- 1‘Lendület’ Landscape and Conservation Ecology, Institute of Ecology and Botany, Centre for Ecological Research, Vácrátót, Hungary
- 2MTA-SZTE ‘Momentum’ Applied Ecology Research Group, University of Szeged, Szeged, Hungary
- 3‘Lendület’ Seed Ecology Research Group, Institute of Ecology and Botany, Centre for Ecological Research, Vácrátót, Hungary
Introduction: Land use changes have seriously fragmented grasslands leading to extensive biodiversity loss worldwide. Habitat fragmentation affects grasslands at both local and landscape scales, adversely affecting the probability of species colonisation and extinction. In our study, we addressed the effects of fragment size and landscape-scale habitat connectivity on the vegetation composition in two grassland types, i.e., loess steppe fragments (situated on kurgans) and sand steppe fragments of the Pannonian forest steppe region.
Methods: We collected frequency data on vascular plant species in 12 1 m × 1 m quadrats in altogether 60 fragments along a connectivity gradient in sand steppes and loess steppe fragments. We analysed whether habitat specialists, generalists and exotic species were affected by the level of fragmentation based on species richness and traits related to local persistence (life span, clonality and soil seed bank type) and dispersal (dispersal strategy and seed mass) in the two grassland types using linear mixed-effects models.
Results: Based on our results, both fragment size and landscape-scale connectivity were important in shaping the trait composition of the vegetation. We observed more fragmentation effects in generalist than in specialist species. We found that isolation resulted in fewer specialist species with autochorous dispersal strategy in loess steppe fragments, but, at the same time, also fewer exotics. Isolated loess steppe fragments harboured fewer generalist species with persistent seed bank. Large loess steppe fragments supported more wind-dispersed species than smaller ones. In isolated sand steppe fragments, generalists were more frequent with endozoochorous dispersal strategy and without clonal propagation. Life span, clonal propagation and seed mass did not depend on the level of fragmentation in the two grassland types.
Discussion: Our results imply that both sand and loess steppe fragments can rely to some extent on the persistence of clonal perennial specialist and generalist species in small and isolated patches to mitigate fragmentation effects. In conclusion, these processes should be supported by the preservation of large fragments, increase of habitat connectivity combined with targeted management of exotic species.
1. Introduction
Land use change is one of the primary drivers of biodiversity loss worldwide, causing degradation and reduced habitat availability (Isbell et al., 2022). Temperate grasslands represent exceptionally high conservation values as they are among the most species-rich communities when considering small (<50 m2) spatial scales (Wilson et al., 2012). However, they have been increasingly fragmented due to ploughing and afforestation (Habel et al., 2013). Despite the drastic level of habitat loss, grasslands still contribute significantly to global and local biodiversity by harbouring many endangered animal and plant species confined to these habitats (Squires and Feng, 2018). Species preservation is essential to provide an insurance biodiversity capacity to reduce the risk caused by the temporal variability of ecosystem processes under current global changes (Loreau et al., 2001).
Habitat fragmentation may affect species at both local and larger spatial scales (Auffret et al., 2018). At the landscape scale, the compositional and configurational heterogeneity of the surrounding habitats shape the species pool by affecting diaspore flow (Gaujour et al., 2012). At the local scale, species pool is filtered primarily by altered abiotic conditions and species interactions (Maurer et al., 2003). Increasing fragmentation leads to an increased distance among habitat patches as it can considerably limit dispersal processes (Taylor et al., 1993) and reduce gene flow (Heinken and Weber, 2013). At the local scale, fragmentation results in reduced habitat patch sizes (Uroy et al., 2019) increasing the extinction risk of species populations due to reduced habitat quality and stronger edge effects (Soons et al., 2005). Unpredictable catastrophic events and demographic stochasticity can also lead to population extinctions more frequently in small patches (Turner, 1996). As isolation and fragment size reduction can amplify each other’s effect (Didham et al., 2012), we can gain deeper insights with their joint assessment.
Functional plant traits are effective tools for investigating fragmentation effects offering more general and mechanistic insights into ecosystem processes and higher predictability than taxonomic approaches (McGill et al., 2006). Colonisation and extinction processes cause direct changes in community composition in the course of fragmentation (Jackson and Sax, 2010). These processes are driven by species-specific functional traits linked to population biological processes (Janečková et al., 2017). We might expect that species with efficient propagule dispersal or local persistence traits are more successful and well-preserved in habitat fragments than species without these traits (Marini et al., 2012).
Dispersal potential can be best captured by assessing the main dispersal modes and propagule characteristics of the species, such as seed mass, shape and size (Ottaviani et al., 2020). The long-distance dispersal ability is critically important for species survival in fragmented landscapes (Bacles et al., 2006). Species dispersing by wind or animals efficiently are assumed to be able to colonise more isolated fragments (Vittoz and Engler, 2007). Species with small seed mass have higher dispersal potential by wind because light seeds have lower terminal velocity (Lindborg et al., 2012), whereas heavy seeds with appendices may disperse by animals more effectively (Amartuvshin et al., 2019). Plant species with high dispersal potential are expected to be less vulnerable to fragmentation (Eriksson, 1996), although some studies detected similar (Hemrová and Münzbergová, 2015) or even higher sensitivity to fragmentation of long-distance than short-distance dispersers (Deák et al., 2018).
Long life span, clonal reproduction and persistent soil seed bank are the main traits that are likely to contribute to local persistence (Maurer et al., 2003). Long-lived species are assumed to be less susceptible to fragmentation because of their smaller fluctuations in abundance (Heinken and Weber, 2013). Species capable of clonal propagation with a potentially unlimited life span are not dependent on sexual reproduction for population survival; therefore, they are expected to be less impacted by isolation (Heinken and Weber, 2013). However, environmental suppression of sexual propagation can also lead to monoclonal populations lowering long-term population viability (Honnay and Bossuyt, 2005). The persistent seed bank in the soil also enables plant populations to survive disturbances and tolerate environmental fluctuations, thus prolonging species persistence in the community (Eriksson, 1996). Based on the contradictory results of the different case studies, fragmentation effects on plant traits seem to be context and habitat specific, highlighting the need to assess them in various ecosystems (Heinken and Weber, 2013).
Inspecting only total species richness patterns can hide important aspects of underlying mechanisms of responses to fragmentation (Lindborg et al., 2012). Depending on habitat specialisation, fragmentation effects vary on plant species (Yan et al., 2022). Specialist species have narrow environmental tolerances and thus are limited to specific habitat types (Pandit et al., 2009), and are more susceptible to disturbances than generalist species (Vázquez and Simberloff, 2002). Habitat generalists are important grassland constituents contributing to the total richness and abundance of the vegetation but do not indicate directly good or bad ecological status (Deák et al., 2020). Generalists can persist under a wide range of abiotic conditions; therefore, they are assumed to be less affected by fragmentation (Yan et al., 2022). Non-indigenous species may behave similarly to generalists but have exceptionally high practical importance as drivers of unfavourable ecosystem changes and degradation indicators (Didham et al., 2005). In this paper, we used species groups based on habitat specialisation and origin to understand how the functional composition of the vegetation changes due to fragmentation.
The forest-steppe fragments of the Pannonic biogeographical region provide an ideal setting to assess the effects of fragmentation. The region is the westernmost part of the Eurasian forest-steppe biome, a distinct vegetation zone between closed forests and steppes in subhumid-semi arid climate zones (Erdős et al., 2018a,b). The vegetation is of a mosaic nature where both the forest and steppe are stable vegetation components (Fekete et al., 2002). Two substrate types, sand and loess, are widely distributed in the region, and both harbour valuable habitats listed as Natura 2000 priority habitat types (Pannonic loess steppic grasslands and Pannonic sand steppes) in Annex I of EU’s Habitat Directive (European Commission, 2013). Loess and sand steppes represent two extremes in terms of abiotic conditions and productivity (Molnár et al., 2012). Sandy substrates create highly stressed abiotic conditions, whereas water and nutrient cycling is more favourable for plant life on loess (Fekete et al., 2002). Loess and sand steppes have been exposed to extensive anthropogenic habitat loss and fragmentation (Gallé et al., 2022). Because of their clearly defined borders and unsuitable matrix, they can be regarded as terrestrial habitat islands (Deák et al., 2016), serving as excellent model systems to study the effects of fragmentation (Ottaviani et al., 2020).
In our study, we tested if species groups with different origin and habitat specialisation (i.e., specialists, generalists and exotics) show general response patterns against fragmentation in two contrasting steppe types of the Pannonian forest steppe region. We focused on the vegetation of open sand steppe and loess steppe fragments on forest steppes and kurgans (ancient burial mounds) in the Great Hungarian Plain. We assessed the effects of fragment size (large vs. small fragments) and landscape-scale connectivity (Hanski’s connectivity index) on the richness and frequency of species with traits related to local persistence (life span, clonal propagation and soil seed bank type) and propagule dispersal (dispersal strategy and seed mass). We expected that (i) specialist plant species would experience more significant effects of connectivity and fragment size than generalists or exotics because they are more dependent on the fragmented steppe habitats; (ii) the importance of connectivity would be higher for dispersal-limited species and (iii) fragment size would affect local persistence trait distribution primarily.
