- 1State Key Laboratory for Conservation and Utilization of Agro-bioresources, Guangxi Key Laboratory of Forest Ecology and Conservation, College of Forestry, Guangxi University, Nanning, China
- 2Laboratoire sur les écosystèmes terrestres boréaux, Département des Sciences Fondamentales, Université du Québec à Chicoutimi, Chicoutimi, QC, Canada
- 3Institut de recherche sur les forêts (IRF), Université du Québec en Abitibi-Témiscamingue, Rouyn-Noranda, QC, Canada
- 4Department of Silviculture and Genetics, Forest Research Institute, Raszyn, Poland
- 5Department of Biology, University of Quebec in Outaouais, Ripon, QC, Canada
- 6Key Laboratory of Conservation Biology for Endangered Wildlife of the Ministry of Education, College of Life Sciences, Zhejiang University, Hangzhou, China
- 7Department of Natural Sciences, University of Quebec in Outaouais Ripon, Gatineau, QC, Canada
Global changes affect the growing conditions of terrestrial ecosystems, causing a mismatch between plant phenology and local climates in Northern regions. Due to their long lifespan and irregular regeneration periods, trees cannot respond quickly enough to climate variability through long-term genetic adaptation. In this study, we explored the phenological plasticity and genetic variation among populations of bud burst in sugar maple (Acer saccharum Marsh.) seedlings from 30 Canadian provenances with contrasting climates planted in two common gardens near and at the northern limit of the species’ range. We tested the hypothesis that phenotypic plasticity and genetic variation among populations affect bud phenology. We expect that phenotypic plasticity is more important in regulating bud phenology due to the high variability in short-term weather events characterizing this part of North America. Bud development and leafing occurred in April–May, with complete bud burst lasting between 21 and 29 days. On average, bud swelling differed by 12 days between common gardens. Both factors site (common gardens) and provenance significantly affected bud burst, demonstrating phenological plasticity and genetic variation of sugar maple, respectively. A significant interaction between site and provenance was also found. Overall, the site (11.8–90.3%) contributed more than provenance (0–3.1%) to the variance in timings of bud burst, indicating a dominant role of plasticity in regulating spring phenology. Our study demonstrated the concurring effects of genetic variation and phenological plasticity of sugar maple and revealed the dominant role of the latter factor. The high plasticity observed in sugar maple has a crucial role in the phenological adaptation of maple and the survival of its local populations in a context of changing climate.
Introduction
Climate change is altering the growing conditions of plants in terrestrial ecosystems, and mainly tree growth at mid and high latitudes. In order to survive under such a rapid change, plants can either track their usual conditions through migration (Chen et al., 2011) or alter their growth strategies through genetic variation or plasticity to match novel environmental conditions (Aitken et al., 2008). However, given their long lifespan, slow regeneration cycles, the presence of geographical barriers (Scheller and Mladenoff, 2008), and the rapid rates of climate change (Gray and Hamann, 2013), trees could be unable to match the new environmental conditions. Under such a context, the ability to cope locally through plasticity may be a critical factor for the long-term survival of populations.
Phenology describes the recurring sequence of biological events during the plant cycle, such as bud burst, flowering, and leaf senescence (Richardson et al., 2013; Liu et al., 2017). Within this sequence, the timings of bud burst in spring and the beginning of the growing season have received a lot of attention given the close relationship with carbon sequestration and growth. An earlier bud burst is related to a longer growing season (Leinonen and Hanninen, 2002), allowing plants to produce more carbohydrates to invest in growth and reserves. Previous studies have demonstrated that climate warming has strongly advanced bud phenology due to the earlier fulfilment of forcing, resulting in an increased carbon sequestration in trees (Bronson et al., 2009; Richardson et al., 2009). Understanding the adaptive potential of phenology to climate variations could help to predict the fate of species under climate change.
The genetic variation among populations is a long-term evolutionary process driven by natural selection. In a given location, the genotypes that are better suited to local growing conditions are able to survive and persist in the population. Thus, species with distributions spreading across wide and heterogeneous environments exhibit populations differentiated genetically. Numerous studies have shown the genetic variation regarding a number of functional traits, such as plant diameter (Gárate-Escamilla et al., 2019), height (Beaulieu et al., 2004), leaf area (Roybal and Butterfield, 2018), and bud phenology (Silvestro et al., 2019).
Phenotypic plasticity is the ability of a genotype to express different phenotypes (alternative reaction norms) within a range of environmental conditions (Nicotra et al., 2010). As an important component of fitness, phenotypic plasticity enables species to respond rapidly and more quickly than genotypic variation to environmental changes (Chevin et al., 2013; Franks et al., 2014). However, high phenotypic plasticity is expensive in terms of maintenance costs (Van Buskirk and Steiner, 2009), engendering developmental instability (Auld et al., 2010), and potential occurrence of maladaptation (Ghalambor et al., 2007).
Genetic variation and plasticity are two important strategies to cope with changing environments (Franks et al., 2014), and the relative contribution of these two processes during evolution is complex. For example, some studies have shown that genetic variation plays a dominant role in mediating the relationship between functional traits and environment (Leimu and Fischer, 2008). However, given that genetic changes occur too slowly, plasticity plays a dominant role in regulating plant response (Chevin et al., 2013). In fact, the relative contribution of genetic variation and plasticity also depends on the studied traits and environmental variability. Traits that are under strong environmental control, such as leaf and physiological traits, exhibit higher plasticity (Xu et al., 2015). Under stable local environments, well-adapted populations can maintain competitive fitness to survive if migration rates are sufficiently low (Chevin et al., 2013). However, in a context of rapidly changing conditions, which requires fast response mechanisms (Van Kleunen and Fischer, 2005), phenotypic plasticity can be favoured. The interaction between plasticity and genetic variation was also confirmed in recent studies, demonstrating the genetic basis of plasticity (Ren et al., 2021). Thus, an accurate estimation of the relative contribution of genetic variation and plasticity can quantify the ability of species to persist across broad distribution ranges, and predict the potential changes of this range under global warming.
Due to its wide distribution range, strong sensitivity to climate, and the economic importance for eastern North America, sugar maple (Acer saccharum Marsh.) has been regarded as a model to study the response of species to climate change (Putnam and Reich, 2017). Previous studies showed evidence of ecotypic differentiation of bud phenology, mainly regarding the minimum temperatures occurring in spring at the provenance (Guo et al., 2020). In this study, we explored the genetic variation among populations and plasticity of sugar maple on seedlings from 30 Canadian origins planted in two common gardens at the center and northern edge of the distribution of sugar maple. We tested the hypothesis that phenotypic plasticity and genetic variation among populations affect bud phenology. We also expect that phenotypic plasticity is more important in regulating bud phenology, due to the high variability in short-term weather events in this part of North America.
