- 1Division of Agriculture, Graduate School of Arts and Sciences, Iwate University, Morioka, Japan
- 2Department of Bioresources Science, The United Graduate School of Agricultural Sciences, Iwate University, Morioka, Japan
- 3Kitakyushu Museum of Natural History and Human History, Kitakyushu, Japan
- 4Joint Faculty of Veterinary Medicine, Kagoshima University, Kagoshima, Japan
Two subspecies of mainland leopard cats (Prionailurus bengalensis), Iriomote cats (Prionailurus bengalensis iriomotensis), and Tsushima leopard cats (Prionailurus bengalensis euptilurus), are small, endangered felids that are endemic to the Japanese islands, Iriomote-jima and Tsushima, respectively. Both subspecies have an estimated population size of only about 100 individuals each. Therefore, it is crucial to expand our understanding of these subspecies to successfully conserve them. While their morphology, anatomy, ecology, pathology, and genetics are well studied, little is known about their physiology regulated in subspecies-specific manners. This study characterized their urinary volatile and nonvolatile organic compounds, with which individuals express their physiological status and territorial ownership. We found significantly higher levels of 3-mercapto-3-methyl-1-butanol and its structural analogs, which are scent signals of domestic cats, in the urinary headspace of Iriomote cats than in that of Tsushima leopard cats. There were no differences in the urinary levels of felinine, an amino acid that acts as a precursor of 3-mercapto-3-methyl-1-butanol, suggesting the variation of catalytic activities for decomposing felinine to 3-mercapto-3-methyl-1-butanol between subspecies. Both subspecies exhibited physiological proteinuria due to the secretion of carboxylesterase 5A, also known as cauxin, for the production of felinine. In conclusion, while the two endangered Japanese subspecies of mainland leopard cats share similar genetic backgrounds, urinary contents of metabolites vary between the subspecies. We suggest that after the separation into two subspecies, the regulation of the metabolic pathway for 3-mercapto-3-methyl-1-butanol production likely evolved differently in each subspecies in response to their specific environments.
1. Introduction
The estimated number of species on Earth is approximately 8.7 million (Mora et al., 2011), and new organisms are discovered every year. For instance, the number of known mammalian species increased by 19.9% from 2005 to 2018 (Burgin et al., 2018). Unfortunately, many species are decreasing in population, and some have become extinct (IUCN, 2022). These declines are primarily caused by habitat reduction due to land development, poaching, and global environmental changes (Alan et al., 2006; Newbold et al., 2015; Everatt et al., 2019). The endangered species contain members of the Felidae family, which are classified into 41 species (80 subspecies) (Kitchener et al., 2017). Some larger Felidae species, such as the tiger (Panthera tigris), the lion (Panthera leo), and the Javan leopard (Panthera pardus melas), have experienced significant population declines in recent decades (IUCN, 2022). Two endangered insular subspecies of the mainland leopard cat (Prionailurus bengalensis), Iriomote cats (Prionailurus bengalensis iriomotensis) and Tsushima leopard cats (Prionailurus bengalensis euptilurus) (Figures 1A,B), are endemic to Japan with an estimated population size of about 100 individuals each (Izawa et al., 2000, 2009).
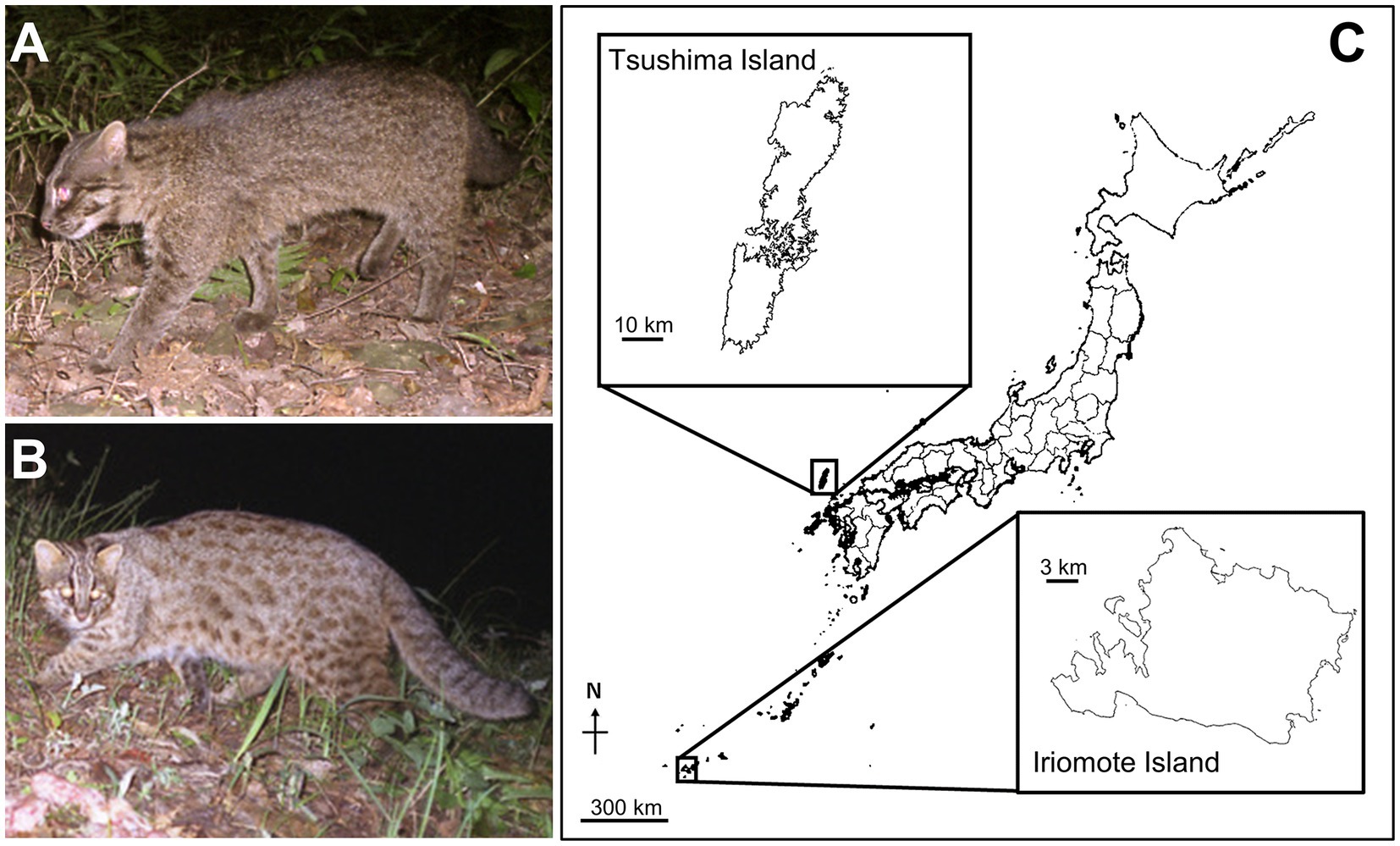
Figure 1. Two Japanese small leopard cats and their habitat. (A,B) Images of an Iriomote cat (Prionailurus bengalensis iriomotensis; A) and a Tsushima leopard cat (Prionailurus bengalensis euptilurus; B). (C) Locations of Iriomote-jima Island and Tsushima Islands in Japan (modified the map from the Geospatial Information Authority of Japan; https://maps.gsi.go.jp/). Each island that distributed Iriomote cats and Tsushima leopard cats is depicted enlarged on the map. Photo credit: Animal Ecology Laboratory, University of the Ryukyus.
The distributions of Iriomote cats and Tsushima leopard cats are limited to small Japanese islands, Iriomote-jima Island (24° 20′ N 123° 48′ E, 284 km2), and Tsushima Islands (34° 25′ N 129° 20′ E, 708.7 km2), respectively (Figure 1C). Both subspecies are listed on the Red List prepared by the Ministry of the Environment in Japan, which has established programs for their protection since 1995. Factors of conservation concern are habitat loss, traffic accidents, and transmission of infectious diseases from domestic cats, such as protozoan diseases and feline immunodeficiency virus (Nishimura et al., 1999; Izawa et al., 2000; Hirata et al., 2012). To prevent the extinction of these subspecies, it is necessary to protect the islands’ wildlife and ecosystems; for examples, building underpasses for animals that can cross under roads without traffic accidents, and management of domestic cats through neutering and an education program for pet owners (Izawa et al., 2000, 2009). In addition, improving our understanding of their feeding habits, nutrition, physiology, pathophysiology, and behavior is crucial to manage them.
Iriomote cats and Tsushima leopard cats have different habitats and feeding habits (Sakaguchi and Ono, 1994; Tatara and Doi, 1994; Izawa et al., 2009; Watanabe, 2012; Díaz-Sacco and Izawa, 2013; Nakanishi and Izawa, 2016; Hisano and Newman, 2020). Climates of distribution areas of Iriomote cats and Tsushima leopard cats are subtropical and temperate zones, respectively. Iriomote cats are considered to live with higher density in coastal lowlands including damp grasslands and mangrove forests, where they feed not only on small mammals and birds but also on reptiles, amphibians, and insects. By contrast, Tsushima leopard cats live in montane forests and occasionally appear in agricultural areas, and they have meat-based diets and mainly feed on mammals and birds. Given these differences, we wondered whether there may be variation in physiology and metabolism between the two subspecies despite their genetic similarity and official classification as mainland leopard cats (Masuda and Yoshida, 1995; Kitchener et al., 2017; Patel et al., 2017). However, little is known about the physiological properties of these two subspecies due to their low abundance.
