- 1Institute of Wetland Ecology & Clone Ecology, Zhejiang Provincial Key Laboratory of Plant Evolutionary Ecology and Conservation, Taizhou University, Taizhou, China
- 2College of Forestry, Inner Mongolia Agricultural University, Huhhot, China
- 3Forest Dynamics, Swiss Federal Institute for Forest, Snow and Landscape Research WSL, Birmensdorf, Switzerland
- 4Key Laboratory of Geographical Processes and Ecological Security in Changbai Mountains, Ministry of Education, School of Geographical Sciences, Northeast Normal University, Changchun, China
- 5School of Life Science, Hebei University, Baoding, China
Biochar has been considered as a cost-effective amendment to improve the soil water content and thus to mitigate the effects of drought on plants. However, less is known about the mitigating effects of biochar application on the negative effect of longer drought duration on trees. We investigated the effects of drought duration in combination with biochar application on the growth, tissue non-structural carbohydrate (NSC) concentrations, needle photosynthesis, and δ13C content of 1-year-old Pinus massoniana saplings in a greenhouse experiment. We found that the height and total biomass of P. massoniana saplings were significantly decreased with increasing drought duration, which was likely attributed to the suppressed photosynthetic capacity. The longer drought duration (e.g., 1 month) significantly decreased needle photosynthesis and increased the value of needle δ13C but did not deplete tissue NSC concentrations at the expense of growth, suggesting that plants prioritize carbon storage over growth in response to a long duration of drought. Surprisingly, the application of biochar significantly decreased the survival of P. massoniana saplings and aggravated the negative effect of drought duration on the growth of P. massoniana, which is probably attributed to the increased soil pH value of >7.5, as P. massoniana is adapted to mildly acid to neutral soils. Our results suggest that the effect of biochar application might be species specific, and it can aggravate the negative effect of drought duration on plants that are less tolerant to alkaline soils.
Introduction
Extreme drought events have rapidly increased worldwide (IPCC, 2021), such as in Europe (Spinoni et al., 2018) and subtropical China (Hu et al., 2021). Induced drought intensity, duration, and frequency have resulted in widespread forest dieback and tree mortality (Allen et al., 2010; Spinoni et al., 2015; Choat et al., 2018; O'Donnell et al., 2021). The subtropical forests in South China have a relatively high carbon uptake and an extensive carbon reserve (Yu et al., 2014). Thus, tree mortality in such ecosystem may have substantial impacts on carbon balance. Despite a large number of studies about drought intensity and frequency (Duan et al., 2019; Chen et al., 2020), only a limited number of works about drought duration and frequency have been conducted (Yang et al., 2022). In particular, the growth and physiological dynamics of trees in subtropical China under prolonged drought duration have been less studied.
Widespread forest dieback and tree mortality have been observed under prolonged drought (Allen et al., 2010; Choat et al., 2018). The physiological dynamics, such as photosynthesis and carbon assimilation, are highly related to the survival of trees under drought (Ouyang et al., 2021; Sapes et al., 2021; Yang et al., 2022)—for example, leaf photosynthesis and leaf stable carbon isotope (δ13C) have been used to infer a plant’s response to atmospheric demand for water (Farquhar et al., 1989; Lawson and Blatt, 2014) and can also help characterize species-specific plant water use strategies (Moreno-Gutierrez et al., 2012; McAusland et al., 2016). The concentration of non-structural carbohydrate (NSC) can play an important role in enhancing drought resistance, given its great effect on energy supply and cellular turgor maintenance (O'Brien et al., 2014; Wiley, 2020). Thus, studying physiological dynamics in trees under drought is helpful in understanding drought-induced tree mortality (Adams et al., 2017).
Biochar has been considered as an ideal amendment to improve the soil quality with active functional groups, high porous structure, and cation exchange capacity (Godlewska et al., 2017; Kavitha et al., 2018). Moreover, biochar could increase the soil’s water holding capacity (Verheijen et al., 2010; Fischer et al., 2019), and thus the application of biochar may mitigate the effects of drought on plants—for example, the application of biochar application at a rate of 15 t ha−1 can slightly increase the soil’s water holding capacity (Paneque et al., 2016). The improved water use efficiency and growth of Abelmoschus esculentus L. Moench have been observed with biochar application under drought stress (Batool et al., 2015). On the other hand, biochar addition may inhibit the growth of plants by influencing the soil nutrients and soil microbial processes (Kuppusamy et al., 2016; Cai et al., 2021; Liang et al., 2021a)—for instance, it has been observed that the application of biochar could significantly decreased the AM fungal abundance in the soils (Warnock et al., 2010; George et al., 2012). Thus, the application of biochar may be a double-edged sword for the growth of plants, especially in drought stress.
Pinus massoniana Lamb. (Masson pine) is a native tree species in southern China. P. massoniana prefers to grow in well-drained, mildly acidic to neutral soils (Chai and Feng, 1984). In this study, we carried out a greenhouse experiment to investigate the interactive effects of drought duration and biochar application on the growth and physiological dynamics of P. massoniana saplings. We recorded the survival, growth, tissue levels of NSC, needle net photosynthesis, and needle δ13C to test our hypotheses that (1) the photosynthesis and tissue NSC—thus, the growth—of P. massoniana saplings decrease along with increasing drought duration and (2) the application of biochar alleviates the negative effects of drought duration on P. massoniana saplings.
Materials and methods
Study site
The experiment was carried out in a greenhouse at Taizhou University (121°23′ E and 28°39′ N) in Taizhou, Zhejiang Province, China. The mean temperature was 22°C (0–45°C), and the mean relative humidity was 80% (25–98°C, measured using a humidity/temperature datalogger, UNI-T, UT330B) inside the greenhouse during the experiment period.
Experimental design
The experiment had four levels of drought treatments fully crossed with two levels of biochar treatments, and this was continued for 14 months from April 10, 2021 to June 21, 2022. Each treatment had eight replicates, with 64 pots in total. The 1-year-old P. massoniana saplings with naked roots used in the present study were obtained from a field nursery in Hechi, Guangxi Province, China.
On March 1, 2021, saplings with similar height (33.2 ± 0.3 cm) were planted in pots (one sapling per pot). Each pot (11 cm in diameter and 10 cm in height) with holes at the bottom was filled with a 1:1 (v:v) mixture of local soil and river sand. The local soil was collected in the mountainous area of Taizhou, containing 0.62 ± 0.17 (mean ± SE) g kg−1 total nitrogen and 0.13 ± 0.03 g kg−1 total phosphorus. All the pots were watered every 2 days for 1 month.
On April 10, 2021, after 1 month of recovery growth, the saplings were transplanted into larger pots with holes at the bottom (24 cm in diameter, 25.5 cm in height, and 9 L in volume) and were randomly arranged according to different biochar treatments, and then these were watered every 2 days. The substrate of the larger pots was also a 1:1 (v:v) mixture of local soil and river sand as mentioned above. In the biochar treatments, two levels of biochar treatments were included, i.e., without biochar (no biochar was applied into the pots) and with biochar (biochar was applied into the pots). The biochar was purchased from a market in Zhengzhou, Henan Province, China. The biochar was derived from maize straw that was pyrolyzed at 550°C in a muffle furnace, containing 375.30 ± 67.99 (mean ± SE) g kg−1 total carbon and 1.36 ± 0.15 g kg−1 total nitrogen, pH 9.76 ± 0.57. The application of biochar was at a rate of 1% (w/w) as frequently used in many studies (Jain et al., 2020; Yao et al., 2021), and the biochar was mixed with the substrate.