Materials and methods
Provenances, seed collections and common garden tests
A total of 30 natural stands of sugar maple located across the species’ range in Canada were used in this study (Figure 1). This area is included in the bioclimatic domains of deciduous and mixed forests of the northern temperate zone, which is dominated by both broadleaves and conifers, mainly sugar maple, red maple (Acer rubrum L.), yellow birch (Betula alleghaniensis Britt) and fir (Abies balsamea (L.) Mill.). The climate is continental, with cold winters and warm summers.
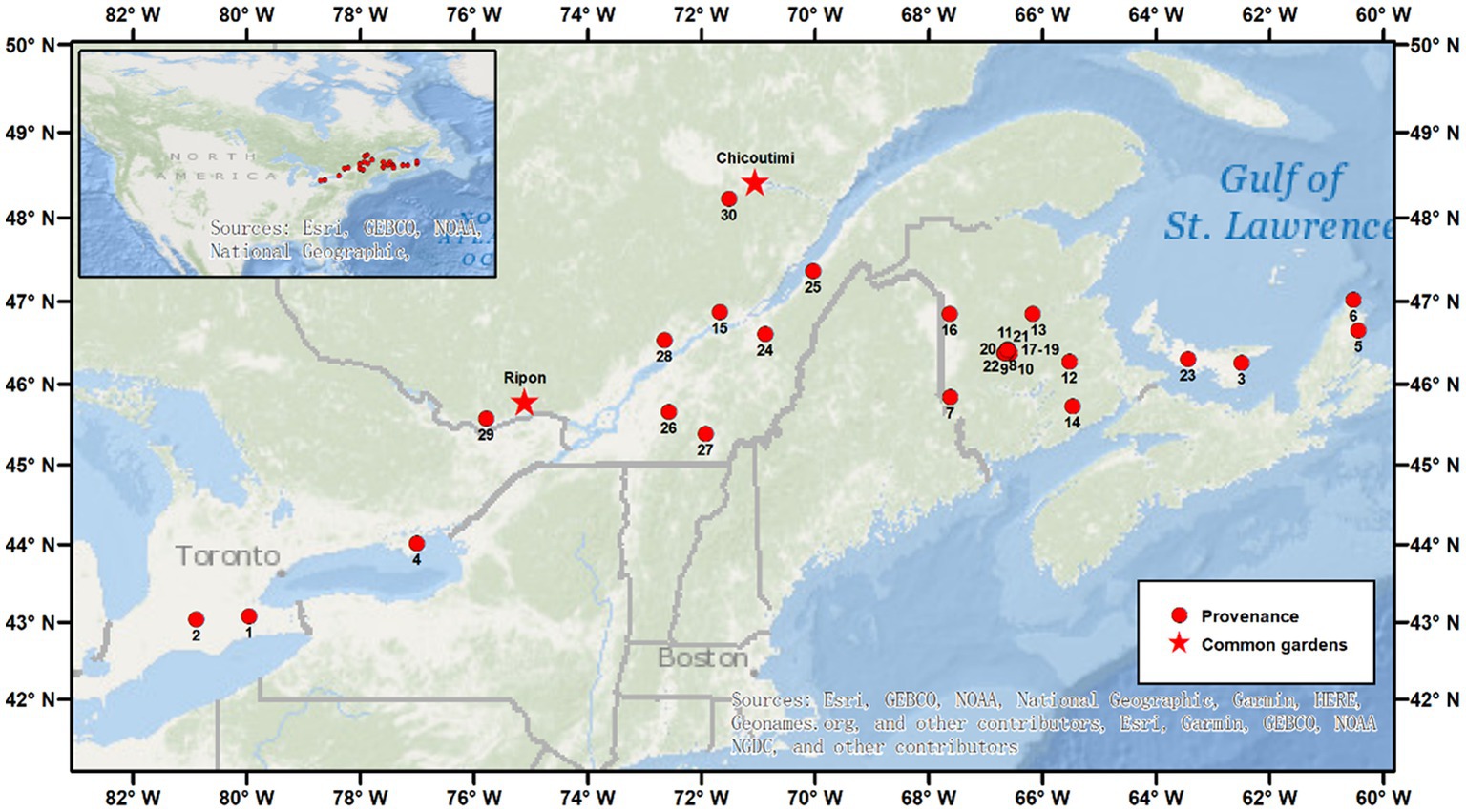
Figure 1. Location of the 30 provenances of sugar maple. The stars mark the locations of the two common gardens in Chicoutimi and Ripon.
Climate of the study sites
Minimum, maximum, mean temperature and precipitation during 1979–2020 were extracted according to the coordinates of all provenances and common gardens, from ERA5 dataset on Google Earth Engine (Copernicus Climate Change Service 2017; Gorelick et al., 2017). The 19 bioclimatic variables proposed by O’Donnell and Ignizio (2012) were extracted with the biovars function of the dismo package (Hijmans et al., 2017).
The mean annual temperature of the 30 provenances ranged between 2.7 and 8.4°C, recorded at provenance 30 and 1, respectively (Supplementary Table S1). With a minimum mean annual temperature of-10.9°C, and a maximum mean annual temperature of 16.6°C, provenance 30 was the coldest site. Provenance 1 was the warmest site, showing a minimum mean annual temperature of −4.7°C, and a maximum mean annual temperature of 22.0°C (Supplementary Table S1). The range in annual temperature between the coldest and warmest site was 5.7°C. The mean annual temperature in Ripon was 6.3°C, warmer than that in Chicoutimi (3.7°C). Annual precipitation ranged between 947 and 4,924 mm, increasing towards east.
Seed collection and plant material
The tested provenances were represented by two types of seeds collection (Supplementary Table S1), S – from one single mother tree (provenance 1–23; obtained from National Tree Seed Centre, Natural Resources Canada), or M – seed mixture from multiple mother trees (provenance 24–30 obtained from Ministère des Forêts, de la Faune et des Parcs). Given the highly heterogeneous topography of Mavis Mills, the natural selection effect was stronger than gene flow among populations, thus, the increasing sampling could be considered as discrete provenances.
Common garden tests
In spring 2019, one-year-old seedlings were planted in two common gardens located in Chicoutimi and Ripon (QC, Canada). This is a half-sib family design where plants from the same mother tree were planted in each common garden. Ripon and Chicoutimi are located at the center and northern edge of the distribution of sugar maple, respectively. With different growing conditions of the two common gardens, this experimental design could not only help to study the effects of genetic variations and plasticity of bud phenology, but also provide possible surviving mechanism of sugar maple under the northward shift scenario in the future. The trials were established according to a single tree plot design with 3 m × 3.5 m spacing. Between 6 and 10 seedlings per provenance were planted, a total of 217 and 242 seedlings in Chicoutimi and Ripon, respectively.