Here, we compared urinary components between Iriomote cats and Tsushima leopard cats. Urinary components in mammals are important not only for expressing physiological status but also for scent communication among intraspecies and interspecies (Hurst and Beynon, 2004; Apfelbach et al., 2005; Wyatt, 2014). The urine of Iriomote cats has a stronger odor to the human nose than that of Tsushima leopard cats, so we first examined the chemical contents of the volatile organic compounds emitted from their urine. In this study, we found the variation in the emission amounts of sulfur-containing volatile organic compounds, 3-mercapto-3-methyl-1-butanol (MMB) and its structural analogs, between two subspecies. MMB is known as a scent signal of domestic cats (Felis silvestris catus) (Miyazaki et al., 2008; Miyazaki et al., 2018). Then we investigated the urinary levels of felinine, which is an amino acid of the MMB precursor (Datta and Harris, 1952; Westall, 1953; Miyazaki et al., 2006), as well as its related compounds. Finally, we examined the excretion levels of cauxin, a member of the carboxylesterase family that is a major urinary protein in domestic cats and produces felinine (Miyazaki et al., 2003; Miyazaki et al., 2006). These studies improve our understanding of the physiology of Japanese leopard cats, which can contribute to the conservation and management of these endangered subspecies.
2. Materials and methods
2.1. Urine sampling
Thirty and twenty urine samples were collected from 27 Iriomote cats (18 males and 9 females) and 17 Tsushima leopard cats (10 males and 7 females), respectively. Three of the 30 Iriomote cat urine samples and three of the 20 Tsushima leopard cat urine samples were collected from same individuals at different days (see Supplementary Table S1). Using box-traps, the Iriomote cats were captured on Iriomote-jima Island, Okinawa Prefecture, between 2006 and 2022 in December and early February, and the Tsushima leopard cats were captured on Tsushima Islands, Nagasaki Prefecture between 2011 and 2018 in December and January when females were not pregnant. All individuals were anesthetized with medetomidine (Domitor, Nippon Zenyaku Kogyo Co., Ltd, Fukushima, Japan) [0.05 mg/kg intramuscularly (IM)] and ketamine hydrochloride (Ketalar, Daiichi Sankyo Co., LTD, Tokyo, Japan) (10 mg/kg IM), and then veterinarians pressed their bladders gently to collect their urine samples. The mean ± standard error of urine volume were 9.0 ± 0.7 mL and 10.5 ± 0.6 mL in urine samples of Iriomote cats and Tsushima leopard cats, respectively (Supplementary Table S1). They were implanted with identification microchips in the scapular region and then released back to their natural habitat. Domestic cat urine samples were collected from a healthy intact male cat, a healthy intact female cat, and a male cat exhibiting hematuria by compressible urination. These cats were kept as experimental animals at Iwate University. All urine samples were stored at −80°C until analysis without freezing and thawing. The Iriomote cat and Tsushima leopard cat were captured under the permission of the Ministry of the Environment and the Agency for Cultural Affairs in Japan. The Animal Research Committee of Iwate University approved urinary sampling from the cats.
2.2. Analysis of urine
After thawing the urine samples, they were centrifuged for 5 min at 500 × g and 4°C to remove debris. Urinary glucose, blood, protein, and pH were evaluated using a Hemacombistix urine test strip (Siemens Healthcare Japan Co., Ltd., Tokyo, Japan). Urinary-specific gravity was measured using a master refractometer (Atago Co., Ltd., Tokyo, Japan). Urinary concentrations of proteins and creatinine were measured using Quick Start Bradford 1× Dye Reagent (BioRad Laboratories, Inc., Hercules, CA, United States) and LabAssay™ Creatinine (Fujifilm Wako Pure Chemical Co.), respectively.
2.3. Thermal desorption gas chromatography/mass spectrometry
Urine samples (2 mL) and 1 g dried sodium chloride (Fujifilm Wako Pure Chemical Corp., Osaka, Japan) for salting out were transferred to a 20 mL screw-cap vial (GESTEL, Mülheim an der Ruhr, Germany) and incubated at 40°C; the volatile organic compounds emitted from each sample were concentrated into an adsorption glass tube containing 300 mg Tenax TA (Shimadzu Co., Kyoto, Japan) by purging with pure nitrogen gas at 60 mL/min for 90 min. Volatile organic compounds trapped in Tenax TA were desorbed at 250°C for 10 min using a thermal desorption system (TD-20, Shimadzu Co.), which was directly injected into a QP-2010 Ultra gas chromatograph/mass spectrometer (GC/MS) equipped with a Stabiliwax capillary column (60 m × 0.25 mm × 0.25 μm, Restek, Bellefonte, PA, USA) in splitless mode. The GC oven temperature was held at 40°C for 2 min, increased to 238°C at a rate of 6°C/min, and held at 238°C for 20 min. The MS was operated in electron impact mode with the electron energy set to 70 eV and the ion source temperature set to 200°C. Mass spectra were obtained in full-scan mode from m/z 35 to 500. Artificial synthetic compounds MMB (Sigma Aldrich, St Louis, MO, United States) and 3-mercapto-3-methyl-1-butyl-1-formate (MMBF, Seikodo Ishida Co., Ltd., Osaka, Japan), were used to generate a standard curve. Calibration curves for MMB and MMBF were generated using 0.0195, 0.0781, 0.3125, 1.25, and 5.00 ng of each, respectively. Data acquisition and processing were carried out with GCMSsolution ver. 4.53 (Shimadzu Co.). MMB and MMBF concentrations were quantified based on the areas of the m/z 86 and 102 peaks of the full-scan data, respectively. The limit of detection was assessed through a signal-to-noise ratio value of 5. The lower limit of quantification was 0.0195 ng for both MMB and MMBF. The NIST08 MS library and our previous paper (Miyazaki et al., 2006) were used for the initial identification of compounds.
2.4. Liquid chromatography/mass spectrometry
Urine samples (200 μL) were deproteinized using Vivaspin 500–5 k (Sartorius Stedim Lab Ltd., Stonehouse, United Kingdom). The filtrate was diluted 200 or 1,000 times with 0.1% formic acid in Milli-Q (MQ) water and then applied to an LCMS-8040 system (Shimadzu Co.) to quantify felinine and its related compounds, 3-methylbutanol glutathione (MBG), 3-methylbutanol cysteinyl-glycine (MBCG), and N-acetylfelinine, based on a previous report (Futsuta et al., 2018). Chromatographic separation of these compounds was performed using L-colomn2 (Chemical Evaluation and Research Institute, Tokyo, Japan) with solvent A (0.1% formic acid in MQ water) and solvent B [0.1% formic acid in methanol (LC/MS grade, Fujifilm Wako Pure Chemical Corp.)] at a flow rate of 0.4 mL/min. The time program for separation was as follows: initial incubation for 2.5 min at 0% solvent B followed by an increase in solvent B to 10% in 7.5 min and a final incubation for 7 min at 100% solvent B. The effluent from the high-performance liquid chromatography step was introduced online into a triple-quadrupole MS. The MS was operated in the multiple reaction-monitoring (MRM) mode using the following parameters: MRM transitions: m/z 208.2 → 122.0 [collision energy (CE) 12 eV] for felinine, m/z 265.1 → 178.9 (CE 11 eV) for MBCG, m/z 394.2 → 179.0 (CE 17 eV) for MBG, and m/z 250.1 → 121.9 for N-acetylfelinine; dwell time 100 ms, 230 kPa collision-induced dissociation gas, ESI in positive mode, 3 L/min nebulizer gas, and 15 L/min drying gas heated at 250°C. Data acquisition and processing were performed in LabSolutions ver. 5.118 (Shimadzu Co.).
2.5. SDS-PAGE and Western blotting
The compositions of 100 μL urine samples were added equal volume of SDS loading buffer with or without 2-mercaptoethanol as a reductant and, after boiling for a few minutes, 5 and 15 μL solution were analyzed by SDS-PAGE (10% gels), respectively. Gels were stained using Coomassie brilliant blue (CBB) staining solution (EzStain Aqua, ATTO Co., Tokyo, Japan). Western blotting using anti-cauxin polyclonal antibodies (Miyazaki et al., 2003) was carried out to detect cauxin in the urine. In brief, 0.2 μL urine samples were analyzed by SDS-PAGE (10% gels) under reducing or non-reducing conditions and then transferred to polyvinylidene fluoride [PVDF (Immobilon®-P Transfer Membrane, Merk Millipore Ltd., Burlington, MA, United States)] membranes by wet blotting (Mini Trans-Blot® Electrophoretic Transfer Cell, BioRad Laboratories, Inc., Hercules, CA, United States). PVDF membranes were blocked with tris-buffered saline with Tween 20 (10 mM Tris–HCl, pH 7.4, 150 mM sodium chloride, 0.05% Tween 20) containing 2% skim milk (Fujifilm Wako Pure Chemical Corp.) and then incubated with a 1:6,000 dilution with rabbit anti-domestic cat cauxin antibody followed by a 1:6,000 dilution with horseradish peroxidase-conjugated goat anti-rabbit immunoglobulin G (BioRad Laboratories, Inc.). The cauxin band was detected using Enhanced chemiluminescence reagents (GE HealthCare Technologies Inc., Chicago, IL, United States) and analyzed with the Image Quant™ LAS 4000 imaging system (GE HealthCare Technologies Inc.).