2. Materials and methods
2.1. Study area
Our study region lies in the Southern part of the Great Hungarian Plain (Figure 1). This is a lowland region with a continental climate characterised by mean annual temperature of 11°C and mean annual precipitation of 550 mm. The semi-natural vegetation of the Pannonic forest-steppe zone can be characterised by a mosaic of dry and open oak (Quercus spp.), and poplar (Populus spp.) forests and Festuca and Stipa species dominated steppes (Molnár et al., 2012). Here we focused on two major grassland components of the vegetation, i.e., open sand steppe and loess steppe.
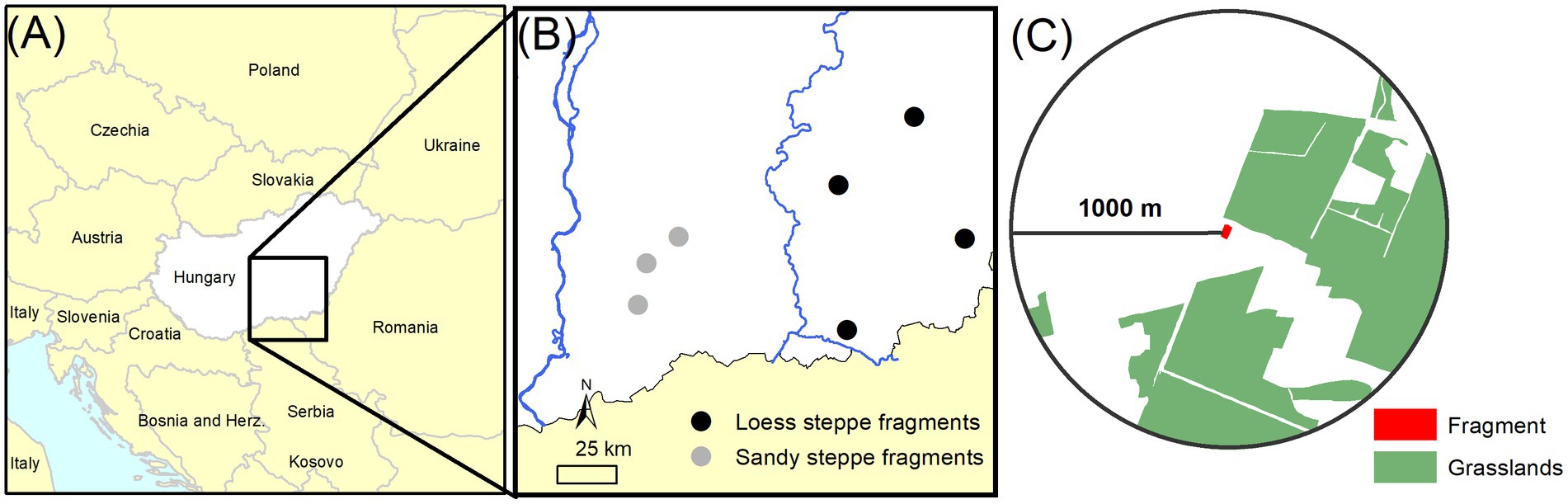
Figure 1. (A) Map of Central Europe showing Hungary, (B) map of the Great Hungarian Plain in Southeast Hungary with the study sites and (C) a loess fragment study site with the 1,000 m-buffer that was used to estimate Hanski’s connectivity. Note that for sand steppe fragments a 500 m-buffer was used.
Open sand steppes are endemic communities (Festucetum vaginatae) to the Pannonic biogeographical region. They are semi-desert-like perennial grasslands occurring in inland sand dune ridges. The average cover of vascular plants is relatively sparse, ranging between 40 and 70% (Erdős et al., 2018a,b). The rest of the surface is usually covered by cryptogams (lichens and mosses) and bare ground. Tussock-forming Festuca vaginata and Stipa borysthenica dominate the vegetation, with many rare and threatened forbs confined to this vegetation type (Csecserits et al., 2011). The soil is wind-blown arenosol with extremely low (below 3%) humus content and low water-retention capacity (Csecserits et al., 2011). Because of the low productivity of the soil, open sand steppes were mostly grazed by sheep or remained unmanaged until the 19th century (Molnár et al., 2012). In the 1920s, intensive lowland afforestation programmes started, and by the end of the 20th century, most of the open sand grasslands were converted to non-native tree plantations in the region (Biró et al., 2008).
Loess steppes (Salvio-Festucetum rupicolae) are highly productive perennial vegetation types developed on chernozem soil (Fekete et al., 2002). The vegetation is diverse, dominated by several perennial grasses (e.g., Festuca rupicola, Brachypodium pinnatum and Stipa spp.) and are rich in threatened forbs (Deák et al., 2016). Because of their favourable edaphic conditions and flat topography, loess steppe transformation most probably started already in the Neolithic, and was accelerated from the 18th century due to agricultural expansion and intensification (Deák et al., 2021a,b). It resulted in the loss of 90% of their original area by the end of the 20th century (Deák et al., 2016). In this study, we focused on kurgans, i.e., ancient burial mounds created in the IV-I millennia BC (Deák et al., 2016). Kurgans usually take on a hemispherical shape with a height ranging between 0.5 and 15 m, and a diameter between 10 and 110 m (Dembicz et al., 2021). As most kurgans are small and isolated in the region, they are usually left unmanaged resulting in the survival of semi-natural vegetation but also unfavourable spontaneous shrub encroachment and litter accumulation (Deák et al., 2016).
The sand and loess steppe fragments of our study could be characterised by contrasting surrounding landscape (Figure 1). The sand fragments were embedded into the matrix of small-scale plantation forests planted with non-native Pinus sylvestris, P. nigra and Robinia pseudoacacia trees, and some native Populus canescens (Biró et al., 2008). These were even-aged plantation forests created after ploughing and harvested with clear-cutting (Csecserits et al., 2016). The loess fragments were surrounded by intensively used large-scale agricultural fields, with some farmhouses and pastures. The region’s main crops are winter cereals, maize, sunflower and alfalfa (Gallé et al., 2022).
2.2. Study sites
We selected 15 small and 15 large fragments for each steppe type along a connectivity gradient (Figure 1). We considered fragment size as a patch-scale characteristic and connectivity as a landscape-scale predictor. We visited 160 fragments around three (Fischerbócsa, Kéleshalom and Pirtó) and four villages (Dévaványa, Kunágota, Makó and Szentes) for sand and loess steppes, respectively. The available fragment sizes differed in the two types; therefore, we applied differing size ranges for the selection. For sand steppes, the size of the small and large fragments ranged between 0.16 and 0.48 ha, and 0.93–6.88 ha, respectively. In the case of loess steppes, the size of the small and large fragments varied between 0.01–0.10 ha, and 0.20–0.44 ha, respectively.
For measuring connectivity, we calculated Hanski’s connectivity index (Hanski and Ovaskainen, 2000) for all potential fragments:
where dij stands for the shortest edge-to-edge distance (in km) between fragment i and j within the search radius, Aj is the size of habitat patch j (in m2), α is a constant scaling the species-specific dispersal ability over distance dij and β is an emigration parameter scaled over the size of patch j as a source of migration (Moilanen and Hanski, 2001). Following Rösch et al. (2013), both parameters were set to 0.5 as we applied the index to entire communities. The grain size of the landscape matrix differed in the two steppe types, with a small-scale forest plantation matrix in the first, and a large-scale agricultural matrix in the latter case (Kuli-Révész et al., 2021). Therefore, we applied different buffer radii for the connectivity index calculations (500 for sand and 1,000 m for loess steppes) based on the ecosystem base map of Hungary (Tanács et al., 2021) and Google aerial photographs by using Quantum GIS 3.6.1 (QGIS Development Team, 2019). We considered all semi-natural vegetation patches (open sand grasslands, loess steppe, other forest-steppe fragments and alkali grasslands) as sources of immigration. The connectivity values ranged between 24 and 811 for sand steppe (mean = 394, SD = 206), and from 0 to 2,637 for loess steppe fragments (mean = 689, SD = 748).
2.3. Vegetation sampling
We sampled the vegetation in 12 1 × 1 m quadrats in each fragment. Six quadrats were placed 1–2 m from fragment edges, and six were positioned in the centre to cover habitat heterogeneity. They were located at least 5 m from each other to avoid spatial autocorrelation. We recorded the presence of each vascular plant species in the quadrats in May–June 2019 and 2020. We summed up the occurrences of each plant species for all quadrats in the two sampling periods for each fragment. Thus, the frequency of a species (used as a proxy for abundance) could range between zero and 24 in a fragment.
We divided the plant species according to their habitat specialisation into the following categories: (i) steppe specialists with a regional preference for open sand or loess steppes; (ii) generalist species including all other native species not confined exclusively to sand or loess steppe habitats and (iii) exotic species. Species were assigned to groups based on field guides and expert knowledge (Király, 2009; Gallé et al., 2022). We assessed five traits related to local persistence and dispersal potential to reveal whether functional traits of the three species groups respond differently to local- and landscape-scale fragmentation effects in the two grassland types. We chose traits that are rather stable within species because we collected trait values from databases that were not observed in the field.