Phenological observations
From mid-April to mid-June in 2020 (a total of 9 weeks), we monitored the timing of bud burst of all seedlings twice per week. On average, two-year-old sugar maples have five buds. Given that the location of the bud on the sugar maple tree could affect phenology, we selected and observed the apical one, which is the acknowledged standard to avoid confounding factors for the successive analyses (Rosique-Esplugas et al., 2022). Eight phases of bud and leaf development of all seedlings were recorded according to Skinner and Parker (1994): (1) bud swell, with reddish scales and enlarging bud; (2) bud elongation, with a yellowish color between the scales; (3) green tip stage, with the tip and area between the scales light green but closed bud; (4) bud break, with loosened scales but barely visible expanding leaf tips; (5) extended bud break, with leaf bundle expanded beyond the scales but no separated leaves; (6) initial leaf emergence, with the leaves starting to expand perpendicularly to the base of the bud; (7) initial leaf expansion, with light green, small, and wrinkled leaves; and (8) full leaf expansion, with flattened and fully expanded leaves. The onset of each phenological phase was defined as the first day (DOY) when a given phase was observed in each individual. Given the low survival rate and low-quality data, plants originating from provenance of 2, 4, 8, 10 and 30 were excluded in the further analysis.
Statistical analyses
We used a bioclimatic index to interpret the climatic difference among provenances and two common gardens. A Principal Component Analysis (PCA) was performed based on the 19 bioclimatic variables to describe the annual trend, seasonality and extreme conditions across provenances and between common gardens. The contribution of each bioclimatic variable to the total variance explained by the principal components was determined.
Plasticity could be calculated at individual or population level. For each common garden, the average bud phenology of all individuals of the same provenance was calculated, and the difference in leaf development of each provenance between the two common gardens was used to present population-level plasticity. In order to show the general difference between the two common gardens of all provenances, the averaged bud phenology and standard deviation for each phase among all provenances were calculated.
According to previous studies, the effect of site (common garden) and provenance could be used to indicate plasticity and genetic variation among provenances, respectively. Thus, we applied an ANOVA to test the effects of site, provenance and their interaction on bud phenology of sugar maple. In order to test whether the seed collection type affects bud phenology, we also added this factor in the ANOVA analysis. One ANOVA was performed for each phenological phase.
Further, we tested the relative contribution of site, provenance, their interaction, and residuals to the variance for the different phenological phases using the non-parametric procedure PERmutational Multivariate Analysis Of Variance (PERMANOVA) (Anderson, 2017). Eight PERMANOVA were used for the phenological phases. This model was based on the adonis function, with metric Euclidean distance matrix (McArdle and Anderson, 2001) and 10,000 permutations. Consideration of the seed collection type in this model depends on the results of the previous ANOVA analysis. If the effect of seed collection type was not significant, this factor will be dropped in the PERMANOVA. All the statistics were performed in R (R Core Team, 2020) using the vegan packages (Oksanen et al., 2013).
Results
PCA results of bioclimate of the study sites
PCA extracted three main principal components (PC), explaining 44.4, 26.3, and 21.2% of the variability in bioclimatic parameters for provenance and common gardens for PC1, PC2, and PC3, respectively (Table 1). PC1 separated oceanic and continental climates, located on the left and right parts of the biplot, respectively (Figure 2A). PC2 separated locations characterized by different precipitation regimes. PC3 separated provenance by isothermality, i.e., the ratio between mean diurnal range and annual temperature range. Ripon and Chicoutimi, the two common gardens, were located in the upper parts of the biplot on the right side of PCA (Figure 2B). Compared to Ripon, Chicoutimi had a higher temperature seasonality (bio 4) and a higher annual temperature range (bio 7).
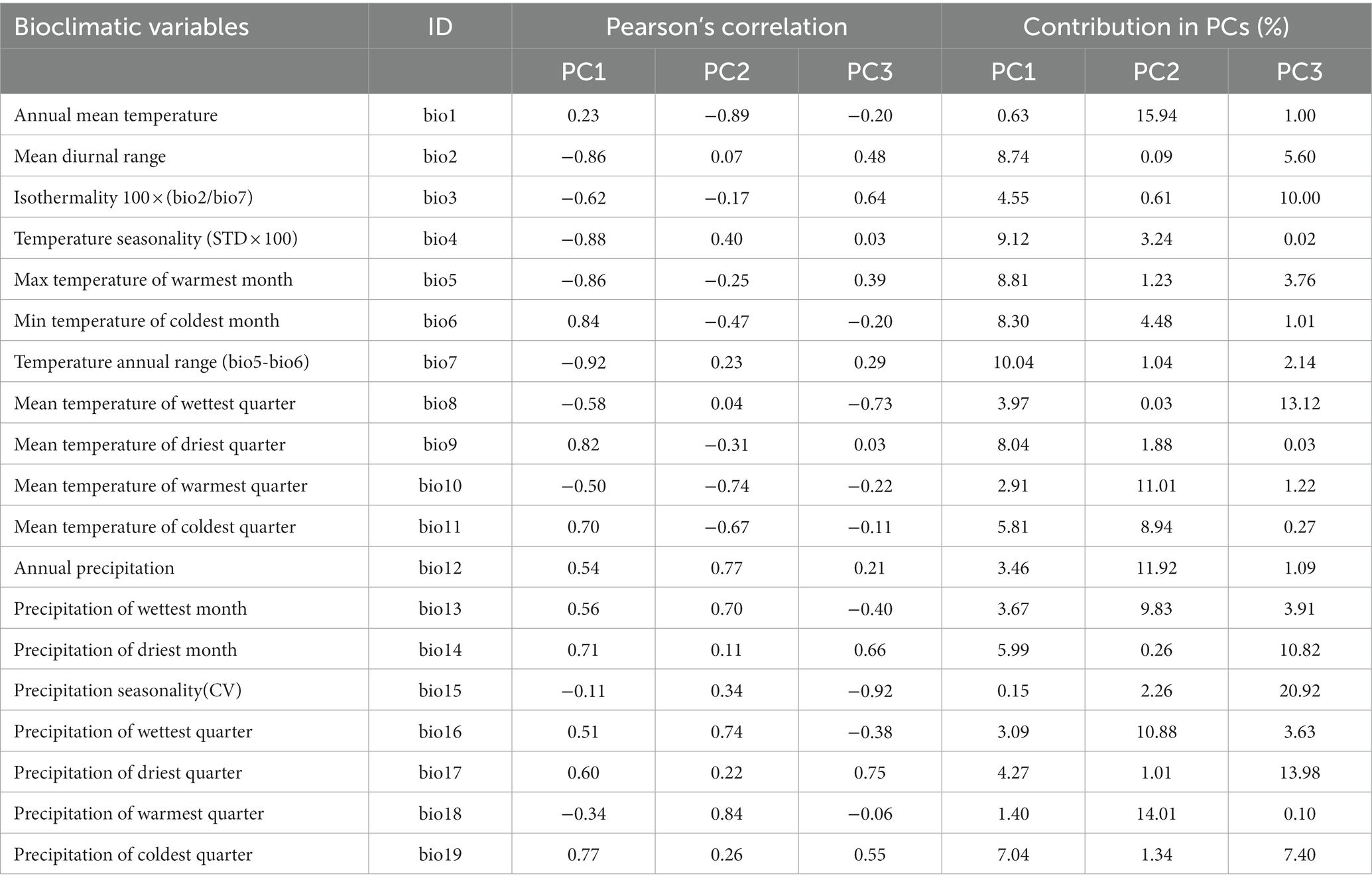
Table 1. Pearson’s correlation coefficient and contribution of bioclimatic variables to the three principal components.