2.6. Statistical analysis
Data analyses were performed in R ver. 4.2.1 (The R Foundation for Statistical Computing, Vienna, Austria), SPSS ver. 29.0.0.0 (IBM Corp., Armonk, NY, United States), and JMP v12.0.0 (SAS Institute Inc., Cary, NC, United States). Parametric analyses were carried out after checking approximated normality of the values by Shapiro-Wilks test. Each felinine-related compound was compared between each subspecies and sex using a non-parametric Kruskal-Wallis test (asymptotic p-value) with Steel-Dwass post-hoc test comparing each group. Differences in urinary protein concentration between each subspecies and sex were determined using a one-way ANOVA with the Tukey’s HSD test. The significance level was set to 0.05.
3. Result
3.1. Analysis of urine
We found urinary glucose in 1 of 18 male and 1 of 12 female Iriomote cat urine samples and in 3 of 11 male and 3 of 9 female Tsushima leopard cat urine samples (see Supplementary Table S1). Urinary blood was detected in 8 Iriomote cat urine samples (4 males and 4 females) and 3 male Tsushima leopard cat urine samples. Urinary pH ranged from 6 to 7.5 (mode: 6.5) in the Iriomote cats and from 6 to 7.5 (mode: 6.0) in the Tsushima leopard cats. The urine of all but one leopard cats showed proteinuria. Urine specific gravity ranged from 1.029 to 1.074 (mean: 1.053) in Iriomote cats and from 1.015 to 1.070 (mean: 1.049) in Tsushima leopard cats.
3.2. Urinary volatile organic compounds in the two leopard cats
Volatile organic compounds emitted from the urine were concentrated into an adsorbent and analyzed them using a thermal desorption GC/MS system. Figures 2B,C show representative total ion chromatograms of volatile organic compounds emitted from the male urine samples of Iriomote cats and Tsushima leopard cats, respectively. The urine of Iriomote cats had substantially more peaks than that of Tsushima leopard cats in both sexes. MMB was detectable in all of the 18 male Iriomote cats as one of the major peaks (Figures 2B,D,F). By contrast, it was detectable only in 5 out of 10 females as a minor peak. In this subspecies, the medians of MMB emission were 8.6 ng/mL/90 min in the 18 males (range: 0.05–17.33 ng/mL/90 min) and 3.18 ng/mL/90 min (range: 0.04–11.34 ng/mL/90 min) in the 5 females (Figure 2H). In addition, other sulfur-containing volatile organic compounds, candidates of felinine derivatives, such as MMBF, 3-methyl-3-methylthio-1-butanol, and 3-methyl-3-(2-methyldisulfanyl)-1-butanol (Miyazaki et al., 2006), were also emitted from Iriomote cat urine (Figures 2B,E,G). Although MMBF was detectable in 17 male samples, its levels were below the quantification limit in 13 of the 17 urine samples, and therefore, the median MMBF concentration calculated for the 4 male samples was 0.03 ng/mL/90 min (range: 0.01–0.07 ng/mL/90 min) (Figure 2I). In contrast to Iriomote cats, MMB and MMBF were not detectable in both sexes of Tsushima leopard cats in which only 3-methyl-3-methylthio-1-butanol and/or 3-methyl-3-(2-methyldisulfanyl)-1-butanol were emitted from their urine. Other characteristic volatile organic compounds for small Felidae animals, such as 3-methyl-3-buten-1-ol and 3-methyl-2-buten-1-ol (Mattina et al., 1991; Miyazaki et al., 2017), were detected in both leopard cats.
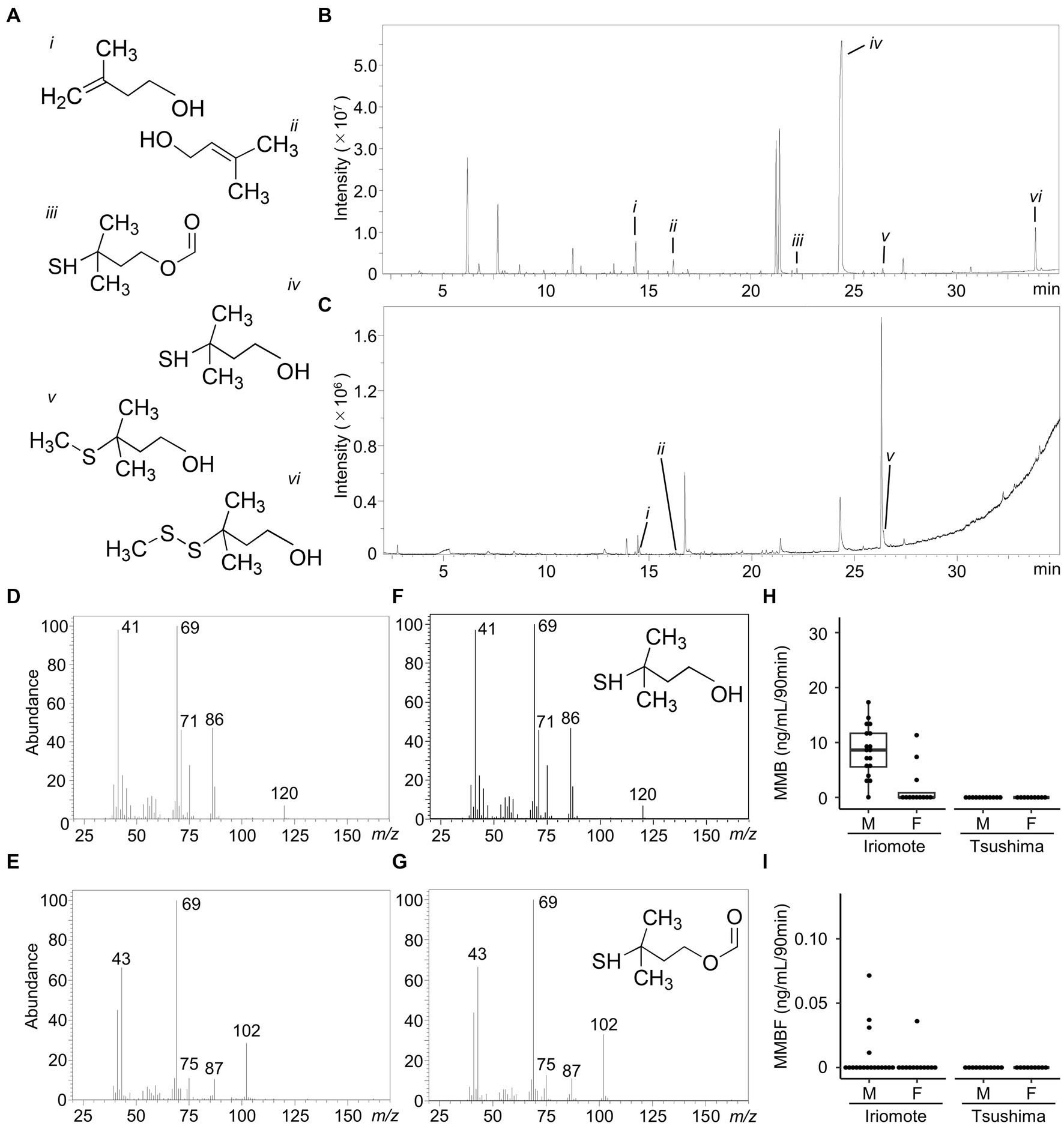
Figure 2. Chemical profiles of urinary volatile organic compounds in the two Japanese leopard cats. (A) Chemical structure of compounds. (i) 3-methyl-3-buten-1-ol, (ii) 3-Methyl-2-buten-1-ol, (iii) 3-mercapto-3-methyl-1-butyl-1-formate (MMBF), (iv) 3-mercapto-3-methyl-1-butanol (MMB), (v) 3-methyl-3-methylthio-1-butanol, (vi) 3-methyl-3-(2-methyldisulfanyl)-1-butanol. (B,C) Representative GC/MS total ion chromatograms of volatile organic compounds emitted from urine samples of a male Iriomote cat (B) and a male Tsushima leopard cat (C). Numbers in peaks are corresponding to (A). (D–G) Mass spectra of the compounds detected at 24.356 min (D) and 22.291 min (E) in the Iriomote cat urine and authentic samples of MMB (F) and MMBF (G). (H,I) Box and whisker plots of MMB (H) and MMBF (I) emission levels from urine samples of Iriomote cats and Tsushima leopard cats. M and F indicate male and female, respectively. Boxes show median and interquartile range, whiskers are first data points within 1.5 interquartile ranges, and points are individual values.