On May 5, 2021, we started the drought treatments and continued it for about 13 months. Four levels of drought treatments were included, i.e., D1 (watering once every week, 60 times), D2 (watering once every 2 weeks, 30 times), D3 (watering once every 3 weeks, 20 times), and D4 (watering once every 4 weeks, 15 times) throughout the experimental period. For each watering, plenty of water was applied, and the excess water could spill out from the bottom of the pots. The soil water condition was not measured because we only focused on the duration between two watering events (Yang et al., 2022). The extra 50 mL of nutrients (1 g/L, Osmocote Plus, 20-20-20+TE, ICL Belgium, N.V.) was applied to the pots at 2 days after the simultaneous application of four drought treatments during the experiment, for five times and 250 mL in total.
On June 21, 2022, before the last watering (four drought treatments watered simultaneously), individual sapling height and photosynthesis were measured. Then, the saplings were harvested and oven-dried at 65°C for 72 h, and the dry weight of the needles, stems, and roots were separately measured. After that, the oven-dried samples of needles, stems, and roots were ground into fine powder and then stored at room temperature for analysis of NSC and δ13C content. A composite soil sample was collected from each pot, sieved (2 mm), and air-dried for pH analysis.
Non-structural carbohydrate analysis
The total non-structural carbohydrate is defined as the sum of soluble sugars and starch within a sample (i.e., organ or tissue). The NSC concentrations were measured according to the method of Wong (1990) as modified by Hoch et al. (2002). In short, 10–12 mg of fine sample powder was boiled in 2 mL distilled water for 30 min. Then, after centrifugation, invertase and isomerase (Sigma-Aldrich, St Louis, MO, USA) were added into a 200-μL aliquot of the extract to degrade sucrose and convert glucose into fructose. The concentration of soluble sugars was determined photometrically at 340 nm using a multiscan spectrum (Readmax 1900, Shanpu, Beijing, China). The total amount of NSC was measured by taking 500 μL of the extract (including sugars and starch) incubated with a fungal amyloglucosidase from Aspergillus niger (Sigma-Aldrich, St. Louis, MO, USA) for 15 h at 49°C to digest starch into glucose. The starch concentration was calculated as total NSC minus soluble sugars. The NSC concentrations are expressed on a dry mass basis.
Measurements of gas exchange
The net photosynthetic rate was measured between 9:00 and 11:00 h with a LI-6400XT portable photosynthesis system (Li-Cor Inc., Lincoln, NE, USA) at the following chamber conditions: 2 × 3-cm chamber, LED lamp, 40%–70% relative humidity, 400 pm CO2 concentration, 1,500 umol m−2s−1 photosynthetically active radiation, 25°C leaf temperature, and 500 umol s−1 flow rate.
Stable carbon isotope analysis
The 13C abundance in needles was analyzed in an elemental analyzer (Elementar Vario MICRO cube, Hanau, Germany) coupled with a stable isotope ratio mass spectrometer (Isoprime 100, Stockport, UK).
δ13C (‰) = [(Rsample − Rstandard)/Rstandard] × 103
where Rsample and Rstandard are the molecular abundance ratios of the carbon isotopes (13C/12C) of the sample and the standard, respectively. The precision of duplicate measurements was <0.2%.
Soil pH analysis
Soil pH was measured with a soil to water ratio of 1:2.5 (w/v).
Data analysis
To investigate the changes in height at different growth stages, one-way ANOVA was used to determine the height on April 10 and August 23, 2021 and on March 1 and June 21, 2022, respectively. The relative height increment was calculated based on the height measured on April 10, 2021 and June 21, 2022. To investigate the interactive effects of drought duration and biochar on variables, two-way ANOVA was used to determine the effects of drought duration, biochar, and their interactions on sapling biomass and height, concentrations of tissue NSC, needle photosynthesis, and δ13C of P. massoniana saplings as well as the soil pH. All data were checked for normality using the Kolmogorov–Smirnov test and for homogeneity of variance using Levene’s test. The data for NSC in both needles and stems, soluble sugars in stems, and starch in both roots and stems that did not meet the assumption were transformed into log (x + 1) prior to analysis. All statistical analyses were performed using SPSS software version 22.0 (IBM Corp., Armonk, NY, USA).
Results
The growth response
The survival of P. massoniana saplings was significantly decreased along with increasing drought duration ranging from D1 to D4, especially under the application of biochar (Figure 1A). Across the biochar addition, the height of P. massoniana saplings increased with the increasing progress of the experiment; however, it was significantly higher under D1 (122% increment) than the other three drought treatments and showed the lowest value under D4 (70% increment) in the second year (Figure 1B).
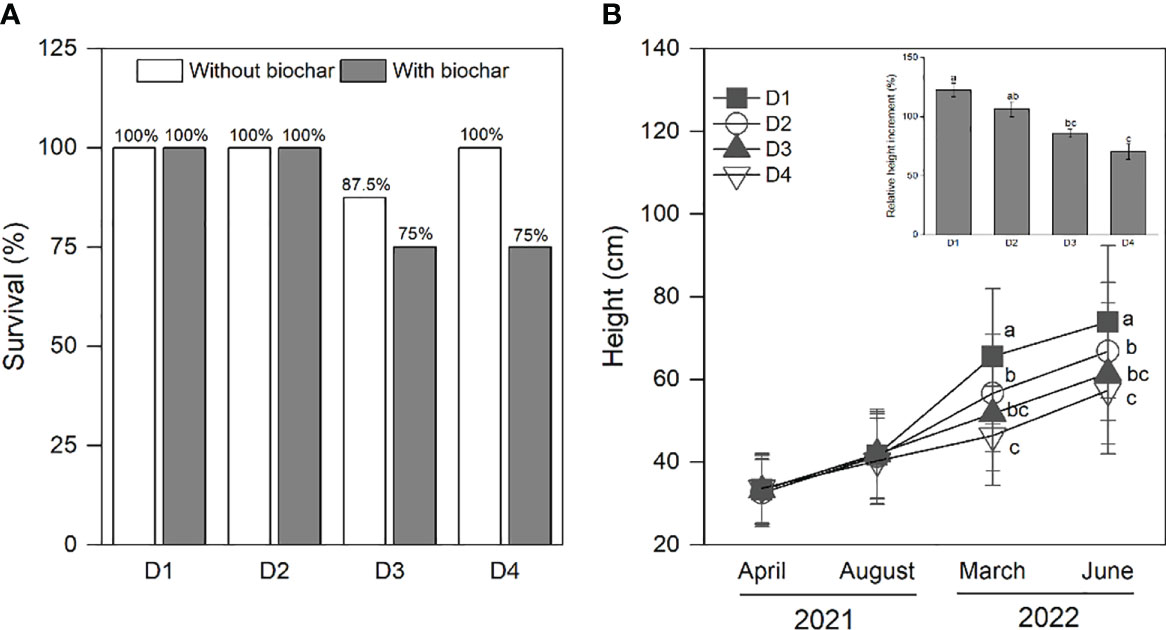
Figure 1 Survival of P. massoniana saplings under the treatments of drought duration and biochar addition (A). Height and relative height increment of P. massoniana saplings under drought duration (B). Biochar addition had no effect on height and relative height increment, and thus saplings without biochar and with biochar were pooled. The mean ± SE of the two-way ANOVAs is given. D represents drought duration. Different letters indicate significant differences among the drought durations.