For local persistence, these were the following: life span, clonal propagation and soil seed bank longevity. Life span was categorised into two levels, short-lived species, including annuals and biennials, and long-lived species. The life span of some species could be both short and long. For these, we used the most common life span category according to Király (2009). Clonality was used as a categorical trait with two values (clonal and non-clonal). We differentiated between transient and persistent soil seed bank types, where transient stands for longevity shorter than 1 year and persistent longer than a year, including short- and long-term persistent seed banking. In cases where more than one value was available, we assigned the longer term value for the species. We used seed dispersal strategies as a functional trait related to diaspore dispersal potential, according to Sádlo et al. (2018). They described dispersal mode combinations with different relative importance corresponding to similar diaspore morphological features and ecological demands of the species. They characterised nine strategies based on dispersal mode combinations. In our fragments, four strategy types occurred [dispersal strategy names follow the nomenclature suggested by Sádlo et al. (2018)]: (i) Allium type was the most common strategy with mainly autochory and to a small degree epi-, endozoochory and anemochory; (ii) Bidens type is dominated by autochory and epizoochory, and to a lesser extent endozoochory; (iii) in Cornus type, species disperse predominantly with autochory and endozoochory and (iv) Epilobium type can be characterised by anemochory beside autochory, and rarely zoochory. Seed mass has an important role in both local persistence and dispersal potential, and we considered it in relation to both processes. Seed mass was measured as thousand seed weight. Trait values were collected from various databases such as PADAPT (Sonkoly et al., 2022), Pladias (Wild et al., 2019), LEDA (Kleyer et al., 2008), CLO-PLA (Klimešová and de Bello, 2009), TRY (Kattge et al., 2020) and regional seed mass records (Csontos et al., 2003, 2007; Török et al., 2013, 2016), and the field guide of the Hungarian flora (Király, 2009). For details of species list and trait values see Supplementary Table 1.
2.4. Statistical analyses
We analysed the effects of fragment size and connectivity and their interaction on the richness of the three species groups (i.e., specialists, generalists and exotics) and the richness and frequency of plant species with different trait categories. Dispersal strategy categories with less than three species were not tested statistically (i.e., Bidens type and Cornus type specialists and exotics except for Cornus type exotics in loess steppe fragments). For each fragment, we calculated the number and frequency of species assigned to each species group and trait category. We also quantified the fragment-level community-weighted mean for the seed mass weighted by species frequency. Statistical analyses were performed using R version 4.1.1 (R Core Team, 2022). We implemented the analyses for the two grassland types separately. We modelled the relationships with generalised linear mixed-effects models (GLMMs) using Poisson error terms and log link function for the categorical traits (Bates et al., 2021) and linear mixed-effects models for the continuous variable, community-weighted mean of seed mass after log transformation (Pinheiro et al., 2017). Hanski’s connectivity index values were log-transformed and ranged between 0 and 1. We treated fragment size as a categorical fixed variable (small vs. large), the ranged connectivity index as a continuous explanatory variable and location (village) served as a random factor in the models. The models were visually inspected for violations of homoscedasticity by checking residuals against fitted values. We tested the models for overdispersion and zero inflation using the DHARMa package (Hartig, 2022). When we detected overdispersion, we recalculated the models with negative binomial error term. In the case of significant zero-inflation, we refitted the model as a zero-inflated Poisson or negative binomial model using fragment size as the explanatory variable in the zero part of the model, while fragment size and connectivity and their interaction were used in the non-zero part of the models using the glmmTMB package (Brooks et al., 2017). We calculated the conditional coefficient of determination (i.e., variance explained by the entire model including both the fixed and random effects) for the models via the delta method with the MuMIn package (Barton, 2022). Data were visualised using the ggplot2 package (Wickham, 2011).
3. Results
We recorded a total of 178 species in the sand steppe fragments and 270 species in the loess grassland fragments during the 2 years of sampling (Table 1). Sandy fragments harboured 67 specialists, 97 generalists and 14 exotic species, while loess grassland fragments were more diverse, with altogether 43 specialist, 193 generalist and 34 exotic species. The mean richness was 22.3 (SD = 3.9) for specialist, 32.7 (SD = 7.9) for generalist and 4.4 (SD = 1.7) for exotic species in the sand steppe fragments, and 4.4 (SD = 2.9), 54.3 (SD = 8.0) and 5.5 (SD = 2.7) in the loess steppe fragments, respectively. We found that the specialist species pool was dominated by perennial and clonal species as opposed to generalist species in both grassland types (Table 1). In both grassland types, species with a persistent seed bank and autochory-dominated Allium-type dispersal strategy dominated the vegetation in every species group. The average seed mass was the lowest in the case of specialists and the highest in exotics in both grassland types.
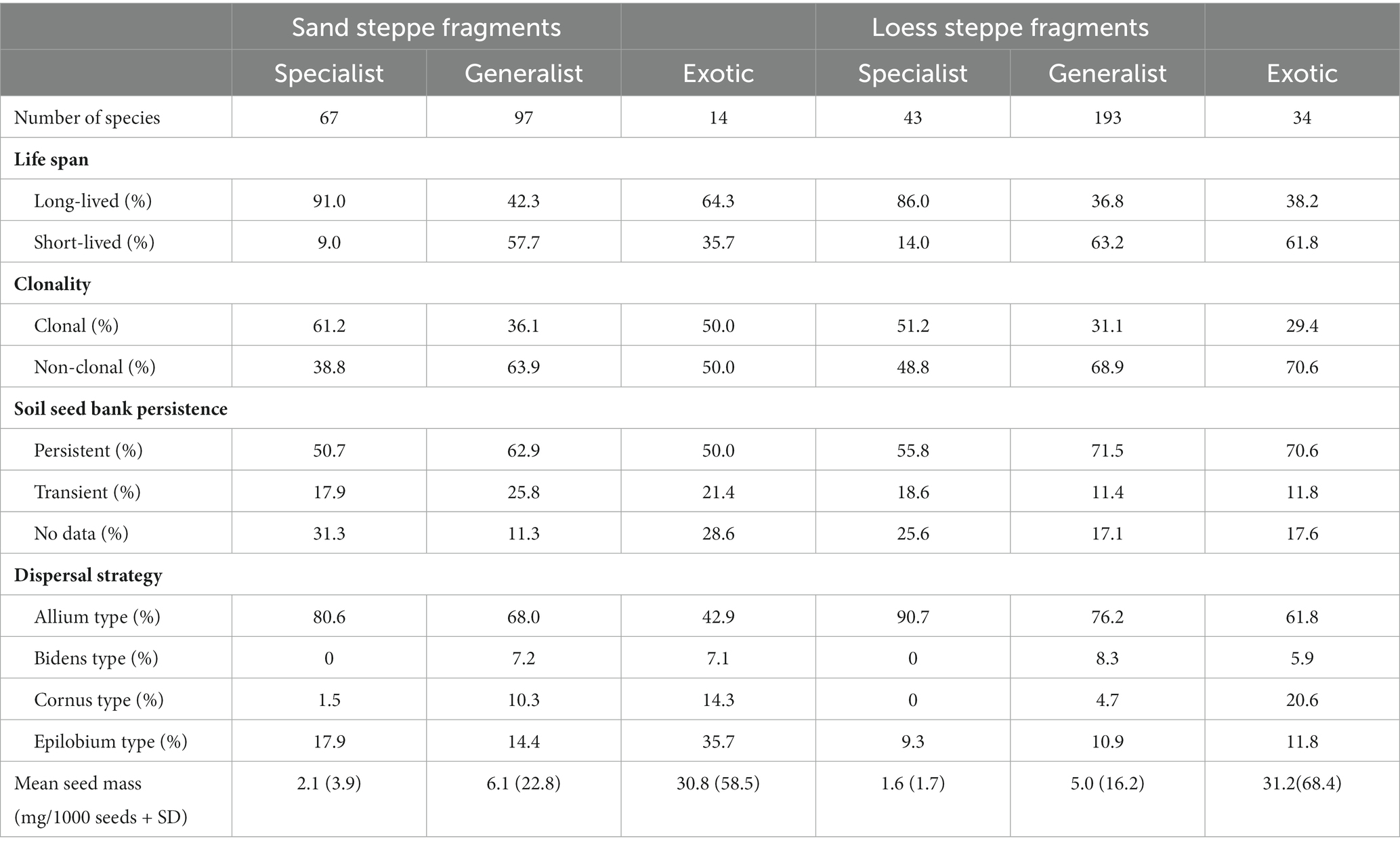
Table 1. Number of species occurred in each species groups, percentage of species assigned to each category of local persistence and dispersal potential traits, and average thousand seed weight of the species for each species groups found in sand and loess steppe fragments.
In the sand steppe fragments, we found no effect of either fragment size or connectivity on the richness of the three species groups (Supplementary Table 2). In contrast, the species richness of specialist and exotic species was higher with 69 and 31% in the most connected fragments compared to the most isolated ones in loess steppe fragments regardless of their size (Figures 2A,B).