3.3. Urinary excretions of felinine and its related compounds in the two leopard cats
MMB is a derivative of felinine (Figure 3A) found in domestic cats (Miyazaki et al., 2006; Starkenmann et al., 2015). Felinine is produced through the hydrolysis of a dipeptide, MBCG using cauxin. A glutathione-conjugate, MBG is an upstream precursor of felinine and is converted into MBCG by γ-glutamyl transferase. A portion of felinine produced in vivo is metabolized to N-acetylfelinine. Based on the urinary volatile organic compound analysis, we examined the excretion levels of felinine and its related compounds, MBCG, MBG, and N-acetylfelinine in both subspecies.
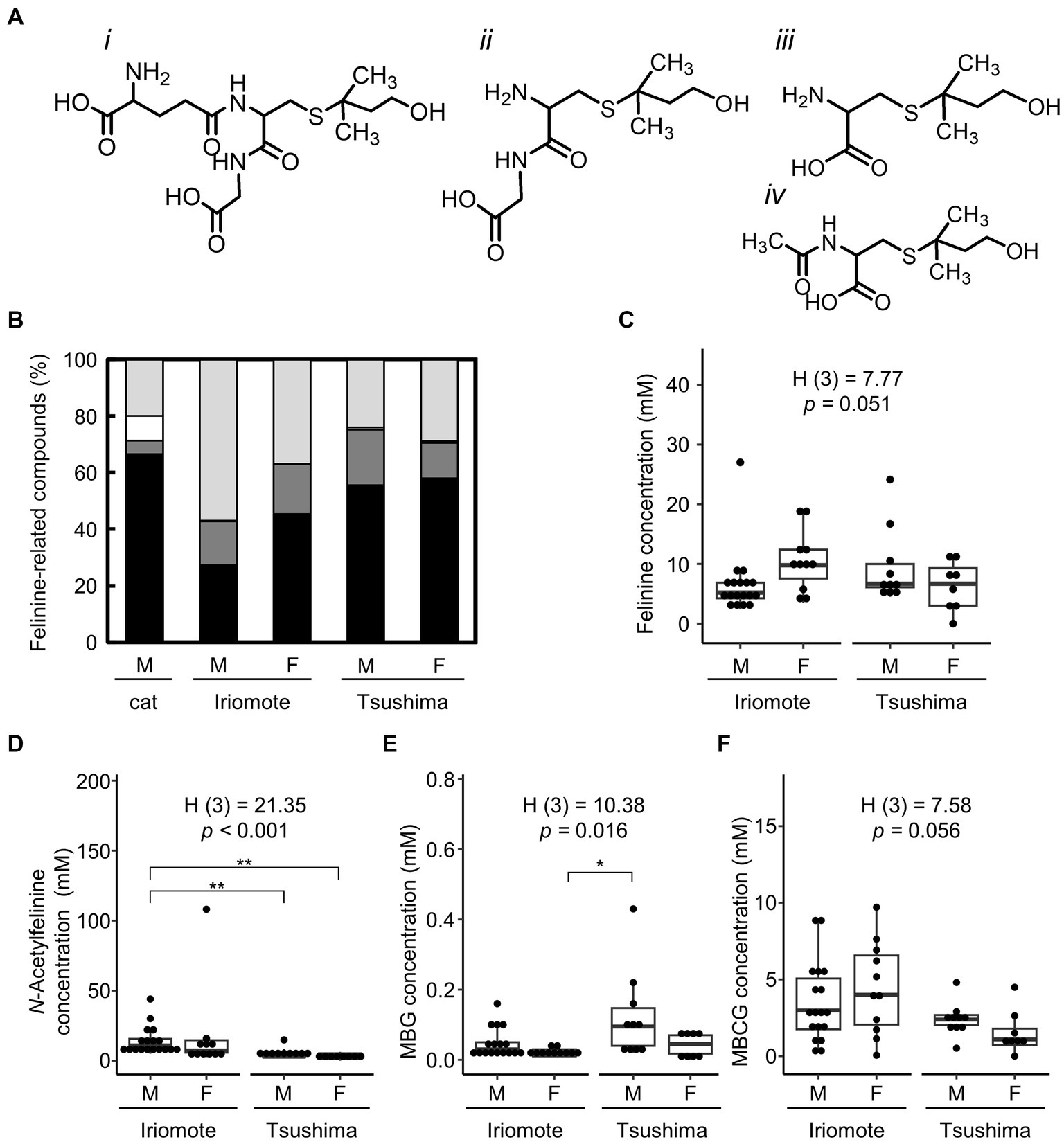
Figure 3. Urinary excretion levels of felinine and its related compounds in the two Japanese leopard cats. (A) Chemical structures of 3-methylbutanol glutathione (MBG, i), 3-methylbutanol cysteinyl-glycine (MBCG, ii), felinine (iii), and N-acetylfelinine (iv). (B) Percentage of urinary contents of felinine and its related compounds in domestic cats (cat), Iriomote cats (Iriomote), and Tsushima leopard cats (Tsushima). Data on domestic cats are used from the previous report (Hendriks et al., 2004). Black, dark gray, white, and light gray indicate felinine, MBCG, MBG, and N-acetylfelinine, respectively. (C–F) Urinary excretion levels (mM) of felinine, N-acetylfelinine, MBG, and MBCG in Iriomote cats (Iriomote) and Tsushima leopard cats (Tsushima). M and F mean males and females, respectively. Boxes show median and interquartile range, whiskers are first data points within 1.5 interquartile ranges, and points are individual values. H test statistics, degrees of freedom, and p-values are from Kruskal-Wallis tests. Asterisks show significant differences calculated with Steel-Dwass test between each subspecies and sex (*p < 0.05, **p < 0.01).
Although previous studies showed that the concentration of felinine is much higher than that of its related compounds in domestic cats (Hendriks et al., 2004, 2008), there were no significant differences in excretion levels between felinine and MBCG or N-acetylfelinine in either subspecies (Figure 3B). Figure 3C shows urinary felinine levels in the two subspecies. The median of urinary felinine concentration was 5.21 mM (range: 2.41–27.02 mM) in 18 male Iriomote cats, 10.07 (range: 3.52–19.05) in 12 female Iriomote cats, 6.52 (range: 0.98–24.13) in 11 male Tsushima leopard cats, and 5.79 (range: 0.01–11.31) in 9 female Tsushima leopard cats. In contrast to the differences in MMB levels between the two subspecies and sexes in Iriomote cats, there were no significant differences in felinine excretion levels between male Iriomote cats and male Tsushima leopard cats (p = 0.298) or between males and females in Iriomote cats (p = 0.054).
Urinary N-acetylfelinine levels were significantly higher in male Iriomote cats than in male Tsushima leopard cats (Figure 3D). Urinary levels of the felinine precursors MBG (Figure 3E) and MBCG (Figure 3F) showed no significant differences between subspecies in each sex or between sexes in each subspecies.
3.4. Major urinary proteins in the two leopard cats
We examined the urinary excretion of cauxin in Iriomote cats and Tsushima leopard cats. Figures 4A,B show CBB-stained gels representative of urine samples obtained from Iriomote cats and Tsushima leopard cats, respectively. In both subspecies, a 70 kDa band was detectable, representing a major urinary protein of the same molecular weight as cauxin in domestic cats (Miyazaki et al., 2003). Western blotting using an anti-cauxin antibody confirmed that the 70 kDa protein was cauxin (Figure 4C). Some bands with over 150 kDa were also detected for both subspecies under non-reducing conditions but not under reducing conditions and such bands were stained by Western blotting using the antibody under non-reducing condition (Figure 4C). Considering also a previous study (McLean et al., 2007), we determined that these bands were oligomers of cauxin. In addition, a 66 kDa albumin band was detected in urine samples with blood of the leopard cats, as detected in hematuria of domestic cats.