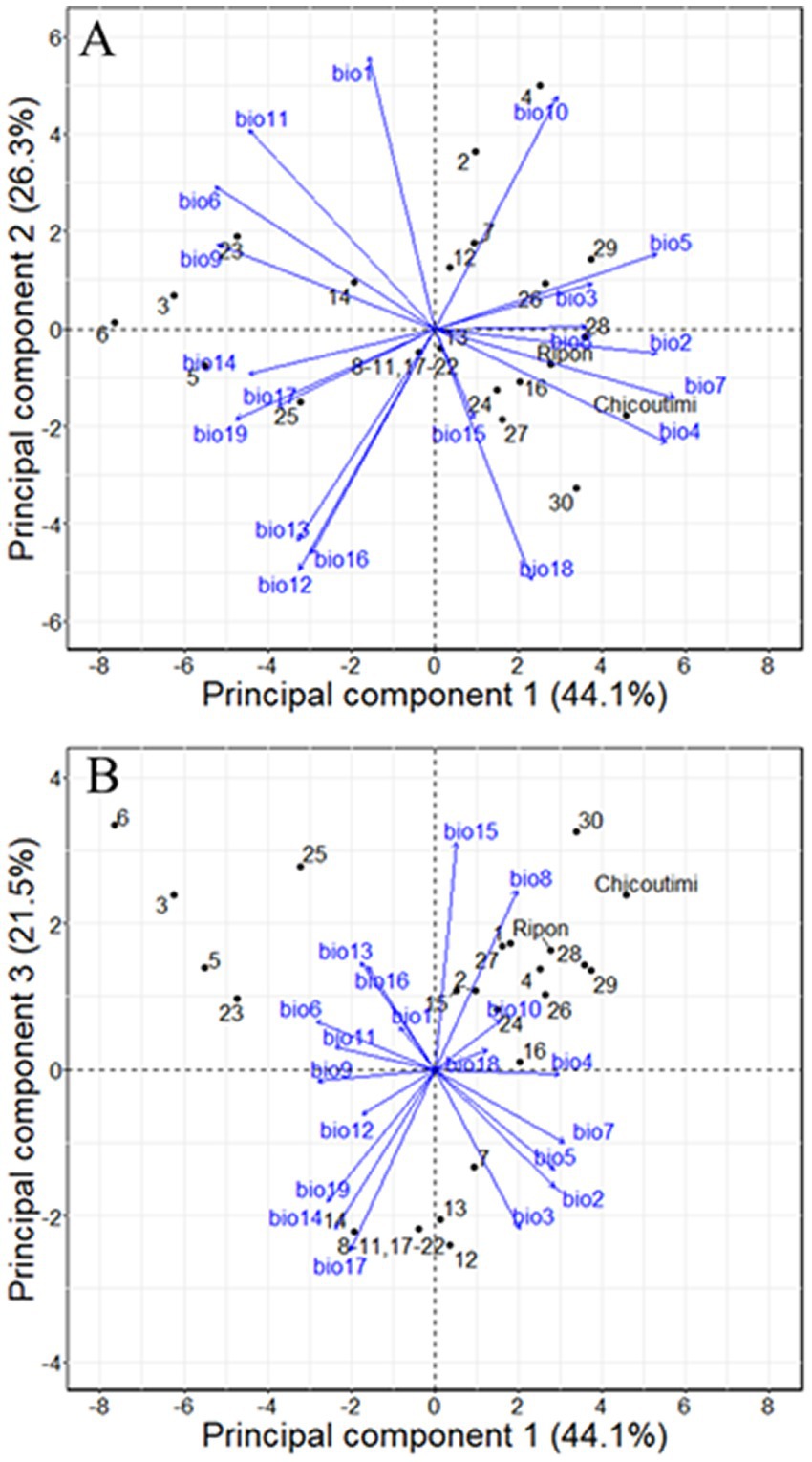
Figure 2. Principal component analysis of the climatic variability between provenance and common gardens. Bio1: annual mean temperature; bio 2: mean diurnal range; bio3: isothermality; bio4: temperature seasonality; bio5: max temperature of warmest month; bio6: min temperature of coldest month; bio7: annual temperature range; bio8: mean temperature of wettest quarter; bio9: mean temperature of driest quarter; bio10: mean temperature of warmest quarter; bio11: mean temperature of coldest quarter; bio12: annual precipitation; bio13: precipitation of wettest month; bio14: precipitation of driest month; bio15: precipitation seasonality; bio16: precipitation of wettest quarter; bio17: precipitation of driest quarter; bio18: precipitation of warmest quarter; bio19: precipitation of coldest quarter.
Bud and leaf phenology difference between two common gardens
Bud and leaf phenology occurred earlier in Ripon than Chicoutimi (Figure 3). On average, phase 1 started on DOY (day of the year) 121 in Ripon, 12 days earlier than Chicoutimi (DOY 133). The difference in bud phenology between the two sites decreased with the successive phases. Seedlings in Ripon showed phase 8 on DOY 150, only 4 days earlier than Chicoutimi (DOY 154). Overall, the period of leafing lasted 29 and 21 days in Ripon and Chicoutimi, respectively.
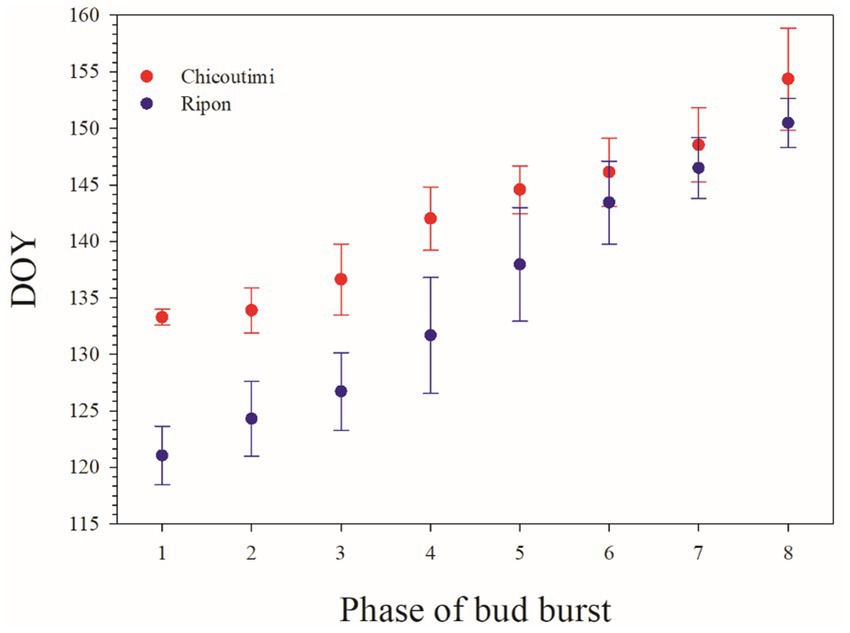
Figure 3. Occurrence of the different leafing phases of sugar maple in the two common gardens. Values are reported as average and standard deviation.