The biomass of P. massoniana saplings was significantly affected by drought duration, showing a significant decrease in total (Figure 2A) and its component biomass (Figures 2B–D) along with increasing drought duration. Biochar addition significantly decreased the total biomass and needle biomass (Figures 2A, B) but had no effect on the biomass of both stems and roots (Figures 2C, D). There was no significantly interactive effect of biochar and drought duration on the biomass of P. massoniana saplings (Figure 2).
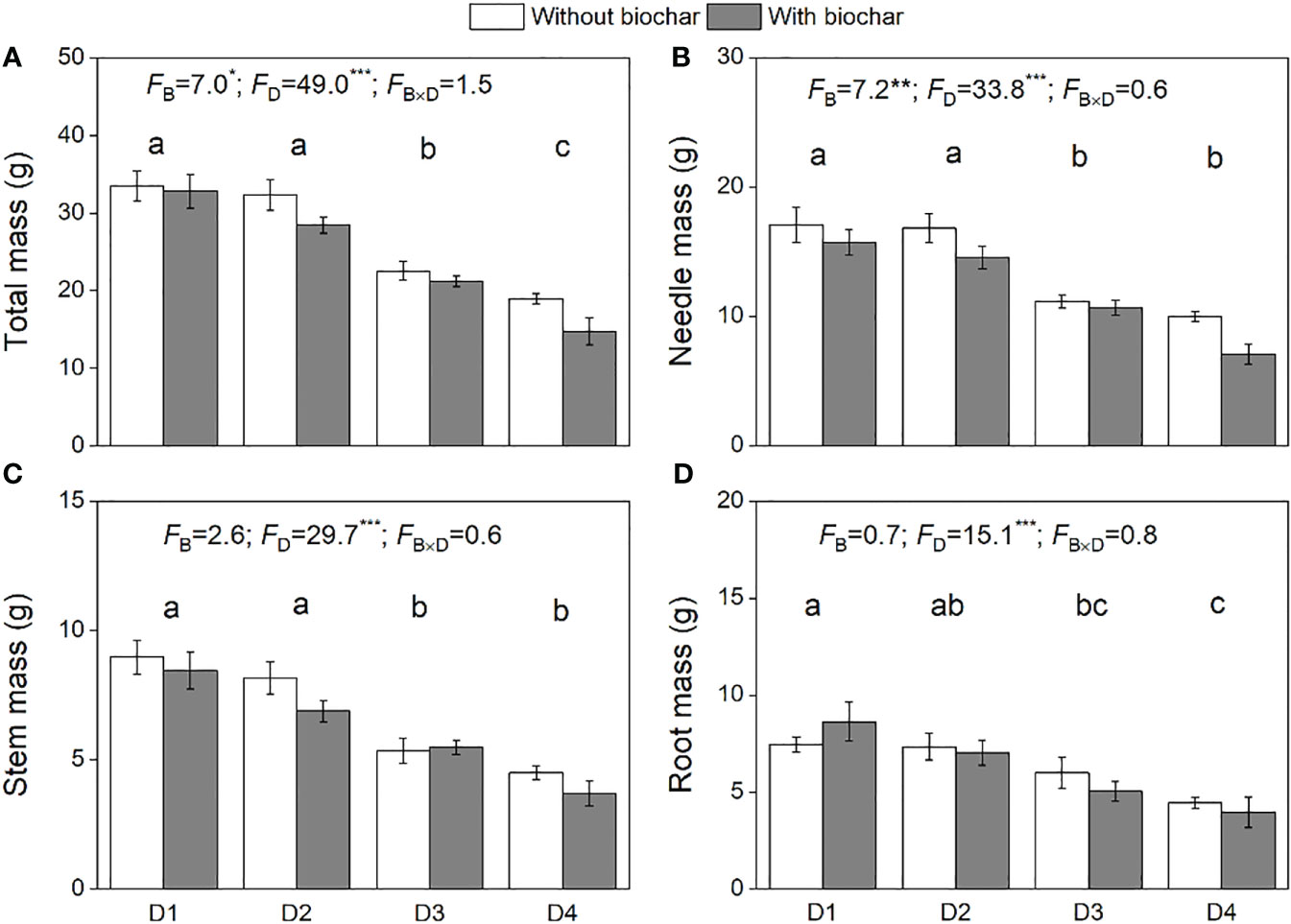
Figure 2 Total biomass (A), needle biomass (B), stem biomass (C), and root biomass (D) of P. massoniana saplings under the treatments of drought duration and biochar addition. The mean ± SE and F values of the two-way ANOVAs are given. B and D represent biochar and drought durations, respectively. Different letters indicate significant differences among the drought durations. *P < 0.05, **P < 0.01 and ***P < 0.001.
The non-structural carbohydrate response
Increasing drought duration did not decrease the NSC concentration in needles (Figure 3A) nor roots (Figure 3C) but significantly increased the NSC concentration in stems (Figure 3B). The concentration of soluble sugars in roots was lower under D4 than in any of the other three drought duration treatments (Figure 3F). Increasing drought duration significantly decreased the ratio of soluble sugars to starch in roots (Figure 3L). Biochar addition significantly decreased the concentrations of NSC in roots (Figure 3C) and soluble sugars in needles (Figure 3D) and roots (Figure 3F) but increased the soluble sugars in stems (Figure 3E). Biochar addition significantly decreased the concentration of starch in stems (Figure 3H) but had no effect on that in needles (Figure 3G) nor in roots (Figure 3I). Neither biochar addition nor drought duration had significant effects on the ratio of soluble sugars to starch (Figure 3J). Biochar addition significantly increased the ratio of soluble sugars to starch in stems (Figure 3K). Drought duration and its combination with biochar had no effect on the concentration of starch in all tissues (Figures 3G–I) but significantly affected the ratio of soluble sugars to starch in roots (Figure 3L).