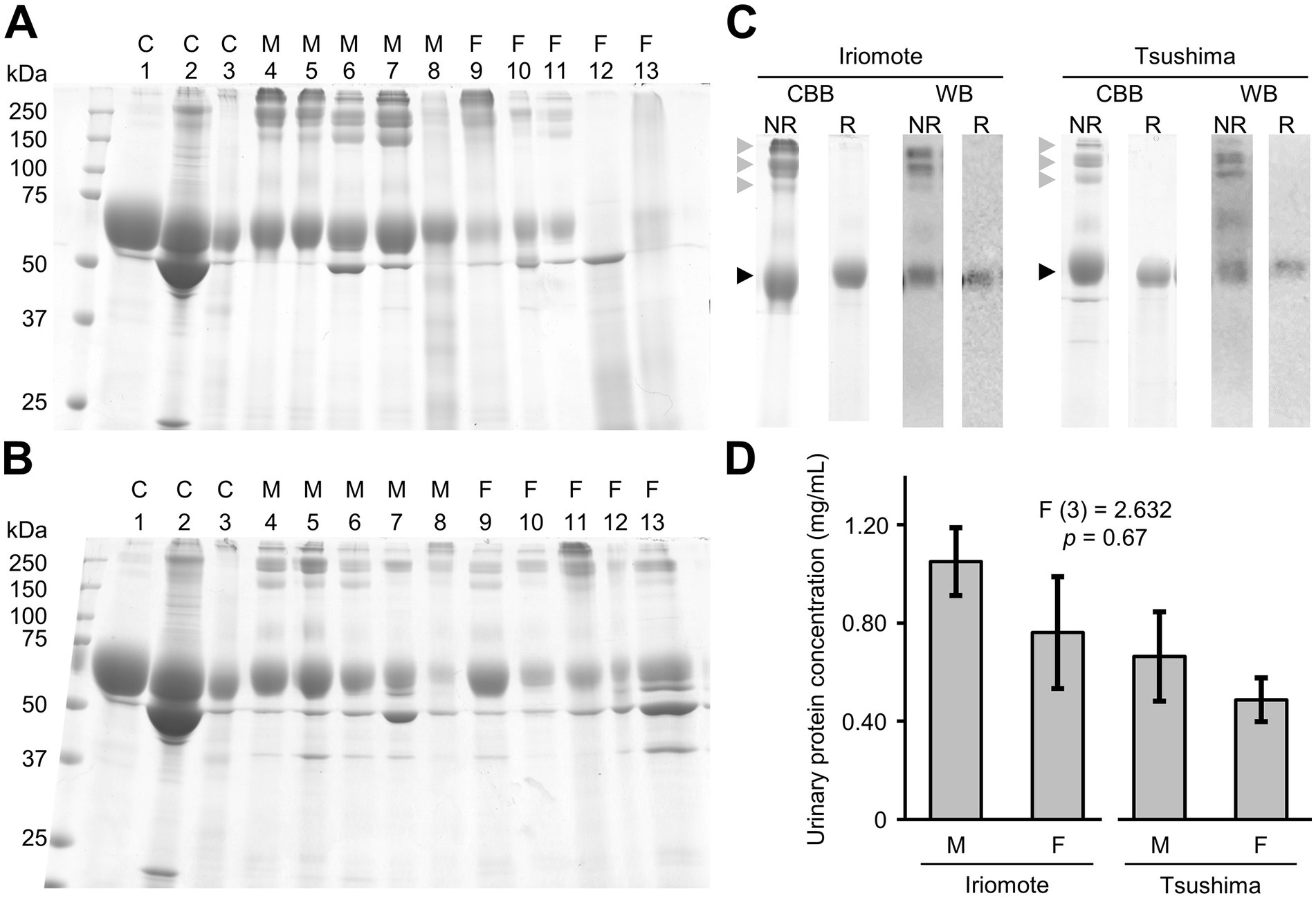
Figure 4. Urinary cauxin excretion of the two Japanese leopard cats. (A,B) Urine samples (7.5 μL) of Iriomote cats (A) and Tsushima leopard cats (B) were resolved by SDS-PAGE analysis under non-reducing condition. Gels were stained using CBB staining solution. C1, C2, and C3 mean male domestic cat urine, male domestic cat with hematuria, and female domestic cat urine, respectively. M and F mean samples of males and females, respectively. Individual information was described in Supplementary Table S1. (C) CBB-staining (CBB) and Western blotting (WB) using anti-cauxin antibodies of urine samples obtained from Iriomote cats (Iriomote) and Tsushima leopard cats (Tsushima) under non-reducing (NR) and reducing conditions (R). Black and gray triangles indicate a monomer and oligomers of cauxin, respectively. (D) Each urinary protein concentration to urinary creatinine concentration (mean ± standard error) of 14 males and 6 females in Iriomote cats and 8 males and 9 females in Tsushima leopard cats. Since the stained gels showed that most urine samples in both subspecies contained cauxin and its oligomers, the urinary protein concentration (mg/mL) was used to estimate urinary cauxin levels in this study. Albuminuria or hematuria was excluded from the data analysis. F-test statistics, degrees of freedom, and p-values are from one-way ANOVA. There was no significant difference among each group in Tukey’s HSD test.
CBB-stained gels showed that most urine samples in both subspecies contained cauxin and its oligomers, indicating that excretion levels of urinary cauxin could be approximately estimated based on urinary protein concentration. Therefore, urine samples, except for those with blood and albuminuria, were compared between the two subspecies in each sex and between sexes in each subspecies. The mean ± standard error (SE) of urinary protein concentration (mg/mL) of male Iriomote cats and male Tsushima leopard cats were 1.05 ± 0.14 (n = 14) and 0.66 ± 0.18 (n = 8), respectively, and those of female Iriomote cats and Tsushima leopard cats were 0.76 ± 0.23 (n = 6) and 0.49 ± 0.09 (n = 8), respectively. There were no significant differences between each group (Figure 4D).
4. Discussion
This study characterized the urinary components of two endangered Japanese subspecies of the mainland leopard cat (Figure 5). A major effort in this study was the collection of urine samples from 27 and 17 endangered leopard cats in two islands where there are only about 100 individuals each. Analyses of urine using urine test strips and a refractometer indicate that the urine of most individuals exhibited moderate compound levels based on the typical levels of domestic cats (glucose-negative, blood-negative, protein-positive, weakly acidic pH, and urine specific gravity of 1.015–1.060). Some individuals exhibited high urinary levels of glucose and blood. These abnormal values are likely artifacts caused by urinary sampling. A transient increase in glucose levels unrelated to feeding is widely recognized as a stress indicator in animals (Nelson, 2002). We presume that some leopard cats experienced severe stress immediately before urine sampling, considering that urinary samples were collected from leopard cats captured in live traps. Furthermore, because urine samples were collected by compressible urination in anesthetized animals in the fields, blood might have contaminated the urine due to injuries of bladder epithelial cells. In a previous study, we found that serum levels of felinine and its related compounds were under 100 μM in domestic cats (Futsuta et al., 2018), indicating that blood contamination should have little effect on urinary levels of felinine and its related compounds. Considering that samples from endangered species whose population includes only about 100 individuals are valuable, we decided to analyze urinary metabolites in all urine samples collected in this study and urinary proteins in urine samples without the blood.
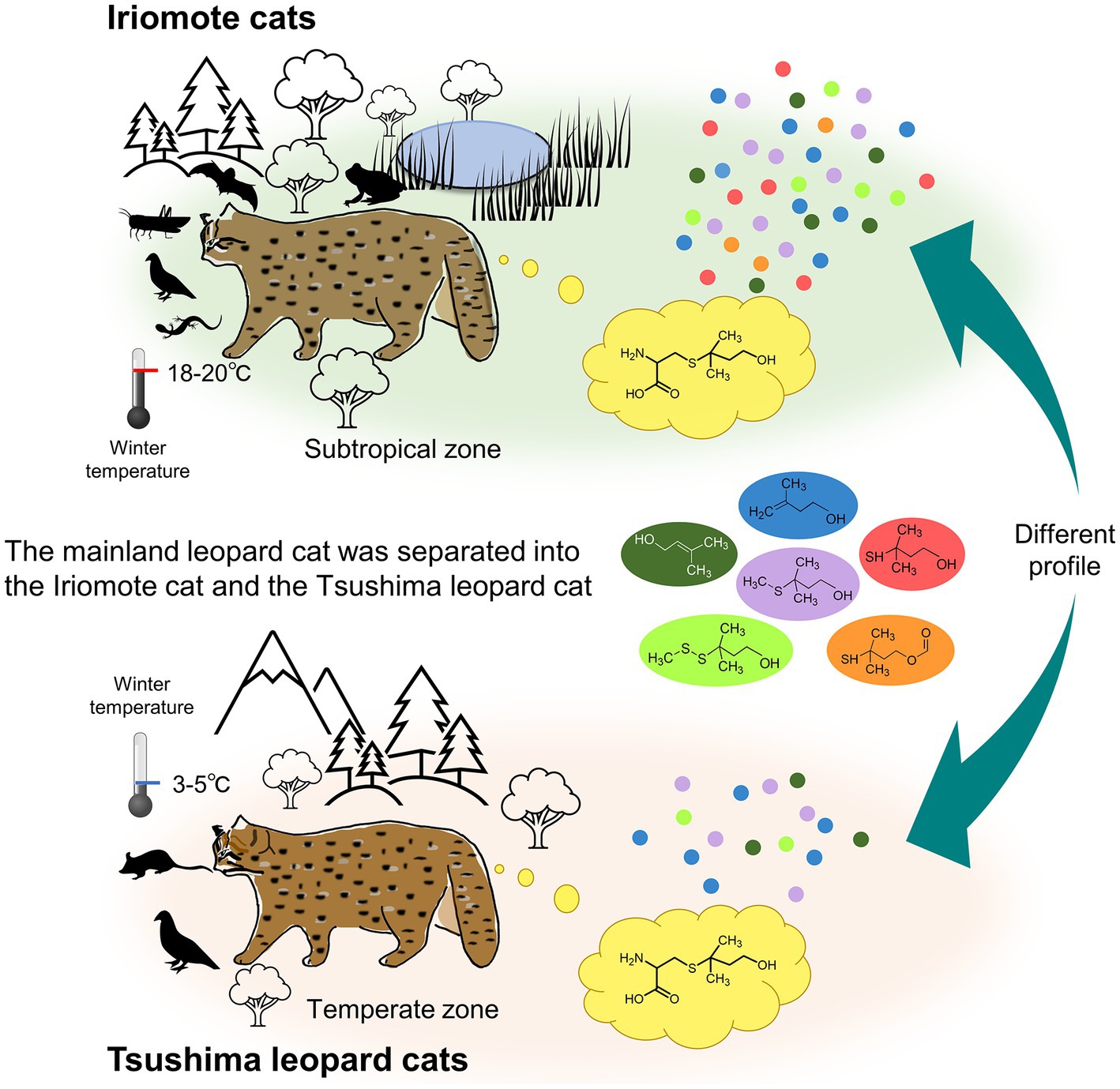
Figure 5. An outline of the present study showing differences between Iriomote cats and Tsushima leopard cats. Since climates of distribution areas of Iriomote cats and Tsushima leopard cats are the subtropical and the temperate zones, respectively, the average lowest temperature of the sampling season in the winter is warmer in Iriomote-jima island than in Tsushima Islands. The difference would cause that Iriomote cats can consume a wider range of prey animals, even in the winter season, in contrast to Tsushima leopard cats, who live in areas with less available prey. A major finding of this study was that sulfur-containing volatile compounds, MMB and its structural analogs, were emitted at higher levels from Iriomote cat urine than Tsushima leopard cat urine, while urinary excretion levels of felinine, a precursor of the volatile compounds, were not different between the subspecies. The variation in the emission levels might be attributed to that metabolic pathways are differently regulated between Iriomote cats and Tsushima leopard cats to adapt to different environments.