Phenological plasticity on population level
Provenance with the highest or lowest phenological plasticity varied among the eight developmental phases. For phases 1–5, provenance 22 showed the highest plasticity, with a difference of 9.1–12.8 days between the two common gardens. For phases 6–8, provenance 14 showed the greatest difference of 6.1–8.5 days between the two common gardens. For phases 1–4, provenance 1 showed the lower plasticity, with a difference of 3.2–5.8 days between the two common gardens. For phases 5–8, provenance 9 showed the least plasticity, with a difference of 1.1–2.5 days between the two common gardens.
Effects of plasticity and genetic variation
The effect of site (df = 1) and provenance (df = 23) was significant for all phases (Table 2). The interaction site×provenance (df = 24) was significant for phases 2, 6, 7, and 8 (p < 0.05), suggesting that the difference in bud phenology between the two common gardens changed as a function of provenance. The effect of seed collection type was not significant, indicating that the uneven seed collection was unable affect the results and was not considered in the successive analyses.
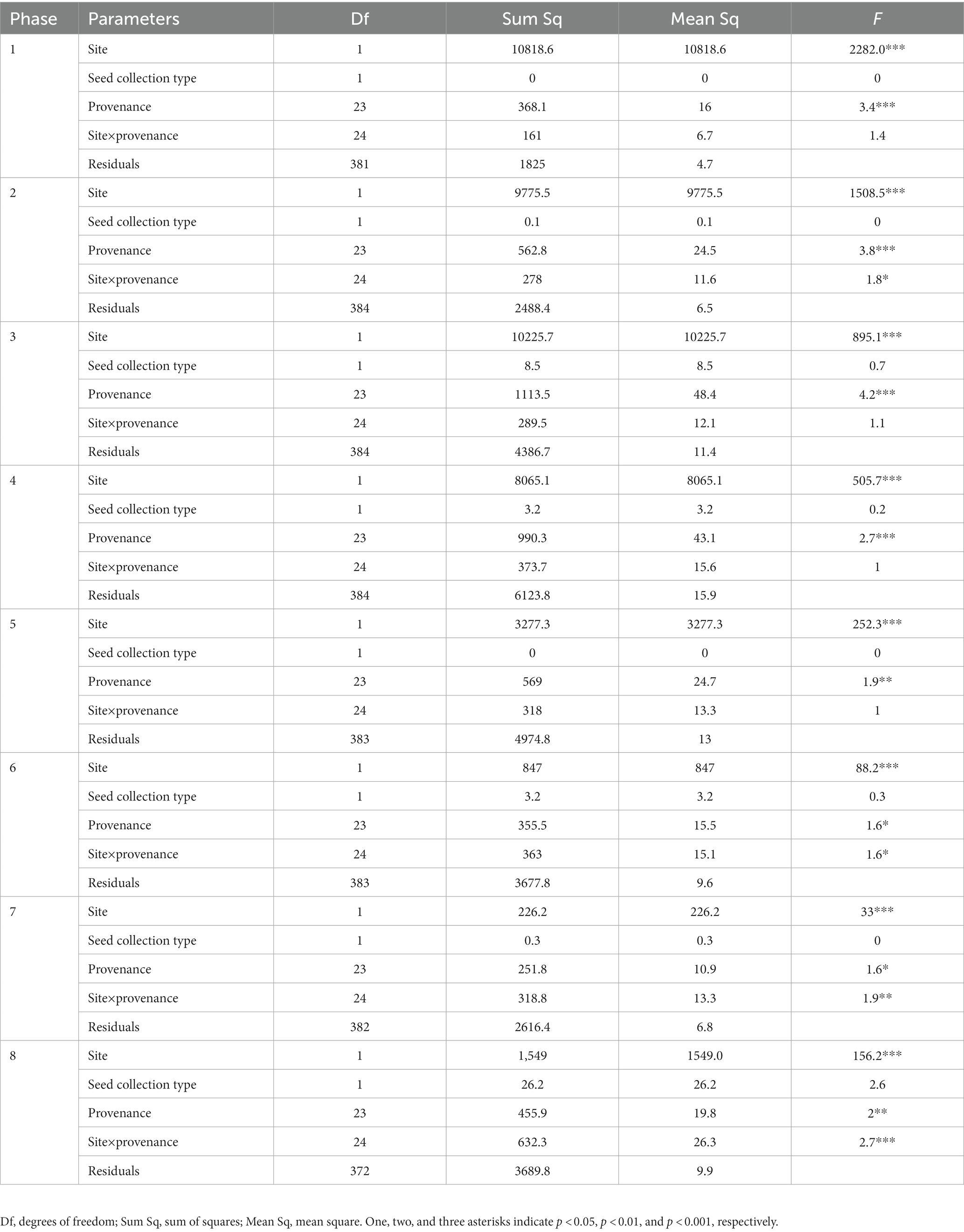
Table 2. Effect of site, seed collection type (single mother tree or multiple mother trees), provenance, site×provenance, and residuals on bud and leaf phenology in sugar maple seedlings.
Variance components of bud phenology
The factor site explained most variance of leaf development, between 11.8 and 90.3% (Figure 4). The variance of the factor site was highest at the beginning, decreasing with the successive phenological phases. Provenance contributed to a lower proportion of the variance of leaf development, between 0 and 3.1%. For all phases, the variance component of the interaction site×provenance was between 0.3 and 8.4%. The variance components of provenance and site×provenance both decreased along the leaf development. The residuals accounted for an increasing variance in bud phenology, from 8.3% for phase 1 to 81% for phase 7.
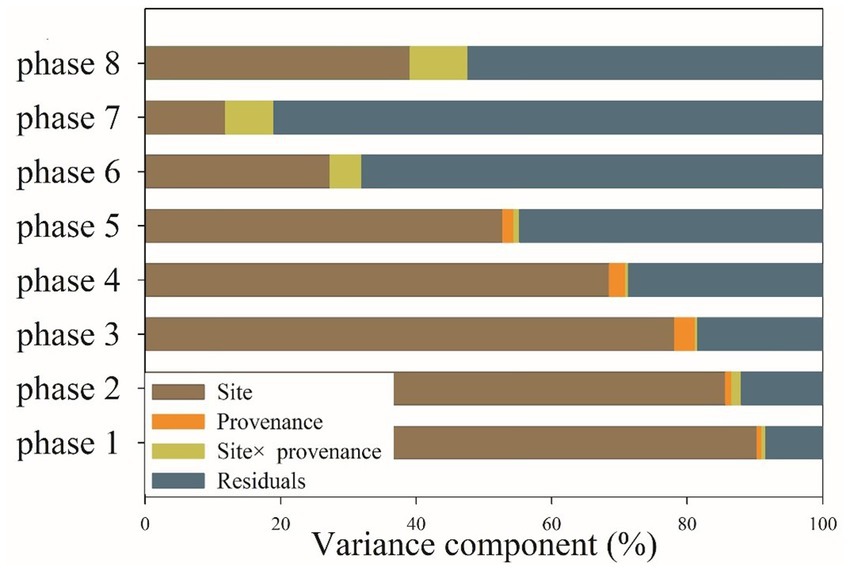
Figure 4. Relative contribution of the effects of site, provenance, interaction of site×provenance, and residuals to the variance of bud phenology in sugar maple.
