnik, R., and Trontelj, P. (2015). Parallels between two geographically and ecologically separate cave invasions by the same species, Asellus aquaticus (Isopoda, Crustacea). J. Evol. Biol. 28, 864–875. doi: 10.1111/jeb.12610
Kratochwíl, L., and Frynta, D. (2002). Body size, male combat, and the evolution of sexual dimorphism in eublepharid geckos (Squamata: Eublepharidae). Biol. J. Linn. Soc. 76, 303–314. doi: 10.1046/j.1095-8312.2002.00064.x
Kuznetsova, A., Brockhoff, P. B., and Christensen, R. H. B. (2017). lmerTest package: tests in linear mixed effects models. J. Stat. Softw. 82, 1–26. doi: 10.18637/jss.v082.i13
Lafuente, E., Lürig, M. D., Rövekamp, M., Matthews, B., Buser, C., Vorburger, C., et al. (2021). Building on 150 years of knowledge: the freshwater isopod Asellus aquaticus as an integrative eco-evolutionary model system. Front. Ecol. Evol. 9:748212. doi: 10.3389/fevo.2021.748212
Legendre, P. (2018). lmodel2: Model II Regression. R package version 1.7-3. Available at: https://CRAN.R-project.org/package=lmodel2
Lenth, R. V. (2020). Emmeans: estimated marginal means, aka least-squares means. R package version 1.3. Available at: https://CRAN.R-project.org/package=emmeans
Liang, T., Meiri, S., and Shi, L. (2022). Sexual size dimorphism in lizards: Rensch’s rule, reproductive mode, clutch size, and line fitting method effects. Integr. Zool. 17, 787–803. doi: 10.1111/1749-4877.12569
Liao, W. B., Liu, W. C., and Merilä, J. (2015). Andrew meets Rensch: sexual size dimorphism and the inverse of Rensch’s rule in Andrew’s toad (Bufo andrewsi). Oecologia 177, 389–399. doi: 10.1007/s00442-014-3147-8
Lomolino, M. V. (1985). Body size of mammals on islands: the island rule re-examined. Am. Nat. 125, 310–316. doi: 10.1086/284343
Lomolino, M. V. (2005). Body size evolution in insular vertebrates: generality of the island rule. J. Biogeogr. 32, 1683–1699. doi: 10.1111/j.1365-2699.2005.01314.x
Lunghi, E., and Bilandžija, H. (2022). Longevity in cave animals. Front. Ecol. Evol. 10:874123. doi: 10.3389/fevo.2022.874123
MacColl, A. D. C., Nagar, A. E., and de Roij, J. (2013). The evolutionary ecology of dwarfism in three-spined sticklebacks. J. Anim. Ecol. 82, 642–652. doi: 10.1111/1365-2656.12028
Mammola, S. (2019). Finding answers in the dark: caves as models in ecology fifty years after Poulson and White. Ecography 42, 1331–1351. doi: 10.1111/ecog.03905
Mammola, S., Enrico, L., Helena, B., Pedro, C., Volker, G., Susanne, I., et al. (2021). Collecting eco-evolutionary data in the dark: impediments to subterranean research and how to overcome them. Ecol. Evol. 11, 5911–5926. doi: 10.1002/ece3.7556
McNab, B. K. (1994). Resource use and the survival of land and freshwater vertebrates on oceanic islands. Am. Nat. 144, 643–660. doi: 10.1086/285698
Meiri, S., and Liang, T. (2021). Rensch’s rule – definitions and statistics. Glob. Ecol. Biogeogr. 30, 573–577. doi: 10.1111/geb.13255
Mojaddidi, H., Fernandez, F. E., Erickson, P. A., and Protas, M. E. (2018). Embryonic origin and genetic basis of cave associated phenotypes in the isopod crustacean Asellus aquaticus. Sci. Rep. 8:16589. doi: 10.1038/s41598-018-34405-8
Payton, M. E., Greenstone, M. H., and Schenker, N. (2003). Overlapping confidence intervals or standard errors: what do they mean in terms of statistical significance? J. Insect Sci. 3:34. doi: 10.1093/jis/3.1.34
Pérez-Moreno, J. L., Balázs, G., Wilkins, B., Herczeg, G., and Bracken-Grissom, H. D. (2017). The role of isolation on contrasting phylogeographic patterns in two cave crustaceans. BMC Evol. Biol. 17:247. doi: 10.1186/s12862-017-1094-9
Peters, R. H. (1983), The ecological implications of body size. Cambridge: Cambridge University Press.