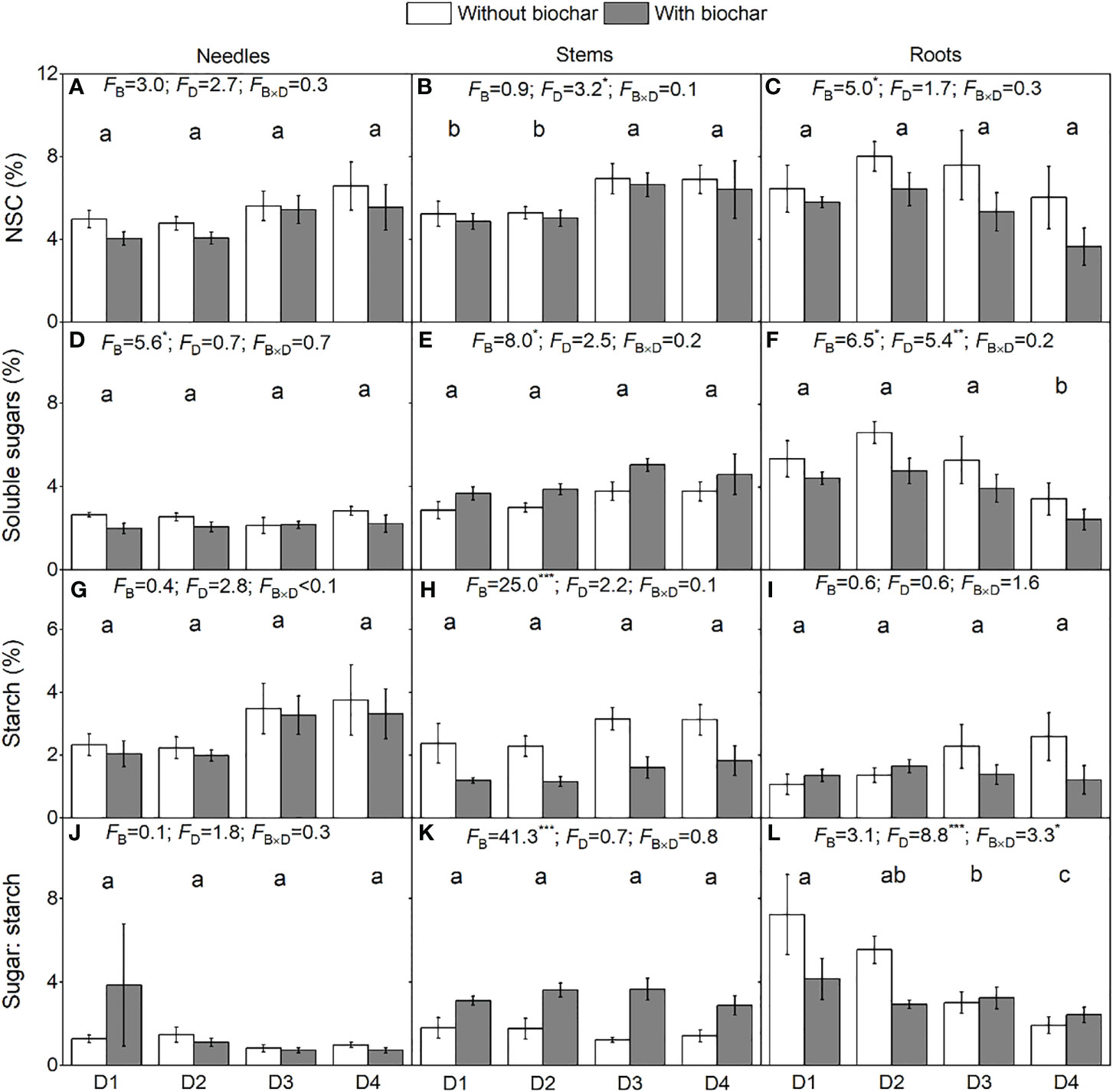
Figure 3 Concentrations of non-structural carbohydrates, soluble sugars, and starch and ratio of soluble sugars to starch in needles (A, D, G, J), in stems (B, E, H, K), and in roots (C, F, I, L) of P. massoniana saplings under the treatments of drought duration and biochar addition. The mean ± SE and F values of the two-way ANOVAs are given. B and D represent biochar and drought durations, respectively. Different letters indicate significant differences among the drought durations. *P < 0.05, **P < 0.01 and ***P < 0.001.
The net photosynthesis and carbon isotope response
Increasing drought duration significantly decreased the net photosynthesis (Figure 4A) but significantly increased the value of δ13C in needles (Figure 4B). However, neither biochar addition nor its combination with drought duration had a significant effect on the net photosynthesis (Figure 4A) and the value of δ13C in needles (Figure 4B).
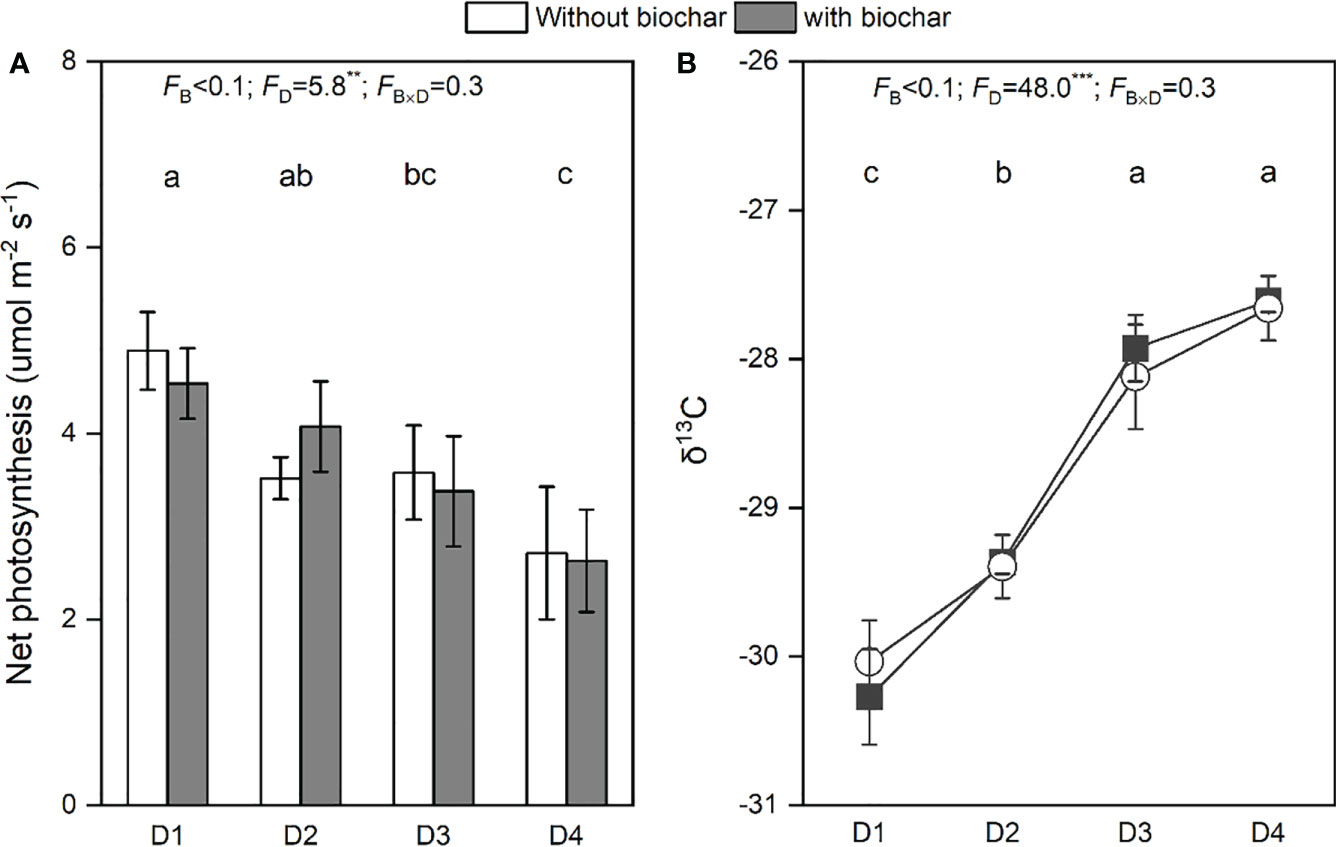
Figure 4 Net photosynthesis (A) and δ13C (B) in needles of P. massoniana saplings under the treatments of drought duration and biochar addition. The mean ± SE and F-values of the two-way ANOVAs are given. B and D represent biochar and drought duration, respectively. Different letters indicate significant differences among the drought durations. **P < 0.01 and ***P < 0.001.