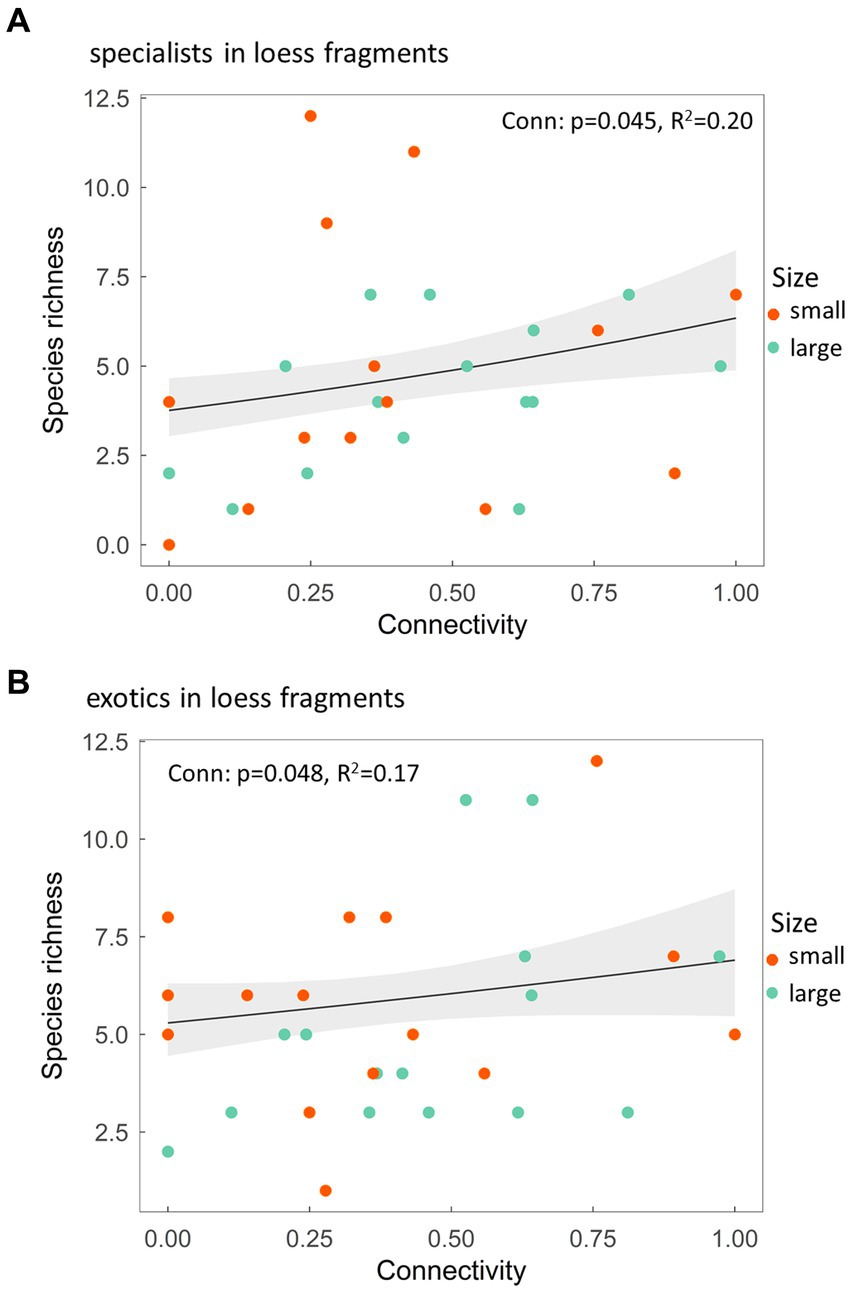
Figure 2. (A) Species richness of specialist and (B) exotic species along a connectivity gradient in loess steppe fragments. p-value shows the statistical significance of the explanatory variable, R2 stands for the conditional coefficient of determination of the model, whereas the grey shaded area represent the standard error of the mean.
We did not find any significant effect of connectivity and fragment size on the richness or the frequency of short-lived, long-lived or clonal species in any species groups of the two steppe types (Supplementary Tables 3, 4). In contrast, isolated sand steppe fragments had a higher richness of non-clonal generalists than connected patches (Figure 3A). In the case of loess steppe specialists, we found that non-clonal species were more abundant in smaller fragments than in the larger ones (Figure 3B). Exotic species did not show any significant pattern in clonality along connectivity gradient or fragment size in either of the grassland types (Supplementary Table 4). We did not observe any effects of connectivity or fragment size on the richness or frequency of specialists with different soil seed bank persistence in either fragment type (Supplementary Table 5). We found that both generalist and exotic species forming persistent seed bank were more frequent in well-connected loess fragments (Figures 3C,D). This difference was not observed in the case of sand steppe fragments. Species with transient seed bank did not show any trend along the connectivity gradient or fragment size in either species groups or grassland types (Supplementary Table 5).
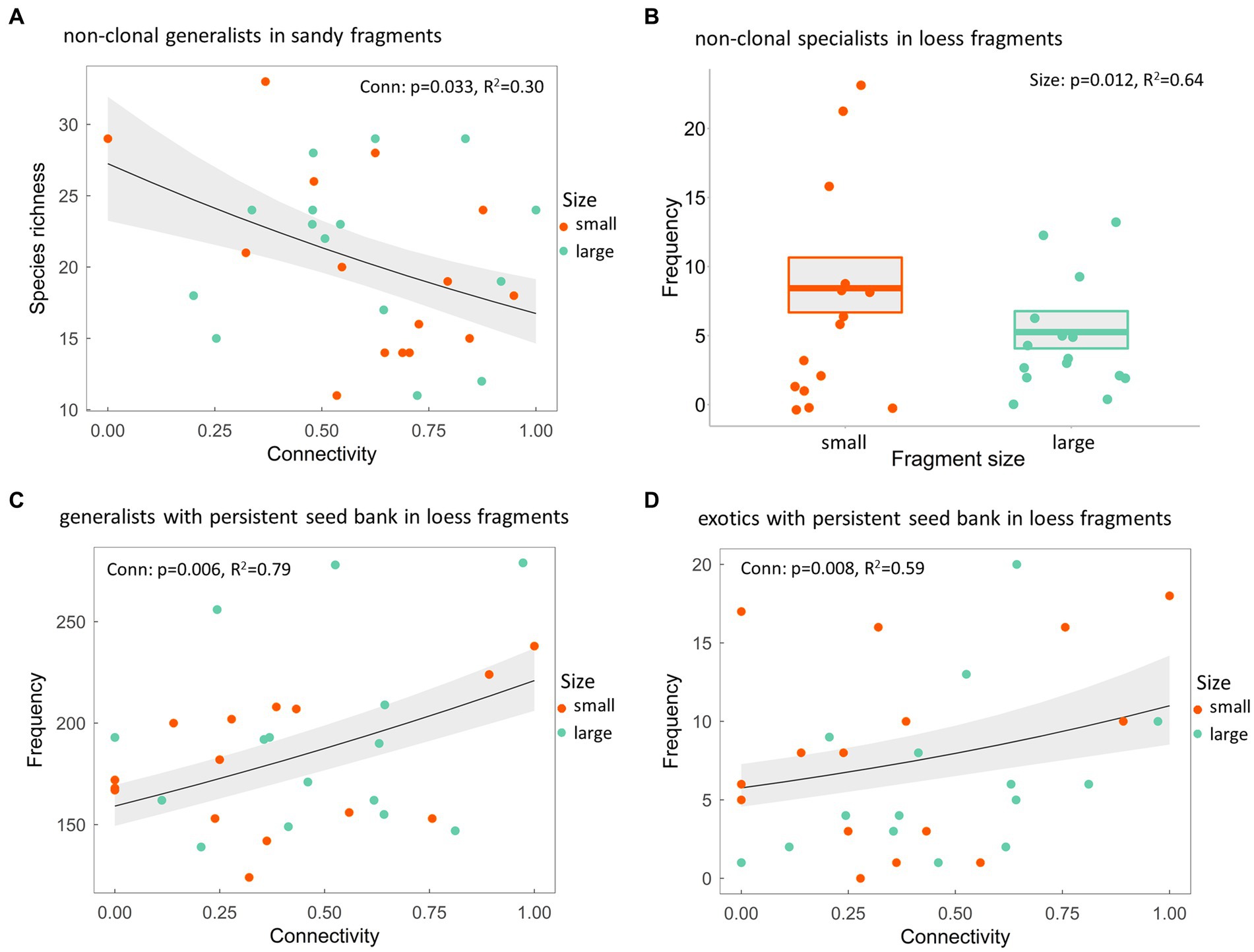
Figure 3. (A) Species richness of non-clonal generalists in sand grassland fragments, (B) frequency of non-clonal specialists, (C) generalists with persistent seed bank and (D) exotics with persistent soil seed bank in loess steppe fragments in small and large fragments along a connectivity gradient. p-value shows the statistical significance of the explanatory variable, R2 stands for the conditional coefficient of determination of the model, whereas the grey shaded area represent the standard error of the mean.
Species with Allium and Epilobium dispersal strategies (i.e., autochory and anemochory) dominated both grasslands with altogether 71% and 17%, and 77% and 11% in sand and loess fragments, respectively. Autochorous Allium-type species did not respond to either connectivity or fragment size in our study, except for loess fragments, where increased connectivity resulted in a higher richness of Allium-type specialists (Supplementary Table 6; Figure 4A). Isolation resulted in a higher frequency of generalist species with autochory- and endozoochory-dominated Cornus-type dispersal in smaller compared to larger fragments in the sand steppes (Figure 4B). We observed more species and higher frequency of generalists Epilobium dispersal strategy characterised by anemochory and autochory in large than in small loess steppe fragments (Figures 4C,D). We showed that Cornus-type exotic species were more abundant in terms of both richness and frequency in small compared to large isolated fragments (Figures 4E,F). Bidens dispersal type with autochory and epizoochory dispersal modes was not different based on fragment size and connectivity in either species group and fragment type (Supplementary Table 6). Community-weighted means of seed mass did not have any trend along the connectivity gradient or with increasing fragment size for either species group in the two grassland types (Supplementary Table 7).