The urinary odor of both subspecies was similar to that of domestic cats by human olfaction, but we felt a stronger smell in Iriomote cat urine than in Tsushima leopard cat urine. Thus, we tested whether contents of characteristic odorants such as MMB and its structural analogs (Figure 2A) were higher in Iriomote cats than in Tsushima leopard cats. We found a substantial difference in urinary emission levels of sulfur-containing volatile organic compounds between Iriomote cats and Tsushima leopard cats. Our analysis showed that MMB, MMBF, 3-methyl-3-methylthio-1-butanol, and 3-methyl-3-(2-methyldisulfanyl)-1-butanol in the urinary headspace were much higher in male Iriomote cats than in male Tsushima leopard cats. These results suggest that differences in emission levels of MMB and MMBF contribute to the difference in urinary odor between these subspecies.
End-products of the felinine biosynthetic pathway are MMB and MMBF. Thus, larger emission of MMB in Iriomote cats than in Tsushima leopard cats would indicate that the pathway is upregulated in Iriomote cats as compared to Tsushima leopard cats. The difference may be related to the prey intakes and habitat environment of the two subspecies, which were observed during the winter season when the subjects were captured on each island. Iriomote cats have a more diverse diet, including mammals, birds, insects, frogs, reptiles (Sakaguchi and Ono, 1994; Díaz-Sacco and Izawa, 2013; Nakanishi and Izawa, 2016), while Tsushima leopard cats mainly feed on small mammals, birds, and sometimes insects (Tatara and Doi, 1994). The average lowest temperature of Iriomote-jima Island during December and January (18.3–19.5°C) is warmer than that of Tsushima Islands (2.5–4.9°C). These suggest that Iriomote cats may have a greater potential to consume a wider range of prey animals, even in the winter season, in contrast to Tsushima leopard cats, who live in areas with less available prey. A previous study suggested that MBG is produced by a conjugation reaction between glutathione and isopentenyl diphosphate, which is an end-product of the mevalonate pathway for cholesterol biosynthesis (Rutherfurd et al., 2002). This indicates that felinine production downregulates cholesterol biosynthesis, as the in vivo synthesis of cholesterol is affected by cholesterol intake, resulting that Tsushima leopard cats may use more isopentenyl diphosphate for the cholesterol biosynthesis rather than felinine production in winter season as compared to Iriomote cats. We suggest that the different levels of MMB production between the two subspecies may be attributable to differences in prey intake during the winter season.
It is possibility that volatile compounds generated in the urine may be affected by the bacterial contamination from environments carried out the sampling and the laboratory conditions, but we suggest that the major cause of different MMB levels between the two subspecies is variation of their metabolic pathway for the MMB production rather than the contamination. Although this study did not carry out bacteriological examinations of their urine samples, the samples were obtained from their bladders by direct manual pressures and were stored at −80°C until analysis, which would minimize the bacterial contamination and action for the production of urinary volatile compounds before and during the volatile analysis. Since this study showed the marked trend that MMB is much higher in Iriomote cats than Tsushima leopard cats, suggesting that the subspecies difference rather than urinary conditions with or without bacterial contamination are important to interpret the results.
In this study, we evaluated excretion levels of felinine and its related compounds and urinary proteins as these urinary concentrations without normalizing using urinary creatinine concentrations, which is a common veterinarian practice to evaluate proteinuria to diagnose renal diseases in domestic cats (Adams et al., 1992). Our analysis indicated that there were considerable variations in urinary creatinine concentrations between individuals of both subspecies (Supplementary Table S1). As obligate carnivores consume prey animals containing creatine and creatinine, these would be excreted in the urine after absorption of these compounds. A previous study reported that a lack of food intake results in a decrease in daily excretion of urinary creatinine in domestic cats (Hendriks et al., 1999). These suggest that differences in prey consumption and in the duration in the box-traps would be the impact on urinary creatinine concentration in the leopard cats. In conclusion, urinary creatinine excretion may be not suitable for normalizing in the wild animals especially obligate carnivores such as Felidae species. However, since our study concentrated on understanding scent communications in the wildcats, we think that direct evaluation using their urinary concentrations would be important for territorial animals.
Considering the excretion level of felinine, an MMB precursor, was not different between the two subspecies, other possibility to explain the variation in the MMB emission level is that most of the felinine is excreted in Tsushima leopard cats without decomposing into MMB. A previous study suggested that C-S lyase degrades felinine to MMB in domestic cats (Starkenmann et al., 2015). We suggest that the urinary activity of C-S lyase might not be sufficient for MMB production in the Tsushima leopard cat. Further studies to identify enzymes involved in MMB production from urine in each subspecies are necessary to understand MMB production and differences among subspecies.
In addition to physiological factors of cats for the breakdown of felinine to MMB, the outer microenvironment and ecology in their habitats may be also involved in the MMB production in these subspecies. There is a possibility for external degradation of felinine in the urine marked in the environment by enzymes not only of cats but also of particular environmental bacteria (Rutherfurd et al., 2007; Starkenmann et al., 2015), which is possibly another pathway to utilize the felinine to convey signals associated with territorial marking or special recognition. Since chemical communication is a coordinated event of species and environment (Wyatt, 2014), specific microflora and microclimate in the urinary marked area may be also caused for variations in amounts and duration of MMB emission, which is essentially important to be the scent signal in both intra- and inter-species. In this study, for the collection of urine samples from many individuals of the endangered wildcats, we captured them using live traps and carried out direct manual pressure of their bladders, although these procedures caused for their stresses and blood contamination to urine from the epithelial rupture of bladders. Therefore, our next challenge will be metabolome and proteome analyses of urine samples collected from environmental scent marks. Such studies will provide useful information to understand scent signals under normal conditions in the leopard cats. Since the two endangered leopard cats live in different climatic conditions, other compounds than MMB may be required to improve the persistent and longevity of MMB in the scent marks in each species. Although urine samples collected from their territories would contaminate several compounds derived from environments, our findings in this study help us to distinguish urinary compounds of wildcats and others such as urinary compounds of other mammals and plant metabolites.
The emission of MMB and its structural analogs are likely used for chemical communication in these small leopard cats, particularly in Iriomote cats with high MMB emission levels. Previous studies have reported MMB emissions from the urine of domestic cats, bobcats (Lynx rufus), and leopards (Panthera pardus pardus) (Mattina et al., 1991; Apps et al., 2014). In addition, one study found that the MMB precursor felinine was excreted in the urine of ocelots (Leopardus pardalis) and Indian leopard cats (Prionailurus bengalensis) (Hendriks et al., 1995), suggesting that their urine might also emit MMB. Because these felids are the only known mammals to emit MMB or its structural analogs from excretions, these compounds seem to be felid-specific. In a previous study, we showed that domestic cats can distinguish between excretions with and without MMB by olfaction (Miyazaki et al., 2018). In field trials using a dispenser releasing MMB, the African wildcat (Felis lybica) and non-felids such as the African civet (Civettictis civetta), the small spotted genet (Genetta genetta), and the slender mongoose (Galerella sanguinea) exhibited scent marking with MMB (Apps et al., 2017). Considering that Iriomote cats and Tsushima leopard cats are the only species on each island using scent marks that emit MMB and its structural analogs, except for domestic cats, we propose that these compounds play a role as scent signals for intra- and interspecies communication in the two subspecies. Because the levels of both MMB and MMBF were higher in males than in females in Iriomote cats, these compounds might be also used for sex recognition.