Soil pH
Drought duration had no significant effect on soil pH (Figure 5). However, biochar addition and its combination with drought duration had a significant effect on soil pH, showing a greater increase in soil pH under biochar addition (Figure 5).
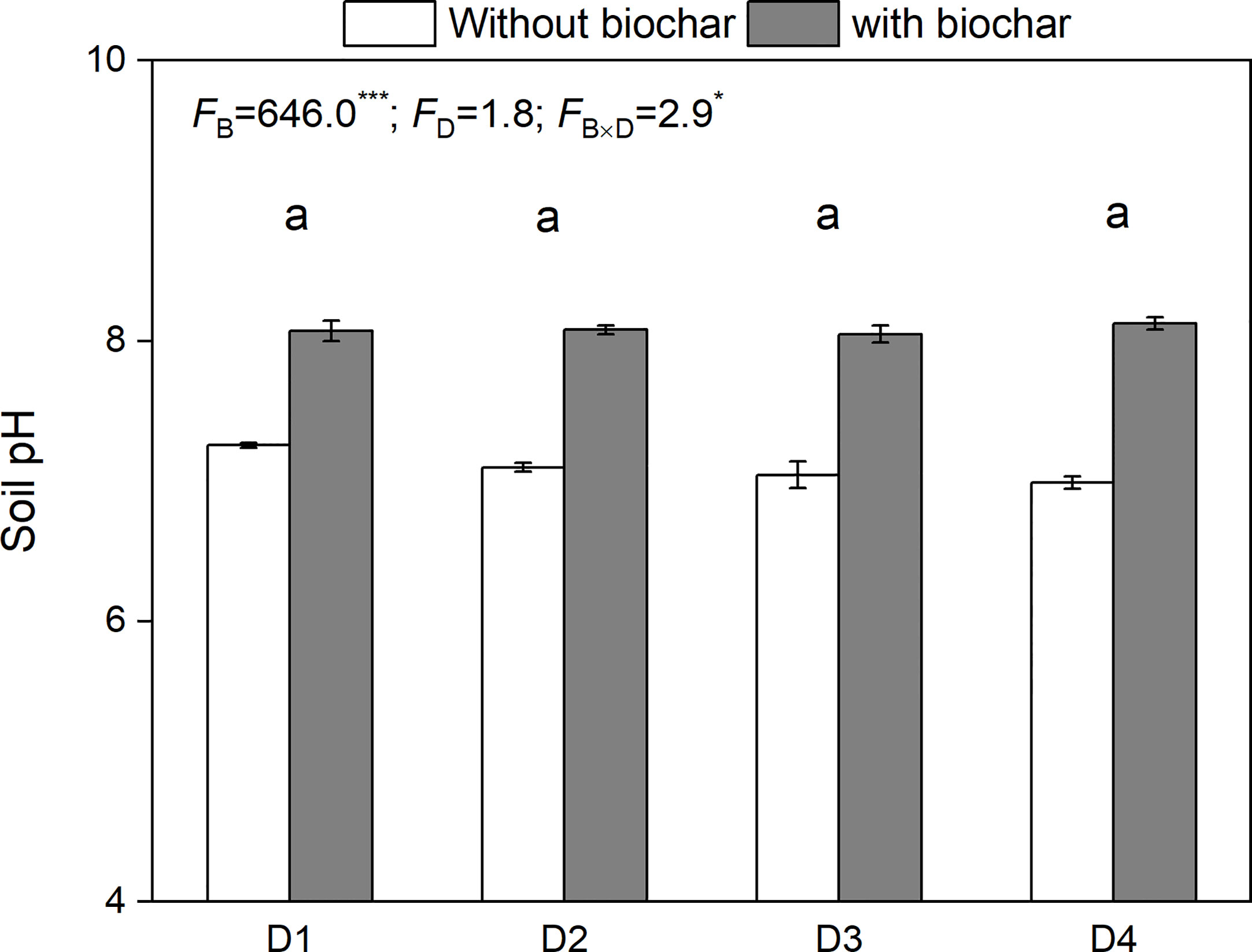
Figure 5 Soil pH under the treatments of drought duration and biochar addition. The mean ± SE and F-values of the two-way ANOVAs are given. B and D represent biochar and drought duration, respectively. Different letters indicate significant differences among the drought durations. *P < 0.05 and ***P < 0.001.
Discussion
Increasing drought duration had a negative effect on the survival of P. massoniana saplings (Figure 1A). We found that the relative height increment and the biomass of P. massoniana saplings were significantly decreased with increasing drought duration (Figures 1B, 2). Similar findings have been reported by many previous studies involving intense drought intensity and a long drought duration (Schönbeck et al., 2020; Ouyang et al., 2021; Yang et al., 2022)—for example, the decreased total biomass of Quercus pubescens (Ouyang et al., 2021) and Quercus castaneifolia (Zoghi et al., 2019) under extreme drought intensity and of Robinia pseudoacacia (Yang et al., 2019), Metrosideros polymorpha (Westerband et al., 2019), and both Quercus petraea and Fagus sylvatica (Yang et al., 2022) under increasing drought duration. The decline in growth under longer drought duration (e.g., the treatment of D4) was likely due to the direct water limitation which inhibited cell expansion and division (Eilmann et al., 2011; Martinez-Sancho et al., 2022). Alternatively, the leaf δ13C value has been used to reflect the ratio of intercellular to atmospheric CO2 concentrations when the carbon is fixed (Farquhar et al., 1982; Farquhar et al., 1989). Under a long drought duration, low water availability generally promotes stomatal closure, decreases the intercellular CO2 concentrations, and subsequently increases the value of δ13C (Sarris et al., 2013; Martinez-Sancho et al., 2022). Thus, the saplings might indirectly increase the stomatal closure to reduce water loss under longer drought duration at the expense of the growth for survival, and the decreased net photosynthesis and less negative value of δ13C under a long drought duration seemed to prove it in the present study (Figures 4A, B). Our results highlight the importance of drought duration in further manipulating the drought experiment.