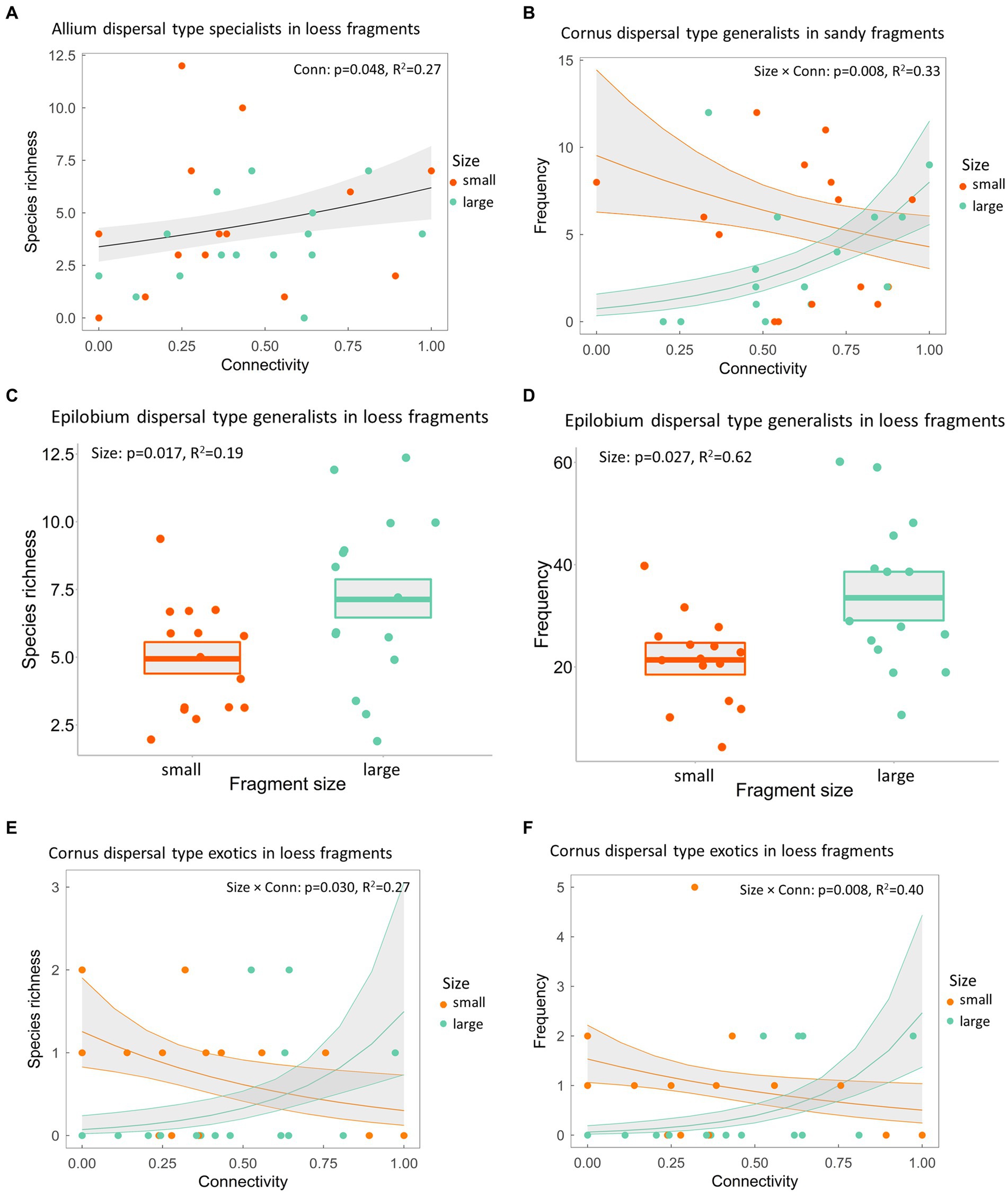
Figure 4. (A) Richness of specialist species with Allium-type dispersal strategy in loess steppe fragments, (B) frequency of generalist species with Cornus-type dispersal strategy in sandy fragments and (C) species richness and (D) frequency of generalists with Epilobium-type dispersal strategy, (E) species richness and (F) frequency of exotic species with Cornus-type dispersal in loess grassland fragments. p-value shows the statistical significance of the explanatory variable(s), R2 stands for the conditional coefficient of determination of the model, whereas the grey shaded area represent the standard error of the mean.
4. Discussion
Our results highlighted that both local and landscape-scale factors were important in shaping the vegetation composition of Pannonic steppe fragments. We observed more fragmentation effects in generalists than in specialist species. We found that isolation resulted in fewer specialist species with autochorous (Allium type) dispersal in loess steppe fragments. Isolated loess steppe fragments also harboured fewer generalist species with persistent seed bank but, at the same time, also fewer exotics. Large loess steppe fragments supported more species with Epilobium-type dispersal strategy than smaller ones. We did not find any systematic change along the two fragmentation factors in the sand fragments, except for the high frequency of generalists without clonal propagation and Cornus-type (endozoochory- and autochory-dominated) dispersal strategy in small and isolated fragments.
4.1. Fragmentation effects on species groups
In sand steppes, we did not observe any fragmentation effects on the trait composition of the specialist flora. This was contrary to our expectations, as several studies showed that habitat specialists are sensitive to fragmentation (e.g., Auffret et al., 2018; Yan et al., 2022). The absence of an effect of sand steppe fragmentation on the distribution of specialists may refer to their better dispersal abilities (Hemrová and Münzbergová, 2015), long-term species persistence (Tilman et al., 1994) or their combination. This is in line with our results, as specialist species of the sand steppes have a high potential for local persistence because the majority of the species are long-lived clonal perennials and have lighter seeds helping dispersal among remnant patches (Bakker et al., 1996).
We observed more fragmentation effects in generalists and exotic species than in specialists. Rösch et al. (2013) found that community composition changes were primarily driven by the responses of generalist species during the fragmentation of dry calcareous grasslands as they compose the dominant part of the vegetation. Pandit et al. (2009) also suggested that generalists should respond strongly to patch configuration and connectivity gradients. In line with them, we observed most habitat fragmentation effects in the case of the generalist species group. We found that clonality and dispersal strategies of generalist species depended on fragment size, connectivity or both factors in the two grassland types, and soil seed bank persistence in loess fragments. Reduced patch size results in a high edge-core area ratio that can be exploited by generalists as opposed to specialists (Brückmann et al., 2010). In such case, habitat generalists offer insurance biodiversity, especially if the habitat is disturbed (Deák et al., 2020). However, the increasing dominance of generalist species following fragmentation relative to specialists can lead to biotic homogenisation and be considered an unfavourable degradation process (Öckinger et al., 2010).
We detected a two-fold frequency increase of exotic species in loess steppe fragments along the increasing connectivity gradient, but we found no such effect in sand fragments. This result suggests that exotic species in the sand steppes are mostly good dispersing invasives that are not hindered by fragmentation (Csecserits et al., 2016), while the exotics of loess remnants are dispersal limited to some extent. Moreover, forest plantations around sand fragments also facilitate the invasion of alien plants (Szitár et al., 2014). We found differential responses of the species groups, suggesting that focusing on only one group (e.g., specialists) may be insufficient to evaluate vegetation responses to habitat fragmentation.
4.2. Fragmentation effects on dispersal traits
Local species persistence and dispersal traits have been shown to influence plant species sensitivity to habitat fragmentation by several studies (e.g., Lindborg, 2007; Marini et al., 2012). Plant species with high dispersal potential are expected to be disproportionally overrepresented in isolated patches (Dupré and Ehrlén, 2002). We found that not only isolation but also fragment size affected dispersal strategies according to Sádlo et al. (2018). Endozoochorous dispersal (Cornus type) strategy was more frequent in small and isolated sites among sand generalists and exotics in loess fragments. Most of these species were shrubs and trees with efficient bird dispersal (e.g., Celtis occidentalis, Elaeagnus angustifolia, and Morus alba). Extensive shrub encroachment is considered unfavourable in this region as they take up the space from the herbaceous vegetation (Ónodi et al., 2021), and if they are non-native, transformative vegetation changes and biotic homogenisation can occur (Didham et al., 2005). Generalists dispersing predominantly with anemochory and autochory (Epilobium dispersal strategy type) in loess fragments were more species rich and frequent in large fragments showing that successful arrival and establishment by wind is proportional to habitat area; therefore, this strategy is less efficient in small remnants. We did not find any fragmentation impacts for the epizoochory- and autochory-dominated Bidens-type dispersal strategy, maybe because of the general absence of grazing in both ecosystem types, similar to the results of Soons et al. (2005). In fragmented landscapes, many habitat remnants can remain unoccupied by bad disperser species (Lindborg et al., 2012). In line with this, we found that isolation also negatively affected loess specialist species with autochorous Allium-type dispersal. This has important nature conservation implications as these species have the least efficient autochorous dispersal (Vittoz and Engler, 2007). Therefore, they are particularly sensitive to fragmentation and may need active interventions to mitigate dispersal limitation or species reintroduction.