The chemical profile of felinine and its related compounds in leopard cats did not match that of domestic cats. In domestic cats, felinine has the highest excretion level among felinine-related compounds (Hendriks et al., 2004, 2008). Felinine excretion level is much higher in intact males than in females, however, the present study showed that there was no sex difference in felinine and cauxin excretions. This is unexpected result because felinine and its synthetic enzyme, cauxin, is regulated by testosterone in domestic cats (Hendriks et al., 2008). Morphological observation of the captured leopard cats confirmed testicular descent in male individuals, indicating that they were intact males fully developed sex hormone levels. We suggest that the regulation for the felinine production is different between domestic cats and the leopard cats.
However, in the case of the two leopard cat subspecies, there were no significant differences in excretion levels between felinine and MBCG and/or N-acetylfelinine because the excretion levels of the latter were higher in the two leopard cats than in domestic cats. MBCG is converted into urinary felinine in the renal tubules through the catalysis of cauxin secreted from the proximal tubular epithelial cells (Miyazaki et al., 2006). Felinine produced in the brush borders via renal dipeptidase-dependent hydrolysis of MBCG is absorbed into the proximal tubular epithelial cells and then secreted into the urine after N-acetylation of felinine. The difference in the ratio of felinine and its related compounds between the two leopard cats and domestic cats might be due to differences of catalytic activities and expression levels of cauxin, renal dipeptidase, or N-acetyltransferase.
In conclusion, we demonstrate that chemical analyses of animal excretions and secretions contribute to our understanding of species-specific chemical ecology of endangered species. Because both subspecies are officially classified as the mainland leopard cat (Prionailurus bengalensis), we assumed that the metabolic pathways for biosynthesis of these volatile organic compounds were regulated in the same level under the similar genetic backgrounds (Masuda and Yoshida, 1995). However, our data suggest that the pathway of each subspecies has been regulated separately as part of their adaptation to different environments after the mainland leopard cat was separated into the Iriomote cat and the Tsushima leopard cat. While recent studies have attempted whole genome sequencing of endangered species for conservation purposes (vonHoldt et al., 2016; Árnason et al., 2018; Supple and Shapiro, 2018; Hu et al., 2020), genome analysis cannot be used to determine differences in the chemical profiles of urinary metabolites between two subspecies with similar genetic backgrounds. Our findings provide useful information for managing Japanese leopard cats with regard to nutrition, behavior, reproduction, and veterinary medicine, for example, evaluation of their physiological status and determining species and sexes of scent owners by analyzing urinary marks of unknown animals in the fields.
Data availability statement
The original contributions presented in the study are included in the article/Supplementary material, further inquiries can be directed to the corresponding author.
Ethics statement
The animal study was reviewed and approved by the Ministry of the Environment and the Agency for Cultural Affairs in Japan and the Animal Research Committee of Iwate University.
Author contributions
MI and MM conceived the study, designed the experiments, and supervised the project. NN, YE, and MI collected urine samples. SI, RU, AS, and MM analyzed the samples. SI, RU, and MM wrote the draft manuscript, which was edited by MI and NN. All authors read and approved the final manuscript.
Funding
This work was supported by JSPS under KAKENHI (Grant no. 22K19321) to MM. RU was supported by the Grant-in-Aid for JSPS Fellows.
Conflict of interest
The authors declare that the research was conducted in the absence of any commercial or financial relationships that could be construed as a potential conflict of interest.
Publisher’s note
All claims expressed in this article are solely those of the authors and do not necessarily represent those of their affiliated organizations, or those of the publisher, the editors and the reviewers. Any product that may be evaluated in this article, or claim that may be made by its manufacturer, is not guaranteed or endorsed by the publisher.
Supplementary material
The Supplementary material for this article can be found online at: https://www.frontiersin.org/articles/10.3389/fevo.2023.1194611/full#supplementary-material
References
Adams, L. G., Polzin, D. J., Osborne, C. A., and O'brien, T. D. (1992). Correlation of urine protein/creatinine ratio and twenty-four-hour urinary protein excretion in normal cats and cats with surgically induced chronic renal failure. J. Vet. Intern. Med. 6, 36–40. doi: 10.1111/j.1939-1676.1992.tb00983.x
Alan, P. J., Bustamante, M. R., Coloma, L. A., Consuegra, J. A., Fogden, M. P. L., Foster, P. N., et al. (2006). Widespread amphibian extinctions from epidemic disease driven by global warming. Nature 439, 161–167. doi: 10.1038/nature04246
Apfelbach, R., Blanchard, C. D., Blanchard, R. J., Hayes, R. A., and Mcgregor, I. S. (2005). The effects of predator odors in mammalian prey species. Neurosci. Biobehav. Rev. 29, 1123–1144. doi: 10.1016/j.neubiorev.2005.05.005
Apps, P. J., Claase, M., Yexley, B., and McNutt, J. W. (2017). Interspecific responses of wild African carnivores to odour of 3-mercapto-3-methylbutanol, a component of wildcat and leopard urine. J. Ethol. 35, 153–159. doi: 10.1007/s10164-016-0503-7
Apps, P., Mmualefe, L., Jordan, N. R., Golabek, K. A., and Mcnutt, J. W. (2014). The “tomcat compound” 3-mercapto-3-methylbutanol occurs in the urine of free-ranging leopards but not in African lions or cheetahs. Biochem. Syst. Ecol. 53, 17–19. doi: 10.1016/j.bse.2013.12.013
Árnason, Ú., Lammers, F., Kumar, V., Nilsson, M. A., and Janke, A. (2018). Whole-genome sequencing of the blue whale and other rorquals finds signatures for introgressive gene flow. Sci. Adv. 4:eaap9873. doi: 10.1126/sciadv.aap9873
Burgin, C. J., Colella, J. P., Kahn, P. L., and Upham, N. S. (2018). How many species of mammals are there? J. Mammal. 99, 1–14. doi: 10.1093/jmammal/gyx147
Datta, S. P., and Harris, H. (1952). Urinary aminoacid patterns of some mammals. Ann. Eugenics 17, 107–116. doi: 10.1111/j.1469-1809.1952.tb02503.x
Díaz-Sacco, J. J., and Izawa, M. (2013). Road use by the Iriomote cat Prionailurus bengalensis iriomotensis on Iriomote-Jima Island in relation to its Herpetile prey. Mamm. Study 38, 73–80. doi: 10.3106/041.038.0202
Everatt, K. T., Kokes, R., and Lopez Pereira, C. (2019). Evidence of a further emerging threat to lion conservation; targeted poaching for body parts. Biodivers. Conserv. 28, 4099–4114. doi: 10.1007/s10531-019-01866-w
Futsuta, A., Hojo, W., Miyazaki, T., Yamashita, T., and Miyazaki, M. (2018). LC-MS/MS quantification of felinine metabolites in tissues, fluids, and excretions from the domestic cat (Felis catus). J. Chromatogr. B Analyt. Technol. Biomed. Life Sci. 1072, 94–99. doi: 10.1016/j.jchromb.2017.11.006
Hendriks, W. H., Harding, D. R., and Rutherfurd-Markwick, K. J. (2004). Isolation and characterisation of renal metabolites of gamma-glutamylfelinylglycine in the urine of the domestic cat (Felis catus). Comp. Biochem. Physiol. B Biochem. Mol. Biol. 139, 245–251. doi: 10.1016/j.cbpc.2004.07.007
Hendriks, W. H., Moughan, P. J., Tarttelin, M. F., and Woolhouse, A. D. (1995). Felinine: a urinary amino acid of Felidae. Comp. Biochem. Physiol. B Biochem. Mol. Biol. 112, 581–588. doi: 10.1016/0305-0491(95)00130-1
Hendriks, W. H., Rutherfurd-Markwick, K. J., Weidgraaf, K., Ugarte, C., and Rogers, Q. R. (2008). Testosterone increases urinary free felinine, N-acetylfelinine and methylbutanolglutathione excretion in cats (Felis catus). Ther. Ber. 92, 53–62. doi: 10.1111/j.1439-0396.2007.00710.x
Hendriks, B. W., Wamberg, S., and Tarttelin, M. (1999). A metabolism cage for quantitative urine collection and accurate measurement of water balance in adult cats (Felis catus). J. Anim. Physiol. Anim. Nutr. 82, 94–105. doi: 10.1111/j.1439-0396.1999.00214.x
Hirata, M., Tateno, M., Sakuma, M., Nakanishi, N., Izawa, M., Asari, Y., et al. (2012). An in Iriomote cats Prionailurus bengalensis iriomotensis. J. Vet. Med. Sci. 74, 1531–1537. doi: 10.1292/jvms.12-0094
Hisano, M., and Newman, C. (2020). Adaptations to prey base in the hypercarnivorous leopard cat Prionailurus bengalensis. Ethol. Ecol. Evol. 32, 324–335. doi: 10.1080/03949370.2020.1711816
Hu, Y., Thapa, A., Fan, H., Ma, T., Wu, Q., Ma, S., et al. (2020). Genomic evidence for two phylogenetic species and long-term population bottlenecks in red pandas. Sci. Adv. 6:eaax5751. doi: 10.1126/sciadv.aax5751
Hurst, J. L., and Beynon, R. J. (2004). Scent wars: the chemobiology of competitive signalling in mice. Bioessays 26, 1288–1298. doi: 10.1002/bies.20147
IUCN (2022). The IUCN Red List of Threatened Species. Version 2022-2 [Online]. Available: https://www.iucnredlist.org/en (Accessed 22 February 2023).