However, the decline in the growth of P. massoniana saplings was accompanied by unchanged or even increased concentrations of non-structural carbohydrates (Figure 3). The decline in the trees’ growth can be explained by carbon starvation, hydraulic failure, and the interaction between the two (Sala et al., 2012; Sevanto et al., 2014). Carbon starvation occurs when photosynthesis is inhibited through stomatal closure, which can lead to a shortage of mobile carbohydrates for growth (Sala et al., 2012; Adams et al., 2013). Alternatively, under a long drought duration, the formation of embolisms and xylem damage can directly lead to a decline in the trees’ growth from hydraulic failure (Hammond et al., 2019), and when it is prior to the inhibition of leaf photosynthesis, it can further contribute to the accumulation of mobile carbohydrates. In the present study, the concentrations of NSC were higher in aboveground tissues under a longer drought duration, which might be a passive storage of growth inhibition. Additionally, the lower soluble sugar levels in roots under a longer drought duration was likely due to the damage of the top-down transport on the one hand and the more energy devotion to root growth and water absorption on the other hand. Although the decreased levels of soluble sugars in roots occurred under a longer drought duration, the levels of NSC in roots remained unchanged (Figure 3C). The unchanged and even increased NSC in tissues indicated that the response of growth to drought is more sensitive than photosynthesis, resulting in a higher accumulation of NSC in needles, and the shortage of NSC could not be the reason for the decline in growth of P. massoniana saplings in the present study.
Biochar addition impeded the effect of drought duration on the growth and survival of P. massoniana saplings. Many previous studies have reported the beneficial effects of biochar under drought stress (Akhtar et al., 2014; Batool et al., 2015; Paneque et al., 2016; Cai et al., 2020; Liang et al., 2019; Liang et al., 2021b)—for example, the dry weight of Quercus castaneifolia in water stress was significantly increased by biochar addition due to the improvement of both the soil parameters and leaf photosynthesis (Zoghi et al., 2019). Biochar addition could alleviate the negative effects of drought stress on soybean by increasing the leaf photosynthetic rate and stomatal conductance (Zhang et al., 2020) and on rapeseed by enhancing the activities of antioxidants, osmoprotectants, and soil fertility (Khan et al., 2021). In the present study, biochar addition had no effect on the photosynthesis of P. massoniana saplings (Figure 4A); however, it significantly increased the soil pH (Figure 5). Thus, the increased soil pH led to an alkaline soil environment that was not suitable for the growth of P. massoniana saplings, which was similar with our previous study of P. massoniana under pulsed watering (Wang et al., 2022). The result indicated that biochar had negative effects on the growth of P. massoniana saplings under drought in the present study, which implied that the beneficial effects of biochar, the environmentally friendly amendment, might not be applicable for all plant species. However, our study had limitations, such that only one type of biochar or tree sapling was considered, as the response of plants to biochar may vary according to the type of plants as well as the type of soil biochar amendments.
NSC storage, particularly in the roots, could help trees survive drought by being transported to the other organs/tissues where needed because drought might lead to phloem transport malfunction and, subsequently, the possible cessation of photosynthesis (Sevanto et al., 2014; Wiley, 2020). We found that the application of biochar significantly decreased the NSC levels in the roots of P. massoniana saplings, which was largely caused by the decline in soluble sugars (Figure 3F). Soluble sugars can serve as osmolytes to protect the membranes from desiccation injury (Li et al., 2013), and the decline in soluble sugars induced by biochar might affect the water uptake of roots, which subsequently inhibited the growth of P. massoniana saplings.
Conclusions
We found that the height and biomass of Pinus massoniana saplings significantly decreased with increasing drought duration as a result of significantly decreased needle photosynthesis and increased drought stress (i.e., increased needle δ13C values). Additionally, drought duration did not deplete the tissue NSC concentrations, suggesting that the growth inhibition was prior to the consumption of NSC and led to the passive storage of NSC. However, the application of biochar significantly decreased the survival rate of Pinus massoniana saplings and aggravated the negative effect of drought duration on the saplings, which might be due to the increased soil pH. Our results suggest that the effect of biochar addition on mitigating the drought’s effects on plants might be species specific, depending on the species’ biological properties.
Data availability statement
The raw data supporting the conclusions of this article will be made available by the authors without undue reservation.
Author contributions
M-HL and F-HY designed the experiment. XW and W-LZ conducted the greenhouse experiment. XW conducted the chemical analysis and wrote the original manuscript. XM and M-HL reviewed the manuscript. All authors contributed to the article and approved the submitted version.
Funding
This work was supported by the Natural Science Foundation of Zhejiang Province of China (LQ20C160008), Taizhou Scientific and Technological Project (22gya05), and the CAS Strategic Priority Research Programme (A) (XDA20050103). Open access funding by Swiss Federal Institute for Forest, Snow and Landscape Research (WSL).
Conflict of interest
The authors declare that the research was conducted in the absence of any commercial or financial relationships that could be construed as a potential conflict of interest.
Publisher’s note
All claims expressed in this article are solely those of the authors and do not necessarily represent those of their affiliated organizations, or those of the publisher, the editors and the reviewers. Any product that may be evaluated in this article, or claim that may be made by its manufacturer, is not guaranteed or endorsed by the publisher.
References
Adams H. D., Germino M. J., Breshears D. D., Barron-Gafford G. A., Guardiola-Claramonte M., Zou C. B., et al. (2013). Nonstructural leaf carbohydrate dynamics of Pinus edulis during drought-induced tree mortality reveal role for carbon metabolism in mortality mechanism. New Phytol. 197, 1142–1151. doi: 10.1111/nph.12102
Adams H. D., Zeppel M. J. B., Anderegg W. R. L., Hartmann H., Landhausser S. M., Tissue D. T., et al. (2017). A multi-species synthesis of physiological mechanisms in drought-induced tree mortality. Nat. Ecol. Evol. 1, 1285–1291. doi: 10.1038/s41559-017-0248-x