Propagule size is an important functional trait for both species persistence and dispersal, as small diaspores are expected to have higher colonisation potential (Marini et al., 2012), whereas longer longevity but also higher local colonisation rates are predicted for larger ones (Fröborg and Eriksson, 1997). However, similar to our results, seed mass was not always found to be correlated with remnant area or isolation (Dupré and Ehrlén, 2002; Lindborg, 2007).
4.3. Fragmentation effects on local persistence traits
Species longevity, the presence of persistent seed bank and clonal reproduction represent a potentially important form of temporal functional connectivity extending the life span of remnant populations and rescuing those that have gone locally extinct (Auffret et al., 2015). We did not find any fragmentation impact on the longevity of plant species in our study. This was opposing to our expectations, as several studies proved that perennial species are less sensitive to habitat loss because of their long generation time (e.g., Heinken and Weber, 2013), whereas short-lived species are more susceptible to fragmentation, especially fragment size, because of their larger natural fluctuations in abundance (Marini et al., 2012).
Fragmentation is expected to affect clonally reproducing species less than non-clonal species (Hemrová and Münzbergová, 2015). According to that, we did not observe any response of species with the ability of clonal propagation against fragmentation. However, we found that non-clonal species were more abundant in isolated and small patches in both sand and loess fragments. Likewise, Lindborg (2007) observed that long-lived species with clonal ability were unrelated to contemporary grassland connectivity and area whereas non-clonal species were more abundant in isolated fragments. However, they also found that small and isolated fragments in the 50 years’ historical landscape had a high share of species with clonal propagation, inferring that an extinction debt existed in clonal plants and may exist in our case, too (Tilman et al., 1994).
The persistent seed bank can create a temporal rescue effect for communities (Honnay et al., 2008). We did not find any association of fragmentation with seed bank persistence in sand fragments. Lindborg (2007) suggested that in the long term, connectivity loss and area reduction would create a plant community dominated by long-lived and clonal plants and plants with a persistent seed bank. In the case of loess fragments, this potential seems to be depleted as we found a lower frequency of generalists with persistent seed bank in isolated sites.
4.4. Fragmentation effects on the two grassland types
Overall, the vegetation of loess steppe fragments seemed to be more affected by fragmentation than that of the sand steppe fragments, although we did not make a direct comparison of the two grassland types. In sand steppe fragments, the taxonomic richness of the species groups was similar in fragments with different sizes and levels of connectivity. In contrast, specialist and exotic species occurring in loess fragments were more species rich in connected fragments. This difference was most probably due to the smaller size of loess fragments and their functionally more hostile large-scale agricultural matrix compared to sand fragments (Gallé et al., 2022). Furthermore, we can consider sand fragments functionally less isolated because there are more road verges, forest fringes (Rédei et al., 2014), and thin grassland strips between forestry units that can serve plant population persistence by supporting meta-population dynamics and thus immigration processes (Szitár et al., 2014). In contrast, road verges preserved only a small portion of grassland specialist plant species near loess grasslands in our study area because of frequent mowing and trampling (Deák et al., 2020, 2021b).
The absence of fragmentation effects of the current landscape can also refer to an extinction debt, that is a time-delayed extinction to be realised in the future without further habitat modification after habitat loss in the past (Kuussaari et al., 2009). A weak extinction debt was shown in the sandy steppe study region by Rédei et al. (2014), who found that the richness of sand specialist flora reflected the historical landscape composition in the 18th century, but they did not find any correlation with the present composition. Deák et al. (2021a) also showed for kurgans that the landscape structure of the 1970s best explained the species richness of loess grassland specialists, referring to an unpaid extinction debt. Their results are not in contradiction with our results, as they showed that contemporary connectivity explained specialist richness almost as well as that in the 1970s. It is also possible that the local management regime (e.g., mowing or grazing) not assessed in this study overrode and obscured fragmentation impacts (Gallé et al., 2022).
5. Conclusion
We found that habitat specialist plant species were less dependent on connectivity and habitat area than generalists and exotic species in sand and loess steppe fragments. However, there may be an extinction debt in the effect of fragmentation. Both habitat connectivity and fragment size, two important measures of habitat fragmentation, affected vegetation composition regarding local persistence and dispersal potential-related traits. Based on our analyses, we can predict how shifted species composition would enable communities to respond to further habitat fragmentation if they still own regeneration and resilience potential. We may rely on the persistence of long-lived and clonal specialist and generalist species in fragmented sand and loess steppes, as they showed no response for fragment size and connectivity loss. The regeneration potential of the vegetation following fragmentation can be improved by preserving large fragments and increasing habitat connectivity in parallel. However, given the fact that these also do not buffer against exotic species, active and targeted management of exotic species is also important, especially in times of climate change extremely threatening these habitats.
Data availability statement
The raw data supporting the conclusions of this article will be made available by the authors, without undue reservation.
Author contributions
PB, RG, and KS designed the study. CT, BD, RG, and DK performed data collection and preparation. KS performed data analyses with substantial input from DK. KS wrote the first draft of the manuscript. All authors contributed to the article and approved the submitted version.
Funding
This study was supported by the Hungarian National Research, Development and Innovation Office (NKFIH KKP 133839).
Conflict of interest
The authors declare that the research was conducted in the absence of any commercial or financial relationships that could be construed as a potential conflict of interest.
Publisher’s note
All claims expressed in this article are solely those of the authors and do not necessarily represent those of their affiliated organizations, or those of the publisher, the editors and the reviewers. Any product that may be evaluated in this article, or claim that may be made by its manufacturer, is not guaranteed or endorsed by the publisher.
Supplementary material
The Supplementary material for this article can be found online at: https://www.frontiersin.org/articles/10.3389/fevo.2023.1155885/full#supplementary-material
References
Amartuvshin, N., Hülber, K., Plutzar, C., and Tserenbaljid, G. (2019). Functional traits but not environmental gradients explain seed weight in Mongolian plant species. Plant Biol. 21, 559–562. doi: 10.1111/plb.12938
Auffret, A. G., Kimberley, A., Plue, J., and Waldén, E. (2018). Super-regional land-use change and effects on the grassland specialist flora. Nat. Commun. 9:3464. doi: 10.1038/s41467-018-05991-y
Auffret, A. G., Plue, J., and Cousins, S. A. (2015). The spatial and temporal components of functional connectivity in fragmented landscapes. Ambio 44, 51–59. doi: 10.1007/s13280-014-0588-6
Bacles, C. F., Lowe, A. J., and Ennos, R. A. (2006). Effective seed dispersal across a fragmented landscape. Science 311:628. doi: 10.1126/science.1121543
Bakker, J. P., Poschlod, P., Strykstra, R. J., Bekker, R. M., and Thompson, K. (1996). Seed banks and seed dispersal: important topics in restoration ecology. Acta Bot. Neerl. 45, 461–490. doi: 10.1111/j.1438-8677.1996.tb00806.x
Barton, K. (2022). MuMIn: Multi-model inference. R package version 1.1. Available at: https://cran.r-project.org/package=MuMIn (Accessed 15 January 2023).
Bates, D., Maechler, M., Bolker, B., Walker, S., Christensen, R. H. B., Singmann, H., et al. (2021). lme4: Linear Mixed-Effects Models using “Eigen” and S4. ver 1.1-27.1 https://cran.r-project.org/web/packages/lme4/index.html (Accessed 20 January 2022).
Biró, M., Révész, A., Molnár, Z., Horváth, F., and Czúcz, B. (2008). Regional habitat pattern of the Duna-Tisza köze in Hungary II. The sand, the steppe and the riverine vegetation: degraded and ruined habitats. Acta Bot. Hung. 50, 19–60. doi: 10.1556/ABot.50.2008.1-2.2
Brooks, M. E., Kristensen, K., Van Benthem, K. J., Magnusson, A., Berg, C. W., Nielsen, A., et al. (2017). glmmTMB balances speed and flexibility among packages for zero-inflated generalized linear mixed modeling. R. I. Dent. J. 9, 378–400. doi: 10.3929/ethz-b-000240890
Brückmann, S. V., Krauss, J., and Steffan-Dewenter, I. (2010). Butterfly and plant specialists suffer from reduced connectivity in fragmented landscapes. J. Appl. Ecol. 47, 799–809. doi: 10.1111/j.1365-2664.2010.01828.x
Csecserits, A., Botta-Dukát, Z., Kröel-Dulay, G., Lhotsky, B., Ónodi, G., Rédei, T., et al. (2016). Tree plantations are hot-spots of plant invasion in a landscape with heterogeneous land-use. Agric. Ecosyst. Environ. 226, 88–98. doi: 10.1016/j.agee.2016.03.024
Csecserits, A., Czúcz, B., Halassy, M., Kröel-Dulay, G., Rédei, T., Szabó, R., et al. (2011). Regeneration of sandy old-fields in the forest steppe region of Hungary. Plant Biosyst. 145, 715–729. doi: 10.1080/11263504.2011.601340
Csontos, P., Tamás, J., and Balogh, L. (2003). Thousand seed weight records of species from the flora of Hungary, I. Monocotyledonopsida. Stud. Bot. Hung. 34, 121–126.