Discussion
Plasticity vs genetic variation
In our study, both provenance and site had a significant influence on bud phenology, demonstrating that genetic variation and plasticity are concurring processes involved in the evolution of sugar maple. Sugar maple is distributed over a wide geographical area, at different latitudes and distances from the sea. Under these varying conditions, this species faces complex climate dynamics, involving different patterns of growing season length and frost risk (Guo et al., 2020). Thus, the genetic variation may be an important factor that enables a broad tolerance of the species to various environmental conditions, and likely contributes to the species’ wide latitudinal range in eastern North America.
Our study found a substantially higher contribution of plasticity to the phenological variation than genetic variation of sugar maple, which supports our hypothesis. Similar results were also observed in other species of boreal and temperate ecosystems (Baliuckas and Pliura, 2003; Vitasse et al., 2010). For example, the contribution of plasticity to bud burst phenology ranged from 55 to 86% in seven deciduous species in Europe, one order of magnitude higher than that of local adaptation (0.3–9%) (Vitasse et al., 2013). In another study combining experiments in situ and common garden, the genetic differentiation explained <28% of variance in the morphological and physiological traits of leaves of sessile oak and European beech, suggesting a minor effect of local adaptation on leaf functional traits (Bresson et al., 2011). In a common garden, environmental conditions also exerted the strongest effect on various functional traits of Phragmites australis, including shoot height, shoot density, node number per stem, leaf life span, flowering occurrence and date.
The dominant role of plasticity in regulating bud phenology could be explained by a number of reasons. Because of the long lifespan and slow and intermittent regeneration periods (masting years) of trees, the survival of individuals could rely on phenotypic plasticity rather than adaptation (Fox et al., 2019). As a sensitive indicator of climate change, the study of phenology allows the effects of extreme events on forest growth to be tracked. Previous studies demonstrated that, for reversible functional traits such as phenology and physiology, plasticity is an important driver of variation within-species (Xu et al., 2015). In effect, reversible traits are under strong environmental control, and plasticity could help plants to respond quickly to environmental cues, which is essential for plant survival. Moreover, under continental climatic conditions, sugar maple can experience a wide inter-annual variability in weather, principally for the temperature, one of the main limiting factors for bud phenology in spring (Guo et al., 2020). Individuals with high plasticity can respond to weather events quickly and lengthen the leafing period to improve the time window for carbon fixation.
The interaction between genetic variation and plasticity
The interaction between site and provenance at phenological phases 2, 7, and 8 indicated differences in phenological plasticity among sugar maple populations. Compared to the others, the initial and final phases are more sensitive to the environment, which in turn allows the interaction site×provenance to be detected. No clear geographic pattern of plasticity was observed for the eight phases of leaf development. At the beginning of leaf development, provenance 1, which is located at the southern latitude and inland, showed the lowest plasticity. This result contrasts with Vitasse et al. (2013), who found that the phenological plasticity of six temperate deciduous species was lower in populations originating from the higher elevations. The results of our study are consistent with those reported by Ren et al. (2021), who found that phenotypic plasticity in final shoot height and maximum biomass per shoot increased towards the higher latitudes. Plasticity correlates with climatic variability, and species experiencing a wider climatic fluctuation also exhibit higher plasticity. In general, the range of climatic variability increases with latitude. Therefore, plants from the higher latitudes exhibit a higher plasticity, which may help them to respond suitably to rapid changes in climate (Jason, 2003).
In this study, we observed a high contribution of residuals to the variance in bud phenology, which is in agreement with previous studies (Sole-Medina et al., 2020; Varsamis et al., 2018). This large heterogeneity in phenology unexplained by our factors may suggest a high variability among individuals, in addition to the potential effect of microsite conditions and sampling errors during field observations. An important effect of microsite on plant phenology seems unlikely, because the two common gardens are located in cropland areas, which produced crops in the past, and are therefore expected to be more homogeneous than natural sites. The wide variation in bud phenology within the same population may represent a diverse gene reservoir for the long-term survival of the species (Rousi and Heinonen, 2007). These various genotypes ensure a potential matching between some individuals of the populations and environmental conditions, thus allowing local persistence of the species.
Application under climate change
It has been predicted that the mid-latitudes of North America will have experienced warming up to 7°C at the end of the 21st century (Feng et al., 2014). In addition, under the ongoing global changes, climatic variability increases in magnitude, resulting in more frequent extreme weather events such as heat waves (Hegerl and Zwiers, 2011) or cooling (Wang et al., 2011). A previous study demonstrated that the recent advance of 13 flowering days under global warming has helped trees to improve their fitness by 40% (Anderson et al., 2012). However, recent studies also revealed that the advanced phenology seriously increases the risk of frost damage to the young developing tissues and leaves. Thus, the ability to respond quickly to environmental changes, matching the optimal moment of the year for flushing, and avoiding the negative consequences of early or late growth reactivations is essential for plants under climate change (Allevato et al., 2019).
Conclusion
Genetic variation and phenotypic plasticity act as concurring processes in the response of plants to environment, whose changes are critical for the survival of local populations. In this study, we explored the genetic variation and phenological plasticity of sugar maple seedlings from 30 Canadian origins planted in two common gardens near and at the northern boundary of the species range. Our study assessed the importance of plasticity and genetic variation and interpreted their role under different temporal and spatial scales, respectively. The high plasticity observed in sugar maple has a crucial role in the phenological adaptation of maple and the survival of its local populations in a context of changing climate. Accounting for both plasticity and the genetic variation within a species could increase the predictive accuracy of plant phenology to climate change.
Data availability statement
The raw data supporting the conclusions of this article will be made available by the authors, without undue reservation.
Author contributions
SR and JH: funding acquisition. SR, YS-G, and SD: acquisition of the seed materials and data collection. SR: designing the experiments. XG, VB, MK, and VM: data analysis. XG, SR, and JH: writing with contributions from all authors. All authors contributed to the article and approved the submitted version.
Funding
This work was funded by Ministère des Forêts, de la Faune et des Parcs du Québec, Natural Sciences and Engineering Research Council of Canada (Alliance Grants and Engage Grants), Fonds de Recherche Nature et Technologies Québec (Établissement de nouveaux chercheurs), Observatoire régional de recherche en forêt boréale, National Natural Science Foundation of China (32201543 and 41861124001), Natural Science Foundation of Guangxi Province (2021 AC19325), the International Collaborative Key Project of the CAS (GJHZ1752).