Akhtar S. S., Li G., Andersen M. N., Liu F. (2014). Biochar enhances yield and quality of tomato under reduced irrigation. Agr. Water Manage. 138, 37–44. doi: 10.1016/j.agwat.2014.02.016
Allen C. D., Macalady A. K., Chenchouni H., Bachelet D., Mcdowell N., Vennetier M., et al. (2010). A global overview of drought and heat-induced tree mortality reveals emerging climate change risks for forests. For. Ecol. Manage. 259, 660–684. doi: 10.1016/j.foreco.2009.09.001
Batool A., Taj S., Rashid A., Khalid A., Qadeer S., Saleem A. R., et al. (2015). Potential of soil amendments (biochar and gypsum) in increasing water use efficiency of Abelmoschus esculentus L. Moench. Front. Plant Sci. 6. doi: 10.3389/fpls.2015.00733
Cai J.-F., Zhang L., Zhang Y., Li X., Xia H. L., Kong H.-J., et al. (2020). Remediation of cadmium-contaminated coastal saline-alkaline soil by Spartina alterniflora derived biochar. Ecotox. Environ. Safe 205, 111172. doi: 10.1016/j.ecoenv.2020.111172
Cai J.-F., Jiang F., Liu X.-S., Sun K., Wang W., Zhang M.-X., Li H.-L., Xu H.-F., Kong W.-J., Yu F.-H. (2021). Biochar-amended coastal wetland soil enhances growth of Suaeda salsa and alters rhizosphere soil nutrients and microbial communities. Sci. Total Environ. 788, 147707. doi: 0.1016/j.scitotenv.2021.147707
Chen X., Zhao P., Ouyang L., Zhu L., Ni G., Schafer K. V. R. (2020). Whole-plant water hydraulic integrity to predict drought-induced Eucalyptus urophylla mortality under drought stress. For. Ecol. Manage. 468, 118179. doi: 10.1016/j.foreco.2020.118179
Choat B., Brodribb T. J., Brodersen C. R., Duursma R. A., Lopez R., Medlyn B. E. (2018). Triggers of tree mortality under drought. Nature 558, 531–539. doi: 10.1038/s41586-018-0240-x
Duan H., Li Y., Xu Y., Zhou S., Liu J., Tissue D. T., et al. (2019). Contrasting drought sensitivity and post-drought resilience among three co-occurring tree species in subtropical China. Agr. For. Meteorol. 272, 55–68. doi: 10.1016/j.agrformet.2019.03.024
Eilmann B., Zweifel R., Buchmann N., Graf Pannatier E., Rigling A. (2011). Drought alters timing, quantity, and quality of wood formation in Scots pine. J. Exp. Bot. 62, 2763–2771. doi: 10.1093/jxb/erq443
Farquhar G. D., Ehleringer J. R., Hubick K. T. (1989). Carbon isotope discrimination and photosynthesis. Annu. Rev. Plant Biol. 40, 503–537. doi: 10.1146/annurev.pp.40.060189.002443
Farquhar G. D., O'leary M., Berry J. (1982). On the relationship between carbon isotope discrimination and the intercellular carbon dioxide concentration in leaves. Funct. Plant Biol. 9 (2), 121–137. doi: 10.1071/pp9820121
Fischer B. M. C., Manzoni S., Morillas L., Garcia M., Johnson M. S., Lyon S. W. (2019). Improving agricultural water use efficiency with biochar — a synthesis of biochar effects on water storage and fluxes across scales. Sci. Total Environ. 657, 853–862. doi: 10.1016/j.scitotenv.2018.11.312
George C., Wagner M., Kücke M., Rillig M. C. (2012). Divergent consequences of hydrochar in the plant–soil system: arbuscular mycorrhiza, nodulation, plant growth and soil aggregation effects. Appl. Soil Ecol. 59, 68–72. doi: 10.1016/j.apsoil.2012.02.021
Godlewska P., Schmidt H. P., Yong S. O., Oleszczuk P. (2017). Biochar for composting improvement and contaminants reduction. a review. Bioresource Technol. 246, 193–202. doi: 10.1016/j.biortech.2017.07.095
Hammond W. M., Yu K., Wilson L. A., Will R. E., Anderegg W. R. L., Adams H. D. (2019). Dead or dying? quantifying the point of no return from hydraulic failure in drought-induced tree mortality. New Phytol. 223, 1834–1843. doi: 10.1111/nph.15922
Hoch G., Popp M., Körner C. (2002). Altitudinal increase of mobile carbon pools in pinus cembra suggests sink limitation of growth at the Swiss treeline. Oikos 98, 361–374. doi: 10.1034/j.1600-0706.2002.980301.x
Hu W., Zheng Y., Xia P., Liang Z. (2021). The research progresses and future prospects of Tetrastigma hemsleyanum Diels et Gilg: a valuable Chinese herbal medicine. J. Ethnopharmacol. 271, 113836. doi: 10.1016/j.jep.2021.113836
IPCC (2021). Climate change 2021: the physical science basis. Contribution of Working Group I to the Sixth Assessment Report of the Intergovernmental Panel on Climate Change. Eds. Masson-Delmotte V., Zhai P., Pirani A., Connors S. L., Péan C., Berger S., Caud N., Chen Y., Goldfarb L., Gomis M. I., Huang M., Leitzell K., Lonnoy, Matthews J. B. R., Maycock T. K., Waterfield T., Yelekçi O., Yu R., Zhou B. (Cambridge, UK: Cambridge University Press).
Jain S., Khare P., Mishra D., Shanker K., Singh P., Singh R. P., et al. (2020). Biochar aided aromatic grass [Cymbopogon martini (Roxb.) Wats.] vegetation: a sustainable method for stabilization of highly acidic mine waste. J. Hazard. Mater. 390, 121799. doi: 10.1016/j.jhazmat.2019.121799
Kavitha B., Reddy P. V. L., Kim B., Lee S. S., Pandey S. K., Kim K. H. (2018). Benefits and limitations of biochar amendment in agricultural soils: a review. J. Environ. Manage. 227, 146–154doi: 10.1016/j.jenvman.2018.08.082
Khan Z., Khan M. N., Zhang K., Luo T., Zhu K., Hu L. (2021). The application of biochar alleviated the adverse effects of drought on the growth, physiology, yield and quality of rapeseed through regulation of soil status and nutrients availability. Ind.Crop. Prod. 171, 113878. doi: 10.1016/j.indcrop.2021.113878
Kuppusamy S., Thavamani P., Megharaj M., Venkateswarlu K., Naidu R. (2016). Agronomic and remedial benefits and risks of applying biochar to soil: current knowledge and future research directions. Environ. Int. 87, 1–12. doi: 10.1016/j.envint.2015.10.018
Lawson T., Blatt M. R. (2014). Stomatal size, speed, and responsiveness impact on photosynthesis and water use efficiency. Plant Physiol. 164, 1556–1570. doi: 10.1104/pp.114.237107
Li M. H., Cherubini P., Dobbertin M., Arend M., Xiao W. F., Rigling A. (2013). Responses of leaf nitrogen and mobile carbohydrates in different Quercus species/provenances to moderate climate changes. Plant Biol. 15, 177–184. doi: 10.1111/j.1438-8677.2012.00579.x
Liang J.-F., An J., Gao J.-Q., Zhang X.-Y., Song M.-H., Yu F.-H. (2019). Interactive effects of biochar and AMF on plant growth and greenhouse gas emissions from wetland microcosms. Geoderma 346, 11–17. doi: 10.1016/j.geoderma.2019.03.033
Liang J.-F., Li Q.-W., Gao J.-Q., Feng J.-G., Zhang X.-Y., Hao Y.-J., et al. (2021a). Biochar rhizosphere addition promoted Phragmites australis growth and changed soil properties in the Yellow River Delta. Sci. Total Environ. 761, 143291. doi: 10.1016/j.scitotenv.2020.143291
Liang J.-F., Li Q.-W., Gao J.-Q., Feng J.-G., Zhang X.-Y., Hao Y.-J., et al. (2021b). Biochar-compost addition benefits Phragmites australis growth and soil property in coastal wetlands. Sci. Total Environ. 769, 145166. doi: 10.1016/j.scitotenv.2021.145166