Csontos, P., Tamás, J., and Balogh, L. (2007). Thousand seed weight records of species from the flora of Hungary, II. Dicotyledonopsida. Stud. Bot. Hung. 38, 179–189.
Deák, B., Bede, Á., Rádai, Z., Tóthmérész, B., Török, P., Torma, A., et al. (2021a). Different extinction debts among plants and arthropods after loss of grassland amount and connectivity. Biol. Conserv. 264:109372. doi: 10.1016/j.biocon.2021.109372
Deák, B., Rádai, Z., Bátori, Z., Kelemen, A., Lukács, K., Kiss, R., et al. (2021b). Ancient burial mounds provide safe havens for grassland specialist plants in transformed landscapes—a trait-based analysis. Front. Ecol. Evol. 9:619812. doi: 10.3389/fevo.2021.619812
Deák, B., Rádai, Z., Lukács, K., Kelemen, A., Kiss, R., Bátori, Z., et al. (2020). Fragmented dry grasslands preserve unique components of plant species and phylogenetic diversity in agricultural landscapes. Biodivers. Conserv. 29, 4091–4110. doi: 10.1007/s10531-020-02066-7
Deák, B., Tóthmérész, B., Valkó, O., Sudnik-Wójcikowska, B., Bragina, T. M., Moysiyenko, I. I., et al. (2016). Cultural monuments and nature conservation: the role of kurgans in maintaining steppe vegetation. Biodivers. Conserv. 25, 2473–2490. doi: 10.1007/s10531-016-1081-2
Deák, B., Valkó, O., Török, P., Kelemen, A., Bede, Á., Csathó, A. I., et al. (2018). Landscape and habitat filters jointly drive richness and abundance of specialist plants in terrestrial habitat islands. Landsc. Ecol. 33, 1117–1132. doi: 10.1007/s10980-018-0660-x
Dembicz, I., Moysiyenko, I. I., Kozub, Ł., Dengler, J., Zakharova, M., and Sudnik-Wójcikowska, B. (2021). Steppe islands in a sea of fields: where island biogeography meets the reality of a severely transformed landscape. J. Veg. Sci. 32:e12930. doi: 10.1111/jvs.12930
Didham, R. K., Kapos, V., and Ewers, R. M. (2012). Rethinking the conceptual foundations of habitat fragmentation research. Oikos 121, 161–170. doi: 10.1111/j.1600-0706.2011.20273.x
Didham, R. K., Tylianakis, J. M., Hutchison, M. A., Ewers, R. M., and Gemmell, N. J. (2005). Are invasive species the drivers of ecological change? Trends Ecol. Evolution 20, 470–474. doi: 10.1016/j.tree.2005.07.006
Dupré, C., and Ehrlén, J. (2002). Habitat configuration, species traits and plant distributions. J. Ecol. 90, 796–805. doi: 10.1046/j.1365-2745.2002.00717.x
Erdős, L., Ambarlı, D., Anenkhonov, O. A., Bátori, Z., Cserhalmi, D., Kiss, M., et al. (2018a). The edge of two worlds: a new review and synthesis on Eurasian forest-steppes. Appl. Veg. Sci. 21, 345–362. doi: 10.1111/avsc.12382
Erdős, L., Kröel-Dulay, G., Bátori, Z., Kovács, B., Németh, C., Kiss, P. J., et al. (2018b). Habitat heterogeneity as a key to high conservation value in forest-grassland mosaics. Biol. Conserv. 226, 72–80. doi: 10.1016/j.biocon.2018.07.029
Eriksson, O. (1996). Regional dynamics of plants: a review of evidence for remnant, source-sink and metapopulations. Oikos 77, 248–258. doi: 10.2307/3546063
European Commission (2013). Interpretation manual of European Union habitats – EUR28. European Commission. Available at: https://ec.europa.eu/environment/nature/legislation/habitatsdirective/docs/Int_Manual_EU28.pdf (Accessed 15 December 2022).
Fekete, G., Molnár, Z., Kun, A., and Botta-Dukát, Z. (2002). On the structure of the Pannonian forest steppe: grasslands on sand. Acta Zool. Hung. 48, 137–150.
Fröborg, H., and Eriksson, O. (1997). Local colonization and extinction of field layer plants in a deciduous forest and their dependence upon life history features. J. Veg. Sci. 8, 395–400. doi: 10.2307/3237329
Gallé, R., Korányi, D., Tölgyesi, C., Lakatos, T., Marcolin, F., Török, E., et al. (2022). Landscape-scale connectivity and fragment size determine species composition of grassland fragments. Basic Appl. Ecol. 65, 39–49. doi: 10.1016/j.baae.2022.10.001
Gaujour, E., Amiaud, B., Mignolet, C., and Plantureux, S. (2012). Factors and processes affecting plant biodiversity in permanent grasslands. A review. Agron. Sustain. Dev. 32, 133–160. doi: 10.1007/s13593-011-0015-3
Habel, J. C., Dengler, J., Janišová, M., Török, P., Wellstein, C., and Wiezik, M. (2013). European grassland ecosystems: threatened hotspots of biodiversity. Biodivers. Conserv. 22, 2131–2138. doi: 10.1007/s10531-013-0537-x
Hanski, I., and Ovaskainen, O. (2000). The metapopulation capacity of a fragmented landscape. Nature 404, 755–758. doi: 10.1038/35008063
Hartig, F. (2022). DHARMa: residual diagnostics for hierarchical (multi-level/mixed) regression models. R project. R package version 0.4.5. Available at: https://cran.r-project.org/web/packages/DHARMa/vignettes/DHARMa.html (Accessed 10 January 2022).
Heinken, T., and Weber, E. (2013). Consequences of habitat fragmentation for plant species: do we know enough? Perspect. Plant Ecol. Evol. Syst. 15, 205–216. doi: 10.1016/j.ppees.2013.05.003
Hemrová, L., and Münzbergová, Z. (2015). The effects of plant traits on species’ responses to present and historical patch configurations and patch age. Oikos 124, 437–445. doi: 10.1111/oik.01130
Honnay, O., and Bossuyt, B. (2005). Prolonged clonal growth: escape route or route to extinction? Oikos 108, 427–432. doi: 10.1111/j.0030-1299.2005.13569.x
Honnay, O., Bossuyt, B., Jacquemyn, H., Shimono, A., and Uchiyama, K. (2008). Can a seed bank maintain the genetic variation in the above ground plant population? Oikos 117, 1–5. doi: 10.1111/j.2007.0030-1299.16188.x
Isbell, F., Balvanera, P., Mori, A. S., He, J. S., Bullock, J. M., Regmi, G. R., et al. (2022). Expert perspectives on global biodiversity loss and its drivers and impacts on people. Front. Ecol. Environ. 21, 94–103. doi: 10.1002/fee.2536
Jackson, S. T., and Sax, D. F. (2010). Balancing biodiversity in a changing environment: extinction debt, immigration credit and species turnover. Trends Ecol. Evol. 25, 153–160. doi: 10.1016/j.tree.2009.10.001
Janečková, P., Janeček, S., Klimešová, J., Götzenberger, L., Horník, J., Lepš, J., et al. (2017). The plant functional traits that explain species occurrence across fragmented grasslands differ according to patch management, isolation, and wetness. Landsc. Ecol. 32, 791–805. doi: 10.1007/s10980-017-0486-y
Kattge, J., Bönisch, G., Díaz, S., Lavorel, S., Prentice, I. C., Leadley, P., et al. (2020). TRY plant trait database–enhanced coverage and open access. Glob. Chang. Biol. 26, 119–188. doi: 10.1111/gcb.14904
Király, G. (2009). New Hungarian herbal. The vascular plants of Hungary. Identification keys. Aggteleki National Park Directorate, Jósvafő, Hungary. 616.