Acknowledgments
The authors thank P. Benoît, F. Gagnon and P. Ren for technical support, Y. Gobeil for permitting the study on his property, and A. Garside for checking the English text.
Conflict of interest
The authors declare that the research was conducted in the absence of any commercial or financial relationships that could be construed as a potential conflict of interest.
Publisher’s note
All claims expressed in this article are solely those of the authors and do not necessarily represent those of their affiliated organizations, or those of the publisher, the editors and the reviewers. Any product that may be evaluated in this article, or claim that may be made by its manufacturer, is not guaranteed or endorsed by the publisher.
Supplementary material
The Supplementary material for this article can be found online at: https://www.frontiersin.org/articles/10.3389/fevo.2023.1217871/full#supplementary-material
References
Aitken, S. N., Yeaman, S., Holliday, J. A., Wang, T., and Curtis-McLane, S. (2008). Adaptation, migration or extirpation: climate change outcomes for tree populations. Evol. Appl. 1, 95–111. doi: 10.1111/j.1752-4571.2007.00013.x
Allevato, E., Saulino, L., Cesarano, G., Chirico, G. B., D'Urso, G., Bolognesi, S. F., et al. (2019). Canopy damage by spring frost in European beech along the Apennines: effect of latitude, altitude and aspect. Remote Sens. Environ. 225, 431–440.
Anderson, J. T., Inouye, D. W., McKinney, A. M., Colautti, R. I., and Mitchell-Olds, T. (2012). Phenotypic plasticity and adaptive evolution contribute to advancing flowering phenology in response to climate change. Proc. Biol. Sci. 279, 3843–3852. doi: 10.1098/rspb.2012.1051
Anderson, M. J. (2017). “Permutational Multivariate Analysis of Variance (PERMANOVA)” in Wiley StatsRef: Statistics Reference Online. eds. N. Balakrishnan, T. Colton, B. Everitt, W. Piegorsch, F. Ruggeri, and J. L. Teugels https://doi.org/10.1002/9781118445112.stat07841
Auld, J. R., Agrawal, A. A., and Relyea, R. A. (2010). Re-evaluating the costs and limits of adaptive phenotypic plasticity. Proc. Biol. Sci. 277, 503–511. doi: 10.1098/rspb.2009.1355
Baliuckas, V., and Pliura, A. (2003). Genetic variation and phenotypic plasticity of Quercus robur populations and open-pollinated families in Lithuania. Scand. J. For. Res. 18, 305–319.
Beaulieu, J., Perron, M., and Bousquet, J. (2004). Multivariate patterns of adaptive genetic variation and seed source transfer in Picea mariana. Can. J. For. Res. 34, 531–545. doi: 10.1139/x03-224
Bresson, C. C., Vitasse, Y., Kremer, A., and andDelzon, S. (2011). To what extent is altitudinal variation of functional traits driven by genetic adaptation in European oak and beech? Tree Physiol. 31, 1164–1174. doi: 10.1093/treephys/tpr084
Bronson, D. R., Gower, S. T., Tanner, M., and Van Herk, I. (2009). Effect of ecosystem warming on boreal black spruce bud burst and shoot growth. Glob. Chang. Biol. 15, 1534–1543. doi: 10.1111/j.1365-2486.2009.01845.x
Chen, I.-C., Hill, J. K., Ohlemüller, R., Roy, D. B., and Thomas, C. D. (2011). Rapid range shifts of species associated with high levels of climate warming. Science 333, 1024–1026. doi: 10.1126/science.1206432
Chevin, L. M., Collins, S., and Lefèvre, F. (2013). Phenotypic plasticity and evolutionary demographic responses to climate change: taking theory out to the field. Funct. Ecol. 27, 967–979. doi: 10.1111/j.1365-2435.2012.02043.x
Feng, S., Hu, Q., Huang, W., Ho, C.-H., Li, R., and Tang, Z. (2014). Projected climate regime shift under future global warming from multi-model, multi-scenario CMIP5 simulations. Glob. Planet. Chang. 112, 41–52. doi: 10.1016/j.gloplacha.2013.11.002
Fox, R. J., Donelson, J. M., Schunter, C., Ravasi, T., and Gaitan-Espitia, J. D. (2019). Beyond buying time: the role of plasticity in phenotypic adaptation to rapid environmental change. Philos. Trans. R. Soc. B 374:20180174. doi: 10.1098/rstb.2018.0174
Franks, S. J., Weber, J. J., and Aitken, S. N. (2014). Evolutionary and plastic responses to climate change in terrestrial plant populations. Evol. Appl. 7, 123–139. doi: 10.1111/eva.12112
Gárate-Escamilla, H., Hampe, A., Vizcaíno-Palomar, N., Robson, T. M., and Benito, G. M. (2019). Range-wide variation in local adaptation and phenotypic plasticity of fitness-related traits in Fagus sylvatica and their implications under climate change. Glob. Ecol. Biogeogr. 28, 1336–1350. doi: 10.1111/geb.12936
Ghalambor, C. K., McKay, J. K., Carroll, S. P., and Reznick, D. N. (2007). Adaptive versus non-adaptive phenotypic plasticity and the potential for contemporary adaptation in new environments. Funct. Ecol. 21, 394–407. doi: 10.1111/j.1365-2435.2007.01283.x
Gorelick, N., Hancher, M., Dixon, M., Ilyushchenko, S., Thau, D., and Moore, R. (2017). Google earth engine: planetary-scale geospatial analysis for everyone. Remote Sens. Environ. 202, 18–27. doi: 10.1016/j.rse.2017.06.031
Gray, L. K., and Hamann, A. (2013). Tracking suitable habitat for tree populations under climate change in western North America. Clim. Chang. 117, 289–303. doi: 10.1007/s10584-012-0548-8
Guo, X., Khare, S., Silvestro, R., Huang, J., Sylvain, J.-D., Delagrange, S., et al. (2020). Minimum spring temperatures at the provenance origin drive leaf phenology in sugar maple populations. Tree Physiol. 40, 1639–1647. doi: 10.1093/treephys/tpaa096
Hegerl, G., and Zwiers, F. (2011). Use of models in detection and attribution of climate change. Wiley Interdiscip. Rev. Clim. Chang. 2, 570–591.
Hijmans, R. J., Phillips, S., Leathwick, J., Elith, J., and Hijmans, M. R. J. (2017). Package ‘dismo’. Circles 9, 1–68.
Jason, P. (2003). Climate tolerance and interspecific variation in geographic range size Proc. R. Soc. Lond., B.270475–B.270481. http://doi.org/10.1098/rspb.2002.2275
Leimu, R., and Fischer, M. (2008). A meta-analysis of local adaptation in plants. PLoS One 3:e4010. doi: 10.1371/journal.pone.0004010
Leinonen, I., and Hanninen, H. (2002). Adaptation of the timing of bud burst of Norway spruce to temperate and boreal climates. Silva Fenn. 36, 695–701.