Izawa, M., Doi, T., Nakanishi, N., and Teranishi, A. (2009). Ecology and conservation of two endangered subspecies of the leopard cat (Prionailurus bengalensis) on Japanese islands. Biol. Conserv. 142, 1884–1890. doi: 10.1016/j.biocon.2009.05.005
Izawa, M., Sakaguchi, N., and Doi, T. (2000). For the Iriomote Felis iriomotensis. Tropics 10, 79–85. doi: 10.3759/tropics.10.79
Kitchener, A. C., Breitenmoser-Würsten, C., Eizirik, E., Gentry, A., Werdelin, L., Wilting, A., et al. (2017). A revised taxonomy of the Felidae. The final report of the Cat Classification Task Force of the IUCN/SSC Cat Specialist Group. Cat News Special Issue 11:80.
Masuda, R., and Yoshida, M. C. (1995). Two Japanese wildcats, the Tsushima cat and the Iriomote cat, show the same mitochondrial DNA lineage as the leopard cat Felis bengalensis. Zoolog Sci 12, 655–659. doi: 10.2108/zsj.12.655
Mattina, M. J., Pignatello, J. J., and Swihart, R. K. (1991). Identification of volatile components of bobcat (Lynx rufus) urine. J Chem Ecol 17, 451–462. doi: 10.1007/BF00994344
Mclean, L., Hurst, J. L., Gaskell, C. J., Lewis, J. C., and Beynon, R. J. (2007). Characterization of cauxin in the urine of domestic and big cats. J Chem Ecol 33, 1997–2009. doi: 10.1007/s10886-007-9354-6
Miyazaki, M., Kamiie, K., Soeta, S., Taira, H., and Yamashita, T. (2003). Molecular cloning and characterization of a novel carboxylesterase-like protein that is physiologically present at high concentrations in the urine of domestic cats (Felis catus). Biochem J 370, 101–110. doi: 10.1042/bj20021446
Miyazaki, M., Miyazaki, T., Nishimura, T., Hojo, W., and Yamashita, T. (2018). Basis of species, sex, and individual recognition using feces in the domestic cat. J. Chem. Ecol. 44, 364–373. doi: 10.1007/s10886-018-0951-3
Miyazaki, M., Nishimura, T., Hojo, W., Miyazaki, T., Laine, R. A., and Yamashita, T. (2017). Potential use of domestic cat (Felis catus) urinary extracts for manipulating the behavior of free-roaming cats and wild small felids. Appl. Anim. Behav. Sci. 196, 52–60. doi: 10.1016/j.applanim.2017.07.003
Miyazaki, M., Yamashita, T., Hosokawa, M., Taira, H., and Suzuki, A. (2006). Species-, sex-, and age-dependent urinary excretion of cauxin, a mammalian carboxylesterase. Comp. Biochem. Physiol. B Biochem. Mol. Biol. 145, 270–277. doi: 10.1016/j.cbpb.2006.05.015
Miyazaki, M., Yamashita, T., Suzuki, Y., Saito, Y., Soeta, S., Taira, H., et al. (2006b). A major urinary protein of the domestic cat regulates the production of felinine, a putative pheromone precursor. Chem Biol 13, 1071–1079. doi: 10.1016/j.chembiol.2006.08.013
Miyazaki, M., Yamashita, T., Taira, H., and Suzuki, A. (2008). The biological function of cauxin, a major urinary protein of the domestic cat (Felis catus). Springer. doi: 10.1007/978-0-387-73945-8_4
Mora, C., Tittensor, D. P., Adl, S., Simpson, A. G. B., and Worm, B. (2011). How many species are there on earth and in the ocean? PLoS Biol 9:e1001127. doi: 10.1371/journal.pbio.1001127
Nakanishi, N., and Izawa, M. (2016). Importance of frogs in the diet of the Iriomote cat based on stomach content analysis. Mamm. Res. 61, 35–44. doi: 10.1007/s13364-015-0246-9
Nelson, R. (2002). Editorial: stress hyperglycemia and diabetes mellitus in cats. J. Vet. Intern. Med. 16, 121–122. doi: 10.1111/j.1939-1676.2002.tb02342.x
Newbold, T., Hudson, L. N., Hill, S. L. L., Contu, S., Lysenko, I., Senior, R. A., et al. (2015). Global effects of land use on local terrestrial biodiversity. Nature 520, 45–50. doi: 10.1038/nature14324
Nishimura, Y., Goto, Y., Yoneda, K., Endo, Y., Mizuno, T., Hamachi, M., et al. (1999). Interspecies transmission of feline immunodeficiency virus from the domestic cat to the Tsushima cat (Felis bengalensis euptilura) in the wild. J. Virol. 73, 7916–7921. doi: 10.1128/JVI.73.9.7916-7921.1999
Patel, R. P., Wutke, S., Lenz, D., Mukherjee, S., Ramakrishnan, U., Veron, G., et al. (2017). Genetic structure and phylogeography of the leopard cat (Prionailurus bengalensis) inferred from mitochondrial genomes. J Hered 108, 349–360. doi: 10.1093/jhered/esx017
Rutherfurd, S. M., Kitson, T. M., Woolhouse, A. D., Mcgrath, M. C., and Hendriks, W. H. (2007). Felinine stability in the presence of selected urine compounds. Amino Acids 32, 235–242. doi: 10.1007/s00726-006-0369-z
Rutherfurd, K. J., Rutherfurd, S. M., Moughan, P. J., and Hendriks, W. H. (2002). Isolation and characterization of a felinine-containing peptide from the blood of the domestic cat (Felis catus). J Biol Chem 277, 114–119. doi: 10.1074/jbc.M107728200
Sakaguchi, N., and Ono, Y. (1994). Seasonal change in the food habits of the Iriomote. Ecol. Res. 9, 167–174. doi: 10.1007/BF02347492
Starkenmann, C., Niclass, Y., Cayeux, I., Brauchli, R., and Gagnon, A. (2015). Odorant volatile sulfur compounds in cat urine: occurrence of 3, 7dimethyloct3sulfanyl1ol and its cysteine conjugate precursor. Flavour Fragr. J. 30, 91–100. doi: 10.1002/ffj.3216
Supple, M. A., and Shapiro, B. (2018). Conservation of biodiversity in the genomics era. Genome Biol. 19:131. doi: 10.1186/s13059-018-1520-3
Tatara, M., and Doi, T. (1994). Comparative analyses on food habits of Japanese marten, Siberian weasel and leopard cat in the Tsushima islands, Japan. Ecol. Res. 9, 99–107. doi: 10.1007/BF02347247
vonHoldt, B. M., Cahill, J. A., Fan, Z., Gronau, I., Robinson, J., Pollinger, J. P., et al. (2016). Whole-genome sequence analysis shows that two endemic species of north American wolf are admixtures of the coyote and gray wolf. Sci. Adv. 2:e1501714. doi: 10.1126/sciadv.1501714
Watanabe, S. (2012). “Ecological Flexibility of the Top Predator in an Island Ecosystem - Food Habit of the Iriomote Cat,” in Diversity of Ecosystems, ed. A. Mahamane . (Rijeka: IntechOpen), Ch. 20 465–485. doi: 10.5772/38368
Westall, R. G. (1953). The amino acids and other ampholytes of urine. 2. The isolation of a new sulphur-containing amino acid from cat urine. Biochem J 55, 244–248. doi: 10.1042/bj0550244
Keywords: endangered species, Iriomote cat, Tsushima leopard cat, volatile organic compounds, 3-mercapto-3-methyl-1-butanol, felinine, carboxylesterase, urine
Citation: Ichizawa S, Uenoyama R, Nakanishi N, Endo Y, Suka A, Izawa M and Miyazaki M (2023) A comparative profile of urinary scent signals of two endangered Japanese populations of leopard cat. Front. Ecol. Evol. 11:1194611. doi: 10.3389/fevo.2023.1194611
Edited by:
Pavel Stopka, Charles University, CzechiaReviewed by:
Mousumi Poddar Sarkar, University of Calcutta, IndiaDevaraj Sankarganesh, Vellore Institute of Technology, India
Copyright © 2023 Ichizawa, Uenoyama, Nakanishi, Endo, Suka, Izawa and Miyazaki. This is an open-access article distributed under the terms of the Creative Commons Attribution License (CC BY). The use, distribution or reproduction in other forums is permitted, provided the original author(s) and the copyright owner(s) are credited and that the original publication in this journal is cited, in accordance with accepted academic practice. No use, distribution or reproduction is permitted which does not comply with these terms.
*Correspondence: Masao Miyazaki, bW1hc2FvQGl3YXRlLXUuYWMuanA=