Pipan, T., and Culver, D. C. (2012). Convergence and divergence in the subterranean realm: a reassessment. Biol. J. Linn. Soc. 107, 1–14. doi: 10.1111/j.1095-8312.2012.01964.x
Piross, I. S., Harnos, A., and Rózsa, L. (2019). Rensch’s rule in avian lice: contradictory allometric trends for sexual size dimorphism. Sci. Rep. 9:7908. doi: 10.1038/s41598-019-44370-5
Poulson, T., and Lavoie, K. (2000). “The trophic basis of subsurface ecosystems” in Ecosystems of the world: Subterranean ecosystems. eds. H. Wilkens, D. C. Culver, and F. W. Humphreys (New York: Elsevier), 231–249.
Poulson, T. L., and White, W. B. (1969). The cave environment. Science 165, 971–981. doi: 10.1126/science.165.3897.971
R Core Team (2020). R: A language and environment for statistical computing. R Foundation for Statistical Computing, Vienna, Austria.
Rensch, B. (1950). Die Abhängigkeit der relativen Sexualdifferenz von der Körpergrösse. Bonn. Zool. Beitr. 1, 58–69.
Ridley, M., and Thompson, D. J. (1979). Size and mating in Asellus aquaticus (Crustacea, Isopoda). Z. Tierpsychol. 51, 380–397.
Romero, A. (2011). The evolution of cave life: new concepts are challenging conventional ideas about life underground. Am. Sci. 99, 144–151. doi: 10.1511/2011.89.144
Runemark, A., Sagonas, K., and Sevensson, E. I. (2015). Ecological explanations to island gigantism: dietary niche divergence, predation, and size in an endemic lizard. Ecology 96, 2077–2092. doi: 10.1890/14-1996.1
Sarbu, S. M., Kane, T. C., and Kinkle, B. K. (1996). A chemoatotrophically based cave ecosystem. Science 272, 1953–1955. doi: 10.1126/science.272.5270.1953
Shine, R. (1989). Ecological causes for the evolution of sexual size dimorphism: a review of the evidence. Q. Rev. Biol. 64, 419–461. doi: 10.1086/416458
Simberloff, D., Dayan, T., Jones, C., and Ogura, G. (2000). Character displacement and release in the small Indian mongoose, Herpestes javanicus. Ecology 81, 2086–2099. doi: 10.1890/0012-9658(2000)081[2086:CDARIT]2.0.CO;2
Simčič, T., and Sket, B. (2019). Comparison of some epigean and troglobiotic animals regarding their metabolism intensity. Examination of a classical assertion. Int. J. Speleol. 48, 133–144. doi: 10.5038/1827-806X.48.2.2251
Sket, B. (1994). Distribution of Asellus aquaticus (Crustacea: Isopoda: Asellidae) and its hypogean populations at different geographic scales, with a note on Proasellus istrianus. Hydrobiologia 287, 39–47. doi: 10.1007/BF00006895
Stamps, J. A., and Andrews, R. M. (1992). Estimating asymptotic size using the largest individual per sample. Oecologia 92, 503–512. doi: 10.1007/BF00317842
Székely, T., Freckleton, R. B., and Reynolds, J. D. (2004). Sexual selection explains Rensch’s rule of size dimorphism in shorebirds. Proc. Natl. Acad. Sci. U. S. A. 101, 12224–12227. doi: 10.1073/pnas.0404503101
Thiel, M., and Duffy, J. E. (2007). “The behavioural ecology of crustaceans: a primer in taxonomy, morphology and biology,” in Evolutionary Ecology of Social and Sexual Systems: Crustaceans as Model Organisms. eds. J. E. Duffy and M. Thiel (New York: Oxford University Press).
Thompson, D. J. (1986). Heritability for body size in the isopod Asellus aquaticus (L.). Crustaceana 51, 241–244. doi: 10.1163/156854086X00386
Turk-Prevorčnik, S., and Blejec, A. (1998). Asellus aquaticus infrenus, new subspecies (Isopoda: Asellota: Asellidae), from Romanian hypogean waters. J. Crust. Biol. 18, 763–773. doi: 10.1163/193724098X00647
Van Valen, L. M. (1973a). Body size and the number of plants and animals. Evolution 27, 27–35. doi: 10.2307/2407116
Verovnik, R., and Konec, M. (2019). “Asellus aquaticus: a model system for historical biogeography” in Encyclopedia of caves. eds. W. B. White, D. C. Culver, and T. Pipan. 3rd ed (London: Elsevier Academic), 76–84.