Liu, Y., Miao, R., Chen, A., Miao, Y., Liu, Y., and Wu, X. (2017). Effects of nitrogen addition and mowing on reproductive phenology of three early-flowering forb species in a Tibetan alpine meadow. Ecol. Eng. 99, 119–125. doi: 10.1016/j.ecoleng.2016.11.033
McArdle, B. H., and Anderson, M. J. (2001). Fitting multivariate models to community data: A comment on distance-based redundancy analysis. Ecology 82, 290–297.
Nicotra, A. B., Atkin, O. K., Bonser, S. P., Davidson, A. M., Finnegan, E. J., Mathesius, U., et al. (2010). Plant phenotypic plasticity in a changing climate. Trends Plant Sci. 15, 684–692. doi: 10.1016/j.tplants.2010.09.008
O’Donnell, M. S., and Ignizio, D. A. (2012). Bioclimatic predictors for supporting ecological applications in the conterminous United States. US Geo. Survey Data Series 691, 4–9.
Oksanen, J., Blanchet, F., Kindt, R., Legendre, P., Minchin, P., O’hara, R., et al. (2013). Package ‘vegan’. Community ecology package, version 2.
Putnam, R. C., and Reich, P. B. (2017). Climate and competition affect growth and survival of transplanted sugar maple seedlings along a 1700-km gradient. Ecol. Monogr. 87, 130–157. doi: 10.1002/ecm.1237
R Development Core Team, 2020. R: A language and environment for statistical computing. R Foundation for Statistical Computing, Vienna, Austria. http://www.Rproject.org/
Ren, P., Liang, E., Raymond, P., and Rossi, S. (2021). Bud break in sugar maple submitted to changing conditions simulating a northward migration. Can. J. For. Res. 51, 842–847. doi: 10.1139/cjfr-2020-0365
Richardson, A. D., Hollinger, D. Y., Dail, D. B., Lee, J. T., Munger, J. W., and O’keefe, J. (2009). Influence of spring phenology on seasonal and annual carbon balance in two contrasting New England forests. Tree Physiol. 29, 321–331. doi: 10.1093/treephys/tpn040
Richardson, A. D., Keenan, T. F., Migliavacca, M., Ryu, Y., Sonnentag, O., and Toomey, M. (2013). Climate change, phenology, and phenological control of vegetation feedbacks to the climate system. Agricultural and Forest Meteorology. 169, 156–173.
Rosique-Esplugas, C., Cottrell, J., Cavers, S., Whittet, R., and Ennos, R. (2022). Clinal genetic variation and phenotypic plasticity in leaf phenology, growth and stem form in common ash (Fraxinus excelsior L.). For. Int. J. For. Res. 95, 83–94. doi: 10.1093/forestry/cpab026
Rousi, M., and Heinonen, J. (2007). Temperature sum accumulation effects on within-population variation and long-term trends in date of bud burst of European white birch (Betula pendula). Tree Physiol. 27, 1019–1025. doi: 10.1093/treephys/27.7.1019
Roybal, C. M., and Butterfield, B. J. (2018). Functional trait heritability and local climatic adaptation among grasses: a meta-analysis. Plant Ecol. 219, 369–379. doi: 10.1007/s11258-018-0801-y
Scheller, R. M., and Mladenoff, D. J. (2008). Simulated effects of climate change, fragmentation, and inter-specific competition on tree species migration in northern Wisconsin, USA. Clim. Res. 36, 191–202. doi: 10.3354/cr00745
Silvestro, R., Rossi, S., Zhang, S., Froment, I., Huang, J. G., and Saracino, A. (2019). From phenology to forest management: ecotypes selection can avoid early or late frosts, but not both. For. Ecol. Manag. 436, 21–26. doi: 10.1016/j.foreco.2019.01.005
Skinner, M., and Parker, B. L. (1994). “Field Guide For Monitoring Sugar Maple Bud Development” in Vermont Agricultural Experiment Station RR 70 & Vermont Monitoring Cooperative RR. 8th ed (Burlington: University of Vermont)
Sole-Medina, A., Heer, K., Opgenoorth, L., Kaldewey, P., Danusevicius, D., Notivol, E., et al. (2020). Genetic variation in early fitness traits across European populations of silver birch (Betula pendula). AoB Plants 12:plaa019. doi: 10.1093/aobpla/plaa019
Van Buskirk, J., and Steiner, U. (2009). The fitness costs of developmental canalization and plasticity. J. Evol. Biol. 22, 852–860. doi: 10.1111/j.1420-9101.2009.01685.x
Van Kleunen, M., and Fischer, M. (2005). Constraints on the evolution of adaptive phenotypic plasticity in plants. New Phytol. 166, 49–60. doi: 10.1111/j.1469-8137.2004.01296.x
Varsamis, G., Papageorgiou, A. C., Merou, T., Takos, I., Malesios, C., Manolis, A., et al. (2018). Adaptive Diversity of Beech Seedlings Under Climate Change Scenarios. Front. Plant Sci. 9:1918. doi: 10.3389/fpls.2018.01918
Vitasse, Y., Bresson, C. C., Kremer, A., Michalet, R., and Delzon, S. (2010). Quantifying phenological plasticity to temperature in two temperate tree species. Funct. Ecol. 24, 1211–1218. doi: 10.1111/j.1365-2435.2010.01748.x
Vitasse, Y., Hoch, G., Randin, C. F., Lenz, A., Kollas, C., Scheepens, J. F., et al. (2013). Elevational adaptation and plasticity in seedling phenology of temperate deciduous tree species. Oecologia 171, 663–678. doi: 10.1007/s00442-012-2580-9
Wang, X., Piao, S., Ciais, P., Li, J., Friedlingstein, P., Koven, C., et al. (2011). Spring temperature change and its implication in the change of vegetation growth in North America from 1982 to 2006. Proc. Natl. Acad. Sci. U.S.A. 108, 1240–1245. doi: 10.1073/pnas.1014425108
Keywords: Acer saccharum , bud burst, common garden, climate change, ecotype, temperature
Citation: Guo X, Buttò V, Mohytych V, Klisz M, Surget-Groba Y, Huang J, Delagrange S and Rossi S (2023) Plasticity plays a dominant role in regulating the phenological variations of sugar maple populations in Canada. Front. Ecol. Evol. 11:1217871. doi: 10.3389/fevo.2023.1217871
Edited by:
Jie Gao, Xinjiang Normal University, ChinaReviewed by:
Guilin Wu, South China Botanical Garden (CAS), ChinaTaotao Han, Chinese Research Academy of Environmental Sciences, China
Copyright © 2023 Guo, Buttò, Mohytych, Klisz, Surget-Groba, Huang, Delagrange and Rossi. This is an open-access article distributed under the terms of the Creative Commons Attribution License (CC BY). The use, distribution or reproduction in other forums is permitted, provided the original author(s) and the copyright owner(s) are credited and that the original publication in this journal is cited, in accordance with accepted academic practice. No use, distribution or reproduction is permitted which does not comply with these terms.
*Correspondence: Jianguo Huang, aHVhbmcwOTEwQHpqdS5lZHUuY24=