Kleyer, M., Bekker, R. M., Knevel, I. C., Bakker, J. P., Thompson, K., Sonnenschein, M., et al. (2008). The LEDA Traitbase: a database of life-history traits of the northwest European flora. J. Ecol. 96, 1266–1274. doi: 10.1111/j.1365-2745.2008.01430.x
Klimešová, J., and de Bello, F. (2009). CLO-PLA: the database of clonal and bud bank traits of central European flora. J. Veg. Sci. 20, 511–516. doi: 10.1111/j.1654-1103.2009.01050.x
Kuli-Révész, K., Korányi, D., Lakatos, T., Szabó, Á. R., Batáry, P., and Gallé, R. (2021). Smaller and isolated grassland fragments are exposed to stronger seed and insect predation in habitat edges. Forests 12:54. doi: 10.3390/f12010054
Kuussaari, M., Bommarco, R., Heikkinen, R. K., Helm, A., Krauss, J., Lindborg, R., et al. (2009). Extinction debt: a challenge for biodiversity conservation. Trends Ecol. Evol. 24, 564–571. doi: 10.1016/j.tree.2009.04.011
Lindborg, R. (2007). Evaluating the distribution of plant life-history traits in relation to current and historical landscape configurations. J. Ecol. 95, 555–564. doi: 10.1111/j.1365-2745.2007.01232.x
Lindborg, R., Helm, A., Bommarco, R., Heikkinen, R. K., Kühn, I., Pykälä, J., et al. (2012). Effect of habitat area and isolation on plant trait distribution in European forests and grasslands. Ecography 35, 356–363. doi: 10.1111/j.1600-0587.2011.07286.x
Loreau, M., Naeem, S., Inchausti, P., Bengtsson, J., Grime, J. P., Hector, A., et al. (2001). Biodiversity and ecosystem functioning: current knowledge and future challenges. Science 294, 804–808. doi: 10.1126/science.1064088
Marini, L., Bruun, H. H., Heikkinen, R. K., Helm, A., Honnay, O., Krauss, J., et al. (2012). Traits related to species persistence and dispersal explain changes in plant communities subjected to habitat loss. Divers. Distrib. 18, 898–908. doi: 10.1111/j.1472-4642.2012.00893.x
Maurer, K., Durka, W., and Stöcklin, J. (2003). Frequency of plant species in remnants of calcareous grassland and their dispersal and persistence characteristics. Basic Appl. Ecol. 4, 307–316. doi: 10.1078/1439-1791-00162
McGill, B. J., Enquist, B. J., Weiher, E., and Westoby, M. (2006). Rebuilding community ecology from functional traits. Trends Ecol. Evol. 21, 178–185. doi: 10.1016/j.tree.2006.02.002
Moilanen, A., and Hanski, I. (2001). On the use of connectivity measures in spatial ecology. Oikos 95, 147–151. doi: 10.1034/j.1600-0706.2001.950116.x
Molnár, Z., Biró, M., Bartha, S., and Fekete, G. (2012). “Past trends, present state and future prospects of Hungarian forest-steppes” in Eurasian steppes. Ecological problems and livelihoods in a changing world. eds. M. J. A. Werger and M. A. van Staalduinen (Dordrecht, Heidelberg, New York: Springer), 209–252.
Öckinger, E., Schweiger, O., Crist, T. O., Debinski, D. M., Krauss, J., Kuussaari, M., et al. (2010). Life-history traits predict species responses to habitat area and isolation: a cross-continental synthesis. Ecol. Lett. 13, 969–979. doi: 10.1111/j.1461-0248.2010.01487.x
Ónodi, G., Kertész, M., Lengyel, A., Pándi, I., Somay, L., Szitár, K., et al. (2021). The effects of woody plant encroachment and wildfire on plant species richness and composition: temporal changes in a forest–steppe mosaic. Appl. Veg. Sci. 24:e12546. doi: 10.1111/avsc.12546
Ottaviani, G., Keppel, G., Götzenberger, L., Harrison, S., Opedal, Ø. H., Conti, L., et al. (2020). Linking plant functional ecology to island biogeography. Trends Plant Sci. 25, 329–339. doi: 10.1016/j.tplants.2019.12.022
Pandit, S. N., Kolasa, J., and Cottenie, K. (2009). Contrasts between habitat generalists and specialists: an empirical extension to the basic metacommunity framework. Ecology 90, 2253–2262. doi: 10.1890/08-0851.1
Pinheiro, J., Bates, D., DebRoy, S., Sarkar, D., Heisterkamp, S., Van Willigen, B., et al. (2017). Package ‘nlme’. Linear and nonlinear mixed effects models, version, 3. Available at: https://cran.r-project.org/web/packages/nlme/nlme.pdf (Accessed 20 January 2022).
QGIS Development Team. (2019). Quantum GIS geographic information system. Open source geospatial foundation project. Available at: http://qgis.osgeo.org (Accessed 1 January 2021).
R Core Team. (2022). R: A language and environment for statistical computing. Vienna, Austria: R Foundation for Statistical Computing.
Rédei, T., Szitár, K., Czúcz, B., Barabás, S., Lellei-Kovács, E., Pándi, I., et al. (2014). Weak evidence of long-term extinction debt in Pannonian dry sand grasslands. Agric. Ecosyst. Environ. 182, 137–143. doi: 10.1016/j.agee.2013.07.016
Rösch, V., Tscharntke, T., Scherber, C., and Batáry, P. (2013). Landscape composition, connectivity and fragment size drive effects of grassland fragmentation on insect communities. J. Appl. Ecol. 50, 387–394. doi: 10.1111/1365-2664.12056
Sádlo, J., Chytrý, M., Pergl, J., and Pyšek, P. (2018). Plant dispersal strategies. Preslia 90, 1–22. doi: 10.23855/preslia.2018.001
Sonkoly, J., Tóth, E., Balogh, N., Balogh, L., Bartha, D., Bata, K., et al. (2022). PADAPT 1.0–the Pannonian database of plant traits. bioRxiv [Preprint].
Soons, M. B., Messelink, J. H., Jongejans, E., and Heil, G. W. (2005). Habitat fragmentation reduces grassland connectivity for both short-distance and long-distance wind-dispersed forbs. J. Ecol. 93, 1214–1225. doi: 10.1111/j.1365-2745.2005.01064.x
Squires, V. R., and Feng, H. (2018). “Brief history of grassland utilization and its significance to humans” in Grasslands of the world. eds. V. R. Squires, J. Dengler, L. Hua, and H. Feng (Boca Raton, FL: CRC Press), 17–26.
Szitár, K., Ónodi, G., Somay, L., Pándi, I., Kucs, P., and Kröel-Dulay, G. (2014). Recovery of inland sand dune grasslands following the removal of alien pine plantation. Biol. Conserv. 171, 52–60. doi: 10.1016/j.biocon.2014.01.021
Tanács, E., Belényesi, M., Lehoczki, R., Pataki, R., Petrik, O., Standovár, T., et al. (2021). Compiling a high-resolution country-level ecosystem map to support environmental policy: methodological challenges and solutions from Hungary. Geocarto Int. 37, 8746–8769. doi: 10.1080/10106049.2021.2005158
Taylor, P. D., Fahrig, L., Henein, K., and Merriam, G. (1993). Connectivity is a vital element of landscape structure. Oikos 68, 571–573. doi: 10.2307/3544927
Tilman, D., May, R. M., Lehman, C. L., and Nowak, M. A. (1994). Habitat destruction and the extinction debt. Nature 371, 65–66. doi: 10.1038/371065a0
Török, P., Miglécz, T., Valkó, O., Tóth, K., Kelemen, A., Albert, Á. J., et al. (2013). New thousand-seed weight records of the Pannonian flora and their application in analysing social behaviour types. Acta Bot. Hung. 55, 429–472. doi: 10.1556/abot.55.2013.3-4.17
Török, P., Tóth, E., Tóth, K., Valkó, O., Deák, B., Kelbert, B., et al. (2016). New measurements of thousand-seed weights of species in the Pannonian flora. Acta Bot. Hung. 58, 187–198. doi: 10.1556/034.58.2016.1-2.10
Turner, I. M. (1996). Species loss in fragments of tropical rain forest: a review of the evidence. J. Appl. Ecol. 33, 200–209. doi: 10.2307/2404743
Uroy, L., Ernoult, A., and Mony, C. (2019). Effect of landscape connectivity on plant communities: a review of response patterns. Landsc. Ecol. 34, 203–225. doi: 10.1007/s10980-019-00771-5
Vázquez, D. P., and Simberloff, D. (2002). Ecological specialization and susceptibility to disturbance: conjectures and refutations. Am. Nat. 159, 606–623. doi: 10.1086/339991
Vittoz, P., and Engler, R. (2007). Seed dispersal distances: a typology based on dispersal modes and plant traits. Bot. Helv. 117, 109–124. doi: 10.1007/s00035-007-0797-8
Wickham, H. (2011). ggplot2. Wiley Interdiscip. Rev. Comput. Stat. 3, 180–185. doi: 10.1002/wics.147
Wild, J., Kaplan, Z., Danihelka, J., Petřík, P., Chytrý, M., Novotný, P., et al. (2019). Plant distribution data for the Czech Republic integrated in the Pladias database. Preslia 91, 1–24. doi: 10.23855/preslia.2019.001
Wilson, J. B., Peet, R. K., Dengler, J., and Pärtel, M. (2012). Plant species richness: the world records. J. Veg. Sci. 23, 796–802. doi: 10.1111/j.1654-1103.2012.01400.x
Keywords: adventive species, functional trait, habitat specialist, habitat isolation, terrestrial habitat island, kurgan, Pannonic loess steppic grassland, Pannonic sand grassland
Citation: Szitár K, Tölgyesi C, Deák B, Gallé R, Korányi D and Batáry P (2023) Connectivity and fragment size drive plant dispersal and persistence traits in forest steppe fragments. Front. Ecol. Evol. 11:1155885. doi: 10.3389/fevo.2023.1155885
Edited by:
Thierry Dutoit, UMR7263 Institut méditerranéen de biodiversité et d’écologie marine et continentale (IMBE), FranceReviewed by:
Ádám Lovas-Kiss, Hungarian Academy of Sciences, HungaryStephen Bonser, University of New South Wales, Australia
Copyright © 2023 Szitár, Tölgyesi, Deák, Gallé, Korányi and Batáry. This is an open-access article distributed under the terms of the Creative Commons Attribution License (CC BY). The use, distribution or reproduction in other forums is permitted, provided the original author(s) and the copyright owner(s) are credited and that the original publication in this journal is cited, in accordance with accepted academic practice. No use, distribution or reproduction is permitted which does not comply with these terms.
*Correspondence: Katalin Szitár, c3ppdGFyLmthdGFsaW5AZWNvbHJlcy5odQ==