Martinez-Sancho E., Treydte K., Lehmann M. M., Rigling A., Fonti P. (2022). Drought impacts on tree carbon sequestration and water use — evidence from intra-annual tree-ring characteristics. New Phytol. 236, 58–70doi: 10.1111/nph.18224
McAusland L., Vialet-Chabrand S., Davey P., Baker N. R., Brendel O., Lawson T. (2016). Effects of kinetics of light-induced stomatal responses on photosynthesis and water-use efficiency. New Phytol. 211, 1209–1220. doi: 10.1111/nph.14000
Moreno-Gutierrez C., Dawson T. E., Nicolas E., Ignacio Querejeta J. (2012). Isotopes reveal contrasting water use strategies among coexisting plant species in a Mediterranean ecosystem. New Phytol. 196, 489–496. doi: 10.1111/j.1469-8137.2012.04276.x
O'Brien M. J., Leuzinger S., Philipson C. D., Tay J., Hector A. (2014). Drought survival of tropical tree seedlings enhanced by non-structural carbohydrate levels. Nat. Clim. Change 4, 710–714. doi: 10.1038/nclimate2281
O'Donnell A. J., Renton M., Allen K. J., Grierson P. F. (2021). The role of extreme rain events in driving tree growth across a continental-scale climatic range in Australia. Ecography 44, 1–12. doi: 10.1111/ecog.05671
Ouyang S. N., Gessler A., Saurer M. (2021). Root carbon and nutrient homeostasis determines downy oak sapling survival and recovery from drought. Tree Physiol. 41, 1400–1412. doi: 10.1093/treephys/tpab019
Paneque M., de la Rosa J. M., Franco-Navarro J. D., Colmenero-Flores J. M., Knicker H. (2016). Effect of biochar amendment on morphology, productivity and water relations of sunflower plants under non-irrigation conditions. Catena 147, 280–287. doi: 10.1016/j.catena.2016.07.037
Sala A., Woodruff D. R., Meinzer F. C. (2012). Carbon dynamics in trees: feast or famine? Tree Physiol. 32, 764–775. doi: 10.1093/treephys/tpr143
Sapes G., Demaree P., Lekberg Y., Sala A. (2021). Plant carbohydrate depletion impairs water relations and spreads via ectomycorrhizal networks. New Phytol. .229, 3172–3183. doi: 10.1111/nph.17134
Sarris D., Siegwolf R., Koerner C. (2013). Inter- and intra-annual stable carbon and oxygen isotope signals in response to drought in Mediterranean pines. Agr. For. Meteorol. 168, 59–68. doi: 10.1016/j.agrformet.2012.08.007
Schönbeck L., Gessler A., Schaub M., Rigling A., Hoch G., Kahmen A., et al. (2020). Soil nutrients and lowered source: sink ratio mitigate effects of mild but not of extreme drought in trees. Environ. Exp. Bot. 169, 103905. doi: 10.1016/j.envexpbot.2019.103905
Sevanto S., McDowell N. G., Dickman L. T., Pangle R., Pockman W. T. (2014). How do trees die? A test of the hydraulic failure and carbon starvation hypotheses. Plant Cell Environ. 37, 153–161. doi: 10.1111/pce.12141
Spinoni J., Naumann G., Vogt J., Barbosa P. (2015). European drought climatologies and trends based on a multi-indicator approach. Global Planet. Change 127, 50–57. doi: 10.1016/j.gloplacha.2015.01.012
Spinoni J., Vogt J. V., Naumann G., Barbosa P., Dosio A. (2018). Will drought events become more frequent and severe in Europe? Int. J. Climatol. 38, 1718–1736. doi: 10.1002/joc.5291
Verheijen F. G. A., Jeffery S., Bastos A. C., Velde M. V. D., Diafas I. (2010). Biochar application to soils — a critical scientific review of effects on soil properties, processes and functions. EUR 24099 EN (Luxembourg: Office for the Official Publications of the European Communities), 149pp.
Wang X., Tao Y.-M., Fang N.-N., Zheng W.-L., Yu F.-H., Li M.-H. (2022). Impact of growing season precipitation regime on the performance of Masson pine saplings. Forests 13, 627. doi: 10.3390/f13040627
Warnock D. D., Mummey D. L., McBride B., Major J., Lehmann J., Rillig M. C. (2010). Influences of non-herbaceous biochar on arbuscular mycorrhizal fungal abundances in roots and soils: results from growth-chamber and field experiments. Appl. Soil Ecol. 46, 450–456. doi: 10.1016/j.apsoil.2010.09.002
Westerband A. C., Kagawa-Viviani A. K., Bogner K. K., Beilman D. W., Knight T. M., Barton K. E. (2019). Seedling drought tolerance and functional traits vary in response to the timing of water availability in a keystone Hawaiian tree species. Plant Ecol. 220, 321–344. doi: 10.1007/s11258-019-00917-0
Wiley E. (2020). Do carbon reserves increase tree survival during stress and following disturbance? Curr. For. Rep. 6, 14–25. doi: 10.1007/s40725-019-00106-2
Wong S.-C. (1990). Elevated atmospheric partial pressure of CO2 and plant growth. Photosynth. Res. 23, 171–180. doi: 10.1007/BF00035008
Yang Y., Ouyang S., Gessler A., Wang X., Na R., He H. S., et al. (2022). Root carbon resources determine survival and growth of young trees under long drought in combination with fertilization. Front. Plant Sci. 13. doi: 10.3389/fpls.2022.929855
Yang B., Peng C., Zhu Q., Zhou X., Liu W., Duan M., et al. (2019). The effects of persistent drought and waterlogging on the dynamics of nonstructural carbohydrates of Robinia pseudoacacia L seedlings in Northwest China. For. Ecosyst. 6, 23. doi: 10.1186/s40663-019-0181-3
Yao P., Zhou H., Li X., Wei L., Wang J., Zhang S., et al. (2021). Effect of biochar on the accumulation and distribution of cadmium in tobacco (Yunyan 87) at different developmental stages. Ecotox. Environ. Safe 207, 111295. doi: 10.1016/j.ecoenv.2020.111295
Yu G., Chen Z., Piao S., Peng C., Ciais P., Wang Q., et al. (2014). High carbon dioxide uptake by subtropical forest ecosystems in the East Asian monsoon region. P. Natl. Acad. Sci. U.S.A. 111, 4910–4915. doi: 10.1073/pnas.1317065111
Zhang Y., Ding J., Wang H., Su L., Zhao C. (2020). Biochar addition alleviate the negative effects of drought and salinity stress on soybean productivity and water use efficiency. BMC Plant Biol. 20, 288. doi: 10.1186/s12870-020-02493-2
Keywords: carbon isotope, drought duration, Masson pine, non-structural carbohydrate, photosynthesis
Citation: Wang X, Zheng W-L, Ma X, Yu F-H and Li M-H (2023) Biochar aggravates the negative effect of drought duration on the growth and physiological dynamics of Pinus massoniana. Front. Ecol. Evol. 11:1166538. doi: 10.3389/fevo.2023.1166538
Received: 15 February 2023; Accepted: 19 June 2023;
Published: 06 July 2023.
Edited by:
Deliang Kong, Henan Agricultural University, ChinaReviewed by:
Ahmed Kenawy, Mansoura University, EgyptYongyong Zhang, Shenyang Agricultural University, China
Heyong Liu, Hebei University, China
Copyright © 2023 Wang, Zheng, Ma, Yu and Li. This is an open-access article distributed under the terms of the Creative Commons Attribution License (CC BY). The use, distribution or reproduction in other forums is permitted, provided the original author(s) and the copyright owner(s) are credited and that the original publication in this journal is cited, in accordance with accepted academic practice. No use, distribution or reproduction is permitted which does not comply with these terms.
*Correspondence: Fei-Hai Yu, ZmVpaGFpeXVAMTI2LmNvbQ==; Mai-He Li, bWFpaGUubGlAd3NsLmNo
†These authors have contributed equally to this work