Verovnik, R., Prevorčnik, S., and Jugovic, J. (2009). Description of a neotype for Asellus aquaticus Linné, 1758 (Crustacea: Isopoda: Asellidae), with description of a new subterranean Asellus species from Europe. Zool. Anz. 248, 101–118. doi: 10.1016/j.jcz.2009.03.001
Verovnik, R., Sket, B., Prevorčnik, S., and Trontelj, P. (2003). Random amplified polymorphic DNA diversity among surface and subterranean populations of Asellus aquaticus (Crustacea: Isopoda). Genetica 119, 155–165. doi: 10.1023/A:1026085716777
Verovnik, R., Sket, B., and Trontelj, P. (2004). Phylogeography of subterranean and surface populations of water lice Asellus aquaticus (Crustacea: Isopoda). Mol. Ecol. 13, 1519–1532. doi: 10.1111/j.1365-294X.2004.02171.x
Voituron, Y., de Fraipont, M., Issartel, J., Guillaume, O., and Clobert, J. (2011). Extreme lifespan of the human fish (Proteus anguinus): a challenge for ageing mechanisms. Biol. Lett. 7, 105–107. doi: 10.1098/rsbl.2010.0539
Wasserzug, R. J., Yang, H., Sepkoski, J. J., and Raup, D. M. (1979). The evolution of body size on islands: a computer simulation. Am. Nat. 114, 287–295. doi: 10.1086/283475
Webb, T. J., and Freckleton, R. P. (2007). Only half right: species with femalebiased sexual size dimorphism consistently break Rensch’s rule. PLoS One 2:e897. doi: 10.1371/journal.pone.0000897
Wilson, D. S. (1975). The adequacy of body size as a niche difference. Am. Nat. 109, 769–784. doi: 10.1086/283042
Wu, H., Jiang, T., Huang, X., Lin, H., Wang, H., Wang, L., et al. (2014). A test of Rensch’s rule in greater horseshoe bats (Rhinolophus ferrumequineum) with female-biased sexual size dimorphism. PLoS One 9:e86085. doi: 10.1371/journal.pone.0086085
Wu, Z., Li, Y., and Murray, B. R. (2006). Insular shifts in body size of rice frogs in the Zhoushan archipelago, China. J. Anim. Ecol. 75, 1071–1080. doi: 10.1111/j.1365-2656.2006.01126.x
Yu, T. L., Li, Y., and Zhang, J. D. (2022). Evolution of sexual dimorphism in the plateau brown frog fails to obey Rensch’s rule. Herp. J. 32, 27–33. doi: 10.33256/32.1.2733
Keywords: competition, body size, isopod, predation, sexual size dimorphism, adaptation, evolution
Citation: Herczeg G, Balázs G, Biró A, Fišer &, Kralj-Fišer S and Fišer C (2023) Island and Rensch’s rules do not apply to cave vs. surface populations of Asellus aquaticus. Front. Ecol. Evol. 11:1155261. doi: 10.3389/fevo.2023.1155261
Edited by:
Enrico Lunghi, University of L'Aquila, ItalyCopyright © 2023 Herczeg, Balázs, Biró, Fišer, Kralj-Fišer and Fišer. This is an open-access article distributed under the terms of the Creative Commons Attribution License (CC BY). The use, distribution or reproduction in other forums is permitted, provided the original author(s) and the copyright owner(s) are credited and that the original publication in this journal is cited, in accordance with accepted academic practice. No use, distribution or reproduction is permitted which does not comply with these terms.
*Correspondence: Gábor Herczeg, Z2Fib3IuaGVyY3plZ0B0dGsuZWx0ZS5odQ==
†ORCID: Gábor Herczeg, https://orcid.org/0000-0003-0441-342X
Gergely Balázs, https://orcid.org/0000-0001-6372-387X
Anna Biró, https://orcid.org/0000-0001-8106-4716
Žiga Fišer, https://orcid.org/0000-0003-4576-5173
Simona Kralj-Fišer, https://orcid.org/0000-0001-6604-2332
Cene Fišer, https://orcid.org/0000-0003-1982-8724